- 1Reproductive Medical Center, Renmin Hospital of Wuhan University & Hubei Clinic Research Center for Assisted Reproductive Technology and Embryonic Development, Wuhan, China
- 2Department of Clinical Laboratory, Renmin Hospital of Wuhan University, Wuhan, China
- 3Shenzhen Key Laboratory of Reproductive Immunology for Peri-implantation, Shenzhen Zhongshan Institute for Reproduction and Genetics, Shenzhen Zhongshan Urology Hospital, Shenzhen, China
Successful pregnancy requires the maternal immune system to tolerate the semi-allogeneic embryo. A good trophoblast function is also essential for successful embryo implantation and subsequent placental development. Chemokines are initially described in recruiting leukocytes. There are rich chemokines and chemokine receptor system at the maternal–fetal interface. Numerous studies have reported that they not only regulate trophoblast biological behaviors but also participate in the decidual immune response. At the same time, the chemokine system builds an important communication network between fetally derived trophoblast cells and maternally derived decidual cells. However, abnormal functions of chemokines or chemokine receptors are involved in a series of pregnancy complications. As growing evidence points to the roles of chemokines in pregnancy, there is a great need to summarize the available data on this topic. This review aimed to describe the recent research progress on the regulation and function of the main chemokines in pregnancy at the maternal–fetal interface. In addition, we also discussed the potential relationship between chemokines and pregnancy complications.
1 Introduction
Pregnancy is a complex and highly coordinated physiological process. Successful pregnancy involves the cooperation of multi-step crucial events at the maternal–fetal interface. First, at the early stages of pregnancy, the proper invasion, proliferation, and differentiation function of trophoblast cells are particularly important for achieving placental formation and embryonic development (Burrows et al., 1996; Anin et al., 2004; Staun-Ram and Shalev, 2005). In addition, the maternal immune system should also be modulated to tolerate the semi-allogeneic embryo (Finn, 1975; Trowsdale and Betz, 2006). However, the mechanisms responsible for maternal tolerance remain incompletely elucidated. Chemokines are a superfamily of small-molecule cytokines, widely expressed in trophoblast cells, decidual stromal cells (DSCs), and decidual immune cells (DICs) at the maternal–fetal interface. Emerging evidence has identified chemokines and chemokine receptors as essential contributors in pregnancy, participating in trophoblast invasion, decidualization, and immune cell recruitment (Hannan and Salamonsen, 2007; Ramhorst et al., 2016; Ashley et al., 2021). Moreover, the aforementioned functional abnormalities of chemokines have been reported in several pregnancy complications, including preeclampsia (PE), recurrent spontaneous abortion (RSA), and preterm birth (PTB) (Whitcomb et al., 2007; Kwak et al., 2014; Ali et al., 2021; Wang et al., 2021; Zheng et al., 2021). In this review, we summarized the crucial regulatory roles of chemokines in pregnancy in detail and highlighted their importance on specific cellular processes at the maternal–fetal interface. We also investigated the main chemokines and chemokine receptors related to pregnancy complications, hoping to provide a better understanding of these diseases.
2 Overview of Chemokines and Chemokine Receptors
Chemokines are a group of small secretory proteins of 8–10 kDa, well known for their chemotactic abilities (Zlotnik et al., 2006). Structurally, they are divided into the C chemokine ligand (XCL1-2), the CC chemokine ligand (CCL1-28), the CXC chemokine ligand (CXCL1-17), and the CX3C chemokine ligand (CX3CL). More than 50 chemokines have been identified since the late 1980s (Rollins, 1997; Zlotnik and Yoshie, 2000; Yoshie et al., 2001). They are widely expressed in humans (Sarkar et al., 2012), pigs (Hu et al., 2016), murine (Sarkar et al., 2012), and sheep (Ashley et al., 2011). Certain viruses also express molecules similar to chemokines (Penfold et al., 1999; Pontejo and Murphy, 2017; Pontejo et al., 2018). Correspondingly, there are about 20 chemokine receptors, including the CC chemokine receptor (CCR), the CXC chemokine receptor (CXCR), the C chemokine receptor (XCR), and the CX3CR chemokine receptor (CX3CR) (Zlotnik et al., 2006). In addition, there is a limited set of atypical chemokine receptors (ACKR1-4), which act as chemokine scavengers without eliciting chemotaxis (Stone et al., 2017). Together, chemokines and chemokine receptors constitute a rich chemokine system. It is generally accepted that there is a redundancy characteristic in the chemokine system. In other words, most chemokine receptors tend to bind to more than one ligand, and at the same time, a single ligand can also interact with different receptors. However, a recent study questions this general and oversimplified point of view (Ellwanger et al., 2020). Functionally, chemokines have been widely reported to be involved in inflammation, tumor, or metabolic diseases (Charo and Ransohoff, 2006; Nagarsheth et al., 2017; Chen et al., 2018).
3 Regulation and Function of Chemokines at the Maternal–Fetal Interface
In recent years, many studies have highlighted the importance of chemokines in pregnancy. Compared to non-pregnant endometrium, the decidual tissue shows increased chemokine levels (Engert et al., 2007; Segerer et al., 2009). During labor, some inflammatory chemokines are also upregulated in the uterus myometrium (Huang et al., 2021). At the maternal–fetal interface, the trophoblast cells, DSCs, and DICs establish rich chemokines and chemokine receptor network (Du et al., 2014). This chemokine network not only regulates specific recruitment and activation of appropriate leucocytes but also coordinates precisely orchestrated invasion of trophoblast through the decidua and maternal vasculature (Jones et al., 2004; Red-Horse et al., 2004; Hannan and Salamonsen, 2007; Fraccaroli et al., 2009). In this section, we will provide a detailed overview of the regulation and function of crucial chemokines at the maternal–fetal interface, as shown in Figure 1.
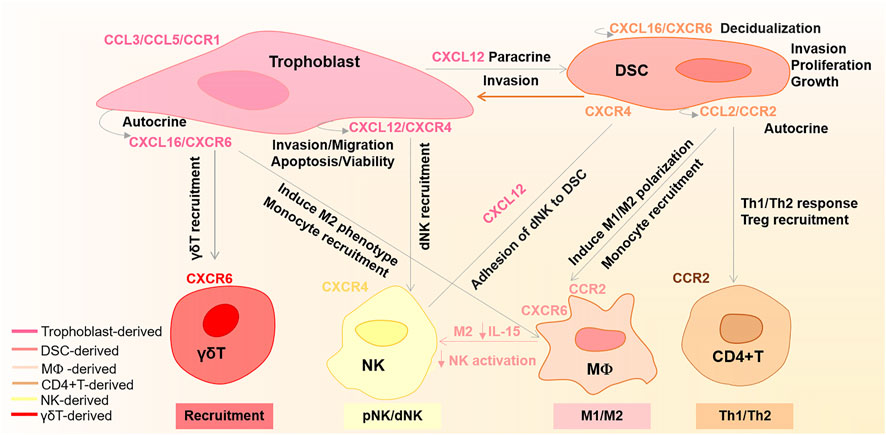
FIGURE 1. Regulation of main chemokines and chemokine receptors at the maternal–fetal interface. CXCL16 binding to CXCR6 promotes trophoblast invasion and proliferation, as well as endometrium decidualization in an autocrine and paracrine manner, respectively. CXCL16 is also involved in recruiting γδT cells and monocytes. Moreover, CXCL16/CXCR6 can induce M2 phenotype macrophages and decrease their IL-15 levels, which in turn induce the inactivation of NK cells. Trophoblast-derived CXCL12 with CXCR4 not only promotes trophoblast cell migration, invasion, and apoptosis via an autocrine manner but also enhances DSC invasion by upregulating the CXCR4 expression in a paracrine manner. Additionally, the trophoblast-derived CXCL12 also participates in NK cell recruitment and enhances the adhesive abilities of dNK cells to DSCs. CCL2/CCR2 enhances DSC invasion, proliferation, and growth in an autocrine manner. Moreover, CCL2/CCR2 also shows roles in M1/M2 phenotype polarization, monocyte recruitment, Treg recruitment, and Th1/Th2 immune response. CCL3/CCL5/CCR1 plays an essential function in trophoblast invasion.
3.1 CCL3/CCL5/CCR1
Trophoblasts, which form at the early stages of pregnancy, subsequently differentiate along the villous or extravillous trophoblast (EVT) pathway. In detail, at the tip of the anchoring villi, the trophoblasts proliferate and differentiate into EVTs while on the border layer of the floating villi, they differentiate into syncytiotrophoblasts. The former participates in endometrium decidualization and spiral artery remodeling, while the latter is responsible for nutrition transport, waste elimination, and placental endocrine functions. All of these aforementioned processes are related to the trophoblast biological function of invasion, migration, proliferation, and apoptosis. Chemokines and chemokine receptors have shown crucial regulatory roles in these aspects (Fujiwara et al., 2005a). It has been found that trophoblasts can acquire chemokine receptors CCR1 as they differentiate into invasive EVTs. Sato et al. (2003) collected the human placental tissue at 9–10 weeks of gestation for immunohistochemical detection. They found that the EVTs highly expressed CCR1 while the syncytiotrophoblasts and cytotrophoblasts hardly expressed CCR1 (Sato et al., 2003). They further demonstrated that CCR1 combined with its ligand CCL5 or CCL3 promoted the migration of the EVTs, which were isolated from the explant cultures in vitro (Sato et al., 2003). The primary EVTs can also trap CCL5 from maternal platelets, thereby enhancing their invasion abilities (Sato et al., 2005; Sato et al., 2010; Sato, 2020). Consistently, in the first trimester of pregnancy, CCL3 also showed a rapid increase during trophoblast differentiation toward EVTs (Drake et al., 2001). In contrast, the expression of CCR1 showed a significant decrease after EVTs migrated to the decidua. Interestingly, Fujiwara et al. (2005b) found that the EVTs also expressed dipeptidyl peptidase IV to metabolize CCL5, therefore inhibiting the excessive cell invasion. This regulation of trophoblast invasion and differentiation function by the chemokine-CCR1 system is considered a key molecular mechanism of maternal vascular remodeling during human early pregnancy (Sato et al., 2012).
