- 1Longgang District People´s Hospital of Shenzhen, The Second Affiliated Hospital of The Chinese University of Hong Kong, Shenzhen, China
- 2School of Basic Medical Sciences, Anhui Medical University, Hefei, China
Tumor-derived exosomes (TDEs) are actively produced and released by tumor cells and carry messages from tumor cells to healthy cells or abnormal cells, and they participate in tumor metastasis. In this review, we explore the underlying mechanism of action of TDEs in tumor metastasis. TDEs transport tumor-derived proteins and non-coding RNA to tumor cells and promote migration. Transport to normal cells, such as vascular endothelial cells and immune cells, promotes angiogenesis, inhibits immune cell activation, and improves chances of tumor implantation. Thus, TDEs contribute to tumor metastasis. We summarize the function of TDEs and their components in tumor metastasis and illuminate shortcomings for advancing research on TDEs in tumor metastasis.
Background
Exosomes are extracellular vesicles, approximately 30–150 nm in diameter, that contain functional biomolecules, such as proteins, RNA, DNA, and lipids, and can interact with recipient cells (Balaj et al., 2011; Choi et al., 2013; Peinado et al., 2011; Raposo and Stoorvogel, 2013; Skog et al., 2008; Thakur et al., 2014; Thery et al., 2009; Valadi et al., 2007). Exosomes are present in various body fluids and are regarded as a key component of intercellular communication. Tumor cell-, stromal cell-, or even normal cell–derived exosomes play an important role in tumor progression and can induce angiogenesis and accelerate metastasis (Hood et al., 2011; Luga et al., 2012; Peinado et al., 2012). The components and functions of the exosomes depend on the cell types; some studies have shown many differences in the contents and release rate in different types of cells. But, the complete mechanism and process have not yet been elucidated and need to be further explored. Metastasis is the leading cause of tumor-induced death and is a complex process involving local invasion, survival, and evasion from immunosurveillance, invasion into circulation, and extravasation at secondary organs (Fidler and Kripke, 2015; Wan et al., 2013). Tumor-derived exosomes (TDEs) are a significant component of the tumor microenvironment and are involved in promoting tumor metastasis through several mechanisms, including acquiring primary tumor migration capacity, tumor angiogenesis, escaping immune system organotropic metastasis, forming the pre-metastatic niche, and metastatic tumor growth in the secondary site.
In this review, we summarize the function of exosomes in every aspect of cancer metastasis (Figure 1) to provide a better systematic comprehension of the role of exosomes in tumor metastasis and propose practical implications of early diagnosis, treatment, and prognostic methods for cancer.
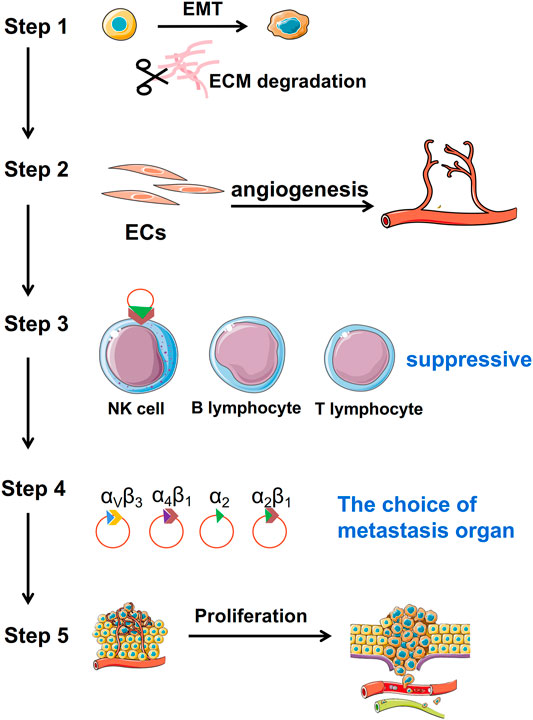
FIGURE 1. Function of TDEs in tumor metastasis. TDEs are mainly involved in tumor metastasis through five aspects. Step 1: acquisition of tumor migration ability; Step 2: angiogenesis; Step 3: immunosuppression; Step 4: localization of metastatic sites; and Step 5: enhancement of proliferation ability of tumor cells after migration.
Tumor-Derived Exosomes Enhance the Migration Ability of Tumor Cells
Tumor-Derived Exosomes Promote Epithelial–Mesenchymal Transition to Initiate Metastasis
Epithelial–mesenchymal transition (EMT) frequently initiates the metastatic process (Li et al., 2021). Epithelial tumor cells acquire mesenchymal characteristics under the influence of cancer-associated fibroblasts (CAFs) in the tumor stroma (Diepenbruck and Christofori, 2016). Epithelial markers, including E-cadherin, zona occludens 1 (ZO-1), cytokeratins, desmoplakin, and laminin, are downregulated, and mesenchymal markers, including N-cadherin, β-catenin, and vimentin, are upregulated (Sommers et al., 1994; Li Y. et al., 2019). During EMT, tumor cells lose their apical–basal polarity, basement anchoring, and cell–cell junctions and switch to a low proliferation state with enhanced migratory and invasion capabilities (Basil et al., 2020). Once the tumor cells reach a distant pre-metastatic niche, the reversed process takes place (Maren, 2016). This so-called mesenchymal–epithelial transition (MET) returns tumor cells to a high proliferative state and enables the formation of micrometastases (Bakir et al., 2019). TDEs play an important regulatory role in mediating the EMT and MET transformation (Bigagli et al., 2019). There has been increasing research showing the signaling pathway involved in inducing cancer-related EMT. We propose that the critical components in TDEs can serve to promote EMT.
The latest hypothesis is TDEs may be conduits for initiating signals for EMT. For example, TDEs carry EMT derivers, such as transforming growth factor-beta (TGF-β), tumor necrosis factor-alpha (TNF-α), hypoxia-inducible factor 1 alpha (HIF-1α), protein kinase B (AKT), caveolin-1, platelet-derived growth factors (PDGFs), and β-catenin Wnt pathway modulators, that directly enhance the process of EMT (Aga et al., 2014; Kucharzewska et al., 2013; Luga et al., 2012; Ramteke et al., 2015). TDEs transmit non-coding RNA, such as, miR-128-3p, miR-27, LINC00960, linc02470, circ-PVT1, and circ-CPA4, that upregulate EMT (Huang C.-S. et al., 2020; Liu et al., 2019; Wang J. et al., 2018). Therefore, many studies have shown that tumor cells can secrete exosomes into the extracellular space and promote the EMT through their effectors: proteins, miRNAs, circRNAs, and lncRNAs (Figure 2A).
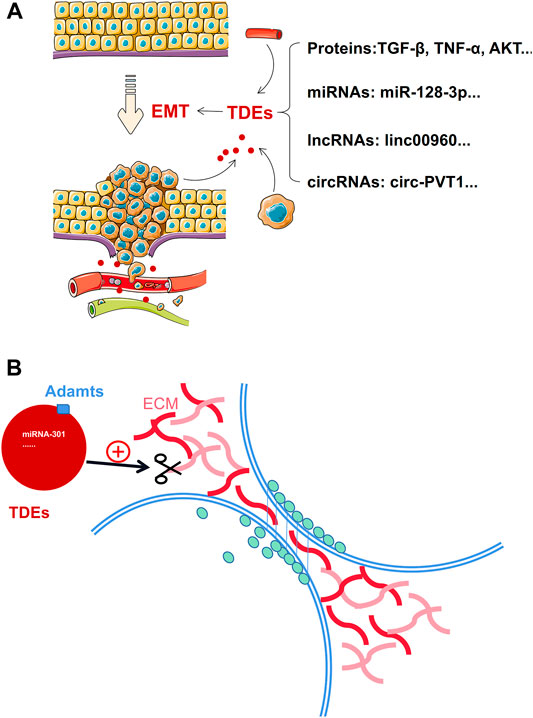
FIGURE 2. TDEs enhance the migration ability of tumor cells by promoting EMT and degrading the ECM. (A): Exosomes carry proteins, miRNA, lncRNA, and circRNA to promote the occurrence and development of EMT. (B): TDEs carry proteins or non-coding RNA to initiate degradation of the ECM.
Tumor-Derived Exosomes Promote Extracellular Matrix Degradation
The extracellular matrix (ECM) is a scaffold for tissues and organs (Eble and Niland, 2019). The ECM is a complex network combined with proteins, proteoglycans, and glycoproteins that can regulate cell growth, survival, motility, and differentiation through specific receptors, such as integrin, syndecan, and discoidin receptors (Leitinger, 2011; Xian et al., 2010). Cancer-associated ECM is not only an integral feature of a tumor but also actively contributes to its histopathology and malignant behavior (Levental et al., 2009; Provenzano et al., 2008). From tumor initiation to metastasis, ECM molecules bind with cell surface receptors and activate intracellular signaling pathways. ECM adhesion–induced signals promote self-sufficient growth through mitogen-activated protein kinase (MAPK) and phosphatidylinositol 3-kinase (PI3K) (Pylayeva et al., 2009). Focal adhesion kinase (FAK) signaling inhibits p15 and p21, which are growth suppressors, and p53 to limit the induction of apoptosis (Kim et al., 2008). TGF-β and RhoA/Rac signaling promote EMT induction and enhance promigratory pathways (Leight et al., 2012). The ECM can also enhance angiogenesis and strengthen vascular endothelial growth factor (VEGF) signaling in endothelial cells (Liu and Agarwal, 2010).
TDEs mediate tumor–tumor and tumor–host cell crosstalk (Kalluri, 2016). TDEs interact with and regulate the synthesis of ECM components and are involved in ECM remodeling (Rackov et al., 2018). The proteins on the surface of TDEs promote the activation of membrane-associated proteinases, such as Adamts1, Adamts4, and Adamts5, thus improving proteolytic activity (Ginestra et al., 1997; Lo Cicero et al., 2012). In addition, matrix metalloproteinases (MMPs) derived from TDEs participate in localized degradation and ECM proteolysis during cellular migration and metastasis (Ginestra et al., 1997; Atay et al., 2014). However, besides exosomal surface proteins, non-coding RNA also mediates ECM degradation. For example, breast cancer–derived exosomes carry miR-301 to regulate matrix modulation (Morad et al., 2020). Gastric cancer cell–derived exosomal miR-27a reshapes the ECM at adjacent sites and promotes tumor progression by downregulating CSRP2 expression and upregulating α-SMA expression (Wang et al., 2018). Currently, there are no direct reports on other non-coding RNAs, such as lncRNA and cicrRNA, but TDE-derived lncRNA and circRNA can influence fibroblast, chondrocyte, and epithelial cell function, secreting ECM components into the extracellular space (Tan et al., 2020).
Some data suggested that the ECM is a prerequisite for tumor cell invasion and metastasis (Tan et al., 2020). When the tumor cells metastasize, they detach from the ECM. Furthermore, the exosomes participate in this process (Figure 2B).
Tumor-Derived Exosomes Promote Angiogenesis Directly or Indirectly
Regardless of tumor size, metastasis may occur; however, in most cases, metastasis is associated with large primary neoplasms (Fidler and Kripke, 2015). If a tumor mass exceeds 1 mm in diameter, angiogenesis is bound to occur (Folkman, 1971; Nagy and Dvorak, 2012). Therefore, exploring tumor angiogenesis is an important way to understand tumor metastasis.
Tumor-Derived Exosomes Promote Angiogenesis by Activating Macrophages
Cancer-derived exosomes stimulate macrophage infiltration and polarization for establishing a pre-metastatic niche. For example, exosomes derived from CT-26, a colon cancer cell, can provoke macrophages to secrete significantly high levels of monocyte chemoattractant protein-1 (MCP-1) and TNF, thus promoting the growth and migration of colorectal cancer cells. Lung cancer cell–derived exosomes containing miRNA-103 upregulate angiogenic VEGF-A and angiopoietin-1 expression from M2 macrophages (Hsu et al., 2018; Wu et al., 2019). Therefore, TDEs can motivate the angiogenic property of macrophages such as secretion of VEGF (Wu et al., 2019). It can induce angiogenesis by tumor cells. In addition, other immune cells also contribute to tumor angiogenesis, such as neutrophils, myeloid precursor cells (MPCs), and dendritic cells (DCs) (Albini et al., 2018). But, there are no reports about TDE-educated neutrophils, MPCs, or DCs to promote angiogenesis in metastasis.
Tumor-Derived Exosomes Carry Non-coding RNA and Proteins to Promote Angiogenesis Directly
TDEs carry non-coding RNAs, including microRNA, lncRNA, and circRNA, that play an indispensable role in angiogenesis. TDEs carry miR-25-3p that regulates VEGFR2, ZO-1, occludin, and claudin5 expression in ECs by targeting KLF2 and KLF4 and eventually promotes vascular permeability and angiogenesis (Felcht et al., 2012; Wu et al., 2019). TDEs deliver miR-130a to vascular cells to promote angiogenesis by targeting c-MYB (Yang et al., 2018). Exosomal miR-155-5p can induce angiogenesis through the SOCS1/JAK2/STAT3 signaling pathway (Zhou X. et al., 2018). Exosomal miR-135b promotes angiogenesis by inhibiting FOXO1 expression. Exosomal miR-23a induces angiogenesis by targeting TSGA10, prolyl hydroxylase, tight junction protein ZO-1, and SIRT1 (Sruthi et al., 2018; Bai et al., 2019). Exosomal miR-1229 promotes angiogenesis by targeting HIPK2. Exosomal miR-21 promotes angiogenesis by targeting STAT3 (Liu and Cao et al., 2016). In addition, lncRNA containing lncCCAT2, lncMALAT1, lncRNA-p21, and lncPOU3F3 or circRNA, such as TDE-derived circRNA-100338, also promote angiogenesis (Castellano et al., 2020; Huang X.-Y. et al., 2020; Lang, Hu, & Chen et al., 2017; Lang, Hu, &; Zhang Z. et al., 2017; Qiu J.-J. et al., 2018). LncRNA and circRNA are often used as “sponges” to regulate miRNA expression in cells. Moreover, TDEs carry a variety of angiogenic proteins, such as VEGF, IGFBP3, MMP2, ICAM-1, and IL-8, thus enhancing angiogenesis through in vitro and in vivo ligand/receptor signaling (Ludwig and Whiteside, 2018). Therefore, a combination of multiple non-coding RNAs and exosomal proteins promotes tumor angiogenesis.
The importance of angiogenesis in tumor metastasis cannot be understated, TDEs can carry proteins and non-coding RNAs that directly promote angiogenesis or they can mediate angiogenesis indirectly by “educating” macrophages to release proangiogenic factors (Figure 3).
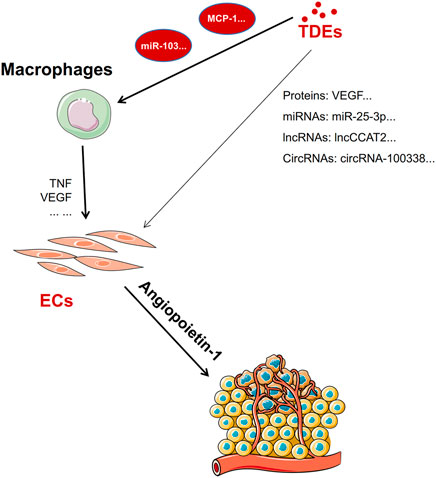
FIGURE 3. Exosomes directly or indirectly promote angiogenesis. TDEs promote macrophages to release TNF and VEGF to promote angiogenesis factors by carrying miRNAs or proteins. In addition, TDEs carry proteins, miRNAs, lncRNA, or circRNA to promote angiogenesis directly by targeting endothelial cells.
Tumor-Derived Exosomes can Protect Tumor Cells During Metastasis
Tumor cells shed from primary or secondary tumors are called circulating tumor cells (CTCs) (Paoletti and Hayes, 2016). CTCs invade the bloodstream and attach to the endothelium in the target organ. They then invade the surrounding parenchyma to form new tumors (Garcia et al., 2018). Blood is an unfavorable environment for CTCs, and they struggle with circulating immune cells (Agarwal et al., 2018). TDEs help CTCs metastasize smoothly by inhibiting immune cell activity and conferring a protective layer on them, thus maintaining cellular integrity (Figure 4).
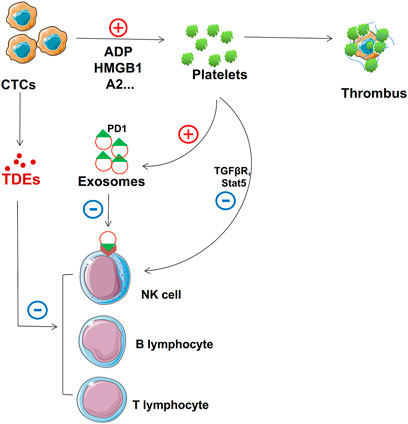
FIGURE 4. Role of TDEs in protecting CTCs. TDEs from CTCs and activated platelets suppress NK cell, T cell, and B cell function. In addition, activated platelets can form thrombi that adhere to the surface to protect CTCs.
Tumor-Derived Exosomes can Suppress Immune Cells to Protect CTCs
The immune system inhibits the progression of cancer cells. Many immune cells are found circulating in human blood, including T lymphocytes, natural killer (NK) cells, and B lymphocytes (de la Cruz-Merino et al., 2008; Grivennikov et al., 2010; McCarthy, 2001). These immune cells play crucial roles in immune surveillance, immunosuppression, and killing effects and mainly act on CTCs (Deepak and Acharya, 2010; Pahl and Cerwenka, 2017; Wernersson and Pejler, 2014; Ye et al., 2017). Immune cells can recognize and attack CTCs under normal circumstances; therefore, immunosuppression is necessary for the metastasis of CTCs (Guo et al., 2019). Many researchers have found that TDEs can suppress immune cells. Exosomes carry bioactive molecules that can impair immune cell function (Becker et al., 2016; Kalluri, 2016; Robbins and Morelli, 2014). Programmed cell death receptor ligand 1 (PD-L1) can bind to programmed cell death protein 1 (PD-1) to inactivate T cells through its extracellular domain (L. Chen and Han, 2015; Chen et al., 2015; Garcia-Diaz et al., 2019). TDEs carry PD-L1 on their surface and suppress CD8+ T cell function in metastatic melanoma (Chen et al., 2018). In addition to PD-1, TDEs can also carry others to inhibit T cell function, and prolyl hydroxylase can inhibit CD4+ and CD8+ T cell functions by oxygen sensing (Clever et al., 2016). TDEs block T cell activation and enhance T cell apoptosis (Czernek and Dutchler, 2017; Ludwig et al., 2017). TDEs can also cause NK cell dysfunction. NK cells do not express PD-1; however, TDEs interfere with the TGFβ/TGFβRI/II pathway and other common molecular pathways, such as the adenosine pathway, eventually driving NK cell responses (Hong et al., 2017). In addition, TDEs can inhibit NK cell cytotoxicity by suppressing STAT5 activation (Zhang et al., 2007). B cells play a critical role in immunoglobulin, antigen, and proinflammatory cytokine secretion (Mauri and Bosma, 2012). TDE HMGB1 regulates the proliferation of T cell Ig and mucin domain-1+ (TIM-1+) B cells and fosters cancer cell immune evasion (Ye et al., 2018). We can design therapeutic modalities to enhance immune cell surveillance and killing of these tumors by understanding these signaling pathways.
CTCs Activate Platelets Directly or by Releasing Exosomes
Platelets play major roles in hemostasis and coagulation and regulate the efficiency of canceration, tumor angiogenesis, tumor metastasis, and chemotherapy (Sharma et al., 2014). Platelets and cancer cells interact, thus affecting tumor growth and metastasis (Sharma P. et al., 2018). During blood circulation, other nontumor help is essential, for example, platelets can protect CTCs from blood flow shear forces by providing a protective layer. CTCs release soluble mediators, such as adenosine diphosphate (ADP), thromboxane (TX) A2, or high-mobility group box 1 (HMGB1), that can ligate toll-like receptor 4 (TLR4) to instigate localized platelet activation and form thrombus encasing tumor cells, thus protecting them from cytolysis by NK cells (Aitokallio-Tallberg et al., 1985; Nieswandt et al., 1999; Yu et al., 2014; Zucchella et al., 1989).
The interaction between platelets and CTCs can lead to platelet activation, and platelets release cytokines conducive to the survival and proliferation of tumor cells. When platelets combine with circulating tumor cells, platelet-derived soluble factors (TGF β and PDGF) mediate and prevent NK cells from detecting and dissolving tumor cells (Lambert et al., 2017; Lee J.-K. et al., 2013).
