- 1Xiyuan Hospital, China Academy of Chinese Medical Sciences, Beijing, China
- 2Graduate School of Beijing University of Chinese Medicine, Beijing, China
- 3National Cardiovascular Clinical Medical Research Center of TCM, Beijing, China
- 4Beijing Xibeiwang Town Community Health Service Center, Beijing, China
Aim: This study was designed to systematically evaluate the effects of growth factor (GF) for therapeutic angiogenesis on ischemic heart disease (IHD) by pooling the results of randomized controlled trials (RCTs).
Methods and Results: PubMed, EMBASE, and CENTRAL databases were searched from inception to October 2022. RCTs, investigating the effects of GF therapy on IHD, were included. The risk bias of included study was assessed according to Cochrane tool. Weighted mean difference (WMD), calculated with fixed effect model or random effect model, was used to evaluate the effects of GF therapy on left ventricular ejection fraction (LVEF) and Canadian Cardiovascular Society (CCS) angina class. Relative risk (RR) was used to evaluate the effects of GF therapy on all-cause mortality, major adverse cardiovascular events (MACE) and revascularization. Meta-analysis, meta-regression analysis and publication bias analysis were performed by RevMan 5.3 or Stata 15.1 software. Twenty-nine studies involving 2899 IHD patients (1,577 patients in GF group and 1,322 patients in control group) were included. Compared with the control group, GF therapy did not reduce all-cause mortality (RR: 0.82; 95% CI: 0.54–1.24; p = 0.341), MACE [(RR: 0.83; 95% CI: 0.61–1.12; p = 0.227), revascularization (RR: 1.27, 95% CI: 0.82–1.96, p = 0.290) and CCS angina class (WMD: −0.08, 95% CI: −0.36 to 0.20, p = 0.560). However, GF therapy could increase LVEF during short-term follow-up (<1 year).
Conclusion: GF for therapeutic angiogenesis was beneficial for increasing LVEF during short-term follow-up (<1 year), however, the therapy was not efficacious in decreasing all-cause mortality, MACE and revascularization.
1 Introduction
Ischemic heart disease (IHD) is the major cause of death all over the world according to the report of World Health Organization (WHO) (Roth et al., 2020). Even though guideline-based medical therapy and percutaneous coronary intervention (PCI) have been widely used in IHD patients, the 4-year rate of death and myocardial infarction of the patients remain about 10% (Maron et al., 2020). Lots of patients could not benefit from PCI (Ng et al., 2022), and some patients also suffer from refractory angina despite intensive medical therapy (Park and Conti, 2018).
IHD is characterized by decreased coronary blood flow, and increasing blood flow in the area of ischemic myocardium is main therapeutic aim (Heusch, 2016). Therapeutic angiogenesis, including vascular endothelial growth factor (VEGF), placental growth factor (PLGF), fibroblast growth factor (FGF), hepatocyte growth factor (HGF), platelet-derived growth factor (PDGF), angiopoietin (Ang) and erythropoietin (EPO), might be novel treatment options for IHD patients. Previous animal study demonstrated that therapeutic angiogenesis could increase blood flow and local blood vessel numbers in the area of ischemia (Shams et al., 2022). However, the results are controversial in clinical trials. Voors et al. (2010) found that GF for therapeutic angiogenesis represented by EPO was related to less major adverse cardiovascular events (MACE). Nevertheless, the study performed by Steppich et al. (2017) showed that GF for therapeutic angiogenesis did not promote target vessel revascularization in patients with IHD compared with placebo. Therefore, the present meta-analysis of randomized controlled trials (RCTs) was designed to assess the effect of therapeutic angiogenesis on IHD patients.
2 Methods
2.1 Data source and searches
The meta-analysis was performed according to the Preferred Reporting Items for Systematic Reviews and Meta-Analyses (PRISMA) guidelines (Liberati et al., 2009), and the protocol was registered in INPLASY (No.2022110041, https://inplasy.com/). Two investigators (TL and ZSS) independently performed the database search and study selection. PubMed, EMBASE, and CENTRAL database were searched with the terms: (“vascular endothelial growth factor” or “placental growth factor” or “fibroblast growth factor” “hepatocyte growth factor” or “platelet-derived growth factor” or “angiopoietin” or “erythropoietin”) and (“ischemic heart disease” or “coronary artery disease” or “coronary heart disease”) and (“randomized controlled trial” or “clinical trial”) from inception to October 2022. The detailed search strategies are listed in Supplementary Table S1. We also performed a manual search according to associated published review.
2.2 Study selection
Studies were included if they met the following inclusion criteria: 1) RCT studies comparing GF therapy and standard treatments for IHD; 2) the participants have myocardial hypoperfusion according to perfusion imaging, and have been diagnosed as acute coronary syndrome or chronic ischemic heart disease (Buja and Vander Heide, 2016); 3) reported the outcome including all-cause mortality, MACE, revascularization, left ventricular ejection fraction (LVEF), or Canadian Cardiovascular Society (CCS) angina class at least one. The studies were excluded if: 1) the data of outcome was not available 2) the studies were published as comments, conference abstracts, or letters to the editor.
2.3 Data extraction and quality assessment
Two investigators (TL and ZSS) extracted data from included studies independently. The disagreements would be resolved by consulting a third investigator (FCG). The following study characteristics were collected: first author, publication year, follow-up duration, type of IHD, categories of growth factors for interventions, control, sample size, age at entry, percentage of male participants, and key outcomes.
The primary outcomes of this study were all-cause mortality and MACE, and the second outcomes were revascularization, LVEF and CCS angina class. When the data of outcome was unavailable, we will try to connect the corresponding author. Two investigators (TL and ZSS) assessed the risk of bias of the included studies with the Cochrane tool. The categorization of “low risk,” “high risk,” or “some concerns” was applied to the included studies according to the following domains: random sequence generation, allocation concealment, blinding of participants and personnel, blinding for outcome assessment, incomplete outcome data, selective reporting, and other potential sources of bias. Disagreements were resolved through discussing with a third investigator (FCG).
