- 1Institut Cochin, INSERM, CNRS, Université de Paris, Paris, France
- 2Ecole Normale Supérieure (ENS), Université Paris Sciences et Lettres (PSL), CNRS, Sorbonne Université, Université de Paris, Paris, France
- 3Department of Histo-embryology, Genetics and Developmental Biology, Shanghai Jiao Tong University School of Medicine, Shanghai, China
- 4Service d’histologie, d’embryologie, Biologie de la Reproduction, AP-HP, Hôpital Cochin, Paris, France
In mammalian fertilization, the link between the oocyte plasma membrane and underneath cytoskeleton has often been associated to key elements of successful gamete fusion, like microvilli shaping or CD9 function, but its effective role has poorly been studied. EWI-2 and EWI-F as cis partners of CD9, and ERM proteins (Ezrin, Radixin and Moesin) that both attach to the actin cytoskeleton and to the EWI are part of the molecules that make the link between the oocyte membrane and its cytoskeleton. This study aims to assay through siRNA inhibition, the involvement of these ERM and EWI molecules in mouse fertilization, their role in the microvilli morphology of the egg but also their possible contribution to the cortical tension, a parameter that reflects the mechanical behavior of the oocyte cortex. Whereas inhibiting separately the expression of each protein had no effect on fertilization, the combined inhibition of either EWI-2/EWI-F or the three ERM triggered a significant decrease of the fertilization index. This inhibition seems to correlate with an increase in the radius of curvature of the oocyte microvilli. It also causes a decrease of the oocyte cortical tension. These results show the importance of EWI-2 and EWI–F and ERM proteins in the smooth running of a fertilization event and support their involvement in the microvilli architecture of the oocyte and in its mechanical properties.
Introduction
Fertilization is the process of union of two gametes. It occurs in several steps and culminates by the encounter of highly differentiated gametes. This interaction follows specific steps including recognition, adhesion and fusion. On the mammalian oocyte, JUNO and CD9 are the only two molecules known to be essential for adhesion/fusion during fertilization (Kaji et al., 2000; Le Naour et al., 2000; Miyado et al., 2000; Bianchi et al., 2014). While JUNO and its sperm partner IZUMO1 are now clearly identified as adhesion molecules (Aydin et al., 2016), the mechanism by which CD9 functions is still not completely understood, with most evidence pointing to an architectural or organizational role with other proteins embedded within the membrane (Runge et al., 2007; Jegou et al., 2011; Chalbi et al., 2014; Benammar et al., 2017). Indeed, the tetraspanin family, including CD9, contributes to the structural organization and plasticity of the plasma membrane. Regarding the architectural aspect, the concentration of CD9 molecules on microvillar membrane was found to be greater than that on the planar membrane. In addition, the complete absence of CD9 results in altered microvillar morphology, particularly a change of the radius of curvature of microvilli that are two fold higher than on wild-type oocytes (Runge et al., 2007) while a small radius of curvature is thought to promote fusibility (Wilson and Snell, 1998; McMahon and Gallop, 2005). An isolated large extracellular loop of CD9 was shown to inhibit fusion when preincubated with eggs, but not with sperm (Zhu et al., 2002) suggesting that CD9 is not a receptor for a sperm molecule. Only one article suggested that CD9 may function in a trans manner during gamete fusion by binding to an immunoglobulin (Ig) super family member, namely PSG17 (Ellerman et al., 2003). But this protein has never been found on sperm. Conversely, and in the sense of its cis action, CD9 associates with other tetraspanins, integrins, Igs and other adhesion receptors and proteins (reviewed in ((Charrin et al., 2014)). There are other members of Ig superfamily expressed by the oocytes with which CD9 interacts. Oocyte CD9 co-immunoprecipitates with two Ig superfamily cis partners, EWI-2 and EWI-F, which could have a role in linking CD9 to the oocyte microvillar actin core. Recently, crystal and cryo-electron microscopic structure of human CD9 and CD9-EWI-2 complex showed the interaction between CD9 and EWI-2, mediated by small residues in the transmembrane region and protein/lipid interaction suggesting the possible role of EWI-2 as a bridge between tetraspanins and other proteins, required for remodeling of membrane resulting in the formation of complex protein network. Cryo-electron microscopic structure of the CD9-EWI-2 complex and the mutation analysis revealed the importance of the small residues in the transmembrane region for the complex formation between CD9 and EWI-2 and the partial involvement of the CD9 large extracellular loop in the complex formation with EWI-2 (Umeda et al., 2020).
EWI-2 and EWI-F are tetraspanin CD9 and CD81 partners. They are immunoglobulins that share a Glu-Trp-Ile (EWI) extracellular motif. EWI-2 is also called IGSF8, PGRL, CD316 or KCT-4 and EWI-F is also known as FPRP or CD9-P1. Both associate directly with tetraspanins CD9 and CD81 while they do not associate with other tetraspanins or integrins (Charrin et al., 2001; Stipp et al., 2001; Sala-Valdes et al., 2006). In somatic cells, they induce tetraspanin relocalization into filopodia and they interfere in cell motility and aggregation (Charrin et al., 2001; Sala-Valdes et al., 2006; He et al., 2009). EWI-2 association with α-actinin regulates T cell immune synapses and HIV viral infection (Gordon-Alonso et al., 2012). Moreover, the expression level of membrane EWI-2 in Cd9-null oocytes is drastically reduced (He et al., 2009).
We have previously shown that CD9 generates fusion competent sites on the egg membrane for mammalian fertilization. These CD9-induced adhesion sites, named pro-fusional (i.e., able to lead to fusion), with strong adhesion, would be the actual location where fusion occurs. Indeed, in the absence of CD9, the adhesion between the two gametes exists but remains abortive (Jegou et al., 2011). Regarding the adhesive strength that we measured experimentally, the crucial difference between wild type (WT) and Cd9-null eggs comes from the strong interaction events that are absent when CD9 is absent. These adhesions are stronger because of the strong connection between the membrane and the cytoskeleton, that is not realized directly but through other molecules suggesting an involvement of CD9 in this process. Under these conditions, the link would likely occur via CD9-direct partners such as EWI-2 and EWI-F present in both somatic cells and oocyte (Rubinstein et al., 2006; Sala-Valdes et al., 2006; Runge et al., 2007). In turn, these CD9 partners bind directly to Ezrin-Radixin-Moesin (ERM) that connect the actin cytoskeleton to the plasma membrane (Sala-Valdes et al., 2006). Actin filaments cross-linked with the plasma membrane are important for generating cell-surface specialization areas. This is achieved through the regulation of actin-binding proteins. ERM proteins are thought to belong to the molecules linking the actin cytoskeleton and the plasma membrane. ERM proteins are widely distributed proteins located in the cellular cortex, in microvilli and adherens junctions. They contain a membrane binding domain and an actin binding domain. They have been implicated in mediating actin-membrane linkage and in regulating signaling molecules. In T lymphocytes for example, phosphorylated ERM are located at the posterior part of the cell where they have a potential role in the induction of uropod formation (Serrador et al., 1997; Lee et al., 2004). Indeed, ERM can have two conformations: a non-phosphorylated inactive cytoplasmic form and a phosphorylated active form located beneath the plasma membrane where it interacts with the cytoplasmic domain of adhesion proteins (For review (Louvet-Vallee, 2000; Fehon et al., 2010)). Furthermore, the function of lymphocyte Moesin is required for efficient HIV-1 viral fusion and infection (Barrero-Villar et al., 2009). More specifically, Dmoesin, the only member of the ERM family present in Drosophila, is required during oogenesis to anchor microfilaments to the oocyte cortex (Polesello et al., 2002) and Ezrin-deficient mice show microvilli disorganization in the developing intestine (Saotome et al., 2004). Mouse oocytes express all three ERM family members but seem to be particularly enriched in Radixin (Louvet et al., 1996; Larson et al., 2010). Finally, it has been shown that the disruption of ERM function reduces cortical tension in metaphase II eggs (Larson et al., 2010). It is known that cortical tension decreases during meiotic divisions and that a soft cortex is essential for asymmetric spindle positioning in mouse (Chaigne et al., 2013) which is guided by a narrow window of cortical tension (Chaigne et al., 2015). Recently it has been shown that aneuploidy in mouse oocytes can be generated by artificially decreasing cortical tension (Bennabi et al., 2020).
The purpose of our work was to analyze the role of ERM and EWI-2 and EWI-F proteins in gamete fertilization. We injected small interfering RNA (siRNA) to inhibit ERM and/or EWI-2 and EWI-F gene expression into oocyte cytoplasm. To assess the functional and morphological effects of inhibition, we assessed the fertilization index and studied oocyte microvilli morphology and measured cortical tension.
