- 1Aurora BioSolutions Inc., Medicine Hat, AB, Canada
- 2Simon Fraser University, Burnaby, BC, Canada
Retrospective analysis of clinical trial outcomes is a vital exercise to facilitate efficient translation of cellular therapies. These analyses are particularly important for mesenchymal stem/stromal cell (MSC) products. The exquisite responsiveness of MSCs, which makes them attractive candidates for immunotherapies, is a double-edged sword; MSC clinical trials result in inconsistent outcomes that may correlate with underlying patient biology or procedural differences at trial sites. Here we review 45 North American MSC clinical trial results published between 2015 and 2021 to assess whether these reports provide sufficient information for retrospective analysis. Trial reports routinely specify the MSC tissue source, autologous or allogeneic origin and administration route. However, most methodological aspects related to cell preparation and handling immediately prior to administration are under-reported. Clinical trial reports inconsistently provide information about cryopreservation media composition, delivery vehicle, post-thaw time and storage until administration, duration of infusion, and pre-administration viability or potency assessments. In addition, there appears to be significant variability in how cell products are formulated, handled or assessed between trials. The apparent gaps in reporting, combined with high process variability, are not sufficient for retrospective analyses that could potentially identify optimal cell preparation and handling protocols that correlate with successful intra- and inter-trial outcomes. The substantial preclinical data demonstrating that cell handling affects MSC potency highlights the need for more comprehensive clinical trial reporting of MSC conditions from expansion through delivery to support development of globally standardized protocols to efficiently advance MSCs as commercial products.
Introduction
Mesenchymal stromal cell (MSC) products are rapidly advancing as clinical treatments for a range of inflammatory diseases and regenerative medicine applications (Davies et al., 2017; Martin et al., 2019; Levy et al., 2020; Wright et al., 2021). MSC therapies have consistently proven safe (Levy et al., 2020; Krampera and le Blanc, 2021), but clinical outcomes from both autologous and allogeneic MSC trials have been variable and often less beneficial than in preclinical studies (Galipeau and Sensébé, 2018; Martin et al., 2019; Levy et al., 2020; Krampera and le Blanc, 2021). The inconsistent performance of MSC products has been attributed to numerous factors, most of which remain poorly understood or controlled. These have been comprehensively reviewed by others and include MSC heterogeneity between donors, tissues of origin and expansion level (Martin et al., 2019; le Blanc and Davies, 2018; Wiese et al., 2019a; Galipeau et al., 2021), preparation/manufacturing protocols (de Wolf et al., 2017; Mennan et al., 2019; Yin et al., 2019; Levy et al., 2020), administration route (Braid et al., 2018; Giri and Galipeau, 2020; Levy et al., 2020; Moll et al., 2020; Galipeau et al., 2021) and the underlying biological differences between patient recipients (Martin et al., 2019; Levy et al., 2020; Moll et al., 2020; Galipeau et al., 2021).
The realization of MSCs as advanced therapy medicinal products/advanced medicinal products (ATMP/AMP) requires global standardization of MSC manufacturing protocols, critical quality attributes, release criteria, and product preparation and delivery protocols at treatment sites (Mendicino et al., 2014; de Wolf et al., 2017; Viswanathan et al., 2019; Galipeau et al., 2021; Wilson et al., 2021; Wright et al., 2021). Retrospective analysis of clinical trial outcomes is a vital exercise to identify the practices that correlate with successful outcomes and those that result in variable outcomes or unsatisfactory efficacy. Statistically powered comparisons of trial procedures and outcomes are limited, however, by the degree to which clinical trial data are recorded and reported.
In this review, we analyze the product and procedural information provided in peer-reviewed clinical trial reports published since 2015. Our analysis focuses on reporting of cell handling procedures from dose preparation–either fresh or thawed–through completion of cell transfer. Surprisingly, we discovered that few clinical trials specify and/or report the handling of MSC products during this window in which the cells are vulnerable to insult and may experience uncontrolled conditions. This lack of information precludes retrospective analysis of the influence of product handling and delivery with clinical outcomes.
Methods
Search Strategy
The search terms mesenchymal stromal cell clinical trial and mesenchymal stem cell clinical trial were searched in PubMed and Google Scholar with filters to include the clinical trial article type, published from 2015 to 2021 inclusive, with an available abstract and full text. These queries returned 471 articles effective 21 January 2022.
Report Selection and Data Extraction
The reports were filtered to include only trials using human-derived live MSC products for human use. Because reporting standards can vary by region, we further limited the scope of our analysis to clinical trials performed in North America. Rationale and Design articles were excluded. These refinements produced 45 peer-reviewed clinical trial reports for analysis.
Data was extracted verbatim from the curated reports according to four categories:
1) Trial and report particulars: Authors, article doi, trial location, publication year, trial phase, product name, affiliate company and clinical trial identifier
2) Study design: Disease or injury indication, administration route, MSC tissue of origin, selected MSC population (if any), MSC state (fresh, cryopreserved or culture-rescued after thaw) and donor relationship (allogeneic or autologous)
3) Dose preparation and handling: MSC dose (per kg and/or mean number), MSC concentration, delivery buffer, rate and duration of cell transfer, dose scheme, storage conditions and duration between dose preparation and administration, and miscellaneous handling details as listed
Where applicable: cryopreservation mode (aliquot or bag), cryomedia formulation, thaw procedures and cell recovery protocols.
4) MSC product characterization: culture media formulation, MSC population doubling level or passage, and quality control attributes including safety (sterility, endotoxin, mycoplasma, viral pathogens, karyotyping, residual FBS, tumorigenesis and others as listed), identity (morphology, surface marker profiles, multilineage potential, HLA profiling, clonogenicity and others as listed), functional attributes (PMBC suppression, cytokine expression, IDO-1 expression, T-cell proliferation, others as listed) and viability including post-thaw viability for cryopreserved products.
Results
Clinical Trial Parameters
The reports predominantly described Phase 1 clinical trials (44%) performed in the United States (90%). The therapeutic indication and clinical trial identifier associated with each publication are listed in Supplementary Table S1. The trials spanned a range of indications, including Graft versus Host Disease (GVHD), autoimmune diseases, cardiovascular injury and disease, sepsis, cancer and others (Supplementary Table S1). The majority of trials used bone marrow-derived (BM) MSCs (71%) delivered intravenously (IV; 40%).
All the clinical trial reports specified the MSC tissue of origin, whether the cell source was autologous or allogeneic, and the administration route (Supplementary Table S1; Tables 1, 2). Most of the trials (93%) reported the dose of MSCs in units of cells/kg patient weight, or mean cells per patient (Table 1). Three trials (7%) did not disclose or even quantify the number of cells per dose (Table 1). Twenty-three trials (51%) included dose-escalation schemes. Twenty-six trials (58%) used fixed doses rather than a dose/kg scheme (Table 1).
