- 1Department of Orthopaedics, Nanfang Hospital, Southern Medical University, Guangzhou, China
- 2Guangdong Provincial Key Laboratory of Bone and Cartilage Regenerative Medicine, Nanfang Hospital, Southern Medical University, Guangzhou, China
- 3Department of Respiratory and Critical Care Medicine, Nanfang Hospital, Southern Medical University, Guangzhou, China
As the population of most nations have a large proportion of older individuals, there is an increase in the prevalence of osteoporosis. Consequently, scientists have focused their attention on the pathogenic mechanisms of osteoporosis. Owing to an increase in studies on cellular senescence in recent years, research has begun to focus on the function of the senescent microenvironment in osteoporosis. With chronic inflammation, senescent cells in the bone marrow secrete a series of factors known as senescence-associated secretory phenotype (SASP) factors, acting on their own or surrounding healthy cells and consequently exacerbating ageing.The components of the SASP may differ depending on the cause of osteoporosis. This review aimed to summarize the relationship between SASP factors and osteoporosis and suggest new insights into the mechanistic investigation of osteoporosis.
Introduction
Osteoporosis is a systemic skeletal condition characterized by decreased bone mass and degeneration of the bone tissue microstructure. Osteoporosis-related fractures are becoming more prevalent in the elderly and result in a series of problems and a higher mortality risk. There is a strong association between ageing and osteoporosis (Li et al., 2021). New approaches to osteoporosis treatment have resulted from research on the fundamental mechanisms of bone resorption and production (Compston et al., 2019). Cellular senescence was first proposed by Hayflick and Moorhead (1961) (Hayflick and Moorhead 1961) and is described by cell cycle arrest. Cellular senescence occurs when cells lose the ability to proliferate and differentiate over time or in response to external stresses (Lopez-Otin et al., 2013). Cell death due to senescence and growth of new cells in living organisms are in a dynamic balance (Perez-Figueroa et al., 2021). The connection between cellular senescence and chronic disease is associated with a variety of chronic diseases such as atherosclerosis, diabetes, and osteoporosis (Stojanovic et al., 2020; Crespo-Garcia et al., 2021; Deng et al., 2021)). The concept of the senescence-associated secretory phenotype (SASP) was first introduced by Jean-Philippe Copper in 2008 for research on human malignancies. This research demonstrated that senescent cells can promote precancerous cells to become cancerous by secreting some substances (Coppe et al., 2008). These substances were defined as SASP factors (Coppe et al., 2008). In recent years, the role of SASP factors in the disease microenvironment has been emphasized in the study of tumors and chronic inflammatory diseases (Acosta et al., 2013). SASP has detrimental paracrine and systemic effects in chronic inflammation, including the induction of senescence in healthy cells (Coppe et al., 2008). By contrast, recent research has demonstrated that senescent human dermal fibroblasts accelerate the healing of keratinocyte scratches and stimulate fibroblast differentiation (Hou and Kim 2018). The SASP factors can also recruit and activate immune cells during tumour development (Acosta et al., 2013).
Senescence-Associated Secretory Phenotype Functional Classification
The SASP affects cellular interactions in vivo and is inextricably linked to cellular senescence, ageing, and age-related diseases (Hubackova et al., 2012; Zhao et al., 2021). The SASP factors can activate the body’s immune system (Jin et al., 2021). This activation may promote the repair of damaged tissues or contribute to a chronic inflammatory response (Jin et al., 2021). Chronic inflammation is associated with many age-related diseases, such as cardiovascular diseases (Ritschka et al., 2017; Ferrucci and Fabbri 2018). The composition of SASP depends on the cell type and the nature of the initial stimulus (Di Micco et al., 2021). Although the core components remain similar, there are differences in the quality and quantity of the SASP in different tissues and ageing models (Aquino-Martinez et al., 2020; Di Micco et al., 2021). The SASP is composed of a series of proinflammatory factors, chemokines, growth factors and proteases, which is produced by stimulation of multiple factors in vivo and ex vivo when cells become senescent (Ortiz-Montero et al., 2017; Wang et al., 2019; Basisty et al., 2020; Kawagoe et al., 2020). These in vivo and ex vivo factors include tumour necrosis factor-α(TNF-α), interleukin (IL)-6, IL-1, IL-8, matrix metalloproteinase (MMP), granulocyte colony-stimulating factor (G-CSF) and plasminogen activator inhibitor-1 (PAI-1) (Coppe et al., 2008; Lim et al., 2015; Maciel-Baron et al., 2016; Oubaha et al., 2016; Ruscetti et al., 2018; You et al., 2019) (Table 1). Depending on the type and function of the SASP components, they can be divided into the following categories: Table 1
The Biological Role of Senescence-Associated Secretory Phenotype
The SASP can have both positive and negative effects on an organism (Ritschka et al., 2017). Cellular senescence and the SASP can repair cells, restore tissue integrity, and promote wound healing (Demaria et al., 2014). It has also been shown that cellular senescence inhibits tumor growth by inhibiting cell proliferation and differentiation (Acosta et al., 2008). However, senescent cells do not lose their ability to interact with other cells and can secrete factors that activate the immune system (Mosteiro et al., 2016). Senescent cells can recruit large numbers of immune cells such as macrophages and natural killer cells to remove senescent cells (Mosteiro et al., 2016). Simultaneously, senescent cells can release cytokines that transmit senescence signals to surrounding cells to inhibit the proliferation of senescent cells (Hubackova et al., 2012). SASP factor release occurs due to the DNA damage response triggered by external stimuli (Slawinska and Krupa 2021). Recently, research has focused on the role of SASP in chronic degenerative diseases, such as neurodegenerative lesions, osteoarthritis, and osteoporosis (Faust et al., 2020; Sharma et al., 2020; Gaikwad et al., 2021).