Materials and Methods
Ethical Standards
All animal experiments were performed in accordance with national guidelines for the care and use of laboratory animals. Authorizations were obtained from local and governmental ethical review committees: Authorization APAFIS #14124-2017072510448522, A. Ziyyat (2018–2023).
Sperm Preparation
Sperm from 8 to 10 weeks old male mice (B6CBAF1, Janvier, France) were expelled from cauda epididymis and vas deferens into a 500 μl drop of Ferticult medium (FertiPro N.V, Belgium) containing 3% BSA under mineral oil, resulting in a concentration of approximately 107 sperm/ml. Sperm were then incubated at 37°C, 5% CO2 in air for 90 min to induce capacitation.
Oocytes Retrieval and siRNA Micro-injection
Germinal Vesicle stage (GV) oocytes were collected from 5 to 8 weeks old B6CBAF1 (Janvier Labs, France) female mice at 39 h post-PMSG (Pregnant mare’s serum gonadotrophin, Intervet, France) injection. GV oocytes pick-up was performed in M2 medium (Sigma) supplemented with 2.5 μM Milrinone (Sigma) to maintain prophase I arrest. Cumulus cells were removed by repeated pipetting. Ten pl of siRNA solution were injected into the cytoplasm of denuded GV oocytes 1 hour after their retrieval, using a 1.2 µm inner diameter pipette (BioMedical Instruments, Germany) connected to a FemtoJet injector (Eppendorf, Germany) under a Nikon inverted microscope in the same M2 medium containing Milrinone at room temperature. Oocytes were then washed several times to remove Milrinone and matured in vitro in M16 medium (Sigma) during 24 or 48 h at 37°C, 5% CO2 in air. siRNA against Ezrin (sc-35350), Radixin (sc-36367), Moesin (sc-35956), ERM (sc-37850) EWI-2 (sc-105564) and EWI-F (sc-142204) siRNA from Santa Cruz biotechnology were used at 1 μM final concentration. Control oocytes were injected following the same protocol with the same volume and concentration of control (CTRL) siRNA-A solution (sc-37007, Santa Cruz biotechnology). Figure 1 represents the experimental design of the study.
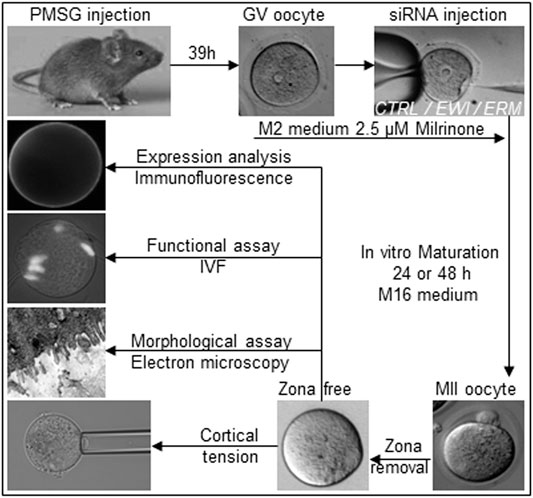
FIGURE 1. Experimental design. Germinal Vesicle stage (GV) oocytes were recovered from 5 to 8 weeks old B6CBAF1 mice 39 h post-PMSG injection. GV oocytes were maintained in M2 medium supplemented with 2.5 μM Milrinone to maintain meiotic arrest and placed on a Nikon inverted microscope at room temperature. Ten pl of siRNA solution (1 μM final concentration) were injected into the cytoplasm using a 1.2 µm inner diameter pipette connected to an injector. After microinjection, oocytes were washed several times to remove Milrinone and let in M16 medium to resume meiosis during 24 or 48 h at 37°, 5% CO2 in air. Then, after zona pellucida removing, oocytes were either assayed for their fertilizability or cortical tension measurement or fixed for electron microscopy or immunofluorescence studies.
Immunofluorescence Staining
Oocytes were fixed in PBS containing 1% BSA, 2% paraformaldehyde, 0.5% saponin (Sigma, France) (for membrane permeabilization when it was necessary). They were stained with anti-pERM antibodies at 5 μg/ml (#3726, Cell-Signaling technology), anti-Radixin at 4 μg/ml (Santa-Cruz biotechnologies), anti-EWI-2, anti-EWI-F at 5 or 10 μg/ml respectively (R&D systems, United States) or rat monoclonal anti-mouse CD9 at 20 μg/ml (KMC8; BD PharMingen, San Diego, MD, United States). Secondary antibodies used were anti-rabbit antibody for pERM at 4 μg/ml (GE Healthcare, United Kingdom), anti-goat Alexa 488 at 10 μg/ml for Radixin (Invitrogen, United States), anti-goat IgG TRITC Conjugate for EWI-2 at 2 μg/ml (ImmunoReagents, Inc, United States), anti-sheep Alexa 488 at 1 μg/ml for EWI-F (Molecular Probes, United States) and goat anti-rat Alexa green antibody for CD9 at 10 μg/ml (Molecular Probes). Stained eggs were then washed and mounted in Vectashield/DAPI for observation under UV light (Nikon Eclipse 600 microscope). Images of stained eggs were collected using the NIS-Elements D (Nikon) software and analyzed using ImageJ. Integrated density measurements of the entire oocyte were taken, using the elliptical selection tool to draw an ellipse around the cell. In order to eliminate any size-effect of the measured oocytes, we divided each result by the pixel size of the area analyzed. Results were first expressed in intensity per 105 pixels then bring to a percentage considering the CTRL siRNA group at 100%.
Zona Pellucida Removal and in vitro Fertilization
Oocytes were assessed after 24 h in M16 medium. Only Metaphase II (MII) oocytes were selected on the basis of the presence of the first polar body. Depending on the experiment, at 24 or 48 h, the zona pellucida (ZP) was subsequently removed by rapid treatment (<30 s) with acidic Tyrode’s solution (Sigma). Oocytes were then incubated for 2 h at 37°C, 5% CO2 in air to recover from the treatment, contemporaneously to sperm capacitation. ZP-free MII oocytes were washed and then placed in a 100 μl Ferticult medium drop containing 3% Bovine Serum Albumin (BSA, Sigma). ZP-free MII oocytes were inseminated with capacitated sperm for 2 h at a final concentration of 105/ml, then washed and mounted in Vectashield/DAPI for observation under UV light (Nikon Eclipse 600 microscope). The fertilization index was evaluated as the total number of fused sperm/total number of eggs.
Microvilli Morphology Assessment
Transmission Electron Microscopy
For transmission electron microscopy, ERM, EWI (EWI-2 and EWI-F), Mix (ERM and EWI) or CTRL siRNA injected oocytes were fixed in a 100 µl drop of 0.25% glutaraldehyde (Sigma) in PBS 1% BSA for 30 min. After three washes in PBS 1% BSA, oocytes were fixed in 2.5% glutaraldehyde in Sorensen buffer supplemented with 1% BSA for 30 min at RT and 1 h at 4°C. After three washes in Sorensen buffer with 1% BSA, oocytes were post-fixed with 1% osmium tetroxide in 0.1 M phosphate buffer and then dehydrated in 70, 90 and 100% ethanol. After 10 min in a 1:2 mixture of epoxy propane and epoxy resin, oocytes were embedded in gelatin capsules with freshly prepared epoxy resin and polymerized at 60°C for 24 h. Samples were then mounted into epon blocks and sections of 90 nm were performed with an ultramicrotome (Reichert ultracut S), stained with uranyl acetate and Reynold’s lead citrate, and observed under a transmission electron microscope (Jeol 1011 microscope), with a Gatan camera. Pictures were taken all around the perimeter of each section.
Measuring of the Microvillar Curvature
The radius of curvature at the tips of microvilli was determined by analyzing the images using the ImageJ software. The radius of curvature was determined to be the radius of a circle that fits the curve at the tip of the microvillus and correspond to the half of the diameter as illustrated in the zoomed area (Figure 6) as described previously (Runge et al., 2007). Only microvilli with distinct tips were used.
Micropipette Aspiration for Cortical Tension Measurement
Micropipette aspiration (MPA) was adopted to measure the oocytes cortical tension as previously described (Larson et al., 2010; Evans and Robinson, 2018). Briefly, the zona pellucida of oocytes was removed using acid Tyrode’s solution. Oocytes were transferred into M2 medium with 3% BSA. The temperature of the medium was maintained at 37°C. A glass micropipette of a 12∼15 μm diameter was connected to a water reservoir of adjustable height. The micropipette and the tubing connections for the entire system were tested to confirm that no air bubbles were present. Zero aspiration pressure was set prior to each experiment by checking the absence of visible flow inside the pipette. Then, we measured the critical aspiration pressure (Δp) according to which the length of the protrusion pulled into the micropipette (Lp) is equal to the pipette radius (Rp). The cortical tension (Tc) was calculated using the Law of Laplace (Δp = 2Tc (1/Rp − 1/Rc)), where Rc is the radius of the oocyte. Oocytes during MPA were observed and images were captured using an inverted microscope equipped with a charge-coupled device (CCD) camera. Tc were measured on the microvillar regions of oocytes.
