- Department of Gastrointestinal Surgery, The First Hospital of Jilin University, Chang Chun, China
Inflammatory bowel disease (IBD), comprising Crohn’s disease and ulcerative colitis, is a refractory disease with many immune abnormalities and pathologies in the gastrointestinal tract. Because macrophages can distinguish innocuous antigens from potential pathogens to maintain mucosa barrier functions, they are essential cells in the intestinal immune system. With numerous numbers in the intestinal tract, tissue-resident macrophages have a significant effect on the constant regeneration of intestinal epithelial cells and maintaining the immune homeostasis of the intestinal mucosa. They also have a significant influence on IBD through regulating pro-(M1) or anti-inflammatory (M2) phenotype polarization according to different environmental cues. The disequilibrium of the phenotypes and functions of macrophages, disturbed by intracellular or extracellular stimuli, influences the progression of disease. Further investigation of macrophages’ role in the progression of IBD will facilitate deciphering the pathogenesis of disease and exploring novel targets to develop novel medications. In this review, we shed light on the origin and maintenance of intestinal macrophages, as well as the role of macrophages in the occurrence and development of IBD. In addition, we summarize the interaction between gut microbiota and intestinal macrophages, and the role of the macrophage-derived exosome. Furthermore, we discuss the molecular and cellular mechanisms participating in the polarization and functions of gut macrophages, the potential targeted strategies, and current clinical trials for IBD.
1 Introduction
In recent years, the morbidity of inflammatory bowel disease (IBD) has increased globally, especially in Africa and Asia, and is spreading worldwide with accelerated speed (Windsor and Kaplan, 2019). IBD is an idiopathic, refractory and chronic disease, with clinical symptoms including abdominal pain, rectal bleeding, weight loss, diarrhea, and anemia, that requires life-long medication (Roda et al., 2020; Seyed Tabib et al., 2020). Conventionally, IBD is mainly divided into Crohn’s disease (CD) and ulcerative colitis (UC), along with different locations and inflammatory types, respectively (Torres et al., 2017; Ungaro et al., 2017). CD affects almost every part of the gastrointestinal tract and tissue layers, and the most frequent locations are the terminal ileum and colon (Torres et al., 2017; Roda et al., 2020). However, UC mainly involves the superficial intestinal mucosa of the colon, including the proximal end of the rectum (Ungaro et al., 2017; Kobayashi et al., 2020). Approximately 5 million people worldwide are affected with IBD, yet the etiology of IBD is not known, and there are no cures so far (Kaplan, 2015). The continuation of this trend is likely to increase the worldwide population of patients affected by IBD to tens of millions or more in the next few decades (Ananthakrishnan, 2015). More importantly, recent discoveries revealed that patients with IBD are more susceptible to colorectal cancer (Nadeem et al., 2020). Thus, the prevention and treatment of IBD are extremely necessary and important.
The etiology of IBD arises from intricate interactions between the immune system, genetic predisposition, environmental cues, and the gut microbiome (Kobayashi et al., 2020; Roda et al., 2020). Under ideal conditions, intestinal inflammation is a self-limiting process that combats harmful pathogens and rapidly returns to homeostasis (Schett and Neurath, 2018). However, a disequilibrium between the mucosal immune system and the commensal ecosystem occurs in the exacerbation of IBD, because of the imbalance of the immunological mechanisms underlying the resolution of inflammation (Hunter, 2012; Schett and Neurath, 2018; Graham and Xavier, 2020). Intestinal macrophages are an integral part of the normal intestinal tissues that can engulf microorganisms and present antigens to activate T cells, as well as prevent excessive inflammation through specific molecular and cellular mechanisms (Smythies et al., 2005; Garrett et al., 2010; Mowat, 2018). According to the microenvironmental cues, macrophages could polarize into two different phenotypes: the classically activated (M1) or alternatively activated (M2) macrophages (Funes et al., 2018; Locati et al., 2020). When the intestinal barrier function is impaired, the efferocytosis effect leads to macrophages skewing toward M2 subtypes functionally, producing cytokines, chemokines and lipid mediators that participate in healing the intestinal mucosal barrier and maintaining homeostasis (Elliott et al., 2017; Na et al., 2019). Various cytokines and other soluble factors, including prostaglandin E2 (PGE2), bone morphogenetic protein 2 (BMP2), and WNT ligands, can be produced by gut macrophages that stimulate the expansion of epithelial cells and participate in the enteric nervous system or intestinal mucosal barrier to help maintain tissue homeostasis (Bain and Mowat, 2014). Meanwhile, intestinal macrophages maintain tolerance via promoting the proliferation of antigen-specific CD4+CD25+ regulatory T (T-reg) cells primarily by producing interleukin (IL)-10 in order to inhibit unrestrained inflammation as a response to innocuous commensal microbes (O'Garra et al., 2004; Hedrich and Bream, 2010).
In addition, the lack of response in patients with IBD to anti-tumor necrosis factor (TNF) therapy was found to be related to a defect in the IL-10 signaling pathway, which is crucial for biologics-induced macrophages skewing toward M2 subsets (Vos et al., 2012; Roda et al., 2016; Koelink et al., 2020). Therefore, intestinal macrophages play major roles in the establishment and maintenance of intestinal homeostasis. As a consequence, disequilibrium of intestinal macrophages could lead to a loss of tolerance to harmless microbes and food antigens, which is considered to underlie the excessive inflammation in IBD (de Souza and Fiocchi, 2016). In view of these findings, it is important to analyze the potential contribution of macrophages to intestinal chronic inflammation, which may offer us novel insights into the pathogenesis and therapeutic strategies in IBD.
In this review, we outline the characteristics of the origin and maintenance of macrophages, as well as their interaction with other immune cells. Then, we delineate the cellular and molecular mechanisms that underlie the differentiation and function of intestinal macrophages. Furthermore, we describe the interaction between gut microbiota and intestinal macrophages, and the role of the macrophage-derived exosome. Finally, potential treatment targets, applicable drugs and current clinical trials for IBD are discussed.
2 Origin and Maintenance of Intestinal Macrophages
The gastrointestinal tract harbors copious amounts of tissue macrophages, orchestrating innate and adaptive immune systems, helping to maintain homeostasis via tolerance to commensal microbes, as well as resisting potentially harmful pathogens (Yip et al., 2021). In the past, the origin of tissue macrophages was considered to be the blood monocytes, deriving from precursors in the bone marrow (van Furth et al., 1972; Bain and Mowat, 2014). With the development of novel techniques, there is mounting evidence showing that monocytes are not the exclusive origin of tissue-resident macrophages (Wu and Hirschi, 2020). Macrophages arise in two different ways: they can be differentiated from blood-derived monocytes and embryo-derived precursors, deriving from the yolk sac and/or fetal liver, established in the tissue before birth (Shaw et al., 2018; Wu and Hirschi, 2020). Intestinal tissue-resident macrophages, derived from embryonic precursors, maintain themselves via self-renewal in situ throughout adulthood but also require constant replenishment by circulating monocytes in the blood (Shaw et al., 2018). This is in contrast to macrophages in other tissues, such as microglia in the central nervous system and Langerhans cells in the liver, that maintain themselves independently and self-renew in situ throughout adult life (Yona et al., 2013; Ginhoux and Guilliams, 2016).
With progress in recent research, traditional understanding has been challenged, and embryo-derived macrophages were found to sustain themselves in the adult intestine (Shaw et al., 2018). In adult mice, parts of intestinal macrophages, characterized by high expression levels of CD4 and apoptotic cell-uptake receptor Tim-4 (Tim-4+CD4+ macrophages), were demonstrated to be long-lived and locally self-renewed independent of monocytes, while Tim-4−CD4+ macrophages were found to have a slow turnover from blood monocytes (Shaw et al., 2018). In contrast, Tim-4−CD4− macrophages were short-lived and relied on continuous replenishment of blood monocytes (Shaw et al., 2018). Similar results have been found in duodenal transplant patients’ mucosa, along with short-lived monocyte-derived macrophage subsets, different and dominant subsets with a slower replenishment rate were confirmed (Bujko et al., 2018). Similarly, another study in mice found that self-renewal embryo-derived macrophages and bone marrow-derived monocytes concomitantly reside in the lamina propria and muscularis externa layer of the intestine, persisting throughout adulthood (De Schepper et al., 2018). Depletion of self-maintaining macrophages could lead to a series of problems, such as injury of the submucosal vascular network, damage of enteric neurons, impaired neuro-evoked gastrointestinal section, and weakened intestinal motility (De Schepper et al., 2018). As noted above, parabiosis experiments (Guilliams et al., 2013; Hashimoto et al., 2013; Jakubzick et al., 2013; Epelman et al., 2014) and genetic fate-mapping experiments (Ginhoux et al., 2010; Hoeffel et al., 2012; Schulz et al., 2012; Yona et al., 2013) have proved that macrophages in the adult gut originating from yolk-sac precursors maintain self-renewal throughout life, thus existing independently of bone-marrow precursors for long-lasting maintenance. Given that there are different intestinal macrophage subsets with strong heterogeneity and distinct functional characteristics, future studies on macrophages in IBD should be focusing on altering the inflammatory phenotype, in parallel with keeping the protective tissue-resident subpopulations intact.
It has been proposed that macrophage precursors might compete for a limited number of niches, and the balance between embryo-derived macrophages and bone-marrow derived monocytes is delicately orchestrated by niche accessibility, availability and precursor plasticity (Guilliams and Scott, 2017; Guilliams and Svedberg, 2021). The orchestration of the niche assures that monocytes can differentiate efficiently into macrophages based on tissue-specific microenvironments only when the niche is available (Guilliams and Scott, 2017). Niche imprints play a strategic role in dictating macrophage identity and capacity for self-maintenance rather than only their origin, as was described previously (Mass and Gentek, 2021; Viola and Boeckxstaens, 2021). When the body is in a steady state, embryonic macrophages continuously renew and maintain themselves in adult tissues (Louwe et al., 2021). In the case of infection and tissue damage causing large consumption of tissue-resident macrophages, blood circulating monocytes can be used for long-term reconstruction of tissue-resident macrophages (Louwe et al., 2021). Intestinal macrophages are replenished by blood-derived monocytes through a series of differential intermediates in a step-wise continuum way, which is a process taking around 5–6 days, and has become known as the monocyte waterfall (Bain et al., 2013; Bain et al., 2014). (Figure 1). Lymphocyte antigen 6C-high (Ly6Chi) CX3C-chemokine receptor 1- intermediate (CX3CR1int) major histocompatibility complex II (MHCII−) (P1) monocytes seem similar to their counterparts in blood in aspects of both phenotype and morphology (Schridde et al., 2017). As these cells enter the lamina propria, they first acquire the expression of MHCII (P2), and then downregulate Ly6C expression (P3), together with the other proteins of extravasation, and finally, upregulating the expression of CX3CR1, CD64, and F4/80, giving rise to mature macrophages (P4) (Bain et al., 2013; Bain et al., 2014) (Figure 1). Notably, more and more evidences suggest that there is an analogous waterfall in the human intestinal mucosa, with classical CD14hiCCR2+CD11chi monocytes gradually differentiating into CD14lowCCR2-CD11clow macrophages (Bain et al., 2013; Bernardo et al., 2018; Bujko et al., 2018). However, there are still many questions to be answered about this process. How do macrophages differentiate into specific compartments in the distinct micro-environment of the duodenum with powerful absorptive ability or the cecum with myriad microbes? Is there a role for tissue-resident macrophages in memorizing past inflammatory injury within the intestinal tissue? Based on the aforementioned findings and potential challenges, facilitating the restoration of inflammation-imprinted resident macrophages and their niche in homeostasis may bring about a promising therapeutic avenue to prevent secondary complications in these patients.
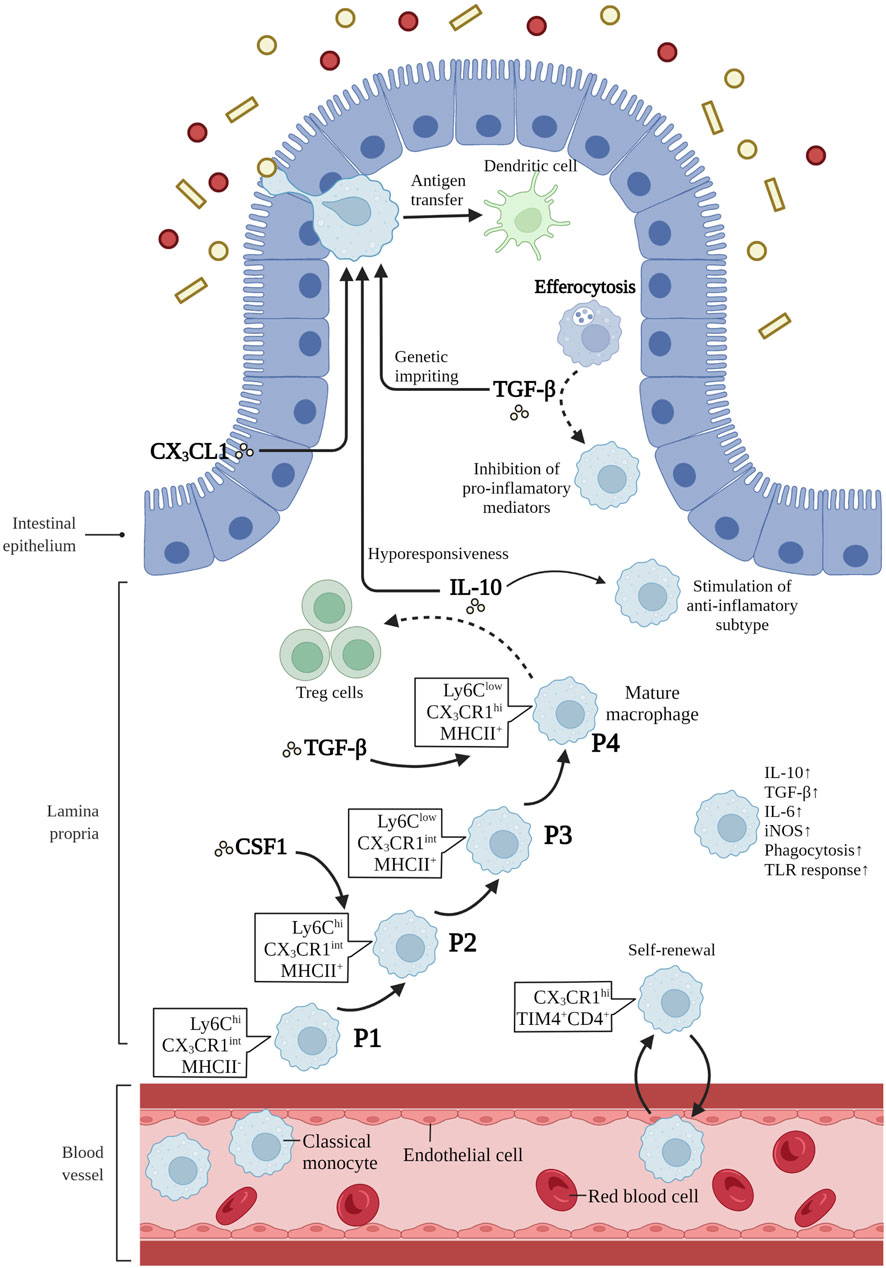
FIGURE 1. Differentiation and function of intestinal macrophages. Intestinal macrophages are replenished by blood-derived monocytes through a series of differential intermediates in a way of “monocyte waterfall”. lymphocyte antigen 6C-high (Ly6Chi) CX3C-chemokine receptor 1-intermediate (CX3CR1int) major histocompatibility complex II (MHCII−) (P1) monocytes enter the lamina propria, acquiring the expression of MHCII (P2), and then downregulating Ly6C expression (P3). Finally, these cells upregulate the expression of CX3CR1, CD64, and F4/80, giving rise to mature macrophages (P4). T cell immunoglobulin mucin receptor 4-positive (Tim-4+) CD4+ macrophages have been demonstrated to be long-lived and locally self-renewed independent of monocytes. Macrophage colony-stimulating factor 1 (CSF1) is a critical cytokine in the survival, proliferation and differentiation of intestinal macrophages. IL-10 is crucial in inducing subtype of anti-inflammatory macrophages, maintaining the hyporesponsiveness to toll-like receptors (TLRs) stimulation, and promoting antigen-specific regulatory T (Treg) cells. CX3C-chemokine ligand 1 (CX3CL1), secreted by intestinal epithelial cells, plays a critical role in the differentiation and function of mouse intestinal macrophages. In addition, CX3CR1+ macrophages form transepithelial dendrites (TEDs) with the ability to capture potential pathogens and transfer antigens to dendritic cells. The transforming growth factor-β (TGF-β) is essential for the terminal differentiation of macrophages in intestinal mucosa, and genetic imprinting of intestinal macrophages in mice. Besides, efferocytosis produce the secretion of TGF-β by macrophages, restraining the production of pro-inflammatory mediators. Mature intestinal macrophages are accompanied with the upregulation of IL-10, TGF-β, IL-6, iNOS, and the ability of phagocytosis and TLR responsiveness. Created with BioRender.com.
