- 1Department of Medical Genetics, School of Medicine, Shahid Beheshti University of Medical Sciences, Tehran, Iran
- 2Phytochemistry Research Center, Shahid Beheshti University of Medical Sciences, Tehran, Iran
- 3Department of Pharmacognosy, College of Pharmacy, Hawler Medical University, Erbil, Iraq
- 4Center of Research and Strategic Studies, Lebanese French University, Erbil, Iraq
- 5Institute of Human Genetics, Jena University Hospitals, Jena, Germany
- 6Malopolska Centre of Biotechnology, Jagiellonian University, Krakow, Poland
- 7Urology and Nephrology Research Center, Shahid Beheshti University of Medical Sciences, Tehran, Iran
- 8Anesthesiology Research Center, Mofid Children Hospital, Shahid Beheshti University of Medical Sciences, Tehran, Iran
Senescence is defined as a gradual weakening of functional features of a living organism. Cellular senescence is a process that is principally aimed to remove undesirable cells by prompting tissue remodeling. This process is also regarded as a defense mechanism induced by cellular damage. In the course of oncogenesis, senescence can limit tumor progression. However, senescence participates in the pathoetiology of several disorders such as fibrotic disorders, vascular disorders, diabetes, renal disorders and sarcopenia. Recent studies have revealed contribution of different classes of non-coding RNAs in the cellular senescence. Long non-coding RNAs, microRNAs and circular RNAs are three classes of these transcripts whose contributions in this process have been more investigated. In the current review, we summarize the available literature on the impact of these transcripts in the cellular senescence.
Introduction
Living organisms encounter a gradual weakening of functional features. This phenomenon is called senescence or biological aging. This word may refer to either cellular senescence or to organismal senescence, the latter being characterized by an increase in death rate and/or a reduction in fertility parallel with increase in age, at least in the later portion of the life cycle of an organism. Cellular senescence is a process that is principally aimed to remove undesirable cells by prompting tissue remodeling (Muñoz-Espín and Serrano, 2014). This process induces tissue remodeling via three consecutive processes: a constant proliferative arrest; a secretory phenotype that leads to recruitment of immune cells and induces changes in the extracellular matrix; and recruitment of neighboring progenitors that activate tissue repopulation (Muñoz-Espín and Serrano, 2014). Cellular senescence has a role in morphogenesis and patterning in the course of embryogenesis through removal of temporary structures and regulation of the relative quantities of diverse cell populations. This process is also regarded as a defense mechanism induced by cellular damage. In the course of oncogenesis, senescence can limit tumor progression. However, senescence participates in the pathoetiology of several disorders such as fibrotic disorders, vascular disorders, diabetes, renal disorders and sarcopenia. While in the initial phases of these pathologies, senescence can limit the fibrotic responses and trigger immune responses that clear the injured cells, at advanced phases, accumulation of senescent cells can lead to aggravation of the pathological manifestations (Muñoz-Espín and Serrano, 2014).
Diverse mechanisms have been found to affect cellular senescence. DNA damage or telomere shortening can induce DNA damage response which can activate senescence. Moreover, secretion of high quantities of certain proteins such as chemokines, pro-inflammatory cytokines and matrix metalloproteinases by senescent cells can activate this process in other cells (Sławińska and Krupa, 2021).
Recent studies have revealed contribution of different classes of non-coding RNAs in the cellular senescence. Long non-coding RNAs (lncRNAs), microRNAs (miRNAs) and circular RNAs (circRNAs) are three classes of these transcripts whose contributions in this process have been more investigated. These three classes of transcript can regulate gene expression through different mechanisms. While miRNAs mostly act at post-transcriptional level through binding with mRNAs and inducing mRNA degradation or suppressing translation, lncRNAs can act at different levels to affect gene expression. Both lncRNAs and circRNA can act as molecular sponges for miRNAs to influence bioavailability of miRNAs and release miRNA targets from their suppressive effects. In addition to this “Decoy” function, lncRNAs can control nuclear hormone receptor abundance or telomere length, or can modulate expression of tumor suppressors by influencing specific stages of their transcription or translation (Dianatpour and Ghafouri-Fard, 2017; Ghafouri-Fard et al., 2020). Expression of these transcript can be modulated by DNA methylation (Ji et al., 2020).
Notably, therapeutic targeting of miRNAs and lncRNAs has been recently suggested as a striking way for management of several disorders with antisense oligonucleotides and small interfering RNAs being the most promising modalities in this regard (Winkle et al., 2021). In the current review, we summarize the available literature on the impact of these transcripts in the cellular senescence.
LncRNAs and Senescence
Expressions of lncRNAs have been found to be altered during the course of senescence. Moreover, a number of lncRNAs can induce or de-activate cellular senescence. TERRA is an example of lncRNAs contributing in cellular senescence (Grammatikakis et al., 2014). This lncRNA inhibits telomere elongation. Most notably, TERRA lncRNA is transcribed from telomere, a region which has been supposed to be transcriptionally silent. Inhibition of elongation of telomeric RNA is associated with the existence of several copies of the telomere UUAGGG repeat in the TERRA transcript (Porro et al., 2014) which offers TERRA a competitive inhibitory effect for TERT (Redon et al., 2010).
Expressions of ANRIL and Sirt1-AS have been shown to be down-regulated, while expression of miR-181a has been found to be increased in aging vascular smooth muscle cells (VSMCs). Functional studies in young and aging VSMCs have shown that up-regulation of ANRIL leads to down-regulation of miR-181a, up-regulation of Sirt1-AS and enhancement of cell viability and suppression of senescence in VSMCs. miR-181a directly targets both ANRIL and Sirt1-AS transcripts. Besides, the up-regulation of ANRIL is able to suppress cell cycle arrest and decrease activity of p53-p21 pathway (Tan et al., 2019a).
Another study in cervical cancer cell lines has shown up-regulation of ANRIL and down-regulation of miR-181a-5p. Short hairpin RNA-mediated silencing of ANRIL has led to inhibition of cell proliferation, invasiveness, migratory potential and enhancement of apoptosis and senescence. This study has also confirmed direct interaction between ANRIL and miR-181a-5p. Notably, TGFβI has been found to be targeted by miR-181a-5p (Zhu et al., 2019a).
LncRNAs might also regulate senescence process of endothelial progenitor cells (EPCs), a group of cells with potential for repair of injured endothelial cells. However, EPC senescence might lead to the failure in EPC function. GAS5 has been found to sponge miR-223, thus decreasing its expression. GAS5 silencing suppressed proliferation of EPCs and increased their senescence through counteracting with miR-223-mediated inhibition of NAMPT expression. Therefore, GAS5 can regulate proliferation and senescence of EPCS through modulation of the PI3K/AKT signaling (Yao et al., 2019). Another study has indicated that suppression of miR-665 by GAS5 modulates VSMC senescence through down-regulating SDC1 (Chen et al., 2021a). GAS5 has also been shown to be differentially expressed between young and old mouse brain samples, indicating its putative impact on senescence and brain aging. Expression of GAS5 has been significantly higher in the hippocampus region of old mouse compared with young mouse. In the hippocampus-derived HT22 cell line, GAS5 is localized in the nucleoplasm and cytoplasm. Functionally, GAS5 could inhibit cell cycle progression and induce apoptosis. Based on the results of RNA-Seq analyses, GAS5 can regulate expression of genes participating in cell proliferation. Moreover, GAS5 interacting proteins have been shown to be involved in senescence-associated disorders (Wang et al., 2021a). Figure 1 shows lncRNAs that participate in the senescence process.
Tano et al. have investigated the noncoding transcriptome of hepatocytes and cardiomyocytes in young and adult mice. Through this approach, they could isolate 62 lncRNAs associated with juvenility with selective expression in these two cell types. Gm14230 is one of these transcripts which has important roles for cellular juvenescence. Gm14230 knock-down has impaired cell growth and induced cellular senescence. Functionally, Gm14230 protects cellular juvenescence via recruitment of histone methyltransferase Ezh2 to Tgif2, thus suppressing the impact of Tgif2 in cellular senescence (Tano et al., 2019). GUARDIN is another lncRNA which can affect senescence. This lncRNA is an indispensable constituent of a transcriptional repressor complex containing LRP130 and PGC1α. In fact, this lncRNA serves as a scaffold to increase stability of LRP130/PGC1α heterodimers and their recruitment on the FOXO4 promoter. GUARDIN knock-down leads to destabilization of this complex and subsequent increase in FOXO4 expression. This also increases expression of p21, thus inducing cellular senescence. Notably, expression of GUARDIN is increased by rapamycin, a substance that inhibits senescence. Finally, FOSL2 can repress expression of GUARDIN (Sun et al., 2020).
H19 is another lncRNA that participates in the process of aging. This lncRNA has been found to be under-expressed in the endothelial cells of aged mice. Similarly, expression of H19 has been down-regulated in atherosclerotic plaques compared with normal carotid artery. Mecahnistically, H19 silencing has resulted in the upregulation of p16 and p21, thus reducing cell proliferation and inducing senescence in vitro. Moreover, H19 silencing has suppressed sprouting capacity of aortic rings in young mice. Endothelial-specific H19 deficiency has resulted in elevation of systolic blood pressure and susceptibility to hindlimb ischemia. Exon array analyses have indicated participation of H19 in IL-6 signaling. Moreover, ICAM1 and VCAM1 have been found to be upregulated following H19 silencing. Based on the results of luciferase reporter screening, STAT3 is activated after H19 silencing and suppressed upon H19 up-regulation (Hofmann et al., 2019). In addition to this mechanism, H19 targeting miR-22 has an impact in modulation of H2O2-induced dysregulation in cellular senescence via Wnt signaling (Figure 2) (Wang et al., 2018a).
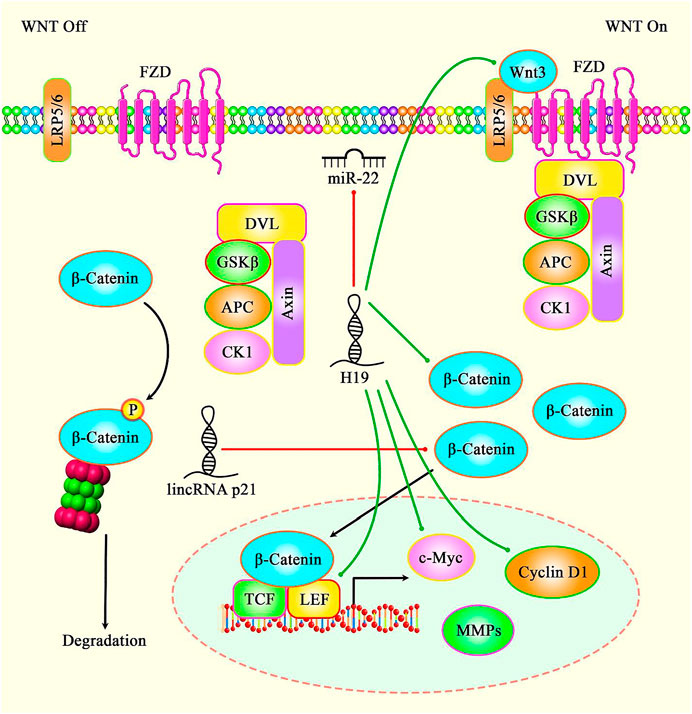
FIGURE 2. A schematic diagram of the role of several lncRNAs in the modulation of cellular senescence through Wnt/β-catenin signaling pathway. Accumulating evidence has illustrated that various lncRNAs are important regulatory factors in the biological aging. It has been reported that lncRNA H19 via directly targeting miR-22 could promote H2O2-induced deregulation in nucleus pulposus cell senescence, proliferation, and ECM synthesis through Wnt/β-catenin signaling cascade. Thereby, lncRNA H19 could enhance the expression levels of LEF1, c-Myc, and Cyclin D1 in NPCs (Wang et al., 2018b). Moreover, another research has figured out that lncRNA-p21 via downregulating β-catenin expression could regulate cellular senescence in mesenchymal stem cells (Xia et al., 2017). Green lines indicate the positive regulatory effect among lncRNAs and their targets, and red lines depict negative one among them.