2.4 Statistical analysis
Dichotomous outcomes were analyzed with the relative risk (RR) and 95% confidence interval (CI). Continuous outcomes were analyzed with the weighted mean difference (WMD) and 95% CI. I2 statistic was used to measure the heterogeneity among the studies. When I2 ≤ 50%, we will consider that the heterogeneity was not significant among the studies, and fixed-effect models will be used. I2 > 50% indicated that the heterogeneity among the studies was statistically significant, random-effect models will be applied. Meta-regression analysis was conducted for the factors that affected the research results, such as the baseline LVEF values and baseline CCS angina class, to observe their impact on outcomes. If necessary, subgroup analysis based on factors such as type of IHD, categories of growth factors, injection methods and follow-up duration was conducted to clarify their impact on outcome. Sensitivity analysis was used to observe whether the results were reliable after the studies were excluded one by one. Publication bias was evaluated using funnel plots and egger’s test. The data were analyzed with Stata (version 15.1) or Cochrane Collaboration software (RevMan 5.3).
3 Results
3.1 Study selection
The process of study selection was shown as Figure 1. Six hundred and fifty-nine articles (114 from PubMed, 306 from EMBASE, 226 from CENTRAL, and 13 additional records identified through literature review) were identified. Ninety-four articles were excluded for duplication, and remained 484 irrelevant articles were excluded after screening titles and abstracts. The remained 81 full-text articles were then assessed for eligibility, and 52 articles were excluded for no randomized controlled trials (n = 15), improper comparisons (n = 9), irrelevant outcomes and/or unavailable outcomes (n = 16) and inclusion of other confounding factors (n = 12). Finally, 29 articles were included in this meta-analysis (Voors et al., 2010; Steppich et al., 2017; Kukuła et al., 2019; Orii et al., 2018; Minamino et al., 2018; Meng et al., 2018; Ziabakhsh-Tabary et al., 2014; Fokkema et al., 2013; Prunier et al., 2012; Chih et al., 2012; Najjar et al., 2011; Kukuła et al., 2011; Ludman et al., 2011; Kastrup et al., 2011; Ozawa et al., 2010; Ott et al., 2010; Wang et al., 2009; Stewart et al., 2009; Hedman et al., 2009; Kastrup et al., 2005; Henry et al., 2003; Stewart et al., 2006).
3.2 Quality assessment
The quality of included studies were assessed based on seven aspects of risk biases, including random sequence generation, allocation concealment, blinding of participants and personnel, blinding for outcome assessment, incomplete outcome data, selective reporting, and other potential sources of bias. The results of quality assessment were shown as Figure 2. Overall, no attrition bias or reporting bias was observed, and the methods of random and blinding were considered to be adequate in this meta-analysis, but there was an unclear risk in allocation concealment.
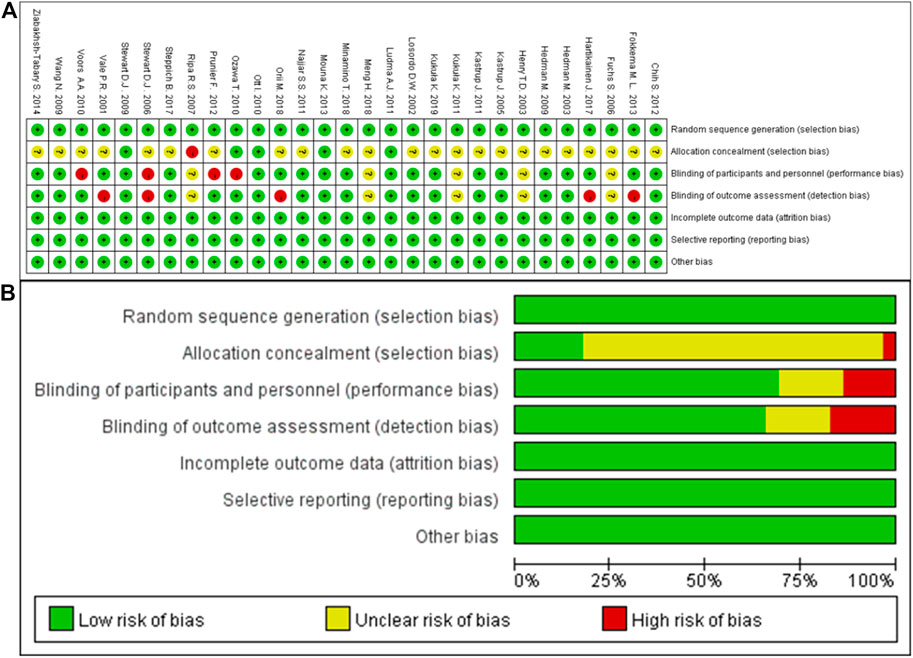
FIGURE 2. Risk of bias. (A), Risk of bias summary: each risk of bias item for each included study; (B), risk of bias graph: each risk of bias item presented as percentages across all included studies.
3.3 Characteristics of included studies
The studies were published between 2001 and 2019. A total of 2899 IHD patients, aged from 56 to 72 years old, were included in the analysis. Six of the 29 studies contained 2 arms (Hedman et al., 2003; Henry et al., 2003; Ripa et al., 2006; Hedman et al., 2009; Minamino et al., 2018; Orii et al., 2018). The GFs involved VEGF, EPO, HGF, and Granulocyte Colony-Stimulating Factor (G-CSF), of which VEGF was subtyped into phVEGF, VEGF-A165, VEGF-A121, rhVEGF, and VEGF-D. Overall, 29 studies involving 2899 IHD patients (1,577 patients in GF group and 1,322 patients in control group) were included (Table 1).
3.4 Primary outcomes
3.4.1 All-cause mortality
Fourteen studies (Steppich et al., 2017; Kukuła et al., 2019; Fokkema et al., 2013; Prunier et al., 2012; Najjar et al., 2011; Ludman et al., 2011; Ott et al., 2010; Stewart et al., 2009; Hedman et al., 2009; Henry et al., 2003; Hedman et al., 2003; Hartikainen et al., 2017), including 1872 IHD patients (952 patients in GF group vs. 920 patients in control group), evaluated the effects of GF on all-cause mortality. The results demonstrated that there was no statistical difference between the GF therapy group and the control group in decreasing all-cause mortality (RR: 0.82; 95% CI: 0.54–1.24; p = 0.341) (Figure 3). And no obvious heterogeneity between studies was observed (I2 = 0.0%, p = 0.972). Results from sensitivity analysis showed that exclusion of any single study did not affect the overall estimate for the effects of GF on all-cause mortality. We also performed subgroup analysis based on type of IHD, categories of growth factors, injection methods and follow-up duration, and the results showed that those factors did not influence the final effect size (Supplementary Figures S1–S4; Table 2).