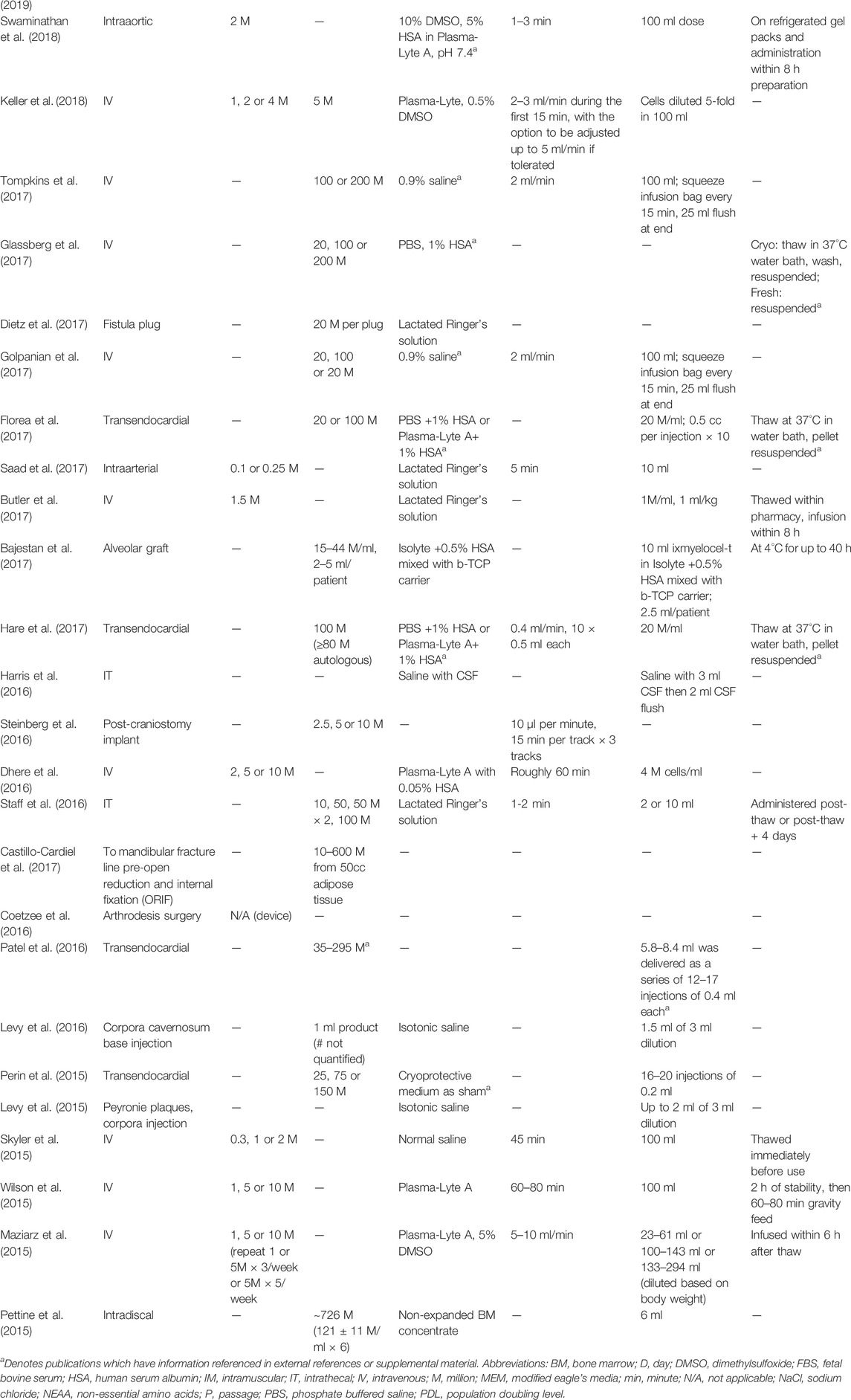
TABLE 1. Clinical trial publications inconsistently report details relevant to MSC dose preparation and bedside handling. Dashed lines represent unreported data.
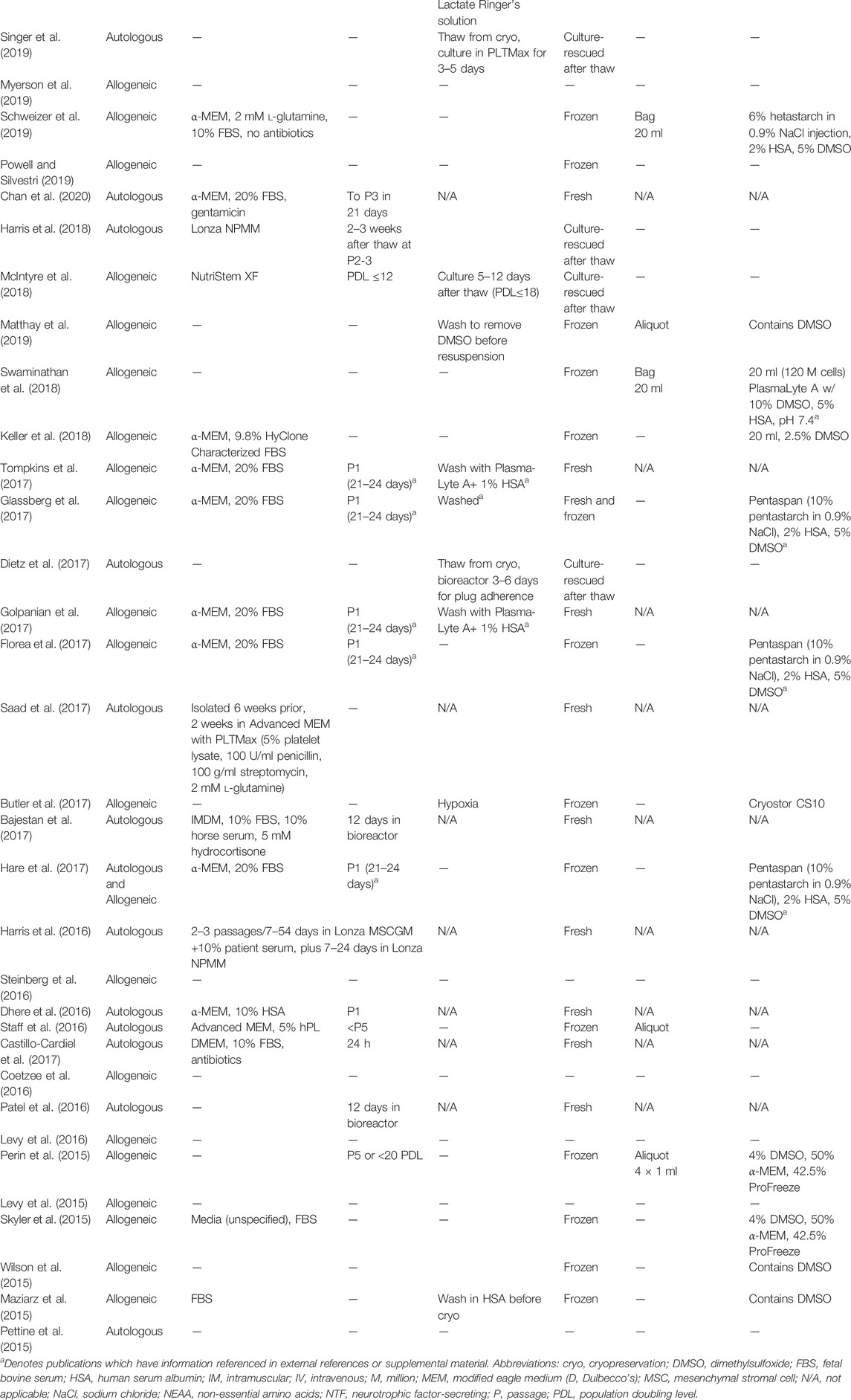
TABLE 2. Clinical trial publications underreport MSC manufacturing details. Dashed lines represent unreported data.