3 Function of Intestinal Macrophages in IBD
IBD is characterized by a destructive and relapsing inflammation of the gastrointestinal tract that harbors many macrophages that accumulate in the mucosa and are involved in the pathogenetic process. In IBD, the inflamed colonic mucosa can recruit a large number of macrophages, vigorously secreting IL-1, IL-6, TNF-α, IL-12, and IL-23, as well as producing reactive oxygen species (ROS), reactive nitrogen intermediates (RNIs), and proteases that could degrade the extracellular matrix. However, the characteristics of intestinal-resident macrophages in CD and UC are distinct with respect to distribution, ability to clear pathogens, and involvement in fibrosis. Macrophages mainly spread within the intestinal mucosa in UC, however, in CD, these cells also infiltrate in the muscular layer and mesenteric fat tissue (Mahida et al., 1989; Kredel et al., 2013). In the past, there was a simplified statement that CD has even been regarded as a primary immunodeficiency of macrophage (Casanova and Abel, 2009). Therefore, impaired cytokine secretion by macrophages was thought to be the reason for defective pathogenic clearance in CD (Smith et al., 2009). It is common to observe that intestinal macrophages contain E. coli in CD, but not in UC (Elliott et al., 2015). In contrary, bacterial pathogens were responded actively by macrophages in patients with UC (Rahman et al., 2010). As a consequence, the formation of granulomas is generally found in CD patients but not the UC patients, because of the failure to eradicate invading pathogens effectively (Cook and Dixon, 1973). Notably, the degree of fibrosis is more pronounced in CD than in UC (Yamagata et al., 2011). Excessive wound-healing processes involve various steps with macrophages, resulting in the development of fibrosis and fibrotic strictures subsequently. In fibrotic CD, several factors produced by macrophages promote the formation of fibrosis, such as MMP-2, IL-13, TNF-α, and transforming growth factor-β (TGF-β) (Fichtner-Feigl et al., 2006; Bailey et al., 2012).
3.1 Macrophage Subsets in IBD
Mature macrophage phenotypes and functions are highly plastic and heterogeneous in response to environmental cues (Mosser and Edwards, 2008). Conventionally, a dichotomic nomenclature describes activated macrophages as having two opposite states: classically activated (M1) and alternatively activated (M2) macrophages (Gordon, 2003; Aggarwal et al., 2014). The imbalance of M1/M2 phenotypes has been demonstrated to be involved in colitis exacerbation in the murine model of IBD (Zhu et al., 2014). Here, we summarize the stimulators of M1 and M2 macrophages, molecular marker expression, signaling pathways involved, major cytokines and chemokines secreted, as well as their functions in IBD.
In general, classically activated (M1) macrophages are characterized by secreting cytokines such as IL-12, IL-23, IL-1β, TNF-α, ROS, and RNIs, inducing inflammation and clearing pathogens. In lamina propria of inflamed gut, pro-inflammatory M1 macrophages break down the tight junction proteins, damage the epithelial barrier, and induce epithelial cell apoptosis, leading to excessive inflammation (Cosín-Roger et al., 2013; Steinbach and Plevy, 2014; Lissner et al., 2015). In addition, the M1 subsets are also involved in the induction of T helper 1 cells (Th1) and Th17 responses. The transformation of macrophages into the M1 phenotype involves many key transcription factors, such as Janus kinase/signal transducer and transcription activator 1 (JAK/STAT1) (Hu et al., 2002; Gordon and Martinez, 2010), phosphoinositide 3-kinase (PI3K)/Akt1 (Lu et al., 2017; Vergadi et al., 2017), nuclear factor (NF)-κB (Oeckinghaus et al., 2011; Zhang et al., 2018), interferon regulatory factor 5 (IRF5) (Chistiakov et al., 2018), and Notch (Xu et al., 2012; Siebel and Lendahl, 2017). On the other hand, sustained M1 phenotype expression has been shown to aggravate the inflammatory response and ultimately cause tissue damage (Figure 2).
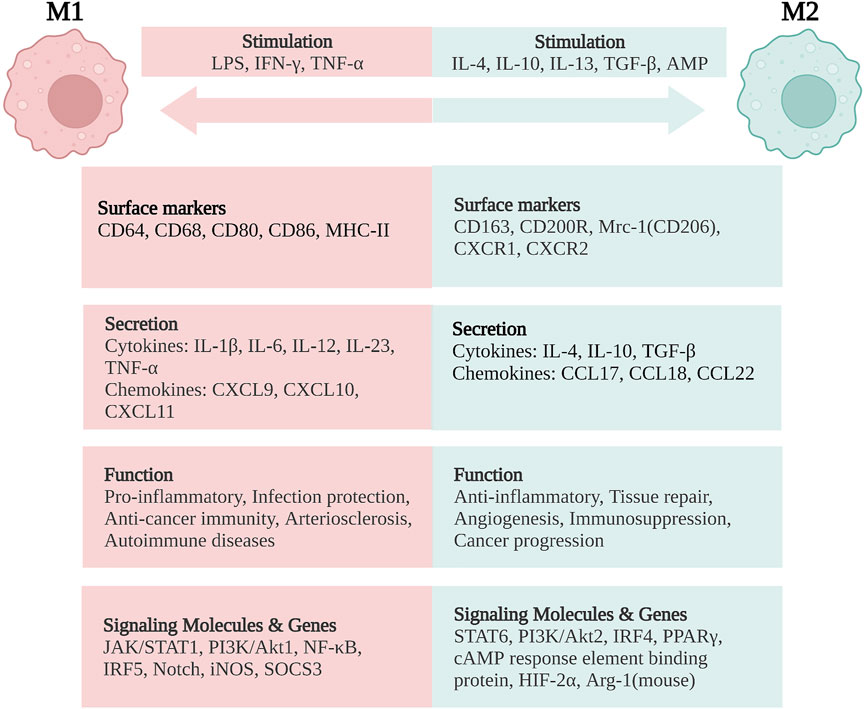
FIGURE 2. Macrophage polarization and phenotypes. M1 macrophages are stimulated by LPS, IFN-γ, and TNF-α, while M2 macrophages are stimulated by IL-4, IL-10, IL-13, TGF-β, and AMP. M1 macrophages are characterized by the functions of pro-inflammatory, infection protection, anti-cancer immunity, arteriosclerosis, and autoimmune diseases. M2 macrophages exert the functions of anti-inflammatory, tissue repair, angiogenesis, and immunosuppression, but are related with cancer progression. LPS, lipopolysaccharide; IFN, interferon; TNF, tumor necrosis factor; IL, interleukin; TGF, transforming growth factor; AMP, adenosine monophosphate; CD, cluster of differentiation; MHC, major histocompatibility complex; Mrc-1, mannose receptor C-type 1 (also known as CD206); CXCR, CXC chemokine receptor; CXCL, CXC chemokine ligand; CCL, CC chemokine ligand; JAK/STAT, Janus kinase/signal transducer and activator of transcription; PI3K/Akt, phosphatidylinositol-3-kinase/Akt; NF-κB, nuclear factor-κB; IRF, interferon regulatory factor; iNOS, inducible nitric oxide synthase; SOCS3, suppressor of cytokine signaling-3; PPARγ, peroxisome proliferator activated receptor-γ; HIF-2α, hypoxia-inducible factor-2α; Arg-1, arginase-1. Created with BioRender.com.
On the contrary, alternatively activated (M2) macrophages are usually characterized by upregulated factors such as IL-4, IL-10, mannose receptor C-type 1 (Mrc-1, also known as CD206), CD163, arginase-I, IL-1 receptor antagonist, and stabilin-1, which could prevent excessive inflammation, and promote tissue healing. M2 polarization is mainly induced by transcription factors including STAT6 (Ohmori and Hamilton, 1997; Martinez et al., 2009), PI3K/Akt2 (Lu et al., 2017; Vergadi et al., 2017), IRF4 (Satoh et al., 2010; Chistiakov et al., 2018), peroxisome proliferator-activated receptor (PPAR)γ (Bouhlel et al., 2007; Odegaard et al., 2007), and cyclic adenosine monophosphate (cAMP) response element binding protein (Ruffell et al., 2009; Luan et al., 2015). M2 macrophages promote resolving inflammation and remodeling tissue by producing several factors, especially IL-10. Some research has shown that the inhibition of M2 polarization or the synthesis of its cytokines may result in the exacerbation of colitis (Hunter et al., 2010; Zhu et al., 2014). M2 macrophages activate TGF-β by promoting Th2 responses to further promote fibrosis that is intimately involved in tissue remodeling (Murray and Wynn, 2011; Locati et al., 2013; Wynn and Vannella, 2016). In addition, M2 macrophages promote matrix metalloproteinases (MMPs) that control extracellular matrix (ECM) turnover to remove debris, apoptotic cells, and various ECM components, which could help to prevent tissue-damaging M1 macrophage responses (Murray and Wynn, 2011). Moreover, the expression of Arg1, Fizz1, and Ym1 has been shown to decrease inflammatory responses and promote tissue remodeling (Lawrence and Natoli, 2011; Kühl et al., 2015). According to different stimuli and transcriptional changes, the M2 macrophages could be subdivided into several subsets (M2a, M2b, M2c, and M2d subsets) with distinct pathophysiological characteristics (The details are summarized in Table 1) (Rőszer, 2015; Wang et al., 2019; Du et al., 2021). Based on these findings, the importance of macrophage polarization in the progression and prognosis of inflammation is becoming increasingly appreciated (Figure 2).
3.2 Intestinal Macrophages and Gut Microbiota in IBD
Gut commensal microbiota has continuous interaction with epithelial cells, which is essential in shaping the function of intestinal barrier structure (Hayes et al., 2018). The dysbiosis of gut microbiota has been considered as the key of IBD etiopathology (Gilbert et al., 2018). Pathogen/microbial-associated molecular patterns (PAMPs/MAMPs) are microbial signature molecules, which are recognized and attached by the innate intracellular receptors of macrophages. The macrophages present antigens to activate T cells, leading to the production of cytokines, chemokines, and antimicrobial peptides (AMPs) to maintain the integrity of intestinal barrier (Wells et al., 2011). Besides, the recruitment and differentiation of intestinal macrophage also need the crucial mediator of the microbiota, aside from the induction of local cytokines, growth factors, and chemokines (Fiocchi, 2008). A few of studies have shown in germ-free mice or in mice with depletion of microbiota with broad-spectrum antibiotics, could decrease the number of monocyte-derived or tissue-resident macrophages (Bain et al., 2014; Muller et al., 2014; Shaw et al., 2018). The metagenomic sequencing of microbial RNA has revealed that there are significant alterations in microbial composition, location and biodiversity between IBD patients and healthy individuals (Wallace et al., 2014).
For instance, the adherent invasive E. coli (AIEC) showed significant increased amount in IBD patients, and it is involved in IBD pathogenesis through evading the immune system of the host, linking to intestinal epithelial cells, and promoting excessive inflammation (Lloyd-Price et al., 2019). Besides, AIEC is associated with the ability to transverse the intestinal wall into the lamina propria, even survive and replicate within macrophages by escaping autophagy (Palmela et al., 2018). Campylobacter concisus (C. concisus) is a kind of adherent and invasive proteobacterium, participating in the pathogenesis of IBD (Underwood et al., 2016). Some C. concisus strains acquired zonula occludens toxin (zot) gene from a virus (prophage), damaging epithelial tight junctions, inducing macrophage production of undue pro-inflammatory cytokines, such as TNF-α, and ultimately causing the chronic relapse of IBD (Zhang et al., 2014). Akkermansia muciniphila (A. muciniphila) is a Gram-negative anaerobic bacterium, that revealed protective potential with reduced abundance both in CD and UC (Png et al., 2010). A. muciniphila or a specific outer membrane protein Amuc_1100 could improve colitis, with the decreased number of infiltrating macrophages and CD8+ cytotoxic T lymphocytes in the gut (Wang et al., 2020). In a word, it is recognized as a crucial challenge to reconstitute the relationship between the host and the microbiome to an effective symbiotic state.
3.3 Macrophage-Derived Exosome
Macrophage-derived exosome has been shown to play an important role in the pathogenesis of inflammatory exacerbation and resolution by interacting with intestinal epithelial cells (IECs) and other cells. Exosome-derived miR-21a-5p from M1 macrophages could be absorbed by IECs, correlated with downregulation of E-cadherin and activation of group 2 innate lymphoid cells, aggravating dextran sulfate sodium (DSS)-induced colitis in mice (Lu et al., 2021a). In addition, the M2 macrophage-derived exosome miR-590–3p targets large tumor suppressor kinase 1 and then activates Yes-associated protein/β-catenin transcription in IECs, hence reducing colonic inflammation, strengthening mucosal healing, and alleviating DSS-induced colitis in mice (Deng et al., 2021).
4 Regulation Mechanism of Macrophages in IBD
Generally speaking, as specialized phagocytic cells of the innate immune system, macrophages exhibit multiple functions such as the maintenance of systematic homeostasis, protective defense of the host, and regulation of inflammatory response, as well as re-establishing tissue barriers upon mucosal disruption (You et al., 2016; Dionne et al., 2017). In addition, intestinal macrophages have also been found to secrete various cytokines to maintain tissue homeostasis (Grainger et al., 2017). Here, we summarize the critical regulation mechanisms of macrophages in IBD.
CSF-1. Macrophage colony-stimulating factor 1 (CSF1) is the primary cytokine, which shows a critical role in the survival, proliferation and differentiation of intestinal macrophages. It has been demonstrated that the number of intestinal macrophages appears significant decrease in Csf1-null mice and these with the administration of anti-CSFR1 antibody (MacDonald et al., 2010). Besides, following anti-CSF1R treatment, the depletion of macrophage leads to restraining Paneth cell differentiation, reducing Lrg5+ stem cells, and ultimately impairing the development of IECs (Sehgal et al., 2018).
IL-10/IL-10R Axis. The IL-10/IL-10-receptor (IL-10R) axis is crucial in regulating phenotype of intestinal macrophages in both mice and humans. Lack of IL-10 signaling results in macrophage hyper-responsiveness to toll-like receptors (TLRs) stimulation, causing the development of spontaneous intestinal inflammation (Zigmond et al., 2014). Disrupted IL-10/IL-10R axis can affect several regulatory pathways involved in macrophage activation, such as STAT1, STAT3, nuclear factor-κB (NF-κB), and TREM-1, as well as increase chromatin accessibility to pro-inflammatory genes (Hirotani et al., 2005; Schenk et al., 2005; Simon et al., 2016). Besides, macrophage-derived IL-10 has been demonstrated that it is crucial for maintaining and promoting antigen-specific Treg cells in the intestinal mucosa in mice (Hadis et al., 2011).
CX3CL1-CX3CR1. The CX3CL1-CX3CR1 axis plays a critical role in the differentiation and function of mouse intestinal macrophages, considering that the high expression of CX3CR1 and their special localization near by the CX3CL1-positive intestinal epithelial cells. Lack of CX3CR1 expression of intestinal macrophages leads to the decrease of IL-10 and defect in promoting Treg cells in the lamina propria, which may exacerbate the DSS-induced colitis in mice (Hadis et al., 2011). In patients with CD, a missense mutation in the CX3CR1 gene was identified, which was related with an impaired antifungal ability and may lead to progression of extra-intestinal inflammatory diseases (Leonardi et al., 2018). In addition, CX3CR1+ macrophages can form transepithelial dendrites (TEDs) with the ability to capture soluble antigens or potential pathogens, and even migrate into the colonic lumen to execute the mission (Rescigno et al., 2001; Vallon-Eberhard et al., 2006).