The Role of Senescence-Associated Secretory Phenotype in Bone Loss
Senescence-Associated Secretory Phenotype in the Bone Marrow Cavity
In the field of age-related osteoporosis research, the study of SASP in the bone marrow senescent microenvironment is still in its early stages. The presence of senescent cells and their release of SASP factors were demonstrated in an age-related osteoporosis mouse model (Farr et al., 2016). This study extracted cells from the marrow cavities of young and old mice and demonstrated that several SASP factor mRNA levels were increased in osteoblasts (e.g., Mmp12, Mmp3, etc.). Given that osteocytes are key factors in bone remodeling, the role of senescent osteocytes and their production of SASP factors may help explain the pathogenesis of age-related bone loss (Farr et al., 2016). Evidence suggests that age-related bone loss can be attenuated by eliminating senescent cells from the bone marrow microenvironment in vivo. In vitro experiments have shown that conditioned media produced by senescent cells suppress osteoblast mineralization and that this process can be alleviated by JAK inhibitors (Figure 1A). These experiments suggest that cellular senescence and the release of SASP factors may play key roles in age-related osteoporosis (Farr et al., 2017) (Figure 1B). Radiation-induced bone loss has received increasing attention in recent years and some scholars have investigated specific cells that are associated with the pathways involved. Evidence suggests that radiation causes bone marrow mesenchymal stem cells (BMSCs) to senesce and activates the januskinase 1/signal transducer and activator of transcription 3 pathway in these cells, which, in turn, secrete SASP factors. The conditioned medium of senescent BMSCs was shown to have a negative effect on osteogenic differentiation. By contrast, the addition of a JAK1 inhibitor to the medium of senescent BMSCs can decrease the senescent cell secretion of negative SASP factors and slow down the adverse effects on osteoblast osteogenic differentiation (Bai et al., 2020). Radiation may also lead to the release of SASP factors from ageing osteoblasts and act on BMSCs to interfere with their osteogenic differentiation. The osteogenic differentiation potential of BMSCs is affected by the release of SASP factors through the paracrine pathway when ageing murine long bone osteocyte Y4 (MLO-Y4) cells are induced by radiation (Xu et al., 2021) (Figure 1C). However, treatment of ageing MLO-Y4 cells with a JAK1 pathway inhibitor blocks the secretion of SASP factors and partially alleviates the inhibition of osteogenic differentiation of BMSCs (Xu et al., 2021). Radiation can also cause the ageing of osteoblasts and BMSCs and lead to the secretion of SASP factors. These SASP factors can then affect osteoblast and BMSC osteogenic differentiation. The effect of the toxic heavy metal cadmium on BMSCs has recently been investigated. Cadmium induced senescence in BMSCs by upregulating the NF-κB signalling pathway, and these cells subsequently released SASP factors. It was also demonstrated that cadmium exposure delayed bone repair and regeneration after cranial defect surgery. This research elucidates the role and mechanism of cadmium in osteoporosis, and it could lead to a new treatment option for cadmium-related bone loss. (Luo et al., 2021). Studies have also demonstrated an association between obesity, ageing, and abnormal skeletal development in offspring. During early maternal pregnancy, maternal obesity can lead to abnormal foetal and postnatal skeletal development. High fat diet-induced maternal obesity reduced foetal skeletal development and enhanced foetal osteoblast senescence signaling. In the bone progenitors of the offspring of pregnant obese, senescent bone progenitors released SASP factors This may be explained by the epigenetic regulation (via histone acetylation) of the genes involved in senescence signalling in developing foetal osteoblasts (Chen et al., 2018).
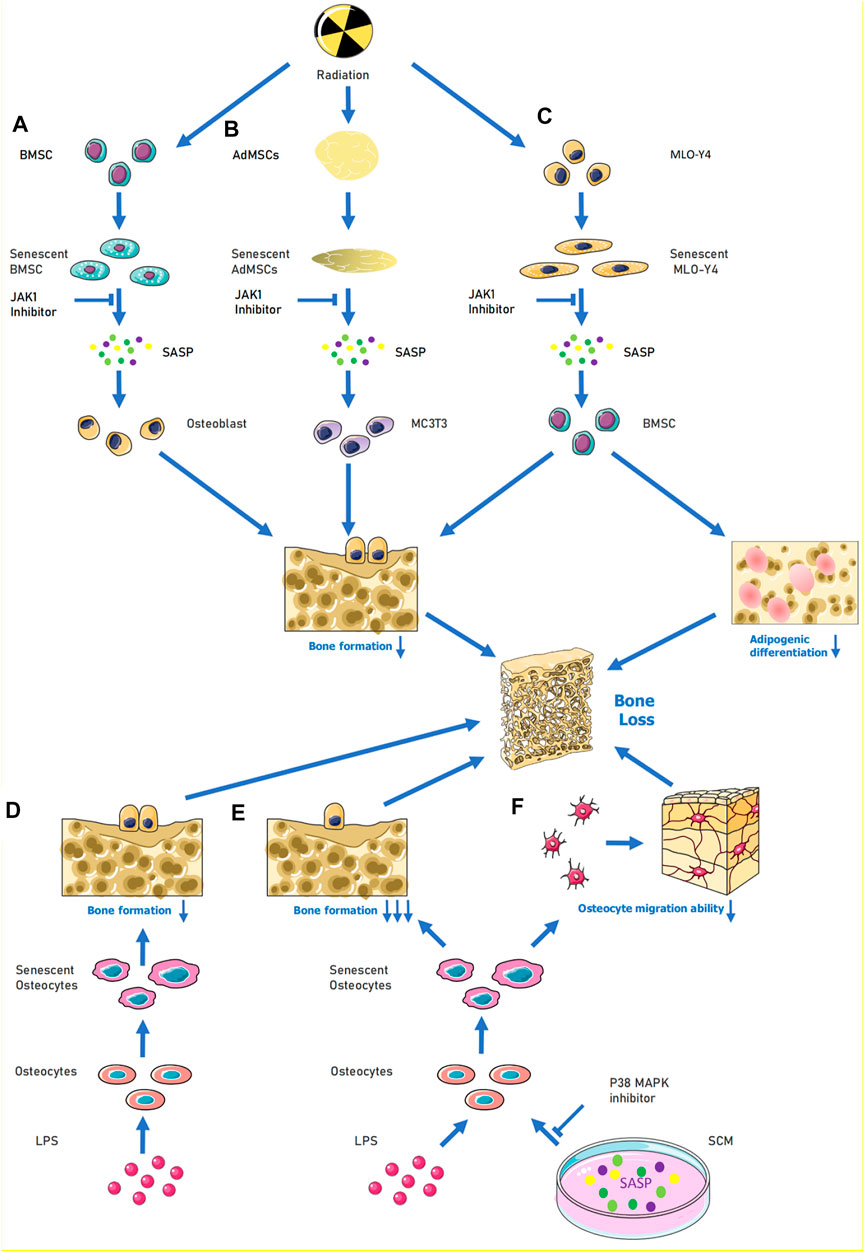
