- 1Department of Zoology, HNB Garhwal University, A Central University, Srinagar Campus, Uttarakhand, India
- 2Department of Biotechnology, Goa University, Taleigao, India
- 3Department of Biological Sciences, KK Birla, Goa Campus, BITS Pilani, Zuarinagar, India
Germ cells (Gc) propagate the genetic information to subsequent generations. Diploid (2n) Gc get transformed to specialized haploid (n) gametes by mitotic and meiotic divisions in adult gonads. Retinoic acid (RA), an active derivative of vitamin A (retinol), plays a critical role in organ morphogenesis and regulates the meiotic onset in developing Gc. Unlike ovaries, fetal testes express an RA-degrading enzyme CYP26B1, and thereby, male Gc fail to enter into meiosis and instead get arrested at G0/G1 stage, termed as gonocytes/pro-spermatogonia by embryonic (E) 13.5 days. These gonocytes are transformed into spermatogonial stem/progenitor cells after birth (1–3 days of neonatal age). During post-natal testicular maturation, the differentiating spermatogonia enter into the meiotic prophase under the influence RA, independent of gonadotropic (both FSH and LH) support. The first pulse of RA ensures the transition of undifferentiated type A spermatogonia to differentiated A1 spermatogonia and upregulates STRA8 expression in Gc. Whereas, the second pulse of RA induces the meiotic prophase by augmenting MEIOSIN expression in differentiated spermatogonia B. This opinion article briefly reviews our current understanding on the RA-driven spermatogonial differentiation in murine testes.
Introduction
Spermatogenesis occurs within testes where male germ cells (Gc) undergo both mitotic and meiotic divisions to form haploid (n) spermatozoa (Griswold, 2016). This is a tightly regulated asynchronized process, broadly sub-divided into four major developmental stages, namely, 1) pre-meiotic proliferation of undifferentiated spermatogonia, 2) meiotic entry of differentiated spermatogonia 3) meiotic completion and formation of round spermatid, and 4) maturation of elongated spermatid to spermatozoa (Griswold, 2016). Testicular Sertoli cells (Sc) provide the structural and biochemical support to all the stages of male Gc within the seminiferous tubules (Griswold, 2018; Bhattacharya et al., 2019). Both Gc-specific intrinsic and Sc-derived extrinsic factors collectively determine the Gc developmental fate (Griswold, 2018; Bhattacharya et al., 2019).
Retinoic acid (RA) is the oxidized derivative/metabolite of vitamin A [retinol (ROL)] and plays a crucial role in pattern formation during organogenesis (Cunningham and Duester, 2015). In fetal ovaries (not in fetal testes), RA triggers the meiotic onset in developing Gc (Endo et al., 2019). During post-natal testicular maturation, RA induces the transition of undifferentiated spermatogonia A to differentiating spermatogonia A1 and governs the meiotic entry of differentiated spermatogonia B, and this action continues in successive spermatogenic waves (Griswold, 2016). Furthermore, RA also has been shown to be essential for maintaining the remodeling and permeability of blood–testis barrier, meiotic recombination and progression, and spermiation (Gewiss et al., 2020). We here briefly discuss our current understanding on the critical role of RA in regulating male Gc differentiation in fetal and post-natal testes considering mouse as a model organism.
Biosynthesis of Retinoic Acid and Signaling
Vitamin A or ROL is synthesized in the liver and delivered to testes via a complex formed by retinol-binding protein 4 (RBP4) and transthyretin (TT4) (Gewiss et al., 2020). ROL is taken up by the membrane receptor STRA6 (stimulated by retinoic acid gene 6) expressed by Sc (Gewiss et al., 2020). The first-rate limiting step of RA production is the reversible conversion of ROL to retinal (RAL) by NAD+-dependent retinol dehydrogenase 10 (RDH10) expressed by Sc (Gewiss et al., 2020). The second step is an irreversible oxidation of RAL to RA catalyzed by the retinaldehyde dehydrogenase (s) (ALDH1A1, ALDH1A2, and ALDH1A3) expressed by both Sc and meiotic Gc (Gewiss et al., 2020).
RA signaling involves two families of nuclear hormone receptors, namely, the retinoic acid receptors (RARs) and the retinoid X receptors (RXRs), each having three isotypes [RARα, RARβ, and RARγ or RXRα, RXRβ, and RXRγ (Endo et al., 2019; Gewiss et al., 2020)]. In target cells, ligand (i.e., RA)-activated RAR-RXR heterodimers bind to retinoic acid response elements (RAREs) located in the cis-acting regulatory sequences of the RA-responsive genes and induce/modulate their transcriptions (Endo et al., 2019; Gewiss et al., 2020). Figure 1A shows the biosynthesis and signaling of cascade RA.
