Erratum: The consequences of assisted reproduction technologies on the offspring health throughout life: A placental contribution
- 1Faculty of Medicine, Institute of Biochemistry and Molecular Medicine, University of Bern, Bern, Switzerland
- 2Centre for Trophoblast Research, Department of Physiology, Development, and Neuroscience, University of Cambridge, Cambridge, United Kingdom
The use of assisted reproductive technologies (ART) worldwide has led to the conception and birth of over eight million babies since being implemented in 1978. ART use is currently on the rise, given growing infertility and the increase in conception age among men and women in industrialized countries. Though obstetric and perinatal outcomes have improved over the years, pregnancies achieved by ART still bear increased risks for the mother and the unborn child. Moreover, given that the first generation of ART offspring is now only reaching their forties, the long-term effects of ART are currently unknown. This is important, as there is a wealth of data showing that life-long health can be predetermined by poor conditions during intrauterine development, including irregularities in the structure and functioning of the placenta. In the current review, we aim to summarize the latest available findings examining the effects of ART on the cardiometabolic, cognitive/neurodevelopmental, and behavioral outcomes in the perinatal period, childhood and adolescence/adulthood; and to examine placental intrinsic factors that may contribute to the developmental outcomes of ART offspring. Altogether, the latest knowledge about life outcomes beyond adolescence for those conceived by ART appears to suggest a better long-term outcome than previously predicted. There are also changes in placenta structure and functional capacity with ART. However, more work in this area is critically required, since the potential consequences of ART may still emerge as the offspring gets older. In addition, knowledge of the placenta may help to foresee and mitigate any adverse outcomes in the offspring.
Introduction
Assisted reproductive technologies (ART) are increasingly used worldwide, to help couples conceive a child, most notably in Europe, where the largest number of ART treatments are performed (De Geyter, 2018; De Geyter et al., 2020). It is estimated that between 1978 - when the first in vitro fertilized child was born - and 2018, over eight million babies were born following ART worldwide (De Geyter, 2018; Sunderam et al., 2019). Though obstetric and perinatal outcomes have improved over the years through the advent of single embryo transfer (Hoyos and Ory, 2021), pregnancies achieved by ART still appear to bear increased risks for the mother and the unborn child (rewiewed in Qin et al., 2015, 2016). Whether the additional maternal and neonatal risks are due to the technology itself or underlying infertility-linked factors remains unresolved (Hoyos and Ory, 2021). When the obstetric and perinatal outcomes of ART and spontaneously conceived (SC) pregnancies were compared using the same mother as control, the results were similar (Ganer Herman et al., 2021), suggesting that health outcomes may be predominantly linked to the parents and not necessarily to ART (Hwang et al., 2018; Molinaro, 2021).
The phenomenon of an adverse in utero environment leading to the development of diseases later in life is well-known and referred to as the “developmental origin of health and disease” hypothesis. This states that the fetus undergoes adaptive changes to maintain homeostasis and to prepare the body for postnatal life. These adaptations depend on numerous factors, such as the type, length, and timing of the insult. Depending on their specific developmental windows, some organs may be programmed differently than others (Barker, 2012). To date, many conditions are believed to lead to fetal programming, including maternal under/overnutrition, smoking, physical inactivity, and psychological stress (Ravelli et al., 1999; Fleming et al., 2018; Lahti-Pulkkinen et al., 2018), preterm birth (Pandey et al., 2012; Qin et al., 2015; Ombelet et al., 2016; Qin et al., 2016; Hwang et al., 2018; Chang et al., 2020; Cochrane et al., 2020), gestational diabetes mellitus (GDM) (Chaveeva et al., 2011; Pandey et al., 2012; Qin et al., 2015, 2016; Mohammadi et al., 2020), preeclampsia (Chaveeva et al., 2011; Pandey et al., 2012; Qin et al., 2015, 2016; Almasi-Hashiani et al., 2019; Petersen et al., 2020), and infections (Fleming et al., 2018; Hwang et al., 2018; Lahti-Pulkkinen et al., 2018) (Figure 1). Gestational insults lead to a 2–10-fold increase in the susceptibility to cardiovascular disease (CVD), type-2 diabetes mellitus (T2DM), obesity, cognitive dysfunction, and developmental disorders (e.g., autism, Asperger’s, and Rett’s syndromes) (Godfrey and Barker, 2000; Jirtle and Skinner, 2007; Van Den Bergh, 2011; Reynolds and Caton, 2012). In addition, heritable environmentally-induced epigenetic modifications resulting from gestational insults may also be transmitted across generations (Jirtle and Skinner, 2007; Nilsson et al., 2018; Beck et al., 2022).
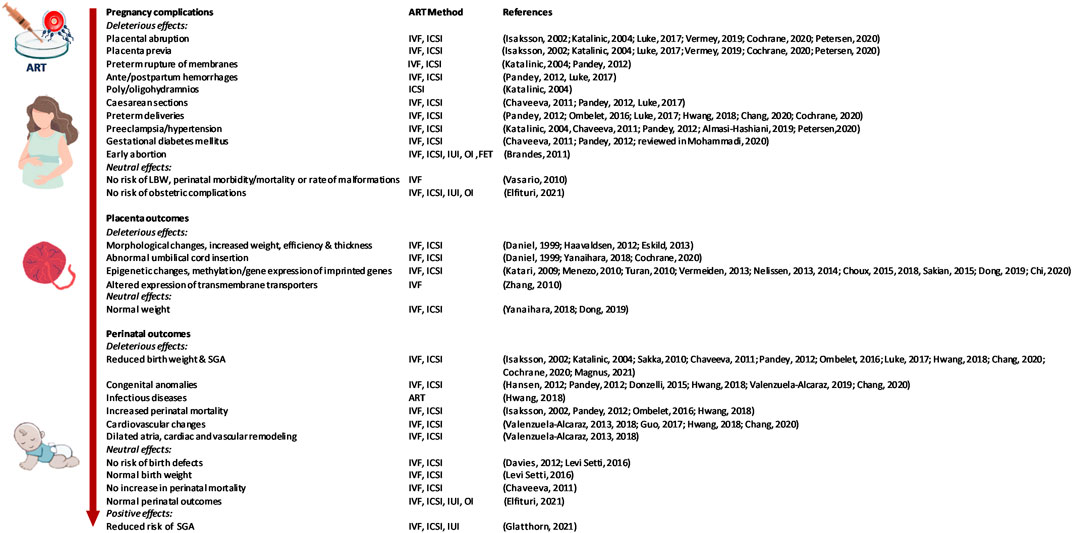
FIGURE 1. The effect of the use of ART on pregnancy complications, placental and fetal adaptations, and perinatal health outcomes. Legend: SGA: Small for Gestational Age. FET: Frozen Embryo Transfer. IUI: Intra-Uterine Insemination, OI: Ovulation Induction with Clomiphene citrate.
While the exact mechanisms underlying developmental programming for each disease are yet to be elucidated, the placenta is believed to play a crucial role in this process (Reynolds and Caton, 2012). Placental nutrient and oxygen supply are vital for fetal development, thus an alteration in placental structure or function is of key relevance for fetal programming. Under adverse environmental conditions, the placenta undergoes adaptive changes to optimize nutrient and oxygen transport to the fetus (Burton et al., 2016). Such adaptions include changes in placental blood flow, morphology, transporter expression, and alterations in the epigenetic profile (Barker et al., 2010a, 2010b, 2012; Eriksson et al., 2011; Barker and Thornburg, 2013). These may, in turn, be exacerbated in pregnancies achieved through ART, considering the increased risks for pregnancy complications compared to SC pregnancies, and that pregnancies achieved by ART imply more stress, due to the many procedures the mother and fetus endure (Lahti-Pulkkinen et al., 2018).
In the current review, we aim to summarize the available knowledge on the effects of ART on offspring cardiometabolic, cognitive/neurodevelopmental, and behavioral outcomes, and to examine placental morphological, functional and epigenetic factors that may contribute to the developmental outcomes of ART offspring in humans. ART includes procedures like in vitro fertilization (IVF), intra-cytoplasmatic sperm injection (ICSI), surgical sperm retrieval, ovarian hyperstimulation, embryo culture, and embryo freezing. In the current review, we will focus on data reporting the effects of IVF and ICSI, as these are the most used.