Statistical Analysis
To compare the fluorescence intensities, an unpaired Student t test was used. To compare the Fertilization Indexes (mean ± s. e.m.), a Mann-Whitney test was used. To compare the cortical tensions and microvilli radius, a one-way ANOVA test with multiple comparisons was used. Differences were considered significant at p < 0.05. All experiments were realized at least three times.
Results
Figure 1 illustrates the experimental set-up including the different steps, ranging from oocytes preparation, siRNA injection, in vitro maturation and zona removal to the various tests that have been applied, namely immunofluorescence staining, zona-free in vitro fertilization assay, electron microscopy analyzes and measurement of cortical tension.
ERM and EWI-2 and EWI-F siRNAs Are Effective to Reduce Expression of Their Respective Proteins at the Oocyte Membrane Level
To evaluate the efficiency of siRNA inhibition on protein expression, we compared by immunofluorescence the microinjected GV oocytes, by one or a combination of siRNAs and then matured in vitro.
Effect of the EWI-2 and EWI-F siRNA Injection on the Corresponding Protein Membrane Expression
When observed by epifluorescence after immunostaining 24 h after microinjection of combined EWI-2 and EWI-F siRNA or CTRL siRNA, EWI-2 was localized on the egg membrane with no difference detectable to the naked eye between the two groups. However, further quantification of the fluorescence with ImageJ revealed a weak (13%) but significant (p = 0.02) decrease of fluorescence intensity of the EWI-F protein after EWI-2/EWI-F siRNA (n = 18) compared to CTRL siRNA (n = 23) injection (Figure 2B). This small reduction of EWI-F expression should be due to a poor efficiency of siRNA, to its degradation or to a high stability of the EWI-F protein.
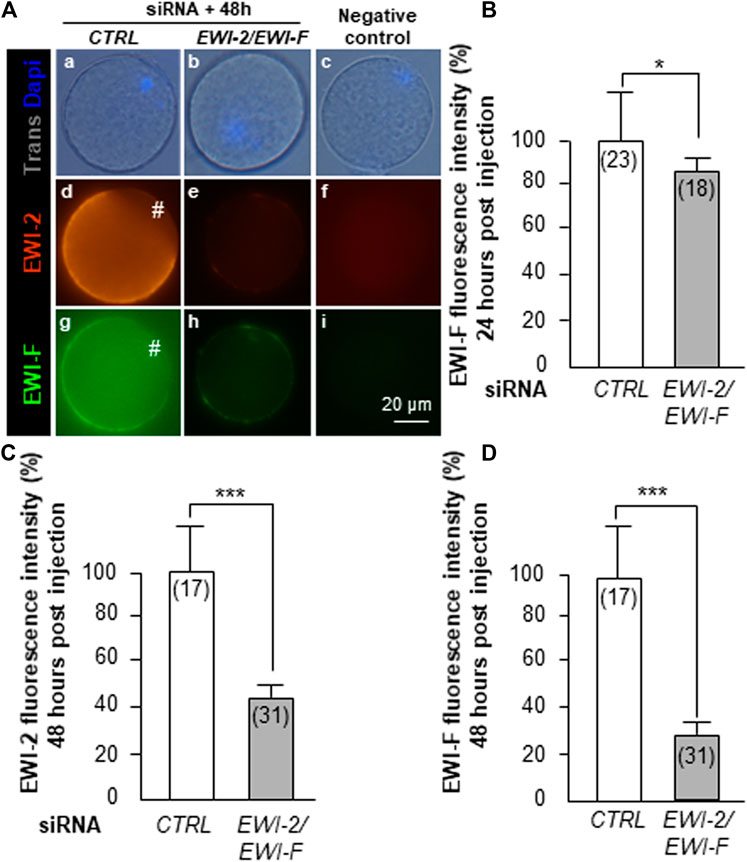
FIGURE 2. EWI-2 and EWI-F oocyte membrane expression 24 and 48 h post EWI-2/EWI-F siRNA injection. CTRL siRNA (A a,d,g) or EWI-2/EWI-F siRNA (A b,e,h) together were microinjected into GV oocytes, which were let to mature 24 or 48 h in vitro, to improve the expression inhibition. They were then fixed and incubated with the anti-EWI-2 or the anti-EWI-F antibodies and stained by the secondary antibodies anti-goat IgG TRITC or anti-sheep Alexa 488 respectively. Negative controls (A) c,f,i without primary antibodies. Analysis of the fluorescence intensity (B,C,D) was performed and EWI-2/EWI-F siRNA microinjected oocytes showed, after 24 h in vitro maturation, a weak (13%) but a significant (p = 0.02) decrease of fluorescence intensity of the EWI-F protein compared to CTRL siRNA group (B). After 48 h in vitro maturation, greater reductions of the EWI-2 55%, (C) and EWI-F 68%, (D) staining compared to the CTRL siRNA injected oocytes were observed (p < 0.0001). # amicrovillar zona; ( ) number of oocytes.
To check this last point and try to improve the expression inhibition, we left the siRNAs acting for 48 h (instead of 24 h) before performing immunostaining of EWI-2 and EWI-F. After this delay, a clear reduction of expression of both EWI-2 and EWI-F was observed on the oocytes injected with EWI-2 and EWI-F siRNA compared to the CTRL group (Figure 2A d vs. e and g vs. h). While negative controls (without primary antibodies) did not reveal any signal (Figure 2A f, i), significant decreases (55 and 68%; p < 0.0001; Figures 2C,D) in the fluorescence intensity of EWI-2 and EWI-F were observed respectively (n = 31) compared with CTRL siRNA oocytes (n = 17). This result suggested the stability of EWI-2 and EWI-F proteins.
ERM siRNA Injection Reduces Radixin and Phosphorylated ERM Membrane Expression
Three groups of GV oocytes were injected either with Radixin, or Ezrin, or Moesin siRNA, and 24 h later, they were immunostained with respective antibodies. The Radixin siRNA group (n = 5), showed a significant decrease (56%; p = 0.03; Figure 3C) of the fluorescence due to Radixin compared to the CTRL group (n = 5) (Figure 3A d vs. e). Unfortunately, because of nonspecific signals obtained with the antibodies directed against Ezrin and Moesin, we could not estimate the influence of Ezrin and Moesin siRNA on Ezrin and Moesin protein expression. To circumvent this difficulty, we injected combined ERM siRNA and 24 h later we used antibodies recognizing the phosphorylated form of the three ERM proteins (pERM). Immunostaining results showed a dramatic decrease of pERM membrane expression since no fluorescence could be detected in the ERM siRNA injected group (n = 13) contrary to the CTRL group (n = 20) (Figure 3B j vs. k). We also assessed the specific expression of Radixin at the oocyte membrane after ERM siRNA injection (n = 7). We found a significant decrease (62%) of its immunofluorescence intensity compared to the CTRL siRNA injected group (p = 0.01; Figure 3C).
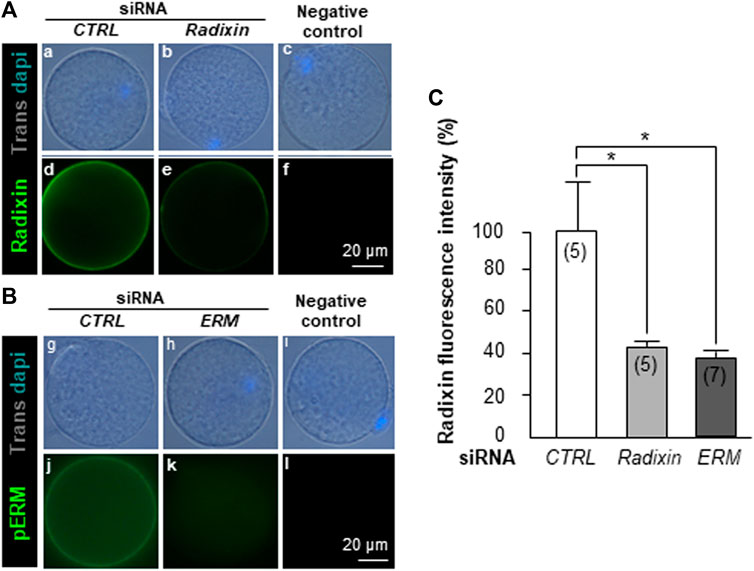
FIGURE 3. Radixin and ERM siRNA injection on Radixin and phosphorylated ERM (pERM) membrane expression, respectively. (A). CTRL siRNA (a,d) or Radixin siRNA (b,e) were microinjected into GV oocytes, which were let to mature 24 h in vitro, then fixed, incubated with anti-Radixin antibodies and then with secondary antibodies anti-goat Alexa 488. Negative control without primary antibodies (c,f). (B). CTRL siRNA (g,j) or ERM siRNA (h,k) were microinjected into GV oocytes, which were let to mature 24 h in vitro, then fixed, incubated with anti-pERM antibodies and then with secondary antibodies anti-rabbit Alexa 488. Negative control without primary antibody (i,l). (C). Radixin siRNA compared to the CTRL siRNA injection resulted in a significant decrease (p = 0.03) of fluorescence intensity of Radixin. Combined ERM siRNA, compared to CTRL siRNA injection, dramatically decreased Radixin oocyte membrane expression. ( ) number of oocytes.
