- Food and Nutritional Sciences, Chubu University, Kasugai, Japan
Schistosomiasis is a life-threatening parasitic disease caused by blood flukes, Schistosomes. In its intestinal type, the parasites reside in visceral/portal veins of the human hosts and lay eggs to excrete in feces via intestinal tracts, and some of the aberrant eggs plug into the liver via the portal blood flow. Ectopic growth of these eggs causes fatal granulomatosis and cirrhosis of the liver. The parasites ingest nutrients from the host blood plasma by using nonspecific and specific transport via their body surface and alimentary tracts. It is especially important for the female adults to obtain lipid molecules because they synthesize neither fatty acids nor sterols and yet produce egg yolk. Low-density lipoprotein receptors have been identified in the body of the Schistosomes but their functions in the parasite life cycle have not clearly been characterized. On the other hand, CD36-related protein was identified in the body and the eggs of Asian blood fluke, Schistosoma japonicum, and characterized as a molecule that mediates selective uptake of cholesteryl ester from the host plasma high-density lipoproteins (HDLs). This reaction was shown crucial for their eggs to grow to miracidia. Interestingly, abnormal large HDL generated in lack of cholesteryl ester transfer protein (CETP) is a poor substrate for this reaction, and, therefore, CETP deficiency resists pathogenic ectopic growth of the aberrant parasite eggs in the liver. This genetic mutation is exclusively found in East Asia, overlapping with the current and historic regions of Schistosoma japonicum epidemic, so that this infection could be related to high prevalence of CETP deficiency in East Asia.
Introduction: Schistosomiasis
Infection of blood flukes, schistosomiasis, is a life-threatening parasitosis widely spread in the world, caused by species such as Schistosoma mansoni (S. mansoni) in Africa and South America, Schistosoma japonicum (S. japonicum) or its subtypes in East Asia (Gryseels et al., 2006; McManus et al., 2018), and Schistosoma haematobium (S. haematobium) in Africa and Middle East. The former two cause intestinal schistosomiasis where the adult parasites reside in portal/visceral veins of the host animals and lay eggs which penetrate into intestinal lumen to be excreted in feces. The latter causes urogenital schistosomiasis where the worms infect in pelvic vein and the eggs penetrate into urinary tract and genital organs to be excreted (McManus et al., 2018). The eggs grow to miracidia by using their yolk and hatch in environmental fresh water. They reach the intermediate hosts, specific fresh water snails such as Oncomelenia nosophora, where they grow to sporocysts and then to cercariae (McManus et al., 2018). The cercariae go into the water again and infect the final mammalian host by penetrating their skins and migrating into their blood stream to reach the final parasitic position. Thus, endemic of schistosomes seems associated with extensive exposure to natural fresh water reserve of the hosts in everyday life. S. japonicum has been endemic widespread in East Asia such as Indonesia, Philippines, China, and Japan as far as traced in recorded history. Its Mekong strain or S. mekongi is also found in the Mekong basin in Indochina peninsula. S. japonicum was first identified in Japan early 20th century when some intensively infected regions were still apparent (Iida et al., 1999). It used to be one of the major infectious diseases among rice farmers in certain areas of Japan but has been eradicated by eliminating the intermediate hosts of fresh water snails by transforming irrigation channels for rice fields into concrete (Iida et al., 1999; Ohmae et al., 2003). The similar environmental strategy has been adopted in China, where the number of the infected patients has sharply come down in the past decades from 11 million to several hundred thousand (Wang et al., 2009; Qian et al., 2018), but it still remains as one of the major public health problems there (Wang et al., 2018), especially in such occasions as flooding (McManus et al., 2010; Yang et al., 2018; Xue et al., 2021). S. japonicum is still active in Philippines (Bergquist and Tanner, 2010; Gordon et al., 2012), Cambodia, Laos, Thailand, Malaysia, and Indonesia (Harinasuta, 1984) accounting for 2 million patients, and it remains as the second major intestinal schistosomiasis next to the African blood fluke, S. mansoni.
One of the major fatal complications of intestinal schistosomiasis is the cirrhosis of the liver in the intestinal type. Although the eggs laid are to be excreted and get into the normal life cycle, substantial portion of them aberrantly reaches and plugs into the liver by the upward flow of the portal blood and ectopically grow to miracidia there (Cheever et al., 1994). This process causes granulomatosis lesions and eventually fatal cirrhosis in the liver. In the urogenital type, the eggs cause granulomatosis in urinary tract or genital organs. Therefore, the egg embryonation is a potential target for prevention of fatal development of the schistosomiasis although the mechanism to induce this lesion is still unknown. Specific antibodies against the various egg antigens have been identified as markers of the infection, but their relationship to granulomatogenesis is unclear (Mohda et al., 1998). A potential pathogenesis stage is egg embryonation to miracidium, because the eggs only after this stage cause the hepatic lesion when transplanted (Hirata et al., 1991). Host L-selectin binds to the eggs only at the stage of miracidium (El Ridi et al., 1996). Accordingly, vaccination to stabilize the embryonation has been proposed as an anti-schistosomiasis therapy (Mitchell et al., 1994).
Schistosomes ingest nutrients from the host blood by nonspecific and specific absorption via the body surface tegument and alimentary tract (Skelly et al., 2014). It is especially important for them to gain lipid nutrients because the parasites de novo synthesize neither fatty acid nor sterol molecules (Smith et al., 1970; Brouwers et al., 1997), and more so in female as they need to produce the yolk for the eggs they lay. As these parasites reside in blood stream, it is rational to assume that the major sources of the lipid nutrients are the host plasma lipoproteins. Mammalian plasma lipoproteins are largely classified to those containing apolipoprotein B (apoB), such as low-density lipoproteins (LDLs), very low-density lipoproteins (VLDLs), and high-density lipoproteins (HDLs). ApoB lipoproteins mainly function to deliver fatty acids and cholesterol from the liver to the tissues and HDL recovers cholesterol from the tissues to transport back to the liver for catabolic conversion to bile acids. The former are taken up by the endocytotic LDL receptor pathway, whereas cholesteryl ester (CE) molecules of HDL are selectively taken up by the CD36-like molecules such as scavenger receptor B1 (SR-B1) in rodents (Acton et al., 1996) or LIMPIIanalogous-1 (CLA-1) in humans (Imachi et al., 1999a; Imachi et al., 1999b). The LDL receptor–like molecules have been identified both in S. mansoni (Rumjanek et al., 1983; Rumjanek et al., 1985; Rumjanek et al., 1988; Bennett and Caulfield, 1991; Xu and Caulfield, 1992; Furlong et al., 1995; Tempone et al., 1997) and S. japonicum (Rogers et al., 1989; Rogers et al., 1990; Fan et al., 2003), and CD36-like molecules have also been identified in S. mansoni (Dinguirard and Yoshino, 2006) and S. japonicum (Okumura-Noji et al., 2013; Zhang et al., 2013). The latter was shown critical for the eggs to grow miracidia (Okumura-Noji et al., 2013) and abnormal large HDL particles generated in patients with CE transfer protein (CETP) deficiency were shown to be a poor substrate for this reaction (Okumura-Noji et al., 2001). The gene mutations to cause this disorder are exclusively found in East Asia, potentially being associated with the historical endemic range of S. japonicum (Yokoyama, 2014; Yokoyama, 2015; Yokoyama et al., 2015).
LDL Receptor–Like Molecules in Schistosomes
LDL is one of the major lipid protein complexes in blood plasma and thought to transport lipid molecules from the liver to the peripheral tissues via circulating blood plasma. Cells take up LDL particles by the endocytotic LDL receptor to transport them by endosome to lysosome, where the lipid and protein molecules are hydrolyzed and cholesterol molecules are then intracellularly redistributed (Brown and Goldstein, 1986). The LDL receptor was defined as the major catabolic pathway to clear plasma LDL by identifying that its functional deficiency is the causative factor of familial hypercholesterolemia (Brown and Goldstein, 1974a). This pathway is the first well-characterized reactions at cellular and molecular biology levels in detail for trafficking and metabolic regulation of cholesterol (Brown and Goldstein, 1974b). As LDL is the major lipoprotein in human plasma and the LDL receptor is the best characterized molecules for the uptake of extracellular cholesterol, it is naturally conceivable that this most abundant lipid carrier would be a major source of lipid nutrients for blood flukes as well. LDL-specific binding components were, in fact, identified in the tegument of S. mansoni cercariae as a protein of 45 kDa(Rumjanek et al., 1983; Rumjanek et al., 1985; Rumjanek et al., 1988), much smaller than the mammalian LDL receptor of 95 kDa (Yamamoto et al., 1984), being induced by incubation with human serum. Other reports demonstrated the proteins with even smaller molecular weights of some 15.7 and 17.8 kDa (Xu and Caulfield, 1992) or 14, 35, and 60 kDa (Tempone et al., 1997) to interact with human LDL in a specific manner. A similar molecule of 43 kDa was identified in the S. japonicum tegument for specific interaction with human LDL (Rogers et al., 1989; Rogers et al., 1990). Ingestion and subsequent intracorporeal distribution of LDL were also demonstrated in S. mansoni by using LDL containing fluorescence-labeled hydrophobic molecules of 3,3′-diindolylmethene (DiI) (Bennett and Caulfield, 1991) or boron-dipyrromethene– and nitrobenzoxadiazole-coupled phosphatidylcholines (Furlong et al., 1995). Another molecule was identified in the Asian blood fluke S. japonicum from its cDNA library deduced to the sequence of 207–amino acid residues containing a transmembrane domain in the C-terminal and a Cys-rich motif in the N-terminal homologous to the Cys-rich repeats of the ligand binding domains in the mammalian LDL/VLDL receptors (Fan et al., 2003). However, recent proteomics analysis of surface proteins of S. mansoni failed to prove the presence of any homologous protein to the mammalian LDL receptors (Braschi et al., 2006a; Braschi et al., 2006b; Braschi and Wilson, 2006). No functional analysis has been successful to demonstrate physiological relevance of any of these LDL receptor–like molecules for a major ingesting mechanism of the lipid nutrients for schistosomes (Skelly et al., 2014).
HDL as a Potential Functional Source of Lipid Nutrient for Schistosomes
HDL is another major lipid-protein particle in human blood plasma to carry lipids. HDL is thought to transport lipid molecules from the somatic cells to the liver, especially cholesterol molecules as a part of their catabolic pathway. Cholesterol molecule is not catabolized in most of somatic cells in animals so that it must be exported from the cells and transported to the liver where it is converted to bile acids for excretion. However, cellular uptake of the lipid molecules carried by HDL is much less characterized than that of LDL. It has been implicated that lipid uptake pathway by cells is different between LDL and HDL. Unlike endocytotic uptake of LDL particles by the LDL receptor and subsequent lysosomal hydrolysis of its lipid molecules (Brown and Goldstein, 1986), cells have been shown to take up selectively CE from HDL particles (Rinninger and Pittman, 1987; Goldberg et al., 1991; Green and Pittman, 1991; Khoo et al., 1995). CE derived from HDL was shown hydrolyzed even in the fibroblasts of patients with Wolman’s disease where LDL-CE is not hydrolyzed due to deficiency of lysosomal acid lipase (Sparrow and Pittman, 1990). SR-B1, a membrane protein closely related to CD36 widely functional in cellular immune response, has been identified as a mediator for selective uptake of CE from HDL particle in mouse (Acton et al., 1996). This reaction was demonstrated crucial for the mouse adrenal glands to generate glucocorticoid in vivo (Hoekstra et al., 2012). CLA-1 as a human counterpart of SR-B1 was also shown to function in cellular CE uptake from HDL (Imachi et al., 1999a; Imachi et al., 1999b; Hoekstra et al., 2012). However, it is puzzling that large increase is found in unesterified cholesterol but not CE in the HDL fraction of SR-B1–deficient mice (Hildebrand et al., 2010). More recently, Aster proteins were found to facilitate transfer of HDL-derived cholesterol from plasma membrane to endoplasmic reticulum, but no information is provided for CE (Sandhu et al., 2018). Thus, study of this pathway is still preliminary, and the mechanisms are largely unknown for selective CE uptake, its intracellular trafficking and its extralysosomal hydrolysis (Connolly et al., 1996; Pollard et al., 2015; Gillard et al., 2017; Shen et al., 2018). To make it more confusing, scavenger receptors have been proposed to play a key role in atherogenesis by unregulated uptake of modified LDL such as oxidized LDL generated by retention of LDL in blood plasma (Dhaliwal and Steinbrecher, 1999; Van Berkel et al., 2000; Greaves and Gordon, 2009).