FIGURE 1. When cells are senescence induced by external stimulation, SASP can be released to aggravate their own senescence or induce the senescence of surrounding normal cells. For (A), under the influence of radiation, BMSCs become senescent, causing activation of the JAK1/STAT3 pathway and release of SASP (e.g., IL-6, IL-8, MMP9) to reduce the osteogenic differentiation ability of osteoblasts. (B) When MLO-Y4 cells were irradiated, MLO-Y4 was induced to senesce, which in turn released SASP (e.g,. IL-1α, IL-6, MMP-3, IGFBP-6, etc.) thus affecting the normal growth of BMSC and reducing their osteogenic differentiation ability and adipogenic differentiation ability. In (C), human adipose MSCs were induced to undergo senescence by radiation, and the released SASP could inhibit MC3T3 osteogenic differentiation, and the process could be alleviated by JAK inhibitors. (D–F), LPS acting on osteoblasts induced senescence and thus inhibited osteogenic differentiation of osteoblasts; when LPS and SCM (senescence conditioned medium) were combined to act on osteoblasts, the osteogenic differentiation and migration ability of osteoblasts were greatly reduced, and this process could be alleviated by P38-MAPK inhibitor. Abbreviations: SCM, senescence-conditioned mediators, LPS, Lipopolysaccharide.
Senescence-Associated Secretory Phenotype in Periodontal Tissue
Periodontitis is characterized by chronic inflammation of periodontal supporting tissues and can lead to bone loss in the teeth when inflammation occurs in the alveolar bone and jaws. In a model of hyperglycaemia-induced periodontitis, bone loss has been shown to be associated with ageing. A transgenic diabetic model has demonstrated that periodontal senescence in young diabetic mice is accompanied by the accumulation of senescent macrophages and enhanced early macrophage SASP responses. GLUT1 sensors are important for hyperglycaemia-induced macrophage senescence and SASP responses. Hyperglycaemia-induced macrophage senescence releases SASP factors into other tissues in the periodontium to induce immune responses. This may highlight a potential molecular mechanism of bone loss in diabetic periodontitis (Wang et al., 2021). Periodontitis can also occur due to a progressive change from commensal to pathogenic oral flora. Bacterial-derived lipopolysaccharide (LPS) induces the accumulation of senescent osteoblasts in the alveolar bone of young mice and leads to upregulation of genes (Icam1, Il6, Il17, Mmp13, and Tnfα) involved in SASP. The secretion of SASP factors promotes the proliferation of certain oral bacteria, which, in turn, produce more LPS (Aquino-Martinez et al., 2020). This exacerbates the senescence of alveolar bone cells and may lead to alveolar bone loss (Aquino-Martinez et al., 2020). In age-related alveolar bone loss, the accumulation of senescent bone cells contributes to the deterioration of the periodontal environment by exacerbating chronic inflammation and reducing the regeneration of older bone cells. Moreover, cellular senescence can enhance the inflammation induced by bacterial components. In osteoblasts, IL6, IL17, IGFBP4, and MMP13 levels are significantly higher with age. In vitro senescence-conditioned mediates enhanced LPS-induced expression of IL1α, IL1β, and IL6 in osteoblasts. This, in turn, affects cell migration and osteogenic differentiation These in vitro effects were partially ameliorated by the p38 mitogen-activated protein kinase (MAPK) inhibitor (Aquino-Martinez et al., 2021) (Figure 1D–F). Melatonin protects osteoblasts from ethanol-induced cellular senescence in human alveolar bone and inhibits osteoclast differentiation. Melatonin blocks the ethanol-induced activation of mammalian target of rapamycin, AMP-activated protein kinase, MAPK, and nuclear factor of activated T cells c-1 pathways. This downregulated the expression of SASP-related genes (including Il1β, Il6, Il8, and Tnf) and possibly the secretion of SASP factors, thereby maintaining homeostasis (Bae et al., 2018). This reversed the osteogenic differentiation of the suppressed cells.
