- 1The Key Laboratory of Developmental Genes and Human Disease, School of Life Science and Technology, Southeast University, Nanjing, China
- 2School of Biological and Medical Engineering, Southeast University, Nanjing, China
Protein phosphorylation is crucial for a variety of biological functions, but how it is involved in sexual development and behavior is rarely known. In this study, we performed a screen of RNA interference targeting 177 protein kinases in Drosophila and identified 13 kinases involved in sexual development in one or both sexes. We further identified that PKA and CASK promote female sexual behavior while not affecting female differentiation. Knocking down PKA or CASK in about five pairs of pC1 neurons in the central brain affects the fine projection but not cell number of these pC1 neurons and reduces virgin female receptivity. We also found that PKA and CASK signaling is required acutely during adulthood to promote female sexual behavior. These results reveal candidate kinases required for sexual development and behaviors and provide insights into how kinases would regulate neuronal development and physiology to fine tune the robustness of sexual behaviors.
Introduction
Protein phosphorylation is an important post-translational modification (PTM). Many enzymes and receptors are activated/deactivated by phosphorylation and dephosphorylation events through kinases and phosphatases (Humphrey, James et al., 2015). Putative orthologous kinase groups have been classified in different species according to the amino acid residue that it phosphorylates, such as STKs (serine/threonine kinases) and TKs (Tyrosine kinases), in which fly and human share several kinases families involved in neurobiology, cell cycle and morphogenesis (Manning, Plowman et al., 2002). In humans, aberrations of kinases have been reported in different types of cancer, as well as neurodegenerative diseases (Ardito, Giuliani et al., 2017; Khan, Kulasiri et al., 2021). Studies in Drosophila melanogaster have provided invaluable insights for identifying conserved kinase pathway components and mechanisms that control signaling events involved in development and behaviors (Giese and Mizuno 2013; Mele and Johnson 2019).
Extensive communication between kinases is a prominent feature of signaling networks. For example, Ca2+/calmodulin-dependent protein kinase II (CaMKII) is regulated by CASK, a membrane-associated guanylate kinase, in neuronal growth, calcium signaling and learning (Gillespie and Hodge 2013). cAMP-dependent protein kinase (PKA) is a critical kinase involved in several signaling processes in the central nervous system (CNS) (Khan, Kulasiri et al., 2021). The Drosophila PKA consists of three catalytic subunits, PKA-C1-3, of which PKA-C1 expressed in the mushroom body (MB) plays important roles in different behaviors, including sleep, learning and memory (Skoulakis, Kalderon et al., 1993; Machado Almeida, Lago Solis et al., 2021). Besides, the loss of PKA-C3 causes copulation defects in male flies (Cassar, Sunderhaus et al., 2018). cAMP binding to the regulatory domain of PKA causes the protein to dissociate, exposing the catalytic subunits, which triggers PKA kinase activity. Both PKA and CaMKII induce cAMP Responsive Element Binding (CREB) activation and enhance specific transcript activity (Borovac, Bosch et al., 2018; Lee, Lin et al., 2018).
The sex determination hierarchy specifies sexually dimorphic aspects of development and behaviors in Drosophila (Robinett, Vaughan et al., 2010; Sato and Yamamoto 2014). Female-specific expression of transformer (tra) along with the non-sex-specific transformer 2 (tra2) control sex-specific splicing of two pivotal downstream genes, doublesex (dsx) and fruitless (fru). dsx and fru are sex-specifically spliced to yield male-specific DsxM and FruM, and female-specific DsxF transcription factors (Baker and Ridge 1980; Burtis and Baker 1989; Ito, Fujitani et al., 1996; Ryner, Goodwin et al., 1996). DsxM and DsxF are expressed in various tissues and cells, directing most aspects of somatic sex differentiation outside the CNS in both sexes (Lee, Hall et al., 2002; Rideout, Dornan et al., 2010). In the CNS, FruM is expressed in ∼2000 neurons, controlling sexual behavior in males (Lee, Foss et al., 2000; Manoli, Foss et al., 2005; Stockinger, Kvitsiani et al., 2005; Yu, Kanai et al., 2010), while DsxM is expressed in ∼900 neurons, most of which co-express FruM. DsxM regulates courtship intensity as well as sine song production in the presence of FruM (Villella and Hall 1996; Shirangi, Wong et al., 2016), and the experience-dependent courtship acquisition in the absence of FruM (Pan and Baker 2014). In females, DsxF is expressed in ∼700 neurons in the CNS. These dsxF-expressing neurons, particularly a cluster of pC1 neurons in the central brain, regulate virgin female receptive, post-mating behavior and aggression (Zhou, Pan et al., 2014; Rezaval, Pattnaik et al., 2016; Palavicino-Maggio, Chan et al., 2019; Wang, Wang et al., 2020; Wang, Wang et al., 2021).
Protein phosphorylation controls many cellular processes to coordinate complex functions including sexual development. In Drosophila, somatic sexual identity is under the control of a genetic cascade, which requires Hopscotch (Hop), a JAK kinase, to transduce the 2-fold expression differences in a X-linked signal element unpaired (upd), augmenting the initial sex-determination signal, the expression of Sex lethal (Sxl) (Avila and Erickson 2007). It has also been found that the kinase Doa (Darkener of Apricot) phosphorylates SR (serine/arginine) proteins, including Tra and Tra2, and is required for proper alternative splicing of dsx in both sexes (Du, McGuffin et al., 1998). Doa mutant males made fewer copulation attempts and success with both control and Doa mutant females (Fumey and Wicker-Thomas 2017). Nevertheless, how different kinases regulate sexual development and behaviors is still rarely known.
To systematically investigate the functions of protein kinases in sexual development and sexual behavior, we screened 177 kinase RNAi lines and identified several kinases, such as Akt and Prpk (p53-related protein kinase, encoded by Tcs5), that are crucial in dsx-expressing cells for sexual development. We also identified two kinases, PKA and CASK, that regulate virgin female receptivity. We further found that PKA and CASK promote virgin female receptivity by regulating the neuronal projection of ∼5 pairs of pC1 neurons during development and modulating the neuronal physiology of these neurons during adulthood. Our results reveal how specific protein kinases are involved in sexual development and behavior.
Results
Identification of Specific Protein Kinases in Sexual Development and Behavior
To explore the functions of protein kinases in regulating sexual development and sexual behavior, we performed an RNAi screen to knock down the expression of different kinases driven by dsxGAL4 in all dsx-expressing cells. We found a small number of kinases (13 out of 177) that were required in dsx-expressing cells for sexual development. Among them, 9 kinases were required in both sexes and 4 kinases were required only in males for the development of external sexual morphology (Figures 1A,B). Knocking down most kinases (162 out of 177) in dsx-expressing cells did not result in obvious changes in external sexual morphology in either sex (Figures 1A,B).