3.2 CXCL12/CXCR4/CXCR7
CXCL12, also known as stromal cell-derived factor 1, is initially discovered in the bone marrow–derived stromal cells (Balabanian et al., 2005). According to reports, CXCL12 is widely expressed in cytotrophoblasts, syncytiotrophoblasts, and EVTs (Douglas et al., 2001; Red-Horse et al., 2001; Wu et al., 2004). Its receptors CXCR4 and CXCR7 are detected in DSCs (Zhou et al., 2008), trophoblast cells (Wu et al., 2004), and decidual natural killer (dNK) cells (Tao et al., 2015). Studies have shown that the CXCL12/CXCR4/CXCR7 axis is the critical signaling component of pregnancy through participating in multiple processes at the maternal–fetal interface.
3.2.1 Regulation and Function in Trophoblast Cells and Decidual Stromal Cells
The CXCL12/CXCR4/CXCR7 axis shows multiple roles in trophoblast cells during pregnancy. Wu et al. (2004) first isolated human placental trophoblast cells at 5–10 weeks of gestation, and they found that CXCL12/CXCR4 increased the trophoblast viability in an autocrine manner in vitro. Tripathi et al. (2014) reported that CXCL12/CXCR7 can promote JAR cell survival in vitro. Lu et al. (2016) observed that with decreased CXCR4 and CXCL12 levels, term human placental trophoblasts isolated from PE patients showed a significant apoptosis tendency, suggesting their roles in trophoblast apoptosis. Specific knockdown of CXCR4 in mouse trophectoderm cells of blastocysts significantly decreased the implantation rate of embryos (Bao et al., 2016). Further analysis indicated that CXCR4 is required upstream of trophectoderm cell apoptosis and migration (Bao et al., 2016). CXCL12/CXCR4 also shows regulation in trophoblast invasion. Both CXCR4 and CXCR7 showed increased expression during the cytotrophoblast differentiation toward the invasive phenotype (Schanz et al., 2011a). In vitro experiments showed that CXCR4 favored JEG-3 cell migration and invasion (Zhang et al., 2018). Correspondingly, downregulated CXCL12 showed direct suppression in HTR-8 cell invasion (Tamaru et al., 2015). In these aforementioned methods, CXCL12/CXCR4/CXCR7 widely regulates the trophoblast cell biological function.
The migratory and invasive capacities of human endometrial stromal cells (ESCs) are increasingly recognized as important features in the reproductive function (Weimar et al., 2013). Decidualized ESCs even perform enhanced motility and invasive capacity (Gellersen et al., 2010). By using an embryo coculture model, Grewal et al. (2008) reported this motility of decidualized ESCs. Interestingly, CXCL12 shows a functional role in DSC invasion. Ren et al. (2012) found that primary trophoblast-derived CXCL12 promoted the invasion of human first-trimester DSCs in a paracrine manner (Ren et al., 2012). Further investigation showed that this effect was mediated via CXCR4 but not CXCR7 (Zheng et al., 2018). CXCR7 may play the role of decoy in trophoblast invasion. At the same time, the invasiveness activity of trophoblast cells in coculture with DSCs also increased significantly and could be inhibited by an anti-CXCR4 neutralizing antibody (Zhou et al., 2008). These studies altogether suggest that CXCL12/CXCR4/CXCR7 not only participates in regulating trophoblast cells and the DSC biological function but also constructs a cross-talk between trophoblast cells and DSCs during pregnancy.
3.2.2 Regulation and Function in Decidual Immune Cells
3.2.2.1 Natural Killer Cells
Up to 70% of DICs are NK cells (King et al., 1989; Verma et al., 2000). In contrast to CD56dimCD16+ peripheral NK (pNK) cells, dNK cells are mainly CD56brightCD16− cells (Jabrane-Ferrat, 2019). DNK cells have poor cytotoxic activity and are believed to be critical in maintaining maternal–fetal tolerance and placental vascular remodeling. CXCR4 is essential for the composition of dNK cells. Hanna et al. (2003) found that CXCR4 was preferentially expressed on CD16− dNK subsets. Wu et al. (2005) also revealed that CD56brightCD16−dNK cells highly transcribed CXCR4. Moreover, CXCL12/CXCR4 shows crucial roles in regulating NK cell recruitment and differentiation during pregnancy. According to a previous report, the CXCL12/CXCR4 axis promoted the recruitment of CD25+ NK cells and the accumulation of CD3− CD56brightCD25+ dNK cells at the maternal–fetal interface (Tao et al., 2015). Subsequently, Piao et al. (2015) found that it is the first-trimester human trophoblast-derived CXCL12 that induced pNK cell recruitment and differentiation toward dNK cells. A recent study also reported that trophoblast-derived CXCL12 enhanced the adhesive abilities of CD56brightCD82−CD29+ NK cells to DSCs via the CXCL12/CD82/CD29 signaling pathway and thus contributed to CD56bright NK cell enrichment in decidua (Lu et al., 2020a). In particular, decidual CXCR4+CD56bright NK cells have been identified as a novel NK subset, which plays vital immune-modulatory roles in the Th1/Th2 response. It has long been established that dynamic deviations in Th1 and Th2 profiles are closely associated with pregnancy maintenance (Raghupathy, 2001). In the initial stages of pregnancy, there is a clear need for an active Th1 inflammatory response to achieve embryo implantation (Granot et al., 2012). Subsequently, a continuous prevalence of anti-inflammatory Th2 bias helps the mother to accommodate the semi-allogeneic embryo until a progressive shift toward Th1 predominance for labor (Challis et al., 2009). According to the report, CXCR4+CD56bright dNK cells can promote the Th2 shift in an IL-4-dependent manner (Tao et al., 2021). Diminished CXCR4+ dNK cells and their impaired ability to induce Th2 differentiation were already found in RSA patients and mouse models (Tao et al., 2021). Moreover, the adoptive transfer of CXCR4+ dNK cells to NK-deficient mice showed their great therapeutic potential in recovering the Th2/Th1 bias and reducing embryo resorption rates (Tao et al., 2021). Collectively, these studies suggest a crucial role of CXCL12/CXCR4 in NK cell recruitment and the Th1/Th2 response, providing the foundation for understanding the regulation of NK cells in maternal–fetal immune tolerance.
3.2.2.2 T Cells
A previous study investigated the role of CXCL12 in T-cell recruitment and differentiation. According to this report, the percentage of embryo loss was markedly decreased in the pregnant non-obese diabetic mice by exogenous regulatory T (Treg) cell transfer along with a CXCL12 injection (Lin et al., 2009). Subsequent in vitro cell migratory experiments showed that T-cell migration cannot be detected when no CXCL12 was added beforehand. In contrast, a considerable percentage of T cells were attracted after CXCL12 addition (Lin et al., 2009). These results indicate that CXCL12 may regulate the migration of T cells into the pregnant uterus and differentiation toward Treg, therefore establishing a beneficial environment for allogeneic pregnant nonobese diabetic mice. The CXCL12/CXCR4 axis is also involved in the Th1/Th2 balance at the maternal–fetal interface in early human pregnancy. By the bioplex assay, Piao et al. (2012) found that human recombinant CXCL12 alone increased Th2-type IL-4 and IL-10 production while decreasing the Th1-type TNF-α expression in primary DICs isolated from the first-trimester decidua. Further anti-CXCR4 antibody re-treatment eliminated the effect of CXCL12 on cytokine production in DICs, suggesting that the CXCL12/CXCR4 axis is involved in the development of the Th2 bias at the maternal–fetal interface (Piao et al., 2012).
3.2.2.3 Dendritic Cells
Dendritic cells (DCs) are a heterogeneous population and have a dual immune regulatory role. They not only initiate primary immune response but also induce immunological tolerance (Banchereau and Steinman, 1998). The immune-suppressive phenotype and function of DCs are critical for pregnancy (Blois et al., 2004; Bizargity and Bonney, 2009). However, research on human decidual DCs is quite sparse since the difficulty of small cell proportion and no single specific marker for DCs. Limited research reported the function of CXCL12/CXCR4 in DCs. Human monocyte-derived DCs can express CXCR4, responsible for chemotaxis to CXCL12. A subsequent study showed that CXCL12/CXCR4 can enhance DC maturation and survival to initiate acquired immune response in non-pregnant mice (Kabashima et al., 2007). Remarkably, impaired homing of CXCR4+ DCs during early gestation provoked a disorganized decidual vasculature with impaired spiral artery remodeling later (Barrientos et al., 2013). Conversely, the adoptive transfer of CXCR4+ DCs rescued early pregnancy (Barrientos et al., 2013).