HCP5 is another lncRNA that regulates cellular senescence through modulation of expression of iR-128. In fact, HCP5 silencing can induce senescence in glioma cells through this rout, thus enhancing their radiosensitivity (Wang et al., 2021b). In addition to these lncRNAs, a number of long intergenic RNAs (lincRNAs) such as LINC00623, LINC00673, LINC01255, Linc-ASEN and lincRNA p21 have been found to affect cellular senescence. For instance, LINC00623 affects IL-1β-mediated degradation of extracellular matrix, thus being involved in the process of senescence of osteoarthritis chondrocytes through acting as a sponge for miR-101 and influencing expression of HRAS (Lü et al., 2020). The role of LINC00673 in cellular senesence has been well established in the context of lung camcer (Roth et al., 2018). Moreover, LINC01255 has been shown to modulate senesence of mesenchymal stromal cell and proliferation of acute myeloid leukemia cells via inhibition of expression of MCP-1 (Liu et al., 2021a). Supplementary Table S1 summarizes the role of lncRNAs in senescence.
miRNAs and Senescence
Several miRNAs can influence senescence process through different mechanisms. Figure 3 depicts a number of mechanisms through which miRNAs regulate this process.
Let-7g is an example of miRNAs with anti-aging impact on endothelial cells. This miRNA can inhibit expression of LOX-1, a gene that activates aging process. An in vitro study in endothelial cells has shown that Ang II can increase expression of aging markers, while decreasing expression of the anti-aging gene SIRT1. Let-7g could exert anti-aging effects on endothelial cells through a LOX-1-independent route (Hsu et al., 2018).
miRNAs can also regulate senescence in the neoplastic conditions. miR-7 is an example of miRNAs being down-regulated in gemcitabine-resistant pancreatic cancer cells. This miRNA can regulate cellular senescence through influencing PARP1/NF-κB pathway. Restoration of miR-7 expression has induced gemcitabine sensitivity in pancreatic cancer cells to. Taken together, miR-7 can regulate cellular senescence and ameliorate resistance to gemcitabine through influencing PARP1/NF-κB pathway (Ye et al., 2021).
Experiments in an animal model of acute myocardial infarction have shown over-expression of miR-18a. Moreover, BDNF has been found to be down-regulated. Suppression of miR-18a expression has led to increase in the expression levels of p-AKT and p-mTOR and the quantities of senescent cells. Moreover, this intervention has reduced levels of Beclin1, LC3-II, p62 decreased and autophagy (Locatelli et al., 2019).
Virus-encoded miRNAs can also affect senescence process in host cells. For instance, EBV-miR-BART3-3p (BART3-3p) has been shown to promote growth of gastric cancer and inhibit the senescence process of these cells. Moreover, BART3-3p inhibits senescence of these cells in a mouse model of gastric cancer and suppress infiltration of NK cells and macrophages in tumors through changing the senescence-associated secretory phenotype. Functionally, BART3-3p targets TP53 and induces down-regulation of p21 (Xu et al., 2019a).
miRNAs can also participate in cytokine-induced premature senescence in endothelium. Expression assay in cellular model of this type of senescence has shown differential expression of eight miRNAs in TNF-α-induced premature senescence cells. Among dysregulated miRNAs have been members of the miR-17-92 cluster. These miRNAs have been known to regulate angiogenesis. hsa-miR-20b participates in this process through targeting RBL1. Silencing of hsa-miR-20b has reduced premature senescence in the TNF-α-treated cells, enhanced their proliferation, elevated RBL1 levels but reduced levels the senescence marker p16INK4a (Wong et al., 2017).
miR-20b is another miRNA that regulates cellular senescence. This miRNA suppresses senescence of endothelial cells via modulating Wnt/β-catenin signals through the TXNIP/NLRP3 axis (Dong et al., 2020). Similarly, delivery of miR-21 and miR-217 by extracellular vesicles can induce cellular senescence in endothelial cells (Mensà et al., 2020). Figure 4 demonstrates the role of various miRNAs in modulating cellular senescence through PI3K/AKT/mTOR signaling pathway.
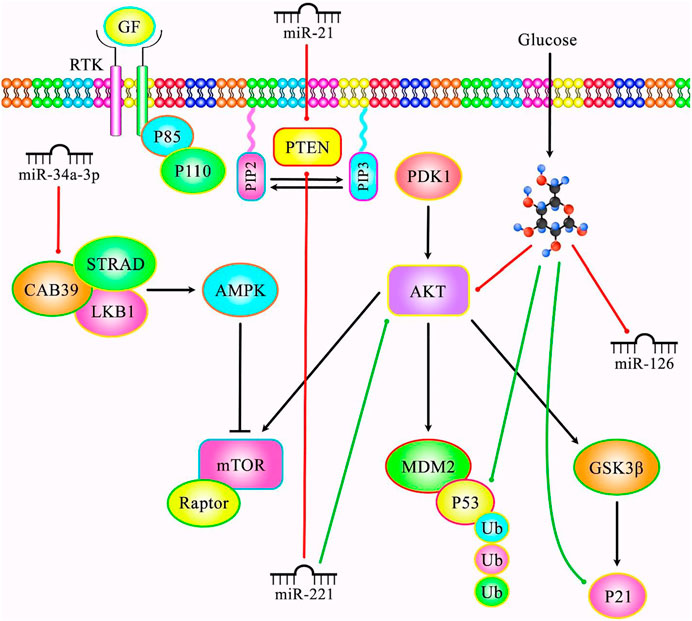
FIGURE 4. A schematic diagram of the role of several miRNAs in the senescence process through regulating PI3K/AKT/mTOR signaling cascade. Mounting evidence has demonstrated that aberrant expression of various miRNAs could be correlated with cellular senescence. As an illustration, a recent study has detected that miR-221 could play a crucial role in modulating proliferation, chemotherapy sensitivity and senescence in lung cancer cells via downregulating PTEN and upregulating AKT expression levels (Wang et al., 2018c). Moreover, another research has revealed that high glucose could result in premature senescence of human glomerular mesangial cells via reducing miR-126 and p-Akt expression levels and promote in the expression of p53, p21 and Rb proteins in the high-dose d-glucose group (Cao et al., 2019). Furthermore, another study has detected that miR-21 could elevate cardiac aging induced by D-gal and Dox via suppressing PTEN (Bei et al., 2018). In addition, miR-34a-3p could enhance the senescence of dental pulp stem cells via inhibiting CAB39 expression through the AMPK/mTOR signaling pathway (Zhang et al., 2021). Green lines indicate the positive regulatory effect among miRNAs and their targets, and red lines depict negative one among them.
Supplementary Table S2 summarizes the role of miRNAs in cellular senescence.
circRNAs and Senescence
Recent investigations have verified contribution of a number of circRNAs in regulation of cellular senescence in different tissues (Figure 5). For instance, circRNA-0077930 secreted from HG-HUVEs-Exos has been shown to increase senescence of VSMCs by reducing miR-622 expression and up-regulation of KRAS, p21, p53 and p16 expression (Wang et al., 2020c). Another study has shown over-expression of circ-Foxo3 in heart tissue of aged humans and mice. Up-regulation of this circRNA has been associated with expression of cellular senescence markers. Forced over-expression of circ-Foxo3 could aggravate doxorubicin-induced cardiomyopathy, while its silencing has relieved this process. Notably, circ-Foxo3 silencing inhibited the senescence process in embryonic fibroblasts of mice. Circ-Foxo3 is able to interact with ID-1, E2F1, FAK, and HIF1α (Du et al., 2017).
Regulation of cellular senescence by circRNAs has also investigated in neoplastic conditions. For instance, in hepatocellular carcinoma cells, up-regulation of circLARP4 could induce cellular senescence and cell cycle arrest through modulation of miR-761/RUNX3 axis (Du et al., 2019).
Comparison of expression profile of circRNAs in proliferating and senescent fibroblasts has shown differential expression of several circRNAs among being circPVT1. This circRNA is produced through circularization of a PVT1 exon. Expression of circPVT1 is decreased in senescent fibroblasts. Mechanistically, down-regulation of circPVT1 in proliferating fibroblasts triggers cellular senescence, enhanced senescence-related β-galactosidase activity, increased CDKN1A/P21 and p53 levels, and decreased cell proliferation. CircPVT1 has been predicted to sequester numerous miRNAs, among them being let-7 which has been enriched following circPVT1 pulldown. Consistent with this interaction, antagonizing endogenous let-7 has induced cell proliferation. CircPVT1 silencing has enhanced cellular senescence and overturned the proliferative effect of let-7. Expression of IGF2BP1, KRAS and HMGA2 have been decreased by circPVT1 silencing (Panda et al., 2017). CircCCNB1 is another circRNA that inhibits cellular senscence via sponging miR-449a and affecting CCNE2 (Du et al., 2019). Moreover, circACTA2 participates cellular senescence through regulation of interaction between ILF3 and CDK4 (Ma et al., 2021).
Table 1 summarizes the role of circRNAs in regulation of cellular senescence.
Discussion
The data presented above indicated contribution of miRNAs, lncRNAs and circRNAs in the modulation of senescence. The effective role of some lncRNAs such as ANRIL on cellular senescence has been verified in both malignant and non-malignant conditions. LncRNAs can affect senescence through sponging miRNAs. For instance, ANRIL/miR-181a, GAS5/miR-223, GAS5/miR-665, H19/miR-22, HCP5/miR-128, HEIH/miR-3619-5p, LINC00623/miR-101, MALAT1/miR-92a-3p, MEG3/miR-16-5p, MEG3/miR-128, MIAT/miR-22-3p, MIAT/miR-302, NEAT1/miR-221-3p, RP11-670E13.6/miR-663a and TRPC7-AS1/miR-4769-5p are examples of lncRNAs/miRNAs that corporately participate in the senescence. Similarly, there are several examples of circRNAs that participate in this process through sponging miRNAs. CircRNA-0077930/miR-622, circLARP4/miR-761, circPVT1/let-7 and circCCNB1/miR-449a are few uncovered circRNAs/miRNAs axes functioning in the regulation of senescence. Thus, it is obvious that lncRNAs, circRNAs and miRNAs work in a complex network to influence cellular senescence.
