- 1Division of Nephrology, Department of Internal Medicine, Kaohsiung Chang Gung Memorial Hospital and Chang Gung University College of Medicine, Kaohsiung, Taiwan
- 2Medical Laboratory, Medical Education and Research Center, Kaohsiung Armed Forces General Hospital, Kaohsiung, Taiwan
Hypoxia-inducible factor-prolyl hydroxylase inhibitors (HIF-PHIs) emerge as promising agents to treat anemia in chronic kidney disease (CKD) but the major concern is their correlated risk of cancer development and progression. The Wilms’ tumor gene, WT1, is transcriptionally regulated by HIF and is known to play a crucial role in tumorigenesis and invasiveness of certain types of cancers. From the mechanism of action of HIF–PHIs, to cancer hypoxia and the biological significance of WT1, this review will discuss the link between HIF, WT1, anemia correction, and cancer. We aimed to reveal the research gaps and offer a focused strategy to monitor the development and progression of specific types of cancer when using HIF–PHIs to treat anemia in CKD patients. In addition, to facilitate the long-term use of HIF–PHIs in anemic CKD patients, we will discuss the strategy of WT1 inhibition to reduce the development and progression of cancer.
Introduction
Anemia is a common complication of chronic kidney disease (CKD) (Stauffer and Fan, 2014; Sofue et al., 2020). It causes reduced quality of life and increased morbidity and mortality in CKD patients (Coresh et al., 2007; Cases et al., 2018; Lee et al., 2018). Anemia in CKD is caused by many factors, including inadequate production of erythropoietin, functional iron deficiency, chronic inflammation, metabolic acidosis, hyperparathyroidism, dietary deficiency of folic acid and vitamin B12, and the side effects of concurrent medications (Babitt and Lin, 2012; Gluba-Brzozka et al., 2020). In spite of its complex pathogenesis, erythropoiesis-stimulating agents (ESA) have improved the quality of life of patients, reduced anemia-associated cardiovascular morbidity and the requirement for blood transfusion (Stone et al., 1988; Drueke et al., 2006; Finkelstein et al., 2009; Lewis et al., 2011). Despite the clinical success of current injected ESA, several large studies have established that supraphysiologic dosing of ESA is associated with increased risk of cardiovascular events, vascular access thrombosis, and overall mortality (Szczech et al., 2008; Solomon et al., 2010).
Cardiovascular complications and safety concerns from current injected ESA have led to the development of alternative strategies for the treatment of renal anemia. One of the most promising approaches is the development of hypoxia-inducible factor-prolyl hydroxylase inhibitors (HIF–PHIs), which offer a more consistent physiological level of erythropoietin (EPO) to stimulate red blood cell production. Despite the promising data from clinical trials of HIF–PHIs on anemia correction in CKD patients, the increased HIF raises the concern of the cancer risks. It is known that hundreds of genes induced by hypoxia in an HIF-dependent manner encode proteins that play key roles in many aspects of cancer biology including proliferation, cell survival, epithelial-to-mesenchymal transition (EMT), angiogenesis, invasion and metastasis (Talks et al., 2000). Notably, a substantial proportion of these genes are regulated by the Wilms’ tumor gene (WT1) (Zhang et al., 2005; Wagner et al., 2008; Meyer et al., 2019; Le et al., 2020).Besides, WT1 is critically regulated by HIF and plays a crucial role in tumorigenesis and metastasis. In this review, we will discuss the mechanisms of action, the outcome of the clinical trials, and the theoretical concerns regarding HIF-PHIs and malignancies. We will discuss HIF-PHIs and malignancies from the perspective of WT1.
HIF-PHIs as a Promising Treatment for Anemia in CKD
Mechanism of Action of HIF–PHIs
HIF, consisting of an oxygen-sensitive α-subunit and a constitutively expressed β-subunit, is a heterodimeric transcription factor responsible for activating the expression of EPO and genes involved in iron metabolism (Rankin et al., 2007; Kapitsinou et al., 2010; Kobayashi et al., 2016). HIF prolyl hydroxylase (HIF-PHD) enzymes affect the stability of the α subunit of HIF by promoting post-translational hydroxylation in an oxygen-dependent manner. HIF-PHIs temporarily inhibit PHD catalysis and contribute to a transient increase in HIF expression, regulating the function of many genes, including EPO, EPO receptor, proteins promoting iron absorption, iron transport, and heme synthesis (Bernhardt et al., 2010; Provenzano et al., 2016).
Clinical Outcomes of HIF-PHIs in CKD
HIF-PHIs stimulate erythropoiesis in a dose-dependent manner and have consistently shown clinical efficacy in patients with anemia of non-dialysis-dependent and dialysis-dependent CKD in phase II and III studies. The HIF-PHI roxadustat, orally administered three times a week for 8 weeks, effectively corrected hemoglobin levels in a small double-blinded, placebo-controlled phase III study in China (Chen et al., 2019), and in a two-arm, randomized, open-label study in Japan (Akizawa et al., 2020). Preliminary results were comparable to those of darbepoetin alfa in a 52-weeks, randomized, open-label study in Japan (Akizawa et al., 2021). Besides, HIF-PHI administration in CKD patients was associated with an increase in total iron binding capacity in most phase II and III studies (Pergola et al., 2016; Akizawa et al., 2019). A comprehensive review on the clinical trial data of well-investigated HIF-PHIs has recently been published (Haase, 2021). There are four compounds being licensed for marketing in Asia. An investigation on their long-term safety, including the occurrence and progression of cancer in extended trials, and a post-marketing analysis are yet to be performed.
Theoretical Concerns on HIF-PHIs and Malignancies
In addition to promoting erythropoiesis, the HIF pathway is essential for cellular survival under hypoxic conditions and regulates an array of biological processes, including cell growth and differentiation, angiogenesis, vascular tone, and metabolic processes (Semenza, 1999; Kewley et al., 2004; Wenger et al., 2005). The major concern is its effects on tumor growth and invasion as well as resistance to therapeutic agents. Activation of HIF-1α and HIF-2 has been shown to increase tumor survival in colorectal and breast cancers through different mechanisms (Kaidi et al., 2006; Choudhry et al., 2015). In addition, activation of HIF pathway has been reported to be associated with tumor aggressiveness, invasion, and metastasis through the c-Myc pathway in osteosarcomas (Yoo et al., 2011). It is also known to promote EMT in pancreatic cancer (Yang et al., 2016). In hematologic malignancies, overexpression of HIF-1α has been reported in acute myeloid leukemia (AML), acute lymphoblastic leukemia (ALL) and chronic myeloid leukemia (CML). HIF-2α overexpression has been demonstrated in both AML and ALL (Deeb et al., 2011; Frolova et al., 2012; Zhang et al., 2012; Forristal et al., 2015). Furthermore, roxadustat has been reported to increase the incidence of lung cancer in male mice and breast cancer in female mice compared with that in the control group (Beck et al., 2017). This evidence raises the theoretical concerns regarding HIF-PHIs and malignancies. Cancers currently known to be associated with the activated HIF pathway are summarized in Table 1.