Perinatal Outcome in Newborns Conceived by ART
Many studies have investigated pregnancy outcomes, as well as short- (i.e. perinatal) and long-term (early life, adolescence, and young adulthood) outcomes in people born after ART procedures. Although most children born through ART are healthy, conception by ART has been linked to a variety of health complications and conflicting reports exist (See Figures 1, 2). For example, an increased risk for adverse perinatal outcomes including low birth weight (LBW) and small for gestational age (SGA) was shown when compared to SC pregnancies (Chaveeva et al., 2011; Pandey et al., 2012; Ombelet et al., 2016; Luke et al., 2017; Hwang et al., 2018; Chang et al., 2020; Cochrane et al., 2020). In contrast, a recent report found no differences and even reduced risk of SGA in ART offspring (Glatthorn et al., 2021). Findings pertaining birth weight were usually analyzed including the maternal weight/height as a covariate. Congenital anomalies also appear to be more prevalent in ART offspring compared to SC pregnancies (Hansen et al., 2012; Donzelli et al., 2015; Qin et al., 2016; Hwang et al., 2018; Valenzuela-Alcaraz et al., 2019; Chang et al., 2020), most notably for the cardiovascular (Valenzuela-Alcaraz et al., 2013, 2018, 2019), gastrointestinal and central nervous systems (Qin et al., 2015; Chang et al., 2020). Importantly, further studies reporting on birth defects showed these were linked to underlying infertility and not necessarily to ART, since children born to sub-fertile parents show similar health outcomes compared to ART conceived offspring (Davies et al., 2012; Levi Setti et al., 2016; Hwang et al., 2018). Finally, it has been suggested that ART may lead to an increased risk of cancer in the offspring, specifically for hematological malignancies, leukemia, neural and hepatic tumors (Hargreave et al., 2013; Wang et al., 2019). However, further studies showed no increased risk of cancer (Raimondi et al., 2005; Levi-Setti and Patrizio, 2018).
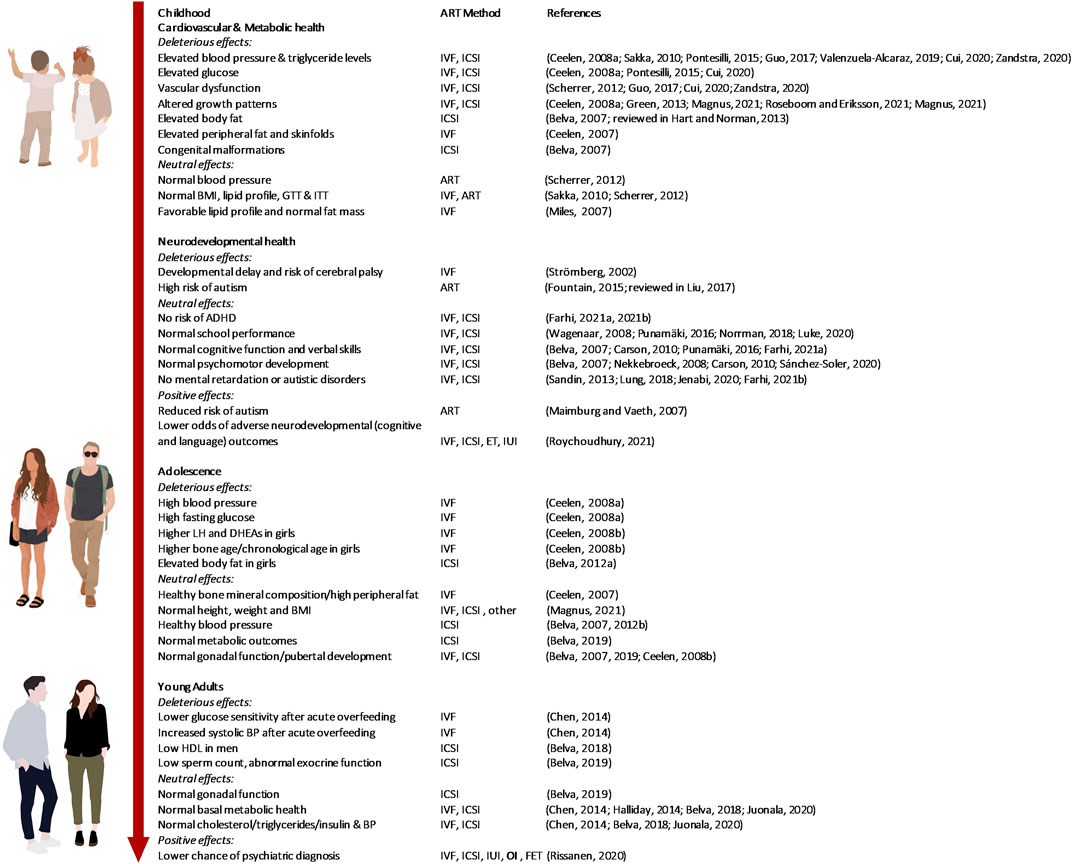
FIGURE 2. The effect of the use of ART on childhood, adolescent and adult health outcomes. Legend: LH: Luteinizing Hormone; DHEAS: Dehydroepiandrosterone sulfate; HDL: High-density lipoprotein; ADHD: Attention-deficit/hyperactivity disorder; BP: Blood pressure; BMI: Body Mass Index, GTT: Glucose Tolerance Test, ITT: Insulin Tolerance Test, ET: Embryo Transfer.
Metabolic and Cardiovascular Health of Children Conceived by ART
The cardiovascular changes observed among ART-conceived children starting at the age of 3 years include dilated atria, more globular ventricles, endothelial dysfunction, signs of systolic and diastolic dysfunction, and systemic and pulmonary hypertension (Sakka et al., 2010; Zhou et al., 2014; Liu et al., 2015; von Arx et al., 2015; Guo et al., 2017; Valenzuela-Alcaraz et al., 2019; Zandstra et al., 2020). At the age of 6–10 years, ART offspring also presented a higher risk for metabolic dysfunction, with elevated fasting glucose, insulin, and insulin resistance compared to SC children (Chen et al., 2014; Pontesilli et al., 2015; Guo et al., 2017; Cui et al., 2020). They also presented elevated body fat and skinfolds (Ceelen et al., 2007; Hart and Norman, 2013). In contrast, Scherrer et al reported no differences in lipid profile, basal glucose, glucose and insulin tolerance, blood pressure, or body mass index (BMI) in ART children compared to SC controls. However, they found general and pulmonary vascular dysfunction in a cohort of 11-year-old children born through ART (Scherrer et al., 2012). In addition, despite presenting similar gross body size, the growth patterns of ART children appear strikingly similar to those of SC children who develop T2DM and CVD later in life (Barker et al., 2005; Magnus et al., 2021; Roseboom and Eriksson, 2021). These adverse outcomes persist when looking at singleton ART pregnancies only and thus, cannot be explained by multiple pregnancies after the use of ART (Pandey et al., 2012; Qin et al., 2015; Ombelet et al., 2016; Qin et al., 2016) (Figure 2).
Cognitive and Neurodevelopmental Health of Newborns and Children Conceived by ART
ART children show similar neurological and psychomotor developmental faculties up to 3 years of age even when born preterm (Roychoudhury et al., 2021) compared with SC children (Nekkebroeck et al., 2008; Carson et al., 2010; Sánchez-Soler et al., 2020). Moreover, singletons and twins of both sexes conceived by ART perform as well as SC children once they reach school (Wagenaar et al., 2008; Punamäki et al., 2016; Norrman et al., 2018; Luke et al., 2020). Cognitive function, visual-motor ability, attention, and verbal skills of ART children were similar to SC children (Farhi et al., 2021a). Some reports suggested that ART does exert some negative influences on cognitive development. One study found a 4-fold higher risk of suspected developmental delay and cerebral palsy in IVF versus SC singletons. However, the effect disappeared when only twins were taken into account (Strömberg et al., 2002). Another study found a significant increase in the risk of mental retardation in ART children during their first year of life, but this association disappeared when the analysis was restricted to singletons (Sandin et al., 2013). There have been broad discussions about whether the use of ART is associated with the diagnosis of autism spectrum disorders (ASD) in the offspring. While most studies were unable to find any associations (Sandin et al., 2013; Lung et al., 2018; Jenabi et al., 2020; Farhi et al., 2021b), a few studies reported a significantly higher risk for ASD in ART offspring (Fountain et al., 2015; reviewed in Liu et al., 2017). However, the effect was substantially reduced when adverse prenatal and perinatal outcomes and demographics were taken into account (Fountain et al., 2015). Remarkably, a further study reported that ART children had a considerably lower risk of developing infantile autism compared to SC children (Maimburg and Vaeth, 2007). Altogether, the mental and sensory health outcomes and communication skills of children born through ART are reassuring and like that from SC pregnancies (Figure 2).
Effects of ART on Long-Term Health (Adolescents and Young Adults)
Given that the first generation of ART children is now only reaching their early forties, there are, to date, no studies investigating health outcomes in adults of older age, i.e., when developmental programming would be expected to manifest more robustly. However, a few recent studies reporting on the health of adolescents and young adults suggest the long-term health outcomes of ART may be less deleterious than anticipated. Accordingly, the early-life abnormalities in blood pressure, reported in ART children, disappeared by the time they reached adolescence. Fourteen-year-old boys and girls conceived by ICSI had comparable resting systolic and diastolic blood pressure as SC controls (Belva et al., 2007; 2012b). A further comprehensive investigation of metabolic syndrome in a cohort of 18–22-year-olds conceived through ART found similar outcomes to SC individuals for both sexes. Only high-density lipoprotein cholesterol concentrations were lower in ART men (Belva et al., 2018). Another recent study investigated various cardiovascular and metabolic outcomes in an ART cohort including 22–35-year-old men and women (Juonala et al., 2020) and reported no evidence of an altered risk factors, including markers of subclinical atherosclerosis. An investigation including 18–28-year-old adults from ART pregnancies self-reporting on their perceived current quality of life, BMI, pubertal development, and educational achievement found no differences when compared to SC adults (Halliday et al., 2014). Finally, Chen et al reported normal metabolic parameters in 20–21-year-old ART offspring. However, when exposed to a 3-day overfeeding protocol,, ART offspring showed increased systolic blood pressure and reduced peripheral glucose sensitivity, suggesting ART offspring may be at increased risk to develop metabolic diseases when challenged (Chen et al., 2014). In addition, increased peripheral adiposity (Ceelen et al., 2007) and higher systolic and diastolic blood pressure levels were reported in 8–18-year-old IVF offspring (Ceelen et al., 2008a).