The eggs of S. japonicum undergo growth to miracidia by using their yolk after they are laid and excreted and hatch in fresh water in the environment (Skelly et al., 2014). This embryonation process to miracidia was found to require the presence of plasma HDL but not apoB lipoproteins (LDL and VLDL) in direct culture, and pre-exposure of the parents to HDL partially rescues their eggs to grow (Figure 1) (Okumura-Noji et al., 2013). In addition, the eggs were shown to take up selectively CE from HDL but not from LDL (Figure 2) (Okumura-Noji et al., 2013). The nature of the reaction is consistent with CE uptake by SR-B1 (Acton et al., 1996). Thereby, HDL was implicated to be more important nutrients source than LDL for generating the egg yolk of S. japonicum.
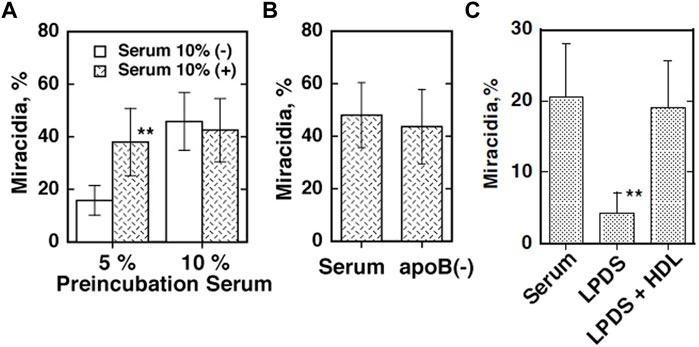
FIGURE 1. Embryonation/maturation of the S. japonicum eggs in culture (Okumura-Noji et al., 2013). The percentage of miracidia in the total eggs per one pair of the adult parasites cultured for 10 days was counted and estimated as efficiency of embryonation. (A) The eggs were separated from the adults after 2 days of culture in the medium containing five or 10% human serum and cultured for 8 days in the fresh media with or without 10% serum. (B) The eggs were removed from the parents cultured in the same condition as A with 5% serum and further cultured in 10% of whole serum and its d = 1.063 bottom fraction [apoB (−)]. (C) A pair of the parent adults was cultured for 10 days in the media with lipoprotein-depleted serum (LPDS) (4 mg protein/ml) with or without isolated HDL fraction (150 μg cholesterol/ml). The numbers of the adult pairs assayed were 6 (A) and 8 (B,C). The data represented the average and SE. **p < 0.005 and *p < 0.05.
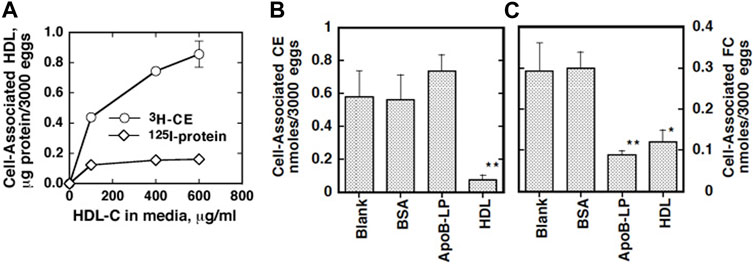
FIGURE 2. Specific cholesterol uptake from lipoproteins by S. japonicum eggs (Okumura-Noji et al., 2013). (A) Human HDL was labeled with [3H]CE or 125iodine. The S. japonicum eggs were incubated with [3H]CE-HDL or 125I-HDL at 37 and 4°C for 20 h. Association of HDL the eggs was estimated as HDL protein calculated from each specific activity. Specific association was estimated by displacement by 10 times of the amount of non-labeled HDL and difference between the results at 37°C and 4°C. (B and C) Selective uptake of cholesterol by S. japonicum eggs from HDL. HDL was double-labeled with [3H]CE and [14C]cholesterol and incubated with the S. japonicum eggs in the presence of 10 times of excess of non-labeled HDL, non-labeled apoB lipoprotein (ApoB-Lp) or BSA (1 μg/ml). Uptakes of CE (B) or cholesterol [FC, (C)] were calculated as the difference between the specific values at 37°C and 4°C. The data represent the average and SE of the triplicate assay. **p < 0.01 and *p < 0.05 different from “Blank”.
CD36-like class B scavenger receptor proteins were identified in schistosomes. Dinguirard and Yoshino reported a CD36-like protein in S. mansoni and demonstrated its binding to acetylated LDL in the sporocytes (Dinguirard and Yoshino, 2006). However, it has never been characterized for either the interaction with HDL or any physiological function. The presence of a similar molecule was demonstrated as a tegument-exposed protein in both male and female adults of S. japonicum (Zhang et al., 2013). This CD36-related protein (CD36RP) has been fully identified in S. japonicum by cloning from its cDNA library with 1,880 bp encoding 506–amino acid residues, including the CD36 domains and two transmembrane regions, and expressed in the adults and the eggs (Okumura-Noji et al., 2013). This protein was demonstrated to interact selectively with HDL by its extracellular loop domain, and the antibody against this region was shown to inhibit selective CE uptake from HDL and embryonation to miracidia of the eggs of S. japonicum (Figure 3) (Okumura-Noji et al., 2013). Thus, this protein likely mediates CE uptake by the parasites from HDL as a key reaction for growth and embryonation to miracidia of the eggs of S. japonicum. The two proteins from S. mansoni and S. japonicum are homologous including highly conserved four Cys and four Pro residues in the domains from IPB002159D to IPB002159F like other CD36 family proteins (Figure 4) (Okumura-Noji et al., 2013), so that CD36-like protein in S. mansoni may also function to interact with HDL.
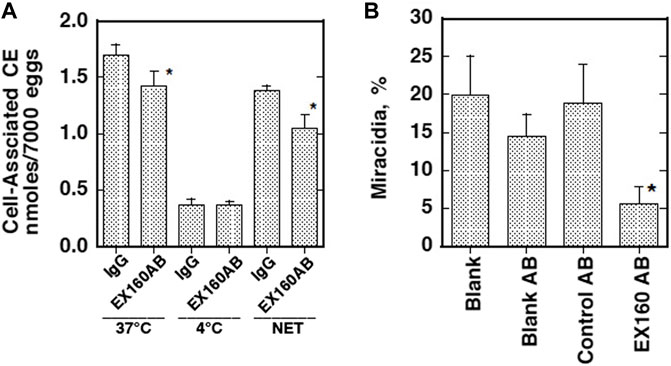
FIGURE 3. Suppression of HDL-CE uptake and maturation of the eggs by the antibody against extracellular loop of CD36RP (EX160AB) (Okumura-Noji et al., 2013). (A) Uptake of HDL-CE was measured in the same system as used in Figure 2, in the presence of the antibody. (B) Maturation of S. japonicum eggs was estimated in the same condition as Figure 1 except for using 5% serum, in the presence of the antibodies. IgG, non-immune rabbit IgG; EX160AB, the antibody against Ex160. Blank, with no additional antibody/antisera. Blank AB, with 1/100 volume of non-immune rabbit serum; Control AB, with 1/100 volume of rabbit antisera against the intracellular domain peptide of CD36RP (anti-peptide 331–348, anti-P); the titer of Ex160 was adjusted to less than 1/25 of the anti-P antisera. Data represent M ± SE of n = 6 for each group. An asterisk indicates p < 0.05 against IgG, Blank, Blank AB, and Control AB.
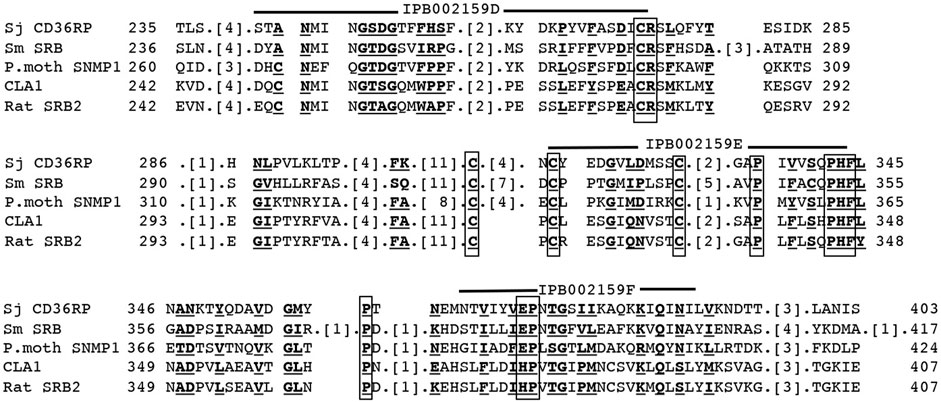
FIGURE 4. Multi-alignment of the amino acid sequences of CD36RP of S. Japonicum (Sj CD36RP) (Okumura-Noji et al., 2013) in comparison to other CD36 family proteins, S. mansoni scavenger receptor B (CD36-like protein) (Sm SRB) (Dinguirard and Yoshino, 2006), sensory neuron membrane protein of Polyphemus moth (P.moth SNMP-1), CLA1 as a human counterpart of SR-B1, and rat SR-B1. The amino acid residues in bald letters and underlined letters are conserved in 32 proteins from a variety of organisms. Conserved Cys and Pro residues in CD36 blocks IPB002159D to F regions are indicated as boxed.
HDL has been claimed to have “beneficial” effects other than cholesterol transport, such as antioxidative or anti-inflammatory functions, which may be mediated by sphingosine-1-phosphate concentrated on HDL to induce intracellular signaling pathway (Nagao et al., 2018). However, these reactions have not been claimed for association with CD36-type HDL receptor.
The findings above were supportive to and providing molecular basis for the preceding interesting observations about the function of HDL from the patients CETP deficiency for embryonation of S. japonicum eggs. The eggs of S. japonicum require HDL to embryonate to miracidia as mentioned above. Growth of the S. japonicum eggs to miracidia was retarded in the plasma of patients with CETP deficiency (Okumura-Noji et al., 2001). The HDL taken from the homozygous CETP deficiency patients, however, failed to substitute lacking of HDL for embryonation of the eggs (Figure 5) (Okumura-Noji et al., 2001). Take-up of CE by the eggs was less from the HDL isolated from a patient with CETP deficiency (Figure 6). The findings were reproduced by using the plasma of mouse, where the wild type is a CETP deficiency model lacking plasma CETP activity and the CETP transgenic mouse is accordingly a model for normal human (Okumura-Noji et al., 2001). In the infection of S. japonicum in the mouse model, the number of the aberrant eggs in the liver was not different between the wild-type and CETP-transgenic mice, but the ectopic embryonation to miracidia was significantly more in the CETP-transgenic mice (Figure 7) (Okumura-Noji et al., 2001). Most interestingly, development of granulomatosis was found more also in the transgenic mice, indicating that lack of CETP leads to resistance to the fatal hepatic complication in Schistosomiasis by S. japonicum (Table 1) (Okumura-Noji et al., 2001).
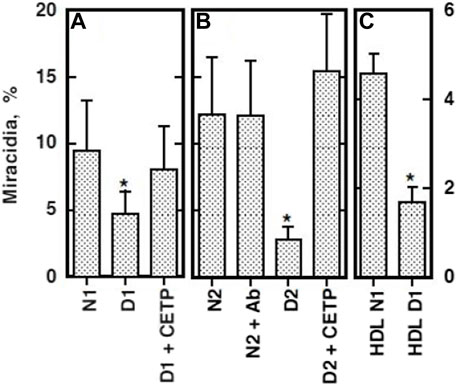
FIGURE 5. Embryonation of the eggs of S. japonicum in vitro in the presence of HDL from patients with CETP deficiency (Okumura-Noji et al., 2001). Egg embryonation was estimated when an adult pair of the parasite was cultured for 8 and 10 days (A–C), respectively). The culture media contained 10% normal human sera (N1 and N2) and CETP-deficient human sera (D1 and D2). Patients with CETP deficiency were both identified as intron 14 splicing defect. Ab indicates the presence of anti-CETP inhibitory monoclonal antibody (IgG). CETP indicates presence of purified human CETP. HDL N1 and HDL D1 indicate the HDL isolated from normal human plasma (N1) or the CETP-deficient plasma (D1). Each panel represents an independent experiment, in which the data points are the average ± SE of the four (A, C) and eight (B) measurements. *p < 0.05 different from N. *p = 0.059 between HDL N1 and HDL D1.
