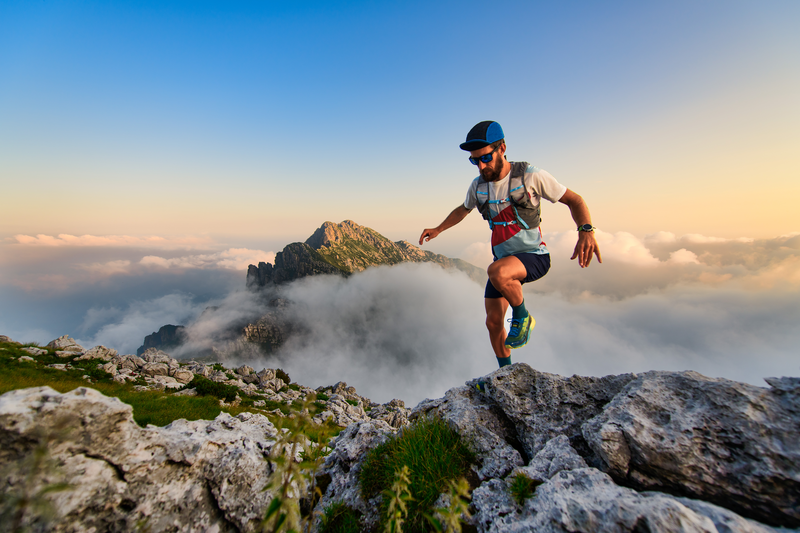
95% of researchers rate our articles as excellent or good
Learn more about the work of our research integrity team to safeguard the quality of each article we publish.
Find out more
ORIGINAL RESEARCH article
Front. Cell Dev. Biol. , 19 August 2022
Sec. Morphogenesis and Patterning
Volume 10 - 2022 | https://doi.org/10.3389/fcell.2022.947376
Specification and elaboration of proximo-distal (P-D) axes for structures or tissues within a body occurs secondarily from that of the main axes of the body. Our understanding of the mechanism(s) that pattern P-D axes is limited to a few examples such as vertebrate and invertebrate limbs. Drosophila Malpighian/renal tubules (MpTs) are simple epithelial tubules, with a defined P-D axis. How this axis is patterned is not known, and provides an ideal context to understand patterning mechanisms of a secondary axis. Furthermore, epithelial tubules are widespread, and their patterning is not well understood. Here, we describe the mechanism that establishes distal tubule and show this is a radically different mechanism to that patterning the proximal MpT. The distal domain is patterned in two steps: distal identity is specified in a small group of cells very early in MpT development through Wingless/Wnt signalling. Subsequently, this population is expanded by proliferation to generate the distal MpT domain. This mechanism enables distal identity to be established in the tubule in a domain of cells much greater than the effective range of Wingless.
Some tubular epithelia, such as nephrons of the kidney, and the oviduct, possess functional polarities along their length, in which distinct domains of cells execute specialised physiological activities. The order of these domains can be important for the emergent properties of the system, for example the sequential processing of primary urine by functionally distinct domains along the length of the nephron. For this reason, the correct and precise establishment of cells with specialised physiological activities along the length of the tube is an important aspect for how the system will ultimately function. Developmental mechanisms that establish and pattern these axes act secondary to those that the establish and pattern the main body axes of the animal. Important insights have been gained into the patterning of epithelial tubules such as kidney nephrons, and the zebrafish pronephros. These insights include transcription factors involved in segment identity (Reggiani et al., 2007; Wingert et al., 2007; Cheng and Wingert, 2015), and signalling molecules such as retinoic acid, BMP, Notch and Wnt necessary for patterning (Wingert et al., 2007; Lindstrom et al., 2015; Schneider et al., 2015; Deacon et al., 2019). There are indications that some of these may act as graded morphogens (Wingert et al., 2007; Lindstrom et al., 2015). However in general these patterning mechanisms remain poorly understood.
Insect Malpighian, or renal tubules (MpTs) are tubular epithelia with a distinct functional axis along their proximo-distal (P-D) length including distinct regions for calcium homeostasis, fluid secretion and reabsorption (Wessing and Eichelberg, 1978; Dow et al., 1994; O’donnell and Maddrell, 1995; Sozen et al., 1997). During embryogenesis, clusters of MpT primordial cells bud out from the wall of the gut, before extending to form each of the MpTs (Skaer, 1993). Functional polarity along the P-D axis is established during this period. It occurs several hours after the patterning of anterior-posterior and dorso-ventral axes of embryo and therefore presents an interesting system to explore how such secondary axes are established and patterned during development. This provides an alternative and therefore informative comparison, for example with P-D patterning of insect appendages which are well-studied examples of secondary axis formation. The MpTs also provide an example of how developmental patterning occurs in the context of a tubular epithelium.
In our previous paper we discovered a mechanism by which the proximal region of the MpT P-D axis is patterned in Drosophila. We determined that the signalling ligand Wingless/Wnt (Wg), produced in gut cells abutting the end of the proximal tubule, patterns the proximal part of the tubule. The ability of the Wg ligand to disperse from its source is essential for this function (Beaven and Denholm, 2018).
The mechanism(s) that establish distal identity is not known, and we therefore turned our attention to this problem here. We considered two putative mechanisms by which patterning of the distal segments of the MpTs could be achieved (Figure 1). In the first, an asymmetric signal from one or other end of the MpT, would play an instructive role in defining distal tubule identity. The signal could either be a distal identity-inducing signal emanating from the distal end of the developing MpT (Figure 1A’), or a distal identity-repressing signal emanating from the gut at the proximal MpT end (Figure 1A’’). We have previously shown that cells derived from the tip cell lineage are a signalling hub that establish a gradient of EGF signalling activity in the distal tubule (Saxena et al., 2014), and thus might be an inductive signal for distal identity. As mentioned above, Wg emanating from the gut at the proximal tubule end induces proximal identity by regulating the expression of genes required for the differentiation of proximal cells (Beaven and Denholm, 2018). It is conceivable that Wg also acts to restrict distal identity by inhibiting the expression of genes required for the differentiation of distal cells.
FIGURE 1. Hypothetical mechanisms for distal patterning in the developing Malpighian tubules. (A′) The distal tubule domain is specified by an inductive signal emanating from the distal tubule end, or (A′′) an inhibitory signal from the proximal tubule end. (B) The distal tubule domain is pre-patterned in the primordium.
The second mechanism we considered is that MpT primordia are already pre-patterned at a stage before they bud from the gut wall and that these domain identities are retained as the MpTs bud out, proliferate and elongate as a tubular structure (Figure 1B). This would be conceptually similar to the way in which the Drosophila legs are patterned, with positional information defined in the leg disc which endures as the disc telescopes out to form the mature leg (Meinhardt, 1983b; Basler and Struhl, 1994; Diaz-Benjumea et al., 1994; Lecuit and Cohen, 1997; Giorgianni and Mann, 2011; refs. Within Ruiz-Losada et al., 2018).
Previous studies suggested this as a plausible mechanism, and indicated that Wg could also be involved in this earlier process. There is a proneural cluster of cells within the MpT primordium, one of which gives rise to the tip-cell and sibling-cell (Hoch et al., 1994; Wan et al., 2000). Wg is expressed in the hindgut, as well as the MpT primordium from the start of tubule development (Skaer and Martinez Arias, 1992), and is required for expression of Achaete in the proneural cluster. Wg is also required for expression of Seven up and PointedP2 (Wan et al., 2000; Sudarsan et al., 2002), two distal transcription factors considered to play roles in promoting cell proliferation (Kerber et al., 1998; Sudarsan et al., 2002).
Here we set out to test these alternative models of how the distal MpT is patterned during development, to gain insights into the principles by which secondary axes are generated and patterned.