PI3K/AKT, Wnt/β-catenin, AMPK/mTOR/ULK1, MAPK, p53/p21, p16/pRb, NF-κB/TNF-α, RAF/MEK/ERK, JNK, Smad3/IκBα and Smad3/NF-κB signaling pathways are among pathways, which are regulated by non-coding RNAs in the process of cellular senescence.
Several non-coding RNAs that regulate cellular senescence interact with p53/p21 and/or phosphorylation of pRb/p16 pathways. These two interconnected and partly exclusive processes participate in all terminating events of senescence irrespective of varied initiating factors (Cai et al., 2021). Stress-dependent up-regulation of p53 induces PTEN, which in turn suppresses two p53 inhibitors, namely, PI3K/AKT and SIRT1/FOXO3a signals (Hekmatimoghaddam et al., 2017). Thus, interaction of non-coding RNAs with these pathways is another route of participation of non-coding RNAs in the cellular senescence.
Induction of cellular senescence can enhance response of cancer cells to therapeutic options. Thus, this strategy can be used in combination with conventional anti-cancer therapies. Identification of the impact of non-coding RNAs in the regulation of cellular senescence has a practical significance in cancer treatment. Moreover, since abnormal expression of non-coding RNAs in cancer cells can have anti-senescence effects, evaluation of levels of these transcripts in tumor samples can facilitate prior evaluation of response of tumor cells to chemoradiotherapy.
Manipulation of senescence process has been suggested as a therapeutic option for diverse disorders. While pro-senescent treatments are desirable therapies in malignant conditions and for continuing tissue repair processes, anti-senescent treatments can be helpful for removal of senescent cells in the contexts of ageing or chronic injuries (Muñoz-Espín and Serrano, 2014).
Taken together, non-coding RNAs have essential roles in the modulation of cellular senescence and pathoetiology of senescence-related disorders. This field represents an unexplored research area for design of novel therapies for a wide variety of human disorders, including both aging-related disorders and cancers.
Author Contributions
SG-F wrote the draft and revised it. MT designed and supervised the study. WB, AB, and TK collected the data and designed the figures and tables. All the authors approved and read the submitted version.
Conflict of Interest
The authors declare that the research was conducted in the absence of any commercial or financial relationships that could be construed as a potential conflict of interest.
Publisher’s Note
All claims expressed in this article are solely those of the authors and do not necessarily represent those of their affiliated organizations, or those of the publisher, the editors and the reviewers. Any product that may be evaluated in this article, or claim that may be made by its manufacturer, is not guaranteed or endorsed by the publisher.
Supplementary Material
The Supplementary Material for this article can be found online at: https://www.frontiersin.org/articles/10.3389/fcell.2022.869011/full#supplementary-material
References
Al-Khalaf, H. H., and Aboussekhra, A. (2017). p16INK4Ainduces Senescence and Inhibits EMT through microRNA-141/microRNA-146b-5p-dependent Repression of AUF1. Mol. Carcinog. 56 (3), 985–999. doi:10.1002/mc.22564
Alipoor, F. J., Asadi, M. H., and Torkzadeh‐Mahani, M. (2018). MIAT lncRNA Is Overexpressed in Breast Cancer and its Inhibition Triggers Senescence and G1 Arrest in MCF7 Cell Line. J. Cell. Biochem. 119 (8), 6470–6481. doi:10.1002/jcb.26678
Alique, M., Bodega, G., Giannarelli, C., Carracedo, J., and Ramírez, R. (2019). MicroRNA-126 Regulates Hypoxia-Inducible Factor-1α Which Inhibited Migration, Proliferation, and Angiogenesis in Replicative Endothelial Senescence. Sci. Rep. 9 (1), 7381–7419. doi:10.1038/s41598-019-43689-3
Asadi, M. H., and Yaghoobi, M. M. (2018). Long Noncoding RNA VIM-AS1 Promotes Colorectal Cancer Progression and Metastasis by Inducing EMT. Eur. J. Cell Biol. 97 (4), 279–288.
Auler, M., Bergmeier, V., Georgieva, V. S., Pitzler, L., Frie, C., Nüchel, J., et al. (2021). miR-127-3p Is an Epigenetic Activator of Myofibroblast Senescence Situated within the MicroRNA-Enriched Dlk1-Dio3‒Imprinted Domain on Mouse Chromosome 12. J. Investigative Dermatology 141 (4), 1076–1086. e3. doi:10.1016/j.jid.2020.11.011
Badi, I., Mancinelli, L., Polizzotto, A., Ferri, D., Zeni, F., Burba, I., et al. (2018). miR-34a Promotes Vascular Smooth Muscle Cell Calcification by Downregulating SIRT1 (Sirtuin 1) and Axl (AXL Receptor Tyrosine Kinase). Atvb 38 (9), 2079–2090. doi:10.1161/atvbaha.118.311298
Bae, Y.-U., Son, Y., Kim, C.-H., Kim, K. S., Hyun, S. H., Woo, H. G., et al. (2019). Embryonic Stem Cell-Derived Mmu-miR-291a-3p Inhibits Cellular Senescence in Human Dermal Fibroblasts through the TGF-β Receptor 2 Pathway. Journals Gerontology Ser. A 74 (9), 1359–1367. doi:10.1093/gerona/gly208
Bae, Y., Hwang, J. S., and Shin, Y. J. (2021). miR-30c-1 Encourages Human Corneal Endothelial Cells to Regenerate through Ameliorating Senescence. Aging 13 (7), 9348–9372. doi:10.18632/aging.202719
Baker, J. R., Vuppusetty, C., Colley, T., Hassibi, S., Fenwick, P. S., Donnelly, L. E., et al. (2019). MicroRNA‐570 Is a Novel Regulator of Cellular Senescence and Inflammaging. FASEB J. 33 (2), 1605–1616. doi:10.1096/fj.201800965r
Banerjee, J., Roy, S., Dhas, Y., and Mishra, N. (2020). Senescence-associated miR-34a and miR-126 in Middle-Aged Indians with Type 2 Diabetes. Clin. Exp. Med. 20 (1), 149–158. doi:10.1007/s10238-019-00593-4
Bastard, P., Rosen, L. B., Zhang, Q., Michailidis, E., Hoffmann, H. H., Zhang, Y., et al. (2020). Autoantibodies against Type I IFNs in Patients with Life-Threatening COVID-19. Science 370 (6515), 370. PubMed PMID: 32972996. Pubmed Central PMCID: PMC7857397. Epub 2020/09/26. eng. doi:10.1126/science.abd4585
Bei, Y., Wu, X., Cretoiu, D., Shi, J., Zhou, Q., Lin, S., et al. (2018). miR-21 Suppression Prevents Cardiac Alterations Induced by D-Galactose and Doxorubicin. J. Mol. Cell. Cardiol. 115, 130–141. doi:10.1016/j.yjmcc.2018.01.007
Cai, J., Qi, H., Yao, K., Yao, Y., Jing, D., Liao, W., et al. (2021). Non-coding RNAs Steering the Senescence-Related Progress, Properties, and Application of Mesenchymal Stem Cells. Front. Cell Dev. Biol. 9, 441. doi:10.3389/fcell.2021.650431
Cao, D.-w., Jiang, C.-m., Wan, C., Zhang, M., Zhang, Q.-y., Zhao, M., et al. (2018). Upregulation of MiR-126 Delays the Senescence of Human Glomerular Mesangial Cells Induced by High Glucose via Telomere-P53-P21-Rb Signaling Pathway. Curr. Med. Sci. 38 (5), 758–764. doi:10.1007/s11596-018-1942-x
Cao, D. W., Han, W. B., He, J. S., Zhao, M., Jiang, C. M., Zhang, Q. Y., et al. (2018). Tea Polyphenols Delays Human Glomerular Mesangial Cells Senescence Induced by High Glucose via Regulating STAT3/miR-126/telomere Signaling Pathway Activation. Zhongguo Zhong Yao Za Zhi 43 (23), 4678–4684. doi:10.19540/j.cnki.cjcmm.20181031.003
Cao, D., Zhao, M., Wan, C., Zhang, Q., Tang, T., Liu, J., et al. (2019). Role of Tea Polyphenols in Delaying Hyperglycemia-Induced Senescence in Human Glomerular Mesangial Cells via miR-126/Akt-P53-P21 Pathways. Int. Urol. Nephrol. 51 (6), 1071–1078. doi:10.1007/s11255-019-02165-7
Chatterjee, A., Jana, S., Chatterjee, S., Wastall, L. M., Mandal, G., Nargis, N., et al. (2019). MicroRNA-222 Reprogrammed Cancer-Associated Fibroblasts Enhance Growth and Metastasis of Breast Cancer. Br. J. Cancer 121 (8), 679–689. doi:10.1038/s41416-019-0566-7
Chen, T., Liang, Q., Xu, J., Zhang, Y., Zhang, Y., Mo, L., et al. (2021). MiR-665 Regulates Vascular Smooth Muscle Cell Senescence by Interacting with LncRNA GAS5/SDC1. Front. Cell Dev. Biol. 9. doi:10.3389/fcell.2021.700006
Chen, Y., Wang, S., Yang, S., Li, R., Yang, Y., Chen, Y., et al. (2021). Inhibitory Role of Ginsenoside Rb2 in Endothelial Senescence and Inflammation Mediated by microRNA-216a. Mol. Med. Rep. 23 (6), 1–11. doi:10.3892/mmr.2021.12054
Chen, Y. L., Sheu, J. J., Sun, C. K., Huang, T. H., Lin, Y. P., and Yip, H. K. (2020). MicroRNA-214 Modulates the Senescence of Vascular Smooth Muscle Cells in Carotid Artery Stenosis. Mol. Med. 26, 46–12. doi:10.1186/s10020-020-00167-1