Male adolescents conceived by ART showed normal endocrine gonadal function at puberty, but their sperm concentration and quality were significantly lower (Ceelen et al., 2008b; Belva et al., 2019). These findings suggest a possible impact of ART on multigenerational outcomes, which should be further investigated. Pubertal stage and age at menarche were similar in IVF and SC female adolescents, but IVF females presented elevated dehydroepiandrosterone and luteinizing hormone levels (Ceelen et al., 2008b) particularly if they were also SGA (Sakka et al., 2010), indicating impaired hypothalamic-pituitary-gonadal axis.
Finally, the risk of developing psychiatric disorders was studied in a Finish cohort, comparing ART and SC offspring from childhood until young adulthood (Rissanen et al., 2020). This study showed a modest increase in the likelihood of a general psychiatric diagnosis. In addition, ART children received their diagnoses on average 2 years earlier than SC children, probably because ART-treated individuals/couples might be more likely to seek medical help for their children. Of note, the reported effect disappeared with time, and ART young adults ended up displaying a lower cumulative incidence of psychiatric diagnoses than SC offspring regardless of sex (Rissanen et al., 2020).
In conclusion, while the knowledge about health outcomes beyond adolescence for those conceived by ART is still scarce, most of the findings are encouraging and suggest that the abnormalities reported in ART babies and children seem transitory, and are no longer observed when they become older (Halliday et al., 2014; Rissanen et al., 2020; Magnus et al., 2021) (Figure 2).
Intrauterine Mechanisms–Placental Programming in ART
Placental Morphology, Gene Expression and Function
In the case of ART, there are limited data available regarding morphological changes in the human placenta (Figure 1). Some studies reported increased placental weight along with reduced birthweight and, thus, an increased placental weight to birthweight ratio in ART compared to SC pregnancies (Daniel et al., 1999; Haavaldsen et al., 2012; Eskild et al., 2013). Increased placental thickness and elevated rate of abnormal cord insertion were also reported after ART (Daniel et al., 1999; Cochrane et al., 2020). In contrast, other groups were unable to detect any placental differences when comparing IVF and SC newborns (Yanaihara et al., 2018). Of note, many studies investigating morphological changes in the placenta after ART were performed using murine models. The outcomes of these studies with regard to the effects of ART on fetal and placental development have been discussed elsewhere (Hemberger et al., 2020).
Beyond morphological changes, abnormalities in gene expression that could reflect alterations in placental function have also been reported in ART. Using a selective twin-to-singleton fetal reduction strategy and collection of first-trimester placentas in vivo, Zhao et al showed that 1910 and 1,495 genes were up- and down-regulated, respectively in the placenta by IVF (Zhao et al., 2019). This included alterations in genes involved in biological pathways, like the immune response, transmembrane signaling, carbon, fatty acid and amino acid metabolism, cell cycle, stress control, invasion, and vascularization (Zhao et al., 2019). A second study investigated first-trimester samples from chorionic villus sampling and included a non-IVF fertility treatment group in addition to the IVF and SC groups. Herein the authors reported modest differences in the transcriptome and suggested that underlying infertility, in addition to treatment-related factors, might be key contributors to the observed gene expression abnormalities in the IVF group (Lee et al., 2019). Similarly, a recent study that compared first-trimester maternal plasma metabolomic profiles in women undergoing IVF, non-IVF fertility treatment, and SC (Sun et al., 2019) found elevated circulating levels of several lipid and lipid-related components (e.g., steroid metabolites, and lipids with docosahexaenoyl acyl chains, and acylcholines) in the infertile groups of women, especially when IVF was performed. Such changes may have consequences for placental lipid transfer and steroid hormone production, and may contribute to the adverse fetal outcomes associated with ART and infertility (Sun et al., 2019).
At term, IVF placentas show altered global gene expression, leading to an over-representation of certain biological pathways as observed in the first-trimester samples, such as immune response, transmembrane transport, cell cycle control, stress control, invasion, vascularization, and amino acid and cholesterol metabolism (Zhang et al., 2010). Several of the genes whose expression differed the most between IVF and the control groups have been implicated in chronic metabolic disorders like obesity and T2DM, supporting the theory that ART induces fetal programming of metabolic diseases and may do so via alterations in the placenta (Katari et al., 2009). In contrast, another study reported modest effects in the expression of 108 imprinted genes in the placenta (Litzky et al., 2017) and suggested that differences in gene expression are more likely associated with infertility rather than the IVF procedure itself.
Placental Epigenetics
ART procedures occur during a developmental time that is critical for epigenetic reprogramming. Hence, perturbations due to technical manipulations during this sensitive time may lead to changes in the epigenetic profile of the conceptus (Monk et al., 2019). This is relevant for the finely-tuned expression of imprinted genes, of which about 100 have been identified in humans. These genes are stimulated by fetal signals and have an impact on transplacental nutrient allocation, placental growth, and vascularization, directly affecting fetal growth and long-term health (Reik et al., 2003; Morison et al., 2005). They are a subset of epigenetically-regulated genes that are selectively expressed from the maternal or paternal allele (Thamban et al., 2020). Imprinted genes are, by definition, functionally haploid and are thereby potentially more susceptible to mutations (Fowden et al., 2011). Epigenetic modifications might occur either at the DNA level via methylation/hydroxymethylation, at the protein level via histone modifications, or at the mRNA level via short and long non-coding RNAs (Ghai and Kader, 2021). Imprinted genes are abundantly expressed in fetal and placental tissues, and DNA methylation of imprinted genes is established in a parent-specific manner during gametogenesis. Several studies highlighted an altered epigenetic status in gametes from infertile couples, raising the possibility of a heightened risk of imprinting defects and somatic epigenetic changes in ART-conceived children (Van Montfoort et al., 2012; Lazaraviciute et al., 2014; Choux et al., 2015, 2018; Cortessis et al., 2018). Specifically, the DNA methylation level of several imprinted genes was altered in ART compared to SC placentas (Van Montfoort et al., 2012; Choux et al., 2015, 2018). Furthermore, these differences were associated with gene expression differences at both imprinted and non-imprinted genes (Katari et al., 2009). Thus, aberrant methylation of imprinted genes may be an indicator of more global epigenetic instability (Denomme and Mann, 2012). Specifically, H19/IGF2, LINE-1Hs, ERVFRD-1, and KCNQ1OT1 are affected in ART through changes in placental DNA methylation (Turan et al., 2010; Nelissen et al., 2013; Choux et al., 2018; Dong et al., 2019). Expression of H19 is linked with fetal and placental growth suppression (Gao et al., 2012) and was significantly higher (Turan et al., 2010; Nelissen et al., 2013, 2014; Sakian et al., 2015; Chi et al., 2020), while IGF2 expression, which increases fetal and placental growth (Sakian et al., 2015; Chi et al., 2020) was significantly lower in ART compared with SC placentas (Nelissen et al., 2014; Sakian et al., 2015; Chi et al., 2020). However, changes in DNA methylation do not always correlate with alterations in transcriptional levels (Rancourt et al., 2012). A further study reported no significant differences in gene expression despite methylation changes between placentas from ART and SC pregnancies (Litzky et al., 2017). Finally, a recent longitudinal study that assessed genome-wide changes in DNA methylation in blood collected from newborns and adults conceived by ART showed that variations observed at birth largely resolved by the time offspring reached adulthood and found no evidence of any impact on development and general health (Novakovic et al., 2019).
An additional factor to consider when assessing impacts of ART is the medium used during embryo culture, since using media that lacks essential amino acids may affect placental DNA methylation and can cause aberrant imprinting in the embryo (Menezo et al., 2010; Eskild et al., 2013). Moreover, the available evidence further indicates that subfertility itself is a risk factor for imprinting diseases and that methylation errors are already present in sperm and oocytes. Thus, the unequivocal proof of a causal relationship between imprinted diseases and IVF or ICSI treatments is still lacking (Vermeiden and Bernardus, 2013) (Figure 1).
Conclusion
In the current review, we aimed to examine the latest available findings examining the effects of ART on behavioral and health related outcomes in the offspring throughout the lifespan, including the potential contribution of the placenta. It is hypothesized that ART may affect the development of gametes and embryo, and epigenetic adaptations aiming to protect the fetus may exacerbate vulnerability to diseases in the offspring. In fact, a combination of genetics, the intergenerational and the current environments in addition to the ART procedure are all involved in disease causation (Hochberg et al., 2011). The long-term effects remain to be seen once the first-generation of ART offspring reaches an older age (i.e. > 65), a time-point where fetal programming effects may still emerge.