Combined, But Not Isolated EWI-2 and EWI-F or Ezrin, Radixin, Moesin siRNAs Injection Decrease the Fertilization Ability of the Oocyte Without Decreasing CD9 Expression
Oocyte Fertilization After EWI-2 and/or EWI-F siRNA Injection
After 48 h of in vitro maturation following EWI-2 or CTRL siRNA injection, zona removal and in vitro fertilization, the fertilization indexes (FI) observed were 2.03 ± 0.19 (n = 67) and 1.66 ± 0.16 (n = 89) respectively (Figure 4Aa). After EWI-F siRNA injection, FI was 2.16 ± 0.15 (n = 80) compared to 2.03 ± 0.16 after CTRL siRNA injection (n = 74) (Figure 4Ab). These differences after EWI-2 or EWI-F siRNA isolated injection were not significant.
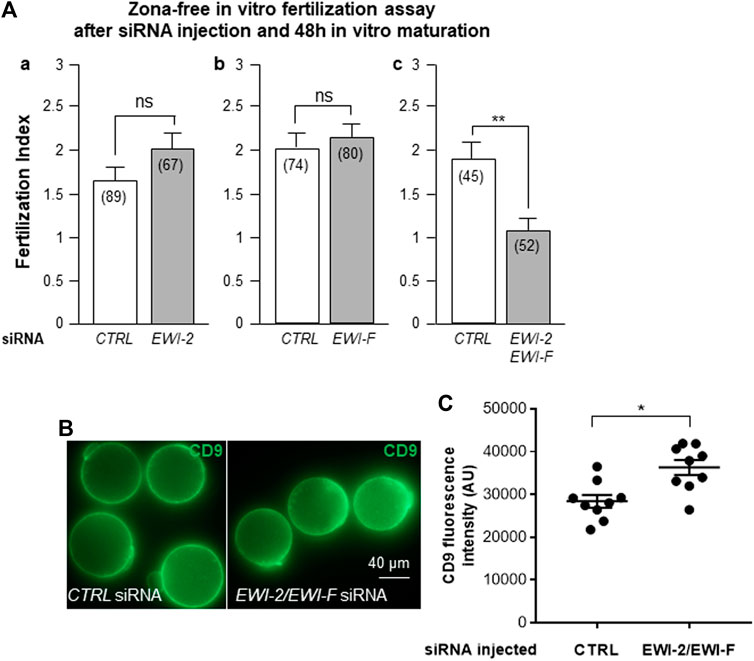
FIGURE 4. Combined, but not isolated EWI-2 and EWI-F siRNAs injection on oocyte inhibits fertilization without disrupting CD9 expression. (A). a. After EWI-2 siRNA injection, in vitro maturation (48 h), zona removal and in vitro fertilization, the oocyte fertilization index observed was not different from the one of the CTRL siRNA oocyte group. b. After EWI-F siRNA injection, in vitro maturation (48 h), zona removal and in vitro fertilization, the oocyte fertilization index observed was not different from the one of the CTRL siRNA injected oocytes. c. After combined EWI-2 and EWI-F siRNA injection, in vitro maturation (48 h), zona removal and in vitro fertilization, a significant reduction of the FI (43%, p = 0.0011) was observed compared to the CTRL siRNA group. ( ) number of oocytes from at least three different experiments. (B). Images illustrating CD9 immunofluorescence on oocytes microinjected with CTRL or EWI-2/EWI-F siRNA and in vitro matured during 48 h. (C). Statistical comparison (T-test) using ImageJ quantification of CD9 fluorescence intensity between CTRL and EWI-2/EWI-F siRNA groups (*p = 0.03).
On the contrary, simultaneous decrease in proteins EWI-2 and EWI-F expression after combined siRNA injection was associated with a significant reduction of the FI (43%, p = 0.0011). Indeed, fertilization index was 1.91 ± 0.19 sperm per egg in CTRL siRNA microinjected oocytes (n = 45) compared to 1.08 ± 0.14 in EWI-2 and EWI-F combined siRNAs injected oocytes (n = 52) (Figure 4Ac).
Inhibition of EWI-2 and EWI-F did Not Decrease CD9 Expression
Considering that EWI-2 and EWI-F are direct partners of CD9, we sought to find out if their effects on fertilization were dependant on a reduction in the expression of CD9, itself directly involved in this process. Immunofluorescence of CD9 in oocytes microinjected with CTRL or EWI-2/EWI-F siRNA (Figure 4B) unexpectedly revealed a slight increase in CD9 expression in oocytes microinjected with EWI-2/EWI-F siRNA (Figure 4C). No difference in CD9 localization was observed between the two groups.
Combined Injection of Ezrin, Radixin and Moesin siRNA Decreases Fertilization Index
As detailed in Figure 5, after 24 h of in vitro maturation, while isolated injection of either Ezrin, Radixin or Moesin siRNA did not affect the FI (Figures 5A–C), combined ERM siRNA injection did induce a significant decrease of the FI (59%) from 2.82 ± 0.23 after CTRL siRNA injection (n = 82) to 1.15 ± 0.16 (n = 67) (p < 0.001, Figure 5D).
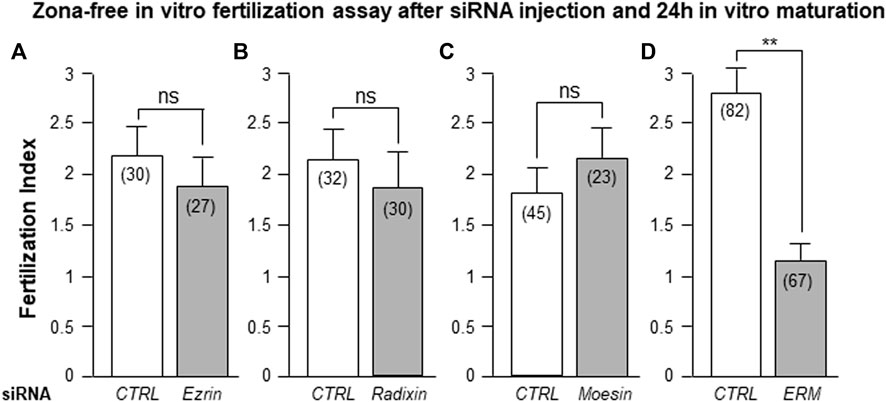
FIGURE 5. Combined, but not isolated, ERM siRNAs injection in oocyte inhibited fertilization (A). After Ezrin siRNA injection, in vitro maturation (24 h), zona removal and in vitro fertilization, the oocyte fertilization index observed was not different from the one of the CTRL siRNA oocyte group. (B). After Radixin siRNA injection, zona removal and in vitro fertilization, the oocyte fertilization index observed was not different from the one of the CTRL siRNA oocyte group (C). After Moesin siRNA injection, zona removal and in vitro fertilization, the oocyte fertilization index observed was not different from the one of the CTRL siRNA oocyte group (D). After combined ERM siRNA injection, zona removal and in vitro fertilization, a significant reduction of the FI (59%; p < 0.001) was observed compared to the CTRL siRNA group. ( ) number of oocytes from at least three different experiments.
EWI-2, EWI-F, ERM or Combined (EWI/ERM) siRNAs Injection on Oocyte Alter Microvilli Morphology and Density
After CTRL, ERM, EWI-2/EWI-F or Mix (ERM and EWI-2/EWI-F) siRNA injection and 48 h in vitro maturation, we used transmission electron microscopy to analyze Metaphase II oocytes microvilli in terms of radius of curvature and density. Figure 6 provides a typical image of each group. The mean radius of curvature of the microvilli increased from 47.44 ± 0.63 nm for CTRL siRNA injected oocytes (553 microvilli on 35 oocytes) to 53.08 ± 0.75 nm (931 microvilli on 29 oocytes, p < 0.0001), 55.26 ± 0.69 nm (425 microvilli on 48 oocytes, p < 0.0001) and 57.78 ± 0.81 nm (493 microvilli on 36 oocytes, p < 0.0001) for ERM, EWI-2/EWI-F and Mix siRNA injected oocytes respectively. ANOVA tests show a significant increase of microvilli radius in all groups as compared to the CTRL siRNA group and between the EWI-2/EWI-F siRNA and Mix siRNA groups and between the ERM siRNA and EWI-2/EWI-F siRNA groups (Figure 7A). To estimate the actual error made during the measurements, we took at random two microvilli in each group that we measured three times. The average standard deviation between the three measurements ranged between 0.255 and 1.326 nm which is significantly lower than the reported difference between the groups.
