- 1Department of Biology, Indiana University-Purdue University Indianapolis, Indianapolis, IN, United States
- 2Stark Neurosciences Research Institute, Indiana University, Indianapolis, IN, United States
- 3Center for Diabetes and Metabolic Diseases, Indiana University School of Medicine, Indianapolis, IN, United States
A subset of genetic disorders termed ciliopathies are associated with obesity. The mechanisms behind cilia dysfunction and altered energy homeostasis in these syndromes are complex and likely involve deficits in both development and adult homeostasis. Interestingly, several cilia-associated gene mutations also lead to morbid obesity. While cilia have critical and diverse functions in energy homeostasis, including their roles in centrally mediated food intake and peripheral tissues, many questions remain. Here, we briefly discuss syndromic ciliopathies and monogenic cilia signaling mutations associated with obesity. We then focus on potential ways neuronal cilia regulate energy homeostasis. We discuss the literature around cilia and leptin-melanocortin signaling and changes in ciliary G protein-coupled receptor (GPCR) signaling. We also discuss the different brain regions where cilia are implicated in energy homeostasis and the potential for cilia dysfunction in neural development to contribute to obesity. We close with a short discussion on the challenges and opportunities associated with studies looking at neuronal cilia and energy homeostasis. This review highlights how neuronal cilia-mediated signaling is critical for proper energy homeostasis.
Introduction
Primary cilia are sensory, cellular appendages that regulate many signaling pathways (Wheway et al., 2018). Dysfunction of cilia leads to many pleiotropic syndromes collectively known as ciliopathies (Reiter and Leroux, 2017). Several ciliopathies such as Bardet-Biedl syndrome (BBS) and Alström Syndrome (ALMS) share a defining clinical feature of pediatric obesity. Additionally, there are many cilia-associated proteins, including G-protein coupled receptors (GPCRs) and signaling molecules [e.g., adenylyl cyclase 3 (ADCY3)], whose functions within the cilium are crucial for regulating energy homeostasis (Figure 1) (Vaisse et al., 2017; Engle et al., 2021). As obesity is a growing health concern, understanding how cilia regulate energy homeostasis and how their dysfunction contributes to this disease proves to be an important endeavor.
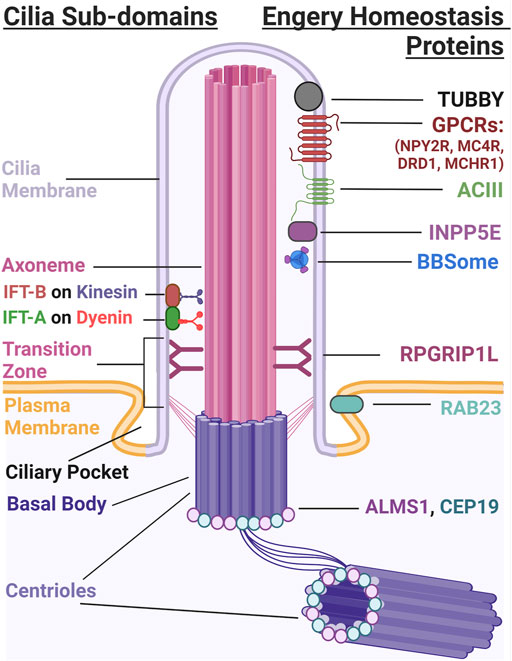
FIGURE 1. The Primary Cilium. The primary cilium has several subdomains that include the cilia membrane, axoneme, transition zone, ciliary pocket, and the basal body. IFT-A and IFT-B use dynein and kinesin motors to build and maintain cilia structure. Several different proteins localize to the cilium that are important for energy homeostasis. These include TUBBY (Mukhopadhyay and Jackson, 2013), GPCRs such as NPY2R, MCHR1, DRD1, and MC4R, ADCY3, and INPP5E which localize to the ciliary membrane. BBSome proteins traffic proteins to and from the ciliary membrane and RGRIP1L, RAB23, ALMS1 and CEP19 localize to the transition zone and base of the cilium, respectively.
This review will briefly describe human ciliopathies associated with obesity and currently available data on clinical trials. We also describe genetic mouse models of ciliopathies and altered cilia-associated proteins that have led to new insights on mechanisms cilia use to regulate body weight. We will focus on the importance of neuronal cilia in regulating energy homeostasis by looking at conditional genetic mouse models which have implicated the hypothalamus and other brain regions. We also address potential neurodevelopmental roles for cilia in obesity, followed by a discussion suggesting that neuronal cilia within the hypothalamus play a prominent role in regulating energy homeostasis. Future studies addressing how cilia influence neuronal activity through various mechanisms may reveal targets to treat this health concern.
Human ciliopathies associated with obesity
Defects in cilia formation, structure, maintenance, and function are associated with a syndromic group of diseases called ciliopathies. Ciliopathies present with a wide variety of clinical features affecting nearly all tissues and organ systems. One shared clinical feature of several ciliopathies is childhood obesity (Table 1) (Reiter and Leroux, 2017).
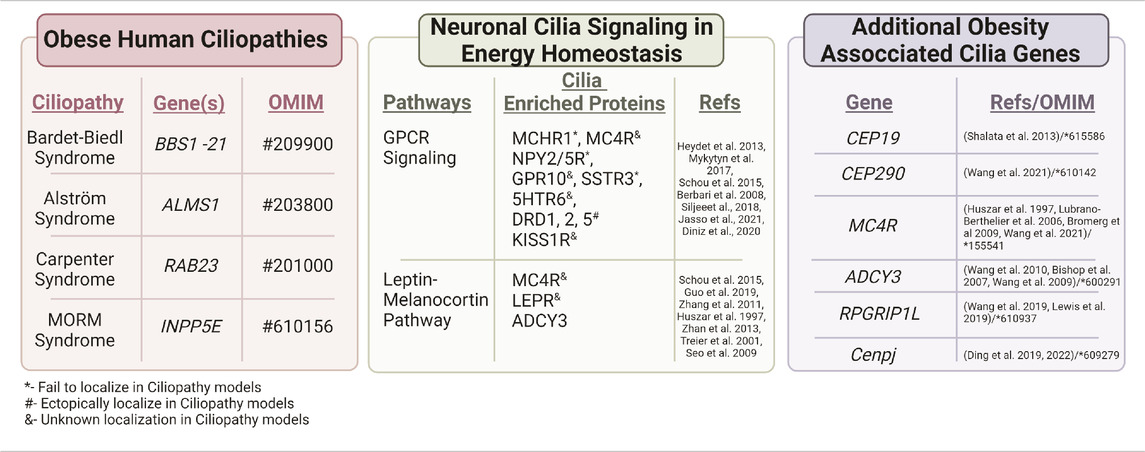
TABLE 1. Obesity associated ciliopathies and genes. Several human ciliopathies share a common clinical feature, obesity. These include Bardet-Biedl syndrome, Alström syndrome, Carpenter syndrome, and MORM syndrome. Neuronal cilia also regulate energy homeostasis through different signaling pathways such as GPCR signaling and the Leptin-melanocortin Pathway (Seo et al., 2009; Obradovic et al., 2021; Yang et al., 2022b) that have proteins enriched along the cilia membrane. Additional cilia genes that are associated with obesity include CEP19, CEP290 (Leitch et al., 2008), MC4R, ADCY 3, and RPGRIP1L. Several of these cilia-enriched proteins show altered localization in ciliopathy models.
Alström syndrome (ALMS, OMIM #203800)
ALMS was first characterized in 1959 as an autosomal recessive disorder that affects less than one out of one million individuals (Tsang et al., 2018). Unlike most ciliopathies, ALMS is associated with mutations in a single gene, ALMS1. ALMS1 protein localizes to the base of the cilium and is suggested to have a role in cilia formation and stability (Hearn et al., 2005). Interestingly, most human mutations of ALMS1 lead to a truncated form of the protein that does not affect cilia formation (Hearn et al., 2005). However, these ALMS1 mutations inhibit proper cilia function and affect the long-term maintenance of the cilium (Hearn et al., 2002; Hearn et al., 2005; Knorz et al., 2010). Along with obesity, ALMS features severe insulin resistance associated with Type 2 diabetes mellitus, rod-cone dystrophy, cardiomyopathy, male infertility, and hearing loss (Mihai et al., 2008). ALMS patients do not necessarily show outward signs of neurological issues; however, brain abnormalities such as enlarged ventricles, grey and white matter atrophy, and diffuse supratentorial white matter derangement, demonstrates cilia play critical roles in the human CNS (Citton et al., 2013).
Bardet-Biedl syndrome (BBS, OMIM #209900)
Similar to ALMS, BBS is another autosomal recessive disorder characterized by pediatric obesity; however, this syndrome is more common than ALMS, affecting around 1 in 100,000 individuals in North America and Europe (Forsythe et al., 2018). Many BBS patients have normal birth weight with obesity occurring in early childhood. Currently, more than 20 genes are implicated in cases of BBS, including BBS1-21 (Rohrschneider and Bolz, 2020), and most BBS proteins localize at cilia or near the base of the cilium. Eight of these gene products (BBS1, 2, 4, 5, 7, 8, 9, and 18) form the BBSome (Niederlova et al., 2019). The BBSome is a ciliary transport protein complex that mediates cargo trafficking to the base of, and removal from, the cilia membrane (Nachury et al., 2007; Jin and Nachury, 2009; Jin et al., 2010; Ye et al., 2018; Shinde et al., 2020). Different BBS mutations result in different degrees of obesity and other diagnostic phenotypes such as polydactyly, retinal degeneration, renal cysts, and hypogonadism (Forsythe et al., 2017; Niederlova et al., 2019). For example, mutations in BBS10 are more often associated with an earlier onset of obesity when compared to mutations in BBS1 (Pomeroy et al., 2021). Interestingly, BBS genes are important for the development of the central nervous system. For example, MRI scans of BBS patients show brain abnormalities including hypothalamic hypoplasia (Keppler-Noreuil et al., 2011), and empty sella of the pituitary (Soliman et al., 1996), both of which could contribute to growth and energy homeostasis features of the syndrome. Mouse models of BBS also show brain phenotypes like hippocampal hypoplasia and hydrocephalus (Bennouna-Greene et al., 2011; Singh et al., 2019).