Finally, platelets prevent tumor cells from being eliminated by the immune system. Platelet-derived TGF-β can downregulate NKG2D expression and inactivate NK cells (Y. Chen et al., 2015; Kopp et al., 2009). The platelet expression profile in tumor and nontumor patients varies substantially (Santarpia et al., 2018). The interaction between CTCs and platelets can protect CTCs from immune surveillance during circulation and help tumor cells adhere to the endothelial cells at the metastasis site (Santarpia et al., 2018). Kuznetsov et al. showed that luminal breast cancer cells carried platelets that loaded factors with the effect of pro-inflammatory and pro-angiogenic activities and confirmed that these factors were released at distant tumors sites (Kuznetsov et al., 2012). Platelets are essential for releasing proangiogenic cytokines and recruiting angiogenic vascular endothelial growth factor receptor 2+ (VEGFR2+) cells that promote malignant progression (Schlesinger, 2018). Moreover, studies have shown that platelets may not just have a secondary role but may also drive malignant progression (or metastasis) (Kuo et al., 2011).
In human blood, platelets are considered to be the major contributors of exosomes (Caby et al., 2005). Goetzl et al. showed that endothelial cells absorb platelet-derived exosomes and enhance their adhesion by increasing endothelial cell adhesion protein expression and anti-adhesion factor production, thereby promoting CTC adhesion in vascular endothelial cells (Goetzl et al., 2016). Platelet-derived exosomes also increase platelet adhesion to monocytes and consequently monocyte activation, thus promoting the formation of inflammatory phenotypes (Goetzl et al., 2016).
Therefore, many researchers believe that blood platelets may be a potential source of biomarkers to aid cancer diagnosis. Nonetheless, the mechanism using which CTC-educated platelets mediate CTCs to avoid damage in the circulatory system still needs further research. We firmly believe that these CTC-educated platelet-derived exosomes play an important role in preventing damage to CTCs.
Integrins of Tumor-Derived Exosomes Determine Organotropic Metastasis
That different types of cancer cells preferentially colonize and metastasize to different organs is the salient feature of metastasis (Nguyen et al., 2009). Current research shows that tumors mainly metastasize to lung, brain, lymph node, bone, and liver tissues. We have summarized organotropic metastasis with respect to cancer types (Table 1). Many studies focus on tumor cell adhesion function, and extracellular matrix molecules, such as integrins, have been determined to be related to the choice of organotropic metastasis (Valastyan and Weinberg, 2011).
Integrins, a large family of adhesion molecules, can mediate cell–cell and cell–extracellular matrix interactions (Desgrosellier and Cheresh, 2010). Many integrins are associated with tumor angiogenesis, such as αvβ3, αvβ5, and α5β1 (Cascone et al., 2005; Lee et al., 2013a; Huang and Rofstad, 2018). β1 integrins bind to vascular cell adhesion molecule 1 (VCAM-1) on ECs and play an important role in trans-endothelial migration (Klemke et al., 2007). Integrins participate in tumor angiogenesis by interacting with the VEGF–VEGFR and ANG–TIE pathways (Klemke et al., 2007). αvβ3 integrin binds to the adhesion molecule L1 on ECs driving trans-endothelial migration (Voura et al., 2001). αvβ3 integrin is the most abundant and influential receptor among integrins on ECs and can regulate angiogenesis (De et al., 2005; Mahabeleshwar et al., 2008; Shattil and Ginsberg, 1997). It can be activated and colocalized with VEGFR-2 on ECs of proliferating blood vessels (Mahabeleshwar et al., 2008). VEGF-stimulated c-Src can be the phosphorylate β3 subunit on ECs, promoting VEGFR-2 phosphorylation and activation (De et al., 2005; Mahabeleshwar et al., 2008; Mahabeleshwar et al., 2007). In addition, αvβ3 is necessary for the survival and maturation of new blood vessels, and proliferative angiogenic EC apoptosis occurs after treatment with αvβ3 antagonists (Brooks et al., 1994). Briefly, integrin subunits α1, α2, α3, α4, α5, α6, α9, αv, β1, β3, and β5 are involved in physiological or pathological angiogenesis. Exosomes affect several steps of angiogenesis including motility, cytokine production, cell adhesion, and cell signaling (Taverna et al., 2012). These can improve the tumor survival environment before metastasis.
Although integrins are secreted by tumor cells, it is transported by exosomes to a distant organ (Peinado et al., 2017). Lyden et al. showed that tumor exosome integrins can determine organotropic metastasis. They suggested that tumor exosome integrins can fuse with organ-specific resident cells and activate Src phosphorylation and proinflammatory S100 expression to establish a pre-metastatic niche (Hoshino et al., 2015). In addition, more bodies of evidence identified that different integrins on the surface of exosomes play varied roles in metastasis to specific organs (Alderton, 2015; Hoshino et al., 2015; Paolillo and Schinelli, 2017). For instance, exosomal integrins α6β4 and α6β1 preferentially direct tumor cells to the lungs, and αvβ5 induces liver metastasis (Hoshino et al., 2015). Tumor exosomes can prepare pre-metastatic niches to facilitate organ-specific metastasis, even for cancer cells equipped to metastasize (Figure 5).
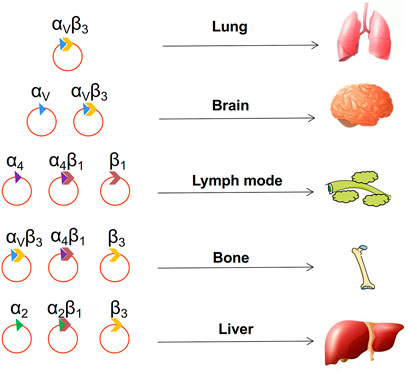
FIGURE 5. Choice of metastatic organs. The integrin on the surface of the exosome determines where the tumor metastasizes.
Tumor Cell Growth at the Metastasis Site
Once tumor cells migrate to tissues and organs, TDEs provide them with a good growth environment and the ability to promote their growth.
Tumor-Derived Exosomes Promote Pre-metastatic Niche Formation
A pre-metastasis niche is a primary tumor in secondary organs and tissues that creates a favorable microenvironment for subsequent metastasis. Tumor-derived molecules secreted by primary tumors play a key role in preparing distant sites for the formation of new pre-metastasis niches, promoting metastasis and even determining the orientation of metastatic organs. These major tumor-derived molecules are usually tumor-derived secretory factors, extracellular vesicles (EVs), and other molecular components (Minciacchi et al., 2015). Exosomes containing protein, mRNA, or DNA fragments promote the pre-metastasis niche formation by mediating the communication between tumor cells and surrounding components or transferring their contents to recipient cells (Chin and Wang, 2016; Zhou et al., 2014).
Tumor cells are “seeds”. With tumor-secreting factors, tumor cell–secreting vesicles, and exosomes acting as catalysts, tumor cells can promote the formation of the “soil” (host microenvironment) in a distant metastasis site and promote the growth of cancer cells. Cancer metastasis is preceded by the interaction between the seed and soil (Y. Chen et al., 2015; Lambert et al., 2017; Liu and Cao, 2016; Minciacchi et al., 2015). Primary tumor cells influence and change the microenvironment at secondary organs by promoting pre-metastasis niche factor before tumor cells arrive (Chin and Wang, 2016; He et al., 2017).
The characteristics of a pre-metastasis niche include the following six aspects. First, pre-metastasis niche formation is accompanied by the recruitment of bone marrow–derived cells (BMDC) (Y. Chen et al., 2015; Minciacchi et al., 2015). Literature suggests that extracellular matrix metalloproteinase inducer (EMMPRIN) in cancer cells can induce the secretion and expression of many factors, such as SDF and VEGF, which mediate the recruitment of BMDC to the liver and lungs (Y. Chen et al., 2015; Minciacchi et al., 2015). Second, the immune cells involved in the pre-transfer niche formation are heterogenous. Pre-metastasis niche formation involves not only the recruitment of foreign cells but also the reprogramming of resident stromal cells, promoting metastasis. Pre-metastasis niche formation also involves the change of the ECM. Niche formation before transfer is accompanied by a change in the vascular system. Metastatic breast cancer cells reduce tight junctions between endothelial cells by secreting exosomes containing mir-105, thus inducing systemic vascular leakage and promoting metastasis (Kong et al., 2019). Breast cancer cells secrete exosomes containing miR-122, which are absorbed by niche cells, and reduce glucose consumption by targeting pyruvate kinase, thus increasing the proliferation rate and survival rate of cancer cells and promoting metastasis (Fong et al., 2015). Pancreatic cancer–derived exosomes, rich in macrophage migration inhibitory factors, recruit macrophages and induce pre-metastasis niche formation in the liver (Costa-Silva et al., 2015). Modulation of the pre-metastatic niche formation by controlling TDEs is a new area for future chemotherapy research.
Tumor-Derived Exosomes Promote the Growth of Metastasis Tumor
The growth of metastatic tumors requires suitable “soil”. MET returns the cancer cells to a highly proliferative state but with the loss of their migration characteristics, enabling tumor growth at the metastasis site (Li K. et al., 2019). The characteristics of MET are increased expression of mesenchymal markers, such as vimentin, and decreased expression of epithelial markers, such as E-cadherin, compared with that of EMT (Wells et al., 2008). MET supports the reacquisition of epithelial features to promote metastasis (Brabletz et al., 2001). Several signaling pathways are involved in regulating MET, including transforming growth factor (TGF), fibroblast growth factors (FGFs), bone morphogenic protein (BMP), epidermal growth factor receptor (EGFR), hepatocyte growth factor (HGF), Wnt/β-catenin, and Notch pathways (Said and Williams, 2011). TDEs can support tumor progression and remodel surrounding parenchymal tissues at the metastatic site (Greening et al., 2015). TDEs play an important regulatory role mediating EMT and transforming MET (Bigagli et al., 2019). Gastric cancer cell–derived exosomes can mediate the stimulation of proinflammatory cytokine IL-1β secretion and activate the Akt and MAPK pathways to promote tumor growth at the metastatic site (Che et al., 2018; Wang et al., 2019e). In addition, TDEs can transform stromal cells into tumor-associated stromal cells (TASCs) that can secrete many pro-tumorigenic factors, including IL-6 and IL-8. These factors can enhance the proliferation ability of tumor cells (Bussard et al., 2016). Hence, TDEs can enable tumor cells to acquire proliferation capacity directly through the MET process or promote tumor proliferation by inducing TASC formation and releasing related factors (Figure 6). Nevertheless, there is still a dearth of research on exosomes and their contribution to MET.
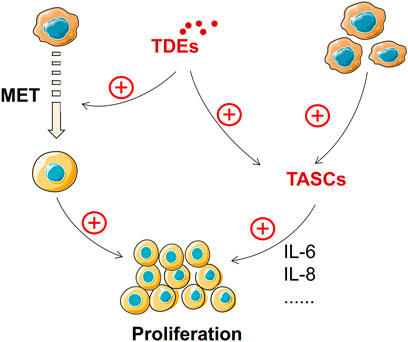
FIGURE 6. TDEs promote tumor growth at the metastasis site. MET and TASCs promote tumor growth at metastatic sites, and TDEs can derive MET and TASC formation.
Conclusion
Exosomes play an important role in every step leading to tumor metastasis. Although there are many reports on the role of exosomes in metastasis, much is left to be explored of the potential mechanisms underlying metastasis. Although a few studies still have unclear results, we have summarized the published literature on the substances that exosomes carry, their main functions in different tumors, the target cells affected, and steps involved in metastasis. (Table 2). Exploring these underlying mechanisms will enlighten us about cancer biology and contribute to the prevention of and therapeutic strategies for malignancies. We can manipulate TDEs to impede not just metastasis formation but even established metastases.
Author Contributions
SB and ZW developed the first draft of the manuscript. All authors contributed to the planning, organization, data collection, and writing of the manuscript. JD completed all figures and provided critical edits. The final version of the manuscript was approved by all authors.
Funding
The study was supported by grants from the National Natural Science Foundation of China (Grant Nos. 81972539 and U1732157).
Conflict of Interest
The authors declare that the research was conducted in the absence of any commercial or financial relationships that could be construed as a potential conflict of interest.
Publisher’s Note
All claims expressed in this article are solely those of the authors and do not necessarily represent those of their affiliated organizations, or those of the publisher, the editors, and the reviewers. Any product that may be evaluated in this article, or claim that may be made by its manufacturer, is not guaranteed or endorsed by the publisher.
References
Abdel Rahman, A. R. M., Gaafar, R. M., Baki, H. A., El Hosieny, H. M., Aboulkasem, F., Farahat, E. G., et al. (2008). Prevalence and Pattern of Lymph Node Metastasis in Malignant Pleural Mesothelioma. Ann. Thorac. Surg. 86 (2), 391–395. doi:10.1016/j.athoracsur.2008.04.012
Abe, Y., Suzuki, M., Tsuji, K., Sato, M., Kimura, H., Kimura, H., et al. (2020). Lung Metastasis from Gastric Cancer Presenting as Diffuse Ground-Glass Opacities. Respir. Med. Case Rep. 30, 101104. doi:10.1016/j.rmcr.2020.101104
Aga, M., Bentz, G. L., Raffa, S., Torrisi, M. R., Kondo, S., Wakisaka, N., et al. (2014). Exosomal HIF1α Supports Invasive Potential of Nasopharyngeal Carcinoma-Associated LMP1-Positive Exosomes. Oncogene 33 (37), 4613–4622. doi:10.1038/onc.2014.66
Agarwal, A., Balic, M., El-Ashry, D., and Cote, R. J. (2018). Circulating Tumor Cells. Cancer J. 24 (2), 70–77. doi:10.1097/PPO.0000000000000310
Ai, C., Ma, G., Deng, Y., Zheng, Q., Gen, Y., Li, W., et al. (2020). Nm23‐H1 Inhibits Lung Cancer Bone‐specific Metastasis by Upregulating miR‐660‐5p Targeted SMARCA5. Thorac. Cancer 11 (3), 640–650. doi:10.1111/1759-7714.13308
Aitokallio-Tallberg, A., Kärkkäinen, J., Pantzar, P., Wahlström, T., and Ylikorkala, O. (1985). Prostacyclin and Thromboxane in Breast Cancer: Relationship between Steroid Receptor Status and Medroxyprogesterone Acetate. Br. J. Cancer 51 (5), 671–674. doi:10.1038/bjc.1985.101
Aktan, M., Koc, M., Yavuz, B. B., and Kanyilmaz, G. (2015). Two Cases of Gastrointestinal Stromal Tumor of the Small Intestine with Liver and Bone Metastasis. Ann. Transl Med. 3 (17), 259. doi:10.3978/j.issn.2305-5839.2015.09.46
Al-Nedawi, K., Meehan, B., Micallef, J., Lhotak, V., May, L., Guha, A., et al. (2008). Intercellular Transfer of the Oncogenic Receptor EGFRvIII by Microvesicles Derived from Tumour Cells. Nat. Cel Biol 10 (5), 619–624. doi:10.1038/ncb1725
Albini, A., Bruno, A., Noonan, D. M., and Mortara, L. (2018). Contribution to Tumor Angiogenesis from Innate Immune Cells within the Tumor Microenvironment: Implications for Immunotherapy. Front. Immunol. 9, 527. doi:10.3389/fimmu.2018.00527
Alderton, G. K. (2015). Directions to Metastatic Sites. Nat. Rev. Cancer 15 (12), 696–697. doi:10.1038/nrc4046
Alhoulaiby, S., Abdulrahman, A., Alouni, G., Mahfoud, M., and Shihabi, Z. (2020). Extra‐CNS Metastasis of Glioblastoma Multiforme to Cervical Lymph Nodes and Parotid Gland: A Case Report. Clin. Case Rep. 8 (9), 1672–1677. doi:10.1002/ccr3.2985
Ali, A., Kim, S. H., Kim, M. J., Choi, M. Y., Kang, S. S., Cho, G. J., et al. (2017). O-GlcNAcylation of NF-kappaB Promotes Lung Metastasis of Cervical Cancer Cells via Upregulation of CXCR4 Expression. Mol. Cell 40 (7), 476–484. doi:10.14348/molcells.2017.2309
Aljohani, H. M., Aittaleb, M., Furgason, J. M., Amaya, P., Deeb, A., Chalmers, J. J., et al. (2018). Genetic Mutations Associated with Lung Cancer Metastasis to the Brain. Mutagenesis 33 (2), 137–145. doi:10.1093/mutage/gey003
AlShammari, A., Almasri, T., Sarraj, J., AlAshgar, O., Ahmed, M. H., AlKattan, K., et al. (2020). Pulmonary Metastasis of Head and Neck Cancer: Surgical Removal Outcomes from a Tertiary Care center. Indian J. Thorac. Cardiovasc. Surg. 36 (3), 199–206. doi:10.1007/s12055-019-00866-3