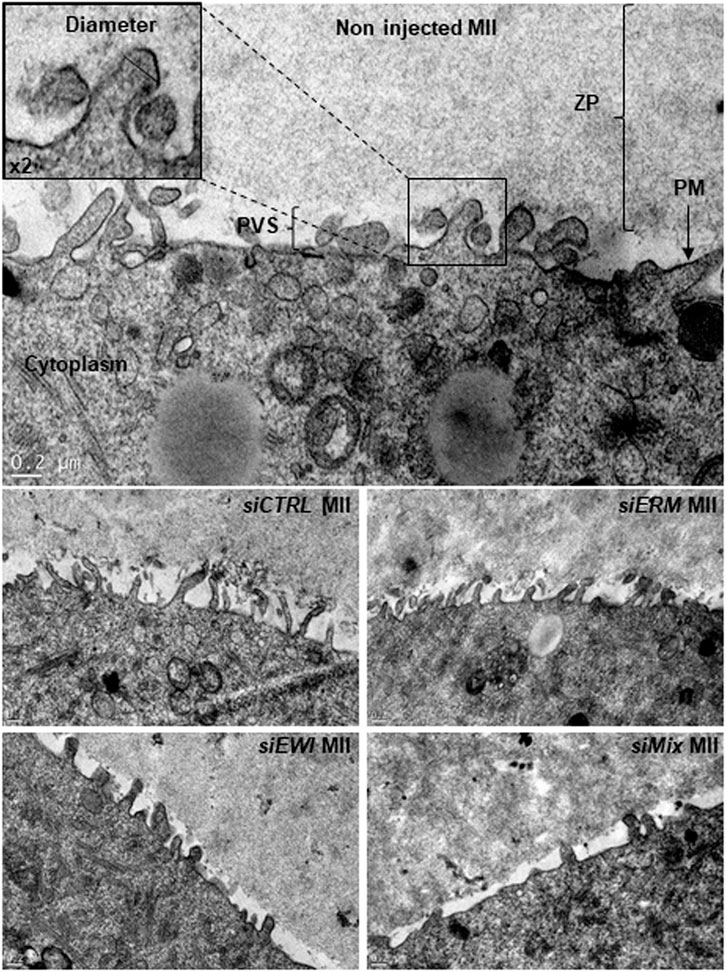
FIGURE 6. ERM and/or EWI siRNAs injection alters microvilli morphology. After CTRL, ERM, EWI or Mix siRNA injection and 48 h in vitro maturation, Metaphase II oocytes were fixed and their microvilli analyzed by electron microscopy. Zona Pellucida (ZP), Plasma Membrane (PM), Perivitelline Space (PVS), Cytoplasm and Radius of curvature are shown in a non-microinjected MII oocyte. An example image is shown for each microinjected MII oocyte group. Scale bar: 0.2 µm.
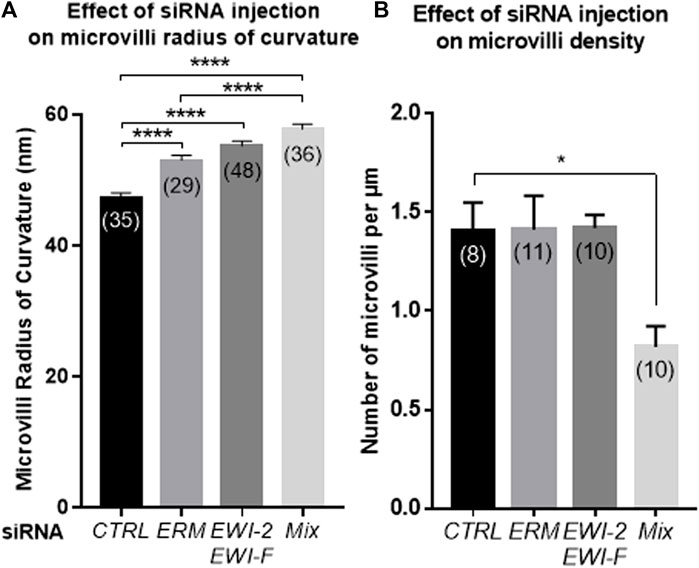
FIGURE 7. Effect of CTRL, ERM, EWI or combined ERM-EWI siRNAs injection on microvilli morphology. After siRNA injection and 48 h in vitro maturation, Metaphase II oocytes were fixed and their microvilli analyzed by electron microscopy. (A). Microvilli radius of curvature increased after ERM, EWI or combined ERM-EWI (Mix) siRNAs injection and maturation compared to CTRL siRNA injected oocytes (****p < 0.0001). (B). Decrease of microvilli density after Mix siRNA (combined ERM and EWI siRNA) injection and maturation (*p < 0.01). ( ): Oocyte number from at least three different experiments.
Regarding the density of the microvilli, the only significant difference (p < 0.01) obtained was between the Mix siRNA group (0.81 ± 0.10/µm) and the three other groups (1.41 ± 0.13/µm, 1.41 ± 0.17/µm and 1.42 ± 0.06/µm for CTRL, ERM and EWI-2/EWI-F siRNA microinjected groups respectively). In each group, between 8 and 11 oocytes from at least three different experiments were analyzed. The counts were carried out on approximately 300 images per group that represent more than one thousand microvilli per group (Figure 7B).
Partial Inhibition of ERM and EWI-2/EWI-F Expression Decreases Metaphase II Cortical Tension
Previous studies identified important changes in the cortical tension of oocytes during their progression through meiotic maturation and egg activation (Larson et al., 2010). It has also been shown that a perturbation of cortical tension through the function disruption of the family of actin-to-membrane tethering (ERM) causes significant defects in spindle function during exit from metaphase II arrest. Here we studied the effect of the inhibition of ERM, EWI-2/EWI-F expression on the oocyte cortical tension. When microinjected, the oocytes were at the germinal vesicle (GV) stage. Then, they were matured in vitro up to the MII stage. During the microinjection, few oocytes did not tolerate this mechanical trauma and lysed quickly. Those that resisted to this step survived and over 80% of them, regardless of the siRNA injected, matured and reached the MII stage. All microinjected oocytes were measured at MII stage.
First, we measured cortical tension without any microinjection on oocytes at the VG stage and obtained an average of 3.574 ± 0.454 nN/μm. This value dropped drastically (p < 0.0001) with the oocyte maturation towards the MI matured in vitro (0.174 ± 0.020 nN/μm) or in vivo (0.142 ± 0.013 nN/μm) and MII stages matured in vitro (0.139 ± 0.008 nN/μm) or in vivo (0.091 ± 0.004 nN/μm). Comparisons of cortical tension between MI and MII oocytes in vitro and in vivo showed no significant differences (Figure 8A). Then we measured the cortical tension at the MII stage after CTRL, ERM or EWI-2/EWI-F siRNA injection at the VG stage and in vitro maturation during 24 h. Only EWI-2/EWI-F combined siRNA injection gave a significant decrease of the cortical tension from 0.109 ± 0.008 for the CTRL siRNA group to 0.069 ± 0.015 nN/μm (p = 0.02). Cortical tensions were comparable for the ERM and CTRL siRNA groups (p = 0.8, Figure 8B). When oocytes were matured in vitro during 48 h instead of 24 h the cortical tensions remained non-significantly different between the CTRL siRNA group (0.141 ± 0.006 nN/μm) and ERM siRNA group (0.116 ± 0.005 nN/μm; p = 0.9) but became very significant after injection of EWI-2/EWI-F siRNA (0.093 ± 0.005 nN/μm; p < 0.0001) or Mix (EWI-2/EWI-F–ERM) siRNA (0.078 ± 0.003, p < 0.0001). Moreover, there was no significant difference between the EWI-2/EWI-F and Mix siRNA groups (0.093 ± 0.005 versus 0.078 ± 0.003; p = 0.8) but the difference between ERM and Mix siRNA appeared significant (p = 0.03) (Figure 8C).

FIGURE 8. ERM and EWI siRNAs injection decreased oocyte cortical tension. (A) A drastic drop in cortical tension was observed between the Germinal Vesicle stage (GV) oocytes and metaphase I (MI) or metaphase II (MII) oocytes whether the latter were matured in vivo or in vitro without any injection (****p < 0.0001). (B). A slight decrease in cortical tension was observed after injection of siRNA EWI (EWI-2 and EWI-F) and 24 h of maturation compared to oocytes injected with CTRL siRNA (*p = 0.02). (C). When the injected oocytes had matured for 48 h, the decrease of the cortical tension after the injection of siRNA EWI or siRNA Mix was more pronounced compared to the CTRL siRNA group (****p < 0.0001 and *p = 0.03). ( ): number of analyzed oocytes from at least three different experiments.