TGF-β.The TGF-β/TGF-β-receptor (TGF-βR) axis is essential for the terminal differentiation of macrophages in intestinal mucosa, and genetic imprinting of intestinal macrophages in mice, including CX3CR1, IL-10, and αvβ5 integrin genes (Schridde et al., 2017). In line with this, the expression of the Runt-related transcription factor 3 (RUNX3), which is regulated by TGF-β, is a unique feature of intestinal macrophages (Lavin et al., 2014). In addition, macrophage turnover is regulated by the TGF-β/TGF-βR axis by controlling the expression of CCL8 (Schridde et al., 2017). Besides, efferocytosis produce the secretion of TGF-β by macrophages, restraining the production of pro-inflammatory mediators by a mechanism of autocrine/paracrine way involving TGF-β (Fadok et al., 1998).
5 Targeting Macrophages for IBD
There is a huge driving force for exploring innovative treatment options in IBD, because existing therapeutic strategies remain ineffective in many patients (Papamichael et al., 2015). It has been shown that modulating the polarization of M2 macrophages or suppressing inflammation-related cytokines could be an innovative therapeutic strategy to regulate intestinal inflammation and restore tissue homeostasis (Na et al., 2019; Du et al., 2021). Modulating the phenotypes of macrophages by inhibiting the pro-inflammatory M1 subset and/or inducing the anti-inflammatory M2 subset, may ameliorate IBD effectively (Ma et al., 2019). It has also been shown that macrophage functions can be affected and modulated by classic IBD agents (such as, infliximab or mesalazine) in many ways, for instance, by downregulating inflammatory signaling pathways and/or skewing to the M2 subpopulation (Bantel et al., 2000; Vos et al., 2012). In the following paragraphs, the current treatment strategies involving macrophages and potential new options to enhance the accumulation of the pro-resolving subpopulation in IBD are summed up in detail (Figure 3).
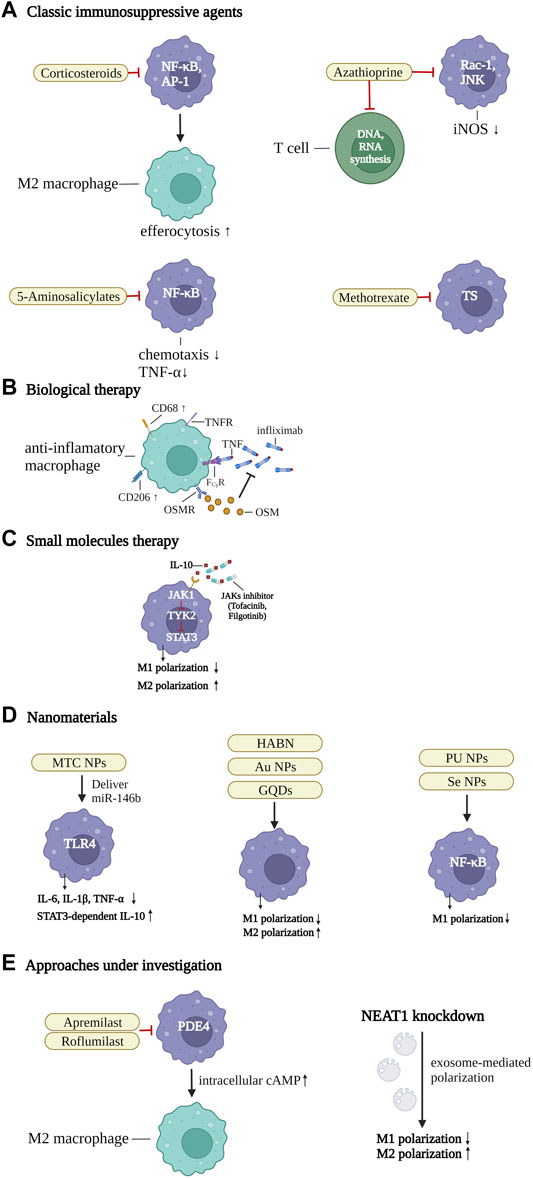
FIGURE 3. Therapeutic strategies of IBD by targeting the macrophages. (A) classic immunosuppressive agents. Corticosteroids inhibit nuclear factor-κB (NF-κB) and activator protein 1 (AP-1), thereby enhancing efferocytosis and inducing the differentiation of M2 macrophages. 5-Aminosalicylate suppresses NF-κB to downregulate TNF-α effects and control the chemotaxis of macrophages. Azathioprine not only restricts T-cell activation by abolishing DNA or RNA synthesis, but also suppresses Ras-related C3 botulinum toxin substrate 1 (Rac1) activity and the phosphorylation of JUN N-terminal kinase, and the expression of iNOS. Methotrexate regulates pro-inflammatory gene expression of macrophages, by controlling thymidylate synthase (TS), (B) biological therapy. Infliximab blocks TNF and TNF receptor (TNFR)-mediated inflammatory response in intestinal macrophages, by binding with Fcγ receptor (FcγR), and inducing CD68+CD206+ regulatory macrophages. The numbers of oncostatin M (OSM) and the receptor (OSMR-β) are associated with unresponsiveness to TNF-α blockers. (C) small molecules. JAK inhibitors inhibit the IL-10-JAK1-tyrosine kinase 2-STAT3 signaling pathway, which is crucial for maintaining mature macrophages in intestinal homeostasis. In addition, they can downregulate M1 and promote the differentiation of M2 macrophages. (D) nanomaterials. A series of macrophage targeting nanoparticles (NPs) could be synthesized to deliver specific pathway inhibitors or agonists to control inflammatory-related pathways and promote the polarization of anti-inflammatory macrophages, such as mannose-modified trimethyl chitosan (MTC) NPs, Hyaluronic acid-bilirubin nanomedicine (HABN), gold (Au) NPs, graphene quantum dots (GQDs), polyurethane (PU) NPs, and selenium (Se) NPs. (E) approaches under investigation. Apremilast and roflumilast are two new phosphodiesterase 4 (PDE4) blockers. The intracellular cAMP level was increased by inhibition of PDE4, thereby blocking inflammation-related factors and promoting pro-resolving macrophages. Inhibition of the Nuclear Enriched Abundant Transcript 1 (NEAT1), a new nuclear long non-coding RNA, can regulate the intestinal mucosal barrier and induce exosome-mediated differentiation of macrophages. Created with BioRender.com.
5.1 Classic Immunosuppressive Agents
Conventional treatment strategies usually rely on modulating the systemic immune responses by mitigating progression of mucosal inflammation. These conventional strategies are mainly based on immunomodulatory medications such as corticosteroids, aminosalicylates, antibiotics, and thiopurines, as well as folic acid antagonists (methotrexate) (Sales-Campos et al., 2015; Chen et al., 2021).
As the central medication for acute exacerbations of IBD patients, both UC and CD, corticosteroids remain the cornerstone of therapeutic options at present (Mowat et al., 2011). Corticosteroids comprise a series of drugs that exerts anti-inflammatory effects by binding to particular cytosolic receptors and suppressing transcription factors, for instance, NF-κB and activator protein 1 (Oakley and Cidlowski, 2013). Moreover, the activation of immune cells and the production of adhesion molecules are suppressed by corticosteroids in inflamed sites (Sales-Campos et al., 2015; Chen et al., 2021). In addition, corticosteroids have several effects on macrophages, ranging from enhancing efferocytosis to inducing the polarization of the M2 subpopulation (Giles et al., 2001; Ehrchen et al., 2007). Nevertheless, if corticosteroids are administrated chronically, the occurrence of side effects (e.g., osteoporosis, metabolic disease, cardiovascular syndrome), along with the non-responsive subpopulation, will appear and remain a primary obstruction to sustained remission (Ford et al., 2011; Sales-Campos et al., 2015).
5-Aminosalicylates could control the chemotaxis of macrophages or promote the proliferation of intestinal mucosa cells by downregulating TNF-α effects as well as suppressing NF-κB signaling pathways directly, which play an integral role in pro-inflammatory macrophages in UC patients (Kaiser et al., 1999; Bantel et al., 2000). In addition, 5-aminosalicylates are often combined with corticosteroids in UC patients to reinforce the anti-inflammatory effects (Allgayer, 2003).
Azathioprine and methotrexate are the other two classic immunosuppressive drugs for IBD (Feagan et al., 2000). They not only restrict T-cell activation by abolishing DNA or RNA synthesis but suppress RAC1 activity via binding to 6-thioguanine triphosphates, resulting in anti-inflammatory functions in the mouse model by inhibiting the phosphorylation of JUN N-terminal kinase and the expression of iNOS. (Elion, 1989; Marinković et al., 2014). By controlling thymidylate synthase, methotrexate regulates pro-inflammatory gene expression of macrophages (Municio et al., 2016). Based on the aforementioned evidence, macrophage-modulating strategies play an important role in treating IBD patients.
5.2 Biological Therapy
TNF-α is a pro-inflammatory mediator that can be produced by macrophages and other cells including B cells, T cells, natural killer (NK) cells, dendritic cells (DCs), neutrophils, and epithelial cells (Kany et al., 2019). Macrophages are the major producers of TNF-α and interestingly are also highly responsive to TNF-α (Parameswaran and Patial, 2010).There is increasing evidence reinforcing that TNF-α plays a crucial role in the pathogenesis of IBD (Targan et al., 1997; D'Haens et al., 1999; Rutgeerts et al., 1999). The anti-TNF treatment remains a crucial strategy for IBD patients, given that it has the ability to promote the differentiation of regulatory macrophages (Vos et al., 2012).
TNF-α blockers have been used to prevent TNF-α-mediated intestinal mucosal damage and reduce or terminate the biological effects of TNF-α. Since the anti-TNF agent infliximab was approved by U.S. Food and Drug Administration in 1997, biologics have become the primary medications in the treatment of IBD patients (Stack et al., 1997; Targan et al., 1997). Thalidomide is a TNF-α inhibitor, which has been demonstrated to be effective for inducing remission in pediatric CD and adults with refractory CD (Sabate et al., 2002; Lazzerini et al., 2013). By blocking the production of interferon-regulatory factor-5 (IRF-5), thalidomide significantly inhibits the differentiation of M1 macrophage, with downregulated surfaced markers including CD86 and CCR7, as well as decreased pro-inflammatory cytokines including IL-12 and IFN-γ, that attenuates intestinal inflammation and facilitates mucosal healing (Lu et al., 2021b). Several new anti-TNFα agents, such as adalimumab, certolizumab pegol, and golimumab, have been applied in clinical research (Danese et al., 2015; Billmeier et al., 2016; Holleran et al., 2017; Chudy-Onwugaje et al., 2019; Na et al., 2019). The ENVISION I study is a phase Ⅲ double-blind randomized controlled clinical trial (RCT) investigating the clinical efficacy of adalimumab in pediatric patients with moderate-to-severe UC (Croft et al., 2021). After 8 weeks of treatment, the adalimumab group had significantly more frequent clinical remission than the placebo group (adalimumab group vs. placebo group; 60 vs. 19.8%, p = 0.0001) (Croft et al., 2021). Therefore, moderate-to-severe UC in children could be efficaciously and safely treated by adalimumab (Croft et al., 2021).
TNF-α inhibitors can specifically bind to receptors to form immune complexes (ICs) to neutralize TNF-α. Once bound to the antibody, TNF-α receptor activation is blocked, leading to decreased permeability of cytomembranes and paracellular tight junctions, as well as blocking the accumulation of inflammatory cells in the local intestinal mucosa (Baert et al., 1999; D'Haens et al., 1999; Rutgeerts et al., 1999; Koch and Nusrat, 2012; Vos et al., 2012; Olesen et al., 2016). In addition, by hindering lipid rafts, infliximab could rebuild equilibrium between the intestinal mucosal barrier and adherent invasive E. coli in CD (Yakymenko et al., 2018). In addition, anti-TNF medications could not only reduce the secretion of inflammatory cytokines and infiltration of T cells but also skew monocytes to differentiation into regulatory macrophages (CD68+CD206+), concurrently (Vos et al., 2012; Olesen et al., 2016). Compared to anti-TNF-α monotherapy, TNF-α inhibitors combined with thiopurines have been demonstrated to promote the differentiation of regulatory macrophages and enhance immunosuppressive efficacy (Vos et al., 2012). However, a majority of IBD patients are intolerant to the therapeutic regimen of anti-TNFα combined with thiopurine, which makes the strategy difficult to carry out (Jharap et al., 2010). Hence, ongoing studies should focus on finding novel alternatives for anti-TNFα combined treatment.
In addition, anti-TNF agents can induce alternative macrophage polarization via Fcγ receptor ligation (Lucas et al., 2005; Colombel et al., 2010; Pander et al., 2011; Vos et al., 2011; Vos et al., 2012) and increase IL-10 production of macrophages (Pander et al., 2011; Vos et al., 2011; Levin et al., 2016; Bloemendaal et al., 2017; Koelink et al., 2020). After TNF-α blocker treatment, there was obvious differentiation of the M2 subpopulation in patients with mucosal healing, whereas those without mucosal healing had no such induction (Vos et al., 2012). However, the anti-inflammatory subpopulation cannot be induced by biologics without the Fc fragment, for example, certolizumab pegol, indicating that the research on novel antibody-based strategies may be a broad avenue for IBD therapy (Boyer et al., 2016).
Moreover, the expression of oncostatin M (OSM) is elevated in IBD patients and is associated with unresponsiveness to TNF-α blockers (West et al., 2017). As a member of the IL-6 cytokine family, OSM comprises IL-11, IL-31, and leukemia inhibitory factor (Rose and Bruce, 1991). When the OSM gene was deleted or blocked, experimental colitis was significantly alleviated in animal models with resistance to TNF-α blockers (West et al., 2017). It's worth noting that OSM aggravates the inflammatory response and damages the intestinal mucosal barrier via initiating the expression of cytokines, chemokines, and adhesion factors, which produce large numbers of the receptor (OSMR-β) (Pothoven and Schleimer, 2017; West et al., 2017). Thus, OSM emerges as a novel therapeutic target for IBD patients, especially for those who are non-responsive to TNF-α inhibitors, and it may also promote remodeling of the intestinal tissue.
Even if some protocols of IBD therapy use biologics in an early top-down way in high-risk CD patients, the ineffectiveness of the therapy is still an intractable problem that drives patients to increase dosage of medications or try other therapeutic regimens (Berg et al., 2019; Maronek et al., 2020; Roda et al., 2020). As mentioned above, TNF-α blockers can exert anti-inflammatory effects and remodel the intestinal mucosal barrier by inducing the polarization of M2 macrophages, but further research is needed, especially in unresponsive patients.
5.3 Small Molecules
Janus kinases (JAKs) are non-receptor tyrosine kinases that comprise JAK1, JAK2, JAK3, and tyrosine kinase 2 (TYK2) (Darnell et al., 1994). Once the JAK cytokines bond to the cell-membrane receptors, the signaling transducers with activators of transcription (STATs) are activated, leading to STAT homo-dimerization and activate downstream transcription (Boland and Vermeire, 2017). Cytokines essential for intestinal homeostasis and those that are well-described mediators of pathological responses in IBD are all dependent on JAK/STAT-mediated signaling (Villarino et al., 2017; Paramsothy et al., 2018; Salas et al., 2020). Inhibition of the JAK/STAT pathway results in a decrease in several pro-inflammatory factors, for instance, IL-6, IL-12, and IL-23 (Schwartz et al., 2017). Therefore, JAK inhibitors may represent an emerging option for treating IBD (Na et al., 2019; Salas et al., 2020).
Tofacitinib, an oral pan-JAK blocker, has recently been approved for oral treatment of moderate-to-severe active UC (Kremer et al., 2012; Machado et al., 2018; Tripathi and Feuerstein, 2019; Rogler, 2020). It inhibits JAK1 and JAK3 preferentially, parts of the tyrosine kinase family, modulates cytokine secretion, and controls immunomodulation of IBD (Shivaji et al., 2019; Sandborn et al., 2021). Three phase Ⅲ trials, with 1,732 patients in total, showed that tofacitinib was more effective efficacy than placebo in inducing and maintaining remission in patients with moderately to severely active UC (Sandborn et al., 2017). However, another two phase Ⅱ double-blind RCT in CD patients, tofacitinib did not show significant results compared with placebo (Sandborn et al., 2014; Panés et al., 2017). Patients on high steroid dosages, some distinct disease characteristics, and details in research design (e.g., no endoscopic confirmation in center) may explain why the lack of efficacy was observed in CD, as opposed to UC.