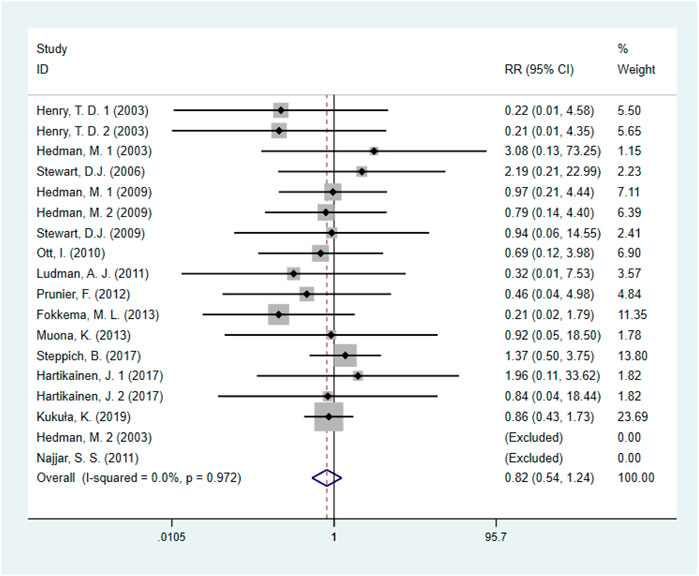
FIGURE 3. Forest plot for all-cause mortality, GF vs. control. RR, relative risk; CI, confidence interval; ID, identification.
3.4.2 Major adverse cardiovascular events
The effect of GF on MACE was evaluated in five studies with 1074 IHD patients (Hedman et al., 2009; Voors et al., 2010; Hartikainen et al., 2017; Steppich et al., 2017; Minamino et al., 2018) (550 patients in GF group vs. 524 patients in control group). The results showed that GF therapy did not significantly decrease the risk of MACE compared to the control group (RR: 0.83; 95% CI: 0.61–1.12; p = 0.227). In addition, low significant heterogeneity was observed regarding this outcome (I2 = 13.4%, p = 0.328) (Figure 4). Subgroup analyses based on type of IHD, categories of growth factors, injection methods and follow-up duration were performed, and the final results were not influenced (Supplementary Figures S5–S8; Table 2). Sensitivity analysis showed that deletion of any one study did not alter the overall estimate for the impact of GF on MACE.
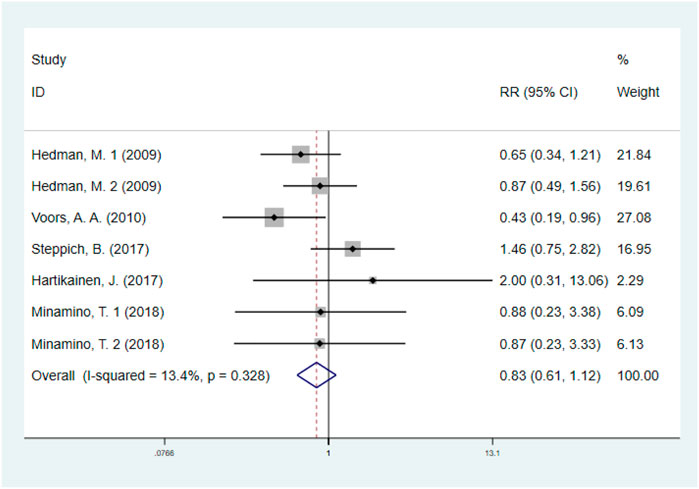
FIGURE 4. Forest plot for MACE, GF vs. control. MACE, major adverse cardiovascular events; RR, relative risk; CI, confidence interval; ID, identification.
3.5 Secondary outcomes
3.5.1 Revascularization
A total of eight studies (Henry et al., 2003; Stewart et al., 2009; Ott et al., 2010; Ludman et al., 2011; Prunier et al., 2012; Fokkema et al., 2013; Steppich et al., 2017; Minamino et al., 2018), including 1513 IHD patients (755 patients in GF group vs. 758 patients in control group), investigated the effects of GF therapy on revascularization. Pooled effect sizes from the eligible studies indicated that there was no significant difference on revascularization between the GF group and control group (RR: 1.27, 95% CI: 0.82–1.96, p = 0.290) (Figure 5). And no obvious heterogeneity was found regarding the outcome (I2 = 0.0%, p = 0.730). Subgroup analyses were performed by type of IHD, categories of GF, injection methods, and follow-up duration, and the results were unchanged, indicating that GF does not increase revascularization in IHD patients (p > 0.05) (Supplementary Figures S9–S12; Table 2). The sensitivity analysis showed that exclusion of any single study did not affect the overall estimate for the effect of GF on revascularization.
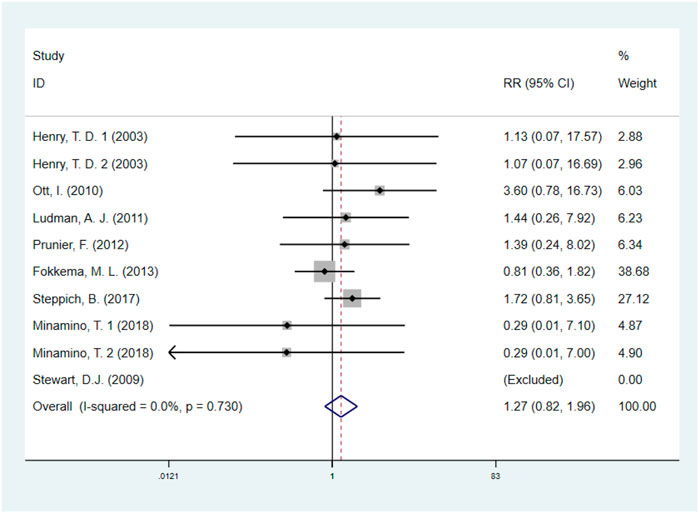
FIGURE 5. Forest plot for revascularization, GF vs. control. RR, relative risk; CI, confidence interval; ID, identification.
