- Epigeneres Biotech Pvt Ltd, Mumbai, India
The concept of dedifferentiation and reprogramming of mature somatic cells holds much promise for the three-front “war” against tissue damage, cancer, and aging. It was hoped that reprogramming human somatic cells into the induced pluripotent state, along with the use of embryonic stem cells, would transform regenerative medicine. However, despite global efforts, clinical applications remain a distant dream, due to associated factors such as genomic instability, tumorigenicity, immunogenicity, and heterogeneity. Meanwhile, the expression of embryonic (pluripotent) markers in multiple cancers has baffled the scientific community, and it has been suggested that somatic cells dedifferentiate and “reprogram” into the pluripotent state in vivo to initiate cancer. It has also been suggested that aging can be reversed by partial reprogramming in vivo. However, better methods are needed; using vectors or Yamanaka factors in vivo, for example, is dangerous, and many potential anti-aging therapies carry the same risks as those using induced pluripotent cells, as described above. The present perspective examines the potential of endogenous, pluripotent very small embryonic-like stem cells (VSELs). These cells are naturally present in multiple tissues; they routinely replace diseased tissue and ensure regeneration to maintain life-long homeostasis, and they have the ability to differentiate into adult counterparts. Recent evidence suggests that cancers initiate due to the selective expansion of epigenetically altered VSELs and their blocked differentiation. Furthermore, VSEL numbers have been directly linked to lifespan in studies of long- and short-lived transgenic mice, and VSEL dysfunction has been found in the ovaries of aged mice. To conclude, a greater interest in VSELs, with their potential to address all three fronts of this war, could be the “light at the end of the tunnel.”
Introduction
Over two decades ago, stem cells were thought to be “magic bullets” and were expected to completely revolutionize the field of medicine. However, despite huge investments, stem cells are not yet making significant contributions in the clinic (Bhartiya, 2019; Harvard Stem Cell Institute, 2022; Mallapaty, 2022). Despite the progress made in using human embryonic stem (hES) cells and induced pluripotent stem (iPS) cells, the use of hES cells continues to face challenges over ethical concerns; meanwhile, the existing roadblocks of tumorigenicity, immunogenicity, and heterogeneity prevent the clinical translation of hES/iPS cells. iPS cell lines show varying degrees of differentiation potential, associated genomic instability, and abnormal epigenetic status (Yamanaka, 2020).
Our team is especially intrigued by the recent attempts to convert iPS cells into mesenchymal stem/stromal cells (MSCs) (Eto et al., 2018; Xu et al., 2019; Liu, 2021; Rajasingh et al., 2021). Efforts like these make one wonder why iPS cells are used for paracrine support, rather than tapping into their regenerative potential. MSCs can be easily expanded from several sources, and even the microvesicles/exosomes derived from MSCs produce similar beneficial/regenerative effects upon transplantation (Zhang et al., 2020; Hade et al., 2021; Moghadasi et al., 2021). Does this mean that the future role of iPS cells will be reduced to providing exosomes? Several roadblocks still have to be overcome to bring iPS cells into the clinic.
How MSCs fit into the landscape of regenerative medicine: The connection to very small embryonic-like stem cells
Unlike hES and iPS cells, MSCs have entered the clinics, and have shown abundant promise (Jovic et al., 2022). As we recently discussed (Bhartiya et al., 2022a), the beneficial effects of transplanting MSCs, as reported in multiple clinical trials (Squillaro et al., 2016; Esfandyari et al., 2020), come from their capacity to rejuvenate (i.e., improve the microenvironment/niche of) diseased tissues by providing paracrine support to the tissue-resident, endogenous very small embryonic-like stem cells (VSELs), which in turn regenerate the diseased tissues. There is an ongoing debate on the true nature of MSCs, particularly whether they are stem or stromal cells (Caplan, 2017). They provide a niche for the hematopoietic stem cells (HSCs) in the bone marrow (Kfoury and Scadden, 2015), MSCs are indeed not stem cells; they overlap with the body’s population of pericytes (Caplan, 2008). Similar beneficial effects are also obtained by transplanting MSC-derived exosomes (Zhao et al., 2020; Rezaie et al., 2022). MSCs have the characteristic property of being adherent in nature and show the potential to rapidly expand in culture. Similar to HSCs, which differentiate into multiple types of blood cells, MSCs differentiate into adipocytes, osteoblasts, myocytes, and chondrocytes; however, neither HSCs nor MSCs have regenerative potential, which implies they have no potential to transdifferentiate and regenerate diseased tissues of other tissue-type and lineages. HSCs, better defined as “lineage-restricted” and “tissue-committed” progenitors, obtained from the cord blood or the bone marrow have had a tremendous clinical effect on cell regeneration in blood-related diseases (Gluckman et al., 1989; Ratajczak et al., 2022). Earlier studies reported MSC differentiation into cell types of other lineages (ectoderm, mesoderm, and endoderm), but this can be explained by the presence of VSELs or multilineage-differentiating stress-enduring (MUSE) cells, which exist as a sub-population; MSCs themselves are not pluripotent (Bhartiya, 2013; Dezawa, 2016). MSCs provide a niche and the required paracrine support for tissue-resident stem cells to function and to undergo normal expansion/differentiation.