Asoh, Y., Nakamura, M., Maeda, T., Shiogai, T., Ogashiwa, M., Takeuchi, K., et al. (1990). Brain Metastasis from Primary Pericardial Mesothelioma. Neurol. Med. Chir.(Tokyo) 30 (11 Spec No), 884–887. doi:10.2176/nmc.30.884
Atay, S., Banskota, S., Crow, J., Sethi, G., Rink, L., and Godwin, A. K. (2014). Oncogenic KIT-Containing Exosomes Increase Gastrointestinal Stromal Tumor Cell Invasion. Proc. Natl. Acad. Sci. 111 (2), 711–716. doi:10.1073/pnas.1310501111
Bai, M., Li, J., Yang, H., Zhang, H., Zhou, Z., Deng, T., et al. (2019). miR-135b Delivered by Gastric Tumor Exosomes Inhibits FOXO1 Expression in Endothelial Cells and Promotes Angiogenesis. Mol. Ther. 27 (10), 1772–1783. doi:10.1016/j.ymthe.2019.06.018
Bakir, B., Chiarella, A. M., Pitarresi, J. R., and Rustgi, A. K. (2020). EMT, MET, Plasticity, and Tumor Metastasis. Trends Cel Biol. 30 (10), 764–776. doi:10.1016/j.tcb.2020.07.003
Balaj, L., Lessard, R., Dai, L., Cho, Y.-J., Pomeroy, S. L., Breakefield, X. O., et al. (2011). Tumour Microvesicles Contain Retrotransposon Elements and Amplified Oncogene Sequences. Nat. Commun. 2, 180. doi:10.1038/ncomms1180
Bale, R., Putzer, D., and Schullian, P. (2019). Local Treatment of Breast Cancer Liver Metastasis. Cancers 11 (9), 1341. doi:10.3390/cancers11091341
Bao, L., You, B., Shi, S., Shan, Y., Zhang, Q., Yue, H., et al. (2018). Metastasis-associated miR-23a from Nasopharyngeal Carcinoma-Derived Exosomes Mediates Angiogenesis by Repressing a Novel Target Gene TSGA10. Oncogene 37 (21), 2873–2889. doi:10.1038/s41388-018-0183-6
Basu, S., and Bhattacharyya, S. N. (2014). Insulin-like Growth Factor-1 Prevents miR-122 Production in Neighbouring Cells to Curtail its Intercellular Transfer to Ensure Proliferation of Human Hepatoma Cells. Nucleic Acids Res. 42 (11), 7170–7185. doi:10.1093/nar/gku346
Becker, A., Thakur, B. K., Weiss, J. M., Kim, H. S., Peinado, H., and Lyden, D. (2016). Extracellular Vesicles in Cancer: Cell-To-Cell Mediators of Metastasis. Cancer Cell 30 (6), 836–848. doi:10.1016/j.ccell.2016.10.009
Berish, R. B., Ali, A. N., Telmer, P. G., Ronald, J. A., and Leong, H. S. (2018). Translational Models of Prostate Cancer Bone Metastasis. Nat. Rev. Urol. 15 (7), 403–421. doi:10.1038/s41585-018-0020-2
Bhagirath, D., Yang, T. L., Bucay, N., Sekhon, K., Majid, S., Shahryari, V., et al. (2018). microRNA-1246 Is an Exosomal Biomarker for Aggressive Prostate Cancer. Cancer Res. 78 (7), 1833–1844. doi:10.1158/0008-5472.CAN-17-2069
Bhandari, V., and Jain, R. (2013). A Retrospective Study of Incidence of Bone Metastasis in Head and Neck Cancer. J. Can. Res. Ther. 9 (1), 90–93. doi:10.4103/0973-1482.110385
Biagioni, A., Laurenzana, A., Menicacci, B., Peppicelli, S., Andreucci, E., Bianchini, F., et al. (2021). uPAR-Expressing Melanoma Exosomes Promote Angiogenesis by VE-Cadherin, EGFR and uPAR Overexpression and Rise of ERK1,2 Signaling in Endothelial Cells. Cell. Mol. Life Sci. 78 (6), 3057–3072. doi:10.1007/s00018-020-03707-4
Bigagli, E., Cinci, L., D'Ambrosio, M., and Luceri, C. (2019). Transcriptomic Characterization, Chemosensitivity and Regulatory Effects of Exosomes in Spontaneous EMT/MET Transitions of Breast Cancer Cells. Cancer Genomics Proteomics 16 (3), 163–173. doi:10.21873/cgp.20122
Brabletz, T., Jung, A., Reu, S., Porzner, M., Hlubek, F., Kunz-Schughart, L. A., et al. (2001). Variable -catenin Expression in Colorectal Cancers Indicates Tumor Progression Driven by the Tumor Environment. Proc. Natl. Acad. Sci. 98 (18), 10356–10361. doi:10.1073/pnas.171610498
Brooks, P. C., Montgomery, A. M. P., Rosenfeld, M., Reisfeld, R. A., Hu, T., Klier, G., et al. (1994). Integrin αvβ3 Antagonists Promote Tumor Regression by Inducing Apoptosis of Angiogenic Blood Vessels. Cell 79 (7), 1157–1164. doi:10.1016/0092-8674(94)90007-8
Bussard, K. M., Mutkus, L., Stumpf, K., Gomez-Manzano, C., and Marini, F. C. (2016). Tumor-associated Stromal Cells as Key Contributors to the Tumor Microenvironment. Breast Cancer Res. 18 (1), 84. doi:10.1186/s13058-016-0740-2
Bustamante, P., Piquet, L., Landreville, S., and Burnier, J. V. (2021). Uveal Melanoma Pathobiology: Metastasis to the Liver. Semin. Cancer Biol. 71, 65–85. doi:10.1016/j.semcancer.2020.05.003
Caby, M.-P., Lankar, D., Vincendeau-Scherrer, C., Raposo, G., and Bonnerot, C. (2005). Exosomal-like Vesicles Are Present in Human Blood Plasma. Int. Immunol. 17 (7), 879–887. doi:10.1093/intimm/dxh267
Cai, J., Gong, L., Li, G., Guo, J., Yi, X., and Wang, Z. (2021). Exosomes in Ovarian Cancer Ascites Promote Epithelial-Mesenchymal Transition of Ovarian Cancer Cells by Delivery of miR-6780b-5p. Cell Death Dis 12 (2), 210. doi:10.1038/s41419-021-03490-5
Cai, Q., Zhu, A., and Gong, L. (2018). Exosomes of Glioma Cells Deliver miR-148a to Promote Proliferation and Metastasis of Glioblastoma via Targeting CADM1. Bull. du Cancer 105 (7-8), 643–651. doi:10.1016/j.bulcan.2018.05.003
Cai, Z., Yang, F., Yu, L., Yu, Z., Jiang, L., Wang, Q., et al. (2012). Activated T Cell Exosomes Promote Tumor Invasion via Fas Signaling Pathway. J.I. 188 (12), 5954–5961. doi:10.4049/jimmunol.1103466
Caldaria, A., Giuffrida, R., di Meo, N., Massari, L., Dianzani, C., Cannavò, S. P., et al. (2020). Diagnosis and Treatment of Melanoma Bone Metastasis: A Multidisciplinary Approach. Dermatol. Ther. 33 (6), e14193. doi:10.1111/dth.14193
Campos, A., Salomon, C., Bustos, R., Díaz, J., Martínez, S., Silva, V., et al. (2018). Caveolin-1-containing Extracellular Vesicles Transport Adhesion Proteins and Promote Malignancy in Breast Cancer Cell Lines. Nanomedicine 13 (20), 2597–2609. doi:10.2217/nnm-2018-0094
Canda, A. E., Ozsoy, Y., Nalbant, O. A., and Sagol, O. (2008). Gastrointestinal Stromal Tumor of the Stomach with Lymph Node Metastasis. World J. Surg. Onc 6, 97. doi:10.1186/1477-7819-6-97
Cao, S.-Q., Zheng, H., Sun, B.-C., Wang, Z.-L., Liu, T., Guo, D.-H., et al. (2019). Long Non-coding RNA Highly Up-Regulated in Liver Cancer Promotes Exosome Secretion. Wjg 25 (35), 5283–5299. doi:10.3748/wjg.v25.i35.5283
Carvalho, J., Teixeira, M., Silva, F. T., Esteves, A., Ribeiro, C., and Guerra, D. (2020). Esophageal Gastrointestinal Stromal Tumor with Rare Intracranial Metastasis. Case Rep. Gastrointest. Med. 2020, 1–4. doi:10.1155/2020/8842006
Cascone, I., Napione, L., Maniero, F., Serini, G., and Bussolino, F. (2005). Stable Interaction between α5β1 Integrin and Tie2 Tyrosine Kinase Receptor Regulates Endothelial Cell Response to Ang-1. J. Cel Biol 170 (6), 993–1004. doi:10.1083/jcb.200507082
Castellano, J. J., Marrades, R. M., Molins, L., Viñolas, N., Moises, J., Canals, J., et al. (2020). Extracellular Vesicle lincRNA-P21 Expression in Tumor-Draining Pulmonary Vein Defines Prognosis in NSCLC and Modulates Endothelial Cell Behavior. Cancers 12 (3), 734. doi:10.3390/cancers12030734
Cavanna, L., Seghini, P., Di Nunzio, C., Orlandi, E., Michieletti, E., Stroppa, E., et al. (2018). Gastric Cancer with Brain Metastasis and the Role of Human Epidermal Growth Factor 2 Status. Oncol. Lett. 15 (4), 5787–5791. doi:10.3892/ol.2018.8054
Chalmin, F., Ladoire, S., Mignot, G., Vincent, J., Bruchard, M., Remy-Martin, J.-P., et al. (2010). Membrane-associated Hsp72 from Tumor-Derived Exosomes Mediates STAT3-dependent Immunosuppressive Function of Mouse and Human Myeloid-Derived Suppressor Cells. J. Clin. Invest. 120 (2), 457–471. doi:10.1172/JCI40483
Chang, G., Shi, L., Ye, Y., Shi, H., Zeng, L., Tiwary, S., et al. (2020). YTHDF3 Induces the Translation of m6A-Enriched Gene Transcripts to Promote Breast Cancer Brain Metastasis. Cancer Cell 38 (6), 857–871. doi:10.1016/j.ccell.2020.10.004
Che, Y., Geng, B., Xu, Y., Miao, X., Chen, L., Mu, X., et al. (2018). Helicobacter pylori -induced Exosomal MET Educates Tumour-Associated Macrophages to Promote Gastric Cancer Progression. J. Cel Mol Med 22 (11), 5708–5719. doi:10.1111/jcmm.13847
Chen, D., Chen, G., Jiang, W., Fu, M., Liu, W., Sui, J., et al. (2019). Association of the Collagen Signature in the Tumor Microenvironment with Lymph Node Metastasis in Early Gastric Cancer. JAMA Surg. 154 (3), e185249. doi:10.1001/jamasurg.2018.5249
Chen, G., Huang, A. C., Zhang, W., Zhang, G., Wu, M., Xu, W., et al. (2018). Exosomal PD-L1 Contributes to Immunosuppression and Is Associated with Anti-PD-1 Response. Nature 560 (7718), 382–386. doi:10.1038/s41586-018-0392-8
Chen, L., and Han, X. (2015). Anti-PD-1/PD-L1 Therapy of Human Cancer: Past, Present, and Future. J. Clin. Invest. 125 (9), 3384–3391. doi:10.1172/JCI80011
Chen, P. G., Schoeff, S. S., Watts, C. A., Reibel, J. F., Levine, P. A., Shonka, D. C., et al. (2014). Utility of Abdominal Imaging to Assess for Liver Metastasis in Patients with Head and Neck Cancer and Abnormal Liver Function Tests. Am. J. Otolaryngol. 35 (2), 137–140. doi:10.1016/j.amjoto.2013.10.004
Chen, X., Chen, L., Zhu, H., and Tao, J. (2020). Risk Factors and Prognostic Predictors for Cervical Cancer Patients with Lung Metastasis. J. Cancer 11 (20), 5880–5889. doi:10.7150/jca.46258
Chen, Y., Gou, X., Kong, D. K., Wang, X., Wang, J., Chen, Z., et al. (2015). EMMPRIN Regulates Tumor Growth and Metastasis by Recruiting Bone Marrow-Derived Cells through Paracrine Signaling of SDF-1 and VEGF. Oncotarget 6 (32), 32575–32585. doi:10.18632/oncotarget.5331
Chen, Z., Yang, L., Cui, Y., Zhou, Y., Yin, X., Guo, J., et al. (2016). Cytoskeleton-centric Protein Transportation by Exosomes Transforms Tumor-Favorable Macrophages. Oncotarget 7 (41), 67387–67402. doi:10.18632/oncotarget.11794
Cheng, Z., Lei, Z., Yang, P., Si, A., Xiang, D., Tang, X., et al. (2019). Exosome‐transmitted P120‐catenin Suppresses Hepatocellular Carcinoma Progression via STAT3 Pathways. Mol. Carcinog 58 (8), 1389–1399. doi:10.1002/mc.23022
Chi, C., Fan, Z., Yang, B., Sun, H., and Zheng, Z. (2021). The Clinical Characteristics and Prognostic Nomogram for Head and Neck Cancer Patients with Bone Metastasis. J. Oncol. 2021, 1–12. doi:10.1155/2021/5859757
Chin, A. R., and Wang, S. E. (2016). Cancer Tills the Premetastatic Field: Mechanistic Basis and Clinical Implications. Clin. Cancer Res. 22 (15), 3725–3733. doi:10.1158/1078-0432.CCR-16-0028
Choi, D.-S., Kim, D.-K., Kim, Y.-K., and Gho, Y. S. (2013). Proteomics, Transcriptomics and Lipidomics of Exosomes and Ectosomes. Proteomics 13 (10-11), 1554–1571. doi:10.1002/pmic.201200329
Clever, D., Roychoudhuri, R., Constantinides, M. G., Askenase, M. H., Sukumar, M., Klebanoff, C. A., et al. (2016). Oxygen Sensing by T Cells Establishes an Immunologically Tolerant Metastatic Niche. Cell 166 (5), 1117–1131 e1114. doi:10.1016/j.cell.2016.07.032
Costa-Silva, B., Aiello, N. M., Ocean, A. J., Singh, S., Zhang, H., Thakur, B. K., et al. (2015). Pancreatic Cancer Exosomes Initiate Pre-metastatic Niche Formation in the Liver. Nat. Cel Biol 17 (6), 816–826. doi:10.1038/ncb3169
Cumsille, P., Godoy, M., Gerdtzen, Z. P., and Conca, C. (2019). Parameter Estimation and Mathematical Modeling for the Quantitative Description of Therapy Failure Due to Drug Resistance in Gastrointestinal Stromal Tumor Metastasis to the Liver. PLoS One 14 (5), e0217332. doi:10.1371/journal.pone.0217332
Czernek, L., and Düchler, M. (2017). Functions of Cancer-Derived Extracellular Vesicles in Immunosuppression. Arch. Immunol. Ther. Exp. 65 (4), 311–323. doi:10.1007/s00005-016-0453-3
da Silva, G. T., Bergmann, A., and Thuler, L. C. S. (2019). Incidence and Risk Factors for Bone Metastasis in Non-small Cell Lung Cancer. Asian Pac. J. Cancer Prev. 20 (1), 45–51. doi:10.31557/APJCP.2019.20.1.45
Dai, J., Escara-Wilke, J., Keller, J. M., Jung, Y., Taichman, R. S., Pienta, K. J., et al. (2019). Primary Prostate Cancer Educates Bone Stroma through Exosomal Pyruvate Kinase M2 to Promote Bone Metastasis. J. Exp. Med. 216 (12), 2883–2899. doi:10.1084/jem.20190158
Das, S. K., Pradhan, A. K., Bhoopathi, P., Talukdar, S., Shen, X.-N., Sarkar, D., et al. (2018). The MDA-9/Syntenin/IGF1R/STAT3 Axis Directs Prostate Cancer Invasion. Cancer Res. 78 (11), 2852–2863. doi:10.1158/0008-5472.CAN-17-2992
Datta, C. K., Weinstein, J. D., Bland, J. E., Brager, P. M., and Stewart, M. A. (1998). A Case of Cervical Lymph Node Metastasis Resulting from Glioblastoma Multiforme. W V Med. J. 94 (5), 276–278.
de la Cruz-Merino, L., Grande-Pulido, E., Albero-Tamarit, A., and Codes-Manuel de Villena, M. E. (2008). Cancer and Immune Response: Old and New Evidence for Future Challenges. Oncologist 13 (12), 1246–1254. doi:10.1634/theoncologist.2008-0166
De, S., Razorenova, O., McCabe, N. P., O'Toole, T., Qin, J., and Byzova, T. V. (2005). VEGF-integrin Interplay Controls Tumor Growth and Vascularization. Proc. Natl. Acad. Sci. 102 (21), 7589–7594. doi:10.1073/pnas.0502935102
Deepak, P., and Acharya, A. (2010). Anti-tumor Immunity and Mechanism of Immunosuppression Mediated by Tumor Cells: Role of Tumor-Derived Soluble Factors and Cytokines. Int. Rev. Immunol. 29 (4), 421–458. doi:10.3109/08830185.2010.483027
Demory Beckler, M., Higginbotham, J. N., Franklin, J. L., Ham, A.-J., Halvey, P. J., Imasuen, I. E., et al. (2013). Proteomic Analysis of Exosomes from Mutant KRAS colon Cancer Cells Identifies Intercellular Transfer of Mutant KRAS. Mol. Cell Proteomics 12 (2), 343–355. doi:10.1074/mcp.M112.022806
Deng, Z., Rong, Y., Teng, Y., Zhuang, X., Samykutty, A., Mu, J., et al. (2017). Exosomes miR-126a Released from MDSC Induced by DOX Treatment Promotes Lung Metastasis. Oncogene 36 (5), 639–651. doi:10.1038/onc.2016.229
Desgrosellier, J. S., and Cheresh, D. A. (2010). Integrins in Cancer: Biological Implications and Therapeutic Opportunities. Nat. Rev. Cancer 10 (1), 9–22. doi:10.1038/nrc2748
Dey, M., Fetcko, K., Gondim, D., and Bonnin, J. (2017). Cervical Cancer Metastasis to the Brain: A Case Report and Review of Literature. Surg. Neurol. Int. 8, 181. doi:10.4103/sni.sni_111_17
Di Modica, M., Regondi, V., Sandri, M., Iorio, M. V., Zanetti, A., Tagliabue, E., et al. (2017). Breast Cancer-Secreted miR-939 Downregulates VE-Cadherin and Destroys the Barrier Function of Endothelial Monolayers. Cancer Lett. 384, 94–100. doi:10.1016/j.canlet.2016.09.013
Diepenbruck, M., and Christofori, G. (2016). Epithelial-mesenchymal Transition (EMT) and Metastasis: Yes, No, Maybe? Curr. Opin. Cel Biol. 43, 7–13. doi:10.1016/j.ceb.2016.06.002
Ding, J., Xu, Z., Zhang, Y., Tan, C., Hu, W., Wang, M., et al. (2018). Exosome-mediated miR-222 Transferring: An Insight into NF-Κb-Mediated Breast Cancer Metastasis. Exp. Cel Res. 369 (1), 129–138. doi:10.1016/j.yexcr.2018.05.014
Dolman, C. L. (1974). Lymph Node Metastasis as First Manifestation of Glioblastoma. J. Neurosurg. 41 (5), 607–609. doi:10.3171/jns.1974.41.5.0607
Doshi, T. V., Doshi, J. V., Makaryus, J. N., and Makaryus, A. N. (2013). A Rare Case of Successfully Treated Cardiac Metastasis from Transitional Cell Bladder Cancer. Am. J. Ther. 20 (3), 307–310. doi:10.1097/MJT.0b013e3181f6c131
Du, Q., Ye, X., Lu, S.-R., Li, H., Liu, H.-Y., Zhai, Q., et al. (2021). Exosomal miR-30a and miR-222 Derived from colon Cancer Mesenchymal Stem Cells Promote the Tumorigenicity of colon Cancer through Targeting MIA3. J. Gastrointest. Oncol. 12 (1), 52–68. doi:10.21037/jgo-20-513
Eble, J. A., and Niland, S. (2019). The Extracellular Matrix in Tumor Progression and Metastasis. Clin. Exp. Metastasis 36 (3), 171–198. doi:10.1007/s10585-019-09966-1
Ekström, E. J., Bergenfelz, C., von Bülow, V., Serifler, F., Carlemalm, E., Jönsson, G., et al. (2014). WNT5A Induces Release of Exosomes Containing Pro-angiogenic and Immunosuppressive Factors from Malignant Melanoma Cells. Mol. Cancer 13, 88. doi:10.1186/1476-4598-13-88
El-Halabi, M. M., Chaaban, S. A., Meouchy, J., Page, S., and Salyers, W. J. (2014). Colon Cancer Metastasis to Mediastinal Lymph Nodes without Liver or Lung Involvement: A Case Report. Oncol. Lett. 8 (5), 2221–2224. doi:10.3892/ol.2014.2426
Elsner, L., Muppala, V., Gehrmann, M., Lozano, J., Malzahn, D., Bickeböller, H., et al. (2007). The Heat Shock Protein HSP70 Promotes Mouse NK Cell Activity against Tumors that Express Inducible NKG2D Ligands. J. Immunol. 179 (8), 5523–5533. doi:10.4049/jimmunol.179.8.5523
Estelles Piera, F., Peris Godoy, M., Saro Pérez, E., and Calabuig Alborch, J. R. (1993). Multiple Myeloma and Adenocarcinoma Liver Metastasis. Med. Interna 10 (7), 363.