3.3 CXCL16/CXCR6
3.3.1 Regulation and Function in Trophoblast Cells and Decidualization
Huang et al. (2006a) detected that CXCL16 and CXCR6 are widely expressed in syncytiotrophoblasts, EVTs, and cytotrophoblasts of placentas at 7–9 weeks of gestation by immunohistochemistry. Moreover, they found that CXCR6/CXCL16 stimulated the first-trimester human trophoblast proliferation and invasion in an autocrine manner (Huang et al., 2006a). Moreover, CXCL16 can also upregulate the expression of antiapoptotic markers in trophoblast cells, suggesting its potential for trophoblast apoptosis (Fan et al., 2019). It has reported the role of CXCL16/CXCR6 in decidualization. Compared to ESCs, the primary human DSCs secreted and expressed higher CXCL16 and CXCR6 (Mei et al., 2019). Meanwhile, the decidualized ESCs showed a significant decidual response after being treated with exogenous recombinant human CXCL16 or trophoblast-secreted CXLC16 in vitro. These results indicated that the CXCL16/CXCR6 axis contributed to the progression of ESC decidualization (Mei et al., 2019).
3.3.2 Regulation and Function in Decidual Immune Cells
3.3.2.1 Macrophages
Macrophages are heterogeneous and are generally divided into two categories: classically activated macrophages (M1) and alternatively activated macrophages (M2) (Mills et al., 2000). Decidual macrophages perform a mixed immune status of M1 and M2 phenotypes according to reports. Successful pregnancy depends on the spatial and temporal balance of M1 and M2 polarization (Brown et al., 2014). This regulation of macrophages is susceptible to changes in the maternal–fetal microenvironment. It has been found that CXCL16/CXCR6 can regulate macrophage polarization (Mantovani et al., 2004; Yao et al., 2019). Wang et al. revealed that first-trimester human trophoblast-derived CXCL16 induced the M2 phenotype of macrophages in vitro. Moreover, the polarized M2 macrophages can downregulate IL-15 levels, thereby facilitating the inactivation of NK cells and contributing to the immunotolerance at the maternal–fetal interface (Wang et al., 2019). In addition, CXCL16 also exhibits its action in monocytes. By using an enzyme-linked immunosorbent assay (ELISA), Huang et al. (2008) first detected CXCL16 secretion in the conditioned medium of primary cytotrophoblasts isolated from villi at 7–9 weeks of gestation (Huang et al., 2008). Next, by flow cytometry, they demonstrated that both exogenous and cytotrophoblast-conditioned medium-derived CXCL16 can direct the migration and recruitment of the monocyte subtype in peripheral blood mononuclear cell or decidua leukocytes (Huang et al., 2008). This chemotactic response of monocyte subtypes to CXCL16 was largely parallel to their receptor CXCR6 expression (Huang et al., 2008). These findings suggest that the fetus-derived trophoblasts can attract monocytes by CXCL16/CXCR6 in the first-trimester pregnancy, forming a specialized immune milieu at the maternofetal interface.
3.3.2.2 T Cells
CXCL16/CXCR6 can recruit and migrate T cells toward decidua, participating in the immune regulation of pregnancy. Using multiple-color flow cytometry, Huang et al. (2008) demonstrated that the CXCL16 sole receptor CXCR6 is preferentially expressed on decidual γδT cells (Huang et al., 2008). Furthermore, they confirmed that fetal trophoblast-produced CXCL16 directed the migration and recruitment of peripheral and decidual T lymphocytes into decidua at 7–9 weeks of gestation, thereby leading to a specialized immune milieu formation at the maternal–fetal interface (Huang et al., 2008). Fan et al. (2019) reported that by reducing the secretion of the cytotoxic factor granzyme B of decidual γδ T cells, the CXCL16/CXCR6 axis may contribute to maintaining normal pregnancy.
3.4 CCL2/CCR2
Previous research has showed that the primary trophoblasts did not express CCR2, while the primary isolated human DSCs highly transcribed CCR2 (Wu et al., 2004). He et al. (2007) found the co-expressions of CCR2 and CCL2 in human first-trimester DSCs and the decidual tissue. They detected high levels of CCL2 secretion in the supernatant of primary DSCs with an ELISA (He et al., 2007). Subsequently, Meng et al. (2013) reported that elevated CCL2/CCR2 promoted primary human DSC proliferation and growth. Hu et al. (2014) found that upregulated CCL2/CCR2 enhanced primary human DSC invasion. These two studies together emphasized the function of CCL2/CCR2 on DSC invasion, proliferation, and growth. In addition, CCL2/CCR2 also shows key functions in DICs. Wei et al. (2021) found that CCL2/CCR2 determined the polarization phenotype of decidual macrophages in a monocyte-DSC coculture system in a paracrine manner during early pregnancy. They detected changes both in decidual macrophages’ percentage and the M1 and M2 marker expressions after treatment with the CCR2 inhibitor by the flow cytometry assay in vivo (Wei et al., 2021). Another report exhibited that the first trimester decidual cell-derived CCL2 promoted monocyte migration and thus mediated excessive macrophage infiltration of the decidua (Huang et al., 2006b). CCL2 also shows an indirect role in the T-cell response. Huang et al. (2020)reported that human chorionic gonadotropin promoted the recruitment of regulatory T cells in the endometrium through increasing CCL2 levels in human ESCs. Yu et al. (2021) revealed that Toll-like receptors induced Th1/Th2 responses by affecting the CCL2 secretion of DSCs at the maternal–fetal interface.
4 Chemokines and Pregnancy Complications
The abnormal expression of chemokines and chemokine receptors can interrupt the trophoblast function, uterus angiogenesis, and maternal–fetal immune tolerance, thereby participating in pregnancy complications (Hannan and Salamonsen, 2007). In this part, we will focus on the relationship between chemokines and pregnancy-associated diseases, including PE, RSA, and PTB.
4.1 Preeclampsia
PE is defined as hypertension after 20 weeks of gestation and proteinuria with maternal multisystem dysfunction or fetal growth restriction (Chappell et al., 2021). PE is a major cause of maternal and perinatal mortality and morbidity, affecting approximately 5% of pregnancies (Bibbins-Domingo et al., 2017). However, the pathological mechanism of PE remains unclear. A prevailing view holds that PE is related to inadequate trophoblast invasion and placental malperfusion with releasing of soluble factors into the circulation, which causes maternal vascular endothelial injury and further leads to hypertension and multi-organ dysfunction (Chappell et al., 2021). CXC chemokines have unique abilities in angiogenesis and trophoblast function and are believed to play a potential role in the pathogenesis of PE. Decreased placental CXCL3 damaged the invasion and angiogenesis of trophoblast, thus leading to shallow implantation, which may be the main cause of severe PE (Gui et al., 2014; Wang et al., 2018). Reports are conflicting about the role of CXC12/CXCR4/CXCR7 in PE. Previous research has reported higher CXCL12 levels in the placenta of PE patients compared to the normal control group (Schanz et al., 2011b; Hwang et al., 2012). However, recent research has showed that CXCL12 and its receptors CXCR4 and CXCR7 levels were downregulated in the placenta of severe PE patients (Lu et al., 2016). Further studies have found that CXCL12 was able to decrease term trophoblast cells’ apoptosis rate (Lu et al., 2016; Lu et al., 2020b). Therefore, downregulation of CXC12/CXCR4/CXCR7 may disturb trophoblast apoptosis, participating in the occurrence of severe PE. Remarkably, CXCL12 levels were elevated in the mid-trimester amniotic fluid of pregnant women with PE, while the mechanism remains unknown (Tseng et al., 2009). In summary, these findings suggest that the CXCL12/CXCR7/CXCR4 axis may be a crucial molecular clue of PE that is worth to be further studied. Interestingly, the chemokines also show their effects on DICs in PE (Valencia ‐ Ortega et al., 2020). Excess CXCL10 and CXCL11 in decidua blunted pNK cell recruitment, contributing to the genesis of shallow placentation in PE (Lockwood et al., 2013). The increased CCL2 in first-trimester decidual cells showed association with the accumulation of decidual macrophages in the preeclamptic decidua (Lockwood et al., 2006).
4.2 Recurrent Spontaneous Abortion
RSA is defined as two or more times consecutive miscarriages before 20 weeks of gestation and impacts approximately 5% of childbearing-age women (Pereza et al., 2017; Practice Committee of the American Society for Reproductive Medicine, 2020). A recent transcriptomic analysis proposed chemokines as a common pathogenic mechanism in pregnancy loss (Wang et al., 2021). Abnormal secretion of CXCL5 was reported as an early indicator of miscarriage risk (Whitcomb et al., 2007). Recent research also showed that CXCL5 levels were downregulated in villous tissue of RSA patients than those of the controls (Zhang et al., 2021). Upregulation of CXCL5 can lead to poor trophoblast invasion and thus may be correlated with RSA (Zhang et al., 2021). CCR7 levels showed a decrease in the villous of RSA women (Luan et al., 2020). Knockdown of CCR7 caused an obvious reduction of migration and invasion in JAR and JEG-3 cells (Luan et al., 2020). These studies suggest that the chemokine system-induced trophoblast invasion dysfunction may be a potential pathological mechanism of RSA. CXCL12/CXCR4 also showed important roles in pregnancy loss. Das et al. found significantly reduced CXCR4 levels in chorionic villi of women with a number of previous miscarriages (Zangmo et al., 2021). This may induce insufficient trophoblast invasion, defective decidualization, or an imbalance of maternal–fetal immune tolerance and thus act on miscarriages (Ren et al., 2012; Piao et al., 2015; Ao et al., 2020). Women who exhibited recurrent implantation failure also performed lower levels of CXCR4 in the endometrium compared with fertile women (Tapia et al., 2008). Interestingly, CXCL12 from bone marrow-derived cells or the stem cells can improve the thin endometrium in a mouse model (Yi et al., 2019). Intrauterine CXCL12 administration in C57BL/6 mice also promoted embryo implantation rates and induced endometrial angiogenesis in vitro (Koo et al., 2021). These studies suggested that CXC12/CXCR4 may act on the endometria and angiogenesis, mediating its role in pregnancy loss (Wang et al., 2015). Some studies have also reported decreased CXCL16 protein levels in the villus of RSA patients compared with normal pregnant women (Fan et al., 2019; Mei et al., 2019). However, the fact whether the abnormal expression of CXCL16 at the maternal–fetal interface is the cause of miscarriage remains unclear. Kuroda et al. (2021) reported the relationship between the increasing number of pregnancy losses and the elevated ratio of Th1/Th2 in blood samples. Moreover, the ratio of Th1 and Th2-related chemokine receptors seems to have a crucial association with RSA. By flow cytometry, Kheshtchin et al. (2010) analyzed the expression of Th1-related (CCR5 and CXCR3) and Th2-related (CCR3 and CCR4) chemokine receptors on peripheral CD4+ or CD8+ T cells from RSA and control group women before 20 weeks of gestation. They reported a higher ratio of Th1/Th2 chemokine receptors in RSA women, indicating the Th1 dominant immune responses in the circulation of RSA women (Kheshtchin et al., 2010). Compared with fertile women, chemokine CCL5 performed decreased serum levels in patients with RSA while increased after immunization with paternal leukocytes. CCL5 can inhibit the mixed lymphocyte reaction in a dose-dependent manner in vitro (Ramhorst et al., 2004). These studies emphasized that the chemokines may exert immunological effects and thus take part in RSA. However, these aforementioned studies are only based on peripheral blood data, and further studies on the maternal–fetal interface are necessary.