Filgotinib, a selective JAK1 inhibitor, has emerged as a potential reliable therapy strategy in IBD patients. A phase Ⅱ double-blind RCT, the FITZROY study, included patients with moderate-to-severe CD (Vermeire et al., 2017). After 10 weeks of treatment, patients who were given filgotinib (200 mg, once daily) showed significantly more frequent clinical remission than patients taking placebo (filgotinib group vs. placebo group; 47 vs. 23%, p = 0.0077) (Vermeire et al., 2017). Another phase Ⅱb/Ⅲ double-blind RCT in patients with moderate-to-severe UC, the SELECTION study, showed that filgotinib (200 mg, once daily) was well tolerated and effective in induction and maintenance of clinical remission compared with placebo (Feagan et al., 2021). A number of studies focusing on the efficacy of this JAK inhibitor in CD patients are under way. The Divergence 2 study (NCT03077412), focusing on analyzing the efficacy of filgotinib in the treatment of perianal fistula in CD, has been completed but no results have yet been reported. Another phase Ⅲ double-blind RCT, The DIVERSITY1 (NCT02914561), will evaluate the effectiveness and safety of filgotinib in induction and maintenance therapy in patients with moderate-to-severe CD groups, who are biologic-naive and biologic-experienced. In addition, the DIVERSITYLTE trial (NCT02914600), aimed at evaluating the long-term safety in CD patients, is the extension of the DIVERSITY1 study.
JAK blockers could modulate functions of T cells, but also inhibit the IL-10-JAK1-tyrosine kinase 2-STAT3 signaling pathway, which is crucial for maintaining mature macrophages in intestinal homeostasis (Pattison et al., 2012). Interestingly, recent studies have shown that tofacitinib may affect the phenotype of macrophages, which was not investigated in previous related studies (Shiratori et al., 2018; De Vries et al., 2019). Studies have found that tofacitinib can downregulate M1 and promote M2 polarization (CD206, CD163, and IL-10) in murine bone marrow-derived or blood-derived macrophages (Shiratori et al., 2018; De Vries et al., 2019). In UC patients, tofacitinib significantly inhibited the M1 pro-inflammatory pathway, resulting in reduced expression of inflammatory factors; the M2 anti-inflammatory pathway was not affected, such as IL-10 secretion and expression of CD39 and CD206, even though the M2 markers were not upregulated (Cordes et al., 2020).
Targeting multiple pro-inflammatory cytokines, especially the JAK-STAT signaling pathway, may play an important role in novel IBD strategies, especially when patients are unresponsive to current regimens. The therapeutic mechanism of macrophage involvement in JAK blockers, especially in the process of resolving intestinal inflammation, needs to be confirmed in further research. In addition, side effects of JAK blockers are still of concern, such as the threat of thromboembolic events, opportunistic infections, or unknown long-term effects. The potential value of JAK blockers in the treatment of IBD needs to be further evaluated in high-quality and long-term RCTs, based on the risk-benefit ratio criterion.
5.4 Nanomaterials
Traditional medical regimens mainly rely on immunosuppressive agents, which could result in off-target systemic adverse effects and toxic reactions. As an emerging and prospective strategy, nanotechnology could be used for targeted delivery of medications to the inflamed lesions, enhancing local concentration as well as minimizing systematic adverse effects (Hur et al., 2012; Beloqui et al., 2016; Sohail et al., 2019). It has been shown that macrophage-targeted drug delivery systems can improve drug-delivery efficiency and decrease side effects by making full use of the phagocytic function of macrophages. This strategy has been widely explored in rheumatoid arthritis (Barrera et al., 2000), adiposis (Bu et al., 2013), and malignant tumors such as hepatocellular carcinoma and melanoma (Piaggio et al., 2016).
Intriguingly, macrophage-targeting nanoparticles have been synthesized to deliver inhibitors or agonists of inflammation-related pathways to modulate transition of macrophage phenotype (Singh et al., 2017). Nanomaterials-based therapeutics have shown great promise in the therapy of IBD, because of their useful properties in food and medicine (Cui et al., 2020). For example, miR-146b can be specifically delivered to intestinal macrophages by mannose-modified trimethyl chitosan (MTC)-conjugated nanoparticles (NPs), resulting in remarkable restoration of mucosal barrier function in the mice models (Deng et al., 2019). MTC-NPs can dramatically inhibit the activation of the M1 subset and induce the M2 phenotype by suppressing the TLR4 signaling pathway, thereby inhibiting the secretion of IL-6, IL-1β, and TNF-α (Deng et al., 2019). Moreover, by producing STAT3-dependent IL-10, the proliferation of co-cultured colonic epithelial cells was significantly increased (Deng et al., 2019). In addition, surface-functionalized polyurethane (PU) NPs showed remarkable immunosuppression of THP-1 macrophages, suppressing the secretion of inflammation-related cytokines in phenotypic M1, controlling the NF-κB signaling pathways, and ultimately modulating the differentiation of macrophages (Huang et al., 2018; Nakkala et al., 2021). Additionally, oral nanomedicine delivery systems for treating IBD have great promise, because of their expandability, biocompatibility, and potential targeted efficacy. Hyaluronic acid-bilirubin nanomedicine (HABN) is responsive to ROS, accumulating in inflammatory mucosa and remodeling the colonic tissue in experimental colitis. Importantly, HABN showed significant treatment efficacy via differentiating pro-inflammatory M1-like subtypes into the M2-like subtypes and elevating the expression of anti-inflammatory phenotypes such as CD3+CD4+Foxp3+ Tregs and CD11b+Ly6C−Ly6G−MHCII+ tissue-resident macrophages (Lee Y. et al., 2020; Xiao et al., 2021).
In recent years, metal-based engineered nanomaterials have shown advantageous properties in medicine. A classical case is that gold NPs (AuNPs) can induce M2 macrophage polarization, leading to inhibition of pro-inflammatory cytokines and increasing IL-10, Arg1, and PPARγ with decreased iNOS (Taratummarat et al., 2018). In addition, lines of evidence have demonstrated that nonmetal-based nanomaterials are capable of regulating macrophage phenotype. For example, graphene quantum dots (GQDs) could effectively alleviate intestinal inflammation and prevent tissue degeneration, via suppressing TH1/TH17 polarization (Lee B.-C. et al., 2020). Furthermore, GQDs can not only switch the differentiation of macrophages from pro-inflammatory M1 to anti-inflammatory M2 but also induce infiltration of intestinal Tregs (Lee B.-C. et al., 2020). A similar study also investigated the effect of nonmetal-based nanomaterials on macrophage polarization by using selenium (Se) (Zhu et al., 2017). Se is an indispensable nutrient and its deceased level may be associated with the exacerbation of IBD (Castro Aguilar-Tablada et al., 2016; Zhu et al., 2017; Ala and Kheyri, 2021). SeNPs have anti-inflammatory activity and low toxicity. Mechanistically, by inhibiting the nuclear translocation of NF-κB, SeNPs strongly suppressed M1 subsets, leading to alleviation of DSS-induced acute colitis in mice models (Zhu et al., 2017). Nanomaterials could be a promising therapeutic strategy for IBD with great potential in the near future.
5.5 Approaches Under Investigation
Inflammatory events are like a complex cascade, modulated by various cytokines and chemokines. Enhancing the pro-resolving phenotype by pharmacological modulation of the Inflammation-related molecules is of high importance to modulate macrophages in IBD. cAMP plays a key role in the modulation of inflammation-related process. Phosphodiesterase-4 (PDE4) is a critical enzyme, regulating intracellular signaling by controlling cAMP, emerging as a novel therapeutic target. Apremilast and roflumilast are two new PDE4 blockers, introduced as new regulators of intracellular signaling pathways in treating IBD patients (Spadaccini et al., 2017; Sabino et al., 2019). The intracellular cAMP level was upregulated by inhibition of PDE4, thereby blocking inflammation-related factors and increasing secretion of anti-inflammatory proteins (Mazur et al., 2015; Spadaccini et al., 2017). Moreover, a double-blind phase II trial of apremilast in patients with active UC showed significant remission of clinical symptoms, changes in endoscopic features, and decrease in markers of inflammation than in the placebo group (Danese et al., 2020).
Nuclear Enriched Abundant Transcript 1 (NEAT1), a new nuclear long non-coding RNA, anchors on particular nuclear structures and participates in various aspects of the immune process (Liu et al., 2018). It has been demonstrated that suppression of the NEAT1 can regulate the intestinal mucosal barrier and induce exosome-mediated differentiation of macrophages, thereby inhibiting the inflammatory response in IBD (Liu et al., 2018).
Targeting PDE4 or NEAT1 might represent a novel strategy in the future, but more high-quality clinical trials are still required to be carried out.
Summary and Prospect
IBD is a prevalent gastrointestinal inflammatory disease, characterized by chronic and excessive inflammation, alternates states of relapse and remission, and needs for lifelong medical therapy (Hazel and O'Connor, 2020). Therefore, new strategies to prevent inflammatory relapse, tissular damage, and non-responsiveness to medications, are urgently needed.
The infiltration and activation of macrophages can phagocytize pathogens, as well as produce various cytokines under certain circumstances, and cooperate with distinct immune cells in many aspects of the pathogenesis of IBD. Disequilibrium of macrophage polarization results in the exacerbation of IBD, and the production of particular cytokines and/or chemokines relies on the ratio of the pro-inflammatory M1 and anti-inflammatory M2 subsets. Therefore, targeted therapy of macrophages is a novel option to modulate the immune microenvironment and remodel intestinal tissue.
In recent years, remarkable advances have been achieved in the understanding of intestinal macrophage immunobiology. The advancement of single-cell sequencing technologies and fate-mapping approaches has helped to confirm new subsets and distinct transcriptional profiles of intestinal macrophages (Ginhoux et al., 2010; Yona et al., 2013; Bian et al., 2020). Emerging evidence strongly points to an accumulation in the pro-inflammatory monocyte/macrophage in the chronic relapse and remission in patients with IBD. Therefore, it is very crucial to elucidate the mechanisms of new molecules and signaling pathways that participate in the polarization and increase of pro-resolving macrophages. However, many aspects of intestinal macrophages are still under-investigated, including mechanisms underlying macrophage polarization and heterogeneity, the signaling pathways of macrophage functions, environmental cues regulating phenotypes, and interactions during the process of IBD. For instance, it will be crucial to explore the characteristics of distinct subpopulations of macrophages, particularly the tissue-resident macrophages deriving from the embryo. This will facilitate finding out differences among distinct macrophages, expanding our comprehension of the functions of macrophages. In addition, the identification of microenvironmental factors that modulate phenotypes and functions of intestinal macrophage has helped us to understand their fundamental role in homeostasis and autoimmune disease. Moreover, research on cross-talk of intestinal macrophages, gut microflora, and metabolites will be conducive to decreasing chronic inflammation. It is also crucial that future investigations probe the synergistic mechanisms of cross-talk between macrophages and other immune cells in IBD.
Nanomedicine-based approaches have emerged as novel and targeted therapeutic strategies for IBD, by improving delivery efficiency to the intestinal inflammatory sites (Hua et al., 2015; Giron et al., 2019). Even though potential application of NPs has been broadly investigated in mammalian models in vitro or in vivo, human studies are still insufficient, and some findings in humans are distinct from the ones observed in murine models to some degree (Nunes et al., 2019). In addition, since the studies of toxicity and interaction of NPs have been mainly observed in animal models, efficacy in human intestinal cells is not fully applicable. The complexity of NP design and suitable methods for administration are two main difficulties with NPs, which impede the manufacturing process. Up to now, there has been no optimal size range of NPs determined to enable effective delivery to the cells in inflamed sites. In addition, the fate of the NPs after the body’s uptake is still unknown, because the majority of the studies have focused on delivery efficiency but not safety of the nanoparticle. Safety issues should be addressed first before moving toward clinical studies. Moreover, evaluation of the effectiveness of drug delivery is also critical. Using non-invasive methods, such as positron emission tomography, to image and trace macrophages in pharmacological responses is a potential option to evaluate the safety and delivery efficacy (Weissleder et al., 2014; Kim et al., 2018; Heo et al., 2019).
Many investigations have explored inventing novel medications and repurposing classical drugs, as well as searching for alternative strategies or improving the existing ones in the effort to eradicate IBD. More efforts are needed to create new strategies for treatment options, incorporating existing therapies, and adjusting the therapeutic schedules to better fit individualized treatment. Hence, future research needs to focus on translating the results from pre-clinical studies to be further explored in clinical trials, ultimately benefiting patients with IBD.
Author Contributions
SM contributed significantly to complete manuscript writing. JZ and HL contributed to the constructive discussions. SL and QW contributed to the supervision of the review. All authors read and approved the submitted version.
Funding
This work has been supported by the grants from National Natural Science Foundation of China (82002498), Jilin Provincial Finance Department (JLSWSRCZX 2020–0016), and Jilin Provincial Science and Technology Department (20210508042RQ).
Conflict of Interest
The authors declare that the research was conducted in the absence of any commercial or financial relationships that could be construed as a potential conflict of interest.
Publisher’s Note
All claims expressed in this article are solely those of the authors and do not necessarily represent those of their affiliated organizations, or those of the publisher, the editors and the reviewers. Any product that may be evaluated in this article, or claim that may be made by its manufacturer, is not guaranteed or endorsed by the publisher.
