- 1Jilin Provincial Key Laboratory on Molecular and Chemical Genetic, Second Hospital of Jilin University, Changchun, China
- 2Engineering Lab on Screening of Antidepressant Drugs, Jilin Province Development and Reform Commission, Changchun, China
- 3Central Laboratory, The Second Hospital of Jilin University, Jilin University, Changchun, China
- 4Department of Pediatrics, The First Hospital of Jilin University, Changchun, China
Depression has an alarmingly high prevalence worldwide. A growing body of evidence indicates that environmental factors significantly affect the neural development and function of the central nervous system and then induce psychiatric disorders. Early life stress (ELS) affects brain development and has been identified as a major cause of depression. It could promote susceptibility to stress in adulthood. Recent studies have found that ELS induces epigenetic changes that subsequently affect transcriptional rates of differentially expressed genes. The epigenetic modifications involved in ELS include histone modifications, DNA methylation, and non-coding RNA. Understanding of these genetic modifications may identify mechanisms that may lead to new interventions for the treatment of depression. Many reports indicate that different types of ELS induce epigenetic modifications of genes involved in the neurotransmitter systems, such as the dopaminergic system, the serotonergic system, the gamma-aminobutyric acid (GABA)-ergic system, and the glutamatergic system, which further regulate gene expression and ultimately induce depression-like behaviors. In this article, we review the effects of epigenetic modifications on the neurotransmitter systems in depression-like outcomes produced by different types of ELS in recent years, aiming to provide new therapeutic targets for patients who suffer from depression.
Introduction
Early life stress (ELS) includes adverse gestational (maternal stress and maternal infection) and adverse childhood experiences (parental loss, emotional abuse, and physical abuse) (Oh et al., 2013; Provenzi et al., 2018). Early life is a critical time for axonal growth and synaptic growth, and in this period, the interaction with mothers is crucial. Studies have pointed out that offspring form special and strong emotional bonds with their caregivers in early life. When this attachment relationship is destroyed, it affects the offspring’s emotional, cognitive, and behavioral responsiveness (Sroufe, 2005; Feifel et al., 2017). When exposed to a long-term maternal separation, rat pups become increasingly marked with slower developing changes in behavioral reactivity, unresponsiveness, reduced movement, reduced alertness, food neglect, and indifference to new stimuli (Hofer, 1970, Hofer, 1973; Hofer, 1994). As Harlow indicated, one of the main roles of the mother is to shape the behavioral responsiveness of young monkeys and to guide the infant in age-appropriate social behavior with peers and adults (Harlow et al., 1971; Hall and Perona, 2012). When rhesus monkeys were exposed to maternal deprivation and then grew up to become mothers, they were extremely abusive and neglectful of their infants (Seay et al., 1964; Arling and Harlow, 1967). This pattern of abnormal behavior may be passed on from generation to generation, as maternal deprivation can lead to abnormal behaviors in offspring, resulting in abnormal behaviors in the next generation of mothers (Hall and Perona, 2012). It has been found that ELS increases susceptibility to psychiatric disorders in adulthood, including depression, anxiety, schizophrenia, autism, and attention deficit hyperactivity disorder (Gilbert et al., 2009; van Velzen and Toth, 2010; Deslauriers et al., 2013; Oh et al., 2013; Shepard and Nugent, 2020; Kronman et al., 2021). Depressed patients who suffered childhood trauma have faster brain aging and have a longer duration of illness than those who have not suffered childhood trauma (Klein et al., 2009; Miniati et al., 2010). In addition, the effect of ELS on depression-like behaviors is related to the duration of stress exposure. It has been reported that long-term maternal separation increases despair-like behaviors, while short-term maternal separation produces better adaptation to stress in adulthood (Köhler et al., 2019). The hypothalamic-pituitary-adrenal (HPA) axis is the primary stress response system. Cortisol mediates numerous tissue-specific effects through the glucocorticoid receptor (Young et al., 2003; Farrell et al., 2018). It has been found that early-life adversity leads to a lifelong increase in glucocorticoid secretion and disruption of the homeostasis of HPA axis activity, and a disturbance of the HPA axis will lead to vulnerability to depression (Heim and Binder, 2012). Animal studies indicate that ELS results in a site-specific upregulation of multiple glucocorticoid receptor transcripts, a net increase in receptor mRNA, and enhanced transcriptional regulation of target genes [for example, increased glucocorticoid receptor occupancy at the intronic glucocorticoid response element (GRE) of FK506 binding protein 51 (Fkbp5)] (Bockmühl et al., 2015). In addition, exposure to ELS interferes with the function and innervation of serotonergic and dopaminergic neurons in the prefrontal-limbic system neural circuit (Gos et al., 2006; Jezierski et al., 2007; Kunzler et al., 2015). Multiple sources of evidence support the regulatory roles of the serotonergic system, the dopaminergic system and other neurotransmitter systems in the pathogenesis of depression (Gershon et al., 2007; van der Doelen et al., 2015; Wang et al., 2017; Marrocco et al., 2019; Alameda et al., 2022). Most of the current commonly used antidepressants, especially selective serotonin reuptake inhibitors (SSRIs), serotonin-norepinephrine reuptake inhibitors, and monoamine oxidase inhibitors, primarily target monoamine neurotransmitter function (Harmer et al., 2017). However, there is no drug aimed at depression-like outcomes produced by different types of ELS or reducing susceptibility to depression. Therefore, the mechanism of different types of ELS-induced depression-like behaviors and their interfering factors have received extensive attention in recent years.
Epigenetic modification is considered a promising pathway to counteract the onset of depression by modulating persistent changes in gene expression in response to ELS. Epigenetics refers to potentially heritable but environmentally modifiable changes in gene expression mediated by non-DNA-encoded mechanisms (Sun et al., 2013; Park et al., 2019). These modifications, including DNA methylation, histone modification, and non-coding RNA, may result in the following changes in genetic transcription, synaptic plasticity, and behavior (Tesone-Coelho et al., 2015; Palmisano and Pandey, 2017; Alameda et al., 2022). By nature, epigenetic mechanisms are dynamic and reversible, and they can be used as a new intervention strategy to treat psychiatric disorders (Lesch, 2011). Stress and depression are primarily associated with epigenetic alterations in genes involved in regulating resilience and/or susceptibility to stress, including stress response-related genes (crf) and genes involved in neurotransmission (SLC6A4) (Park et al., 2019). In recent years, many studies have focused on the role of epigenetics on depression-like outcomes produced by different types of ELS (Ptak and Petronis, 2010; Alameda et al., 2022). Among various epigenetic modifications, cytosine-phosphate-guanine (CpG) methylation has the longest duration (Guo et al., 2011). In both animal and clinical studies, an unfavorable maternal environment causes epigenetic changes in neurons that are often persistent (Oberlander et al., 2008; McGowan et al., 2009; Oh et al., 2013). Some ELS, such as maternal separation, can induce long-term epigenetic changes in gene expression and even persist into adulthood (Bhansali et al., 2007). Maternal effects indirectly regulate gene expression by regulating splicing selectivity, promoter usage, and microRNAs (miRNAs) expression, rather than regulating transcription from proximal promoters (Oh et al., 2013). Under the action of maternal effect, neurotransmitter receptors (corticotropin-releasing factor receptor type 1 (CRF1), dopamine D3 receptor (DRD3), adenosine A2 receptor (ADORA2A), acetylcholine alpha 4 subunit (CHRNA4), gamma-aminobutyric acid A receptor gamma 3 (GABRG3), and GABAB receptor subunit 2 (GABBR2)) are methylated to varying degrees (Oh et al., 2013).
In this article, we aimed to review recent studies on the epigenetic mechanisms of different types of ELS-induced depression-like behavior, especially focusing on the neurotransmitter systems such as the dopaminergic system, the serotonergic system, and the glutamatergic system. We hoped to find therapeutic targets for people who have experienced childhood stress and trauma.
Serotonergic System
Periodic maternal separation during pre-weaning leads to altered serotonin concentration and serotonergic function in selective brain regions [nucleus accumbens (NAc), hippocampus, and raphe] (Matthews et al., 2001; van Riel et al., 2004; Jahng et al., 2010). Serotonin (5-HT) level can be regulated by multiple factors, such as synthesis, release, and reuptake (Calabrese et al., 2013). At present, 14 different 5-HT receptor subtypes have been identified, and they belong to seven families (termed 5-HT1 through 5-HT7). Among them, 5-HT1 was divided into six subtypes (termed 5-HT1A through 5HT1F), and 5-HT2 was divided into three subtypes (termed 5-HT2A through 5-HT2C) (Fink and Göthert, 2007; Björk et al., 2010). Many studies indicate that the serotonergic system is involved in the pathogenesis and therapy of depression (Hoyer et al., 2002; Stockmeier, 2003). Experiencing social isolation rearing (single cage feeding on post-natal day 21) results in a decrease in 5-HT and its metabolite 5-hydroxyindole-acetic acid (5-HIAA) in the prefrontal cortex of adult rats (Möller et al., 2013).
5-HT1AR
The serotonin type 1A receptor (5-HT1AR) is considered to be an important specific therapeutic target for depression. 5-HT1AR and serotonin type 2C receptor (5-HT2CR) modulate reward behavior by modulating dopamine release in the NAc (Leventopoulos et al., 2009). Repeated early deprivation leads to reduce reward motivation and a decrease in 5-HT1AR binding in the anterior cingulate cortex (ACC), CA1, and dorsal raphe nucleus (DRN) in adulthood (Leventopoulos et al., 2009). Epigenetic mechanisms of anxiety- or depression-like behavioral changes are associated with maternal 5-HT1AR deficiency. In the offspring, the immobility behavior of the second-filial generation of male mice was particularly reduced in the forced swimming test. In addition, the behavioral changes in the first- and second-filial generation of female mice were in opposite directions. These suggest that there may be sex differences in epigenetic mechanisms resulting from maternal 5-HT1AR deficiency (Mitchell et al., 2016). Immune system dysregulation in 5-HT1AR+/− heterozygote and first-filial generation females was associated with immune activation in their offspring and the transmission of somatic anxiety trait. Non-genetic traits of complex psychiatric-like phenotypes were independently transmitted across multiple generations through parallel non-genetic mechanisms. The features of anxiety and hypoactivity were transmitted through somatic mechanisms, while the altered stress-reactivity was transmitted through gamete mechanisms (Mitchell et al., 2016). In vitro experiments further found that differentially methylated regions existed in the first-, second-, and third-filial generations of neurons, and 95% of the methylation changes occurred in CpG dinucleotides (among them, hypomethylation accounts for 55% and hypermethylation accounts for 45%), and 87% of differentially methylated sites were unidirectional (Mitchell et al., 2016).
In clinical studies, ELS induced hypermethylation of 5-HT1AR, whereas patients with high 5-HT1AR methylation from −340 to −149 bp upstream of the transcription start site (TSS) showed a decrease in 5-HT1AR expression (Xu et al., 2022), which further reduced 5-HT1AR availability (Xu et al., 2011; David and Gardier, 2016). These are consistent with the results of animal studies.