3.5.2 Left ventricular ejection fraction
Eighteen studies (Minamino et al., 2018; Ziabakhsh-Tabary et al., 2014; Prunier et al., 2012; Chih et al., 2012; Najjar et al., 2011; Kukuła et al., 2011; Kastrup et al., 2011; Ozawa et al., 2010; Ott et al., 2010; Wang et al., 2009; Kastrup et al., 2005; Henry et al., 2003), including 1,446 patients (750 individuals in GF group vs. 696 individuals in control group) assessed the effect of GF on LVEF. Pooled results showed that GF therapy led to a significantly increase in LVEF (WMD: 2.05; 95% CI: 1.64–2.46; p < 0.001) without significant heterogeneity (I2 = 11.4%, p = 0.301) (Figure 6). Meta-regression analysis demonstrated that there was no significant positive correlation between the effect of GF on LVEF and baseline LVEF (regression = 0.81, p = 0.429) (Figure 7). And subgroup analyses showed that GF therapy increased LVEF both in baseline LVEF ≥50% (WMD 2.81; 95% CI: 2.21–3.42, p = 0.630) and baseline LVEF <50% (WMD 1.42; 95% CI: 0.88–1.97, p = 0.865). In addition, GF therapy increased LVEF only during short-time follow-up (<1 year) (WMD: 2.05; 95% CI: 1.64–2.46; p < 0.001) (Supplementary Figures S13–S17; Table 2).
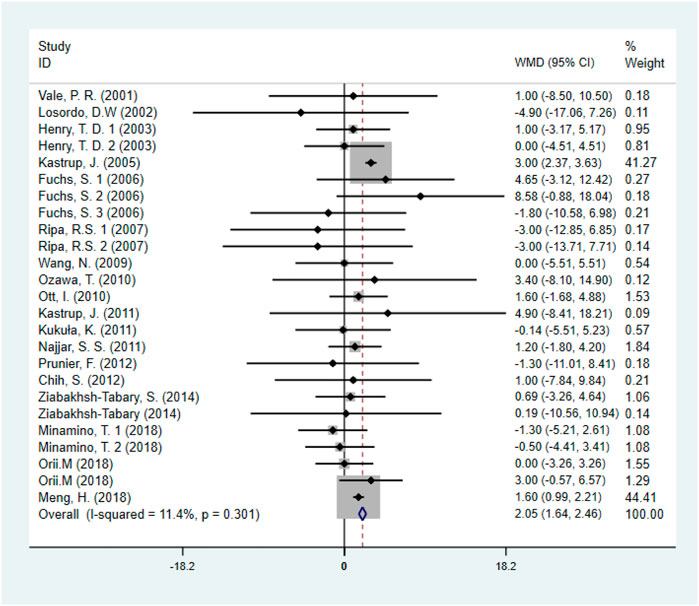
FIGURE 6. Forest plot for LVEF, GF vs. control. LVEF, left ventricular ejection fraction; RR, relative risk; CI, confidence interval; ID, identification.
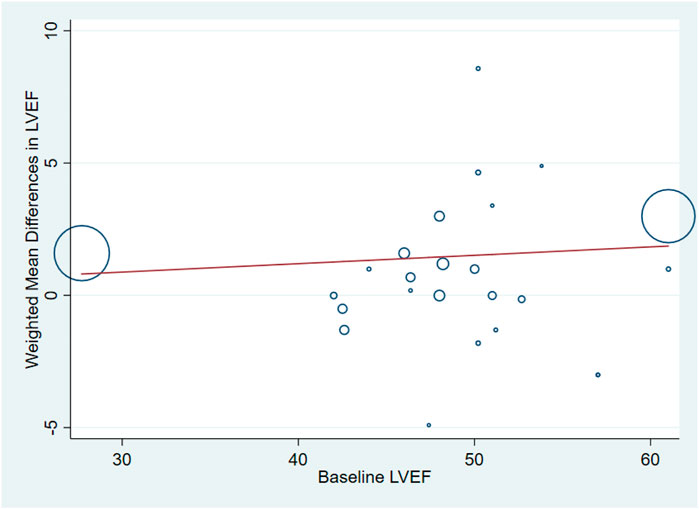
FIGURE 7. Meta-regression plots of the weighted mean difference in LVEF according to baseline LVEF (p = 0.429). LVEF, left ventricular ejection fraction.
3.5.3 Canadian cardiovascular society angina class
Five studies with 531 IHD patients (Losordo et al., 2002; Henry et al., 2003; Kastrup et al., 2005; Kastrup et al., 2011; Hartikainen et al., 2017) (291 individuals in GF group vs. 240 individuals in placebo group), evaluated the effect of GF on CCS angina class. The results demonstrated that there was no significant difference on CCS angina class between GF group and control group (WMD: −0.08, 95% CI: −0.36 to 0.20, p = 0.560) (Figure 8). Obvious heterogeneity between studies was observed (I2 = 96.5%, p < 0.001). Sensitivity analyses showed no obvious changes in overall effect size with the summary WMDs ranged from −0.175 (95% CI: −0.481 to 0.131) to 0.069 (95% CI: −0.195–0.332), when individual studies were eliminated from the analysis. Subgroup analyses were carried out based on type of IHD, injection methods, follow-up duration and baseline CCS angina class, and the results indicated that those factors did not influence the final effect estimates (Supplementary Figures S18–S21; Table 2). Meta-regression analysis showed no statistical correlation between baseline CCS angina class and WMD in CCS angina class (regression = −1.70, p = 0.127) (Figure 9).
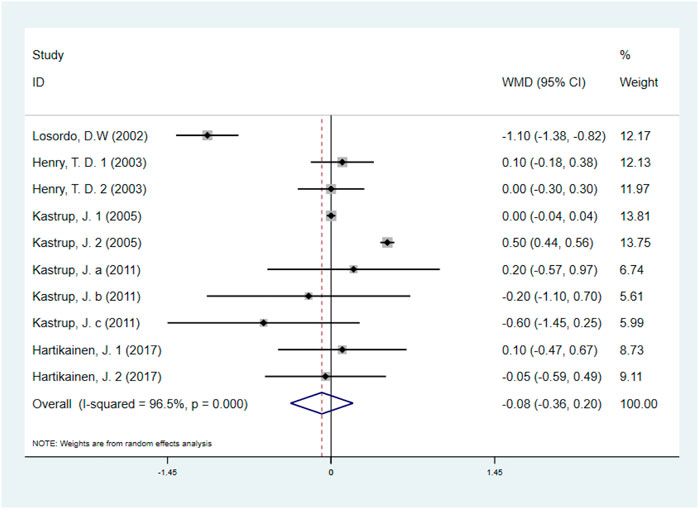
FIGURE 8. Forest plot for CCS angina class, GF vs. control. CCS, Canadian Cardiovascular Society; RR, relative risk; CI, confidence interval; ID, identification.