References
Aggarwal, N. R., King, L. S., and D'Alessio, F. R. (2014). Diverse Macrophage Populations Mediate Acute Lung Inflammation and Resolution. Am. J. Physiology-Lung Cell. Mol. Physiology 306 (8), L709–L725. doi:10.1152/ajplung.00341.2013
Ala, M., and Kheyri, Z. (2021). The Rationale for Selenium Supplementation in Inflammatory Bowel Disease: A Mechanism-Based Point of View. Nutrition 85, 111153. doi:10.1016/j.nut.2021.111153
Allgayer, H. (2003). Review Article: Mechanisms of Action of Mesalazine in Preventing Colorectal Carcinoma in Inflammatory Bowel Disease. Aliment. Pharmacol. Ther. 18 (Suppl. 2), 10–14. doi:10.1046/j.1365-2036.18.s2.1.x
Ananthakrishnan, A. N. (2015). Epidemiology and Risk Factors for IBD. Nat. Rev. Gastroenterol. Hepatol. 12 (4), 205–217. doi:10.1038/nrgastro.2015.34
Baert, F. J., D'Haens, G. R., Peeters, M., Hiele, M. I., Schaible, T. F., Shealy, D., et al. (1999). Tumor Necrosis Factor α Antibody (Infliximab) Therapy Profoundly Down-Regulates the Inflammation in Crohn's Ileocolitis. Gastroenterology 116 (1), 22–28. doi:10.1016/s0016-5085(99)70224-6
Bailey, J. R., Bland, P. W., Tarlton, J. F., Peters, I., Moorghen, M., Sylvester, P. A., et al. (2012). IL-13 Promotes Collagen Accumulation in Crohn's Disease Fibrosis by Down-Regulation of Fibroblast MMP Synthesis: a Role for Innate Lymphoid Cells? PLoS One 7 (12), e52332. doi:10.1371/journal.pone.0052332
Bain, C. C., Bravo-Blas, A., Scott, C. L., Gomez Perdiguero, E., Geissmann, F., Henri, S., et al. (2014). Constant Replenishment from Circulating Monocytes Maintains the Macrophage Pool in the Intestine of Adult Mice. Nat. Immunol. 15 (10), 929–937. doi:10.1038/ni.2967
Bain, C. C., and Mowat, A. M. (2014). Macrophages in Intestinal Homeostasis and Inflammation. Immunol. Rev. 260 (1), 102–117. doi:10.1111/imr.12192
Bain, C. C., Scott, C. L., Uronen-Hansson, H., Gudjonsson, S., Jansson, O., Grip, O., et al. (2013). Resident and Pro-inflammatory Macrophages in the Colon Represent Alternative Context-dependent Fates of the Same Ly6Chi Monocyte Precursors. Mucosal Immunol. 6 (3), 498–510. doi:10.1038/mi.2012.89
Bantel, H., Berg, C., Vieth, M., Stolte, M., Kruis, W., and Schulze-Osthoff, K. (2000). Mesalazine Inhibits Activation of Transcription Factor Nf-Κb in Inflamed Mucosa of Patients with Ulcerative Colitis. Am. J. Gastroenterol. 95 (12), 3452–3457. doi:10.1111/j.1572-0241.2000.03360.x
Barrera, P., Blom, A., van Lent, P. L. E. M., van Bloois, L., Beijnen, J. H., van Rooijen, N., et al. (2000). Synovial Macrophage Depletion with Clodronate‐containing Liposomes in Rheumatoid Arthritis. Arthritis & Rheumatism 43 (9), 1951–1959. doi:10.1002/1529-0131(200009)43:9<1951::Aid-anr5>3.0.Co;2-k
Beloqui, A., Memvanga, P. B., Coco, R., Reimondez-Troitiño, S., Alhouayek, M., Muccioli, G. G., et al. (2016). A Comparative Study of Curcumin-Loaded Lipid-Based Nanocarriers in the Treatment of Inflammatory Bowel Disease. Colloids Surfaces B Biointerfaces 143, 327–335. doi:10.1016/j.colsurfb.2016.03.038
Berg, D. R., Colombel, J.-F., and Ungaro, R. (2019). The Role of Early Biologic Therapy in Inflammatory Bowel Disease. Inflamm. Bowel Dis. 25 (12), 1896–1905. doi:10.1093/ibd/izz059
Bernardo, D., Marin, A. C., Fernández-Tomé, S., Montalban-Arques, A., Carrasco, A., Tristán, E., et al. (2018). Human Intestinal Pro-inflammatory CD11chighCCR2+CX3CR1+ Macrophages, but Not Their Tolerogenic CD11c−CCR2−CX3CR1− Counterparts, Are Expanded in Inflammatory Bowel Disease. Mucosal Immunol. 11 (4), 1114–1126. doi:10.1038/s41385-018-0030-7
Bian, Z., Gong, Y., Huang, T., Lee, C. Z. W., Bian, L., Bai, Z., et al. (2020). Deciphering Human Macrophage Development at Single-Cell Resolution. Nature 582 (7813), 571–576. doi:10.1038/s41586-020-2316-7
Billmeier, U., Dieterich, W., Neurath, M. F., and Atreya, R. (2016). Molecular Mechanism of Action of Anti-tumor Necrosis Factor Antibodies in Inflammatory Bowel Diseases. Wjg 22 (42), 9300–9313. doi:10.3748/wjg.v22.i42.9300
Bloemendaal, F. M., Levin, A. D., Wildenberg, M. E., Koelink, P. J., McRae, B. L., Salfeld, J., et al. (2017). Anti-Tumor Necrosis Factor with a Glyco-Engineered Fc-Region Has Increased Efficacy in Mice with Colitis. Gastroenterology 153 (5), 1351–1362. e1354. doi:10.1053/j.gastro.2017.07.021
Boland, B. S., and Vermeire, S. (2017). Janus Kinase Antagonists and Other Novel Small Molecules for the Treatment of Crohn's Disease. Gastroenterology Clin. N. Am. 46 (3), 627–644. doi:10.1016/j.gtc.2017.05.015
Bouhlel, M. A., Derudas, B., Rigamonti, E., Dièvart, R., Brozek, J., Haulon, S., et al. (2007). PPARγ Activation Primes Human Monocytes into Alternative M2 Macrophages with Anti-inflammatory Properties. Cell Metab. 6 (2), 137–143. doi:10.1016/j.cmet.2007.06.010
Boyer, J. F., Baron, M., Constantin, A., Degboé, Y., Cantagrel, A., and Davignon, J.-L. (2016). Anti-TNF Certolizumab Pegol Induces Antioxidant Response in Human Monocytes via Reverse Signaling. Arthritis Res. Ther. 18, 56. doi:10.1186/s13075-016-0955-8
Bu, L., Gao, M., Qu, S., and Liu, D. (2013). Intraperitoneal Injection of Clodronate Liposomes Eliminates Visceral Adipose Macrophages and Blocks High-Fat Diet-Induced Weight Gain and Development of Insulin Resistance. Aaps J. 15 (4), 1001–1011. doi:10.1208/s12248-013-9501-7
Bujko, A., Atlasy, N., Landsverk, O. J. B., Richter, L., Yaqub, S., Horneland, R., et al. (2018). Transcriptional and Functional Profiling Defines Human Small Intestinal Macrophage Subsets. J. Exp. Med. 215 (2), 441–458. doi:10.1084/jem.20170057
Casanova, J.-L., and Abel, L. (2009). Revisiting Crohn's Disease as a Primary Immunodeficiency of Macrophages. J. Exp. Med. 206 (9), 1839–1843. doi:10.1084/jem.20091683
Castro Aguilar-Tablada, T., Navarro-Alarcón, M., Quesada Granados, J., Samaniego Sánchez, C., Rufián-Henares, J., and Nogueras-Lopez, F. (2016). Ulcerative Colitis and Crohn's Disease Are Associated with Decreased Serum Selenium Concentrations and Increased Cardiovascular Risk. Nutrients 8 (12), 780. doi:10.3390/nu8120780
Chen, F., Liu, Q., Xiong, Y., and Xu, L. (2021). Current Strategies and Potential Prospects of Nanomedicine-Mediated Therapy in Inflammatory Bowel Disease. Ijn 16, 4225–4237. doi:10.2147/ijn.S310952
Chistiakov, D. A., Myasoedova, V. A., Revin, V. V., Orekhov, A. N., and Bobryshev, Y. V. (2018). The Impact of Interferon-Regulatory Factors to Macrophage Differentiation and Polarization into M1 and M2. Immunobiology 223 (1), 101–111. doi:10.1016/j.imbio.2017.10.005
Chudy-Onwugaje, K. O., Christian, K. E., Farraye, F. A., and Cross, R. K. (2019). A State-Of-The-Art Review of New and Emerging Therapies for the Treatment of IBD. Inflamm. Bowel Dis. 25 (5), 820–830. doi:10.1093/ibd/izy327
Colombel, J. F., Sandborn, W. J., Reinisch, W., Mantzaris, G. J., Kornbluth, A., Rachmilewitz, D., et al. (2010). Infliximab, Azathioprine, or Combination Therapy for Crohn's Disease. N. Engl. J. Med. 362 (15), 1383–1395. doi:10.1056/NEJMoa0904492
Cook, M. G., and Dixon, M. F. (1973). An Analysis of the Reliability of Detection and Diagnostic Value of Various Pathological Features in Crohn's Disease and Ulcerative Colitis. Gut 14 (4), 255–262. doi:10.1136/gut.14.4.255
Cordes, F., Lenker, E., Spille, L. J., Weinhage, T., Bettenworth, D., Kessel, C., et al. (2020). Tofacitinib Reprograms Human Monocytes of IBD Patients and Healthy Controls toward a More Regulatory Phenotype. Inflamm. Bowel Dis. 26 (3), 391–406. doi:10.1093/ibd/izz213
Cosín-Roger, J., Ortiz-Masiá, D., Calatayud, S., Hernández, C., Álvarez, A., Hinojosa, J., et al. (2013). M2 Macrophages Activate WNT Signaling Pathway in Epithelial Cells: Relevance in Ulcerative Colitis. PLoS One 8 (10), e78128. doi:10.1371/journal.pone.0078128
Croft, N. M., Faubion, W. A., Kugathasan, S., Kierkus, J., Ruemmele, F. M., Shimizu, T., et al. (2021). Efficacy and Safety of Adalimumab in Paediatric Patients with Moderate-To-Severe Ulcerative Colitis (ENVISION I): a Randomised, Controlled, Phase 3 Study. Lancet Gastroenterology Hepatology 6 (8), 616–627. doi:10.1016/s2468-1253(21)00142-4
Cui, X., Bao, L., Wang, X., and Chen, C. (2020). The Nano-Intestine Interaction: Understanding the Location‐Oriented Effects of Engineered Nanomaterials in the Intestine. Small 16 (21), 1907665. doi:10.1002/smll.201907665
D'Haens, G., Van Deventer, S., Van Hogezand, R., Chalmers, D., Kothe, C., Baert, F., et al. (1999). Endoscopic and Histological Healing with Infliximab Anti-tumor Necrosis Factor Antibodies in Crohn's Disease: A European Multicenter Trial. Gastroenterology 116 (5), 1029–1034. doi:10.1016/s0016-5085(99)70005-3
Danese, S., Neurath, M. F., Kopoń, A., Zakko, S. F., Simmons, T. C., Fogel, R., et al. (2020). Effects of Apremilast, an Oral Inhibitor of Phosphodiesterase 4, in a Randomized Trial of Patients with Active Ulcerative Colitis. Clin. Gastroenterology Hepatology 18 (11), 2526–2534. e2529. doi:10.1016/j.cgh.2019.12.032
Danese, S., Vuitton, L., and Peyrin-Biroulet, L. (2015). Biologic Agents for IBD: Practical Insights. Nat. Rev. Gastroenterol. Hepatol. 12 (9), 537–545. doi:10.1038/nrgastro.2015.135
Darnell, J. E., Kerr, l. M., and Stark, G. R. (1994). Jak-STAT Pathways and Transcriptional Activation in Response to IFNs and Other Extracellular Signaling Proteins. Science 264 (5164), 1415–1421. doi:10.1126/science.8197455
De Schepper, S., Verheijden, S., Aguilera-Lizarraga, J., Viola, M. F., Boesmans, W., Stakenborg, N., et al. (2018). Self-Maintaining Gut Macrophages Are Essential for Intestinal Homeostasis. Cell 175 (2), 400–415. e413. doi:10.1016/j.cell.2018.07.048
de Souza, H. S. P., and Fiocchi, C. (2016). Immunopathogenesis of IBD: Current State of the Art. Nat. Rev. Gastroenterol. Hepatol. 13 (1), 13–27. doi:10.1038/nrgastro.2015.186
De Vries, L. C. S., Duarte, J. M., De Krijger, M., Welting, O., Van Hamersveld, P. H. P., Van Leeuwen-Hilbers, F. W. M., et al. (2019). A JAK1 Selective Kinase Inhibitor and Tofacitinib Affect Macrophage Activation and Function. Inflamm. Bowel Dis. 25 (4), 647–660. doi:10.1093/ibd/izy364
Deng, F., He, S., Cui, S., Shi, Y., Tan, Y., Li, Z., et al. (2019). A Molecular Targeted Immunotherapeutic Strategy for Ulcerative Colitis via Dual-Targeting Nanoparticles Delivering miR-146b to Intestinal Macrophages. J. Crohns Colitis 13 (4), 482–494. doi:10.1093/ecco-jcc/jjy181
Deng, F., Yan, J., Lu, J., Luo, M., Xia, P., Liu, S., et al. (2021). M2 Macrophage-Derived Exosomal miR-590-3p Attenuates DSS-Induced Mucosal Damage and Promotes Epithelial Repair via the LATS1/YAP/ β-Catenin Signalling Axis. J. Crohns Colitis 15 (4), 665–677. doi:10.1093/ecco-jcc/jjaa214
Dionne, S., Duchatelier, C.-F., and Seidman, E. G. (2017). The Influence of Vitamin D on M1 and M2 Macrophages in Patients with Crohn's Disease. Innate Immun. 23 (6), 557–565. doi:10.1177/1753425917721965
Du, Y., Rong, L., Cong, Y., Shen, L., Zhang, N., and Wang, B. (2021). Macrophage Polarization: an Effective Approach to Targeted Therapy of Inflammatory Bowel Disease. Expert Opin. Ther. Targets 25 (3), 1–19. doi:10.1080/14728222.2021.1901079
Ehrchen, J., Steinmüller, L., Barczyk, K., Tenbrock, K., Nacken, W., Eisenacher, M., et al. (2007). Glucocorticoids Induce Differentiation of a Specifically Activated, Anti-inflammatory Subtype of Human Monocytes. Blood 109 (3), 1265–1274. doi:10.1182/blood-2006-02-001115
Elion, G. B. (1989). The Purine Path to Chemotherapy. Science 244 (4900), 41–47. doi:10.1126/science.2649979
Elliott, M. R., Koster, K. M., and Murphy, P. S. (2017). Efferocytosis Signaling in the Regulation of Macrophage Inflammatory Responses. J. I. 198 (4), 1387–1394. doi:10.4049/jimmunol.1601520
Elliott, T. R., Rayment, N. B., Hudspith, B. N., Hands, R. E., Taylor, K., Parkes, G. C., et al. (2015). Lamina Propria Macrophage Phenotypes in Relation to Escherichia coli in Crohn's Disease. BMC Gastroenterol. 15, 75. doi:10.1186/s12876-015-0305-3
Epelman, S., Lavine, K. J., and Randolph, G. J. (2014). Origin and Functions of Tissue Macrophages. Immunity 41 (1), 21–35. doi:10.1016/j.immuni.2014.06.013
Fadok, V. A., Bratton, D. L., Konowal, A., Freed, P. W., Westcott, J. Y., and Henson, P. M. (1998). Macrophages that Have Ingested Apoptotic Cells In Vitro Inhibit Proinflammatory Cytokine Production through Autocrine/paracrine Mechanisms Involving TGF-Beta, PGE2, and PAF. J. Clin. Invest. 101 (4), 890–898. doi:10.1172/jci1112
Feagan, B. G., Danese, S., Loftus, E. V., Vermeire, S., Schreiber, S., Ritter, T., et al. (2021). Filgotinib as Induction and Maintenance Therapy for Ulcerative Colitis (SELECTION): a Phase 2b/3 Double-Blind, Randomised, Placebo-Controlled Trial. Lancet 397 (10292), 2372–2384. doi:10.1016/s0140-6736(21)00666-8
Feagan, B. G., Fedorak, R. N., Irvine, E. J., Wild, G., Sutherland, L., Steinhart, A. H., et al. (2000). A Comparison of Methotrexate with Placebo for the Maintenance of Remission in Crohn's Disease. N. Engl. J. Med. 342 (22), 1627–1632. doi:10.1056/nejm200006013422202
Fichtner-Feigl, S., Strober, W., Kawakami, K., Puri, R. K., and Kitani, A. (2006). IL-13 Signaling through the IL-13α2 Receptor Is Involved in Induction of TGF-Β1 Production and Fibrosis. Nat. Med. 12 (1), 99–106. doi:10.1038/nm1332