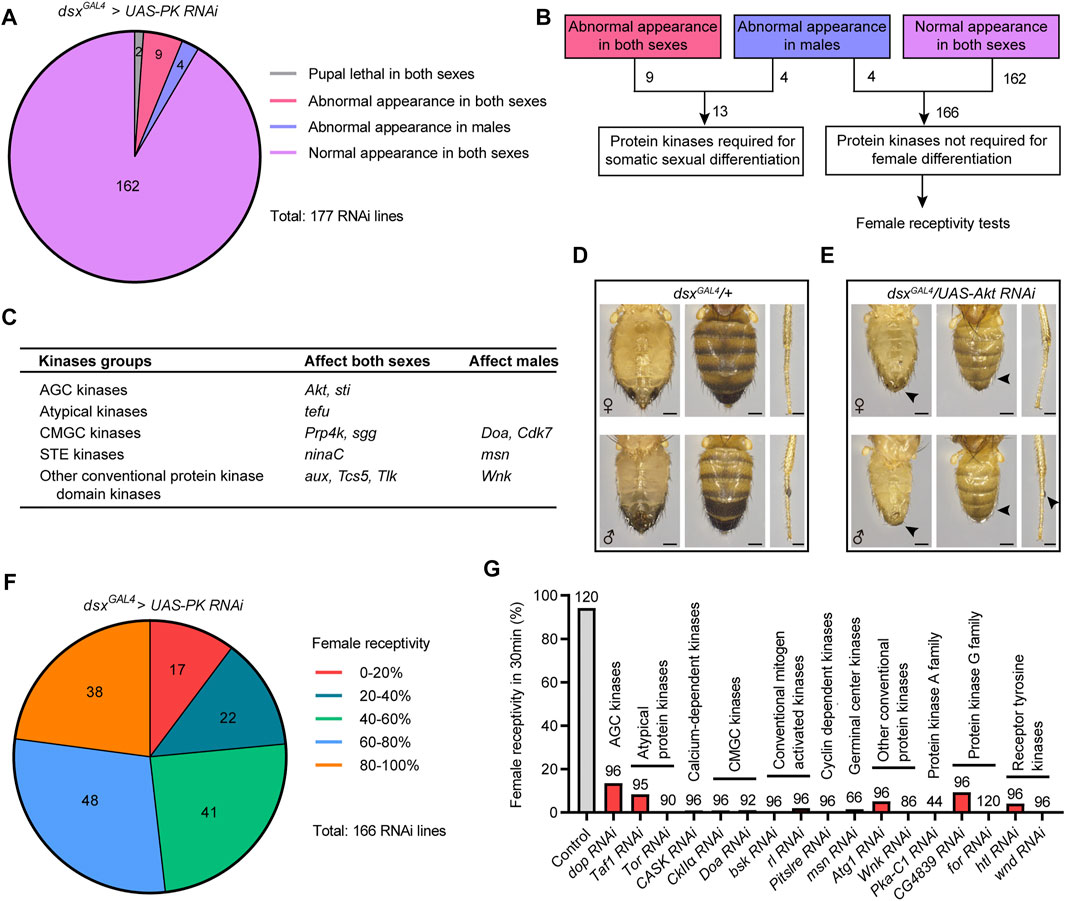
FIGURE 1. Specific kinases are required for sexual development and female receptivity. (A) Statistical results of screening for developmental defects of sex traits. The number in the colored graph represents the number of different RNAi lines targeting protein kinases. (B) Protein kinases were divided into two categories, one crucial for somatic sexual development, and the other not required for female differentiation, which were further assayed for virgin female receptivity. (C) A summary of protein kinases that are required for somatic sexual development. (D,E) Knockdown of Akt in dsx-expressing cells led to developmental defects in external genitalia, abdominal cuticular pigmentation (scale bar, 0.2 mm) and sex comb (scale bar, 0.1 mm), as indicated by arrowheads. (F) Statistical results of screening for unreceptive females. The number in the colored graph represents the number of different RNAi lines targeting protein kinases. (G) Knockdown of 17 protein kinases in dsx-expressing cells severely reduced female receptivity. The number at the top of the bar indicates the number of tested flies. The genotype of the control group was dsxGAL4/+; and other genotypes were abbreviations of specific kinase RNAi driven by dsxGAL4.
The kinases required for somatic sexual differentiation belong to different groups of protein kinases, including AGC kinases, atypical kinases, CMGC kinases, STE kinases, and other conventional protein kinase domain kinases (Figure 1C). Knocking down the expression of specific kinase resulted in different intersex phenotypes in flies, often with malformed external genitals and intermedial pigmentation of abdomens (Supplementary Figure S1). For example, when Akt was knocked down under the control of dsxGAL4, flies displayed similar intersex phenotypes between females (w−/yv; dsxGAL4/UAS-Akt RNAi) and males (w−/Y; dsxGAL4/UAS-Akt RNAi), including feminized external genitals and abdominal pigmentations in both sexes, and the significant reduction of sex combs in the forelegs of males (Figures 1D,E). These results suggest that specific kinases are crucial for somatic sexual development.
As above mentioned, knocking down 166 out of 177 protein kinases did not affect somatic sexual differentiation in females, but whether they would regulate female behaviors was unknown. Virgin female receptivity is one of the most important female behaviors that are crucial for reproduction. Thus, we initiated another screen of the 166 protein kinases to identify kinases involved in female receptivity (Figure 1B). We tested virgin receptivity in females with specific protein kinases knocked down in dsx-expressing cells over a 30 min period, and calculated the percentage of females successfully mated with males within the test. We found that a large number of kinases were crucial for virgin female receptivity: knocking down 80 kinases reduced female receptivity by ∼40%; in particular, knocking down 17 specific kinases reduced female receptivity by ∼80% (Figures 1F,G and Supplementary Figure S2). Based on the above screening results, we focused on these 17 candidate kinases that did not regulate sexual development but significantly reduced female receptivity after knockdown in dsx-expressing cells.
Identification of Protein Kinases That are Required in the Central Brain for Virgin Female Receptivity
dsxGAL4 is expressed in a variety of tissues with sexually dimorphic features or physiologies, such as the foreleg, cuticle, fat body, reproductive organs, and the CNS. To further investigate the functions of protein kinases in the CNS for female receptivity, we next targeted dsx neurons specifically in the brain using Otd-Flp expressing FLP specifically in the central brain (Otd-Flp; dsxGAL4,tub > GAL80>), hereafter referred to as dsxbrain neurons (Figure 2A). We asked whether knocking down the above 17 candidate protein kinases specifically in dsxbrain neurons would affect virgin female receptivity and found that knocking down 14 out of 17 kinases did not affect female receptivity, which suggests that these kinases may regulate female receptivity in dsx-expressing cells out of the central brain. Interestingly, knocking down CASK, msn or PKA in dsxbrain neurons significantly reduced female receptivity compared to control female flies (Figures 2B,C), but did not lead to obvious post-mating behaviors, which led us to focus on these three kinases for further study.