Depending on later life outcomes, the need to identify those at risk from an early stage will be imperative to treat and prevent their development throughout the lifespan in individuals conceived by ART. Though it is difficult to establish the mechanisms underlying the changes observed among ART newborns and children, it is plausible that the placenta could play a key role in the process. Since placental size and shape are indicative of its efficiency and function, and the imprinted genes in the placenta appear to regulate nutrient allocation, the observed changes could cause potential epigenetic adaptations in the fetus that may further exacerbate disease susceptibility.
In addition, each step utilized in ART (i.e. ovarian stimulation, in vitro culture, culture media, cryopreservation technique) could represent a risk for the pregnancy (Palomba et al., 2016) and in turn, the placental response to environmental stress can further define the outcome for the offspring (Litzky and Marsit, 2019). E.g., the use of fresh versus thawed embryos in IVF can affect weight, height, and circulating growth factor and lipid profiles in the resulting children (Green et al., 2013). In addition, evolving laboratory procedures used for ART, and sometimes inappropriate choice of control groups, make comparisons between studies difficult. Since ART is predominantly used on infertile couples, distinguishing between the effects of ART procedures and those of underlying infertility is challenging. Further disregarded aspects that should be considered when assessing the long-term impacts of ART include, e.g. differences in lifestyle and the high anxiety and stress levels experienced by couples that are unable to conceive naturally (Litzky and Marsit, 2019).
Altogether, our work highlights the need for further study into the role of potential confounding factors when assessing the short- and long-term effects of ART for the offspring, and whether these effects could be passed to the next generation. While there is a need for additional studies to investigate the effects of ART on the offspring when they are >65 years, based on the currently available literature, ART offspring until around 40 years of age do not appear to be at greater risk of developing persistent life-long health complications.
Author Contributions
MS and GB wrote the article. AS and CA revised and commented on the article.
Funding
This work was supported by the Swiss National Science Foundation (Grant No. 310030_149958) and via the National Center of Competence in Research (NCCR) TransCure, University of Bern, Switzerland (Grant No. 51NF40-185544). CA and ASP were supported by the Sigrist Foundation. Open access funding provided by University Of Bern.
Conflict of Interest
The authors declare that the research was conducted in the absence of any commercial or financial relationships that could be construed as a potential conflict of interest.
Publisher’s Note
All claims expressed in this article are solely those of the authors and do not necessarily represent those of their affiliated organizations, or those of the publisher, the editors and the reviewers. Any product that may be evaluated in this article, or claim that may be made by its manufacturer, is not guaranteed or endorsed by the publisher.
References
Almasi-Hashiani, A., Omani-Samani, R., Mohammadi, M., Amini, P., Navid, B., Alizadeh, A., et al. (2019). Assisted Reproductive Technology and the Risk of Preeclampsia: An Updated Systematic Review and Meta-Analysis. BMC Pregnancy Childbirth 19, 1–13. doi:10.1186/s12884-019-2291-x
Barker, D. J., Larsen, G., Osmond, C., Thornburg, K. L., Kajantie, E., and Eriksson, J. G. (2012). The Placental Origins of Sudden Cardiac Death. Int. J. Epidemiol. 41, 1394–1399. doi:10.1093/ije/dys116
Barker, D. J. P. (2012). Developmental Origins of Chronic Disease. Public Health 126, 185–189. doi:10.1016/j.puhe.2011.11.014
Barker, D. J. P., Osmond, C., Forsén, T. J., Kajantie, E., and Eriksson, J. G. (2005). Trajectories of Growth Among Children Who Have Coronary Events as Adults. N. Engl. J. Med. 353, 1802–1809. doi:10.1056/nejmoa044160
Barker, D. J. P., Thornburg, K. L., Osmond, C., Kajantie, E., and Eriksson, J. G. (2010b). The Prenatal Origins of Lung Cancer. II. The Placenta. Am. J. Hum. Biol. 22, 512–516. doi:10.1002/AJHB.21041
Barker, D. J. P., Thornburg, K. L., Osmond, C., Kajantie, E., and Eriksson, J. G. (2010a). The Surface Area of the Placenta and Hypertension in the Offspring in Later Life. Int. J. Dev. Biol. 54, 525–530. doi:10.1387/ijdb.082760db
Barker, D. J. P., and Thornburg, K. L. (2013). Placental Programming of Chronic Diseases, Cancer and Lifespan: A Review. Placenta 34, 841–845. doi:10.1016/j.placenta.2013.07.063
Beck, D., Nilsson, E. E., Ben Maamar, M., and Skinner, M. K. (2022). Environmental Induced Transgenerational Inheritance Impacts Systems Epigenetics in Disease Etiology. Sci. Rep. 12, 5452. doi:10.1038/S41598-022-09336-0
Belva, F., Bonduelle, M., Provyn, S., Painter, R. C., Tournaye, H., Roelants, M., et al. (20182018). Metabolic Syndrome and its Components in Young Adults Conceived by ICSI. Int. J. Endocrinol. 2018, 1–8. doi:10.1155/2018/8170518
Belva, F., Bonduelle, M., and Tournaye, H. (2019). Endocrine and Reproductive Profile of Boys and Young Adults Conceived after ICSI. Curr. Opin. Obstet. Gynecol. 31, 163–169. doi:10.1097/GCO.0000000000000538
Belva, F., Henriet, S., Liebaers, I., Van Steirteghem, A., Celestin-Westreich, S., and Bonduelle, M. (2007). Medical Outcome of 8-Year-Old Singleton ICSI Children (Born >=32 Weeks' Gestation) and a Spontaneously Conceived Comparison Group. Hum. Reprod. 22, 506–515. doi:10.1093/HUMREP/DEL372
Belva, F., Painter, R., Bonduelle, M., Roelants, M., Devroey, P., and De Schepper, J. (2012a). Are ICSI Adolescents at Risk for Increased Adiposity? Hum. Reprod. 27, 257–264. doi:10.1093/HUMREP/DER375
Belva, F., Roelants, M., De Schepper, J., Roseboom, T. J., Bonduelle, M., Devroey, P., et al. (2012b). Blood Pressure in ICSI-Conceived Adolescents. Hum. Reprod. 27, 3100–3108. doi:10.1093/humrep/des259
Brandes, M., Verzijden, J. C. M., Hamilton, C. J. C. M., De Weys, N. P. C., De Bruin, J. P., Bots, R. S. G. M., et al. (2011). Is the Fertility Treatment Itself a Risk Factor for Early Pregnancy Loss? Reprod. Biomed. Online 22, 192–199. doi:10.1016/j.rbmo.2010.10.013
Burton, G. J., Fowden, A. L., and Thornburg, K. L. (2016). Placental Origins of Chronic Disease. Physiol. Rev. 96, 1509–1565. doi:10.1152/physrev.00029.2015
Carson, C., Kurinczuk, J. J., Sacker, A., Kelly, Y., Klemetti, R., Redshaw, M., et al. (2010). Cognitive Development Following ART: Effect of Choice of Comparison Group, Confounding and Mediating Factors. Hum. Reprod. 25, 244–252. doi:10.1093/HUMREP/DEP344
Ceelen, M., Van Weissenbruch, M. M., Roos, J. C., Vermeiden, J. P. W., Van Leeuwen, F. E., and Delemarre-van De Waal, H. A. (2007). Body Composition in Children and Adolescents Born Afterin VitroFertilization or Spontaneous Conception. J. Clin. Endocrinol. Metab. 92, 3417–3423. doi:10.1210/jc.2006-2896
Ceelen, M., Van Weissenbruch, M. M., Vermeiden, J. P. W., Van Leeuwen, F. E., and Delemarre-Van De Waal, H. A. (2008a). Cardiometabolic Differences in Children Born after In Vitro Fertilization: Follow-Up Study. J. Clin. Endocrinol. Metab. 93, 1682–1688. doi:10.1210/jc.2007-2432
Ceelen, M., Van Weissenbruch, M. M., Vermeiden, J. P. W., Van Leeuwen, F. E., and Delemarre-Van De Waal, H. A. (2008b). Pubertal Development in Children and Adolescents Born after IVF and Spontaneous Conception. Hum. Reprod. 23, 2791–2798. doi:10.1093/humrep/den309