Taken together, these studies suggest that cellular senescence and the release of SASP markers by various factors may be key mechanisms leading to senescence-associated bone loss. Therefore, it is important to define the SASP at the proteomic level in senescent cells and to develop ways to slow down the progression of osteoporosis.
Discussion
In the ageing milieu of the bone marrow cavity, SASP is primarily generated by BMSCs and osteoblasts, with senescent cells secreting the majority of SASP factors in the setting of chronic inflammation. Furthermore, SASP that is released from senescent BMSCs and osteoblasts has an extremely inhibitory effect on bone formation. Cells in the bone marrow cavity include BMSCs, osteoblasts and vascular endothelial cells, etc. However, the relationship between SASP that is released from senescent vascular endothelial cells in the bone marrow lumen and bone loss has been less frequently reported. The link between SASP and vascular endothelial cell senescence is extremely strong in other diseases associated with vascular senescence (Prattichizzo et al., 2016). This suggests that senescent vascular endothelial cells may play an important role in senescence-associated osteoporosis. Overall, researchers are now increasingly interested in the role of SASP in osteoporosis, but most studies have concluded that SASP has an inhibitory effect on bone mass formation. Does SASP contribute to bone mass formation under certain conditions? We don’t know, and perhaps subsequent studies will change our current view of SASP. This review also establishes a framework for future work that can investigate the role of SASP in the bone marrow microenvironment.
Author Contributions
RZ drafted the manuscript; RZ, HW, HY and MS drew the chart ; BY and YC supervised the research and revised the manuscript.
Funding
This study was supported by grants from the Major Program of National Natural Science Foundation of China (No. 81830079 to BY), China Postdoctoral Science Foundation (Grant number: 2020M672720), Natural Science Foundation of Guangdong (Grant number: 2019A1515011778), President Foundation of Nanfang Hospital, Southern Medical University (Grant number: 2019Z011).
Conflict of Interest
The authors declare that the research was conducted in the absence of any commercial or financial relationships that could be construed as a potential conflict of interest.
Publisher’s Note
All claims expressed in this article are solely those of the authors and do not necessarily represent those of their affiliated organizations, or those of the publisher, the editors, and the reviewers. Any product that may be evaluated in this article, or claim that may be made by its manufacturer, is not guaranteed or endorsed by the publisher.
Abbreviations
AREG, amphiregulin; BMSCs, bone marrow mesenchymal stem cells; CCL27 cc motif chemokine ligands; ENA-78 epithelial neutrophil-activating protein 78; EGF epidermal growth factor; GRO growth-regulated oncogene; HGF hepatocyte growth factor; IGFBP insulin-like growth factor binding protein; LPS lipopolysaccharide; MIP-3a macrophage inflammatory protein-3 alpha; MMP matrix metalloproteinase; MLO-Y4, murine long bone osteocyte Y4; SASP, senescence-associated secretory phenotype; TIMP tissue inhibitor of metalloproteinases; VEGF vascular endothelial growth factor.
References
Acosta, J. C., Banito, A., Wuestefeld, T., Georgilis, A., Janich, P., Morton, J. P., et al. (2013). A Complex Secretory Program Orchestrated by the Inflammasome Controls Paracrine Senescence. Nat. Cel Biol 15, 978–990. doi:10.1038/ncb2784
Acosta, J. C., O'Loghlen, A., Banito, A., Guijarro, M. V., Augert, A., Raguz, S., et al. (2008). Chemokine Signaling via the CXCR2 Receptor Reinforces Senescence. Cell 133, 1006–1018. doi:10.1016/j.cell.2008.03.038
Alessio, N., Squillaro, T., Di Bernardo, G., Galano, G., De Rosa, R., Melone, M. A., et al. (2020). Increase of Circulating IGFBP-4 Following Genotoxic Stress and its Implication for Senescence. Elife 9. doi:10.7554/elife.54523
Andriani, G. A., Almeida, V. P., Faggioli, F., Mauro, M., Tsai, W. L., Santambrogio, L., et al. (2016). Whole Chromosome Instability Induces Senescence and Promotes SASP. Sci. Rep. 6, 35218. doi:10.1038/srep35218
Aquino-Martinez, R., Eckhardt, B. A., Rowsey, J. L., Fraser, D. G., Khosla, S., Farr, J. N., et al. (2021). Senescent Cells Exacerbate Chronic Inflammation and Contribute to Periodontal Disease Progression in Old Mice. J. Periodontol. 92, 1483–1495. doi:10.1002/JPER.20-0529