An introduction to VSELs
VSELs were first reported in 2005 and were recently reviewed by Ratajczak et al. (2013) and Ratajczak et al. (2019). The germ-line origin of VSELs is the primordial germ cells (PGCs); being pluripotent, VSELs show the ability to differentiate into three germ layers, viz., the ectoderm, endoderm, and mesoderm, and in gametes both in vitro and in vivo, in both mice and humans (Havens et al., 2014; Monti et al., 2017a; Lahlil et al., 2018; Shaikh et al., 2017; Ratajczak et al., 2019). They reside in all adult tissues and participate in regular regeneration and remodeling under physiological conditions (Figure 1A). They remain undifferentiated, maintain their distinct spherical shape, possess euchromatin, show dark-blue-stained nuclei upon hematoxylin–eosin staining, have a high nucleo-cytoplasmic ratio, and express pluripotent transcription factors with open chromatin at the OCT-4 promoter (Shin et al., 2009). VSELs have been studied for their global gene expression in mice (Shin et al., 2012) as well as in humans (Virant-Klun et al., 2013; Lahlil et al., 2018). The gene expression of VSELs has been summarized and compared with ES, iPS, and HSCs (Bhartiya et al., 2016). As with ES cells, the Ezh2-dependent bivalent domain mechanism contributes to the pluripotent state of VSELs (Shin et al., 2012). However, unlike ES and iPS cells, VSELs do not readily divide in culture, and upon transplantation in vivo, they neither form teratoma upon injection into immuno-deficient mice nor complement a growing embryo, two gold-standard tests for proving a pluripotent state. The inability of VSELs to achieve this state is basically because of their unique epigenetic status and their quiescent state due to the erasure of differently methylated regions (DMRs) at some of the paternally imprinted genes involved in embryogenesis (Shin et al., 2010; Ratajczak et al., 2019), which allow them to achieve what the hES/iPS cells cannot (Figure 1A). Recent attempts to expand VSELs in vitro by treating with epigenetic modulators, such as pyrimidoindole derivative (UM171) and nicotinamide acid, were successful (Lahlil et al., 2018; Domingues et al., 2022). CD34+ cells are now being produced on an industrial scale in a “good manufacturing practice” facility with an increased fraction of LIN–CD45–CD133+ VSELs (Henon et al., 2022). Meanwhile, Domingues et al. (2022) showed that CD34+ VSELs represent an excellent source of true vasculogenic endothelial progenitors for vascular repair, especially since both ES and iPS cells have failed to show similar potential. Ratajczak et al. (2011) differentiated VSELs into HSCs in vitro by culturing on OP9 feeder support. VSELs sit at the top of the hematopoietic system hierarchy and give rise to HSCs, MSCs, and EPCs (Figure 1B), which further differentiate into all types of blood cells, pericytes, and endothelial cells (Taichman et al., 2010; Domingues et al., 2022).
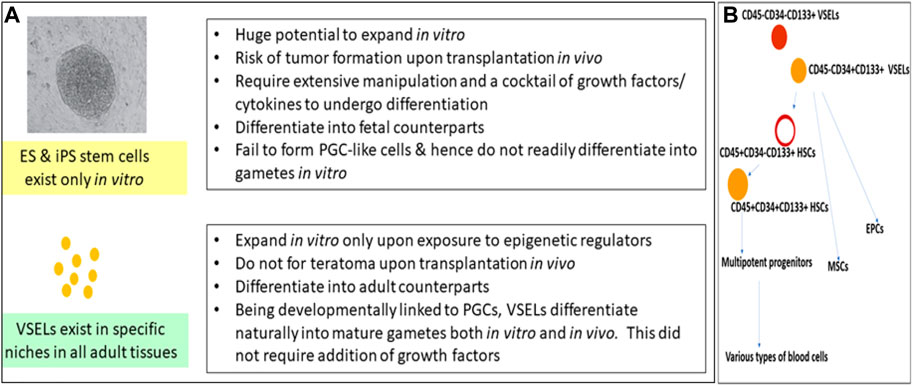
FIGURE 1. (A) compares VSELs with ES and iPS cells. Pluripotent VSELs exist in adult tissues; they regularly participate in adult tissue regeneration and remodeling, with no risk of teratoma formation. More focused research efforts are required to make use of their regenerative potential. (B) shows that VSELs sit at the top of the hematopoietic system hierarchy, and that they give rise to HSCs, MSCs, and EPCs. VSELs, very small embryonic-like stem cells; ES cells, embryonic stem cells; iPS cells, induced pluripotent stem cells; HSCs, hematopoietic stem cells; MSCs, mesenchymal stem cells; EPCs, endothelial progenitor cells.
VSELs ensure replacement of tissue-committed progenitors throughout life and are activated to regenerate damaged organ tissue upon injury in vivo, e.g., in the lungs (Ciechanowicz et al., 2021), pancreas (Mohammad et al., 2020), bone (Leppik et al., 2020), and endometrial epithelium (Singh and Bhartiya, 2022). Being developmentally linked to PGCs, which are natural precursors for gametes, VSELs differentiate into oocytes and sperm in vitro (Bhartiya et al., 2017); however, it has proven difficult to differentiate human ES and iPS cells in vitro into PGC-like cells (PGCLCs), and thus, the hype surrounding the potential conversion hES and iPS cells into gametes for clinical use has not yet translated into reality. It is easy to regulate cellular differentiation by exposing stem cells to growth factors and cytokines in vitro, but modulating their epigenetic status is not yet possible. VSELs are also responsible for the regeneration of diseased organs in conditions where transplanted mesenchymal stromal cells provide paracrine support (Bhartiya et al., 2022a). Complete restoration of spermatogenesis from VSELs that survived chemotherapy was observed in vivo in busulfan-treated mice when MSCs were transplanted (Anand et al., 2016). VSELs and OSCs together are active in adult ovaries, resulting in oogenesis that is similar to spermatogenesis observed in males (Sharma and Bhartiya, 2021a). However, VSELs have remained elusive during the lineage-tracing studies reported so far because of their quiescent state; our recent work uncover their role in various biological processes in vivo, we have had success tracking fate of GFP-tagged VSELs (Bhartiya et al., 2022b).