5-HT2R
Maternal separation induces the development of adult depression and increases 5-HT2CR pre-mRNA editing significantly (Bhansali et al., 2007). Fluoxetine administration in adolescence reduced depression-like behaviors and suppressed the increase in the phenotype of 5-HT2CR pre-mRNA editing; however, fluoxetine administration in adult mice did not affect either depression-like behaviors or the 5-HT2CR pre-mRNA editing phenotype (Bhansali et al., 2007). Mice exposed to ELS showed significantly increased expression of mRNA and protein-encoding the Gαq subunit of G-protein that couples to 5-HT2A/2CRs. The aforementioned results suggest that compensatory changes in Gαq expression occur in mice with persistent changes in 5-HT2CR pre-mRNA editing (Bhansali et al., 2007).
5-HT2AR genotype was associated with the methylation of 5-HT2AR at CpG-1420 and CpG-1224 in a sample of preschoolers with ELS (Parade et al., 2017). Contextual stress was positively correlated with the methylation of A homozygotes at the CpG-1420 site and negatively correlated with the methylation of G homozygotes at the CpG-1420 site. Depression-like behaviors were negatively correlated with methylation of CpG-1420 and positively correlated with methylation of CpG-1224. Collectively, environmental factors and DNA variation influence the epigenetic process of 5-HT2AR (Parade et al., 2017).
5-HT3R
Serotonin type 3A receptor (5-HT3AR) is required for exercise-induced neurogenesis and antidepressant effects, and it modulates cortical interneuron migration and dendritic morphology in pyramidal neurons (Murthy et al., 2014; Kondo et al., 2015; Perroud et al., 2016). In clinical studies, early life trauma interacts with 5-HT3AR and brain-derived neurotrophic factor (Bdnf) genes to exacerbate the risk for depression (Gatt et al., 2010a; Gatt et al., 2010b). The study found that emotional neglect in children was inversely correlated with methylation levels of CpG1_I (located in the GRE element upstream of 5-HT3AR). In addition, a functional 5HT3AR single nucleotide polymorphism (SNP) (rs1062613) selectively affects the methylation of a CpG located at 1 bp of the SNP (Perroud et al., 2016). However, the relationship between depression-like outcomes produced by different types of ELS and the epigenetic modification of 5HT3AR and its mechanism still needs to be explored.
SERT
The serotonin transporter is encoded by a single gene, SERT (also known as 5-HTT or SLC6A4) (Caspi et al., 2003), located in the presynaptic 5-HT nerve terminal, axons, and cell bodies (Blakely et al., 1998). In the brain, SERT modulates the intensity and duration of serotonergic neurotransmission (Gaspar et al., 2003). Clinical study results show that methylation of the SLC6A4 promoter is associated with increased susceptibility to depression (Olsson et al., 2010), and higher SLC6A4 promoter methylation is significantly associated with childhood adversity (Kang et al., 2013). Methylation of SLC6A4 was positively associated with depression severity in women but not with depression severity and age of onset (Sanwald et al., 2021).
There is a common polymorphic region in the SERT gene, the 5-HT transporter-linked polymorphic region (5-HTTLPR), and carriers of the 5-HTTLPR short (S) variant have an increased susceptibility to depression under adversity (Uher and McGuffin, 2010). Interestingly, one study found that sex determined neonatal SLC6A4 methylation independent of ELS and 5-HTTLPR genotype, and the methylation was higher in females than males (Dukal et al., 2015). The short allele of the 5-HTTLPR polymorphism and maternal prenatal stress/child maltreatment showed an additive relationship to the reduction of SLC6A4 mRNA expression in vivo (Wankerl et al., 2014). Deletion of the SERT led to depression-like behavior, which may be associated with reduced neuronal plasticity (Lira et al., 2003). Female offspring of mice after maternal immune activation have enhanced anhedonia behavior, as manifested by a reduced preference for sucrose (Reisinger et al., 2016). In addition, in the hippocampus, the binding of acH3 and acH4 histones to the SERT promoter was increased nearly twofold, suggesting that SERT is a specific target for the regulation of epigenetic changes induced by maternal immune activation (Reisinger et al., 2016).
In the prefrontal cortex, Bdnf mRNA levels were more sensitive to the changes in SERT. SERT deficiency significantly reduced Bdnf mRNA expression in rat prefrontal cortex during the first week of life, whereas changes in the ventral hippocampus were not seen until the second week (Pezawas et al., 2008; Calabrese et al., 2013). This may be related to the degree of promoter methylation of Bdnf exon IV in SERT−/− rats. Further study found that the level of DNA methyltransferase, DNA (cytosine-5)-methyltransferase 1 (Dnmt1) was increased in SERT−/− rats, while the level of demethylase growth arrest and DNA-damage-inducible beta (Gadd45β) was decreased. In addition, the induction of depression- or anxiety-like behaviors by SERT deletion is closely associated with increased DNA methylation of Bdnf and decreased availability of transcription factors such as cAMP-response element-binding protein (Creb), neuronal PAS domain protein 4 (Npas4), and calcium-responsive transcription factor (Carf) (Molteni et al., 2010; Calabrese et al., 2013). Furthermore, the study found that serum SERT mRNA levels were reduced in individuals exposed to maternal prenatal stress or child maltreatment, but this phenomenon was not observed in stressed adults, suggesting that this change occurs during a sensitive period of early development (Wankerl et al., 2014). In addition, depressive symptoms in second-trimester women were positively associated with methylation within subregions of SERT CpG island (Devlin et al., 2010), and the methylation status of the gene promoter was closely related to the volume of the hippocampus, especially in the dentate gyrus, CA2, and CA3 of in the hippocampus (Booij et al., 2015).
CRF
Changes in 5-HT content regulate crf mRNA level in the paraventricular nucleus of the hypothalamus (PVN) (Jørgensen et al., 2002), and SSRIs administration reverses stress-induced Crf transcription elevation (Pan et al., 2013). CRF is a 41 amino acid peptide. Hyperactivity of the CRF neuronal system appears to be a pathological hallmark of depression, and CRF is a key mediator of the hypothalamic-pituitary-adrenal (HPA) axis (Hasan and Hasan, 2011). In response to stress, CRF initiates a series of physiological processes and ultimately releases glucocorticoids from the adrenal cortex (Smith and Vale, 2006), and HPA axis hyperactivity returns to normal after antidepressant treatment (Arborelius et al., 1999). It has been found that maternal separation alters CRF expression in brain regions such as the central amygdala (CeA), PVN, and bed nucleus of the stria terminalis (BNST) (Chen et al., 2012). In the female mice which were exposed to impoverished housing, increased risk-taking behavior during a reward-related task (predator-odor risk-taking, PORT) was associated with increased CRF receptor 1 (Crfr1) gene expression in the medial prefrontal cortex. Further studies revealed that the levels of the protein marker histone H3 at arginine 2 (H3R2me2s) in the proximal promoter region of the Crfr1 gene were elevated, whereas these changes were not observed in male mice (Viola et al., 2019). The deposition of histone-modified H3R2me2s results in a stable euchromatin structure that is essentially associated with transcriptional activation (Migliori et al., 2012), which further confirms the phenomenon of elevated Crfr1 mRNA levels after PORT testing (Schreiber et al., 2017; Viola et al., 2019). In addition, maternal separation and 5-HT genotypes affect the epigenetic modification of the urocortin 1 (Ucn1) gene (a member of the CRF peptide family). Studies have found that maternal separation induces methylation at CpG-156 and CpG-49 of the Ucn1 promoter region in the Edinger–Westphal nuclei, and 5-HTT deficiency induces DNA methylation at CpG-171 in the Ucn1 promoter region; however, DNA methylation at other CpG sites was not affected (van der Doelen et al., 2017). The interaction of maternal separation and 5-HTT genotype affects DNA methylation of the Crf gene promoter in adult rat CeA, while DNA methylation at specific sites in the Crf promoter was related to Crf mRNA levels in CeA, and CpG 36 negatively correlated with CeA Crf mRNA levels (van der Doelen et al., 2015).
TPH2
The tryptophan hydroxylase 2 (TPH2) gene encodes a rate-limiting enzyme involved in the 5-HT synthesis (Tesoro-Cruz et al., 2021). Clinical studies have shown that the association of TPH2 methylation changes with ELS only manifests at specific CpG sites. Men with depression who experienced ELS had hypermethylation at the TPH2-5-203 CpG site, whereas depressed women who experienced ELS had hypermethylation at the TPH2-10-60 CpG site. These methylations alter the transcription of TPH2, further disrupting 5-HT levels, thereby counteracting the effects of antidepressants (Shen et al., 2020).
Dopaminergic System
Dopaminergic system dysfunction is a pathological hallmark of many neuropsychiatric diseases, such as depression, anxiety, and drug addiction. Stress induces changes in dopamine release or metabolism in the dopaminergic system, especially in the mesolimbic dopaminergic system. The release of dopamine typically varies according to the intensity, duration, and avoidance ability of stress (Baik, 2020). The reduction of dopaminergic function and dopaminergic activity may lead to depression-like behaviors in rodents (Willner et al., 1992; Di Chiara et al., 1999; Jahng et al., 2010). Dopamine receptors are divided into two categories, the dopamine 1 family [divided into dopamine D1 receptor (DRD1) and DRD5 subtypes] and the dopamine 2 family (divided into DRD2, DRD3, and DRD4 subtypes) (Dunlop and Nemeroff, 2007).
DRD1
It has been reported that maternal separation combined with social isolation stress reduced Drd1a mRNA expression, while Drd2 mRNA expression did not change significantly (Sasagawa et al., 2017). In addition, stress increased methylation of the promoter of the Drd1a gene in mice NAc, and 29 of 31 CpG sites (CpG sites 1–5, 8–16, and 18–31) in the Drd1a gene were more frequently methylated than normal mice (Table 1) (Sasagawa et al., 2017). It has been found that maternal separation induces an increase in DNA methyltransferase expression in pups and adult rats NAc (Anier et al., 2014; Todkar et al., 2015). According to a recent study, demethylation of histone H3 on lysine 79 (H3K79me2) was a key regulator of transcriptional abnormalities in the adult NAc induced by maternal separation (Kronman et al., 2021). In addition, short-term ELS increased DRD1 expression in the hippocampus and decreased the expression of histone H3 acetylation and dopamine- and cAMP-regulated neuronal phosphoprotein (DARPP-32) but did not change the level of histone H4 acetylation. In contrast, long-term maternal separation upregulated DARPP-32 expression but did not alter DRD1 expression and histone H3/H4 acetylation (Köhler et al., 2019). Furthermore, it was confirmed that the short-term maternal separation induced a decrease in the expression of DARPP-32 was related to the decrease of acetylation of H3 in the promoter region but not to the acetylation of H4 (Köhler et al., 2019).