Flies were cultured on standard media at 25°C unless otherwise stated. Controls were w1118 or Oregon-R, or balanced sibling embryos. Mutations were balanced over YFP bearing chromosomes (dfd-GMR-nvYFP balancers (Le et al., 2006)), in order that homozygous mutant embryos could be selected. The following stocks were also used: wg-GFP (Port et al., 2014), CtB-Gal4;CtB-Gal4/TM3 (Sudarsan et al., 2002), byn-Gal4 (gift from R. Reuter), UAS-λtop (gift from T. Schüpbach), UAS-EGFR-DN (#5364, BSC), UAS-pangolin/dTCF∆N (Van De Wetering et al., 1997), UAS-armadilloS10 (Pai et al., 1997), wg1-17 (Baker, 1987), cv-cC524 and cv-cM62 (Denholm et al., 2005), Df(1)sc-B57 (González et al., 1989), stg4 (#2500, BSC), dac RE-lacZ (Giorgianni and Mann, 2011), ∆PRE2 (Ogiyama et al., 2018), UAS-hth-dsRNA (TRiP line HMS01112), Nrt-wg, a wg mutant bearing a transgenic insertion of wg fused to Nrt (wg[KO; NRT–Wg; pax-Cherry] (Alexandre et al., 2014)), UAS-Nrt-wg (gift from J-P. Vincent), and a temperature sensitive allele of wg (wg1-12 (Nüsslein-Volhard et al., 1984)). For temperature shift experiments with wg1-12 a restrictive temperature of 25°C was used for depletion of Wg and a permissive temperature of 18°C was used for recovery of Wg levels, with timings as indicated in the text and Figures 7A–C.
Embryos were collected on grape juice agar plates with yeast paste. Embryos were fixed and antibody stained using standard techniques (Weavers and Skaer, 2013). Antibodies were diluted in, and washing steps performed with, PBST + BSA (PBS with 0.3% Triton X-100 and 0.5% bovine serum albumin). The following antibodies were used: anti-Cut (mouse, 1:200, 2B10-c from DSHB), anti-Cut (rabbit, 1:1000, (Grueber et al., 2003)), anti-GFP (goat, 1:500, ab6673, from Abcam), either of two anti-Hth antibodies which produced comparable results (rabbit, 1:1000, AS 1924, (Kurant et al., 1998)) or (goat, 1:100, (dG-20) sc-26187 from Santa Cruz), anti-Dac (mouse, 1:100, mAbdac1-1-c from DSHB), anti-β-galactosidase (rabbit, 1:10,000, ICN Biomedicals), anti-pHH3 (1:1000, Upstate Biotechnology). For fluorescence stainings, secondary antibodies from Jackson ImmunoResearch of the appropriate species tagged with 488, Cy3 or Cy5 fluorophores were used, and DAPI (Molecular Probes) was used 1:1000. Embryos were mounted in Vectashield (Vector Laboratories) or 85% glycerol, 2.5% propyl gallate. In cases where DAB staining was used, following incubation with the first primary antibody, embryos were incubated for 1h with a biotinylated antibody of the appropriate species, washed 3 × 10 min, incubated for 30 min in ABC solution (Vector Elite ABC kit, Vector Laboratories), washed 3 × 10 min and then incubated in 600 μL PBST to which was added 30 μL of 10 mg/ml DAB, 30 μL 0.06% H202, and 60 μL of 1% Nickel (II) chloride in order to develop a black stain. After rinsing 3x in PBST the second primary antibody was added, and the DAB staining protocol outlined above was repeated, except that Nickel (II) chloride was not added so that a brown stain developed. After rinsing 3x in PBST, embryos were dehydrated with 5 min incubations in 50, 70, 90% and 3 × 100% ethanol. Embryos were left overnight in Histoclear, rinsed 2x in acetone and mounted in DPX new (Merck). In situ hybridisation was carried out using the hybridisation chain reaction v3.0 (HCR v3.0) technique using established protocols (Choi et al., 2018) with a probe set size of 20 for cut and Irk3 (Molecular Instruments).
Tubules were dissected from young (<10 day old) adults, and viewed immediately (without fixation) on a slide in PBS.
Fluorescence images were taken using either a Nikon A1R or Zeiss LSM800 confocal microscope. Maximum intensity projection images were generated using Fiji. Images of DAB-stained embryos were imaged with a Zeiss Axioplan light microscope with a Leica DFC 425C camera. Fiji was used for quantification of Wg-GFP localisation along the MpT. Grey values were taken from maximum intensity projections of the anti-GFP images from ∼stage 15–16 embryos, along a line from the gut at the proximal tubule end, extending along a MpT. Following background subtraction, the intensity values were normalised to give the brightest pixel a value of 100. Means were calculated for each pixel position and finally the data was adjusted to give a maximum staining intensity of 100 (arbitrary units).
To assess patterning of the distal MpT, we used markers of the transcription factor Homothorax (Hth), which is expressed in the distal segment of the MpTs (Zohar-Stoopel et al., 2014; Figures 2A–D), and Dachshund (Dac), which we found to also be expressed in this segment (Figures 2E,F). These genes are likely to be involved in normal differentiation of the distal cells, leading to their specialised structure and physiological function. Indeed, Hth has been implicated in correct positioning of the kink in each anterior MpT (Zohar-Stoopel et al., 2014), and we found that following depletion of Hth by RNAi, excessive uric acid can be observed in the adult MpT, suggesting a disruption in normal physiological function (Supplementary Figures S1A,B).
FIGURE 2. Homothorax and Dachshund are expressed in a distal domain of developing Malpighian tubules. (A-D) Stage 11–16 embryos (as indicated) stained with anti-Cut to show MpT nuclei, and with anti-Homothorax (Hth). Homothorax starts to be detectable in nuclei of the distal MpT from about stage 11 onwards (arrowheads). Single channel images equate to region in blue box of merged image. Posterior MpTs are also indicated (*), as are posterior spiracles (ps), which also express Cut and Hth. Scale bar = 50 μm. (E,F) Stage 14–16 embryos (as indicated) stained with anti-Dachshund. Scale bar = 50 μm.
We then used genetic approaches to manipulate EGF and Wg signalling (Figures 3A–E and Supplementary Figures S2A–E). We drove expression of constitutively active EGFR (UAS-λtop) or dominant negative EGFR (UAS-EGFR-DN) in the developing tubules using CtB-Gal4 which expresses in all MpT cells from the stage when they bud from the gut (Sudarsan et al., 2002). If EGF signalling is required to induce distal identity it would be expected that the constitutively active form would induce expression of distal markers throughout the MpT, whilst distal marker expression would be abolished by the dominant negative form. Distal tubule identity, indicated by the pattern of distal marker expression, was maintained in both of these conditions (Figures 3A–C, Figure 4A, and Supplementary Figures S2A–C). This argues against a mechanism whereby EGF signalling is required for distal MpT cell identity.
FIGURE 3. EGF or Wg pathway activity do not appear to pattern the distal Malpighian tubule. (A–E) ∼Stage 16 embryos stained to show MpT nuclei (anti-Cut) and the nuclei of cells from the distal MpT segment (anti-Dac, arrowheads). In each example at least one of the pair of anterior MpTs is clearly visible. (A) Control (w1118) embryo. (B) CtB-Gal4>UAS-λtop embryo. (C) CtB-Gal4>UAS-EGFR-DN embryo. (D) CtB-Gal4>UAS-pan/dTCF∆N embryo. (E) CtB > UAS-armS10 embryo. Scale bar = 50 μm.
FIGURE 4. Wingless is required for expression of distal tubule markers. (A) Counts of the number of Malpighian tubule cell nuclei expressing distal marker, Dachshund. Generally, for each embryo the number of nuclei staining with anti-Dac in a single anterior Malpighian tubule were counted. This was not possible in all genotypes depending on the morphology, and in this case the count was from a single tubule if discernible, or the entire mass of tubule cells if not. W1118 (control) n = 16. OrR (control) n = 10. CtB>λtop n = 20, p = 0.087. CtB > EGFR-DN n = 12, p < 0.00001***. CtB > pan∆N = 12, p = 0.000012***. CtB > armS10 n = 16, p < 0.00001***. Wg1-17 n = 20, p < 0.00001***. Byn > pan∆N = 19, p < 0.00001*** (p = 0.81 comparing to wg1-17). Byn > armS10 n = 7, p = 0.040*. Byn > Nrt-wg n = 13, p = 0.00070***. Cv-cM62 embryos n = 16, p < 0.00001*** (p < 0.00001*** comparing to wg1-17). Df(1)sc-B57 n = 20, p < 0.00001*** (p = 0.00018*** comparing to wg1-17). Stg4 n = 22, p < 0.00001*** (p = 0.062 comparing to Df(1)sc-B57, p = 0.020* comparing to wg1-17). Nrt-wg n = 6, p = 0.28. N numbers represent number of embryos. p-values calculated using Mann-Whitney U test comparing to the w1118 control group, except where stated otherwise. (B–D) and (G–H) ∼Stage 16 embryos stained to show MpT nuclei (anti-Cut, brown) and the nuclei of cells from the distal MpT segment (anti-Dac, black, arrowheads). Arrows indicate MpTs, in cases where anti-Dac staining is absent. (E, F) ∼stage 16–17 embryos stained for cut and Irk3 mRNA. (B) Control embryo (w1118). (C) Homozygous wg1-17 embryo. (D) Byn-Gal4>UAS-pan/dTCF∆N embryo. (E) Control (w1118) embryo. (F) Homozygous wg1-17 embryo. (G) Byn-Gal4>UAS-armS10 embryo. (H) Byn-Gal4>UAS-Nrt-wg embryo. Scale bars = 50 μm. Scale bar in H applies to (B–D), (G, H). Scale bar in F applies to (E, F).