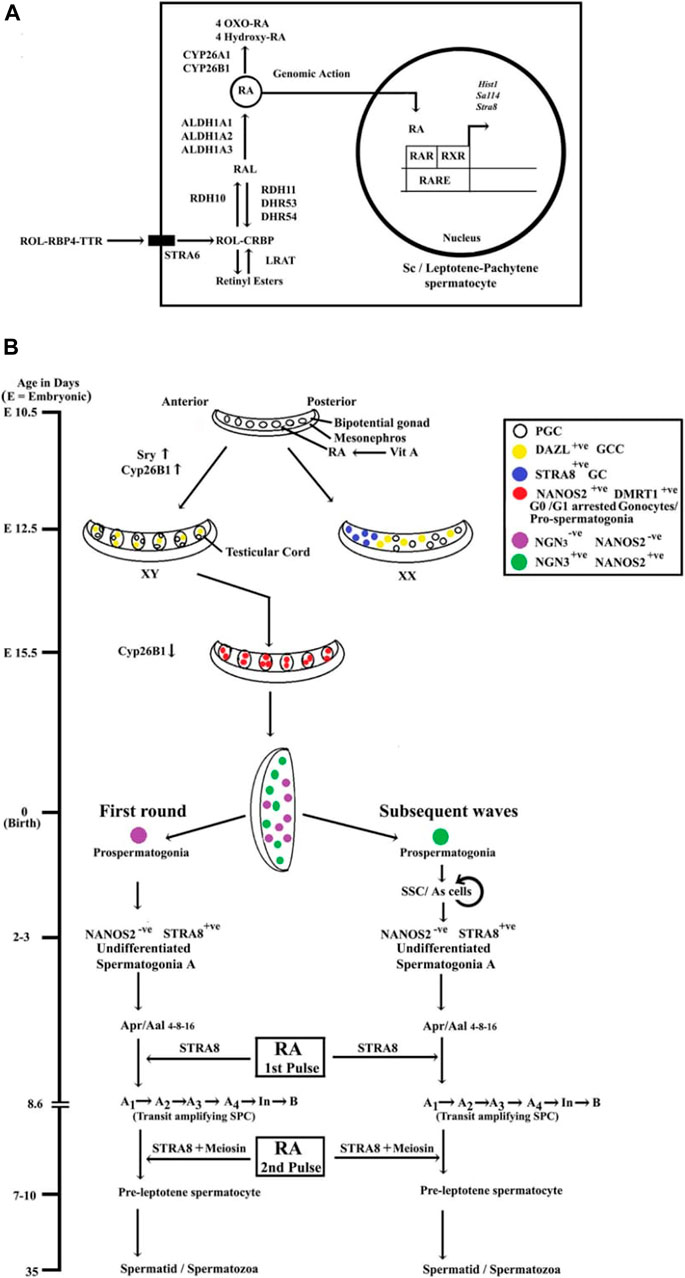
FIGURE 1. (A) The biosynthesis and signaling of RA in testicular Sc and/or meiotic Gc. Vitamin A or retinol (ROL) is synthesized in the liver and delivered to testes via retinol-binding protein 4 (RBP4) and transthyretin (TTR). ROL is uptaken by a membrane-bound receptor termed stimulated by retinoic acid gene 6 (STRA6) expressed by Sc. In Sc, lecithin retinol acyltransferase (LRAT) can convert ROL [bound with cellular retinol-binding protein (CRBP)] into retinyl esters, or ROL gets converted into retinal (RAL) by NAD+-dependent retinol dehydrogenase 10 (RDH10). The reverse step, i.e., conversion of RAL to ROL, is also mediated by NADPH-dependent retinal reductases (RDH11, DHRS3, and DHRS4). In both Sc and meiotic Gc, the second step, i.e., the irreversible oxidation of RAL to RA, is catalyzed by the retinaldehyde dehydrogenase(s) (ALDH1A1, ALDH1A2, and ALDH1A3). RA gets degraded and further oxidized to 4-hydroxy RA/4-oxo-RA by CYP26A1 or CYP26B1 enzymes. RA signaling involves two families of nuclear hormone receptors, namely, the retinoic acid receptors (RARs) and the retinoid X receptors (RXRs). In target cells, RA-activated RAR-RXR heterodimers bind to retinoic acid response elements (RAREs) located in the cis-acting regulatory sequences of the RA-responsive genes (Stra8, Hist1, Sall4, etc.) and induce/modulate their transcriptions. (B) Summary of the developmental time schedule of male Gc development and the cellular target sites of RA actions in murine testes. Retinoic acid (RA) is produced by embryonic mesonephros and diffused to the adjacent bipotential gonad establishing an anterior to posterior gradient. During embryonic (E) 10.5–12.5 days, primordial germ cells (PGC) get transformed into gametogenesis-competent cells (GCC) by induction of RNA-binding proteins Dazl. In XY gonad, which has been differentiated into fetal testis by Sry during E 11.5–12.5 days, RA gets degraded by an enzyme CYP26B1. By E 13.5 days, the Gc get arrested into G0/G1 stage and termed as gonocytes/pro-spermatogonia until birth (day 0). Although the expression of CYP26B1 gets downregulated in fetal testes by E 13.5 days, Gc are maintained at this quiescent stage (G0/G1 stage) of pro-spermatogonia via destabilizing DAZL by an intrinsic factor NANOS2. By post-natal days 2–3, the SSC (designated As spermatogonia) system gets established from neurogenin 3 (NGN3)+ lineage with specific markers, e.g., ID4, GFRa1, NANOS2, etc. Whereas, the first round of differentiated spermatogonia appears from a unique NGN3−, NANOS2−/low lineage of pro-spermatogonia, independent from the SSC pool and directly form the STRA8+ A1 spermatogonial cells. During subsequent spermatogenic waves, STRA8+ A1 spermatogonia originate from the NGN3+, NANOS2+ SSC. The first pulse of RA from Sc (by 2 days of post-natal age) induces the formations of the transit-amplifying spermatogonial progenitor cells (SPC) including A1, A2, A3, A4, intermediate (In), and B spermatogonia population and upregulates STRA8 expression, covering 8.6 days. The second pulse of RA in pre-pubertal testes augments both STRA8 and MEIOSIN, which act as the gatekeepers of meiotic entry for differentiated spermatogonia B.