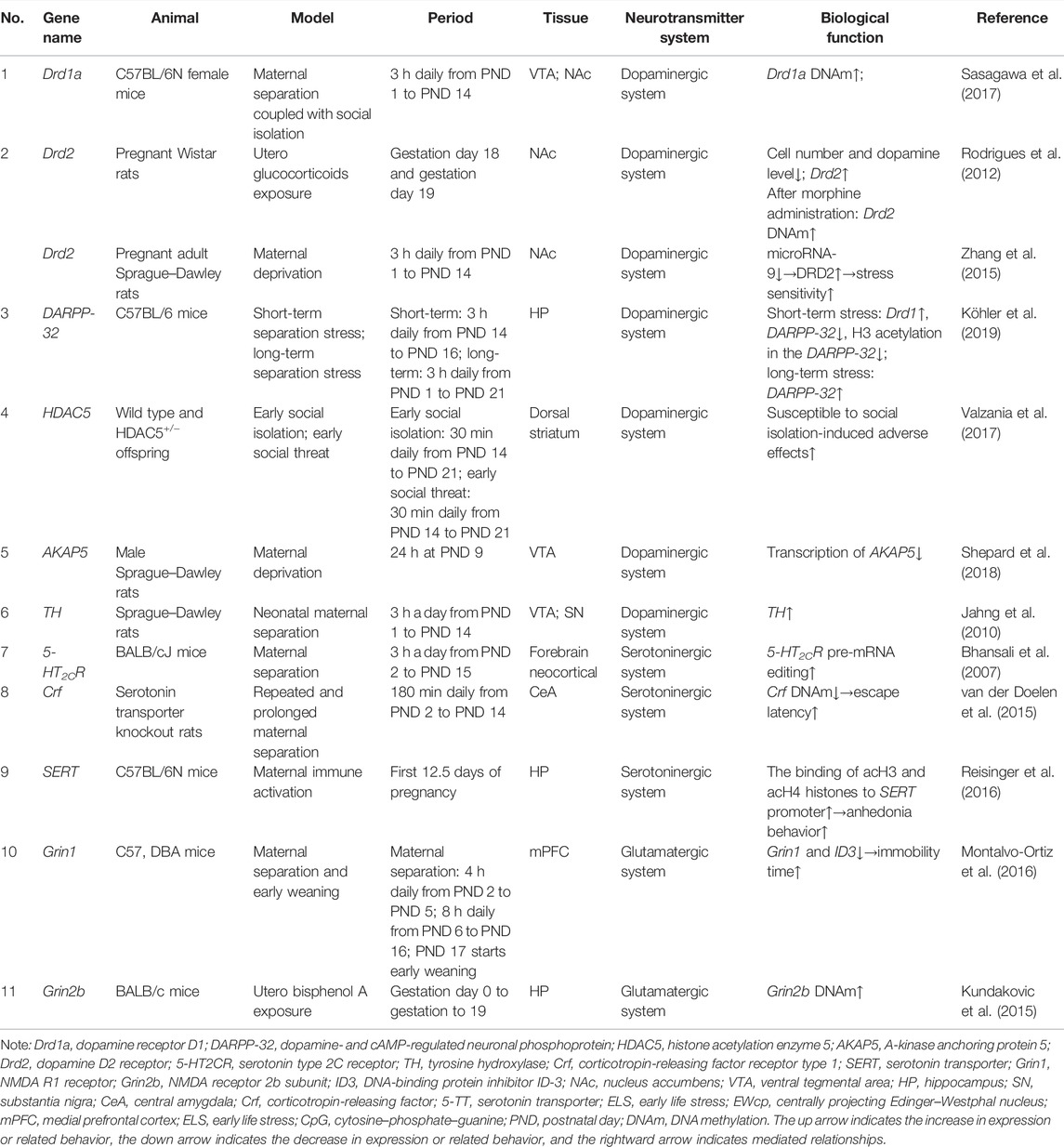
TABLE 1. Summary of different types of ELS-induced epigenetic changes in neurotransmitter system-related genes in animal models mentioned in this review.
DRD2
H3K79me2 demethylation and the enzymes that control this demethylation [disruptor of telomeric silencing 1-like (DOT1L) and lysine-specific demethylase 2B (KDM2B)] in D2-medium spiny neurons (MSNs) are critical for maternal separation-induced stress sensitivity (Kronman et al., 2021). In adult mice, knockout of the DOT1L or overexpression of the KDM2B in NAc D2-MSN neurons attenuated maternal separation-induced depression-like behavior, including increased social interaction, increased open field exploration, and decreased despair-like behaviors, whereas the same treatment in Nac D1-MSNs had no changes in depression-like behaviors (Kronman et al., 2021). Increased DOT1L after maternal separation is associated with H3K29me2 deposition at numerous genomic loci. Meanwhile, systemic administration of a small-molecule DOT1L inhibitor reversed maternal separation-induced behavioral deficits (Kronman et al., 2021). This provides strong evidence support for the treatment of maternal separation-induced depression.
One of the closely related and persistent histone modifications in ELS is a histone deacetylase (HDAC)-mediated histone acetylation (Levine et al., 2012; Valzania et al., 2017; Shepard et al., 2018). Histone acetylation, the most reported histone modification in neurological diseases, is dynamically regulated by two distinct types of enzymes, histone acetyltransferases (HATs) and HDACs (Marmorstein and Zhou, 2014; Alameda et al., 2022). HAT transferred acetyl groups to histone tails, leading to chromatin relaxation and subsequent increase in transcription rate; the latter increased chromatin-histone interactions by removing acetyl groups and ultimately decreased transcription rate (Shepard and Nugent, 2020). Studies have shown that HDAC inhibitors exert antidepressant-like effects and alleviate depression-like and addictive behaviors (Covington et al., 2009). Studies have found that spatial and associative memory functions are impaired in histone acetylation enzyme 5 (HDAC5) knockout mice and in early-life stressed mice (Agis-Balboa et al., 2013; Suri et al., 2014; Valzania et al., 2017). In addition, a heterozygous null mutation in HDAC5 increased the time of conditioned place preference in mice that suffered from social isolation in early life but not in socially threatened mice (Valzania et al., 2017). The dorsal striatum may be involved in mediating this effect (Valzania et al., 2017). In brief, individuals are more susceptible to social isolation-induced adverse effects when heterozygous null mutations in HDAC5 are present in the organism.
In another study, it was found that maternal deprivation-induced GABAergic neuroplasticity (not glutamatergic) and aberrant A-kinase anchoring protein (AKAP) signaling could be reversed by injection of HDAC inhibitors in the ventral tegmental area (VTA), and researchers proposed these effects may be related to dopamine neurons in the VTA (Authement et al., 2015; Shepard et al., 2018). Further studies found that maternal deprivation increased HDAC2 expression in VTA dopaminergic neurons and increased BDNF (a biological indicator closely related to the onset of depression) expression in VTA (Shepard et al., 2018). In addition, prenatal stress regulated gene expression in the hippocampus, such as decreased early growth response 1 (Egr1) and RAC-alpha serine/threonine-protein kinase (Akt1) mRNA expression, which were important for regulating cell proliferation and cell survival (Boulle et al., 2016). On the other hand, maternal deprivation also induced histone hypoacetylation in VTA. In addition, maternal deprivation increased the intrasynaptic AKAP150 level and decreased the protein kinase A (PKA)-RIIβ level, and these effects can be reversed by an HDAC inhibitor (Shepard et al., 2018). Maternal deprivation-induced AKAP150-anchored signaling changes may be closely linked with HDAC2-mediated epigenetic modifications, and these modifications prevent transcription of the AKAP5 gene (Shepard et al., 2018; Shepard et al., 2020). In recent research, the interaction of PKA and AKAP150 regulated the cellular excitability and intrinsic membrane properties of VTA dopaminergic neurons, and the disruption of the AKAP150-PKA complex increased VTA action potential generation in normal animals, and it decreased in maternal deprivation animals (Shepard et al., 2020). Therefore, by targeting AKAP150 and HDACs to reduce changes in histone acetylation, they can modulate not only neuronal excitability through synaptic transmission but also ion channel activity and postsynaptic ion channel transport (Shepard et al., 2020).
ELS enhances stress sensitivity in adulthood by reducing microRNA-9 expression, which, in turn, upregulates DRD2 expression in the NAc (Zhang et al., 2015). It has been found that chronic unpredictable stress increased Drd2 mRNA levels within the NAc and decreased microRNA-9 expression, while maternal deprivation synergistically enhanced the effects of chronic unpredictable stress on Drd2 and microRNA-9 expression. In vitro studies have shown that microRNA-9 directly targets the 3′ untranslated region of Drd2 mRNA and then inhibits DRD2 expression (Zhang et al., 2015).
Exposure to in utero glucocorticoids significantly decreased cell number and dopamine levels and significantly upregulated Drd2 mRNA levels in the NAc of adult rats, while repeated morphine administration downregulated the levels of DRD2 expression while increasing the DNA methylation of the Drd2 gene. Administration of therapeutic doses of levodopa restored a hypodopaminergic state, normalized DRD2 expression, and resisted morphine-induced methylation of the Drd2 promoter in the NAc of animals exposed to in utero glucocorticoids. In addition, levodopa treatment also promoted the dendritic and synaptic plasticity of the NAc (Rodrigues et al., 2012).
Other Dopamine-Related Genes
Intrasynaptic dopamine transmission is primarily regulated by dopamine transporter (DAT) uptake of released dopamine, and it is sensitive to changes in DAT density and its function (Wightman and Zimmerman, 1990). A lower density of DAT sites in mice NAc may be the reason why maternal separation animals are more susceptible to stress responses (Brake et al., 2004). In addition, in the same experiment, it was found that Drd3 mRNA levels were greatly reduced in the NAc of maternal stress model mice (Brake et al., 2004).
Tyrosine hydroxylase (TH) is regarded as a rate-limiting enzyme in catecholamine biosynthesis. It has been found that stress response is associated with TH activity in the central nervous system (Masserano and Weiner, 1983). A study found that restraint stress increased TH mRNA level and enhanced the rate-limiting enzyme in dopamine synthesis in the midbrain VTA and substantia nigra (SN). In addition, restraint stress-induced increase in TH mRNA expression was significantly attenuated in the VTA and SN of neonatal maternal separation-treated rats (Jahng et al., 2010). However, there are few studies on the mechanism of different types of ELS-induced TH expression changes, and further exploration is needed.
GABAergic System
There are three subtypes of GABA receptors: GABAA receptors, GABAB receptors, and GABAC receptors. GABAB receptors are metabotropic receptors, and GABAA receptors and GABAC receptors are ionotropic receptors (Bormann, 2000). GABA receptors play a pivotal role in the regulation of psychiatric disorders such as depression, epilepsy, and anxiety. Maternal deprivation-induced GABAergic neuronal plasticity and aberrant AKAP signaling could be reversed by the injection of HDAC inhibitors in the VTA (Authement et al., 2015). Maternal separation altered the levels of 24 miRNAs in the hippocampus. Among them, antidepressant treatment attenuated maternal separation-induced changes in the levels of miR-451, a miRNA that regulates many important genes, including GABAergic (GABAA receptor-associated protein) and cholinergic neurotransmission (muscarinic cholinergic receptors 5) (O’ Connor et al., 2013). In the ventral hippocampus of SERT−/− rats at the third week of life, SERT deficiency resulted in a significant decrease in γ2 subunit of GABAA receptor (GABAAγ2) and glutamic acid decarboxylase 1 (Gad67) mRNA levels, while vesicular-GABA transporter (Vgat) only showed a trend of decreasing but not statistically significant. On the other hand, in the prefrontal cortex, SERT deficiency only reduced GABAAγ2 mRNA levels (Calabrese et al., 2013). The effects of the prenatal environment on early childhood neurodevelopment are gender-mediated in both animal and human studies (Bale, 2011), in which maternal care and ELS in rats are associated with GABAergic synaptic function and GABAA receptor expression (Diorio and Meaney, 2007). In addition, GABAB receptor subunit 1 (Gabbr1) gene expression was reduced by 36% in the hippocampus of female rat pups exposed to prenatal stress (Van den Hove et al., 2013). In clinical studies, DNA methylation of Gabbr1 was positively associated with anxiety during pregnancy in male neonates (Vangeel et al., 2017). The glutamatergic and GABAergic system was critical for stress response and emotion regulation (Popoli et al., 2011). In the results of RNA sequencing, although the expression of the N-methyl-D-aspartic acid receptor (NMDA) receptor subunit genes NMDA R1 receptor (Grin1), Grin2a, GABAB receptor 2 (Gabbr2), and GABAARα1 (Gabra1) was reduced in CA3 after exposure to acute-swim stress, the expression levels of these genes did not change after acute-swim stress in mice exposed to maternal separation (Marrocco et al., 2017; Marrocco et al., 2019).