We also tested the effect of driving expression of a construct which inhibits Wg signalling (UAS-pan/dTCF∆N) or which constitutively activates Wg signalling (UAS-armS10) using CtB-Gal4. We previously reported that both of these genotypes disrupt the normal proximal patterning of the MpTs (Beaven and Denholm, 2018). If Wg signalling is required to repress distal identity it would be expected that distal identity markers would be expressed throughout the MpT upon inhibition of Wg signalling, and that their expression would be lost when constitutively activating Wg signalling. However distal marker expression was maintained in the distal tubule in these conditions (Figures 3A,D,E, Figure 4A, Supplementary Figures S2A,D,E). Therefore we find no evidence that Wg in the proximal MpT acts to inhibit distal tubule identity.
These experiments extinguish EGF and Wg signalling pathways, the two strongest candidate pathways, as signals emanating from one or other end of the MpT to define distal identity. However they do not rule out the possibility that other signalling pathways could be involved in such a mechanism.
In contrast to our findings when manipulating Wg using reagents driven by the CtB-Gal4 driver, we observed that in a null mutant of wg (wg1-17), expression of distal markers in the MpTs is abolished in nearly all cases (Figures 4A–C, and Supplementary Figures S3A,B). Note that MpTs are much shorter in this mutant, as previously reported (Skaer and Martinez Arias, 1992), relating to roles of Wg in specifying the tip-cell and driving cell proliferation. This seeming contradiction to the data reported above could be explained by the timing of Wg manipulation when using the CtB-Gal4 driver line. The CtB enhancer is expressed in the developing MpTs from stage 11 or earlier, and would be expected to be expressed from the very start of their development (Jack and Delotto, 1995; Skaer, 2002; Sudarsan et al., 2002), however it may be that the Gal4 protein does not reach sufficient levels to induce good inhibition of Wg signalling until later in MpT development. It therefore seems plausible that Wg participates in specifying distal identity early on in MpT development, in the period before CtB-Gal4 is effective. This notion is also supported by the normal size of the MpTs when manipulating Wg using the CtB-Gal4 driver, suggesting that Wg’s early roles, for example in cell division, are not significantly perturbed by manipulations using CtB-Gal4.
To further test the hypothesis that Wg acts in early MpT development, we repeated the UAS-pan/dTCF∆N experiment using brachyenteron-Gal4 (byn-Gal4), which is expressed in the proctodeum in the shared primordia that gives rise to the hindgut and MpTs, and subsequently in the developing MpTs (Hatton-Ellis et al., 2007; Weavers and Skaer, 2013). This produced a similar result to wg1-17 in that MpTs were greatly reduced in size, and distal identity, as indicated by Dac and Hth expression, was generally abolished (Figures 4A–D). Wg is therefore required to establish distal identity.
These findings suggest a role for Wg in correctly specifying the identity of the distal MpT, and this would be expected to be necessary for the differentiation of the distal tubule to acquire its specific physiological functions. Inwardly rectifying potassium channels have reported roles in potassium transport and fluid secretion in MpTs (Evans et al., 2005; Wu et al., 2015), and one member, Irk3, is expressed in the distal tubules by late embryogenesis (Döring et al., 2002). We therefore looked at the expression of Irk3 mRNA expression, and found that the distal tubule expression observed in late embryos was absent in almost all (9/10) wg1-17 embryos observed (Figures 4E,F).
We also tested whether the distal tubule domain could be expanded by ectopic activation of Wg signalling. We expressed either UAS-armS10 or membrane tethered Wg (UAS-Nrt-wg), using byn-Gal4 (Nrt-Wg is known to function at least as well in activating Wg signalling as untethered wild-type Wg (Zecca et al., 1996; Beaven and Denholm, 2018; Chaudhary et al., 2019)). Neither of these manipulations led to an expansion of the distal expression of Dac (Figures 4B,G,H). Together, these data show Wg function is necessary but not sufficient for distal tubule identity. The lack of sufficiency suggests other factors act in concert with Wg to establish distal identity.
Our findings therefore support a model that Wg acts to specify distal cell identity in the MpT primordium early in MpT development (Figure 1B). However alternative hypotheses could also explain this phenotype. Firstly, we have not ruled out a signal other than Wg emanating from the gut to repress distal MpT gene expression (Figure 1A”). This would be another way to explain the loss of distal identity in the wg mutant tubules, as the tubules are much smaller which could bring all the MpT cells within range of the putative inhibitory signal. We previously found that crossveinless c mutant tubules (cv-cC524 or cv-cM62) are morphologically abnormal and often do not extend far from the gut (Denholm et al., 2005), providing an ideal means to test this hypothesis. We found that regions of these short cv-c mutant tubules expressed the distal markers Hth and Dac (Figure 4A and Figures 5A–D). This provides further evidence against a model for an inhibitory signal emanating from the proximal end of the MpT to inhibit distal identity (Figure 1A’’), and also indicating that such an inhibitory signal does not account for the loss of distal identity in the shortened wg mutant.
FIGURE 5. Reducing Malpighian tubule length does not lead to inhibition of distal transcription factor expression. (A,B) ∼Stage 16 embryos stained to show MpT nuclei (anti-Cut) and the nuclei of cells from the distal MpT segment (anti-Dac, arrowheads). (C,D) ∼Stage 16 embryos stained to show MpT nuclei (anti-Cut) and the nuclei of cells from the distal MpT segment (anti-Hth, arrowheads). (A) Control cv-cM62/+ or +/+ embryo. (B) Homozygous cv-cM62 embryo. (C) Control cv-cC524/+ or +/+ embryo. (D) Homozygous cv-cC524 embryo. Single channel images equate to region in blue box of merged image. Scale bar = 50 μm.
A second alternative hypothesis to explain the loss of Dac expression in the wg mutant MpTs relates to the role of the tip- and sibling-cells. As well as producing the EGF ligand Spitz (Saxena et al., 2014), the tip-/sibling-cell(s) could act to produce other signals which induce distal MpT identity. It is known that Wg is required for formation of the tubule tip/sibling-cells through its role in activating expression of achaete-scute complex (as-c) genes (Wan et al., 2000), so it is conceivable that the loss of Dac expression in the wg mutant is an indirect consequence of losing the tip and sibling-cells.
We therefore analysed embryos mutant for the as-c genes (Df(1)sc-B57) which fail to develop the tip/sibling-cells (Hoch et al., 1994; Sudarsan et al., 2002). In mutant embryos, the MpTs still expressed distal transcription factors (Figure 4A, Figures 6A,B, Supplementary Figures S4A,B). This experiment is informative in two ways: it provides clear evidence against a requirement for the tip-cell lineage in specifying distal identity downstream of Wg, i.e., it points to a more direct role for Wg in specifying distal identity. Secondly, it provides further evidence against the model of distal identity induction by a signal originating from the tip/sibling-cells (Figure 1A’).
FIGURE 6. Achaete-scute complex genes are not required to specify distal tubule identity, but are required to expand the pool of distal identity cells. (A,B,D) ∼Stage 16 embryos stained to show the nuclei of the MpT cells (anti-Cut, brown) and nuclei from the distal MpT cells (anti-Dac, black, arrowheads). (A) Control (w1118) embryo. (B) Homozygous Df(1)sc-B57 embryo. (C) Homozygous stg4 embryo. (D) Wild-type (OrR) embryo stained to show dividing cell nuclei (anti-pHH3) in relation to nuclei of the distal MpT cells (anti-Dac). For tissue context all nuclei are stained with DAPI. Single channel images equate to region in blue box of merged image. Scale bars = 50 μm.