Cheng, T., Ding, S., Liu, S., Li, Y., and Sun, L. (2021). Human Umbilical Cord-Derived Mesenchymal Stem Cell Therapy Ameliorate Lupus through Increasing CD4+ T Cell Senescence via MiR-199a-5p/Sirt1/p53 axis. Theranostics 11 (2), 893–905. doi:10.7150/thno.48080
Cheng, X., Shihabudeen Haider Ali, M. S., Moran, M., Viana, M. P., Schlichte, S. L., Zimmerman, M. C., et al. (2021). Long Non-coding RNA Meg3 Deficiency Impairs Glucose Homeostasis and Insulin Signaling by Inducing Cellular Senescence of Hepatic Endothelium in Obesity. Redox Biol. 40, 101863. doi:10.1016/j.redox.2021.101863
Choi, J. Y., Shin, H. J., and Bae, I. H. (2018). miR-93-5p Suppresses Cellular Senescence by Directly Targeting Bcl-W and P21. Biochem. biophysical Res. Commun. 505 (4), 1134–1140. doi:10.1016/j.bbrc.2018.10.010
Cui, H., Ge, J., Xie, N., Banerjee, S., Zhou, Y., Antony, V. B., et al. (2017). miR-34a Inhibits Lung Fibrosis by Inducing Lung Fibroblast Senescence. Am. J. Respir. Cell Mol. Biol. 56 (2), 168–178. doi:10.1165/rcmb.2016-0163OC
Cui, H., Ge, J., Xie, N., Banerjee, S., Zhou, Y., Liu, R.-M., et al. (2017). miR-34a Promotes Fibrosis in Aged Lungs by Inducing Alveolarepithelial Dysfunctions. Am. J. Physiology-Lung Cell. Mol. Physiology 312 (3), L415–L424. doi:10.1152/ajplung.00335.2016
Davis, C., Dukes, A., Drewry, M., Helwa, I., Johnson, M. H., Isales, C. M., et al. (2017). MicroRNA-183-5p Increases with Age in Bone-Derived Extracellular Vesicles, Suppresses Bone Marrow Stromal (Stem) Cell Proliferation, and Induces Stem Cell Senescence. Tissue Eng. Part A 23 (21-22), 1231–1240. doi:10.1089/ten.TEA.2016.0525
de Yébenes, V. G., Briones, A. M., Martos-Folgado, I., Mur, S. M., Oller, J., Bilal, F., et al. (2020). Aging-associated miR-217 Aggravates Atherosclerosis and Promotes Cardiovascular Dysfunction. Arterioscler. Thromb. Vasc. Biol. 40 (10), 2408–2424. doi:10.1161/ATVBAHA.120.314333
Deng, S., Wang, H., Jia, C., Zhu, S., Chu, X., Ma, Q., et al. (2017). MicroRNA-146a Induces Lineage-Negative Bone Marrow Cell Apoptosis and Senescence by Targeting Polo-like Kinase 2 Expression. Atvb 37 (2), 280–290. doi:10.1161/atvbaha.116.308378
Dhahri, W., Dussault, S., Légaré, É., Rivard, F., Desjarlais, M., Mathieu, R., et al. (2020). Reduced Expression of microRNA-130a Promotes Endothelial Cell Senescence and Age-dependent Impairment of Neovascularization. Aging 12 (11), 10180–10193. doi:10.18632/aging.103340
Dianatpour, A., and Ghafouri-Fard, S. (2017). Long Non Coding RNA Expression Intersecting Cancer and Spermatogenesis: a Systematic Review. Asian Pac J. Cancer Prev. 18 (10), 2601–2610. doi:10.22034/APJCP.2017.18.10.2601
Dong, C., Zhou, Q., Fu, T., Zhao, R., Yang, J., Kong, X., et al. (2019). Circulating Exosomes Derived-miR-146a from Systemic Lupus Erythematosus Patients Regulates Senescence of Mesenchymal Stem Cells. Biomed. Res. Int. 2019, 6071308. doi:10.1155/2019/6071308
Dong, F., Dong, S., Liang, Y., Wang, K., Qin, Y., and Zhao, X. (2020). miR-20b I-nhibits the S-enescence of H-uman U-mbilical V-ein E-ndothelial C-ells through R-egulating the Wnt/β-catenin P-athway via the TXNIP/NLRP3 axis. Int. J. Mol. Med. 45 (3), 847–857. doi:10.3892/ijmm.2020.4457
Dong, R., Wang, X., Wang, L., Wang, C., Huang, K., Fu, T., et al. (2021). Yangonin Inhibits Ethanol-Induced Hepatocyte Senescence via miR-194/FXR axis. Eur. J. Pharmacol. 890, 173653. doi:10.1016/j.ejphar.2020.173653
Dong, X., Hu, X., Chen, J., Hu, D., and Chen, L. F. (2018). BRD4 Regulates Cellular Senescence in Gastric Cancer Cells via E2F/miR-106b/p21 axis. Cell Death Dis. 9 (2), 203–213. doi:10.1038/s41419-017-0181-6
Du, W. W., Yang, W., Chen, Y., Wu, Z. K., Foster, F. S., Yang, Z., et al. (2017). Foxo3 Circular RNA Promotes Cardiac Senescence by Modulating Multiple Factors Associated with Stress and Senescence Responses. Eur. Heart J. 38 (18), 1402–1412. doi:10.1093/eurheartj/ehw001
Du, Y., Yu, Y., Hu, Y., Li, X.-W., Wei, Z.-X., Pan, R.-Y., et al. (2019). Genome-Wide, Integrative Analysis Implicates Exosome-Derived MicroRNA Dysregulation in Schizophrenia. Schizophr. Bull. 45 (6), 1257–1266. PubMed PMID: 30770930. Pubmed Central PMCID: 6811837. doi:10.1093/schbul/sby191
Fang, C., Chen, Y. X., Wu, N. Y., Yin, J. Y., Li, X. P., Huang, H. S., et al. (2017). MiR-488 Inhibits Proliferation and Cisplatin Sensibility in Non-small-cell Lung Cancer (NSCLC) Cells by Activating the eIF3a-Mediated NER Signaling Pathway. Sci. Rep. 7 (1), 40384–40411. doi:10.1038/srep40384
Fu, R., Yang, P., Sajid, A., and Li, Z. (2019). Avenanthramide A Induces Cellular Senescence via miR-129-3p/Pirh2/p53 Signaling Pathway to Suppress Colon Cancer Growth. J. Agric. Food Chem. 67 (17), 4808–4816. doi:10.1021/acs.jafc.9b00833
Ghafouri-Fard, S., Shoorei, H., and Taheri, M. (2020). The Role of Long Non-coding RNAs in Cancer Metabolism: A Concise Review. Front. Oncol. 10, 555825. doi:10.3389/fonc.2020.555825
Grammatikakis, I., Panda, A. C., Abdelmohsen, K., and Gorospe, M. (2014). Long Noncoding RNAs (lncRNAs) and the Molecular Hallmarks of Aging. Aging 6 (12), 992–1009. PubMed PMID: 25543668. eng. doi:10.18632/aging.100710
Guo, L., Chen, J., Liu, D., and Liu, L. (2020). OIP5-AS1/miR-137/ZNF217 Axis Promotes Malignant Behaviors in Epithelial Ovarian Cancer. Cmar 12, 6707–6717. doi:10.2147/cmar.s237726
Guo, Q., Zhang, H., Zhang, B., Zhang, E., and Wu, Y. (2019). Tumor Necrosis Factor-Alpha (TNF-α) Enhances miR-155-Mediated Endothelial Senescence by Targeting Sirtuin1 (SIRT1). Med. Sci. Monit. 25, 8820–8835. doi:10.12659/msm.919721
Guo, Y., Chao, L., and Chao, J. (2018). Kallistatin Attenuates Endothelial Senescence by Modulating Let‐7g‐mediated miR‐34a‐ SIRT 1‐ eNOS Pathway. J. Cell. Mol. Med. 22 (9), 4387–4398. doi:10.1111/jcmm.13734
Guo, Y., Li, P., Gao, L., Zhang, J., Yang, Z., Bledsoe, G., et al. (2017). Kallistatin Reduces Vascular Senescence and Aging by Regulating microRNA-34a-SIRT1 Pathway. Aging Cell 16 (4), 837–846. doi:10.1111/acel.12615
Guo, Y., Xing, L., Chen, N., Gao, C., Ding, Z., and Jin, B. (2019). Total Flavonoids from the Carya Cathayensis Sarg. Leaves Inhibit HUVEC Senescence through the miR‐34a/SIRT1 Pathway. J Cell. Biochem. 120 (10), 17240–17249. doi:10.1002/jcb.28986
Gupta, S., Silveira, D. A., and Mombach, J. C. M. (2020). ATM/miR‐34a‐5p axis Regulates a P21‐dependent Senescence‐apoptosis Switch in Non‐small Cell Lung Cancer: a Boolean Model of G1/S Checkpoint Regulation. FEBS Lett. 594 (2), 227–239. doi:10.1002/1873-3468.13615
Han, C., Zhou, J., Liu, B., Liang, C., Pan, X., Zhang, Y., et al. (2019). Delivery of miR-675 by Stem Cell-Derived Exosomes Encapsulated in Silk Fibroin Hydrogel Prevents Aging-Induced Vascular Dysfunction in Mouse Hindlimb. Mater. Sci. Eng. C 99, 322–332. doi:10.1016/j.msec.2019.01.122
He, J., Feng, X., Hua, J., Wei, L., Lu, Z., Wei, W., et al. (2017). miR-300 Regulates Cellular Radiosensitivity through Targeting P53 and Apaf1 in Human Lung Cancer Cells. Cell Cycle 16 (20), 1943–1953. doi:10.1080/15384101.2017.1367070
He, X., Yang, A., McDonald, D. G., Riemer, E. C., Vanek, K. N., Schulte, B. A., et al. (2017). MiR-34a Modulates Ionizing Radiation-Induced Senescence in Lung Cancer Cells. Oncotarget 8 (41), 69797–69807. doi:10.18632/oncotarget.19267
Hekmatimoghaddam, S., Dehghani Firoozabadi, A., Zare-Khormizi, M. R., and Pourrajab, F. (2017). Sirt1 and Parp1 as Epigenome Safeguards and microRNAs as SASP-Associated Signals, in Cellular Senescence and Aging. Ageing Res. Rev. 40, 120–141. doi:10.1016/j.arr.2017.10.001
Hemmings, K. E., Riches-Suman, K., Bailey, M. A., O’Regan, D. J., Turner, N. A., and Porter, K. E. (2021). Role of microRNA-145 in DNA Damage Signalling and Senescence in Vascular Smooth Muscle Cells of Type 2 Diabetic Patients. Cells 10 (4), 919. doi:10.3390/cells10040919
Herman, A. B., Anerillas, C., Harris, S. C., Munk, R., Martindale, J. L., Yang, X., et al. (2021). Reduction of Lamin B Receptor Levels by miR-340-5p Disrupts Chromatin, Promotes Cell Senescence and Enhances Senolysis. Nucleic acids Res. 49 (13), 7389–7405. doi:10.1093/nar/gkab538
Hofmann, P., Sommer, J., Theodorou, K., Kirchhof, L., Fischer, A., Li, Y., et al. (2019). Long Non-coding RNA H19 Regulates Endothelial Cell Aging via Inhibition of STAT3 Signalling. Cardiovasc. Res. 115 (1), 230–242. doi:10.1093/cvr/cvy206
