- 1College of Medicine, Mohammed Bin Rashid University of Medicine and Health Sciences, Dubai, United Arab Emirates
- 2Vascular Biology Research Centre, Molecular and Clinical Research Institute, University of London, London, United Kingdom
- 3Department of Biomedical Sciences, University of Sassari, Sassari, Italy
- 4Rheumatology Unit, Department of Clinical and Experimental Medicine, University Hospital (AOUSS) and University of Sassari, Sassari, Italy
- 5Department of Basic Medical Sciences, College of Medicine, Q.U. Health. Qatar University, Doha, Qatar
- 6Discipline of Clinical Pharmacology, College of Medicine and Public Health, Flinders University, Adelaide, SA, Australia
- 7Department of Clinical Pharmacology, Flinders Medical Centre, Adelaide, SA, Australia
- 8Department of Medical Laboratory Sciences, College of Health Sciences and Sharjah Institute for Medical Research, University of Sharjah, Sharjah, United Arab Emirates
There have been significant advances, particularly over the last 20 years, in the identification of non-coding RNAs (ncRNAs) and their pathophysiological role in a wide range of disease states, particularly cancer and other chronic conditions characterized by excess inflammation and oxidative stress such as atherosclerosis, diabetes, obesity, multiple sclerosis, osteoporosis, liver and lung fibrosis. Such discoveries have potential therapeutic implications as a better understanding of the molecular mechanisms underpinning the effects of ncRNAs on critical homeostatic control mechanisms and biochemical pathways might lead to the identification of novel druggable targets. In this context, increasing evidence suggests that several natural compounds can target ncRNAs at different levels and, consequently, influence processes involved in the onset and progression of disease states. The natural phenol resveratrol has been extensively studied for therapeutic purposes in view of its established anti-inflammatory and antioxidant effects, particularly in disease states such as cancer and cardiovascular disease that are associated with human aging. However, increasing in vitro and in vivo evidence also suggests that resveratrol can directly target various ncRNAs and that this mediates, at least in part, its potential therapeutic effects. This review critically appraises the available evidence regarding the resveratrol-mediated modulation of different ncRNAs in a wide range of disease states characterized by a pro-inflammatory state and oxidative stress, the potential therapeutic applications, and future research directions.
Introduction
Over the last two decades of the post-genomic era, it has become evident that only about 1.5% of the human genome corresponds to protein-coding sequences whereas the remaining 98.5% is non-coding (Lander et al., 2001). Large consortia, such as the Encyclopedia of DNA Elements (ENCODE) and its official Gencode gene catalog (www.gencodegenes.org) (Derrien et al., 2012), have been instrumental in the expansion of the human gene catalog with the addition of thousands of validated and annotated non-coding genes. These initiatives have also facilitated the generation of evidence supporting the participation of non-coding genes, once merely regarded as “junk”, in the regulation of the activity of protein-coding genes (Palazzo and Lee, 2015). Specifically, the discovery of several types of non-coding RNAs (ncRNAs) and their involvement in a wide range of homeostatic processes has revolutionized our understanding of the pathogenesis of disease states (Costa, 2005). NcRNAs are a heterogenous group of RNA molecules with different biological function, biogenesis, structure, and length. Based on the arbitrary length of 200 nucleotides (nt), ncRNAs are divided into two classes: small (sncRNAs) (<200 nt) and long (>200 nt) (lncRNAs) (Zhang P. et al., 2019). lncRNAs are widely expressed in various tissues and cells lines and their spatial and temporal expression profiles as well as their specific interactions with DNA, RNA and proteins, reflect distinct functions (Statello et al., 2021). Indeed, lncRNAs participate in regulating cell cycle and cell differentiation, physiological processes such as development, aging, and immunity, and pathological processes such as cancer, cardiovascular disease and diabetes (Leung and Natarajan, 2018; Fu P.-F. et al., 2019; Dehaini et al., 2019; Wehbe et al., 2019; Zhang C. et al., 2020; Giordo et al., 2021a; Statello et al., 2021). lncRNAs regulate gene expression at both transcriptional and post-transcriptional level. At the transcriptional level, they can affect target genes either in cis, regulating the expression of neighboring genes, or in trans, away from their loci, interacting with chromatin at several thousand different locations across multiple chromosomes (Zhang X. et al., 2019). Another category of ncRNAs (a subtype of the sncRNAs class), the microRNAs (miRNAs), have also gained significant popularity over the last two decades and revolutionized our knowledge of the mechanisms regulating health and disease states. miRNAs have a length of approximately 22 nt and typically bind to the 3′ untranslated region (3′UTR) of mRNAs, inducing mRNA degradation and translational repression, although miRNAs inducing mRNA translation have been also reported (Dragomir et al., 2018). miRNAs can target multiple mRNAs and, at the same time, mRNAs are targeted by multiple miRNAs (Peter, 2010; O'Brien et al., 2018). The complexity of these regulatory networks is further compounded by the involvement of lncRNAs as competing endogenous RNAs (ceRNAs), or miRNAs sponges, with the consequent reduction or inhibition of the regulatory effect of miRNAs on their mRNA target(s) (Tay et al., 2014; López-Urrutia et al., 2019). A wide range of biological processes is regulated through the crosstalk lncRNAs-miRNAs and the aberrant expression of miRNAs is associated with various pathological states. In addition, miRNAs can be packed into extracellular vesicles such as exosomes, serving as messengers that mediate cell-cell communication or function through intercellular signaling pathways. Emerging evidence, mainly in the context of cancer research, suggests that the expression of ncRNAs, as well as their downstream targets, can be modulated by the intake of specific nutrients and natural compounds (Ferrero et al., 2021; Scoditti et al., 2021). For instance, natural products such as curcumin, resveratrol, and epigallocatechin-3-gallate, have been shown to exhibit anti-cancer effects by modulating the expression and the function of several ncRNAs, with consequent inhibition of cancer cell growth, induction of apoptosis, and increased therapeutic efficacy of conventional cancer therapy (Zhang B. et al., 2020; Sabo et al., 2021). Within the above mentioned natural compounds, resveratrol (3, 5, 4′-trihydroxystilbene), a nonflavonoid polyphenol contained in various foods, e.g., grapes, apples, blueberries, cocoa and peanuts, has been extensively studied in view of its numerous biological effects (Galiniak et al., 2019). Such effects are mediated by multiple pathways and targets and include antioxidant, anti-inflammatory, neuroprotective, cardioprotective, immunomodulatory, anti-platelet, and anticancer properties (Koushki et al., 2018; Shaito et al., 2020; Giordo et al., 2021b). Moreover, resveratrol has been found to modulate several aspects of cancer biology, such as tumor cell growth, migration, invasion, and resistance/evasion to apoptosis, by controlling the expression of miRNAs and lncRNAs (Wang et al., 2019). However, the ability of resveratrol to modulate the expression profile of ncRNAs has been also reported in other pathophysiological states (Latruffe et al., 2015; Zhu et al., 2020b; Shu et al., 2021). In this review, we critically appraise the available evidence regarding the resveratrol-mediated regulation of miRNAs and lncRNAs in various pathological conditions, particularly cancer, metabolic disorders, inflammation and ischemia.
Insulin Resistance, Diabetes, and Obesity
The hormone insulin, secreted by pancreatic β-cells in response to high blood glucose, lowers serum glucose concentrations by stimulating glucose uptake into the cells (Fu et al., 2013). Insulin resistance, a condition associated with reduced cell sensitivity to insulin, predisposes to type 2 diabetes (T2D) (Cerf, 2013). Recent studies have shown that resveratrol and metformin (MET), a first-line drug for the management of type 2 diabetes (Giannarelli et al., 2003), exert glucose-lowering effects through similar mechanisms, i.e., reduced hepatic gluconeogenesis, inflammation and oxidative stress, mainly through the activation of NAD-dependent deacetylase SIRT1 and AMP-activated protein kinase (AMPK) (Dludla et al., 2020). Based on this finding, a study has sought to determine whether such effects are mediated by lncRNAs, performing a comparative analysis of liver tissue lncRNAs expression profiles by high-throughput sequencing from high-fat diet (HFD)-fed mice treated with resveratrol and MET (Shu et al., 2021). Both compounds attenuated liver insulin resistance by regulating lncRNAs, although MET exerted a more prominent effect. Moreover, both resveratrol and MET affected the PI3K/Akt signaling pathway through the NONMMUT034936.2 and G6PC target genes (Shu et al., 2021). By using the same experimental model (HFD-fed mice), another study identified mmu-miR-363-3p as the major miRNA involved in the beneficial effects of resveratrol on insulin resistance. Specifically, resveratrol improved insulin resistance by upregulating mmu-miR-363-3p via the PI3K-Akt pathway, and reducing the expression of FOXO1 and G6PC, the downstream effectors of the PI3K-Akt pathway (Shu et al., 2020). The modulation of miR-33a/b and miR-122 has also been proposed as a treatment strategy for insulin resistance and dyslipidemia associated with obesity and metabolic syndrome; miR-122 is strongly associated with the risk of developing metabolic syndrome and type 2 diabetes (Willeit et al., 2017) whereas the downregulation of hepatic miR-33 improves metabolic homeostasis and liver function (Price et al., 2021). Proanthocyanidins, a polyphenolic compounds extracted from grape seeds, have been shown to suppress these key miRNAs in the liver of healthy and obese rats (Baselga-Escudero et al., 2015). In addition, Baselga-Escudero et al. investigated the modulatory effects of resveratrol and epigallocatechin gallate, polyphenols that are instead mainly found in the grape skin, toward these miRNAs (Baselga-Escudero et al., 2013). Both resveratrol and epigallocatechin gallate modulated miR-33 and miR-122 concentrations by direct binding, and the effect on miRNA expression was structure-dependent. The specific binding of polyphenols to miRNAs represents a new post-transcriptional mechanism by which polyphenols can modulate metabolism (Baselga-Escudero et al., 2013). Finally, resveratrol treatment in diabetic mice upregulated miR-18a-5p, a promising therapeutic target for preventing and attenuating diabetic nephropathy (Xu et al., 2017). Insulin resistance is also closely associated with the metabolic syndrome, a cluster of risk factors specific for cardiovascular disease such as obesity, high blood pressure, and dyslipidemia (Roberts et al., 2013). In the context of obesity, the main adipogenic genes involved in weight gain are regulated by different mechanisms, including miRNAs (Brandao et al., 2021). Another reported beneficial effect of resveratrol is body-fat lowering through the combined suppression of adipogenesis and lipogenesis (Aguirre et al., 2014). To identify the mechanisms underpinning this effect, Eseberri et al. investigated the possible involvement of miRNAs in the regulation of adipogenic transcription factors, peroxisome proliferator-activated receptor γ (pparγ) and CCAAT enhancer-binding proteins α and β (cebpβ and cebpα), by resveratrol and its glucuronide metabolites (Eseberri et al., 2017). Among all selected miRNAs (validated and predicted) targeting the adipogenic transcription factors, only miR-155 was modified by resveratrol and its metabolites. Specifically, up-regulation of miR-155, with the consequent down-regulation of the expression of the cebpβ gene, was observed (Eseberri et al., 2017). Additionaly, a miRNA microarray analysis in perirenal adipose tissue disclosed that resveratrol treatment led to the overexpression of miR-539-5p in the white adipose tissue of rats, and consequent inhibition of de novo lipogenesis, providing additional evidence of the anti-obesity effect of this flavonoid (Gracia et al., 2016). See Table 1 for summary.
