- 1Department of Orthopaedics, The First Affiliated Hospital of Nanjing Medical University, Nanjing, Jiangsu, China
- 2Jiangsu Institute of Functional Reconstruction and Rehabilitation, Nanjing, Jiangsu, China
- 3Spinal Cord Disease Research Center, Nanjing Medical University, Nanjing, Jiangsu, China
- 4Department of Orthopaedics, People’s Hospital Affiliated to Jiangsu University, Zhenjiang, Jiangsu, China
- 5Department of Anesthesia and Perioperative Medicine, The First Affiliated Hospital of Nanjing Medical University, Nanjing, Jiangsu, China
Deep venous thrombosis (DVT) is a common medical complication in patients with lumbar fractures. The current study aimed to investigate the predictive value of neutrophil extracellular traps (NETs) in postoperative DVT formation in patients with lumbar fractures and to develop a nomogram relating clinical admission information for prediction. Patients who underwent open reduction and pedicle screw internal fixation in the treatment of single-segment lumbar fracture in the Department of Spine Surgery, the First Affiliated Hospital of Nanjing Medical University, from December 2020 to June 2022 were enrolled in this study. Baseline data and laboratory results were collected from enrollees, and the primary study endpoint event was the occurrence of DVT in patients after surgery. Multivariable logistic regression analysis was used to identify risk factors associated with higher odds of DVT after surgery. A nomogram was constructed using the results of the multivariable model. The calibration plot and receiver operating characteristics (ROC) curve were used to show the satisfactory predictive capacity of the model. Of these 393 patients who did not have DVT preoperatively, 79 patients developed it postoperatively, and 314 did not, respectively. Multivariate analysis showed that higher body mass index (BMI) (BMI between 24 and 28: RR = 1.661, 95% CI = 0.891–3.094; BMI ≤28: RR = 5.625, 95% CI = 2.590–12.217; reference: BMI <24), neutrophils (RR = 1.157, 95% CI 1.042–1.285), D-dimer (RR = 1.098, 95% CI 1.000–1.206), and citrullinated histone H3 (CitH3) (RR = 1.043, 95% CI 1.026–1.060) were independent risk factors for postoperative DVT. Using the multivariable analysis, we then constructed a nomogram to predict DVT, which was found to have an area under the curve of 0.757 (95% CI = 0.693–0.820). Calibration plots also showed the satisfied discrimination and calibration of the nomogram. In conclusion, patients with lumbar fractures with postoperative DVT had higher levels of NETs in the circulation preoperatively compared to those without postoperative DVT. Furthermore, based on BMI, D-dimer, neutrophils, and CitH3, we developed a predictive model to predict postoperative DVT incidence in these patients.
Introduction
Besides tremendously impacting the patient’s quality of life, lumbar fractures burden families and society. Spine fractures account for 5%–6% of total body fractures, with the lumbar spine being a high prevalence location for spine fractures (Liu et al., 2012; Wang et al., 2012). Deep venous thrombosis (DVT) is one of the most common complications of lumbar fractures. Studies have shown that the incidence of venous thromboembolism after spine surgery ranges from 0.3% to 31% (Fineberg et al., 2013; Mosenthal et al., 2018). If not timely diagnosed or treated, DVT would develop into post-thrombotic syndrome, which leads to ulcers, necrosis, and pigmentation in lower limbs (Galanaud et al., 2018). Furthermore, due to the sizeable vascular diameter of the proximal lower extremity, the thrombus in this site is prone to dislodge and develop into fatal pulmonary thromboembolism (PE) (Qiu et al., 2021). Therefore, to reduce the incidence and mortality of DVT and ease the socioeconomic stress, there is an urgent demand for discovering predictors of DVT and taking corresponding precautions in the perioperative period of spine surgery besides improving the diagnostic technique (Le Gal et al., 2017; Ruskin, 2018; Wang et al., 2021).
Neutrophil extracellular traps (NETs), released by activated neutrophils, contain double-stranded DNA, histone, and granule proteins, which include neutrophil elastase (NE), cathepsin G, and myeloperoxidase (MPO) (Urban et al., 2009; Papayannopoulos, 2018; Yang and Liu, 2021). Increased NETs can be a double-edged sword. On the one hand, these components are essential to innate immune defense against pathogens (Jorch and Kubes, 2017). On the other hand, when not well-regulated, NETs have the potential to facilitate inflammatory pathologies of several diseases, such as deep vein thrombosis, by providing a scaffold for blood cells to attach to and activating coagulation pathways (Martinod et al., 2013; Savchenko et al., 2014). Researchers have recently conducted preliminary explorations of the relationship between NETs and thrombosis (Martinod et al., 2013; Kimball et al., 2016; Liu et al., 2021). It is assumed that the plasma level of citrullinated histone H3 (CitH3), a specific marker of NETs, could assist in diagnosing and predicting DVT in traumatic lower extremity fractures (Liu et al., 2021). Nevertheless, to our knowledge, the relationship between the preoperative level of NETs and postoperative DVT in patients undergoing spine surgery for lumbar fractures has not been fully elucidated.
In the present study, NETs released by neutrophils were observed in the circulation of patients with lumbar fractures. Our results also demonstrated that high levels of NETs in patients with lumbar fractures before surgery predict an increased risk of postoperative DVT. Notably, we developed a model for predicting the formation of DVT after surgery for lumbar fractures, and this model might be put into clinical application.