The Link Between WT1 and Cancer
The WT1 gene, located at chromosome 11p13 (Call et al., 1990), encodes for 10 exons and generates a 3 kb mRNA. There are two major alternative splicing events. These include splicing of exon 5 (17 amino acids), and of a stretch of nine nucleotides (three amino acids, lysine, threoine, and serine (KTS)) in the 3’ end of exon 9. Alternative splicing of these two sites results in four different protein isoforms designated A, B, C and D, representing the presence or absence of exon 5 and KTS insert, respectively. Under normal physiological conditions, the expression of KTS(+)/KTS(-) ratio is maintained at approximately 2:1 (Haber et al., 1991). The N-terminal domain of WT1 is comprised of proline-glutamine-rich sequences and is critical for the transcriptional regulatory function of WT1. The C-terminal domain of WT1 is composed of four zinc fingers, which allow binding to target DNA sequences but are also involved in RNA and protein interactions. Through the C-terminal half of the protein, WT1 has been reported to be a potent transcriptional regulator targeting genes responsible for cellular growth and metabolism (Menke et al., 1998; Yang et al., 2007).
WT1 in concert with a variety of genes and proteins plays important roles in tumorigenesis and cancer metastasis. It is known to transcriptionally activate the proto-oncogene, c-Myc in human leukemic K562 cells and in several human breast cancer cell lines (Han et al., 2004). By protein-protein interactions, WT1 interacts with p53 and modulates their ability to transactivate their respective targets (Maheswaran et al., 1993). The clinical significance of the interaction between WT1 and p53 has been demonstrated in ovarian cancers (Carter et al., 2018). In addition, in solid tumors, WT1 activation has been shown in tumors originating from tissues that do not express WT1 in adults. The role of WT1 in controlling the balance between the mesenchymal and epithelial state of the cells might provide a critical link between WT1 and EMT, which is a key process for the metastasis of carcinomas. The two better studied major roles of WT1 in cancer development and metastasis are discussed below.
WT1 as an Oncogene
WT1 was originally discovered as a tumor suppressor because of its loss-of-function mutations in a subset of pediatric renal neoplasms, known as nephroblastomas or Wilms’ tumors (Huff et al., 1991). It is well known that WT1 is fundamental to mammalian organ development, including blood vessels, heart, spleen, liver and genitourinary system (Kreidberg et al., 1993; Herzer et al., 1999; Moore et al., 1999; Ijpenberg et al., 2007). On the other hand, although WT1 behaves as a tumor suppressor gene in Wilms’ tumors, increasing data suggest a role for WT1 as an oncogene in both leukemia and solid tumors (Osaka et al., 1997; Sera et al., 2008; Desmedt et al., 2009; Brett et al., 2013). These accumulating data has been summarized in comprehensive reviews (Yang et al., 2007; Huff, 2011; Chau and Hastie, 2012).
Compared with normal human tissues, WT1 is expressed at a rather high level in various malignancies including ovarian (Hylander et al., 2006; Yamamoto et al., 2007; Andersson et al., 2014), breast (Loeb et al., 2001; McGregor et al., 2018), uterine (Coosemans et al., 2011; Guntupalli et al., 2013), lung (Oji et al., 2002; Hayashi et al., 2012), colon (Koesters et al., 2004; Bejrananda et al., 2010) cancers and malignant pleural mesothelioma (Cedres et al., 2014). In breast cancer, WT1 upregulates the expression of human epidermal growth factor receptor 2 (HER2), leading to estrogen-independent tumor growth and anti-estrogen resistance. Silencing of WT1 inhibits the growth of MCF-7 cell line (Navakanit et al., 2007; Nasomyon et al., 2014). HER2 has been shown to upregulate WT1 expression through the AKT signaling pathway, promoting breast cancer cell proliferation and inhibiting cellular apoptosis (Tuna et al., 2005). In lung cancer, there is a positive feedback loop between WT1 and AKT-1. Cisplatin treatment downregulates the WT1 expression through the PI3K/AKT signaling pathway (Wang et al., 2013).
Although the molecular mechanisms that account for the increased expression of WT1 in these cancers are not fully characterized, it has been reported that the proximal WT1 promoter contains a hypoxia-responsive element (HRE), which is a binding site of HIF-1 (Wagner et al., 2003). Considering the relatively low oxygen tensions in rapidly growing tumors (Hockel and Vaupel, 2001), it would be reasonable to speculate that intratumoral hypoxia could lead to enhanced HIF expression, which transcriptionally activates WT1 in these cancers. Supporting this perspective, the upregulation of both HIF1α and WT1 has been reported in patients with myelodysplastic syndrome (MDS) or acute leukemia (Rosenfeld et al., 2003; Mpakou et al., 2021).
WT1 Regulates the EMT
Despite mounting evidence demonstrating high levels of WT1 expression in leukemia and solid tumors as described above, the exact functional implications of increased WT1 expression in tumorigenesis are not fully understood. Nevertheless, WT1 has been shown to regulate cell proliferation, apoptosis, and blood vessels formation (Hartkamp and Roberts, 2008; Scholz et al., 2009), which all are well known biological processes leading to tumorigenesis when go awry. Furthermore, WT1 is known to control the cell transition between the mesenchymal and epithelial states by transcriptionally regulating major EMT mediators Snail (Snai1) and E-cadherin (Cadh1) during embryonic development (Martinez-Estrada et al., 2010). Uncontrolled EMT is a hallmark of various pathologies, including cancer, while disruption of mesenchymal-to-epithelial transition has been associated with a number of developmental abnormalities (Davies et al., 2004; Wessels and Perez-Pomares, 2004; Hohenstein and Hastie, 2006). Gain-of-function and loss-of-function approaches have been used to investigate the role of WT1 and its effect on EMT marker expression and cancer cell migrations. Silencing of WT1 has been demonstrated to reduce proliferation, chemotaxis and invasiveness of human malignant mesothelioma cell lines (Plones et al., 2017). In cultured ovarian cancer cells and xenograft mouse models, WT1 depletion significantly reversed EMT, inhibited cell migration and invasion, and prevented metastasis of cancer cells (Han et al., 2020).
An important observation is that EMT tends to occur in a hypoxic microenvironment. Exposure of breast cancer cells to a low-oxygen microenvironment facilitates cell migration by inducing the upregulation of vimentin and downregulation of epithelial marker proteins (Lester et al., 2007). This evidence collectively suggests the crucial roles of HIFs and WT1 in modulating EMT in cancer hypoxia. The hypothetical roles of WT1 in the development and progression of cancers with the activated HIF pathway are depicted in Figure 1. Although the pathogenesis of each cancer in Figure 1 could be more complex than depicted, this figure aims to highlight the link between HIFs and WT1 in these cancers.