Aquino-Martinez, R., Rowsey, J. L., Fraser, D. G., Eckhardt, B. A., Khosla, S., Farr, J. N., et al. (2020). LPS-induced Premature Osteocyte Senescence: Implications in Inflammatory Alveolar Bone Loss and Periodontal Disease Pathogenesis. Bone 132, 115220. doi:10.1016/j.bone.2019.115220
Bae, W. J., Park, J. S., Kang, S. K., Kwon, I. K., and Kim, E. C. (2018). Effects of Melatonin and its Underlying Mechanism on Ethanol-Stimulated Senescence and Osteoclastic Differentiation in Human Periodontal Ligament Cells and Cementoblasts. Int. J. Mol. Sci. 19. doi:10.3390/ijms19061742
Bai, J., Wang, Y., Wang, J., Zhai, J., He, F., and Zhu, G. (2020). Irradiation-induced Senescence of Bone Marrow Mesenchymal Stem Cells Aggravates Osteogenic Differentiation Dysfunction via Paracrine Signaling. Am. J. Physiology-Cell Physiol. 318, C1005–C1017. doi:10.1152/ajpcell.00520.2019
Bao, X., and Hu, X. (2018). Up-regulated Expression of E2F2 Is Necessary for p16INK4a-Induced Cartilage Injury. BMC Musculoskelet. Disord. 19, 334. doi:10.1186/s12891-018-2253-x
Basisty, N., Kale, A., Jeon, O. H., Kuehnemann, C., Payne, T., Rao, C., et al. (2020). A Proteomic Atlas of Senescence-Associated Secretomes for Aging Biomarker Development. Plos Biol. 18, e3000599. doi:10.1371/journal.pbio.3000599
Behnia, F., Taylor, B. D., Woodson, M., Kacerovsky, M., Hawkins, H., Fortunato, S. J., et al. (2015). Chorioamniotic Membrane Senescence: a Signal for Parturition? Am. J. Obstet. Gynecol. 213, 359–416. doi:10.1016/j.ajog.2015.05.041
Cao, Z., Xie, Y., Yu, L., Li, Y., and Wang, Y. (2020). Hepatocyte Growth Factor (HGF) and Stem Cell Factor (SCF) Maintained the Stemness of Human Bone Marrow Mesenchymal Stem Cells (hBMSCs) during Long-Term Expansion by Preserving Mitochondrial Function via the PI3K/AKT, ERK1/2, and STAT3 Signaling Pathways. Stem Cel Res Ther 11, 329. doi:10.1186/s13287-020-01830-4
Chen, J.-R., Lazarenko, O. P., Zhao, H., Alund, A. W., and Shankar, K. (2018). Maternal Obesity Impairs Skeletal Development in Adult Offspring. J. Endocrinol. 239, 33–47. doi:10.1530/joe-18-0244
Compston, J. E., McClung, M. R., and Leslie, W. D. (2019). Osteoporosis. The Lancet 393, 364–376. doi:10.1016/s0140-6736(18)32112-3
Coppé, J. P., Patil, C. K., Rodier, F., Sun, Y., Muñoz, D. P., Goldstein, J., et al. (2008). Senescence-associated Secretory Phenotypes Reveal Cell-Nonautonomous Functions of Oncogenic RAS and the P53 Tumor Suppressor. Plos Biol. 6, 2853–2868. doi:10.1371/journal.pbio.0060301
Crespo-Garcia, S., Tsuruda, P. R., Dejda, A., Ryan, R. D., Fournier, F., Chaney, S. Y., et al. (2021). Pathological Angiogenesis in Retinopathy Engages Cellular Senescence and Is Amenable to Therapeutic Elimination via BCL-xL Inhibition. Cel Metab. 33, 818–832. doi:10.1016/j.cmet.2021.01.011
Degos, C., Heinemann, M., Barrou, J., Boucherit, N., Lambaudie, E., Savina, A., et al. (2019). Endometrial Tumor Microenvironment Alters Human NK Cell Recruitment, and Resident NK Cell Phenotype and Function. Front. Immunol. 10, 877. doi:10.3389/fimmu.2019.00877
Demaria, M., Ohtani, N., Youssef, S. A., Rodier, F., Toussaint, W., Mitchell, J. R., et al. (2014). An Essential Role for Senescent Cells in Optimal Wound Healing through Secretion of PDGF-AA. Dev. Cel 31, 722–733. doi:10.1016/j.devcel.2014.11.012
Deng, P., Yuan, Q., Cheng, Y., Li, J., Liu, Z., Liu, Y., et al. (2021). Loss of KDM4B Exacerbates Bone-Fat Imbalance and Mesenchymal Stromal Cell Exhaustion in Skeletal Aging. Cell Stem Cell 28, 1057–1073. doi:10.1016/j.stem.2021.01.010
Di Micco, R., Krizhanovsky, V., Baker, D., and d’Adda di Fagagna, F. (2021). Cellular Senescence in Ageing: from Mechanisms to Therapeutic Opportunities. Nat. Rev. Mol. Cel Biol 22, 75–95. doi:10.1038/s41580-020-00314-w
Farr, J. N., Fraser, D. G., Wang, H., Jaehn, K., Ogrodnik, M. B., Weivoda, M. M., et al. (2016). Identification of Senescent Cells in the Bone Microenvironment. J. Bone Miner Res. 31, 1920–1929. doi:10.1002/jbmr.2892
Farr, J. N., Xu, M., Weivoda, M. M., Monroe, D. G., Fraser, D. G., Onken, J. L., et al. (2017). Targeting Cellular Senescence Prevents Age-Related Bone Loss in Mice. Nat. Med. 23, 1072–1079. doi:10.1038/nm.4385
Faust, H. J., Zhang, H., Han, J., Wolf, M. T., Jeon, O. H., Sadtler, K., et al. (2020). IL-17 and Immunologically Induced Senescence Regulate Response to Injury in Osteoarthritis. J. Clin. Invest. 130, 5493–5507. doi:10.1172/jci134091