4.3 Preterm Birth
PTB is defined as giving birth to babies before 37 weeks of gestation and is the leading cause of perinatal morbidity and mortality in developed countries (Goldenberg et al., 2008). Multiple factors attribute to the occurrence of PTB, such as inflammation, stress, and hormonal disorders (Romero et al., 2006; Goldenberg et al., 2008). Aberrant levels of chemokines have been reported in women with PTB. By ELISA, Laudanski et al. (2014) detected unusually high CCL16 levels in the blood serum samples of women with PTB. Subsequently, another study showed that lower CCL16 in umbilical cord blood was associated with spontaneous PTB, with 94.7% prediction sensitivity and 46.9% specificity (Kaukola et al., 2011). This indicates that CCL16 may be one of the potential pathological factors of PTB. Studies of chemokines in the amniotic fluid of women with PTB provide additional clues. For example, increased CXCL10 in the amniotic fluid showed the risk of spontaneous PTB after 32 weeks of gestation (Gervasi et al., 2012). Other studies also reported that CXCL12 (Tseng et al., 2009), CXCL8 (Hamilton et al., 2013), CCL5 (Hamilton et al., 2013), CCL20 (Hamill et al., 2008; Hua et al., 2012), CXCL5 (Hua et al., 2012), and CCL7 (Jacobsson et al., 2005) in the amniotic fluid were associated with microbial invasion and amniotic cavity inflammation. Studies from animals showed that broad-spectrum chemokine inhibitors can inhibit infection-mediated PTB (Shynlova et al., 2014; Coleman et al., 2020). These reports suggest that the chemokines may be involved in PTB through the inflammatory response. However, Esplin et al. (2005) found that CCL2 was increased in the amniotic fluid of PTB women with or without intra-amniotic infection. Interestingly, a prospective immunohistochemical analysis of 203 chorionic villus sampling specimens showed that the scores of syndecan-1, a regulator of chemokine function, are correlated with PTB (Schmedt et al., 2012). Recently, a transcriptomic analysis has also reported that the chemokine pathway may present a common pathogenic mechanism in spontaneous PTB (Wang et al., 2021). Taken together, these studies implied that chemokines play important roles in the pathological mechanism of PTB.
5 Conclusion
Successful pregnancy requires participation and cooperation of multiple crucial events, including good trophoblast function, decidualization, and balanced maternal–fetal immune tolerance. In this review, the available evidence shows that chemokines and chemokine receptors have wide regulatory effects in these events surrounding the trophoblast cells, DSCs, and DICs. Abnormalities of chemokines are related to trophoblast dysfunction, impaired angiogenesis, and disturbances in the maternal–fetal immune tolerance, which therefore may lead to pregnancy complications. Reviewing the regulation and function of chemokines in pregnancy may provide some potential targets for the clinical treatment of abnormal pregnancies in the future.
Author Contributions
TY, JY, and SL supported the research. SZ, JD, and YZ conceived the structure of the manuscript. SZ drafted the manuscript. JD revised the first version of the manuscript. SL provided critical feedback and helped revise the manuscript. All authors contributed to the article and approved the submitted version.
Funding
This work was supported by the National Key Research and Developmental Program of China (2018YFC1004601/2018YFC1003900/2018YFC1003904), the National Natural Science Foundation of China (Nos. 82101749, 81771662, and 82001547), and the Hubei Provincial Natural Science Foundation of China (2021CFB105).
Conflict of Interest
The authors declare that the research was conducted in the absence of any commercial or financial relationships that could be construed as a potential conflict of interest.
Publisher’s Note
All claims expressed in this article are solely those of the authors and do not necessarily represent those of their affiliated organizations, or those of the publisher, the editors, and the reviewers. Any product that may be evaluated in this article, or claim that may be made by its manufacturer, is not guaranteed or endorsed by the publisher.
References
Ali, S., Majid, S., Ali, M. N., Taing, S., Rehman, M. U., and Arafah, A. (2021). Cytokine Imbalance at Materno-Embryonic Interface as a Potential Immune Mechanism for Recurrent Pregnancy Loss. Int. Immunopharmacol. 90, 107118. doi:10.1016/j.intimp.2020.107118
Anin, S., Vince, G., and Quenby, S. (2004). Trophoblast Invasion. Hum. Fertil. 7 (3), 169–174. doi:10.1080/14647270400006911
Ao, D., Li, D. J., and Li, M. Q. (2020). CXCL12 in Normal and Pathological Pregnancies: A Review. Am. J. Reprod. Immunol. 84 (3), e13280. doi:10.1111/aji.13280
Ashley, R. L., Antoniazzi, A. Q., Anthony, R. V., and Hansen, T. R. (2011). The Chemokine Receptor CXCR4 and its Ligand CXCL12 Are Activated during Implantation and Placentation in Sheep. Reprod. Biol. Endocrinol. 9, 148. doi:10.1186/1477-7827-9-148
Ashley, R. L., Runyan, C. L., Maestas, M. M., Trigo, E., and Silver, G. (2021). Inhibition of the C-X-C Motif Chemokine 12 (CXCL12) and its Receptor CXCR4 Reduces Utero-Placental Expression of the VEGF System and Increases Utero-Placental Autophagy. Front. Vet. Sci. 8, 650687. doi:10.3389/fvets.2021.650687
Balabanian, K., Lagane, B., Infantino, S., Chow, K. Y. C., Harriague, J., Moepps, B., et al. (2005). The Chemokine SDF-1/CXCL12 Binds to and Signals through the Orphan Receptor RDC1 in T Lymphocytes. J. Biol. Chem. 280 (42), 35760–35766. doi:10.1074/jbc.M508234200
Banchereau, J., and Steinman, R. M. (1998). Dendritic Cells and the Control of Immunity. Nature 392 (6673), 245–252. doi:10.1038/32588
Bao, S., Li, T., Long, X., Zhang, J., Zhao, H., Ren, Y., et al. (2016). Chemokine Receptor Type 4 Regulates Migration and Invasion of Trophectoderm Cell in the Human Blastocyst. Biol. Reproduction 95 (1), 21. doi:10.1095/biolreprod.116.138826
Barrientos, G., Tirado-González, I., Freitag, N., Kobelt, P., Moschansky, P., Klapp, B. F., et al. (2013). CXCR4(+) Dendritic Cells Promote Angiogenesis during Embryo Implantation in Mice. Angiogenesis 16 (2), 417–427. doi:10.1007/s10456-012-9325-6
Bibbins-Domingo, K., Bibbins-Domingo, K., Grossman, D. C., Curry, S. J., Barry, M. J., Davidson, K. W., et al. (2017). Screening for Preeclampsia: US Preventive Services Task Force Recommendation Statement. Jama 317 (16), 1661–1667. doi:10.1001/jama.2017.3439
Bizargity, P., and Bonney, E. A. (2009). Dendritic Cells: a Family Portrait at Mid-gestation. Immunology 126 (4), 565–578. doi:10.1111/j.1365-2567.2008.02918.x
Blois, S. M., Alba Soto, C. D., Tometten, M., Klapp, B. F., Margni, R. A., and Arck, P. C. (2004). Lineage, Maturity, and Phenotype of Uterine Murine Dendritic Cells throughout Gestation Indicate a Protective Role in Maintaining Pregnancy1. Biol. Reprod. 70 (4), 1018–1023. doi:10.1095/biolreprod.103.022640
Brown, M. B., von Chamier, M., Allam, A. B., and Reyes, L. (2014). M1/M2 Macrophage Polarity in Normal and Complicated Pregnancy. Front. Immunol. 5, 606. doi:10.3389/fimmu.2014.00606
Burrows, T., King, A., and Loke, Y. W. (1996). Trophoblast Migration during Human Placental Implantation. Hum. Reprod. Update 2 (4), 307–321. doi:10.1093/humupd/2.4.307
Challis, J. R., Lockwood, C. J., Myatt, L., Norman, J. E., Strauss, J. F., and Petraglia, F. (2009). Inflammation and Pregnancy. Reprod. Sci. 16 (2), 206–215. doi:10.1177/1933719108329095
Chappell, L. C., Cluver, C. A., Kingdom, J., and Tong, S. (2021). Pre-eclampsia. Lancet 398 (10297), 341–354. doi:10.1016/s0140-6736(20)32335-7