Etoh, T., Baba, H., Adachi, E., Kohnoe, S., Seo, Y., Saito, T., et al. (1997). A Case of Liver Metastasis of Malignant Peritoneal Mesothelioma Successfully Treated by Surgery. Oncol. Rep. 4 (4), 803–805. doi:10.3892/or.4.4.803
Fan, Z., Huang, Z., Hu, C., Tong, Y., and Zhao, C. (2020). Risk Factors and Nomogram for Newly Diagnosis of Bone Metastasis in Bladder Cancer. Medicine (Baltimore) 99 (42), e22675. doi:10.1097/MD.0000000000022675
Fang, J. H., Zhang, Z. J., Shang, L. R., Luo, Y. W., Lin, Y. F., Yuan, Y., et al. (2018). Hepatoma Cell‐secreted Exosomal microRNA‐103 Increases Vascular Permeability and Promotes Metastasis by Targeting junction Proteins. Hepatology 68 (4), 1459–1475. doi:10.1002/hep.29920
Fang, T., Lv, H., Lv, G., Li, T., Wang, C., Han, Q., et al. (2018). Tumor-derived Exosomal miR-1247-3p Induces Cancer-Associated Fibroblast Activation to foster Lung Metastasis of Liver Cancer. Nat. Commun. 9 (1), 191. doi:10.1038/s41467-017-02583-0
Fang, W.-Y., Kuo, Y.-Z., Chang, J.-Y., Hsiao, J.-R., Kao, H.-Y., Tsai, S.-T., et al. (2020). The Tumor Suppressor TGFBR3 Blocks Lymph Node Metastasis in Head and Neck Cancer. Cancers 12 (6), 1375. doi:10.3390/cancers12061375
Faries, M. B., Han, D., Reintgen, M., Kerivan, L., Reintgen, D., and Caracò, C. (2018). Lymph Node Metastasis in Melanoma: a Debate on the Significance of Nodal Metastases, Conditional Survival Analysis and Clinical Trials. Clin. Exp. Metastasis 35 (5-6), 431–442. doi:10.1007/s10585-018-9898-6
Felcht, M., Luck, R., Schering, A., Seidel, P., Srivastava, K., Hu, J., et al. (2012). Angiopoietin-2 Differentially Regulates Angiogenesis through TIE2 and Integrin Signaling. J. Clin. Invest. 122 (6), 1991–2005. doi:10.1172/JCI58832
Fidler, I. J., and Kripke, M. L. (2015). The challenge of Targeting Metastasis. Cancer Metastasis Rev. 34 (4), 635–641. doi:10.1007/s10555-015-9586-9
Fong, M. Y., Zhou, W., Liu, L., Alontaga, A. Y., Chandra, M., Ashby, J., et al. (2015). Breast-cancer-secreted miR-122 Reprograms Glucose Metabolism in Premetastatic Niche to Promote Metastasis. Nat. Cel Biol 17 (2), 183–194. doi:10.1038/ncb3094
Fu, Q., Zhang, Q., Lou, Y., Yang, J., Nie, G., Chen, Q., et al. (2018). Primary Tumor-Derived Exosomes Facilitate Metastasis by Regulating Adhesion of Circulating Tumor Cells via SMAD3 in Liver Cancer. Oncogene 37 (47), 6105–6118. doi:10.1038/s41388-018-0391-0
Fujimoto, T., Nakagawa, S., Morofuji, Y., Watanabe, D., Ujifuku, K., Horie, N., et al. (2020). Pericytes Suppress Brain Metastasis from Lung Cancer In Vitro. Cell Mol Neurobiol 40 (1), 113–121. doi:10.1007/s10571-019-00725-0
Fujita, I., Toyokawa, T., Makino, T., Matsueda, K., Omote, S., and Horii, J. (2020). Small Early Gastric Cancer with Synchronous Bone Metastasis: A Case Report. Mol. Clin. Onc 12 (3), 202–207. doi:10.3892/mco.2020.1985
García, S. A., Weitz, J., and Schölch, S. (2018). Circulating Tumor Cells. Methods Mol. Biol. 1692, 213–219. doi:10.1007/978-1-4939-7401-6_18
Garcia-Diaz, A., Shin, D. S., Moreno, B. H., Saco, J., Escuin-Ordinas, H., Rodriguez, G. A., et al. (2019). Interferon Receptor Signaling Pathways Regulating PD-L1 and PD-L2 Expression. Cel Rep. 29 (11), 3766. doi:10.1016/j.celrep.2019.11.113
Garnier, D., Magnus, N., Lee, T. H., Bentley, V., Meehan, B., Milsom, C., et al. (2012). Cancer Cells Induced to Express Mesenchymal Phenotype Release Exosome-like Extracellular Vesicles Carrying Tissue Factor. J. Biol. Chem. 287 (52), 43565–43572. doi:10.1074/jbc.M112.401760
Ginestra, A., Monea, S., Seghezzi, G., Dolo, V., Nagase, H., Mignatti, P., et al. (1997). Urokinase Plasminogen Activator and Gelatinases Are Associated with Membrane Vesicles Shed by Human HT1080 Fibrosarcoma Cells. J. Biol. Chem. 272 (27), 17216–17222. doi:10.1074/jbc.272.27.17216
Goetzl, E. J., Goetzl, L., Karliner, J. S., Tang, N., and Pulliam, L. (2016). Human Plasma Platelet‐derived Exosomes: Effects of Aspirin. FASEB j. 30 (5), 2058–2063. doi:10.1096/fj.201500150R
Greening, D. W., Gopal, S. K., Xu, R., Simpson, R. J., and Chen, W. (2015). Exosomes and Their Roles in Immune Regulation and Cancer. Semin. Cel Dev. Biol. 40, 72–81. doi:10.1016/j.semcdb.2015.02.009
Grivennikov, S. I., Greten, F. R., and Karin, M. (2010). Immunity, Inflammation, and Cancer. Cell 140 (6), 883–899. doi:10.1016/j.cell.2010.01.025
Grossniklaus, H. E. (2019). Understanding Uveal Melanoma Metastasis to the Liver: The Zimmerman Effect and the Zimmerman Hypothesis. Ophthalmology 126 (4), 483–487. doi:10.1016/j.ophtha.2018.09.031
Guan, Z., Lan, H., Chen, X., Jiang, X., Wang, X., and Jin, K. (2017). Individualized Drug Screening Based on Next Generation Sequencing and Patient Derived Xenograft Model for Pancreatic Cancer with Bone Metastasis. Mol. Med. Rep. 16 (4), 4784–4790. doi:10.3892/mmr.2017.7213
Guo, Y., Ji, X., Liu, J., Fan, D., Zhou, Q., Chen, C., et al. (2019). Effects of Exosomes on Pre-metastatic Niche Formation in Tumors. Mol. Cancer 18 (1), 39. doi:10.1186/s12943-019-0995-1
Han, Q., Lv, L., Wei, J., Lei, X., Lin, H., Li, G., et al. (2019). Vps4A Mediates the Localization and Exosome Release of β-catenin to Inhibit Epithelial-Mesenchymal Transition in Hepatocellular Carcinoma. Cancer Lett. 457, 47–59. doi:10.1016/j.canlet.2019.04.035
Hashimoto, K., Ochi, H., Sunamura, S., Kosaka, N., Mabuchi, Y., Fukuda, T., et al. (2018). Cancer-secreted Hsa-miR-940 Induces an Osteoblastic Phenotype in the Bone Metastatic Microenvironment via Targeting ARHGAP1 and FAM134A. Proc. Natl. Acad. Sci. USA 115 (9), 2204–2209. doi:10.1073/pnas.1717363115
He, J., Shi, J., Zhang, K., Xue, J., Li, J., Yang, J., et al. (2017). Sox2 Inhibits Wnt-β-Catenin Signaling and Metastatic Potency of Cisplatin-Resistant Lung Adenocarcinoma Cells. Mol. Med. Rep. 15 (4), 1693–1701. doi:10.3892/mmr.2017.6170
Hernández-Esquivel, M. A., Pérez-Torres, A., Romero-Romero, L., Reyes-Matute, A., Loaiza, B., Mellado-Sánchez, G., et al. (2018). The Dialyzable Leukocyte Extract TransferonTM Inhibits Tumor Growth and Brain Metastasis in a Murine Model of Prostate Cancer. Biomed. Pharmacother. 101, 938–944. doi:10.1016/j.biopha.2018.03.012
Hirooka, A., Tamiya, A., Kanazu, M., Nonaka, J., Yonezawa, T., Asami, K., et al. (2016). Brain Metastasis of Pleural Mesothelioma after a Subarachnoid Hemorrhage. Intern. Med. 55 (7), 779–781. doi:10.2169/internalmedicine.55.3765
Ho, T. T. B., Nasti, A., Seki, A., Komura, T., Inui, H., Kozaka, T., et al. (2020). Combination of Gemcitabine and Anti-PD-1 Antibody Enhances the Anticancer Effect of M1 Macrophages and the Th1 Response in a Murine Model of Pancreatic Cancer Liver Metastasis. J. Immunother. Cancer 8 (2), e001367. doi:10.1136/jitc-2020-001367
Hoffman, H. A., Li, C. H., Everson, R. G., Strunck, J. L., Yong, W. H., and Lu, D. C. (2017). Primary Lung Metastasis of Glioblastoma Multiforme with Epidural Spinal Metastasis: Case Report. J. Clin. Neurosci. 41, 97–99. doi:10.1016/j.jocn.2017.03.033
Hong, C.-S., Sharma, P., Yerneni, S. S., Simms, P., Jackson, E. K., Whiteside, T. L., et al. (2017). Circulating Exosomes Carrying an Immunosuppressive Cargo Interfere with Cellular Immunotherapy in Acute Myeloid Leukemia. Sci. Rep. 7 (1), 14684. doi:10.1038/s41598-017-14661-w
Hong, L., Qiu, H., Mei, Z., Zhang, H., Liu, S., and Cao, H. (2018). Ovarian Cancer Initially Presenting with Supra-clavicular L-ymph N-ode M-etastasis: A C-ase R-eport. Oncol. Lett. 16 (1), 505–510. doi:10.3892/ol.2018.8664
Hood, J. L., San, R. S., and Wickline, S. A. (2011). Exosomes Released by Melanoma Cells Prepare sentinel Lymph Nodes for Tumor Metastasis. Cancer Res. 71 (11), 3792–3801. doi:10.1158/0008-5472.CAN-10-4455
Hoshino, A., Costa-Silva, B., Shen, T.-L., Rodrigues, G., Hashimoto, A., Tesic Mark, M., et al. (2015). Tumour Exosome Integrins Determine Organotropic Metastasis. Nature 527 (7578), 329–335. doi:10.1038/nature15756
Hosonaga, M., Saya, H., and Arima, Y. (2020). Molecular and Cellular Mechanisms Underlying Brain Metastasis of Breast Cancer. Cancer Metastasis Rev. 39 (3), 711–720. doi:10.1007/s10555-020-09881-y
Hsieh, Y.-S., Chu, S.-C., Huang, S.-C., Kao, S.-H., Lin, M.-S., and Chen, P.-N. (2021). Gossypol Reduces Metastasis and Epithelial-Mesenchymal Transition by Targeting Protease in Human Cervical Cancer. Am. J. Chin. Med. 49 (1), 181–198. doi:10.1142/S0192415X21500105
Hsu, Y.-L., Hung, J.-Y., Chang, W.-A., Jian, S.-F., Lin, Y.-S., Pan, Y.-C., et al. (2018). Hypoxic Lung-Cancer-Derived Extracellular Vesicle MicroRNA-103a Increases the Oncogenic Effects of Macrophages by Targeting PTEN. Mol. Ther. 26 (2), 568–581. doi:10.1016/j.ymthe.2017.11.016
Hsu, Y.-L., Hung, J.-Y., Chang, W.-A., Lin, Y.-S., Pan, Y.-C., Tsai, P.-H., et al. (2017). Hypoxic Lung Cancer-Secreted Exosomal miR-23a Increased Angiogenesis and Vascular Permeability by Targeting Prolyl Hydroxylase and Tight junction Protein ZO-1. Oncogene 36 (34), 4929–4942. doi:10.1038/onc.2017.105
Hu, C., Yang, J., Huang, Z., Liu, C., Lin, Y., Tong, Y., et al. (2020). Diagnostic and Prognostic Nomograms for Bone Metastasis in Hepatocellular Carcinoma. BMC Cancer 20 (1), 494. doi:10.1186/s12885-020-06995-y
Hu, H.-Y., Yu, C.-H., Zhang, H.-H., Zhang, S.-Z., Yu, W.-Y., Yang, Y., et al. (2019). Exosomal miR-1229 Derived from Colorectal Cancer Cells Promotes Angiogenesis by Targeting HIPK2. Int. J. Biol. Macromolecules 132, 470–477. doi:10.1016/j.ijbiomac.2019.03.221
Hu, J. L., Wang, W., Lan, X. L., Zeng, Z. C., Liang, Y. S., Yan, Y. R., et al. (2019). CAFs Secreted Exosomes Promote Metastasis and Chemotherapy Resistance by Enhancing Cell Stemness and Epithelial-Mesenchymal Transition in Colorectal Cancer. Mol. Cancer 18 (1), 91. doi:10.1186/s12943-019-1019-x
Hu, Y., Li, D., Wu, A., Qiu, X., Di, W., Huang, L., et al. (2017). TWEAK-stimulated Macrophages Inhibit Metastasis of Epithelial Ovarian Cancer via Exosomal Shuttling of microRNA. Cancer Lett. 393, 60–67. doi:10.1016/j.canlet.2017.02.009
Huang, C.-S., Ho, J.-Y., Chiang, J.-H., Yu, C.-P., and Yu, D.-S. (2020a). Exosome-Derived LINC00960 and LINC02470 Promote the Epithelial-Mesenchymal Transition and Aggressiveness of Bladder Cancer Cells. Cells 9 (6), 1419. doi:10.3390/cells9061419
Huang, R., and Rofstad, E. K. (2018). Integrins as Therapeutic Targets in the Organ-specific Metastasis of Human Malignant Melanoma. J. Exp. Clin. Cancer Res. 37 (1), 92. doi:10.1186/s13046-018-0763-x
Huang, R., Wu, J., Zheng, Z., Wang, G., Song, D., Yan, P., et al. (2019). The Construction and Analysis of ceRNA Network and Patterns of Immune Infiltration in Mesothelioma with Bone Metastasis. Front. Bioeng. Biotechnol. 7, 257. doi:10.3389/fbioe.2019.00257
Huang, X.-Y., Huang, Z.-L., Huang, J., Xu, B., Huang, X.-Y., Xu, Y.-H., et al. (2020b). Exosomal circRNA-100338 Promotes Hepatocellular Carcinoma Metastasis via Enhancing Invasiveness and Angiogenesis. J. Exp. Clin. Cancer Res. 39 (1), 20. doi:10.1186/s13046-020-1529-9
Hwang, J. H., Yoo, H. J., Lim, M. C., Seo, S.-S., Kang, S., Kim, J.-Y., et al. (2013). Brain Metastasis in Patients with Uterine Cervical Cancer. J. Obstet. Gynaecol. Res. 39 (1), 287–291. doi:10.1111/j.1447-0756.2012.01927.x
Hyun, Y.-M., Seo, S.-U., Choi, W. S., Kwon, H.-J., Kim, D.-Y., Jeong, S., et al. (2020). Endogenous DEL-1 Restrains Melanoma Lung Metastasis by Limiting Myeloid Cell-Associated Lung Inflammation. Sci. Adv. 6 (45). doi:10.1126/sciadv.abc4882
Ikegami, T., Yoshizumi, T., Kawasaki, J., Nagatsu, A., Uchiyama, H., Harada, N., et al. (2017). Surgical Resection for Lymph Node Metastasis after Liver Transplantation for Hepatocellular Carcinoma. Ar 37 (2), 891–896. doi:10.21873/anticanres.11395
Imura, Y., Tateiwa, D., Sugimoto, N., Inoue, A., Wakamatsu, T., Outani, H., et al. (2020). Prognostic Factors and Skeletal-related E-vents in P-atients with B-one M-etastasis from G-astric C-ancer. Mol. Clin. Oncol. 13 (4), 31. doi:10.3892/mco.2020.2101
Jamjoom, A. B., Jamjoom, Z. A. B., Rahman, N.-U., and Al-Rikabi, A. C. (1997). Cervical Lymph Node Metastasis from a Glioblastoma Multiforme in a Child: Report of a Case and a Review of the Literature. Ann. Saudi Med. 17 (3), 340–343. doi:10.5144/0256-4947.1997.340
Ji, L., Fan, L., Zhu, X., Gao, Y., and Wang, Z. (2020). A Prognostic Model for Breast Cancer with Liver Metastasis. Front. Oncol. 10, 1342. doi:10.3389/fonc.2020.01342
Jiang, K., Dong, C., Yin, Z., Li, R., Mao, J., Wang, C., et al. (2020). Exosome-derived ENO1 Regulates Integrin α6β4 Expression and Promotes Hepatocellular Carcinoma Growth and Metastasis. Cel Death Dis 11 (11), 972. doi:10.1038/s41419-020-03179-1
Jie, G. N., Sing, W. C., Ching, C. Y., and Tiffany, fnm. (2011). Is Lymph Node Metastasis a Common Feature of Gastrointestinal Stromal Tumor? Clin. Nucl. Med. 36 (8), 678–682. doi:10.1097/RLU.0b013e318219ad31
Kalluri, R. (2016). The Biology and Function of Exosomes in Cancer. J. Clin. Invest. 126 (4), 1208–1215. doi:10.1172/JCI81135
Kanayama, T., Mabuchi, S., Shimura, K., Hisamatsu, T., Isohashi, F., Hamasaki, T., et al. (2015). Prognostic Factors for Survival in Cervical Cancer Patients with Bone Metastasis. Eur. J. Gynaecol. Oncol. 36 (3), 290–293.
Kanda, M., Fujii, T., Nagai, S., Kodera, Y., Kanzaki, A., Sahin, T. T., et al. (2011). Pattern of Lymph Node Metastasis Spread in Pancreatic Cancer. Pancreas 40 (6), 951–955. doi:10.1097/MPA.0b013e3182148342
Kapoor, H., Sanampudi, S., Owen, J., and Raissi, D. (2020). Lung Metastasis Postradioembolization of Hepatocellular Carcinoma with Tumor in Vein. ACG Case Rep. J. 7 (2), e00322. doi:10.14309/crj.0000000000000322
Katona, F., Murnyák, B., Marko-Varga, G., and Hortobágyi, T. (2017). A Melanoma És Az Agyi Áttétképződés Molekuláris Háttere. Orvosi Hetilap 158 (28), 1083–1091. doi:10.1556/650.2017.30793
Kawai, A., Nagasaka, Y., Muraki, M., Fukuoka, M., Satou, T., Kimura, M., et al. (1997). Brain Metastasis in Malignant Pleural Mesothelioma. Intern. Med. 36 (8), 591–594. doi:10.2169/internalmedicine.36.591
Khan, S., Aspe, J. R., Asumen, M. G., Almaguel, F., Odumosu, O., Acevedo-Martinez, S., et al. (2009). Extracellular, Cell-Permeable Survivin Inhibits Apoptosis while Promoting Proliferative and Metastatic Potential. Br. J. Cancer 100 (7), 1073–1086. doi:10.1038/sj.bjc.6604978
Khan, S., Jutzy, J. M. S., Aspe, J. R., McGregor, D. W., Neidigh, J. W., and Wall, N. R. (2011). Survivin Is Released from Cancer Cells via Exosomes. Apoptosis 16 (1), 1–12. doi:10.1007/s10495-010-0534-4
Kim, E.-K., Song, M.-J., Jung, Y., Lee, W.-S., and Jang, H. H. (2019a). Proteomic Analysis of Primary Colon Cancer and Synchronous Solitary Liver Metastasis. Cancer Genomics Proteomics 16 (6), 583–592. doi:10.21873/cgp.20161
Kim, H., Lee, K. K., Heo, M. H., and Kim, J. Y. (2019b). The Prognostic Factors Influencing Overall Survival in Uterine Cervical Cancer with Brain Metastasis. Korean J. Intern. Med. 34 (6), 1324–1332. doi:10.3904/kjim.2018.051
Kim, J., Piao, H.-L., Kim, B.-J., Yao, F., Han, Z., Wang, Y., et al. (2018). Long Noncoding RNA MALAT1 Suppresses Breast Cancer Metastasis. Nat. Genet. 50 (12), 1705–1715. doi:10.1038/s41588-018-0252-3
Kim, J. W., Wieckowski, E., Taylor, D. D., Reichert, T. E., Watkins, S., and Whiteside, T. L. (2005). Fas Ligand-Positive Membranous Vesicles Isolated from Sera of Patients with Oral Cancer Induce Apoptosis of Activated T Lymphocytes. Clin. Cancer Res. 11 (3), 1010–1020. doi:10.1158/1078-0432.ccr-05-1346
Kim, R., Song, J., and Kim, S. B. (2020). Concurrent Hepatocellular Carcinoma Metastasis to Stomach, colon, and Brain: A Case Report. Wjcc 8 (16), 3534–3541. doi:10.12998/wjcc.v8.i16.3534
Kim, S., Bae, W. J., Ahn, J. M., Heo, J.-H., Kim, K.-M., Choi, K. W., et al. (2021). MicroRNA Signatures Associated with Lymph Node Metastasis in Intramucosal Gastric Cancer. Mod. Pathol. 34 (3), 672–683. doi:10.1038/s41379-020-00681-x
Kim, W., Seok Kang, Y., Soo Kim, J., Shin, N.-Y., Hanks, S. K., and Song, W. K. (2008). The Integrin-Coupled Signaling Adaptor p130Cas Suppresses Smad3 Function in Transforming Growth Factor-β Signaling. MBoC 19 (5), 2135–2146. doi:10.1091/mbc.E07-10-0991
Kircher, D., Silvis, M., Cho, J., and Holmen, S. (2016). Melanoma Brain Metastasis: Mechanisms, Models, and Medicine. Ijms 17 (9), 1468. doi:10.3390/ijms17091468
Klemke, M., Weschenfelder, T., Konstandin, M. H., and Samstag, Y. (2007). High Affinity Interaction of Integrin α4β1 (VLA-4) and Vascular Cell Adhesion Molecule 1 (VCAM-1) Enhances Migration of Human Melanoma Cells across Activated Endothelial Cell Layers. J. Cel. Physiol. 212 (2), 368–374. doi:10.1002/jcp.21029
Kleppe, M., Wang, T., Van Gorp, T., Slangen, B. F. M., Kruse, A. J., and Kruitwagen, R. F. P. M. (2011). Lymph Node Metastasis in Stages I and II Ovarian Cancer: a Review. Gynecol. Oncol. 123 (3), 610–614. doi:10.1016/j.ygyno.2011.09.013
Klingenberg, S., Jochumsen, M. R., Ulhøi, B. P., Fredsøe, J., Sørensen, K. D., Borre, M., et al. (2021). 68Ga-PSMA PET/CT for Primary Lymph Node and Distant Metastasis NM Staging of High-Risk Prostate Cancer. J. Nucl. Med. 62 (2), 214–220. doi:10.2967/jnumed.120.245605
Kofler, B., Kerschbaumer, J., Schartinger, V. H., Posch, A., Gizewski, E. R., and Widmann, G. (2017). Hirnmetastase versus Strahlennekrose nach Kopf-Hals-Karzinom. Radiologe 57 (5), 392–396. doi:10.1007/s00117-017-0219-x
Kogure, T., Lin, W.-L., Yan, I. K., Braconi, C., and Patel, T. (2011). Intercellular Nanovesicle-Mediated microRNA Transfer: a Mechanism of Environmental Modulation of Hepatocellular Cancer Cell Growth. Hepatology 54 (4), 1237–1248. doi:10.1002/hep.24504
Kong, J., Tian, H., Zhang, F., Zhang, Z., Li, J., Liu, X., et al. (2019). Extracellular Vesicles of Carcinoma-Associated Fibroblasts Creates a Pre-metastatic Niche in the Lung through Activating Fibroblasts. Mol. Cancer 18 (1), 175. doi:10.1186/s12943-019-1101-4
Kong, M., Jin, J., Cai, X., Shen, J., Ma, D., Ye, M., et al. (2017). Characteristics of Lymph Node Metastasis in Resected Adenosquamous Lung Cancer. Medicine (Baltimore) 96 (48), e8870. doi:10.1097/MD.0000000000008870
Kong, X., Zhang, J., Li, J., Shao, J., and Fang, L. (2018). MiR-130a-3p Inhibits Migration and Invasion by Regulating RAB5B in Human Breast Cancer Stem Cell-like Cells. Biochem. Biophysical Res. Commun. 501 (2), 486–493. doi:10.1016/j.bbrc.2018.05.018
Kopp, H.-G., Placke, T., and Salih, H. R. (2009). Platelet-Derived Transforming Growth Factor-β Down-Regulates NKG2D Thereby Inhibiting Natural Killer Cell Antitumor Reactivity. Cancer Res. 69 (19), 7775–7783. doi:10.1158/0008-5472.CAN-09-2123
Kosaka, N., Iguchi, H., Hagiwara, K., Yoshioka, Y., Takeshita, F., and Ochiya, T. (2013). Neutral Sphingomyelinase 2 (nSMase2)-dependent Exosomal Transfer of Angiogenic microRNAs Regulate Cancer Cell Metastasis. J. Biol. Chem. 288 (15), 10849–10859. doi:10.1074/jbc.M112.446831
Kubo, N., and Takeuchi, N. (2017). Gastrointestinal Stromal Tumor of the Stomach with Axillary Lymph Node Metastasis: A Case Report. Wjg 23 (9), 1720–1724. doi:10.3748/wjg.v23.i9.1720
Kucharzewska, P., Christianson, H. C., Welch, J. E., Svensson, K. J., Fredlund, E., Ringner, M., et al. (2013). Exosomes Reflect the Hypoxic Status of Glioma Cells and Mediate Hypoxia-dependent Activation of Vascular Cells during Tumor Development. Proc. Natl. Acad. Sci. 110 (18), 7312–7317. doi:10.1073/pnas.1220998110
Kumar, L., Bhargava, V. L., Rao, R. C. K., Rath, G. K., and Kataria, S. P. (1992). Bone Metastasis in Ovarian Cancer. Asia Oceania J. Obstet. Gynaecol. 18 (4), 309–313. doi:10.1111/j.1447-0756.1992.tb00324.x
Kuo, S.-W., Ke, F.-C., Chang, G.-D., Lee, M.-T., and Hwang, J.-J. (2011). Potential Role of Follicle-Stimulating Hormone (FSH) and Transforming Growth Factor (TGFβ1) in the Regulation of Ovarian Angiogenesis. J. Cel. Physiol. 226 (6), 1608–1619. doi:10.1002/jcp.22491
Kuznetsov, H. S., Marsh, T., Markens, B. A., Castaño, Z., Greene-Colozzi, A., Hay, S. A., et al. (2012). Identification of Luminal Breast Cancers that Establish a Tumor-Supportive Macroenvironment Defined by Proangiogenic Platelets and Bone Marrow-Derived Cells. Cancer Discov. 2 (12), 1150–1165. doi:10.1158/2159-8290.CD-12-0216
Lal, P., Tiwari, A., Kumar, N., and Bajpai, R. (2007). Bone Metastasis from Ovarian Cancer. J. Can. Res. Ther. 3 (1), 34–36. doi:10.4103/0973-1482.31969
Lambert, A. W., Pattabiraman, D. R., and Weinberg, R. A. (2017). Emerging Biological Principles of Metastasis. Cell 168 (4), 670–691. doi:10.1016/j.cell.2016.11.037
Lan, J., Sun, L., Xu, F., Liu, L., Hu, F., Song, D., et al. (2019). M2 Macrophage-Derived Exosomes Promote Cell Migration and Invasion in Colon Cancer. Cancer Res. 79 (1), 146–158. doi:10.1158/0008-5472.CAN-18-0014
Lang, H.-L., Hu, G.-W., Zhang, B., Kuang, W., Chen, Y., Wu, L., et al. (2017b). Glioma Cells Enhance Angiogenesis and Inhibit Endothelial Cell Apoptosis through the Release of Exosomes that Contain Long Non-coding RNA CCAT2. Oncol. Rep. 38 (2), 785–798. doi:10.3892/or.2017.5742
Lang, H. L., Hu, G. W., Chen, Y., Liu, Y., Tu, W., Lu, Y. M., et al. (2017a). Glioma Cells Promote Angiogenesis through the Release of Exosomes Containing Long Non-coding RNA POU3F3. Eur. Rev. Med. Pharmacol. Sci. 21 (5), 959–972.