In addition to VSELs, adult tissues also harbor actively dividing and lineage-restricted tissue-committed stem cells (TCSCs) or the “progenitors” with limited plasticity, such as HSCs in the hematopoietic system and spermatogonial stem cells (SSCs) in the testes. VSELs serve as a back-up pool to give rise to tissue-committed progenitors and maintain life-long tissue homeostasis (Figure 2), and both VSELs and the tissue-specific progenitors are also mobilized when tissue function is affected. VSELs and progenitors express ERα, ERβ, and FSHR, and are thus vulnerable to various endocrine insults during early development. VSELs therefore have the potential to carry these insults into adult life, (since other somatic cells that also get exposed have limited life span and are regularly replaced by the VSELs), where these insults can manifest as a wide spectrum of pathologies, including cancer. It is the excessive self-renewal of pluripotent VSELs and their blocked differentiation (Figure 2) that initiate various diseases, including cancer (Bhartiya et al., 2022c).
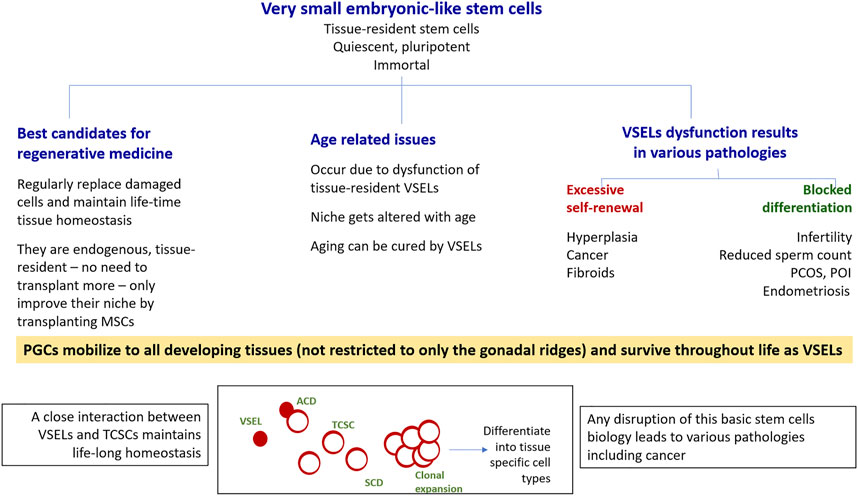
FIGURE 2. VSELs have the potential to win the war for human health on three major fronts. As shown, VSELs have the ability to regenerate adult tissues with none of the associated problems currently facing human embryonic and induced pluripotent stem cells, including ethical concerns as well as genomic instability, tumorigenicity, immunogenicity, and heterogeneity. Age-related disorders occur due to VSEL dysfunction. Various pathologies, including cancer, occur due to the dysfunction of the stem/progenitor cell compartment in adult tissues, as shown in the bottom panel. Excessive self-renewal of VSELs results in cancer, and the blocking of stem/progenitor cells differentiation (to different extents) gives rise to a wide spectrum of pathologies. This was first revealed through studying the effects of neonatal exposure to endocrine disruption on mouse reproductive tissues, but similar mechanisms could exist in other organs as well. More focused research on various aspects of VSELs is needed. VSELs, very small embryonic-like stem cells; PGCs, primordial germ cells; TCSCs, tissue-committed stem cells (progenitors); ACD, asymmetrical cell division; SCD, symmetrical cell division.
VSELs and HSCs: The CD34 connection
CD34 is a transmembrane phosphoglycoprotein, first identified on hematopoietic stem and progenitor cells. VSELs are at the top of the hierarchy that comprises HSCs, MSCs, and EPCs. VSELs are more primitive and give rise to progenitor HSCs (Taichman et al., 2010; Ratajczak et al., 2011a; Domingues et al., 2022) by undergoing asymmetrical cell divisions (Ganguly et al., 2018). VSELs are 2–6 µm cells with a surface phenotype of LIN–CD45–CD133+ in humans and LIN–CD45–SCA-1+ in mice, whereas HSCs have a surface phenotype of LIN–CD45+CD133+ in humans and LIN–CD45+SCA-1+ in mice. There is heterogeneity among VSELs and HSCs based on the expression of markers. Most intriguing is the expression of CD34 on the cell surface. Earlier, CD34+ HSCs had been thought to be the most primitive, but CD34– HSCs have recently been reported, altering the stem cell hierarchy (Sonoda, 2021). This hierarchy of stem cells in the hematopoietic system needs further modification, as VSELs are the most primitive stem cells (Figure 1B).
We undertook a study on human cord blood and peripheral blood to evaluate the expression of CD34 on LIN–CD45– VSELs and LIN–CD45+ HSCs via flow cytometry (Shaikh et al., 2017, unpublished data). The most primitive VSELs are LIN–CD45–CD34–, which exist along with LIN–CD45−CD34+ VSELs. A small fraction of LIN–CD45+ HSCs are CD34–, but the majority of them express CD34. CD34 is also expressed by MSCs, EPCs, and several other cells, but all these cells are also positive for lineage markers. Thus, as shown in Figure 1B, LIN–CD45–CD34– CD133+ VSELs are the most primitive stem cells in the hematopoietic system, with the potential to regenerate all types of tissues due to their pluripotent state. On the other hand, HSCs possess limited potential in that they can only regenerate tissue damaged by blood diseases. The many failed efforts to regenerate other tissues using bone marrow- or cord blood-derived mononuclear cells taught the global scientific community that progenitors become lineage-committed and tissue-restricted, and have no potential to cross boundaries or transdifferentiate.