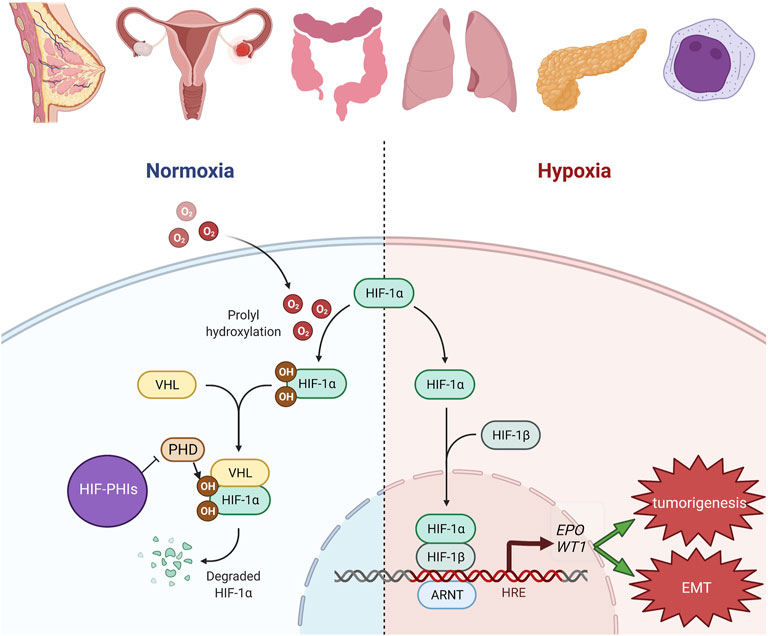
FIGURE 1. Hypothetic roles of WT1 in the development and progression of cancers with HIF pathway activation. In breast, uterine, ovarian, colon, lung, pleural, pancreatic and hematologic malignancies, HIF pathway is known to be activated especially in the hypoxic milieu. HIF-PHIs inhibit PHD catalysis and stabilize more HIF. The increased HIF resulted from either condition will bind to HRE and then transcriptionally activate its downstream genes, including EPO and WT1. Upregulation of WT1 will contribute to tumorigenesis and EMT. The figure was created with BioRender.com.
WT1 and Anemia
The exact mechanism by which WT1 benefits anemia remains largely unknown. It has been demonstrated that Wt1 is required for the differentiation of the red blood cells. In conditional Wt1 knockout mice, diminished extramedullary hematopoiesis within the red pulp compartment of the spleen was found. In addition, the Wt1-mutant bone marrow cells failed to differentiate into the erythrocyte lineage (Chau et al., 2011). In genetically manipulated cultured cell models, WT1 was shown to be the transcriptional activator of the EPO gene (Dame et al., 2006). Recently, by using WT1 conditional knockout mice, Ji et al. demonstrated that WT1 recruits Tet2 to the promoter of EPO, which results in enhanced 5-hydroxymethylcytosine levels and the promotion of EPO expression (Ji et al., 2021). These important findings shed light on the potential beneficial role of WT1 in anemia.
Strategies to Target WT1 to Avoid Cancer Development While Using HIF-PHIs to Treat Anemia
Currently available data from clinical trials on HIF-PHIs do not show cancer occurrence. In the phase II study of vadadustat in CKD patients, there were no reports of cancer (Pergola et al., 2016). In a study of 252 patients with non-dialysis CKD and 216 patients under dialysis treated with daprodustat, no malignancies were observed during the study (Holdstock et al., 2019). Recent data of large clinical trials on roxadustat have not shown the development of cancer (Chen et al., 2019). However, all these clinical studies were performed for less than 26 weeks. Long-term observations in humans will be required to examine the cancer-related risks of HIF-PHIs. Therefore, the first step of current strategies for using HIF-PHIs is carefully monitoring the occurrence of HIF-related cancers. As listed in Table 1, attention needs to be paid to the development of breast, lung, colorectal, pancreatic and hematologic malignancies. In addition, as WT1 is one of the HIF downstream oncogene targets, it will be mandatory to monitor the development and progression of WT1-mediated cancers including ovarian, breast, lung, uterine, colon cancers, pleural mesothelioma and hematologic malignancies. In addition to monitoring, more research into WT1 inhibition in these cancers is required. WT1 peptide vaccine is known to induce clinical responses in MDS, AML, CML, ALL, multiple myeloma and various types of solid tumors including lung and breast cancers (Oka et al., 2004; Oka et al., 2017). Further investigations on the efficacy and safety of the WT1 peptide vaccine in other WT1-related cancers are required. Besides, vorinostat and bortezomib have been reported to significantly inhibit WT1 gene expression in MO7-e and P39 cell lines, which are in vitro models for leukemia and MDS, respectively (Galimberti et al., 2008). In addition, curcumin is reported to decrease WT1 expression in patients’ leukemic cells (Anuchapreeda et al., 2006). Recently, the deubiquitinase inhibitor degrasyn was reported to promptly induce the degradation of endogenous and exogenous WT1 in pancreatic ductal adenocarcinoma (Li et al., 2020). However, the therapeutic potential and the underlying mechanisms of these agents are yet to be investigated in ovarian, breast, lung, uterine, pancreatic, colon cancers, pleural mesothelioma and hematologic malignancies. More investigations are also required to examine if WT1 inhibition reduces the survival effects of HIF-PHIs in these cancers.
Conclusion and Perspectives
HIF-PHIs activate HIF transcription factors, leading to an increase in endogenous EPO production and modulation of iron metabolism. Data on clinical trials has demonstrated their efficacy and short-term safety. HIF-PHIs have the potential to revolutionize the treatment of anemia in CKD but careful monitoring of the development or progression of cancer is required. Despite the persuasive links between hypoxia, HIF pathways, EMT and high levels of WT1 expression being observed in solid tumors, it is still yet to be answered in full whether WT1 is necessary or its overexpression alone is sufficient to drive tumorigenesis in human. In the coming era of using HIF-PHIs in renal anemia, a better understanding of the link between HIF and WT1 will help focus on the specific types of cancers to be monitored. In addition, more research on WT1 inhibition in ovarian, breast, lung, uterine, pancreatic, colon cancers, and pleural mesothelioma will contribute to the treatment of HIF-PHI-induced WT1-mediated cancers.
Author Contributions
WL and CC conceptualized and wrote the manuscript. TC and YC reviewed and edited the manuscript before submission. WL, CC and TC analysed the literature. WL prepared the figure. All authors read and approved the final version of the manuscript.
Funding
This research was funded by Kaohsiung Chang Gung Memorial Hospital, grant number CORPG8L0351.
Conflict of Interest
The authors declare that the research was conducted in the absence of any commercial or financial relationships that could be construed as a potential conflict of interest.