Reported MSC Product Characterization
Some form of cell product characterization was usually reported (89%), although the assessment criteria used was mixed (Supplementary Table S2). Viability was the most commonly reported metric, but the acceptable threshold ranged from 50 to 98% between trials (Supplementary Table S2). Studies using frozen cells stipulate whether viability assessments were made before cryopreservation, on a sample thawed lot, or per vial/bag at the time of use. Safety criteria, including tests for bacterial, fungal and viral contamination, chromosomal stability and residual FBS, were reported in 32 studies (71%; Supplementary Table S2). Thirty-three reports (73%) listed cell identity tests, including surface marker profiling, multi-lineage differentiation, and clonogenicity (Supplementary Table S2). Functional assessments were only reported for 12 clinical trials (27%) and included peripheral blood mononuclear cell (PBMC) and T-cell suppression, IDO-1 expression after IFN-γ stimulation, or secretion of other relevant proteins (Supplementary Table S2).
Details related to product formulation and handling were poorly documented. Twenty-five publications (55%) failed to fully define the medium in which the MSCs were expanded or administered, and 23 reports (51%) provided no information about the population doubling level (culture age) of the cells (Table 2). Of the 21 reports (47%) that provided some description of MSC expansion level, 10 (22%) only provided number of days in culture. Three (7%) reports provided discrete population doubling levels; the remaining studies reported passage number.
Reported MSC Product Handling
Most trials (62%) used previously frozen MSCs, while six publications (13%) did not stipulate whether their MSC products were derived from fresh cultures or had been thawed (Table 2). Of the 28 publications that used previously frozen MSC products, nearly half did not list the cryopreservation media (Table 2). Cryo-rescue procedures were essentially unreported, even though all but four trials administered MSCs directly following thaw without a recovery period or transfer of cells from cryopreservation media to delivery buffer/vehicle. Only seven papers stated that a wash step was performed, but no further details of the wash procedures were provided (Table 1).
Injection/infusion buffers were fairly well reported (91%) and predominately consisted of Plasma-Lyte, Plasma-Lyte A, Lactated Ringer’s solution, and saline with or without human serum albumin (HSA) or dimethylsulfoxide (DMSO) at varying concentrations (Table 1). Buffer solution was not used in an AD MSC bone allograft device in arthrodesis surgery (Coetzee et al., 2016; Myerson et al., 2019). One publication reported intradiscal injection of non-expanded BM concentrate (Pettine et al., 2015).
Duration of cell transfer was reported for the majority (78%) of trials that used IV infusion, either in minutes or ml/min (Table 1). Infusion time ranged from 5 min to 1 h. Of the trials using other administration routes, 28% reported the duration or rate of administration (Table 1). Most reports (84%) provided no information about the elapsed time from when the dose was prepared until cell transfer was complete (Table 1). Seven (16%) reports specified a maximum elapsed time from dose prep or thaw to administration, which ranged from 90 min to 12 h (Table 1). The three studies that included product handling protocols each used different methods; prepared doses were held in refrigeration, on cold packs or at room temperature (Table 1).
Discussion
MSCs are fundamentally responsive to subtle changes in their environment. MSCs respond to changes in atmospheric gases (Lin et al., 2014; Gorgun et al., 2021; Roemeling-Van Rhijn et al., 2013; Ejtehadifar et al., 2015; Kang et al., 2019; von Bahr et al., 2019), temperature (Stolzing et al., 2006; Kubrova et al., 2020; Shimoni et al., 2020), hydrostatic pressure (Steward et al., 2012; Becquart et al., 2016; Pattappa et al., 2019) and aggregation (Robb et al., 2019; Yuan et al., 2019; Burand et al., 2020; Xie et al., 2021). It is surprising then, that the steps and duration between dose preparation and delivery of MSC therapies are ill-defined and under-reported. We predict that bedside handling of MSC products may contribute substantially to the variability and reduced efficacy documented in clinical trials. Retrospective analysis to test this hypothesis, however, is currently impossible due to the absence of relevant information (Sart et al., 2014).
As example, MSCs have a natural tendency to self-assemble and form aggregates [reviewed in (Myerson et al., 2019)]. It has been reported that spontaneous aggregation can alter the immunosuppressive properties of MSCs, rendering them incapable of T cell suppression (Lanzoni et al, 2021). Thus, steps must be taken to control MSC aggregation between dose preparation and the completion of cell transfer. Even though cell doses were held for up to 12 h in the reviewed clinical trials, almost no measures to manage cell aggregation were described. Two studies reported squeezing the bag every 15 min during infusion, but no other reports described strategies to mitigate spontaneous aggregation. If the reports had documented the steps taken (if any) to prevent MSC aggregation during administration, retrospective analysis could potentially reveal whether implementing these strategies improves clinical outcomes.
Retrospective analysis could similarly be used to determine whether wash number, wash duration, centrifugation speed and buffer composition correlates with clinical outcomes. Thawed cells are fragile so thaw temperatures, duration and subsequent wash steps likely impact MSC fitness. The steps used to reconstitute frozen MSCs thawed immediately prior to administration were never reported. Moreover, few trials that thawed frozen MSCs immediately prior to administration stated the density at which the cells were cryopreserved, composition of the cryopreservation media, how the cells were thawed, whether or not they were washed, frequency of washing and the wash buffer used.
Currently, any changes in MSC fitness and performance in the hours between dose preparation and completion of infusion or injection is a black box devoid of data. To our knowledge, few studies have formally tested potential loss of function through sampling of MSC products during this window, or by recapitulating these conditions in laboratory tests (Pal et al., 2008; Chen et al., 2013; Niu et al., 2013). Intermittent bedside product testing admittedly is a logistical challenge. Thus, we suggest that clinical trial design include laboratory development of defined bedside procedures to ensure that the patient receives the same quality of MSC product that was prepared earlier and was subject to quality testing. Establishing and reporting these cell handling procedures, as well as any deviations from these protocols, may provide invaluable insight for retrospective analysis and ultimately ensure that patients consistently receive high quality MSC treatments.
There is a global movement towards standardization of MSC products. Such standardization includes development of tests to establish minimum cell performance criteria (Chinnadurai et al., 2018; Galipeau and Sensébé, 2018; Wiese et al., 2019b; Martin et al., 2019; Wiese and Braid, 2020a; Wiese and Braid, 2020b; Moll et al., 2020; Galipeau et al., 2021; Krampera and le Blanc, 2021), which are a critical to obtain regulatory approval for commercialization (Mendicino et al., 2014; Galipeau et al., 2015; de Wolf et al., 2017; Galipeau and Sensébé, 2018). Consistent with this movement, we found that most clinical trials reported some type of cell characterization. Viability and cell identity, based on accepted MSC cell surface profiles, were the most commonly reported tests. Consistent with a recent review of MSC characterization in clinical trials (Wilson et al., 2021), cell performance in functional assays or surrogate potency assays was documented infrequently, and performance thresholds were not disclosed. Post-thaw viability was also reported far less frequently than expected, especially since most of the trials used cryo-rescued cells.