VSELs and cancer
The somatic mutation theory (SMT) of cancer suggesting that mutations initiate cancer has recently been challenged by several groups in favor of the tissue organization field theory (TOFT), which describes cancer as “development gone wrong.” There have been calls for abandoning the SMT in favor of TOFT (Bizzarri and Cucina, 2016; Sonnenschein and Soto, 2020; Monti et al., 2022). The presence of embryonic markers in multiple cancers has intrigued the research community, and several researchers have postulated concepts such as the reprogramming and dedifferentiation of somatic cells to their pluripotent, OCT-4–expressing stem-like state to initiate cancer (Carvalho, 2020; Shivdasani et al., 2021). They postulate that a process similar to reprogramming somatic cells in a Petri dish to produce iPS cells may occur naturally in the body tissue, producing cancer stem cells and therefore leading to cancer initiation. Cancer is not a “genetic disease” but rather a stem cell disease; OCT-4–positive VSELs have been implicated in the initiation of cancers (rather than the random reprogramming of somatic cells) in multiple organs (Bhartiya et al., 2022c; Kaushik and Bhartiya 2022; Singh and Bhartiya 2022). Mutations may possibly occur as a consequence of the cancer, as the cells undergo rapid expansion.
We can make progress in the war against cancer by understanding the potential role of VSELs, as embryonic remnants, in initiating cancer (Ratajczak et al., 2009). Restoring the quiescence of VSELs and their normal functioning provides an interesting therapeutic target for the treatment of cancer.
VSELs and aging
Aging is an inevitable part of life, and many different diseases, including cancers, Alzheimer’s disease, osteoporosis, cardiovascular diseases, and cognitive dysfunction, manifest as age increases. Interest in developing methods that can reverse the aging process, and thereby facilitate an extended period of life with rejuvenated organs, is growing; indeed, these efforts have attracted enormous amounts of attention and huge investments in recent years (Eisenstein, 2022). It has been demonstrated that short-term induction of Yamanaka factors has the potential to roll back cellular aging and repair tissues without reverting to pluripotency (Eisenstein, 2022). Partial reprogramming has reversed age-related phenotypes in eye, muscle, and other tissues in cultured mammalian cells, and even in genetically engineered rodent models, by countering epigenetic changes (Eisenstein, 2022). However, while these therapies have huge therapeutic potential to reverse age-related diseases, scientists are currently focused on more fundamental research. The exact mechanisms responsible for cellular reprogramming and how the epigenetic state becomes reversed remain to be understood. Ratajczak’s group has shown that the number of VSELs is correlated with longevity and caloric restriction, and that regular exercise and metformin have a positive effect on maintaining VSELs in adult tissues (Shin et al., 2010; Ratajczak et al., 2017). Laron and Ames dwarf mice with a deficiency of the GH receptor (GHR) were found to have severely reduced levels of plasma Igf1 and to live 30%–40% longer than their normal littermates; the number of VSELs in their bone marrow was 3-fold to 4-fold higher than normal during aging, However, both short-lived transgenic mice expressing bovine growth hormone (bGH) and wild-type animals injected with bGH for 2 months experienced an accelerated depletion of VSELs from the bone marrow, as well as premature aging (Ratajczak et al., 2011).
The ovary has been described as the pacemaker for physiological aging. Ovarian aging sets in earlier than aging in other organs of the body and, in turn, ushers in the start of various other diseases. Bhartiya’s group studied the potential role of VSELs in ovarian aging. VSELs reside in the surface epithelium of the ovary, along with the “progenitor” ovarian stem cells (OSCs) (Bhartiya and Patel, 2018). Under normal physiological conditions, VSELs and OSCs undergo neo-oogenesis and primordial follicle assembly in a regular manner across the estrous cycle (Sharma and Bhartiya, 2021b). In contrast, one study found that neonatal exposure to endocrine disruption in mouse pups (by treating them with estradiol and diethylstilbestrol) resulted in polycystic ovarian syndrome and primary ovarian insufficiency in adult life (Sharma and Bhartiya, 2022a). Epithelial cell smears from aged ovaries have shown VSELs, OSCs, and increased numbers of germ cell nests. Moreover, meiosis and further differentiation into oocytes were blocked. Thus, ovarian aging occurs due to VSEL dysfunction and a compromised niche (Sharma and Bhartiya, 2022b). The conversion of a pluripotent VSEL with open chromatin into OSCs involves extensive epigenetic changes, and this process is affected by aging. Ovarian aging can be reversed by transplanting MSCs directly into the ovaries (Tian et al., 2021; Wu et al., 2022) and by providing a younger niche. Aged ovaries possess rare premeiotic germ cells that can generate oocytes, following transplantation into a young host environment (Niikura et al., 2009). Tissue and organ rejuvenation and senescence/aging are closely related to the function of stem cells.
Discussion
It has been more than 10 years since the publication of few studies that failed to detect VSELs via flow cytometry (Abbott 2013). But progress is the essence of life! The protocols to isolate VSELs have become more robust and easily replicable, and VSEL biology has matured over the last decade. VSELs have now been reported in multiple adult tissues by 60 independent groups (Ratajczak et al., 2019), and it is time to create a strong case for VSELs in the field and to resolve the question as to which are the “ideal” stem cell candidates for regenerative medicine. The resolution of the controversy surrounding VSELs is not an inconsequential “academic” pursuit; it directly impacts human health on three major fronts – i.e., regenerative medicine and the wars against cancer and aging (in terms of both diagnosis and treatment).