Chang, H.-Y., Hwu, W.-L., Chen, C.-H., Hou, C.-Y., and Cheng, W. (2020). Children Conceived by Assisted Reproductive Technology Prone to Low Birth Weight, Preterm Birth, and Birth Defects: A Cohort Review of More Than 50,000 Live Births during 2011-2017 in Taiwan. Front. Pediatr. 8, 1–5. doi:10.3389/fped.2020.00087
Chaveeva, P., Carbone, I. F., Syngelaki, A., Akolekar, R., and Nicolaides, K. H. (2011). Contribution of Method of Conception on Pregnancy Outcome after the 11-13 Weeks Scan. Fetal diagn. Ther. 30, 9–22. doi:10.1159/000323921
Chen, M., Wu, L., Zhao, J., Wu, F., Davies, M. J., Wittert, G. A., et al. (2014). Altered Glucose Metabolism in Mouse and Humans Conceived by IVF. Diabetes 63, 3189–3198. doi:10.2337/db14-0103
Chi, F., Zhao, M., Li, K., Lin, A.-Q., Li, Y., and Teng, X. (2020). DNA Methylation Status of Imprinted H19 and KvDMR1 Genes in Human Placentas after Conception Using Assisted Reproductive Technology. Ann. Transl. Med. 8, 854. doi:10.21037/atm-20-3364
Choux, C., Binquet, C., Carmignac, V., Bruno, C., Chapusot, C., Barberet, J., et al. (2018). The Epigenetic Control of Transposable Elements and Imprinted Genes in Newborns Is Affected by the Mode of Conception: ART versus Spontaneous Conception without Underlying Infertility. Hum. Reprod. 33, 331–340. doi:10.1093/humrep/dex366
Choux, C., Carmignac, V., Bruno, C., Sagot, P., Vaiman, D., and Fauque, P. (2015). The Placenta: Phenotypic and Epigenetic Modifications Induced by Assisted Reproductive Technologies throughout Pregnancy. Clin. Epigenet 7, 1–20. doi:10.1186/s13148-015-0120-2
Cochrane, E., Pando, C., Kirschen, G. W., Soucier, D., Fuchs, A., and Garry, D. J. (2020). Assisted Reproductive Technologies (ART) and Placental Abnormalities. J. Perinat. Med. 48, 825–828. doi:10.1515/jpm-2020-0141
Cortessis, V. K., Azadian, M., Buxbaum, J., Sanogo, F., Song, A. Y., Sriprasert, I., et al. (2018). Comprehensive Meta-Analysis Reveals Association between Multiple Imprinting Disorders and Conception by Assisted Reproductive Technology. J. Assist. Reprod. Genet. 35, 943–952. doi:10.1007/s10815-018-1173-x
Cui, L., Zhou, W., Xi, B., Ma, J., Hu, J., Fang, M., et al. (2020). Increased Risk of Metabolic Dysfunction in Children Conceived by Assisted Reproductive Technology. Diabetologia 63, 2150–2157. doi:10.1007/s00125-020-05241-1
Daniel, Y., Schreiber, L., Geva, E., Amit, A., Pausner, D., Kupferminc, M. J., et al. (1999). Do placentae of Term Singleton Pregnancies Obtained by Assisted Reproductive Technologies Differ from Those of Spontaneously Conceived Pregnancies? ? 14, 1107–1110.doi:10.1093/humrep/14.4.1107
Davies, M. J., Moore, V. M., Willson, K. J., Van Essen, P., Priest, K., Scott, H., et al. (2012). Reproductive Technologies and the Risk of Birth Defects. N. Engl. J. Med. 366, 1803–1813. doi:10.1056/NEJMOA1008095
De Geyter, C., Wyns, C., Bergh, C., Calhaz-Jorge, C., De Geyter, C., Kupka, M. S., et al. (2020). ART in Europe, 2016: Results Generated from European Registries by ESHRE. Hum. Reprod. Open 2020, hoaa032–17. doi:10.1093/HROPEN/HOZ03810.1093/hropen/hoaa032
Denomme, M. M., and Mann, M. R. W. (2012). Genomic Imprints as a Model for the Analysis of Epigenetic Stability during Assisted Reproductive Technologies. Reproduction 144, 393–409. doi:10.1530/REP-12-0237
Dong, J., Wen, L., Guo, X., Xiao, X., Jiang, F., Li, B., et al. (2019). The Increased Expression of Glucose Transporters in Human Full-Term Placentas from Assisted Reproductive Technology without Changes of mTOR Signaling. Placenta 86, 4–10. doi:10.1016/j.placenta.2019.08.087
Donzelli, G., Carnesecchi, G., Amador, C., Di Tommaso, M., Filippi, L., Caporali, R., et al. (2015). Fetal Programming and Systemic Sclerosis. Am. J. Obstetrics Gynecol. 213, 839e1–839. doi:10.1016/j.ajog.2015.07.034
Elfituri, A., Bakker, W., Viswanatha, R., Robinson, E., Jan, H., and Ganapathy, R. (2021). Maternal and Perinatal Outcomes of Dichorionic Diamniotic Twins in Women after Spontaneous and Assisted Conception. Eur. J. Obstetrics Gynecol. Reproductive Biol. 263, 247–251. doi:10.1016/J.EJOGRB.2021.06.044
Eriksson, J. G., Kajantie, E., Thornburg, K. L., Osmond, C., and Barker, D. J. P. (2011). Mother's Body Size and Placental Size Predict Coronary Heart Disease in Men. Eur. Heart J. 32, 2297–2303. doi:10.1093/EURHEARTJ/EHR147
Eskild, A., Monkerud, L., and Tanbo, T. (2013). Birthweight and Placental Weight; Do Changes in Culture Media Used for IVF Matter? Comparisons with Spontaneous Pregnancies in the Corresponding Time Periods. Hum. Reprod. 28, 3207–3214. doi:10.1093/humrep/det376
Farhi, A., Gabis, L. V., Frank, S., Glasser, S., Hirsh-Yechezkel, G., Brinton, L., et al. (2021a). Cognitive Achievements in School-Age Children Born Following Assisted Reproductive Technology Treatments: A Prospective Study. Early Hum. Dev. 155, 105327. doi:10.1016/j.earlhumdev.2021.105327
Farhi, A., Glasser, S., Gabis, L. V., Hirsh-Yechezkel, G., Frank, S., Brinton, L., et al. (2021b). How Are They Doing? Neurodevelopmental Outcomes at School Age of Children Born Following Assisted Reproductive Treatments. J. Child. Neurol. 36, 262–271. doi:10.1177/0883073820967169
Fleming, T. P., Watkins, A. J., Velazquez, M. A., Mathers, J. C., Prentice, A. M., Stephenson, J., et al. (2018). Origins of Lifetime Health Around the Time of Conception: Causes and Consequences. Lancet 391, 1842–1852. doi:10.1016/S0140-6736(18)30312-X
Fountain, C., Zhang, Y., Kissin, D. M., Schieve, L. A., Jamieson, D. J., Rice, C., et al. (2015). Association between Assisted Reproductive Technology Conception and Autism in California, 1997-2007. Am. J. Public Health 105, 963–971. doi:10.2105/AJPH.2014.302383
Fowden, A. L., Coan, P. M., Angiolini, E., Burton, G. J., and Constancia, M. (2011). Imprinted Genes and the Epigenetic Regulation of Placental Phenotype. Prog. Biophysics Mol. Biol. 106, 281–288. doi:10.1016/j.pbiomolbio.2010.11.005
Ganer Herman, H., Mizrachi, Y., Shevach Alon, A., Farhadian, Y., Gluck, O., Bar, J., et al. (2021). Obstetric and Perinatal Outcomes of In Vitro Fertilization and Natural Pregnancies in the Same Mother. Fertil. Steril. 115, 940–946. doi:10.1016/J.FERTNSTERT.2020.10.060
Gao, W.-l., Liu, M., Yang, Y., Yang, H., Liao, Q., Bai, Y., et al. (2012). The Imprinted H19 Gene Regulates Human Placental Trophoblast Cell Proliferation via Encoding miR-675 that Targets Nodal Modulator 1 (NOMO1). RNA Biol. 9, 1002–1010. doi:10.4161/rna.20807
Ghai, M., and Kader, F. (2021). A Review on Epigenetic Inheritance of Experiences in Humans. Biochem. Genet. doi:10.1007/S10528-021-10155-7
Glatthorn, H. N., Sauer, M. V., Brandt, J. S., and Ananth, C. V. (2021). Infertility Treatment and the Risk of Small for Gestational Age Births: a Population-Based Study in the United States. F&S Rep. 2, 413–420. doi:10.1016/J.XFRE.2021.05.002
Godfrey, K. M., and Barker, D. J. (2000). Fetal Nutrition and Adult Disease. Am. J. Clin. Nutr. Am. Soc. Nutr. 71, 1344S–1352S. doi:10.1093/ajcn/71.5.1344s
Green, M. P., Mouat, F., Miles, H. L., Hopkins, S. A., Derraik, J. G. B., Hofman, P. L., et al. (2013). Phenotypic Differences in Children Conceived from Fresh and Thawed Embryos in In Vitro Fertilization Compared with Naturally Conceived Children. Fertil. Steril. 99, 1898–1904. doi:10.1016/J.FERTNSTERT.2013.02.009
Guo, X.-Y., Liu, X.-M., Jin, L., Wang, T.-T., Ullah, K., Sheng, J.-Z., et al. (2017). Cardiovascular and Metabolic Profiles of Offspring Conceived by Assisted Reproductive Technologies: a Systematic Review and Meta-Analysis. Fertil. Steril. 107, 622–631. doi:10.1016/j.fertnstert.2016.12.007