Hong, Y., He, H., Jiang, G., Zhang, H., Tao, W., Ding, Y., et al. (2020). miR-155-5p Inhibition Rejuvenates Aged Mesenchymal Stem Cells and Enhances Cardioprotection Following Infarction. Aging Cell 19 (4), e13128. doi:10.1111/acel.13128
Houri, K., Mori, T., Onodera, Y., Tsujimoto, T., Takehara, T., Nakao, S., et al. (2020). miR-142 Induces Accumulation of Reactive Oxygen Species (ROS) by Inhibiting Pexophagy in Aged Bone Marrow Mesenchymal Stem Cells. Sci. Rep. 10 (1), 3735–3813. doi:10.1038/s41598-020-60346-2
Hsu, P. Y., Lin, W. Y., Lin, R. T., and Juo, S. H. (2018). MicroRNA Let-7g Inhibits Angiotensin II-Induced Endothelial Senescence via the LOX-1-independent Mechanism. Int. J. Mol. Med. 41 (4), 2243–2251. doi:10.3892/ijmm.2018.3416
Iwagami, Y., Zou, J., Zhang, H., Cao, K., Ji, C., Kim, M., et al. (2018). Alcohol‐mediated miR‐34a Modulates Hepatocyte Growth and Apoptosis. J. Cell. Mol. Medi 22 (8), 3987–3995. doi:10.1111/jcmm.13681
Jain, N., Roy, J., Das, B., and Mallick, B. (2019). miR‐197‐5p Inhibits Sarcomagenesis and Induces Cellular Senescence via Repression of KIAA0101. Mol. Carcinog. 58 (8), 1376–1388. doi:10.1002/mc.23021
Ji, J., Zhao, L., Zhao, X., Li, Q., An, Y., Li, L., et al. (2020). Genome-wide DNA M-ethylation R-egulation A-nalysis of L-ong N-on-coding RNAs in G-lioblastoma. Int. J. Mol. Med. 46 (1), 224–238. PubMed PMID: 32319552. Epub 04/15. eng. doi:10.3892/ijmm.2020.4579
Jia, Y., Jin, H., Gao, L., Yang, X., Wang, F., Ding, H., et al. (2020). A Novel lncRNA PLK4 Up‐regulated by Talazoparib Represses Hepatocellular Carcinoma Progression by Promoting YAP‐mediated Cell Senescence. J. Cell. Mol. Medi 24 (9), 5304–5316. doi:10.1111/jcmm.15186
Jiang, J., Hu, H., Chen, Q., Zhang, Y., Chen, W., Huang, Q., et al. (2021). Long Non-coding RNA SNHG29 Regulates Cell Senescence via P53/p21 Signaling in Spontaneous Preterm Birth. Placenta 103, 64–71. doi:10.1016/j.placenta.2020.10.009
Jiang, X., Ruan, X. L., Xue, Y. X., Yang, S., Shi, M., and Wang, L. N. (2020). Metformin Reduces the Senescence of Renal Tubular Epithelial Cells in Diabetic Nephropathy via the MBNL1/miR-130a-3p/STAT3 Pathway. Oxid. Med. Cell Longev. 2020, 8708236. doi:10.1155/2020/8708236
Jung, Y.-d., Park, S.-K., Kang, D., Hwang, S., Kang, M.-H., Hong, S.-W., et al. (2020). Epigenetic Regulation of miR-29a/miR-30c/DNMT3A axis Controls SOD2 and Mitochondrial Oxidative Stress in Human Mesenchymal Stem Cells. Redox Biol. 37, 101716. doi:10.1016/j.redox.2020.101716
Kalwa, M., Hänzelmann, S., Otto, S., Kuo, C.-C., Franzen, J., Joussen, S., et al. (2016). The lncRNA HOTAIR Impacts on Mesenchymal Stem Cellsviatriple Helix Formation. Nucleic Acids Res. 44 (22), 10631–10643. doi:10.1093/nar/gkw802
Kang, D., Shin, J., Cho, Y., Kim, H. S., Gu, Y. R., Kim, H., et al. (2019). Stress-activated miR-204 Governs Senescent Phenotypes of Chondrocytes to Promote Osteoarthritis Development. Sci. Transl. Med. 11 (486). doi:10.1126/scitranslmed.aar6659
Kim, N.-H., Choi, S.-H., Yi, N., Lee, T. R., and Lee, A.-Y. (2017). Arginase-2, a miR-1299 Target, Enhances Pigmentation in Melasma by Reducing Melanosome Degradation via Senescence-Induced Autophagy Inhibition. Pigment. Cell Melanoma Res. 30 (6), 521–530. doi:10.1111/pcmr.12605
Lai, X. H., Lei, Y., Yang, J., and Xiu, C. K. (2018). [Effect of microRNA-34a/SIRT1/p53 Signal Pathway on Notoginsenoside R₁ Delaying Vascular Endothelial Cell Senescence]. Zhongguo Zhong Yao Za Zhi 43 (3), 577–584. doi:10.19540/j.cnki.cjcmm.20180110.001
Lan, Y., Li, Y.-J., Li, D.-J., Li, P., Wang, J.-Y., Diao, Y.-P., et al. (2019). Long Noncoding RNA MEG3 Prevents Vascular Endothelial Cell Senescence by Impairing miR-128-dependent Girdin Downregulation. Am. J. Physiology-Cell Physiology 316 (6), C830–C843. doi:10.1152/ajpcell.00262.2018
Lee, H. C., Kang, D., Han, N., Lee, Y., Hwang, H. J., Lee, S.-B., et al. (2020). A Novel Long Noncoding RNA Linc-ASEN Represses Cellular Senescence through Multileveled Reduction of P21 Expression. Cell Death Differ. 27 (6), 1844–1861. doi:10.1038/s41418-019-0467-6
Lee, J. W., Chun, Y. L., Kim, A. Y., Lloyd, L. T., Ko, S., Yoon, J.-H., et al. (2021). Accumulation of Mitochondrial RPPH1 RNA Is Associated with Cellular Senescence. Ijms 22 (2), 782. doi:10.3390/ijms22020782
Li, B., Zhang, G., Wang, Z., Yang, Y., Wang, C., Fang, D., et al. (2021). c-Myc-activated USP2-AS1 Suppresses Senescence and Promotes Tumor Progression via Stabilization of E2F1 mRNA. Cell death Dis. 12 (11), 1–14. doi:10.1038/s41419-021-04330-2
Li, L., van Breugel, P. C., Loayza-Puch, F., Ugalde, A. P., Korkmaz, G., Messika-Gold, N., et al. (2018). LncRNA-OIS1 Regulates DPP4 Activation to Modulate Senescence Induced by RAS. Nucleic acids Res. 46 (8), 4213–4227. doi:10.1093/nar/gky087
Li, M., Li, L., Zhang, X., Zhao, H., Wei, M., Zhai, W., et al. (2019). LncRNA RP11-670E13.6, Interacted with hnRNPH, Delays Cellular Senescence by Sponging microRNA-663a in UVB Damaged Dermal Fibroblasts. Aging 11 (16), 5992–6013. doi:10.18632/aging.102159
Li, T., Luo, Z., Lin, S., Li, C., Dai, S., Wang, H., et al. (2020). MiR-185 Targets POT1 to Induce Telomere Dysfunction and Cellular Senescence. Aging 12 (14), 14791–14807. doi:10.18632/aging.103541
Li, X., Zhang, J., Yang, Y., Wu, Q., and Ning, H. (2021). MicroRNA-340-5p Increases Telomere Length by Targeting Telomere Protein POT1 to Improve Alzheimer's Disease in Mice. Cell Biol. Int. 45 (6), 1306–1315. doi:10.1002/cbin.11576
Li, X., Wu, J., Liu, S., Zhang, K., Miao, X., Li, J., et al. (2019). miR-384-5p Targets Gli2 and Negatively Regulates Age-Related Osteogenic Differentiation of Rat Bone Marrow Mesenchymal Stem Cells. Stem cells Dev. 28 (12), 791–798. doi:10.1089/scd.2019.0044
Li, Z.-y., Zhu, S.-s., Chen, X.-j., Zhu, J., Chen, Q., Zhang, Y.-q., et al. (2017). ARID1A Suppresses Malignant Transformation of Human Pancreatic Cells via Mediating Senescence-Associated miR-503/CDKN2A Regulatory axis. Biochem. biophysical Res. Commun. 493 (2), 1018–1025. doi:10.1016/j.bbrc.2017.09.099
Li, Z., Ge, X., Lu, J., Bian, M., Li, N., Wu, X., et al. (2019). MiR-141-3p Regulates Proliferation and Senescence of Stem Cells from Apical Papilla by Targeting YAP. Exp. Cell Res. 383 (2), 111562. doi:10.1016/j.yexcr.2019.111562
Liang, D., Hong, D., Tang, F., Wang, Y., Li, J., Li, L., et al. (2020). Upregulated lnc-HRK-2:1 P-rompts N-ucleus P-ulposus C-ell S-enescence in I-ntervertebral D-isc D-egeneration. Mol. Med. Rep. 22 (6), 5251–5261. doi:10.3892/mmr.2020.11603
Lin, N., Yao, Z., Xu, M., Chen, J., Lu, Y., Yuan, L., et al. (2019). Long Noncoding RNA MALAT1 Potentiates Growth and Inhibits Senescence by Antagonizing ABI3BP in Gallbladder Cancer Cells. J. Exp. Clin. Cancer Res. 38 (1), 244–315. doi:10.1186/s13046-019-1237-5
Lin, X., Zhan, J. K., Zhong, J. Y., Wang, Y. J., Wang, Y., Li, S., et al. (2019). lncRNA-ES3/miR-34c-5p/BMF axis Is Involved in Regulating High-Glucose-Induced Calcification/senescence of VSMCs. Aging (Albany NY) 11 (2), 523. doi:10.18632/aging.101758
Liu, C., Gao, J., Su, G., Xiang, Y., and Wan, L. (2020). MicroRNA-1202 Plays a Vital Role in Osteoarthritis via KCNQ1OT1 Has-miR-1202-ETS1 Regulatory Pathway. J. Orthop. Surg. Res. 15 (1), 130–139. doi:10.1186/s13018-020-01655-0
Liu, J., He, J., Ge, L., Xiao, H., Huang, Y., Zeng, L., et al. (2021). Hypoxic Preconditioning Rejuvenates Mesenchymal Stem Cells and Enhances Neuroprotection Following Intracerebral Hemorrhage via the miR-326-Mediated Autophagy. Stem Cell Res. Ther. 12 (1), 1–17. doi:10.1186/s13287-021-02480-w
Liu, J., Zhang, J., Zhang, G., Zhou, T., Zou, X., Guan, H., et al. (2021). CircMRE11A_013 Binds to UBXN1 and Integrates ATM Activation Enhancing Lens Epithelial Cells Senescence in Age-Related Cataract. Aging 13 (4), 5383–5402. doi:10.18632/aging.202470
Liu, Q., Dong, J., Li, J., Duan, Y., Wang, K., Kong, Q., et al. (2021). LINC01255 Combined with BMI1 to Regulate Human Mesenchymal Stromal Senescence and Acute Myeloid Leukemia Cell Proliferation through Repressing Transcription of MCP-1. Clin. Transl. Oncol. 23 (6), 1105–1116. doi:10.1007/s12094-020-02505-5
Liu, Y., Liu, Z., Xie, Y., Zhao, C., and Xu, J. (2019). Serum Extracellular Vesicles Retard H9C2 Cell Senescence by Suppressing miR-34a Expression. J. Cardiovasc. Trans. Res. 12 (1), 45–50. doi:10.1007/s12265-018-9847-4