Other ciliopathies associated with obesity
Other rare disorders associated with cilia dysfunction and obesity are Carpenter and MORM Syndrome. Carpenter syndrome (CRPT1 OMIM #201000) is a developmental disorder characterized by obesity, craniofacial malformations, polysyndactyly, and intellectual disability (Hor et al., 2018). MORM syndrome (OMIM #610156) is classified by Mental retardation, Obesity, Retinal dystrophy, and Micropenis (Hakim et al., 2012). Homozygous null mutations in the Rab-GTPase, RAB23, lead to Carpenter syndrome. RAB23 protein is important for ciliary trafficking of receptors and proteins and is a negative regulator of hedgehog (HH) signaling. Mutations in the inositol polyphosphate-5-phosphatase E (INPP5E) gene lead to MORM syndrome (Zhang et al., 2022). INPP5E is a ciliary transition zone protein that is associated with establishing the different ciliary membrane compartments required to regulate signaling (Figure 1).
All of these syndromes are associated with mutations in genes important for cilia function, structure, and maintenance. Thus, cilia function is required for proper feeding behaviors and their dysfunction leads to obesity. However, the mechanisms that would link altered cilia function to obesity remain to be elucidated. It is likely that there are both developmental and adult homeostatic roles for cilia in energy homeostasis, and that cilia dysfunction impinges on their roles in the neuronal control of food intake and in peripheral tissues associated with energy homeostasis. Further studies to identify direct associations between ciliopathy gene function and energy homeostasis could identify potential therapeutic targets for these diseases.
Clinical trials for ciliopathy associated obesity
Despite over 70% of BBS patients being overweight and obese, studies to elucidate the relationship between BBS genotypes and obese phenotypes have not revealed strong genotype-phenotype correlations (Pomeroy et al., 2021). This suggests that genetic background and the nature of the ciliopathy mutations and environment can play roles in the energy homeostasis phenotypes. Recently, one group used the Clinical Registry Investigating Bardet-Biedl Syndrome (CRIBBS) to look at the prevalence of obesity in BBS patients (Pomeroy et al., 2021). Children with BBS are often born with normal gestational length and weight but go on to show rapid weight gain and obesity in early childhood through adolescence. Loss of function variants in BBS genes are associated with a high risk for severe obesity (Pomeroy et al., 2021). Uncovering the specific genotypes of ciliopathy patients may help optimize the timing of potential therapies used to treat obesity. Previous trials for weight loss studies in Prader-Willi syndrome (PWS, OMIM #176270) used Methionine aminopeptidase 2 inhibitors (MetAP2i). These same inhibitors were shown to reduce hyperphagia in obese ciliopathy mouse models (Pottorf et al., 2020). While these studies showed reductions in weight and food intake in PWS patients, these trials have been suspended. More recently, a melanocortin-4 receptor (MC4R) agonist, Setmelanotide, has progressed to phase 3 trials (Clément et al., 2020). This drug is being tested to treat obesity in BBS and ALMS patients by reducing appetite and increasing energy expenditure (ClinicalTrials.gov, NCT04966741). Additionally, another clinical study is aimed at implementing caloric restriction in obese patients with autosomal dominant polycystic kidney disease (ADPKD, OMIM #173900), a disease characterized by mutations in cilia associated proteins polycystin-1 and -2 (Kim and Park, 2016). Here, the goal is to determine if weight loss can alter the progression of cystic disease (ClinicalTrials.gov, NCT04907799). Although these diseases are rare, development of both drug and behavioral interventions could be beneficial for ciliopathy patients. Ultimately, understanding how cilia are involved in energy homeostasis may also reveal novel targets for the general population of obese patients.
Genetic mouse models of cilia associated obesity
Many genetic mouse models of obesity recapitulate phenotypes observed in humans, thus making them essential tools for studying the pathogenesis of obesity. A better understanding of cilia and their roles in obesity comes from mouse models of BBS and ALMS, as well as by mice carrying mutations in specific GPCRs known to localize to cilia and regulate energy homeostasis, such as melanin-concentrating hormone receptor 1 (MCHR1) and neuropeptide-Y 2 receptor (NPY2R). Additionally, mice with mutations in genes important for the formation and maintenance of primary cilia have proved essential to building our understanding of cilia regulated processes and pathways.
BBS and ALMS mouse models are obese
Congenital mouse models of BBS and ALMS are obese, see (Vaisse et al., 2017) for BBS gene and obese mouse model elaboration. Two new obese mouse models for BBS include a gene trap allele (Bbs5−/−) thought to be a congenital null mutation and a conditional (Bbs5flox/flox) allele of Bbs5. Interestingly, the Bbs5 conditional mutants become obese, independent of the age of Bbs5 loss implying homeostatic roles for Bbs5 in energy homeostasis (Bentley-Ford et al., 2021; Bentley-Ford et al., 2022). Mouse models of ALMS have included a whole-body Alms1 knockout (Almsflin/flin) (Geberhiwot et al., 2021) and the fat aussie mouse which carries a spontaneous 11 bp deletion resulting in a frameshift and truncating mutation in Alms1 (Arsov et al., 2006; Kim et al., 2020). Both mice become obese and exhibit adipocyte hypertrophy, hyperglycemia, glucose intolerance, and leptin resistance. Interestingly, when Alms1 was reintroduced to adipose tissue in the Almsflin/flin mouse, insulin sensitivity and glucose tolerance were restored (Geberhiwot et al., 2021). In fat aussie, Alms1 fails to localize to the base of the cilium in hypothalamic neurons and there is a significant reduction of adenylyl cyclase 3 (ADCY3) positive cilia (Heydet et al., 2013). It is unclear if the altered ciliary ADCY3 in this Alms1 model is due to loss of cilia or failure of ADCY3 to localize to the cilia membrane. This highlights a challenge for studies of neuronal cilia, the lack of a universal cilia marker in the central nervous system.
GPCRs and neuronal cilia
There are several GPCRs enriched in neuronal cilia (Schou et al., 2015; Mykytyn and Askwith, 2017). If odorant and opsin receptors are included, then most GPCRs function at cilia. However, there is an emerging set of GPCRs that localize to cilia on neurons deep within the brain including MCHR1, melanocortin 4 receptor (MC4R), NPY2R, NPY5R, somatostatin receptor 3 (SSTR3), kisspeptin 1 receptor (KISS1R), serotonin receptor 6 (5HT6), and dopamine receptor 1 (DRD1) (Berbari et al., 2008a; Loktev and Jackson, 2013; Koemeter-Cox et al., 2014; Siljee et al., 2018) (Table 1). Many of these GPCRs have known roles in feeding behaviors, energy homeostasis, and altered cilia localization is observed in obese ciliopathy mouse models.
For instance, the MCH/MCHR1 signaling pathway has a well-defined role in energy homeostasis [for a review see (Al-Massadi et al., 2021)]. MCHR1 localizes to cilia in many areas of the mouse and rat brain, including the olfactory bulb, hippocampus, amygdala, hypothalamus, and spinal cord (Niño-Rivero et al., 2019; Diniz et al., 2020; Brewer et al., 2022). Using optogenetics, and chemogenetics, activation and inhibition of the MCH pathway causes cilia shortening and lengthening, respectively, in the brain as measured with ADCY3s staining (Alhassen et al., 2022). Interestingly, MCHR1 ligand, MCH, expression changes based on feeding status. For example, under fasted conditions, MCH increases in the lateral hypothalamus (Segal-Lieberman et al., 2003; Simon et al., 2018). There is also growing evidence to support the biological importance of ciliary GPCR signaling, such as MCHR1, in regulating cilia length and neuronal function (Miki et al., 2019; Kobayashi et al., 2020; Kobayashi et al., 2021). Ciliary MCHR1 may regulate different physiological conditions, such as feeding and be a potential target for conditions with impaired cilia function, such as ciliopathies. New mouse models, such as a fusion mCherry protein on the N-terminus of MCHR1 (Jasso et al., 2021) and an inducible MCHR1 promoter driven cre allele (Engle et al., 2018), will aid in visual and additional functional analysis of this GPCR in the brain.
Many models of ciliopathies associated with obesity appear to mislocalize ciliary GPCRs. The BBSome plays an essential role in dynamically trafficking GPCRs to and from the cilia membrane [(Ye et al., 2018); (Nozaki et al., 2018; Nozaki et al., 2019; Zhou et al., 2022)]. BBS mouse models fail to localize MCHR1 and SSTR3 to the cilium in areas of the brain involved in feeding and reward pathways, such as the nucleus accumbens, olfactory bulb, and the hypothalamus (Berbari et al., 2008a). NPY2R and SSTR3 also fail to localize to the cilium in the absence of BBSome subunit BBIP10, a protein required for BBSome stability. These mice also fail to activate c-fos and decrease food intake in response to NPY2R ligand PYY3-36. Depletion of Bbs3 showed normal NPY2R cilia localization (Loktev and Jackson, 2013); however, Bbs1 mutants have decreased NPY2R cilia localization and NPY2R expression, specifically in POMC and AgRP neurons (Guo et al., 2019). Interestingly, Bbs3 mutant mice have increased fat mass but do not develop overt obesity, and loss of Bbs3 allows for normal formation of the BBSome (Zhang et al., 2011). ALMS mouse models do not appear to mislocalize the GPCRs MCHR1 and SSTR3; however, fat aussie mouse models have a significant reduction in total cilia labeled with ADCY3 (Heydet et al., 2013). Together, ALMS and BBS may serve different functions in regulation of GPCR signaling in the cilium.