Publisher’s Note
All claims expressed in this article are solely those of the authors and do not necessarily represent those of their affiliated organizations, or those of the publisher, the editors and the reviewers. Any product that may be evaluated in this article, or claim that may be made by its manufacturer, is not guaranteed or endorsed by the publisher.
References
Akizawa, T., Iwasaki, M., Otsuka, T., Yamaguchi, Y., and Reusch, M. (2021). Phase 3 Study of Roxadustat to Treat Anemia in Non-dialysis-dependant CKD. Kidney Int. Rep. 6 (7), 1810–1828. doi:10.1016/j.ekir.2021.04.003
Akizawa, T., Macdougall, I. C., Berns, J. S., Yamamoto, H., Taguchi, M., Iekushi, K., et al. (2019). Iron Regulation by Molidustat, a Daily Oral Hypoxia-Inducible Factor Prolyl Hydroxylase Inhibitor, in Patients with Chronic Kidney Disease. Nephron 143 (4), 243–254. doi:10.1159/000502012
Akizawa, T., Yamaguchi, Y., Otsuka, T., and Reusch, M. (2020). A Phase 3, Multicenter, Randomized, Two-Arm, Open-Label Study of Intermittent Oral Dosing of Roxadustat for the Treatment of Anemia in Japanese Erythropoiesis-Stimulating Agent-Naïve Chronic Kidney Disease Patients Not on Dialysis. Nephron 144 (8), 372–382. doi:10.1159/000508100
Andersson, C., Oji, Y., Ohlson, N., Wang, S., Li, X., Ottander, U., et al. (2014). Prognostic Significance of Specific Anti‐ WT 1 IgG Antibody Level in Plasma in Patients with Ovarian Carcinoma. Cancer Med. 3 (4), 909–918. doi:10.1002/cam4.244
Anuchapreeda, S., Limtrakul, P., Thanarattanakorn, P., Sittipreechacharn, S., and Chanarat, P. (2006). Inhibitory Effect of Curcumin onWT1 Gene Expression in Patient Leukemic Cells. Arch. Pharm. Res. 29 (1), 80–87. doi:10.1007/BF02977473
Babitt, J. L., and Lin, H. Y. (2012). Mechanisms of Anemia in CKD. Jasn 23 (10), 1631–1634. doi:10.1681/ASN.2011111078
Beck, J., Henschel, C., Chou, J., Lin, A., and Del Balzo, U. (2017). Evaluation of the Carcinogenic Potential of Roxadustat (FG-4592), a Small Molecule Inhibitor of Hypoxia-Inducible Factor Prolyl Hydroxylase in CD-1 Mice and Sprague Dawley Rats. Int. J. Toxicol. 36 (6), 427–439. doi:10.1177/1091581817737232
Bejrananda, T., Phukaoloun, M., Boonpipattanapong, T., Wanitsuwan, W., Kanngern, S., Sangthong, R., et al. (2011). WT1 Expression as an Independent Marker of Poor Prognosis in Colorectal Cancers. Cbm 8 (1), 35–42. doi:10.3233/DMA-2011-0822
Bernhardt, W. M., Wiesener, M. S., Scigalla, P., Chou, J., Schmieder, R. E., Günzler, V., et al. (2010). Inhibition of Prolyl Hydroxylases Increases Erythropoietin Production in ESRD. Jasn 21 (12), 2151–2156. doi:10.1681/ASN.2010010116
Brett, A., Pandey, S., and Fraizer, G. (2013). The Wilms' Tumor Gene (WT1) Regulates E-Cadherin Expression and Migration of Prostate Cancer Cells. Mol. Cancer 12, 3. doi:10.1186/1476-4598-12-3
Call, K. M., Glaser, T., Ito, C. Y., Buckler, A. J., Pelletier, J., Haber, D. A., et al. (1990). Isolation and Characterization of a Zinc finger Polypeptide Gene at the Human Chromosome 11 Wilms' Tumor Locus. Cell 60 (3), 509–520. doi:10.1016/0092-8674(90)90601-a
Carter, J. H., Deddens, J. A., Mueller, G., Lewis, T. G., Dooley, M. K., Robillard, M. C., et al. (2018). Transcription Factors WT1 and P53 Combined: a Prognostic Biomarker in Ovarian Cancer. Br. J. Cancer 119 (4), 462–470. doi:10.1038/s41416-018-0191-x
Cases, A., Egocheaga, M. I., Tranche, S., Pallarés, V., Ojeda, R., Górriz, J. L., et al. (2018). Anemia en la enfermedad renal crónica: protocolo de estudio, manejo y derivación a Nefrología. Atención Primaria 50, 60–64. doi:10.1016/j.aprim.2017.09.007
Cedrés, S., Montero, M. A., Zamora, E., Martínez, A., Martínez, P., Fariñas, L., et al. (2014). Expression of Wilms' Tumor Gene (WT1) Is Associated with Survival in Malignant Pleural Mesothelioma. Clin. Transl Oncol. 16 (9), 776–782. doi:10.1007/s12094-013-1146-6
Chau, Y.-Y., Brownstein, D., Mjoseng, H., Lee, W.-C., Buza-Vidas, N., Nerlov, C., et al. (2011). Acute Multiple Organ Failure in Adult Mice Deleted for the Developmental Regulator Wt1. Plos Genet. 7 (12), e1002404. doi:10.1371/journal.pgen.1002404
Chau, Y.-Y., and Hastie, N. D. (2012). The Role of Wt1 in Regulating Mesenchyme in Cancer, Development, and Tissue Homeostasis. Trends Genet. 28 (10), 515–524. doi:10.1016/j.tig.2012.04.004
Chen, N., Hao, C., Peng, X., Lin, H., Yin, A., Hao, L., et al. (2019). Roxadustat for Anemia in Patients with Kidney Disease Not Receiving Dialysis. N. Engl. J. Med. 381 (11), 1001–1010. doi:10.1056/NEJMoa1813599
Choudhry, H., Albukhari, A., Morotti, M., Haider, S., Moralli, D., Smythies, J., et al. (2015). Erratum: Tumor Hypoxia Induces Nuclear Paraspeckle Formation through HIF-2α Dependent Transcriptional Activation of NEAT1 Leading to Cancer Cell Survival. Oncogene 34 (34), 4546. doi:10.1038/onc.2014.431
Coosemans, A., Van Calster, B., Verbist, G., Moerman, P., Vergote, I., Van Gool, S. W., et al. (2011). Wilms Tumor Gene 1(WT1) Is a Prognostic Marker in High-Grade Uterine Sarcoma. Int. J. Gynecol. Cancer 21 (2), 302–308. doi:10.1097/IGC.0b013e318207cab5
Coresh, J., Selvin, E., Stevens, L. A., Manzi, J., Kusek, J. W., Eggers, P., et al. (2007). Prevalence of Chronic Kidney Disease in the United States. JAMA 298 (17), 2038–2047. doi:10.1001/jama.298.17.2038