Inflammatory States
Inflammation plays a critical pathophysiological role in a wide range of pathological states, including cancer, diabetes, obesity, and neurodegenerative diseases (Furman et al., 2019; Fois et al., 2020; Malhab et al., 2021). A significant body of research has provided strong evidence that resveratrol has anti-inflammatory properties by targeting transcription factors such as NF-kB and AP-1, and the gene COX-2 (Meng et al., 2021). The link between resveratrol and inflammation correlated-ncRNAs is still under investigation and the molecular mechanisms underpinning this interaction are yet to be identified. Resveratrol seems to modulate several miRNAs involved in inflammatory pathways, such as the proinflammatory miR-155, the anti-inflammatory miR-663, and the oncogenic miR-21 21 (Latruffe et al., 2015). A recent study has also reported the potential involvement of the lncRNA MALAT-1, a biomarker of inflammation (Wang et al., 2021). Treatment with resveratrol in rats with sepsis-induced kidney damage was associated with lower circulating concentrations of this lncRNA compared to untreated animals (Wang et al., 2021). Moreover, resveratrol reduced the intracellular concentrations of key pro-inflammatory cytokines, i.e., TNF-α, IL-1β and IL-6 (Wang et al., 2021). Based on previous studies reporting the participation of several MALAT1-sponged miRNAs, including miR-205 and miR204, in the pathogenesis of acute kidney injury (AKI) (Li et al., 2016; Chen et al., 2018), Wang et al. investigated the potential involvement of these miRNAs in their model of sepsis-induced AKI and the effects of resveratrol (Wang et al., 2021). Resveratrol downregulated miR-205 expression, suggesting that the inhibition of the lncRNA MALAT1/miR-205 axis by this flavonoid protects against sepsis-induced AKI (Wang et al., 2021). High-throughput RNA sequencing of chondrocytes treated with the pro-inflammatory factor IL-1beta, in presence or absence of resveratrol, revealed several differentially expressed lncRNAs, miRNAs, and mRNAs. (Yi et al., 2021). Moreover, combining bioinformatics methods with RT-PCR validation, a competitive endogenous RNA (ceRNA) network, involving the lncRNA LINC00654, miR-210-5p, and the Opioid Growth Factor Receptor Like 1 (OGFRL1), was identified (Yi et al., 2021). In this network, the mutual interaction between lncRNAs and miRNAs may influence cellular metabolic activity and functions as lncRNAs may competitively bind miRNAs, weakening the miRNA responses on the mRNA target and regulating genes at the post-transcriptional level (Yi et al., 2021). Resveratrol treatment upregulated LINC00654 and OGFRL1 and downregulated miR-210-5p in chondrocytes, suggesting that LINC00654 acts as ceRNA by competitively occupying the shared binding sequences of miR-210-5p in the OGFRL1 mRNA, ultimately affecting its gene expression (Yi et al., 2021). Another association between resveratrol and miRNA has been identified by Song et al. (Song et al., 2016) in human THP-1 macrophages. In this study, resveratrol decreased the inflammatory response through the modulation of miR-Let7A, a well-known tumor suppressor miRNA that modulates inflammation and apoptosis. In another study in mice with induced allergic asthma, resveratrol downregulated the expression of miR-34a, with consequent overexpression of FOXP3, a critically important transcription factor for the development and function of asthma-implicated regulatory T-cells (Tregs) (Alharris et al., 2018). Resveratrol may also be effective in models of acute lung damage, e.g., following exposure to staphylococcal enterotoxin B (SEB) (Alghetaa et al., 2018). In mice, Alghetaa et al. (Alghetaa et al., 2018) showed that 5-day resveratrol treatment in SEB-treated animals resulted in 100% survival compared to 0% survival in untreated animals. miRNA expression analysis in lung immune cells showed altered expression of different ncRNAs, particularly miR193-a, which was significantly activated in SEB-treated animals and down-regulated after resveratrol treatment. miR193-a seems to promote anti-inflammatory responses through activation of TGF-beta signaling and death receptor-6 apoptotic pathways (Alghetaa et al., 2018). The authors suggest that the SEB-activated lung injury can be prevented by resveratrol through the modulation of these anti-inflammatory miRNAs (Alghetaa et al., 2018). It is well known that resveratrol is a strong Sirtuins activator, above all SIRT1 that possesses anti-inflammatory activity (Borra et al., 2005; Moraes et al., 2020). SIRT1 seems to exert its effects through miRNAs, particularly miR-204, however the molecular mechanisms involved are still unknown (Li et al., 2015). Using lipopolysaccharide (LPS)-stimulated mice microglia cell lines, Li et al. (Li et al., 2015) demonstrated that SIRT1 overexpression and miR-204 inhibition counteracted LPS-induced inflammation similarly to resveratrol treatment. The latter promoted the activity of SIRT1 through miR-204. A significant number of studies have reported that the anticancer effects of resveratrol are strongly correlated with its anti-inflammatory activity (Ren et al., 2021). In a mice study on chemopreventive mechanisms of colitis-associated tumorigenesis, resveratrol treatment decreased markers of inflammation such as IL-6, tumor necrosis factor-alpha (protein levels), and COX-2 mRNA (mRNA levels) (Altamemi et al., 2014). Over 100 miRNAs appeared involved in these effects. Among them, miRNA-101b and miRNA-455 were significantly upregulated, suggesting that the interplay with different miRNAs may play an important role in the pleiotropic effects of resveratrol (Altamemi et al., 2014). See Table 2 for summary.
Liver Fibrosis and Pulmonary Vascular Remodeling
Factors such as high alcohol consumption, viral infections and autoimmune diseases can lead to persistent inflammation and hepatotoxic effects (Suk and Kim, 2015). Subsequently, fibrosis or scarring of the liver may occur due to overactivation of hepatic stellate cells (HSCs) and excessive deposits of extracellular matrix proteins (Suk and Kim, 2015; Zhu et al., 2020a). The more extreme forms, i.e., cirrhosis, may culminate in fatal systemic inflammation and liver failure. Treatment of the disease mainly involve strategies that mitigate the triggering factors or, ultimately, liver transplant (Zhu et al., 2020a). In this context, resveratrol-elicited reduction of liver failure (LF) severity is mainly associated with its anti-inflammatory and antioxidative activity (Hessin et al., 2017; Liu et al., 2017). In a rat model of LF, the liver tissue of resveratrol treated animals demonstrated well defined hepatocytes, in contrast to the untreated group which displayed necrotic cells with abnormal features such as collagen fiber hyperplasia and pseudolobuli formation (Zhu et al., 2020a). Various concentrations of resveratrol were able to reduce liver cell death, normalize liver lobule structure and decrease excess collagen fiber deposits. In addition, resveratrol at various doses upregulated the expression of the autophagy proteins Beclin1 and Atg7, with consequent increase in autophagy in experimental models of LF (Zhu et al., 2020a). In LF, autophagy is often blocked by preventing full fusion of autophagosomes with lysosomes, resulting in increased liver damage (Zou et al., 2020). Autophagy is an important mechanism that facilitates elimination of misfolded and aggregated proteins and dysfunctional organelles and prevents excessive growth (Ravanan et al., 2017). Thus, a potentially significant therapeutic effect of resveratrol consists in the ability to induce protective autophagy in LF models, mitigating inflammation and excessive growth of HSC cells. In rat models of LF, animals treated with resveratrol at various doses demonstrated a dose-dependent increase in phosphatase and tensin homolog (PTEN), which is an important inducer of apoptosis in hepatic stellate cells in LF (HSCs) (Zhu et al., 2020a). Owing to its ability to inhibit downstream mitogenic PI3K/AKT, PTEN mitigates cell activation, thereby exerting a protective effect against LF (Zhu et al., 2020a). The ability of resveratrol to increase PTEN was partially attributed to a significant reduction in miR-20a expression in rat LF models. This miR is associated with LF and, incidentally, contains binding sites for PTEN. Binding to PTEN results in its reduced activation and thus diminished ability to activate cell autophagy in LF. Thus, resveratrol has the potential to slow the development of LF by suppressing miR-20a (Zhu et al., 2020a). Resveratrol has also demonstrated beneficial effects in pulmonary hypertension (PH), a progressive disease of the pulmonary arteries which ultimately results in pulmonary remodeling, vasoconstriction and increased pulmonary arterial pressure (Thenappan et al., 2018). Vascular remodeling, characterized by excessive proliferation of pulmonary artery smooth muscle cells (PAMCs), is a key feature of PH and a target for intervention (Tao et al., 2019). Interestingly, resveratrol was recently shown to induce a protective effect in vivo against PH (Liu et al., 2020). Specifically, in rat models of monocrotaline (MCT)-induced PH, treatment with resveratrol attenuated pulmonary vascular remodeling (Liu et al., 2020). This was associated with the increased expression of miR-638. In vitro, resveratrol was able to prevent PDGF-induced over-proliferation in pulmonary arterial smooth muscle cells (PASMCs) (Liu et al., 2020). Previous studies have highlighted the crucial role of NR4A3, a type of NR4A nuclear receptor superfamily, in stimulating PASMCs proliferation (Rodríguez-Calvo et al., 2013; Wang et al., 2015). NR4A3 was later shown to upregulate PASMC proliferation in a cyclin D1-dependent manner (Liu et al., 2020). In vitro, miR-638 mimics diminished PASMC proliferation, whereas anti-miR-638 resulted in enhanced cell proliferation, highlighting the role of this miR in halting proliferation (Liu et al., 2020). Luciferase reporter assay ultimately demonstrated the miR-638 directly targets NR4A3, suggesting that its anti-proliferative effect is mediated by the inhibition of NR4A3, thereby preventing downstream activation of cyclin D1 (Liu et al., 2020). Thus, by upregulating the expression of miR-638, resveratrol demonstrates a protective role in subverting pulmonary vascular remodeling related to excessive PASMC proliferation. See Table 3 for summary. See Table 3 for summary.
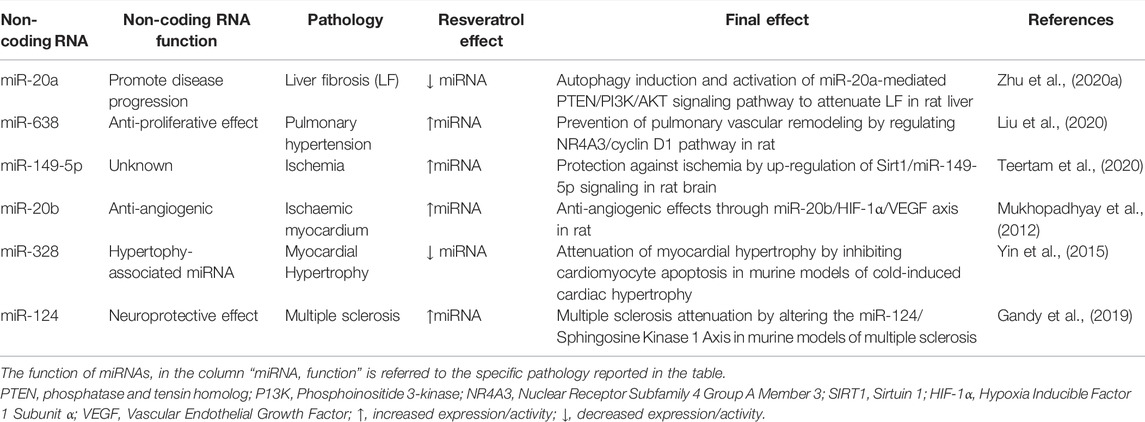
TABLE 3. Liver fibrosis, pulmonary vascular remodeling, low ambient cardiovascular disease, multiple sclerosis, ischemia, and osteoporosis.