Materials and methods
Patient recruitment and study design
Our study included 393 patients who underwent open reduction and pedicle screw internal fixation in the treatment of single-segment lumbar fracture in the Department of Spine Surgery, the First Affiliated Hospital of Nanjing Medical University, from December 2020 to June 2022. Study participants were excluded if they met the following exclusion criteria: age <18 years; pregnant; combined with thrombosis or spinal cord injury; previous history of another vascular embolism such as myocardial infarction; under treatment with anticoagulation and antiplatelet such as heparin, warfarin, and aspirin within 4 weeks before admission; blood coagulation dysfunction; immune dysfunction; history of varicose veins; cardiovascular disease; infection; tumor and incomplete medical records. Once hospitalized, all participants routinely underwent doppler ultrasound (DUS) scanning of bilateral lower extremities to distinguish DVT or not (Suenaga et al., 2010; Hansrani et al., 2017). The same procedure was performed within 3 days after the operation. 79 Patients who were not found DVT when admitted but developed it after surgery were enrolled in the post-DVT group. The non-DVT group enrolled 314 patients with no thrombosis before and after surgery. Clinical and laboratory data were collected, including age, sex, body mass index (BMI), chronic medical diseases (hypertension, diabetes), hemoglobin, platelets, monocytes, neutrophils, lymphocytes, D-dimer, and CitH3, obtained during hospitalization. Figure 1 shows the flow chart of grouping. The study was approved by the Ethical Committee of The First Affiliated Hospital of Nanjing Medical University.
Blood sample analysis
Blood was collected from participants on the second day after hospitalization. 4 ml venous whole blood was anticoagulated with an EDTA tube, gently mixed into a 15 ml centrifuge tube, and centrifugated at 3,000 rpm at 4°C for 20 min. Then the plasma was obtained and stored at −80°C for subsequent use. CitH3 was quantified in plasma using the Citrullinated Histone H3 (clone 11D3) ELISA Kit (Cayman, 501,620) according to the manufacturer’s instructions. All samples were repeated in triplicate. In addition, each patient was inspected for blood routine examination on admission. So we obtain the results directly from the laboratory department.
Neutrophil isolation and neutrophil extracellular traps immunofluorescence
Polymorphonuclear neutrophils (PMNs) were isolated with Human StraightFrom Whole Blood CD15 microbeads kits (130-091-058, Miltenyi Biotech, Germany). Briefly, after erythrocyte lysis, the blood cells were incubated in the presence of CD15 microbeads. Blood cells were washed with separation buffer and centrifuged at room temperature at 300 g for 10 min. The pellets containing magnetically labeled cells were resuspended in a separation buffer and applied to the equilibrated whole blood column placed on the QuadroMACS. After extensive washing, the column was removed from the magnetic separator, and labeled cells were eluted using a whole-blood elution buffer. Finally, the PMNs were centrifuged at 300 g for 10 min and resuspended in Hank’s buffer with calcium and magnesium (Carminita et al., 2021).
After isolation, 1 × 105 PMNs were seeded onto a glass bottom cell culture dish coated with 0.001% poly-l-lysine (Sigma-Aldrich, P4707) and cultured at 37°C and 5% CO2 for 1 h. NETs formation by adherent PMNs was induced by stimulation with 20 nM Phorbol 12-myristate 13-acetate (PMA) (Sigma-Aldrich, P1585) for 1 h at 37°C. Then, the cells were fixed with 4% paraformaldehyde for 10 min at room temperature, followed by washing with PBS and blocking with Blocking Buffer (P0235, Beyotime, China). The cells were incubated overnight in the dark at 4°C with an anti-Histone H3 (citrulline R2 + R8 + R17) antibody (Abcam 5,103, diluted 1:1000) and an anti-Human/Mouse MPO Antibody (R&D Systems, AF3667, 10 µg/ml). After washing, the corresponding secondary antibodies conjugated to green and red fluorescent dyes were added to the cells, and nuclei were stained with DAPI. Images used in the figures were captured using a confocal microscope (Zeiss LSM710, Heidenheim, Germany).
Statistical analysis
SPSS version 26.0 (IBM Corporation, Armonk, NY) was used for statistical data analysis. Continuous variables were displayed as mean ± standard deviation. Categorical variables were displayed as counts (n) and percentages (%). The independent sample t-test evaluated differences in continuous variables between groups, and the Chi-square test was applied for categorical variables. Multivariate logistic regression analysis was performed to calculate the relative risk (RR) and the 95% confidence interval (CI). A nomogram was established to predict postoperative DVT. The calibration curve was used to display the predictive value of the prognostic models. Additionally, the receiver operating characteristic (ROC) curve was constructed, and the area under the ROC curve (AUC) was calculated to illustrate the value for predicting DVT. p < 0.05 was considered statistically significant.
Results
Demographic data of each experimental group
The baseline data of the study subjects are displayed in Table 1. There was no significant difference in age, gender, or chronic medical diseases (hypertension, diabetes) between non-DVT and post-DVT groups. Compared with the non-DVT group, patients in the post-DVT group experienced significantly increased levels of BMI (p < 0.001).
Patients in the post-deep venous thrombosis group had higher preoperative blood concentrations of citrullinated histone H3
To demonstrate the reticular structure of NETs, we stained MPO red and CitH3 green (Figure 2A). We used the proportion of green area (CitH3) in a field of view to measure the degree of NETs formation (Figure 2B). In the unstimulated (US) group, neutrophils isolated from the blood of patients in the post-DVT group showed a significant increase in the percentage of NETs in comparison with the corresponding control group (4.5 ± 1.0 versus 1.0 ± 0.6, p < 0.001). As predicted, this finding was amplified by the stimulation of PMA, and the result of the PMA group was similar to that of the US group (11.1 ± 2.9 versus 5.3 ± 1.3, p < 0.001). Moreover, for the plasma CitH3, the concentration in the post-DVT group was 35.18 ± 23.79 ng/ml, which was statistically higher than 22.49 ± 13.89 ng/ml in the non-DVT group (p < 0.001) (Figure 2C).