Discussion
The aim of our study was to assess the role of the oocyte EWI-2, EWI-F and ERM protein family as potential partners of CD9 in gamete adhesion/fusion processes.
Our data showed that fertilization was not affected by the inhibition of one of these proteins, the fertilization index being not decreased after inhibition of either EWI-2, EWI-F, Ezrin, Radixin or Moesin, separately. Conversely, injection of ERM siRNA or combined EWI-2/EWI-F siRNA inhibited fertilization, decreasing the FI by 59 and 43% respectively. The lack of single siRNA effect did not appear to be due to a lack of siRNA efficiency since the injection of EWI-F or EWI-2 siRNA induced significant decreases in protein expression (55 and 68% respectively), as well as Radixin siRNA alone caused a decrease in Radixin protein expression (56%) similar to that caused by the combined injection of ERM siRNA (62%). Thus, the lack of effect on fertilization could be explained by a redundancy mechanism, like already highlighted in the context of knockout gene experiments. Indeed, EWI-2 and EWI-F are both immunoglobulins known to be associated to tetraspanins in somatic cells as well as in oocytes (Charrin et al., 2001; Stipp et al., 2001; Charrin et al., 2003; Runge et al., 2007; Glazar and Evans, 2009; He et al., 2009). EWI-2-deficient mice have been demonstrated to be fertile. Their eggs retain the normal level and localization of CD9, resulting in normal microvilli formation (Inoue et al., 2012). While CD9 regulates the expression and localization of EWI-2, these results and ours indicate that EWI-2 and EWI-F do not regulate the expression and localization of CD9. These results also suggest a possible compensation by EWI-F. In addition, unlike the anti-CD9 antibody, an anti-EWI-2 antibody has moderate inhibitory effects on sperm-egg binding and fusion (Glazar and Evans, 2009). Similarly, in our previous experiments, a monoclonal antibody directed against the EWI-F (CD9P-1) molecule failed to inhibit fusion (Rubinstein et al., 2006). Otherwise, EWI-2 and EWI-F have been shown to associate directly to ERM in somatic cells (Sala-Valdes et al., 2006). ERM are thought to constitute a bridge between the actin cytoskeleton and the plasma membrane. Ezrin-deficient mice, that die by 3 weeks of age (explaining that their fertility cannot be explored), showed abnormalities in their intestinal epithelium morphogenesis with microvilli disorganization. It should be noted that Ezrin is the only ERM protein detected in the developing intestinal epithelium, and this is likely why no redundancy mechanism may occur in this case (Saotome et al., 2004). Radixin-deficient mice are viable and fertile (Kikuchi et al., 2002). Moesin-deficient mice are normal and fertile without any Ezrin or Radixin hyper-regulation (Doi et al., 1999). However, the mutations of the Dmoesin gene in drosophila have dramatic consequences on embryo development. Dmoesin is required during oogenesis for anchoring microfilaments to the oocyte cortex (Polesello et al., 2002), since in mutant oocytes, the subcortical actin network is detached from the cell membrane (Jankovics et al., 2002). This is consistent with the fact that no redundancy is possible in this non-mammalian species that lacks the ezrin and radixin genes. Indeed, ERM are encoded by three highly homologous genes that have ∼85% similarity at the amino acid level (For review (Louvet-Vallee, 2000; Fehon et al., 2010)).
Given that ERM proteins promote the CD9-cytoskeleton interaction (Sala-Valdes et al., 2006), we can hypothesize that ERM are also critical in gametes adhesion/fusion mechanism via this function. The fertilization inhibition obtained by siRNA injection was however less dramatic than the one we have reported in Cd9-knockout eggs (Jegou et al., 2011). This discrepancy could be due to the fact that although almost no pERM expression was detected by immunofluorescence after siRNA injection, it cannot be ruled out that few ERM proteins remained or that further ERM phosphorylation may take place. In this case, our results would underestimate their role. A significant decrease of protein expression necessitated 48 h after the injection of EWI-2/EWI-F siRNA, while 24 h post injection of ERM siRNA were sufficient to detect an important decrease suggesting that the EWI-2 and EWI-F proteins would be more stable than ERM proteins.
It has been shown that overexpression of ERMBMPs (ERM-binding membrane proteins) in collaboration with activated ERM proteins in several cell lines, induced formation and elongation of microvilli (Yonemura et al., 1999). A mixture of ezrin/radixin/moesin antisense oligonucleotides could induce the destruction of both cell-cell and cell-substrate adhesion, as well as the disappearance of microvilli (Takeuchi et al., 1994).
In addition, it has been shown that the mechanical properties of the cell cortex can predict the developmental potential of oocytes after fertilization in mice in humans (Yanez et al., 2016). Cortical tension is highly regulated during oocyte maturation and could also play a role during gamete adhesion/fusion.
In order to check if the functional finding, the fertilization decrease, resulted from microvilli morphological alteration and/or cortical tension deregulation, we measured the radius of curvature of microvilli on oocyte membranes and cortical tension after injection of ERM and/or EWI-2/EWI-F siRNA compared to CTRL siRNA. Indeed, the inhibition of the expression of these proteins also caused an increase in the radius of curvature of the oocyte microvilli and a decrease in cortical tension.
Several works have shown the link between the radius of curvature and fusion. A small radius of curvature at the tip of microvilli maximizes the efficiency of membrane fusion (Wilson and Snell, 1998; McMahon and Gallop, 2005). In several cases of cell fusion, a small radius of curvature of the membrane protrusions promotes fusion (Wilson and Snell, 1998). Similarly, the fusion efficiency is higher when vesicles have a smaller radius of curvature (Lentz et al., 1992). Otherwise, several studies showed the involvement of Ezrin in microvilli morphology (Saotome et al., 2004; Bonilha et al., 2006). Moreover, in accordance with our results, in thymoma cells cultured in presence of antisense oligonucleotides complementary to ERM sequences, microvilli disappear, whereas when each one of the ERM sequences was inhibited separately, microvilli remain (Takeuchi et al., 1994). Finally, the involvement of oocyte microvilli in gamete interaction is strongly suggested by the fact that this interaction has been reported to be restricted to the microvilli-rich region (Yanagimachi, 1978; Runge et al., 2007). In addition, consistently with our results, the radius of curvature of microvillar tips on wild-type oocytes was found to be half that of the Cd9-null oocytes (Runge et al., 2007). But contrary to our results, Cd9-knockout oocytes were also shown to have denser arrays of microvilli than wild-type oocytes. Surprisingly, we did not observe any difference in terms of density of microvilli after injection of ERM or EWI-2/EWI-F siRNA whereas this decreased after the combined injection of the two (Mix siRNA). This result seems to indicate that ERM and EWI-2/EWI-F would be involved in the formation of microvilli while CD9 would negatively regulate this one, probably by acting indirectly on the organization of other proteins. The absence of this negative regulation in the context of Cd9-KO could explain microvilli density increase.
If a correlation between the increase in the radius of curvature of the microvilli and the decrease in the fertilizing ability of the oocytes seems to be rational and very likely (Runge et al., 2007), the link between the decrease in cortical tension and that in the fertilization index is more questionable. Cortical tension measures the mechanical characteristics of both the membrane and the subcortical cytoskeleton. But the major contribution of cortical tension comes from the cytoskeleton. Therefore, an inhibition of the links between the two may result in a slight drop in cortical tension. Indeed, the difference we obtained in terms of cortical tension between the oocytes microinjected by CTRL siRNAs and those microinjected by EWI-2/EWI-F and ERM siRNAs were too small to explain the important difference in fertilization index between these two groups. Especially since even more important differences of cortical tension, such as those observed between GV and metaphase I or metaphase II oocytes do not completely prevent these oocytes, whatever their stage of maturation, from fusing with sperm (Kryzak et al., 2013). However, it is possible that optimal adhesion/fusion during fertilization requires a narrow range of cortical tension, but additional investigations are necessary to prove it.
Since we cannot totally exclude the existence of other molecules able to overcome the lack of EWI-2/EWI-F or ERM proteins, the confirmation of redundancy mechanism on one hand between EWI-2 and EWI-F and on the other hand between Ezrin, Radixin and Moesin remains to be provided by experiments using EWI-2 and EWI-F double-knockout mice and ERM conditional oocyte specific triple-knockout mice respectively.
In conclusion, we highlighted here the role of oocyte EWI-2, EWI-F and ERM in fertilization, specifically in the step of gamete adhesion/fusion. The ERM and EWI-2/EWI-F proteins seem involved in gamete interaction through their role in the formation and maintenance of functional oocyte microvilli. These results confirm the role of microvilli in gamete interaction and support the hypothesis of the existence of a link between CD9 and the cytoskeleton through EWI-2/EWI-F and ERM proteins.