MC4R signaling at the cilium
MC4R mutations compromise 3%–5% of cases of monogenic obesity in humans, making MC4R signaling and its downstream circuitry an appealing target for obesity therapeutics (Huszar et al., 1997; Lubrano-Berthelier et al., 2006; Bromberg et al., 2009). Neurons in the paraventricular nucleus of the hypothalamus (PVN) localize MC4R to cilia (Siljee et al., 2018). Loss of cilia in MC4R-expressing neurons causes obesity, hyperphagia, and increased body lengths (Siljee et al., 2018). AAV vector driven expression of normal and mutated forms of MC4R, localizes this GPCR with ADCY3 at the primary cilium (Siljee et al., 2018). This localization is significantly reduced in human MC4R mutants, p.P230L and p.R236C (Siljee et al., 2018). Interestingly, these mutations are found in the third intracellular loop of the GPCR, a region implicated in ciliary localization (Berbari et al., 2008b). Inhibition of ADCY3 activity at the cilia membrane using constitutively active Gαi coupled GPR88 to inhibit MC4R at cilia caused mice to increase their food intake and become obese (Wang et al., 2020), demonstrating the importance of MC4R signaling at cilia.
Melanocortin receptors, like MC4R, require accessory proteins to regulate their activity and function (Rouault et al., 2017). For example, melanocortin receptor accessory protein 2 (MRAP2) plays a critical role in energy homeostasis in both mice and humans (Asai et al., 2013; Jackson et al., 2015; Bruschetta et al., 2018). Additionally, MC4R and MRAP2 are co-expressed in many cells within the PVN (Asai et al., 2013). Mice with global null mutations of MRAP2 are significantly heavier than littermate controls. MRAP2 deletion specifically in Sim1-expressing neurons of the PVN causes obesity in mice (Asai et al., 2013). MRAP2 and MC4R interact directly with each other to enhance MC4R stimulated cyclic adenosine monophosphate (cAMP) production (Asai et al., 2013; Jackson et al., 2015). Recent data also shows that MRAP2 promotes the cilia localization of MC4R (Bernard et al., 2020). This raises the question if other neuronal GPCRs, such as MCHR1 and NPY2R, are regulated by MRAPs or other accessory proteins. Understanding the molecular mechanisms of neuronal GPCR localization and function will aid in the development of drug treatments for metabolic disorders.
Altered cilia signaling machinery and obesity
Although GPCR signaling is a common paradigm that cilia use to regulate different physiological processes like vision and olfaction, it is emerging that cilia may utilize other signaling mechanisms in the brain. For example, a recent study has proposed the idea of axo-ciliary synapses. Here, they show that artificial stimulation of serotonergic axons releases serotonin directly onto the ciliary receptor 5-hydroxytrptamine receptor 6 (5-HTR6) in hippocampal neurons to activate the Gαq/11 RhoA pathway (Sheu et al., 2022). Further research could elucidate other neuronal populations that may use their cilium to form axo-ciliary synapses to regulate different functions, perhaps those involved in energy homeostasis.
Other alternate mechanisms for ciliary signaling could involve downstream effectors of GPCRs such as ADCY3. Polymorphisms in ADCY3 are associated with obesity in humans (Nordman et al., 2008; Wang et al., 2010). ADCY3 is highly expressed in the hypothalamus and shown to localize to neuronal primary cilia (Bishop et al., 2007; Domire and Mykytyn, 2009; Caspary et al., 2016). Mice lacking ADCY3 exhibit obesity that is caused by a decrease in activity, hyperphagia, and leptin resistance. Additionally, ADCY3 activity in the hypothalamus was reduced upon forskolin stimulation (Wang et al., 2009). Similar results are observed in male and female mice using an AAV-CRE GFP injection into the hypothalamus of conditional ADCY3 animals (Cao et al., 2016). These data point toward a role for hypothalamic ADCY3 in regulating feeding behaviors in both mice and humans.
Furthermore, when ADCY3 deletion is specific to the ventromedial hypothalamus (VMH), weight gain is pronounced in animals on a high fat diet (HFD). In addition, ADCY3 regulates autophagy by binding to gamma-aminobutyric acid A receptor-associated protein (GABARAP) (Yang et al., 2022a). Interestingly, autophagy is another mechanism thought to be used by cilia to regulate signaling (Pampliega et al., 2013; Orhon et al., 2015), and there is growing evidence to support a bi-directional relationship between ciliogenesis and autophagy (Ávalos et al., 2017). In ADCY3 knockout mice, there is a reduction of p62 and an increase in LC3-II, two proteins that regulate autophagy (Cao et al., 2016). Similarly, an increase in LC3-II and decrease in p62 is observed when overexpression of ADCY3 is inhibited using an AVV carrying constitutively active GPR88, a Gi- protein coupled receptor ((Siljee et al., 2018); (Yang et al., 2022a)).
High fat diets are rich in saturated fatty acids, mainly palmitic acid, and known to be the main cause of visceral obesity, glucose intolerance, and insulin resistance (Tchernof and Després, 2013). Palmitic acid is significantly increased in the hypothalamus of mice under chronic HFD conditions and in the plasma of obese humans (Kang et al., 2017). Additionally, chronic HFD in mice decreases the number and length of cilia of POMC neurons (Ávalos et al., 2022). Interestingly, treatment of hypothalamic neurons with palmitic acid impairs autophagy [(Hernández-Cáceres et al., 2019); (Hernández-Cáceres et al., 2020)] and reduces cilia number and length and blocks insulin-dependent signaling [(Ávalos et al., 2022); (Hernández-Cáceres et al., 2020)]. In developing POMC neurons, removal of Intraflagellar Transport 88 (IFT88) or kinesin family member 3A (Kif3A) disrupts axonal projections from the ARC to the PVN and development of POMC neurons through impaired lysosome protein degradation [(Ding et al., 2021); (Croizier and Bouret, 2022)]. Together, these results show that autophagy may regulate ciliary signaling and the proper localization of cilia proteins. Body composition and diet influences autophagy mechanisms important for ciliogenesis and cilia signaling as well as protein degradation in a cilia dependent manner.
Transition zone and basal body in energy homeostasis
The cilium regulates its structure maintenance and extracellular signaling processing through different compartments along its axoneme, such as at the transition zone and basal body. Several centrosome related gene mutations are also associated with obesity. Ciliopathies such as ALMS and BBS have mutations in genes associated with the centrosome. CEP19 is a cilia and centrosome associated protein that is highly conserved in vertebrates and invertebrates. Cep-19 knockout mice are morbidly obese, hyperphagic, glucose intolerant, and insulin resistant which recapitulates CEP19 mutations in humans (Shalata et al., 2013) (OMIM #615586). Centromere protein J (Cenpj) is a protein crucial for centrosome biogenesis and elongation, cilium disassembly, and spindle pole integrity. Depletion of Cenpj results in long cilia and abnormal cilia disassembly in neural progenitor cells in vivo (Ding et al., 2019). Conditional knockout of Cenpj in the hypothalamus results in decreased proliferation and increased apoptosis during embryonic development (Ding et al., 2021). These mice became obese, hyperphagic and less active in adulthood.
The Abelson-helper integration site 1 (Ahi1) gene product is required for localizing proteins to the transition zone of the cilium. When mutated, this gene causes the human ciliopathy Joubert Syndrome (JBTS, OMIM #213300) which does not routinely present with obesity [(Thomas et al., 2015); (Adams et al., 2007)]. In Ahi1 mutant mice, MCHR1 expression in neurons was similar compared to littermates; however, ciliary localization of MCHR1 was significantly reduced. Ahi1 depletion also led to the downregulation of two downstream signaling pathways of MCHR1, cAMP and extracellular signal-regulated kinase (ERK), upon ligand stimulation (Hsiao et al., 2021). Further evidence would be required to see how Ahi1 associated MCHR1 mislocalization impacts physiological processes, such as feeding. Another cilia transition zone protein, retinitis pigmentosa GTPase regulator-interacting protein-1 like (RPGRIP1L), is also implicated in feeding, as conditional ablation of RPGRIP1L leads to obesity in mice [(Lewis et al., 2019); (Wang et al., 2019)]. Congenital RPGRIP1L hypomorphism in POMC neurons leads to hyperphagic obesity and increased adiposity; however, deletion of RPGRIP1L in adult POMC neurons did not result in an obesity phenotype. These studies also report a reduction in the ratio of POMC and Neuropeptide-Y (NPY) neurons with an increase in axonal projections between the arcuate nucleus of the hypothalamus (ARC) and PVN. These findings suggest that hypothalamic RPGRIP1L polymorphisms impact the development of POMC neurons and their derivatives (Wang et al., 2019).
Neuronal cilia populations and feeding behaviors
The brain is vital for integrating and coordinating signals, such as hormones and nutrients, to maintain energy homeostasis. Cilia on neurons are required for normal energy homeostasis as conditional knockout models of ciliogenesis genes, IFT88 and Kif3A, cause obesity (Davenport et al., 2007; Lechtreck, 2015; Lee et al., 2020). Here, we discuss the roles of neuronal cilia in the different nuclei of the hypothalamus and the localization of GPCRs, specifically MC4R, to hypothalamic neuronal cilia in response to feeding. Many of these recent data suggest that neuronal cilia of the hypothalamus may work together to create a metabolic signaling hub critical for proper energy homeostasis (Figure 2).