Intriguingly, Dac and Hth are expressed only in a small cluster of cells at the distal tubule end, in the as-c mutant embryos (Figures 6A,B, Supplementary Figures S4A,B). We considered the best explanation of this result to be that the distal identity is specified in a small population of tubule cells early in tubule development, with this pool of cells then being expanded (during stages 12/13) by cell proliferation driven by the tip-cell. If this hypothesis is correct, then blocking cell division should also reduce the number of tubule cells expressing distal transcription factors. In string mutant embryos (stg4) cell division is arrested in cycle 14 (Edgar and O’farrell, 1990). We found that only a very small number of tubule cells expressed distal markers in this mutant (Figure 4A, Figure 6C, Supplementary Figure S4C), which supports our hypothesis of early specification, and subsequent amplification, of distal identity cells. This model also makes sense in light of the position of the tip-cell at the very distal end of the tubule, and the fact that it is known to drive proliferation by secretion of the EGF ligand, spitz (Sudarsan et al., 2002), which activates the EGFR pathway most highly in the distal-most cells (Saxena et al., 2014). We also examined the pattern of proliferation (using the proliferation marker, anti-phospho-histone H3, anti-pHH3) in relation to the distal marker, Dac, in tubules during tip-cell driven cell proliferation. We observed proliferative cells inside the Dac expression domain in 9/10 cases and outside this domain in only 1/10 (Figure 6D). This supports the model that the distal cell population is expanded by tip-cell driven proliferation. These findings also resolve the possibility that the lack of distal cells seen in the wg mutant condition results from an inability to generate the distal cell population, relating to the known role of Wg in driving MpT cell proliferation. It is known that in wg mutant embryos, MpT cells cease dividing after mitotic cycle 15 (Skaer and Martinez Arias, 1992; Wan et al., 2000), however we do still see cells expressing distal markers in the stg mutant where cell division is arrested in mitotic cycle 14.
As a direct test of the hypothesis that Wg is required in the tubule primordium in the very early stages of tubule development, we made use of a temperature sensitive mutant (wg1-12), which has previously been used to map the temporal requirements of Wg in tubule development (Skaer and Martinez Arias, 1992; Beaven and Denholm, 2018). In batches of wg1-12 embryos which had developed at the restrictive temperature for 195 ± 30 min, before switching to the permissive temperature for the rest of development, tubules still expressed Dac (100%, n = 12; Figure 7A). This establishes that downshift before ∼230 min does not result in a loss of Dac expression. However in batches of wg1-12 embryos which had developed at the restrictive temperature for 260 ± 30 min before shifting to the permissive temperature, few embryos expressed Dac (17%, n = 12; Figure 7B). For embryos raised at the restrictive temperature for 320 ± 30 min before shifting to the permissive temperature, no embryos expressed Dac (100%, n = 12; Figure 7C). Therefore shifting to the permissive temperature at some point between 230–290 min onwards results in a loss of Dac, and we estimate that this point is approximately 240 min. Taking into account that Wg function is considered to take ∼25 min to recover after shifting to a permissive temperature (Skaer and Martinez Arias, 1992), this suggests that Wg must be present from roughly 265 min into embryogenesis to confer distal tubule identity. This equates to early stage 10. In line with our pre-patterning hypothesis (Figure 1B), we have found evidence that the requirement of Wg in conferring distal tubule identity is very early in MpT development: in the tubule primordium before the tubules bud out from the gut. The time of Wg requirement is also comparable, or slightly earlier than, its requirement for allocation of the tip/sibling-cells, early in the development of the MpT (Wan et al., 2000).
FIGURE 7. Wingless is required during early tubule formation for specification of distal tubule identity. (A–C) ∼Stage 15–16 embryos stained to show MpT nuclei (anti-Cut) and the nuclei of cells from the distal MpT segment (anti-Dac, arrowheads). Arrows show distal MpT regions not expressing Dac. Embryos are homozygous for a temperature sensitive allele of wg (wg1-12). (A) Raised at the restrictive temperature until 160–230 min, when shifted to the permissive temperature. (B) Raised at the restrictive temperature until 230–290 min when shifted to the permissive temperature. (C) Raised at the restrictive temperature until 290–350 min when shifted to the permissive temperature. Single channel images equate to region in blue box of merged image. Scale bar = 50 μm. (D) ∼Stage 15–16 embryo stained to show MpT nuclei (anti-Cut, brown) and the nuclei of cells from the distal MpT segment (anti-Dac, black, arrowheads). Embryo is a homozygote in which endogenous wg has been replaced with Nrt-wg. Scale bar = 50 μm.
We were curious to know whether the role of Wg in specifying distal identity in the MpT primordium requires release and spread of the Wg ligand, as we had demonstrated a requirement for Wg dispersal in patterning the proximal tubule (Beaven and Denholm, 2018). Therefore we assessed the expression pattern of Dac in tubules using a fly line in which endogenous Wg is replaced with a non-diffusible membrane tethered form (Nrt-wg) (Alexandre et al., 2014). We found that the pattern of Dac expression appears normal in Nrt-wg tubules (Figure 7D). This suggests that the requirement for Wg in establishing distal identity occurs within the cells which express Wg (autocrine signalling) and/or their immediate neighbours (juxtacrine signalling). This contrasts to the role of Wg in patterning the proximal MpT, where release and spread is essential.
In order to better understand the role of Wg during MpT development, we made use of a line in which Wg has been tagged with GFP at the endogenous genetic locus (Port et al., 2014). In fixed samples, anti-GFP staining of these embryos gave much clearer staining than we have seen using anti-Wg (Beaven and Denholm, 2018). We combined this with the tubule marker anti-Cut, and observed the expression pattern of Wg-GFP in relation to the developing tubules from the tubule primordium at stage 10, until the tubules had formed at stage 16 (Figures 8A–G).
FIGURE 8. Wingless is present in the half of the tubule primordium that abuts the developing hindgut. (A–E) ∼Stage 10–16 embryos expressing Wg-GFP, stained with anti-GFP, along with anti-Cut to show nuclei of MpT cells. Wg-GFP can be observed in (A) MpT cells closest to the hindgut as the MpTs begin budding out at stage 10, (B–D) on one side of the MpTs at stage 11–12 (arrowheads in C + D), (C–E) and a gut region near the midgut-hindgut boundary at the proximal MpT ends from late stage 11. (F) In a late stage (∼stage 15–16) embryo, Wg-GFP localises most strongly to a region near the midgut-hindgut boundary (*) from where a gradient extends into the midgut (arrow) and into the two tubule ureters (arrowheads), which branch into the anterior and posterior pairs of MpTs. Single channel images equate to region in blue box of merged image. In A the developing hindgut (hg) and midgut (mg) are indicated. Scale bar = 50 μm. (G) A graph of the staining intensity of anti-GFP to show the distribution of Wg-GFP from the proximal tubule end. n = 4. Error bars show S.E.M.
At stage 10, we found that Wg-GFP is expressed in the region of the tubule primordium abutting the developing hindgut primordium, but not the region abutting the developing midgut (Figure 8A). This is consistent with the pattern observed with anti-Wg staining (Wan et al., 2000). This region of the tubule primordium is the region from which the tip mother cell segregates (Wan et al., 2000), and is therefore likely to be the population of cells from which the distal cell lineage is derived. This patch of early Wg expression coincides with the period Wg activity is required to establish distal identity in the tubule (previous section; Figure 9A), strengthening the evidence that Wg is the early patterning signal.
FIGURE 9. Model of distal patterning of the Malpighian tubules. (A) During the early stages of MpT formation (stage 9–10) Wg is expressed in the part of the MpT primordium abutting the hindgut, and is required for specifying distal cell identity. (B) As the early MpTs bud out from the wall of the developing gut (stage 11), Wg is expressed on one side of the MpT where it functions in driving cell proliferation. (C) In stage 12 W g is expressed in a further, distinct region in the midgut at the base of the tubule. Wg forms a gradient extending into the proximal tubule from the gut, and this is required to activate expression of the proximal identity factor Odd skipped (orange). During this stage the newly formed tip/sibling-cells produce the EGF ligand, spitz, which drives cell proliferation in a distal domain. This enlarges the distal cell population (D), and is therefore necessary for the distal domain to reach its correct final size.
We have found evidence of a mechanism in which epithelial tubules, the Malpighian tubules of Drosophila, achieve patterning of their P-D axis by early specification of a subgroup of cells which expand during development, giving rise to the distal domain (Figures 9A–D). Wg signalling during early MpT development plays a critical role in specifying their distal identity. This mechanism of early patterning followed by expansion does not rely on dispersal of the Wg ligand. This is in contrast to our previous findings for the role of Wg in patterning the proximal tubule, which does require release and spread of Wg.