Fiocchi, C. (2008). What Is "physiological" Intestinal Inflammation and How Does it Differ from "pathological" Inflammation? Inflamm. Bowel Dis. 14 (Suppl. 2), S77–S78. doi:10.1002/ibd.20618
Ford, A. C., Bernstein, C. N., Khan, K. J., Abreu, M. T., Marshall, J. K., Talley, N. J., et al. (2011). Glucocorticosteroid Therapy in Inflammatory Bowel Disease: Systematic Review and Meta-Analysis. Am. J. Gastroenterol. 106 (4), 590–599. doi:10.1038/ajg.2011.70
Funes, S. C., Rios, M., Escobar-Vera, J., and Kalergis, A. M. (2018). Implications of Macrophage Polarization in Autoimmunity. Immunology 154 (2), 186–195. doi:10.1111/imm.12910
Garrett, W. S., Gordon, J. I., and Glimcher, L. H. (2010). Homeostasis and Inflammation in the Intestine. Cell 140 (6), 859–870. doi:10.1016/j.cell.2010.01.023
Gilbert, J. A., Blaser, M. J., Caporaso, J. G., Jansson, J. K., Lynch, S. V., and Knight, R. (2018). Current Understanding of the Human Microbiome. Nat. Med. 24 (4), 392–400. doi:10.1038/nm.4517
Giles, K. M., Ross, K., Rossi, A. G., Hotchin, N. A., Haslett, C., and Dransfield, I. (2001). Glucocorticoid Augmentation of Macrophage Capacity for Phagocytosis of Apoptotic Cells Is Associated with Reduced p130Cas Expression, Loss of Paxillin/pyk2 Phosphorylation, and High Levels of Active Rac. J. Immunol. 167 (2), 976–986. doi:10.4049/jimmunol.167.2.976
Ginhoux, F., Greter, M., Leboeuf, M., Nandi, S., See, P., Gokhan, S., et al. (2010). Fate Mapping Analysis Reveals that Adult Microglia Derive from Primitive Macrophages. Science 330 (6005), 841–845. doi:10.1126/science.1194637
Ginhoux, F., and Guilliams, M. (2016). Tissue-Resident Macrophage Ontogeny and Homeostasis. Immunity 44 (3), 439–449. doi:10.1016/j.immuni.2016.02.024
Giron, F., Pastó, A., Tasciotti, E., and Abraham, B. P. (2019). Nanotechnology in the Treatment of Inflammatory Bowel Disease. Inflamm. Bowel Dis. 25 (12), 1871–1880. doi:10.1093/ibd/izz205
Gordon, S. (2003). Alternative Activation of Macrophages. Nat. Rev. Immunol. 3 (1), 23–35. doi:10.1038/nri978
Gordon, S., and Martinez, F. O. (2010). Alternative Activation of Macrophages: Mechanism and Functions. Immunity 32 (5), 593–604. doi:10.1016/j.immuni.2010.05.007
Graham, D. B., and Xavier, R. J. (2020). Pathway Paradigms Revealed from the Genetics of Inflammatory Bowel Disease. Nature 578 (7796), 527–539. doi:10.1038/s41586-020-2025-2
Grainger, J. R., Konkel, J. E., Zangerle-Murray, T., and Shaw, T. N. (2017). Macrophages in Gastrointestinal Homeostasis and Inflammation. Pflugers Arch. - Eur. J. Physiol. 469 (3-4), 527–539. doi:10.1007/s00424-017-1958-2
Guilliams, M., De Kleer, I., Henri, S., Post, S., Vanhoutte, L., De Prijck, S., et al. (2013). Alveolar Macrophages Develop from Fetal Monocytes that Differentiate into Long-Lived Cells in the First Week of Life via GM-CSF. J. Exp. Med. 210 (10), 1977–1992. doi:10.1084/jem.20131199
Guilliams, M., and Scott, C. L. (2017). Does Niche Competition Determine the Origin of Tissue-Resident Macrophages? Nat. Rev. Immunol. 17 (7), 451–460. doi:10.1038/nri.2017.42
Guilliams, M., and Svedberg, F. R. (2021). Does Tissue Imprinting Restrict Macrophage Plasticity? Nat. Immunol. 22 (2), 118–127. doi:10.1038/s41590-020-00849-2
Hadis, U., Wahl, B., Schulz, O., Hardtke-Wolenski, M., Schippers, A., Wagner, N., et al. (2011). Intestinal Tolerance Requires Gut Homing and Expansion of FoxP3+ Regulatory T Cells in the Lamina Propria. Immunity 34 (2), 237–246. doi:10.1016/j.immuni.2011.01.016
Hashimoto, D., Chow, A., Noizat, C., Teo, P., Beasley, M. B., Leboeuf, M., et al. (2013). Tissue-Resident Macrophages Self-Maintain Locally throughout Adult Life with Minimal Contribution from Circulating Monocytes. Immunity 38 (4), 792–804. doi:10.1016/j.immuni.2013.04.004
Hayes, C. L., Dong, J., Galipeau, H. J., Jury, J., McCarville, J., Huang, X., et al. (2018). Commensal Microbiota Induces Colonic Barrier Structure and Functions that Contribute to Homeostasis. Sci. Rep. 8 (1), 14184. doi:10.1038/s41598-018-32366-6
Hazel, K., and O’Connor, A. (2020). Emerging Treatments for Inflammatory Bowel Disease. Ther. Adv. Chronic Dis. 11, 204062231989929. doi:10.1177/2040622319899297
Hedrich, C. M., and Bream, J. H. (2010). Cell Type-specific Regulation of IL-10 Expression in Inflammation and Disease. Immunol. Res. 47 (1-3), 185–206. doi:10.1007/s12026-009-8150-5
Heo, G. S., Kopecky, B., Sultan, D., Ou, M., Feng, G., Bajpai, G., et al. (2019). Molecular Imaging Visualizes Recruitment of Inflammatory Monocytes and Macrophages to the Injured Heart. Circ. Res. 124 (6), 881–890. doi:10.1161/circresaha.118.314030
Hirotani, T., Lee, P. Y., Kuwata, H., Yamamoto, M., Matsumoto, M., Kawase, I., et al. (2005). The Nuclear IκB Protein IκBNS Selectively Inhibits Lipopolysaccharide-Induced IL-6 Production in Macrophages of the Colonic Lamina Propria. J. Immunol. 174 (6), 3650–3657. doi:10.4049/jimmunol.174.6.3650
Hoeffel, G., Wang, Y., Greter, M., See, P., Teo, P., Malleret, B., et al. (2012). Adult Langerhans Cells Derive Predominantly from Embryonic Fetal Liver Monocytes with a Minor Contribution of Yolk Sac-Derived Macrophages. J. Exp. Med. 209 (6), 1167–1181. doi:10.1084/jem.20120340
Holleran, G., Lopetuso, L., Petito, V., Graziani, C., Ianiro, G., McNamara, D., et al. (2017). The Innate and Adaptive Immune System as Targets for Biologic Therapies in Inflammatory Bowel Disease. Ijms 18 (10), 2020. doi:10.3390/ijms18102020
Hu, X., Herrero, C., Li, W.-P., Antoniv, T. T., Falck-Pedersen, E., Koch, A. E., et al. (2002). Sensitization of IFN-γ Jak-STAT Signaling during Macrophage Activation. Nat. Immunol. 3 (9), 859–866. doi:10.1038/ni828
Hua, S., Marks, E., Schneider, J. J., and Keely, S. (2015). Advances in Oral Nano-Delivery Systems for Colon Targeted Drug Delivery in Inflammatory Bowel Disease: Selective Targeting to Diseased versus Healthy Tissue. Nanomedicine Nanotechnol. Biol. Med. 11 (5), 1117–1132. doi:10.1016/j.nano.2015.02.018
Huang, Y.-J., Hung, K.-C., Hung, H.-S., and Hsu, S.-h. (2018). Modulation of Macrophage Phenotype by Biodegradable Polyurethane Nanoparticles: Possible Relation between Macrophage Polarization and Immune Response of Nanoparticles. ACS Appl. Mat. Interfaces 10 (23), 19436–19448. doi:10.1021/acsami.8b04718
Hunter, M. M., Wang, A., Parhar, K. S., Johnston, M. J. G., Van Rooijen, N., Beck, P. L., et al. (2010). In Vitro-derived Alternatively Activated Macrophages Reduce Colonic Inflammation in Mice. Gastroenterology 138 (4), 1395–1405. doi:10.1053/j.gastro.2009.12.041
Hunter, P. (2012). The Inflammation Theory of Disease. EMBO Rep. 13 (11), 968–970. doi:10.1038/embor.2012.142
Hur, S. J., Kang, S. H., Jung, H. S., Kim, S. C., Jeon, H. S., Kim, I. H., et al. (2012). Review of Natural Products Actions on Cytokines in Inflammatory Bowel Disease. Nutr. Res. 32 (11), 801–816. doi:10.1016/j.nutres.2012.09.013
Jakubzick, C., Gautier, E. L., Gibbings, S. L., Sojka, D. K., Schlitzer, A., Johnson, T. E., et al. (2013). Minimal Differentiation of Classical Monocytes as They Survey Steady-State Tissues and Transport Antigen to Lymph Nodes. Immunity 39 (3), 599–610. doi:10.1016/j.immuni.2013.08.007
Jharap, B., Seinen, M. L., de Boer, N. K. H., van Ginkel, J. R., Linskens, R. K., Kneppelhout, J. C., et al. (2010). Thiopurine Therapy in Inflammatory Bowel Disease Patients: Analyses of Two 8-year Intercept Cohorts. Inflamm. Bowel Dis. 16 (9), 1541–1549. doi:10.1002/ibd.21221
Kaiser, G. C., Yan, F., and Polk, D. B. (1999). Mesalamine Blocks Tumor Necrosis Factor Growth Inhibition and Nuclear Factor κB Activation in Mouse Colonocytes. Gastroenterology 116 (3), 602–609. doi:10.1016/s0016-5085(99)70182-4
Kany, S., Vollrath, J. T., and Relja, B. (2019). Cytokines in Inflammatory Disease. Ijms 20 (23), 6008. doi:10.3390/ijms20236008
Kaplan, G. G. (2015). The Global Burden of IBD: from 2015 to 2025. Nat. Rev. Gastroenterol. Hepatol. 12 (12), 720–727. doi:10.1038/nrgastro.2015.150
Kim, H.-Y., Li, R., Ng, T. S. C., Courties, G., Rodell, C. B., Prytyskach, M., et al. (2018). Quantitative Imaging of Tumor-Associated Macrophages and Their Response to Therapy Using 64Cu-Labeled Macrin. ACS Nano 12 (12), 12015–12029. doi:10.1021/acsnano.8b04338
Kobayashi, T., Siegmund, B., Le Berre, C., Wei, S. C., Ferrante, M., Shen, B., et al. (2020). Ulcerative Colitis. Nat. Rev. Dis. Prim. 6 (1), 74. doi:10.1038/s41572-020-0205-x
Koch, S., and Nusrat, A. (2012). The Life and Death of Epithelia during Inflammation: Lessons Learned from the Gut. Annu. Rev. Pathol. Mech. Dis. 7, 35–60. doi:10.1146/annurev-pathol-011811-120905
Koelink, P. J., Bloemendaal, F. M., Li, B., Westera, L., Vogels, E. W. M., van Roest, M., et al. (2020). Anti-TNF Therapy in IBD Exerts its Therapeutic Effect through Macrophage IL-10 Signalling. Gut 69 (6), 1053–1063. doi:10.1136/gutjnl-2019-318264
Kredel, L. I., Batra, A., Stroh, T., Kühl, A. A., Zeitz, M., Erben, U., et al. (2013). Adipokines from Local Fat Cells Shape the Macrophage Compartment of the Creeping Fat in Crohn's Disease. Gut 62 (6), 852–862. doi:10.1136/gutjnl-2011-301424
Kremer, J. M., Cohen, S., Wilkinson, B. E., Connell, C. A., French, J. L., Gomez-Reino, J., et al. (2012). A Phase IIb Dose-Ranging Study of the Oral JAK Inhibitor Tofacitinib (CP-690,550) versus Placebo in Combination with Background Methotrexate in Patients with Active Rheumatoid Arthritis and an Inadequate Response to Methotrexate Alone. Arthritis & Rheumatism 64 (4), 970–981. doi:10.1002/art.33419
Kühl, A. A., Erben, U., Kredel, L. I., and Siegmund, B. (2015). Diversity of Intestinal Macrophages in Inflammatory Bowel Diseases. Front. Immunol. 6, 613. doi:10.3389/fimmu.2015.00613
Lavin, Y., Winter, D., Blecher-Gonen, R., David, E., Keren-Shaul, H., Merad, M., et al. (2014). Tissue-resident Macrophage Enhancer Landscapes Are Shaped by the Local Microenvironment. Cell 159 (6), 1312–1326. doi:10.1016/j.cell.2014.11.018
Lawrence, T., and Natoli, G. (2011). Transcriptional Regulation of Macrophage Polarization: Enabling Diversity with Identity. Nat. Rev. Immunol. 11 (11), 750–761. doi:10.1038/nri3088
Lazzerini, M., Martelossi, S., Magazzù, G., Pellegrino, S., Lucanto, M. C., Barabino, A., et al. (2013). Effect of Thalidomide on Clinical Remission in Children and Adolescents with Refractory Crohn Disease. Jama 310 (20), 2164–2173. doi:10.1001/jama.2013.280777
Lee, B.-C., Lee, J. Y., Kim, J., Yoo, J. M., Kang, I., Kim, J.-J., et al. (2020a). Graphene Quantum Dots as Anti-inflammatory Therapy for Colitis. Sci. Adv. 6 (18), eaaz2630. doi:10.1126/sciadv.aaz2630
Lee, Y., Sugihara, K., Gillilland, M. G., Jon, S., Kamada, N., and Moon, J. J. (2020b). Hyaluronic Acid-Bilirubin Nanomedicine for Targeted Modulation of Dysregulated Intestinal Barrier, Microbiome and Immune Responses in Colitis. Nat. Mat. 19 (1), 118–126. doi:10.1038/s41563-019-0462-9
Leonardi, I., Li, X., Semon, A., Li, D., Doron, I., Putzel, G., et al. (2018). CX3CR1 + Mononuclear Phagocytes Control Immunity to Intestinal Fungi. Science 359 (6372), 232–236. doi:10.1126/science.aao1503
Levin, A. D., Wildenberg, M. E., and van den Brink, G. R. (2016). Mechanism of Action of Anti-TNF Therapy in Inflammatory Bowel Disease. Eccojc 10 (8), 989–997. doi:10.1093/ecco-jcc/jjw053
Lissner, D., Schumann, M., Batra, A., Kredel, L.-I., Kühl, A. A., Erben, U., et al. (2015). Monocyte and M1 Macrophage-Induced Barrier Defect Contributes to Chronic Intestinal Inflammation in IBD. Inflamm. Bowel Dis. 21 (6), 1–1305. doi:10.1097/mib.0000000000000384
Liu, R., Tang, A., Wang, X., Chen, X., Zhao, L., Xiao, Z., et al. (2018). Inhibition of lncRNA NEAT1 Suppresses the Inflammatory Response in IBD by Modulating the Intestinal Epithelial Barrier and by Exosome-Mediated Polarization of Macrophages. Int. J. Mol. Med. 42 (5), 2903–2913. doi:10.3892/ijmm.2018.3829
Lloyd-Price, J., Arze, C., Arze, C., Ananthakrishnan, A. N., Schirmer, M., Avila-Pacheco, J., et al. (2019). Multi-omics of the Gut Microbial Ecosystem in Inflammatory Bowel Diseases. Nature 569 (7758), 655–662. doi:10.1038/s41586-019-1237-9
Locati, M., Curtale, G., and Mantovani, A. (2020). Diversity, Mechanisms, and Significance of Macrophage Plasticity. Annu. Rev. Pathol. Mech. Dis. 15, 123–147. doi:10.1146/annurev-pathmechdis-012418-012718
Locati, M., Mantovani, A., and Sica, A. (2013). Macrophage Activation and Polarization as an Adaptive Component of Innate Immunity. Adv. Immunol. 120, 163–184. doi:10.1016/b978-0-12-417028-5.00006-5
Louwe, P. A., Badiola Gomez, L., Webster, H., Perona-Wright, G., Bain, C. C., Forbes, S. J., et al. (2021). Recruited Macrophages that Colonize the Post-inflammatory Peritoneal Niche Convert into Functionally Divergent Resident Cells. Nat. Commun. 12 (1). doi:10.1038/s41467-021-21778-0
Lu, J., Liu, D., Tan, Y., Deng, F., and Li, R. (2021a). M1 Macrophage Exosomes MiR‐21a‐5p Aggravates Inflammatory Bowel Disease through Decreasing E‐cadherin and Subsequent ILC2 Activation. J. Cell Mol. Med. 25 (6), 3041–3050. doi:10.1111/jcmm.16348
Lu, J., Liu, D., Tan, Y., Li, R., Wang, X., and Deng, F. (2021b). Thalidomide Attenuates Colitis and Is Associated with the Suppression of M1 Macrophage Polarization by Targeting the Transcription Factor IRF5. Dig. Dis. Sci. 66 (11), 3803–3812. doi:10.1007/s10620-021-07067-2