A failure to acknowledge the existence of VSELs could leave patients deprived of treatments on all three of these fronts. We therefore wish to inspire a new way of thinking, in the hope that it can spur rapid progress on these three fronts – especially in the war against cancer, which has been ongoing since 1971 – hence, for over five decades. Ignoring the presence of VSELs in adult tissues is no longer justifiable.
VSELs and pluripotent stem cells: The similarities
• They express pluripotent markers.
• They demonstrate an ability to differentiate into three germ layers, as well as into germ cells (Kucia et al., 2006; Monti et al., 2017b; Shaikh et al., 2017).
VSELs and pluripotent stem cells: The differences
• VSELs do not integrate into a developing embryo.
• VSELs do not form teratoma in SCID mice.
• VSELs do not divide and expand in culture; however, there has been some recent success in this regard (Ratajczak et al 2017; Lahlil et al 2018; Henon et al 2022).
How VSELs compare to hES and iPS cells
• VSELs are tissue-resident, endogenous, pluripotent stem cells that are found in the body; hES and iPS cells exist only in vitro, as tissue-culture artefacts (Hasson et al., 2007), with no equivalent cells in the body.
• VSELs have unique features that keep them quiescent in nature; otherwise, cancers will spontaneously occur (Shin et al., 2009; Mierzejewska et al., 2013). The tumorigenic potential of pluripotent VSELs is therefore managed by natural body processes, whereas humans have not yet succeeded in resolving the tumorigenicity-related concerns associated with hES/iPS cells and/or their derivatives upon transplantation.
• VSELs, being developmentally linked to PGCs, differentiate into gametes in vitro—which has not yet been accomplished using hES/iPS cells (Virant-Klun 2018; Bhartiya et al., 2017 and 2014).
• VSELs differentiate into various adult cell types in the body in vivo and also functionally integrate—a feat that has not yet been achieved with transplanted iPS cells or their progenitors.
• Both human and mouse VSELs express steroid and gonadotropin hormone receptors; thus, they function under the influence of hormones within the body (Patel et al., 2013; Abdelbaset-Ismail et al., 2016; Bhartiya et al., 2021) and endocrine insults during early development lead to various pathologies due to VSELs dysfunctions.
• Unlike iPS and hES cells, which undergo symmetrical cell divisions in culture, VSELs possess a unique ability to undergo asymmetrical cell divisions, whereby they self-renew and give rise to a slightly bigger progenitor with a distinct fate (Bhartiya et al., 2018).
The average human life expectancy has increased in recent times, and the burden of age-related diseases has increased along with it. A developing field of research is focused on the reversal of the aging phenotype via in vivo partial reprogramming through introducing Yamanaka factors (Ocampo et al., 2016; Sikder et al., 2022). It is intriguing to see VSELs and Yamanaka factors at the crossroads of aging research, similar to making iPS cells. Many questions about cell reprogramming, both in vitro and in vivo, remain. In particular, it is unclear why only a very small proportion of somatic cells, not all of them, can be reprogrammed to their pluripotent state in vitro. It has been reported that a sub-population of pluripotent stem cells is the primary source of iPS cells in human fibroblast culture (Wakao et al., 2011). We postulate that introducing Yamanaka factors both in vitro and in vivo most likely stimulates VSELs, causing them to overcome quiescence and expand in the culture as iPS cells, allowing them to spontaneously reverse aging in vivo. However, better methods are needed: using vectors or Yamanaka factors in vivo, for example, is dangerous, and many potential anti-aging therapies carry the same risks as those using iPS cells. Similarly, organoids, which are currently attracting a lot of attention in the field of cancer biology, are formed on a regular basis due to clonal expansion and incomplete cytokinesis of tissue-resident VSELs and progenitors cells (Figure 1, Bhartiya et al., 2018).
To conclude, the scientific community seems to be lagging in the three-front war against tissue damage, aging, and cancer, especially where the use of stem cells to treat these conditions is concerned. The debate over which stem cells are “best” for regenerative medicine remains unsettled, and surprisingly, it is still not understood how cancer begins. It is our hope that greater interest in VSELs, and greater consideration of their potential role as a common answer to all three of these problems, will foster the creation of new methods to achieve regeneration, making it possible to both prevent cancer and treat it without causing recurrence. Moreover, aging is also a stem cell disease, and it too can be reversed by manipulating VSELs (Figure 1). A broader consensus in support of VSELs, based on published evidence, has the potential to lead to new ways of thinking that will help mankind.
Data availability statement
The original contributions presented in the study are included in the article/supplementary materials; further inquiries can be directed to the corresponding author.
Ethics statement
This article includes all published data, and necessary approvals were taken.
Author contributions
DB wrote the original draft. NJ reviewed the draft and provided additional inputs. AnT and AsT proofread and provided inputs. All authors read and agreed to the final version of the article.
Funding
This study was supported by EBPL, Manuscript EPI/REV/03/2022. The funder had no involvement in the study design, analysis, interpretation of data.
Conflict of interest
Authors DB, NJ, AnT, and AsT are employees of a startup company in India named Epigeneres Biotech Pvt., Ltd. AnT and AsT are owners of Epigeneres Biotech Pvt., Ltd.
Publisher’s note
All claims expressed in this article are solely those of the authors and do not necessarily represent those of their affiliated organizations, or those of the publisher, the editors, and the reviewers. Any product that may be evaluated in this article, or claim that may be made by its manufacturer, is not guaranteed or endorsed by the publisher.