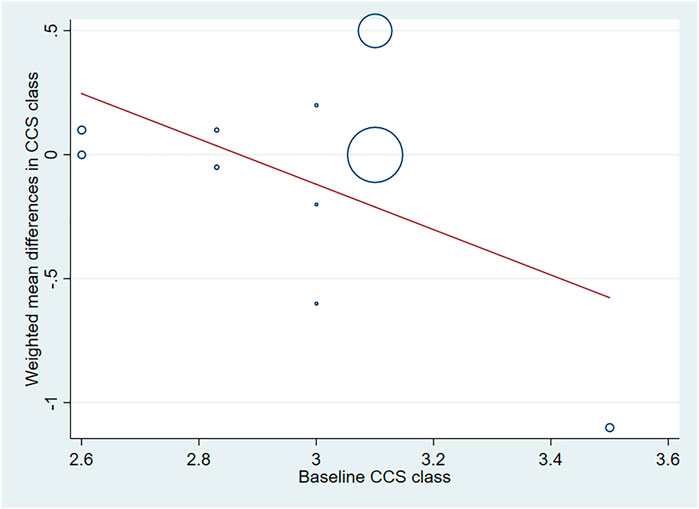
FIGURE 9. Meta-regression plots of the weighted mean difference in CCS angina class according to baseline CCS class (p = 0.127). CCS, Canadian Cardiovascular Society.
3.5.4 Publication bias
According to the visual inspection of funnel plot, a slight asymmetry was observed in the analysis for the effects of GF on LVEF. This was further confirmed by a significant Egger’s test (p < 0.001) (Figure 10). The application of the trim-and-fill method did not change the effect size (WMD 2.051; 95% CI: 1.645–2.457, p < 0.001) (Supplementary Figures S22). The funnel plots created for the visual analysis of the publication bias are presented in Figure 10.
4 Discussion
To our knowledge, the present meta-analysis is the first time to evaluate the effects of GF for therapeutic angiogenesis on IHD patients. The results showed that GF for therapeutic angiogenesis improved LVEF detected by echocardiography, rather than decreased all-cause mortality, MACE and revascularization during follow-up period. Furthermore, GF also did not improve the CCS angina class. Subgroup analysis showed that GF for therapeutic angiogenesis decreased LVEF only in short-term follow-up (<1 year) independent of baseline LVEF. Overall, these evidences supported that GF for therapeutic angiogenesis might be beneficial in improving cardiac function in short-term follow-up, however, they are not effective in decreasing hard endpoints, such as all-cause mortality and MACE.
Developing extensive collateral circulation in ischemic myocardium is a promising therapy for treating IHD. Even though antiplatelet agent and statin are cornerstones for treating IHD, however, previous clinical studies showed that statin and aspirin was effective in decreasing VEGF levels and have no effects on promoting angiogenesis (Dworacka et al., 2014; Cheng et al., 2015). Recently, animal studies showed that VEFG for therapeutic angiogenesis could promotes collateral circulation in mouse heart by recruiting endothelial progenitor cells, and subsequently rescue myocardial tissue after an ischemic insult (Mallick et al., 2022). Transforming growth factor beta (TGF-β1) induces pro-reparative phenotypic changes in epicardial cells in mice after myocardial infarction (Dergilev et al., 2021). However, in the present meta-analysis, we collect most comprehensive data regarding the effects of GF on all-cause mortality, MACE and revascularization, and we found that GF did not decrease the rate of all-cause mortality, MACE and revascularization. Low heterogeneity was observed regarding these outcomes, which increased the robustness of the results. For finding specific population might be beneficial from GF therapy, the subgroup analysis was performed. And we found that the results were stable independent of IHD, categories of growth factors, injection methods and follow-up duration. The effects of GF on CCS angina class were also evaluated, and we found GF therapy could not decrease CCS angina class. Taking together, the results of these studies supported that GF therapy may not effective in improving the prognosis of IHD.
LVEF is a quantitative marker to evaluate cardiac systolic function. previous study showed that patients with preserved left ventricular ejection fraction had lower one and 3-year mortality rates as compared with reduced left ventricular ejection fraction regardless of the acute coronary syndrome period onset (Yahud et al., 2020). Our results showed that GF for therapeutic angiogenesis could increase LVEF by 2.05%. And the subgroup analysis found that GF therapy was only effective during a short-term follow-up (<1 year). The metabolism of GF might lead to a transient effect on cardiac function. What’s more, angiogenesis is a complex biological process that involves degradation of the vascular basement membrane, endothelial cell proliferation, vascular sprouting, lumen formation, and stabilization and maturation of the vascular network. Relying only on a single injection of a singular GF may promote angiogenesis in a short period of time (<1 year), thus improving cardiac function and increasing LVEF, however, the long-term (≥1 year) efficacy on cardiac function is not significant. Moreover, GF showed notable improvement in cardiac function in SCHD and MIHF with stable disease, while it had no efficacy in refractory CAD or STEMI with critical disease. For specific GF categories, VEGF and HGF showed dramatic improvement in LVEF, while EPO and G-CSF had no obvious efficacy. Interestingly, HGF has a beneficial synergistic effect with VEGF. There is a study identified that HGF prominently promotes the effects of VEGF on angiogenesis via the ets-1 pathway (Tomita et al., 2003). Furthermore, in respect to the injection methods, gene transfer therapy is superior to protein injection therapy, because gene transfer can increase LVEF by 2.95%, transcutaneous endocardium injection of GF protein only increased by 1.60%, while intravenous injection, myocardial injection and subcutaneous injection of GF protein could not increase LVEF. We consider that GF should be used more often in the treatment of IHD patients with stable disease conditions. In terms of treatment methods, a combination of multiple synergistic GFs application and more frequent administration can be used in future clinical practice, and the gene transfer mode of delivery is more effective.
There are some limitations in our study. First, there are different injection method of GF, including intramyocardial injections and intracoronary infusion, which might lead to clinical heterogeneity. Thus, we performed subgroup analyses based on injection method, and the effect sizes did not change. Second, the sample size of included studies is relatively small and might lead to less robust results, we would update the meta-analysis when large-scale clinical studies publish. Third, the long-term persistence of the treatment effects is unknown. Most of the trials ranged in duration from 3 to 12 months. Fourth, obvious publication bias was found from egger’s test, which might influence the credibility of present results. Thus, trim-and-fill method was used to evaluate the corrected effect size, and we found that the effect size remained unchanged.