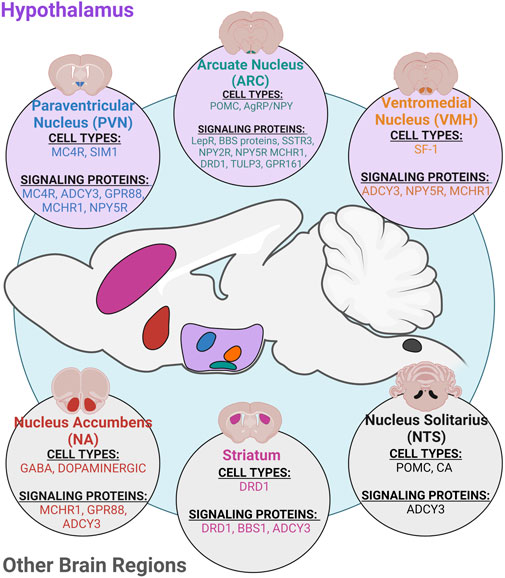
FIGURE 2. Ciliary neuronal populations in the CNS. Primary cilia are found on most neuronal cell types in the CNS and play roles in regulating different physiological processes. Specifically, cilia on different cell types in the hypothalamus and other brain regions such as the nucleus accumbens (NA), striatum, and nucleus solitarius (NTS) have known roles in regulating energy homeostasis and feeding. Cilia on hypothalamic neurons in the paraventricular nucleus (PVN), arcuate nucleus (ARC), and ventromedial nucleus (VMH) have roles in regulating feeding and metabolism (Fetissov et al., 2004; Nguyen et al., 2012; Chávez et al., 2015; Ehrlich et al., 2018; Nishimura et al., 2019; Nyamugenda et al., 2019; Ramos et al., 2021).
Conditional cilia models implicating the hypothalamus
The hypothalamus is a key region in the brain known to regulate feeding behaviors and metabolism, and it consists of many well-defined nuclei, including the ventromedial hypothalamus (VMH), arcuate nucleus (ARC), and paraventricular nucleus (PVN) (Figure 2). The VMH is important for many homeostatic processes including, skeletal homeostasis, mood behavior, reproduction, and regulation of body weight and glucose homeostasis (Krause and Ingraham, 2017). Primary cilia in the VMH are longer than other neuronal cilia types (Sipos et al., 2018) which are significantly shorter under metabolic conditions such as obesity and leptin resistance (Han et al., 2014). Genetic ablation of cilia using Cre-loxP and bilateral AAV-Cre injection in VMH steroidogenic factor-1 (SF1) expressing neurons caused an increase in body weight, subcutaneous and gonadal adipose tissue increase, and elevated serum leptin levels (Sun et al., 2021). Additionally, food intake was significantly increased in VMH cilia mutants, and they showed reduced activity, especially during the dark cycle, and reduced brown adipose tissue thermogenesis (Sun et al., 2021). Interestingly, humanized ACDCY3 knock-in mice are resistant to high-fat diet induced obesity and show an increase in cilia frequency and length in the VMH. Injection of ciliary ADCY3 inhibitor GPR88 (Siljee et al., 2018) resulted in obesity under normal chow and attenuated weight gain under HFD. Similarly, Bbs1 deletion in the VMH using a SIM1 cre approach results in obesity without altering food intake, energy absorption, and digestive efficiency (Rouabhi et al., 2021).
Conditional knockout of IFT88 or Kif3A in neonatal POMC-expressing neurons leads to obesity in adult mice (Davenport et al., 2007). Interestingly, conditional ablation of cilia in adult POMC neurons did not result in significant changes in body weight, food intake, or energy expenditure suggesting that cilia on these neurons are important for their embryonic development and early post-natal circuit organization (Wang et al., 2019). Deletion of Bbs1 in POMC or AgRP neurons disrupts the formation of the BBSome and increases body weight and adiposity. Specifically, this obesity in driven by hyperphagia when the BBSome is disrupted in POMC neurons, and these mice were glucose intolerant and insulin resistant. In AgRP neurons, disruption of the BBSome shows a more pronounced weight gain and increase in fat mass in females compared to males (Guo et al., 2019). It is clear that proper cilia assembly and signaling in the ARC are important for regulating energy balance. Cenpj conditional knockout models lead to hypothalamic defects early on and reduced area size of the ARC and PVN in adults. Additionally, depletion of Cenpj leads to a decrease in POMC neurons, a reduction of POMC neuronal projections into the PVN, and increased spontaneous firing of NPY neurons in the ARC (Ding et al., 2021). It appears that an increase in AgRP/NPY and decrease in alpha melano-stimulating hormone (α-MSH) blocks satiety causing the obese phenotype (Ding et al., 2021).
Cilia in other brain regions associated with energy homeostasis
Although the hypothalamus appears to be the main brain region for neuronal cilia regulation of feeding and metabolism, cilia in other regions in the CNS may contribute to energy homeostasis (Figure 2). For example, in Bbs1 mutant mice, dopamine receptor 1 (DRD1), localizes to the cilium in the striatum, amygdala, and olfactory tubercle (Domire et al., 2011). Interestingly, in BBS mutants, DRD1 localization to the cilium is accompanied by a reduction of ciliary ADCY3 in DRD1-expressing neurons. These mice become obese as a result of reduced locomotor activity which is recapitulated in DRD1 cilia knockout mice (Stubbs et al., 2022). Additionally, the hindbrain includes regions such as the nucleus tractus solitarius (NTS) that have implicated roles in feeding behavior. Here, POMC neurons located in the NTS respond to short term satiety signals in the brain stem to regulate energy homeostasis, whereas POMC neurons in the ARC respond to long-term feeding signals [(Cheng et al., 2021); (Zhan et al., 2013)]. When cilia are ablated from POMC neurons, through Kif3A, mice are obese and present with an increase in adiposity, lean mass, and body length (Davenport et al., 2007). Although this study focused on POMC neurons in the hypothalamus, it is possible that cilia ablation on POMC neurons in the NTS could contribute to the obese phenotype. These data suggest that cilia in other areas of the brain may also regulate energy homeostasis through additional mechanisms, such as influencing locomotion or at specific time points. Future studies should expand upon understanding the role for cilia in these different brain regions.
Neurodevelopmental roles for cilia in obesity
The most well-defined roles for primary cilia come from our understanding of how they mediate hedgehog signaling in embryonic development and tissue patterning [for a review see (Goetz and Anderson, 2010)]. Cilia-mediated hedgehog signaling is critical for patterning many tissues, including the developing hypothalamus (Szabó et al., 2009; Shimogori et al., 2010; Blaess et al., 2014) and pituitary (Treier et al., 2001). In mouse models of BBS, mispatterning of the hypothalamus is associated with a potential loss of 20% of POMC neurons in BBS2 and BBS6 mutants (Seo et al., 2009). In cultured BBS mutant iPSC cells, hedgehog signaling plays a role in differentiation into arcuate and other hypothalamic neuronal fates (Wang et al., 2019; Wang et al., 2021b). In addition, pituitary phenotypes consistent with perturbations in Hedgehog patterning defects occur in a mouse model of BBS5 (Bentley-Ford et al., 2021). These observations indicate the potential for altered hedgehog-mediated patterning to contribute to ciliopathy-associated obesity later in life.
Challenges and future directions and conclusions
There are many challenges to understanding neuronal cilia. Simply visualizing cilia in the CNS is a challenge, as the standard tubulin markers are not specific to the organelle in neurons (Caspary et al., 2016). Even fundamental questions around understanding if neuronal cilia act through slower neuropeptide mediated mechanisms or directly influence neuronal activity, as suggested with the observation that cilia can directly synapse to neurons (Sheu et al., 2022), or perhaps cilia serve as both slow and fast modulators of neurons and circuits. Fascinating work in models like C. elegans has suggested that neuronal cilia length changes can directly impact their ability to sense the external environment (Maurya and Sengupta, 2022). In addition, neuronal cilia also appear to be capable of sending signals not just receiving them pointing to the complexity of neuronal cilia signaling [(Wang et al., 2021c); (Nikonorova et al., 2022)]. In conclusion, how cilia in the brain regulate energy homeostasis has become a complex question with impacts beyond the field of obesity and into general neuroscience and signaling. It is likely that both developmental and homeostatic processes regulated by CNS cilia can contribute to obesity. It is also likely that multiple pathways are perturbed in the context of ciliopathies leading to hyperphagia and obesity.
Author contributions
KMB wrote the manuscript. All authors contributed to the design and editing of the manuscript and figures.
Funding
This work was funded by the National Institute of Diabetes and Digestive and Kidney Diseases R01DK114008 to NFB.
Acknowledgments
NCR was supported by the Arnold and Mabel Beckman Foundation through the Beckman Scholars Award to IUPUI. KMB and KKB were supported by the IUPUI University Fellowship. Figures and Table created with BioRender.com.
Conflict of interest
The authors declare that the research was conducted in the absence of any commercial or financial relationships that could be construed as a potential conflict of interest.
Publisher’s note
All claims expressed in this article are solely those of the authors and do not necessarily represent those of their affiliated organizations, or those of the publisher, the editors and the reviewers. Any product that may be evaluated in this article, or claim that may be made by its manufacturer, is not guaranteed or endorsed by the publisher.