Data Availability Statement
The original contributions presented in the study are included in the article, further inquiries can be directed to the corresponding author.
Ethics Statement
The animal study was reviewed and approved by Local and governmental ethical review committees: Authorization APAFIS #14124-2017072510448522, A. AZ (2018–2023).
Author Contributions
Conceived and designed the experiments: AZ and CG. Performed the experiments: JC, LW, SM, CI-R, BL, CG, and AZ. Analyzed the data: JC, SM, LW, SB, CG and AZ. Contributed reagents/materials/analysis tools: JC, LW, SM, CI-R, SB, BL, CG and AZ. Wrote the paper: AZ, SB, BL, and CG.
Funding
This research received no external funding. This work was supported by the Institut National de la Santé et de la Recherche Médicale (INSERM), the Centre National de la Recherche Scientifique (CNRS) and Université de Paris.
Conflict of Interest
The authors declare that the research was conducted in the absence of any commercial or financial relationships that could be construed as a potential conflict of interest.
Publisher’s Note
All claims expressed in this article are solely those of the authors and do not necessarily represent those of their affiliated organizations, or those of the publisher, the editors and the reviewers. Any product that may be evaluated in this article, or claim that may be made by its manufacturer, is not guaranteed or endorsed by the publisher.
Acknowledgments
The authors thank A. Schmitt for Electron Microscopy analysis (Plate-Forme Electron Microscopy, Institut Cochin, Paris, France). JC was supported by a fellowship from La Fondation pour la Recherche Médicale (DEA20110621579). LW was supported by the Shanghai Jiao Tong University School of Medicine.
References
Aydin, H., Sultana, A., Li, S., Thavalingam, A., and Lee, J. E. (2016). Molecular Architecture of the Human Sperm IZUMO1 and Egg JUNO Fertilization Complex. Nature 534 (7608), 562–565. doi:10.1038/nature18595
Barrero-Villar, M., Cabrero, J. R., Gordón-Alonso, M., Barroso-González, J., Álvarez-Losada, S., Muñoz-Fernández, M. A., et al. (2009). Moesin Is Required for HIV-1-Induced CD4-CXCR4 Interaction, F-Actin Redistribution, Membrane Fusion and Viral Infection in Lymphocytes. J. Cel Sci 122 (Pt 1), 103–113. doi:10.1242/jcs.035873
Benammar, A., Ziyyat, A., Lefèvre, B., and Wolf, J.-P. (2017). Tetraspanins and Mouse Oocyte Microvilli Related to Fertilizing Ability. Reprod. Sci. 24 (7), 1062–1069. doi:10.1177/1933719116678688
Bennabi, I., Crozet, F., Nikalayevich, E., Chaigne, A., Letort, G., Manil-Ségalen, M., et al. (2020). Artificially Decreasing Cortical Tension Generates Aneuploidy in Mouse Oocytes. Nat. Commun. 11 (1), 1649. doi:10.1038/s41467-020-15470-y
Bianchi, E., Doe, B., Goulding, D., and Wright, G. J. (2014). Juno Is the Egg Izumo Receptor and Is Essential for Mammalian Fertilization. Nature 508 (7497), 483–487. doi:10.1038/nature13203
Bonilha, V. L., Rayborn, M. E., Saotome, I., McClatchey, A. I., and Hollyfield, J. G. (2006). Microvilli Defects in Retinas of Ezrin Knockout Mice. Exp. Eye Res. 82 (4), 720–729. doi:10.1016/j.exer.2005.09.013
Chaigne, A., Campillo, C., Gov, N. S., Voituriez, R., Azoury, J., Umaña-Diaz, C., et al. (2013). A Soft Cortex Is Essential for Asymmetric Spindle Positioning in Mouse Oocytes. Nat. Cel Biol 15 (8), 958–966. doi:10.1038/ncb2799
Chaigne, A., Campillo, C., Gov, N. S., Voituriez, R., Sykes, C., Verlhac, M. H., et al. (2015). A Narrow Window of Cortical Tension Guides Asymmetric Spindle Positioning in the Mouse Oocyte. Nat. Commun. 6, 6027. doi:10.1038/ncomms7027
Chalbi, M., Barraud-Lange, V., Ravaux, B., Howan, K., Rodriguez, N., Soule, P., et al. (2014). Binding of Sperm Protein Izumo1 and its Egg Receptor Juno Drives Cd9 Accumulation in the Intercellular Contact Area Prior to Fusion during Mammalian Fertilization. Development 141 (19), 3732–3739. doi:10.1242/dev.111534
Charrin, S., Le Naour, F., Labas, V., Billard, M., Le Caer, J. P., Emile, J. F., et al. (2003). EWI-2 Is a New Component of the Tetraspanin Web in Hepatocytes and Lymphoid Cells. Biochem. J. 373 (Pt 2), 409–421. doi:10.1042/BJ20030343
Charrin, S., Jouannet, S., Boucheix, C., and Rubinstein, E. (2014). Tetraspanins at a Glance. J. Cel Sci 127 (Pt 17), 3641–3648. doi:10.1242/jcs.154906
Charrin, S., Le Naour, F., Oualid, M., Billard, M., Faure, G., Hanash, S. M., et al. (2001). The Major CD9 and CD81 Molecular Partner. J. Biol. Chem. 276 (17), 14329–14337. doi:10.1074/jbc.m011297200
Doi, Y., Itoh, M., Yonemura, S., Ishihara, S., Takano, H., Noda, T., et al. (1999). Normal Development of Mice and Unimpaired Cell Adhesion/cell Motility/actin-Based Cytoskeleton without Compensatory Up-Regulation of Ezrin or Radixin in Moesin Gene Knockout. J. Biol. Chem. 274 (4), 2315–2321. doi:10.1074/jbc.274.4.2315
Ellerman, D. A., Ha, C., Primakoff, P., Myles, D. G., and Dveksler, G. S. (2003). Direct Binding of the Ligand PSG17 to CD9 Requires a CD9 Site Essential for Sperm-Egg Fusion. MBoC 14 (12), 5098–5103. doi:10.1091/mbc.e03-04-0244
Evans, J. P., and Robinson, D. N. (2018). Micropipette Aspiration of Oocytes to Assess Cortical Tension. Methods Mol. Biol. 1818, 163–171. doi:10.1007/978-1-4939-8603-3_17
Fehon, R. G., McClatchey, A. I., and Bretscher, A. (2010). Organizing the Cell Cortex: the Role of ERM Proteins. Nat. Rev. Mol. Cel Biol 11 (4), 276–287. doi:10.1038/nrm2866
Glazar, A. I., and Evans, J. P. (2009). Immunoglobulin Superfamily Member IgSF8 (EWI-2) and CD9 in Fertilisation: Evidence of Distinct Functions for CD9 and a CD9-Associated Protein in Mammalian Sperm - Egg Interaction. Reprod. Fertil. Dev. 21 (2), 293–303. doi:10.1071/rd08158
Gordón-Alonso, M., Sala-Valdés, M., Rocha-Perugini, V., Pérez-Hernández, D., López-Martín, S., Ursa, A., et al. (2012). EWI-2 Association with α-Actinin Regulates T Cell Immune Synapses and HIV Viral Infection. J.I. 189 (2), 689–700. doi:10.4049/jimmunol.1103708
He, Z.-Y., Gupta, S., Myles, D., and Primakoff, P. (2009). Loss of Surface EWI-2 on CD9 Null Oocytes. Mol. Reprod. Dev. 76 (7), 629–636. doi:10.1002/mrd.20991
Inoue, N., Nishikawa, T., Ikawa, M., and Okabe, M. (2012). Tetraspanin-interacting Protein IGSF8 Is Dispensable for Mouse Fertility. Fertil. Sterility 98 (2), 465–470. doi:10.1016/j.fertnstert.2012.04.029
Jankovics, F., Sinka, R., Lukácsovich, T., and Erdélyi, M. (2002). MOESIN Crosslinks Actin and Cell Membrane in Drosophila Oocytes and Is Required for OSKAR Anchoring. Curr. Biol. 12 (23), 2060–2065. doi:10.1016/s0960-9822(02)01256-3
Jegou, A., Ziyyat, A., Barraud-Lange, V., Perez, E., Wolf, J. P., Pincet, F., et al. (2011). CD9 Tetraspanin Generates Fusion Competent Sites on the Egg Membrane for Mammalian Fertilization. Proc. Natl. Acad. Sci. 108 (27), 10946–10951. doi:10.1073/pnas.1017400108