In the context of proximal patterning, Wg activates Odd skipped (Odd) expression to a distance of ∼30 μm (Beaven and Denholm, 2018; Figure 9C). Using Wg-GFP, we find that in late stage embryos (∼stage 15–16) Wg in the proximal tubule clearly forms a gradient, which extends ∼25 μm into the MpT (Figures 8F,G). It also appears to extend ∼40 μm into the midgut (Figures 8F,G). This fits well with the range of Wg dispersal in the wing imaginal disc, which is in the order of ∼30 μm (Chaudhary et al., 2019), as well as the range of the Wg-GFP gradient observed in the adult gut (Tian et al., 2019). These figures may constitute the upper limit of Drosophila Wg dispersal. Considering that the anterior tubules extend to over 300 μm by late embryogenesis, and that the domains of distal transcription factors such as Dac and Hth extend to about half this length, this would make the final size of distal expression domains much greater than the effective range of Wg. It seems that the maximum range of Wg is too small to allow Wg scaling to pattern the distal MpT in the manner of a classical morphogen (as, for example, Decapentaplegic (Dpp) does in the wing disc, scaling to 100 μm as the disc reaches full size (Teleman and Cohen, 2000; Wartlick et al., 2011)). This could explain why Wg defines the distal tubule domain in the tubule primordium whilst it is a small cluster of cells, and these cells then maintain their distal identity as they proliferate and the tubule elongates.
Our findings that Wg acts very early in MpT development to define distal cell identity also provoked us to contemplate whether the Wg signal is the earliest symmetry breaking step by which the axis is established. Intuitively one might expect the distal cells to arise from the centre of the primordium, as these would naturally become distal as the tubule telescopes out during development. Such a mechanism is responsible for P-D patterning of the Drosophila legs; here the discs are patterned as concentric rings, with the centre of the disc ultimately becoming the distal end of the leg (Estella et al., 2012). This patterning is achieved by first specifying the central point of the disc which becomes the distal pole of the P-D axis. This is achieved by Dpp and Wg which are expressed dorsally and ventrally at the anterior-posterior compartment boundary, under regulation of hedgehog from the posterior compartment. The intersection of the Dpp and Wg signals activates Distal-less expression at the centre of the leg disc (Basler and Struhl, 1994; Campbell et al., 1993; Diaz-Benjumea et al., 1994). However, our observations in the MpTs are different, in that the cells that ultimately give rise to the distal MpT originate on one side of the MpT primordium—the side which abuts the hindgut and expresses Wg (Figure 8A, Figure 9A), rather than at the centre of the primordium. It is conceivable that the established axis of the developing gut acts to break symmetry in the MpT primordium and thus establish the P-D axis of the tubules. In this context the expression of Wg on one side of the MpT primordium is likely to be regulated by the processes concomitantly patterning the developing gut, such as the division of the hind- and midgut. Further processes define a proneural cluster of cells in the developing MpT, and specify the tip-cell lineage within this cluster. These processes are perturbed by depletion of Wg in early MpT development (Wan et al., 2000), which may be because only the distal lineage of MpT cells are competent to give rise to the proneural cluster. The emergence of the tip/sibling-cells as a signalling centre could then orchestrate the morphological changes underlying tubule elongation, in a way which results in their surrounding cells coming to have a distal position in the final tubule. The EGF signal which emanates from the tip/sibling-cells drives cell proliferation (Baumann and Skaer, 1993; Kerber et al., 1998; Sudarsan et al., 2002; Figure 9C), as well as defining planar cell polarity in surrounding cells to orchestrate their intercalation and the convergent extension of the distal MpT (Saxena et al., 2014), so orchestrating the morphological changes which propel the tip/sibling-cells, and their neighbouring cells with distal identity, to finally reside at the distal end of the extended MpT. Together these processes could convert an already established asymmetry in the gut, into a P-D axis in the MpTs which grow out perpendicular to the gut axis.
The formation of this secondary axis is conceptually similar to the formation of the body’s main axes, in that both are underpinned by the breaking of symmetry under influence from external source(s). It also shows parallels to formation of other studied secondary axes such as Drosophila legs, where establishment of the P-D axis requires an initial breaking of symmetry which is derived from patterning of the main body axes. In diverse examples, including all the Drosophila imaginal discs, as well as vertebrate limbs, primordia develop straddling compartment boundaries formed along the primary body axes. As the cells of the primordia straddle boundaries, they are conferred a developmental asymmetry from the outset, allowing them to establish secondary axes (Meinhardt, 1983a; Meinhardt, 1983b; Basler and Struhl, 1994; Tabata et al., 1995). This may explain why the MpT primordia arise precisely at the boundary between the developing hindgut and midgut.
The mechanism we have uncovered for patterning the distal tubules, i.e. early patterning and expansion of a distal domain, must require some form of cellular memory. Distal cells appear to begin expressing transcription factors such as Dac and Hth some time after their exposure to the Wg signal, and their expression is maintained into the adult, in the absence of Wg. Therefore, there must be a mechanism for cells to retain their identity in the absence of the initial signal. One means to achieve such cellular memory is transcriptional memory as has been characterised for Hox genes, for example in conferring positional identity along the anterior-posterior axis, as well as for hedgehog expression in the Drosophila wing discs. Transcriptional memory of Hox genes and hedgehog involves binding of Trithorax- and Polycomb-group proteins to Trithorax response elements (TREs) and Polycomb response elements (PREs) which are regulatory chromosomal regions (Francis and Kingston, 2001; Maurange and Paro, 2002; Wang et al., 2009; Pérez et al., 2011).
In the case of patterning mechanisms by Wg, it has been shown in wing discs that the frizzled 2 receptor can function to maintain Wg signalling in cells which are no longer exposed to the Wg ligand (Chaudhary et al., 2019). We do not think that this mechanism is the one used in the MpTs, as it relies on perpetuating activity of the Wg signalling pathway (which we found not to be necessary in our experiment driving dTCF∆N, Figure 3D, Supplementary Figure S2D). It has also been shown in gastruloids that cells retain memory of their exposure to Wnt via an unknown mechanism, which controls their responsiveness to activin (Yoney et al., 2018).
To further understand the mechanisms by which Wg could regulate distal target genes, we looked at the activity of dac enhancers containing dTCF/Pan binding sites, but found that these enhancers, which regulate expression of dac in the leg disc, are not responsible for dac expression in the distal tubule (Supplementary Figure S5). This provides evidence to suggest regulation by Wg is indirect (at least for dac), indicating that Wg might trigger a signalling cascade which activates and maintains expression of distal identity genes. Testing the regulation of Hth may also shed light on this mechanism. A genomic fragment of hth was previously found which contains the enhancer region responsible for its expression in the distal MpT (Zohar-Stoopel et al., 2014). There is a dTCF/Pan binding site within this region (Junion et al., 2012), which provides a putative site for direct Wg regulation of hth expression in the MpT.
We have found that Wg, acting at different times during development, plays roles in patterning both the distal and proximal ends of the MpT, and is required for the expression of different target genes in these two contexts. This highlights how context dependent the outcomes of Wg signalling are, even within a single tissue. We had previously found that constitutively activating Wg signalling throughout the MpT leads to an expansion of the proximal Odd expression domain, but that the majority of the MpT including all of the distal region still lacked Odd expression (Beaven and Denholm, 2018). In contrast, we report here that activating Wg signalling throughout the MpT from the very beginning of its development does not cause expansion of the distal domain, as indicated by Dac expression (Figures 4B,G,H). The different contexts may be underpinned by differences in the signals which proximal and distal MpT cells have encountered during their development, apart from Wg.
We first observed Wg expression in the half of the tubule primordium which abuts the hindgut at stage 10 (Figure 8A, Figure 9A). At stage 11 we observed Wg expression on one side of the MpT (Figures 8B,C). This expression is consistent with a previous report of wg mRNA and Wg protein expression at this stage, and may be a continuation of expression seen at stage 10. At stage 11 Wg drives cell division in this cell population (Skaer and Martinez Arias, 1992; Sudarsan et al., 2002; Figure 9B), as well as acting to specify the tip-cell lineage (Wan et al., 2000). Secondly, from late stage 11, throughout the rest of embryogenesis, we observed another distinct region of Wg expression in the developing gut abutting the proximal tubule (Figures 8C–F, Figures 9C,D). This is the region of Wg that specifies the identity of a proximal tubule domain, being required for expression of Odd (Beaven and Denholm, 2018). This expression of Wg at the midgut-hindgut boundary persists stably through larval and adult stages, where it regulates cellular behaviours of the gut and MpT (Takashima et al., 2008; Fang et al., 2016; Tian et al., 2016; Xu et al., 2018; Tian et al., 2019).