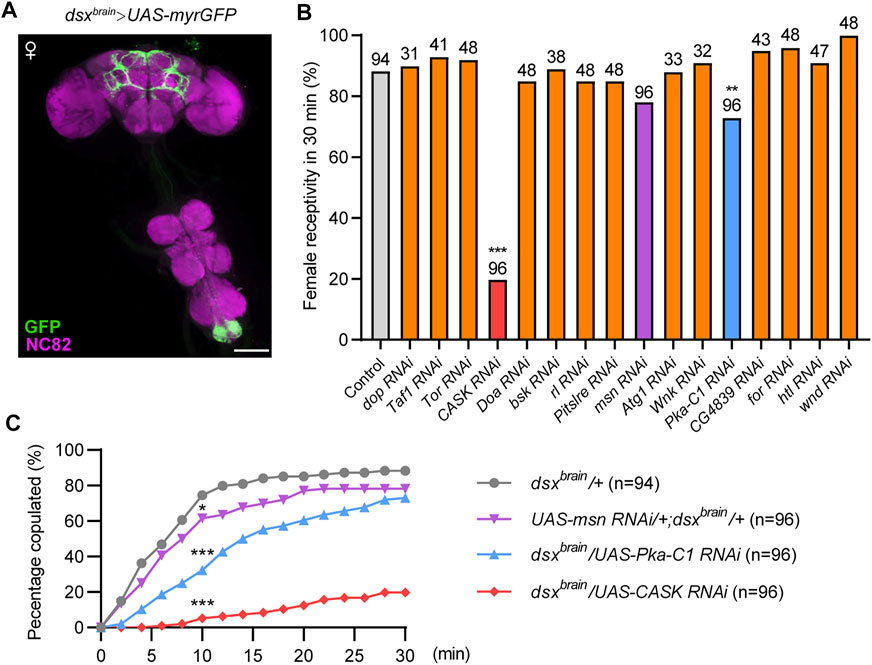
FIGURE 2. Identification of protein kinases that are required in dsxbrain neurons for virgin female receptivity. (A) Expression pattern of dsxbrain in female CNS. Scale bar, 100 μm. (B) Compared to the control, female receptivity was significantly decreased with specific kinases knocked down in dsxbrain neurons. The number at the top of the bar indicates the number of tested flies. The control genotype (dsxbrain/+) is Otd-Flp/+; dsxGAL4,tub > GAL80>/+, and other genotypes were abbreviations of specific kinase RNAi driven by dsxbrain. (C) Copulation rates were significantly decreased in virgin females with PKA, CASK or msn knocked down in dsxbrain neurons compared with control females. *p < 0.05 and ***p < 0.001 at 10 min time point, Chi-square test. The number in parentheses indicates the number of tested virgin females paired with wild-type males.
PKA and CASK Signaling in pC1 Neurons is Essential for Virgin Female Receptivity
Previous findings identified a small number of dsx-expressing pC1 neurons in the central brain for virgin female receptivity. To further investigate whether the three kinases would function in pC1 neurons for female receptivity, we knocked down CASK, msn or PKA using a previously generated split-GAL4 driver pC1-SS2, which specifically labels five pC1 cells (Figure 3A). We found that knockdown of PKA or CASK in pC1 neurons resulted in a dramatic reduction in female receptivity, while knockdown of msn did not affect female receptivity, compared to control females (Figure 3B). The more severe reduction of female receptivity by knocking down PKA or CASK in pC1 neurons than that in all dsxbrain neurons may be due to a stronger expression in pC1 neurons by the pC1-SS2 driver.
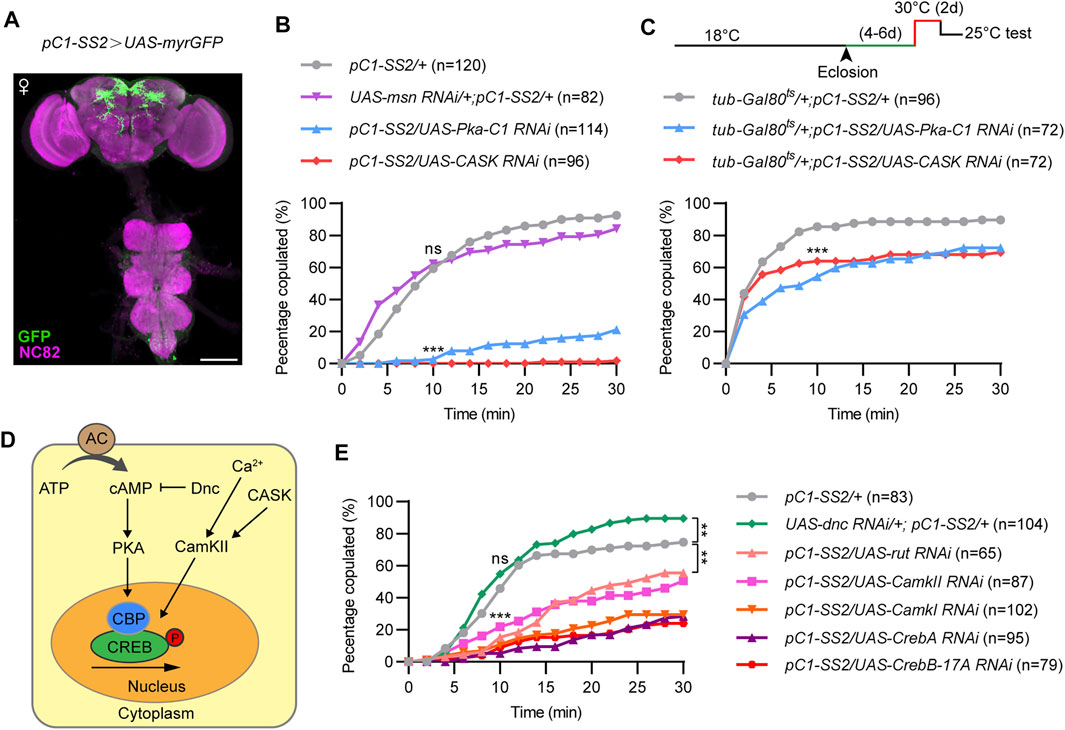
FIGURE 3. PKA and CASK signaling in pC1 neurons is essential for virgin female receptivity. (A) Expression pattern of pC1-SS2 in female CNS. Scale bar, 100 μm. (B) Compared to control females, copulation rates were significantly decreased in virgin females with PKA or CASK knocked down in pC1 neurons, whereas copulation rate was not significantly different in msn knockdown females. ns, not significant; ***p < 0.001 at 10 min time point, Chi-square test. (C) Knocking down PKA or CASK in 4–6 days old virgin females for 2 days significantly reduced female receptivity. ***p < 0.001 at 10 min time point, Chi-square test. (D) Diagram of the PKA and CASK signaling pathway. (E) Knockdown of other positive regulators, rather than the negative regulator (Dnc), of PKA and CASK signaling pathway significantly decreased virgin female receptivity. ns, not significant; ***p < 0.001 at 10 min time point. **p < 0.01 at 30 min time point, Chi-square test. The number in parentheses indicates the number of tested virgin females paired with wild-type males.