References
Adams, N. A., Awadein, A., and Toma, H. S. (2007). The retinal ciliopathies. Ophthalmic Genet. 28 (3), 113–125. doi:10.1080/13816810701537424
Al-Massadi, O., Dieguez, C., Schneeberger, M., López, M., Schwaninger, M., Prevot, V., et al. (2021). Multifaceted actions of melanin-concentrating hormone on mammalian energy homeostasis. Nat. Rev. Endocrinol. 17 (12), 745–755. doi:10.1038/s41574-021-00559-1
Alhassen, W., Kobayashi, Y., Su, J., Robbins, B., Nguyen, H., Myint, T., et al. (2022). Regulation of brain primary cilia length by MCH signaling: Evidence from pharmacological, genetic, optogenetic, and chemogenic manipulations. Mol. Neurobiol. 59 (1), 245–265. doi:10.1007/s12035-021-02511-w
Arsov, T., Silva, D. G., O'Bryan, M. K., Sainsbury, A., Lee, N. J., Kennedy, C., et al. (2006). Fat aussie--a new Alström syndrome mouse showing a critical role for ALMS1 in obesity, diabetes, and spermatogenesis. Mol. Endocrinol. 20 (7), 1610–1622. doi:10.1210/me.2005-0494
Asai, M., Ramachandrappa, S., Joachim, M., Shen, Y., Zhang, R., Nuthalapati, N., et al. (2013). Loss of function of the melanocortin 2 receptor accessory protein 2 is associated with mammalian obesity. Science 341 (6143), 275–278. doi:10.1126/science.1233000
Ávalos, Y., Hernández-Cáceres, M. P., Lagos, P., Pinto-Nuñez, D., Rivera, P., Burgos, P., et al. (2022). Palmitic acid control of ciliogenesis modulates insulin signaling in hypothalamic neurons through an autophagy-dependent mechanism. Cell Death Dis. 13 (7), 659. doi:10.1038/s41419-022-05109-9
Ávalos, Y., Peña-Oyarzun, D., Budini, M., Morselli, E., and Criollo, A. (2017). New roles of the primary cilium in autophagy. Biomed. Res. Int. 2017, 4367019. doi:10.1155/2017/4367019
Bennouna-Greene, V., Kremer, S., Stoetzel, C., Christmann, D., Schuster, C., Durand, M., et al. (2011). Hippocampal dysgenesis and variable neuropsychiatric phenotypes in patients with Bardet-Biedl syndrome underline complex CNS impact of primary cilia. Clin. Genet. 80 (6), 523–531. doi:10.1111/j.1399-0004.2011.01688.x
Bentley-Ford, M. R., Engle, S. E., Clearman, K. R., Haycraft, C. J., Andersen, R. S., Croyle, M. J., et al. (2021). A mouse model of BBS identifies developmental and homeostatic effects of BBS5 mutation and identifies novel pituitary abnormalities. Hum. Mol. Genet. 30 (3-4), 234–246. doi:10.1093/hmg/ddab039
Bentley-Ford, M. R., LaBonty, M., Thomas, H. R., Haycraft, C. J., Scott, M., LaFayette, C., et al. (2022). Evolutionarily conserved genetic interactions between nphp-4 and bbs-5 mutations exacerbate ciliopathy phenotypes. Genetics 220 (1), iyab209. doi:10.1093/genetics/iyab209
Berbari, N. F., Johnson, A. D., Lewis, J. S., Askwith, C. C., and Mykytyn, K. (2008). Identification of ciliary localization sequences within the third intracellular loop of G protein-coupled receptors. Mol. Biol. Cell 19 (4), 1540–1547. doi:10.1091/mbc.e07-09-0942
Berbari, N. F., Lewis, J. S., Bishop, G. A., Askwith, C. C., and Mykytyn, K. (2008). Bardet-Biedl syndrome proteins are required for the localization of G protein-coupled receptors to primary cilia. Proc. Natl. Acad. Sci. U. S. A. 105 (11), 4242–4246. doi:10.1073/pnas.0711027105
Bernard, A., Naharros, I. O., Bourgain-Guglielmetti, F., Ciprin, J., Yue, X., Zhang, S., et al. (2020). The single pass membrane protein MRAP2 regulates energy homeostasis by promoting primary cilia localization of the G protein-coupled receptor MC4R. bioRxiv.
Bishop, G. A., Berbari, N. F., Lewis, J., and Mykytyn, K. (2007). Type III adenylyl cyclase localizes to primary cilia throughout the adult mouse brain. J. Comp. Neurol. 505 (5), 562–571. doi:10.1002/cne.21510
Blaess, S., Szabó, N., Haddad-Tóvolli, R., Zhou, X., and Álvarez-Bolado, G. (2014). Sonic hedgehog signaling in the development of the mouse hypothalamus. Front. Neuroanat. 8, 156. doi:10.3389/fnana.2014.00156
Brewer, K. M., Engle, S. E., Bansal, R., Brewer, K. K., Jasso, K. R., McIntyre, J. C., et al. (2022). Physiological condition dependent changes in ciliary GPCR localization in the brain. bioRxiv.
Bromberg, Y., Overton, J., Vaisse, C., Leibel, R. L., and Rost, B. (2009). In silico mutagenesis: A case study of the melanocortin 4 receptor. Faseb J. 23 (9), 3059–3069. doi:10.1096/fj.08-127530
Bruschetta, G., Kim, J. D., Diano, S., and Chan, L. F. (2018). Overexpression of melanocortin 2 receptor accessory protein 2 (MRAP2) in adult paraventricular MC4R neurons regulates energy intake and expenditure. Mol. Metab. 18, 79–87. doi:10.1016/j.molmet.2018.09.010
Cao, H., Chen, X., Yang, Y., and Storm, D. R. (2016). Disruption of type 3 adenylyl cyclase expression in the hypothalamus leads to obesity. Integr. Obes. Diabetes 2 (2), 225–228. doi:10.15761/IOD.1000149
Caspary, T., Marazziti, D., and Berbari, N. F. (2016). Methods for visualization of neuronal cilia. Methods Mol. Biol. 1454, 203–214. doi:10.1007/978-1-4939-3789-9_13
Chávez, M., Ena, S., Van Sande, J., de Kerchove d'Exaerde, A., Schurmans, S., and Schiffmann, S. N. (2015). Modulation of ciliary phosphoinositide content regulates trafficking and sonic hedgehog signaling output. Dev. Cell 34 (3), 338–350. doi:10.1016/j.devcel.2015.06.016
Cheng, W., Ndoka, E., Maung, J. N., Pan, W., Rupp, A. C., Rhodes, C. J., et al. (2021). NTS Prlh overcomes orexigenic stimuli and ameliorates dietary and genetic forms of obesity. Nat. Commun. 12 (1), 5175. doi:10.1038/s41467-021-25525-3
Citton, V., Favaro, A., Bettini, V., Gabrieli, J., Milan, G., Greggio, N. A., et al. (2013). Brain involvement in Alström syndrome. Orphanet J. Rare Dis. 8, 24. doi:10.1186/1750-1172-8-24
Clément, K., van den Akker, E., Argente, J., Bahm, A., Chung, W. K., Connors, H., et al. (2020). Efficacy and safety of setmelanotide, an MC4R agonist, in individuals with severe obesity due to LEPR or POMC deficiency: Single-arm, open-label, multicentre, phase 3 trials. Lancet. Diabetes Endocrinol. 8 (12), 960–970. doi:10.1016/S2213-8587(20)30364-8
Croizier, S., and Bouret, S. G. (2022). Molecular control of the development of hypothalamic neurons involved in metabolic regulation. J. Chem. Neuroanat. 123, 102117. doi:10.1016/j.jchemneu.2022.102117
Davenport, J. R., Watts, A. J., Roper, V. C., Croyle, M. J., van Groen, T., Wyss, J. M., et al. (2007). Disruption of intraflagellar transport in adult mice leads to obesity and slow-onset cystic kidney disease. Curr. Biol. 17 (18), 1586–1594. doi:10.1016/j.cub.2007.08.034
Ding, W., Wu, Q., Sun, L., Pan, N. C., and Wang, X. (2019). Cenpj regulates cilia disassembly and neurogenesis in the developing mouse cortex. J. Neurosci. 39 (11), 1994–2010. doi:10.1523/JNEUROSCI.1849-18.2018
Ding, W., Zhang, C., Wang, B., Zhou, X., Sun, L., Zhong, S., et al. (2021). Loss of the centrosomal protein Cenpj leads to dysfunction of the hypothalamus and obesity in mice. Sci. China. Life Sci. 64 (3), 419–433. doi:10.1007/s11427-020-1767-5
Diniz, G. B., Battagello, D. S., Klein, M. O., Bono, B. S. M., Ferreira, J. G. P., Motta-Teixeira, L. C., et al. (2020). Ciliary melanin-concentrating hormone receptor 1 (MCHR1) is widely distributed in the murine CNS in a sex-independent manner. J. Neurosci. Res. 98 (10), 2045–2071. doi:10.1002/jnr.24651
Domire, J. S., Green, J. A., Lee, K. G., Johnson, A. D., Askwith, C. C., and Mykytyn, K. (2011). Dopamine receptor 1 localizes to neuronal cilia in a dynamic process that requires the Bardet-Biedl syndrome proteins. Cell. Mol. Life Sci. 68 (17), 2951–2960. doi:10.1007/s00018-010-0603-4
Domire, J. S., and Mykytyn, K. (2009). Markers for neuronal cilia. Methods Cell Biol. 91, 111–121. doi:10.1016/S0091-679X(08)91006-2
Ehrlich, A. T., Semache, M., Bailly, J., Wojcik, S., Arefin, T. M., Colley, C., et al. (2018). Mapping GPR88-Venus illuminates a novel role for GPR88 in sensory processing. Brain Struct. Funct. 223 (3), 1275–1296. doi:10.1007/s00429-017-1547-3
Engle, S. E., Antonellis, P. J., Whitehouse, L. S., Bansal, R., Emond, M. R., Jontes, J. D., et al. (2018). A CreER mouse to study melanin concentrating hormone signaling in the developing brain. Genesis 56 (8), e23217. doi:10.1002/dvg.23217
Engle, S. E., Bansal, R., Antonellis, P. J., and Berbari, N. F. (2021). Cilia signaling and obesity. Semin. Cell Dev. Biol. 110, 43–50. doi:10.1016/j.semcdb.2020.05.006
Fetissov, S. O., Byrne, L. C., Hassani, H., Ernfors, P., and Hökfelt, T. (2004). Characterization of neuropeptide Y Y2 and Y5 receptor expression in the mouse hypothalamus. J. Comp. Neurol. 470 (3), 256–265. doi:10.1002/cne.11047