Origin and Migration of Germ Line Cells in Fetal Testes
In mice, primordial germ cells (PGC) originate from epiblast at embryonic age (E) 6.5 days, whereas the somatic genital ridge (GR) is formed from the coelomic epithelium of the mesonephros by E 8.5–9.5 days (Endo et al., 2019). PGC migrate from the base of the allantois to the GR, and this process is completed by E 10.5–11 days (Endo et al., 2019). Migratory PGC are mitotically active expressing pluripotency markers (e.g., OCT4, SOX2, and NANOG) and can form teratomas (Endo et al., 2019). However, after the arrival at the GR/bipotential gonad, PGC undergo a massive global demethylation during E 10.5–12.5 days (Seisenberger et al., 2012). This extensive epigenetic reprogramming leads to the induction of RNA-binding proteins Dazl (deleted in azoospermia-like) and Ddx4 (DEAD box polypeptide 4, also called mouse vasa homolog), and PGC get transformed into gametogenesis-competent cells (GCC) (Lin et al., 2008). GATA-4 plays a critical role in the formation of somatic GR and substantially supports the licensing of PGC to be responsive towards RA signal (Hu et al., 2015). On E 11.5 days, Sry gene gets activated in SF-1/Nr5a1+ somatic cells of XY embryos, induces the genetic cascade of male programming genes (Sox9, Amh, Fgf9, Dmrt1, etc.) essential for sex determination, and initiates the testicular morphogenesis by influx of mesonephric endothelial/perivascular cells to XY gonads to form male-specific vasculature pattern and eventually testicular cord (Koopman et al., 1990; Martineau et al., 1997; Svingen and Koopman, 2013; DeFalco et al., 2014; Stévant et al., 2018). By E 12.5 days, the fetal Sc are completely specified with SOX9 as molecular marker in fetal testis (Svingen and Koopman, 2013).
Degradation of Retinoic Acid in Fetal Testes
RA is produced by adjacent mesonephros and diffuses through the fetal gonad, establishing a robust anterior-to-posterior gradient (Endo et al., 2019). Under the influence of RA, the GCC enter into meiotic prophase by upregulating transcription factor Stra8 (stimulated by retinoic acid gene 8) and Rec 8 (coding for REC8 meiotic recombination protein) in the XX gonads, which have been specified as fetal ovary by E 12.5 days (Bowles et al., 2006; Koubova et al., 2006; Endo et al., 2019). However, in XY gonad, which is differentiated into fetal testis, male GCS fail to initiate the meiotic prophase due to the degradation of RA by CYP26B1 (Griswold, 2016; Endo et al., 2019). Ectopic expression of STRA8+ meiotic Gc has been observed in CYP26B1-deficient male embryos, confirming the role of RA in inducing Gc meiosis in males (MacLean et al., 2007). By E 13.5 days of age, male Gc get arrested into G0/G1 stage and termed as gonocytes/pro-spermatogonia (Griswold, 2016; Du et al., 2021). However, although the expression of CYP26B1 gets downregulated in fetal testes by E 13.5 days, Gc are maintained at this quiescent stage (G0/G1 stage) of pro-spermatogonia via destabilizing DAZL by Gc intrinsic factor NANOS2 (Griswold, 2016; Endo et al., 2019; Du et al., 2021). It is important here to note that in Nanos2-null XY embryos, Gc initiate ectopic meiosis by expressing STRA8 by E14.5 (Suzuki and Saga, 2008).