Glutamatergic System
In patients with depression, alterations in the glutamatergic system can lead to alterations in related excitatory neurotransmission, and it plays a vital role in the pathogenesis of neurological disorders (Lener et al., 2017; Alameda et al., 2022). It has been found different types of ELS change oxidative stress and redox balance, including elevated levels of the oxidative stress marker Nox2, which controls glutamate release in the prefrontal cortex (Schiavone et al., 2009; Sorce et al., 2010; Möller et al., 2013). In addition, glutamate can bind to ionotropic receptors [including NMDA receptors, α-amino-3-hydroxy-5-methyl-4-isoxazole-propionic acid receptor (AMPA) receptors, and kainate receptors] and metabotropic receptors (mGluRs) (Groc and Choquet, 2006; Lener et al., 2017).
NMDA
In clinical studies, ELS decreased NMDA receptor expression and increased glutamate-mediated excitotoxicity, which further induced neuronal loss and ultimately reduced NMDA receptor binding (Underwood et al., 2020). In animal studies, it has been found that exposure to maternal separation at 2–3 weeks postnatally altered synaptic plasticity in susceptible rats and inhibited the effect of antidepressants on CA1, accompanied by a lifetime upregulation of synaptic NMDA receptor 1 (NR1) levels in sensitive rats (Ryan et al., 2009). In the epigenome study of children, methylation at CpG sites in DNA-binding protein inhibitor ID-3 (ID3), tubulin polymerization promoting protein (TPPP), and Grin1 were inversely related to depression severity in maltreated children (Weder et al., 2014). Similarly, in maternal separation and early weaning (MSEW)–exposed mice, TPPP gene expression in the prefrontal cortex was inversely proportional to dwell time in the open arm and proportional to dwell time in the closed arm. The expression of Grin1 and ID3 genes in the medial prefrontal cortex were inversely proportional to the immobility time in the forced swimming test (Montalvo-Ortiz et al., 2016), and these were consistent with previous results.
Bisphenol A (BPA) exposure induced sex difference in methylation of the CREB-binding site (CpG1) and a site adjacent to CpG1 of the NMDA receptor 2b subunit (Grin2b) promoter, and BPA also induced hypermethylation of CpG3 in Grin2b promoter in males in the mice hippocampus (Kundakovic et al., 2015). Similarly, the effect of ELS on the methylation of the Grin2b gene has been demonstrated in clinical studies, and the Grin2b regulatory region has a higher degree of methylation in adults who have experienced childhood adversity, especially the changes at the CpG3 site in the Grin2b gene (Engdahl et al., 2021). These studies confirm that the methylation of the Grin2b gene is very sensitive to ELS, and these findings may increase the understanding of the impact of ELS on neurodevelopment at the molecular level.
AMPA
Studies have found that exposure to early-life social isolation for 6 weeks in 21-day-old rats led to depression-like behaviors, including anhedonia and increased despair-like behavior (Fone and Porkess, 2008; Haj-Mirzaian et al., 2015; Wang et al., 2017). Further studies found that early-life social isolation increased the levels of di-methylation of histone H3 at lysine9 (H3K9me2) in the rat hippocampus, but not tri-methylation of histone H3 at lysine4 (H3K4me3). H3K9me2 has been reported to lead to impairment of synaptic plasticity and glutamatergic neurotransmission and is regarded as a risk factor for major depressive disorder (Muchimapura et al., 2002; Peter and Akbarian, 2011). Furthermore, early-life social isolation reduced the levels of glutamate receptor subunits (NMDA receptor subunits, NR1, and AMPA receptor subunits, GluR1 and GluR2) in the rat hippocampus, which could be rescued by minocycline (Wang et al., 2017). In contrast, maternal isolation increased NMDA receptor 2 expression in adolescent mice (Wieck et al., 2013).
Treatment of Different Types of ELS-Induced Epigenetic Changes in the Neurotransmitter Systems
At present, studies have found that the epigenetic effects of fluoxetine, citalopram, and other drugs on the serotonergic pathway further reduce depression-like behaviors (Boulle et al., 2016; Unroe et al., 2021). It has been found that developmental fluoxetine exposure (a selective 5-HT reuptake inhibitor for perinatal depression) increased despair-like behaviors in adult rats (Boulle et al., 2016). Further research found that developmental fluoxetine exposure increased Histone H3 Lys 27 trimethylation (H3K27me3, a repressive histone marker) at Bdnf promoter IV in the hippocampus in prenatally stressed female offspring. However, this effect was only observed in female offspring exposed to prenatal stress, suggesting that prenatal stress increases vulnerability to developmental fluoxetine-induced epigenetic reprogramming in the hippocampus (Boulle et al., 2016). In addition, in clinical studies, it was found that exposure to SSRIs during pregnancy not only resulted in neonatal abstinence syndrome but also increased the risk of anxiety and autism spectrum disorder in neonates. This may be due to the polymorphism of the SLC6A4 promoter affecting gene transcription and ultimately altering SERT function. Similarly, offspring of rodents exposed to SSRIs during pregnancy exhibited depression-like and anxiety-like behaviors and increased expression of histone deacetylase 4 (Hdac4) in the hippocampus, accompanied by increased H3 and H4 acetylation at the Hdac4 promoter. In addition, overexpression of Hdac4 in the hippocampus reversed the depression-like behaviors induced by SSRI exposure during pregnancy. These studies provide a potential therapeutic target for depression-like behaviors induced by exposure to SSRIs during pregnancy. On the other hand, these studies could rationalize antidepressant use during pregnancy to avoid adverse effects on offspring (Sarkar et al., 2014; Glover and Clinton, 2016).
Early maternal separation increases susceptibility to depression in chronic mild stressed rats (Choi et al., 2021). N-3 PUFA ameliorated HPA axis dysregulation and BDNF-serotonergic pathway, decreased hippocampal miRNA-218 and miRNA-132 expression, and increased miRNA-155 expression; thus, it had a therapeutic effect on depression induced by maternal separation in childhood combined with chronic mild stress in adulthood (Kim et al., 2020; Choi et al., 2021). Furthermore, quetiapine treatment reversed depression-like behavior and reduced DNA methyltransferase activity induced by maternal deprivation (Ignácio et al., 2017). LPM570065, a 5-HT/NE/DA triple reuptake inhibitor with a high binding affinity for SERT, norepinephrine transporter (NET) and DAT, has been shown to be effective in major depressive disorder treatment in clinical studies. A study found that LPM570065 significantly ameliorated maternal separation combined with social defeat-induced depression susceptibility in adulthood, and this effect was shown to be mediated by reversing Oxtr methylation and regulating the expression of DNMT1 and DNMT3a in the hippocampus (Meng et al., 2022). In addition, electroconvulsive shock therapy and ketamine treatment shared 43 miRNA targets after maternal separation, seven of which were found to reverse stress-induced changes after treatment. This also suggests that the antidepressant effects of electroconvulsive shock therapy and ketamine are mediated through a common pathway that converges on the same miRNAs. Whether these miRNAs are worthwhile as therapeutic targets still requires more research (O’Connor et al., 2013).
Conclusion
In this article, we reviewed the important role of epigenetics in the treatment of depression-like outcomes produced by different types of ELS, particularly the evidence for the neurotransmitter system. We believe that there is a strong link between ELS, epigenetic modifications, neurotransmitters system, and depression. Current studies have demonstrated that epigenetic changes (DNA methylation and acetylation) in the dopaminergic system, serotoninergic system, GABAergic system, and glutamatergic system have a regulatory effect on depression-like outcomes produced by different types of ELS (Figure 1). On the other hand, the neuronal function can be improved by modulating environmental factors, and different types of ELS-induced gene defects can also be normalized by pharmacological intervention. These studies provide direct evidence for the epigenetic mechanism of depression-like outcomes produced by different types of ELS, provide new targets for the treatment of depression, and provide a theoretical basis for the development of more effective drugs in the future.
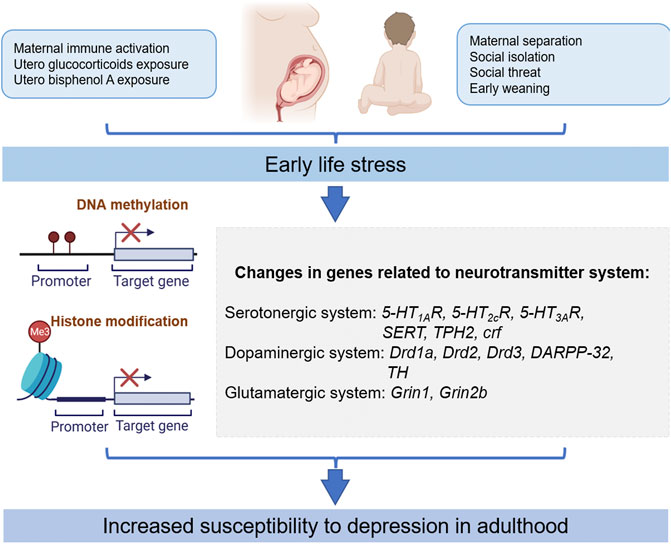
FIGURE 1. Different types of ELS-induced epigenetic modifications contribute to susceptibility to depression in adults. Drd1a, dopamine receptor D1; Drd2, dopamine D2 receptor; Drd3, dopamine D3 receptor; DARPP-32, dopamine- and cAMP-regulated neuronal phosphoprotein; TH, tyrosine hydroxylase; 5-HT1AR, serotonin type 1A receptor; 5-HT2CR, serotonin type 2C receptor; 5-HT3AR, serotonin type 3A receptor; SERT, serotonin transporter; TPH2, tryptophan hydroxylase 2; Grin1, NMDA R1 receptor; Grin2b, NMDA receptor 2b subunit; CRF, corticotropin-releasing factor.
Author Contributions
ZC, JS, and KZ wrote the manuscript. BL and HJ provided the critical revisions. All authors approved the final version of the manuscript for submission.
Funding
This work was supported by the National Natural Science Foundation of China (Grant No. 81871070), Jilin Province Medical and Health Talents (2019SCZT013) and the science and technology development program of Jilin province (No.20200201504JC).
Conflict of Interest
The authors declare that the research was conducted in the absence of any commercial or financial relationships that could be construed as a potential conflict of interest.
Publisher’s Note
All claims expressed in this article are solely those of the authors and do not necessarily represent those of their affiliated organizations, or those of the publisher, the editors, and the reviewers. Any product that may be evaluated in this article, or claim that may be made by its manufacturer, is not guaranteed or endorsed by the publisher.