Dame, C., Kirschner, K. M., Bartz, K. V., Wallach, T., Hussels, C. S., and Scholz, H. (2006). Wilms Tumor Suppressor, Wt1, Is a Transcriptional Activator of the Erythropoietin Gene. Blood 107 (11), 4282–4290. doi:10.1182/blood-2005-07-2889
Davies, J. A., Ladomery, M., Hohenstein, P., Michael, L., Shafe, A., Spraggon, L., et al. (2004). Development of an siRNA-Based Method for Repressing Specific Genes in Renal Organ Culture and its Use to Show that the Wt1 Tumour Suppressor Is Required for Nephron Differentiation. Hum. Mol. Genet. 13 (2), 235–246. doi:10.1093/hmg/ddh015
Deeb, G., Vaughan, M. M., McInnis, I., Ford, L. A., Sait, S. N. J., Starostik, P., et al. (2011). Hypoxia-inducible Factor-1α Protein Expression Is Associated with Poor Survival in normal Karyotype Adult Acute Myeloid Leukemia. Leuk. Res. 35 (5), 579–584. doi:10.1016/j.leukres.2010.10.020
Desmedt, C., Sotiriou, C., and Piccart-Gebhart, M. J. (2009). Development and Validation of Gene Expression Profile Signatures in Early-Stage Breast Cancer. Cancer Invest. 27 (1), 1–10. doi:10.1080/07357900802574710
Drüeke, T. B., Locatelli, F., Clyne, N., Eckardt, K.-U., Macdougall, I. C., Tsakiris, D., et al. (2006). Normalization of Hemoglobin Level in Patients with Chronic Kidney Disease and Anemia. N. Engl. J. Med. 355 (20), 2071–2084. doi:10.1056/NEJMoa062276
Finkelstein, F. O., Story, K., Firanek, C., Mendelssohn, D., Barre, P., Takano, T., et al. (2009). Health-related Quality of Life and Hemoglobin Levels in Chronic Kidney Disease Patients. Cjasn 4 (1), 33–38. doi:10.2215/CJN.00630208
Forristal, C. E., Brown, A. L., Helwani, F. M., Winkler, I. G., Nowlan, B., Barbier, V., et al. (2015). Hypoxia Inducible Factor (HIF)-2α Accelerates Disease Progression in Mouse Models of Leukemia and Lymphoma but Is Not a Poor Prognosis Factor in Human AML. Leukemia 29 (10), 2075–2085. doi:10.1038/leu.2015.102
Frolova, O., Samudio, I., Benito, J. M., Jacamo, R., Kornblau, S. M., Markovic, A., et al. (2012). Regulation of HIF-1α Signaling and Chemoresistance in Acute Lymphocytic Leukemia under Hypoxic Conditions of the Bone Marrow Microenvironment. Cancer Biol. Ther. 13 (10), 858–870. doi:10.4161/cbt.20838
Galimberti, S., Canestraro, M., Khan, R., Buda, G., Orciuolo, E., Guerrini, F., et al. (2008). Vorinostat and Bortezomib Significantly Inhibit WT1 Gene Expression in MO7-e and P39 Cell Lines. Leukemia 22 (3), 628–631. doi:10.1038/sj.leu.2404918
` Gluba-Brzózka, A., Franczyk, B., Olszewski, R., and Rysz, J. (2020). The Influence of Inflammation on Anemia in CKD Patients. Ijms 21 (3), 725. doi:10.3390/ijms21030725
Guntupalli, S. R., Cao, D., Shroff, R., Gao, F., Menias, C., Stewart Massad, L., et al. (2013). Wilms' Tumor 1 Protein and Estrogen Receptor Beta Expression Are Associated with Poor Outcomes in Uterine Carcinosarcoma. Ann. Surg. Oncol. 20 (7), 2373–2379. doi:10.1245/s10434-012-2838-9
Haase, V. H. (2021). Hypoxia-inducible Factor-Prolyl Hydroxylase Inhibitors in the Treatment of Anemia of Chronic Kidney Disease. Kidney Int. Supplements 11 (1), 8–25. doi:10.1016/j.kisu.2020.12.002
Haber, D. A., Sohn, R. L., Buckler, A. J., Pelletier, J., Call, K. M., and Housman, D. E. (1991). Alternative Splicing and Genomic Structure of the Wilms Tumor Gene WT1. Proc. Natl. Acad. Sci. U.S.A. 88 (21), 9618–9622. doi:10.1073/pnas.88.21.9618
Han, Y., San-Marina, S., Liu, J., and Minden, M. D. (2004). Transcriptional Activation of C-Myc Proto-Oncogene by WT1 Protein. Oncogene 23 (41), 6933–6941. doi:10.1038/sj.onc.1207609
Han, Y., Song, C., Zhang, T., Zhou, Q., Zhang, X., Wang, J., et al. (2020). Wilms' Tumor 1 (WT1) Promotes Ovarian Cancer Progression by Regulating E-Cadherin and ERK1/2 Signaling. Cell Cycle 19 (20), 2662–2675. doi:10.1080/15384101.2020.1817666
Hartkamp, J., and Roberts, S. G. E. (2008). The Role of the Wilms' Tumour-Suppressor Protein WT1 in Apoptosis. Biochem. Soc. Trans. 36 (Pt 4), 629–631. doi:10.1042/BST0360629
Hayashi, S., Oji, Y., Kanai, Y., Teramoto, T., Kitaichi, M., Kawaguchi, T., et al. (2012). Low Wilms' Tumor Gene Expression in Tumor Tissues Predicts Poor Prognosis in Patients with Non-small-cell Lung Cancer. Cancer Invest. 30 (2), 165–171. doi:10.3109/07357907.2011.633291
Herzer, U., Crocoll, A., Barton, D., Howells, N., and Englert, C. (1999). The Wilms Tumor Suppressor Gene Wt1 Is Required for Development of the Spleen. Curr. Biol. 9 (15), 837–S1. doi:10.1016/s0960-9822(99)80369-8
Hockel, M., and Vaupel, P. (2001). Tumor Hypoxia: Definitions and Current Clinical, Biologic, and Molecular Aspects. JNCI J. Natl. Cancer Inst. 93 (4), 266–276. doi:10.1093/jnci/93.4.266
Hohenstein, P., and Hastie, N. D. (2006). The many Facets of the Wilms' Tumour Gene, WT1. Hum. Mol. Genet. 15, R196–R201. doi:10.1093/hmg/ddl196
Holdstock, L., Cizman, B., Meadowcroft, A. M., Biswas, N., Johnson, B. M., Jones, D., et al. (2019). Daprodustat for Anemia: a 24-week, Open-Label, Randomized Controlled Trial in Participants with Chronic Kidney Disease. Clin. Kidney J. 12 (1), 129–138. doi:10.1093/ckj/sfy013
Huff, V., Miwa, H., Haber, D. A., Call, K. M., Housman, D., Strong, L. C., et al. (1991). Evidence for WT1 as a Wilms Tumor (WT) Gene: Intragenic Germinal Deletion in Bilateral WT. Am. J. Hum. Genet. 48 (5), 997–1003.