Haavaldsen, C., Tanbo, T., and Eskild, A. (2012). Placental Weight in Singleton Pregnancies with and without Assisted Reproductive Technology: a Population Study of 536 567 Pregnancies. Hum. Reprod. 27, 576–582. doi:10.1093/humrep/der428
Halliday, J., Wilson, C., Hammarberg, K., Doyle, L. W., Bruinsma, F., McLachlan, R., et al. (2014). Comparing Indicators of Health and Development of Singleton Young Adults Conceived with and without Assisted Reproductive Technology. Fertil. Steril. 101, 1055–1063. doi:10.1016/j.fertnstert.2014.01.006
Hansen, M., Kurinczuk, J. J., De Klerk, N., Burton, P., and Bower, C. (2012). Assisted Reproductive Technology and Major Birth Defects in Western Australia. Obstetrics Gynecol. 120, 852–863. doi:10.1097/AOG.0B013E318269C282
Hargreave, M., Jensen, A., Toender, A., Andersen, K. K., and Kjaer, S. K. (2013). Fertility Treatment and Childhood Cancer Risk: a Systematic Meta-Analysis. Fertil. Steril. 100, 150–161. doi:10.1016/J.FERTNSTERT.2013.03.017
Hart, R., and Norman, R. J. (2013). The Longer-Term Health Outcomes for Children Born as a Result of Ivf Treatment: Part I-General Health Outcomes. Hum. Reprod. Update 19, 232–243. doi:10.1093/humupd/dms062
Hemberger, M., Hanna, C. W., and Dean, W. (2020). Mechanisms of Early Placental Development in Mouse and Humans. Nat. Rev. Genet. 21, 27–43. doi:10.1038/s41576-019-0169-4
Hochberg, Z., Feil, R., Constancia, M., Fraga, M., Junien, C., Carel, J.-C., et al. (2011). Child Health, Developmental Plasticity, and Epigenetic Programming. Endocr. Rev. 32, 159–224. doi:10.1210/ER.2009-0039
Hoyos, L. R., and Ory, S. J. (2021). The Influence of Assisted Reproductive Technologies on Obstetric and Perinatal Outcomes: the Chicken, the Egg, or Both? Fertil. Steril. 115, 884–885. doi:10.1016/J.FERTNSTERT.2021.01.022
Hwang, S. S., Dukhovny, D., Gopal, D., Cabral, H., Missmer, S., Diop, H., et al. (2018). Health of Infants after ART-Treated, Subfertile, and Fertile Deliveries. Pediatrics 142. doi:10.1542/peds.2017-4069
Isaksson, R., Gissler, M., and Tiitinen, A. (2002). Obstetric Outcome Among Women with Unexplained Infertility after IVF: A Matched Case-Control Study. Hum. Reprod. 17, 1755–1761. doi:10.1093/HUMREP/17.7.1755
Jenabi, E., Seyedi, M., Hamzehei, R., Bashirian, S., Rezaei, M., Razjouyan, K., et al. (2020). Association between Assisted Reproductive Technology and Autism Spectrum Disorders in iran: A Case-Control Study. Clin. Exp. Pediatr. 63, 368–372. doi:10.3345/cep.2020.00073
Jirtle, R. L., and Skinner, M. K. (2007). Environmental Epigenomics and Disease Susceptibility. Nat. Rev. Genet. 8, 253–262. doi:10.1038/nrg2045
Juonala, M., Lewis, S., McLachlan, R., Hammarberg, K., Kennedy, J., Saffery, R., et al. (2020). American Heart Association Ideal Cardiovascular Health Score and Subclinical Atherosclerosis in 22-35-Year-Old Adults Conceived with and without Assisted Reproductive Technologies. Hum. Reprod. 35, 232–239. doi:10.1093/humrep/dez240
Katalinic, A., Rösch, C., and Ludwig, M. (2004). Pregnancy Course and Outcome after Intracytoplasmic Sperm Injection: a Controlled, Prospective Cohort Study. Fertil. Steril. 81, 1604–1616. doi:10.1016/J.FERTNSTERT.2003.10.053
Katari, S., Turan, N., Bibikova, M., Erinle, O., Chalian, R., Foster, M., et al. (2009). DNA Methylation and Gene Expression Differences in Children Conceived In Vitro or In Vivo. Hum. Mol. Genet. 18, 3769–3778. doi:10.1093/hmg/ddp319
Lahti-Pulkkinen, M., Cudmore, M. J., Haeussner, E., Schmitz, C., Pesonen, A.-K., Hämäläinen, E., et al. (2018). Placental Morphology Is Associated with Maternal Depressive Symptoms during Pregnancy and Toddler Psychiatric Problems. Sci. Rep. 8, 1–12. doi:10.1038/s41598-017-19133-9
Lazaraviciute, G., Kauser, M., Bhattacharya, S., Haggarty, P., and Bhattacharya, S. (2014). A Systematic Review and Meta-Analysis of DNA Methylation Levels and Imprinting Disorders in Children Conceived by IVF/ICSI Compared with Children Conceived Spontaneously. Hum. Reprod. Update 20, 840–852. doi:10.1093/humupd/dmu033
Lee, B., Koeppel, A. F., Wang, E. T., Gonzalez, T. L., Sun, T., Kroener, L., et al. (2019). Differential Gene Expression during Placentation in Pregnancies Conceived with Different Fertility Treatments Compared with Spontaneous Pregnancies. Fertil. Steril. 111, 535–546. doi:10.1016/j.fertnstert.2018.11.005
Levi Setti, P. E., Moioli, M., Smeraldi, A., Cesaratto, E., Menduni, F., Livio, S., et al. (2016). Obstetric Outcome and Incidence of Congenital Anomalies in 2351 IVF/ICSI Babies. J. Assist. Reprod. Genet. 33, 711–717. doi:10.1007/S10815-016-0714-4
Levi-Setti, P. E., and Patrizio, P. (2018). Assisted Reproductive Technologies (ART) and Childhood Cancer: Is the Risk Real? J. Assist. Reprod. Genet. 35, 1773–1775. doi:10.1007/S10815-018-1274-6
Litzky, J. F., Deyssenroth, M. A., Everson, T. M., Armstrong, D. A., Lambertini, L., Chen, J., et al. (2017). Placental Imprinting Variation Associated with Assisted Reproductive Technologies and Subfertility. Epigenetics 12, 653–661. doi:10.1080/15592294.2017.1336589
Litzky, J. F., and Marsit, C. J. (2019). Epigenetically Regulated Imprinted Gene Expression Associated with IVF and Infertility: Possible Influence of Prenatal Stress and Depression. J. Assist. Reprod. Genet. 36, 1299–1313. doi:10.1007/s10815-019-01483-0
Liu, H., Zhang, Y., Gu, H.-T., Feng, Q.-L., Liu, J.-Y., Zhou, J., et al. (2015). Association between Assisted Reproductive Technology and Cardiac Alteration at Age 5 Years. JAMA Pediatr. 169, 603–605. doi:10.1001/jamapediatrics.2015.0214
Liu, L., Gao, J., He, X., Cai, Y., Wang, L., and Fan, X. (2017). Association between Assisted Reproductive Technology and the Risk of Autism Spectrum Disorders in the Offspring: a Meta-Analysis. Sci. Rep. 7, 46207. doi:10.1038/SREP46207
Luke, B., Brown, M. B., Ethen, M. K., Canfield, M. A., Watkins, S., Wantman, E., et al. (2020). Third Grade Academic Achievement Among Children Conceived with the Use of In Vitro Fertilization: a Population-Based Study in Texas. Fertil. Steril. 113, 1242–1250. doi:10.1016/j.fertnstert.2020.01.015
Luke, B., Gopal, D., Cabral, H., Stern, J. E., and Diop, H. (2017). Pregnancy, Birth, and Infant Outcomes by Maternal Fertility Status: the Massachusetts Outcomes Study of Assisted Reproductive Technology. Am. J. Obstetrics Gynecol. 217, e1–e14. doi:10.1016/J.AJOG.2017.04.006
Lung, F.-W., Chiang, T.-L., Lin, S.-J., Lee, M.-C., and Shu, B.-C. (2018). Assisted Reproductive Technology Has No Association with Autism Spectrum Disorders: The Taiwan Birth Cohort Study. Autism 22, 377–384. doi:10.1177/1362361317690492
Luo, W., Friedman, M. S., Shedden, K., Hankenson, K. D., and Woolf, P. J. (2009). GAGE: Generally Applicable Gene Set Enrichment for Pathway Analysis. BMC Bioinforma. 10, 161. doi:10.1186/1471-2105-10-161
Magnus, M. C., Wilcox, A. J., Fadum, E. A., Gjessing, H. K., Opdahl, S., Juliusson, P. B., et al. (2021). Growth in Children Conceived by ART. Hum. Reprod. 36, 1074–1082. doi:10.1093/HUMREP/DEAB007
Maimburg, R. D., and Vaeth, M. (2007). Do children Born after Assisted Conception Have Less Risk of Developing Infantile Autism? Hum. Reprod. 22, 1841–1843. doi:10.1093/HUMREP/DEM082
Menezo, Y., Elder, K., Benkhalifa, M., and Dale, B. (2010). DNA Methylation and Gene Expression in IVF. Reprod. Biomed. Online 20, 709–710. doi:10.1016/j.rbmo.2010.02.016