Charo, I. F., and Ransohoff, R. M. (2006). The Many Roles of Chemokines and Chemokine Receptors in Inflammation. N. Engl. J. Med. 354 (6), 610–621. doi:10.1056/NEJMra052723
Chen, K., Bao, Z., Tang, P., Gong, W., Yoshimura, T., and Wang, J. M. (2018). Chemokines in Homeostasis and Diseases. Cell Mol. Immunol. 15 (4), 324–334. doi:10.1038/cmi.2017.134
Coleman, M., Orvis, A., Wu, T.-Y., Dacanay, M., Merillat, S., Ogle, J., et al. (2020). A Broad Spectrum Chemokine Inhibitor Prevents Preterm Labor but Not Microbial Invasion of the Amniotic Cavity or Neonatal Morbidity in a Non-human Primate Model. Front. Immunol. 11, 770. doi:10.3389/fimmu.2020.00770
Douglas, G. C., Thirkill, T. L., Sideris, V., Rabieh, M., Trollinger, D., and Nuccitelli, R. (2001). Chemokine Receptor Expression by Human Syncytiotrophoblast. J. Reproductive Immunol. 49 (2), 97–114. doi:10.1016/s0165-0378(00)00083-8
Drake, P. M., Gunn, M. D., Charo, I. F., Tsou, C.-L., Zhou, Y., Huang, L., et al. (2001). Human Placental Cytotrophoblasts Attract Monocytes and Cd56(bright) Natural Killer Cells via the Actions of Monocyte Inflammatory Protein 1α. J. Exp. Med. 193 (10), 1199–1212. doi:10.1084/jem.193.10.1199
Du, M.-R., Wang, S.-C., and Li, D.-J. (2014). The Integrative Roles of Chemokines at the Maternal-Fetal Interface in Early Pregnancy. Cell Mol. Immunol. 11 (5), 438–448. doi:10.1038/cmi.2014.68
Ellwanger, J. H., Kaminski, V. d. L., and Chies, J. A. (2020). What We Say and what We Mean when We Say Redundancy and Robustness of the Chemokine System - How CCR 5 Challenges These Concepts. Immunol. Cell Biol. 98 (1), 22–27. doi:10.1111/imcb.12291
Engert, S., Rieger, L., Kapp, M., Becker, J. C., Dietl, J., and Kämmerer, U. (2007). Profiling Chemokines, Cytokines and Growth Factors in Human Early Pregnancy Decidua by Protein Array. Am. J. Reprod. Immunol. 58 (2), 129–137. doi:10.1111/j.1600-0897.2007.00498.x
Esplin, M. S., Romero, R., Chaiworapongsa, T., Kim, Y. M., Edwin, S., Gomez, R., et al. (2005). Monocyte Chemotactic Protein-1 Is Increased in the Amniotic Fluid of Women Who Deliver Preterm in the Presence or Absence of Intra-amniotic Infection. J. Maternal-Fetal Neonatal Med. 17 (6), 365–373. doi:10.1080/14767050500141329
Fan, D.-X., Zhou, W.-J., Jin, L.-P., Li, M.-Q., Xu, X.-H., and Xu, C.-J. (2019). Trophoblast-Derived CXCL16 Decreased Granzyme B Production of Decidual γδ T Cells and Promoted Bcl-xL Expression of Trophoblasts. Reprod. Sci. 26 (4), 532–542. doi:10.1177/1933719118777638
Finn, R. (1975). Survival of the Genetically Incompatible Fetal Allograft. Lancet 305 (7911), 835–838. doi:10.1016/s0140-6736(75)93006-8
Fraccaroli, L., Alfieri, J., Larocca, L., Calafat, M., Mor, G., Leirós, C. P., et al. (2009). A Potential Tolerogenic Immune Mechanism in a Trophoblast Cell Line through the Activation of Chemokine-Induced T Cell Death and Regulatory T Cell Modulation. Hum. Reprod. 24 (1), 166–175. doi:10.1093/humrep/den344
Fujiwara, H., Higuchi, T., Sato, Y., Nishioka, Y., Zeng, B.-X., Yoshioka, S., et al. (2005). Regulation of Human Extravillous Trophoblast Function by Membrane-Bound Peptidases. Biochimica Biophysica Acta (BBA) - Proteins Proteomics 1751 (1), 26–32. doi:10.1016/j.bbapap.2005.04.007
Fujiwara, H., Sato, Y., Nishioka, Y., Yoshioka, S., Kosaka, K., Fujii, H., et al. (2005). New Regulatory Mechanisms for Human Extravillous Trophoblast Invasion. Reprod. Med. Biol. 4 (3), 189–195. doi:10.1111/j.1447-0578.2005.00104.x
Gellersen, B., Reimann, K., Samalecos, A., Aupers, S., and Bamberger, A.-M. (2010). Invasiveness of Human Endometrial Stromal Cells Is Promoted by Decidualization and by Trophoblast-Derived Signals. Hum. Reprod. 25 (4), 862–873. doi:10.1093/humrep/dep468
Gervasi, M.-T., Romero, R., Bracalente, G., Erez, O., Dong, Z., Hassan, S. S., et al. (2012). Midtrimester Amniotic Fluid Concentrations of Interleukin-6 and Interferon-Gamma-Inducible Protein-10: Evidence for Heterogeneity of Intra-amniotic Inflammation and Associations with Spontaneous Early (<32 Weeks) and Late (>32 Weeks) Preterm Delivery. J. Perinat. Med. 40 (4), 329–343. doi:10.1515/jpm-2012-0034
Goldenberg, R. L., Culhane, J. F., Iams, J. D., and Romero, R. (2008). Epidemiology and Causes of Preterm Birth. Lancet 371 (9606), 75–84. doi:10.1016/s0140-6736(08)60074-4
Granot, I., Gnainsky, Y., and Dekel, N. (2012). Endometrial Inflammation and Effect on Implantation Improvement and Pregnancy Outcome. Reproduction 144 (6), 661–668. doi:10.1530/rep-12-0217
Grewal, S., Carver, J. G., Ridley, A. J., and Mardon, H. J. (2008). Implantation of the Human Embryo Requires Rac1-dependent Endometrial Stromal Cell Migration. Proc. Natl. Acad. Sci. U.S.A. 105 (42), 16189–16194. doi:10.1073/pnas.0806219105
Gui, S., Ni, S., Jia, J., Gong, Y., Gao, L., Zhang, L., et al. (2014). Inconformity of CXCL3 Plasma Level and Placenta Expression in Preeclampsia and its Effect on Trophoblast Viability and Invasion. PLoS One 9 (12), e114408. doi:10.1371/journal.pone.0114408
Hamill, N., Romero, R., Gotsch, F., Kusanovic, J. P., Edwin, S., Erez, O., et al. (2008). Exodus-1 (CCL20): Evidence for the Participation of This Chemokine in Spontaneous Labor at Term, Preterm Labor, and Intrauterine Infection. J. Perinat. Med. 36 (3), 217–227. doi:10.1515/jpm.2008.034
Hamilton, S. A., Tower, C. L., and Jones, R. L. (2013). Identification of Chemokines Associated with the Recruitment of Decidual Leukocytes in Human Labour: Potential Novel Targets for Preterm Labour. PLoS One 8 (2), e56946. doi:10.1371/journal.pone.0056946
Hanna, J., Wald, O., Goldman-Wohl, D., Prus, D., Markel, G., Gazit, R., et al. (2003). CXCL12 Expression by Invasive Trophoblasts Induces the Specific Migration of CD16- Human Natural Killer Cells. Blood 102 (5), 1569–1577. doi:10.1182/blood-2003-02-0517
Hannan, N. J., and Salamonsen, L. A. (2007). Role of Chemokines in the Endometrium and in Embryo Implantation. Curr. Opin. Obstet. Gynecol. 19 (3), 266–272. doi:10.1097/GCO.0b013e328133885f
He, Y.-Y., Du, M.-R., Guo, P.-F., He, X.-J., Zhou, W.-h., Zhu, X.-Y., et al. (2007). Regulation of C-C Motif Chemokine Ligand 2 and its Receptor in Human Decidual Stromal Cells by Pregnancy-Associated Hormones in Early Gestation. Hum. Reprod. 22 (10), 2733–2742. doi:10.1093/humrep/dem208
Hu, J., Yang, D., Wang, H., Li, C., Zeng, Y., and Chen, W. (2016). CpG Oligodeoxynucleotides Induce Differential Cytokine and Chemokine Gene Expression Profiles in Dapulian and Landrace Pigs. Front. Microbiol. 7, 1992. doi:10.3389/fmicb.2016.01992
Hu, W.-T., Li, M.-Q., Liu, W., Jin, L.-P., Li, D.-J., and Zhu, X.-Y. (2014). IL-33 Enhances Proliferation and Invasiveness of Decidual Stromal Cells by Up-Regulation of CCL2/CCR2 via NF-Κb and ERK1/2 Signaling. Mol. Hum. Reprod. 20 (4), 358–372. doi:10.1093/molehr/gat094
Hua, R., Pease, J. E., Sooranna, S. R., Viney, J. M., Nelson, S. M., Myatt, L., et al. (2012). Stretch and Inflammatory Cytokines Drive Myometrial Chemokine Expression via NF-Κb Activation. Endocrinology 153 (1), 481–491. doi:10.1210/en.2011-1506
Huang, Q., Jin, X., Li, P., Zheng, Z., Jiang, Y., and Liu, H. (2021). Elevated Inflammatory Mediators from the Maternal-Fetal Interface to Fetal Circulation during Labor. Cytokine 148, 155707. doi:10.1016/j.cyto.2021.155707
Huang, S. J., Schatz, F., Masch, R., Rahman, M., Buchwalder, L., Niven-Fairchild, T., et al. (2006). Regulation of Chemokine Production in Response to Pro-inflammatory Cytokines in First Trimester Decidual Cells. J. Reproductive Immunol. 72 (1-2), 60–73. doi:10.1016/j.jri.2006.03.002