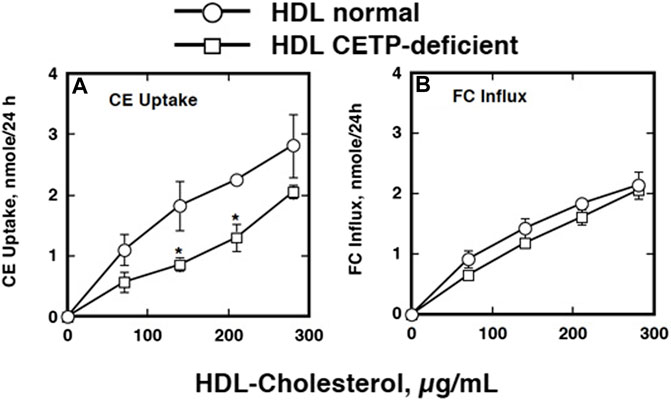
FIGURE 6. Active CE uptake by the eggs of S. japonicum from normal HDL and that isolated from CETP deficiency (Okumura-Noji et al., 2001). HDL was labeled with [3H]CE and active uptake was determined by the difference between the values at 37°C and 4°C. The data represent the average and SE of the triplicate assay. Symbols are as follows: circles, HDL prepared from normal human plasma; squares, CETP-deficient human plasma. *p < 0.05 between normal HDL and CETP-deficient HDL. Panel (A), CE uptake. Panel (B), FC influx.
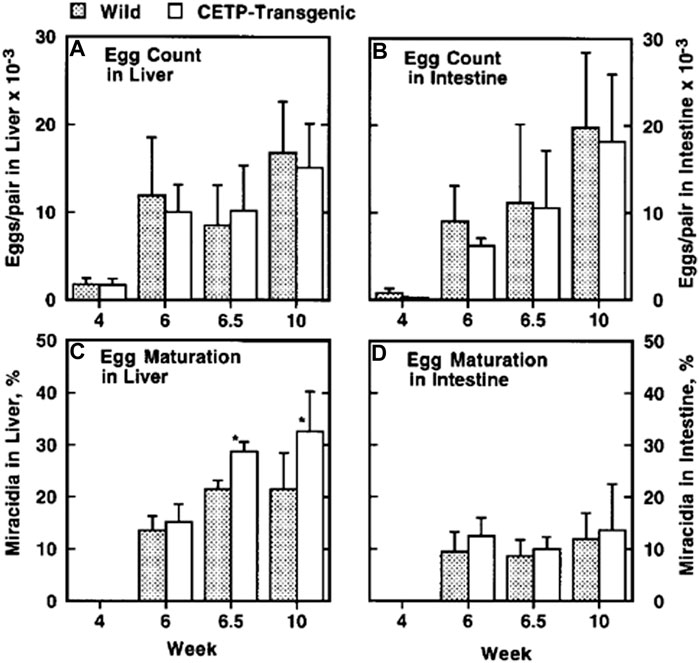
FIGURE 7. Egg analysis in the mice infected by S. japonicum (Okumura-Noji et al., 2001). The CETP transgenic and wild-type mice were infected. Total eggs recovered were counted in the liver and intestine and the egg number per an adult pair of the parasite in the portal vein was calculated for individual mouse. Egg embryonation to miracidia was microscopically counted for 100 eggs, for the liver and intestine in each mouse. (A,B) The egg count, in the liver and in the intestine. No difference was observed between wild type C57BL/6 mice and human CETP-transgenic mice. (C,D) The egg embryonation as indicated by the relative content of miracidia. In the CETP-transgenic mice, the eggs were matured significantly more in the liver by *p < 0.001, whereas there is no difference in the intestine. N = 10.

TABLE 1. Granulomatosis lesions in the liver (Okumura-Noji et al., 2001).
Endemic of CETP Deficiency
CETP is a plasma protein to catalyze equimolar non-directional exchange of CE and triglyceride (TG) among lipoproteins (Ohnishi et al., 1994a; Ohnishi et al., 1994b; Ko et al., 1994; Qiu et al., 2007) in certain species of mammalians including humans (Bruce et al., 1995; Jiang et al., 1995; Tall, 1995; Wang et al., 1995). Human CETP is composed of 476–amino acid residues (Drayna et al., 1987) with a glycosylated molecular weight of 74 kDa. The reaction equalizes distribution of the core lipids among lipoprotein particles and accordingly causes net transfer of CE from HDL to TG-rich lipoproteins such as VLDL and TG reversely to HDL and LDL (Ko et al., 1994). CE is generated on HDL in plasma by enzymatic cholesterol acyl-esterification and TG in VLDL and chylomicron originates in the liver and the intestine, and CETP reaction moves CE out of HDL and, in turn, transfers TG both to HDL and LDL where TG is hydrolyzed by hepatic or lipoprotein lipases. Accordingly, the size of HDL and LDL becomes smaller to produce HDL3 and “small dense” LDL. The increase in plasma TG, in fact, decreases plasma HDL and increases small dense LDL in the presence of CETP, and HDL-CE increases by decrease of CETP reaction (Föger et al., 1996).
Generation of CE in HDL is one of the driving forces to remove cell cholesterol (Czarnecka and Yokoyama, 1993) to play one of the key roles in cholesterol transport from peripheral cells to the liver for its catabolism. CETP reaction facilitates this pathway by transferring HDL-CE to LDL for its efficient recovery by the hepatic LDL receptor (Francis et al., 1991). On the other hand, increase of HDL surface by decrease of CETP activity may provide more capacity to accept cell cholesterol.
The patients with CETP deficiency were first described in Japan in 1985 as the cases with hyperalphalipoproteinemia (Koizumi et al., 1985; Kurasawa et al., 1985). Its genetic background was soon established (Brown et al., 1989), and many cases were found in Japan thereafter. Two major genotypes have been identified as intron 14 G (+1)-to-A (Int14A) and exon 15 missense mutation (D442G) (Inazu et al., 1994; Sakai et al., 1995; Hirano et al., 1997). Prevalence of these two mutants was found very high among Japanese general population as 1%–2% and 6%–7%, respectively. Many sporadic cases were also identified with more than 10 other types of mutations among Japanese (Yamashita et al., 2000; Maruyama et al., 2004; Nagano et al., 2004). The heterozygotes can therefore be estimated around 10 million, and the homozygotes would be as many as 150,000 to 250,000 in Japan. CETP deficiency may account for 27.6% of the people with HDL cholesterol ≥60 mg/dl and 31.4%–32.5% of those with HDL ≥80 mg/dl in Japan (Inazu et al., 1994; Nagano et al., 2002). City of Omagari, Akita district in Northern Japan, was found with high accumulation of the Int14A mutant, showing the prevalence of the heterozygote 27% (Hirano et al., 1997). Thus, genetic CETP deficiency is highly common among Japanese.
The first non-Japanese patient was found in Switzerland as a Chinese descendant (Ritsch et al., 1997). Several reports thereafter described CETP deficiency among Asians. Prevalence of D442G mutant heterozygotes was found as 3.3%–10.8% among the mainland Chinese (Chiba et al., 1997; Hui, 1997; Chen et al., 2008) and 4.5%–7.7% in the population of Taiwan (Hsu et al., 2002; Jap et al., 2002; Nagano et al., 2002; Yang et al., 2006). It could be estimated as 12% among Koreans based on its allele frequency 6% (Song et al., 1997). Vietnamese D442G mutants were estimated as 6.9% in their general population (Thu et al., 2005). Nine cases were found as D442G heterozygotes among the 35 hyperalphalipoproteinemia individuals in Thailand accounting for 26% (Plengpanich et al., 2009) same as Japanese (Inazu et al., 1994; Nagano et al., 2002). Detailed information should be referred to the previous review article (Thompson et al., 2009). Interestingly, analysis of elderly Siberian Yakuts showed the prevalence of D442G mutant 16.2% in the native Yakuts and 5.2% among the non-indigenous (Arkhipova et al., 2013), mostly Russians and Ukrainians whose intermarriage with Yakuts is 10%–20% (Arkhipova et al., 2013; Yokoyama et al., 2015). No reliable information is available for Int14A mutation, except for two out of the 145 subjects (1.4%) in Hong Kong Chinese (Ma et al., 2001; Okumura-Noji et al., 2001; Takahashi et al., 2001; Zhang et al., 2001; Hsu et al., 2002; Nihei et al., 2006; Finkelstein et al., 2008) and none in the 346 Vietnamese (Thu et al., 2005). CETP deficiency is thus highly prevalent widely in East Asia, predominantly with D442G mutant. Int14A may be second common but it is unclear except in Japan. Many other types of mutations have been found in Japan but information from other Asian regions is not adequate. The data are summarized in Table 2.
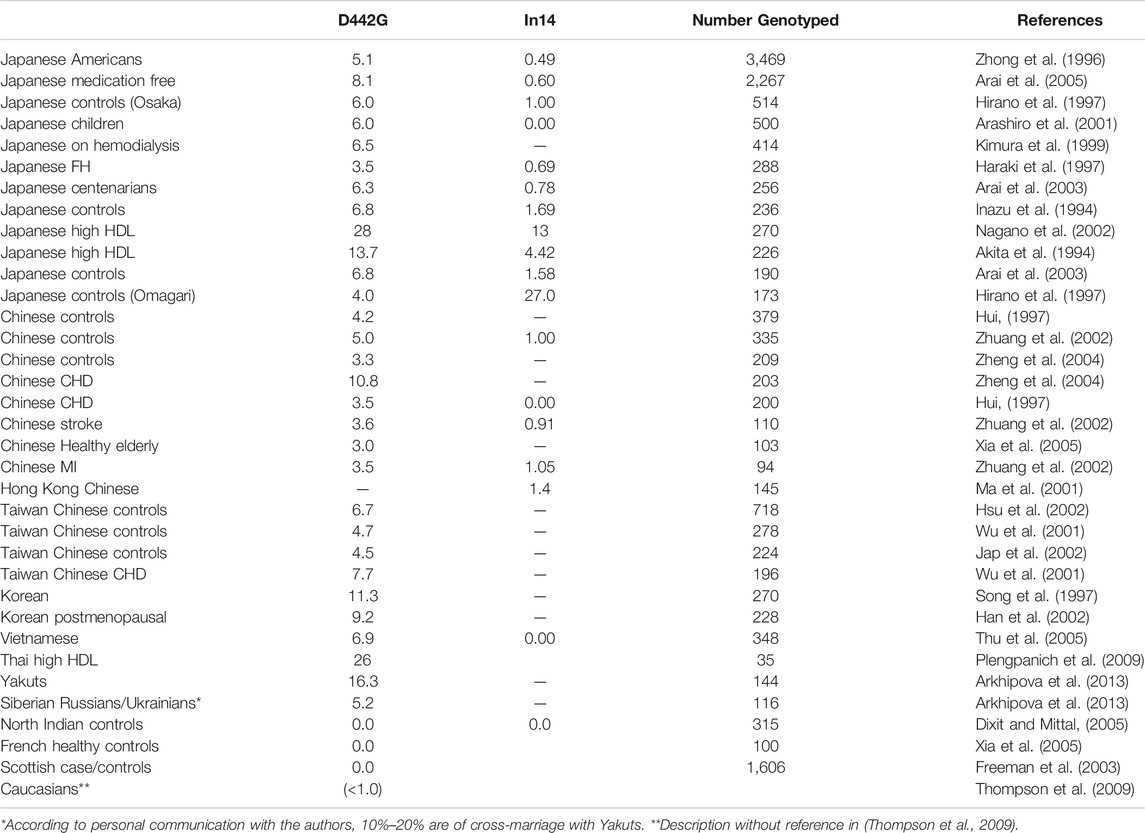
TABLE 2. Frequency of CETP deficiency in various regions of the world as prevalence of mutants in population (%).
In contrast, CETP deficiency seems rare in any other ethnic groups. The first Caucasian case was reported in 1997 in North America (Teh et al., 1998), and one case with Int14A was reported in 1998 in Canada without ethnic identification (Hill et al., 1997). It was thus said that CETP deficiency is rare among North American Caucasians (van der Steeg et al., 2007). A few studies later added sporadic cases of CETP deficiency in the United States (Rhyne et al., 2006), Italy (Calabresi et al., 2009; Cefalù et al., 2009), and the Netherlands (van der Steeg et al., 2007; Chantepie et al., 2012). There was an estimation of D442G mutant among Caucasians “less than 1%” without evident grounds (Thompson et al., 2008) (Table 2).