Huff, V. (2011). Wilms' Tumours: about Tumour Suppressor Genes, an Oncogene and a Chameleon Gene. Nat. Rev. Cancer 11 (2), 111–121. doi:10.1038/nrc3002
Hylander, B., Repasky, E., Shrikant, P., Intengan, M., Beck, A., Driscoll, D., et al. (2006). Expression of Wilms Tumor Gene (WT1) in Epithelial Ovarian Cancer. Gynecol. Oncol. 101 (1), 12–17. doi:10.1016/j.ygyno.2005.09.052
Ijpenberg, A., Pérez-Pomares, J. M., Guadix, J. A., Carmona, R., Portillo-Sánchez, V., Macías, D., et al. (2007). Wt1 and Retinoic Acid Signaling Are Essential for Stellate Cell Development and Liver Morphogenesis. Dev. Biol. 312 (1), 157–170. doi:10.1016/j.ydbio.2007.09.014
Ji, F., Wang, W., Feng, C., Gao, F., and Jiao, J. (2021). Brain-specific Wt1 Deletion Leads to Depressive-like Behaviors in Mice via the Recruitment of Tet2 to Modulate Epo Expression. Mol. Psychiatry 26 (8), 4221–4233. doi:10.1038/s41380-020-0759-8
Kaidi, A., Qualtrough, D., Williams, A. C., and Paraskeva, C. (2006). Direct Transcriptional Up-Regulation of Cyclooxygenase-2 by Hypoxia-Inducible Factor (HIF)-1 Promotes Colorectal Tumor Cell Survival and Enhances HIF-1 Transcriptional Activity during Hypoxia. Cancer Res. 66 (13), 6683–6691. doi:10.1158/0008-5472.CAN-06-0425
Kapitsinou, P. P., Liu, Q., Unger, T. L., Rha, J., Davidoff, O., Keith, B., et al. (2010). Hepatic HIF-2 Regulates Erythropoietic Responses to Hypoxia in Renal Anemia. Blood 116 (16), 3039–3048. doi:10.1182/blood-2010-02-270322
Kewley, R. J., Whitelaw, M. L., and Chapman-Smith, A. (2004). The Mammalian Basic helix-loop-helix/PAS Family of Transcriptional Regulators. Int. J. Biochem. Cel Biol. 36 (2), 189–204. doi:10.1016/s1357-2725(03)00211-5
Kobayashi, H., Liu, Q., Binns, T. C., Urrutia, A. A., Davidoff, O., Kapitsinou, P. P., et al. (2016). Distinct Subpopulations of FOXD1 Stroma-Derived Cells Regulate Renal Erythropoietin. J. Clin. Invest. 126 (5), 1926–1938. doi:10.1172/JCI83551
Koesters, R., Linnebacher, M., Coy, J. F., Germann, A., Schwitalle, Y., Findeisen, P., et al. (2004). WT1 Is a Tumor-Associated Antigen in colon Cancer that Can Be Recognized Byin Vitro Stimulated Cytotoxic T Cells. Int. J. Cancer 109 (3), 385–392. doi:10.1002/ijc.11721
Kreidberg, J. A., Sariola, H., Loring, J. M., Maeda, M., Pelletier, J., Housman, D., et al. (1993). WT-1 Is Required for Early Kidney Development. Cell 74 (4), 679–691. doi:10.1016/0092-8674(93)90515-r
Le, F., Luo, P., Ouyang, Q., and Zhong, X. (2020). LncRNA WT1-AS Downregulates Survivin by Upregulating miR-203 in Papillary Thyroid Carcinoma. Cmar 12, 443–449. doi:10.2147/CMAR.S232294
Lee, W.-C., Lee, Y.-T., Li, L.-C., Ng, H.-Y., Kuo, W.-H., Lin, P.-T., et al. (2018). The Number of Comorbidities Predicts Renal Outcomes in Patients with Stage 3-5 Chronic Kidney Disease. Jcm 7 (12), 493. doi:10.3390/jcm7120493
Lewis, E. F., Pfeffer, M. A., Feng, A., Uno, H., McMurray, J. J. V., Toto, R., et al. (2011). Darbepoetin Alfa Impact on Health Status in Diabetes Patients with Kidney Disease: a Randomized Trial. Cjasn 6 (4), 845–855. doi:10.2215/CJN.06450710
Li, J., Li, H., Zhu, W., Zhou, B., Ying, J., Wu, J., et al. (2020). Deubiquitinase Inhibitor Degrasyn Suppresses Metastasis by Targeting USP5‐WT1‐E‐cadherin Signalling Pathway in Pancreatic Ductal Adenocarcinoma. J. Cel Mol Med 24 (2), 1370–1382. doi:10.1111/jcmm.14813
Loeb, D. M., Evron, E., Patel, C. B., Sharma, P. M., Niranjan, B., Buluwela, L., et al. (2001). Wilms' Tumor Suppressor Gene (WT1) Is Expressed in Primary Breast Tumors Despite Tumor-specific Promoter Methylation. Cancer Res. 61 (3), 921–925.