Laurini, R. N. (1974). Diffuse Pleural Mesothelioma with Distant Bone Metastasis. Acta Pathol. Microbiol. Scand. A. 82A (2), 296–298. doi:10.1111/j.1699-0463.1974.tb03854.x
Lázaro‐Ibáñez, E., Sanz‐Garcia, A., Visakorpi, T., Escobedo‐Lucea, C., Siljander, P., Ayuso‐Sacido, Á., et al. (2014). Different gDNA Content in the Subpopulations of Prostate Cancer Extracellular Vesicles: Apoptotic Bodies, Microvesicles, and Exosomes. Prostate 74 (14), 1379–1390. doi:10.1002/pros.22853
Lee, J.-K., Park, S.-R., Jung, B.-K., Jeon, Y.-K., Lee, Y.-S., Kim, M.-K., et al. (2013b). Exosomes Derived from Mesenchymal Stem Cells Suppress Angiogenesis by Down-Regulating VEGF Expression in Breast Cancer Cells. PLoS One 8 (12), e84256. doi:10.1371/journal.pone.0084256
Lee, J., Kim, K. E., Choi, D.-K., Jang, J. Y., Jung, J.-J., Kiyonari, H., et al. (2013a). Angiopoietin-1 Guides Directional Angiogenesis through Integrin α V β 5 Signaling for Recovery of Ischemic Retinopathy. Sci. Transl. Med. 5 (203), 203ra127. doi:10.1126/scitranslmed.3006666
Leight, J. L., Wozniak, M. A., Chen, S., Lynch, M. L., and Chen, C. S. (2012). Matrix Rigidity Regulates a Switch between TGF-Β1-Induced Apoptosis and Epithelial-Mesenchymal Transition. MBoC 23 (5), 781–791. doi:10.1091/mbc.E11-06-0537
Leitinger, B. (2011). Transmembrane Collagen Receptors. Annu. Rev. Cel Dev. Biol. 27, 265–290. doi:10.1146/annurev-cellbio-092910-154013
Lemke, J., Scheele, J., Kapapa, T., Wirtz, C., Henne-Bruns, D., and Kornmann, M. (2013). Brain Metastasis in Pancreatic Cancer. Ijms 14 (2), 4163–4173. doi:10.3390/ijms14024163
Levental, K. R., Yu, H., Kass, L., Lakins, J. N., Egeblad, M., Erler, J. T., et al. (2009). Matrix Crosslinking Forces Tumor Progression by Enhancing Integrin Signaling. Cell 139 (5), 891–906. doi:10.1016/j.cell.2009.10.027
Li, B., Mao, R., Liu, C., Zhang, W., Tang, Y., and Guo, Z. (2018). LncRNA FAL1 Promotes Cell Proliferation and Migration by Acting as a CeRNA of miR-1236 in Hepatocellular Carcinoma Cells. Life Sci. 197, 122–129. doi:10.1016/j.lfs.2018.02.006
Li, F., Dai, Y., Xu, H., Huang, K., Zhou, Y., Luo, D., et al. (2019). XPNPEP2 Is Associated with Lymph Node Metastasis in Prostate Cancer Patients. Sci. Rep. 9 (1), 10078. doi:10.1038/s41598-019-45245-5
Li, J., Li, Z., Jiang, P., Peng, M., Zhang, X., Chen, K., et al. (2018). Circular RNA IARS (Circ-IARS) Secreted by Pancreatic Cancer Cells and Located within Exosomes Regulates Endothelial Monolayer Permeability to Promote Tumor Metastasis. J. Exp. Clin. Cancer Res. 37 (1), 177. doi:10.1186/s13046-018-0822-3
Li, K., Chen, Y., Li, A., Tan, C., and Liu, X. (2019). Exosomes Play Roles in Sequential Processes of Tumor Metastasis. Int. J. Cancer 144 (7), 1486–1495. doi:10.1002/ijc.31774
Li, R., Wang, Y., Zhang, X., Feng, M., Ma, J., Li, J., et al. (2019). Exosome-mediated Secretion of LOXL4 Promotes Hepatocellular Carcinoma Cell Invasion and Metastasis. Mol. Cancer 18 (1), 18. doi:10.1186/s12943-019-0948-8
Li, Y., Li, Q., Li, D., Gu, J., Qian, D., Qin, X., et al. (2021). Exosome Carrying PSGR Promotes Stemness and Epithelial-Mesenchymal Transition of Low Aggressive Prostate Cancer Cells. Life Sci. 264, 118638. doi:10.1016/j.lfs.2020.118638
Li, Y., Liang, Y., Sang, Y., Song, X., Zhang, H., Liu, Y., et al. (2018). MiR-770 Suppresses the Chemo-Resistance and Metastasis of Triple Negative Breast Cancer via Direct Targeting of STMN1. Cel Death Dis 9 (1), 14. doi:10.1038/s41419-017-0030-7
Li, Y., Xi, Y., Zhu, G., Jia, J., Huang, H., Liu, Y., et al. (2019). Downregulated IGFBP7 Facilitates Liver Metastasis by Modulating Epithelial-mesenchymal T-ransition in colon C-ancer. Oncol. Rep. 42 (5), 1935–1945. doi:10.3892/or.2019.7303
Li, Z., Jiang, P., Li, J., Peng, M., Zhao, X., Zhang, X., et al. (2018). Tumor-derived Exosomal Lnc-Sox2ot Promotes EMT and Stemness by Acting as a ceRNA in Pancreatic Ductal Adenocarcinoma. Oncogene 37 (28), 3822–3838. doi:10.1038/s41388-018-0237-9
Lin, W.-H., Chang, Y.-W., Hong, M.-X., Hsu, T.-C., Lee, K.-C., Lin, C., et al. (2021). STAT3 Phosphorylation at Ser727 and Tyr705 Differentially Regulates the EMT-MET Switch and Cancer Metastasis. Oncogene 40 (4), 791–805. doi:10.1038/s41388-020-01566-8
Lin, Y., Huang, S.-T., Jiang, Y.-M., and Pan, X.-B. (2017). Hemorrhage of Brain Metastasis Is a Poor Prognostic Factor in Hepatocellular Carcinoma Patients. Oncotarget 8 (54), 93245–93250. doi:10.18632/oncotarget.21449
Liu, D., Kang, H., Gao, M., Jin, L., Zhang, F., Chen, D., et al. (2020). Exosome‐transmitted circ_MMP2 Promotes Hepatocellular Carcinoma Metastasis by Upregulating MMP2. Mol. Oncol. 14 (6), 1365–1380. doi:10.1002/1878-0261.12637
Liu, H., Ye, X., Li, D., Yao, Q., and Li, Y. (2021). Incidence, Clinical Risk and Prognostic Factors for Liver Metastasis in Patients with Cervical Cancer: a Population-Based Retrospective Study. BMC Cancer 21 (1), 421. doi:10.1186/s12885-021-08127-6
Liu, J., and Agarwal, S. (2010). Mechanical Signals Activate Vascular Endothelial Growth Factor Receptor-2 to Upregulate Endothelial Cell Proliferation during Inflammation. J.I. 185 (2), 1215–1221. doi:10.4049/jimmunol.0903660
Liu, T., Zhang, X., Du, L., Wang, Y., Liu, X., Tian, H., et al. (2019). Exosome-transmitted miR-128-3p Increase Chemosensitivity of Oxaliplatin-Resistant Colorectal Cancer. Mol. Cancer 18 (1), 43. doi:10.1186/s12943-019-0981-7
Liu, Y., and Cao, X. (2016). Characteristics and Significance of the Pre-metastatic Niche. Cancer Cell 30 (5), 668–681. doi:10.1016/j.ccell.2016.09.011
Liu, Y., Gu, Y., Han, Y., Zhang, Q., Jiang, Z., Zhang, X., et al. (2016a). Tumor Exosomal RNAs Promote Lung Pre-metastatic Niche Formation by Activating Alveolar Epithelial TLR3 to Recruit Neutrophils. Cancer Cell 30 (2), 243–256. doi:10.1016/j.ccell.2016.06.021
Liu, Y., Luo, F., Wang, B., Li, H., Xu, Y., Liu, X., et al. (2016b). STAT3-regulated Exosomal miR-21 Promotes Angiogenesis and Is Involved in Neoplastic Processes of Transformed Human Bronchial Epithelial Cells. Cancer Lett. 370 (1), 125–135. doi:10.1016/j.canlet.2015.10.011
Liu, Y., Ren, W., Bai, Y., Wan, L., Sun, X., Liu, Y., et al. (2018a). Oxyresveratrol Prevents Murine H22 Hepatocellular Carcinoma Growth and Lymph Node Metastasis via Inhibiting Tumor Angiogenesis and Lymphangiogenesis. J. Nat. Med. 72 (2), 481–492. doi:10.1007/s11418-018-1173-2
Liu, Y., Wu, T., Lu, D., Zhen, J., and Zhang, L. (2018b). CD44 Overexpression Related to Lymph Node Metastasis and Poor Prognosis of Pancreatic Cancer. Int. J. Biol. Markers 33 (3), 308–313. doi:10.1177/1724600817746951
Liu, Z.-L., Wang, C., Chen, H.-J., Li, X., Dai, L.-J., and Ding, Z.-Y. (2017). Bone Metastasis from Lung Cancer Identified by Genetic Profiling. Oncol. Lett. 13 (2), 847–850. doi:10.3892/ol.2016.5458
Lo Cicero, A., Majkowska, I., Nagase, H., Di Liegro, I., and Troeberg, L. (2012). Microvesicles Shed by Oligodendroglioma Cells and Rheumatoid Synovial Fibroblasts Contain Aggrecanase Activity. Matrix Biol. 31 (4), 229–233. doi:10.1016/j.matbio.2012.02.005
Longo, R., Bastien, C., Campitiello, M., Plastino, F., and Rozzi, A. (2020). Breast and Axillary Lymph Node Metastasis from Ovarian Cancer: A Case Report. Am. J. Case Rep. 21, e925089. doi:10.12659/AJCR.925089
Lu, Y.-J., Yang, Y., Yuan, Y.-H., Wang, W.-J., Cui, M.-T., Tang, H.-Y., et al. (2020). A Novel Nomogram Based on SEER Database for the Prediction of Liver Metastasis in Patients with Small-Cell Lung Cancer. Ann. Palliat. Med. 9 (5), 3123–3137. doi:10.21037/apm-20-886
Luan, W., Ding, Y., Xi, H., Ruan, H., Lu, F., Ma, S., et al. (2021). Exosomal miR-106b-5p Derived from Melanoma Cell Promotes Primary Melanocytes Epithelial-Mesenchymal Transition through Targeting EphA4. J. Exp. Clin. Cancer Res. 40 (1), 107. doi:10.1186/s13046-021-01906-w
Ludwig, N., and Whiteside, T. L. (2018). Potential Roles of Tumor-Derived Exosomes in Angiogenesis. Expert Opin. Ther. Targets 22 (5), 409–417. doi:10.1080/14728222.2018.1464141
Ludwig, S., Floros, T., Theodoraki, M.-N., Hong, C.-S., Jackson, E. K., Lang, S., et al. (2017). Suppression of Lymphocyte Functions by Plasma Exosomes Correlates with Disease Activity in Patients with Head and Neck Cancer. Clin. Cancer Res. 23 (16), 4843–4854. doi:10.1158/1078-0432.CCR-16-2819
Luga, V., Zhang, L., Viloria-Petit, A. M., Ogunjimi, A. A., Inanlou, M. R., Chiu, E., et al. (2012). Exosomes Mediate Stromal Mobilization of Autocrine Wnt-PCP Signaling in Breast Cancer Cell Migration. Cell 151 (7), 1542–1556. doi:10.1016/j.cell.2012.11.024
Luo, K.-J., Chen, C.-X., Yang, J.-P., Huang, Y.-C., Cardenas, E. R., and Jiang, J. X. (2020a). Connexins in Lung Cancer and Brain Metastasis. Front. Oncol. 10, 599383. doi:10.3389/fonc.2020.599383
Luo, Y., Ma, J., Liu, F., Guo, J., and Gui, R. (2020b). Diagnostic Value of Exosomal circMYC in Radioresistant Nasopharyngeal Carcinoma. Head & Neck 42 (12), 3702–3711. doi:10.1002/hed.26441
Luo, Z., Rong, Z., and Huang, C. (2019). Surgery Strategies for Gastric Cancer with Liver Metastasis. Front. Oncol. 9, 1353. doi:10.3389/fonc.2019.01353
Ma, B., Wells, A., Wei, L., and Zheng, J. (2021). Prostate Cancer Liver Metastasis: Dormancy and Resistance to Therapy. Semin. Cancer Biol. 71, 2–9. doi:10.1016/j.semcancer.2020.07.004
Ma, J., Zhang, J., Weng, Y. C., and Wang, J. C. (2018). EZH2-Mediated microRNA-139-5p Regulates Epithelial-Mesenchymal Transition and Lymph Node Metastasis of Pancreatic Cancer. Mol. Cell 41 (9), 868–880. doi:10.14348/molcells.2018.0109
Ma, R., Ye, X., Cheng, H., Cui, H., and Chang, X. (2021). Tumor-derived Exosomal circRNA051239 Promotes Proliferation and Migration of Epithelial Ovarian Cancer. Am. J. Transl Res. 13 (3), 1125–1139.
Ma, R.-Y., Zhang, H., Li, X.-F., Zhang, C.-B., Selli, C., Tagliavini, G., et al. (2020a). Monocyte-derived Macrophages Promote Breast Cancer Bone Metastasis Outgrowth. J. Exp. Med. 217 (11). doi:10.1084/jem.20191820
Ma, R., Feng, Y., Lin, S., Chen, J., Lin, H., Liang, X., et al. (2015). Mechanisms Involved in Breast Cancer Liver Metastasis. J. Transl Med. 13, 64. doi:10.1186/s12967-015-0425-0
Ma, Z.-j., Wang, Y., Li, H.-f., Liu, M.-H., Bi, F.-r., Ma, L., et al. (2020b). LncZEB1-AS1 Regulates Hepatocellular Carcinoma Bone Metastasis via Regulation of the miR-302b-EGFR-Pi3k-AKT axis. J. Cancer 11 (17), 5118–5128. doi:10.7150/jca.45995
Mahabeleshwar, G. H., Chen, J., Feng, W., Somanath, P. R., Razorenova, O. V., and Byzova, T. V. (2008). Integrin Affinity Modulation in Angiogenesis. Cell Cycle 7 (3), 335–347. doi:10.4161/cc.7.3.5234
Mahabeleshwar, G. H., Feng, W., Reddy, K., Plow, E. F., and Byzova, T. V. (2007). Mechanisms of Integrin-Vascular Endothelial Growth Factor Receptor Cross-Activation in Angiogenesis. Circ. Res. 101 (6), 570–580. doi:10.1161/CIRCRESAHA.107.155655
Makino, H., Nishio, S., Tsubamoto, H., Shimada, M., Nishikawa, R., Kai, K., et al. (2016). Treatment and Prognosis of Bone Metastasis from Cervical Cancer (KCOG-G1202s). J. Obstet. Gynaecol. Res. 42 (6), 701–706. doi:10.1111/jog.12956
Malla, R. R., Pandrangi, S., Kumari, S., Gavara, M. M., and Badana, A. K. (2018). Exosomal Tetraspanins as Regulators of Cancer Progression and Metastasis and Novel Diagnostic Markers. Asia-pac J. Clin. Oncol. 14 (6), 383–391. doi:10.1111/ajco.12869
Mannavola, F., Mandala, M., Todisco, A., Sileni, V. C., Palla, M., Minisini, A. M., et al. (2020). An Italian Retrospective Survey on Bone Metastasis in Melanoma: Impact of Immunotherapy and Radiotherapy on Survival. Front. Oncol. 10, 1652. doi:10.3389/fonc.2020.01652
Marchand Crety, C., Ettalhaoui, L., and Servagi Vernat, S. (2020). Cystic Brain Metastasis from Prostate Cancer: A Case Report and Literature Review. Urol. Case Rep. 32, 101219. doi:10.1016/j.eucr.2020.101219
Marzullo, A., Serio, G., Pezzuto, F., Fortarezza, F., Cazzato, G., Caporusso, C., et al. (2020). A Single Liver Metastasis from Pleural Biphasic Mesothelioma. Diagnostics 10 (8), 555. doi:10.3390/diagnostics10080555
Matsumoto, H., and Yoshida, Y. (2015). Brain Metastasis from Pancreatic Cancer: A Case Report and Literature Review. Asian J. Neurosurg. 10 (1), 35–39. doi:10.4103/1793-5482.151507
Matsumura, T., Ohzato, H., Yamamoto, T., Ota, K., Mabuchi, E., Miwa, H., et al. (2009). A Case of Postoperative Brain Metastasis Originated from Pancreatic Cancer Which Was Successfully Treated by Resection and Postoperative Irradiation. Gan To Kagaku Ryoho 36 (12), 2433–2435.