Miles, H. L., Hofman, P. L., Peek, J., Harris, M., Wilson, D., Robinson, E. M., et al. (2007). In Vitro fertilization Improves Childhood Growth and Metabolism. J. Clin. Endocrinol. Metab. 92, 3441–3445. doi:10.1210/JC.2006-2465
Mohammadi, M., Khedmati Morasae, E., Maroufizadeh, S., Almasi-Hashiani, A., Navid, B., Amini, P., et al. (2020). Assisted Reproductive Technology and the Risk of Gestational Diabetes Mellitus: a Systematic Review and Meta-Analysis. Middle East Fertil. Soc. J. 25, 1–12. doi:10.1186/S43043-020-0018-6/FIGURES/6
Molinaro, T. A. (2021). Taking a Second Look at Obstetrical Outcomes after Assisted Reproductive Technologies. Fertil. Steril. Rep. 2, 368–369. doi:10.1016/j.xfre.2021.08.014
Monk, D., Mackay, D. J. G., Eggermann, T., Maher, E. R., and Riccio, A. (2019). Genomic Imprinting Disorders: Lessons on How Genome, Epigenome and Environment Interact. Nat. Rev. Genet. 20, 235–248. doi:10.1038/s41576-018-0092-0
Morison, I. M., Ramsay, J. P., and Spencer, H. G. (2005). A Census of Mammalian Imprinting. Trends Genet. 21, 457–465. doi:10.1016/j.tig.2005.06.008
Nekkebroeck, J., Bonduelle, M., Desmyttere, S., Van Den Broeck, W., and Ponjaert-Kristoffersen, I. (2008). Mental and Psychomotor Development of 2-Year-Old Children Born after Preimplantation Genetic Diagnosis/screening. Hum. Reprod. 23, 1560–1566. doi:10.1093/humrep/den033
Nelissen, E. C. M., Dumoulin, J. C. M., Busato, F., Ponger, L., Eijssen, L. M., Evers, J. L. H., et al. (2014). Altered Gene Expression in Human Placentas after IVF/ICSI. Hum. Reprod. 29, 2821–2831. doi:10.1093/humrep/deu241
Nelissen, E. C. M., Dumoulin, J. C. M., Daunay, A., Evers, J. L. H., Tost, J., and Van Montfoort, A. P. A. (2013). Placentas from Pregnancies Conceived by IVF/ICSI Have a Reduced DNA Methylation Level at the H19 and MEST Differentially Methylated Regions†. Hum. Reprod. 28, 1117–1126. doi:10.1093/humrep/des459
Nilsson, E. E., Sadler-Riggleman, I., and Skinner, M. K. (2018). Environmentally Induced Epigenetic Transgenerational Inheritance of Disease. Environ. epigenetics 4, 1–13. doi:10.1093/EEP/DVY016
Norrman, E., Petzold, M., Bergh, C., and Wennerholm, U.-B. (2018). School Performance in Singletons Born after Assisted Reproductive Technology. Hum. Reprod. 33, 1948–1959. doi:10.1093/humrep/dey273
Novakovic, B., Lewis, S., Halliday, J., Kennedy, J., Burgner, D. P., Czajko, A., et al. (2019). Assisted Reproductive Technologies Are Associated with Limited Epigenetic Variation at Birth that Largely Resolves by Adulthood. Nat. Commun. 10, 1–12. doi:10.1038/s41467-019-11929-9
Ombelet, W., Martens, G., and Bruckers, L. (2016). Pregnant after Assisted Reproduction: a Risk Pregnancy Is Born! 18-years Perinatal Outcome Results from a Population-Based Registry in Flanders. Facts, views Vis. ObGyn 8, 193–204. Available at: http://www.ncbi.nlm.nih.gov/pubmed/28210479 (Accessed April 13, 2021).
Ong, Y. Y., Sadananthan, S. A., Aris, I. M., Tint, M. T., Yuan, W. L., Huang, J. Y., et al. (2020). Mismatch between Poor Fetal Growth and Rapid Postnatal Weight Gain in the First 2 Years of Life Is Associated with Higher Blood Pressure and Insulin Resistance without Increased Adiposity in Childhood: The GUSTO Cohort Study. Int. J. Epidemiol. 49, 1591–1603. doi:10.1093/ije/dyaa143
Palomba, S., Homburg, R., Santagni, S., La Sala, G. B., and Orvieto, R. (2016). Risk of Adverse Pregnancy and Perinatal Outcomes after High Technology Infertility Treatment: A Comprehensive Systematic Review. Reprod. Biol. Endocrinol. 14, 1–25. doi:10.1186/s12958-016-0211-8
Pandey, S., Shetty, A., Hamilton, M., Bhattacharya, S., and Maheshwari, A. (2012). Obstetric and Perinatal Outcomes in Singleton Pregnancies Resulting from Ivf/icsi: A Systematic Review and Meta-Analysis. Hum. Reprod. Update 18, 485–503. doi:10.1093/humupd/dms018
Petersen, S. H., Bergh, C., Gissler, M., Åsvold, B. O., Romundstad, L. B., Tiitinen, A., et al. (2020). Time Trends in Placenta-Mediated Pregnancy Complications after Assisted Reproductive Technology in the Nordic Countries. Am. J. Obstetrics Gynecol. 223, e1–e19. doi:10.1016/j.ajog.2020.02.030
Pontesilli, M., Painter, R. C., Grooten, I. J., Van Der Post, J. A., Mol, B. W., Vrijkotte, T. G. M., et al. (2015). Subfertility and Assisted Reproduction Techniques Are Associated with Poorer Cardiometabolic Profiles in Childhood. Reprod. Biomed. Online 30, 258–267. doi:10.1016/j.rbmo.2014.11.006
Punamäki, R.-L., Tiitinen, A., Lindblom, J., Unkila-Kallio, L., Flykt, M., Vänskä, M., et al. (2016). Mental Health and Developmental Outcomes for Children Born after ART: A Comparative Prospective Study on Child Gender and Treatment Type. Hum. Reprod. 31, 100–107. doi:10.1093/humrep/dev273
Qin, J., Liu, X., Sheng, X., Wang, H., and Gao, S. (2016). Assisted Reproductive Technology and the Risk of Pregnancy-Related Complications and Adverse Pregnancy Outcomes in Singleton Pregnancies: A Meta-Analysis of Cohort Studies. Fertil. Steril. 105, 73–85. doi:10.1016/j.fertnstert.2015.09.007
Qin, J., Wang, H., Sheng, X., Liang, D., Tan, H., and Xia, J. (2015). Pregnancy-related Complications and Adverse Pregnancy Outcomes in Multiple Pregnancies Resulting from Assisted Reproductive Technology: A Meta-Analysis of Cohort Studies. Fertil. Steril. 103, 1492–1508. doi:10.1016/j.fertnstert.2015.03.018
Raimondi, S., Pedotti, P., and Taioli, E. (2005). Meta-analysis of Cancer Incidence in Children Born after Assisted Reproductive Technologies. Br. J. Cancer 93, 1053–1056. doi:10.1038/SJ.BJC.6602838
Rancourt, R. C., Harris, H. R., and Michels, K. B. (2012). Methylation Levels at Imprinting Control Regions Are Not Altered with Ovulation Induction or In Vitro Fertilization in a Birth Cohort. Hum. Reprod. 27, 2208–2216. doi:10.1093/HUMREP/DES151
Ravelli, A. C., Van Der Meulen, J. H., Osmond, C., Barker, D. J., and Bleker, O. P. (1999). Obesity at the Age of 50 Y in Men and Women Exposed to Famine Prenatally. Am. J. Clin. Nutr. 70, 811–816. doi:10.1093/ajcn/70.5.811
Reik, W., Constância, M., Fowden, A., Anderson, N., Dean, W., Ferguson-Smith, A., et al. (2003). Regulation of Supply and Demand for Maternal Nutrients in Mammals by Imprinted Genes. J. Physiology 547, 35–44. doi:10.1113/jphysiol.2002.033274
Reynolds, L. P., and Caton, J. S. (2012). Role of the Pre- and Post-natal Environment in Developmental Programming of Health and Productivity. Mol. Cell. Endocrinol. 354, 54–59. doi:10.1016/j.mce.2011.11.013
Rissanen, E., Gissler, M., Lehti, V., and Tiitinen, A. (2020). The Risk of Psychiatric Disorders Among Finnish ART and Spontaneously Conceived Children: Finnish Population-Based Register Study. Eur. Child. Adolesc. Psychiatry 29, 1155–1164. doi:10.1007/S00787-019-01433-2
Roseboom, T. J., and Eriksson, J. G. (2021). Children Conceived by ART Grow Differently in Early Life Than Naturally Conceived Children but Reach the Same Height and Weight by Age 17. Reassuring? Not So Sure. Hum. Reprod. 36, 847–849. doi:10.1093/humrep/deab048
Roychoudhury, S., Lodha, A., Synnes, A., Abou Mehrem, A., Canning, R., Banihani, R., et al. (2021). Neurodevelopmental Outcomes of Preterm Infants Conceived by Assisted Reproductive Technology. Am. J. Obstetrics Gynecol. 225, e1–e1276. doi:10.1016/J.AJOG.2021.03.027
Sakian, S., Louie, K., Wong, E. C., Havelock, J., Kashyap, S., Rowe, T., et al. (2015). Altered Gene Expression of H19 and IGF2 in Placentas from ART Pregnancies. Placenta 36, 1100–1105. doi:10.1016/j.placenta.2015.08.008