Maheswaran, S., Park, S., Bernard, A., Morris, J. F., Rauscher, F. J., Hill, D. E., et al. (1993). Physical and Functional Interaction between WT1 and P53 Proteins. Proc. Natl. Acad. Sci. U.S.A. 90 (11), 5100–5104. doi:10.1073/pnas.90.11.5100
Martínez-Estrada, O. M., Lettice, L. A., Essafi, A., Guadix, J. A., Slight, J., Velecela, V., et al. (2010). Wt1 Is Required for Cardiovascular Progenitor Cell Formation through Transcriptional Control of Snail and E-Cadherin. Nat. Genet. 42 (1), 89–93. doi:10.1038/ng.494
McGregor, R. J., Chau, Y.-Y., Kendall, T. J., Artibani, M., Hastie, N., and Hadoke, P. W. F. (2018). WT1 Expression in Vessels Varies with Histopathological Grade in Tumour-Bearing and Control Tissue from Patients with Breast Cancer. Br. J. Cancer 119 (12), 1508–1517. doi:10.1038/s41416-018-0317-1
Menke, A. L., van der Eb, A. J., and Jochemsen, A. G. (1998). The Wilms' Tumor 1 Gene: Oncogene or Tumor Suppressor Gene? Int. Rev. Cytol. 181, 151–212. doi:10.1016/s0074-7696(08)60418-0
Meyer, S. N., Galván, J. A., Zahnd, S., Sokol, L., Dawson, H., Lugli, A., et al. (2019). Co-expression of Cytokeratin and Vimentin in Colorectal Cancer Highlights a Subset of Tumor Buds and an Atypical Cancer-Associated Stroma. Hum. Pathol. 87, 18–27. doi:10.1016/j.humpath.2019.02.002
Moore, A. W., McInnes, L., Kreidberg, J., Hastie, N. D., and Schedl, A. (1999). YAC Complementation Shows a Requirement for Wt1 in the Development of Epicardium, Adrenal Gland and throughout Nephrogenesis. Development 126 (9), 1845–1857. doi:10.1242/dev.126.9.1845
Mpakou, V., Spathis, A., Bouchla, A., Tsakiraki, Z., Kontsioti, F., Papageorgiou, S., et al. (2021). Upregulated Hypoxia Inducible Factor 1α Signaling Pathway in High Risk Myelodysplastic Syndrome and Acute Myeloid Leukemia Patients Is Associated with Better Response to 5‐azacytidine-Data from the Hellenic Myelodysplastic Syndrome Study Group. Hematological Oncol. 39 (2), 231–242. doi:10.1002/hon.2834
Nasomyon, T., Samphao, S., Sangkhathat, S., Mahattanobon, S., and Graidist, P. (2014). Correlation of Wilms' Tumor 1 Isoforms with HER2 and ER-α and its Oncogenic Role in Breast Cancer. Anticancer Res. 34 (3), 1333–1342.
Navakanit, R., Graidist, P., Leeanansaksiri, W., and Dechsukum, C. (2007). Growth Inhibition of Breast Cancer Cell Line MCF-7 by siRNA Silencing of Wilms Tumor 1 Gene. J. Med. Assoc. Thai 90 (11), 2416–2421.
Oji, Y., Miyoshi, S., Maeda, H., Hayashi, S., Tamaki, H., Nakatsuka, S.-I., et al. (2002). Overexpression of the Wilms' Tumor geneWT1 Inde Novo Lung Cancers. Int. J. Cancer 100 (3), 297–303. doi:10.1002/ijc.10476
Oka, Y., Tsuboi, A., Nakata, J., Nishida, S., Hosen, N., Kumanogoh, A., et al. (2017). Wilms' Tumor Gene 1 (WT1) Peptide Vaccine Therapy for Hematological Malignancies: From CTL Epitope Identification to Recent Progress in Clinical Studies Including a Cure-Oriented Strategy. Oncol. Res. Treat. 40 (11), 682–690. doi:10.1159/000481353
Oka, Y., Tsuboi, A., Taguchi, T., Osaki, T., Kyo, T., Nakajima, H., et al. (2004). Induction of WT1 (Wilms' Tumor Gene)-specific Cytotoxic T Lymphocytes by WT1 Peptide Vaccine and the Resultant Cancer Regression. Proc. Natl. Acad. Sci. U.S.A. 101 (38), 13885–13890. doi:10.1073/pnas.0405884101
Osaka, M., Koami, K., and Sugiyama, T. (1997). WT1 Contributes to Leukemogenesis: Expression Patterns in 7,12-dimethylbenz[a]anthracene (DMBA)-induced Leukemia. Int. J. Cancer 72 (4), 696–699. doi:10.1002/(sici)1097-0215(19970807)72:4<696::aid-ijc23>3.0.co;2-8
Pergola, P. E., Spinowitz, B. S., Hartman, C. S., Maroni, B. J., and Haase, V. H. (2016). Vadadustat, a Novel Oral HIF Stabilizer, Provides Effective Anemia Treatment in Nondialysis-dependent Chronic Kidney Disease. Kidney Int. 90 (5), 1115–1122. doi:10.1016/j.kint.2016.07.019
Plönes, T., Fischer, M., Höhne, K., Sato, H., Müller-Quernheim, J., and Zissel, G. (2017). Turning Back the Wheel: Inducing Mesenchymal to Epithelial Transition via Wilms Tumor 1 Knockdown in Human Mesothelioma Cell Lines to Influence Proliferation, Invasiveness, and Chemotaxis. Pathol. Oncol. Res. 23 (4), 723–730. doi:10.1007/s12253-016-0181-3
Provenzano, R., Besarab, A., Wright, S., Dua, S., Zeig, S., Nguyen, P., et al. (2016). Roxadustat (FG-4592) versus Epoetin Alfa for Anemia in Patients Receiving Maintenance Hemodialysis: A Phase 2, Randomized, 6- to 19-Week, Open-Label, Active-Comparator, Dose-Ranging, Safety and Exploratory Efficacy Study. Am. J. Kidney Dis. 67 (6), 912–924. doi:10.1053/j.ajkd.2015.12.020
Rankin, E. B., Biju, M. P., Liu, Q., Unger, T. L., Rha, J., Johnson, R. S., et al. (2007). Hypoxia-inducible Factor-2 (HIF-2) Regulates Hepatic Erythropoietin In Vivo. J. Clin. Invest. 117 (4), 1068–1077. doi:10.1172/JCI30117
Rosenfeld, C., Cheever, M. A., and Gaiger, A. (2003). WT1 in Acute Leukemia, Chronic Myelogenous Leukemia and Myelodysplastic Syndrome: Therapeutic Potential of WT1 Targeted Therapies. Leukemia 17 (7), 1301–1312. doi:10.1038/sj.leu.2402988
Scholz, H., Wagner, K.-D., and Wagner, N. (2009). Role of the Wilms' Tumour Transcription Factor, Wt1, in Blood Vessel Formation. Pflugers Arch. - Eur. J. Physiol. 458 (2), 315–323. doi:10.1007/s00424-008-0621-3
Semenza, G. L. (1999). Regulation of Mammalian O2 Homeostasis by Hypoxia-Inducible Factor 1. Annu. Rev. Cel Dev. Biol. 15, 551–578. doi:10.1146/annurev.cellbio.15.1.551
Sera, T., Hiasa, Y., Mashiba, T., Tokumoto, Y., Hirooka, M., Konishi, I., et al. (2008). Wilms' Tumour 1 Gene Expression Is Increased in Hepatocellular Carcinoma and Associated with Poor Prognosis. Eur. J. Cancer 44 (4), 600–608. doi:10.1016/j.ejca.2008.01.008