References
Agis-Balboa, R. C., Pavelka, Z., Kerimoglu, C., and Fischer, A. (2012). Loss of HDAC5 Impairs Memory Function: Implications for Alzheimer's Disease. J. Alzheimers Dis. 33, 35–44. doi:10.3233/JAD-2012-121009
Alameda, L., Trotta, G., Quigley, H., Rodriguez, V., Gadelrab, R., Dwir, D., et al. (2022). Can Epigenetics Shine a Light on the Biological Pathways Underlying Major Mental Disorders? Psychol. Med., 1–21. [online ahead of print]. doi:10.1017/S0033291721005559
Anier, K., Malinovskaja, K., Pruus, K., Aonurm-Helm, A., Zharkovsky, A., and Kalda, A. (2014). Maternal Separation Is Associated with DNA Methylation and Behavioural Changes in Adult Rats. Eur. Neuropsychopharmacol. 24, 459–468. doi:10.1016/j.euroneuro.2013.07.012
Arborelius, L., Owens, M., Plotsky, P., and Nemeroff, C. (1999). The Role of Corticotropin-Releasing Factor in Depression and Anxiety Disorders. J. Endocrinol. 160, 1–12. doi:10.1677/joe.0.1600001
Arling, G. L., and Harlow, H. F. (1967). Effects of Social Deprivation on Maternal Behavior of Rhesus Monkeys. J. Comp. physiological Psychol. 64, 371–377. doi:10.1037/h0025221
Authement, M. E., Kodangattil, J. N., Gouty, S., Rusnak, M., Symes, A. J., Cox, B. M., et al. (2015). Histone Deacetylase Inhibition Rescues Maternal Deprivation-Induced GABAergic Metaplasticity through Restoration of AKAP Signaling. Neuron 86, 1240–1252. doi:10.1016/j.neuron.2015.05.024
Baik, J.-H. (2020). Stress and the Dopaminergic Reward System. Exp. Mol. Med. 52, 1879–1890. doi:10.1038/s12276-020-00532-4
Bale, T. L. (2011). Sex Differences in Prenatal Epigenetic Programing of Stress Pathways. Stress 14, 348–356. doi:10.3109/10253890.2011.586447
Bhansali, P., Dunning, J., Singer, S. E., David, L., and Schmauss, C. (2007). Early Life Stress Alters Adult Serotonin 2C Receptor Pre-mRNA Editing and Expression of the Subunit of the Heterotrimeric G-Protein Gq. J. Neurosci. 27, 1467–1473. doi:10.1523/JNEUROSCI.4632-06.2007
Björk, K., Sjögren, B., and Svenningsson, P. (2010). Regulation of Serotonin Receptor Function in the Nervous System by Lipid Rafts and Adaptor Proteins. Exp. Cell Res. 316, 1351–1356. doi:10.1016/j.yexcr.2010.02.034
Blakely, R. D., Ramamoorthy, S., Schroeter, S., Qian, Y., Apparsundaram, S., Galli, A., et al. (1998). Regulated Phosphorylation and Trafficking of Antidepressant-Sensitive Serotonin Transporter Proteins. Biol. psychiatry 44, 169–178. doi:10.1016/s0006-3223(98)00124-3
Bockmühl, Y., Patchev, A. V., Madejska, A., Hoffmann, A., Sousa, J. C., Sousa, N., et al. (2015). Methylation at the CpG Island Shore Region upregulatesNr3c1promoter Activity after Early-Life Stress. Epigenetics 10, 247–257. doi:10.1080/15592294.2015.1017199
Booij, L., Szyf, M., Carballedo, A., Frey, E.-M., Morris, D., Dymov, S., et al. (2015). DNA Methylation of the Serotonin Transporter Gene in Peripheral Cells and Stress-Related Changes in Hippocampal Volume: a Study in Depressed Patients and Healthy Controls. PloS one 10, e0119061. doi:10.1371/journal.pone.0119061
Bormann, J. (2000). The 'ABC' of GABA Receptors. Trends Pharmacol. Sci. 21, 16–19. doi:10.1016/s0165-6147(99)01413-3
Boulle, F., Pawluski, J. L., Homberg, J. R., Machiels, B., Kroeze, Y., Kumar, N., et al. (2016). Developmental Fluoxetine Exposure Increases Behavioral Despair and Alters Epigenetic Regulation of the Hippocampal BDNF Gene in Adult Female Offspring. Hormones Behav. 80, 47–57. doi:10.1016/j.yhbeh.2016.01.017
Brake, W. G., Zhang, T. Y., Diorio, J., Meaney, M. J., and Gratton, A. (2004). Influence of Early Postnatal Rearing Conditions on Mesocorticolimbic Dopamine and Behavioural Responses to Psychostimulants and Stressors in Adult Rats. Eur. J. Neurosci. 19, 1863–1874. doi:10.1111/j.1460-9568.2004.03286.x
Calabrese, F., Guidotti, G., Middelman, A., Racagni, G., Homberg, J., and Riva, M. A. (2013). Lack of Serotonin Transporter Alters BDNF Expression in the Rat Brain during Early Postnatal Development. Mol. Neurobiol. 48, 244–256. doi:10.1007/s12035-013-8449-z
Caspi, A., Sugden, K., Moffitt, T. E., Taylor, A., Craig, I. W., Harrington, H., et al. (2003). Influence of Life Stress on Depression: Moderation by a Polymorphism in the 5-HTT Gene. Science 301, 386–389. doi:10.1126/science.1083968
Chen, J., Evans, A. N., Liu, Y., Honda, M., Saavedra, J. M., and Aguilera, G. (2012). Maternal Deprivation in Rats Is Associated with Corticotrophin-Releasing Hormone (CRH) Promoter Hypomethylation and Enhances CRH Transcriptional Responses to Stress in Adulthood. J. Neuroendocrinol. 24, 1055–1064. doi:10.1111/j.1365-2826.2012.02306.x
Choi, J.-E., Borkowski, K., Newman, J. W., and Park, Y. (2021). N-3 PUFA Improved Post-menopausal Depression Induced by Maternal Separation and Chronic Mild Stress through Serotonergic Pathway in Rats-Effect Associated with Lipid Mediators. J. Nutr. Biochem. 91, 108599. doi:10.1016/j.jnutbio.2021.108599
Covington, H. E., Maze, I., LaPlant, Q. C., Vialou, V. F., Ohnishi, Y. N., Berton, O., et al. (2009). Antidepressant Actions of Histone Deacetylase Inhibitors. J. Neurosci. 29, 11451–11460. doi:10.1523/JNEUROSCI.1758-09.2009
David, D. J., and Gardier, A. M. (2016). The Pharmacological Basis of the Serotonin System: Application to Antidepressant Response. L'Encéphale 42, 255–263. doi:10.1016/j.encep.2016.03.012
Deslauriers, J., Larouche, A., Sarret, P., and Grignon, S. (2013). Combination of Prenatal Immune Challenge and Restraint Stress Affects Prepulse Inhibition and dopaminergic/GABAergic Markers. Prog. Neuro-Psychopharmacology Biol. Psychiatry 45, 156–164. doi:10.1016/j.pnpbp.2013.05.006
Devlin, A. M., Brain, U., Austin, J., and Oberlander, T. F. (2010). Prenatal Exposure to Maternal Depressed Mood and the MTHFR C677T Variant Affect SLC6A4 Methylation in Infants at Birth. PloS one 5, e12201. doi:10.1371/journal.pone.0012201
Di Chiara, G., Loddo, P., and Tanda, G. (1999). Reciprocal Changes in Prefrontal and Limbic Dopamine Responsiveness to Aversive and Rewarding Stimuli after Chronic Mild Stress: Implications for the Psychobiology of Depression. Biol. psychiatry 46, 1624–1633. doi:10.1016/s0006-3223(99)00236-x
Diorio, J., and Meaney, M. J. (2007). Maternal Programming of Defensive Responses through Sustained Effects on Gene Expression. J. Psychiatry Neurosci. 32, 275–284.
Dukal, H., Frank, J., Lang, M., Treutlein, J., Gilles, M., Wolf, I. A., et al. (2015). New-born Females Show Higher Stress- and Genotype-independent Methylation of SLC6A4 Than Males. Bord. Personal. Disord. Emot. dysregul 2, 8. doi:10.1186/s40479-015-0029-6
Dunlop, B. W., and Nemeroff, C. B. (2007). The Role of Dopamine in the Pathophysiology of Depression. Arch. Gen. Psychiatry 64, 327–337. doi:10.1001/archpsyc.64.3.327
Engdahl, E., Alavian-Ghavanini, A., Forsell, Y., Lavebratt, C., and Rüegg, J. (2021). Childhood Adversity Increases Methylation in the GRIN2B Gene. J. psychiatric Res. 132, 38–43. doi:10.1016/j.jpsychires.2020.09.022
Farrell, C., Doolin, K., O’ Leary, N., Jairaj, C., Roddy, D., Tozzi, L., et al. (2018). DNA Methylation Differences at the Glucocorticoid Receptor Gene in Depression Are Related to Functional Alterations in Hypothalamic-Pituitary-Adrenal axis Activity and to Early Life Emotional Abuse. Psychiatry Res. 265, 341–348. doi:10.1016/j.psychres.2018.04.064
Feifel, A. J., Shair, H. N., and Schmauss, C. (2017). Lasting Effects of Early Life Stress in Mice: Interaction of Maternal Environment and Infant Genes. Genes, Brain, Behav. 16, 768–780. doi:10.1111/gbb.12395
Fink, K. B., and Gothert, M. (2007). 5-HT Receptor Regulation of Neurotransmitter Release. Pharmacol. Rev. 59, 360–417. doi:10.1124/pr.107.07103
Fone, K. C. F., and Porkess, M. V. (2008). Behavioural and Neurochemical Effects of Post-weaning Social Isolation in Rodents-Relevance to Developmental Neuropsychiatric Disorders. Neurosci. Biobehav. Rev. 32, 1087–1102. doi:10.1016/j.neubiorev.2008.03.003
Gaspar, P., Cases, O., and Maroteaux, L. (2003). The Developmental Role of Serotonin: News from Mouse Molecular Genetics. Nat. Rev. Neurosci. 4, 1002–1012. doi:10.1038/nrn1256
Gatt, J. M., Nemeroff, C. B., Schofield, P. R., Paul, R. H., Clark, C. R., Gordon, E., et al. (2010a). Early Life Stress Combined with Serotonin 3A Receptor and Brain-Derived Neurotrophic Factor Valine 66 to Methionine Genotypes Impacts Emotional Brain and Arousal Correlates of Risk for Depression. Biol. psychiatry 68, 818–824. doi:10.1016/j.biopsych.2010.06.025
Gatt, J. M., Williams, L. M., Schofield, P. R., Dobson-Stone, C., Paul, R. H., Grieve, S. M., et al. (2010b). Impact of the HTR3A Gene with Early Life Trauma on Emotional Brain Networks and Depressed Mood. Depress. Anxiety 27, 752–759. doi:10.1002/da.20726
Gershon, A. A., Vishne, T., and Grunhaus, L. (2007). Dopamine D2-like Receptors and the Antidepressant Response. Biol. psychiatry 61, 145–153. doi:10.1016/j.biopsych.2006.05.031
Gilbert, R., Widom, C. S., Browne, K., Fergusson, D., Webb, E., and Janson, S. (2009). Burden and Consequences of Child Maltreatment in High-Income Countries. Lancet 373, 68–81. doi:10.1016/S0140-6736(08)61706-7
Glover, M. E., and Clinton, S. M. (2016). Of Rodents and Humans: A Comparative Review of the Neurobehavioral Effects of Early Life SSRI Exposure in Preclinical and Clinical Research. Int. J. Dev. Neurosci. 51, 50–72. doi:10.1016/j.ijdevneu.2016.04.008
Gos, T., Becker, K., Bock, J., Malecki, U., Bogerts, B., Poeggel, G., et al. (2006). Early Neonatal and Postweaning Social Emotional Deprivation Interferes with the Maturation of Serotonergic and Tyrosine Hydroxylase-Immunoreactive Afferent Fiber Systems in the Rodent Nucleus Accumbens, hippocampus and Amygdala. Neuroscience 140, 811–821. doi:10.1016/j.neuroscience.2006.02.078
Groc, L., and Choquet, D. (2006). AMPA and NMDA Glutamate Receptor Trafficking: Multiple Roads for Reaching and Leaving the Synapse. Cell Tissue Res. 326, 423–438. doi:10.1007/s00441-006-0254-9
Guo, J. U., Ma, D. K., Mo, H., Ball, M. P., Jang, M.-H., Bonaguidi, M. A., et al. (2011). Neuronal Activity Modifies the DNA Methylation Landscape in the Adult Brain. Nat. Neurosci. 14, 1345–1351. doi:10.1038/nn.2900
Haj-Mirzaian, A., Amiri, S., Kordjazy, N., Rahimi-Balaei, M., Haj-Mirzaian, A., Marzban, H., et al. (2015). Blockade of NMDA Receptors Reverses the Depressant, but Not Anxiogenic Effect of Adolescence Social Isolation in Mice. Eur. J. Pharmacol. 750, 160–166. doi:10.1016/j.ejphar.2015.01.006
Hall, F. S., and Perona, M. T. G. (2012). Have Studies of the Developmental Regulation of Behavioral Phenotypes Revealed the Mechanisms of Gene-Environment Interactions? Physiology Behav. 107, 623–640. doi:10.1016/j.physbeh.2012.05.014
Harlow, H. F., Harlow, M. K., and Suomi, S. J. (1971). From Thought to Therapy: Lessons from a Primate Laboratory. Am. Sci. 59, 538–549.