Lo Curto, A., Taverna, S., Costa, M. A., Passantino, R., Augello, G., Adamo, G., et al. (2021). Can Be miR-126-3p a Biomarker of Premature Aging? an Ex Vivo and In Vitro Study in Fabry Disease. Cells 10 (2), 356. doi:10.3390/cells10020356
Locatelli, S. L., Careddu, G., Serio, S., Consonni, F. M., Maeda, A., Viswanadha, S., et al. (2019). Targeting Cancer Cells and Tumor Microenvironment in Preclinical and Clinical Models of Hodgkin Lymphoma Using the Dual PI3Kδ/γ Inhibitor RP6530. Clin. Cancer Res. 25 (3), 1098–1112. doi:10.1158/1078-0432.ccr-18-1133
Lou, K. X., Li, Z. H., Wang, P., Liu, Z., Chen, Y., Wang, X. L., et al. (2018). Long Non-coding RNA BANCR Indicates Poor Prognosis for Breast Cancer and Promotes Cell Proliferation and Invasion. Eur. Rev. Med. Pharmacol. Sci. 22 (5), 1358–1365. PubMed PMID: 29565494. Epub 2018/03/23. eng. doi:10.26355/eurrev_201803_14479
Lü, G., Li, L., Wang, B., and Kuang, L. (2020). LINC00623/miR-101/HRAS axis Modulates IL-1β-mediated ECM Degradation, Apoptosis and Senescence of Osteoarthritis Chondrocytes. Aging (Albany NY) 12 (4), 3218–3237. doi:10.18632/aging.102801
Lu, Q., Qin, H., Tan, H., Wei, C., Yang, X., He, J., et al. (2021). Senescence Osteoblast-Derived Exosome-Mediated miR-139-5p Regulates Endothelial Cell Functions. Biomed. Res. Int. 2021, 5576023. doi:10.1155/2021/5576023
Lyu, G., Guan, Y., Zhang, C., Zong, L., Sun, L., Huang, X., et al. (2018). TGF-β Signaling Alters H4K20me3 Status via miR-29 and Contributes to Cellular Senescence and Cardiac Aging. Nat. Commun. 9 (1), 2560–2613. doi:10.1038/s41467-018-04994-z
Ma, Y., Zheng, B., Zhang, X.-H., Nie, Z.-Y., Yu, J., Zhang, H., et al. (2021). circACTA2 Mediates Ang II-Induced VSMC Senescence by Modulation of the Interaction of ILF3 with CDK4 mRNA. Aging 13 (8), 11610–11628. doi:10.18632/aging.202855
Mao, G. X., Xu, X. G., Wang, S. Y., Li, H. F., Zhang, J., Zhang, Z. S., et al. (2019). Salidroside Delays Cellular Senescence by Stimulating Mitochondrial Biogenesis Partly through a miR-22/SIRT-1 Pathway. Oxid. Med. Cell Longev. 2019, 5276096. doi:10.1155/2019/5276096
Mensà, E., Guescini, M., Giuliani, A., Bacalini, M. G., Ramini, D., Corleone, G., et al. (2020). Small Extracellular Vesicles Deliver miR-21 and miR-217 as Pro-senescence Effectors to Endothelial Cells. J. Extracell. Vesicles 9 (1), 1725285. doi:10.1080/20013078.2020.1725285
Mokhberian, N., Bolandi, Z., Eftekhary, M., Hashemi, S. M., Jajarmi, V., Sharifi, K., et al. (2020). Inhibition of miR-34a Reduces Cellular Senescence in Human Adipose Tissue-Derived Mesenchymal Stem Cells through the Activation of SIRT1. Life Sci. 257, 118055. doi:10.1016/j.lfs.2020.118055
Montes, M., Lubas, M., Arendrup, F. S., Mentz, B., Rohatgi, N., Tumas, S., et al. (2021). The Long Non-coding RNA MIR31HG Regulates the Senescence Associated Secretory Phenotype. Nat. Commun. 12 (1), 2459–2517. doi:10.1038/s41467-021-22746-4
Muñoz-Espín, D., and Serrano, M. (2014). Cellular Senescence: from Physiology to Pathology. Nat. Rev. Mol. Cell Biol. 15 (7), 482–496.
Nunes, A. D., Weigl, M., Schneider, A., Noureddine, S., Yu, L., Lahde, C., et al. (2021). miR-146a-5p Modulates Cellular Senescence and Apoptosis in Visceral Adipose Tissue of Long-Lived Ames Dwarf Mice and in Cultured Pre-adipocytes. GeroScience 44, 1–16. doi:10.1007/s11357-021-00490-3
Panda, A. C., Grammatikakis, I., Kim, K. M., De, S., Martindale, J. L., Munk, R., et al. (2017). Identification of Senescence-Associated Circular RNAs (SAC-RNAs) Reveals Senescence Suppressor CircPVT1. Nucleic acids Res. 45 (7), 4021–4035. doi:10.1093/nar/gkw1201
Peng, C., Hu, W., Weng, X., Tong, R., Cheng, S., Ding, C., et al. (2017). Over Expression of Long Non-coding RNA PANDA Promotes Hepatocellular Carcinoma by Inhibiting Senescence Associated Inflammatory Factor IL8. Sci. Rep. 7 (1), 4186–4211. doi:10.1038/s41598-017-04045-5
Peng, D., Wang, H., Li, L., Ma, X., Chen, Y., Zhou, H., et al. (2018). miR-34c-5p Promotes Eradication of Acute Myeloid Leukemia Stem Cells by Inducing Senescence through Selective RAB27B Targeting to Inhibit Exosome Shedding. Leukemia 32 (5), 1180–1188. doi:10.1038/s41375-018-0015-2
Pi, C., Ma, C., Wang, H., Sun, H., Yu, X., Gao, X., et al. (2021). MiR-34a Suppression Targets Nampt to Ameliorate Bone Marrow Mesenchymal Stem Cell Senescence by Regulating NAD+-Sirt1 Pathway. Stem Cell Res. Ther. 12 (1), 1–17. doi:10.1186/s13287-021-02339-0
Piegari, E., Cozzolino, A., Ciuffreda, L. P., Cappetta, D., De Angelis, A., Urbanek, K., et al. (2020). Cardioprotective Effects of miR-34a Silencing in a Rat Model of Doxorubicin Toxicity. Sci. Rep. 10 (1), 12250–12312. doi:10.1038/s41598-020-69038-3
Porro, A., Feuerhahn, S., and Lingner, J. (2014). TERRA-reinforced Association of LSD1 with MRE11 Promotes Processing of Uncapped Telomeres. Cell Rep. 6 (4), 765–776. doi:10.1016/j.celrep.2014.01.022
Ramalho-Carvalho, J., Graça, I., Gomez, A., Oliveira, J., Henrique, R., Esteller, M., et al. (2017). Downregulation of miR-130b∼301b Cluster Is Mediated by Aberrant Promoter Methylation and Impairs Cellular Senescence in Prostate Cancer. J. Hematol. Oncol. 10 (1), 43–13. doi:10.1186/s13045-017-0415-1
Redon, S., Reichenbach, P., and Lingner, J. (2010). The Non-coding RNA TERRA Is a Natural Ligand and Direct Inhibitor of Human Telomerase. Nucleic acids Res. 38 (17), 5797–5806. doi:10.1093/nar/gkq296
Roth, A., Boulay, K., Groß, M., Polycarpou-Schwarz, M., Mallette, F. A., Regnier, M., et al. (2018). Targeting LINC00673 Expression Triggers Cellular Senescence in Lung Cancer. RNA Biol. 15 (12), 1499–1511. doi:10.1080/15476286.2018.1553481
Sang, B., Zhang, Y. Y., Guo, S. T., Kong, L. F., Cheng, Q., Liu, G. Z., et al. (2018). Dual Functions for OVAAL in Initiation of RAF/MEK/ERK Prosurvival Signals and Evasion of P27-Mediated Cellular Senescence. Proc. Natl. Acad. Sci. U. S. A. 115 (50), E11661–E70. doi:10.1073/pnas.1805950115
Satoh, M., Nasu, T., Takahashi, Y., Osaki, T., Hitomi, S., Morino, Y., et al. (2017). Expression of miR-23a Induces Telomere Shortening and Is Associated with Poor Clinical Outcomes in Patients with Coronary Artery Disease. Clin. Sci. 131 (15), 2007–2017. doi:10.1042/cs20170242
Shaosheng, W., Shaochuang, W., Lichun, F., Na, X., and Xiaohong, Z. (2021). ITPKA Induces Cell Senescence, Inhibits Ovarian Cancer Tumorigenesis and Can Be Downregulated by miR-203. Aging 13 (8), 11822–11832. doi:10.18632/aging.202880
Shen, J., Zhu, X., and Liu, H. (2020). MiR-483 Induces Senescence of Human Adipose-Derived Mesenchymal Stem Cells through IGF1 Inhibition. Aging 12 (15), 15756–15770. doi:10.18632/aging.103818
Shen, Z., Xuan, W., Wang, H., Sun, F., Zhang, C., Gong, Q., et al. (2020). miR-200b Regulates Cellular Senescence and Inflammatory Responses by Targeting ZEB2 in Pulmonary Emphysema. Artif. cells, nanomedicine, Biotechnol. 48 (1), 656–663. doi:10.1080/21691401.2020.1725029
Shi, L., Han, Q., Hong, Y., Li, W., Gong, G., Cui, J., et al. (2021). Inhibition of miR-199a-5p Rejuvenates Aged Mesenchymal Stem Cells Derived from Patients with Idiopathic Pulmonary Fibrosis and Improves Their Therapeutic Efficacy in Experimental Pulmonary Fibrosis. Stem Cell Res. Ther. 12 (1), 1–17. doi:10.1186/s13287-021-02215-x
Shiu, T.-Y., Shih, Y.-L., Feng, A.-C., Lin, H.-H., Huang, S.-M., Huang, T.-Y., et al. (2017). HCV Core Inhibits Hepatocellular Carcinoma Cell Replicative Senescence through Downregulating microRNA-138 Expression. J. Mol. Med. 95 (6), 629–639. doi:10.1007/s00109-017-1518-4
Shuai, T., Khan, M. R., Zhang, X. D., Li, J., Thorne, R. F., Wu, M., et al. (2021). lncRNA TRMP-S Directs Dual Mechanisms to Regulate P27-Mediated Cellular Senescence. Mol. Ther. - Nucleic Acids 24, 971–985. doi:10.1016/j.omtn.2021.04.004
Si, L., Chen, J., Yang, S., Liu, Z., Chen, Y., Peng, M., et al. (2020). lncRNA HEIH Accelerates Cell Proliferation and Inhibits Cell Senescence by Targeting miR-3619-5p/CTTNBP2 axis in Ovarian Cancer. Menopause 27 (11), 1302–1314. doi:10.1097/gme.0000000000001655
Sławińska, N., and Krupa, R. (2021). Molecular Aspects of Senescence and Organismal Ageing—DNA Damage Response, Telomeres, Inflammation and Chromatin. Int. J. Mol. Sci. 22 (2), 590.