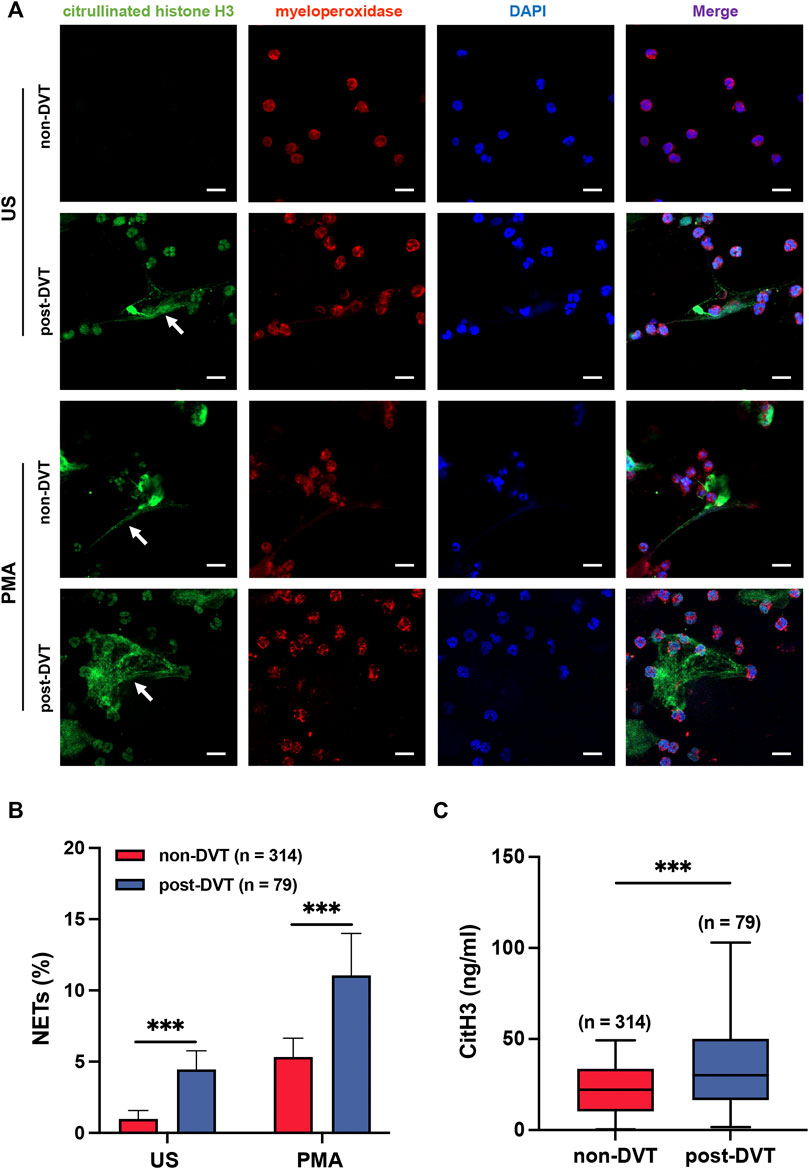
FIGURE 2. Neutrophils from the blood of patients in the post-DVT group form more NETs compared to the non-DVT group. (A) Representative images of Immunofluorescence staining of peripheral blood neutrophils from patients in non-DVT and post-DVT groups for MPO (red) and CitH3 (green) are shown, and the cell nuclei were stained with DAPI (blue). The white arrows indicate the typical NETs structure. Scale bars, 30 μm. US, unstimulated. (B) Results of the statistical analysis show the percentage of NETs in each group (n = 314 in the non-DVT group and n = 79 in the post-DVT group). ***p < 0.001. (C) Plasma samples were analyzed for CitH3 (n = 314 in the non-DVT group and n = 79 in the post-DVT group). ***p < 0.001.
Plasma citrullinated histone H3 acts as a predictive marker for deep venous thrombosis
All patients were routinely inspected for blood routine examination and D-dimer upon admission, and we obtained the clinical data of these indices from the laboratory department. As shown in Figure 3, compared with the non-DVT group, patients in the post-DVT group experienced significantly increased levels of neutrophils (p = 0.001), Neutrophil–lymphocyte ratio (NLR) (p = 0.010) and D-dimer (p = 0.002). The levels of other parameters, including hemoglobin, platelets, monocytes, and lymphocytes, were not statistically significant between the two groups (Supplementary Table S1).
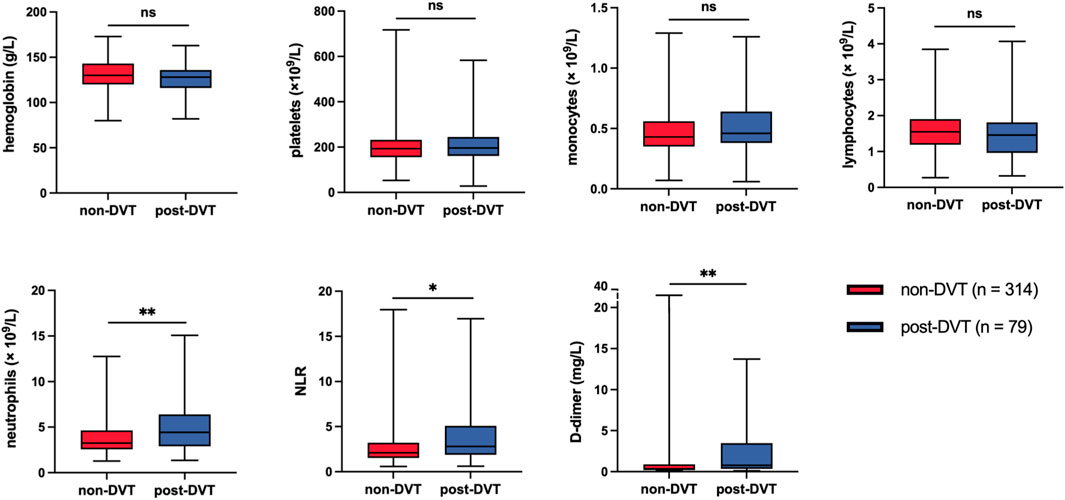
FIGURE 3. Statistical analysis of laboratory data after admission to hospital between the two study groups. ns, no significance; *p < 0.05; **p < 0.01; NLR, neutrophil–lymphocyte ratio.