References
Abdelbaset-Ismail, A., Suszynska, M., Borkowska, S., Adamiak, M., Ratajczak, J., Kucia, M., et al. (2016). Human haematopoietic stem/progenitor cells express several functional sex hormone receptorss. J. Cell. Mol. Med. 20 (1), 134–146. doi:10.1111/jcmm.12712
Anand, S., Bhartiya, D., Sriraman, K., and Mallick, A. (2016). Underlying mechanisms that restore spermatogenesis on transplanting healthy niche cells in busulphan treated mouse testis. Stem Cell Rev. Rep. 12 (6), 682–697. doi:10.1007/s12015-016-9685-1
Bhartiya, D., Anand, S., Patel, H., and Parte, S. (2017). Making gametes from alternate sources of stem cells: Past, present and future. Reprod. Biol. Endocrinol. 15 (1), 89. doi:10.1186/s12958-017-0308-8
Bhartiya, D., Mohammad, S. A., Singh, P., Sharma, D., and Kaushik, A. (2022b). GFP tagged VSELs help delineate novel stem cells biology in multiple adult tissues. Stem Cell Rev. Rep. 18 (5), 1603–1613. doi:10.1007/s12015-022-10401-4
Bhartiya, D., Patel, H., Ganguly, R., Shaikh, A., Shukla, Y., Sharma, D., et al. (2018). Novel insights into adult and cancer stem cell biology. Stem Cells Dev. 27 (22), 1527–1539. doi:10.1089/scd.2018.0118
Bhartiya, D., Shaikh, A., Anand, S., Patel, H., Kapoor, S., Sriraman, K., et al. (2016). Endogenous, very small embryonic-like stem cells: Critical review, therapeutic potential and a look ahead. Hum. Reprod. Update 23 (1), 41–76. doi:10.1093/humupd/dmw030
Bhartiya, D., Singh, P., Sharma, D., and Kaushik, A. (2022a). Very small embryonic-like stem cells (VSELs) regenerate whereas mesenchymal stromal cells (MSCs) rejuvenate diseased reproductive tissues. Stem Cell Rev. Rep. 18 (5), 1718–1727. doi:10.1007/s12015-021-10243-6
Bhartiya, D., Anand, S., Patel, H., and Parte, S. (2017). Making gametes from alternate sources of stem cells: Past, present and future. Reprod. Biol. Endocrinol. 15 (1), 89–14. doi:10.1186/s12958-017-0308-8
Bhartiya, D. (2013). Are mesenchymal cells indeed pluripotent stem cells or just stromal cells? OCT-4 and VSELs biology has led to better understanding. Stem Cells Int. 2013, 547501. doi:10.1155/2013/547501
Bhartiya, D. (2019). Clinical translation of stem cells for regenerative medicine: A comprehensive analysis. Circ. Res. 124 (6), 840–842. doi:10.1161/CIRCRESAHA.118.313823
Bhartiya, D., Hinduja, I., Patel, H., and Bhilawadikar, R. (2014). Making gametes from pluripotent stem cells–a promising role for very small embryonic-like stem cells. Reprod. Biol. Endocrinol. 12 (1), 114. doi:10.1186/1477-7827-12-114
Bhartiya, D., Kaushik, A., Singh, P., and Sharma, D. (2022c). Cancer initiates due to excessive self-renewal and blocked differentiation of tissue-resident, OCT-4 positive VSELs. Stem Cell Rev. Rep. 18, 3112–3114. doi:10.1007/s12015-022-10424-x
Bhartiya, D., and Patel, H. (2021). An overview of FSH-FSHR biology and explaining the existing conundrums. J. Ovarian Res. 14 (1), 144. doi:10.1186/s13048-021-00880-3
Bhartiya, D., Patel, H., Ganguly, R., Shaikh, A., Shukla, Y., Sharma, D., et al. (2018). Novel insights into adult and cancer stem cell biology. Stem Cells Dev. 27 (22), 1527–1539. doi:10.1089/scd.2018.0118
Bhartiya, D., Patel, H., Kaushik, A., Singh, P., and Sharma, D. (2021). Endogenous, tissue-resident stem/progenitor cells in gonads and bone marrow express FSHR and respond to FSH via FSHR-3. J. Ovarian Res. 14 (1), 145. doi:10.1186/s13048-021-00883-0
Bizzarri, M., and Cucina, A. (2016). SMT and TOFT: Why and how they are opposite and incompatible paradigms. Acta Biotheor. 64 (3), 221–239. doi:10.1007/s10441-016-9281-4
Caplan, A. I. (2008). All MSCs are pericytes. Cell Stem Cell 3 (3), 229–230. doi:10.1016/j.stem.2008.08.008
Caplan, A. I. (2017). Mesenchymal stem cells: Time to change the name. Stem Cells Transl. Med. 6 (6), 1445–1451. doi:10.1002/sctm.17-0051
Carvalho, J. (2020). Cell reversal from a differentiated to a stem-like state at cancer initiation. Front. Oncol. 10, 541. doi:10.3389/fonc.2020.00541
Ciechanowicz, A. K., Sielatycka, K., Cymer, M., Skoda, M., Suszyńska, M., Bujko, K., et al. (2021). Bone marrow-derived VSELs engraft as lung epithelial progenitor cells after bleomycin-induced lung injury. Cells 10, 1570. doi:10.3390/cells10071570
Dezawa, M. (2016). Muse cells provide the pluripotency of mesenchymal stem cells: Direct contribution of muse cells to tissue regeneration. Cell Transpl. 25 (5), 849–861. doi:10.3727/096368916X690881