Mauri, C., and Bosma, A. (2012). Immune Regulatory Function of B Cells. Annu. Rev. Immunol. 30, 221–241. doi:10.1146/annurev-immunol-020711-074934
McCarthy, D. H. (2001). Letters to the Editor. Prog. Transpl. 11 (3), 162. doi:10.7182/prtr.11.3.hh6665126211678310.1177/152692480101100302
Merkow, J., Paniccia, A., Jones, E., Jones, T., Hodges, M., Stovall, R., et al. (2016). Association of sentinel Lymph Node Diameter with Melanoma Metastasis. Am. J. Surg. 212 (2), 315–320. doi:10.1016/j.amjsurg.2015.09.022
Mermod, M., Bongiovanni, M., Petrova, T., Goun, E., Simon, C., Tolstonog, G., et al. (2019). Prediction of Occult Lymph Node Metastasis in Head and Neck Cancer with CD31 Vessel Quantification. Otolaryngol. Head Neck Surg. 160 (2), 277–283. doi:10.1177/0194599818791779
Mikami, J., Kimura, Y., Makari, Y., Fujita, J., Kishimoto, T., Sawada, G., et al. (2017). Clinical Outcomes and Prognostic Factors for Gastric Cancer Patients with Bone Metastasis. World J. Surg. Onc 15 (1), 8. doi:10.1186/s12957-016-1091-2
Minciacchi, V. R., Freeman, M. R., and Di Vizio, D. (2015). Extracellular Vesicles in Cancer: Exosomes, Microvesicles and the Emerging Role of Large Oncosomes. Semin. Cel Dev. Biol. 40, 41–51. doi:10.1016/j.semcdb.2015.02.010
Moy, A. P., Mochel, M. C., Muzikansky, A., Duncan, L. M., and Kraft, S. (2017). Lymphatic Invasion Predicts sentinel Lymph Node Metastasis and Adverse Outcome in Primary Cutaneous Melanoma. J. Cutan. Pathol. 44 (9), 734–739. doi:10.1111/cup.12969
Muhsin-Sharafaldine, M.-R., Saunderson, S. C., Dunn, A. C., and McLellan, A. D. (2017). Melanoma Growth and Lymph Node Metastasis Is Independent of Host CD169 Expression. Biochem. Biophysical Res. Commun. 486 (4), 965–970. doi:10.1016/j.bbrc.2017.03.138
Nabet, B. Y., Qiu, Y., Shabason, J. E., Wu, T. J., Yoon, T., Kim, B. C., et al. (2017). Exosome RNA Unshielding Couples Stromal Activation to Pattern Recognition Receptor Signaling in Cancer. Cell 170 (2), 352 e313–366. doi:10.1016/j.cell.2017.06.031
Nagata, A., Kanemasa, Y., Kikuchi, M., Otani, R., Yamada, R., Motoi, T., et al. (2020). Bone Marrow Metastasis of Glioblastoma Multiforme Mimicking Acute Myeloid Leukemia. Oxf Med. Case Rep. 2020 (6), omaa040. doi:10.1093/omcr/omaa040
Nagy, J. A., and Dvorak, H. F. (2012). Heterogeneity of the Tumor Vasculature: the Need for New Tumor Blood Vessel Type-specific Targets. Clin. Exp. Metastasis 29 (7), 657–662. doi:10.1007/s10585-012-9500-6
Nakamara, N., Matsui, T., Ishibashi, Y., Sotokawauchi, A., Fukami, K., Higashimoto, Y., et al. (2017b). RAGE-aptamer Attenuates the Growth and Liver Metastasis of Malignant Melanoma in Nude Mice. Mol. Med. 23, 295–306. doi:10.2119/molmed.2017.00099
Nakamura, K., Sawada, K., Kinose, Y., Yoshimura, A., Toda, A., Nakatsuka, E., et al. (2017a). Exosomes Promote Ovarian Cancer Cell Invasion through Transfer of CD44 to Peritoneal Mesothelial Cells. Mol. Cancer Res. 15 (1), 78–92. doi:10.1158/1541-7786.MCR-16-0191
Nam, H. C., Sung, P. S., Song, D. S., Kwon, J. H., Nam, S. W., Yoon, D. J., et al. (2019). Control of Intracranial Disease Is Associated with Improved Survival in Patients with Brain Metastasis from Hepatocellular Carcinoma. Int. J. Clin. Oncol. 24 (6), 666–676. doi:10.1007/s10147-019-01407-z
Namburi, S., Broxmeyer, H. E., Hong, C.-S., Whiteside, T. L., and Boyiadzis, M. (2021). DPP4+ Exosomes in AML Patients' Plasma Suppress Proliferation of Hematopoietic Progenitor Cells. Leukemia 35 (7), 1925–1932. doi:10.1038/s41375-020-01047-7
Nance, M. E., Biedermann, G. B., Bhat, A. P., and Davis, R. M. (2020). Chemorefractory Liver Metastasis from Cervical Cancer Successfully Treated with a Combination of Yttrium-90 and Immunotherapy. Radiol. Case Rep. 15 (8), 1359–1365. doi:10.1016/j.radcr.2020.06.010
Naoe, H., Kaku, E., Ido, Y., Gushima, R., Maki, Y., Saito, H., et al. (2011). Brain Metastasis from Gastrointestinal Stromal Tumor: a Case Report and Review of the Literature. Case Rep. Gastroenterol. 5 (3), 583–589. doi:10.1159/000333403
Nartthanarung, A., Thanapprapasr, K., Udomsubpayakul, U., and Thanapprapasr, D. (2014). Age and Survival of Cervical Cancer Patients with Bone Metastasis. Asian Pac. J. Cancer Prev. 15 (19), 8401–8404. doi:10.7314/apjcp.2014.15.19.8401
Nguyen, D. X., Bos, P. D., and Massagué, J. (2009). Metastasis: from Dissemination to Organ-specific Colonization. Nat. Rev. Cancer 9 (4), 274–284. doi:10.1038/nrc2622
Nienstedt, J. C., Schroeder, C., Clauditz, T., Simon, R., Sauter, G., Muenscher, A., et al. (2018). EZH2 Overexpression in Head and Neck Cancer Is Related to Lymph Node Metastasis. J. Oral Pathol. Med. 47 (3), 240–245. doi:10.1111/jop.12673
Nieswandt, B., Hafner, M., Echtenacher, B., and Männel, D. N. (1999). Lysis of Tumor Cells by Natural Killer Cells in Mice Is Impeded by Platelets. Cancer Res. 59 (6), 1295–1300.
Nishino, H., Abe, K., Igarashi, M., Miyata, M., and Kitamura, K. (1996). Chemotherapy with Low-Dose Consecutive Cisplatin (Cddp) for Patients with Recurrent and Distant Metastasis of Head and Neck Cancer. Nippon Jibiinkoka Gakkai Kaiho 99 (9), 1218–1222. doi:10.3950/jibiinkoka.99.1218
Nishio, N., van den Berg, N. S., Martin, B. A., van Keulen, S., Fakurnejad, S., Rosenthal, E. L., et al. (2021). Photoacoustic Molecular Imaging for the Identification of Lymph Node Metastasis in Head and Neck Cancer Using an Anti-EGFR Antibody-Dye Conjugate. J. Nucl. Med. 62 (5), 648–655. doi:10.2967/jnumed.120.245241
Ohara, H., Ishibashi, Y., Yoshimura, S., Yamazaki, R., Hatao, F., Koshiishi, T., et al. (2020). Intratumoral Pseudoaneurysm within a Liver Metastasis of Gastric Cancer: a Case Report. Surg. Case Rep. 6 (1), 39. doi:10.1186/s40792-020-00806-z
Okabe, H., Aoki, K., Yogosawa, S., Saito, M., Marumo, K., and Yoshida, K. (2018). Downregulation of CD24 Suppresses Bone Metastasis of Lung Cancer. Cancer Sci. 109 (1), 112–120. doi:10.1111/cas.13435
Outani, H., Akita, H., Nakai, T., Takada, R., Imura, Y., Tanaka, T., et al. (2018). Clinical Features and Prognosis of Patients with the Bone Metastasis of Pancreatic Cancer. Pancreas 47 (7), e43–e46. doi:10.1097/MPA.0000000000001098
Pace, K. R., Dutt, R., and Galileo, D. S. (2019). Exosomal L1CAM Stimulates Glioblastoma Cell Motility, Proliferation, and Invasiveness. Ijms 20 (16), 3982. doi:10.3390/ijms20163982
Pahl, J., and Cerwenka, A. (2017). Tricking the Balance: NK Cells in Anti-cancer Immunity. Immunobiology 222 (1), 11–20. doi:10.1016/j.imbio.2015.07.012
Pakneshan, S., Safarpour, D., Tavassoli, F., and Jabbari, B. (2014). Brain Metastasis from Ovarian Cancer: a Systematic Review. J. Neurooncol. 119 (1), 1–6. doi:10.1007/s11060-014-1447-9
Paoletti, C., and Hayes, D. F. (2016). Circulating Tumor Cells. Adv. Exp. Med. Biol. 882, 235–258. doi:10.1007/978-3-319-22909-6_10
Paolillo, M., and Schinelli, S. (2017). Integrins and Exosomes, a Dangerous Liaison in Cancer Progression. Cancers 9 (8), 95. doi:10.3390/cancers9080095
Park, J.-M., Han, Y.-M., Jeong, M., Chung, M. H., Kwon, C. I., Ko, K. H., et al. (2017). Synthetic 8-hydroxydeoxyguanosine Inhibited Metastasis of Pancreatic Cancer through Concerted Inhibitions of ERM and Rho-GTPase. Free Radic. Biol. Med. 110, 151–161. doi:10.1016/j.freeradbiomed.2017.06.003
Park, S., Byun, H. K., and Seong, J. (2019). Irradiation-Related Lymphopenia for Bone Metastasis from Hepatocellular Carcinoma. Liver Cancer 8 (6), 468–479. doi:10.1159/000500461
Park, S. M., Do-Thi, V. A., Lee, J. O., Lee, H., and Kim, Y. S. (2020). Interleukin-9 Inhibits Lung Metastasis of Melanoma through Stimulating Anti-tumor M1 Macrophages. Mol. Cell 43 (5), 479–490. doi:10.14348/molcells.2020.0047
Pedrosa, R. M. S. M., Mustafa, D. A., Soffietti, R., and Kros, J. M. (2018). Breast Cancer Brain Metastasis: Molecular Mechanisms and Directions for Treatment. Neuro Oncol. 20 (11), 1439–1449. doi:10.1093/neuonc/noy044
Peinado, H., Alečković, M., Lavotshkin, S., Matei, I., Costa-Silva, B., Moreno-Bueno, G., et al. (2012). Melanoma Exosomes Educate Bone Marrow Progenitor Cells toward a Pro-metastatic Phenotype through MET. Nat. Med. 18 (6), 883–891. doi:10.1038/nm.2753
Peinado, H., Lavotshkin, S., and Lyden, D. (2011). The Secreted Factors Responsible for Pre-metastatic Niche Formation: Old Sayings and New Thoughts. Semin. Cancer Biol. 21 (2), 139–146. doi:10.1016/j.semcancer.2011.01.002
Peinado, H., Zhang, H., Matei, I. R., Costa-Silva, B., Hoshino, A., Rodrigues, G., et al. (2017). Pre-metastatic Niches: Organ-specific Homes for Metastases. Nat. Rev. Cancer 17 (5), 302–317. doi:10.1038/nrc.2017.6
Peng, Z., Xu, S., Li, H., Sun, C., Fu, M., and Gao, M. (2014). Advanced Gastric Cancer with Brain Metastasis Effectively Treated by Arterial Infusion Chemotherapy: A Case Report. Oncol. Lett. 7 (2), 449–451. doi:10.3892/ol.2013.1699
Polistina, G. E., Matarese, A., Cariello, P., Caroppo, D., and Zamparelli, A. S. (2020). Cavitary Lung Metastasis as Relapse of Prostate Cancer. Respir. Med. Case Rep. 29, 100973. doi:10.1016/j.rmcr.2019.100973
Provenzano, P. P., Inman, D. R., Eliceiri, K. W., Knittel, J. G., Yan, L., Rueden, C. T., et al. (2008). Collagen Density Promotes Mammary Tumor Initiation and Progression. BMC Med. 6, 11. doi:10.1186/1741-7015-6-11
Pylayeva, Y., Gillen, K. M., Gerald, W., Beggs, H. E., Reichardt, L. F., and Giancotti, F. G. (2009). Ras- and PI3K-dependent Breast Tumorigenesis in Mice and Humans Requires Focal Adhesion Kinase Signaling. J. Clin. Invest. 119 (2), 252–266. doi:10.1172/JCI37160
Qian, B.-Z., and Pollard, J. W. (2010). Macrophage Diversity Enhances Tumor Progression and Metastasis. Cell 141 (1), 39–51. doi:10.1016/j.cell.2010.03.014
Qiu, J.-J., Lin, X.-J., Tang, X.-Y., Zheng, T.-T., Lin, Y.-Y., and Hua, K.-Q. (2018a). Exosomal Metastasis-Associated Lung Adenocarcinoma Transcript 1 Promotes Angiogenesis and Predicts Poor Prognosis in Epithelial Ovarian Cancer. Int. J. Biol. Sci. 14 (14), 1960–1973. doi:10.7150/ijbs.28048
Qiu, M.-Z., Shi, S.-M., Chen, Z.-H., Yu, H.-E., Sheng, H., Jin, Y., et al. (2018b). Frequency and Clinicopathological Features of Metastasis to Liver, Lung, Bone, and Brain from Gastric Cancer: A SEER-Based Study. Cancer Med. 7 (8), 3662–3672. doi:10.1002/cam4.1661
Qiu, X., Jiang, Y., Zhao, Q., Yan, C., Huang, M., and Jiang, T. a. (2020). Could Ultrasound‐Based Radiomics Noninvasively Predict Axillary Lymph Node Metastasis in Breast Cancer? J. Ultrasound Med. 39 (10), 1897–1905. doi:10.1002/jum.15294
Rackov, G., Garcia-Romero, N., Esteban-Rubio, S., Carrión-Navarro, J., Belda-Iniesta, C., and Ayuso-Sacido, A. (2018). Vesicle-Mediated Control of Cell Function: The Role of Extracellular Matrix and Microenvironment. Front. Physiol. 9, 651. doi:10.3389/fphys.2018.00651
Ramteke, A., Ting, H., Agarwal, C., Mateen, S., Somasagara, R., Hussain, A., et al. (2015). Exosomes Secreted under Hypoxia Enhance Invasiveness and Stemness of Prostate Cancer Cells by Targeting Adherens junction Molecules. Mol. Carcinog. 54 (7), 554–565. doi:10.1002/mc.22124
Raposo, G., and Stoorvogel, W. (2013). Extracellular Vesicles: Exosomes, Microvesicles, and Friends. J. Cel Biol 200 (4), 373–383. doi:10.1083/jcb.201211138
Redmer, T. (2018). Deciphering Mechanisms of Brain Metastasis in Melanoma - the Gist of the Matter. Mol. Cancer 17 (1), 106. doi:10.1186/s12943-018-0854-5
Ren, J., Ding, L., Zhang, D., Shi, G., Xu, Q., Shen, S., et al. (2018). Carcinoma-associated Fibroblasts Promote the Stemness and Chemoresistance of Colorectal Cancer by Transferring Exosomal lncRNA H19. Theranostics 8 (14), 3932–3948. doi:10.7150/thno.25541
Ricard, J. A., Cramer, S. W., Charles, R., Gil Tommee, C., Le, A., Bell, W. R., et al. (2019). Infratentorial Glioblastoma Metastasis to Bone. World Neurosurg. 131, 90–94. doi:10.1016/j.wneu.2019.07.142
Robbins, P. D., and Morelli, A. E. (2014). Regulation of Immune Responses by Extracellular Vesicles. Nat. Rev. Immunol. 14 (3), 195–208. doi:10.1038/nri3622
Roccaro, A. M., Sacco, A., Maiso, P., Azab, A. K., Tai, Y.-T., Reagan, M., et al. (2013). BM Mesenchymal Stromal Cell-Derived Exosomes Facilitate Multiple Myeloma Progression. J. Clin. Invest. 123 (4), 1542–1555. doi:10.1172/JCI66517
Rodrigues, G., Hoshino, A., Kenific, C. M., Matei, I. R., Steiner, L., Freitas, D., et al. (2019). Tumour Exosomal CEMIP Protein Promotes Cancer Cell Colonization in Brain Metastasis. Nat. Cel Biol 21 (11), 1403–1412. doi:10.1038/s41556-019-0404-4
Roegel, J. C., Bressollette, L., Fouilhoux, A. C., Martin, F., Morand, C., and Mottier, D. (1993). Peritoneal Mesothelioma with Bone Metastasis: a Radionuclide Follow-Up. Ann. Med. Interne (Paris) 144 (6), 425.
Ryu, S.-H., Heo, S.-H., Park, E. Y., Choi, K.-C., Ryu, J.-W., Lee, S. H., et al. (2017). Selumetinib Inhibits Melanoma Metastasis to Mouse Liver via Suppression of EMT-Targeted Genes. Ar 37 (2), 607–614. doi:10.21873/anticanres.11354
Said, N. A. B. M., and Williams, E. D. (2011). Growth Factors in Induction of Epithelial-Mesenchymal Transition and Metastasis. Cells Tissues Organs 193 (1-2), 85–97. doi:10.1159/000320360
Saif, M. W., Galanina, N., Ravage-Mass, L., Kaley, K., Cornfeld, D., Lamb, L., et al. (20102010). Bone Metastasis as the Only Metastatic Site in a Patient with Pancreatic Cancer Following Distal Pancreatectomy. Case Rep. Med. 2010, 1–3. doi:10.1155/2010/634975
Sakimura, C., Hori, T., Deguchi, S., Masuda, G., Tendo, M., Nakata, B., et al. (2019). A Case Report of Lung Resection for Lung Metastasis from Pancreatic Cancer. Gan To Kagaku Ryoho 46 (13), 1934–1936.
Salvador, F., Llorente, A., and Gomis, R. R. (2019). From Latency to Overt Bone Metastasis in Breast Cancer: Potential for Treatment and Prevention. J. Pathol. 249 (1), 6–18. doi:10.1002/path.5292
Santarpia, M., Liguori, A., D’Aveni, A., Karachaliou, N., Gonzalez-Cao, M., Daffinà, M. G., et al. (2018). Liquid Biopsy for Lung Cancer Early Detection. J. Thorac. Dis. 10 (Suppl. 7), S882–S897. doi:10.21037/jtd.2018.03.81
Sasaki, T., Sato, T., Nakai, Y., Sasahira, N., Isayama, H., and Koike, K. (2019). Brain Metastasis in Pancreatic Cancer. Medicine (Baltimore) 98 (4), e14227. doi:10.1097/MD.0000000000014227
Sato, Y., Tanaka, K., Kobayashi, Y., Shibuya, H., Nishigaya, Y., Momomura, M., et al. (2015). Uterine Cervical Cancer with Brain Metastasis as the Initial Site of Presentation. J. Obstet. Gynaecol. Res. 41 (7), 1145–1148. doi:10.1111/jog.12668
Schwarz, D., Niederle, T., Münch, P., Hielscher, T., Hassel, J. C., Schlemmer, H. P., et al. (2019). Susceptibility‐weighted Imaging in Malignant Melanoma Brain Metastasis. J. Magn. Reson. Imaging 50 (4), 1251–1259. doi:10.1002/jmri.26692
Seifert, A. M., Eymer, A., Heiduk, M., Wehner, R., Tunger, A., von Renesse, J., et al. (2020). PD-1 Expression by Lymph Node and Intratumoral Regulatory T Cells Is Associated with Lymph Node Metastasis in Pancreatic Cancer. Cancers 12 (10), 2756. doi:10.3390/cancers12102756
Sharma, D., Brummel-Ziedins, K. E., Bouchard, B. A., and Holmes, C. E. (2014). Platelets in Tumor Progression: a Host Factor that Offers Multiple Potential Targets in the Treatment of Cancer. J. Cel. Physiol 229 (8), 1005–1015. doi:10.1002/jcp.24539
Sharma, P., Ludwig, S., Muller, L., Hong, C. S., Kirkwood, J. M., Ferrone, S., et al. (2018a). Immunoaffinity-based Isolation of Melanoma Cell-Derived Exosomes from Plasma of Patients with Melanoma. J. Extracellular Vesicles 7 (1), 1435138. doi:10.1080/20013078.2018.1435138
Sharma, S., Alharbi, M., Kobayashi, M., Lai, A., Guanzon, D., Zuñiga, F., et al. (2018b). Proteomic Analysis of Exosomes Reveals an Association between Cell Invasiveness and Exosomal Bioactivity on Endothelial and Mesenchymal Cell Migration In Vitro. Clin. Sci. (Lond) 132 (18), 2029–2044. doi:10.1042/CS20180425
Shattil, S. J., and Ginsberg, M. H. (1997). Integrin Signaling in Vascular Biology. J. Clin. Invest. 100 (11 Suppl. l), S91–S95. doi:10.1172/jci119500
Sherwood, L. M., Parris, E. E., and Folkman, J. (1971). Tumor Angiogenesis: Therapeutic Implications. N. Engl. J. Med. 285 (21), 1182–1186. doi:10.1056/NEJM197111182852108
Shida, Y., Hakariya, T., Miyata, Y., and Sakai, H. (2020). Three Cases of Brain Metastasis from Castration‐resistant Prostate Cancer. Clin. Case Rep. 8 (1), 96–99. doi:10.1002/ccr3.2587
Shuto, T., Fujino, H., Inomori, S., Nakayama, S., Satoh, H., Ideguchi, H., et al. (1995). Glioblastoma Multiforme with Liver Metastasis-Ccase Report. No To Shinkei 47 (8), 772–777.