In total, we selected five factors (BMI, neutrophils, NLR, D-dimer, and CitH3) that were significantly different between the two groups to perform multivariate logistic regression analysis. Four predictors remained significant in the multivariate logistic regression model after multivariable adjustment. Table 2 shows the independent predictors of DVT after lumbar surgery, which include BMI, neutrophils, D-dimer, and CitH3. Respectively, BMI between 24 and 28 group (versus BMI <24 group, RR = 1.661, 95% CI = 0.891–3.094), BMI >28 group (versus BMI <24 group, RR = 5.625, 95% CI = 2.590–12.217), D-dimer (RR = 1.098, 95% CI 1.000–1.206), neutrophils (RR = 1.157, 95% CI 1.042–1.285), and CitH3 (RR = 1.043, 95% CI 1.026–1.060) predicted an increased risk of DVT. The Hosmer-Lemeshow test showed the good fitness (X2 = 4.765, p = 0.782, Nagelkerke R2 = 0.236). To visualize the logistic regression model, we constructed a nomogram in which a graphic score was statistically assigned to each significant predictor for quick reference (Figure 4A). The total points of summed scores referred to an individual probability of DVT after lumbar surgery. For instance, red points in Figure 4 showed a patient’s scores for each item, and his probability of deep vein thrombosis after surgery was 74.7%. The calibration plot revealed good predictive accuracy between the nomogram prediction and actual observation (Figure 4B).
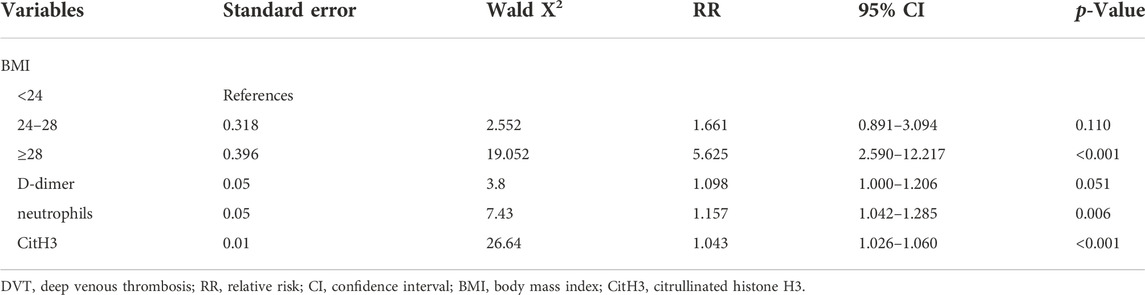
TABLE 2. Multivariate logistic regression analyses of risk factors for predicting postoperative DVT.
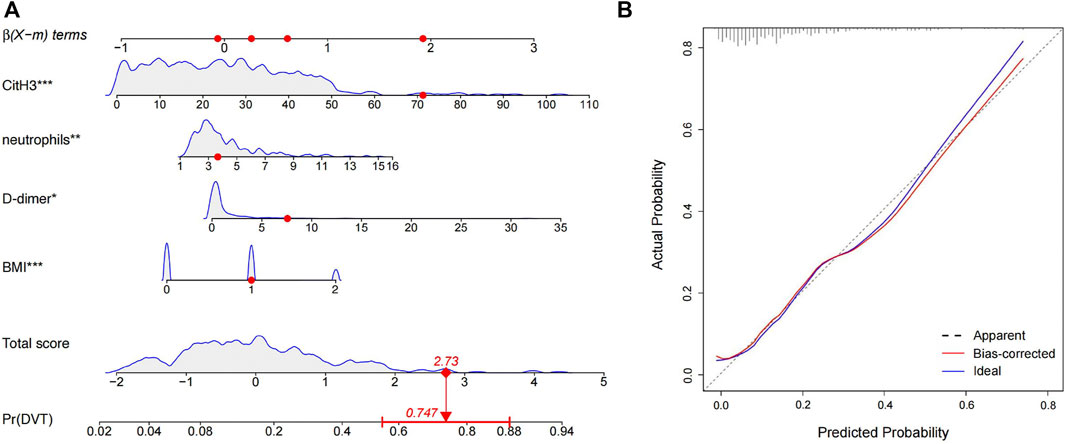
FIGURE 4. Nomogram and the corresponding calibration curve for predicting the risk of operative DVT. (A) Four predictors were involved in the predicting model, and each of them was assigned a graphic score. The sum of these four scores generated a result on the “total score” axis, and the “Pr (DVT)” axis shows the probability of DVT. Red dots represented the scores of a random patient. (B) The calibration curve of the predictive model for evaluating the risk of postoperative DVT.