Spermatogonial Stem Cell or Spermatogonial Progenitor Cell Population in Neonatal Testes
The pro-spermatogonia or gonocytes form the spermatogonial stem cell (SSC) or spermatogonial progenitor cell (SPC) population by 1–2 days post-birth (Lord and Oatley, 2017; Yoshida, 2018). By post-natal age of 2–3 days, the SSC (designated As spermatogonia) and/or SPC (undifferentiated spermatogonia A) system get established with specific markers for self-renewal (e.g., ID4, PAX7, RHOX5, BMI1, EOMES, GFRA1, NANOS2, UTF1, ZBTB16, SALL4, LIN28, FOXO1, miR-21, and mirR-17∼92/miRc1) (Lord and Oatley, 2017; Kubota and Brinster, 2018; Yoshida, 2018). The specific topological location of the SSC pool and its unique micro-environment restrict the direct action of RA on SSC (Lord and Oatley, 2017; Kubota and Brinster, 2018; Yoshida, 2018). During the initiation of the spermatogonial differentiation, the SSC/SPC first downregulates these self-renewal-associated genes [Id4, Gfra1, Nanos2, Pou5f1, Zbtb16 (Plzf), Lin28, etc.] and upregulates the genes necessary for differentiation like cKit-receptor, Sohlh1/2, Stra8, Ccnd2, and Sall4 (Griswold, 2016; Lord and Oatley, 2017; Kubota and Brinster, 2018; Yoshida, 2018). Notably, the first round of differentiated spermatogonia appears from a unique neurogenin 3 (NGN3)−, NANOS2−/low lineage of pro-spermatogonia, independent from the SSC/SPC pool and directly form the STRA8+ A1 spermatogonial cells (Griswold, 2016; Yoshida, 2018). However, during subsequent spermatogenic waves, STRA8+ A1 spermatogonia originate from the NGN3+ SSC/SPC cells (Griswold, 2016; Yoshida, 2018). Data from hpg mouse (lacking endogenous GnRH) or mutant mice models having altered/ablated gonadotropic functions demonstrate that the initial expansion/differentiation of the spermatogonial population is not dependent on either FSH or LH (Kumar, 2007; Jonas et al., 2014). However, a significant shift in FSH action with dominant cAMP signaling has been shown to be associated with the rapid transition of spermatogonia A to B in maturing rat Sc between 9 and 12 days of post-natal age (Bhattacharya et al., 2012).
The Pulses of Retinoic Acid in Neonatal/Pre-Pubertal Testes
Historically, in vitamin A-deficient (VAD) rodents, the transition of the undifferentiated spermatogonia A into differentiating spermatogonia A1 has been found to be blocked, and exogenous supplementations of RA in VAD animals restore the initiation of spermatogonial differentiation (Wolbach and Howe, 1925; Mitranond et al., 1979; Morales and Griswold, 1987; van Pelt and de Rooij, 1990; McLean et al., 2002). Pharmacological inhibition of the conversion of RAL to RA by bis-(dichloroacetyl)-diamines (BDAD) compounds like WIN 18446 and/or genetic ablations of the RA synthesizing enzymes have confirmed that RA triggers the differentiation of spermatogonial cells in rodents (Griswold, 2016; Gewiss et al., 2020). RA acts on the differentiating spermatogonia A via both classical genomic or non-genomic pathways to induce 1) genes required for G1/S phase transition (e.g., Hist1 and Ccnd2), 2) genes critical for initiating spermatogonial differentiation (c-Kit and Sall4), and 3) genes to promote the meiotic prophase [Stra8 (transcription factor inducing meiotic entry); Rec8 (a component of the cohesin complex critical for chromosomal segregation and synapsis); and subsequently Dmc1, Sycp3, etc.] (Chen et al., 2016). Very recently, another transcription factor having helix-loop-helix (HLH) and high-mobility-group (HMG) domain termed as MEIOSIN has been shown to be a crucial regulator of meiotic initiation (Ishiguro et al., 2020; Oatley and Griswold, 2020). Although both STRA8 and MEIOSIN are induced by RA signaling, the timing of such inductions differs (Oatley and Griswold, 2020). The transcription of Stra8 appears during the transition of Aal to A1 stage with first pulse of RA from Sc (by 2 days of post-natal age), but the Gc do not directly switch over to meiotic prophase and rather continue with six additional mitotic divisions creating the transit-amplifying SPC [A1, A2, A3, A4, intermediate (In), and B spermatogonia] population (Griswold, 2016; Gewiss et al., 2020). These six mitotic divisions occur over a span of exactly 8.6 days in mice testes (Griswold, 2016). During spermatogonial differentiation, RA signaling directly regulates the expression of replication-dependent core histone genes that is critical for entering into S phase (Chen et al., 2016). However, the second pulse of RA induces the transcription of Meiosin and induces the mitosis-to-meiosis switch in B spermatogonia to form pre-leptotene spermatocytes in pre-pubertal testes (Oatley and Griswold, 2020). It is important to note here that, despite an accumulation of undifferentiated spermatogonia and pre-leptotene spermatocyte arrest, the transition of Aal to A1 stage remains unaffected in Stra8 or Meiosin null mice (Endo et al., 2015; Ishiguro et al., 2020). Therefore, RA can be termed as the “driver” whereas Stra8 or Meiosin as gatekeeper/watchman for meiotic onset.