Huang, X., Cai, Y., Ding, M., Zheng, B., Sun, H., and Zhou, J. (2020). Human Chorionic Gonadotropin Promotes Recruitment of Regulatory T Cells in Endometrium by Inducing Chemokine CCL2. J. Reproductive Immunol. 137, 102856. doi:10.1016/j.jri.2019.102856
Huang, Y., Zhu, X.-Y., Du, M.-R., and Li, D.-J. (2008). Human Trophoblasts Recruited T Lymphocytes and Monocytes into Decidua by Secretion of Chemokine CXCL16 and Interaction with CXCR6 in the First-Trimester Pregnancy. J. Immunol. 180 (4), 2367–2375. doi:10.4049/jimmunol.180.4.2367
Huang, Y., Zhu, X.-Y., Du, M.-R., Wu, X., Wang, M.-Y., and Li, D.-J. (2006). Chemokine CXCL16, a Scavenger Receptor, Induces Proliferation and Invasion of First-Trimester Human Trophoblast Cells in an Autocrine Manner. Hum. Reprod. 21 (4), 1083–1091. doi:10.1093/humrep/dei436
Hwang, H. S., Kwon, H. S., Sohn, I. S., Park, Y. W., and Kim, Y. H. (2012). Increased CXCL12 Expression in the Placentae of Women with Pre-eclampsia. Eur. J. Obstetrics Gynecol. Reproductive Biol. 160 (2), 137–141. doi:10.1016/j.ejogrb.2011.10.007
Jabrane-Ferrat, N. (2019). Features of Human Decidual NK Cells in Healthy Pregnancy and during Viral Infection. Front. Immunol. 10, 1397. doi:10.3389/fimmu.2019.01397
Jacobsson, B., Holst, R.-M., Andersson, B., and Hagberg, H. (2005). Monocyte Chemotactic Protein-2 and -3 in Amniotic Fluid: Relationship to Microbial Invasion of the Amniotic Cavity, Intra-amniotic Inflammation and Preterm Delivery. Acta Obstet. Gynecol. Scand. 84 (6), 566–571. doi:10.1111/j.0001-6349.2005.00830.x
Jones, R. L., Hannan, N. J., Kaitu’u, T. u. J., Zhang, J., and Salamonsen, L. A. (2004). Identification of Chemokines Important for Leukocyte Recruitment to the Human Endometrium at the Times of Embryo Implantation and Menstruation. J. Clin. Endocrinol. Metabolism 89 (12), 6155–6167. doi:10.1210/jc.2004-0507
Kabashima, K., Sugita, K., Shiraishi, N., Tamamura, H., Fujii, N., and Tokura, Y. (2007). CXCR4 Engagement Promotes Dendritic Cell Survival and Maturation. Biochem. Biophysical Res. Commun. 361 (4), 1012–1016. doi:10.1016/j.bbrc.2007.07.128
Kaukola, T., Ojaniemi, M., Tuimala, J., Herva, R., Saarela, T., Kingsmore, S. F., et al. (2011). Cord Blood Chemokines Differentiate between Spontaneous and Elective Preterm Births in Singleton Pregnancies. Cytokine 54 (1), 85–91. doi:10.1016/j.cyto.2011.01.002
Kheshtchin, N., Gharagozloo, M., Andalib, A., Ghahiri, A., Maracy, M. R., and Rezaei, A. (2010). The Expression of Th1- and Th2-Related Chemokine Receptors in Women with Recurrent Miscarriage: the Impact of Lymphocyte Immunotherapy. Am. J. Reprod. Immunol. 64 (2), 104–112. doi:10.1111/j.1600-0897.2010.00829.x
King, A., Wellings, V., Gardner, L., and Loke, Y. W. (1989). Immunocytochemical Characterization of the Unusual Large Granular Lymphocytes in Human Endometrium throughout the Menstrual Cycle. Hum. Immunol. 24 (3), 195–205. doi:10.1016/0198-8859(89)90060-8
Koo, H. S., Yoon, M.-J., Hong, S.-H., Ahn, J., Cha, H., Lee, D., et al. (2021). CXCL12 Enhances Pregnancy Outcome via Improvement of Endometrial Receptivity in Mice. Sci. Rep. 11 (1), 7397. doi:10.1038/s41598-021-86956-y
Kuroda, K., Nakagawa, K., Horikawa, T., Moriyama, A., Ojiro, Y., Takamizawa, S., et al. (2021). Increasing Number of Implantation Failures and Pregnancy Losses Associated with Elevated Th1/Th2 Cell Ratio. Am. J. Reprod. Immunol. 86 (3), e13429. doi:10.1111/aji.13429
Kwak, J.-Y., Mamura, M., Barlic-Dicen, J., and Grage-Griebenow, E. (2014). Pathophysiological Roles of Cytokine-Chemokine Immune Network. J. Immunol. Res. 2014, 1–2. doi:10.1155/2014/615130
Laudanski, P., Lemancewicz, A., Kuc, P., Charkiewicz, K., Ramotowska, B., Kretowska, M., et al. (2014). Chemokines Profiling of Patients with Preterm Birth. Mediat. Inflamm. 2014, 1–7. doi:10.1155/2014/185758
Lin, Y., Xu, L., Jin, H., Zhong, Y., Di, J., and Lin, Q.-d. (2009). CXCL12 Enhances Exogenous CD4+CD25+ T Cell Migration and Prevents Embryo Loss in Non-obese Diabetic Mice. Fertil. Steril. 91 (6), 2687–2696. doi:10.1016/j.fertnstert.2008.01.109
Lockwood, C. J., Huang, S. J., Chen, C.-P., Huang, Y., Xu, J., Faramarzi, S., et al. (2013). Decidual Cell Regulation of Natural Killer Cell-Recruiting Chemokines. Am. J. Pathology 183 (3), 841–856. doi:10.1016/j.ajpath.2013.05.029
Lockwood, C. J., Matta, P., Krikun, G., Koopman, L. A., Masch, R., Toti, P., et al. (2006). Regulation of Monocyte Chemoattractant Protein-1 Expression by Tumor Necrosis Factor-α and Interleukin-1β in First Trimester Human Decidual Cells. Am. J. Pathology 168 (2), 445–452. doi:10.2353/ajpath.2006.050082
Lu, H., Jin, L. P., Huang, H. L., Ha, S. Y., Yang, H. L., Chang, R. Q., et al. (2020). Trophoblast‐derived CXCL12 Promotes CD56(bright) CD82(−) CD29(+) NK Cell Enrichment in the Decidua. Am. J. Reprod. Immunol. 83 (2). doi:10.1111/aji.13203
Lu, J., Wang, Z., Ren, L., Sun, Y., Cao, Y., Xiong, Y., et al. (2020). Expression and Functional Analysis of CXCL12 and its Receptors in Human Term Trophoblast Cells. Reprod. Sci. 27 (1), 46–54. doi:10.1007/s43032-019-00134-0
Lu, J., Zhou, W.-H., Ren, L., and Zhang, Y.-Z. (2016). CXCR4, CXCR7, and CXCL12 Are Associated with Trophoblastic Cells Apoptosis and Linked to Pathophysiology of Severe Preeclampsia. Exp. Mol. Pathology 100 (1), 184–191. doi:10.1016/j.yexmp.2015.12.013
Luan, X., Li, S., Zhao, J., Zhai, J., Liu, X., Chen, Z.-J., et al. (2020). Down-regulation of CCR7 via AKT Pathway and GATA2 Inactivation Suppressed Trophoblast Migration and Invasion in Recurrent Spontaneous Abortion†. Biol. Reprod. 102 (2), 424–433. doi:10.1093/biolre/ioz172
Mantovani, A., Sica, A., Sozzani, S., Allavena, P., Vecchi, A., and Locati, M. (2004). The Chemokine System in Diverse Forms of Macrophage Activation and Polarization. Trends Immunol. 25 (12), 677–686. doi:10.1016/j.it.2004.09.015
Mei, J., Yan, Y., Li, S.-Y., Zhou, W.-J., Zhang, Q., Li, M.-Q., et al. (2019). CXCL16/CXCR6 Interaction Promotes Endometrial Decidualization via the PI3K/AKT Pathway. Reproduction 157 (3), 273–282. doi:10.1530/rep-18-0417
Meng, Y.-H., Li, H., Chen, X., Liu, L.-B., Shao, J., Chang, K.-K., et al. (2013). RANKL Promotes the Growth of Decidual Stromal Cells in an Autocrine Manner via CCL2/CCR2 Interaction in Human Early Pregnancy. Placenta 34 (8), 663–671. doi:10.1016/j.placenta.2013.04.020
Mills, C. D., Kincaid, K., Alt, J. M., Heilman, M. J., and Hill, A. M. (2000). M-1/M-2 Macrophages and the Th1/Th2 Paradigm. J. Immunol. 164 (12), 6166–6173. doi:10.4049/jimmunol.164.12.6166
Nagarsheth, N., Wicha, M. S., and Zou, W. (2017). Chemokines in the Cancer Microenvironment and Their Relevance in Cancer Immunotherapy. Nat. Rev. Immunol. 17 (9), 559–572. doi:10.1038/nri.2017.49
Penfold, M. E. T., Dairaghi, D. J., Duke, G. M., Saederup, N., Mocarski, E. S., Kemble, G. W., et al. (1999). Cytomegalovirus Encodes a Potent α Chemokine. Proc. Natl. Acad. Sci. U.S.A. 96 (17), 9839–9844. doi:10.1073/pnas.96.17.9839
Pereza, N., Ostojić, S., Kapović, M., and Peterlin, B. (2017). Systematic Review and Meta-Analysis of Genetic Association Studies in Idiopathic Recurrent Spontaneous Abortion. Fertil. Steril. 107 (1), 150–159. e152. doi:10.1016/j.fertnstert.2016.10.007
Piao, H.-L., Tao, Y., Zhu, R., Wang, S.-C., Tang, C.-L., Fu, Q., et al. (2012). The CXCL12/CXCR4 axis Is Involved in the Maintenance of Th2 Bias at the Maternal/fetal Interface in Early Human Pregnancy. Cell Mol. Immunol. 9 (5), 423–430. doi:10.1038/cmi.2012.23
Piao, H. L., Wang, S. C., Tao, Y., Fu, Q., Du, M. R., and Li, D. J. (2015). CXCL12/CXCR4 Signal Involved in the Regulation of Trophoblasts on Peripheral NK Cells Leading to Th2 Bias at the Maternal-Fetal Interface. Eur. Rev. Med. Pharmacol. Sci. 19 (12), 2153–2161.