Sofue, T., Nakagawa, N., Kanda, E., Nagasu, H., Matsushita, K., Nangaku, M., et al. (2020). Prevalence of Anemia in Patients with Chronic Kidney Disease in Japan: A Nationwide, Cross-Sectional Cohort Study Using Data from the Japan Chronic Kidney Disease Database (J-CKD-DB). PLoS One 15 (7), e0236132. doi:10.1371/journal.pone.0236132
Solomon, S. D., Uno, H., Lewis, E. F., Eckardt, K.-U., Lin, J., Burdmann, E. A., et al. (2010). Erythropoietic Response and Outcomes in Kidney Disease and Type 2 Diabetes. N. Engl. J. Med. 363 (12), 1146–1155. doi:10.1056/NEJMoa1005109
Stauffer, M. E., and Fan, T. (2014). Prevalence of Anemia in Chronic Kidney Disease in the United States. PLoS One 9 (1), e84943. doi:10.1371/journal.pone.0084943
Stone, W. J., Graber, S. E., Krantz, S. B., Dessypris, E. N., O’Neil, V. L., Olsen, N. J., et al. (1988). Treatment of the Anemia of Predialysis Patients with Recombinant Human Erythropoietin: a Randomized, Placebo-Controlled Trial. Am. J. Med. Sci. 296 (3), 171–179. doi:10.1097/00000441-198809000-00005
Szczech, L. A., Barnhart, H. X., Inrig, J. K., Reddan, D. N., Sapp, S., Califf, R. M., et al. (2008). Secondary Analysis of the CHOIR Trial Epoetin-α Dose and Achieved Hemoglobin Outcomes. Kidney Int. 74 (6), 791–798. doi:10.1038/ki.2008.295
Talks, K. L., Turley, H., Gatter, K. C., Maxwell, P. H., Pugh, C. W., Ratcliffe, P. J., et al. (2000). The Expression and Distribution of the Hypoxia-Inducible Factors HIF-1α and HIF-2α in Normal Human Tissues, Cancers, and Tumor-Associated Macrophages. Am. J. Pathol. 157 (2), 411–421. doi:10.1016/s0002-9440(10)64554-3
Tuna, M., Chavez-Reyes, A., and Tari, A. M. (2005). HER2/neu Increases the Expression of Wilms' Tumor 1 (WT1) Protein to Stimulate S-phase Proliferation and Inhibit Apoptosis in Breast Cancer Cells. Oncogene 24 (9), 1648–1652. doi:10.1038/sj.onc.1208345
Wagner, K.-D., Wagner, N., Wellmann, S., Schley, G., Bondke, A., Theres, H., et al. (2003). Oxygen‐regulated Expression of the Wilms' Tumor suppressorWt1involves Hypoxia‐inducible Factor‐1 (HIF‐1). FASEB j. 17 (10), 1364–1366. doi:10.1096/fj.02-1065fje
Wagner, N., Michiels, J. F., Schedl, A., and Wagner, K.-D. (2008). The Wilms' Tumour Suppressor WT1 Is Involved in Endothelial Cell Proliferation and Migration: Expression in Tumour Vessels In Vivo. Oncogene 27 (26), 3662–3672. doi:10.1038/sj.onc.1211044
Wang, X., Gao, P., Lin, F., Long, M., Weng, Y., Ouyang, Y., et al. (2013). Wilms' Tumour Suppressor Gene 1 (WT1) Is Involved in the Carcinogenesis of Lung Cancer through Interaction with PI3K/Akt Pathway. Cancer Cel Int 13 (1), 114. doi:10.1186/1475-2867-13-114
Wenger, R. H., Stiehl, D. P., and Camenisch, G. (2005). Integration of Oxygen Signaling at the Consensus HRE. Sci. STKE 2005 (306), re12. doi:10.1126/stke.3062005re12
Wessels, A., and Pérez-Pomares, J. M. (2004). The Epicardium and Epicardially Derived Cells (EPDCs) as Cardiac Stem Cells. Anat. Rec. 276A (1), 43–57. doi:10.1002/ar.a.10129
Yamamoto, S., Tsuda, H., Kita, T., Maekawa, K., Fujii, K., Kudoh, K., et al. (2007). Clinicopathological Significance of WT1 Expression in Ovarian Cancer: a Possible Accelerator of Tumor Progression in Serous Adenocarcinoma. Virchows Arch. 451 (1), 27–35. doi:10.1007/s00428-007-0433-4
Yang, J., Zhang, X., Zhang, Y., Zhu, D., Zhang, L., Li, Y., et al. (2016). HIF-2α Promotes Epithelial-Mesenchymal Transition through Regulating Twist2 Binding to the Promoter of E-Cadherin in Pancreatic Cancer. J. Exp. Clin. Cancer Res. 35, 26. doi:10.1186/s13046-016-0298-y
Yang, L., Han, Y., Saurez Saiz, F., and Minden, M. D. (2007). A Tumor Suppressor and Oncogene: the WT1 story. Leukemia 21 (5), 868–876. doi:10.1038/sj.leu.2404624
Yoo, Y.-G., Christensen, J., and Huang, L. E. (2011). HIF-1α Confers Aggressive Malignant Traits on Human Tumor Cells Independent of its Canonical Transcriptional Function. Cancer Res. 71 (4), 1244–1252. doi:10.1158/0008-5472.CAN-10-2360
Zhang, H., Li, H., Xi, H. S., and Li, S. (2012). HIF1α Is Required for Survival Maintenance of Chronic Myeloid Leukemia Stem Cells. Blood 119 (11), 2595–2607. doi:10.1182/blood-2011-10-387381
Zhang, P. J., Goldblum, J. R., Pawel, B. R., Pasha, T. L., Fisher, C., and Barr, F. G. (2005). PDGF-A, PDGF-Rβ, TGFβ3 and Bone Morphogenic Protein-4 in Desmoplastic Small Round Cell Tumors with EWS-WT1 Gene Fusion Product and Their Role in Stromal Desmoplasia: an Immunohistochemical Study. Mod. Pathol. 18 (3), 382–387. doi:10.1038/modpathol.3800264
Keywords: Wilms’ tumor 1, anemia, cancer, chronic kidney disease, hypoxia-inducible factor-prolyl hydroxylase inhibitor
Citation: Lee W-, Chiu C-, Chu T- and Chien Y- (2022) WT1: The Hinge Between Anemia Correction and Cancer Development in Chronic Kidney Disease. Front. Cell Dev. Biol. 10:876723. doi: 10.3389/fcell.2022.876723
Received: 15 February 2022; Accepted: 21 March 2022;
Published: 06 April 2022.
Edited by:
You-Ying Chau, University of Edinburgh, United KingdomReviewed by:
Xiong Zhong Ruan, University College London, United KingdomChien-Liang Chen, Kaohsiung Veterans General Hospital, Taiwan
Ching-Hung Lin, National Taiwan University, Taiwan
Copyright © 2022 Lee, Chiu, Chu and Chien. This is an open-access article distributed under the terms of the Creative Commons Attribution License (CC BY). The use, distribution or reproduction in other forums is permitted, provided the original author(s) and the copyright owner(s) are credited and that the original publication in this journal is cited, in accordance with accepted academic practice. No use, distribution or reproduction is permitted which does not comply with these terms.
*Correspondence: Yu-Shu Chien, Y2hpZW55c0BjZ21oLm9yZy50dw==