Song, S., Yang, Y., Liu, M., Liu, B., Yang, X., Yu, M., et al. (2018). MiR-125b Attenuates Human Hepatocellular Carcinoma Malignancy through Targeting SIRT6. Am. J. Cancer Res. 8 (6), 993–1007.
Su, W., Hong, L., Xu, X., Huang, S., Herpai, D., Li, L., et al. (2018). miR-30 Disrupts Senescence and Promotes Cancer by Targeting Both p16INK4A and DNA Damage Pathways. Oncogene 37 (42), 5618–5632. doi:10.1038/s41388-018-0358-1
Sun, X., Thorne, R. F., Zhang, X. D., He, M., Li, J., Feng, S., et al. (2020). LncRNA GUARDIN Suppresses Cellular Senescence through a LRP130-pgc1α-FOXO4-p21-dependent Signaling axis. EMBO Rep. 21 (4), e48796. doi:10.15252/embr.201948796
Tan, J., Hu, L., Yang, X., Zhang, X., Wei, C., Lu, Q., et al. (2020). miRNA Expression Profiling Uncovers a Role of miR‐302b‐3p in Regulating Skin Fibroblasts Senescence. J. Cell Biochem. 121 (1), 70–80. doi:10.1002/jcb.28862
Tan, P., Wang, H., Zhan, J., Ma, X., Cui, X., Wang, Y., et al. (2019). Rapamycin-induced miR-30a D-ownregulation I-nhibits S-enescence of VSMCs by T-argeting Beclin1. Int. J. Mol. Med. 43 (3), 1311–1320. doi:10.3892/ijmm.2019.4074
Tan, P., Guo, Y.-H., Zhan, J.-K., Long, L.-M., Xu, M.-L., Ye, L., et al. (2019). LncRNA-ANRIL Inhibits Cell Senescence of Vascular Smooth Muscle Cells by Regulating miR-181a/Sirt1. Biochem. Cell Biol. 97 (5), 571–580. doi:10.1139/bcb-2018-0126
Tano, A., Kadota, Y., Morimune, T., Jam, F. A., Yukiue, H., Bellier, J. P., et al. (2019). The Juvenility-Associated Long Noncoding RNA Gm14230 Maintains Cellular Juvenescence. J. Cell Sci. 132 (8), jcs227801. doi:10.1242/jcs.227801
Tao, J., Fan, M., Zhou, D., Hong, Y., Zhang, J., Liu, H., et al. (2019). miR-200c Modulates the Pathogenesis of Radiation-Induced Oral Mucositis. Oxid. Med. Cell Longev. 2019, 2352079. doi:10.1155/2019/2352079
Tao, W., Hong, Y., He, H., Han, Q., Mao, M., Hu, B., et al. (2021). MicroRNA-199a-5p Aggravates Angiotensin II–Induced Vascular Smooth Muscle Cell Senescence by Targeting Sirtuin-1 in Abdominal Aortic Aneurysm. J. Cell. Mol. Med. 25 (13), 6056–6069. doi:10.1111/jcmm.16485
Tao, Y., Yue, P., Miao, Y., Gao, S., Wang, B., Leng, S. X., et al. (2021). The lncRNA MEG3/miR-16-5p/VGLL4 Regulatory axis Is Involved in Etoposide-Induced Senescence of Tumor Cells. J. Gene Med. 23 (2), e3291. doi:10.1002/jgm.3291
Thounaojam, M. C., Jadeja, R. N., Warren, M., Powell, F. L., Raju, R., Gutsaeva, D., et al. (2019). MicroRNA-34a (miR-34a) Mediates Retinal Endothelial Cell Premature Senescence through Mitochondrial Dysfunction and Loss of Antioxidant Activities. Antioxidants 8 (9), 328. doi:10.3390/antiox8090328
Tinaburri, L., D'Errico, M., Sileno, S., Maurelli, R., Degan, P., Magenta, A., et al. (2018). miR-200a Modulates the Expression of the DNA Repair Protein OGG1 Playing a Role in Aging of Primary Human Keratinocytes. Oxid. Med. Cell Longev. 2018, 9147326. doi:10.1155/2018/9147326
Wan, Y., McDaniel, K., Wu, N., Ramos-Lorenzo, S., Glaser, T., Venter, J., et al. (2017). Regulation of Cellular Senescence by miR-34a in Alcoholic Liver Injury. Am. J. pathology 187 (12), 2788–2798. doi:10.1016/j.ajpath.2017.08.027
Wang, B., Du, R., Xiao, X., Deng, Z.-L., Jian, D., Xie, H.-F., et al. (2017). Microrna-217 Modulates Human Skin Fibroblast Senescence by Directly Targeting DNA Methyltransferase 1. Oncotarget 8 (20), 33475–33486. doi:10.18632/oncotarget.16509
Wang, C., Yu, G., Xu, Y., Liu, C., Sun, Q., Li, W., et al. (2021). Knockdown of Long Non-coding RNA HCP5 Increases Radiosensitivity through Cellular Senescence by Regulating microRNA-128 in Gliomas. Cmar 13, 3723–3737. doi:10.2147/cmar.s301333
Wang, D., Cai, G., Wang, H., and He, J. (2020). TRAF3, a Target of microRNA-363-3p, Suppresses Senescence and Regulates the Balance between Osteoblastic and Adipocytic Differentiation of Rat Bone Marrow-Derived Mesenchymal Stem Cells. Stem cells Dev. 29 (11), 737–745. doi:10.1089/scd.2019.0276
Wang, N., Zhu, C., Xu, Y., Qian, W., and Zheng, M. (2018). Negative Regulation of PTEN by microRNA-221 and its Association with Drug Resistance and Cellular Senescence in Lung Cancer Cells. Biomed. Res. Int. 2018, 7908950. doi:10.1155/2018/7908950
Wang, S., Ke, S., Wu, Y., Zhang, D., Liu, B., He, Y-h., et al. (2021). Functional Network of the Long Non-coding RNA Growth Arrest-specific Transcript 5 and its Interacting Proteins in Senescence. Front. Genet. 12, 203. doi:10.3389/fgene.2021.615340
Wang, S., Zhan, J., Lin, X., Wang, Y., Wang, Y., and Liu, Y. (2020). CircRNA ‐0077930 from Hyperglycaemia‐stimulated Vascular Endothelial Cell Exosomes Regulates Senescence in Vascular Smooth Muscle Cells. Cell Biochem. Funct. 38 (8), 1056–1068. doi:10.1002/cbf.3543
Wang, X., Li, D., Wu, H., Liu, F., Liu, F., Zhang, Q., et al. (2020). LncRNA TRPC7-AS1 Regulates Nucleus Pulposus Cellular Senescence and ECM Synthesis via Competing with HPN for miR-4769-5p Binding. Mech. Ageing Dev. 190, 111293. doi:10.1016/j.mad.2020.111293
Wang, X., Zou, M., Li, J., Wang, B., Zhang, Q., Liu, F., et al. (2018). LncRNA H19 Targets miR‐22 to Modulate H 2 O 2 ‐induced Deregulation in Nucleus Pulposus Cell Senescence, Proliferation, and ECM Synthesis through Wnt Signaling. J. Cell. Biochem. 119 (6), 4990–5002. doi:10.1002/jcb.26738
Wang, X., Zou, M., Li, J., Wang, B., Zhang, Q., Liu, F., et al. (2018). LncRNA H19 Targets miR‐22 to Modulate H2 O2 ‐induced Deregulation in Nucleus Pulposus Cell Senescence, Proliferation, and ECM Synthesis through Wnt Signaling. J. Cell. Biochem. 119 (6), 4990–5002. PubMed PMID: 29520849. Epub 2018/03/10. eng. doi:10.1002/jcb.26738
Wang, Y., Chen, J., Chen, X., Jiang, F., Sun, Y., Pan, Y., et al. (2017). MiR-34a Suppresses HNSCC Growth through Modulating Cell Cycle Arrest and Senescence. Neoplasma 64 (4), 543–553. doi:10.4149/neo_2017_408
Winkle, M., El-Daly, S. M., Fabbri, M., and Calin, G. A. (2021). Noncoding RNA Therapeutics - Challenges and Potential Solutions. Nat. Rev. Drug Discov. 20 (8), 629–651. PubMed PMID: 34145432. Pubmed Central PMCID: PMC8212082 declare no competing interests. Epub 2021/06/20. eng. doi:10.1038/s41573-021-00219-z
Wong, P.-F., Jamal, J., Tong, K.-L., Khor, E.-S., Yeap, C.-E., Jong, H.-L., et al. (2017). Deregulation of Hsa-miR-20b Expression in TNF-α-Induced Premature Senescence of Human Pulmonary Microvascular Endothelial Cells. Microvasc. Res. 114, 26–33. doi:10.1016/j.mvr.2017.06.002
Xia, C., Jiang, T., Wang, Y., Chen, X., Hu, Y., and Gao, Y. (2021). The p53/miR-145a axis Promotes Cellular Senescence and Inhibits Osteogenic Differentiation by Targeting Cbfb in Mesenchymal Stem Cells. Front. Endocrinol. 11, 1017. doi:10.3389/fendo.2020.609186
Xia, W., Chen, H., Chen, D., Ye, Y., Xie, C., and Hou, M. (2020). PD-1 Inhibitor Inducing Exosomal miR-34a-5p Expression Mediates the Cross Talk between Cardiomyocyte and Macrophage in Immune Checkpoint Inhibitor-Related Cardiac Dysfunction. J. Immunother. Cancer 8 (2). doi:10.1136/jitc-2020-001293
Xia, W., and Hou, M. (2018). Mesenchymal Stem Cells Confer Resistance to Doxorubicin-Induced Cardiac Senescence by Inhibiting microRNA-34a. Oncol. Lett. 15 (6), 10037–10046. doi:10.3892/ol.2018.8438
Xia, W., Chen, H., Xie, C., and Hou, M. (2020). Long-noncoding RNA MALAT1 Sponges microRNA-92a-3p to Inhibit Doxorubicin-Induced Cardiac Senescence by Targeting ATG4a. Aging 12 (9), 8241–8260. doi:10.18632/aging.103136
Xia, W., Zhuang, L., Deng, X., and Hou, M. (2017). Long Noncoding RNA-P21 Modulates Cellular Senescence via the Wnt/β-Catenin Signaling Pathway in Mesenchymal Stem Cells. Mol. Med. Rep. 16 (5), 7039–7047. doi:10.3892/mmr.2017.7430
Xie, Q., Peng, J., Guo, Y., and Li, F. (2021). MicroRNA-33-5p Inhibits Cholesterol Efflux in Vascular Endothelial Cells by Regulating Citrate Synthase and ATP-Binding Cassette Transporter A1. BMC Cardiovasc Disord. 21 (1), 433–512. doi:10.1186/s12872-021-02228-7