Low Ambient Cardiovascular Disease, Multiple Sclerosis, and Osteoporosis
Cardiovascular disease (CVD) is one of the three leading causes of death worldwide (Yin et al., 2015). There has been an increasing recognition that cold temperature is a risk factor of CVD, particularly as it leads to cardiac hypertrophy and hypertension (Sun, 2010; Yin et al., 2015). In murine models of cold-induced cardiac hypertrophy, resveratrol was shown to mitigate myocardial enlargement, damage caused by fibrosis, and myofibril disarray. Interestingly, heart function, assessed as ejection fraction (EF) using echocardiogram, was also improved with resveratrol. A resveratrol-mediated reduction of the hypertrophy-associated miR-328 was also observed (Li et al., 2014; Yin et al., 2015). miR-328 has been shown to induce cardiac hypertrophy in murine models. This was associated with reduced sarcoplasmic reticulum Ca2+-ATPase (SERCA2a) levels, a major transporter of Ca2+ from the cytosol back into the sarcoplasmic reticulum (Li et al., 2014). As a result, there was increased accumulation of intracellular Ca2+, resulting in elevated calcineurin and NFATc3 nuclear translocation. Activation of this pathway has been shown to regulate cardiac hypertrophy (Molkentin, 2004). Therefore, the suppression of miR-328 expression caused by resveratrol might prevent SERCA2Aa downregulation and, consequently, cardiac hypertrophy.
Protective effects of resveratrol have also been observed in murine models of multiple sclerosis, mainly through a reduction in central nervous system inflammation (Gandy et al., 2019). Interestingly, the miR profile of the encephalitogenic CD4+ T cells from these mice was altered, particularly those related to cellular proliferation. Resveratrol significantly increased miR-124 in these cells, thereby allowing inhibition of its target gene sphingosine kinase 1 (SK1) (Gandy et al., 2019). As SK1 is a lipid enzyme involved in activating signaling pathways leading to cell migration, proliferation, and inflammation, while also preventing apoptosis (Proia and Hla, 2015; Li et al., 2021), its inhibition by miR-124 might account for the neuroprotective effects of resveratrol in these studies. Resveratrol has also shown promising microRNA-mediated effects in models of osteoporosis, a disabling condition characterized by degradation of the bone microstructure and reduction in bone mass (Das and Crockett, 2013). Previously, ovariectomized rats were shown to benefit from treatment with resveratrol, as indicated by enhanced bone mineral density (BMD) of the epiphyses (Liu et al., 2005). In an experiment conducted by Guo et al., female ovariectomized rats treated with resveratrol demonstrated increased BMD compared to controls (Guo et al., 2015). Increased concentrations of serum calcium, osteocalcin, and calcium/phosphate ratios, as well as a reduction in calcium urinary excretion, were also observed (Guo et al., 2015). Examination of the miR profile in the treatment group highlighted a significantly reduced expression of miR-338-3p. miR-338-3p knockdown in human osteoblast (HOB) cells resulted in increased expression of runt-related transcription factor 2 (RUNX2), confirming the TargetScan prediction that this miR can directly bind to and inhibit its expression (Guo et al., 2015). Inhibition of miR-338-3p expression resulted in increased cell proliferation and calcium deposition. These effects are attributed to the effect of RUNX2 on osteoblasts and odontoblasts (Miyazaki et al., 2008; Li et al., 2011). This transcription factor is involved in the signaling pathways associated with bone morphogenetic protein (BMP) and transforming growth factor β (TGF β), both of which contribute to osteoblast development and growth (Guo et al., 2015). Thus, stimulation of RUNX2 ultimately results in downstream proliferation and differentiation of HOB cells. Resveratrol treatment likely removed the miR-338-3p-mediated suppression of RUNX2, facilitating differentiation of the cells which direct bone growth (Guo et al., 2015). See Table 3 for summary.
Ischemia
Ischemic stroke, resulting from vessel obstruction in the brain, represents the most common type of stroke worldwide (Meschia and Brott, 2018). Sirtuin 1 (SIRT1), a member of the nicotinamide adenine dinucleotide-dependent deacetylases, is widely expressed in various tissues and exerts protective effects against cellular stress, especially those associated with ischemia (Pantazi et al., 2013). Such effects include the attenuation of energy depletion, oxidative stress, and inflammation. SIRT1 was previously shown to deacetylate inducers of apoptosis, particularly the tumor-suppressor p53 (Vaziri et al., 2001). This is associated with a neuroprotective effect in the context of brain ischemia, likely by preventing apoptosis and the associated inflammation (Hernández-Jiménez et al., 2013). In a recent study in an animal model of ischemia-reperfusion injury (IRI) via middle cerebral artery occlusion (MCAO), pre-treatment with resveratrol exerted protective effects mediated by SIRT1 modulation (Teertam et al., 2020). Twenty-four hours after injury, there was a significant reduction in p53 and downstream caspase-3 activity (Teertam et al., 2020). This was likely a result of the increased miR-149-5p activity on SIRT1 stimulation. Thus, resveratrol modulation of the miR-149-5p/SIRT1 axis might exert neuroprotective effects against ischemia by preventing the apoptotic and inflammatory actions of p53 (Teertam et al., 2020).
In a related study, resveratrol, and its commercially available form, longevinex, were also investigated in animal models of cardiac ischemia/reperfusion (I/R) (Mukhopadhyay et al., 2012). Both treatments exerted anti-angiogenic effects in the heart, with significant reduction in infarct size, especially when resveratrol was used in combination with γ-tocotrienol. Importantly, this effect was attributed to miR-20b, a miR which is significantly down-regulated in the ischemic heart (Mukhopadhyay et al., 2012). Resveratrol treatment significantly reversed this decrease, once again especially when combination with γ-tocotrienol or given as longevinex. Concomitantly, there was a significant decrease, within the heart tissue, in the expression of hypoxia-inducible factor-1α (HIF-1α) and vascular endothelial growth factor (VEGF) (Mukhopadhyay et al., 2012), both of which modulate angiogenesis (Lee et al., 2019; Sousa Fialho et al., 2019). The reduction in these target proteins was attributed to the resveratrol-induced increase in miR-20b, as treatment with antagomir-miR-20b restored their pre-treatment concentrations (Mukhopadhyay et al., 2012). It is important to highlight that the strongest effect on miR-20b expression, and the consequent reduction in HIF-1α and VEGF, was achieved with longevinex, which contains the phenolic anti-oxidants, quercetin and ferulic acid in addition to resveratrol (Mukhopadhyay et al., 2012). The miR-20b/HIF-1α/VEGF axis represents another important signaling pathway through which resveratrol, especially in combination with other antioxidants, can exert therapeutic effects. See Table 3 for summary.
Cancer
Breast Cancer
A significant number of in vitro and in vivo studies have shown that resveratrol possesses anti-cancer properties through the modulation of key processes such as cell growth, invasion, migration and metastasis (Varoni et al., 2016), as well as the stimulation of various anti-inflammatory, antioxidant, anti-apoptotic and anti-angiogenic mechanisms (Trapp et al., 2010; Varoni et al., 2016). Resveratrol has also shown adjuvant anti-cancer effects by enhancing the sensitivity of cancer cells to chemotherapeutics, consequently overcoming drug resistance (Ko et al., 2017). Recent studies have shown that resveratrol modulates the expression of important oncogenic drivers and tumor suppressors, including miRNAs and lncRNAs (Varoni et al., 2016; Anastasiadou et al., 2018). Breast cancer is the most diagnosed female cancer worldwide and the most common type of cancer in women in developing countries (Gabriel and Domchek, 2010; Bray et al., 2018). The effects of resveratrol on breast cancer are controversial (Bartolacci et al., 2018) due to the fact that, as a phytoestrogen, it possesses both estrogenic and anti-estrogenic activities in ERɑ-positive breast cancer (Bhat et al., 2001). Moreover, the effects of resveratrol are highly dependent of the administration protocol, e.g., dose, frequency, and route (Pasciu et al., 2010; Giordo et al., 2013; Posadino et al., 2015; Bartolacci et al., 2018; Posadino et al., 2019; Shaito et al., 2020). By using a combination of secondary mining of published data and experimental validations (i.e., the mRNA expression profile from the Gene Expression Omnibus database and a miRNA expression profile previously published (Venkatadri et al., 2016)), along with the bioinformatics tools, STITCH, miRDB, and de novo motifs (used to predict the transcription factors motifs for differentially expressed genes), a recent bioinformatics study investigated the transcription factors–miRNA–mRNA coregulatory network involved in the inhibition of breast cancer cell proliferation by resveratrol. (Zhou et al., 2022). This integrative investigation highlights as resveratrol inhibit human breast cancer cells (MCF-7) proliferation by modulating the expression of genes, transcription factors, and miRNAs involved in cell cycle and apoptosis, such as E2F2, JUN, FOS, BRCA1, CDK1, CDKN1A, TNF, and hsa-miR34a-5p. Moreover, the combined assessment of STITCH and miRDB databases revealed that the inhibition of MCF-7 proliferation resulted from a dual action, i.e., activation of TF cell cycle inhibitors and inhibition of antiapoptosis-associated miRNAs (Zhou et al., 2022). An additional anti-cancer effect of resveratrol consists in the increased sensitivity of breast cancer cells to the conventional anti-cancer agent adriamycin. A recent study (Zhang W. et al., 2019) suggested that resveratrol may upregulate miR-122-5p, consequently causing cell-cycle arrest of adriamycin-resistant breast cancer cells by targeting apoptosis and cell cycle key regulators such as B-cell lymphoma 2 (Bcl-2) and cyclin-dependent kinases (CDKs) (Zhang W. et al., 2019). Tumor-suppressive miRNAs modulation is another mechanism used by resveratrol to control breast cancer cell proliferation (Venkatadri et al., 2016; Otsuka et al., 2018). For instance, according to Otsuka et al. (Otsuka et al., 2018), by activating the p53 pathway, resveratrol induces the expression of tumor-suppressive miR-34a, miR-424, and miR-503; this, in turn, suppresses the splicing regulator heterogeneous nuclear ribonucleoprotein A1 (HNRNPA1), which is associated with oncogenesis and tumor progression and is generally up-regulated in several cancer types in addition to breast cancer, e.g., colorectal cancer, lung cancer, and glioma (Park et al., 2016; Kim et al., 2017). This tumor suppressor miRNAs-associated resveratrol antiproliferative effect has been also reported by Venkatadri et al. (Venkatadri et al., 2016). In this study, miR-125b-5p, miR-200c-3p, miR-409-3p, miR-122-5p and miR-542-3p mediated the decreased expression of anti-apoptotic proteins, including Bcl-2 and X-linked inhibitor of apoptosis (XIAP) and CDKs proteins specific for G-phase arrest (Venkatadri et al., 2016). An elegant in vivo study highlighted that resveratrol may differentially influence the expression of DNA methyltransferase 3b (DNMT3b) and miRNAs in tumor tissue vs. normal tissue in a dose-dependent manner (Qin et al., 2014). Specifically, eighty-nine estradiol-(E2)-dependent mammary carcinoma female rats received 21-day treatment with estradiol plus high- or low-dose resveratrol. High doses caused a reduction in DNMT3b, the predominant methyltransferase in breast tumorigenesis, in tumor tissue and a concomitant increase in normal tissue (Qin et al., 2014). In addition, high doses increased, >two-fold, miR21, -129, -204, and -489 in tumor, and caused their reduction, by 10–50%, in normal tissue compared to untreated animals. By contrast, low doses increased the expression of miR10a, a tumor suppressor, and miR10b, associated with metastasis and advanced disease, in tumor tissue (Qin et al., 2014). As emphasized by the authors (Qin et al., 2014), the dose-dependent effect of resveratrol on mammary carcinogenesis warrants further investigations to identify the optimal dose in terms of risk/benefit ratio. This issue is particularly relevant in view of the challenges associated with the inherent poor solubility and bioavailability of resveratrol (Shaito et al., 2020). See summary in Table 4.