Harmer, C. J., Duman, R. S., and Cowen, P. J. (2017). How Do Antidepressants Work? New Perspectives for Refining Future Treatment Approaches. lancet. Psychiatry 4, 409–418. doi:10.1016/S2215-0366(17)30015-9
Hasan, T. F., and Hasan, H. (2011). Anorexia Nervosa: a Unified Neurological Perspective. Int. J. Med. Sci. 8, 679–703. doi:10.7150/ijms.8.679
Heim, C., and Binder, E. B. (2012). Current Research Trends in Early Life Stress and Depression: Review of Human Studies on Sensitive Periods, Gene-Environment Interactions, and Epigenetics. Exp. Neurol. 233, 102–111. doi:10.1016/j.expneurol.2011.10.032
Hofer, M. A. (1994). Early Relationships as Regulators of Infant Physiology and Behavior. Acta Paediatr. 83, 9–18. doi:10.1111/j.1651-2227.1994.tb13260.x
Hofer, M. A. (1970). Physiological Responses of Infant Rats to Separation from Their Mothers. Science 168, 871–873. doi:10.1126/science.168.3933.871
Hofer, M. A. (1973). The Effects of Brief Maternal Separations on Behavior and Heart Rate of Two Week Old Rat Pups. Physiology Behav. 10, 423–427. doi:10.1016/0031-9384(73)90200-x
Hoyer, D., Hannon, J. P., and Martin, G. R. (2002). Molecular, Pharmacological and Functional Diversity of 5-HT Receptors. Pharmacol. Biochem. Behav. 71, 533–554. doi:10.1016/s0091-3057(01)00746-8
Ignácio, Z. M., Réus, G. Z., Abelaira, H. M., Maciel, A. L., de Moura, A. B., Matos, D., et al. (2017). Quetiapine Treatment Reverses Depressive-like Behavior and Reduces DNA Methyltransferase Activity Induced by Maternal Deprivation. Behav. Brain Res. 320, 225–232. doi:10.1016/j.bbr.2016.11.044
Jahng, J. W., Ryu, V., Yoo, S. B., Noh, S. J., Kim, J. Y., and Lee, J. H. (2010). Mesolimbic Dopaminergic Activity Responding to Acute Stress Is Blunted in Adolescent Rats that Experienced Neonatal Maternal Separation. Neuroscience 171, 144–152. doi:10.1016/j.neuroscience.2010.08.063
Jezierski, G., Zehle, S., Bock, J., Braun, K., and Gruss, M. (2007). Early Stress and Chronic Methylphenidate Cross-Sensitize Dopaminergic Responses in the Adolescent Medial Prefrontal Cortex and Nucleus Accumbens. J. Neurochem. 103, 2234–2244. doi:10.1111/j.1471-4159.2007.04927.x
Jørgensen, H., Knigge, U., Kjaer, A., Møller, M., and Warberg, J. (2002). Serotonergic Stimulation of Corticotropin-Releasing Hormone and Pro-opiomelanocortin Gene Expression. J. Neuroendocrinol. 14, 788–795. doi:10.1046/j.1365-2826.2002.00839.x
Kang, H.-J., Kim, J.-M., Stewart, R., Kim, S.-Y., Bae, K.-Y., Kim, S.-W., et al. (2013). Association of SLC6A4 Methylation with Early Adversity, Characteristics and Outcomes in Depression. Prog. Neuro-Psychopharmacology Biol. Psychiatry 44, 23–28. doi:10.1016/j.pnpbp.2013.01.006
Kim, E.-Y., Choi, J.-E., Kim, M., Hong, J., and Park, Y. (2020). N-3 PUFA Have Antidepressant-like Effects via Improvement of the HPA-Axis and Neurotransmission in Rats Exposed to Combined Stress. Mol. Neurobiol. 57, 3860–3874. doi:10.1007/s12035-020-01980-9
Klein, D. N., Arnow, B. A., Barkin, J. L., Dowling, F., Kocsis, J. H., Leon, A. C., et al. (2009). Early Adversity in Chronic Depression: Clinical Correlates and Response to Pharmacotherapy. Depress. Anxiety 26, 701–710. doi:10.1002/da.20577
Köhler, J. C., Gröger, N., Lesse, A., Guara Ciurana, S., Rether, K., Fegert, J., et al. (2019). Early-Life Adversity Induces Epigenetically Regulated Changes in Hippocampal Dopaminergic Molecular Pathways. Mol. Neurobiol. 56, 3616–3625. doi:10.1007/s12035-018-1199-1
Kondo, M., Nakamura, Y., Ishida, Y., and Shimada, S. (2015). The 5-HT3 Receptor Is Essential for Exercise-Induced Hippocampal Neurogenesis and Antidepressant Effects. Mol. Psychiatry 20, 1428–1437. doi:10.1038/mp.2014.153
Kronman, H., Torres-Berrío, A., Sidoli, S., Issler, O., Godino, A., Ramakrishnan, A., et al. (2021). Long-term Behavioral and Cell-type-specific Molecular Effects of Early Life Stress Are Mediated by H3K79me2 Dynamics in Medium Spiny Neurons. Nat. Neurosci. 24, 667–676. doi:10.1038/s41593-021-00814-8
Kundakovic, M., Gudsnuk, K., Herbstman, J. B., Tang, D., Perera, F. P., and Champagne, F. A. (2015). DNA Methylation of BDNF as a Biomarker of Early-Life Adversity. Proc. Natl. Acad. Sci. U.S.A. 112, 6807–6813. doi:10.1073/pnas.1408355111
Kunzler, J., Braun, K., and Bock, J. (2015). Early Life Stress and Sex-specific Sensitivity of the Catecholaminergic Systems in Prefrontal and Limbic Regions of Octodon degus. Brain Struct. Funct. 220, 861–868. doi:10.1007/s00429-013-0688-2
Lener, M. S., Niciu, M. J., Ballard, E. D., Park, M., Park, L. T., Nugent, A. C., et al. (2017). Glutamate and Gamma-Aminobutyric Acid Systems in the Pathophysiology of Major Depression and Antidepressant Response to Ketamine. Biol. psychiatry 81, 886–897. doi:10.1016/j.biopsych.2016.05.005
Lesch, K.-P. (2011). When the Serotonin Transporter Gene Meets Adversity: the Contribution of Animal Models to Understanding Epigenetic Mechanisms in Affective Disorders and Resilience. Curr. Top. Behav. Neurosci. 7, 251–280. doi:10.1007/7854_2010_109
Leventopoulos, M., Russig, H., Feldon, J., Pryce, C. R., and Opacka-Juffry, J. (2009). Early Deprivation Leads to Long-Term Reductions in Motivation for Reward and 5-HT1A Binding and Both Effects Are Reversed by Fluoxetine. Neuropharmacology 56, 692–701. doi:10.1016/j.neuropharm.2008.12.005
Levine, A., Worrell, T. R., Zimnisky, R., and Schmauss, C. (2012). Early Life Stress Triggers Sustained Changes in Histone Deacetylase Expression and Histone H4 Modifications that Alter Responsiveness to Adolescent Antidepressant Treatment. Neurobiol. Dis. 45, 488–498. doi:10.1016/j.nbd.2011.09.005
Lira, A., Zhou, M., Castanon, N., Ansorge, M. S., Gordon, J. A., Francis, J. H., et al. (2003). Altered Depression-Related Behaviors and Functional Changes in the Dorsal Raphe Nucleus of Serotonin Transporter-Deficient Mice. Biol. psychiatry 54, 960–971. doi:10.1016/s0006-3223(03)00696-6
Marmorstein, R., and Zhou, M.-M. (2014). Writers and Readers of Histone Acetylation: Structure, Mechanism, and Inhibition. Cold Spring Harb. Perspect. Biol. 6, a018762. doi:10.1101/cshperspect.a018762
Marrocco, J., Gray, J. D., Kogan, J. F., Einhorn, N. R., O’Cinneide, E. M., Rubin, T. G., et al. (2019). Early Life Stress Restricts Translational Reactivity in CA3 Neurons Associated with Altered Stress Responses in Adulthood. Front. Behav. Neurosci. 13, 157. doi:10.3389/fnbeh.2019.00157
Marrocco, J., Petty, G. H., Ríos, M. B., Gray, J. D., Kogan, J. F., Waters, E. M., et al. (2017). A Sexually Dimorphic Pre-stressed Translational Signature in CA3 Pyramidal Neurons of BDNF Val66Met Mice. Nat. Commun. 8, 808. doi:10.1038/s41467-017-01014-4
Masserano, J., and Weiner, N. (1983). Tyrosine Hydroxylase Regulation in the Central Nervous System. Mol. Cell Biochem. 53-54, 129–152. doi:10.1007/BF00225250
Matthews, K., Dalley, J. W., Matthews, C., Hu Tsai, T., and Robbins, T. W. (2001). Periodic Maternal Separation of Neonatal Rats Produces Region- and Gender-specific Effects on Biogenic Amine Content in Postmortem Adult Brain. Synapse 40, 1–10. doi:10.1002/1098-2396(200104)40:1<1::aid-syn1020>3.0.co;2-e
McGowan, P. O., Sasaki, A., D'Alessio, A. C., Dymov, S., Labonté, B., Szyf, M., et al. (2009). Epigenetic Regulation of the Glucocorticoid Receptor in Human Brain Associates with Childhood Abuse. Nat. Neurosci. 12, 342–348. doi:10.1038/nn.2270
Meng, P., Li, C., Duan, S., Ji, S., Xu, Y., Mao, Y., et al. (2022). Epigenetic Mechanism of 5-HT/NE/DA Triple Reuptake Inhibitor on Adult Depression Susceptibility in Early Stress Mice. Front. Pharmacol. 13, 848251. doi:10.3389/fphar.2022.848251
Migliori, V., Müller, J., Phalke, S., Low, D., Bezzi, M., Mok, W. C., et al. (2012). Symmetric Dimethylation of H3R2 Is a Newly Identified Histone Mark that Supports Euchromatin Maintenance. Nat. Struct. Mol. Biol. 19, 136–144. doi:10.1038/nsmb.2209
Miniati, M., Rucci, P., Benvenuti, A., Frank, E., Buttenfield, J., Giorgi, G., et al. (2010). Clinical Characteristics and Treatment Outcome of Depression in Patients with and without a History of Emotional and Physical Abuse. J. psychiatric Res. 44, 302–309. doi:10.1016/j.jpsychires.2009.09.008
Mitchell, E., Klein, S. L., Argyropoulos, K. V., Sharma, A., Chan, R. B., Toth, J. G., et al. (2016). Behavioural Traits Propagate across Generations via Segregated Iterative-Somatic and Gametic Epigenetic Mechanisms. Nat. Commun. 7, 11492. doi:10.1038/ncomms11492