To further investigate whether PKA or CASK would be required in pC1 neurons during adulthood for female receptivity, we utilized the temperature sensitive GAL80ts that represses GAL4 activity at 18°C but not at 30°C as we previously used. We found that acutely knocking down PKA or CASK for 2 days during adulthood significantly reduced female receptivity by ∼20% (Figure 3C). These results indicate that PKA and CASK play acute roles in pC1 neurons during adulthood for female receptivity. That knocking down PKA or CASK during adulthood only moderately reduced female receptivity also suggested important roles of these kinases in pC1 neurons during development (see below).
PKA and CASK signaling are involved in the phosphorylation and activation of the transcription factor CREB, which regulates neuronal growth, neuronal differentiation, synaptic plasticity, spatial memory, as well as long-term memory formation. Thus, we tested whether other components of the PKA and CASK signaling pathway are involved in regulating virgin female receptivity, including the cAMP specific adenylyl cyclase (AC) rutabaga (rut), the cAMP specific phosphdiesterase dunce (dnc), CamK, and CREB (Figure 3D). As expected, knockdown of the molecules that positively regulate PKA or CASK signaling pathway significantly reduced female receptivity, while knockdown of dnc, a negative regulator, did not reduce, but instead slightly increased female receptivity (Figure 3E). Thus, the cAMP-PKA-CREB pathway is crucial in pC1 neurons to regulate female receptivity.
PKA and CASK Regulate Fine Projection of pC1 Neurons
The above results indicate that PKA and CASK may function both during development and adulthood in pC1 neurons for female receptivity. To determine whether PKA or CASK regulate the development of pC1 neurons, we examined the morphology of pC1 neurons in female flies with PKA or CASK knocked down. In the control females, there were five pC1 neurons in each hemisphere labeled by the pC1-SS2 driver, sending projections anterodorsally to the lateral junction of the lateral protocerebral complex and the superior-medial protecerebrum (SMP) (Figure 4A). These pC1 neurons also send projections vertically in the middle of the brain almost to the SOG region (arrows, Figure 4A), probably from the pC1d neurons based on previous studies. We found that pC1 neurons with PKA knockdown displayed abnormal neuronal projection, with the absence of vertical projection (arrows, Figure 4B) and the increase of nerve endings in the SMP region (arrowheads, Figure 4B). Similar deficits were observed in pC1 neurons with CASK knockdown (Figure 4C). We suspected that the missing of the vertical neuronal projection might be resulted from the decrease in number of pC1 neurons, e.g., the pC1d neurons, and analyzed the number of pC1 neurons. We found that the number of pC1 cells was still about five in each hemisphere after knocking down PKA or CASK (Figure 4D). Thus, the loss of the vertical projection in pC1 neuron and the significant increased nerve endings in the SMP region (Figure 4E) were defects of pC1 neuronal projections. These results indicate that PKA and CASK regulate fine neuronal projections of pC1 neurons to promote female receptivity.
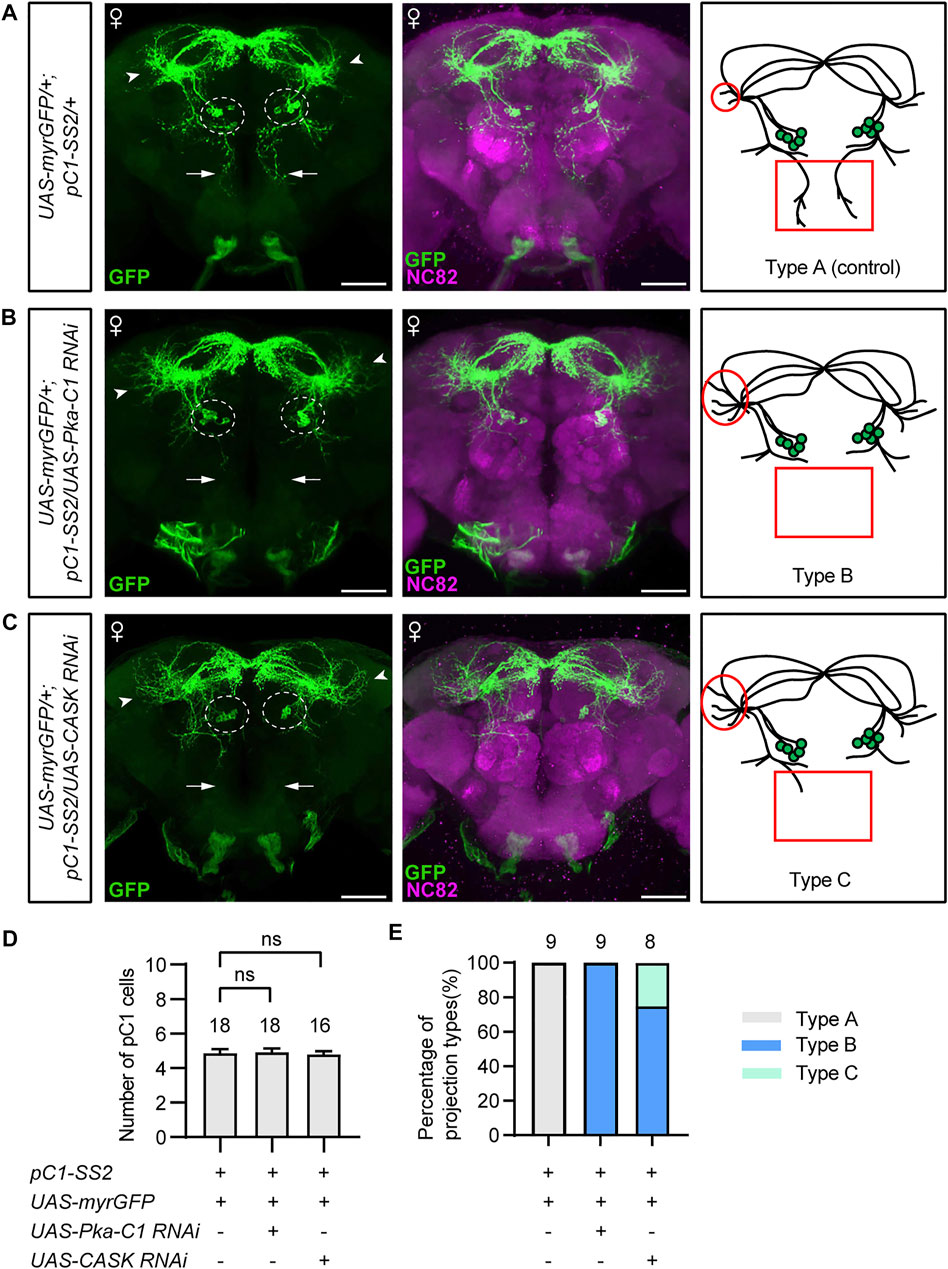
FIGURE 4. PKA and CASK regulate fine neuronal projection of pC1 neurons. (A–C) Morphology of pC1 neurons of different genotypes. Compared with the control (A), pC1 neurons with PKA knockdown (B) or CASK knockdown (C) exhibited unchanged number of pC1 cell bodies (dashed circles), loss of the vertical projection (arrows in the left panels, red rectangles in the right panels), and increase of the nerve endings in SMP region (arrowheads in the left panels, red circles in the right panels). Scale bars, 50 μm. (D) pC1 cell numbers per hemisphere were not affected by knocking down PKA or CASK. n = 18, 18 and 16, respectively from left to right. ns, not significant, Mann-Whitney U test. Error bars indicate SEM. (E) PKA or CASK knockdown resulted in significant projection defects of pC1 neurons. Type A: regular morphology of pC1 neurons; type B: loss of the vertical projection and increase of the nerve endings in SMP region; type C: weak/unilateral vertical projection and increase of the nerve endings in SMP region. n = 9, 9 and 8, respectively from left to right.