Sakka, S. D., Loutradis, D., Kanaka-Gantenbein, C., Margeli, A., Papastamataki, M., Papassotiriou, I., et al. (2010). Absence of Insulin Resistance and Low-Grade Inflammation Despite Early Metabolic Syndrome Manifestations in Children Born after In Vitro Fertilization. Fertil. Steril. 94, 1693–1699. doi:10.1016/j.fertnstert.2009.09.049
Sánchez-Soler, M. J., López-González, V., Ballesta-Martínez, M. J., Gálvez- Pradillo, J., Domingo-Jiménez, R., Pérez-Fernández, V., et al. (2020). Assessment of Psychomotor Development of Spanish Children up to 3 Years of Age Conceived by Assisted Reproductive Techniques: Prospective Matched Cohort Study. An. Pediatría (English Ed. 92, 200–207. doi:10.1016/j.anpedi.2019.07.00610.1016/j.anpede.2019.07.005
Sandin, S., Nygren, K.-G., Iliadou, A., Hultman, C. M., and Reichenberg, A. (2013). Autism and Mental Retardation Among Offspring Born after In Vitro Fertilization. Jama 310, 75–84. doi:10.1001/jama.2013.7222
Scherrer, U., Rimoldi, S. F., Rexhaj, E., Stuber, T., Duplain, H., Garcin, S., et al. (2012). Systemic and Pulmonary Vascular Dysfunction in Children Conceived by Assisted Reproductive Technologies. Circulation 125, 1890–1896. doi:10.1161/CIRCULATIONAHA.111.071183
Strömberg, B., Dahlquist, G., Ericson, A., Finnström, O., Köster, M., and Stjernqvist, K. (2002). Neurological Sequelae in Children Born after In-Vitro Fertilisation: A Population-Based Study. Lancet 359, 461–465. doi:10.1016/S0140-6736(02)07674-2
Sun, T., Lee, B., Kinchen, J., Wang, E. T., Gonzalez, T. L., Chan, J. L., et al. (2019). Differences in First-Trimester Maternal Metabolomic Profiles in Pregnancies Conceived from Fertility Treatments. J. Clin. Endocrinol. Metab. 104, 1005–1019. doi:10.1210/jc.2018-01118
Sunderam, S., Kissin, D. M., Zhang, Y., Folger, S. G., Boulet, S. L., Warner, L., et al. (2019). Assisted Reproductive Technology Surveillance - United States, 2016. MMWR Surveill. Summ. 68, 1–23. doi:10.15585/mmwr.ss6804a1
Thamban, T., Agarwaal, V., and Khosla, S. (2020). Role of Genomic Imprinting in Mammalian Development. J. Biosci. 45, 20. doi:10.1007/s12038-019-9984-1
Turan, N., Katari, S., Gerson, L. F., Chalian, R., Foster, M. W., Gaughan, J. P., et al. (2010). Inter- and Intra-individual Variation in Allele-specific DNA Methylation and Gene Expression in Children Conceived Using Assisted Reproductive Technology. PLoS Genet. 6, e1001033–14. doi:10.1371/JOURNAL.PGEN.1001033
Valenzuela-Alcaraz, B., Serafini, A., Sepulveda-Martínez, A., Casals, G., Rodríguez-López, M., Garcia-Otero, L., et al. (2019). Postnatal Persistence of Fetal Cardiovascular Remodelling Associated with Assisted Reproductive Technologies: a Cohort Study. BJOG Int. J. Obstet. Gy 126, 291–298. doi:10.1111/1471-0528.15246
Valenzuela-Alcaraz, B., Crispi, F., Bijnens, B., Cruz-Lemini, M., Creus, M., Sitges, M., et al. (2013). Assisted Reproductive Technologies Are Associated with Cardiovascular Remodeling In Utero that Persists Postnatally. Circulation 128, 1442–1450. doi:10.1161/CIRCULATIONAHA.113.002428
Valenzuela-Alcaraz, B., Cruz-Lemini, M., Rodríguez-López, M., Goncé, A., García-Otero, L., Ayuso, H., et al. (2018). Fetal Cardiac Remodeling in Twin Pregnancy Conceived by Assisted Reproductive Technology. Ultrasound Obstet. GynecolGynecol 51, 94–100. doi:10.1002/uog.17527
Van Den Bergh, B. R. H. (2011). Developmental Programming of Early Brain and Behaviour Development and Mental Health: a Conceptual Framework. Dev. Med. Child. Neurol. 53, 19–23. doi:10.1111/j.1469-8749.2011.04057.x
Van Montfoort, A. P. A., Hanssen, L. L. P., De Sutter, P., Viville, S., Geraedts, J. P. M., and De Boer, P. (2012). Assisted Reproduction Treatment and Epigenetic Inheritance. Hum. Reprod. Update 18, 171–197. doi:10.1093/humupd/dmr047
Vasario, E., Borgarello, V., Bossotti, C., Libanori, E., Biolcati, M., Arduino, S., et al. (2010). IVF Twins Have Similar Obstetric and Neonatal Outcome as Spontaneously Conceived Twins: a Prospective Follow-Up Study. Reprod. Biomed. Online 21, 422–428. doi:10.1016/J.RBMO.2010.04.007
Vermeiden, J. P. W., and Bernardus, R. E. (2013). Are Imprinting Disorders More Prevalent after Human In Vitro Fertilization or Intracytoplasmic Sperm Injection? Fertil. Steril. 99, 642–651. doi:10.1016/j.fertnstert.2013.01.125
Vermey, B., Buchanan, A., Chambers, G., Kolibianakis, E., Bosdou, J., Chapman, M., et al. (2019). Are Singleton Pregnancies after Assisted Reproduction Technology ( ART ) Associated with a Higher Risk of Placental Anomalies Compared with Non- ART Singleton Pregnancies? A Systematic Review and Meta-analysis. BJOG Int. J. Obstet. Gy 126, 209–218. doi:10.1111/1471-0528.15227
von Arx, R., Allemann, Y., Sartori, C., Rexhaj, E., Cerny, D., de Marchi, S. F., et al. (2015). Right Ventricular Dysfunction in Children and Adolescents Conceived by Assisted Reproductive Technologies. J. Appl. Physiology 118, 1200–1206. doi:10.1152/JAPPLPHYSIOL.00533.2014
Wagenaar, K., Ceelen, M., Van Weissenbruch, M. M., Knol, D. L., Delemarre-Van De Waal, H. A., and Huisman, J. (2008). School Functioning in 8- to 18-Year-Old Children Born after In Vitro Fertilization. Eur. J. Pediatr. 167, 1289–1295. doi:10.1007/s00431-008-0677-2
Wang, T., Chen, L., Yang, T., Wang, L., Zhao, L., Zhang, S., et al. (2019). Cancer Risk Among Children Conceived by Fertility Treatment. Int. J. cancer 144, 3001–3013. doi:10.1002/IJC.32062
Yanaihara, A., Hatakeyama, S., Ohgi, S., Motomura, K., Taniguchi, R., Hirano, A., et al. (2018). Difference in the Size of the Placenta and Umbilical Cord between Women with Natural Pregnancy and Those with IVF Pregnancy. J. Assist. Reprod. Genet. 35, 431–434. doi:10.1007/s10815-017-1084-2
Zandstra, H., van Montfoort, A. P. A., Dumoulin, J. C. M., Zimmermann, L. J. I., and Touwslager, R. N. H. (2020). Increased Blood Pressure and Impaired Endothelial Function after Accelerated Growth in IVF/ICSI Children. Hum. Reprod. Open 2020, 1–13. doi:10.1093/hropen/hoz037
Zhang, Y., Cui, Y., Zhou, Z., Sha, J., Li, Y., and Liu, J. (2010). Altered Global Gene Expressions of Human Placentae Subjected to Assisted Reproductive Technology Treatments. Placenta 31, 251–258. doi:10.1016/j.placenta.2010.01.005
Zhao, L., Zheng, X., Liu, J., Zheng, R., Yang, R., Wang, Y., et al. (2019). The Placental Transcriptome of the First-Trimester Placenta Is Affected by In Vitro Fertilization and Embryo Transfer. Reprod. Biol. Endocrinol. 17, 1–14. doi:10.1186/s12958-019-0494-7
Keywords: assisted reproductive technologies, placenta, epigenetics, metabolism, long-term health, DOHaD, fetal programming
Citation: Schroeder M, Badini G, Sferruzzi-Perri AN and Albrecht C (2022) The Consequences of Assisted Reproduction Technologies on the Offspring Health Throughout Life: A Placental Contribution. Front. Cell Dev. Biol. 10:906240. doi: 10.3389/fcell.2022.906240
Received: 28 March 2022; Accepted: 05 May 2022;
Published: 20 May 2022.
Edited by:
João Ramalho-Santos, University of Coimbra, PortugalReviewed by:
Susana Pereira, Center of Neurosciences and Cell Biology, University of Coimbra, PortugalCopyright © 2022 Schroeder, Badini, Sferruzzi-Perri and Albrecht. This is an open-access article distributed under the terms of the Creative Commons Attribution License (CC BY). The use, distribution or reproduction in other forums is permitted, provided the original author(s) and the copyright owner(s) are credited and that the original publication in this journal is cited, in accordance with accepted academic practice. No use, distribution or reproduction is permitted which does not comply with these terms.
*Correspondence: Mariana Schroeder, mariana.schroeder@ibmm.unibe.ch
†These authors have contributed equally to this work