Möller, M., Du Preez, J. L., Viljoen, F. P., Berk, M., and Harvey, B. H. (2013). N-acetyl Cysteine Reverses Social Isolation Rearing Induced Changes in Cortico-Striatal Monoamines in Rats. Metab. Brain Dis. 28, 687–696. doi:10.1007/s11011-013-9433-z
Molteni, R., Cattaneo, A., Calabrese, F., Macchi, F., Olivier, J. D. A., Racagni, G., et al. (2010). Reduced Function of the Serotonin Transporter Is Associated with Decreased Expression of BDNF in Rodents as Well as in Humans. Neurobiol. Dis. 37, 747–755. doi:10.1016/j.nbd.2009.12.014
Montalvo-Ortiz, J. L., Bordner, K. A., Carlyle, B. C., Gelernter, J., Simen, A. A., and Kaufman, J. (2016). The Role of Genes Involved in Stress, Neural Plasticity, and Brain Circuitry in Depressive Phenotypes: Convergent Findings in a Mouse Model of Neglect. Behav. Brain Res. 315, 71–74. doi:10.1016/j.bbr.2016.08.010
Muchimapura, S., Fulford, A. J., Mason, R., and Marsden, C. A. (2002). Isolation Rearing in the Rat Disrupts the Hippocampal Response to Stress. Neuroscience 112, 697–705. doi:10.1016/s0306-4522(02)00107-0
Murthy, S., Niquille, M., Hurni, N., Limoni, G., Frazer, S., Chameau, P., et al. (2014). Serotonin Receptor 3A Controls Interneuron Migration into the Neocortex. Nat. Commun. 5, 5524. doi:10.1038/ncomms6524
O'Connor, R. M., Grenham, S., Dinan, T. G., and Cryan, J. F. (2013). microRNAs as Novel Antidepressant Targets: Converging Effects of Ketamine and Electroconvulsive Shock Therapy in the Rat hippocampus. Int. J. Neuropsychopharmacol. 16, 1885–1892. doi:10.1017/S1461145713000448
Oberlander, T. F., Weinberg, J., Papsdorf, M., Grunau, R., Misri, S., and Devlin, A. M. (2008). Prenatal Exposure to Maternal Depression, Neonatal Methylation of Human Glucocorticoid Receptor Gene (NR3C1) and Infant Cortisol Stress Responses. Epigenetics 3, 97–106. doi:10.4161/epi.3.2.6034
Oh, J.-e., Chambwe, N., Klein, S., Gal, J., Andrews, S., Gleason, G., et al. (2013). Differential Gene Body Methylation and Reduced Expression of Cell Adhesion and Neurotransmitter Receptor Genes in Adverse Maternal Environment. Transl. Psychiatry 3, e218. doi:10.1038/tp.2012.130
Olsson, C. A., Foley, D. L., Parkinson-Bates, M., Byrnes, G., McKenzie, M., Patton, G. C., et al. (2010). Prospects for Epigenetic Research within Cohort Studies of Psychological Disorder: a Pilot Investigation of a Peripheral Cell Marker of Epigenetic Risk for Depression. Biol. Psychol. 83, 159–165. doi:10.1016/j.biopsycho.2009.12.003
Palmisano, M., and Pandey, S. C. (2017). Epigenetic Mechanisms of Alcoholism and Stress-Related Disorders. Alcohol 60, 7–18. doi:10.1016/j.alcohol.2017.01.001
Pan, Y., Hong, Y., Zhang, Q.-Y., and Kong, L.-D. (2013). Impaired Hypothalamic Insulin Signaling in CUMS Rats: Restored by Icariin and Fluoxetine through Inhibiting CRF System. Psychoneuroendocrinology 38, 122–134. doi:10.1016/j.psyneuen.2012.05.007
Parade, S. H., Novick, A. M., Parent, J., Seifer, R., Klaver, S. J., Marsit, C. J., et al. (2017). Stress Exposure and Psychopathology Alter Methylation of the Serotonin Receptor 2A (HTR2A) Gene in Preschoolers. Dev. Psychopathol. 29, 1619–1626. doi:10.1017/S0954579417001274
Park, C., Rosenblat, J. D., Brietzke, E., Pan, Z., Lee, Y., Cao, B., et al. (2019). Stress, Epigenetics and Depression: A Systematic Review. Neurosci. Biobehav. Rev. 102, 139–152. doi:10.1016/j.neubiorev.2019.04.010
Perroud, N., Zewdie, S., Stenz, L., Adouan, W., Bavamian, S., Prada, P., et al. (2016). Methylation of Serotonin Receptor 3a In Adhd, Borderline Personality, And Bipolar Disorders: Link With Severity of The Disorders and Childhood Maltreatment. Depress Anxiety 33, 45–55. doi:10.1002/da.22406
Peter, C. J., and Akbarian, S. (2011). Balancing Histone Methylation Activities in Psychiatric Disorders. Trends Mol. Med. 17, 372–379. doi:10.1016/j.molmed.2011.02.003
Pezawas, L., Meyer-Lindenberg, A., Goldman, A. L., Verchinski, B. A., Chen, G., Kolachana, B. S., et al. (2008). Evidence of Biologic Epistasis between BDNF and SLC6A4 and Implications for Depression. Mol. Psychiatry 13, 709–716. doi:10.1038/mp.2008.32
Popoli, M., Yan, Z., McEwen, B. S., and Sanacora, G. (2011). The Stressed Synapse: the Impact of Stress and Glucocorticoids on Glutamate Transmission. Nat. Rev. Neurosci. 13, 22–37. doi:10.1038/nrn3138
Provenzi, L., Guida, E., and Montirosso, R. (2018). Preterm Behavioral Epigenetics: A Systematic Review. Neurosci. Biobehav. Rev. 84, 262–271. doi:10.1016/j.neubiorev.2017.08.020
Ptak, C., and Petronis, A. (2010). Epigenetic Approaches to Psychiatric Disorders. Dialogues Clin. Neurosci. 12, 25–35. doi:10.31887/DCNS.2010.12.1/cptak
Reisinger, S. N., Kong, E., Khan, D., Schulz, S., Ronovsky, M., Berger, S., et al. (2016). Maternal Immune Activation Epigenetically Regulates Hippocampal Serotonin Transporter Levels. Neurobiol. stress 4, 34–43. doi:10.1016/j.ynstr.2016.02.007
Rodrigues, A. J., Leão, P., Pêgo, J. M., Cardona, D., Carvalho, M. M., Oliveira, M., et al. (2012). Mechanisms of Initiation and Reversal of Drug-Seeking Behavior Induced by Prenatal Exposure to Glucocorticoids. Mol. Psychiatry 17, 1295–1305. doi:10.1038/mp.2011.126
Ryan, B., Musazzi, L., Mallei, A., Tardito, D., Gruber, S. H. M., El Khoury, A., et al. (2009). Remodelling by Early-Life Stress of NMDA Receptor-dependent Synaptic Plasticity in a Gene-Environment Rat Model of Depression. Int. J. Neuropsychopharm. 12, 553–559. doi:10.1017/S1461145708009607
Sanwald, S., Widenhorn-Müller, K., Schönfeldt-Lecuona, C., Connemann, B. J., Gahr, M., Kammer, T., et al. (2021). Factors Related to Age at Depression Onset: the Role of SLC6A4 Methylation, Sex, Exposure to Stressful Life Events and Personality in a Sample of Inpatients Suffering from Major Depression. BMC psychiatry 21, 167. doi:10.1186/s12888-021-03166-6
Sarkar, A., Chachra, P., and Vaidya, V. A. (2014). Postnatal Fluoxetine-Evoked Anxiety Is Prevented by Concomitant 5-HT2A/C Receptor Blockade and Mimicked by Postnatal 5-HT2A/C Receptor Stimulation. Biol. psychiatry 76, 858–868. doi:10.1016/j.biopsych.2013.11.005
Sasagawa, T., Horii-Hayashi, N., Okuda, A., Hashimoto, T., Azuma, C., and Nishi, M. (2017). Long-term Effects of Maternal Separation Coupled with Social Isolation on Reward Seeking and Changes in Dopamine D1 Receptor Expression in the Nucleus Accumbens via DNA Methylation in Mice. Neurosci. Lett. 641, 33–39. doi:10.1016/j.neulet.2017.01.025
Schiavone, S., Sorce, S., Dubois-Dauphin, M., Jaquet, V., Colaianna, M., Zotti, M., et al. (2009). Involvement of NOX2 in the Development of Behavioral and Pathologic Alterations in Isolated Rats. Biol. psychiatry 66, 384–392. doi:10.1016/j.biopsych.2009.04.033
Schreiber, A. L., Lu, Y.-L., Baynes, B. B., Richardson, H. N., and Gilpin, N. W. (2017). Corticotropin-releasing Factor in Ventromedial Prefrontal Cortex Mediates Avoidance of a Traumatic Stress-Paired Context. Neuropharmacology 113, 323–330. doi:10.1016/j.neuropharm.2016.05.008
Seay, B., Alexander, B. K., and Harlow, H. F. (1964). Maternal Behavior of Socially Deprived Rhesus Monkeys. J. Abnorm. Soc. Psychol. 69, 345–354. doi:10.1037/h0040539
Shen, T., Li, X., Chen, L., Chen, Z., Tan, T., Hua, T., et al. (2020). The Relationship of Tryptophan Hydroxylase-2 Methylation to Early-Life Stress and its Impact on Short-Term Antidepressant Treatment Response. J. Affect. Disord. 276, 850–858. doi:10.1016/j.jad.2020.07.111
Shepard, R. D., Gouty, S., Kassis, H., Berenji, A., Zhu, W., Cox, B. M., et al. (2018). Targeting Histone Deacetylation for Recovery of Maternal Deprivation-Induced Changes in BDNF and AKAP150 Expression in the VTA. Exp. Neurol. 309, 160–168. doi:10.1016/j.expneurol.2018.08.002
Shepard, R. D., Langlois, L. D., Authement, M. E., and Nugent, F. S. (2020). Histone Deacetylase Inhibition Reduces Ventral Tegmental Area Dopamine Neuronal Hyperexcitability Involving AKAP150 Signaling Following Maternal Deprivation in Juvenile Male Rats. J Neurosci. Res. 98, 1457–1467. doi:10.1002/jnr.24613
Shepard, R. D., and Nugent, F. S. (2020). Early Life Stress- and Drug-Induced Histone Modifications within the Ventral Tegmental Area. Front. Cell Dev. Biol. 8, 588476. doi:10.3389/fcell.2020.588476
Smith, S. M., and Vale, W. W. (2006). The Role of the Hypothalamic-Pituitary-Adrenal axis in Neuroendocrine Responses to Stress. Dialogues Clin. Neurosci. 8, 383–395. doi:10.31887/DCNS.2006.8.4/ssmith