Pontejo, S. M., and Murphy, P. M. (2017). Chemokines Encoded by Herpesviruses. J. Leukoc. Biol. 102 (5), 1199–1217. doi:10.1189/jlb.4RU0417-145RR
Pontejo, S. M., Murphy, P. M., and Pease, J. E. (2018). Chemokine Subversion by Human Herpesviruses. J. Innate Immun. 10 (5-6), 465–478. doi:10.1159/000492161
Practice Committee of the American Society for Reproductive Medicine, (2020). Definitions of Infertility and Recurrent Pregnancy Loss: a Committee Opinion. Fertil. Steril. 113 (3), 533–535. doi:10.1016/j.fertnstert.2019.11.025
Raghupathy, R. (2001). Pregnancy: Success and Failure within the Th1/Th2/Th3 Paradigm. Seminars Immunol. 13 (4), 219–227. doi:10.1006/smim.2001.0316
Ramhorst, R. E., Garcı́a, V. E., Corigliano, A., Rabinovich, G. A., and Fainboim, L. (2004). Identification of RANTES as a Novel Immunomodulator of the Maternal Allogeneic Response. Clin. Immunol. 110 (1), 71–80. doi:10.1016/j.clim.2003.09.011
Ramhorst, R., Grasso, E., Paparini, D., Hauk, V., Gallino, L., Calo, G., et al. (2016). Decoding the Chemokine Network that Links Leukocytes with Decidual Cells and the Trophoblast during Early Implantation. Cell Adhesion Migr. 10 (1-2), 197–207. doi:10.1080/19336918.2015.1135285
Red-Horse, K., Drake, P. M., and Fisher, S. J. (2004). Human Pregnancy: the Role of Chemokine Networks at the Fetal-Maternal Interface. Expert Rev. Mol. Med. 6 (11), 1–14. doi:10.1017/s1462399404007720
Red-Horse, K., Drake, P. M., Gunn, M. D., and Fisher, S. J. (2001). Chemokine Ligand and Receptor Expression in the Pregnant Uterus: Reciprocal Patterns in Complementary Cell Subsets Suggest Functional Roles. Am. J. Pathology 159 (6), 2199–2213. doi:10.1016/s0002-9440(10)63071-4
Ren, L., Liu, Y.-Q., Zhou, W.-H., and Zhang, Y.-Z. (2012). Trophoblast-derived Chemokine CXCL12 Promotes CXCR4 Expression and Invasion of Human First-Trimester Decidual Stromal Cells. Hum. Reprod. 27 (2), 366–374. doi:10.1093/humrep/der395
Romero, R., Espinoza, J., Kusanovic, J., Gotsch, F., Hassan, S., Erez, O., et al. (2006). The Preterm Parturition Syndrome. Bjog 113 (Suppl. 3), 17–42. doi:10.1111/j.1471-0528.2006.01120.x
Sarkar, S. A., Lee, C. E., Victorino, F., Nguyen, T. T., Walters, J. A., Burrack, A., et al. (2012). Expression and Regulation of Chemokines in Murine and Human Type 1 Diabetes. Diabetes 61 (2), 436–446. doi:10.2337/db11-0853
Sato, Y. (2020). Endovascular Trophoblast and Spiral Artery Remodeling. Mol. Cell. Endocrinol. 503, 110699. doi:10.1016/j.mce.2019.110699
Sato, Y., Fujiwara, H., and Konishi, I. (2012). Mechanism of Maternal Vascular Remodeling during Human Pregnancy. Reprod. Med. Biol. 11 (1), 27–36. doi:10.1007/s12522-011-0102-9
Sato, Y., Fujiwara, H., and Konishi, I. (2010). Role of Platelets in Placentation. Med. Mol. Morphol. 43 (3), 129–133. doi:10.1007/s00795-010-0508-1
Sato, Y., Fujiwara, H., Zeng, B.-X., Higuchi, T., Yoshioka, S., and Fujii, S. (2005). Platelet-derived Soluble Factors Induce Human Extravillous Trophoblast Migration and Differentiation: Platelets Are a Possible Regulator of Trophoblast Infiltration into Maternal Spiral Arteries. Blood 106 (2), 428–435. doi:10.1182/blood-2005-02-0491
Sato, Y., Higuchi, T., Yoshioka, S., Tatsumi, K., Fujiwara, H., and Fujii, S. (2003). Trophoblasts Acquire a Chemokine Receptor, CCR1, as They Differentiate towards Invasive Phenotype. Development 130 (22), 5519–5532. doi:10.1242/dev.00729
Schanz, A., Baston-Bust, D., Krussel, J. S., Heiss, C., Janni, W., and Hess, A. P. (2011). CXCR7 and Syndecan-4 Are Potential Receptors for CXCL12 in Human Cytotrophoblasts. J. Reproductive Immunol. 89 (1), 18–25. doi:10.1016/j.jri.2011.01.016
Schanz, A., Winn, V. D., Fisher, S. J., Blumenstein, M., Heiss, C., Hess, A. P., et al. (2011). Pre-eclampsia Is Associated with Elevated CXCL12 Levels in Placental Syncytiotrophoblasts and Maternal Blood. Eur. J. Obstetrics Gynecol. Reproductive Biol. 157 (1), 32–37. doi:10.1016/j.ejogrb.2011.02.023
Schmedt, A., Götte, M., Heinig, J., Kiesel, L., Klockenbusch, W., and Steinhard, J. (2012). Evaluation of Placental Syndecan-1 Expression in Early Pregnancy as a Predictive Fetal Factor for Pregnancy Outcome. Prenat. Diagn 32 (2), 131–137. doi:10.1002/pd.2908
Segerer, S., Kämmerer, U., Kapp, M., Dietl, J., and Rieger, L. (2009). Upregulation of Chemokine and Cytokine Production during Pregnancy. Gynecol. Obstet. Invest. 67 (3), 145–150. doi:10.1159/000174291
Shynlova, O., Dorogin, A., Li, Y., and Lye, S. (2014). Inhibition of Infection‐mediated Preterm Birth by Administration of Broad Spectrum Chemokine Inhibitor in Mice. J. Cell. Mol. Med. 18 (9), 1816–1829. doi:10.1111/jcmm.12307
Staun-Ram, E., and Shalev, E. (2005). Human Trophoblast Function during the Implantation Process. Reprod. Biol. Endocrinol. 3, 56. doi:10.1186/1477-7827-3-56
Stone, M., Hayward, J., Huang, C., and E. Huma, J. (2017). Mechanisms of Regulation of the Chemokine-Receptor Network. Ijms 18 (2), 342. doi:10.3390/ijms18020342
Tamaru, S., Mizuno, Y., Tochigi, H., Kajihara, T., Okazaki, Y., Okagaki, R., et al. (2015). MicroRNA-135b Suppresses Extravillous Trophoblast-Derived HTR-8/SVneo Cell Invasion by Directly Down Regulating CXCL12 under Low Oxygen Conditions. Biochem. Biophysical Res. Commun. 461 (2), 421–426. doi:10.1016/j.bbrc.2015.04.055
Tao, Y., Li, Y.-H., Piao, H.-L., Zhou, W.-J., Zhang, D., Fu, Q., et al. (2015). CD56(bright)CD25+ NK Cells Are Preferentially Recruited to the Maternal/fetal Interface in Early Human Pregnancy. Cell Mol. Immunol. 12 (1), 77–86. doi:10.1038/cmi.2014.26
Tao, Y., Li, Y. H., Zhang, D., Xu, L., Chen, J. J., Sang, Y. F., et al. (2021). Decidual CXCR4(+) CD56(bright) NK Cells as a Novel NK Subset in Maternal-Foetal Immune Tolerance to Alleviate Early Pregnancy Failure. Clin. Transl. Med. 11 (10), e540. doi:10.1002/ctm2.540
Tapia, A., Gangi, L. M., Zegers-Hochschild, F., Balmaceda, J., Pommer, R., Trejo, L., et al. (2008). Differences in the Endometrial Transcript Profile during the Receptive Period between Women Who Were Refractory to Implantation and Those Who Achieved Pregnancy. Hum. Reprod. 23 (2), 340–351. doi:10.1093/humrep/dem319
Tripathi, V., Kumar, R., Dinda, A. K., Kaur, J., and Luthra, K. (2014). CXCL12-CXCR7 Signaling Activates ERK and Akt Pathways in Human Choriocarcinoma Cells. Cell Commun. Adhesion 21 (4), 221–228. doi:10.3109/15419061.2013.876013
Trowsdale, J., and Betz, A. G. (2006). Mother's Little Helpers: Mechanisms of Maternal-Fetal Tolerance. Nat. Immunol. 7 (3), 241–246. doi:10.1038/ni1317
Tseng, J.-J., Chen, Y.-F., Hsieh, Y.-T., and Chou, M.-M. (2009). Elevated Amniotic Fluid Stromal Cell-Derived Factor-1α (SDF-1α) Concentration in Mid-gestation as a Predictor of Adverse Birth Outcomes. J. Chin. Med. Assoc. 72 (12), 638–642. doi:10.1016/s1726-4901(09)70446-0