As presented in Figure 5, the ROC curve marked in orange demonstrated that the AUC of the plasma level of CitH3 before surgery for predicting DVT was 0.650 (95% CI = 0.577–0.723). The optimal cutoff value for CitH3 was 47.925 ng/ml, and the sensitivity and specificity for predicting DVT were 29.1% and 97.8%, respectively. The ROC curve of CitH3 combined with the other three predictors was represented by a red line with an AUC of 0.757 (95% CI = 0.693–0.820). The sensitivity was 60.8%, and the specificity was 81.2%, respectively. The AUC for the other predictors were as follows: BMI, 0.621 (0.550–0.692); neutrophils, 0.622 (0.550–0.694); D-dimer, 0.667 (0.598–0.736).
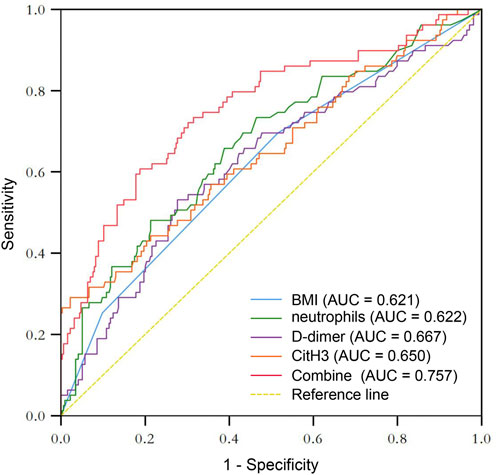
FIGURE 5. ROC curves of the risk factors in DVT, as well as the combination of these factors in predicting DVT.
Discussion
DVT, as a perioperative complication with a high incidence in patients with lumbar fractures, seriously affects the prognosis of patients (Chakravarthy et al., 2019). Clinical diagnosis alone is often unreliable and a large percentage of DVT occurring in the hospital are asymptomatic, leading to DVT being easily undiagnosed (Hansrani et al., 2017). Occasionally, the embolus will endanger the patient’s life once it dislodges from the vessel wall causing PE. Hence, finding a marker that can predict DVT in the early stage of fracture is crucial. In this study, we detected the level of NETs in patients with lumbar fractures. We found that a high level of preoperative NETs could predict postoperative DVT, which led us to develop a predictive model consisting of multiple markers, including NETs.
NETs can promote venous thromboembolism through different mechanisms. By providing a scaffold for erythrocytes, leukocytes, platelets, and activated coagulation factors, NETs stimulate the adhesion and aggregation of these substances directly (Lendvai et al., 2013; Martinod et al., 2013; Li et al., 2020). In addition, the polyanionic surface of NETs activates the intrinsic coagulation pathways, and NETs can also bind and induce the expression of tissue factor to initiate the extrinsic coagulation pathways (Iba et al., 2014; Carminita et al., 2021). Meanwhile, NE, the critical protein of NETs, inactivates anticoagulants in vivo by decomposition of tissue factor pathway inhibitors and thrombomodulin, thereby exacerbating the coagulation response (Iba et al., 2014; Waisberg et al., 2014).
According to the previous reports in the literature, NETs and DVT are inextricably linked. von Bruhl et al. (2012) found that activated neutrophils and NETs took part in thrombosis after inferior vena cava ligation in mice. Similar results suggest that NETs released by neutrophils are present in thrombi in experimental mice and coronary arteries of patients (Mangold et al., 2015). Circulating levels of nucleosomes and activated neutrophils were notably elevated in patients with DVT, and levels of either were associated with an approximately 3-fold increased risk of developing DVT (van Montfoort et al., 2013; Riegger et al., 2016). Clinical studies have further demonstrated that the circulating level of NETs is significantly increased during acute thrombotic episodes (Diaz et al., 2013; van Montfoort et al., 2013; Lee et al., 2018).
In our predictive model, in addition to NETs, BMI, Neutrophils, and D-dimer were also predictors of postoperative DVT. Patients with higher BMI had an increased risk of DVT after spine surgery, and this study noted that the probability of venous thrombosis increased by 9.4% for every 1 kg/m2 increase in BMI (Xu et al., 2020). Patients with high BMI may have relatively more adipose tissue, leading to increased release of inflammatory cytokines and enhanced synthesis of tissue factor, which activates exogenous coagulation pathways and thus promotes thrombus formation (Hunt, 2017). Additionally, obese patients are often associated with hyperlipidemia and slower blood flow than average, further inducing DVT (Vaya et al., 2002). There is a significant relationship between D-dimer and alterations in the hypercoagulability and fibrinolytic system of the organism (Goldenberg et al., 2004; Terpos et al., 2020). With high sensitivity, D-dimer is a well-established diagnostic marker for DVT. Furthermore, a multicenter study showed that the high level of D-dimer is both a diagnostic indicator and a predictor of DVT after spinal surgery (Cohen et al., 2014). Our results further corroborate the effectiveness of D-dimer in predicting DVT. Previous studies have shown that neutrophils act as an indicator of inflammation and contribute to DVT by releasing NETs and neutrophil histone modifications (Brill et al., 2012; Brinkmann, 2018). Notably, there is controversy regarding the relationship between neutrophil counts and venous thrombosis (Martos et al., 2020; Ferrer-Marin et al., 2021; Langiu et al., 2022). Studies suggested that NETs rather than increased neutrophil counts are crucial in promoting DVT formation (Noubouossie et al., 2019). The role of increased neutrophil counts in DVT formation after surgery is still undefined.
Several limitations of this study should be discussed. First, the NETs data in this study were at levels before surgical stimulation and did not examine the expression levels of NETs longitudinally during subsequent thrombolysis in patients. Follow-up studies will be meaningful to detect NETs in the period after surgery. Second, our study has not explored the specific molecular mechanisms of NETs formation. And finally, the conclusions obtained from this study need to be validated by a large sample and multicenter study.