Forsythe, E., Kenny, J., Bacchelli, C., and Beales, P. L. (2018). Managing bardet-biedl syndrome-now and in the future. Front. Pediatr. 6, 23. doi:10.3389/fped.2018.00023
Forsythe, E., Sparks, K., Best, S., Borrows, S., Hoskins, B., Sabir, A., et al. (2017). Risk factors for severe renal disease in bardet-biedl syndrome. J. Am. Soc. Nephrol. 28 (3), 963–970. doi:10.1681/ASN.2015091029
Geberhiwot, T., Baig, S., Obringer, C., Girard, D., Dawson, C., Manolopoulos, K., et al. (2021). Relative adipose tissue failure in Alström syndrome drives obesity-induced insulin resistance. Diabetes 70 (2), 364–376. doi:10.2337/db20-0647
Goetz, S. C., and Anderson, K. V. (2010). The primary cilium: A signalling centre during vertebrate development. Nat. Rev. Genet. 11 (5), 331–344. doi:10.1038/nrg2774
Guo, D. F., Lin, Z., Wu, Y., Searby, C., Thedens, D. R., Richerson, G. B., et al. (2019). The BBSome in POMC and AgRP neurons is necessary for body weight regulation and sorting of metabolic receptors. Diabetes 68 (8), 1591–1603. doi:10.2337/db18-1088
Hakim, S., Bertucci, M. C., Conduit, S. E., Vuong, D. L., and Mitchell, C. A. (2012). Inositol polyphosphate phosphatases in human disease. Curr. Top. Microbiol. Immunol. 362, 247–314. doi:10.1007/978-94-007-5025-8_12
Han, Y. M., Kang, G. M., Byun, K., Ko, H. W., Kim, J., Shin, M. S., et al. (2014). Leptin-promoted cilia assembly is critical for normal energy balance. J. Clin. Invest. 124 (5), 2193–2197. doi:10.1172/JCI69395
Hearn, T., Renforth, G. L., Spalluto, C., Hanley, N. A., Piper, K., Brickwood, S., et al. (2002). Mutation of ALMS1, a large gene with a tandem repeat encoding 47 amino acids, causes Alström syndrome. Nat. Genet. 31 (1), 79–83. doi:10.1038/ng874
Hearn, T., Spalluto, C., Phillips, V. J., Renforth, G. L., Copin, N., Hanley, N. A., et al. (2005). Subcellular localization of ALMS1 supports involvement of centrosome and basal body dysfunction in the pathogenesis of obesity, insulin resistance, and type 2 diabetes. Diabetes 54 (5), 1581–1587. doi:10.2337/diabetes.54.5.1581
Hernández-Cáceres, M. P., Cereceda, K., Hernández, S., Li, Y., Narro, C., Rivera, P., et al. (2020). Palmitic acid reduces the autophagic flux in hypothalamic neurons by impairing autophagosome-lysosome fusion and endolysosomal dynamics. Mol. Cell. Oncol. 7 (5), 1789418. doi:10.1080/23723556.2020.1789418
Hernández-Cáceres, M. P., Toledo-Valenzuela, L., Díaz-Castro, F., Ávalos, Y., Burgos, P., Narro, C., et al. (2019). Palmitic acid reduces the autophagic flux and insulin sensitivity through the activation of the free fatty acid receptor 1 (FFAR1) in the hypothalamic neuronal cell line N43/5. Front. Endocrinol. 10, 176. doi:10.3389/fendo.2019.00176
Heydet, D., Chen, L. X., Larter, C. Z., Inglis, C., Silverman, M. A., Farrell, G. C., et al. (2013). A truncating mutation of Alms1 reduces the number of hypothalamic neuronal cilia in obese mice. Dev. Neurobiol. 73 (1), 1–13. doi:10.1002/dneu.22031
Hor, C. H. H., Tang, B. L., and Goh, E. L. K. (2018). Rab23 and developmental disorders. Rev. Neurosci. 29 (8), 849–860. doi:10.1515/revneuro-2017-0110
Hsiao, Y. C., Muñoz-Estrada, J., Tuz, K., and Ferland, R. J. (2021). The transition zone protein AHI1 regulates neuronal ciliary trafficking of MCHR1 and its downstream signaling pathway. J. Neurosci. 41 (17), 3932–3943. doi:10.1523/JNEUROSCI.2993-20.2021
Huszar, D., Lynch, C. A., Fairchild-Huntress, V., Dunmore, J. H., Fang, Q., Berkemeier, L. R., et al. (1997). Targeted disruption of the melanocortin-4 receptor results in obesity in mice. Cell 88 (1), 131–141. doi:10.1016/s0092-8674(00)81865-6
Jackson, D. S., Ramachandrappa, S., Clark, A. J., and Chan, L. F. (2015). Melanocortin receptor accessory proteins in adrenal disease and obesity. Front. Neurosci. 9, 213. doi:10.3389/fnins.2015.00213
Jasso, K. R., Kamba, T. K., Zimmerman, A. D., Bansal, R., Engle, S. E., Everett, T., et al. (2021). An N-terminal fusion allele to study melanin concentrating hormone receptor 1. Genesis 59 (7-8), e23438. doi:10.1002/dvg.23438
Jin, H., and Nachury, M. V. (2009). The BBSome. Curr. Biol. 19 (12), R472–R473. doi:10.1016/j.cub.2009.04.015
Jin, H., White, S. R., Shida, T., Schulz, S., Aguiar, M., Gygi, S. P., et al. (2010). The conserved Bardet-Biedl syndrome proteins assemble a coat that traffics membrane proteins to cilia. Cell 141 (7), 1208–1219. doi:10.1016/j.cell.2010.05.015
Kang, M., Lee, A., Yoo, H. J., Kim, M., Kim, M., Shin, D. Y., et al. (2017). Association between increased visceral fat area and alterations in plasma fatty acid profile in overweight subjects: A cross-sectional study. Lipids Health Dis. 16 (1), 248. doi:10.1186/s12944-017-0642-z
Keppler-Noreuil, K. M., Blumhorst, C., Sapp, J. C., Brinckman, D., Johnston, J., Nopoulos, P. C., et al. (2011). Brain tissue- and region-specific abnormalities on volumetric MRI scans in 21 patients with Bardet-Biedl syndrome (BBS). BMC Med. Genet. 12, 101. doi:10.1186/1471-2350-12-101
Kim, D. Y., and Park, J. H. (2016). Genetic mechanisms of ADPKD. Adv. Exp. Med. Biol. 933, 13–22. doi:10.1007/978-981-10-2041-4_2
Kim, Y. C., Ganguly, S., Nespoux, J., Freeman, B., Zhang, H., Brenner, D., et al. (2020). Western diet promotes renal injury, inflammation, and fibrosis in a murine model of Alström syndrome. Nephron 144 (8), 400–412. doi:10.1159/000508636
Knorz, V. J., Spalluto, C., Lessard, M., Purvis, T. L., Adigun, F. F., Collin, G. B., et al. (2010). Centriolar association of ALMS1 and likely centrosomal functions of the ALMS motif-containing proteins C10orf90 and KIAA1731. Mol. Biol. Cell 21 (21), 3617–3629. doi:10.1091/mbc.E10-03-0246
Kobayashi, Y., Hamamoto, A., and Saito, Y. (2020). Analysis of ciliary status via G-protein-coupled receptors localized on primary cilia. Microsc. (Oxf) 69 (5), 277–285. doi:10.1093/jmicro/dfaa035
Kobayashi, Y., Tomoshige, S., Imakado, K., Sekino, Y., Koganezawa, N., Shirao, T., et al. (2021). Ciliary GPCR-based transcriptome as a key regulator of cilia length control. FASEB Bioadv. 3 (9), 744–767. doi:10.1096/fba.2021-00029
Koemeter-Cox, A. I., Sherwood, T. W., Green, J. A., Steiner, R. A., Berbari, N. F., Yoder, B. K., et al. (2014). Primary cilia enhance kisspeptin receptor signaling on gonadotropin-releasing hormone neurons. Proc. Natl. Acad. Sci. U. S. A. 111 (28), 10335–10340. doi:10.1073/pnas.1403286111
Krause, W. C., and Ingraham, H. A. (2017). Origins and functions of the ventrolateral VMH: A complex neuronal cluster orchestrating sex differences in metabolism and behavior. Adv. Exp. Med. Biol. 1043, 199–213. doi:10.1007/978-3-319-70178-3_10
Lechtreck, K. F. (2015). IFT-cargo interactions and protein transport in cilia. Trends biochem. Sci. 40 (12), 765–778. doi:10.1016/j.tibs.2015.09.003
Lee, C. H., Song, D. K., Park, C. B., Choi, J., Kang, G. M., Shin, S. H., et al. (2020). Primary cilia mediate early life programming of adiposity through lysosomal regulation in the developing mouse hypothalamus. Nat. Commun. 11 (1), 5772. doi:10.1038/s41467-020-19638-4
Leitch, C. C., Zaghloul, N. A., Davis, E. E., Stoetzel, C., Diaz-Font, A., Rix, S., et al. (2008). Hypomorphic mutations in syndromic encephalocele genes are associated with Bardet-Biedl syndrome. Nat. Genet. 40 (4), 443–448. doi:10.1038/ng.97
Lewis, W. R., Bales, K. L., Revell, D. Z., Croyle, M. J., Engle, S. E., Song, C. J., et al. (2019). Mks6 mutations reveal tissue- and cell type-specific roles for the cilia transition zone. Faseb J. 33 (1), 1440–1455. doi:10.1096/fj.201801149R
Loktev, A. V., and Jackson, P. K. (2013). Neuropeptide Y family receptors traffic via the Bardet-Biedl syndrome pathway to signal in neuronal primary cilia. Cell Rep. 5 (5), 1316–1329. doi:10.1016/j.celrep.2013.11.011
Lubrano-Berthelier, C., Dubern, B., Lacorte, J. M., Picard, F., Shapiro, A., Zhang, S., et al. (2006). Melanocortin 4 receptor mutations in a large cohort of severely obese adults: Prevalence, functional classification, genotype-phenotype relationship, and lack of association with binge eating. J. Clin. Endocrinol. Metab. 91 (5), 1811–1818. doi:10.1210/jc.2005-1411
Maurya, A. K., and Sengupta, P. (2022). The DYF-5 RCK and CDKL-1 CDKL5 kinases contribute differentially to shape distinct sensory neuron cilia morphologies in C. elegans. Micropubl. Biol. 2022, 2022. doi:10.17912/micropub.biology.000619
Mihai, C. M., Catrinoiu, D., Marshall, J., Stoicescu, R., and Tofolean, I. T. (2008). Cilia, Alström syndrome--molecular mechanisms and therapeutic perspectives. J. Med. Life 1 (3), 254–261.