Xie, Z., Xia, W., and Hou, M. (2018). Long Intergenic Non-coding RNA-p21 M-ediates C-ardiac S-enescence via the Wnt/β-catenin S-ignaling P-athway in D-oxorubicin-I-nduced C-ardiotoxicity. Mol. Med. Rep. 17 (2), 2695–2704. doi:10.3892/mmr.2017.8169
Xin, P., Xu, W., Zhu, X., Li, C., Zheng, Y., Zheng, T., et al. (2019). Protective Autophagy or Autophagic Death: Effects of BEZ235 on Chronic Myelogenous Leukemia. Cmar 11, 7933–7951. doi:10.2147/cmar.s204472
Xu, C. L., Sang, B., Liu, G. Z., Li, J. M., Zhang, X. D., Liu, L. X., et al. (2020). SENEBLOC, a Long Non-coding RNA Suppresses Senescence via P53-dependent and Independent Mechanisms. Nucleic acids Res. 48 (6), 3089–3102. doi:10.1093/nar/gkaa063
Xu, S., Wang, P., Zhang, J., Wu, H., Sui, S., Zhang, J., et al. (2019). Ai-lncRNA EGOT Enhancing Autophagy Sensitizes Paclitaxel Cytotoxicity via Upregulation of ITPR1 Expression by RNA-RNA and RNA-Protein Interactions in Human Cancer. Mol. Cancer 18 (1), 89–18. doi:10.1186/s12943-019-1017-z
Xu, S., Wu, W., Huang, H., Huang, R., Xie, L., Su, A., et al. (2019). The p53/miRNAs/Ccna2 Pathway Serves as a Novel Regulator of Cellular Senescence: Complement of the Canonical P53/p21 Pathway. Aging Cell 18 (3), e12918. doi:10.1111/acel.12918
Xu, S., Zhang, B., Zhu, Y., Huang, H., Yang, W., Huang, H., et al. (2017). miR-194 Functions as a Novel Modulator of Cellular Senescence in Mouse Embryonic Fibroblasts. Cell Biol. Int. 41 (3), 249–257. doi:10.1002/cbin.10715
Yan, Y., Qin, D., Hu, B., Zhang, C., Liu, S., Wu, D., et al. (2019). Deletion of miR-126a Promotes Hepatic Aging and Inflammation in a Mouse Model of Cholestasis. Mol. Ther. - Nucleic Acids 16, 494–504. doi:10.1016/j.omtn.2019.04.002
Yang, J., Lu, Y., Yang, P., Chen, Q., Wang, Y., Ding, Q., et al. (2019). MicroRNA‐145 Induces the Senescence of Activated Hepatic Stellate Cells through the Activation of P53 Pathway by ZEB2. J. Cell. Physiology 234 (5), 7587–7599. doi:10.1002/jcp.27521
Yang, S., Mi, X., Chen, Y., Feng, C., Hou, Z., Hui, R., et al. (2018). MicroRNA‐216a Induces Endothelial Senescence and Inflammation via Smad3/IκBα Pathway. J. Cell Mol. Med. 22 (5), 2739–2749. doi:10.1111/jcmm.13567
Yao, J., Shi, Z., Ma, X., Xu, D., and Ming, G. (2019). lncRNA GAS5/miR‐223/NAMPT axis Modulates the Cell Proliferation and Senescence of Endothelial Progenitor Cells through PI3K/AKT Signaling. J Cell. Biochem. 120 (9), 14518–14530. doi:10.1002/jcb.28713
Ye, Z. Q., Chen, H. B., Zhang, T. Y., Chen, Z., Tian, L., and Gu, D. N. (2021). MicroRNA-7 Modulates Cellular Senescence to Relieve Gemcitabine Resistance by Targeting PARP1/NF-Κb Signaling in Pancreatic Cancer Cells. Oncol. Lett. 21 (2), 139. doi:10.3892/ol.2020.12400
Yuan, D., Liu, Y., Li, M., Zhou, H., Cao, L., Zhang, X., et al. (2021). Senescence Associated Long Non-coding RNA 1 Regulates Cigarette Smoke-Induced Senescence of Type II Alveolar Epithelial Cells through Sirtuin-1 Signaling. J. Int. Med. Res. 49 (2), 300060520986049. doi:10.1177/0300060520986049
Yuan, M., Da Silva, A. C. A. L., Arnold, A., Okeke, L., Ames, H., Correa-Cerro, L. S., et al. (2018). MicroRNA (miR) 125b Regulates Cell Growth and Invasion in Pediatric Low Grade Glioma. Sci. Rep. 8 (1), 12506–12514. doi:10.1038/s41598-018-30942-4
Yuan, P., Qi, X., Song, A., Ma, M., Zhang, X., Lu, C., et al. (2021). LncRNA MAYA Promotes Iron Overload and Hepatocyte Senescence through Inhibition of YAP in Non‐alcoholic Fatty Liver Disease. J. Cell Mol. Med. 25 (15), 7354–7366. doi:10.1111/jcmm.16764
Zeng, Q., and Zeng, J. (2021). Inhibition of miR-494-3p Alleviates Oxidative Stress-Induced Cell Senescence and Inflammation in the Primary Epithelial Cells of COPD Patients. Int. Immunopharmacol. 92, 107044. doi:10.1016/j.intimp.2020.107044
Zhan, S., Wang, K., Xiang, Q., Song, Y., Li, S., Liang, H., et al. (2020). lncRNA HOTAIR Upregulates Autophagy to Promote Apoptosis and Senescence of Nucleus Pulposus Cells. J. Cell Physiol. 235 (3), 2195–2208. doi:10.1002/jcp.29129
Zhang, C.-L., Liu, X., He, Q.-J., Zheng, H., Xu, S., Xiong, X.-D., et al. (2017). miR-342-5p Promotes Zmpste24-Deficient Mouse Embryonic Fibroblasts Proliferation by Suppressing GAS2. Mol. Med. Rep. 16 (6), 8944–8952. doi:10.3892/mmr.2017.7731
Zhang, S., Zhang, R., Qiao, P., Ma, X., Lu, R., Wang, F., et al. (2021). Metformin-induced microRNA-34a-3p Downregulation Alleviates Senescence in Human Dental Pulp Stem Cells by Targeting CAB39 through the AMPK/mTOR Signaling Pathway. Stem Cells Int. 2021, 6616240. doi:10.1155/2021/6616240
Zhang, W., Hsu, P., Zhong, B., Guo, S., Zhang, C., Wang, Y., et al. (2018). MiR-34a Enhances Chondrocyte Apoptosis, Senescence and Facilitates Development of Osteoarthritis by Targeting DLL1 and Regulating PI3K/AKT Pathway. Cell Physiol. Biochem. 48 (3), 1304–1316. doi:10.1159/000492090
Zhang, Y., Liang, Q., Zhang, Y., Hong, L., Lei, D., and Zhang, L. (2020). Olmesartan Alleviates Bleomycin-Mediated Vascular Smooth Muscle Cell Senescence via the miR-665/SDC1 axis. Am. J. Transl. Res. 12 (9), 5205–5220.
Zhao, L., Hu, K., Cao, J., Wang, P., Li, J., Zeng, K., et al. (2019). lncRNA Miat Functions as a ceRNA to Upregulate Sirt1 by Sponging miR-22-3p in HCC Cellular Senescence. Aging 11 (17), 7098–7122. doi:10.18632/aging.102240
Zheng, Y., Liu, H., and Kong, Y. (2017). miR-188 Promotes Senescence of Lineage-Negative Bone Marrow Cells by Targeting MAP3K3 Expression. FEBS Lett. 591 (15), 2290–2298. doi:10.1002/1873-3468.12720
Zhong, H., Zhou, Z., Guo, L., Liu, F., Zheng, B., Bi, S., et al. (2021). The miR-623/CXCL12 axis Inhibits LPS-Induced Nucleus Pulposus Cell Apoptosis and Senescence. Mech. Ageing Dev. 194, 111417. doi:10.1016/j.mad.2020.111417
Zhong, J. Y., Cui, X. J., Zhan, J. K., Wang, Y. J., Li, S., Lin, X., et al. (2020). LncRNA‐ES3 Inhibition by Bhlhe40 Is Involved in High Glucose-Induced Calcification/senescence of Vascular Smooth Muscle Cells. Ann. N.Y. Acad. Sci. 1474 (1), 61–72. doi:10.1111/nyas.14381
Zhu, B., Zhang, L., Liang, C., Liu, B., Pan, X., Wang, Y., et al. (2019). Stem Cell-Derived Exosomes Prevent Aging-Induced Cardiac Dysfunction through a Novel Exosome/lncRNA MALAT1/NF-Κb/tnf-α Signaling Pathway. Oxid. Med. Cell Longev. 2019, 9739258. doi:10.1155/2019/9739258
Zhu, L., Zhang, Q., Li, S., Jiang, S., Cui, J., and Dang, G. (2019). Interference of the Long Noncoding RNA CDKN2B‐AS1 Upregulates miR‐181a‐5p/TGFβI axis to Restrain the Metastasis and Promote Apoptosis and Senescence of Cervical Cancer Cells. Cancer Med. 8 (4), 1721–1730. doi:10.1002/cam4.2040
Zhuang, L., Xia, W., Chen, D., Ye, Y., Hu, T., Li, S., et al. (2020). Exosomal LncRNA-NEAT1 Derived from MIF-Treated Mesenchymal Stem Cells Protected against Doxorubicin-Induced Cardiac Senescence through Sponging miR-221-3p. J. Nanobiotechnology 18 (1), 1–16. doi:10.1186/s12951-020-00716-0
Keywords: lncRNA, miRNA, senescence, expression, biomarker, epigenetics
Citation: Ghafouri-Fard S, Khoshbakht T, Hussen BM, Baniahmad A, Branicki W, Taheri M and Eghbali A (2022) Emerging Role of Non-Coding RNAs in Senescence. Front. Cell Dev. Biol. 10:869011. doi: 10.3389/fcell.2022.869011
Received: 03 February 2022; Accepted: 13 June 2022;
Published: 05 July 2022.
Edited by:
Leo Kurian, University of Cologne, GermanyReviewed by:
Cecilia Battistelli, Sapienza University of Rome, ItalyHamed Shoorei, Birjand University of Medical Sciences, Iran
Copyright © 2022 Ghafouri-Fard, Khoshbakht, Hussen, Baniahmad, Branicki, Taheri and Eghbali. This is an open-access article distributed under the terms of the Creative Commons Attribution License (CC BY). The use, distribution or reproduction in other forums is permitted, provided the original author(s) and the copyright owner(s) are credited and that the original publication in this journal is cited, in accordance with accepted academic practice. No use, distribution or reproduction is permitted which does not comply with these terms.
*Correspondence: Aria Baniahmad, aria.baniahmad@med.uni-jena.de; Mohammad Taheri, mohammad.taheri@uni-jena.de; Ahmad Eghbali, aegbali@yahoo.com