To summarize, this study proposed associations between NETs and postoperative DVT in patients with lumbar fractures, suggesting that intervention with NETs is a promising strategy to prevent DVT. And as stated in the results section, the integration of multiple markers into a single signature has the potential to improve predictive value over that of a single one. So our study provides a predictive model mainly composed of BMI, D-dimer, neutrophils, and CitH3, which can predict patient DVT and deserves to be explored in future clinical practice.
Data availability statement
The raw data supporting the conclusion of this article will be made available by the authors, without undue reservation.
Ethics statement
The studies involving human participants were reviewed and approved by the Ethical Committee of The First Affiliated Hospital of Nanjing Medical University. The patients/participants provided their written informed consent to participate in this study.
Author contributions
SZ and GY conceived and designed the study. YL and QJ wrote the first draft and the final manuscript after the edition. XiZ, MW, SD, ZZ, and XuZ contributed to the acquisition of the clinical and laboratory data. ZS, JG, and HZ contributed to data interpretation and statistical analysis. ZH, JC, and HL revised the manuscript and provided valuable suggestions for this study. All authors read and approved the final draft for submission.
Funding
This study was supported by grants from the National Natural Science Foundation of China (81902211, 82030069, 81772351, 8151001184, and 82102570), the Jiangsu Natural Science Foundation (BK20191061), the Jiangsu Committee of Science and Technology-Social Development Plan (BE201755), the Young Scholars Fostering Fund of the First Affiliated Hospital of Nanjing Medical University (PY2021012) and the Postgraduate Research and Practice Innovation Program of Jiangsu Province (JX10214014).
Acknowledgments
The authors would like to thank the Core Facility of the First Affiliated Hospital of Nanjing Medical University for its help in the experiment.
Conflict of interest
The authors declare that the research was conducted in the absence of any commercial or financial relationships that could be construed as a potential conflict of interest.
The handling editor SHJ declared a past co- authorship with the authors JC, HL, ZZ, XZ, GY, SZ.
Publisher’s note
All claims expressed in this article are solely those of the authors and do not necessarily represent those of their affiliated organizations, or those of the publisher, the editors and the reviewers. Any product that may be evaluated in this article, or claim that may be made by its manufacturer, is not guaranteed or endorsed by the publisher.
Supplementary material
The Supplementary Material for this article can be found online at: https://www.frontiersin.org/articles/10.3389/fcell.2022.1071550/full#supplementary-material
References
Brill, A., Fuchs, T. A., Savchenko, A. S., Thomas, G. M., Martinod, K., De Meyer, S. F., et al. (2012). Neutrophil extracellular traps promote deep vein thrombosis in mice. J. Thromb. Haemost. 10 (1), 136–144. doi:10.1111/j.1538-7836.2011.04544.x
Brinkmann, V. (2018). Neutrophil extracellular traps in the second decade. J. Innate Immun. 10 (5-6), 414–421. doi:10.1159/000489829
Carminita, E., Crescence, L., Brouilly, N., Altie, A., Panicot-Dubois, L., and Dubois, C. (2021). DNAse-dependent, NET-independent pathway of thrombus formation in vivo. Proc. Natl. Acad. Sci. U. S. A. 118 (28), e2100561118. doi:10.1073/pnas.2100561118
Chakravarthy, V. B., Yokoi, H., Coughlin, D. J., Manlapaz, M. R., and Krishnaney, A. A. (2019). Development and implementation of a comprehensive spine surgery enhanced recovery after surgery protocol: The cleveland clinic experience. Neurosurg. Focus 46 (4), E11. doi:10.3171/2019.1.FOCUS18696
Cohen, A. T., Spiro, T. E., Spyropoulos, A. C., Desanctis, Y. H., Homering, M., Buller, H. R., et al. (2014). D-Dimer as a predictor of venous thromboembolism in acutely ill, hospitalized patients: A subanalysis of the randomized controlled MAGELLAN trial. J. Thromb. Haemost. 12 (4), 479–487. doi:10.1111/jth.12515
Diaz, J. A., Fuchs, T. A., Jackson, T. O., Kremer Hovinga, J. A., Lammle, B., Henke, P. K., et al. (2013). Plasma DNA is elevated in patients with deep vein thrombosis. J. Vasc. Surg. Venous Lymphatic Disord. 1 (4), 341–348.e1. doi:10.1016/j.jvsv.2012.12.002
Ferrer-Marin, F., Cuenca-Zamora, E. J., Guijarro-Carrillo, P. J., and Teruel-Montoya, R. (2021). Emerging role of neutrophils in the thrombosis of chronic myeloproliferative neoplasms. Int. J. Mol. Sci. 22 (3), 1143. doi:10.3390/ijms22031143
Fineberg, S. J., Oglesby, M., Patel, A. A., Pelton, M. A., and Singh, K. (2013). The incidence and mortality of thromboembolic events in lumbar spine surgery. Spine (Phila Pa 1976) 38 (13), 1154–1159. doi:10.1097/BRS.0b013e318286b7c0