Taken together, we have identified specific protein kinases that regulate sexual development and virgin female receptivity of Drosophila. On the one hand, several kinases, such as Akt, are required in dsx-expressing somatic cells to regulate the external sexual morphology (Figure 5A); on the other hand, PKA, and CASK promote receptivity in virgin females by regulating both neuronal projection and physiology of pC1 neurons in the central brain (Figure 5B).
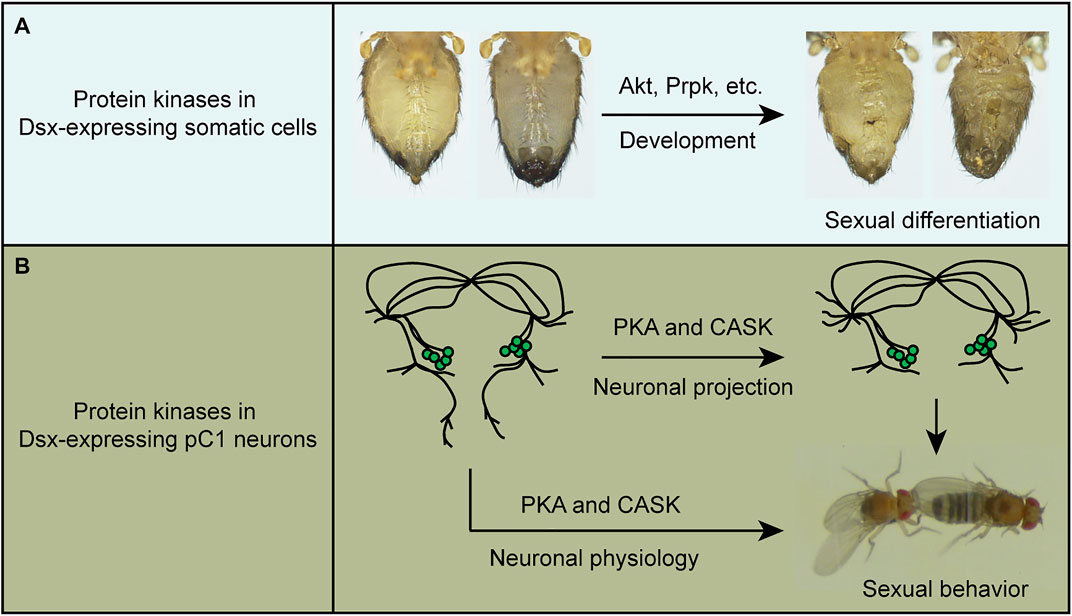
FIGURE 5. A summary of the functions of protein kinases in sexual development and behavior. (A) Protein kinases such as Akt and Prpk are crucial in Dsx-expressing cells for sexual differentiation. (B) PKA and CASK regulate both fine neuronal projection of pC1 neurons and their physiology to promote virgin female receptivity.
Discussion
Previous studies have identified several protein kinases involved in sexual development. The JAK kinase Hop regulates expression of the X-linked signal element Upd, which promotes the expression of the sex determination gene Sxl (Avila and Erickson 2007). Besides, the kinase Doa phosphorylates Tra and Tra2 to ensure the proper alternative splicing of dsx in both sexes (Du, McGuffin et al., 1998). It has been found that hypomorphic Doa mutant females, but not males, displayed subtle intersex phenotypes. However, knocking down Doa in dsx-expressing cells in our study caused a slight abnormality of the external genitals specifically in males. Such differences might be due to the nature of different Doa manipulations. In addition, we have identified Akt, Prpk, and a few other kinases required in sexual development. Knockdown of Akt not only led to developmental defect of external genitals in both sexes, but also resulted in significant reductions in abdominal pigmentation in both sexes such that the two sexes were difficult to distinguish. The decrease in pigmentation is similar to the phenotypes with perturbating InR and PI3K signaling, which depends on the Akt kinase activity (Shakhmantsir, Massad et al., 2014). Similarly, Prpk transduces the PI3K/TOR signaling to regulate its targets and plays important roles in organ and cell growth (Ibar, Cataldo et al., 2013). In addition to these previously identified pathways, our results also reveal a few other protein kinases that play important roles in the regulation of the sexual development. Among the identified kinases, 4 out of 13 specifically regulate male differentiation. However, we used only one RNAi line for each gene in the screen, and it is possible that the developmental defects were caused by potential off-target of RNAi. Future studies would further test how these kinases regulate sexual development in one or both sexes.