Domingues, A., Rossi, E., Bujko, K., Detriche, G., Richez, U., Blandinieres, A., et al. (2022). Human CD34+ very small embryonic-like stem cells can give rise to endothelial colony-forming cells with a multistep differentiation strategy using UM171 and nicotinamide acid. Leukemia 36 (5), 1440–1443. doi:10.1038/s41375-022-01517-0
Eisenstein, M. (2022). Rejuvenation by controlled reprogramming is the latest gambit in anti-aging. Nat. Biotechnol. 40, 144–146. doi:10.1038/d41587-022-00002-4
Esfandyari, S., Chugh, R. M., Park, H. S., Hobeika, E., Ulin, M., and Al-Hendy, A. (2020). Mesenchymal stem cells as a bioorgan for treatment of female infertility. Cells 9 (10), 2253. doi:10.3390/cells9102253
Eto, S., Goto, M., Soga, M., Kaneko, Y., Uehara, Y., Mizuta, H., et al. (2018). Mesenchymal stem cells derived from human iPS cells via mesoderm and neuroepithelium have different features and therapeutic potentials. PLoS One 13 (7), e0200790. doi:10.1371/journal.pone.0200790
Ganguly, R., Metkari, S., and Bhartiya, D. (2018). Dynamics of bone marrow VSELs and HSCs in response to treatment with gonadotropin and steroid hormones, during pregnancy and evidence to support their asymmetric/symmetric cell divisions. Stem Cell Rev. Rep. 14 (1), 110–124. doi:10.1007/s12015-017-9781-x
Gluckman, E., Broxmeyer, H. E., Auerbach, A. D., Friedman, H. S., Douglas, G. W., Devergie, A., et al. (1989). Hematopoietic reconstitution in a patient with Fanconi's anemia by means of umbilical-cord blood from an HLA-identical sibling. N. Engl. J. Med. 321 (17), 1174–1178. doi:10.1056/NEJM198910263211707
Hade, M. D., Suire, C. N., and Suo, Z. (2021). Mesenchymal stem cell-derived exosomes: Applications in regenerative medicine. Cells 10 (8), 1959. doi:10.3390/cells10081959
Hansson, M. G., Helgesson, G., Wessman, R., and Jaenisch, R. (2007). Commentary: Isolated stem cells—patentable as cultural artifacts? Stem Cells 25 (6), 1507–1510. doi:10.1634/stemcells.2006-0684
Harvard Stem Cell Institute. (2022) What stem cell-based therapies are currently available? Available at: https://hsci.harvard.edu/faq/stem-cell-therapies.
Havens, A. M., Sun, H., Shiozawa, Y., Jung, Y., Wang, J., Mishra, A., et al. (2014). Human and murine very small embryonic-like cells represent multipotent tissue progenitors, in vitro and in vivo. Stem Cells Dev. 23 (7), 689–701. doi:10.1089/scd.2013.0362
Hénon, P., Kowalczyk, M., Aries, A., Vignon, C., Trébuchet, G., and Lahlil, R. (2022). Industrialized GMP production of CD34+ cells (ProtheraCytes®) at clinical scale for treatment of ischemic cardiac diseases is feasible and safe. Stem Cell Rev. Rep. 18 (5), 1614–1626. doi:10.1007/s12015-022-10373-5
Jovic, D., Yu, Y., Wang, D., Wang, K., Li, H., Xu, F., et al. (2022). A brief overview of global trends in MSC-based cell therapy. Stem Cell Rev. Rep. 18, 1525–1545. doi:10.1007/s12015-022-10369-1
Kaushik, A., and Bhartiya, D. (2022). Testicular cancer in mice: Interplay between stem cells and endocrine insults. Stem Cell Res. Ther. 13 (1), 243. doi:10.1186/s13287-022-02784-5
Kfoury, Y., and Scadden, D. T. (2015). Mesenchymal cell contributions to the stem cell niche. Cell stem Cell 16 (3), 239–253. doi:10.1016/j.stem.2015.02.019
Kim, Y., Jeong, J., Kang, H., Lim, J., Heo, J., Ratajczak, J., et al. (2014). The molecular nature of very small embryonic-like stem cells in adult tissues. Int. J. Stem Cells 7 (2), 55–62. doi:10.15283/ijsc.2014.7.2.55
Kucia, M., Reca, R., Campbell, F. R., Zuba-Surma, E., Majka, M., Ratajczak, J., et al. (2006). A population of very small embryonic-like (VSEL) CXCR4+ SSEA-1+ Oct-4+ stem cells identified in adult bone marrow. Leukemia 20 (5), 857–869. doi:10.1038/sj.leu.2404171
Lahlil, R., Scrofani, M., Barbet, R., Tancredi, C., Aries, A., and Hénon, P. (2018). VSELs maintain their pluripotency and competence to differentiate after enhanced ex vivo expansion. Stem Cell Rev. Rep. 14 (4), 510–524. doi:10.1007/s12015-018-9821-1
Leppik, L., Sielatycka, K., Henrich, D., Han, Z., Wang, H., Eischen-Loges, M. J., et al. (2020). Role of adult tissue-derived pluripotent stem cells in bone regeneration. Stem Cell Rev. Rep. 16 (1), 198–211. doi:10.1007/s12015-019-09943-x
Liu, T. M. (2021). Application of mesenchymal stem cells derived from human pluripotent stem cells in regenerative medicine. World J. Stem Cells 13 (12), 1826–1844. doi:10.4252/wjsc.v13.i12.1826
Mallapaty, S. (2022). Pioneering stem-cell trials in Japan report promising early results. Nature 609 (7926), 235. doi:10.1038/d41586-022-02232-7