Sorce, S., Schiavone, S., Tucci, P., Colaianna, M., Jaquet, V., Cuomo, V., et al. (2010). The NADPH Oxidase NOX2 Controls Glutamate Release: a Novel Mechanism Involved in Psychosis-like Ketamine Responses. J. Neurosci. 30, 11317–11325. doi:10.1523/JNEUROSCI.1491-10.2010
Sroufe, L. A. (2005). Attachment and Development: a Prospective, Longitudinal Study from Birth to Adulthood. Attachment Hum. Dev. 7, 349–367. doi:10.1080/14616730500365928
Stockmeier, C. A. (2003). Involvement of Serotonin in Depression: Evidence from Postmortem and Imaging Studies of Serotonin Receptors and the Serotonin Transporter. J. psychiatric Res. 37, 357–373. doi:10.1016/s0022-3956(03)00050-5
Sun, H., Kennedy, P. J., and Nestler, E. J. (2013). Epigenetics of the Depressed Brain: Role of Histone Acetylation and Methylation. Neuropsychopharmacol official Publ. Am. Coll. Neuropsychopharmacol. 38, 124–137. doi:10.1038/npp.2012.73
Suri, D., Bhattacharya, A., and Vaidya, V. A. (2014). Early Stress Evokes Temporally Distinct Consequences on the Hippocampal Transcriptome, Anxiety and Cognitive Behaviour. Int. J. Neuropsychopharm. 17, 289–301. doi:10.1017/S1461145713001004
Tesone-Coelho, C., Morel, L. J., Bhatt, J., Estevez, L., Naudon, L., Giros, B., et al. (2015). Vulnerability to Opiate Intake in Maternally Deprived Rats: Implication of MeCP2 and of Histone Acetylation. Addict. Biol. 20, 120–131. doi:10.1111/adb.12084
Tesoro-Cruz, E., Manuel-Apolinar, L., Oviedo, N., Orozco-Suárez, S., Crespo Ramírez, M., Bekker-Méndez, V. C., et al. (2021). Increase of 5-HT Levels Is Induced Both in Mouse Brain and HEK-293 Cells Following Their Exposure to a Non-viral Tryptophan Hydroxylase Construct. Transl. Psychiatry 11, 515. doi:10.1038/s41398-021-01634-x
Todkar, A., Granholm, L., Aljumah, M., Nilsson, K. W., Comasco, E., and Nylander, I. (2015). HPA Axis Gene Expression and DNA Methylation Profiles in Rats Exposed to Early Life Stress, Adult Voluntary Ethanol Drinking and Single Housing. Front. Mol. Neurosci. 8, 90. doi:10.3389/fnmol.2015.00090
Uher, R., and McGuffin, P. (2010). The Moderation by the Serotonin Transporter Gene of Environmental Adversity in the Etiology of Depression: 2009 Update. Mol. Psychiatry 15, 18–22. doi:10.1038/mp.2009.123
Underwood, M. D., Bakalian, M. J., Johnson, V. L., Kassir, S. A., Ellis, S. P., Mann, J. J., et al. (2020). Less NMDA Receptor Binding in Dorsolateral Prefrontal Cortex and Anterior Cingulate Cortex Associated with Reported Early-Life Adversity but Not Suicide. Int. J. Neuropsychopharmacol. 23, 311–318. doi:10.1093/ijnp/pyaa009
Unroe, K. A., Glover, M. E., Shupe, E. A., Feng, N., and Clinton, S. M. (2021). Perinatal SSRI Exposure Disrupts G Protein-Coupled Receptor Bai3 in Developing Dentate Gyrus and Adult Emotional Behavior: Relevance to Psychiatric Disorders. Neuroscience 471, 32–50. doi:10.1016/j.neuroscience.2021.07.007
Valzania, A., Catale, C., Viscomi, M. T., Puglisi-Allegra, S., and Carola, V. (2017). Histone Deacetylase 5 Modulates the Effects of Social Adversity in Early Life on Cocaine-Induced Behavior. Physiology Behav. 171, 7–12. doi:10.1016/j.physbeh.2016.12.027
Van den Hove, D. L. A., Kenis, G., Brass, A., Opstelten, R., Rutten, B. P. F., Bruschettini, M., et al. (2013). Vulnerability versus Resilience to Prenatal Stress in Male and Female Rats; Implications from Gene Expression Profiles in the hippocampus and Frontal Cortex. Eur. Neuropsychopharmacol. 23, 1226–1246. doi:10.1016/j.euroneuro.2012.09.011
van der Doelen, R. H. A., Arnoldussen, I. A., Ghareh, H., van Och, L., Homberg, J. R., and Kozicz, T. (2015). Early Life Adversity and Serotonin Transporter Gene Variation Interact to Affect DNA Methylation of the Corticotropin-Releasing Factor Gene Promoter Region in the Adult Rat Brain. Dev. Psychopathol. 27, 123–135. doi:10.1017/S0954579414001345
van der Doelen, R. H. A., Robroch, B., Arnoldussen, I. A., Schulpen, M., Homberg, J. R., and Kozicz, T. (2017). Serotonin and Urocortin 1 in the Dorsal Raphe and Edinger-Westphal Nuclei after Early Life Stress in Serotonin Transporter Knockout Rats. Neuroscience 340, 345–358. doi:10.1016/j.neuroscience.2016.10.072
van Riel, E., van Gemert, N. G., Meijer, O. C., and Joëls, M. (2004). Effect of Early Life Stress on Serotonin Responses in the hippocampus of Young Adult Rats. Synapse 53, 11–19. doi:10.1002/syn.20033
van Velzen, A., and Toth, M. (2010). Role of Maternal 5-HT(1A) Receptor in Programming Offspring Emotional and Physical Development. Genes, Brain, Behav. 9, 877–885. doi:10.1111/j.1601-183X.2010.00625.x
Vangeel, E. B., Pishva, E., Hompes, T., van den Hove, D., Lambrechts, D., Allegaert, K., et al. (2017). Newborn Genome-wide DNA Methylation in Association with Pregnancy Anxiety Reveals a Potential Role for GABBR1. Clin. Epigenet 9, 107. doi:10.1186/s13148-017-0408-5
Viola, T. W., Wearick-Silva, L. E., Creutzberg, K. C., Kestering-Ferreira, É., Orso, R., Centeno-Silva, A., et al. (2019). Postnatal Impoverished Housing Impairs Adolescent Risk-Assessment and Increases Risk-Taking: A Sex-specific Effect Associated with Histone Epigenetic Regulation of Crfr1 in the Medial Prefrontal Cortex. Psychoneuroendocrinology 99, 8–19. doi:10.1016/j.psyneuen.2018.08.032
Wang, H.-T., Huang, F.-L., Hu, Z.-L., Zhang, W.-J., Qiao, X.-Q., Huang, Y.-Q., et al. (2017). Early-Life Social Isolation-Induced Depressive-like Behavior in Rats Results in Microglial Activation and Neuronal Histone Methylation that Are Mitigated by Minocycline. Neurotox. Res. 31, 505–520. doi:10.1007/s12640-016-9696-3
Wankerl, M., Miller, R., Kirschbaum, C., Hennig, J., Stalder, T., and Alexander, N. (2014). Effects of Genetic and Early Environmental Risk Factors for Depression on Serotonin Transporter Expression and Methylation Profiles. Transl. Psychiatry 4, e402. doi:10.1038/tp.2014.37
Weder, N., Zhang, H., Jensen, K., Yang, B. Z., Simen, A., Jackowski, A., et al. (2014). Child Abuse, Depression, and Methylation in Genes Involved with Stress, Neural Plasticity, and Brain Circuitry. J. Am. Acad. Child Adolesc. Psychiatry 53, 417. doi:10.1016/j.jaac.2013.12.025
Wieck, A., Andersen, S. L., and Brenhouse, H. C. (2013). Evidence for a Neuroinflammatory Mechanism in Delayed Effects of Early Life Adversity in Rats: Relationship to Cortical NMDA Receptor Expression. Brain, Behav. Immun. 28, 218–226. doi:10.1016/j.bbi.2012.11.012
Wightman, R. M., and Zimmerman, J. B. (1990). Control of Dopamine Extracellular Concentration in Rat Striatum by Impulse Flow and Uptake. Brain Res. Res. Rev. 15, 135–144. doi:10.1016/0165-0173(90)90015-g
Willner, P., Muscat, R., and Papp, M. (1992). Chronic Mild Stress-Induced Anhedonia: a Realistic Animal Model of Depression. Neurosci. Biobehav. Rev. 16, 525–534. doi:10.1016/s0149-7634(05)80194-0
Xu, J., Zhang, G., Cheng, Y., Chen, B., Dong, Y., Li, L., et al. (2011). Hypomethylation of the HTR1A Promoter Region and High Expression of HTR1A in the Peripheral Blood Lymphocytes of Patients with Systemic Lupus Erythematosus. Lupus 20, 678–689. doi:10.1177/0961203310394892
Xu, Z., Chen, Z., Shen, T., Chen, L., Tan, T., Gao, C., et al. (2022). The Impact of HTR1A and HTR1B Methylation Combined with Stress/genotype on Early Antidepressant Efficacy. Psychiatry Clin. Neurosci. 76, 51–57. doi:10.1111/pcn.13314
Young, E. A., Lopez, J. F., Murphy-Weinberg, V., Watson, S. J., and Akil, H. (2003). Mineralocorticoid Receptor Function in Major Depression. Arch. Gen. Psychiatry 60, 24–28. doi:10.1001/archpsyc.60.1.24
Keywords: early life stress, depression, epigenetics, neurotransmitter systems, methylation
Citation: Cheng Z, Su J, Zhang K, Jiang H and Li B (2022) Epigenetic Mechanism of Early Life Stress-Induced Depression: Focus on the Neurotransmitter Systems. Front. Cell Dev. Biol. 10:929732. doi: 10.3389/fcell.2022.929732
Received: 27 April 2022; Accepted: 31 May 2022;
Published: 05 July 2022.
Edited by:
Fushun Wang, Nanjing University of Chinese Medicine, ChinaCopyright © 2022 Cheng, Su, Zhang, Jiang and Li. This is an open-access article distributed under the terms of the Creative Commons Attribution License (CC BY). The use, distribution or reproduction in other forums is permitted, provided the original author(s) and the copyright owner(s) are credited and that the original publication in this journal is cited, in accordance with accepted academic practice. No use, distribution or reproduction is permitted which does not comply with these terms.
*Correspondence: Huiyi Jiang, hyjiang@jlu.edu.cn; Bingjin Li, libingjin@jlu.edu.cn