Source of Retinoic Acid
RA employs both juxtracrine/paracrine and autocrine mode of action in testes (Griswold, 2016; Gewiss et al., 2020). Both Sc-derived extrinsic and Gc-specific intrinsic RA collectively determine the Gc developmental fate in adult testes (Griswold, 2016; Gewiss et al., 2020). Although the synthesis of RA occurs in both Sc and pachytene/diplotene spermatocytes (during stages VII–XIII), Sc are the primary site of RA signaling and direct the upregulation of STRA8 during the first round of spermatogenesis (Griswold, 2016; Gewiss et al., 2020). Sc-specific ablation of either Rdh10 or Aldh1a1-3 leads to a complete block of the transition of spermatogonia A to A1 stage (Griswold, 2016; Gewiss et al., 2020). Intriguingly, in Sc-specific Rdh10 mutant mice, Gc do compensate the need of RA as spermatogenesis gets naturally restored in the next round (after 30 days) without any exogenous support of RA (Tong et al., 2013). However, mice with Sc-specific ablation of Aldh1a1, Aldh1a2, and Aldh1a3 require the external stimulation of RA to recover and maintain the spermatogenic continuation (Raverdeau et al., 2012). This data confirmed that RA synthesized/supplied by Sc is sufficient to drive only the first round of spermatogenesis, whereas the Gc intrinsic RA is critically essential for spermatogenic maintenance in subsequent waves (Griswold, 2016; Gewiss et al., 2020). Notably, the lack or unavailability of RA results in the accumulation of SSC or SPC, whereas a high concentration of RA shows no adverse impact on male fertility (Griswold, 2016; Gewiss et al., 2020). Interestingly in post-natal testis, RA-degrading enzymes CYP26A1, CYP26B1, and CYP26C1 are expressed in peritubular myoid cells (PTc) and Gc but not in steroidogenic Leydig cells (Lc) (Gewiss et al., 2020). Gc- or Sc-specific single/double knock-out (KO) mice for Cyp26a1 and Cyp26b1 result into male sub-fertility, confirming the critical requirement of the patchy, non-uniform pulse of RA within the seminiferous tubules in an asynchronous manner for optimal spermatogenesis (Hogarth et al., 2015; Griswold, 2016; Gewiss et al., 2020). It is also important to note that a critical suppressor of RA action on Gc is DMRT1 (double-sex and mab-3-related transcription factor 1) (Matson et al., 2010). Gc-specific Dmrt1 mutant shows precocious induction of STRA8 followed by meiotic entry (Matson et al., 2010).
Concluding Remarks
The pharmacological inhibition of RA biosynthesis and/or in vivo genetic ablations of RA-synthesizing enzymes have confirmed the critical role of RA signaling in regulating male Gc fate decisions. Fetal Sc express CYP26B1 and thereby restrict the action of RA to induce the meiotic onset in male GCG and ensure the formation of mitotically quiescent pro-spermatogonia. Both NANOS2 and DMRT1 act as essential Gc intrinsic factors to prevent the meiotic entry of pro-spermatogonia in late gestation period (after E 13.5 days) and early neonatal age (first 7 days after birth). In post-natal testes, the pulses of RA drives 1) the conversion of undifferentiated spermatogonia A to differentiated A1 stage, 2) the expansion of the transit-amplifying SPC population (spermatogonia A1 to spermatogonia B), and finally 3) the induction of meiotic prophase to form the pre-leptotene spermatocytes. Figure 1B summarizes the developmental time schedule of male Gc and the specific cellular targets of RA in murine testes.