Simons, B. W., Dalrymple, S., Rosen, M., Zheng, L., and Brennen, W. N. (2020). A Hemi‐spleen Injection Model of Liver Metastasis for Prostate Cancer. Prostate 80 (14), 1263–1269. doi:10.1002/pros.24055
Singh, R., Pochampally, R., Watabe, K., Lu, Z., and Mo, Y.-Y. (2014). Exosome-mediated Transfer of miR-10b Promotes Cell Invasion in Breast Cancer. Mol. Cancer 13, 256. doi:10.1186/1476-4598-13-256
Skog, J., Würdinger, T., van Rijn, S., Meijer, D. H., Gainche, L., Curry, W. T., et al. (2008). Glioblastoma Microvesicles Transport RNA and Proteins that Promote Tumour Growth and Provide Diagnostic Biomarkers. Nat. Cel Biol 10 (12), 1470–1476. doi:10.1038/ncb1800
Soler-Cardona, A., Forsthuber, A., Lipp, K., Ebersberger, S., Heinz, M., Schossleitner, K., et al. (2018). CXCL5 Facilitates Melanoma Cell-Neutrophil Interaction and Lymph Node Metastasis. J. Invest. Dermatol. 138 (7), 1627–1635. doi:10.1016/j.jid.2018.01.035
Sommers, C. L., Byers, S. W., Thompson, E. W., Torri, J. A., and Gelmann, E. P. (1994). Differentiation State and Invasiveness of Human Breast Cancer Cell Lines. Breast Cancer Res. Tr 31 (2-3), 325–335. doi:10.1007/BF00666165
Sridhar, S., Paz-Ares, L., Liu, H., Shen, K., Morehouse, C., Rizvi, N., et al. (2019). Prognostic Significance of Liver Metastasis in Durvalumab-Treated Lung Cancer Patients. Clin. Lung Cancer 20 (6), e601–e608. doi:10.1016/j.cllc.2019.06.020
Sruthi, T. V., Edatt, L., Raji, G. R., Kunhiraman, H., Shankar, S. S., Shankar, V., et al. (2018). Horizontal Transfer of miR-23a from Hypoxic Tumor Cell Colonies Can Induce Angiogenesis. J. Cel Physiol 233 (4), 3498–3514. doi:10.1002/jcp.26202
Stansel, T., A. Wickline, S., and Pan, H. (2020). NF-κB Inhibition Suppresses Experimental Melanoma Lung Metastasis. J. Cancer Sci. Clin. Ther. 04 (3), 256–265. doi:10.26502/jcsct.5079070
Stasenko, M., Cybulska, P., Feit, N., Makker, V., Konner, J., O'Cearbhaill, R. E., et al. (2019). Brain Metastasis in Epithelial Ovarian Cancer by BRCA1/2 Mutation Status. Gynecol. Oncol. 154 (1), 144–149. doi:10.1016/j.ygyno.2019.05.004
Su, Y. Y., Sun, L., Guo, Z. R., Li, J. C., Bai, T. T., Cai, X. X., et al. (2019). Upregulated Expression of Serum Exosomal miR-375 and miR-1307 Enhance the Diagnostic Power of CA125 for Ovarian Cancer. J. Ovarian Res. 12 (1), 6. doi:10.1186/s13048-018-0477-x
Sun, H., Wang, C., Hu, B., Gao, X., Zou, T., Luo, Q., et al. (2021). Exosomal S100A4 Derived from Highly Metastatic Hepatocellular Carcinoma Cells Promotes Metastasis by Activating STAT3. Sig Transduct Target. Ther. 6 (1), 187. doi:10.1038/s41392-021-00579-3
Sun, L., He, M., Xu, N., Xu, D.-H., Ben-David, Y., Yang, Z.-Y., et al. (2018). Regulation of RAB22A by Mir-193b Inhibits Breast Cancer Growth and Metastasis Mediated by Exosomes. Int. J. Oncol. 53 (6), 2705–2714. doi:10.3892/ijo.2018.4571
Sun, S., Lian, X., Liu, X., Ma, J., Hou, X., Zhang, F., et al. (2020). Multimodal Therapy Is a Better Choice for Patients with Brain Metastasis from Cervical Cancer. Cmar Vol 12, 12395–12402. doi:10.2147/CMAR.S283673
Sussman, J., and Rosai, J. (1990). Lymph Node Metastasis. Am. J. Surg. Pathol. 14 (9), 819–828. doi:10.1097/00000478-199009000-00003
Suzuki, K., Yasuda, T., Nagao, K., Hori, T., Watanabe, K., Kanamori, M., et al. (2015). Bone Metastasis of a Gastrointestinal Stromal Tumor: A Report of Two Cases. Oncol. Lett. 9 (4), 1814–1818. doi:10.3892/ol.2015.2976
Swayne, L. C., Hediger, R. G., and Wolff, M. (1992). Bone Scan Detection of Pelvic Metastasis from Pleural Mesothelioma. Clin. Nucl. Med. 17 (12), 965–966. doi:10.1097/00003072-199212000-00013
Szczepanski, M. J., Szajnik, M., Welsh, A., Whiteside, T. L., and Boyiadzis, M. (2011). Blast-derived Microvesicles in Sera from Patients with Acute Myeloid Leukemia Suppress Natural Killer Cell Function via Membrane-Associated Transforming Growth Factor- 1. Haematologica 96 (9), 1302–1309. doi:10.3324/haematol.2010.039743
Tahara, R. K., Brewer, T. M., Theriault, R. L., and Ueno, N. T. (2019). Bone Metastasis of Breast Cancer. Adv. Exp. Med. Biol. 1152, 105–129. doi:10.1007/978-3-030-20301-6_7
Takehara, Y., Endo, S., Mori, Y., Nakahara, K., Takayanagi, D., Shimada, S., et al. (2014). Malignant Peritoneal Mesothelioma with Lymph Node Metastasis that Originated in the Transverse colon. World J. Surg. Onc 12, 112. doi:10.1186/1477-7819-12-112
Tan, S., Xia, L., Yi, P., Han, Y., Tang, L., Pan, Q., et al. (2020). Exosomal miRNAs in Tumor Microenvironment. J. Exp. Clin. Cancer Res. 39 (1), 67. doi:10.1186/s13046-020-01570-6
Taverna, S., Flugy, A., Saieva, L., Kohn, E. C., Santoro, A., Meraviglia, S., et al. (2012). Role of Exosomes Released by Chronic Myelogenous Leukemia Cells in Angiogenesis. Int. J. Cancer 130 (9), 2033–2043. doi:10.1002/ijc.26217
Taylor, D. D., Gerçel-Taylor, C., Lyons, K. S., Stanson, J., and Whiteside, T. L. (2003). T-cell Apoptosis and Suppression of T-Cell receptor/CD3-Zeta by Fas Ligand-Containing Membrane Vesicles Shed from Ovarian Tumors. Clin. Cancer Res. 9 (14), 5113–5119.
Teng, Y., Ren, Y., Hu, X., Mu, J., Samykutty, A., Zhuang, X., et al. (2017). MVP-mediated Exosomal Sorting of miR-193a Promotes colon Cancer Progression. Nat. Commun. 8, 14448. doi:10.1038/ncomms14448
Thakur, B. K., Zhang, H., Becker, A., Matei, I., Huang, Y., Costa-Silva, B., et al. (2014). Double-stranded DNA in Exosomes: a Novel Biomarker in Cancer Detection. Cell Res 24 (6), 766–769. doi:10.1038/cr.2014.44
Théry, C., Ostrowski, M., and Segura, E. (2009). Membrane Vesicles as Conveyors of Immune Responses. Nat. Rev. Immunol. 9 (8), 581–593. doi:10.1038/nri2567
Tian, X.-P., Wang, C.-Y., Jin, X.-H., Li, M., Wang, F.-W., Huang, W.-J., et al. (2019). Acidic Microenvironment Up-Regulates Exosomal miR-21 and miR-10b in Early-Stage Hepatocellular Carcinoma to Promote Cancer Cell Proliferation and Metastasis. Theranostics 9 (7), 1965–1979. doi:10.7150/thno.30958
Tian, X., Sun, M., Wu, H., Chen, C., Li, H., Qiu, S., et al. (2021). Exosome-derived miR-Let-7c Promotes Angiogenesis in Multiple Myeloma by Polarizing M2 Macrophages in the Bone Marrow Microenvironment. Leuk. Res. 105, 106566. doi:10.1016/j.leukres.2021.106566
Tokoro, T., Nakamura, K., Hirose, A., Nakanuma, S., Okamoto, K., Kinoshita, J., et al. (2018). A Case of Colon Cancer with Brain, Liver and Lung Metastasis Successfully Treated with Bevacizumab Plus Xelox Therapy. Gan To Kagaku Ryoho 45 (3), 521–523.
Tuncer, M., Faydaci, G., Altin, G., Erdogan, B. A., Kibar, S., Sanli, A., et al. (2014). Metastasis of Non-muscle-invasive Bladder Cancer into the Thyroid Gland: a Literature Review Accompanied by a Rare Case. Korean J. Urol. 55 (3), 222–225. doi:10.4111/kju.2014.55.3.222
Tyagi, A., Sharma, S., Wu, K., Wu, S.-Y., Xing, F., Liu, Y., et al. (2021). Nicotine Promotes Breast Cancer Metastasis by Stimulating N2 Neutrophils and Generating Pre-metastatic Niche in Lung. Nat. Commun. 12 (1), 474. doi:10.1038/s41467-020-20733-9
Ubukata, H., Motohashi, G., Tabuchi, T., Nagata, H., Konishi, S., and Tabuchi, T. (2011). Overt Bone Metastasis and Bone Marrow Micrometastasis of Early Gastric Cancer. Surg. Today 41 (2), 169–174. doi:10.1007/s00595-010-4389-7
Ueda, S., Ooka, H., Yamaguchi, M., Hayashi, H., and Okayasu, T. (1973). Case of Pulmonary Metastasis of Ovarian Cancer Erroneously Diagnosed and Treated as Pulmonary Tuberculosis for a Long Time. Nihon Kyobu Shikkan Gakkai Zasshi 11 (11), 684–687.
Uesato, Y., Tamashiro, K., and Takatsuki, M. (2020). Long-term Survival after Repeated Resection for Lung Metastasis Originating from Pancreatic Cancer: a Case Report. Surg. Case Rep. 6 (1), 66. doi:10.1186/s40792-020-00832-x
Uluçkan, Ö. (2019). Mouse Models of Melanoma Bone Metastasis. Methods Mol. Biol. 1914, 343–348. doi:10.1007/978-1-4939-8997-3_19
Umezu, T., Tadokoro, H., Azuma, K., Yoshizawa, S., Ohyashiki, K., and Ohyashiki, J. H. (2014). Exosomal miR-135b Shed from Hypoxic Multiple Myeloma Cells Enhances Angiogenesis by Targeting Factor-Inhibiting HIF-1. Blood 124 (25), 3748–3757. doi:10.1182/blood-2014-05-576116
Valadi, H., Ekström, K., Bossios, A., Sjöstrand, M., Lee, J. J., and Lötvall, J. O. (2007). Exosome-mediated Transfer of mRNAs and microRNAs Is a Novel Mechanism of Genetic Exchange between Cells. Nat. Cel Biol 9 (6), 654–659. doi:10.1038/ncb1596
Valastyan, S., and Weinberg, R. A. (2011). Tumor Metastasis: Molecular Insights and Evolving Paradigms. Cell 147 (2), 275–292. doi:10.1016/j.cell.2011.09.024
Voura, E. B., Ramjeesingh, R. A., Montgomery, A. M. P., and Siu, C.-H. (2001). Involvement of Integrin αvβ3and Cell Adhesion Molecule L1 in Transendothelial Migration of Melanoma Cells. MBoC 12 (9), 2699–2710. doi:10.1091/mbc.12.9.2699
Wan, L., Pantel, K., and Kang, Y. (2013). Tumor Metastasis: Moving New Biological Insights into the Clinic. Nat. Med. 19 (11), 1450–1464. doi:10.1038/nm.3391
Wang, B., Mao, J.-h., Wang, B.-y., Wang, L.-x., Wen, H.-y., Xu, L.-j., et al. (2020). Exosomal miR-1910-3p Promotes Proliferation, Metastasis, and Autophagy of Breast Cancer Cells by Targeting MTMR3 and Activating the NF-Κb Signaling Pathway. Cancer Lett. 489, 87–99. doi:10.1016/j.canlet.2020.05.038
Wang, C.-F., Peng, S.-J., Liu, R.-Q., Yu, Y.-J., Ge, Q.-M., Liang, R.-B., et al. (2020). The Combination of CA125 and NSE Is Useful for Predicting Liver Metastasis of Lung Cancer. Dis. Markers 2020, 1–10. doi:10.1155/2020/8850873
Wang, G., Xu, J., Qi, Y., Xiu, J., Li, R., and Han, M. (2019a). Distribution of Brain Metastasis from Lung Cancer. Cmar Vol 11, 9331–9338. doi:10.2147/CMAR.S222920
Wang, H., Deng, Q., Lv, Z., Ling, Y., Hou, X., Chen, Z., et al. (2019b). N6-methyladenosine Induced miR-143-3p Promotes the Brain Metastasis of Lung Cancer via Regulation of VASH1. Mol. Cancer 18 (1), 181. doi:10.1186/s12943-019-1108-x
Wang, H., Wei, M., Kang, Y., Xing, J., and Zhao, Y. (2020). Circular RNA circ_PVT1 Induces Epithelial-Mesenchymal Transition to Promote Metastasis of Cervical Cancer. aging 12 (20), 20139–20151. doi:10.18632/aging.103679
Wang, J., Guan, X., Zhang, Y., Ge, S., Zhang, L., Li, H., et al. (2018a). Exosomal miR-27a Derived from Gastric Cancer Cells Regulates the Transformation of Fibroblasts into Cancer-Associated Fibroblasts. Cell Physiol Biochem 49 (3), 869–883. doi:10.1159/000493218
Wang, J., Zhang, Q., Wang, D., Yang, S., Zhou, S., Xu, H., et al. (2020). Microenvironment‐induced TIMP2 Loss by Cancer‐secreted Exosomal miR‐4443 Promotes Liver Metastasis of Breast Cancer. J. Cel Physiol 235 (7-8), 5722–5735. doi:10.1002/jcp.29507
Wang, L., Tantai, J., and Zhu, X. (2020). Katanin P60: a Potential Biomarker for Lymph Node Metastasis and Prognosis for Non-small Cell Lung Cancer. World J. Surg. Onc 18 (1), 157. doi:10.1186/s12957-020-01939-z
Wang, M., Chao, C.-C., Chen, P.-C., Liu, P.-I., Yang, Y.-C., Su, C.-M., et al. (2019c). Thrombospondin Enhances RANKL-dependent Osteoclastogenesis and Facilitates Lung Cancer Bone Metastasis. Biochem. Pharmacol. 166, 23–32. doi:10.1016/j.bcp.2019.05.005
Wang, M., Zhou, J., Zhang, L., Zhao, Y., Zhang, N., Wang, L., et al. (2019d). Surgical Treatment of Ovarian Cancer Liver Metastasis. Hepatobiliary Surg. Nutr. 8 (2), 129–137. doi:10.21037/hbsn.2018.12.06
Wang, N., Feng, Y., Xie, J., Han, H., Dong, Q., and Wang, W. (2020). Long Non-coding RNA ZNF667-AS1 Knockdown Curbs Liver Metastasis in Acute Myeloid Leukemia by Regulating the microRNA-206/AKAP13 Axis. Cmar Vol 12, 13285–13300. doi:10.2147/CMAR.S269258
Wang, Q., Wang, G., Niu, L., Zhao, S., Li, J., Zhang, Z., et al. (2021a). Exosomal MiR-1290 Promotes Angiogenesis of Hepatocellular Carcinoma via Targeting SMEK1. J. Oncol. 2021, 1–13. doi:10.1155/2021/6617700
Wang, X., Hu, L.-P., Qin, W.-T., Yang, Q., Chen, D.-Y., Li, Q., et al. (2021b). Identification of a Subset of Immunosuppressive P2RX1-Negative Neutrophils in Pancreatic Cancer Liver Metastasis. Nat. Commun. 12 (1), 174. doi:10.1038/s41467-020-20447-y
Wang, X., Luo, G., Zhang, K., Cao, J., Huang, C., Jiang, T., et al. (2018). Hypoxic Tumor-Derived Exosomal miR-301a Mediates M2 Macrophage Polarization via PTEN/PI3Kγ to Promote Pancreatic Cancer Metastasis. Cancer Res. 78 (16), 4586–4598. doi:10.1158/0008-5472.CAN-17-3841
Wang, X., Wang, B., Zhan, W., Kang, L., Zhang, S., Chen, C., et al. (2019e). Melatonin Inhibits Lung Metastasis of Gastric Cancer In Vivo. Biomed. Pharmacother. 117, 109018. doi:10.1016/j.biopha.2019.109018
Wang, X., Wang, X., Zhu, Z., Li, W., Yu, G., Jia, Z., et al. (2019f). Prostate Carcinoma Cell-Derived Exosomal MicroRNA-26a Modulates the Metastasis and Tumor Growth of Prostate Carcinoma. Biomed. Pharmacother. 117, 109109. doi:10.1016/j.biopha.2019.109109
Wang, Y., Liu, W., Yu, Y., Liu, J.-j., Xue, H.-d., Qi, Y.-f., et al. (2020). CT Radiomics Nomogram for the Preoperative Prediction of Lymph Node Metastasis in Gastric Cancer. Eur. Radiol. 30 (2), 976–986. doi:10.1007/s00330-019-06398-z
Waqar, S. N., Samson, P. P., Robinson, C. G., Bradley, J., Devarakonda, S., Du, L., et al. (2018). Non-small-cell Lung Cancer with Brain Metastasis at Presentation. Clin. Lung Cancer 19 (4), e373–e379. doi:10.1016/j.cllc.2018.01.007
Webber, J., Steadman, R., Mason, M. D., Tabi, Z., and Clayton, A. (2010). Cancer Exosomes Trigger Fibroblast to Myofibroblast Differentiation. Cancer Res. 70 (23), 9621–9630. doi:10.1158/0008-5472.CAN-10-1722
Wells, A., Yates, C., and Shepard, C. R. (2008). E-cadherin as an Indicator of Mesenchymal to Epithelial Reverting Transitions during the Metastatic Seeding of Disseminated Carcinomas. Clin. Exp. Metastasis 25 (6), 621–628. doi:10.1007/s10585-008-9167-1
Wen, S., Wei, Y., Zen, C., Xiong, W., Niu, Y., and Zhao, Y. (2020). Long Non-coding RNA NEAT1 Promotes Bone Metastasis of Prostate Cancer through N6-Methyladenosine. Mol. Cancer 19 (1), 171. doi:10.1186/s12943-020-01293-4
Wernersson, S., and Pejler, G. (2014). Mast Cell Secretory Granules: Armed for Battle. Nat. Rev. Immunol. 14 (7), 478–494. doi:10.1038/nri3690
Wu, H., Song, S., Yan, A., Guo, X., Chang, L., Xu, L., et al. (2020). RACK1 Promotes the Invasive Activities and Lymph Node Metastasis of Cervical Cancer via Galectin-1. Cancer Lett. 469, 287–300. doi:10.1016/j.canlet.2019.11.002
Wu, J., Li, H., Xie, H., Wu, X., and Lan, P. (2019). The Malignant Role of Exosomes in the Communication Among Colorectal Cancer Cell, Macrophage and Microbiome. Carcinogenesis 40 (5), 601–610. doi:10.1093/carcin/bgy138
Xia, Y., Zhang, B., Zhang, H., Li, W., Wang, K. P., and Shen, H. (2015). Evaluation of Lymph Node Metastasis in Lung Cancer: Who Is the Chief justice? J. Thorac. Dis. 7 (Suppl. 4), S231–S237. doi:10.3978/j.issn.2072-1439.2015.11.63
Xian, X., Gopal, S., and Couchman, J. R. (2010). Syndecans as Receptors and Organizers of the Extracellular Matrix. Cell Tissue Res 339 (1), 31–46. doi:10.1007/s00441-009-0829-3