Ovarian Cancer
Resveratrol’s anti-tumor activity has also been reported in the context of ovarian cancer (OC) both in vitro and in vivo. Proposed mechanisms include autophagocytosis (Opipari et al., 2004), inhibition of proliferation and apoptosis induction (Liu et al., 2018), inhibition of glucose metabolism, and combined induction of autophagy and apoptosis (Tan et al., 2016). A crucial role in OC development is also played by several miRNAs (Alshamrani, 2020). For instance, the miR-34 family was found to be downregulated in OC tissues (Welponer et al., 2020) and one of its three members, miR-34a, was reported to function as a tumor suppressor in OC (Lv et al., 2018). A recent study showed a significant increase in miR-34a expression in resveratrol-treated OC cells (Yao et al., 2021), with consequent enhanced anti-tumor activity. The latter was attenuated by miR-34a-knockdown (Yao et al., 2021). This study also reported that miR-34a-mediated suppression of Bcl-2 in OC cells was responsible for the anti-cancer effects of resveratrol (Yao et al., 2021). Further confirmation of the resveratrol anti-neoplastic activity exerted via the ncRNAs-associated epigenetic modulation of intracellular pathways governing homeostasis, proliferation, death, and motility in OC cells has been provided by Vallino et al. (Vallino et al., 2020). In their study, combining miRNAs and lncRNAs microarray profiling of OC cells exposed to 24-hour resveratrol treatment with literature-based biochemical pathways and functional processes potentially associated with OC, resveratrol up-regulated seven miRNAs (including miR-1207-5p, miR-3665 and miR-4281) and five lncRNAs (including GAS5 and NBR2) and down-regulated two miRNAs and ten lncRNAs (including LINC00092, H19, MALAT1) (Vallino et al., 2020). See summary in Table 4.
Prostate Cancer
Prostate cancer (PC) is one of the leading causes of cancer death among men of all races (Crawford, 2003). Genetic modifications in oncogenes and tumor suppressor genes, mutations in androgen receptors, and alterations in lncRNAs or miRNAs are some of the risk factors associated with PC development (Marcelli et al., 2000; Taylor et al., 2010; Weiss et al., 2014). Pro-inflammatory cytokines, such as IL-6, are also critical for the progression of PC as they are associated with increased activation of the oncogene signal transducer and activator of transcription 3 (STAT3) (Culig et al., 2005) (Rojas et al., 2011). The interplay between lncRNAs and miRNAs has also been reported as key to modulate the expression of genes involved in PC development and progression (Hu et al., 2022). lncRNAs such as PC associated transcript-1 and -3 (PCAT1 and PCAT3) increase proliferation of cancer cells whereas PCAT29 decreases both proliferation and migration of PC cells (Malik et al., 2014). A recent study reported that both gene and protein level expression of PCAT29 were reduced in tumors compared to normal prostate tissues (Al Aameri et al., 2017). The IL-6/STAT3/miR-21 signaling pathway mediated this reduction, and IL-6 was the main inducer of PCAT29 suppression through STAT3 activation. STAT3 then activated the downstream target miR-21, which in turn downregulated PCAT29. By contrast, inhibition of miR-21 restored the basal expression of PCAT29 (Al Aameri et al., 2017). Notably, resveratrol was able to reverse PCAT29 downregulation via abrogation of IL-6/STAT3/miR-21 signaling, and a direct targeting of miR-21 by resveratrol was also suggested (Al Aameri et al., 2017). Other studies have similarly reported the ability of resveratrol to alter PC miRNA profiles. For instance, Dhar et al. (Dhar et al., 2015) showed that both resveratrol and pterostilbene, by repressing several members of the oncogenic miR-17 family, were able to rescue the defective expression of PTEN in prostate cancer. These miRNAs are indeed overexpressed in PC and directly target the 3′UTR of tumor suppressor gene PTEN (Phosphatase and Tensin homolog), reducing its expression (Dhar et al., 2015). Furthermore, Chen et al. (Chen et al., 2021) reported the downregulation of lncRNA DIO3OS by resveratrol in benign prostatic hyperplasia (BPH). Although the pathophysiological role of DIO3OS remains poorly known, Chen et al. demonstrated that DIO3OS is highly expressed in BPH tissues. Within this environment, acting via miR-656-3p and miR-485-5p, DIO3OS promotes epithelial-mesenchymal transition (EMT) by BPH-1 cells through the upregulation of two EMT key effectors, CTGF and ZEB1 (Chen et al., 2021). Moreover, both TGF-β1 and resveratrol modulated DIO3OS in a Smads-dependent manner: TGF-β1 upregulated DIO3OS expression, which favored EMT, whereas resveratrol downregulated it, suppressing EMT (Chen et al., 2021). See summary in Table 4.
Other Cancers
Colorectal cancer (CRC) is the third most common cancer worldwide. Mutations in the oncogene Kras are the most prevalent CRC drivers (Haggar and Boushey, 2009). An in vivo study showed the effects of a resveratrol-supplemented diet on genetically engineered mice, where the Kras locus is activated specifically in the distal colon (Saud et al., 2014). The intervention resulted in a 60% inhibition of tumor production before tumors were visible with colonoscopy. In animals with established tumors, 33% exhibited complete remission whereas 97% showed a decrease in tumor size (Saud et al., 2014). Analysis of miRNA expression in both tumor and non-tumor tissue of resveratrol fed mice indicated an increased expression of miR-96, suggesting that resveratrol may inhibit translation of Kras mRNA by inducing miR-96 overexpression (Saud et al., 2014). Using the human colorectal adenocarcinoma cell line HT-29, a recent in vitro study showed that resveratrol, alone or in combination with the telomerase inhibitor BIBR1532, reduced cell viability and decreased the expression of a set of CRC-associated lncRNAs, including CCAT1, CRNDE, HOTAIR, PCAT1, PVT1, and SNHG16 (Cesmeli et al., 2022). The study of Fu et al. (Fu J. et al., 2019) suggested that the effect of the resveratrol derivative, triacetyl resveratrol (TCRV), in inhibiting pancreatic cancer cells (AsPC-1) migration, invasion, and EMT was mediated by the miR-200 family members. The authors indirectly confirmed the hypothesis that the miR-200 family mediated the biological effects of TCRV as the overexpression of anti-miR-200a/b/c counteracted the inhibitory effects of TCRV on AsPC-1 cells migration and invasion (Fu J. et al., 2019). Besides, treatment with TCRV inhibited the activity of the miR-200 family’s transcriptional target, Zeb1, one of the main EMT inducers (Fu J. et al., 2019). In multiple myeloma (MM) cells, where the lncRNA NEAT1 is highly expressed and induces cells proliferation, migration and invasion, resveratrol reversed the negative effect of NEAT1 through the Wnt/β-catenin signaling pathway (Geng et al., 2018). The anti-tumor effects of resveratrol have been also reported in melanoma, both in vitro and in vivo, where this flavonoid significantly decreased the expression of the oncogenic miR-221 by regulating (NF-κB) (RELA) activity (Wu and Cui, 2017). In the context of lung cancer, a microarray analysis of gene expression reported by Yang et (Yang et al., 2015) indicated that lncRNA expression profiles were altered in resveratrol-treated lung cancer cells, with 21 upregulated and 19 downregulated. The lncRNA AK001796 displayed the most altered expression, which was high in untreated lung cancer cell lines but significantly low with resveratrol (Yang et al., 2015). In the same study, in vitro and in vivo experiments supported the proposition that AK001796 acts as an oncogene in lung cancer carcinogenesis and is involved in the anticancer effects of resveratrol (Yang et al., 2015). Another study investigating the epigenetic effects of resveratrol in lung cancer identified a signaling cascade, resveratrol|miRNA-520h|PP2A/C|Akt|NF-κB|FOXC2, in which resveratrol inhibits both gene and protein expression of the transcriptional factor FOXC2 by downregulating the oncogenic miRNA-520h and the downstream signaling cascade (Yu et al., 2013). FOXC2 is an inducer of EMT, tumor angiogenesis and metastasis, and elevated levels of FOXC2 are associated with advanced cancer and poor prognosis (Yu et al., 2013). Interestingly, treatment with resveratrol reversed the effect of FOXC2 and induced MET (transformation of mesenchyme to epithelium), the opposite of EMT (Yu et al., 2013). See summary in Table 4.
Conclusion
A significant number of studies published over the last 5 years has provided robust evidence that the natural flavonoid resveratrol can modulate critical homeostatic processes by interacting with a wide range of ncRNAs in the context of disease states that are typically characterized by a high inflammatory burden and oxidative stress (Figure 1). The discovery of such resveratrol-ncRNAs interactions adds to the well-known anti-inflammatory and antioxidant effects of this natural compound by allowing the identification of a series of mediators that could serve as additional druggable targets as well as markers of disease onset and progression. Notably, the reported modulatory effects of resveratrol on ncRNAs also applies to disease states with limited available therapeutic options, i.e., ovarian cancer, liver fibrosis and failure, and pulmonary hypertension, providing much-needed information for the discovery of new therapeutic strategies. Furthermore, the resveratrol-ncRNAs interactions seem to also exert beneficial effects that go beyond the established anti-inflammatory and antioxidant effects of resveratrol, e.g., addressing drug resistance in cancer through the increased sensitivity of cancer cells to conventional chemotherapeutic regimens.
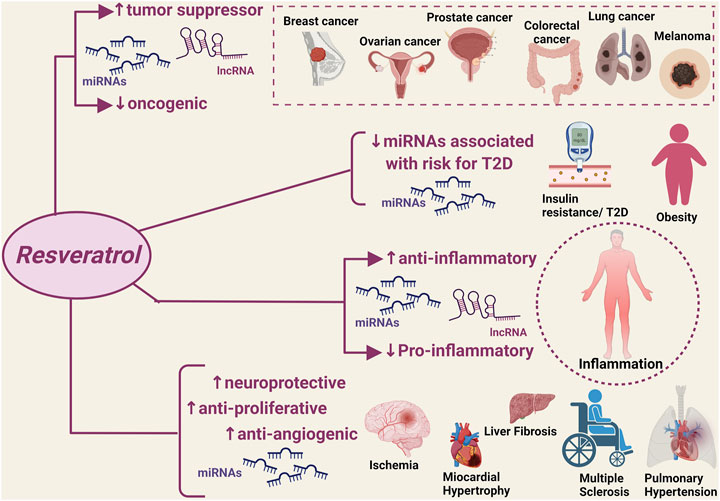
FIGURE 1. Modulatory effect of resveratrol on disease-associated non-coding RNAs. miRNAs, micro RNA; lncRNA, non-coding RNAs. ↑: increased expression/activity; ↓: decreased expression/activity.