We propose that ongoing global efforts to define the critical quality attributes of MSC ATMPs and subsequent release criteria be mindful of the need to identify markers and tests that can rapidly report MSC fitness and potency. These rapid-response markers will enable future development of in-process and bedside testing of MSC products, an important advancement in the realization of MSCs as commercially viable cell therapies.
Finally, retrospective analysis would be better enabled by establishing formal guidelines for clinical trial reporting. A recent clinical trial design by Baker et al. (2021) provides an excellent model to establish reproducible and transparent bedside cell handling procedures. We propose that clinical trial reports include all available cell characterization data and carefully document bedside handling of MSC products. Making this information readily available in the main report rather than citing other publications would facilitate accessibility for statistical analysis of large data sets and improve confidence that the data correlates with actual events and cell doses used in the trial.
Conclusion
We urge the MSC community to incorporate and report bedside MSC handling protocols and best practices in clinical trial design and reporting. The notable lack of information and data surrounding how these exquisitely responsive cells are treated when the cells are most vulnerable is not likely an issue of propriety. Rather, this aspect of the cell therapy journey from vial to vein appears to have been designated as arbitrary, a classification that we argue is flawed. Documenting and reporting bedside cell processing and handling procedures will aid effective retrospective analysis of clinical trial outcomes and expedite the commercialization of MSC products.
Author Contributions
LB conceived the manuscript. DW and CW contributed to literature search and analysis. DW and LB prepared the manuscript with assistance from CW. LB generated financial support for the research. All authors approved the final manuscript submitted for consideration.
Funding
This work was funded in part by the National Research Council of Canada Industrial Research Assistance Program Project 914919.
Conflict of Interest
LB, DW, and CW were employed by the company Aurora BioSolutions Inc.
The authors declare that the research was conducted in the absence of any commercial or financial relationships that could be construed as a potential conflict of interest with the subject matter.
Publisher’s Note
All claims expressed in this article are solely those of the authors and do not necessarily represent those of their affiliated organizations, or those of the publisher, the editors and the reviewers. Any product that may be evaluated in this article, or claim that may be made by its manufacturer, is not guaranteed or endorsed by the publisher.
Acknowledgments
The authors thank Brendon DeGroot for assistance with updating the literature search.
Supplementary Material
The Supplementary Material for this article can be found online at: https://www.frontiersin.org/articles/10.3389/fcell.2022.867426/full#supplementary-material
Abbreviations
CFU, colony forming units; cryo, cryopreservation; ELISA, enzyme linked immunosorbent assay; FBS, fetal bovine serum; h, hour; HLA, human leukocyte antigen; IDO, indoleamine 2,3-deoxygenase; IFN, interferon; IL, interleukin; NTF, neurotrophic factor; PBMC, peripheral blood mononuclear cells; PCR, polymerase chain reaction; QC, quality control; TNF, tumor necrosis factor.
References
Amirdelfan, K., Bae, H., McJunkin, T., DePalma, M., Kim, K., Beckworth, W. J., et al. (2021). Allogeneic Mesenchymal Precursor Cells Treatment for Chronic Low Back Pain Associated with Degenerative Disc Disease: a Prospective Randomized, Placebo-Controlled 36-month Study of Safety and Efficacy. Spine J. 21 (2). doi:10.1016/j.spinee.2020.10.004
Bajestan, M. N., Rajan, A., Edwards, S. P., Aronovich, S., Cevidanes, L. H. S., Polymeri, A., et al. (2017). Stem Cell Therapy for Reconstruction of Alveolar Cleft and Trauma Defects in Adults: A Randomized Controlled, Clinical Trial. Clin. Implant Dent Relat. Res. 19 (5), 793–801. doi:10.1111/cid.12506
Baker, E. K., Wallace, E. M., Davis, P. G., Malhotra, A., Jacobs, S. E., Hooper, S. B., et al. (2021). A Protocol for Cell Therapy Infusion in Neonates. Stem Cell Transl Med 10 (5), 773–780. doi:10.1002/sctm.20-0281
Becquart, P., Cruel, M., Hoc, T., Sudre, L., Pernelle, K., Bizios, R., et al. (2016). Human Mesenchymal Stem Cell Responses to Hydrostatic Pressure and Shear Stress. Eur. Cel Mater 31, 160–73. doi:10.22203/ecm.v031a11
Berry, J. D., Cudkowicz, M. E., Windebank, A. J., Staff, N. P., Owegi, M., Nicholson, K., et al. (2019). NurOwn, Phase 2, Randomized, Clinical Trial in Patients with ALS: Safety, Clinical, and Biomarker Results. Neurology 93 (24), e2294. doi:10.1212/WNL.0000000000008620
Bolli, R., Hare, J. M., March, K. L., Pepine, C. J., Willerson, J. T., Perin, E. C., et al. (2018). Rationale and Design of the CONCERT-HF Trial (Combination of Mesenchymal and C-Kit + Cardiac Stem Cells as Regenerative Therapy for Heart Failure). Circ. Res. 122 (12), 1703–1715. doi:10.1161/circresaha.118.312978
Bolli, R., Mitrani, R. D., Hare, J. M., Pepine, C. J., Perin, E. C., Willerson, J. T., et al. (2021). A Phase II Study of Autologous Mesenchymal Stromal Cells and C‐kit Positive Cardiac Cells, Alone or in Combination, in Patients with Ischaemic Heart Failure: the CCTRN CONCERT‐HF Trial. Eur. J. Heart Fail. 23 (4), 661–674. doi:10.1002/ejhf.2178
Braid, L. R., Wood, C. A., Wiese, D. M., and Ford, B. N. (2018). Intramuscular Administration Potentiates Extended Dwell Time of Mesenchymal Stromal Cells Compared to Other Routes. Cytotherapy 20, 232–244. doi:10.1016/j.jcyt.2017.09.013
Burand, A. J., Di, L., Boland, L. K., Boyt, D. T., Schrodt, M. V., Santillan, D. A., et al. (2020). Aggregation of Human Mesenchymal Stromal Cells Eliminates Their Ability to Suppress Human T Cells. Front. Immunol. 11, 143. doi:10.3389/fimmu.2020.00143
Butler, J., Epstein, S. E., Greene, S. J., Quyyumi, A. A., Sikora, S., Kim, R. J., et al. (2017). Intravenous Allogeneic Mesenchymal Stem Cells for Nonischemic Cardiomyopathy: Safety and Efficacy Results of a Phase II-A Randomized Trial. Circ. Res. 120 (2), 332–340. doi:10.1161/CIRCRESAHA.116.309717
Castillo-Cardiel, G., López-Echaury, A. C., Saucedo-Ortiz, J. A., Fuentes-Orozco, C., Michel-Espinoza, L. R., Irusteta-Jiménez, L., et al. (2017). Bone Regeneration in Mandibular Fractures after the Application of Autologous Mesenchymal Stem Cells, a Randomized Clinical Trial. Dent Traumatol. 33 (1), 38–44. doi:10.1111/edt.12303
Chahal, J., Gómez-Aristizábal, A., Shestopaloff, K., Bhatt, S., Chaboureau, A., Fazio, A., et al. (2019). Bone Marrow Mesenchymal Stromal Cell Treatment in Patients with Osteoarthritis Results in Overall Improvement in Pain and Symptoms and Reduces Synovial Inflammation. Stem Cell Transl Med 8 (8), 746–757. doi:10.1002/sctm.18-0183
Chan, J. L., Miller, J. G., Zhou, Y., Robey, P. G., Stroncek, D. F., Arai, A. E., et al. (2020). “Intramyocardial Bone Marrow Stem Cells in Patients Undergoing Cardiac Surgical Revascularization,” in Annals of Thoracic Surgery. doi:10.1016/j.athoracsur.2019.07.093
Chen, Y., Yu, B., Xue, G., Zhao, J., Li, R. K., Liu, Z., et al. (2013). Effects of Storage Solutions on the Viability of Human Umbilical Cord Mesenchymal Stem Cells for Transplantation. Cel Transpl. 22 (6), 1075–86. doi:10.3727/096368912X657602
Chinnadurai, R., Rajan, D., Qayed, M., Arafat, D., Garcia, M., Liu, Y., et al. (2018). Potency Analysis of Mesenchymal Stromal Cells Using a Combinatorial Assay Matrix Approach. Cel Rep.