The only significant clinical manifestation in CETP deficiency is abnormal profile of plasma lipoprotein spectrum characterized by very high HDL cholesterol and moderate reduction of LDL cholesterol. Lack of CETP reaction accumulates CE in HDL particle to expand the core to make it as large as LDL and rich in apoE (Inazu et al., 1990; Yamashita et al., 1990; Sakai et al., 1991; Yamashita et al., 1991) and causes heterogeneity in size and lipid composition of LDL due to lack of core lipid equilibration (Yamashita et al., 1988; Hirano et al., 1992). The patients do not suffer from any serious clinical symptoms in general. Roles of CETP deficiency in atherogenesis are controversial as potential increase of the risk in the homozygotes (Hirano et al., 1997), whereas overall risk may decrease among the heterozygotes (Moriyama et al., 1998; Goto et al., 2001; Curb et al., 2004). The outcome of its pharmacological inhibition has also been inconclusive so far (Nissen et al., 2007; Schwartz et al., 2012; Bowman et al., 2017).
Is S. japonicum Associated With High Prevalence of CETP Deficiency in East Asia?
Two potential mechanisms are considered for geographic or ethnic accumulation of a genetic abnormality: “founders’ effect” and screening by a regional fatal disease(s), which are mostly infectious in these situations. The former cases are found in relatively isolated communities of the descendants from earlier settlers. Typical examples are familial hypercholesterolemia accumulated in French Canadians (Bétard et al., 1992), in Afrikaners of South Africa (Torrington et al., 1984) and maybe in Lebanese (Abifadel et al., 2009). In this case, the accumulated mutations are not highly diverse as originating in a few carrier families. On the other hand, the latter is represented by sickle cell anemia and other hemoglobinopathies that is resistant to malaria infection and therefore stated to be a reason for its high prevalence among African ethnic groups (Allison, 1954; Hedrick, 2012; Taylor et al., 2012). This case may affect larger populations historically exposed to specific diseases. There are more examples to implicate similar association (Withrock et al., 2015) such as cholera and cystic fibrosis (Muanprasat and Chatsudthipong, 2013), tuberculosis and Tay–Sachs disease (Spyropoulos et al., 1981), mycotic abortions, and phenylketonuria (Woolf et al., 1975) likely causing selective pressure. The others are also suggested resistance but not to a selection level such as enveloped viruses and disorders of glycosylation (Sadat et al., 2014), filoviruses and Niemann–Pick C1 disease (Carette et al., 2011), and rabies and myasthenia gravis (Lafon, 2005).
Majority of the patients with CETP deficiency accumulated in East Asia may be limited to one or two type(s) of mutations but further diversity of mutation is also found in the region. In addition, the region with high prevalence of the mutants is so large in East Asia beyond considering founder’s effect. No specific settler family can be conceivable to account for such large descending population affected. The only exception is extreme accumulation of the mutant int14A in Omagari, Japan, which can be a potential case of the local “founders’ effect” (Hirano et al., 1997). However, the only significant phenotype of CETP deficiency is abnormal plasma lipoprotein profile to which very few infectious diseases are found related. Schistosomiasis is one of the few to meet such criteria as discussed above.
The reason for poor reactivity of the HDL of CETP deficiency is not clear at this moment. CETP is a plasma protein that mediates nondirectional transfer of hydrophobic core lipids like CE and TG between lipoprotein particles (Ohnishi et al., 1994b). As CE is enzymatically generated constantly in HDL particles in plasma, net transfer of CE to LDL and VLDL occurs mainly in exchange with TG by the reaction of CETP (Ko et al., 1994). In the lack of CETP, CE remains and accumulates in HDL to make it enlarged, sometimes as large as LDL as described above (Inazu et al., 2008). Such a large HDL may be dysfunctional in its cellular interaction and selective uptake of CE for some reason. Remodeling of HDL by CETP was shown to result in enhancement of CE uptake from HDL by SR-B1 in cultured cells (Collet et al., 1999) and expression of CETP in mice enhanced HDL-CE uptake in the liver (Harada et al., 2007). These findings are consistent with the observations that CD36-RP is not functional to take up CE from the HDL of CETP-deficient plasma.
The regions the mutations are found with high prevalence of CETP deficiency largely overlap with the areas where S. japonicum infection is either currently still endemic or it has been or potentially been highly endemic in the historic records (Figure 8; Table 2) (Yokoyama, 2014; Yokoyama, 2015; Yokoyama et al., 2015). The high prevalence among Yakuts may indicate genetic drift from their Asian ancestors. Many of these areas have been civilized under the culture of water farming of crops such as rice, so that their common life style fits for incubation of Schistosome life cycle. As the homozygotes of CETP deficiency are apparently resistant for development of the fatal complication of Schistosomiasis japonica, i.e., hepatic granulomatosis and cirrhosis in the mouse model (Okumura-Noji et al., 2001), it is conceivable that this disease was one of the factors that caused high prevalence of the CETP gene mutations in the East Asia.
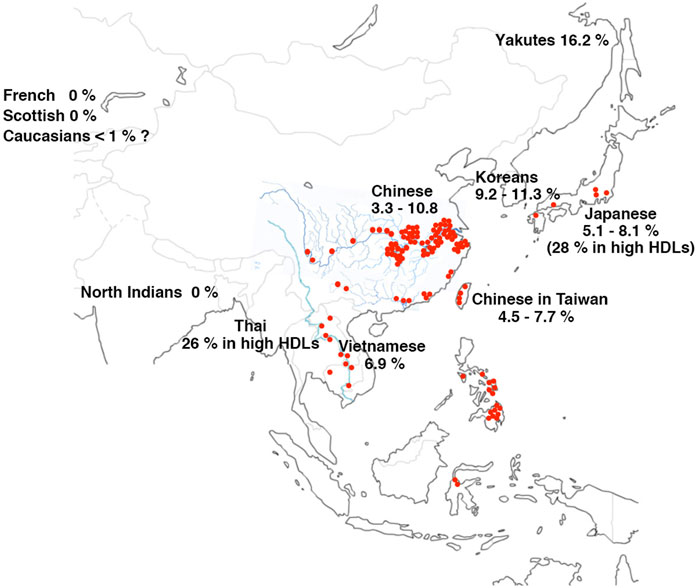
FIGURE 8. Endemics map of S. japonicum and CETP deficiency (D442G). Red spots show the regions where the cases of infection by S. japonicum were found currently and historically since the early 20th century when the parasite was identified. The regions spread over Japan, China including Taiwan, Mekong valleys, Thailand, Philippines, and Indonesia. The endemic was eliminated in many of these regions by the 21st century. Data of the prevalence of G442G available in literature till today are listed as percentage in each ethnic general population as listed in Table 2.
Conclusion
S. japonicum expresses CD36RP in the adults and their eggs, which mediates selective CE uptake from the host plasma HDL as a pathway for the parasite to ingest lipid nutrients (Okumura-Noji et al., 2013). This reaction seems essential for the parasite eggs to embryonate to miracidia, likely not only for generation of the yolk before they are laid but also for replenishment after being laid. Large abnormal HDL caused by CETP deficiency becomes a poor substrate for the CD36RP-mediated CE uptake (Okumura-Noji et al., 2001; Okumura-Noji et al., 2013), so that the aberrant eggs into the liver are to grow less to miracidia and generate less granulomatosis in the patients with CETP deficiency. Thus, S. japonicum infection can be one of the factors to screen CETP deficiency in East Asia. Because the ectopic embryonation is the major fatal complication of schistosomiasis, the CD36RP pathway is an important potential target to inhibit for prevention of the fatal complication of this disease (Okumura-Noji et al., 2013). Inhibition of CETP can be an approach to block this process and prevent the liver complication when the infection outbreak is feared such as a case of major flooding in the endemic region. Alternatively, CD36RP can be a novel vaccination target to prevent the liver complication. The data are still limited to S. japonicum so that S. mansoni should be investigated to find whether the findings are more general. Further studies are required to understand association of S. japonicum infection and CETP gene mutation based on genome-wide analysis for evolutional backgrounds of the human genes in certain regional or ethnic groups, like the recent findings of copy number variants in Melanesians selected from Denisovans and Neanderthals (Hsieh et al., 2019) or lack of inheritance from Neanderthals in East Asians of genetic risk factors for clinical severity of COVID-19 (Zeberg and Pääbo, 2020; Zeberg and Pääbo, 2021).
Author Contributions
SY extensively reviewed works and publications related to the topic and wrote the article.
Funding
This work was supported by the MEXT-Supported Program for Strategic Founding of Research in Private Universities (S1201007) and by Grants-in-aid from MEXT Japan (24614018, 26461370, 15H02903).
Conflict of Interest
The author declares that the research was conducted in the absence of any commercial or financial relationships that could be construed as a potential conflict of interest.
Publisher’s Note
All claims expressed in this article are solely those of the authors and do not necessarily represent those of their affiliated organizations, or those of the publisher, the editors and the reviewers. Any product that may be evaluated in this article, or claim that may be made by its manufacturer, is not guaranteed or endorsed by the publisher.
References
Abifadel, M., Rabès, J.-P., Jambart, S. l., Halaby, G., Gannagé-Yared, M.-H. l. n., Sarkis, A., et al. (2009). The Molecular Basis of Familial Hypercholesterolemia in Lebanon: Spectrum ofLDLRmutations and Role ofPCSK9as a Modifier Gene. Hum. Mutat. 30 (7), E682–E691. doi:10.1002/humu.21002
Acton, S., Rigotti, A., Landschulz, K. T., Xu, S., Hobbs, H. H., and Krieger, M. (1996). Identification of Scavenger Receptor SR-BI as a High Density Lipoprotein Receptor. Science 271 (5248), 518–520. doi:10.1126/science.271.5248.518
Akita, H., Chiba, H., Tsuchihashi, K., Tsuji, M., Kumagai, M., Matsuno, K., et al. (1994). Cholesteryl Ester Transfer Protein Gene: Two Common Mutations and Their Effect on Plasma High-Density Lipoprotein Cholesterol Content. J. Clin. Endocrinol. Metab. 79 (6), 1615–1618. doi:10.1210/jcem.79.6.7989465
Allison, A. C. (1954). Protection Afforded by Sickle-Cell Trait against Subtertian Malarial Infection. Bmj 1 (4857), 290–294. doi:10.1136/bmj.1.4857.290
Arai, H., Yamamoto, A., Matsuzawa, Y., Saito, Y., Yamada, N., Oikawa, S., et al. (2005). Polymorphisms in Four Genes Related to Triglyceride and HDL-Cholesterol Levels in the General Japanese Population in 2000. Jat 12 (5), 240–250. doi:10.5551/jat.12.240
Arai, Y., Hirose, N., Yamamura, K., Nakazawa, S., Shimizu, K.-i., Takayama, M., et al. (2003). Deficiency of Choresteryl Ester Transfer Protein and Gene Polymorphisms of Lipoprotein Lipase and Hepatic Lipase Are Not Associated with Longevity. J. Mol. Med. 81 (2), 102–109. doi:10.1007/s00109-002-0407-6
Arashiro, R., Katsuren, K., Maung, K. K., Fukuyama, S., and Ohta, T. (2001). Effect of a Common Mutation (D442G) of the Cholesteryl Ester Transfer Protein Gene on Lipids and Lipoproteins in Children. Pediatr. Res. 50 (4), 455–459. doi:10.1203/00006450-200110000-00006
Arkhipova, N. S., Ariev, A. L., Popova, E. K., Grigorieva, L. V., and Kozina, L. S. (2013). Association of Polymorphism of Angiotensin-Converting Enzyme Gene I/D and D442G Cholesterol Ester Transfer Protein Gene with Risk Factors for Atherosclerosis Among Elderly and Senile Patients with Coronary Heart Disease in the republic of Sakha (Yakutia). Adv. Gerontol. 3 (4), 277–281. doi:10.1134/s2079057013040024
Bennett, M. W., and Caulfield, J. P. (1991). Specific Binding of Human Low-Density Lipoprotein to the Surface of Schistosomula of Schistosoma Mansoni and Ingestion by the Parasite. Am. J. Pathol. 138 (5), 1173–1182.