Despite the promising results of the studies discussed in this review, additional research is urgently warranted to confirm the potential therapeutic effects of resveratrol and its analogues in robust animal models of disease using a wide range of doses, routes of administration, and treatment duration, and possible combinations with conventional medications and/or other natural compounds. This is particularly important given the complex pharmacokinetics of resveratrol and the delicate balance between efficacy and toxicity with this flavonoid (Salehi et al., 2018; Shaito et al., 2020), necessitating the identification of the most appropriate serum and tissue concentration ranges. Only then can the assessment of the potential therapeutic benefits of the resveratrol-ncRNAs interaction be conducted in patient cohorts using appropriately designed trials.
Author Contributions
Conceptualization, RG and GP; writing—original draft preparation, RG, ZW, AP, GE, and AE; writing—review and editing, RG, GP, and AM; supervision, GP and AM; project administration, GP; funding acquisition, GP and AM.
Funding
This work has been made possible thanks to grants from (Progetto Fondazione di Sardegna—bando 2022-2023 and FAR2020-Pintus) to GP and the (University of Sharjah Collaborative 2101050160) to GP and AM.
Conflict of Interest
The authors declare that the research was conducted in the absence of any commercial or financial relationships that could be construed as a potential conflict of interest.
Publisher’s Note
All claims expressed in this article are solely those of the authors and do not necessarily represent those of their affiliated organizations, or those of the publisher, the editors and the reviewers. Any product that may be evaluated in this article, or claim that may be made by its manufacturer, is not guaranteed or endorsed by the publisher.
References
Aguirre, L., Fernández-Quintela, A., Arias, N., and Portillo, M. (2014). Resveratrol: Anti-obesity Mechanisms of Action. Molecules 19, 18632–18655. doi:10.3390/molecules191118632
Al Aameri, R. F. H., Sheth, S., Alanisi, E. M. A., Borse, V., Mukherjea, D., Rybak, L. P., et al. (2017). Tonic Suppression of PCAT29 by the IL-6 Signaling Pathway in Prostate Cancer: Reversal by Resveratrol. PLoS One 12, e0177198. doi:10.1371/journal.pone.0177198
Alghetaa, H., Mohammed, A., Sultan, M., Busbee, P., Murphy, A., Chatterjee, S., et al. (2018). Resveratrol Protects Mice against SEB‐induced Acute Lung Injury and Mortality by miR‐193a Modulation that Targets TGF‐β Signalling. J. Cell Mol. Med. 22, 2644–2655. doi:10.1111/jcmm.13542
Alharris, E., Alghetaa, H., Seth, R., Chatterjee, S., Singh, N. P., Nagarkatti, M., et al. (2018). Resveratrol Attenuates Allergic Asthma and Associated Inflammation in the Lungs through Regulation of miRNA-34a that Targets FoxP3 in Mice. Front. Immunol. 9, 2992. doi:10.3389/fimmu.2018.02992
Alshamrani, A. A. (2020). Roles of microRNAs in Ovarian Cancer Tumorigenesis: Two Decades Later, what Have We Learned? Front. Oncol. 10, 1084. doi:10.3389/fonc.2020.01084
Altamemi, I., Murphy, E. A., Catroppo, J. F., Zumbrun, E. E., Zhang, J., Mcclellan, J. L., et al. (2014). Role of microRNAs in Resveratrol-Mediated Mitigation of Colitis-Associated Tumorigenesis in ApcMin/+ Mice. J. Pharmacol. Exp. Ther. 350, 99–109. doi:10.1124/jpet.114.213306
Anastasiadou, E., Jacob, L. S., and Slack, F. J. (2018). Non-coding RNA Networks in Cancer. Nat. Rev. Cancer 18, 5–18. doi:10.1038/nrc.2017.99
Bartolacci, C., Andreani, C., Amici, A., and Marchini, C. (2018). Walking a Tightrope: a Perspective of Resveratrol Effects on Breast Cancer. Curr. Protein Pept. Sci. 19, 311–322. doi:10.2174/1389203718666170111115914
Baselga-Escudero, L., Blade, C., Ribas-Latre, A., Casanova, E., Suarez, M., Torres, J. L., et al. (2013). Resveratrol and EGCG Bind Directly and Distinctively to miR-33a and miR-122 and Modulate Divergently Their Levels in Hepatic Cells. Nucleic acids Res. 42, 882–892. doi:10.1093/nar/gkt1011
Baselga-Escudero, L., Pascual-Serrano, A., Ribas-Latre, A., Casanova, E., Salvadó, M. J., Arola, L., et al. (2015). Long-term Supplementation with a Low Dose of Proanthocyanidins Normalized Liver miR-33a and miR-122 Levels in High-Fat Diet-Induced Obese Rats. Nutr. Res. 35, 337–345. doi:10.1016/j.nutres.2015.02.008
Bhat, K. P., Lantvit, D., Christov, K., Mehta, R. G., Moon, R. C., and Pezzuto, J. M. (2001). Estrogenic and Antiestrogenic Properties of Resveratrol in Mammary Tumor Models. Cancer Res. 61, 7456–7463.
Borra, M. T., Smith, B. C., and Denu, J. M. (2005). Mechanism of Human SIRT1 Activation by Resveratrol. J. Biol. Chem. 280, 17187–17195. doi:10.1074/jbc.m501250200
Brandao, B. B., Lino, M., and Kahn, C. R. (2021). Extracellular miRNAs as Mediators of Obesity‐associated Disease. J. Physiology 600 (5), 1155–1169. doi:10.1113/jp280910
Bray, F., Ferlay, J., Soerjomataram, I., Siegel, R. L., Torre, L. A., and Jemal, A. (2018). Global Cancer Statistics 2018: GLOBOCAN Estimates of Incidence and Mortality Worldwide for 36 Cancers in 185 Countries. CA a cancer J. Clin. 68, 394–424. doi:10.3322/caac.21492
Cerf, M. E. (2013). Beta Cell Dysfunction and Insulin Resistance. Front. Endocrinol. 4, 37. doi:10.3389/fendo.2013.00037
Cesmeli, S., Goker Bagca, B., Caglar, H. O., Ozates, N. P., Gunduz, C., and Biray Avci, C. (2022). Combination of Resveratrol and BIBR1532 Inhibits Proliferation of Colon Cancer Cells by Repressing Expression of LncRNAs. Med. Oncol. 39, 1–10. doi:10.1007/s12032-021-01611-w
Chen, Q., Huang, X., and Li, R. (2018). lncRNA MALAT1/miR-205-5p axis Regulates MPP+-induced Cell Apoptosis in MN9D Cells by Directly Targeting LRRK2. Am. J. Transl. Res. 10, 563–572.
Chen, Y., Xu, H., Liu, C., Gu, M., Zhan, M., Chen, Q., et al. (2021). LncRNA DIO3OS Regulated by TGF-Β1 and Resveratrol Enhances Epithelial Mesenchymal Transition of Benign Prostatic Hyperplasia Epithelial Cells and Proliferation of Prostate Stromal Cells. Transl. Androl. Urol. 10, 643–653. doi:10.21037/tau-20-1169
Costa, F. F. (2005). Non-coding RNAs: New Players in Eukaryotic Biology. Gene 357, 83–94. doi:10.1016/j.gene.2005.06.019
Crawford, E. D. (2003). Epidemiology of Prostate Cancer. Urology 62, 3–12. doi:10.1016/j.urology.2003.10.013
Culig, Z., Steiner, H., Bartsch, G., and Hobisch, A. (2005). Interleukin-6 Regulation of Prostate Cancer Cell Growth. J. Cell. Biochem. 95, 497–505. doi:10.1002/jcb.20477
Das, S., and Crockett, J. C. (2013). Osteoporosis - a Current View of Pharmacological Prevention and Treatment. Drug Des. Devel Ther. 7, 435–448. doi:10.2147/DDDT.S31504
Dehaini, H., Awada, H., El-Yazbi, A., Zouein, F. A., Issa, K., Eid, A. A., et al. (2019). MicroRNAs as Potential Pharmaco-Targets in Ischemia-Reperfusion Injury Compounded by Diabetes. Cells 8, 152. doi:10.3390/cells8020152
Derrien, T., Johnson, R., Bussotti, G., Tanzer, A., Djebali, S., Tilgner, H., et al. (2012). The GENCODE V7 Catalog of Human Long Noncoding RNAs: Analysis of Their Gene Structure, Evolution, and Expression. Genome Res. 22, 1775–1789. doi:10.1101/gr.132159.111
Dhar, S., Kumar, A., Rimando, A. M., Zhang, X., and Levenson, A. S. (2015). Resveratrol and Pterostilbene Epigenetically Restore PTEN Expression by Targeting oncomiRs of the miR-17 Family in Prostate Cancer. Oncotarget 6, 27214–27226. doi:10.18632/oncotarget.4877
Dludla, P. V., Silvestri, S., Orlando, P., Gabuza, K. B., Mazibuko-Mbeje, S. E., Nyambuya, T. M., et al. (2020). Exploring the Comparative Efficacy of Metformin and Resveratrol in the Management of Diabetes-Associated Complications: a Systematic Review of Preclinical Studies. Nutrients 12, 739. doi:10.3390/nu12030739
Dragomir, M. P., Knutsen, E., and Calin, G. A. (2018). SnapShot: Unconventional miRNA Functions. Cell 174, 1038. doi:10.1016/j.cell.2018.07.040
Eseberri, I., Lasa, A., Miranda, J., Gracia, A., and Portillo, M. P. (2017). Potential miRNA Involvement in the Anti-adipogenic Effect of Resveratrol and its Metabolites. PLoS One 12, e0184875. doi:10.1371/journal.pone.0184875
Ferrero, G., Carpi, S., Polini, B., Pardini, B., Nieri, P., Impeduglia, A., et al. (2021). Intake of Natural Compounds and Circulating microRNA Expression Levels: Their Relationship Investigated in Healthy Subjects with Different Dietary Habits. Front. Pharmacol. 11, 619200. doi:10.3389/fphar.2020.619200
Fois, A. G., Sotgiu, E., Scano, V., Negri, S., Mellino, S., Zinellu, E., et al. (2020). Effects of Pirfenidone and Nintedanib on Markers of Systemic Oxidative Stress and Inflammation in Patients with Idiopathic Pulmonary Fibrosis: a Preliminary Report. Antioxidants 9, 1064. doi:10.3390/antiox9111064