Coetzee, J. C., Myerson, M. S., and Anderson, J. G. (2016). The Use of Allostem in Subtalar Fusions. Foot Ankle Clin. 21. doi:10.1016/j.fcl.2016.07.011
Davies, J. E., Walker, J. T., and Keating, A. (2017). Concise Review: Wharton's Jelly: The Rich, but Enigmatic, Source of Mesenchymal Stromal Cells. Stem Cell Translational Med. 6 (7), 1620–1630. doi:10.1002/sctm.16-0492
de Wolf, C., van de Bovenkamp, M., and Hoefnagel, M. (2017). Regulatory Perspective on In Vitro Potency Assays for Human Mesenchymal Stromal Cells Used in Immunotherapy. Cytotherapy. doi:10.1016/j.jcyt.2017.03.076
Dhere, T., Copland, I., Garcia, M., Chiang, K. Y., Chinnadurai, R., Prasad, M., et al. (2016). The Safety of Autologous and Metabolically Fit Bone Marrow Mesenchymal Stromal Cells in Medically Refractory Crohn's Disease - a Phase 1 Trial with Three Doses. Aliment. Pharmacol. Ther. 44 (5), 471–81. doi:10.1111/apt.13717
Dietz, A. B., Dozois, E. J., Fletcher, J. G., Butler, G. W., Radel, D., Lightner, A. L., et al. (2017). Autologous Mesenchymal Stem Cells, Applied in a Bioabsorbable Matrix, for Treatment of Perianal Fistulas in Patients with Crohn's Disease. Gastroenterology 153 (1), 59–e2. doi:10.1053/j.gastro.2017.04.001
Dozois, E. J., Lightner, A. L., Mathis, K. L., Chua, H. K., Kelley, S. R., Fletcher, J. G., et al. (2019). Early Results of a Phase I Trial Using an Adipose-Derived Mesenchymal Stem Cell-Coated Fistula Plug for the Treatment of Transsphincteric Cryptoglandular Fistulas. Dis. Colon Rectum 62 (5), 615–622. doi:10.1097/DCR.0000000000001333
Ejtehadifar, M., Shamsasenjan, K., Movassaghpour, A., Akbarzadehlaleh, P., Dehdilani, N., Abbasi, P., et al. (2015). The Effect of Hypoxia on Mesenchymal Stem Cell Biology. Adv. Pharm. Bull. 5. doi:10.15171/apb.2015.021
Florea, V., Rieger, A. C., DiFede, D. L., El-Khorazaty, J., Natsumeda, M., Banerjee, M. N., et al. (2017). Dose Comparison Study of Allogeneic Mesenchymal Stem Cells in Patients with Ischemic Cardiomyopathy (The TRIDENT Study). Circ. Res. 121 (11), 1279–1290. doi:10.1161/CIRCRESAHA.117.311827
Galipeau, J., Krampera, M., Barrett, J., Dazzi, F., Deans, R. J., DeBruijn, J., et al. (2015). International Society for Cellular Therapy Perspective on Immune Functional Assays for Mesenchymal Stromal Cells as Potency Release Criterion for Advanced Phase Clinical Trials. Cytotherapy.
Galipeau, J., Krampera, M., Leblanc, K., Nolta, J. A., Phinney, D. G., Shi, Y., et al. (2021). Mesenchymal Stromal Cell Variables Influencing Clinical Potency: the Impact of Viability, Fitness, Route of Administration and Host Predisposition. Cytotherapy 23 (5). doi:10.1016/j.jcyt.2020.11.007
Galipeau, J., and Sensébé, L. (2018). Mesenchymal Stromal Cells: Clinical Challenges and Therapeutic Opportunities. Cell Stem Cell 22 (6), 824–833. doi:10.1016/j.stem.2018.05.004
Giri, J., and Galipeau, J. (2020). Mesenchymal Stromal Cell Therapeutic Potency Is Dependent upon Viability, Route of Delivery, and Immune Match. Blood Adv. 4 (9), 1987–1997. doi:10.1182/bloodadvances.2020001711
Glassberg, M. K., Minkiewicz, J., Toonkel, R. L., Simonet, E. S., Rubio, G. A., DiFede, D., et al. (2017). Allogeneic Human Mesenchymal Stem Cells in Patients with Idiopathic Pulmonary Fibrosis via Intravenous Delivery (AETHER): A Phase I Safety Clinical Trial. Chest 151. doi:10.1016/j.chest.2016.10.061
Golpanian, S., Difede, D. L., Khan, A., Schulman, I. H., Landin, A. M., Tompkins, B. A., et al. (2017). Allogeneic Human Mesenchymal Stem Cell Infusions for Aging Frailty. J. Gerontol. A. Biol. Sci. Med. Sci. 72 (11), 1505–1512. doi:10.1093/gerona/glx056
Gorgun, C., Ceresa, D., Lesage, R., Villa, F., Reverberi, D., Balbi, C., et al. (2021). Dissecting the Effects of Preconditioning with Inflammatory Cytokines and Hypoxia on the Angiogenic Potential of Mesenchymal Stromal Cell (MSC)-derived Soluble Proteins and Extracellular Vesicles (EVs). Biomaterials, 269.