Lu, J., Xie, L., Liu, C., Zhang, Q., and Sun, S. (2017). PTEN/PI3k/AKT Regulates Macrophage Polarization in Emphysematous Mice. Scand. J. Immunol. 85 (6), 395–405. doi:10.1111/sji.12545
Luan, B., Yoon, Y.-S., Le Lay, J., Kaestner, K. H., Hedrick, S., and Montminy, M. (2015). CREB Pathway Links PGE2 Signaling with Macrophage Polarization. Proc. Natl. Acad. Sci. U.S.A. 112 (51), 15642–15647. doi:10.1073/pnas.1519644112
Lucas, M., Zhang, X., Prasanna, V., and Mosser, D. M. (2005). ERK Activation Following Macrophage FcγR Ligation Leads to Chromatin Modifications at the IL-10 Locus. J. Immunol. 175 (1), 469–477. doi:10.4049/jimmunol.175.1.469
Ma, W.-T., Gao, F., Gu, K., and Chen, D.-K. (2019). The Role of Monocytes and Macrophages in Autoimmune Diseases: A Comprehensive Review. Front. Immunol. 10, 1140. doi:10.3389/fimmu.2019.01140
MacDonald, K. P. A., Palmer, J. S., Cronau, S., Seppanen, E., Olver, S., Raffelt, N. C., et al. (2010). An Antibody against the Colony-Stimulating Factor 1 Receptor Depletes the Resident Subset of Monocytes and Tissue- and Tumor-Associated Macrophages but Does Not Inhibit Inflammation. Blood 116 (19), 3955–3963. doi:10.1182/blood-2010-02-266296
Machado, M. A. d. Á., Moura, C. S. d., Guerra, S. F., Curtis, J. R., Abrahamowicz, M., and Bernatsky, S. (2018). Effectiveness and Safety of Tofacitinib in Rheumatoid Arthritis: a Cohort Study. Arthritis Res. Ther. 20 (1), 60. doi:10.1186/s13075-018-1539-6
Mahida, Y. R., Patel, S., Gionchetti, P., Vaux, D., and Jewell, D. P. (1989). Macrophage Subpopulations in Lamina Propria of Normal and Inflamed Colon and Terminal Ileum. Gut 30 (6), 826–834. doi:10.1136/gut.30.6.826
Marinković, G., Hamers, A. A. J., de Vries, C. J. M., and de Waard, V. (2014). 6-Mercaptopurine Reduces Macrophage Activation and Gut Epithelium Proliferation through Inhibition of GTPase Rac1. Inflamm. Bowel Dis. 20 (9), 1487–1495. doi:10.1097/mib.0000000000000122
Maronek, M., Link, R., Ambro, L., and Gardlik, R. (2020). Phages and Their Role in Gastrointestinal Disease: Focus on Inflammatory Bowel Disease. Cells 9 (4), 1013. doi:10.3390/cells9041013
Martinez, F. O., Helming, L., and Gordon, S. (2009). Alternative Activation of Macrophages: an Immunologic Functional Perspective. Annu. Rev. Immunol. 27, 451–483. doi:10.1146/annurev.immunol.021908.132532
Mass, E., and Gentek, R. (2021). Fetal-Derived Immune Cells at the Roots of Lifelong Pathophysiology. Front. Cell Dev. Biol. 9. doi:10.3389/fcell.2021.648313
Mazur, M., Karczewski, J., Lodyga, M., Żaba, R., and Adamski, Z. (2015). Inhibitors of Phosphodiesterase 4 (PDE 4): A New Therapeutic Option in the Treatment of Psoriasis Vulgaris and Psoriatic Arthritis. J. Dermatological Treat. 26 (4), 326–328. doi:10.3109/09546634.2014.991267
Mosser, D. M., and Edwards, J. P. (2008). Exploring the Full Spectrum of Macrophage Activation. Nat. Rev. Immunol. 8 (12), 958–969. doi:10.1038/nri2448
Mowat, A. M. (2018). To Respond or Not to Respond - a Personal Perspective of Intestinal Tolerance. Nat. Rev. Immunol. 18 (6), 405–415. doi:10.1038/s41577-018-0002-x
Mowat, C., Cole, A., Windsor, A., Ahmad, T., Arnott, I., Driscoll, R., et al. (2011). Guidelines for the Management of Inflammatory Bowel Disease in Adults. Gut 60 (5), 571–607. doi:10.1136/gut.2010.224154
Muller, P. A., Koscsó, B., Rajani, G. M., Stevanovic, K., Berres, M.-L., Hashimoto, D., et al. (2014). Crosstalk between Muscularis Macrophages and Enteric Neurons Regulates Gastrointestinal Motility. Cell 158 (2), 300–313. doi:10.1016/j.cell.2014.04.050
Municio, C., Soler Palacios, B., Estrada-Capetillo, L., Benguria, A., Dopazo, A., García-Lorenzo, E., et al. (2016). Methotrexate Selectively Targets Human Proinflammatory Macrophages through a Thymidylate Synthase/p53 axis. Ann. Rheum. Dis. 75 (12), 2157–2165. doi:10.1136/annrheumdis-2015-208736
Murray, P. J., and Wynn, T. A. (2011). Protective and Pathogenic Functions of Macrophage Subsets. Nat. Rev. Immunol. 11 (11), 723–737. doi:10.1038/nri3073
Na, Y. R., Stakenborg, M., Seok, S. H., and Matteoli, G. (2019). Macrophages in Intestinal Inflammation and Resolution: a Potential Therapeutic Target in IBD. Nat. Rev. Gastroenterol. Hepatol. 16 (9), 531–543. doi:10.1038/s41575-019-0172-4
Nadeem, M. S., Kumar, V., Al-Abbasi, F. A., Kamal, M. A., and Anwar, F. (2020). Risk of Colorectal Cancer in Inflammatory Bowel Diseases. Seminars Cancer Biol. 64, 51–60. doi:10.1016/j.semcancer.2019.05.001
Nakkala, J. R., Li, Z., Ahmad, W., Wang, K., and Gao, C. (2021). Immunomodulatory Biomaterials and Their Application in Therapies for Chronic Inflammation-Related Diseases. Acta Biomater. 123, 1–30. doi:10.1016/j.actbio.2021.01.025
Nunes, R., Neves, J. d., and Sarmento, B. (2019). Nanoparticles for the Regulation of Intestinal Inflammation: Opportunities and Challenges. Nanomedicine 14 (19), 2631–2644. doi:10.2217/nnm-2019-0191
Oakley, R. H., and Cidlowski, J. A. (2013). The Biology of the Glucocorticoid Receptor: New Signaling Mechanisms in Health and Disease. J. Allergy Clin. Immunol. 132 (5), 1033–1044. doi:10.1016/j.jaci.2013.09.007
Odegaard, J. I., Ricardo-Gonzalez, R. R., Goforth, M. H., Morel, C. R., Subramanian, V., Mukundan, L., et al. (2007). Macrophage-specific PPARγ Controls Alternative Activation and Improves Insulin Resistance. Nature 447 (7148), 1116–1120. doi:10.1038/nature05894
Oeckinghaus, A., Hayden, M. S., and Ghosh, S. (2011). Crosstalk in NF-Κb Signaling Pathways. Nat. Immunol. 12 (8), 695–708. doi:10.1038/ni.2065
O’Garra, A., Vieira, P. L., Vieira, P., and Goldfeld, A. E. (2004). IL-10-producing and Naturally Occurring CD4+ Tregs: Limiting Collateral Damage. J. Clin. Invest. 114 (10), 1372–1378. doi:10.1172/jci23215
Ohmori, Y., and Hamilton, T. A. (1997). IL-4-induced STAT6 Suppresses IFN-Gamma-Stimulated STAT1-dependent Transcription in Mouse Macrophages. J. Immunol. 159 (11), 5474–5482.
Olesen, C. M., Coskun, M., Peyrin-Biroulet, L., and Nielsen, O. H. (2016). Mechanisms behind Efficacy of Tumor Necrosis Factor Inhibitors in Inflammatory Bowel Diseases. Pharmacol. Ther. 159, 110–119. doi:10.1016/j.pharmthera.2016.01.001
Palmela, C., Chevarin, C., Xu, Z., Torres, J., Sevrin, G., Hirten, R., et al. (2018). Adherent-invasive Escherichia coli in Inflammatory Bowel Disease. Gut 67 (3), 574–587. doi:10.1136/gutjnl-2017-314903
Pander, J., Heusinkveld, M., van der Straaten, T., Jordanova, E. S., Baak-Pablo, R., Gelderblom, H., et al. (2011). Activation of Tumor-Promoting Type 2 Macrophages by EGFR-Targeting Antibody Cetuximab. Clin. Cancer Res. 17 (17), 5668–5673. doi:10.1158/1078-0432.Ccr-11-0239
Panés, J., Sandborn, W. J., Schreiber, S., Sands, B. E., Vermeire, S., D'Haens, G., et al. (2017). Tofacitinib for Induction and Maintenance Therapy of Crohn's Disease: Results of Two Phase IIb Randomised Placebo-Controlled Trials. Gut 66 (6), 1049–1059. doi:10.1136/gutjnl-2016-312735
Papamichael, K., Gils, A., Rutgeerts, P., Levesque, B. G., Vermeire, S., Sandborn, W. J., et al. (2015). Role for Therapeutic Drug Monitoring during Induction Therapy with TNF Antagonists in IBD. Inflamm. Bowel Dis. 21 (1), 182–197. doi:10.1097/mib.0000000000000202
Parameswaran, N., and Patial, S. (2010). Tumor Necrosis Factor-α Signaling in Macrophages. Crit. Rev. Eukar Gene Expr. 20 (2), 87–103. doi:10.1615/critreveukargeneexpr.v20.i2.10
Paramsothy, S., Rosenstein, A. K., Mehandru, S., and Colombel, J.-F. (2018). The Current State of the Art for Biological Therapies and New Small Molecules in Inflammatory Bowel Disease. Mucosal Immunol. 11 (6), 1558–1570. doi:10.1038/s41385-018-0050-3
Pattison, M. J., Mackenzie, K. F., and Arthur, J. S. C. (2012). Inhibition of JAKs in Macrophages Increases Lipopolysaccharide-Induced Cytokine Production by Blocking IL-10-mediated Feedback. J. I. 189 (6), 2784–2792. doi:10.4049/jimmunol.1200310
Piaggio, F., Kondylis, V., Pastorino, F., Di Paolo, D., Perri, P., Cossu, I., et al. (2016). A Novel Liposomal Clodronate Depletes Tumor-Associated Macrophages in Primary and Metastatic Melanoma: Anti-angiogenic and Anti-tumor Effects. J. Control. Release 223, 165–177. doi:10.1016/j.jconrel.2015.12.037
Png, C. W., Lindén, S. K., Gilshenan, K. S., Zoetendal, E. G., McSweeney, C. S., Sly, L. I., et al. (2010). Mucolytic Bacteria with Increased Prevalence in IBD Mucosa Augment In Vitro Utilization of Mucin by Other Bacteria. Am. J. Gastroenterol. 105 (11), 2420–2428. doi:10.1038/ajg.2010.281
Pothoven, K. L., and Schleimer, R. P. (2017). The Barrier Hypothesis and Oncostatin M: Restoration of Epithelial Barrier Function as a Novel Therapeutic Strategy for the Treatment of Type 2 Inflammatory Disease. Tissue Barriers 5 (3), e1341367. doi:10.1080/21688370.2017.1341367
Rahman, F. Z., Smith, A. M., Hayee, B. H., Marks, D. J. B., Bloom, S. L., and Segal, A. W. (2010). Delayed Resolution of Acute Inflammation in Ulcerative Colitis Is Associated with Elevated Cytokine Release Downstream of TLR4. PLoS One 5 (3), e9891. doi:10.1371/journal.pone.0009891
Rescigno, M., Urbano, M., Valzasina, B., Francolini, M., Rotta, G., Bonasio, R., et al. (2001). Dendritic Cells Express Tight Junction Proteins and Penetrate Gut Epithelial Monolayers to Sample Bacteria. Nat. Immunol. 2 (4), 361–367. doi:10.1038/86373
Roda, G., Chien Ng, S., Kotze, P. G., Argollo, M., Panaccione, R., Spinelli, A., et al. (2020). Crohn's Disease. Nat. Rev. Dis. Prim. 6 (1), 22. doi:10.1038/s41572-020-0156-2
Roda, G., Jharap, B., Neeraj, N., and Colombel, J.-F. (2016). Loss of Response to Anti-TNFs: Definition, Epidemiology, and Management. Clin. Transl. Gastroenterol. 7 (1), e135. doi:10.1038/ctg.2015.63
Rogler, G. (2020). Efficacy of JAK Inhibitors in Crohn's Disease. J. Crohns Colitis 14 (Suppl. ment_2), S746–s754. doi:10.1093/ecco-jcc/jjz186
Rose, T. M., and Bruce, A. G. (1991). Oncostatin M Is a Member of a Cytokine Family that Includes Leukemia-Inhibitory Factor, Granulocyte Colony-Stimulating Factor, and Interleukin 6. Proc. Natl. Acad. Sci. U.S.A. 88 (19), 8641–8645. doi:10.1073/pnas.88.19.8641
Rőszer, T. (2015). Understanding the Mysterious M2 Macrophage through Activation Markers and Effector Mechanisms. Mediat. Inflamm. 2015, 1–16. doi:10.1155/2015/816460
Ruffell, D., Mourkioti, F., Gambardella, A., Kirstetter, P., Lopez, R. G., Rosenthal, N., et al. (2009). A CREB-C/EBPβ Cascade Induces M2 Macrophage-specific Gene Expression and Promotes Muscle Injury Repair. Proc. Natl. Acad. Sci. U.S.A. 106 (41), 17475–17480. doi:10.1073/pnas.0908641106
Rutgeerts, P., D'Haens, G., Targan, S., Vasiliauskas, E., Hanauer, S. B., Present, D. H., et al. (1999). Efficacy and Safety of Retreatment with Anti-tumor Necrosis Factor Antibody (Infliximab) to Maintain Remission in Crohn's Disease. Gastroenterology 117 (4), 761–769. doi:10.1016/s0016-5085(99)70332-x
Sabate, J. M., Villarejo, J., Lemann, M., Bonnet, J., Allez, M., and Modigliani, R. (2002). An Open-Label Study of Thalidomide for Maintenance Therapy in Responders to Infliximab in Chronically Active and Fistulizing Refractory Crohn's Disease. Aliment. Pharmacol. Ther. 16 (6), 1117–1124. doi:10.1046/j.1365-2036.2002.01273.x
Sabino, J., Verstockt, B., Vermeire, S., and Ferrante, M. (2019). New Biologics and Small Molecules in Inflammatory Bowel Disease: an Update. Ther. Adv. Gastroenterol. 12, 175628481985320. doi:10.1177/1756284819853208
Salas, A., Hernandez-Rocha, C., Duijvestein, M., Faubion, W., McGovern, D., Vermeire, S., et al. (2020). JAK-STAT Pathway Targeting for the Treatment of Inflammatory Bowel Disease. Nat. Rev. Gastroenterol. Hepatol. 17 (6), 323–337. doi:10.1038/s41575-020-0273-0
Sales-Campos, H., Basso, P. J., Alves, V. B. F., Fonseca, M. T. C., Bonfá, G., Nardini, V., et al. (2015). Classical and Recent Advances in the Treatment of Inflammatory Bowel Diseases. Braz J. Med. Biol. Res. 48 (2), 96–107. doi:10.1590/1414-431x20143774
Sandborn, W. J., Ghosh, S., Panes, J., Vranic, I., Wang, W., and Niezychowski, W. (2014). A Phase 2 Study of Tofacitinib, an Oral Janus Kinase Inhibitor, in Patients with Crohn's Disease. Clin. Gastroenterol. Hepatol. 12 (9), 1485–e1482. doi:10.1016/j.cgh.2014.01.029
Sandborn, W. J., Feagan, B. G., Danese, S., O’Brien, C. D., Ott, E., Marano, C., et al. (2021). Safety of Ustekinumab in Inflammatory Bowel Disease: Pooled Safety Analysis of Results from Phase 2/3 Studies. Inflamm. Bowel Dis. 27 (7), 994–1007. doi:10.1093/ibd/izaa236
Sandborn, W. J., Su, C., and Panes, J. (2017). Tofacitinib as Induction and Maintenance Therapy for Ulcerative Colitis. N. Engl. J. Med. 377 (5), 496–497. doi:10.1056/NEJMc1707500
Satoh, T., Takeuchi, O., Vandenbon, A., Yasuda, K., Tanaka, Y., Kumagai, Y., et al. (2010). The Jmjd3-Irf4 axis Regulates M2 Macrophage Polarization and Host Responses against Helminth Infection. Nat. Immunol. 11 (10), 936–944. doi:10.1038/ni.1920
Schenk, M., Bouchon, A., Birrer, S., Colonna, M., and Mueller, C. (2005). Macrophages Expressing Triggering Receptor Expressed on Myeloid Cells-1 Are Underrepresented in the Human Intestine. J. Immunol. 174 (1), 517–524. doi:10.4049/jimmunol.174.1.517