Miki, D., Kobayashi, Y., Okada, T., Miyamoto, T., Takei, N., Sekino, Y., et al. (2019). Characterization of functional primary cilia in human induced pluripotent stem cell-derived neurons. Neurochem. Res. 44 (7), 1736–1744. doi:10.1007/s11064-019-02806-4
Mukhopadhyay, S., and Jackson, P. K. (2013). Cilia, tubby mice, and obesity. Cilia 2, 1. doi:10.1186/2046-2530-2-1
Mykytyn, K., and Askwith, C. (2017). G-Protein-Coupled receptor signaling in cilia. Cold Spring Harb. Perspect. Biol. 9 (9), a028183. doi:10.1101/cshperspect.a028183
Nachury, M. V., Loktev, A. V., Zhang, Q., Westlake, C. J., Peränen, J., Merdes, A., et al. (2007). A core complex of BBS proteins cooperates with the GTPase Rab8 to promote ciliary membrane biogenesis. Cell 129 (6), 1201–1213. doi:10.1016/j.cell.2007.03.053
Nguyen, A. D., Mitchell, N. F., Lin, S., Macia, L., Yulyaningsih, E., Baldock, P. A., et al. (2012). Y1 and Y5 receptors are both required for the regulation of food intake and energy homeostasis in mice. PLoS One 7 (6), e40191. doi:10.1371/journal.pone.0040191
Niederlova, V., Modrak, M., Tsyklauri, O., Huranova, M., and Stepanek, O. (2019). Meta-analysis of genotype-phenotype associations in Bardet-Biedl syndrome uncovers differences among causative genes. Hum. Mutat. 40 (11), 2068–2087. doi:10.1002/humu.23862
Nikonorova, I. A., Wang, J., Cope, A. L., Tilton, P. E., Power, K. M., Walsh, J. D., et al. (2022). Isolation, profiling, and tracking of extracellular vesicle cargo in Caenorhabditis elegans. Curr. Biol. 32 (9), 1924–1936. doi:10.1016/j.cub.2022.03.005
Niño-Rivero, S., Torterolo, P., and Lagos, P. (2019). Melanin-concentrating hormone receptor-1 is located in primary cilia of the dorsal raphe neurons. J. Chem. Neuroanat. 98, 55–62. doi:10.1016/j.jchemneu.2019.03.003
Nishimura, Y., Kasahara, K., Shiromizu, T., Watanabe, M., and Inagaki, M. (2019). Primary cilia as signaling hubs in health and disease. Adv. Sci. 6 (1), 1801138. doi:10.1002/advs.201801138
Nordman, S., Abulaiti, A., Hilding, A., Långberg, E. C., Humphreys, K., Ostenson, C. G., et al. (2008). Genetic variation of the adenylyl cyclase 3 (AC3) locus and its influence on type 2 diabetes and obesity susceptibility in Swedish men. Int. J. Obes. 32 (3), 407–412. doi:10.1038/sj.ijo.0803742
Nozaki, S., Castro Araya, R. F., Katoh, Y., and Nakayama, K. (2019). Requirement of IFT-B-BBSome complex interaction in export of GPR161 from cilia. Biol. Open 8 (9), bio043786. doi:10.1242/bio.043786
Nozaki, S., Katoh, Y., Kobayashi, T., and Nakayama, K. (2018). BBS1 is involved in retrograde trafficking of ciliary GPCRs in the context of the BBSome complex. PLoS One 13 (3), e0195005. doi:10.1371/journal.pone.0195005
Nyamugenda, E., Trentzsch, M., Russell, S., Miles, T., Boysen, G., Phelan, K. D., et al. (2019). Injury to hypothalamic Sim1 neurons is a common feature of obesity by exposure to high-fat diet in male and female mice. J. Neurochem. 149 (1), 73–97. doi:10.1111/jnc.14662
Obradovic, M., Sudar-Milovanovic, E., Soskic, S., Essack, M., Arya, S., Stewart, A. J., et al. (2021). Leptin and obesity: Role and clinical implication. Front. Endocrinol. 12, 585887. doi:10.3389/fendo.2021.585887
Orhon, I., Dupont, N., Pampliega, O., Cuervo, A. M., and Codogno, P. (2015). Autophagy and regulation of cilia function and assembly. Cell Death Differ. 22 (3), 389–397. doi:10.1038/cdd.2014.171
Pampliega, O., Orhon, I., Patel, B., Sridhar, S., Díaz-Carretero, A., Beau, I., et al. (2013). Functional interaction between autophagy and ciliogenesis. Nature 502 (7470), 194–200. doi:10.1038/nature12639
Pomeroy, J., Krentz, A. D., Richardson, J. G., Berg, R. L., VanWormer, J. J., and Haws, R. M. (2021). Bardet-Biedl syndrome: Weight patterns and genetics in a rare obesity syndrome. Pediatr. Obes. 16 (2), e12703. doi:10.1111/ijpo.12703
Pottorf, T. S., Fagan, M. P., Burkey, B. F., Cho, D. J., Vath, J. E., and Tran, P. V. (2020). MetAP2 inhibition reduces food intake and body weight in a ciliopathy mouse model of obesity. JCI Insight 5 (2), 134278. doi:10.1172/jci.insight.134278
Ramos, C., Roberts, J. B., Jasso, K. R., Ten Eyck, T. W., Everett, T., Pozo, P., et al. (2021). Neuron-specific cilia loss differentially alters locomotor responses to amphetamine in mice. J. Neurosci. Res. 99 (3), 827–842. doi:10.1002/jnr.24755
Reiter, J. F., and Leroux, M. R. (2017). Genes and molecular pathways underpinning ciliopathies. Nat. Rev. Mol. Cell Biol. 18 (9), 533–547. doi:10.1038/nrm.2017.60
Rohrschneider, K., and Bolz, H. J. (2020). [The bardet-biedl syndrome - diagnosis and follow-up]. Klin. Monbl. Augenheilkd. 237 (3), 239–247. doi:10.1055/a-1118-3748
Rouabhi, M., Guo, D. F., Morgan, D. A., Zhu, Z., López, M., Zingman, L., et al. (2021). BBSome ablation in SF1 neurons causes obesity without comorbidities. Mol. Metab. 48, 101211. doi:10.1016/j.molmet.2021.101211
Rouault, A. A. J., Srinivasan, D. K., Yin, T. C., Lee, A. A., and Sebag, J. A. (2017). Melanocortin receptor accessory proteins (MRAPs): Functions in the melanocortin system and beyond. Biochim. Biophys. Acta. Mol. Basis Dis. 1863 (10), 2462–2467. doi:10.1016/j.bbadis.2017.05.008
Schou, K. B., Pedersen, L. B., and Christensen, S. T. (2015). Ins and outs of GPCR signaling in primary cilia. EMBO Rep. 16 (9), 1099–1113. doi:10.15252/embr.201540530
Segal-Lieberman, G., Bradley, R. L., Kokkotou, E., Carlson, M., Trombly, D. J., Wang, X., et al. (2003). Melanin-concentrating hormone is a critical mediator of the leptin-deficient phenotype. Proc. Natl. Acad. Sci. U. S. A. 100 (17), 10085–10090. doi:10.1073/pnas.1633636100
Seo, S., Guo, D. F., Bugge, K., Morgan, D. A., Rahmouni, K., and Sheffield, V. C. (2009). Requirement of Bardet-Biedl syndrome proteins for leptin receptor signaling. Hum. Mol. Genet. 18 (7), 1323–1331. doi:10.1093/hmg/ddp031
Shalata, A., Ramirez, M. C., Desnick, R. J., Priedigkeit, N., Buettner, C., Lindtner, C., et al. (2013). Morbid obesity resulting from inactivation of the ciliary protein CEP19 in humans and mice. Am. J. Hum. Genet. 93 (6), 1061–1071. doi:10.1016/j.ajhg.2013.10.025
Sheu, S. H., Upadhyayula, S., Dupuy, V., Pang, S., Deng, F., Wan, J., et al. (2022). A serotonergic axon-cilium synapse drives nuclear signaling to alter chromatin accessibility. Cell 185 (18), 3390–3407. e18. doi:10.1016/j.cell.2022.07.026
Shimogori, T., Lee, D. A., Miranda-Angulo, A., Yang, Y., Wang, H., Jiang, L., et al. (2010). A genomic atlas of mouse hypothalamic development. Nat. Neurosci. 13 (6), 767–775. doi:10.1038/nn.2545
Shinde, S. R., Nager, A. R., and Nachury, M. V. (2020). Ubiquitin chains earmark GPCRs for BBSome-mediated removal from cilia. J. Cell Biol. 219 (12), e202003020. doi:10.1083/jcb.202003020
Siljee, J. E., Wang, Y., Bernard, A. A., Ersoy, B. A., Zhang, S., Marley, A., et al. (2018). Subcellular localization of MC4R with ADCY3 at neuronal primary cilia underlies a common pathway for genetic predisposition to obesity. Nat. Genet. 50 (2), 180–185. doi:10.1038/s41588-017-0020-9