Fu, J., Shrivastava, A., Shrivastava, S. K., Srivastava, R. K., and Shankar, S. (2019a). Triacetyl Resveratrol Upregulates miRNA-200 and S-uppresses the Shh P-athway in P-ancreatic C-ancer: A P-otential T-herapeutic A-gent. Int. J. Oncol. 54, 1306–1316. doi:10.3892/ijo.2019.4700
Fu, P.-F., Zheng, X., Fan, X., and Lin, A.-F. (2019b). Role of Cytoplasmic lncRNAs in Regulating Cancer Signaling Pathways. J. Zhejiang Univ. Sci. B 20, 1–8. doi:10.1631/jzus.b1800254
Fu, Z., R. Gilbert, E., and Liu, D. (2013). Regulation of Insulin Synthesis and Secretion and Pancreatic Beta-Cell Dysfunction in Diabetes. Curr. diabetes Rev. 9, 25–53. doi:10.2174/157339913804143225
Furman, D., Campisi, J., Verdin, E., Carrera-Bastos, P., Targ, S., Franceschi, C., et al. (2019). Chronic Inflammation in the Etiology of Disease across the Life Span. Nat. Med. 25, 1822–1832. doi:10.1038/s41591-019-0675-0
Gabriel, C. A., and Domchek, S. M. (2010). Breast Cancer in Young Women. Breast Cancer Res. 12, 212. doi:10.1186/bcr2647
Galiniak, S., Aebisher, D., and Bartusik-Aebisher, D. (2019). Health Benefits of Resveratrol Administration. Acta Biochim. Pol. 66, 13–21. doi:10.18388/abp.2018_2749
Gandy, K. A. O., Zhang, J., Nagarkatti, P., and Nagarkatti, M. (2019). Resveratrol (3, 5, 4′-Trihydroxy-Trans-Stilbene) Attenuates a Mouse Model of Multiple Sclerosis by Altering the miR-124/Sphingosine Kinase 1 Axis in Encephalitogenic T Cells in the Brain. J. Neuroimmune Pharmacol. 14, 462–477. doi:10.1007/s11481-019-09842-5
Geng, W., Guo, X., Zhang, L., Ma, Y., Wang, L., Liu, Z., et al. (2018). Resveratrol Inhibits Proliferation, Migration and Invasion of Multiple Myeloma Cells via NEAT1-Mediated Wnt/β-Catenin Signaling Pathway. Biomed. Pharmacother. 107, 484–494. doi:10.1016/j.biopha.2018.08.003
Giannarelli, R., Aragona, M., Coppelli, A., and Del Prato, S. (2003). Reducing Insulin Resistance with Metformin: the Evidence Today. Diabetes & metabolism 29, 6S28–6S35. doi:10.1016/s1262-3636(03)72785-2
Giordo, R., Ahmed, Y., Allam, H., Abusnana, S., Pappalardo, L., Nasrallah, G. K., et al. (2021a). EndMT Regulation by Small RNAs in Diabetes-Associated Fibrotic Conditions: Potential Link with Oxidative Stress. Front. Cell Dev. Biol. 9, 1275. doi:10.3389/fcell.2021.683594
Giordo, R., Cossu, A., Pasciu, V., Hoa, P. T., Posadino, A. M., and Pintus, G. (2013). Different Redox Response Elicited by Naturally Occurring Antioxidants in Human Endothelial Cells. Open Biochem. J. 7, 44–53. doi:10.2174/1874091x01307010044
Giordo, R., Zinellu, A., Eid, A. H., and Pintus, G. (2021b). Therapeutic Potential of Resveratrol in COVID-19-Associated Hemostatic Disorders. Molecules 26, 856. doi:10.3390/molecules26040856
Gracia, A., Miranda, J., Fernández-Quintela, A., Eseberri, I., Garcia-Lacarte, M., Milagro, F. I., et al. (2016). Involvement of miR-539-5p in the Inhibition of De Novo Lipogenesis Induced by Resveratrol in White Adipose Tissue. Food Funct. 7, 1680–1688. doi:10.1039/c5fo01090j
Guo, D.-W., Han, Y.-X., Cong, L., Liang, D., and Tu, G.-J. (2015). Resveratrol Prevents Osteoporosis in Ovariectomized Rats by Regulating microRNA-338-3p. Mol. Med. Rep. 12, 2098–2106. doi:10.3892/mmr.2015.3581
Haggar, F., and Boushey, R. (2009). Colorectal Cancer Epidemiology: Incidence, Mortality, Survival, and Risk Factors. Clin. colon rectal Surg. 22, 191–197. doi:10.1055/s-0029-1242458
Hernández-Jiménez, M., Hurtado, O., Cuartero, M. I., Ballesteros, I., Moraga, A., Pradillo, J. M., et al. (2013). Silent Information Regulator 1 Protects the Brain against Cerebral Ischemic Damage. Stroke 44, 2333–2337. doi:10.1161/strokeaha.113.001715
Hessin, A., Hegazy, R., Hassan, A., Yassin, N., and Kenawy, S.-B. (2017). Resveratrol Prevents Liver Fibrosis via Two Possible Pathways: Modulation of Alpha Fetoprotein Transcriptional Levels and Normalization of Protein Kinase C Responses. Indian J. Pharmacol. 49, 282–289. doi:10.4103/ijp.ijp_299_16
Hu, C.-Y., Wu, K.-Y., Lin, T.-Y., and Chen, C.-C. (2022). The Crosstalk of Long Non-coding RNA and MicroRNA in Castration-Resistant and Neuroendocrine Prostate Cancer: Their Interaction and Clinical Importance. Int. J. Mol. Sci. 23, 392.
Kim, Y.-J., Kim, B.-R., Ryu, J.-S., Lee, G.-O., Kim, H.-R., Choi, K.-H., et al. (2017). HNRNPA1, a Splicing Regulator, Is an Effective Target Protein for Cervical Cancer Detection: Comparison with Conventional Tumor Markers. Int. J. Gynecol. Cancer 27. doi:10.1097/igc.0000000000000868
Ko, J.-H., Sethi, G., Um, J.-Y., Shanmugam, M. K., Arfuso, F., Kumar, A. P., et al. (2017). The Role of Resveratrol in Cancer Therapy. Ijms 18, 2589. doi:10.3390/ijms18122589
Koushki, M., Amiri-Dashatan, N., Ahmadi, N., Abbaszadeh, H.-A., and Rezaei-Tavirani, M. (2018). Resveratrol: A Miraculous Natural Compound for Diseases Treatment. Food Sci. Nutr. 6, 2473–2490. doi:10.1002/fsn3.855
Lander, E. S., Linton, L. M., Birren, B., Nusbaum, C., Zody, M. C., Baldwin, J., et al. (2001). Initial Sequencing and Analysis of the Human Genome.
Latruffe, N., Lançon, A., Frazzi, R., Aires, V., Delmas, D., Michaille, J.-J., et al. (2015). Exploring New Ways of Regulation by Resveratrol Involving miRNAs, with Emphasis on Inflammation. Ann. N.Y. Acad. Sci. 1348, 97–106. doi:10.1111/nyas.12819
Lee, J. W., Ko, J., Ju, C., and Eltzschig, H. K. (2019). Hypoxia Signaling in Human Diseases and Therapeutic Targets. Exp. Mol. Med. 51, 1–13. doi:10.1038/s12276-019-0235-1
Leung, A., and Natarajan, R. (2018). Long Noncoding RNAs in Diabetes and Diabetic Complications. Antioxidants redox Signal. 29, 1064–1073. doi:10.1089/ars.2017.7315
Li, C., Li, X., Gao, X., Zhang, R., Zhang, Y., Liang, H., et al. (2014). MicroRNA-328 as a Regulator of Cardiac Hypertrophy. Int. J. Cardiol. 173, 268–276. doi:10.1016/j.ijcard.2014.02.035
Li, J., Wang, J., Chen, Y., Li, S., Jin, M., Wang, H., et al. (2016). LncRNA MALAT1 Exerts Oncogenic Functions in Lung Adenocarcinoma by Targeting miR-204. Am. J. Cancer Res. 6, 1099–1107.
Li, L., Sun, Q., Li, Y., Yang, Y., Yang, Y., Chang, T., et al. (2015). Overexpression of SIRT1 Induced by Resveratrol and Inhibitor of miR-204 Suppresses Activation and Proliferation of Microglia. J. Mol. Neurosci. 56, 858–867. doi:10.1007/s12031-015-0526-5
Li, S., Kong, H., Yao, N., Yu, Q., Wang, P., Lin, Y., et al. (2011). The Role of Runt-Related Transcription Factor 2 (Runx2) in the Late Stage of Odontoblast Differentiation and Dentin Formation. Biochem. Biophysical Res. Commun. 410, 698–704. doi:10.1016/j.bbrc.2011.06.065
Li, Z., Zhang, L., Liu, D., and Wang, C. (2021). Ceramide Glycosylation and Related Enzymes in Cancer Signaling and Therapy. Biomed. Pharmacother. 139, 111565. doi:10.1016/j.biopha.2021.111565
Liu, C., Liao, J.-Z., and Li, P.-Y. (2017). Traditional Chinese Herbal Extracts Inducing Autophagy as a Novel Approach in Therapy of Nonalcoholic Fatty Liver Disease. Wjg 23, 1964–1973. doi:10.3748/wjg.v23.i11.1964
Liu, Y.-y., Zhang, W.-y., Wang, C.-g., Huang, J.-a., Jiang, J.-h., and Zeng, D.-x. (2020). Resveratrol Prevented Experimental Pulmonary Vascular Remodeling via miR-638 Regulating NR4A3/cyclin D1 Pathway. Microvasc. Res. 130, 103988. doi:10.1016/j.mvr.2020.103988
Liu, Y., Tong, L., Luo, Y., Li, X., Chen, G., and Wang, Y. (2018). Resveratrol Inhibits the Proliferation and Induces the Apoptosis in Ovarian Cancer Cells via Inhibiting Glycolysis and Targeting AMPK/mTOR Signaling Pathway. J Cell. Biochem. 119, 6162–6172. doi:10.1002/jcb.26822
Liu, Z. P., Li, W. X., Yu, B., Huang, J., Sun, J., Huo, J. S., et al. (2005). Effects of Trans-resveratrol from Polygonum Cuspidatum on Bone Loss Using the Ovariectomized Rat Model. J. Med. Food 8, 14–19. doi:10.1089/jmf.2005.8.14
López-Urrutia, E., Bustamante Montes, L. P., Ladrón de Guevara Cervantes, D., Pérez-Plasencia, C., and Campos-Parra, A. D. (2019). Crosstalk between Long Non-coding RNAs, Micro-RNAs and mRNAs: Deciphering Molecular Mechanisms of Master Regulators in Cancer. Front. Oncol. 9, 669. doi:10.3389/fonc.2019.00669
Lv, T., Song, K., Zhang, L., Li, W., Chen, Y., Diao, Y., et al. (2018). miRNA-34a Decreases Ovarian Cancer Cell Proliferation and Chemoresistance by Targeting HDAC1. Biochem. Cell Biol. 96, 663–671. doi:10.1139/bcb-2018-0031
Malhab, L. J. B., Saber-Ayad, M. M., Al-Hakm, R., Nair, V. A., Paliogiannis, P., Pintus, G., et al. (2021). Chronic Inflammation and Cancer: The Role of Endothelial Dysfunction and Vascular Inflammation. Cpd 27, 2156–2169. doi:10.2174/1381612827666210303143442
Malik, R., Patel, L., Prensner, J. R., Shi, Y., Iyer, M. K., Subramaniyan, S., et al. (2014). The lncRNA PCAT29 Inhibits Oncogenic Phenotypes in Prostate Cancer. Mol. Cancer Res. 12, 1081–1087. doi:10.1158/1541-7786.mcr-14-0257
Marcelli, M., Ittmann, M., Mariani, S., Sutherland, R., Nigam, R., Murthy, L., et al. (2000). Androgen Receptor Mutations in Prostate Cancer. Cancer Res. 60, 944–949.