Future Directions
Despite sincere efforts from various groups, limited RA-responsive gene(s) (e.g., Stra8, Rec8, c-Kit, Hist1, Sall4, and Meiosin) having potential/key role(s) in regulating the meiotic onset have been identified up to date in murine testes (Oatley and Griswold, 2020). Therefore, RA-mediated regulatory genetic networks critical for the induction of spermatogonial differentiation and determining the developmental switch for the transition of Gc from mitosis to meiosis are largely unknown (Tan and Wilkinson, 2020). Future studies are thereby required to investigate the in depth-molecular landscape associated with RA signaling cascade in purified spermatogonial cells employing advanced single-cell transcriptomic analyses (Suzuki et al., 2019).
Author Contributions
IB conceived the idea. IB and AB designed the first draft. IB prepared the text and figure and generated the final form with active support from PS, SP, SK, MD, and AB. All authors contributed to the article and approved the submitted version.
Conflict of Interest
The authors declare that the research was conducted in the absence of any commercial or financial relationships that could be construed as a potential conflict of interest.
Publisher’s Note
All claims expressed in this article are solely those of the authors and do not necessarily represent those of their affiliated organizations, or those of the publisher, the editors and the reviewers. Any product that may be evaluated in this article, or claim that may be made by its manufacturer, is not guaranteed or endorsed by the publisher.
Acknowledgments
IB acknowledges the financial support from University Grants Commission (F.30104/2015BSR) and Department of Science and Technology (ECR/2018/000868). AB thanks Science and Engineering Research Board (EMR/2017/004290), New Delhi, India, for financial assistance. However, the funder was not involved in the study design, collection, analysis, interpretation of data, writing of this review.
References
Bhattacharya, I., Pradhan, B. S., Sarda, K., Gautam, M., Basu, S., and Majumdar, S. S. (2012). A Switch in Sertoli Cell Responsiveness to FSH May Be Responsible for Robust Onset of Germ Cell Differentiation during Prepubertal Testicular Maturation in Rats. Am. J. Physiology-Endocrinology Metab. 303 (7), E886–E898. doi:10.1152/ajpendo.00293.2012
Bhattacharya, I., Sen Sharma, S., and Majumdar, S. S. (2019). Pubertal Orchestration of Hormones and Testis in Primates. Mol. Reprod. Dev. 86 (11), 1505–1530. doi:10.1002/mrd.23246
Bowles, J., Knight, D., Smith, C., Wilhelm, D., Richman, J., Mamiya, S., et al. (2006). Retinoid Signaling Determines Germ Cell Fate in Mice. Science 312 (5773), 596–600. doi:10.1126/science.1125691
Chen, Y., Ma, L., Hogarth, C., Wei, G., Griswold, M. D., and Tong, M.-H. (2016). Retinoid Signaling Controls Spermatogonial Differentiation by Regulating Expression of Replication-dependent Core Histone Genes. Development (Cambridge, England) 143 (9), 1502–1511. doi:10.1242/dev.135939
Cunningham, T. J., and Duester, G. (2015). Mechanisms of Retinoic Acid Signalling and its Roles in Organ and Limb Development. Nat. Rev. Mol. Cell Biol 16 (2), 110–123. doi:10.1038/nrm3932
DeFalco, T., Bhattacharya, I., Williams, A. V., Sams, D. M., and Capel, B. (2014). Yolk-sac-derived Macrophages Regulate Fetal Testis Vascularization and Morphogenesis. Proc. Natl. Acad. Sci. 111 (23), E2384–E2393. doi:10.1073/pnas.1400057111
Du, G., Oatley, M. J., Law, N. C., Robbins, C., Wu, X., and Oatley, J. M. (2021). Proper Timing of a Quiescence Period in Precursor Prospermatogonia Is Required for Stem Cell Pool Establishment in the Male Germline. Development (Cambridge, England) 148 (9), dev194571. doi:10.1242/dev.194571
Endo, T., Mikedis, M. M., Nicholls, P. K., Page, D. C., and de Rooij, D. G. (2019). Retinoic Acid and Germ Cell Development in the Ovary and Testis. Biomolecules 9 (12), 775. doi:10.3390/biom9120775