Ferrucci, L., and Fabbri, E. (2018). Inflammageing: Chronic Inflammation in Ageing, Cardiovascular Disease, and Frailty. Nat. Rev. Cardiol. 15, 505–522. doi:10.1038/s41569-018-0064-2
Freudenberg, U., Zieris, A., Chwalek, K., Tsurkan, M. V., Maitz, M. F., Atallah, P., et al. (2015). Heparin Desulfation Modulates VEGF Release and Angiogenesis in Diabetic Wounds. J. Controlled Release 220, 79–88. doi:10.1016/j.jconrel.2015.10.028
Gaikwad, S., Puangmalai, N., Bittar, A., Montalbano, M., Garcia, S., McAllen, S., et al. (2021). Tau Oligomer Induced HMGB1 Release Contributes to Cellular Senescence and Neuropathology Linked to Alzheimer's Disease and Frontotemporal Dementia. Cel Rep. 36, 109419. doi:10.1016/j.celrep.2021.109419
Gao, C., Ning, B., Sang, C., and Zhang, Y. (2018). Rapamycin Prevents the Intervertebral Disc Degeneration via Inhibiting Differentiation and Senescence of Annulus Fibrosus Cells. Aging 10, 131–143. doi:10.18632/aging.101364
Hayflick, L., and Moorhead, P. S. (1961). The Serial Cultivation of Human Diploid Cell Strains. Exp. Cel Res. 25, 585–621. doi:10.1016/0014-4827(61)90192-6
Hilliard, A., Mendonca, P., Russell, T. D., and Soliman, K. F. A. (2020). 'The Protective Effects of Flavonoids in Cataract Formation through the Activation of Nrf2 and the Inhibition of MMP-9. Nutrients 12. doi:10.3390/nu12123651
Hou, J. G., Jeon, B. M., Yun, Y. J., Cui, C. H., and Kim, S. C. (2019). Ginsenoside Rh2 Ameliorates Doxorubicin-Induced Senescence Bystander Effect in Breast Carcinoma Cell MDA-MB-231 and Normal Epithelial Cell MCF-10A. Int. J. Mol. Sci. 20. doi:10.3390/ijms20051244
Hou, J., and Kim, S. (2018). Possible Role of Ginsenoside Rb1 in Skin Wound Healing via Regulating Senescent Skin Dermal Fibroblast. Biochem. Biophysical Res. Commun. 499, 381–388. doi:10.1016/j.bbrc.2018.03.170
Hubackova, S., Krejcikova, K., Bartek, J., and Hodny, Z. (2012). IL1- and TGFβ-Nox4 Signaling, Oxidative Stress and DNA Damage Response Are Shared Features of Replicative, Oncogene-Induced, and Drug-Induced Paracrine 'Bystander Senescence'. Aging 4, 932–951. doi:10.18632/aging.100520
Jin, W.-N., Shi, K., He, W., Sun, J.-H., Van Kaer, L., Shi, F.-D., et al. (2021). Neuroblast Senescence in the Aged Brain Augments Natural Killer Cell Cytotoxicity Leading to Impaired Neurogenesis and Cognition. Nat. Neurosci. 24, 61–73. doi:10.1038/s41593-020-00745-w
Kawagoe, Y., Kawashima, I., Sato, Y., Okamoto, N., Matsubara, K., and Kawamura, K. (2020). CXCL5-CXCR2 Signaling Is a Senescence-Associated Secretory Phenotype in Preimplantation Embryos. Aging Cell 19, e13240. doi:10.1111/acel.13240
Keane, M. P., Belperio, J. A., Burdick, M. D., Lynch, J. P., Fishbein, M. C., and Strieter, R. M. (2001). ENA-78 Is an Important Angiogenic Factor in Idiopathic Pulmonary Fibrosis. Am. J. Respir. Crit. Care Med. 164, 2239–2242. doi:10.1164/ajrccm.164.12.2104106
Li, C.-J., Xiao, Y., Sun, Y.-C., He, W.-Z., Liu, L., Huang, M., et al. (2021). Senescent Immune Cells Release Grancalcin to Promote Skeletal Aging. Cel Metab. 33, 1957–1973. doi:10.1016/j.cmet.2021.08.009
Li, Y., Sun, B., Zhao, X., Wang, X., Zhang, D., Gu, Q., et al. (2017). MMP-2 and MMP-13 Affect Vasculogenic Mimicry Formation in Large Cell Lung Cancer. J. Cel. Mol. Med. 21, 3741–3751. doi:10.1111/jcmm.13283
Lim, H., Park, H., and Kim, H. P. (2015). Effects of Flavonoids on Senescence-Associated Secretory Phenotype Formation from Bleomycin-Induced Senescence in BJ Fibroblasts. Biochem. Pharmacol. 96, 337–348. doi:10.1016/j.bcp.2015.06.013
López-Otín, C., Blasco, M. A., Partridge, L., Serrano, M., and Kroemer, G. (2013). The Hallmarks of Aging. Cell 153, 1194–1217. doi:10.1016/j.cell.2013.05.039
Luo, H., Gu, R., Ouyang, H., Wang, L., Shi, S., Ji, Y., et al. (2021). Cadmium Exposure Induces Osteoporosis through Cellular Senescence, Associated with Activation of NF-Κb Pathway and Mitochondrial Dysfunction. Environ. Pollut. 290, 118043. doi:10.1016/j.envpol.2021.118043
Maciel-Barón, L. A., Morales-Rosales, S. L., Aquino-Cruz, A. A., Triana-Martínez, F., Galván-Arzate, S., Luna-López, A., et al. (2016). Senescence Associated Secretory Phenotype Profile from Primary Lung Mice Fibroblasts Depends on the Senescence Induction Stimuli. Age 38, 26. doi:10.1007/s11357-016-9886-1
Marazita, M. C., Dugour, A., Marquioni-Ramella, M. D., Figueroa, J. M., and Suburo, A. M. (2016). Oxidative Stress-Induced Premature Senescence Dysregulates VEGF and CFH Expression in Retinal Pigment Epithelial Cells: Implications for Age-Related Macular Degeneration. Redox Biol. 7, 78–87. doi:10.1016/j.redox.2015.11.011