Context dependence would allow Wg to play a complex and rapidly changing set of roles in MpT development, in specifying distal identity and the tip-cell lineage in the primordium, driving cell proliferation on one side of the tubule and in specifying proximal identity in the later MpT. These multiple, sequential phases of Wg activity are reminiscent of findings from the wing discs. Here the first phase of Wg function is to subdivide the identity of the disc, by contributing to specification of wing fate as opposed to wing hinge and notum fate. This occurs during the larval second instar, during which Wg is expressed in a wedge of cells in the ventral/anterior compartment (Couso et al., 1993; Williams et al., 1993; Ng et al., 1996; Klein and Arias, 1998). Wg is expressed in the entire wing primordium until early third instar larvae where it contributes to growth and patterning of the disc, but is not expressed in the rest of the wing disc. By the late third larval instar Wg is only expressed in a narrow line of cells at the presumptive wing margin, in a double ring around the distal region of the wing and in part of the notal region (Couso et al., 1993; Williams et al., 1993; Couso et al., 1994; Ng et al., 1996; Martinez Arias, 2003; Alexandre et al., 2014). The roles of Wg at the presumptive wing margin include patterning the wing (Swarup and Verheyen, 2012 - references within; Chaudhary et al., 2019).
The findings here provide mechanistic insights into how a secondary axis is established and patterned in an epithelial tubule. Epithelial tubules are a fundamental component of many organs, and are frequently patterned along their P-D axis. The oviduct or fallopian tube is one example of this, and recent evidence shows that distinct distal and proximal populations of oviduct cells are specified early in development before the oviduct has extended by cell proliferation. These 2 cell populations form distinct stable lineages (Ford et al., 2021). It may therefore be that early patterning followed by tissue expansion could be a widespread means to pattern the P-D axis of epithelial tubules. Such a mechanism may be important in patterning the P-D axis of developing epithelial tubules of the lungs for which there are indications of a role for Wnt signalling, as well as nephrons in the kidney, which are also known to require β-catenin, most likely acting in the Wnt signalling pathway (Mucenski et al., 2003; Lindstrom et al., 2015; Deacon et al., 2019). In the case of kidney nephrons, there is evidence that a persistent gradient of β-catenin signalling is required for correct patterning (Lindstrom et al., 2015), and this may be more akin to what we observe in later stages of MpT development, where a Wingless gradient patterns the proximal tubule (Beaven and Denholm, 2018; Figures 8F,G, Figure 9C). However in common with the mechanisms we report here, the P-D domains that are defined in the developing nephron, appear to be subsequently expanded by cell proliferation (Lindstrom et al., 2015). Furthermore, β-catenin signalling appears to act reiteratively during nephron development, for example it is required for conferring distal identity, and also for the final differentiation of the proximal tubule domain (Lindstrom et al., 2015; Deacon et al., 2019). This is similar to the highly dynamic, context dependent roles of Wingless signalling which we have characterised in the MpTs. How widespread early patterning and subsequent tissue growth are utilised for patterning P-D axes in other epithelial tubules remains an open question, requiring further investigation.
The original contributions presented in the study are included in the article/Supplementary Material, further inquiries can be directed to the corresponding author.
Conceptualization—BD and RB, Investigation—RB, Visualization—RB and BD, Writing—RB and BD, Funding acquisition—BD.
This work has been funded by the Biotechnology and Biological Sciences Research Council [BB/N001281/1] and The Leverhulme Trust [RPG-2019-167]. For the purpose of open access, the author has applied a CC BY public copyright licence to any Author Accepted Manuscript version arising from this submission.
We are very thankful to the following people and organisations for their help. For fly stocks: Richard Mann and Anthony Gilmore (dac RE-lacZ), Giacomo Cavalli and Bernd Schuttengruber (∆PRE2), Fillip Port (wg-GFP), Jean-Paul Vincent and Joachim Kurth (Nrt-wg), Rolf Reuter (byn-Gal4), Trudi Schüpbach (UAS-λtop), the Bloomington Drosophila Stock Center and Resource Center (National Institutes of Health grants P40OD018537 and 2P40OD010949-10A1) and the Vienna Drosophila Resource Center (VDRC). For rabbit anti-Cut Yuh Nung and Tong Cheng. For assistance with imaging, Anisha Kubasik-Thayil and Marta Czapranska from the IMPACT imaging facility, University of Edinburgh. For useful advice and reagents, Kyra Campbell, members of Andrew Jarman’s lab and the Edinburgh fly club.
The authors declare that the research was conducted in the absence of any commercial or financial relationships that could be construed as a potential conflict of interest.
All claims expressed in this article are solely those of the authors and do not necessarily represent those of their affiliated organizations, or those of the publisher, the editors and the reviewers. Any product that may be evaluated in this article, or claim that may be made by its manufacturer, is not guaranteed or endorsed by the publisher.
The Supplementary Material for this article can be found online at: https://www.frontiersin.org/articles/10.3389/fcell.2022.947376/full#supplementary-material
Alexandre, C., Baena-Lopez, A., and Vincent, J. P. (2014). Patterning and growth control by membrane-tethered Wingless. Nature 505 (7482), 180–185. doi:10.1038/nature12879
Baker, N. E. (1987). Molecular cloning of sequences from wingless, a segment polarity gene in Drosophila: The spatial distribution of a transcript in embryos. EMBO J. 6 (6), 1765–1773. doi:10.1002/j.1460-2075.1987.tb02429.x
Basler, K., and Struhl, G. (1994). Compartment boundaries and the control of Drosophila limb pattern by hedgehog protein. Nature 368 (6468), 208–214. doi:10.1038/368208a0
Baumann, P., and Skaer, H. (1993). The Drosophila EGF receptor homologue (DER) is required for Malpighian tubule development. Development l, 65–75. doi:10.1242/dev.119.supplement.65
Beaven, R., and Denholm, B. (2018). Release and spread of Wingless is required to pattern the proximo-distal axis of Drosophila renal tubules. Elife 7, e35373. doi:10.7554/eLife.35373
Campbell, G., Weaver, T., and Tomlinson, A. (1993). Axis specification in the developing Drosophila appendage: the role of wingless, decapentaplegic, and the homeobox gene aristaless. Cell 74 (6), 1113–1123.