Bergquist, R., and Tanner, M. (2010). Controlling Schistosomiasis in Southeast Asia. Adv. Parasitol. 72, 109–144. doi:10.1016/s0065-308x(10)72005-4
Bétard, C., Kessling, A. M., Roy, M., Chamberland, A., Lussier-Cacan, S., and Davignon, J. (1992). Molecular Genetic Evidence for a Founder Effect in Familial Hypercholesterolemia Among French Canadians. Hum. Genet. 88 (5), 529–536. doi:10.1007/bf00219339
Bowman, L., Hopewell, J. C., Chen, F., Wallendszus, K., Stevens, W., Collins, R., et al. (2017). Effects of Anacetrapib in Patients with Atherosclerotic Vascular Disease. N. Engl. J. Med. 377 (13), 1217–1227. doi:10.1056/NEJMoa1706444
Braschi, S., Borges, W. C., and Wilson, R. A. (2006). Proteomic Analysis of the Shistosome Tegument and its Surface Membranes. Mem. Inst. Oswaldo Cruz 101 (Suppl. 1), 205–212. doi:10.1590/s0074-02762006000900032
Braschi, S., Curwen, R. S., Ashton, P. D., Verjovski-Almeida, S., and Wilson, A. (2006). The Tegument Surface Membranes of the Human Blood parasiteSchistosoma Mansoni: A Proteomic Analysis after Differential Extraction. Proteomics 6 (5), 1471–1482. doi:10.1002/pmic.200500368
Braschi, S., and Wilson, R. A. (2006). Proteins Exposed at the Adult Schistosome Surface Revealed by Biotinylation. Mol. Cell Proteomics 5 (2), 347–356. doi:10.1074/mcp.M500287-MCP200
Brouwers, J. F., Smeenk, I. M., van Golde, L. M., and Tielens, A. G. (1997). The Incorporation, Modification and Turnover of Fatty Acids in Adult Schistosoma Mansoni. Mol. Biochem. Parasitol. 88 (1-2), 175–185. doi:10.1016/s0166-6851(97)00091-1
Brown, M. L., Inazu, A., Hesler, C. B., Agellon, L. B., Mann, C., Whitlock, M. E., et al. (1989). Molecular Basis of Lipid Transfer Protein Deficiency in a Family with Increased High-Density Lipoproteins. Nature 342 (6248), 448–451. doi:10.1038/342448a0
Brown, M. S., and Goldstein, J. L. (1986). A Receptor-Mediated Pathway for Cholesterol Homeostasis. Science 232 (4746), 34–47. doi:10.1126/science.3513311
Brown, M. S., and Goldstein, J. L. (1974). Expression of the Familial Hypercholesterolemia Gene in Heterozygotes: Mechanism for a Dominant Disorder in Man. Science 185 (4145), 61–63. doi:10.1126/science.185.4145.61
Brown, M. S., and Goldstein, J. L. (1974). Familial Hypercholesterolemia: Defective Binding of Lipoproteins to Cultured Fibroblasts Associated with Impaired Regulation of 3-Hydroxy-3-Methylglutaryl Coenzyme A Reductase Activity. Proc. Natl. Acad. Sci. 71 (3), 788–792. doi:10.1073/pnas.71.3.788
Bruce, C., Davidson, W. S., Kussie, P., Lund-Katz, S., Phillips, M. C., Ghosh, R., et al. (1995). Molecular Determinants of Plasma Cholesteryl Ester Transfer Protein Binding to High Density Lipoproteins. J. Biol. Chem. 270 (19), 11532–11542. doi:10.1074/jbc.270.19.11532
Calabresi, L., Nilsson, P., Pinotti, E., Gomaraschi, M., Favari, E., Adorni, M. P., et al. (2009). A Novel Homozygous Mutation in CETP Gene as a Cause of CETP Deficiency in a Caucasian kindred. Atherosclerosis 205 (2), 506–511. doi:10.1016/j.atherosclerosis.2009.01.006
Carette, J. E., Raaben, M., Wong, A. C., Herbert, A. S., Obernosterer, G., Mulherkar, N., et al. (2011). Ebola Virus Entry Requires the Cholesterol Transporter Niemann-Pick C1. Nature 477 (7364), 340–343. doi:10.1038/nature10348
Cefalù, A. B., Noto, D., Magnolo, L., Pinotti, E., Gomaraschi, M., Martini, S., et al. (2009). Novel Mutations of CETP Gene in Italian Subjects with Hyeralphalipoproteinemia. Atherosclerosis 204 (1), 202–207. doi:10.1016/j.atherosclerosis.2008.08.031
Chantepie, S., Bochem, A. E., Chapman, M. J., Hovingh, G. K., and Kontush, A. (2012). High-density Lipoprotein (HDL) Particle Subpopulations in Heterozygous Cholesteryl Ester Transfer Protein (CETP) Deficiency: Maintenance of Antioxidative Activity. PLoS One 7 (11), e49336. doi:10.1371/journal.pone.0049336
Cheever, A. W., Cheever, E. A., Mosimann, J. G. J. E., and Cheever, E. A. (1994). Kinetics of Egg Production and Egg Excretion by Schistosoma Mansoni and S. Japonicum in Mice Infected with a Single Pair of Worms. Am. J. Trop. Med. Hyg. 50 (3), 281–295. doi:10.4269/ajtmh.1994.50.281
Chen, D.-W., Yang, J.-F., Tang, Z., Dong, X.-M., Feng, X.-L., Yu, S., et al. (2008). Cholesteryl Ester Transfer Protein Polymorphism D442G Associated with a Potential Decreased Risk for Alzheimer's Disease as a Modifier for APOE ε4 in Chinese. Brain Res. 1187, 52–57. doi:10.1016/j.brainres.2007.10.054
Chiba, H., Akita, H., Tsuchihashi, K., Hui, S. P., Takahashi, Y., Fuda, H., et al. (1997). Quantitative and Compositional Changes in High Density Lipoprotein Subclasses in Patients with Various Genotypes of Cholesteryl Ester Transfer Protein Deficiency. J. Lipid Res. 38 (6), 1204–1216. doi:10.1016/s0022-2275(20)37202-3
Collet, X., Tall, A. R., Serajuddin, H., Guendouzi, K., Royer, L., Oliveira, H., et al. (1999). Remodeling of HDL by CETP In Vivo and by CETP and Hepatic Lipase In Vitro Results in Enhanced Uptake of HDL CE by Cells Expressing Scavenger Receptor B-I. J. Lipid Res. 40 (7), 1185–1193. doi:10.1016/s0022-2275(20)33480-5
Connolly, D. T., McIntyre, J., Heuvelman, D., Remsen, E. E., McKinnie, R. E., Vu, L., et al. (1996). Physical and Kinetic Characterization of Recombinant Human Cholesteryl Ester Transfer Protein. Biochem. J. 320, 39–47. doi:10.1042/bj3200039
Curb, J. D., Abbott, R. D., Rodriguez, B. L., Masaki, K., Chen, R., Sharp, D. S., et al. (2004). A Prospective Study of HDL-C and Cholesteryl Ester Transfer Protein Gene Mutations and the Risk of Coronary Heart Disease in the Elderly. J. Lipid Res. 45 (5), 948–953. doi:10.1194/jlr.M300520-JLR200
Czarnecka, H., and Yokoyama, S. (1993). Regulation of Lecithin-Cholesterol Acyltransferase Reaction by Acyl Acceptors and Demonstration of its "idling" Reaction. J. Biol. Chem. 268 (26), 19334–19340. doi:10.1016/s0021-9258(19)36518-4
Dhaliwal, B. S., and Steinbrecher, U. P. (1999). Scavenger Receptors and Oxidized Low Density Lipoproteins. Clin. Chim. Acta 286 (1-2), 191–205. doi:10.1016/s0009-8981(99)00101-1
Dinguirard, N., and Yoshino, T. P. (2006). Potential Role of a CD36-like Class B Scavenger Receptor in the Binding of Modified Low-Density Lipoprotein (acLDL) to the Tegumental Surface of Schistosoma Mansoni Sporocysts. Mol. Biochem. Parasitol. 146 (2), 219–230. doi:10.1016/j.molbiopara.2005.12.010
Dixit, M., and Mittal, B. (2005). Frequencies of CETP Gene TaqI B and D442G Polymorphisms in North Indian Population. Curr. Sci. 88, 1973–1976.
Drayna, D., Jarnagin, A. S., McLean, J., Henzel, W., Kohr, W., Fielding, C., et al. (1987). Cloning and Sequencing of Human Cholesteryl Ester Transfer Protein cDNA. Nature 327, 632–634. doi:10.1038/327632a0
El Ridi, R., Velupillai, P., and Harn, D. A. (1996). Regulation of Schistosome Egg Granuloma Formation: Host-Soluble L-Selectin Enters Tissue-Trapped Eggs and Binds to Carbohydrate Antigens on Surface Membranes of Miracidia. Infect. Immun. 64 (11), 4700–4705. doi:10.1128/iai.64.11.4700-4705.1996
Fan, J., Gan, X., Yang, W., Shen, L., McManus, D. P., and Brindley, P. J. (2003). A Schistosoma Japonicum Very Low-Density Lipoprotein-Binding Protein. Int. J. Biochem. Cell Biol. 35 (10), 1436–1451. doi:10.1016/s1357-2725(03)00105-5
Finkelstein, J. L., Schleinitz, M. D., Carabin, H., and McGarvey, S. T. (2008). Decision-model Estimation of the Age-specific Disability Weight for Schistosomiasis Japonica: a Systematic Review of the Literature. Plos Negl. Trop. Dis. 2 (3), e158. doi:10.1371/journal.pntd.0000158
Föger, B., Ritsch, A., Doblinger, A., Wessels, H., and Patsch, J. R. (1996). Relationship of Plasma Cholesteryl Ester Transfer Protein to HDL Cholesterol. Studies in Normotriglyceridemia and Moderate Hypertriglyceridemia. Arterioscler Thromb. Vasc. Biol. 16 (12), 1430–1436. doi:10.1161/01.atv.16.12.1430
Francis, G. A., Ko, K. W. S., Hitoshi, H., and Yokoyama, S. (1991). Regulation of the Uptake of High-Density Lipoprotein-Originated Cholesteryl Ester by HepG2 Cells: Role of Low-Density Lipoprotein and Plasma Lipid Transfer Protein. Biochim. Biophys. Acta (Bba) - Lipids Lipid Metab. 1084 (2), 159–166. doi:10.1016/0005-2760(91)90215-4
Freeman, D., Samani, N. J., Wilson, V., McMahon, A. D., Braund, P. S., Cheng, S., et al. (2003). A Polymorphism of the Cholesteryl Ester Transfer Protein Gene Predicts Cardiovascular Events in Non-smokers in the West of Scotland Coronary Prevention Study. Eur. Heart J. 24 (20), 1833–1842. doi:10.1016/j.ehj.2003.07.001
Furlong, S. T., Thibault, K. S., Morbelli, L. M., Quinn, J. J., and Rogers, R. A. (1995). Uptake and Compartmentalization of Fluorescent Lipid Analogs in Larval Schistosoma Mansoni. J. Lipid Res. 36 (1), 1–12. doi:10.1016/s0022-2275(20)39749-2
Gillard, B. K., Bassett, G. R., Gotto, A. M., Rosales, C., and Pownall, H. J. (2017). Scavenger Receptor B1 (SR-B1) Profoundly Excludes High Density Lipoprotein (HDL) Apolipoprotein AII as it Nibbles HDL-Cholesteryl Ester. J. Biol. Chem. 292 (21), 8864–8873. doi:10.1074/jbc.M117.781963
Goldberg, D. I., Beltz, W. F., and Pittman, R. C. (1991). Evaluation of Pathways for the Cellular Uptake of High Density Lipoprotein Cholesterol Esters in Rabbits. J. Clin. Invest. 87 (1), 331–346. doi:10.1172/jci114991
Gordon, C. A., Acosta, L. P., Gray, D. J., Olveda, R. M., Jarilla, B., Gobert, G. N., et al. (2012). High Prevalence of Schistosoma Japonicum Infection in Carabao from Samar Province, the Philippines: Implications for Transmission and Control. Plos Negl. Trop. Dis. 6 (9), e1778. doi:10.1371/journal.pntd.0001778
Goto, A., Sasai, K., Suzuki, S., Fukutomi, T., Ito, S., Matsushita, T., et al. (2001). Cholesteryl Ester Transfer Protein and Atherosclerosis in Japanese Subjects: a Study Based on Coronary Angiography. Atherosclerosis 159 (1), 153–163. doi:10.1016/s0021-9150(01)00476-2
Greaves, D. R., and Gordon, S. (2009). The Macrophage Scavenger Receptor at 30 Years of Age: Current Knowledge and Future Challenges. J. Lipid Res. 50, S282–S286. doi:10.1194/jlr.R800066-JLR200
Green, S., and Pittman, R. (1991). Comparative Acyl Specificities for Transfer and Selective Uptake of High Density Lipoprotein Cholesteryl Esters. J. Lipid Res. 32 (3), 457–467. doi:10.1016/s0022-2275(20)42069-3
Gryseels, B., Polman, K., Clerinx, J., and Kestens, L. (2006). Human Schistosomiasis. The Lancet 368, 1106–1118. doi:10.1016/s0140-6736(06)69440-3
Han, K. O., Yim, C. H., Jung, H. Y., Jang, H. C., Yoon, H. K., Cho, D. H., et al. (2002). A Common Mutation in Cholesteryl Ester Transfer Protein Gene and Plasma HDL Cholesterol Level before and after Hormone Replacement Therapy in Korean Postmenopausal Women. Korean J. Intern. Med. 17 (2), 83–87. doi:10.3904/kjim.2002.17.2.83
Harada, L. M., Amigo, L., Cazita, P. M., Salerno, A. G., Rigotti, A. A., Quintão, E. C. R., et al. (2007). CETP Expression Enhances Liver HDL-Cholesteryl Ester Uptake but Does Not Alter VLDL and Biliary Lipid Secretion. Atherosclerosis 191 (2), 313–318. doi:10.1016/j.atherosclerosis.2006.05.036
Haraki, T., Inazu, A., Yagi, K., Kajinami, K., Koizumi, J., and Mabuchi, H. (1997). Clinical Characteristics of Double Heterozygotes with Familial Hypercholesterolemia and Cholesteryl Ester Transfer Protein Deficiency. Atherosclerosis 132 (2), 229–236. doi:10.1016/s0021-9150(97)00093-2
Harinasuta, C. (1984). Epidemiology and Control of Schistosomiasis in Southeast Asia. Southeast. Asian J. Trop. Med. Public Health 15 (4), 431–438.