Simon, Á., Németh, J., Jávor, A., Komlósi, I., Bai, P., Oláh, J., et al. (2018). Feeding state and age dependent changes in melanin-concentrating hormone expression in the hypothalamus of broiler chickens. Acta Biochim. Pol. 65 (2), 251–258. doi:10.18388/abp.2017_2362
Singh, M., Garrison, J. E., Wang, K., and Sheffield, V. C. (2019). Absence of BBSome function leads to astrocyte reactivity in the brain. Mol. Brain 12 (1), 48. doi:10.1186/s13041-019-0466-z
Sipos, É., Komoly, S., and Ács, P. (2018). Quantitative comparison of primary cilia marker expression and length in the mouse brain. J. Mol. Neurosci. 64 (3), 397–409. doi:10.1007/s12031-018-1036-z
Soliman, A. T., Rajab, A., AlSalmi, I., and Asfour, M. G. (1996). Empty sellae, impaired testosterone secretion, and defective hypothalamic-pituitary growth and gonadal axes in children with Bardet-Biedl syndrome. Metabolism. 45 (10), 1230–1234. doi:10.1016/s0026-0495(96)90240-1
Stubbs, T., Koemeter-Cox, A., Bingman, J. I., Zhao, F., Kalyanasundaram, A., Rowland, L. A., et al. (2022). Disruption of dopamine receptor 1 localization to primary cilia impairs signaling in striatal neurons. J. Neurosci. 42 (35), 6692–6705. doi:10.1523/JNEUROSCI.0497-22.2022
Sun, J. S., Yang, D. J., Kinyua, A. W., Yoon, S. G., Seong, J. K., Kim, J., et al. (2021). Ventromedial hypothalamic primary cilia control energy and skeletal homeostasis. J. Clin. Invest. 131 (1), 138107. doi:10.1172/JCI138107
Szabó, N. E., Zhao, T., Cankaya, M., Theil, T., Zhou, X., and Alvarez-Bolado, G. (2009). Role of neuroepithelial Sonic hedgehog in hypothalamic patterning. J. Neurosci. 29 (21), 6989–7002. doi:10.1523/JNEUROSCI.1089-09.2009
Tchernof, A., and Després, J. P. (2013). Pathophysiology of human visceral obesity: An update. Physiol. Rev. 93 (1), 359–404. doi:10.1152/physrev.00033.2011
Thomas, S., Cantagrel, V., Mariani, L., Serre, V., Lee, J. E., Elkhartoufi, N., et al. (2015). Identification of a novel ARL13B variant in a Joubert syndrome-affected patient with retinal impairment and obesity. Eur. J. Hum. Genet. 23 (5), 621–627. doi:10.1038/ejhg.2014.156
Treier, M., O'Connell, S., Gleiberman, A., Price, J., Szeto, D. P., Burgess, R., et al. (2001). Hedgehog signaling is required for pituitary gland development. Development 128 (3), 377–386. doi:10.1242/dev.128.3.377
Tsang, S. H., Aycinena, A. R. P., and Sharma, T. (2018). Ciliopathy: Alström syndrome. Adv. Exp. Med. Biol. 1085, 179–180. doi:10.1007/978-3-319-95046-4_35
Vaisse, C., Reiter, J. F., and Berbari, N. F. (2017). Cilia and obesity. Cold Spring Harb. Perspect. Biol. 9 (7), a028217. doi:10.1101/cshperspect.a028217
Wang, H., Wu, M., Zhu, W., Shen, J., Shi, X., Yang, J., et al. (2010). Evaluation of the association between the AC3 genetic polymorphisms and obesity in a Chinese Han population. PLoS One 5 (11), e13851. doi:10.1371/journal.pone.0013851
Wang, J., Nikonorova, I. A., Silva, M., Walsh, J. D., Tilton, P. E., Gu, A., et al. (2021). Sensory cilia act as a specialized venue for regulated extracellular vesicle biogenesis and signaling. Curr. Biol. 31 (17), 3943–3951. e3. doi:10.1016/j.cub.2021.06.040
Wang, L., De Solis, A. J., Goffer, Y., Birkenbach, K. E., Engle, S. E., Tanis, R., et al. (2019). Ciliary gene RPGRIP1L is required for hypothalamic arcuate neuron development. JCI Insight 4 (3), e123337. doi:10.1172/jci.insight.123337
Wang, L., Liu, Y., Stratigopoulos, G., Panigrahi, S., Sui, L., Zhang, Y., et al. (2021). Bardet-Biedl syndrome proteins regulate intracellular signaling and neuronal function in patient-specific iPSC-derived neurons. J. Clin. Invest. 131 (8), 146287. doi:10.1172/JCI146287
Wang, Y., Bernard, A., Comblain, F., Yue, X., Paillart, C., Zhang, S., et al. (2021). Melanocortin 4 receptor signals at the neuronal primary cilium to control food intake and body weight. J. Clin. Invest. 131 (9), 142064. doi:10.1172/JCI142064
Wang, Y., Bernard, A., Comblain, F., Yue, X., Paillart, C., Zhang, S., et al. (2020). The primary cilium is required for MC4R control of food intake and body weight. bioRxiv.
Wang, Z., Li, V., Chan, G. C., Phan, T., Nudelman, A. S., Xia, Z., et al. (2009). Adult type 3 adenylyl cyclase-deficient mice are obese. PLoS One 4 (9), e6979. doi:10.1371/journal.pone.0006979
Wheway, G., Nazlamova, L., and Hancock, J. T. (2018). Signaling through the primary cilium. Front. Cell Dev. Biol. 6, 8. doi:10.3389/fcell.2018.00008
Yang, D., Wu, X., Wang, W., Zhou, Y., and Wang, Z. (2022). Ciliary type III adenylyl cyclase in the VMH is crucial for high-fat diet-induced obesity mediated by autophagy. Adv. Sci. 9 (3), e2102568. doi:10.1002/advs.202102568
Yang, D. J., Tran, L. T., Yoon, S. G., Seong, J. K., Shin, D. M., Choi, Y. H., et al. (2022). Primary cilia regulate adaptive responses to fasting. Metabolism. 135, 155273. doi:10.1016/j.metabol.2022.155273
Ye, F., Nager, A. R., and Nachury, M. V. (2018). BBSome trains remove activated GPCRs from cilia by enabling passage through the transition zone. J. Cell Biol. 217 (5), 1847–1868. doi:10.1083/jcb.201709041
Zhan, C., Zhou, J., Feng, Q., Zhang, J. E., Lin, S., Bao, J., et al. (2013). Acute and long-term suppression of feeding behavior by POMC neurons in the brainstem and hypothalamus, respectively. J. Neurosci. 33 (8), 3624–3632. doi:10.1523/JNEUROSCI.2742-12.2013
Zhang, Q., Nishimura, D., Seo, S., Vogel, T., Morgan, D. A., Searby, C., et al. (2011). Bardet-Biedl syndrome 3 (Bbs3) knockout mouse model reveals common BBS-associated phenotypes and Bbs3 unique phenotypes. Proc. Natl. Acad. Sci. U. S. A. 108 (51), 20678–20683. doi:10.1073/pnas.1113220108
Zhang, R., Tang, J., Li, T., Zhou, J., and Pan, W. (2022). INPP5E and coordination of signaling networks in cilia. Front. Mol. Biosci. 9, 885592. doi:10.3389/fmolb.2022.885592
Keywords: Neuronal cilia, obesity, Leptin-melanocortin signaling, GPCR signaling, energy homeostasis
Citation: Brewer KM, Brewer KK, Richardson NC and Berbari NF (2022) Neuronal cilia in energy homeostasis. Front. Cell Dev. Biol. 10:1082141. doi: 10.3389/fcell.2022.1082141
Received: 27 October 2022; Accepted: 11 November 2022;
Published: 08 December 2022.
Edited by:
Daniel Kopinke, University of Florida, United StatesReviewed by:
Kirk Mykytyn, The Ohio State University, United StatesKeren Hilgendorf, The University of Utah, United States
Copyright © 2022 Brewer, Brewer, Richardson and Berbari. This is an open-access article distributed under the terms of the Creative Commons Attribution License (CC BY). The use, distribution or reproduction in other forums is permitted, provided the original author(s) and the copyright owner(s) are credited and that the original publication in this journal is cited, in accordance with accepted academic practice. No use, distribution or reproduction is permitted which does not comply with these terms.
*Correspondence: Nicolas F. Berbari, bmJlcmJhcmlAaXVwdWkuZWR1