Endo, T., Romer, K. A., Anderson, E. L., Baltus, A. E., de Rooij, D. G., and Page, D. C. (2015). Periodic Retinoic Acid-STRA8 Signaling Intersects with Periodic Germ-Cell Competencies to Regulate Spermatogenesis. Proc. Natl. Acad. Sci. USA 112 (18), E2347–E2356. doi:10.1073/pnas.1505683112
Gewiss, R., Topping, T., and Griswold, M. D. (2020). Cycles, Waves, and Pulses: Retinoic Acid and the Organization of Spermatogenesis. Andrology 8 (4), 892–897. doi:10.1111/andr.12722
Griswold, M. D. (2018). 50 Years of Spermatogenesis: Sertoli Cells and Their Interactions with Germ Cells. Biol. Reprod. 99 (1), 87–100. doi:10.1093/biolre/ioy027
Griswold, M. D. (2016). Spermatogenesis: The Commitment to Meiosis. Physiol. Rev. 96 (1), 1–17. doi:10.1152/physrev.00013.2015
Hogarth, C. A., Evans, E., Onken, J., Kent, T., Mitchell, D., Petkovich, M., et al. (2015). CYP26 Enzymes Are Necessary within the Postnatal Seminiferous Epithelium for Normal Murine Spermatogenesis1. Biol. Reprod. 93 (1), 19. doi:10.1095/biolreprod.115.129718
Hu, Y.-C., Nicholls, P. K., Soh, Y. Q. S., Daniele, J. R., Junker, J. P., van Oudenaarden, A., et al. (2015). Licensing of Primordial Germ Cells for Gametogenesis Depends on Genital ridge Signaling. Plos Genet. 11 (3), e1005019. doi:10.1371/journal.pgen.1005019
Ishiguro, K.-i., Matsuura, K., Tani, N., Takeda, N., Usuki, S., Yamane, M., et al. (2020). MEIOSIN Directs the Switch from Mitosis to Meiosis in Mammalian Germ Cells. Dev. Cel. 52 (4), 429–445. doi:10.1016/j.devcel.2020.01.010
Jonas, K. C., Oduwole, O. O., Peltoketo, H., Rulli, S. B., and Huhtaniemi, I. T. (2014). Mouse Models of Altered Gonadotrophin Action: Insight into Male Reproductive Disorders. Reproduction (Cambridge, England) 148 (4), R63–R70. doi:10.1530/REP-14-0302
Koopman, P., Münsterberg, A., Capel, B., Vivian, N., and Lovell-Badge, R. (1990). Expression of a Candidate Sex-Determining Gene during Mouse Testis Differentiation. Nature 348 (6300), 450–452. doi:10.1038/348450a0
Koubova, J., Menke, D. B., Zhou, Q., Capel, B., Griswold, M. D., and Page, D. C. (2006). Retinoic Acid Regulates Sex-specific Timing of Meiotic Initiation in Mice. Proc. Natl. Acad. Sci. 103 (8), 2474–2479. doi:10.1073/pnas.0510813103
Kubota, H., and Brinster, R. L. (2018). Spermatogonial Stem Cells†. Biol. Reprod. 99 (1), 52–74. doi:10.1093/biolre/ioy077
Kumar, T. R. (2007). Mouse Models for Gonadotropins: a 15-year Saga. Mol. Cell. Endocrinol. 260-262, 249–254. doi:10.1016/j.mce.2006.09.002
Lin, Y., Gill, M. E., Koubova, J., and Page, D. C. (2008). Germ Cell-Intrinsic and -extrinsic Factors Govern Meiotic Initiation in Mouse Embryos. Science 322 (5908), 1685–1687. doi:10.1126/science.1166340
Lord, T., and Oatley, J. M. (2017). A Revised Asingle Model to Explain Stem Cell Dynamics in the Mouse Male Germline. Reproduction (Cambridge, England) 154 (2), R55–R64. doi:10.1530/REP-17-0034
MacLean, G., Li, H., Metzger, D., Chambon, P., and Petkovich, M. (2007). Apoptotic Extinction of Germ Cells in Testes of Cyp26b1 Knockout Mice. Endocrinology 148 (10), 4560–4567. doi:10.1210/en.2007-0492
Martineau, J., Nordqvist, K., Tilmann, C., Lovell-Badge, R., and Capel, B. (1997). Male-specific Cell Migration into the Developing Gonad. Curr. Biol. 7 (12), 958–968. doi:10.1016/s0960-9822(06)00415-5
Matson, C. K., Murphy, M. W., Griswold, M. D., Yoshida, S., Bardwell, V. J., and Zarkower, D. (2010). The Mammalian Doublesex Homolog DMRT1 Is a Transcriptional Gatekeeper that Controls the Mitosis versus Meiosis Decision in Male Germ Cells. Dev. Cel. 19 (4), 612–624. doi:10.1016/j.devcel.2010.09.010
McLean, D. J., Russell, L. D., and Griswold, M. D. (2002). Biological Activity and Enrichment of Spermatogonial Stem Cells in Vitamin A-Deficient and Hyperthermia-Exposed Testes from Mice Based on Colonization Following Germ Cell Transplantation1. Biol. Reprod. 66 (5), 1374–1379. doi:10.1095/biolreprod66.5.1374