Hedrick, P. W. (2012). Resistance to Malaria in Humans: the Impact of strong, Recent Selection. Malar. J. 11, 349. doi:10.1186/1475-2875-11-349
Hildebrand, R. B., Lammers, B., Meurs, I., Korporaal, S. J. A., De Haan, W., Zhao, Y., et al. (2010). Restoration of High-Density Lipoprotein Levels by Cholesteryl Ester Transfer Protein Expression in Scavenger Receptor Class B Type I (SR-BI) Knockout Mice Does Not Normalize Pathologies Associated with SR-BI Deficiency. Atvb 30 (7), 1439–1445. doi:10.1161/atvbaha.110.205153
Hill, S. A., Nazir, D. J., Jayaratne, P., Bamford, K. S., and McQueen, M. J. (1997). Mutations in Cholesteryl Ester Transfer Protein and Hepatic Lipase in a North American Population. Clin. Biochem. 30 (5), 413–418. doi:10.1016/s0009-9120(97)00009-x
Hirano, K.-i., Matsuzawa, Y., Sakai, N., Hiraoka, H., Nozaki, S., Funahashi, T., et al. (1992). Polydisperse Low-Density Lipoproteins in Hyperalphalipoproteinemic Chronic Alcohol Drinkers in Association with Marked Reduction of Cholesteryl Ester Transfer Protein Activity. Metabolism 41 (12), 1313–1318. doi:10.1016/0026-0495(92)90101-f
Hirano, K.-i., Yamashita, S., Nakajima, N., Arai, T., Maruyama, T., Yoshida, Y., et al. (1997). Genetic Cholesteryl Ester Transfer Protein Deficiency Is Extremely Frequent in the Omagari Area of Japan. Atvb 17 (6), 1053–1059. doi:10.1161/01.atv.17.6.1053
Hirata, M., Takushima, M., Kage, M., and Fukuma, T. (1991). Induction of Experimental Murine Granuloma Formation againstSchistosoma Japonicum Eggs Produced by In Vitro Ova Deposition, In Vitro Tissue Extraction, or Lyophilization. Parasitol. Res. 77 (4), 315–319. doi:10.1007/bf00930907
Hoekstra, M., Van Eck, M., and Korporaal, S. J. A. (2012). Genetic Studies in Mice and Humans Reveal New Physiological Roles for the High-Density Lipoprotein Receptor Scavenger Receptor Class B Type I. Curr. Opin. Lipidol. 23 (2), 127–132. doi:10.1097/MOL.0b013e3283508c09
Hsieh, P., Vollger, M. R., Dang, V., Porubsky, D., Baker, C., Cantsilieris, S., et al. (2019). Adaptive Archaic Introgression of Copy Number Variants and the Discovery of Previously Unknown Human Genes. Science 366 (6463). doi:10.1126/science.aax2083
Hsu, L.-A., Ko, Y.-L., Hsu, K.-H., Ko, Y.-H., and Lee, Y.-S. (2002). Genetic Variations in the Cholesteryl Ester Transfer Protein Gene and High Density Lipoprotein Cholesterol Levels in Taiwanese Chinese. Hum. Genet. 110 (1), 57–63. doi:10.1007/s00439-001-0640-z
Hui, S. P. (1997). Frequency and Effect on Plasma Lipoprotein Metabolism of a Mutation in the Cholesteryl Ester Transfer Protein Gene in the Chinese. Hokkaido Igaku Zasshi 72 (3), 319–327.
Iida, F., Iida, R., Kamijo, H., Takaso, K., Miyazaki, Y., Funabashi, W., et al. (1999). Chronic Japanese Schistosomiasis and Hepatocellular Carcinoma: Ten Years of Follow-Up in Yamanashi Prefecture, Japan. Bull. World Health Organ. 77 (7), 573–581.
Imachi, H., Murao, K., Sato, M., Hosokawa, H., Ishida, T., and Takahara, J. (1999). CD36 LIMPII Analogous-1, a Human Homolog of the Rodent Scavenger Receptor B1, Provides the Cholesterol Ester for Steroidogenesis in Adrenocortical Cells. Metabolism 48 (5), 627–630. doi:10.1016/s0026-0495(99)90061-6
Imachi, H., Murao, K., Sayo, Y., Hosokawa, H., Sato, M., Niimi, M., et al. (1999). Evidence for a Potential Role for HDL as an Important Source of Cholesterol in Human Adrenocortical Tumors via the CLA-1 Pathway. Endocr. J. 46 (1), 27–34. doi:10.1507/endocrj.46.27
Inazu, A., Brown, M. L., Hesler, C. B., Agellon, L. B., Koizumi, J., Takata, K., et al. (1990). Increased High-Density Lipoprotein Levels Caused by a Common Cholesteryl-Ester Transfer Protein Gene Mutation. N. Engl. J. Med. 323 (18), 1234–1238. doi:10.1056/nejm199011013231803
Inazu, A., Jiang, X. C., Haraki, T., Yagi, K., Kamon, N., Koizumi, J., et al. (1994). Genetic Cholesteryl Ester Transfer Protein Deficiency Caused by Two Prevalent Mutations as a Major Determinant of Increased Levels of High Density Lipoprotein Cholesterol. J. Clin. Invest. 94 (5), 1872–1882. doi:10.1172/jci117537
Inazu, A., Nakajima, K., Nakano, T., Niimi, M., Kawashiri, M.-a., Nohara, A., et al. (2008). Decreased post-prandial Triglyceride Response and Diminished Remnant Lipoprotein Formation in Cholesteryl Ester Transfer Protein (CETP) Deficiency. Atherosclerosis 196 (2), 953–957. doi:10.1016/j.atherosclerosis.2007.02.028
Jap, T.-S., Wu, Y.-C., Tso, Y.-C., and Chiu, C.-Y. (2002). A Novel Mutation in the Intron 1 Splice Donor Site of the Cholesterol Ester Transfer Protein (CETP) Gene as a Cause of Hyperalphalipoproteinemia. Metabolism 51 (3), 394–397. doi:10.1053/meta.2002.30527
Jiang, X.-C., Bruce, C., Cocke, T., Wang, S., Boguski, M., and Tall, A. R. (1995). Point Mutagenesis of Positively Charged Amino Acids of Cholesteryl Ester Transfer Protein: Conserved Residues within the Lipid Transfer/lipopolysaccharide Binding Protein Gene Family Essential for Function. Biochemistry 34 (21), 7258–7263. doi:10.1021/bi00021a042
Khoo, J. C., Pittman, R. C., and Rubin, E. M. (1995). Selective Uptake of HDL Cholesteryl Esters Is Active in Transgenic Mice Expressing Human Apolipoprotein A-I. J. Lipid Res. 36 (3), 593–600. doi:10.1016/s0022-2275(20)39893-x
Kimura, H., Gejyo, F., Suzuki, S., Yamaguchi, T., Imura, T., Miyazaki, R., et al. (1999). A Common Mutation of Cholesteryl Ester Transfer Protein Gene in Dialysis Patients. Kidney Int. 56, S186–S189. doi:10.1046/j.1523-1755.1999.07148.x
Ko, K. W., Ohnishi, T., and Yokoyama, S. (1994). Triglyceride Transfer Is Required for Net Cholesteryl Ester Transfer between Lipoproteins in Plasma by Lipid Transfer Protein. Evidence for a Hetero-Exchange Transfer Mechanism Demonstrated by Using Novel Monoclonal Antibodies. J. Biol. Chem. 269 (45), 28206–28213. doi:10.1016/s0021-9258(18)46915-3
Koizumi, J., Mabuchi, H., Yoshimura, A., Michishita, I., Takeda, M., Itoh, H., et al. (1985). Deficiency of Serum Cholesteryl-Ester Transfer Activity in Patients with Familial Hyperalphalipoproteinaemia. Atherosclerosis 58 (1-3), 175–186. doi:10.1016/0021-9150(85)90064-4
Kurasawa, T., Yokoyama, S., Miyake, Y., Yamamura, T., and Yamamoto, A. (1985). Rate of Cholesteryl Ester Transfer between High and Low Density Lipoproteins in Human Serum and a Case with Decreased Transfer Rate in Association with Hyperalphalipoproteinemia1. J. Biochem. 98 (6), 1499–1508. doi:10.1093/oxfordjournals.jbchem.a135418
Lafon, M. (2005). Rabies Virus Receptors. J. NeuroVirology 11 (1), 82–87. doi:10.1080/13550280590900427
Ma, Y.-Q., Thomas, G. N., Critchley, J. A., Chan, J. C., Lee, Z. S., and Tomlinson, B. (2001). Identification of the Intron 14 Splicing Defect of the Cholesteryl Ester Transfer Protein Gene in Hong Kong Chinese. Clin. Genet. 59 (4), 287–289. doi:10.1034/j.1399-0004.2001.590413.x
Maruyama, T., Yamashita, S., Matsuzawa, Y., Bujo, H., Takahashi, K., Saito, Y., et al. (2004). Mutations in Japanese Subjects with Primary Hyperlipidemia— Results from the Research Committee of the Ministry of Health and Welfare of Japan since 1996 —. Jat 11 (3), 131–145. doi:10.5551/jat.11.131
McManus, D. P., Dunne, D. W., Sacko, M., Utzinger, J., Vennervald, B. J., and Zhou, X.-N. (2018). Schistosomiasis. Nat. Rev. Dis. Primers 4 (1), 13. doi:10.1038/s41572-018-0013-8
McManus, D. P., Gray, D. J., Li, Y., Feng, Z., Williams, G. M., Stewart, D., et al. (2010). Schistosomiasis in the People's Republic of China: the Era of the Three Gorges Dam. Clin. Microbiol. Rev. 23 (2), 442–466. doi:10.1128/cmr.00044-09
Mitchell, G. F., Garcia, E. G., Rivera, P. T., Tiu, W. U., and Davern, K. M. (1994). Evidence for and Implications of Anti-embryonation Immunity in Schistosomiasis. Exp. Parasitol. 79 (4), 546–549. doi:10.1006/expr.1994.1116
Mohda, J., Redman, C. A., Thormhill, J. A., and Kusel, J. R. (1998). Schstosomes: Unanswered Questions on the Basic Biology of the Host-Parasite Relationship. Prasitol. Today 14, 396–401. doi:10.1016/s0169-4758(98)01321-0
Moriyama, Y., Okamura, T., Inazu, A., Doi, M., Iso, H., Mouri, Y., et al. (1998). A Low Prevalence of Coronary Heart Disease Among Subjects with Increased High-Density Lipoprotein Cholesterol Levels, Including Those with Plasma Cholesteryl Ester Transfer Protein Deficiency. Prev. Med. 27 (5 Pt 1), 659–667. doi:10.1006/pmed.1998.0340