Hare, J. M., DiFede, D. L., Rieger, A. C., Florea, V., Landin, A. M., El-Khorazaty, J., et al. (2017). Randomized Comparison of Allogeneic versus Autologous Mesenchymal Stem Cells for Nonischemic Dilated Cardiomyopathy: POSEIDON-DCM Trial. J. Am. Coll. Cardiol. 69 (5), 526–537. doi:10.1016/j.jacc.2016.11.009
Harris, V. K., Stark, J., Vyshkina, T., Blackshear, L., Joo, G., Stefanova, V., et al. (2018). Phase I Trial of Intrathecal Mesenchymal Stem Cell-Derived Neural Progenitors in Progressive Multiple Sclerosis. EBioMedicine 29, 23–30. doi:10.1016/j.ebiom.2018.02.002
Harris, V. K., Vyshkina, T., and Sadiq, S. A. (2016). Clinical Safety of Intrathecal Administration of Mesenchymal Stromal Cell-Derived Neural Progenitors in Multiple Sclerosis. Cytotherapy 18 (12), 1476–1482. doi:10.1016/j.jcyt.2016.08.007
Kang, T. Y., Kwon, J. S., Kumar, N., Choi, E. H., and Kim, K. M. (2019). Effects of a Non-thermal Atmospheric Pressure Plasma Jet with Different Gas Sources and Modes of Treatment on the Fate of Human Mesenchymal Stem Cells. Appl. Sci. (Switzerland) 9 (22). doi:10.3390/app9224819
Kebriaei, P., Hayes, J., Daly, A., Uberti, J., Marks, D. I., Soiffer, R., et al. (2020). A Phase 3 Randomized Study of Remestemcel-L versus Placebo Added to Second-Line Therapy in Patients with Steroid-Refractory Acute Graft-Versus-Host Disease. Biol. Blood Marrow Transpl. 26 (5), 835–844. doi:10.1016/j.bbmt.2019.08.029
Keller, C. A., Gonwa, T. A., Hodge, D. O., Hei, D. J., Centanni, J. M., and Zubair, A. C. (2018). Feasibility, Safety, and Tolerance of Mesenchymal Stem Cell Therapy for Obstructive Chronic Lung Allograft Dysfunction. Stem Cell Transl Med 7 (2), 161–167. doi:10.1002/sctm.17-0198
Krampera, M., and le Blanc, K. (2021). Mesenchymal Stromal Cells: Putative Microenvironmental Modulators Become Cell Therapy. Cell Stem Cell 28. doi:10.1016/j.stem.2021.09.006
Kubrova, E., Qu, W., Galvan, M. L., Paradise, C. R., Yang, J., Dietz, A. B., et al. (2020). Hypothermia and Nutrient Deprivation Alter Viability of Human Adipose-Derived Mesenchymal Stem Cells. Gene 722, 144058. doi:10.1016/j.gene.2019.144058
Kurtzberg, J., Abdel-Azim, H., Carpenter, P., Chaudhury, S., Horn, B., Mahadeo, K., et al. (2020). A Phase 3, Single-Arm, Prospective Study of Remestemcel-L, Ex Vivo Culture-Expanded Adult Human Mesenchymal Stromal Cells for the Treatment of Pediatric Patients Who Failed to Respond to Steroid Treatment for Acute Graft-Versus-Host Disease. Biol. Blood Marrow Transpl. 26 (5), 845–854. doi:10.1016/j.bbmt.2020.01.018
Lanzoni, G., Linetsky, E., Correa, D., Messinger Cayetano, S., Alvarez, R. A., Kouroupis, D., et al. (2021). Umbilical Cord Mesenchymal Stem Cells for COVID-19 Acute Respiratory Distress Syndrome: A Double-Blind, Phase 1/2a, Randomized Controlled Trial. Stem Cell Translational Med. 10 (5), 660–673. doi:10.1002/sctm.20-0472
Levy, J. A., Marchand, M., Iorio, L., Cassini, W., and Zahalsky, M. P. (2016). Determining the Feasibility of Managing Erectile Dysfunction in Humans with Placental-Derived Stem Cells. J. Am. Osteopath Assoc. 116 (1), e1–5. doi:10.7556/jaoa.2016.007
Levy, J. A., Marchand, M., Iorio, L., Zribi, G., and Zahalsky, M. P. (2015). Effects of Stem Cell Treatment in Human Patients with Peyronie Disease. J. Am. Osteopath Assoc. 115 (10), e8–13. doi:10.7556/jaoa.2015.124
Levy, M. L., Crawford, J. R., Dib, N., Verkh, L., Tankovich, N., and Cramer, S. C. (2019). Phase I/II Study of Safety and Preliminary Efficacy of Intravenous Allogeneic Mesenchymal Stem Cells in Chronic Stroke. Stroke 50 (10), 2835–2841. doi:10.1161/STROKEAHA.119.026318
Levy, O., Kuai, R., Siren, E. M. J., Bhere, D., Milton, Y., Nissar, N., et al. (2020). Shattering Barriers toward Clinically Meaningful MSC Therapies. Sci. Adv. 6 (30), eaba6884. doi:10.1126/sciadv.aba6884
Lin, S. S., Ueng, S. W., Niu, C. C., Yuan, L. J., Yang, C. Y., Chen, W. J., et al. (2014). Effects of Hyperbaric Oxygen on the Osteogenic Differentiation of Mesenchymal Stem Cells. BMC Musculoskelet. Disord. 15 (1), 56. doi:10.1186/1471-2474-15-56
Martin, I., Galipeau, J., Kessler, C., Le Blanc, K., and Dazzi, F. (2019). Challenges for Mesenchymal Stromal Cell Therapies. Sci. Transl Med. 11 (480). doi:10.1126/scitranslmed.aat2189
Matthay, M. A., Calfee, C. S., Zhuo, H., Thompson, B. T., Wilson, J. G., Levitt, J. E., et al. (2019). Treatment with Allogeneic Mesenchymal Stromal Cells for Moderate to Severe Acute Respiratory Distress Syndrome (START Study): a Randomised Phase 2a Safety Trial. Lancet Respir. Med. 7 (2), 154–162. doi:10.1016/S2213-2600(18)30418-1
Maziarz, R. T., Devos, T., Bachier, C. R., Goldstein, S. C., Leis, J. F., Devine, S. M., et al. (2015). Single and Multiple Dose Multistem (Multipotent Adult Progenitor Cell) Therapy Prophylaxis of Acute Graft-Versus-Host Disease in Myeloablative Allogeneic Hematopoietic Cell Transplantation: A Phase 1 Trial. Biol. Blood Marrow Transpl. 21 (4), 720–8. doi:10.1016/j.bbmt.2014.12.025
McIntyre, L. A., Stewart, D. J., Mei, S. H. J., Courtman, D., Watpool, I., Granton, J., et al. (2018). Cellular Immunotherapy for Septic Shock. A Phase I Clinical Trial. Am. J. Respir. Crit. Care Med. 197 (3), 337–347. doi:10.1164/rccm.201705-1006OC
Mendicino, M., Bailey, A. M., Wonnacott, K., Puri, R. K., and Bauer, S. R. (2014). MSC-based Product Characterization for Clinical Trials: An FDA Perspective. Cell Stem Cell 14. doi:10.1016/j.stem.2014.01.013
Mennan, C., Garcia, J., Roberts, S., Hulme, C., and Wright, K. (2019). A Comprehensive Characterisation of Large-Scale Expanded Human Bone Marrow and Umbilical Cord Mesenchymal Stem Cells. Stem Cel Res Ther 10 (1), 99–15. doi:10.1186/s13287-019-1202-4
Moll, G., Drzeniek, N., Kamhieh-Milz, J., Geissler, S., Volk, H. D., and Reinke, P. (2020). MSC Therapies for COVID-19: Importance of Patient Coagulopathy, Thromboprophylaxis, Cell Product Quality and Mode of Delivery for Treatment Safety and Efficacy. Front. Immunol. 11, 1091. doi:10.3389/fimmu.2020.01091
Myerson, C. L., Myerson, M. S., Coetzee, J. C., Stone McGaver, R., and Giveans, M. R. (2019). Subtalar Arthrodesis with Use of Adipose-Derived Cellular Bone Matrix Compared with Autologous Bone Graft: A Multicenter, Randomized Controlled Trial. J. Bone Jt. Surg Am 101 (21), 1904–1911. doi:10.2106/JBJS.18.01300
Niu, Y. H., Chen, Y., Zhang, J. L., Lei, X., Dong, Y. T., Cui, L., et al. (2013). Oxidative Stress Effect on Viability of Umbilical Cord-Derived Mesenchymal Stem Cells in Storage Solution of Transplantation. Chin. J. Tissue Eng. Res. 17 (32).