Schett, G., and Neurath, M. F. (2018). Resolution of Chronic Inflammatory Disease: Universal and Tissue-specific Concepts. Nat. Commun. 9 (1), 3261. doi:10.1038/s41467-018-05800-6
Schridde, A., Bain, C. C., Mayer, J. U., Montgomery, J., Pollet, E., Denecke, B., et al. (2017). Tissue-specific Differentiation of Colonic Macrophages Requires TGFβ Receptor-Mediated Signaling. Mucosal Immunol. 10 (6), 1387–1399. doi:10.1038/mi.2016.142
Schulz, C., Perdiguero, E. G., Chorro, L., Szabo-Rogers, H., Cagnard, N., Kierdorf, K., et al. (2012). A Lineage of Myeloid Cells Independent of Myb and Hematopoietic Stem Cells. Science 336 (6077), 86–90. doi:10.1126/science.1219179
Schwartz, D. M., Kanno, Y., Villarino, A., Ward, M., Gadina, M., and O'Shea, J. J. (2018). Erratum: JAK Inhibition as a Therapeutic Strategy for Immune and Inflammatory Diseases. Nat. Rev. Drug Discov. 17 (1), 78. doi:10.1038/nrd.2017.267
Sehgal, A., Donaldson, D. S., Pridans, C., Sauter, K. A., Hume, D. A., and Mabbott, N. A. (2018). The Role of CSF1R-dependent Macrophages in Control of the Intestinal Stem-Cell Niche. Nat. Commun. 9 (1), 1272. doi:10.1038/s41467-018-03638-6
Seyed Tabib, N. S., Madgwick, M., Sudhakar, P., Verstockt, B., Korcsmaros, T., and Vermeire, S. (2020). Big Data in IBD: Big Progress for Clinical Practice. Gut 69 (8), 1520–1532. doi:10.1136/gutjnl-2019-320065
Shaw, T. N., Houston, S. A., Wemyss, K., Bridgeman, H. M., Barbera, T. A., Zangerle-Murray, T., et al. (2018). Tissue-resident Macrophages in the Intestine Are Long Lived and Defined by Tim-4 and CD4 Expression. J. Exp. Med. 215 (6), 1507–1518. doi:10.1084/jem.20180019
Shiratori, H., Feinweber, C., Luckhardt, S., Wallner, N., Geisslinger, G., Weigert, A., et al. (2018). An In Vitro Test System for Compounds that Modulate Human Inflammatory Macrophage Polarization. Eur. J. Pharmacol. 833, 328–338. doi:10.1016/j.ejphar.2018.06.017
Shivaji, U. N., Sharratt, C. L., Thomas, T., Smith, S. C. L., Iacucci, M., Moran, G. W., et al. (2019). Review Article: Managing the Adverse Events Caused by Anti-TNF Therapy in Inflammatory Bowel Disease. Aliment. Pharmacol. Ther. 49 (6), 664–680. doi:10.1111/apt.15097
Siebel, C., and Lendahl, U. (2017). Notch Signaling in Development, Tissue Homeostasis, and Disease. Physiol. Rev. 97 (4), 1235–1294. doi:10.1152/physrev.00005.2017
Simon, J. M., Davis, J. P., Lee, S. E., Schaner, M. R., Gipson, G. R., Weiser, M., et al. (2016). Alterations to Chromatin in Intestinal Macrophages Link IL-10 Deficiency to Inappropriate Inflammatory Responses. Eur. J. Immunol. 46 (8), 1912–1925. doi:10.1002/eji.201546237
Singh, Y., Pawar, V. K., Meher, J. G., Raval, K., Kumar, A., Shrivastava, R., et al. (2017). Targeting Tumor Associated Macrophages (TAMs) via Nanocarriers. J. Control. Release 254, 92–106. doi:10.1016/j.jconrel.2017.03.395
Smith, A. M., Rahman, F. Z., Hayee, B. H., Graham, S. J., Marks, D. J. B., Sewell, G. W., et al. (2009). Disordered Macrophage Cytokine Secretion Underlies Impaired Acute Inflammation and Bacterial Clearance in Crohn's Disease. J. Exp. Med. 206 (9), 1883–1897. doi:10.1084/jem.20091233
Smythies, L. E., Sellers, M., Clements, R. H., Mosteller-Barnum, M., Meng, G., Benjamin, W. H., et al. (2005). Human Intestinal Macrophages Display Profound Inflammatory Anergy Despite Avid Phagocytic and Bacteriocidal Activity. J. Clin. Invest. 115 (1), 66–75. doi:10.1172/jci200519229
Sohail, M., MudassirMinhas, M. U., Minhas, M. U., Khan, S., Hussain, Z., de Matas, M., et al. (2019). Natural and Synthetic Polymer-Based Smart Biomaterials for Management of Ulcerative Colitis: a Review of Recent Developments and Future Prospects. Drug Deliv. Transl. Res. 9 (2), 595–614. doi:10.1007/s13346-018-0512-x
Spadaccini, M., D’Alessio, S., Peyrin-Biroulet, L., and Danese, S. (2017). PDE4 Inhibition and Inflammatory Bowel Disease: A Novel Therapeutic Avenue. Ijms 18 (6), 1276. doi:10.3390/ijms18061276
Stack, W., Mann, S., Roy, A., Heath, P., Sopwith, M., Freeman, J., et al. (1997). Randomised Controlled Trial of CDP571 Antibody to Tumour Necrosis Factor-α in Crohn's Disease. Lancet 349 (9051), 521–524. doi:10.1016/s0140-6736(97)80083-9
Steinbach, E. C., and Plevy, S. E. (2014). The Role of Macrophages and Dendritic Cells in the Initiation of Inflammation in IBD. Inflamm. Bowel Dis. 20 (1), 166–175. doi:10.1097/MIB.0b013e3182a69dca
Taratummarat, S., Sangphech, N., Vu, C. T. B., Palaga, T., Ondee, T., Surawut, S., et al. (2018). Gold Nanoparticles Attenuates Bacterial Sepsis in Cecal Ligation and Puncture Mouse Model through the Induction of M2 Macrophage Polarization. BMC Microbiol. 18 (1), 85. doi:10.1186/s12866-018-1227-3
Targan, S. R., Hanauer, S. B., van Deventer, S. J. H., Mayer, L., Present, D. H., Braakman, T., et al. (1997). A Short-Term Study of Chimeric Monoclonal Antibody cA2 to Tumor Necrosis Factor α for Crohn's Disease. N. Engl. J. Med. 337 (15), 1029–1036. doi:10.1056/nejm199710093371502
Torres, J., Mehandru, S., Colombel, J.-F., and Peyrin-Biroulet, L. (2017). Crohn's Disease. Lancet 389 (10080), 1741–1755. doi:10.1016/s0140-6736(16)31711-1
Tripathi, K., and Feuerstein, J. D. (2019). New Developments in Ulcerative Colitis: Latest Evidence on Management, Treatment, and Maintenance. Dic 8, 1–11. doi:10.7573/dic.212572
Underwood, A. P., Kaakoush, N. O., Sodhi, N., Merif, J., Seah Lee, W., Riordan, S. M., et al. (2016). Campylobacter Concisus Pathotypes Are Present at Significant Levels in Patients with Gastroenteritis. J. Med. Microbiol. 65 (3), 219–226. doi:10.1099/jmm.0.000216
Ungaro, R., Mehandru, S., Allen, P. B., Peyrin-Biroulet, L., and Colombel, J.-F. (2017). Ulcerative Colitis. Lancet 389 (10080), 1756–1770. doi:10.1016/s0140-6736(16)32126-2
Vallon-Eberhard, A., Landsman, L., Yogev, N., Verrier, B., and Jung, S. (2006). Transepithelial Pathogen Uptake into the Small Intestinal Lamina Propria. J. Immunol. 176 (4), 2465–2469. doi:10.4049/jimmunol.176.4.2465
van Furth, R., Cohn, Z. A., Hirsch, J. G., Humphrey, J. H., Spector, W. G., and Langevoort, H. L. (1972). The Mononuclear Phagocyte System: a New Classification of Macrophages, Monocytes, and Their Precursor Cells. Bull. World Health Organ 46 (6), 845–852.
Vergadi, E., Ieronymaki, E., Lyroni, K., Vaporidi, K., and Tsatsanis, C. (2017). Akt Signaling Pathway in Macrophage Activation and M1/M2 Polarization. J. I. 198 (3), 1006–1014. doi:10.4049/jimmunol.1601515
Vermeire, S., Schreiber, S., Petryka, R., Kuehbacher, T., Hebuterne, X., Roblin, X., et al. (2017). Clinical Remission in Patients with Moderate-To-Severe Crohn's Disease Treated with Filgotinib (The FITZROY Study): Results from a Phase 2, Double-Blind, Randomised, Placebo-Controlled Trial. Lancet 389 (10066), 266–275. doi:10.1016/s0140-6736(16)32537-5
Villarino, A. V., Kanno, Y., and O'Shea, J. J. (2017). Mechanisms and Consequences of Jak-STAT Signaling in the Immune System. Nat. Immunol. 18 (4), 374–384. doi:10.1038/ni.3691
Viola, M. F., and Boeckxstaens, G. (2021). Niche-specific Functional Heterogeneity of Intestinal Resident Macrophages. Gut 70 (7), 1383–1395. doi:10.1136/gutjnl-2020-323121
Vos, A. C. W., Wildenberg, M. E., Arijs, I., Duijvestein, M., Verhaar, A. P., de Hertogh, G., et al. (2012). Regulatory Macrophages Induced by Infliximab Are Involved in Healing In Vivo and In Vitro. Inflamm. Bowel Dis. 18 (3), 401–408. doi:10.1002/ibd.21818
Vos, A. C. W., Wildenberg, M. E., Duijvestein, M., Verhaar, A. P., van den Brink, G. R., and Hommes, D. W. (2011). Anti-Tumor Necrosis Factor-α Antibodies Induce Regulatory Macrophages in an Fc Region-dependent Manner. Gastroenterology 140 (1), 221–230. doi:10.1053/j.gastro.2010.10.008
Wallace, K. L., Zheng, L. B., Kanazawa, Y., and Shih, D. Q. (2014). Immunopathology of Inflammatory Bowel Disease. Wjg 20 (1), 6–21. doi:10.3748/wjg.v20.i1.6
Wang, L., Tang, L., Feng, Y., Zhao, S., Han, M., Zhang, C., et al. (2020). A Purified Membrane Protein from Akkermansia Muciniphila or the Pasteurised Bacterium Blunts Colitis Associated Tumourigenesis by Modulation of CD8+ T Cells in Mice. Gut 69 (11), 1988–1997. doi:10.1136/gutjnl-2019-320105
Wang, L. x., Zhang, S. x., Wu, H. j., Rong, X. l., and Guo, J. (2019). M2b Macrophage Polarization and its Roles in Diseases. J. Leukoc. Biol. 106 (2), 345–358. doi:10.1002/jlb.3ru1018-378rr
Weissleder, R., Nahrendorf, M., and Pittet, M. J. (2014). Imaging Macrophages with Nanoparticles. Nat. Mater 13 (2), 125–138. doi:10.1038/nmat3780
Wells, J. M., Rossi, O., Meijerink, M., and van Baarlen, P. (2011). Epithelial Crosstalk at the Microbiota-Mucosal Interface. Proc. Natl. Acad. Sci. U.S.A. 108 (Suppl. 1Suppl 1), 4607–4614. doi:10.1073/pnas.1000092107
West, N. R., Hegazy, A. N., Hegazy, A. N., Owens, B. M. J., Bullers, S. J., Linggi, B., et al. (2017). Oncostatin M Drives Intestinal Inflammation and Predicts Response to Tumor Necrosis Factor-Neutralizing Therapy in Patients with Inflammatory Bowel Disease. Nat. Med. 23 (5), 579–589. doi:10.1038/nm.4307
Windsor, J. W., and Kaplan, G. G. (2019). Evolving Epidemiology of IBD. Curr. Gastroenterol. Rep. 21 (8), 40. doi:10.1007/s11894-019-0705-6
Wu, Y., and Hirschi, K. K. (2020). Tissue-Resident Macrophage Development and Function. Front. Cell Dev. Biol. 8, 617879. doi:10.3389/fcell.2020.617879
Wynn, T. A., and Vannella, K. M. (2016). Macrophages in Tissue Repair, Regeneration, and Fibrosis. Immunity 44 (3), 450–462. doi:10.1016/j.immuni.2016.02.015
Xiao, Q., Li, X., Li, Y., Wu, Z., Xu, C., Chen, Z., et al. (2021). Biological Drug and Drug Delivery-Mediated Immunotherapy. Acta Pharm. Sin. B 11 (4), 941–960. doi:10.1016/j.apsb.2020.12.018
Xu, H., Zhu, J., Smith, S., Foldi, J., Zhao, B., Chung, A. Y., et al. (2012). Notch-RBP-J Signaling Regulates the Transcription Factor IRF8 to Promote Inflammatory Macrophage Polarization. Nat. Immunol. 13 (7), 642–650. doi:10.1038/ni.2304
Yakymenko, O., Schoultz, I., Gullberg, E., Ström, M., Almer, S., Wallon, C., et al. (2018). Infliximab Restores Colonic Barrier to Adherent-Invasive E. coli in Crohn's Disease via Effects on Epithelial Lipid Rafts. Scand. J. Gastroenterology 53 (6), 677–684. doi:10.1080/00365521.2018.1458146
Yamagata, M., Mikami, T., Tsuruta, T., Yokoyama, K., Sada, M., Kobayashi, K., et al. (2011). Submucosal Fibrosis and Basic-Fibroblast Growth Factor-Positive Neutrophils Correlate with Colonic Stenosis in Cases of Ulcerative Colitis. Digestion 84 (1), 12–21. doi:10.1159/000320773
Yip, J. L. K., Balasuriya, G. K., Spencer, S. J., and Hill-Yardin, E. L. (2021). The Role of Intestinal Macrophages in Gastrointestinal Homeostasis: Heterogeneity and Implications in Disease. Cell. Mol. Gastroenterology Hepatology 12, 1701–1718. doi:10.1016/j.jcmgh.2021.08.021
Yona, S., Kim, K.-W., Wolf, Y., Mildner, A., Varol, D., Breker, M., et al. (2013). Fate Mapping Reveals Origins and Dynamics of Monocytes and Tissue Macrophages under Homeostasis. Immunity 38 (1), 79–91. doi:10.1016/j.immuni.2012.12.001
You, Y., Zhou, C., Li, D., Cao, Z.-L., Shen, W., Li, W.-Z., et al. (2016). Sorting Nexin 10 Acting as a Novel Regulator of Macrophage Polarization Mediates Inflammatory Response in Experimental Mouse Colitis. Sci. Rep. 6, 20630. doi:10.1038/srep20630
Zhang, H., Wu, X., Wang, G., Liu, P., Qin, S., Xu, K., et al. (2018). Macrophage Polarization, Inflammatory Signaling, and NF-Κb Activation in Response to Chemically Modified Titanium Surfaces. Colloids Surfaces B Biointerfaces 166, 269–276. doi:10.1016/j.colsurfb.2018.03.029
Zhang, L., Lee, H., Grimm, M. C., Riordan, S. M., Day, A. S., and Lemberg, D. A. (2014). Campylobacter Concisusand Inflammatory Bowel Disease. Wjg 20 (5), 1259–1267. doi:10.3748/wjg.v20.i5.1259
Zhu, C., Zhang, S., Song, C., Zhang, Y., Ling, Q., Hoffmann, P. R., et al. (2017). Selenium Nanoparticles Decorated with Ulva Lactuca Polysaccharide Potentially Attenuate Colitis by Inhibiting NF-Κb Mediated Hyper Inflammation. J. Nanobiotechnol 15 (1), 20. doi:10.1186/s12951-017-0252-y
Zhu, W., Yu, J., Nie, Y., Shi, X., Liu, Y., Li, F., et al. (2014). Disequilibrium of M1 and M2 Macrophages Correlates with the Development of Experimental Inflammatory Bowel Diseases. Immunol. Investig. 43 (7), 638–652. doi:10.3109/08820139.2014.909456
Keywords: inflammatory bowel disease, ulcerative colitis, Crohn’s disease, tissue-resident macrophage, macrophage polarization
Citation: Ma S, Zhang J, Liu H, Li S and Wang Q (2022) The Role of Tissue-Resident Macrophages in the Development and Treatment of Inflammatory Bowel Disease. Front. Cell Dev. Biol. 10:896591. doi: 10.3389/fcell.2022.896591
Received: 21 March 2022; Accepted: 09 May 2022;
Published: 26 May 2022.
Edited by:
Yuyong Tan, Central South University, ChinaCopyright © 2022 Ma, Zhang, Liu, Li and Wang. This is an open-access article distributed under the terms of the Creative Commons Attribution License (CC BY). The use, distribution or reproduction in other forums is permitted, provided the original author(s) and the copyright owner(s) are credited and that the original publication in this journal is cited, in accordance with accepted academic practice. No use, distribution or reproduction is permitted which does not comply with these terms.
*Correspondence: Shuang Li, c2h1YW5nbGlAamx1LmVkdS5jbg==; Quan Wang, d3F1YW5Aamx1LmVkdS5jbg==