Meng, T., Xiao, D., Muhammed, A., Deng, J., Chen, L., and He, J. (2021). Anti-inflammatory Action and Mechanisms of Resveratrol. Molecules 26, 229. doi:10.3390/molecules26010229
Meschia, J. F., and Brott, T. (2018). Ischaemic Stroke. Eur. J. Neurol. 25, 35–40. doi:10.1111/ene.13409
Miyazaki, T., Kanatani, N., Rokutanda, S., Yoshida, C., Toyosawa, S., Nakamura, R., et al. (2008). Inhibition of the Terminal Differentiation of Odontoblasts and Their Transdifferentiation into Osteoblasts in Runx2 Transgenic Mice. Archives Histology Cytol. 71, 131–146. doi:10.1679/aohc.71.131
Molkentin, J. (2004). Calcineurin?NFAT Signaling Regulates the Cardiac Hypertrophic Response in Coordination with the MAPKs. Cardiovasc. Res. 63, 467–475. doi:10.1016/j.cardiores.2004.01.021
Moraes, D. S., Moreira, D. C., Andrade, J. M. O., and Santos, S. H. S. (2020). Sirtuins, Brain and Cognition: A Review of Resveratrol Effects. IBRO Rep. 9, 46–51. doi:10.1016/j.ibror.2020.06.004
Mukhopadhyay, P., Das, S., Ahsan, M. K., Otani, H., and Das, D. K. (2012). Modulation of microRNA 20b with Resveratrol and Longevinex Is Linked with Their Potent Anti-angiogenic Action in the Ischaemic Myocardium and Synergestic Effects of Resveratrol and γ-tocotrienol. J. Cell. Mol. Med. 16, 2504–2517. doi:10.1111/j.1582-4934.2011.01480.x
O'brien, J., Hayder, H., Zayed, Y., and Peng, C. (2018). Overview of microRNA Biogenesis, Mechanisms of Actions, and Circulation. Front. Endocrinol. 9, 402. doi:10.3389/fendo.2018.00402
Opipari, A. W., Tan, L., Boitano, A. E., Sorenson, D. R., Aurora, A., and Liu, J. R. (2004). Resveratrol-induced Autophagocytosis in Ovarian Cancer Cells. Cancer Res. 64, 696–703. doi:10.1158/0008-5472.can-03-2404
Otsuka, K., Yamamoto, Y., and Ochiya, T. (2018). Regulatory Role of Resveratrol, a microRNA-Controlling Compound, in HNRNPA1 Expression, Which Is Associated with Poor Prognosis in Breast Cancer. Oncotarget 9, 24718–24730. doi:10.18632/oncotarget.25339
Palazzo, A. F., and Lee, E. S. (2015). Non-coding RNA: what Is Functional and what Is Junk? Front. Genet. 6, 2. doi:10.3389/fgene.2015.00002
Pantazi, E., Zaouali, M. A., Bejaoui, M., Folch-Puy, E., Ben Abdennebi, H., and Roselló-Catafau, J. (2013). Role of Sirtuins in Ischemia-Reperfusion Injury. Wjg 19, 7594–7602. doi:10.3748/wjg.v19.i43.7594
Park, W. C., Kim, H. R., Kang, D. B., Ryu, J. S., Choi, K. H., Lee, G. O., et al. (2016). Comparative Expression Patterns and Diagnostic Efficacies of SR Splicing Factors and HNRNPA1 in Gastric and Colorectal Cancer. BMC cancer 16, 358. doi:10.1186/s12885-016-2387-x
Pasciu, V., Posadino, A. M., Cossu, A., Sanna, B., Tadolini, B., Gaspa, L., et al. (2010). Akt Downregulation by Flavin Oxidase-Induced ROS Generation Mediates Dose-dependent Endothelial Cell Damage Elicited by Natural Antioxidants. Toxicol. Sci. 114, 101–112. doi:10.1093/toxsci/kfp301
Peter, M. E. (2010). Targeting of mRNAs by Multiple miRNAs: the Next Step. Oncogene 29, 2161–2164. doi:10.1038/onc.2010.59
Posadino, A. M., Cossu, A., Giordo, R., Zinellu, A., Sotgia, S., Vardeu, A., et al. (2015). Resveratrol Alters Human Endothelial Cells Redox State and Causes Mitochondrial-dependent Cell Death. Food Chem. Toxicol. 78, 10–16. doi:10.1016/j.fct.2015.01.017
Posadino, A. M., Giordo, R., Cossu, A., Nasrallah, G. K., Shaito, A., Abou-Saleh, H., et al. (2019). Flavin Oxidase-Induced ROS Generation Modulates PKC Biphasic Effect of Resveratrol on Endothelial Cell Survival. Biomolecules 9, 209. doi:10.3390/biom9060209
Price, N. L., Zhang, X., Fernández-Tussy, P., Singh, A. K., Burnap, S. A., Rotllan, N., et al. (2021). Loss of Hepatic miR-33 Improves Metabolic Homeostasis and Liver Function without Altering Body Weight or Atherosclerosis. Proc. Natl. Acad. Sci. 118. doi:10.1073/pnas.2006478118
Proia, R. L., and Hla, T. (2015). Emerging Biology of Sphingosine-1-Phosphate: its Role in Pathogenesis and Therapy. J. Clin. Invest. 125, 1379–1387. doi:10.1172/jci76369
Qin, W., Zhang, K., Clarke, K., Weiland, T., and Sauter, E. R. (2014). Methylation and miRNA Effects of Resveratrol on Mammary Tumors vs. Normal Tissue. Nutr. cancer 66, 270–277. doi:10.1080/01635581.2014.868910
Ravanan, P., Srikumar, I. F., and Talwar, P. (2017). Autophagy: The Spotlight for Cellular Stress Responses. Life Sci. 188, 53–67. doi:10.1016/j.lfs.2017.08.029
Ren, B., Kwah, M. X.-Y., Liu, C., Ma, Z., Shanmugam, M. K., Ding, L., et al. (2021). Resveratrol for Cancer Therapy: Challenges and Future Perspectives. Cancer Lett. 515, 63–72. doi:10.1016/j.canlet.2021.05.001
Roberts, C. K., Hevener, A. L., and Barnard, R. J. (2013). Metabolic Syndrome and Insulin Resistance: Underlying Causes and Modification by Exercise Training. Compr. Physiol. 3, 1–58. doi:10.1002/cphy.c110062
Rodríguez-Calvo, R., Guadall, A., Calvayrac, O., Navarro, M. A., Alonso, J., Ferrán, B., et al. (2013). Over-expression of Neuron-Derived Orphan Receptor-1 (NOR-1) Exacerbates Neointimal Hyperplasia after Vascular Injury. Hum. Mol. Genet. 22, 1949–1959. doi:10.1093/hmg/ddt042
Rojas, A., Liu, G., Coleman, I., Nelson, P. S., Zhang, M., Dash, R., et al. (2011). IL-6 Promotes Prostate Tumorigenesis and Progression through Autocrine Cross-Activation of IGF-IR. Oncogene 30, 2345–2355. doi:10.1038/onc.2010.605
Sabo, A. A., Dudau, M., Constantin, G. L., Pop, T. C., Geilfus, C. M., Naccarati, A., et al. (2021). Two Worlds Colliding: The Interplay between Natural Compounds and Non-coding Transcripts in Cancer Therapy. Front. Pharmacol. 12, 652074. doi:10.3389/fphar.2021.652074
Salehi, B., Mishra, A., Nigam, M., Sener, B., Kilic, M., Sharifi-Rad, M., et al. (2018). Resveratrol: A Double-Edged Sword in Health Benefits. Biomedicines 6, 91. doi:10.3390/biomedicines6030091
Saud, S. M., Li, W., Morris, N. L., Matter, M. S., Colburn, N. H., Kim, Y. S., et al. (2014). Resveratrol Prevents Tumorigenesis in Mouse Model of Kras Activated Sporadic Colorectal Cancer by Suppressing Oncogenic Kras Expression. Carcinogenesis 35, 2778–2786. doi:10.1093/carcin/bgu209
Scoditti, E., Naccarati, A., Carpi, S., Polini, B., Ebada, S. S., and Nieri, P. (2021). Non-Coding RNAs as Mediators of the Activity of Natural Compounds. Front. Pharmacol. 12, 751956. doi:10.3389/fphar.2021.751956
Shaito, A., Posadino, A. M., Younes, N., Hasan, H., Halabi, S., Alhababi, D., et al. (2020). Potential Adverse Effects of Resveratrol: A Literature Review. Ijms 21, 2084. doi:10.3390/ijms21062084
Shu, L., Hou, X., Song, G., Wang, C., and Ma, H. (2021). Comparative Analysis of Long Non-coding RNA E-xpression P-rofiles I-nduced by R-esveratrol and M-etformin T-reatment for H-epatic I-nsulin R-esistance. Int. J. Mol. Med. 48, 1–14. doi:10.3892/ijmm.2021.5039
Shu, L., Zhao, H., Huang, W., Hou, G., Song, G., and Ma, H. (2020). Resveratrol Upregulates Mmu-miR-363-3p via the PI3K-Akt Pathway to Improve Insulin Resistance Induced by a High-Fat Diet in Mice. Dmso Vol. 13, 391–403. doi:10.2147/dmso.s240956
Song, J., Jun, M., Ahn, M.-R., and Kim, O. Y. (2016). Involvement of miR-Let7A in Inflammatory Response and Cell Survival/apoptosis Regulated by Resveratrol in THP-1 Macrophage. Nutr. Res. Pract. 10, 377–384. doi:10.4162/nrp.2016.10.4.377
Sousa Fialho, M. d. L., Abd Jamil, A. H., Stannard, G. A., and Heather, L. C. (2019). Hypoxia-inducible Factor 1 Signalling, Metabolism and its Therapeutic Potential in Cardiovascular Disease. Biochimica Biophysica Acta (BBA) - Mol. Basis Dis. 1865, 831–843. doi:10.1016/j.bbadis.2018.09.024
Statello, L., Guo, C.-J., Chen, L.-L., and Huarte, M. (2021). Gene Regulation by Long Non-coding RNAs and its Biological Functions. Nat. Rev. Mol. Cell Biol. 22, 96–118. doi:10.1038/s41580-020-00315-9
Suk, K. T., and Kim, D. J. (2015). Staging of Liver Fibrosis or Cirrhosis: The Role of Hepatic Venous Pressure Gradient Measurement. Wjh 7, 607–615. doi:10.4254/wjh.v7.i3.607
Sun, Z. (2010). Cardiovascular Responses to Cold Exposure. Front. Biosci. E2, 495–503. doi:10.2741/e108
Tan, L., Wang, W., He, G., Kuick, R. D., Gossner, G., Kueck, A. S., et al. (2016). Resveratrol Inhibits Ovarian Tumor Growth in an In Vivo Mouse Model. Cancer 122, 722–729. doi:10.1002/cncr.29793
Tao, W., Sun, W., Zhu, H., and Zhang, J. (2019). miR-205-5p Suppresses Pulmonary Vascular Smooth Muscle Cell Proliferation by Targeting MICAL2-Mediated Erk1/2 Signaling. Microvasc. Res. 124, 43–50. doi:10.1016/j.mvr.2019.03.001