Pal, R., Hanwate, M., and Totey, S. M. (2008). Effect of Holding Time, Temperature and Different Parenteral Solutions on Viability and Functionality of Adult Bone Marrow-Derived Mesenchymal Stem Cells before Transplantation. J. Tissue Eng. Regen. Med. 2 (7), 436–44. doi:10.1002/term.109
Patel, A. N., Henry, T. D., Quyyumi, A. A., Schaer, G. L., Anderson, R. D., Toma, C., et al. (2016). Ixmyelocel-T for Patients with Ischaemic Heart Failure: a Prospective Randomised Double-Blind Trial. Lancet 387 (10036), 2412–21. doi:10.1016/S0140-6736(16)30137-4
Pattappa, G., Zellner, J., Johnstone, B., Docheva, D., and Angele, P. (2019). Cells under Pressure - the Relationship between Hydrostatic Pressure and Mesenchymal Stem Cell Chondrogenesis. Eur. Cell Mater. 37.
Perin, E. C., Borow, K. M., Silva, G. V., DeMaria, A. N., Marroquin, O. C., Huang, P. P., et al. (2015). A Phase II Dose-Escalation Study of Allogeneic Mesenchymal Precursor Cells in Patients with Ischemic or Nonischemic Heart Failure. Circ. Res. 117 (6), 576–84. doi:10.1161/CIRCRESAHA.115.306332
Pettine, K. A., Murphy, M. B., Suzuki, R. K., and Sand, T. T. (2015). Percutaneous Injection of Autologous Bone Marrow Concentrate Cells Significantly Reduces Lumbar Discogenic Pain through 12 Months. Stem Cells 33 (1), 146–56. doi:10.1002/stem.1845
Powell, S. B., and Silvestri, J. M. (2019). Safety of Intratracheal Administration of Human Umbilical Cord Blood Derived Mesenchymal Stromal Cells in Extremely Low Birth Weight Preterm Infants. J. Pediatr. 210, 209–e2. doi:10.1016/j.jpeds.2019.02.029
Robb, K., Gómez-Aristizábal, A., Gandhi, R., and Viswanathan, S. (2019). A Culture Engineering Strategy to Enhance Mesenchymal Stromal Cells for Treatment of Osteoarthritis. Osteoarthritis and Cartilage 27. doi:10.1016/j.joca.2019.02.447
Roemeling-Van Rhijn, M., Mensah, F. K., Korevaar, S. S., Leijs, M. J., van Osch, G. J., IJzermans, J. N., et al. (2013). Effects of Hypoxia on the Immunomodulatory Properties of Adipose Tissue-Derived Mesenchymal Stem Cells. Front. Immunol. 4 (JUL), 203. doi:10.3389/fimmu.2013.00203
Saad, A., Dietz, A. B., Herrmann, S. M. S., Hickson, L. J., Glockner, J. F., McKusick, M. A., et al. (2017). Autologous Mesenchymal Stem Cells Increase Cortical Perfusion in Renovascular Disease. J. Am. Soc. Nephrol. 28 (9), 2777–2785. doi:10.1681/ASN.2017020151
Sart, S., Tsai, A. C., Li, Y., and Ma, T. (2014). Three-dimensional Aggregates of Mesenchymal Stem Cells: Cellular Mechanisms, Biological Properties, and Applications. Tissue Eng. - B: Rev. 20. doi:10.1089/ten.teb.2013.0537
Schlosser, K., Wang, J. P., dos Santos, C., Walley, K. R., Marshall, J., Fergusson, D. A., et al. (2019). Effects of Mesenchymal Stem Cell Treatment on Systemic Cytokine Levels in a Phase 1 Dose Escalation Safety Trial of Septic Shock Patients. Crit. Care Med. 47 (7), 918–925. doi:10.1097/CCM.0000000000003657
Schweizer, M. T., Wang, H., Bivalacqua, T. J., Partin, A. W., Lim, S. J., Chapman, C., et al. (2019). A Phase I Study to Assess the Safety and Cancer-Homing Ability of Allogeneic Bone Marrow-Derived Mesenchymal Stem Cells in Men with Localized Prostate Cancer. Stem Cell Transl Med 8 (5), 441–449. doi:10.1002/sctm.18-0230
Shimoni, C., Goldstein, M., Ribarski-Chorev, I., Schauten, I., Nir, D., Strauss, C., et al. (2020). Heat Shock Alters Mesenchymal Stem Cell Identity and Induces Premature Senescence. Front Cel Dev Biol 8, 565970. doi:10.3389/fcell.2020.565970
Singer, W., Dietz, A. B., Zeller, A. D., Gehrking, T. L., Schmelzer, J. D., Schmeichel, A. M., et al. (2019). Intrathecal Administration of Autologous Mesenchymal Stem Cells in Multiple System Atrophy. Neurology 93 (1), e77. doi:10.1212/WNL.0000000000007720
Skyler, J. S., Fonseca, V. A., Segal, K. R., and Rosenstock, J. (2015). Allogeneic Mesenchymal Precursor Cells in Type 2 Diabetes: A Randomized, Placebo-Controlled, Dose-Escalation Safety and Tolerability Pilot Study. Diabetes Care 38 (9), 1742–9. doi:10.2337/dc14-2830
Soder, R. P., Dawn, B., Weiss, M. L., Dunavin, N., Weir, S., Mitchell, J., et al. (2020). A Phase I Study to Evaluate Two Doses of Wharton's Jelly-Derived Mesenchymal Stromal Cells for the Treatment of De Novo High-Risk or Steroid-Refractory Acute Graft versus Host Disease. Stem Cel Rev Rep 16 (5), 979–991. doi:10.1007/s12015-020-10015-8
Staff, N. P., Madigan, N. N., Morris, J., Jentoft, M., Sorenson, E. J., Butler, G., et al. (2016). Safety of Intrathecal Autologous Adipose-Derived Mesenchymal Stromal Cells in Patients with ALS. Neurology 87 (21), 2230–2234. doi:10.1212/WNL.0000000000003359
Steinberg, G. K., Kondziolka, D., Bates, D., Lunsford, L. D., Coburn, M. L., Billigen, J. B., et al. (2016). Response by Steinberg et al to Letter Regarding Article, "Clinical Outcomes of Transplanted Modified Bone Marrow-Derived Mesenchymal Stem Cells in Stroke: A Phase 1/2A Study". Stroke 47 (7), e269. doi:10.1161/STROKEAHA.116.015209
Steward, A. J., Thorpe, S. D., Vinardell, T., Buckley, C. T., Wagner, D. R., and Kelly, D. J. (2012). Cell-matrix Interactions Regulate Mesenchymal Stem Cell Response to Hydrostatic Pressure. Acta Biomater. 8 (6), 2153–9. doi:10.1016/j.actbio.2012.03.016