Chaudhary, V., Hingole, S., Frei, J., Port, F., Strutt, D., and Boutros, M. (2019). Robust Wnt signaling is maintained by a Wg protein gradient and Fz2 receptor activity in the developing Drosophila wing. Development 146 (15), dev174789. doi:10.1242/dev.174789
Cheng, C., and Wingert, R. (2015). Nephron proximal tubule patterning and corpuscles of Stannius formation are regulated by the sim1a transcription factor and retinoic acid in zebrafish. Dev. Biol. 399 (1), 100–116. doi:10.1016/j.ydbio.2014.12.020
Choi, H. M. T., Schwarzkopf, M., Fornace, M. E., Acharya, A., Artavanis, G., Stegmaier, J., et al. (2018). Third-generation in situ hybridization chain reaction: Multiplexed, quantitative, sensitive, versatile, robust. Development 145 (12), dev165753. doi:10.1242/dev.165753
Couso, J., Bate, M., and Martínez-Arias, A. (1993). A wingless-dependent polar coordinate system in Drosophila imaginal discs. Science 259, 484–489. doi:10.1126/science.8424170
Couso, J. P., Bishop, S. A., Martinez Arias, A., and MArtinez AriAs, A. (1994). The wingless signalling pathway and the patterning of the wing margin in Drosophila. Development 120 (3), 621–636. doi:10.1242/dev.120.3.621
Deacon, P., Concodora, C., Chung, E., and Park, J. (2019). β-catenin regulates the formation of multiple nephron segments in the mouse kidney. Sci. Rep. 9 (1), 15915. doi:10.1038/s41598-019-52255-w
Denholm, B., Brown, S., Ray, R. P., Ruiz-Gomez, M., Skaer, H., and Hombria, J. C. (2005). crossveinless-c is a RhoGAP required for actin reorganisation during morphogenesis. Development 132 (10), 2389–2400. doi:10.1242/dev.01829
Diaz-Benjumea, F. J., Cohen, B., and Cohen, S. M. (1994). Cell interaction between compartments establishes the proximal-distal axis of Drosophila legs. Nature 372 (6502), 175–179. doi:10.1038/372175a0
Döring, F., Wischmeyer, E., Kühnlein, R., Jäckle, H., and Karschin, A. (2002). Inwardly rectifying K+ (Kir) channels in Drosophila. A crucial role of cellular milieu factors Kir channel function. J. Biol. Chem. 277 (28), 25554–25561. doi:10.1074/jbc.M202385200
Dow, J. A., Maddrell, S. H., Gortz, A., Skaer, N. J., Brogan, S., and Kaiser, K. (1994). The malpighian tubules of Drosophila melanogaster: A novel phenotype for studies of fluid secretion and its control. J. Exp. Biol. 197, 421–428. doi:10.1242/jeb.197.1.421
Edgar, B. A., and O’farrell, P. H. (1990). The three postblastoderm cell cycles of Drosophila embryogenesis are regulated in G2 by string. Cell 62 (3), 469–480. doi:10.1016/0092-8674(90)90012-4
Estella, C., Voutev, R., and Mann, R. S. (2012). A dynamic network of morphogens and transcription factors patterns the fly leg. Curr. Top. Dev. Biol. 98, 173–198. doi:10.1016/B978-0-12-386499-4.00007-0
Evans, J. M., Allan, a. K., Davies, S. A., and Dow, J. A. (2005). Sulphonylurea sensitivity and enriched expression implicate inward rectifier K+ channels in Drosophila melanogaster renal function. J. Exp. Biol. 208 (19), 3771–3783. doi:10.1242/jeb.01829
Fang, H. Y., Martinez-Arias, A., and De Navascués, J. (2016). Autocrine and paracrine Wingless signalling in the Drosophila midgut by both continuous gradient and asynchronous bursts of wingless expression. F1000Res. 317 (317), 317. doi:10.12688/f1000research.8170.1
Ford, M., Harwalkar, K., Pacis, A., Maunsell, H., Wang, Y., Badescu, D., et al. (2021). Oviduct epithelial cells constitute two developmentally distinct lineages that are spatially separated along the distal-proximal axis. Cell Rep. 36 (10), 109677. doi:10.1016/j.celrep.2021.109677
Francis, N., and Kingston, R. (2001). Mechanisms of transcriptional memory. Nat. Rev. Mol. Cell Biol. 2 (6), 409–421. doi:10.1038/35073039
Giorgianni, M. W., and Mann, R. S. (2011). Establishment of medial fates along the proximo-distal axis of the Drosophila leg through direct activation of dachshund by Distalless. Dev. Cell 20 (4), 455–468. doi:10.1016/j.devcel.2011.03.017
González, F., Romani, S., Cubas, P., Modolell, J., and Campuzano, S. (1989). Molecular analysis of the asense gene, a member of the achaete-scute complex of Drosophila melanogaster, and its novel role in optic lobe development. EMBO J. 8 (12), 3553–3562. doi:10.1002/j.1460-2075.1989.tb08527.x
Grueber, W., Jan, L., and Jan, Y. (2003). Different levels of the homeodomain protein cut regulate distinct dendrite branching patterns of Drosophila multidendritic neurons. Cell 112 (6), 805–818. doi:10.1016/s0092-8674(03)00160-0
Hatton-Ellis, E., Ainsworth, C., Sushama, Y., Wan, S., Vijayraghavan, K., and Skaer, H. (2007). Genetic regulation of patterned tubular branching in Drosophila. Proc. Natl. Acad. Sci. U. S. A. 104 (1), 169–174. doi:10.1073/pnas.0606933104
Hoch, M., Broadie, K., Jackle, H., and Skaer, H. (1994). Sequential fates in a single cell are established by the neurogenic cascade in the Malpighian tubules of Drosophila. Development 120 (12), 3439–3450. doi:10.1242/dev.120.12.3439
Jack, J., and Delotto, Y. (1995). Structure and regulation of a complex locus: The cut gene of Drosophila. Genetics 139 (4), 1689–1700. doi:10.1093/genetics/139.4.1689
Junion, G., Spivakov, M., Girardot, C., Braun, M., Gustafson, E. H., Birney, E., et al. (2012). A transcription factor collective defines cardiac cell fate and reflects lineage history. Cell 148 (3), 473–486. doi:10.1016/j.cell.2012.01.030
Kerber, B., Fellert, S., and Hoch, M. (1998). Seven-up, the Drosophila homolog of the COUP-TF orphan receptors, controls cell proliferation in the insect kidney. Genes Dev. 12 (12), 1781–1786. doi:10.1101/gad.12.12.1781
Klein, T., and Arias, A. (1998). Different spatial and temporal interactions between Notch, wingless, and vestigial specify proximal and distal pattern elements of the wing in Drosophila. Dev. Biol. 194 (2), 196–212. doi:10.1006/dbio.1997.8829
Kurant, E., Pai, C., Sharf, R., Halachmi, N., Sun, Y., and Salzberg, A. (1998)., 125. Cambridge, England), 1037–1048. doi:10.1242/dev.125.6.1037Dorsotonals/homothorax, the Drosophila homologue of meis1, interacts with extradenticle in patterning of the embryonic PNSDevelopment6
Le, T., Liang, Z., Patel, H., Yu, M. H., Sivasubramaniam, G., Slovitt, M., et al. (2006). Genetics, 2255–2257.
Lecuit, T., and Cohen, S. M. (1997). Proximal-distal axis formation in the Drosophila leg. Nature 388 (6638), 139–145. doi:10.1038/40563
Lindstrom, N. O., Lawrence, M. L., Burn, S. F., Johansson, J. A., Bakker, E. R., Ridgway, R. A., et al. (2015). Integrated beta-catenin, BMP, PTEN, and Notch signalling patterns the nephron. Elife 3, e04000. doi:10.7554/eLife.04000
Martinez Arias, A. (2003). Wnts as morphogens? The view from the wing of Drosophila. Nat. Rev. Mol. Cell Biol. 4 (4), 321–325. doi:10.1038/nrm1078
Maurange, C., and Paro, R. (2002). A cellular memory module conveys epigenetic inheritance of hedgehog expression during Drosophila wing imaginal disc development. Genes Dev. 16 (20), 2672–2683. doi:10.1101/gad.242702
Meinhardt, H. (1983a). A boundary model for pattern formation in vertebrate limbs. Development 76, 115–137. doi:10.1242/dev.76.1.115
Meinhardt, H. (1983b). Cell determination boundaries as organizing regions for secondary embryonic fields. Dev. Biol. 96 (2), 375–385. doi:10.1016/0012-1606(83)90175-6
Mucenski, M., Wert, S., Nation, J., Loudy, D., Huelsken, J., Birchmeier, W., et al. (2003). beta-Catenin is required for specification of proximal/distal cell fate during lung morphogenesis. J. Biol. Chem. 278 (41), 40231–40238. doi:10.1074/jbc.M305892200
Ng, M., Diaz-Benjumea, F., Vincent, J., Wu, J., and Cohen, S. (1996). Specification of the wing by localized expression of wingless protein. Nature 381 (6580), 316–318. doi:10.1038/381316a0
Nüsslein-Volhard, C., Wieschaus, E., and Kluding, H. (1984). Mutations affecting the pattern of the larval cuticle inDrosophila melanogaster : I. Zygotic loci on the second chromosome. Wilehm. Roux. Arch. Dev. Biol. 193 (5), 267–282. doi:10.1007/BF00848156
O'donnell, M. J., and Maddrell, S. H. (1995). Fluid reabsorption and ion transport by the lower Malpighian tubules of adult female Drosophila. J. Exp. Biol. 198 (8), 1647–1653. doi:10.1242/jeb.198.8.1647
Ogiyama, Y., Schuettengruber, B., Papadopoulos, G. L., Chang, J. M., and Cavalli, G. (2018). Polycomb-dependent chromatin looping contributes to gene silencing during Drosophila development. Mol. Cell 71 (1), 73–88. e75. doi:10.1016/j.molcel.2018.05.032
Pai, L. M., Orsulic, S., Bejsovec, A., and Peifer, M. (1997). Negative regulation of armadillo, a wingless effector in Drosophila. Development 124 (11), 2255–2266. doi:10.1242/dev.124.11.2255
Pérez, L., Barrio, L., Cano, D., Fiuza, U., Muzzopappa, M., and Milán, M. (2011)., 138. Cambridge, England), 3125–3134. doi:10.1242/dev.065599Enhancer-PRE communication contributes to the expansion of gene expression domains in proliferating primordiaDevelopment15
Port, F., Chen, H. M., Lee, T., and Bullock, S. L. (2014). Optimized CRISPR/Cas tools for efficient germline and somatic genome engineering in Drosophila. Proc. Natl. Acad. Sci. U. S. A. 111 (29), E2967–E2976. doi:10.1073/pnas.1405500111
Reggiani, L., Raciti, D., Airik, R., Kispert, A., and Brändli, A. (2007). The prepattern transcription factor Irx3 directs nephron segment identity. Genes Dev. 21 (18), 2358–2370. doi:10.1101/gad.450707
Ruiz-Losada, M., Blom-Dahl, D., Córdoba, S., and Estella, C. (2018). Specification and patterning of Drosophila appendages. J. Dev. Biol. 6 (3), E17. doi:10.3390/jdb6030017
Saxena, A., Denholm, B., Bunt, S., Bischoff, M., Vijayraghavan, K., and Skaer, H. (2014). Epidermal growth factor signalling controls myosin II planar polarity to orchestrate convergent extension movements during Drosophila tubulogenesis. PLoS Biol. 12 (12), e1002013. doi:10.1371/journal.pbio.1002013
Schneider, J., Arraf, a. A., Grinstein, M., Yelin, R., and Schultheiss, T. M. (2015). Wnt signaling orients the proximal-distal axis of chick kidney nephrons. Development 142 (15), 2686–2695. doi:10.1242/dev.123968
Skaer, H., and Martinez Arias, A. (1992). The wingless product is required for cell proliferation in the Malpighian tubule anlage of Drosophila melanogaster. Development 116, 745–754. doi:10.1242/dev.116.3.745
Skaer, H. (1993). The alimentary canal. M. Bate, and A. Martinez-Arias and editors. (eds): Cold spring harbor (New York): CSH Press, 941–1012.