Muanprasat, C., and Chatsudthipong, V. (2013). Cholera: Pathophysiology and Emerging Therapeutic Targets. Future Med. Chem. 5 (7), 781–798. doi:10.4155/fmc.13.42
Nagano, M., Yamashita, S., Hirano, K.-i., Ito, M., Maruyama, T., Ishihara, M., et al. (2002). Two Novel Missense Mutations in the CETP Gene in Japanese Hyperalphalipoproteinemic Subjects: High-Throughput Assay by Invader Assay. J. Lipid Res. 43 (7), 1011–1018. doi:10.1194/jlr.m200024-jlr200
Nagano, M., Yamashita, S., Hirano, K.-i., Takano, M., Maruyama, T., Ishihara, M., et al. (2004). Molecular Mechanisms of Cholesteryl Ester Transfer Protein Deficiency in Japanese. Jat 11 (3), 110–121. doi:10.5551/jat.11.110
Nagao, M., Nakajima, H., Toh, R., Hirata, K.-i., and Ishida, T. (2018). Cardioprotective Effects of High-Density Lipoprotein beyond its Anti-atherogenic Action. Jat 25 (10), 985–993. doi:10.5551/jat.RV17025
Nihei, N., Kajihara, N., Kirinoki, M., Chigusa, Y., Matsuda, H., Saitoh, Y., et al. (2006). Establishment of a GIS Monitoring System for Schistosomiasis Japonica in Kofu, Japan. Ann. Trop. Med. Parasitol. 100 (2), 143–153. doi:10.1179/136485906x86293
Nissen, S. E., Tardif, J.-C., Nicholls, S. J., Revkin, J. H., Shear, C. L., Duggan, W. T., et al. (2007). Effect of Torcetrapib on the Progression of Coronary Atherosclerosis. N. Engl. J. Med. 356 (13), 1304–1316. doi:10.1056/NEJMoa070635
Ohmae, H., Sy, O. S., Chigusa, Y., and Portillo, G. P. (2003). Imaging Diagnosis of Schistosomiasis Japonica-The Use in Japan and Application for Field Study in the Present Endemic Area. Parasitol. Int. 52 (4), 385–393. doi:10.1016/s1383-5769(03)00055-2
Ohnishi, T., Hicks, L. D., Oikawa, K., Kay, C. M., and Yokoyama, S. (1994). Properties of Human Plasma Lipid Transfer Protein in Aqueous Solution and at Interfaces. Biochemistry 33 (20), 6093–6099. doi:10.1021/bi00186a008
Ohnishi, T., Tan, C., and Yokoyama, S. (1994). Selective Transfer of Cholesteryl Ester over Triglyceride by Human Plasma Lipid Transfer Protein between Apolipoprotein-Activated Lipid Microemulsions. Biochemistry 33, 4533–4542. doi:10.1021/bi00181a014
Okumura-Noji, K., Miura, Y., Lu, R., Asai, K., Ohta, N., Brindley, P. J., et al. (2013). CD36‐related Protein inSchistosoma Japonicum: Candidate Mediator of Selective Cholesteryl Ester Uptake from High‐density Lipoprotein for Egg Maturation. FASEB j. 27 (3), 1236–1244. doi:10.1096/fj.12-219816
Okumura-Noji, K., Sasai, K., Zhan, R., Kawaguchi, H., Maruyama, H., Tada, T., et al. (2001). Cholesteryl Ester Transfer Protein Deficiency Causes Slow Egg Embryonation of Schistosoma Japonicum. Biochem. Biophysical Res. Commun. 286 (2), 305–310. doi:10.1006/bbrc.2001.5386
Plengpanich, W., Siriwong, S., and Khovidhunkit, W. (2009). Two Novel Mutations and Functional Analyses of the CETP and LIPC Genes Underlying Severe Hyperalphalipoproteinemia. Metabolism 58 (8), 1178–1184. doi:10.1016/j.metabol.2009.03.020
Pollard, R. D., Blesso, C. N., Zabalawi, M., Fulp, B., Gerelus, M., Zhu, X., et al. (2015). Procollagen C-Endopeptidase Enhancer Protein 2 (PCPE2) Reduces Atherosclerosis in Mice by Enhancing Scavenger Receptor Class B1 (SR-BI)-mediated High-Density Lipoprotein (HDL)-Cholesteryl Ester Uptake. J. Biol. Chem. 290 (25), 15496–15511. doi:10.1074/jbc.M115.646240
Qian, C., Zhang, Y., Zhang, X., Yuan, C., Gao, Z., Yuan, H., et al. (2018). Effectiveness of the New Integrated Strategy to Control the Transmission of Schistosoma Japonicum in China: a Systematic Review and Meta-Analysis. Parasite 25, 54. doi:10.1051/parasite/2018058
Qiu, X., Mistry, A., Ammirati, M. J., Chrunyk, B. A., Clark, R. W., Cong, Y., et al. (2007). Crystal Structure of Cholesteryl Ester Transfer Protein Reveals a Long Tunnel and Four Bound Lipid Molecules. Nat. Struct. Mol. Biol. 14 (2), 106–113. doi:10.1038/nsmb1197
Rhyne, J., Ryan, M. J., White, C., Chimonas, T., and Miller, M. (2006). The Two Novel CETP Mutations Gln87X and Gln165X in a Compound Heterozygous State Are Associated with Marked Hyperalphalipoproteinemia and Absence of Significant Coronary Artery Disease. J. Mol. Med. 84 (8), 647–650. doi:10.1007/s00109-006-0070-4
Rinninger, F., and Pittman, R. C. (1987). Regulation of the Selective Uptake of High Density Lipoprotein-Associated Cholesteryl Esters. J. Lipid Res. 28 (11), 1313–1325. doi:10.1016/s0022-2275(20)38596-5
Ritsch, A., Drexel, H., Amann, F. W., Pfeifhofer, C., and Patsch, J. R. (1997). Deficiency of Cholesteryl Ester Transfer Protein. Atvb 17 (12), 3433–3441. doi:10.1161/01.atv.17.12.3433
Rogers, M. V., Henkle, K. J., Fidge, N. H., and Mitchell, G. F. (1989). Identification of a Multispecific Lipoprotein Receptor in Adult Schistosoma Japonicum by Ligand Blotting Analyses. Mol. Biochem. Parasitol. 35 (1), 79–88. doi:10.1016/0166-6851(89)90145-x
Rogers, M. V., Quilici, D., Mitchell, G. F., and Fidge, N. H. (1990). Purification of a Putative Lipoprotein Receptor from Schistosoma Japonicum Adult Worms. Mol. Biochem. Parasitol. 41 (1), 93–100. doi:10.1016/0166-6851(90)90100-z
Rumjanek, F. D., Campos, E. G., and Luis Carlos, C. A. (1988). Evidence for the Occurrence of LDL Receptors in Extracts of Schistosomula of Schistosoma Mansoni. Mol. Biochem. Parasitol. 28 (2), 145–152. doi:10.1016/0166-6851(88)90062-x
Rumjanek, F. D., McLaren, D. J., and Smithers, S. R. (1983). Serum-induced Expression of a Surface Protein in Schistosomula of Schistosoma Mansoni: a Possible Receptor for Lipid Uptake. Mol. Biochem. Parasitol. 9 (4), 337–350. doi:10.1016/0166-6851(83)90090-7
Rumjanek, F., Vidigalsilveira, M. A., and Silveira, A. M. (1985). The Interaction of Human Serum with the Surface Membrane of Schistosomula of Schistosoma Mansoni. Mol. Biochem. Parasitol. 14 (1), 63–73. doi:10.1016/0166-6851(85)90106-9
Sadat, M. A., Moir, S., Chun, T.-W., Lusso, P., Kaplan, G., Wolfe, L., et al. (2014). Glycosylation, Hypogammaglobulinemia, and Resistance to Viral Infections. N. Engl. J. Med. 370 (17), 1615–1625. doi:10.1056/NEJMoa1302846
Sakai, N., Matsuzawa, Y., Hirano, K., Yamashita, S., Nozaki, S., Ueyama, Y., et al. (1991). Detection of Two Species of Low Density Lipoprotein Particles in Cholesteryl Ester Transfer Protein Deficiency. Arterioscler Thromb. 11 (1), 71–79. doi:10.1161/01.atv.11.1.71
Sakai, N., Yamashita, S., Hirano, K.-i., Menju, M., Arai, T., Kobayashi, K., et al. (1995). Frequency of Exon 15 Missense Mutation (442D:G) in Cholesteryl Ester Transfer Protein Gene in Hyperalphalipoproteinemic Japanese Subjects. Atherosclerosis 114 (2), 139–145. doi:10.1016/0021-9150(94)05477-z
Sandhu, J., Li, S., Fairall, L., Pfisterer, S. G., Gurnett, J. E., Xiao, X., et al. (2018). Aster Proteins Facilitate Nonvesicular Plasma Membrane to ER Cholesterol Transport in Mammalian Cells. Cell 175 (2), 514–529. doi:10.1016/j.cell.2018.08.033
Schwartz, G. G., Olsson, A. G., Abt, M., Ballantyne, C. M., Barter, P. J., Brumm, J., et al. (2012). Effects of Dalcetrapib in Patients with a Recent Acute Coronary Syndrome. N. Engl. J. Med. 367 (22), 2089–2099. doi:10.1056/NEJMoa1206797
Shen, W.-J., Asthana, S., Kraemer, F. B., and Azhar, S. (2018). Thematic Review Series: Lipid Transfer Proteins Scavenger Receptor B Type 1: Expression, Molecular Regulation, and Cholesterol Transport Function. J. Lipid Res. 59 (7), 1114–1131. doi:10.1194/jlr.R083121
Skelly, P. J., Da'dara, A. A., Li, X.-H., Castro-Borges, W., and Wilson, R. A. (2014). Schistosome Feeding and Regurgitation. Plos Pathog. 10 (8), e1004246. doi:10.1371/journal.ppat.1004246
Smith, T. M., Brooks, T. J., and Lockard, V. G. (1970). In Vitro studies on Cholesterol Metabolism in the Blood flukeSchistosoma Mansoni. Lipids 5 (10), 854–856. doi:10.1007/bf02531979
Song, G. J., Han, G. H., Chae, J. J., Namkoong, Y., Lee, H. K., Park, Y. B., et al. (1997). The Effects of the Cholesterol Ester Transfer Protein Gene and Environmental Factors on the Plasma High Density Lipoprotein Cholesterol Levels in the Korean Population. Mol. Cell 7 (5), 615–619.
Sparrow, C. P., and Pittman, R. C. (1990). Cholesterol Esters Selectively Taken up from High-Density Lipoproteins Are Hydrolyzed Extralysosomally. Biochim. Biophys. Acta (Bba) - Lipids Lipid Metab. 1043 (2), 203–210. doi:10.1016/0005-2760(90)90297-b
Spyropoulos, B., Moens, P. B., Davidson, J., and Lowden, J. A. (1981). Heterozygote Advantage in Tay-Sachs Carriers? Am. J. Hum. Genet. 33 (3), 375–380.