Tay, Y., Rinn, J., and Pandolfi, P. P. (2014). The Multilayered Complexity of ceRNA Crosstalk and Competition. Nature 505, 344–352. doi:10.1038/nature12986
Taylor, B. S., Schultz, N., Hieronymus, H., Gopalan, A., Xiao, Y., Carver, B. S., et al. (2010). Integrative Genomic Profiling of Human Prostate Cancer. Cancer Cell 18, 11–22. doi:10.1016/j.ccr.2010.05.026
Teertam, S. K., Jha, S., and Prakash Babu, P. (2020). Up-regulation of Sirt1/miR-149-5p Signaling May Play a Role in Resveratrol Induced Protection against Ischemia via P53 in Rat Brain. J. Clin. Neurosci. 72, 402–411. doi:10.1016/j.jocn.2019.11.043
Thenappan, T., Ormiston, M. L., Ryan, J. J., and Archer, S. L. (2018). Pulmonary Arterial Hypertension: Pathogenesis and Clinical Management. BMJ 360, j5492. doi:10.1136/bmj.j5492
Trapp, V., Parmakhtiar, B., Papazian, V., Willmott, L., and Fruehauf, J. P. (2010). Anti-angiogenic Effects of Resveratrol Mediated by Decreased VEGF and Increased TSP1 Expression in Melanoma-Endothelial Cell Co-culture. Angiogenesis 13, 305–315. doi:10.1007/s10456-010-9187-8
Vallino, L., Ferraresi, A., Vidoni, C., Secomandi, E., Esposito, A., Dhanasekaran, D. N., et al. (2020). Modulation of Non-coding RNAs by Resveratrol in Ovarian Cancer Cells: In Silico Analysis and Literature Review of the Anti-cancer Pathways Involved. J. traditional complementary Med. 10, 217–229. doi:10.1016/j.jtcme.2020.02.006
Varoni, E. M., Lo Faro, A. F., Sharifi-Rad, J., and Iriti, M. (2016). Anticancer Molecular Mechanisms of Resveratrol. Front. Nutr. 3, 8. doi:10.3389/fnut.2016.00008
Vaziri, H., Dessain, S. K., Eaton, E. N., Imai, S.-I., Frye, R. A., Pandita, T. K., et al. (2001). hSIR2SIRT1 Functions as an NAD-dependent P53 Deacetylase. Cell 107, 149–159. doi:10.1016/s0092-8674(01)00527-x
Venkatadri, R., Muni, T., Iyer, A. K. V., Yakisich, J. S., and Azad, N. (2016). Role of Apoptosis-Related miRNAs in Resveratrol-Induced Breast Cancer Cell Death. Cell Death Dis. 7, e2104. doi:10.1038/cddis.2016.6
Wang, B., Wang, Y., Xu, K., Zeng, Z., Xu, Z., Yue, D., et al. (2021). Resveratrol Alleviates Sepsis-Induced Acute Kidney Injury by Deactivating the lncRNA MALAT1/MiR-205 axis. cejoi 46, 295–304. doi:10.5114/ceji.2021.109195
Wang, C.-G., Lei, W., Li, C., Zeng, D.-X., and Huang, J.-A. (2015). Neuron-derived Orphan Receptor 1 Promoted Human Pulmonary Artery Smooth Muscle Cells Proliferation. Exp. Lung Res. 41, 208–215. doi:10.3109/01902148.2014.993776
Wang, M., Jiang, S., Yu, F., Zhou, L., and Wang, K. (2019). Noncoding RNAs as Molecular Targets of Resveratrol Underlying its Anticancer Effects. J. Agric. Food Chem. 67, 4709–4719. doi:10.1021/acs.jafc.9b01667
Wehbe, N., Nasser, S., Pintus, G., Badran, A., Eid, A., and Baydoun, E. (2019). MicroRNAs in Cardiac Hypertrophy. Ijms 20, 4714. doi:10.3390/ijms20194714
Weiss, M., Plass, C., and Gerhauser, C. (2014). Role of lncRNAs in Prostate Cancer Development and Progression. Biol. Chem. 395, 1275–1290. doi:10.1515/hsz-2014-0201
Welponer, H., Tsibulak, I., Wieser, V., Degasper, C., Shivalingaiah, G., Wenzel, S., et al. (2020). The miR-34 Family and its Clinical Significance in Ovarian Cancer. J. Cancer 11, 1446–1456. doi:10.7150/jca.33831
Willeit, P., Skroblin, P., Moschen, A. R., Yin, X., Kaudewitz, D., Zampetaki, A., et al. (2017). Circulating microRNA-122 Is Associated with the Risk of New-Onset Metabolic Syndrome and Type 2 Diabetes. Diabetes 66, 347–357. doi:10.2337/db16-0731
Wu, F., and Cui, L. (2017). Resveratrol Suppresses Melanoma by Inhibiting NF-κB/miR-221 and Inducing TFG Expression. Arch. Dermatol Res. 309, 823–831. doi:10.1007/s00403-017-1784-6
Xu, X. H., Ding, D. F., Yong, H. J., Dong, C. L., You, N., Ye, X. L., et al. (2017). Resveratrol Transcriptionally Regulates miRNA-18a-5p Expression Ameliorating Diabetic Nephropathy via Increasing Autophagy. Eur. Rev. Med. Pharmacol. Sci. 21, 4952–4965.
Yang, Q., Xu, E., Dai, J., Liu, B., Han, Z., Wu, J., et al. (2015). A Novel Long Noncoding RNA AK001796 Acts as an Oncogene and Is Involved in Cell Growth Inhibition by Resveratrol in Lung Cancer. Toxicol. Appl. Pharmacol. 285, 79–88. doi:10.1016/j.taap.2015.04.003
Yao, S., Gao, M., Wang, Z., Wang, W., Zhan, L., and Wei, B. (2021). Upregulation of MicroRNA-34a Sensitizes Ovarian Cancer Cells to Resveratrol by Targeting Bcl-2. Yonsei Med. J. 62, 691. doi:10.3349/ymj.2021.62.8.691
Yi, H., Zhang, W., Cui, S. Y., Fan, J. B., Zhu, X. H., and Liu, W. (2021). Identification and Validation of Key Long Non-coding RNAs in Resveratrol Protect against IL-1β-treated Chondrocytes via Integrated Bioinformatic Analysis. J. Orthop. Surg. Res. 16, 421–511. doi:10.1186/s13018-021-02574-4
Yin, K., Zhao, L., Feng, D., Ma, W., Liu, Y., Wang, Y., et al. (2015). Resveratrol Attenuated Low Ambient Temperature-Induced Myocardial Hypertrophy via Inhibiting Cardiomyocyte Apoptosis. Cell Physiol. Biochem. 35, 2451–2462. doi:10.1159/000374045
Yu, Y.-H., Chen, H.-A., Chen, P.-S., Cheng, Y.-J., Hsu, W.-H., Chang, Y.-W., et al. (2013). MiR-520h-mediated FOXC2 Regulation Is Critical for Inhibition of Lung Cancer Progression by Resveratrol. Oncogene 32, 431–443. doi:10.1038/onc.2012.74
Zhang, B., Tian, L., Xie, J., Chen, G., and Wang, F. (2020a). Targeting miRNAs by Natural Products: A New Way for Cancer Therapy. Biomed. Pharmacother. 130, 110546. doi:10.1016/j.biopha.2020.110546
Zhang, C., Han, B., Xu, T., and Li, D. (2020b). The Biological Function and Potential Mechanism of Long Non‐coding RNAs in Cardiovascular Disease. J. Cell. Mol. Med. 24, 12900–12909. doi:10.1111/jcmm.15968
Zhang, P., Wu, W., Chen, Q., and Chen, M. (2019a). Non-coding RNAs and Their Integrated Networks. J. Integr. Bioinform 16. doi:10.1515/jib-2019-0027
Zhang, W., Jiang, H., Chen, Y., and Ren, F. (2019b). Resveratrol Chemosensitizes Adriamycin‐resistant Breast Cancer Cells by Modulating miR‐122‐5p. J Cell. Biochem. 120, 16283–16292. doi:10.1002/jcb.28910
Zhang, X., Wang, W., Zhu, W., Dong, J., Cheng, Y., Yin, Z., et al. (2019c). Mechanisms and Functions of Long Non-coding RNAs at Multiple Regulatory Levels. Ijms 20, 5573. doi:10.3390/ijms20225573
Zhou, Y., Zhang, J., Li, W., Zhang, D., Wang, Z., Zhai, Y., et al. (2022). Integrative Investigation of the TF-miRNA Coregulatory Network Involved in the Inhibition of Breast Cancer Cell Proliferation by Resveratrol. FEBS Open Bio 12, 379–393. doi:10.1002/2211-5463.13344
Zhu, L., Mou, Q., Wang, Y., Zhu, Z., and Cheng, M. (2020a). Resveratrol Contributes to the Inhibition of Liver Fibrosis by Inducing Autophagy via the microRNA-20a-mediated A-ctivation of the PTEN/PI3K/AKT S-ignaling P-athway. Int. J. Mol. Med. 46, 2035–2046. doi:10.3892/ijmm.2020.4748
Zhu, L., Mou, Q., Wang, Y., Zhu, Z., and Cheng, M. (2020b). Resveratrol Contributes to the Inhibition of Liver Fibrosis by Inducing Autophagy via the microRNA-20a-mediated A-ctivation of the PTEN/PI3K/AKT S-ignaling P-athway. Int. J. Mol. Med. 46, 2035–2046. doi:10.3892/ijmm.2020.4748
Keywords: non-coding RNAs, ncRNAs, resveratrol, atherosclerosis, diabetes, obesity, cancer
Citation: Giordo R, Wehbe Z, Posadino AM, Erre GL, Eid AH, Mangoni AA and Pintus G (2022) Disease-Associated Regulation of Non-Coding RNAs by Resveratrol: Molecular Insights and Therapeutic Applications. Front. Cell Dev. Biol. 10:894305. doi: 10.3389/fcell.2022.894305
Received: 11 March 2022; Accepted: 21 June 2022;
Published: 13 July 2022.
Edited by:
Nejat Dalay, Istanbul University, TurkeyReviewed by:
Erika Cione, University of Calabria, ItalyKiesha Wilson, University of South Carolina, United States
Archana Saxena, University of South Carolina, United States
Copyright © 2022 Giordo, Wehbe, Posadino, Erre, Eid, Mangoni and Pintus. This is an open-access article distributed under the terms of the Creative Commons Attribution License (CC BY). The use, distribution or reproduction in other forums is permitted, provided the original author(s) and the copyright owner(s) are credited and that the original publication in this journal is cited, in accordance with accepted academic practice. No use, distribution or reproduction is permitted which does not comply with these terms.
*Correspondence: Arduino A. Mangoni, YXJkdWluby5tYW5nb25pQGZsaW5kZXJzLmVkdS5hdQ==; Gianfranco Pintus, Z3BpbnR1c0B1bmlzcy5pdA==