5 Conclusion
Even though GF for therapeutic angiogenesis was beneficial for increasing LVEF during short-term follow-up (<1 year), the therapy was not efficacious in decreasing all-cause mortality and MACE in IHD patients.
Author contributions
HQ, C-GF, and S-SZ conceived the study. LT and S-SZ searched the databases and checked them according to the eligible criteria and exclusion criteria. HQ and F-FL helped develop search strategies. J-ML and W-WY analyzed the data and wrote the draft of the paper. H-ZL and Y-XP contributed to reviewing or revising the paper. C-GF and X-CM are the guarantors of this work. All authors read and approve the final manuscript.
Funding
This study was supported by the Youth Talent Promotion Project of China Association for Science and Technology (No. 2020-QNRCI-02) and the Science and Technology Innovation Project of China Academy of Chinese Medical Sciences (No. CI 2021A05013).
Conflict of interest
The authors declare that the research was conducted in the absence of any commercial or financial relationships that could be construed as a potential conflict of interest.
The reviewer BZ declared a shared parent affiliation with the authors H-ZL, Y-XP, J-ML, and F-FL to the handling editor at the time of review.
Publisher’s note
All claims expressed in this article are solely those of the authors and do not necessarily represent those of their affiliated organizations, or those of the publisher, the editors and the reviewers. Any product that may be evaluated in this article, or claim that may be made by its manufacturer, is not guaranteed or endorsed by the publisher.
Supplementary material
The Supplementary Material for this article can be found online at: https://www.frontiersin.org/articles/10.3389/fcell.2022.1095623/full#supplementary-material
References
Buja, L. M., and Vander Heide, R. S. (2016). Pathobiology of ischemic heart disease: Past, present and future. Cardiovasc. Pathol. 25 (3), 214–220. doi:10.1016/j.carpath.2016.01.007
Cheng, Y., Liu, J., Wang, Y., Yan, W., and Zhang, M. (2015). Decreased vascular endothelial growth factor expression is associated with cell apoptosis in low-dose aspirin-induced gastric mucosal injury. Am. J. Med. Sci. 349 (2), 110–116. doi:10.1097/MAJ.0000000000000409
Chih, S., Macdonald, P. S., McCrohon, J. A., Ma, D., Moore, J., Feneley, M. P., et al. (2012). Granulocyte colony stimulating factor in chronic angina to stimulate neovascularisation: A placebo controlled crossover trial. Heart (British Card. Soc. 98 (4), 282–290. doi:10.1136/heartjnl-2011-300751
Dergilev, K. V., Tsokolaeva, Z. I., Beloglazova, I. B., Ratner, E. I., and Parfenova, E. V. (2021). Transforming growth factor beta (TGF-β1) induces pro-reparative phenotypic changes in epicardial cells in mice. Bull. Exp. Biol. Med. 170 (4), 565–570. doi:10.1007/s10517-021-05107-5
Dworacka, M., Krzyzagorska, E., Wesolowska, A., Borowska, M., Iskakova, S., and Dworacki, G. (2014). Statins in low doses reduce VEGF and bFGF serum levels in patients with type 2 diabetes mellitus. Pharmacology 93 (1-2), 32–38. doi:10.1159/000357476
Fokkema, M. L., Kleijn, L., van der Meer, P., Belonje, A. M., Achterhof, S. K., Hillege, H. L., et al. (2013). Long term effects of epoetin alfa in patients with ST- elevation myocardial infarction. Cardiovasc. Drugs Ther. 27 (5), 433–439. doi:10.1007/s10557-013-6470-0
Fuchs, S., Dib, N., Cohen, B. M., Okubagzi, P., Diethrich, E. B., Campbell, A., et al. (2006). A randomized, double-blind, placebo-controlled, multicenter, pilot study of the safety and feasibility of catheter-based intramyocardial injection of AdVEGF121 in patients with refractory advanced coronary artery disease. Catheter. Cardiovasc. Interv. 68 (3), 372–378. doi:10.1002/ccd.20859
Hartikainen, J., Hassinen, I., Hedman, A., Kivela, A., Saraste, A., Knuuti, J., et al. (2017). Adenoviral intramyocardial VEGF-ddndc gene transfer increases myocardial perfusion reserve in refractory angina patients: A phase I/IIa study with 1-year follow-up. Eur. Heart J. 38 (33), 2547–2555. doi:10.1093/eurheartj/ehx352
Hedman, M., Hartikainen, J., Syvanne, M., Stjernvall, J., Hedman, A., Kivela, A., et al. (2003). Safety and feasibility of catheter-based local intracoronary vascular endothelial growth factor gene transfer in the prevention of postangioplasty and in-stent restenosis and in the treatment of chronic myocardial ischemia: Phase II results of the kuopio angiogenesis trial (KAT). Circulation 107 (21), 2677–2683. doi:10.1161/01.CIR.0000070540.80780.92
Hedman, M., Muona, K., HedmAn, A., Kivela, A., SyvanneM., , Eranen, J., et al. (2009). Eight-year safety follow-up of coronary artery disease patients after local intracoronary VEGF gene transfer. Gene Ther. 16 (5), 629–634. doi:10.1038/gt.2009.4
Henry, T. D., Annex, B. H., McKendall, G. R., Azrin, M. A., Lopez, J. J., Giordano, F. J., et al. (2003). The VIVA trial: Vascular endothelial growth factor in ischemia for vascular angiogenesis. Circulation 107 (10), 1359–1365. doi:10.1161/01.cir.0000061911.47710.8a
Heusch, G. (2016). Myocardial ischemia: Lack of coronary blood flow or myocardial oxygen supply/demand imbalance? Circ. Res. 119 (2), 194–196. doi:10.1161/CIRCRESAHA.116.308925
Kastrup, J., Jorgensen, E., Fuchs, S., Nikol, S., Botker, H. E., Gyongyosi, M., et al. (2011). A randomised, double-blind, placebo-controlled, multicentre study of the safety and efficacy of BIOBYPASS (AdGVVEGF121.10NH) gene therapy in patients with refractory advanced coronary artery disease: The NOVA trial. EuroIntervention 6 (7), 813–818. doi:10.4244/EIJV6I7A140