Martini, H., Iacovoni, J. S., Maggiorani, D., Dutaur, M., Marsal, D. J., Roncalli, J., et al. (2019). Aging Induces Cardiac Mesenchymal Stromal Cell Senescence and Promotes Endothelial Cell Fate of the CD90 + subset. Aging Cell 18, e13015. doi:10.1111/acel.13015
Matsui, T., Akahoshi, T., Namai, R., Hashimoto, A., Kurihara, Y., Rana, M., et al. (2001). Selective Recruitment of CCR6-Expressing Cells by Increased Production of MIP-3α in Rheumatoid Arthritis. Clin. Exp. Immunol. 125, 155–161. doi:10.1046/j.1365-2249.2001.01542.x
Mosteiro, L., Pantoja, C., Alcazar, N., Marión, R. M., Chondronasiou, D., Rovira, M., et al. (2016). Tissue Damage and Senescence Provide Critical Signals for Cellular Reprogramming In Vivo. Science 354, 354. doi:10.1126/science.aaf4445
Niwa, H., Kanno, Y., Shu, E., and Seishima, M. (2020). Decrease in Matrix Metalloproteinase-3 A-ctivity in S-ystemic S-clerosis F-ibroblasts C-auses α2-antiplasmin and E-xtracellular M-atrix D-eposition, and C-ontributes to F-ibrosis D-evelopment. Mol. Med. Rep. 22, 3001–3007. doi:10.3892/mmr.2020.11358
Orjalo, A. V., Bhaumik, D., Gengler, B. K., Scott, G. K., and Campisi, J. (2009). Cell Surface-Bound IL-1 Is an Upstream Regulator of the Senescence-Associated IL-6/IL-8 Cytokine Network. Proc. Natl. Acad. Sci. 106, 17031–17036. doi:10.1073/pnas.0905299106
Ortiz-Montero, P., Londoño-Vallejo, A., and Vernot, J.-P. (2017). Senescence-associated IL-6 and IL-8 Cytokines Induce a Self- and Cross-Reinforced Senescence/inflammatory Milieu Strengthening Tumorigenic Capabilities in the MCF-7 Breast Cancer Cell Line. Cell Commun Signal 15, 17. doi:10.1186/s12964-017-0172-3
Oubaha, M., Miloudi, K., Dejda, A., Guber, V., Mawambo, G., Germain, M. A., et al. (2016). Senescence-associated Secretory Phenotype Contributes to Pathological Angiogenesis in Retinopathy. Sci. Transl Med. 8, 362ra144. 362ra144. doi:10.1126/scitranslmed.aaf9440
Pérez-Figueroa, E., Álvarez-Carrasco, P., Ortega, E., and Maldonado-Bernal, C. (2021). Neutrophils: Many Ways to Die. Front. Immunol. 12, 631821. doi:10.3389/fimmu.2021.631821
Prattichizzo, F., Giuliani, A., Recchioni, R., Bonafè, M., Marcheselli, F., De Carolis, S., et al. (2016). Anti-TNF-α Treatment Modulates SASP and SASP-Related microRNAs in Endothelial Cells and in Circulating Angiogenic Cells. Oncotarget 7, 11945–11958. doi:10.18632/oncotarget.7858
Ritschka, B., Storer, M., Mas, A., Heinzmann, F., Ortells, M. C., Morton, J. P., et al. (2017). The Senescence-Associated Secretory Phenotype Induces Cellular Plasticity and Tissue Regeneration. Genes Dev. 31, 172–183. doi:10.1101/gad.290635.116
Rohn, F., Kordes, C., Buschmann, T., Reichert, D., Wammers, M., Poschmann, G., et al. (2020). Impaired Integrin α5 /β1 -mediated Hepatocyte Growth Factor Release by Stellate Cells of the Aged Liver. Aging Cell 19, e13131. doi:10.1111/acel.13131
Ruscetti, M., Leibold, J., Bott, M. J., Fennell, M., Kulick, A., Salgado, N. R., et al. (2018). NK Cell-Mediated Cytotoxicity Contributes to Tumor Control by a Cytostatic Drug Combination. Science 362, 1416–1422. doi:10.1126/science.aas9090
Salehinejad, P., Alitheen, N. B., Mandegary, A., Nematollahi-Mahani, S. N., and Janzamin, E. (2013). Effect of EGF and FGF on the Expansion Properties of Human Umbilical Cord Mesenchymal Cells. In Vitro Cell.Dev.Biol.-Animal 49, 515–523. doi:10.1007/s11626-013-9631-3
Schafer, M. J., Zhang, X., Kumar, A., Atkinson, E. J., Zhu, Y., Jachim, S., et al. (2020). The Senescence-Associated Secretome as an Indicator of Age and Medical Risk. JCI Insight 5. doi:10.1172/jci.insight.133668
Severino, V., Alessio, N., Farina, A., Sandomenico, A., Cipollaro, M., Peluso, G., et al. (2013). Insulin-like Growth Factor Binding Proteins 4 and 7 Released by Senescent Cells Promote Premature Senescence in Mesenchymal Stem Cells. Cell Death Dis 4, e911. doi:10.1038/cddis.2013.445
Sharma, A. K., Roberts, R. L., Benson, R. D., Pierce, J. L., Yu, K., Hamrick, M. W., et al. (2020). The Senolytic Drug Navitoclax (ABT-263) Causes Trabecular Bone Loss and Impaired Osteoprogenitor Function in Aged Mice. Front. Cel Dev. Biol. 8, 354. doi:10.3389/fcell.2020.00354
Slawinska, N., and Krupa, R. (2021). 'Molecular Aspects of Senescence and Organismal Ageing-DNA Damage Response, Telomeres, Inflammation and Chromatin. Int. J. Mol. Sci. 22.