Valencia‐Ortega, J., Saucedo, R., Peña‐Cano, M. I., Hernández‐Valencia, M., and Cruz‐Durán, J. G. (2020). Immune Tolerance at the Maternal‐placental Interface in Healthy Pregnancy and Pre‐eclampsia. J. Obstet. Gynaecol. Res. 46 (7), 1067–1076. doi:10.1111/jog.14309
Verma, S., Hiby, S. E., Loke, Y. W., and King, A. (2000). Human Decidual Natural Killer Cells Express the Receptor for and Respond to the Cytokine Interleukin 151. Biol. Reprod. 62 (4), 959–968. doi:10.1095/biolreprod62.4.959
Wang, H., Wang, T., Dai, L., Cao, W., Ye, L., Gao, L., et al. (2018). Effects of CXCL3 on Migration, Invasion, Proliferation and Tube Formation of Trophoblast Cells. Placenta 66, 47–56. doi:10.1016/j.placenta.2018.05.004
Wang, L., Li, X., Zhao, Y., Fang, C., Lian, Y., Gou, W., et al. (2015). Insights into the Mechanism of CXCL12-Mediated Signaling in Trophoblast Functions and Placental Angiogenesis. Acta Biochim. Biophys. Sin. 47 (9), 663–672. doi:10.1093/abbs/gmv064
Wang, P., Pan, J., Tian, X., Dong, X., Ju, W., Wang, Y., et al. (2021). Transcriptomics‐determined Chemokine‐cytokine Pathway Presents a Common Pathogenic Mechanism in Pregnancy Loss and Spontaneous Preterm Birth. Am. J. Reprod. Immunol. 86 (1), e13398. doi:10.1111/aji.13398
Wang, X.-Q., Zhou, W.-J., Hou, X.-X., Fu, Q., and Li, D.-J. (2019). Correction: Trophoblast-Derived CXCL16 Induces M2 Macrophage Polarization that in Turn Inactivates NK Cells at the Maternal-Fetal Interface. Cell Mol. Immunol. 16 (3), 313. doi:10.1038/s41423-018-0194-9
Wei, C. Y., Li, M. Q., Zhu, X. Y., and Li, D. J. (2021). Immune Status of Decidual Macrophages Is Dependent on the CCL2/CCR2/JAK2 Pathway during Early Pregnancy. Am. J Rep Immunol 86 (5), e13480. doi:10.1111/aji.13480
Weimar, C. H. E., Macklon, N. S., Post Uiterweer, E. D., Brosens, J. J., and Gellersen, B. (2013). The Motile and Invasive Capacity of Human Endometrial Stromal Cells: Implications for Normal and Impaired Reproductive Function. Hum. Reprod. Update 19 (5), 542–557. doi:10.1093/humupd/dmt025
Whitcomb, B. W., Schisterman, E. F., Klebanoff, M. A., Baumgarten, M., Rhoton-Vlasak, A., Luo, X., et al. (2007). Circulating Chemokine Levels and Miscarriage. Am. J. Epidemiol. 166 (3), 323–331. doi:10.1093/aje/kwm084
Wu, X., Jin, L.-P., Yuan, M.-M., Zhu, Y., Wang, M.-Y., and Li, D.-J. (2005). Human First-Trimester Trophoblast Cells Recruit CD56brightCD16−NK Cells into Decidua by Way of Expressing and Secreting of CXCL12/Stromal Cell-Derived Factor 1. J. Immunol. 175 (1), 61–68. doi:10.4049/jimmunol.175.1.61
Wu, X., Li, D.-J., Yuan, M.-M., Zhu, Y., and Wang, M.-Y. (2004). The Expression of CXCR4/CXCL12 in First-Trimester Human Trophoblast Cells1. Biol. Reprod. 70 (6), 1877–1885. doi:10.1095/biolreprod.103.024729
Yao, Y., Xu, X.-H., and Jin, L. (2019). Macrophage Polarization in Physiological and Pathological Pregnancy. Front. Immunol. 10, 792. doi:10.3389/fimmu.2019.00792
Yi, K. W., Mamillapalli, R., Sahin, C., Song, J., Tal, R., and Taylor, H. S. (2019). Bone Marrow-Derived Cells or C-X-C Motif Chemokine 12 (CXCL12) Treatment Improve Thin Endometrium in a Mouse Model. Biol. Reprod. 100 (1), 61–70. doi:10.1093/biolre/ioy175
Yoshie, O., Imai, T., and Nomiyama, H. (2001). Chemokines in Immunity. Adv. Immunol. 78, 57–110. doi:10.1016/s0065-2776(01)78002-9
Yu, N., Weng, Y., Liu, W., Chen, L., Iqbal, F., Yin, Z., et al. (2021). TLRs Induce Th1/Th2 Responses by Affecting the Secretion of CCL2 at the Maternal-Foetal Interface. Int. Immunopharmacol. 100, 108070. doi:10.1016/j.intimp.2021.108070
Zangmo, R., Das, A., Agrawal, N., Roy, K., Singh, K., and Bala, R. (2021). Comparison of Expression of Chemokine Receptor 4 in Maternal Decidua and Chorionic Villi in Women with Spontaneous Miscarriages and Women Opting for Termination of Viable Pregnancies. J. Hum. Reprod. Sci. 14 (1), 68–72. doi:10.4103/jhrs.JHRS_64_20
Zhang, S., Ding, J., Wang, J., Yin, T., Zhang, Y., and Yang, J. (2021). CXCL5 Downregulation in Villous Tissue Is Correlated with Recurrent Spontaneous Abortion. Front. Immunol. 12, 717483. doi:10.3389/fimmu.2021.717483
Zhang, Z., Li, P., Wang, Y., and Yan, H. (2018). Hypoxia-induced E-xpression of CXCR4 F-avors T-rophoblast C-ell M-igration and I-nvasion via the A-ctivation of HIF-1α. Int. J. Mol. Med. 42 (3), 1508–1516. doi:10.3892/ijmm.2018.3701
Zheng, J., Wang, H., and Zhou, W. (2018). Modulatory Effects of Trophoblast-Secreted CXCL12 on the Migration and Invasion of Human First-Trimester Decidual Epithelial Cells Are Mediated by CXCR4 rather Than CXCR7. Reprod. Biol. Endocrinol. 16 (1), 17. doi:10.1186/s12958-018-0333-2
Zheng, Z., Chen, H., Zhu, S., and Hu, Y. (2021). CXCR4/CXCR7 Protein Expression Levels in Placentas of Patients with Preeclampsia. Med. Sci. Monit. 27, e931192. doi:10.12659/msm.931192
Zhou, W.-H., Du, M.-R., Dong, L., Yu, J., and Li, D.-J. (2008). Chemokine CXCL12 Promotes the Cross-Talk between Trophoblasts and Decidual Stromal Cells in Human First-Trimester Pregnancy. Hum. Reprod. 23 (12), 2669–2679. doi:10.1093/humrep/den308
Zlotnik, A., and Yoshie, O. (2000). Chemokines: a New Classification System and Their Role in Immunity. Immunity 12 (2), 121–127. doi:10.1016/s1074-7613(00)80165-x
Keywords: chemokine, chemokine receptor, trophoblast, decidual cells, maternal–fetal interface, pregnancy
Citation: Zhang S, Ding J, Zhang Y, Liu S, Yang J and Yin T (2022) Regulation and Function of Chemokines at the Maternal–Fetal Interface. Front. Cell Dev. Biol. 10:826053. doi: 10.3389/fcell.2022.826053
Received: 30 November 2021; Accepted: 22 June 2022;
Published: 22 July 2022.
Edited by:
Zhao-Jia Ge, Qingdao Agricultural University, ChinaReviewed by:
Jose Artur Chies, Federal University of Rio Grande do Sul, BrazilScott Michael Gordon, Children’s Hospital of Philadelphia, United States
Padma Murthi, Monash University, Australia
Copyright © 2022 Zhang, Ding, Zhang, Liu, Yang and Yin. This is an open-access article distributed under the terms of the Creative Commons Attribution License (CC BY). The use, distribution or reproduction in other forums is permitted, provided the original author(s) and the copyright owner(s) are credited and that the original publication in this journal is cited, in accordance with accepted academic practice. No use, distribution or reproduction is permitted which does not comply with these terms.
*Correspondence: Su Liu, c3VubnlzdWUwMzA5QDE2My5jb20=; Jing Yang, ZHJ5YW5namluZ0B3aHUuZWR1LmNu; Tailang Yin, cmVwcm9kdWN0aXZlQHdodS5lZHUuY24=
†These authors have contributed equally to this work and share first authorship