Galanaud, J. P., Monreal, M., and Kahn, S. R. (2018). Epidemiology of the post-thrombotic syndrome. Thromb. Res. 164, 100–109. doi:10.1016/j.thromres.2017.07.026
Goldenberg, N. A., Knapp-Clevenger, R., and Manco-Johnson, M. J. (2004). Mountain States Regional ThrombophiliaElevated plasma factor VIII and D-dimer levels as predictors of poor outcomes of thrombosis in children. N. Engl. J. Med. 351 (11), 1081–1088. doi:10.1056/NEJMoa040161
Hansrani, V., Khanbhai, M., and McCollum, C. (2017). The diagnosis and management of early deep vein thrombosis. Adv. Exp. Med. Biol. 906, 23–31. doi:10.1007/5584_2016_103
Hunt, B. J. (2017). The effect of BMI on haemostasis: Implications for thrombosis in women's health. Thromb. Res. 151 (1), S53–S55. doi:10.1016/S0049-3848(17)30068-3
Iba, T., Miki, T., Hashiguchi, N., Tabe, Y., and Nagaoka, I. (2014). Is the neutrophil a 'prima donna' in the procoagulant process during sepsis? Crit. Care 18 (4), 230. doi:10.1186/cc13983
Jorch, S. K., and Kubes, P. (2017). An emerging role for neutrophil extracellular traps in noninfectious disease. Nat. Med. 23 (3), 279–287. doi:10.1038/nm.4294
Kimball, A. S., Obi, A. T., Diaz, J. A., and Henke, P. K. (2016). The emerging role of NETs in venous thrombosis and immunothrombosis. Front. Immunol. 7, 236. doi:10.3389/fimmu.2016.00236
Langiu, M., Palacios-Acedo, A. L., Crescence, L., Mege, D., Dubois, C., and Panicot-Dubois, L. (2022). Neutrophils, cancer and thrombosis: The new Bermuda triangle in cancer research. Int. J. Mol. Sci. 23 (3), 1257. doi:10.3390/ijms23031257
Le Gal, G., Righini, M., and Wells, P. S. (2017). Scoring systems for diagnosis of acute venous thromboembolism. Semin. Thromb. Hemost. 43 (5), 479–485. doi:10.1055/s-0037-1601332
Lee, K. H., Cavanaugh, L., Leung, H., Yan, F., Ahmadi, Z., Chong, B. H., et al. (2018). Quantification of NETs-associated markers by flow cytometry and serum assays in patients with thrombosis and sepsis. Int. J. Lab. Hematol. 40 (4), 392–399. doi:10.1111/ijlh.12800
Lendvai, D., Morawski, M., Negyessy, L., Gati, G., Jager, C., Baksa, G., et al. (2013). Neurochemical mapping of the human hippocampus reveals perisynaptic matrix around functional synapses in Alzheimer's disease. Acta Neuropathol. 125 (2), 215–229. doi:10.1007/s00401-012-1042-0
Li, T., Peng, R., Wang, F., Hua, L., Liu, S., Han, Z., et al. (2020). Lysophosphatidic acid promotes thrombus stability by inducing rapid formation of neutrophil extracellular traps: A new mechanism of thrombosis. J. Thromb. Haemost. 18 (8), 1952–1964. doi:10.1111/jth.14839
Liu, P., Yao, Y., Liu, M. Y., Fan, W. L., Chao, R., Wang, Z. G., et al. (2012). Spinal trauma in mainland China from 2001 to 2007: An epidemiological study based on a nationwide database. Spine (Phila Pa 1976) 37 (15), 1310–1315. doi:10.1097/BRS.0b013e3182474d8b
Liu, L., Zhang, W., Su, Y., Chen, Y., Cao, X., and Wu, J. (2021). The impact of neutrophil extracellular traps on deep venous thrombosis in patients with traumatic fractures. Clin. Chim. Acta. 519, 231–238. doi:10.1016/j.cca.2021.04.021
Mangold, A., Alias, S., Scherz, T., Hofbauer, M., Jakowitsch, J., Panzenbock, A., et al. (2015). Coronary neutrophil extracellular trap burden and deoxyribonuclease activity in ST-elevation acute coronary syndrome are predictors of ST-segment resolution and infarct size. Circ. Res. 116 (7), 1182–1192. doi:10.1161/CIRCRESAHA.116.304944
Martinod, K., Demers, M., Fuchs, T. A., Wong, S. L., Brill, A., Gallant, M., et al. (2013). Neutrophil histone modification by peptidylarginine deiminase 4 is critical for deep vein thrombosis in mice. Proc. Natl. Acad. Sci. U. S. A. 110 (21), 8674–8679. doi:10.1073/pnas.1301059110
Martos, L., Oto, J., Fernandez-Pardo, A., Plana, E., Solmoirago, M. J., Cana, F., et al. (2020). Increase of neutrophil activation markers in venous thrombosis-contribution of circulating activated protein C. Int. J. Mol. Sci. 21 (16), E5651. doi:10.3390/ijms21165651
Mosenthal, W. P., Landy, D. C., Boyajian, H. H., Idowu, O. A., Shi, L. L., Ramos, E., et al. (2018). Thromboprophylaxis in spinal surgery. Spine (Phila Pa 1976) 43 (8), E474–E481. doi:10.1097/BRS.0000000000002379
Noubouossie, D. F., Reeves, B. N., Strahl, B. D., and Key, N. S. (2019). Neutrophils: Back in the thrombosis spotlight. Blood 133 (20), 2186–2197. doi:10.1182/blood-2018-10-862243
Papayannopoulos, V. (2018). Neutrophil extracellular traps in immunity and disease. Nat. Rev. Immunol. 18 (2), 134–147. doi:10.1038/nri.2017.105
Qiu, T., Zhang, T., Liu, L., Li, W., Li, Q., Zhang, X., et al. (2021). The anatomic distribution and pulmonary embolism complications of hospital-acquired lower extremity deep venous thrombosis. J. Vasc. Surg. Venous Lymphat. Disord. 9 (6), 1391–1398.e3. doi:10.1016/j.jvsv.2021.03.004