Kastrup, J., Jorgensen, E., Ruck, A., Tagil, K., Glogar, D., Ruzyllo, W., et al. (2005). Direct intramyocardial plasmid vascular endothelial growth factor-a165 gene therapy in patients with stable severe angina pectoris A randomized double-blind placebo-controlled study: The euroinject one trial. J. Am. Coll. Cardiol. 45 (7), 982–988. doi:10.1016/j.jacc.2004.12.068
Kukuła, K., Chojnowska, L., Dabrowski, M., Witkowski, A., Chmielak, Z., Skwarek, M., et al. (2011). Intramyocardial plasmid-encoding human vascular endothelial growth factor A165/basic fibroblast growth factor therapy using percutaneous transcatheter approach in patients with refractory coronary artery disease (VIF-CAD). Am. Heart J. 161 (3), 581–589. doi:10.1016/j.ahj.2010.11.023
Kukuła, K., Urbanowicz, A., Klopotowski, M., Dabrowski, M., Pregowski, J., Kadziela, J., et al. (2019). Long-term follow-up and safety assessment of angiogenic gene therapy trial VIF-CAD: Transcatheter intramyocardial administration of a bicistronic plasmid expressing VEGF-A165/bFGF cDNA for the treatment of refractory coronary artery disease. Am. Heart J. 215, 78–82. doi:10.1016/j.ahj.2019.06.009
Liberati, A., Altman, D. G., Tetzlaff, J., Mulrow, C., Gotzsche, P. C., Ioannidis, J. P. A., et al. (2009). The PRISMA statement for reporting systematic reviews and meta-analyses of studies that evaluate health care interventions: Explanation and elaboration. J. Clin. Epidemiol. 62 (10), e1–e34. doi:10.1016/j.jclinepi.2009.06.006
Losordo, D. W., Vale, P. R., Hendel, R. C., Milliken, C. E., Fortuin, F. D., Cummings, N., et al. (2002). Phase 1/2 placebo-controlled, double-blind, dose-escalating trial of myocardial vascular endothelial growth factor 2 gene transfer by catheter delivery in patients with chronic myocardial ischemia. Circulation 105 (17), 2012–2018. doi:10.1161/01.cir.0000015982.70785.b7
Ludman, A. J., Yellon, D. M., Hasleton, J., Ariti, C., Babu, G. G., Boston-Griffiths, E., et al. (2011). Effect of erythropoietin as an adjunct to primary percutaneous coronary intervention: A randomised controlled clinical trial. Heart (British Card. Soc. 97 (19), 1560–1565. doi:10.1136/hrt.2011.223867
Mallick, R., Gurzeler, E., Toivanen, P. I., Nieminen, T., and Yla-Herttuala, S. (2022). Novel designed proteolytically resistant VEGF-B186R127S promotes angiogenesis in mouse heart by recruiting endothelial progenitor cells. Front. Bioeng. Biotechnol. 10, 907538. doi:10.3389/fbioe.2022.907538
Maron, D. J., Hochman, J. S., Reynolds, H. R., Bangalore, S., O'Brien, S. M., Boden, W. E., et al. (2020). Initial invasive or conservative strategy for stable coronary disease. N. Engl. J. Med. 382 (15), 1395–1407. doi:10.1056/NEJMoa1915922
Meng, H., Chen, B., Tao, Z., Xu, Z., Wang, L., Weizhu, J., et al. (2018). Safety and efficacy of adenovirus carrying hepatocyte growth factor gene by percutaneous endocardial injection for treating post-infarct heart failure: A phase IIa clinical trial. Curr. Gene Ther. 18 (2), 125–130. doi:10.2174/1566523218666180404162209
Minamino, T., Higo, S., Araki, R., Hikoso, S., Nakatani, D., Suzuki, H., et al. (2018). Low-dose erythropoietin in patients with ST-segment elevation myocardial infarction (EPO-AMI-II): ― A randomized controlled clinical trial. Circ. J. 82 (4), 1083–1091. doi:10.1253/circj.cj-17-0889
Muona, K., Hedman, M., and Hedman, A. (2013). Intramyocardial adenovirus-mediated VEGF-dî?nî”C gene transfer in patients with No-option coronary artery disease: Interim safety analysis of the kuopio angiogenesis trial 301. Int. J. Cardiovasc. Res. 02. doi:10.4172/2324-8602.1000150
Najjar, S. S., Rao, S. V., Melloni, C., Raman, S. V., Povsic, T. J., Melton, L., et al. (2011). Intravenous erythropoietin in patients with ST-segment elevation myocardial infarction: Reveal: A randomized controlled trial. JAMA 305 (18), 1863–1872. doi:10.1001/jama.2011.592
Ng, A. K., Ng, P. Y., Ip, A., Ling, I. W. H., Lam, L. T., and Siu, C. W. (2022). Incidence, prediction, and outcomes of major bleeding after percutaneous coronary intervention in Chinese patients. JACC. Asia 2 (3), 341–350. doi:10.1016/j.jacasi.2021.12.009
Orii, M., Hirata, K., Takemoto, K., and Akasaka, T. (2018). Effect of erythropoietin administration on myocardial viability and coronary microvascular dysfunction in anterior acute myocardial infarction: Randomized controlled trial in the Japanese population. Cardiol. Ther. 7 (2), 151–162. doi:10.1007/s40119-018-0122-1
Ott, I., Schulz, S., Mehilli, J., Fichtner, S., Hadamitzky, M., Hoppe, K., et al. (2010). Erythropoietin in patients with acute ST-segment elevation myocardial infarction undergoing primary percutaneous coronary intervention: A randomized, double-blind trial. Circ. Cardiovasc. Interv. 3 (5), 408–413. doi:10.1161/CIRCINTERVENTIONS.109.904425
Ozawa, T., Toba, K., Suzuki, H., Kato, K., Iso, Y., Akutsu, Y., et al. (2010). Single-dose intravenous administration of recombinant human erythropoietin is a promising treatment for patients with acute myocardial infarction - randomized controlled pilot trial of EPO/AMI-1 study. Circ. J. 74 (7), 1415–1423. doi:10.1253/circj.cj-10-0109
Park, K. E., and Conti, C. R. (2018). Non-PCI/CABG therapies for refractory angina. Trends cardiovasc. Med. 28 (3), 223–228. doi:10.1016/j.tcm.2017.10.002