Takahashi, H., Takahashi, A., Maki, M., Sasai, H., and Kamada, M. (2001). Effect of CETP on the Plasma Lipoprotein Profile in Four Strains of Transgenic Mouse. Biochem. Biophysical Res. Commun. 283 (1), 118–123. doi:10.1006/bbrc.2001.4743
Tall, A. (1995). Plasma Lipid Transfer Proteins. Annu. Rev. Biochem. 64, 235–257. doi:10.1146/annurev.bi.64.070195.001315
Taylor, S. M., Parobek, C. M., and Fairhurst, R. M. (2012). Haemoglobinopathies and the Clinical Epidemiology of Malaria: a Systematic Review and Meta-Analysis. Lancet Infect. Dis. 12 (6), 457–468. doi:10.1016/s1473-3099(12)70055-5
Teh, E. M., Dolphin, P. J., Breckenridge, W. C., and Tan, M.-H. (1998). Human Plasma CETP Deficiency: Identification of a Novel Mutation in Exon 9 of the CETP Gene in a Caucasian Subject from North America. J. Lipid Res. 39, 442–456. doi:10.1016/s0022-2275(20)33905-5
Tempone, A. J., Bianconi, M. L., and Rumjanek, F. D. (1997). The Interaction of Human LDL with the Tegument of Adult Schistosoma Mansoni. Mol. Cel. Biochem. 177 (1-2), 139–144. doi:10.1023/a:1006801216344
Thompson, A., Di Angelantonio, E., Sarwar, N., Erqou, S., Saleheen, D., Dullaart, R. P., et al. (2008). Association of Cholesteryl Ester Transfer Protein Genotypes with CETP Mass and Activity, Lipid Levels, and Coronary Risk. JAMA 299 (23), 2777–2788. doi:10.1001/jama.299.23.2777
Thompson, J. F., Reynolds, J. M., Williams, S. P., Wood, L. S., Paciga, S. A., and Lloyd, D. B. (2009). Frequency and Function of CETP Variants Among Individuals of Asian Ancestry. Atherosclerosis 202 (1), 241–247. doi:10.1016/j.atherosclerosis.2008.03.013
Thu, N. N., Mai, T. T. T., Ohmori, R., Kuroki, M., Van Chuyen, N., Hung, N. T. K., et al. (2005). Effect of the Cholesteryl Ester Transfer Protein Genotypes on Plasma Lipid and Lipoprotein Levels in Vietnamese Children. Pediatr. Res. 58 (6), 1249–1253. doi:10.1203/01.pdr.0000183782.57705.fc
Torrington, M., Botha, J. L., Pilcher, G. J., and Baker, S. G. (1984). Association between Familial Hypercholesterolaemia and Church Affiliation. Is This the Result of Sociocultural Isolation of Migrant Farmers in 19th-century South Africa? S Afr. Med. J. 65 (19), 762–767.
Van Berkel, T. J. C., Van Eck, M., Herijgers, N., Fluiter, K., and Nion, S. (2000). Scavenger Receptor Classes A and B: Their Roles in Atherogenesis and the Metabolism of Modified LDL and HDL. Ann. N. Y Acad. Sci. 902, 113–127. doi:10.1111/j.1749-6632.2000.tb06306.x
van der Steeg, W. A., Hovingh, G. K., Klerkx, A. H. E. M., Hutten, B. A., Nootenboom, I. C., Levels, J. H. M., et al. (2007). Cholesteryl Ester Transfer Protein and Hyperalphalipoproteinemia in Caucasians. J. Lipid Res. 48 (3), 674–682. doi:10.1194/jlr.M600405-JLR200
Wang, L.-D., Chen, H.-G., Guo, J.-G., Zeng, X.-J., Hong, X.-L., Xiong, J.-J., et al. (2009). A Strategy to Control Transmission ofSchistosoma Japonicumin China. N. Engl. J. Med. 360 (2), 121–128. doi:10.1056/NEJMoa0800135
Wang, S., Kussie, P., Deng, L., and Tall, A. (1995). Defective Binding of Neutral Lipids by a Carboxyl-Terminal Deletion Mutant of Cholesteryl Ester Transfer Protein. J. Biol. Chem. 270 (2), 612–618. doi:10.1074/jbc.270.2.612
Wang, X.-Y., Xu, J., Zhao, S., Li, W., Zhang, J.-F., He, J., et al. (2018). Estimating the Prevalence of Schistosomiasis Japonica in China: a Serological Approach. Infect. Dis. Poverty 7 (1), 62. doi:10.1186/s40249-018-0443-2
Withrock, I. C., Anderson, S. J., Jefferson, M. A., McCormack, G. R., Mlynarczyk, G. S. A., Nakama, A., et al. (2015). Genetic Diseases Conferring Resistance to Infectious Diseases. Genes Dis. 2 (3), 247–254. doi:10.1016/j.gendis.2015.02.008
Woolf, L. I., McBean, M. S., Woolf, F. M., and Cahalanf, S. F. (1975). Phenylketonuria as a Balanced Polymorphism: the Nature of the Heterozygote Advantage. Ann. Hum. Genet 38 (4), 461–469. doi:10.1111/j.1469-1809.1975.tb00635.x
Wu, J. H., Lee, Y.-T., Hsu, H.-C., and Hsieh, L.-L. (2001). Influence of CETP Gene Variation on Plasma Lipid Levels and Coronary Heart Disease: a Survey in Taiwan. Atherosclerosis 159 (2), 451–458. doi:10.1016/s0021-9150(01)00524-x
Xia, Y., Baum, L., Pang, C. P., Siest, G., and Visvikis, S. (2005). Cardiovascular Risk-Associated Allele Frequencies for 15 Genes in Healthy Elderly French and Chinese. Clin. Chem. Lab. Med. 43 (8), 817–822. doi:10.1515/cclm.2005.137
Xu, X., and Caulfield, J. P. (1992). Characterization of Human Low Density Lipoprotein Binding Proteins on the Surface of Schistosomula of Schistosoma Mansoni. Eur. J. Cell Biol. 57 (2), 229–235.
Xue, J.-B., Wang, X.-Y., Zhang, L.-J., Hao, Y.-W., Chen, Z., Lin, D.-D., et al. (2021). Potential Impact of Flooding on Schistosomiasis in Poyang Lake Regions Based on Multi-Source Remote Sensing Images. Parasites Vectors 14 (1), 116. doi:10.1186/s13071-021-04576-x
Yamamoto, T., Davis, C. G., Brown, M. S., Schneider, W. J., Casey, M. L., Goldstein, J. L., et al. (1984). The Human LDL Receptor: a Cysteine-Rich Protein with Multiple Alu Sequences in its mRNA. Cell 39 (1), 27–38. doi:10.1016/0092-8674(84)90188-0
Yamashita, S., Hirano, K., Sakai, N., and Matsuzawa, Y. (2000). Molecular Biology and Pathophysiological Aspects of Plasma Cholesteryl Ester Transfer Protein. Biochim. Biophys. Acta 1529 (1-3), 257–275. doi:10.1016/s1388-1981(00)00164-5
Yamashita, S., Matsuzawa, Y., Okazaki, M., Kako, H., Yasugi, T., Akioka, H., et al. (1988). Small Polydisperse Low Density Lipoproteins in Familial Hyperalphalipoproteinemia with Complete Deficiency of Cholesteryl Ester Transfer Activity. Atherosclerosis 70 (1-2), 7–12. doi:10.1016/0021-9150(88)90094-9
Yamashita, S., Hui, D. Y., Wetterau, J. R., Sprecher, D. L., Harmony, J. A. K., Sakai, N., et al. (1991). Characterization of Plasma Lipoproteins in Patients Heterozygous for Human Plasma Cholesteryl Ester Transfer Protein (CETP) Deficiency: Plasma CETP Regulates High-Density Lipoprotein Concentration and Composition. Metabolism 40 (7), 756–763. doi:10.1016/0026-0495(91)90097-g
Yamashita, S., Sprecher, D. L., Sakai, N., Matsuzawa, Y., Tarui, S., and Hui, D. Y. (1990). Accumulation of Apolipoprotein E-Rich High Density Lipoproteins in Hyperalphalipoproteinemic Human Subjects with Plasma Cholesteryl Ester Transfer Protein Deficiency. J. Clin. Invest. 86 (3), 688–695. doi:10.1172/jci114764
Yang, G.-J., Gemperli, A., Utzinger, J., Zhou, X.-N., Tanner, P. M., and Utzinger, J. (2006). A Growing Degree-Days Based Time-Series Analysis for Prediction of Schistosoma Japonicum Transmission in Jiangsu Province, China. Am. J. Trop. Med. Hyg. 75 (3), 549–555. doi:10.4269/ajtmh.2006.75.549
Yang, Y., Zheng, S.-B., Yang, Y., Cheng, W.-T., Pan, X., Dai, Q.-Q., et al. (2018). The Three Gorges Dam: Does the Flooding Time Determine the Distribution of Schistosome-Transmitting Snails in the Middle and Lower Reaches of the Yangtze River, China? Ijerph 15 (7), 1304. doi:10.3390/ijerph15071304
Yokoyama, S. (2014). A Potential Screening Factor for Accumulation of Cholesteyl Ester Transfer Protein Deficiency in East Asia: Schistosoma Japonicum. Biochim. Biophys. Acta (Bba) - Mol. Cell Biol. Lipids 1841 (4), 495–504. doi:10.1016/j.bbalip.2013.12.014
Yokoyama, S., Okumura-Noji, K., and Lu, R. (2015). Prevention of Fatal Hepatic Complication in Schistosomiasis by Inhibition of CETP. J. Biomed. Res. 29 (3), 176–188. doi:10.7555/jbr.29.20150005
Yokoyama, S. (2015). Unique Features of High-Density Lipoproteins in the Japanese: in Population and in Genetic Factors. Nutrients 7 (4), 2359–2381. doi:10.3390/nu7042359
Zeberg, H., and Pääbo, S. (2021). A Genomic Region Associated with protection against Severe COVID-19 Is Inherited from Neandertals. Proc. Natl. Acad. Sci. USA 118 (9), e2026309118. doi:10.1073/pnas.2026309118
Zeberg, H., and Pääbo, S. (2020). The Major Genetic Risk Factor for Severe COVID-19 Is Inherited from Neanderthals. Nature 587 (7835), 610–612. doi:10.1038/s41586-020-2818-3
Zhang, M., Hong, Y., Han, Y., Han, H., Peng, J., Qiu, C., et al. (2013). Proteomic Analysis of Tegument-Exposed Proteins of Female and Male Schistosoma Japonicum Worms. J. Proteome Res. 12 (11), 5260–5270. doi:10.1021/pr400476a
Zhang, R., Yoshida, A., Kumagai, T., Kawaguchi, H., Maruyama, H., Suzuki, T., et al. (2001). Vaccination with Calpain Induces a Th1-Biased Protective Immune Response against Schistosoma Japonicum. Infect. Immun. 69 (1), 386–391. doi:10.1128/iai.69.1.386-391.2001
Zheng, K.-Q., Zhang, S.-Z., Zhang, L., Huang, D.-J., Liao, L.-C., and Hou, Y.-P. (2004). A Novel Missense Mutation (L296Q) in Cholesteryl Ester Transfer Protein Gene Related to Coronary Heart Disease. Acta Biochim. Biophys. Sin (Shanghai) 36 (1), 33–36. doi:10.1093/abbs/36.1.33
Zhong, S., Sharp, D. S., Grove, J. S., Bruce, C., Yano, K., Curb, J. D., et al. (1996). Increased Coronary Heart Disease in Japanese-American Men with Mutation in the Cholesteryl Ester Transfer Protein Gene Despite Increased HDL Levels. J. Clin. Invest. 97 (12), 2917–2923. doi:10.1172/jci118751
Keywords: Schistosoma japonicum, HDL, egg embryonation, CD36, cholesteryl ester, CETP, East Asia, hepatic granulomatosis
Citation: Yokoyama S (2022) HDL Receptor in Schistosoma japonicum Mediating Egg Embryonation: Potential Molecular Basis for High Prevalence of Cholesteryl Ester Transfer Protein Deficiency in East Asia. Front. Cell Dev. Biol. 10:807289. doi: 10.3389/fcell.2022.807289
Received: 02 November 2021; Accepted: 21 February 2022;
Published: 17 March 2022.
Edited by:
Paul J. Brindley, George Washington University, United StatesReviewed by:
Akihiro Inazu, Kanazawa University, JapanVictoria Mann, George Washington University, United States
Copyright © 2022 Yokoyama. This is an open-access article distributed under the terms of the Creative Commons Attribution License (CC BY). The use, distribution or reproduction in other forums is permitted, provided the original author(s) and the copyright owner(s) are credited and that the original publication in this journal is cited, in accordance with accepted academic practice. No use, distribution or reproduction is permitted which does not comply with these terms.
*Correspondence: Shinji Yokoyama, c3lva295YW1AaXNjLmNodWJ1LmFjLmpw