Mitranond, V., Sobhon, P., Tosukhowong, P., and Chindaduangrat, W. (1979). Cytological Changes in the Testes of Vitamin-A-Deficient Rats. Acta anatomica 103 (2), 159–168. doi:10.1159/000145007
Morales, C., and Griswold, M. D. (1987). Retinol-induced Stage Synchronization in Seminiferous Tubules of the Rat. Endocrinology 121 (1), 432–434. doi:10.1210/endo-121-1-432
Oatley, J. M., and Griswold, M. D. (2020). MEIOSIN: A New Watchman of Meiotic Initiation in Mammalian Germ Cells. Dev. Cel. 52 (4), 397–398. doi:10.1016/j.devcel.2020.02.002
Raverdeau, M., Gely-Pernot, A., Feret, B., Dennefeld, C., Benoit, G., Davidson, I., et al. (2012). Retinoic Acid Induces Sertoli Cell Paracrine Signals for Spermatogonia Differentiation but Cell Autonomously Drives Spermatocyte Meiosis. Proc. Natl. Acad. Sci. 109 (41), 16582–16587. doi:10.1073/pnas.1214936109
Seisenberger, S., Andrews, S., Krueger, F., Arand, J., Walter, J., Santos, F., et al. (2012). The Dynamics of Genome-wide DNA Methylation Reprogramming in Mouse Primordial Germ Cells. Mol. Cel. 48 (6), 849–862. doi:10.1016/j.molcel.2012.11.001
Stévant, I., Neirijnck, Y., Borel, C., Escoffier, J., Smith, L. B., Antonarakis, S. E., et al. (2018). Deciphering Cell Lineage Specification during Male Sex Determination with Single-Cell RNA Sequencing. Cell Rep. 22 (6), 1589–1599. doi:10.1016/j.celrep.2018.01.043
Suzuki, A., and Saga, Y. (2008). Nanos2 Suppresses Meiosis and Promotes Male Germ Cell Differentiation. Genes Dev. 22 (4), 430–435. doi:10.1101/gad.1612708
Suzuki, S., Diaz, V. D., and Hermann, B. P. (2019). What Has Single-Cell RNA-Seq Taught Us about Mammalian Spermatogenesis? Biol. Reprod. 101 (3), 617–634. doi:10.1093/biolre/ioz088
Svingen, T., and Koopman, P. (2013). Building the Mammalian Testis: Origins, Differentiation, and Assembly of the Component Cell Populations. Genes Dev. 27 (22), 2409–2426. doi:10.1101/gad.228080.113
Tan, K., and Wilkinson, M. F. (2020). A Single-Cell View of Spermatogonial Stem Cells. Curr. Opin. Cel. Biol. 67, 71–78. doi:10.1016/j.ceb.2020.07.005
Tong, M.-H., Yang, Q.-E., Davis, J. C., and Griswold, M. D. (2013). Retinol Dehydrogenase 10 Is Indispensible for Spermatogenesis in Juvenile Males. Proc. Natl. Acad. Sci. 110 (2), 543–548. doi:10.1073/pnas.1214883110
van Pelt, A. M. M., and de Rooij, D. G. (1990). Synchronization of the Seminiferous Epithelium after Vitamin A Replacement in Vitamin A-Deficient Mice. Biol. Reprod. 43 (3), 363–367. doi:10.1095/biolreprod43.3.363
Wolbach, S. B., and Howe, P. R. (1925). Tissue Changes Following Deprivation of Fat-Soluble A Vitamin. J. Exp. Med. 42 (6), 753–777. doi:10.1084/jem.42.6.753
Keywords: Sertoli cell, germ cell, androgen receptor, retinoic acid, meiosis, spermatogenesis
Citation: Bhattacharya I, Sharma P, Purohit S, Kothiyal S, Das M and Banerjee A (2022) Recent Update on Retinoic Acid-Driven Initiation of Spermatogonial Differentiation. Front. Cell Dev. Biol. 10:833759. doi: 10.3389/fcell.2022.833759
Received: 12 December 2021; Accepted: 24 January 2022;
Published: 16 March 2022.
Edited by:
Souvik Dey, Manipal Academy of Higher Education (MAHE), IndiaReviewed by:
Suresh Yenugu, University of Hyderabad, IndiaCopyright © 2022 Bhattacharya, Sharma, Purohit, Kothiyal, Das and Banerjee. This is an open-access article distributed under the terms of the Creative Commons Attribution License (CC BY). The use, distribution or reproduction in other forums is permitted, provided the original author(s) and the copyright owner(s) are credited and that the original publication in this journal is cited, in accordance with accepted academic practice. No use, distribution or reproduction is permitted which does not comply with these terms.
*Correspondence: Indrashis Bhattacharya, aW5kcmFzaGlzLmJoYXR0YWNoYXJ5YUBnbWFpbC5jb20=; Arnab Banerjee, YXJuYWJiQGdvYS5iaXRzLXBpbGFuaS5hYy5pbg==