Kaji, K., Oda, S., Shikano, T., Ohnuki, T., Uematsu, Y., Sakagami, J., et al. (2000). The Gamete Fusion Process Is Defective in Eggs of Cd9-Deficient Mice. Nat. Genet. 24 (3), 279–282. doi:10.1038/73502
Kikuchi, S., Hata, M., Fukumoto, K., Yamane, Y., Matsui, T., Tamura, A., et al. (2002). Radixin Deficiency Causes Conjugated Hyperbilirubinemia with Loss of Mrp2 from Bile Canalicular Membranes. Nat. Genet. 31 (3), 320–325. doi:10.1038/ng905
Kryzak, C. A., Moraine, M. M., Kyle, D. D., Lee, H. J., Cubeñas-Potts, C., Robinson, D. N., et al. (2013). Prophase I Mouse Oocytes Are Deficient in the Ability to Respond to Fertilization by Decreasing Membrane Receptivity to Sperm and Establishing a Membrane Block to Polyspermy1. Biol. Reprod. 89 (2), 44. doi:10.1095/biolreprod.113.110221
Larson, S. M., Lee, H. J., Hung, P.-h., Matthews, L. M., Robinson, D. N., and Evans, J. P. (2010). Cortical Mechanics and Meiosis II Completion in Mammalian Oocytes Are Mediated by Myosin-II and Ezrin-Radixin-Moesin (ERM) Proteins. MBoC 21 (18), 3182–3192. doi:10.1091/mbc.e10-01-0066
Le Naour, F., Rubinstein, E., Jasmin, C., Prenant, M., and Boucheix, C. (2000). Severely Reduced Female Fertility in CD9-Deficient Mice. Science 287 (5451), 319–321. doi:10.1126/science.287.5451.319
Lee, J.-H., Katakai, T., Hara, T., Gonda, H., Sugai, M., and Shimizu, A. (2004). Roles of P-ERM and Rho-ROCK Signaling in Lymphocyte Polarity and Uropod Formation. J. Cel Biol 167 (2), 327–337. doi:10.1083/jcb.200403091
Lentz, B. R., McIntyre, G. F., Parks, D. J., Yates, J. C., and Massenburg, D. (1992). Bilayer Curvature and Certain Amphipaths Promote Poly(ethylene Glycol)-Induced Fusion of Dipalmitoylphosphatidylcholine Unilamellar Vesicles. Biochemistry 31 (10), 2643–2653. doi:10.1021/bi00125a003
Louvet, S., Aghion, J., Santa-Maria, A., Mangeat, P., and Maro, B. (1996). Ezrin Becomes Restricted to Outer Cells Following Asymmetrical Division in the Preimplantation Mouse Embryo. Developmental Biol. 177 (2), 568–579. doi:10.1006/dbio.1996.0186
Louvet-Vallée, S. (2000). ERM Proteins: from Cellular Architecture to Cell Signaling. Biol. Cel 92 (5), 305–316. doi:10.1016/s0248-4900(00)01078-9
McMahon, H. T., and Gallop, J. L. (2005). Membrane Curvature and Mechanisms of Dynamic Cell Membrane Remodelling. Nature 438 (7068), 590–596. doi:10.1038/nature04396
Miyado, K., Yamada, G., Yamada, S., Hasuwa, H., Nakamura, Y., Ryu, F., et al. (2000). Requirement of CD9 on the Egg Plasma Membrane for Fertilization. Science 287 (5451), 321–324. doi:10.1126/science.287.5451.321
Polesello, C., Delon, I., Valenti, P., Ferrer, P., and Payre, F. (2002). Dmoesin Controls Actin-Based Cell Shape and Polarity during Drosophila melanogaster Oogenesis. Nat. Cel Biol 4 (10), 782–789. doi:10.1038/ncb856
Rubinstein, E., Ziyyat, A., Prenant, M., Wrobel, E., Wolf, J.-P., Levy, S., et al. (2006). Reduced Fertility of Female Mice Lacking CD81. Developmental Biol. 290 (2), 351–358. doi:10.1016/j.ydbio.2005.11.031
Runge, K. E., Evans, J. E., He, Z.-Y., Gupta, S., McDonald, K. L., Stahlberg, H., et al. (2007). Oocyte CD9 Is Enriched on the Microvillar Membrane and Required for normal Microvillar Shape and Distribution. Developmental Biol. 304 (1), 317–325. doi:10.1016/j.ydbio.2006.12.041
Sala-Valdés, M., Ursa, Á., Charrin, S., Rubinstein, E., Hemler, M. E., Sánchez-Madrid, F., et al. (2006). EWI-2 and EWI-F Link the Tetraspanin Web to the Actin Cytoskeleton through Their Direct Association with Ezrin-Radixin-Moesin Proteins. J. Biol. Chem. 281 (28), 19665–19675. doi:10.1074/jbc.m602116200
Saotome, I., Curto, M., and McClatchey, A. I. (2004). Ezrin Is Essential for Epithelial Organization and Villus Morphogenesis in the Developing Intestine. Developmental Cel 6 (6), 855–864. doi:10.1016/j.devcel.2004.05.007
Serrador, J. M., Alonso-Lebrero, J. L., Pozo, M. A. d., Furthmayr, H., Schwartz-Albiez, R., Calvo, J., et al. (1997). Moesin Interacts with the Cytoplasmic Region of Intercellular Adhesion Molecule-3 and Is Redistributed to the Uropod of T Lymphocytes during Cell Polarization. J. Cel Biol 138 (6), 1409–1423. doi:10.1083/jcb.138.6.1409
Stipp, C. S., Kolesnikova, T. V., and Hemler, M. E. (2001). EWI-2 Is a Major CD9 and CD81 Partner and Member of a Novel Ig Protein Subfamily. J. Biol. Chem. 276 (44), 40545–40554. doi:10.1074/jbc.m107338200
Takeuchi, K., Sato, N., Kasahara, H., Funayama, N., Nagafuchi, A., Yonemura, S., et al. (1994). Perturbation of Cell Adhesion and Microvilli Formation by Antisense Oligonucleotides to ERM Family Members. J. Cel Biol 125 (6), 1371–1384. doi:10.1083/jcb.125.6.1371
Umeda, R., Satouh, Y., Takemoto, M., Nakada-Nakura, Y., Liu, K., Yokoyama, T., et al. (2020). Structural Insights into Tetraspanin CD9 Function. Nat. Commun. 11 (1), 1606. doi:10.1038/s41467-020-15459-7
Wilson, N. F., and Snell, W. J. (1998). Microvilli and Cell-Cell Fusion during Fertilization. Trends Cel Biol. 8 (3), 93–96. doi:10.1016/s0962-8924(98)01234-3
Yanagimachi, R. (1978). Chapter 4 Sperm-Egg Association in Mammals. Curr. Top. Dev. Biol. 12, 83–105. doi:10.1016/s0070-2153(08)60594-3
Yanez, L. Z., Han, J., Behr, B. B., Pera, R. A. R., and Camarillo, D. B. (2016). Human Oocyte Developmental Potential Is Predicted by Mechanical Properties within Hours after Fertilization. Nat. Commun. 7, 10809. doi:10.1038/ncomms10809
Yonemura, S., Tsukita, S., and Tsukita, S. (1999). Direct Involvement of Ezrin/radixin/moesin (ERM)-binding Membrane Proteins in the Organization of Microvilli in Collaboration with Activated ERM Proteins. J. Cel Biol 145 (7), 1497–1509. doi:10.1083/jcb.145.7.1497
Keywords: CD9, EWI-2, EWI-F, ERM, fertilization, microvilli, cortical tension
Citation: Cohen J, Wang L, Marques S, Ialy-Radio C, Barbaux S, Lefèvre B, Gourier C and Ziyyat A (2022) Oocyte ERM and EWI Proteins Are Involved in Mouse Fertilization. Front. Cell Dev. Biol. 10:863729. doi: 10.3389/fcell.2022.863729
Received: 27 January 2022; Accepted: 25 February 2022;
Published: 14 March 2022.
Edited by:
Dolores Busso, University of the Andes, ChileReviewed by:
Debora Cohen, Consejo Nacional de Investigaciones Científicas y Técnicas (CONICET), ArgentinaWilliam Kinsey, University of Kansas Medical Center, United States
Copyright © 2022 Cohen, Wang, Marques, Ialy-Radio, Barbaux, Lefèvre, Gourier and Ziyyat. This is an open-access article distributed under the terms of the Creative Commons Attribution License (CC BY). The use, distribution or reproduction in other forums is permitted, provided the original author(s) and the copyright owner(s) are credited and that the original publication in this journal is cited, in accordance with accepted academic practice. No use, distribution or reproduction is permitted which does not comply with these terms.
*Correspondence: A Ziyyat, YWhtZWQueml5eWF0QHUtcGFyaXMuZnI=
†These authors share first authorship
‡These authors share senior authorship