It has been found in rodents that tropomyosin receptor kinase B (TrkB) modulates male sexual motivation (Hawley and Mosura 2019), and the activities of PKA and extracellular signal-regulated kinase (ERK) in distinct brain regions regulate the rapid behavioral shift in male mating reactions (Goto, Nakahara et al., 2015). In Drosophila, loss of PKA-C3 or Doa also causes copulation defects in male flies (Fumey and Wicker-Thomas 2017; Cassar, Sunderhaus et al., 2018). However, the role of kinases in female mating behavior is rarely known. We identified that PKA and CASK function in ∼5 pairs of pC1 neurons in the central brain to promote virgin female receptivity. There are at least two categories of function by PKA and CASK. First, we found that PKA and CASK regulate fine projections of pC1 neurons to promote female receptivity. PKA has been found to regulate the fundamental CNS functions including neuronal survival, axonal outgrowth, neuronal development and cognition (Khan, Kulasiri et al., 2021). Our results are generally consistent with these findings regarding to neuronal development. The loss of vertical projection in pC1 neurons in females with PKA or CASK knocked down is likely due to the single pair of pC1d neurons from previous studies on the morphology of single pC1a-e neurons (Wang, Wang et al., 2020). PKA and CASK may specifically modulate the development of pC1d but not other pC1 neurons. Alternatively, PKA and CASK also regulate the development of other pC1 neurons, but the morphological change is trivial and not obviously seen in our study. Second, PKA and CASK also acutely modulate pC1 physiology to promote female receptivity, as knocking down PKA or CASK for 2 days just before behavioral assays significantly reduced female receptivity. pC1 neurons have been found to regulate several vital functions including virgin female receptivity, egg-laying decision, and aggression (Wang, Wang et al., 2020; Chiu, Hoopfer et al., 2021; Wang, Wang et al., 2021). That PKA and CASK acutely modulate pC1 function provides a molecular pathway that fine tunes the robustness of female sexual and aggressive behaviors.
How kinases function in sexual development and behavior? Regarding to the regulation of sexual development, we speculate that Dsx may be phosphorylated by specific kinases to regulate its function as transcription factor, but the kinases that would phosphorylate Dsx are unknown. In vitro phosphorylation experiments would confirm whether Dsx is phosphorylated by specific kinases. Regarding to the regulation of sexual behavior, kinases may function in a general way to affect the formation and activity of neural circuits, like functions of PKA and CASK in pC1 neurons. We speculate that PKA and CASK may act on the transcription factor CREB through different signaling pathways to regulate the neuronal projection and physiology of pC1 neurons. Future studies would testify how the cAMP-PKA-CREB pathway regulates the activity of pC1 neurons, possibly by phosphorylating proteins involved in neurotransmission, to acutely adjust female sexual behaviors.
Materials and Methods
Fly Stocks
Key resources used in this study, such as antibodies and fly stocks, are listed as Table 1. All flies used in this study were raised at 25°C and in 60% humidity with a 12 h light/12 h dark cycle. dsxGAL4 (Rideout, Dornan et al., 2010), Otd-Flp (Asahina, Watanabe et al., 2014), pC1-SS2 (Wang, Wang et al., 2020) and UAS-myrGFP (Pfeiffer, Ngo et al., 2010) was used as described previously. tub-GAL80ts (BDSC_7019) and tub > GAL80> (BDSC_38881) were from Bloomington Drosophila Stock Center. The UAS-for RNAi2dsf07 (Figures 1G, 2B) was used in the previous study (Peng, Wang et al., 2016). Other RNAi lines used in this study were obtained from the Tsinghua Fly Center at Tsinghua University, with the detailed information listed in the Supplementary Table S1.
Female Receptivity Assay
Tester virgin females and 4–6 days old wild-type virgin males were gently aspirated into two layers of the round courtship chambers (diameter: 10 mm; height: 3 mm per layer) and separated by a transparent film between the layers. After about 1 h at 25°C, the film was removed, and behavior was recorded by camera for 30 min. Female receptivity was measured every 2 min as the cumulative percentage of females engaging in copulation within 30 min (Figures 2C, 3B,C,E). Female receptivity at 30 min point was presented in Figures 1G, 2B and Supplementary Figure S2.
Tissue Imaging
For visualizing the morphological appearances of flies, the wings and legs of 3–5 days old flies with control genotype or specific kinase knocked down were removed under a microscope. The abdomens and the forelegs (Figures 1D,E and Supplementary Figure S1) were captured by a Nikon ShuttlePix P-400R digital microscope.
Immunostaining and Imaging
Brains of flies were dissected in Schneider’s insect medium (Thermo Fisher Scientific, Waltham, MA) and fixed in 4% paraformaldehyde (PFA) in phosphate-buffered saline (PBS) for 1 h at 4°C. After washing four times in PBST (0.5% Triton X-100 in PBS), Brains were blocked with 3% normal goat serum (NGS) in PBST for 60 min, then incubated with primary antibody diluted in 3% NGS for circa 24 h at 4°C. After washed four times in PBST, Brains were incubated with secondary antibodies diluted in 3% NGS for circa 24 h at 4°C. Primary antibodies used: rabbit polyclonal anti-GFP antibody (1:1000; A-11122, Thermo Fisher Scientific) and mouse monoclonal anti-Bruchpilot antibody (1:50; nc82, DSHB). Secondary antibody used: donkey anti-rabbit IgG conjugated to Alexa 488 (1:500, A21206, Invitrogen) and donkey anti-mouse IgG conjugated to Alexa 555 (1:500, A31570, Invitrogen). Brains were then washed four times in PBST and mounted in Vectorshield (Vector Laboratories H-1000). Stacks of images were obtained by a Zeiss 700 confocal microscope and processed with ImageJ (National Institutes of Health).
Statistical Analyses
Experimental flies and genetic controls were tested at the same condition. Statistical analysis was performed using GraphPad Prism 8 and indicated inside each figure legend. For female receptivity assay, Chi-square tests were performed to compare two different groups. Mann-Whitney U test was performed for pairwise comparisons of the number of pC1 cells. For the quantification of pC1 projection defects, three different types were defined as type A (regular morphology of pC1 neurons), type B (loss of the vertical projection and increase of the nerve endings in SMP region) and type C (weak/unilateral vertical projection and increase of the nerve endings in SMP region). Type B and C were never observed in control females.
Data Availability Statement
The original contributions presented in the study are included in the article/Supplementary Material, further inquiries can be directed to the corresponding author.
Author Contributions
HZ and QP performed the initial RNAi screen experiment. JC, XS, and QP performed additional behavioral experiments. JC and RW performed tissue dissection, immunochemistry, and confocal microscopy imaging. QP, ZR, and YP conceived and supervised the project. QP and YP wrote the manuscript with comments from all authors.
Funding
This work was supported by grants from National Natural Science Foundation of China (31700905) and Jiangsu Innovation and Entrepreneurship Team Program.
Conflict of Interest
The authors declare that the research was conducted in the absence of any commercial or financial relationships that could be construed as a potential conflict of interest.
Publisher’s Note
All claims expressed in this article are solely those of the authors and do not necessarily represent those of their affiliated organizations, or those of the publisher, the editors and the reviewers. Any product that may be evaluated in this article, or claim that may be made by its manufacturer, is not guaranteed or endorsed by the publisher.