Sozen, M. A., Armstrong, J. D., Yang, M., Kaiser, K., and Dow, J. A. (1997). Functional domains are specified to single-cell resolution in a Drosophila epithelium. Proc. Natl. Acad. Sci. U. S. A. 94 (10), 5207–5212. doi:10.1073/pnas.94.10.5207
Sudarsan, V., Pasalodos-Sanchez, S., Wan, S., Gampel, A., and Skaer, H. (2002). A genetic hierarchy establishes mitogenic signalling and mitotic competence in the renal tubules of Drosophila. Development 129 (4), 935–944. doi:10.1242/dev.129.4.935
Swarup, S., and Verheyen, E. M. (2012). Cold spring harb perspect Biology. Cold Spring Harbour, New York: CSH.
Tabata, T., Schwartz, C., Gustavson, E., Ali, Z., and Kornberg, T. (1995)., 121. Cambridge, England), 3359–3369. doi:10.1242/dev.121.10.3359Creating a Drosophila wing de novo, the role of engrailed, and the compartment border hypothesisDevelopment10
Takashima, S., Mkrtchyan, M., Younossi-Hartenstein, A., Merriam, J., and Hartenstein, V. (2008). The behaviour of Drosophila adult hindgut stem cells is controlled by Wnt and Hh signalling. Nature 454 (7204), 651–655. doi:10.1038/nature07156
Teleman, A., and Cohen, S. (2000). Dpp gradient formation in the Drosophila wing imaginal disc. Cell 103 (6), 971–980. doi:10.1016/s0092-8674(00)00199-9
Tian, A., Benchabane, H., Wang, Z., and Ahmed, Y. (2016). Regulation of stem cell proliferation and cell fate specification by wingless/wnt signaling gradients enriched at adult intestinal compartment boundaries. PLoS Genet. 12 (2), e1005822. doi:10.1371/journal.pgen.1005822
Tian, A., Duwadi, D., Benchabane, H., and Ahmed, Y. (2019). Essential long-range action of Wingless/Wnt in adult intestinal compartmentalization. PLoS Genet. 15 (6), e1008111. doi:10.1371/journal.pgen.1008111
Van De Wetering, M., Cavallo, R., Dooijes, D., Van Beest, M., Van Es, J., Loureiro, J., et al. (1997). Armadillo coactivates transcription driven by the product of the Drosophila segment polarity gene dTCF. Cell 88 (6), 789–799. doi:10.1016/s0092-8674(00)81925-x
Wan, S., Cato, a. M., and Skaer, H. (2000). Multiple signalling pathways establish cell fate and cell number in Drosophila malpighian tubules. Dev. Biol. 217 (1), 153–165. doi:10.1006/dbio.1999.9499
Wang, K., Helms, J., and Chang, H. (2009). Regeneration, repair and remembering identity: The three rs of Hox gene expression. Trends Cell Biol. 19 (6), 268–275. doi:10.1016/j.tcb.2009.03.007
Wartlick, O., Mumcu, P., Kicheva, A., Bittig, T., Seum, C., Julicher, F., et al. (2011). Dynamics of Dpp signaling and proliferation control. Science 331 (6021), 1154–1159. doi:10.1126/science.1200037
Weavers, H., and Skaer, H. (2013). Tip cells act as dynamic cellular anchors in the morphogenesis of looped renal tubules in Drosophila. Dev. Cell 27 (3), 331–344. doi:10.1016/j.devcel.2013.09.020
Wessing, A., and Eichelberg, D. (1978). Malpighian tubules, rectal papillae and excretion. Genet. Biol. Drosophila 2c, 1–42.
Williams, J., Paddock, S., and Carroll, S. (1993). Pattern formation in a secondary field: A hierarchy of regulatory genes subdivides the developing Drosophila wing disc into discrete subregions. Dev. Camb. Engl. 117 (2), 571–584. doi:10.1242/dev.117.2.571
Wingert, R., Selleck, R., Yu, J., Song, H., Chen, Z., Song, A., et al. (2007). The cdx genes and retinoic acid control the positioning and segmentation of the zebrafish pronephros. PLoS Genet. 3 (10), 1922–1938. doi:10.1371/journal.pgen.0030189
Wu, Y., Baum, M., Huang, C. L., and Rodan, a. R. (2015). Two inwardly rectifying potassium channels, Irk1 and Irk2, play redundant roles in Drosophila renal tubule function. Am. J. Physiol. Regul. Integr. Comp. Physiol. 309 (7), R747–R756. doi:10.1152/ajpregu.00148.2015
Xu, K., Liu, X., Wang, Y., Wong, C., and Song, Y. (2018). Temporospatial induction of homeodomain gene cut dictates natural lineage reprogramming. eLife 7, e33934. doi:10.7554/eLife.33934
Yoney, A., Etoc, F., Ruzo, A., Carroll, T., Metzger, J., Martyn, I., et al. (2018). WNT signaling memory is required for ACTIVIN to function as a morphogen in human gastruloids. eLife 7, e38279. doi:10.7554/eLife.38279
Zecca, M., Basler, K., and Struhl, G. (1996). Direct and long-range action of a wingless morphogen gradient. Cell 87 (5), 833–844. doi:10.1016/s0092-8674(00)81991-1
Keywords: proximo-distal patterning, Wnt/Wingless, epithelial tubule, tubulogenesis, Dachshund, Homothorax, patterning
Citation: Beaven R and Denholm B (2022) Early patterning followed by tissue growth establishes distal identity in Drosophila Malpighian tubules. Front. Cell Dev. Biol. 10:947376. doi: 10.3389/fcell.2022.947376
Received: 18 May 2022; Accepted: 28 July 2022;
Published: 19 August 2022.
Edited by:
Carlos Estella, Centre for Molecular Biology Severo Ochoa (CSIC), SpainReviewed by:
Brooke Elizabeth Chambers, University of Notre Dame, United StatesCopyright © 2022 Beaven and Denholm. This is an open-access article distributed under the terms of the Creative Commons Attribution License (CC BY). The use, distribution or reproduction in other forums is permitted, provided the original author(s) and the copyright owner(s) are credited and that the original publication in this journal is cited, in accordance with accepted academic practice. No use, distribution or reproduction is permitted which does not comply with these terms.
*Correspondence: Barry Denholm, QmFycnkuRGVuaG9sbUBlZC5hYy51aw==
Disclaimer: All claims expressed in this article are solely those of the authors and do not necessarily represent those of their affiliated organizations, or those of the publisher, the editors and the reviewers. Any product that may be evaluated in this article or claim that may be made by its manufacturer is not guaranteed or endorsed by the publisher.
Research integrity at Frontiers
Learn more about the work of our research integrity team to safeguard the quality of each article we publish.