Stolzing, A., Sethe, S., and Scutt, A. M. (2006). Stressed Stem Cells: Temperature Response in Aged Mesenchymal Stem Cells. Stem Cell Dev 15 (4), 478–87. doi:10.1089/scd.2006.15.478
Swaminathan, M., Stafford-Smith, M., Chertow, G. M., Warnock, D. G., Paragamian, V., Brenner, R. M., et al. (2018). Allogeneic Mesenchymal Stem Cells for Treatment of AKI after Cardiac Surgery. J. Am. Soc. Nephrol. 29 (1), 260–267. doi:10.1681/ASN.2016101150
Tompkins, B. A., Difede, D. L., Khan, A., Landin, A. M., Schulman, I. H., Pujol, M. V., et al. (2017). Allogeneic Mesenchymal Stem Cells Ameliorate Aging Frailty: A Phase II Randomized, Double-Blind, Placebo-Controlled Clinical Trial. J. Gerontol. A. Biol. Sci. Med. Sci. 72 (11), 1513–1522. doi:10.1093/gerona/glx137
Viswanathan, S., Shi, Y., Galipeau, J., Krampera, M., Leblanc, K., Martin, I., et al. (2019). Mesenchymal Stem versus Stromal Cells: International Society for Cell & Gene Therapy (ISCT®) Mesenchymal Stromal Cell Committee Position Statement on Nomenclature. Cytotherapy.
von Bahr, V., Millar, J. E., Malfertheiner, M. V., Ki, K. K., Passmore, M. R., Bartnikowski, N., et al. (2019). Mesenchymal Stem Cells May Ameliorate Inflammation in an Ex Vivo Model of Extracorporeal Membrane Oxygenation. Perfusion 34 (1_Suppl. l), 15–21. doi:10.1177/0267659119830857
Wiese, D., and Braid, L. R. (2020). Towards a Consensus Potency Assay for Mesenchymal Stromal Cells: Identification of Activation Markers Reliable across media Formulations, Donor and Tissue Source. Cytotherapy 22 (5). doi:10.1016/j.jcyt.2020.03.109
Wiese, D., Ford, B., and Braid, L. R. (2019). Towards a Consensus Potency Assay for Mesenchymal Stromal Cells: a Matrix Analysis of Cell Source, Donor Variability and Inflammatory Stimuli to Refine Surrogate Markers of Immunomodulation. Cytotherapy 21 (5). doi:10.1016/j.jcyt.2019.03.322
Wiese, D. M., and Braid, L. R. (2020). Transcriptome Profiles Acquired during Cell Expansion and Licensing Validate Mesenchymal Stromal Cell Lineage Genes. Stem Cel Res. Ther. 2020. doi:10.1186/s13287-020-01873-7
Wiese, D. M., Ruttan, C. C., Wood, C. A., Ford, B. N., and Braid, L. R. (2019). Accumulating Transcriptome Drift Precedes Cell Aging in Human Umbilical Cord-Derived Mesenchymal Stromal Cells Serially Cultured to Replicative Senescence. Stem Cell Transl Med 8 (9), 945–958. doi:10.1002/sctm.18-0246
Wilson, A. J., Rand, E., Webster, A. J., and Genever, P. G. (2021). Characterisation of Mesenchymal Stromal Cells in Clinical Trial Reports: Analysis of Published Descriptors. Stem Cel Res Ther 12 (1), 360. doi:10.1186/s13287-021-02435-1
Wilson, J. G., Liu, K. D., Zhuo, H., Caballero, L., McMillan, M., Fang, X., et al. (2015). Mesenchymal Stem (Stromal) Cells for Treatment of ARDS: A Phase 1 Clinical Trial. Lancet Respir. Med. 3 (1), 24–32. doi:10.1016/S2213-2600(14)70291-7
Wright, A., Arthaud-Day, M. L., and Weiss, M. L. (2021). Therapeutic Use of Mesenchymal Stromal Cells: The Need for Inclusive Characterization Guidelines to Accommodate All Tissue Sources and Species. Front. Cel Developmental Biol. 9. doi:10.3389/fcell.2021.632717
Xie, A. W., Zacharias, N. A., Binder, B. Y. K., and Murphy, W. L. (2021). Controlled Aggregation Enhances Immunomodulatory Potential of Mesenchymal Stromal Cell Aggregates. Stem Cell Transl Med 10 (8), 1184–1201. doi:10.1002/sctm.19-0414
Yau, T. M., Pagani, F. D., Mancini, D. M., Chang, H. L., Lala, A., Woo, Y. J., et al. (2019). Intramyocardial Injection of Mesenchymal Precursor Cells and Successful Temporary Weaning from Left Ventricular Assist Device Support in Patients with Advanced Heart Failure: A Randomized Clinical Trial. JAMA 321 (12), 1176–1186. doi:10.1001/jama.2019.2341
Yin, J. Q., Zhu, J., and Ankrum, J. A. (2019). Manufacturing of Primed Mesenchymal Stromal Cells for Therapy. Nat. Biomed. Eng.doi:10.1038/s41551-018-0325-8
Keywords: mesenchymal stromal (stem) cell (MSC), ATMP, clinical trial, retrospective analysis, cell therapy (CT), regulatory approval, cell fitness, cell potency
Citation: Wiese DM, Wood CA and Braid LR (2022) From Vial to Vein: Crucial Gaps in Mesenchymal Stromal Cell Clinical Trial Reporting. Front. Cell Dev. Biol. 10:867426. doi: 10.3389/fcell.2022.867426
Received: 01 February 2022; Accepted: 07 March 2022;
Published: 13 April 2022.
Edited by:
Mayasari Lim, Fujifilm Irvine Scientific, Inc., United StatesReviewed by:
Courtney Anne McDonald, Hudson Institute of Medical Research, AustraliaCopyright © 2022 Wiese, Wood and Braid. This is an open-access article distributed under the terms of the Creative Commons Attribution License (CC BY). The use, distribution or reproduction in other forums is permitted, provided the original author(s) and the copyright owner(s) are credited and that the original publication in this journal is cited, in accordance with accepted academic practice. No use, distribution or reproduction is permitted which does not comply with these terms.
*Correspondence: Lorena R. Braid, bG9yZW5hQGF1cm9yYWJpb3NvbHV0aW9ucy5jb20=, bHJicmFpZEBzZnUuY2E=