Riegger, J., Byrne, R. A., Joner, M., Chandraratne, S., Gershlick, A. H., Ten Berg, J. M., et al. (2016). Histopathological evaluation of thrombus in patients presenting with stent thrombosis. A multicenter European study: A report of the prevention of late stent thrombosis by an interdisciplinary global European effort consortium. Eur. Heart J. 37 (19), 1538–1549. doi:10.1093/eurheartj/ehv419
Ruskin, K. J. (2018). Deep vein thrombosis and venous thromboembolism in trauma. Curr. Opin. Anaesthesiol. 31 (2), 215–218. doi:10.1097/ACO.0000000000000567
Savchenko, A. S., Martinod, K., Seidman, M. A., Wong, S. L., Borissoff, J. I., Piazza, G., et al. (2014). Neutrophil extracellular traps form predominantly during the organizing stage of human venous thromboembolism development. J. Thromb. Haemost. 12 (6), 860–870. doi:10.1111/jth.12571
Suenaga, M., Mizunuma, N., Kobayashi, K., Shinozaki, E., Matsusaka, S., Chin, K., et al. (2010). Management of venous thromboembolism in colorectal cancer patients treated with bevacizumab. Med. Oncol. 27 (3), 807–814. doi:10.1007/s12032-009-9289-6
Terpos, E., Ntanasis-Stathopoulos, I., Elalamy, I., Kastritis, E., Sergentanis, T. N., Politou, M., et al. (2020). Hematological findings and complications of COVID-19. Am. J. Hematol. 95 (7), 834–847. doi:10.1002/ajh.25829
Urban, C. F., Ermert, D., Schmid, M., Abu-Abed, U., Goosmann, C., Nacken, W., et al. (2009). Neutrophil extracellular traps contain calprotectin, a cytosolic protein complex involved in host defense against Candida albicans. PLoS Pathog. 5 (10), e1000639. doi:10.1371/journal.ppat.1000639
van Montfoort, M. L., Stephan, F., Lauw, M. N., Hutten, B. A., Van Mierlo, G. J., Solati, S., et al. (2013). Circulating nucleosomes and neutrophil activation as risk factors for deep vein thrombosis. Arterioscler. Thromb. Vasc. Biol. 33 (1), 147–151. doi:10.1161/ATVBAHA.112.300498
Vaya, A., Mira, Y., Ferrando, F., Contreras, M., Estelles, A., Espana, F., et al. (2002). Hyperlipidaemia and venous thromboembolism in patients lacking thrombophilic risk factors. Br. J. Haematol. 118 (1), 255–259. doi:10.1046/j.1365-2141.2002.03563.x
von Bruhl, M. L., Stark, K., Steinhart, A., Chandraratne, S., Konrad, I., Lorenz, M., et al. (2012). Monocytes, neutrophils, and platelets cooperate to initiate and propagate venous thrombosis in mice in vivo. J. Exp. Med. 209 (4), 819–835. doi:10.1084/jem.20112322
Waisberg, M., Molina-Cruz, A., Mizurini, D. M., Gera, N., Sousa, B. C., Ma, D., et al. (2014). Plasmodium falciparum infection induces expression of a mosquito salivary protein (Agaphelin) that targets neutrophil function and inhibits thrombosis without impairing hemostasis. PLoS Pathog. 10 (9), e1004338. doi:10.1371/journal.ppat.1004338
Wang, H., Zhang, Y., Xiang, Q., Wang, X., Li, C., Xiong, H., et al. (2012). Epidemiology of traumatic spinal fractures: Experience from medical university-affiliated hospitals in chongqing, China, 2001-2010. J. Neurosurg. Spine 17 (5), 459–468. doi:10.3171/2012.8.SPINE111003
Wang, Q., Yuan, L., Ding, X., and Zhou, Z. (2021). Prediction and diagnosis of venous thromboembolism using artificial intelligence approaches: A systematic review and meta-analysis. Clin. Appl. Thromb. Hemost. 27, 10760296211021162. doi:10.1177/10760296211021162
Xu, A., Sibai, H., Atenafu, E. G., Japs, K., and Seki, J. T. (2020). Universal venous thromboembolism policy is effective but may not adequately protect hospitalized cancer patients with larger BMI. J. Thromb. Thrombolysis 49 (1), 113–120. doi:10.1007/s11239-019-01975-x
Keywords: deep venous thrombosis, neutrophil extracellular traps, lumbar fracture, predicting marker, citrullinated histone H3
Citation: Li Y, Jiang Q, Zhou X, Wu M, Chen J, Liu H, Dai S, Zheng Z, Zhao X, Zhang C, Shi Z, Zhang H, Gu J, Huang Z, Yin G and Zhao S (2022) A prospective marker for the prediction of postoperative deep venous thrombosis: Neutrophil extracellular traps. Front. Cell Dev. Biol. 10:1071550. doi: 10.3389/fcell.2022.1071550
Received: 16 October 2022; Accepted: 01 November 2022;
Published: 16 November 2022.
Edited by:
Shu-Heng Jiang, Shanghai Cancer Institute, ChinaReviewed by:
Yuan Xiaoqiu, Changzheng Hospital, ChinaYanlun Zhu, The Chinese University of Hong Kong, China
Copyright © 2022 Li, Jiang, Zhou, Wu, Chen, Liu, Dai, Zheng, Zhao, Zhang, Shi, Zhang, Gu, Huang, Yin and Zhao. This is an open-access article distributed under the terms of the Creative Commons Attribution License (CC BY). The use, distribution or reproduction in other forums is permitted, provided the original author(s) and the copyright owner(s) are credited and that the original publication in this journal is cited, in accordance with accepted academic practice. No use, distribution or reproduction is permitted which does not comply with these terms.
*Correspondence: Zhenfei Huang, MTg4MDAxNjE3NTVAMTYzLmNvbQ==; Guoyong Yin, Z3VveW9uZ195aW5Ac2luYS5jb20=; Shujie Zhao, emhhb3NodWppZUBuam11LmVkdS5jbg==
†These authors have contributed equally to this work