Supplementary Material
The Supplementary Material for this article can be found online at: https://www.frontiersin.org/articles/10.3389/fcell.2022.923171/full#supplementary-material
References
Ardito, F., Giuliani, M., Perrone, D., Troiano, G., and Muzio, L. L. (2017). The Crucial Role of Protein Phosphorylation in Cell Signaling and its Use as Targeted Therapy (Review). Int. J. Mol. Med. 40 (2), 271–280. doi:10.3892/ijmm.2017.3036
Asahina, K., Watanabe, K., Duistermars, B. J., Hoopfer, E., González, C. R., Eyjólfsdóttir, E. A., et al. (2014). Tachykinin-expressing Neurons Control Male-specific Aggressive Arousal in Drosophila. Cell 156 (1-2), 221–235. doi:10.1016/j.cell.2013.11.045
Avila, F. W., and Erickson, J. W. (2007). Drosophila JAK/STAT Pathway Reveals Distinct Initiation and Reinforcement Steps in Early Transcription of Sxl. Curr. Biol. 17 (7), 643–648. doi:10.1016/j.cub.2007.02.038
Baker, B. S., and Ridge, K. A. (1980). Sex and the Single Cell I on the Action of Major Loci Affecting Sex Determination in Drosophila melanogaster. Genetics 94 (2), 383–423. doi:10.1093/genetics/94.2.383
Borovac, J., Bosch, M., and Okamoto, K. (2018). Regulation of Actin Dynamics during Structural Plasticity of Dendritic Spines: Signaling Messengers and Actin-Binding Proteins. Mol. Cell. Neurosci. 91, 122–130. doi:10.1016/j.mcn.2018.07.001
Burtis, K. C., and Baker, B. S. (1989). Drosophila Doublesex Gene Controls Somatic Sexual Differentiation by Producing Alternatively Spliced mRNAs Encoding Related Sex-specific Polypeptides. Cell 56 (6), 997–1010. doi:10.1016/0092-8674(89)90633-8
Cassar, M., Sunderhaus, E., Wentzell, J. S., Kuntz, S., Strauss, R., and Kretzschmar, D. (2018). The PKA-C3 Catalytic Subunit Is Required in Two Pairs of Interneurons for Successful Mating of Drosophila. Sci. Rep. 8 (1), 2458. doi:10.1038/s41598-018-20697-3
Chiu, H., Hoopfer, E. D., Coughlan, M. L., Pavlou, H. J., Goodwin, S. F., and Anderson, D. J. (2021). A Circuit Logic for Sexually Shared and Dimorphic Aggressive Behaviors in Drosophila. Cell 184 (3), 847. doi:10.1016/j.cell.2021.01.021
Du, C., McGuffin, M. E., Dauwalder, B., Rabinow, L., and Mattox, W. (1998). Protein Phosphorylation Plays an Essential Role in the Regulation of Alternative Splicing and Sex Determination in Drosophila. Mol. Cell 2 (6), 741–750. doi:10.1016/s1097-2765(00)80289-0
Fumey, J., and Wicker-Thomas, C. (2017). Mutations at the Darkener of Apricot Locus Modulate Pheromone Production and Sex Behavior in Drosophila melanogaster. J. Insect Physiology 98, 182–187. doi:10.1016/j.jinsphys.2017.01.005
Giese, K. P., and Mizuno, K. (2013). The Roles of Protein Kinases in Learning and Memory. Learn. Mem. 20 (10), 540–552. doi:10.1101/lm.028449.112
Gillespie, J. M., and Hodge, J. J. L. (2013). CASK Regulates CaMKII Autophosphorylation in Neuronal Growth, Calcium Signaling, and Learning. Front. Mol. Neurosci. 6, 27. doi:10.3389/fnmol.2013.00027
Goto, A., Nakahara, I., Yamaguchi, T., Kamioka, Y., Sumiyama, K., Matsuda, M., et al. (2015). Circuit-dependent Striatal PKA and ERK Signaling Underlies Rapid Behavioral Shift in Mating Reaction of Male Mice. Proc. Natl. Acad. Sci. U.S.A. 112 (21), 6718–6723. doi:10.1073/pnas.1507121112
Hawley, W. R., and Mosura, D. E. (2019). Sexual Motivation in Male Rats Is Modulated by Tropomyosin Receptor Kinase B (TrkB). Behav. Neurosci. 133 (1), 32–38. doi:10.1037/bne0000286
Humphrey, S. J., James, D. E., and Mann, M. (2015). Protein Phosphorylation: A Major Switch Mechanism for Metabolic Regulation. Trends Endocrinol. Metabolism 26 (12), 676–687. doi:10.1016/j.tem.2015.09.013
Ibar, C., Cataldo, V. F., Vásquez-Doorman, C., Olguín, P. Á., and Glavic, A. (2013). Drosophila p53-related Protein Kinase Is Required for PI3K/TOR Pathway-dependent Growth. Development 140 (6), 1282–1291. doi:10.1242/dev.086918
Ito, H., Fujitani, K., Usui, K., Shimizu-Nishikawa, K., Tanaka, S., and Yamamoto, D. (1996). Sexual Orientation in Drosophila Is Altered by the Satori Mutation in the Sex-Determination Gene Fruitless that Encodes a Zinc Finger Protein with a BTB Domain. Proc. Natl. Acad. Sci. U.S.A. 93 (18), 9687–9692. doi:10.1073/pnas.93.18.9687
Kulasiri, D., Khan, R., and Samarasinghe, S. (2021). Functional Repertoire of Protein Kinases and Phosphatases in Synaptic Plasticity and Associated Neurological Disorders. Neural Regen. Res. 16 (6), 1150–1157. doi:10.4103/1673-5374.300331
Lee, G., Foss, M., Goodwin, S. F., Carlo, T., Taylor, B. J., and Hall, J. C. (2000). Spatial, Temporal, and Sexually Dimorphic Expression Patterns of The Fruitless Gene in the Drosophila Central Nervous System. J. Neurobiol. 43 (4), 404–426. doi:10.1002/1097-4695(20000615)43:4<404::aid-neu8>3.0.co;2-d
Lee, G., Hall, J. C., and Park, J. H. (2002). Doublesex Gene Expression in the Central Nervous System of Drosophila melanogaster. J. Neurogenetics 16 (4), 229–248. doi:10.1080/01677060216292
Lee, P.-T., Lin, G., Lin, W.-W., Diao, F., White, B. H., and Bellen, H. J. (2018). A Kinase-dependent Feedforward Loop Affects CREBB Stability and Long Term Memory Formation. Elife 7, e33007. doi:10.7554/eLife.33007
Machado Almeida, P., Lago Solis, B., Stickley, L., Feidler, A., and Nagoshi, E. (2021). Neurofibromin 1 in Mushroom Body Neurons Mediates Circadian Wake Drive through Activating cAMP-PKA Signaling. Nat. Commun. 12 (1), 5758. doi:10.1038/s41467-021-26031-2
Manning, G., Plowman, G. D., Hunter, T., and Sudarsanam, S. (2002). Evolution of Protein Kinase Signaling from Yeast to Man. Trends Biochem. Sci. 27 (10), 514–520. doi:10.1016/s0968-0004(02)02179-5