Prunier, F., Biere, L., Gilard, M., Boschat, J., Mouquet, F., Bauchart, J. J., et al. (2012). Single high-dose erythropoietin administration immediately after reperfusion in patients with ST-segment elevation myocardial infarction: Results of the erythropoietin in myocardial infarction trial. Am. Heart J. 163 (2), 200–207. doi:10.1016/j.ahj.2011.11.005
Ripa, R. S., Wang, Y., Jorgensen, E., Johnsen, H. E., Hesse, B., and Kastrup, J. (2006). Intramyocardial injection of vascular endothelial growth factor-A165 plasmid followed by granulocyte-colony stimulating factor to induce angiogenesis in patients with severe chronic ischaemic heart disease. Eur. Heart J. 27 (15), 1785–1792. doi:10.1093/eurheartj/ehl117
Roth, G. A., Mensah, G. A., Johnson, C. O., Addolorato, G., Ammirati, E., Baddour, L. M., et al. (2020). Global burden of cardiovascular diseases and risk factors, 1990-2019: Update from the GBD 2019 study. J. Am. Coll. Cardiol. 76 (25), 2982–3021. doi:10.1016/j.jacc.2020.11.010
Shams, F., Moravvej, H., Hosseinzadeh, S., Mostafavi, E., Bayat, H., Kazemi, B., et al. (2022). Overexpression of VEGF in dermal fibroblast cells accelerates the angiogenesis and wound healing function: In vitro and in vivo studies. Sci. Rep. 12 (1), 18529. doi:10.1038/s41598-022-23304-8
Steppich, B., Groha, P., Ibrahim, T., Schunkert, H., Laugwitz, K. L., Hadamitzky, M., et al. (2017). Effect of erythropoietin in patients with acute myocardial infarction: Five-year results of the REVIVAL-3 trial. BMC Cardiovasc. Disord. 17 (1), 38. doi:10.1186/s12872-016-0464-3
Stewart, D. J., Hilton, J. D., Arnold, J. M. O., Gregoire, J., RivArd, A., Archer, S. L., et al. (2006). Angiogenic gene therapy in patients with nonrevascularizable ischemic heart disease: A phase 2 randomized, controlled trial of AdVEGF(121) (AdVEGF121) versus maximum medical treatment. Gene Ther. 13 (21), 1503–1511. doi:10.1038/sj.gt.3302802
Stewart, D. J., Kutryk, M. J. B., Fitchett, D., Freeman, M., Camack, N., Su, Y., et al. (2009). VEGF gene therapy fails to improve perfusion of ischemic myocardium in patients with advanced coronary disease: Results of the NORTHERN trial. Mol. Ther. 17 (6), 1109–1115. doi:10.1038/mt.2009.70
Tomita, N., Morishita, R., Taniyama, Y., Koike, H., Aoki, M., Shimizu, H., et al. (2003). Angiogenic property of hepatocyte growth factor is dependent on upregulation of essential transcription factor for angiogenesis, ets-1. Circulation 107 (10), 1411–1417. doi:10.1161/01.cir.0000055331.41937.aa
Vale, P. R., Losordo, D. W., Milliken, C. E., McDonald, M. C., Gravelin, L. M., Curry, C. M., et al. (2001). Randomized, single-blind, placebo-controlled pilot study of catheter-based myocardial gene transfer for therapeutic angiogenesis using left ventricular electromechanical mapping in patients with chronic myocardial ischemia. Circulation 103 (17), 2138–2143. doi:10.1161/01.cir.103.17.2138
Voors, A. A., Belonje, A. M. S., Zijlstra, F., Hillege, H. L., Anker, S. D., Slart, R. H. J. A., et al. (2010). A single dose of erythropoietin in ST-elevation myocardial infarction. Eur. Heart J. 31 (21), 2593–2600. doi:10.1093/eurheartj/ehq304
Wang, N., Tong, G., Yang, J., Zhou, Z., Pan, H., Huo, Y., et al. (2009). Effect of hepatocyte growth-promoting factors on myocardial ischemia during exercise in patients with severe coronary artery disease. Int. Heart J. 50 (3), 291–299. doi:10.1536/ihj.50.291
Yahud, E., Tzuman, O., Fink, N., Goldenberg, I., Goldkorn, R., Peled, Y., et al. (2020). Trends in long-term prognosis according to left ventricular ejection fraction after acute coronary syndrome. J. Cardiol. 76 (3), 303–308. doi:10.1016/j.jjcc.2020.03.012
Ziabakhsh-Tabary, S., Jalalian, R., Mokhtari-Esbuie, F., and Habibi, M. R. (2014). Echocardiographic evaluation of the effects of a single bolus of erythropoietin on reducing ischemia-reperfusion injuries during coronary artery bypass graft surgery; a randomized, double-blind, placebo-control study. Iran. J. Med. Sci. 39 (2), 94–101.
Keywords: growth factor, therapeutic angiogenesis, ischemic heart disease, meta-analysis, randomized controlled study
Citation: Tan L, Long L-Z, Li H-Z, Yang W-W, Peng Y-X, Lu J-M, Liao F-F, Ma X-C, Qu H, Fu C-G and Zhang S-S (2022) Growth factor for therapeutic angiogenesis in ischemic heart disease: A meta-analysis of randomized controlled trials. Front. Cell Dev. Biol. 10:1095623. doi: 10.3389/fcell.2022.1095623
Received: 11 November 2022; Accepted: 24 November 2022;
Published: 09 December 2022.
Edited by:
Chao Li, Shandong University of Traditional Chinese Medicine, ChinaReviewed by:
Baochen Zhu, Dongzhimen Hospital, Beijing University of Chinese Medicine, ChinaLiu Zhao, China-Japan Friendship Hospital, China
Copyright © 2022 Tan, Long, Li, Yang, Peng, Lu, Liao, Ma, Qu, Fu and Zhang. This is an open-access article distributed under the terms of the Creative Commons Attribution License (CC BY). The use, distribution or reproduction in other forums is permitted, provided the original author(s) and the copyright owner(s) are credited and that the original publication in this journal is cited, in accordance with accepted academic practice. No use, distribution or reproduction is permitted which does not comply with these terms.
*Correspondence: Hua Qu, hua_qu@yeah.net; Chang-Geng Fu, fucgbs@163.com; Shan-Shan Zhang, zhangshanshan0533@163.com
†These authors have contributed equally to this work and share first authorship