Xiao, D., Barry, S., Kmetz, D., Egger, M., Pan, J., Rai, S. N., et al. (2016). Melanoma Cell-Derived Exosomes Promote Epithelial-Mesenchymal Transition in Primary Melanocytes through Paracrine/autocrine Signaling in the Tumor Microenvironment. Cancer Lett. 376 (2), 318–327. doi:10.1016/j.canlet.2016.03.050
Xie, Z., Gao, Y., Ho, C., Li, L., Jin, C., Wang, X., et al. (2021). Exosome-delivered CD44v6/C1QBP Complex Drives Pancreatic Cancer Liver Metastasis by Promoting Fibrotic Liver Microenvironment. Gut 71, 568–579. doi:10.1136/gutjnl-2020-323014
Xing, F., Liu, Y., Wu, S.-Y., Wu, K., Sharma, S., Mo, Y.-Y., et al. (2018). Loss of XIST in Breast Cancer Activates MSN-C-Met and Reprograms Microglia via Exosomal miRNA to Promote Brain Metastasis. Cancer Res. 78 (15), 4316–4330. doi:10.1158/0008-5472.CAN-18-1102
Xu, D., Lin, X., and Qiu, X. (2020a). The Epithelioid Gastrointestinal Stromal Tumor with Pulmonary Metastasis. Medicine (Baltimore) 99 (9), e19346. doi:10.1097/MD.0000000000019346
Xu, H., Xu, G. L., Li, X. D., Su, Q. H., and Dong, C. Z. (2021). Correlation between the Contrast-Enhanced Ultrasound Image Features and Axillary Lymph Node Metastasis of Primary Breast Cancer and its Diagnostic Value. Clin. Transl Oncol. 23 (1), 155–163. doi:10.1007/s12094-020-02407-6
Xu, L., Xue, F., Wang, B., Yan, D., Ding, W., Yin, J., et al. (2016). Hoarseness Due to Lymph Node Metastasis of Hepatocellular Carcinoma: A Case Report. Oncol. Lett. 12 (2), 918–920. doi:10.3892/ol.2016.4687
Xu, N., Chen, S. H., Lin, T. T., Cai, H., Ke, Z. B., Dong, R. N., et al. (2020b). Development and Validation of Hub Genes for Lymph Node Metastasis in Patients with Prostate Cancer. J. Cel Mol Med 24 (8), 4402–4414. doi:10.1111/jcmm.15098
Xu, Y. Y., Lu, X., Mao, Y. L., Xiong, J. P., Bian, J., Huang, H. C., et al. (2017). The Surgical Treatment of Ovarian Cancer Metastasis between Liver and Diaphragm: a Report of 83 Cases. Zhonghua Wai Ke Za Zhi 55 (11), 838–841. doi:10.3760/cma.j.issn.0529-5815.2017.11.004
Yamakawa, Y., Moriguchi, M., Aramaki, T., Mitsuya, K., Asakura, K., Sawada, A., et al. (2015). Brain Metastasis from Hepatocellular Carcinoma: The Impact of Radiotherapy on Control of Intracranial Hemorrhage. Hepatol. Res. 45 (11), 1071–1075. doi:10.1111/hepr.12457
Yamanaka, T., Takaki, H., Nakatsuka, A., Uraki, J., Fujimori, M., Hasegawa, T., et al. (2013). Radiofrequency Ablation for Liver Metastasis from Gastrointestinal Stromal Tumor. J. Vasc. Interv. Radiol. 24 (3), 341–346. doi:10.1016/j.jvir.2012.11.021
Yamashita, K., Baba, Y., Kurashige, J., Iwatsuki, M., Imai, K., Hashimoto, D., et al. (2016). Co-occurrence of Liver Metastasis of Gastrointestinal Stromal Tumor and Hepatocellular Carcinoma: a Case Report. Surg. Case Rep. 2 (1), 86. doi:10.1186/s40792-016-0212-z
Yan, T. D., Yoo, D., and Sugarbaker, P. H. (2006). Significance of Lymph Node Metastasis in Patients with Diffuse Malignant Peritoneal Mesothelioma. Eur. J. Surg. Oncol. (Ejso) 32 (9), 948–953. doi:10.1016/j.ejso.2006.05.009
Yang, B., Feng, X., Liu, H., Tong, R., Wu, J., Li, C., et al. (2020a). High-metastatic Cancer Cells Derived Exosomal miR92a-3p Promotes Epithelial-Mesenchymal Transition and Metastasis of Low-Metastatic Cancer Cells by Regulating PTEN/Akt Pathway in Hepatocellular Carcinoma. Oncogene 39 (42), 6529–6543. doi:10.1038/s41388-020-01450-5
Yang, G.-L., Luo, T.-H., Zhang, H.-Q., Ling, C.-Q., and Li, B. (2016). A Case Report of Gastric Cancer with Brain Metastasis: Rare Peripheral Nervous System Symptoms. Oncol. Lett. 11 (4), 2893–2895. doi:10.3892/ol.2016.4288
Yang, H., Zhang, H., Ge, S., Ning, T., Bai, M., Li, J., et al. (2018). Exosome-Derived miR-130a Activates Angiogenesis in Gastric Cancer by Targeting C-MYB in Vascular Endothelial Cells. Mol. Ther. 26 (10), 2466–2475. doi:10.1016/j.ymthe.2018.07.023
Yang, S.-S., Ma, S., Dou, H., Liu, F., Zhang, S.-Y., Jiang, C., et al. (2020b). Breast Cancer-Derived Exosomes Regulate Cell Invasion and Metastasis in Breast Cancer via miR-146a to Activate Cancer Associated Fibroblasts in Tumor Microenvironment. Exp. Cel Res. 391 (2), 111983. doi:10.1016/j.yexcr.2020.111983
Yao, K., Peng, C., Zhang, Y., Zykova, T. A., Lee, M.-H., Lee, S.-Y., et al. (2017). RSK2 Phosphorylates T-Bet to Attenuate colon Cancer Metastasis and Growth. Proc. Natl. Acad. Sci. USA 114 (48), 12791–12796. doi:10.1073/pnas.1710756114
Ye, L., Zhang, F., Li, H., Yang, L., Lv, T., Gu, W., et al. (2017). Circulating Tumor Cells Were Associated with the Number of T Lymphocyte Subsets and NK Cells in Peripheral Blood in Advanced Non-small-cell Lung Cancer. Dis. Markers 2017, 1–6. doi:10.1155/2017/5727815
Ye, L., Zhang, Q., Cheng, Y., Chen, X., Wang, G., Shi, M., et al. (2018). Tumor-derived Exosomal HMGB1 Fosters Hepatocellular Carcinoma Immune Evasion by Promoting TIM-1+ Regulatory B Cell Expansion. J. Immunotherapy Cancer 6 (1), 145. doi:10.1186/s40425-018-0451-6
Yi, H., Ye, J., Yang, X. M., Zhang, L. W., Zhang, Z. G., and Chen, Y. P. (2015). High-grade Ovarian Cancer Secreting Effective Exosomes in Tumor Angiogenesis. Int. J. Clin. Exp. Pathol. 8 (5), 5062–5070.
Yin, Z., Ma, T., Huang, B., Lin, L., Zhou, Y., Yan, J., et al. (2019). Macrophage-derived Exosomal microRNA-501-3p Promotes Progression of Pancreatic Ductal Adenocarcinoma through the TGFBR3-Mediated TGF-β Signaling Pathway. J. Exp. Clin. Cancer Res. 38 (1), 310. doi:10.1186/s13046-019-1313-x
Yoon, A., Choi, C. H., Kim, H.-J., Park, J.-Y., Lee, Y.-Y., Kim, T.-J., et al. (2013). Contributing Factors for Bone Metastasis in Uterine Cervical Cancer. Int. J. Gynecol. Cancer 23 (7), 1311–1317. doi:10.1097/IGC.0b013e31829da127
York, J. E., Stringer, J., Ajani, J. A., Wildrick, D. M., and Gokaslan, Z. L. (1999). Gastric Cancer and Metastasis to the Brain. Ann. Surg. Oncol. 6 (8), 771–776. doi:10.1007/s10434-999-0771-3
Yoshida, Y., Hoshino, S., Miyake, T., Fukuda, S., Yamada, K., Naoya, A., et al. (2012). Early Start of Chemotherapy after Resection of Brain Metastasis from colon Cancer with Synchronous Multiple Liver Metastases. Case Rep. Oncol. 5 (2), 290–295. doi:10.1159/000339614
You, L.-N., Tai, Q.-W., Xu, L., Hao, Y., Guo, W.-J., Zhang, Q., et al. (2021a). Exosomal LINC00161 Promotes Angiogenesis and Metastasis via Regulating miR-590-3p/ROCK axis in Hepatocellular Carcinoma. Cancer Gene Ther. 28 (6), 719–736. doi:10.1038/s41417-020-00269-2
You, X., Wang, Y., Meng, J., Han, S., Liu, L., Sun, Y., et al. (2021b). Exosomal miR-663b E-xposed to TGF-β1 P-romotes C-ervical C-ancer M-etastasis and E-pithelial-mesenchymal T-ransition by T-argeting MGAT3. Oncol. Rep. 45 (4). doi:10.3892/or.2021.7963
You, Y., Shan, Y., Chen, J., Yue, H., You, B., Shi, S., et al. (2015). Matrix Metalloproteinase 13‐containing Exosomes Promote Nasopharyngeal Carcinoma Metastasis. Cancer Sci. 106 (12), 1669–1677. doi:10.1111/cas.12818
Yousefi, M., Nosrati, R., Salmaninejad, A., Dehghani, S., Shahryari, A., and Saberi, A. (2018). Organ-specific Metastasis of Breast Cancer: Molecular and Cellular Mechanisms Underlying Lung Metastasis. Cell Oncol. 41 (2), 123–140. doi:10.1007/s13402-018-0376-6
Yu, L.-X., Yan, L., Yang, W., Wu, F.-Q., Ling, Y., Chen, S.-Z., et al. (2014). Platelets Promote Tumour Metastasis via Interaction between TLR4 and Tumour Cell-Released High-Mobility Group Box1 Protein. Nat. Commun. 5, 5256. doi:10.1038/ncomms6256
Yue, S., Mu, W., Erb, U., and Zöller, M. (2015). The Tetraspanins CD151 and Tspan8 Are Essential Exosome Components for the Crosstalk between Cancer Initiating Cells and Their Surrounding. Oncotarget 6 (4), 2366–2384. doi:10.18632/oncotarget.2958
Yung, W. K. A., Tepper, S. J., and Young, D. F. (1983). Diffuse Bone Marrow Metastasis by Glioblastoma: Premortem Diagnosis by Peroxidase-Antiperoxidase Staining for Glial Fibrillary Acidic Protein. Ann. Neurol. 14 (5), 581–585. doi:10.1002/ana.410140514
Zeng, Z., Li, Y., Pan, Y., Lan, X., Song, F., Sun, J., et al. (2018). Cancer-derived Exosomal miR-25-3p Promotes Pre-metastatic Niche Formation by Inducing Vascular Permeability and Angiogenesis. Nat. Commun. 9 (1), 5395. doi:10.1038/s41467-018-07810-w
Zhang, C., Liao, Y., Liu, P., Du, Q., Liang, Y., Ooi, S., et al. (2020). FABP5 Promotes Lymph Node Metastasis in Cervical Cancer by Reprogramming Fatty Acid Metabolism. Theranostics 10 (15), 6561–6580. doi:10.7150/thno.44868
Zhang, G., Zhang, W., Li, B., Stringer-Reasor, E., Chu, C., Sun, L., et al. (2017). MicroRNA-200c and microRNA- 141 Are Regulated by a FOXP3-Kat2b axis and Associated with Tumor Metastasis in Breast Cancer. Breast Cancer Res. 19 (1), 73. doi:10.1186/s13058-017-0858-x
Zhang, H.-G., Kim, H., Liu, C., Yu, S., Wang, J., Grizzle, W. E., et al. (2007). Curcumin Reverses Breast Tumor Exosomes Mediated Immune Suppression of NK Cell Tumor Cytotoxicity. Biochim. Biophys. Acta (Bba) - Mol. Cel Res. 1773 (7), 1116–1123. doi:10.1016/j.bbamcr.2007.04.015
Zhang, H., Deng, T., Liu, R., Bai, M., Zhou, L., Wang, X., et al. (2017). Exosome-delivered EGFR Regulates Liver Microenvironment to Promote Gastric Cancer Liver Metastasis. Nat. Commun. 8, 15016. doi:10.1038/ncomms15016
Zhang, J., Gao, Y., Han, H., Zou, C., Feng, Y., and Zhang, H. (2019a). Matrine Suppresses Lung Metastasis of Human Hepatocellular Carcinoma by Directly Targeting Matrix Metalloproteinase-9. Biochem. Biophysical Res. Commun. 515 (1), 57–63. doi:10.1016/j.bbrc.2019.04.063
Zhang, L., Niu, H., Ma, J., Yuan, B.-Y., Chen, Y.-H., Zhuang, Y., et al. (2019b). The Molecular Mechanism of LncRNA34a-Mediated Regulation of Bone Metastasis in Hepatocellular Carcinoma. Mol. Cancer 18 (1), 120. doi:10.1186/s12943-019-1044-9
Zhang, M., and Sun, J. (2013). Bone Metastasis from Ovarian Cancer. Clinical Analysis of 26 Cases. Saudi Med. J. 34 (12), 1270–1273.
Zhang, Q., Len, T. Y., Zhang, S. X., Zhao, Q. H., and Yang, L. H. (2020). Exosomes Transferring Long Non-coding RNA FAL1 to Regulate Ovarian Cancer Metastasis through the PTEN/AKT Signaling Pathway. Eur. Rev. Med. Pharmacol. Sci. 24 (21), 10921. doi:10.26355/eurrev_202011_23560
Zhang, Q., Suo, J., Chang, W., Shi, J., and Chen, M. (2017). Dual-modal Computer-Assisted Evaluation of Axillary Lymph Node Metastasis in Breast Cancer Patients on Both Real-Time Elastography and B-Mode Ultrasound. Eur. J. Radiol. 95, 66–74. doi:10.1016/j.ejrad.2017.07.027
Zhang, X. (2019). Interactions between Cancer Cells and Bone Microenvironment Promote Bone Metastasis in Prostate Cancer. Cancer Commun. 39 (1), 76. doi:10.1186/s40880-019-0425-1
Zhang, Y.-F., Zhou, Y.-Z., Zhang, B., Huang, S.-F., Li, P.-P., He, X.-M., et al. (2019c). Pancreatic Cancer-Derived Exosomes Promoted Pancreatic Stellate Cells Recruitment by Pancreatic Cancer. J. Cancer 10 (18), 4397–4407. doi:10.7150/jca.27590
Zhang, Z., Li, X., Sun, W., Yue, S., Yang, J., Li, J., et al. (2017). Loss of Exosomal miR-320a from Cancer-Associated Fibroblasts Contributes to HCC Proliferation and Metastasis. Cancer Lett. 397, 33–42. doi:10.1016/j.canlet.2017.03.004
Zhao, Z., Liang, S., and Sun, F. (2020). LncRNA DLX6-AS1 Promotes Malignant Phenotype and Lymph Node Metastasis in Prostate Cancer by Inducing LARGE Methylation. Front. Oncol. 10, 1172. doi:10.3389/fonc.2020.01172
Zheng, X., Ma, N., Wang, X., Hu, J., Ma, X., Wang, J., et al. (2020). Exosomes Derived from 5-Fluorouracil-Resistant colon Cancer Cells Are Enriched in GDF15 and Can Promote Angiogenesis. J. Cancer 11 (24), 7116–7126. doi:10.7150/jca.49224
Zhong, Y., Lu, Q., Qiu, W., and Luo, Y. (2020). LINC00636 Promotes Lymph Node Metastasis and Cervical Cancer through Targeting NM23. Biosci. Rep. 40 (10). doi:10.1042/BSR20200367
Zhou, C.-F., Ma, J., Huang, L., Yi, H.-Y., Zhang, Y.-M., Wu, X.-G., et al. (2019). Cervical Squamous Cell Carcinoma-Secreted Exosomal miR-221-3p Promotes Lymphangiogenesis and Lymphatic Metastasis by Targeting VASH1. Oncogene 38 (8), 1256–1268. doi:10.1038/s41388-018-0511-x
Zhou, J., Sun, J.-Y., Wu, S.-G., Wang, X., He, Z.-Y., Chen, Q.-H., et al. (2016). Risk Factors for Lymph Node Metastasis in Ovarian Cancer: Implications for Systematic Lymphadenectomy. Int. J. Surg. 29, 123–127. doi:10.1016/j.ijsu.2016.03.039
Zhou, W., Fong, M. Y., Min, Y., Somlo, G., Liu, L., Palomares, M. R., et al. (2014). Cancer-secreted miR-105 Destroys Vascular Endothelial Barriers to Promote Metastasis. Cancer Cell 25 (4), 501–515. doi:10.1016/j.ccr.2014.03.007
Zhou, X., Yan, T., Huang, C., Xu, Z., Wang, L., Jiang, E., et al. (2018). Melanoma Cell-Secreted Exosomal miR-155-5p Induce Proangiogenic Switch of Cancer-Associated Fibroblasts via SOCS1/JAK2/STAT3 Signaling Pathway. J. Exp. Clin. Cancer Res. 37 (1), 242. doi:10.1186/s13046-018-0911-3
Zhou, Z., Chen, L., Sher, D., Zhang, Q., Shah, J., Pham, N.-L., et al. (2018). Predicting Lymph Node Metastasis in Head and Neck Cancer by Combining Many-objective Radiomics and 3-dimensioal Convolutional Neural Network through Evidential Reasoning*. Annu. Int. Conf. IEEE Eng. Med. Biol. Soc., 1–4. doi:10.1109/EMBC.2018.8513070
Zhu, H., Jia, Z., Trush, M. A., and Li, Y. R. (2016). Nrf2 Deficiency Promotes Melanoma Growth and Lung Metastasis. Ros 2 (4), 308–314. doi:10.20455/ros.2016.853
Zhu, Y.-J., Chen, Y., Hu, H.-Y., Zhou, Y.-W., Zhu, Y.-T., and Liu, J.-Y. (2020). Predictive Risk Factors and Online Nomograms for Synchronous Colon Cancer with Liver Metastasis. Front. Oncol. 10, 1681. doi:10.3389/fonc.2020.01681
Zhuo, S., Zhou, J., Ruan, G., Zeng, S., Ma, H., Xie, C., et al. (2020). Percutaneous Microwave Ablation versus Surgical Resection for Ovarian Cancer Liver Metastasis. Int. J. Hyperthermia 37 (1), 28–36. doi:10.1080/02656736.2019.1706767
Zong, Z.-H., Du, Y.-P., Guan, X., Chen, S., and Zhao, Y. (2019). CircWHSC1 Promotes Ovarian Cancer Progression by Regulating MUC1 and hTERT through Sponging miR-145 and miR-1182. J. Exp. Clin. Cancer Res. 38 (1), 437. doi:10.1186/s13046-019-1437-z
Keywords: tumor-derived exosomes, metastasis, pre-metastatic niche, angiogenesis, immunosuppression
Citation: Bai S, Wang Z, Wang M, Li J, Wei Y, Xu R and Du J (2022) Tumor-Derived Exosomes Modulate Primary Site Tumor Metastasis. Front. Cell Dev. Biol. 10:752818. doi: 10.3389/fcell.2022.752818
Received: 03 August 2021; Accepted: 10 February 2022;
Published: 02 March 2022.
Edited by:
Peti Thuwajit, Mahidol University, ThailandReviewed by:
Nabanita Chatterjee, The Ohio State University, United StatesPaola Massimi, International Centre for Genetic Engineering and Biotechnology, Italy
Copyright © 2022 Bai, Wang, Wang, Li, Wei, Xu and Du. This is an open-access article distributed under the terms of the Creative Commons Attribution License (CC BY). The use, distribution or reproduction in other forums is permitted, provided the original author(s) and the copyright owner(s) are credited and that the original publication in this journal is cited, in accordance with accepted academic practice. No use, distribution or reproduction is permitted which does not comply with these terms.
*Correspondence: Juan Du, ZHVqdWFuQGN1aGsuZWR1LmNu
†These authors have contributed equally to this work and share first authorship