Stephan, R. P., Reilly, C. R., and Witte, P. L. (1998). Impaired Ability of Bone Marrow Stromal Cells to Support B-Lymphopoiesis with Age. Blood 91, 75–88. doi:10.1182/blood.v91.1.75.75_75_88
Stojanovic, S. D., Fiedler, J., Bauersachs, J., Thum, T., and Sedding, D. G. (2020). 'Senescence-induced Inflammation: an Important Player and Key Therapeutic Target in Atherosclerosis. Eur. Heart J. 41, 2983–2996.
Wang, Q., Nie, L., Zhao, P., Zhou, X., Ding, Y., Chen, Q., et al. (2021). Diabetes Fuels Periodontal Lesions via GLUT1-Driven Macrophage Inflammaging. Int. J. Oral Sci. 13, 11. doi:10.1038/s41368-021-00116-6
Wang, S.-Y., Wang, W.-J., Liu, J.-Q., Song, Y.-H., Li, P., Sun, X.-F., et al. (2019). Methionine Restriction Delays Senescence and Suppresses the Senescence-Associated Secretory Phenotype in the Kidney through Endogenous Hydrogen Sulfide. Cell Cycle 18, 1573–1587. doi:10.1080/15384101.2019.1618124
Wiley, C. D., Velarde, M. C., Lecot, P., Liu, S., Sarnoski, E. A., Freund, A., et al. (2016). Mitochondrial Dysfunction Induces Senescence with a Distinct Secretory Phenotype. Cel Metab. 23, 303–314. doi:10.1016/j.cmet.2015.11.011
Wu, Y., Tang, Y., Zhang, X., Chu, Z., Liu, Y., and Tang, C. (2020). MMP-1 Promotes Osteogenic Differentiation of Human Bone Marrow Mesenchymal Stem Cells via the JNK and ERK Pathway. Int. J. Biochem. Cel Biol. 129, 105880. doi:10.1016/j.biocel.2020.105880
Xu, L., Wang, Y., Wang, J., Zhai, J., Ren, L., and Zhu, G. (2021). 'Radiation-Induced Osteocyte Senescence Alters Bone Marrow Mesenchymal Stem Cell Differentiation Potential via Paracrine Signaling. Int. J. Mol. Sci. 22. doi:10.3390/ijms22179323
Xu, Q., Long, Q., Zhu, D., Fu, D., Zhang, B., Han, L., et al. (2019). Targeting Amphiregulin (AREG) Derived from Senescent Stromal Cells Diminishes Cancer Resistance and Averts Programmed Cell Death 1 Ligand (PD-L1)-Mediated Immunosuppression. Aging Cell 18, e13027. doi:10.1111/acel.13027
Yang, G., Rosen, D. G., Zhang, Z., Bast, R. C., Mills, G. B., Colacino, J. A., et al. (2006). The Chemokine Growth-Regulated Oncogene 1 (Gro-1) Links RAS Signaling to the Senescence of Stromal Fibroblasts and Ovarian Tumorigenesis. Proc. Natl. Acad. Sci. 103, 16472–16477. doi:10.1073/pnas.0605752103
Yokose, U., Hachiya, A., Sriwiriyanont, P., Fujimura, T., Visscher, M. O., Kitzmiller, W. J., et al. (2012). The Endogenous Protease Inhibitor TIMP-1 Mediates Protection and Recovery from Cutaneous Photodamage. J. Invest. Dermatol. 132, 2800–2809. doi:10.1038/jid.2012.204
You, K., Parikh, P., Khandalavala, K., Wicher, S. A., Manlove, L., Yang, B., et al. (2019). Moderate Hyperoxia Induces Senescence in Developing Human Lung Fibroblasts. Am. J. Physiology-Lung Cell Mol. Physiol. 317, L525–L536. doi:10.1152/ajplung.00067.2019
Keywords: senescence, senescence-associated secretory phenotype (SASP), osteoporosis, bone loss, stem cell
Citation: Zhu R, Wan H, Yang H, Song M, Chai Y and Yu B (2022) The Role of Senescence-Associated Secretory Phenotype in Bone Loss. Front. Cell Dev. Biol. 10:841612. doi: 10.3389/fcell.2022.841612
Received: 22 December 2021; Accepted: 27 January 2022;
Published: 09 February 2022.
Edited by:
Changjun Li, Xiangya Hospital, Central South University, ChinaReviewed by:
Ling Liu, Xiangya Hospital, Central South University, ChinaSeiji Yamamoto, University of Toyama, Japan
Copyright © 2022 Zhu, Wan, Yang, Song, Chai and Yu. This is an open-access article distributed under the terms of the Creative Commons Attribution License (CC BY). The use, distribution or reproduction in other forums is permitted, provided the original author(s) and the copyright owner(s) are credited and that the original publication in this journal is cited, in accordance with accepted academic practice. No use, distribution or reproduction is permitted which does not comply with these terms.
*Correspondence: Bin Yu, eXViaW5Ac211LmVkdS5jbg==; Yu Chai, MTcyNzIzNDUwQHFxLmNvbQ==