Manoli, D. S., Foss, M., Villella, A., Taylor, B. J., Hall, J. C., and Baker, B. S. (2005). Male-specific Fruitless Specifies the Neural Substrates of Drosophila Courtship Behaviour. Nature. 436 (7049), 395–400. doi:10.1038/nature03859
Mele, S., and Johnson, T. K. (2019). Receptor Tyrosine Kinases in Development: Insights from Drosophila. Ijms. 21 (1), 188. doi:10.3390/ijms21010188
Palavicino-Maggio, C. B., Chan, Y.-B., McKellar, C., and Kravitz, E. A. (2019). A Small Number of Cholinergic Neurons Mediate Hyperaggression in Female Drosophila. Proc. Natl. Acad. Sci. U.S.A. 116 (34), 17029–17038. doi:10.1073/pnas.1907042116
Pan, Y., and Baker, B. S. (2014). Genetic Identification and Separation of Innate and Experience-dependent Courtship Behaviors in Drosophila. Cell. 156 (1-2), 236–248. doi:10.1016/j.cell.2013.11.041
Peng, Q., Wang, Y., Li, M., Yuan, D., Xu, M., Li, C., et al. (2016). cGMP-Dependent Protein Kinase Encoded by Foraging Regulates Motor Axon Guidance in Drosophila by Suppressing Lola Function. J. Neurosci. 36 (16), 4635–4646. doi:10.1523/JNEUROSCI.3726-15.2016
Pfeiffer, B. D., Ngo, T.-T. B., Hibbard, K. L., Murphy, C., Jenett, A., Truman, J. W., et al. (2010). Refinement of Tools for Targeted Gene Expression in Drosophila. Genetics. 186 (2), 735–755. doi:10.1534/genetics.110.119917
Rezával, C., Pattnaik, S., Pavlou, H. J., Nojima, T., Brüggemeier, B., D’Souza, L. A. D., et al. (2016). Activation of Latent Courtship Circuitry in the Brain of Drosophila Females Induces Male-like Behaviors. Curr. Biol. 26 (18), 2508–2515. doi:10.1016/j.cub.2016.07.021
Rideout, E. J., Dornan, A. J., Neville, M. C., Eadie, S., and Goodwin, S. F. (2010). Control of Sexual Differentiation and Behavior by the Doublesex Gene in Drosophila melanogaster. Nat. Neurosci. 13 (4), 458–466. doi:10.1038/nn.2515
Robinett, C. C., Vaughan, A. G., Knapp, J.-M., and Baker, B. S. (2010). Sex and the Single Cell. II. There Is a Time and Place for Sex. PLoS Biol. 8 (5), e1000365. doi:10.1371/journal.pbio.1000365
Ryner, L. C., Goodwin, S. F., Castrillon, D. H., Anand, A., Villella, A., Baker, B. S., et al. (1996). Control of Male Sexual Behavior and Sexual Orientation in Drosophila by the Fruitless Gene. Cell. 87 (6), 1079–1089. doi:10.1016/s0092-8674(00)81802-4
Sato, K., and Yamamoto, D. (2014). An Epigenetic Switch of the Brain Sex as a Basis of Gendered Behavior in Drosophila. Adv. Genet. 86, 45–63. doi:10.1016/B978-0-12-800222-3.00003-6
Shakhmantsir, I., Massad, N. L., and Kennell, J. A. (2014). Regulation of Cuticle Pigmentation In Drosophila by the Nutrient Sensing Insulin and TOR Signaling Pathways. Dev. Dyn. 243 (3), 393–401. doi:10.1002/dvdy.24080
Shirangi, T. R., Wong, A. M., Truman, J. W., and Stern, D. L. (2016). Doublesex Regulates the Connectivity of a Neural Circuit Controlling Drosophila Male Courtship Song. Dev. Cell. 37 (6), 533–544. doi:10.1016/j.devcel.2016.05.012
Skoulakis, E. M. C., Kalderon, D., and Davis, R. L. (1993). Preferential Expression in Mushroom Bodies of the Catalytic Subunit of Protein Kinase A and its Role in Learning and Memory. Neuron. 11 (2), 197–208. doi:10.1016/0896-6273(93)90178-t
Stockinger, P., Kvitsiani, D., Rotkopf, S., Tirián, L., and Dickson, B. J. (2005). Neural Circuitry that Governs Drosophila Male Courtship Behavior. Cell. 121 (5), 795–807. doi:10.1016/j.cell.2005.04.026
Villella, A., and Hall, J. C. (1996). Courtship Anomalies Caused by Doublesex Mutations in Drosophila melanogaster. Genetics. 143 (1), 331–344. doi:10.1093/genetics/143.1.331
Wang, F., Wang, K., Forknall, N., Patrick, C., Yang, T., Parekh, R., et al. (2020). Neural Circuitry Linking Mating and Egg Laying in Drosophila Females. Nature. 579 (7797), 101–105. doi:10.1038/s41586-020-2055-9
Wang, K., Wang, F., Forknall, N., Yang, T., Patrick, C., Parekh, R., et al. (2021). Neural Circuit Mechanisms of Sexual Receptivity in Drosophila Females. Nature. 589 (7843), 577–581. doi:10.1038/s41586-020-2972-7
Yu, J. Y., Kanai, M. I., Demir, E., Jefferis, G. S. X. E., and Dickson, B. J. (2010). Cellular Organization of the Neural Circuit that Drives Drosophila Courtship Behavior. Curr. Biol. 20 (18), 1602–1614. doi:10.1016/j.cub.2010.08.025
Keywords: protein kinase, PKA, Akt, CASK, female receptivity, sexual dimorphism, Drosophila
Citation: Chen J, Zhu H, Wang R, Su X, Ruan Z, Pan Y and Peng Q (2022) Functional Dissection of Protein Kinases in Sexual Development and Female Receptivity of Drosophila. Front. Cell Dev. Biol. 10:923171. doi: 10.3389/fcell.2022.923171
Received: 19 April 2022; Accepted: 13 May 2022;
Published: 09 June 2022.
Edited by:
Shun-Fan Wu, Nanjing Agricultural University, ChinaReviewed by:
Margaret Su-chun Ho, ShanghaiTech University, ChinaLuoying Zhang, Huazhong University of Science and Technology, China
Copyright © 2022 Chen, Zhu, Wang, Su, Ruan, Pan and Peng. This is an open-access article distributed under the terms of the Creative Commons Attribution License (CC BY). The use, distribution or reproduction in other forums is permitted, provided the original author(s) and the copyright owner(s) are credited and that the original publication in this journal is cited, in accordance with accepted academic practice. No use, distribution or reproduction is permitted which does not comply with these terms.
*Correspondence: Qionglin Peng, cGVuZ3Fpb25nbGluQHNldS5lZHUuY24=
†These authors have contributed equally to this work