Mierzejewska, K., Borkowska, S., Suszynska, E., Suszynska, M., Poniewierska-Baran, A., Maj, M., et al. (2015). Hematopoietic stem/progenitor cells express several functional sex hormone receptors—novel evidence for a potential developmental link between hematopoiesis and primordial germ cells. Stem Cells Dev. 24 (8), 927–937. doi:10.1089/scd.2014.0546
Mierzejewska, K., Heo, J., Kang, J. W., Kang, H., Ratajczak, J., Ratajczak, M. Z., et al. (2013). Genome-wide analysis of murine bone marrow-derived very small embryonic-like stem cells reveals that mitogenic growth factor signaling pathways play a crucial role in the quiescence and ageing of these cells. Int. J. Mol. Med. 32 (2), 281–290. doi:10.3892/ijmm.2013.1389
Moghadasi, S., Elveny, M., Rahman, H. S., Suksatan, W., Jalil, A. T., Abdelbasset, W. K., et al. (2021). A paradigm shift in cell-free approach: The emerging role of MSCs-derived exosomes in regenerative medicine. J. Transl. Med. 19 (1), 302–321. doi:10.1186/s12967-021-02980-6
Mohammad, S. A., Metkari, S., and Bhartiya, D. (2020). Mouse pancreas stem/progenitor cells get augmented by streptozotocin and regenerate diabetic pancreas after partial pancreatectomy. Stem Cell Rev. Rep. 16 (1), 144–158. doi:10.1007/s12015-019-09919-x
Monti, M., Imberti, B., Bianchi, N., Pezzotta, A., Morigi, M., Del Fante, C., et al. (2017b). A novel method for isolation of pluripotent stem cells from human umbilical cord blood. Stem Cells Dev. 26 (17), 1258–1269. doi:10.1089/scd.2017.0012
Monti, M., Imberti, B., Bianchi, N., Pezzotta, A., Morigi, M., Del Fante, C., et al. (2017a). A novel method for isolation of pluripotent stem cells from human umbilical cord blood. Stem Cells Dev. 26 (17), 1258–1269. doi:10.1089/scd.2017.0012
Monti, N., Verna, R., Piombarolo, A., Querqui, A., Bizzarri, M., and Fedeli, V. (2022). Paradoxical behavior of oncogenes undermines the somatic mutation theory. Biomolecules 12 (5), 662. doi:10.3390/biom12050662
Niikura, Y., Niikura, T., and Tilly, J. L. (2009). Aged mouse ovaries possess rare premeiotic germ cells that can generate oocytes following transplantation into a young host environment. Aging (Albany NY) 1 (12), 971–978. doi:10.18632/aging.100105
Ocampo, A., Reddy, P., Martinez-Redondo, P., Platero-Luengo, A., Hatanaka, F., Hishida, T., et al. (2016). In vivo amelioration of age-associated hallmarks by partial reprogramming. Cell 167 (7), 1719–1733. doi:10.1016/j.cell.2016.11.052
Patel, H., Bhartiya, D., Parte, S., Gunjal, P., Yedurkar, S., and Bhatt, M. (2013). Follicle stimulating hormone modulates ovarian stem cells through alternately spliced receptor variant FSH-R3. J. Ovarian Res. 6, 52. doi:10.1186/1757-2215-6-52
Rajasingh, S., Sigamani, V., Selvam, V., Gurusamy, N., Kirankumar, S., Vasanthan, J., et al. (2021). Comparative analysis of human induced pluripotent stem cell-derived mesenchymal stem cells and umbilical cord mesenchymal stem cells. J. Cell. Mol. Med. 25 (18), 8904–8919. doi:10.1111/jcmm.16851
Ratajczak, J., Wysoczynski, M., Zuba-Surma, E., Wan, W., Kucia, M., Yoder, M. C., et al. (2011). Adult murine bone marrow-derived very small embryonic-like stem cells differentiate into the hematopoietic lineage after coculture over OP9 stromal cells. Exp. Hematol. 39 (2), 225–237. doi:10.1016/j.exphem.2010.10.007
Ratajczak, J., Shin, D. M., Wan, W., Liu, R., Masternak, M. M., Piotrowska, K., et al. (2011a). Higher number of stem cells in the bone marrow of circulating low igf-1 level laron dwarf mice—Novel view on igf-1, stem cells and aging. Leukemia 25 (4), 729–733. doi:10.1038/leu.2010.314
Ratajczak, J., Wysoczynski, M., Zuba-Surma, E., Wan, W., Kucia, M., Yoder, M. C., et al. (2011b). Adult murine bone marrow-derived very small embryonic-like stem cells differentiate into the hematopoietic lineage after coculture over OP9 stromal cells. Exp. Hematol. 39 (2), 225–237. doi:10.1016/j.exphem.2010.10.007
Ratajczak, M., Gluckman, É., and Gale, R. P. (2022). Hal E. Broxmeyer PhD: Experimental haematologist and umbilical cord blood cell transplant pioneer (27 November, 1944–8 December, 2021). Stem Cell Rev. Rep. 57 (4), 1–2. doi:10.1007/s12015-021-10310-y
Ratajczak, M. Z., Bartke, A., and Darzynkiewicz, Z. (2017). Prolonged growth hormone/insulin/insulin-like growth factor nutrient response signaling pathway as a silent killer of stem cells and a culprit in aging. Stem Cell Rev. Rep. 13 (4), 443–453. doi:10.1007/s12015-017-9728-2
Ratajczak, M. Z., Ratajczak, J., and Kucia, M. (2019). Very small embryonic-like stem cells (VSELs). Circ. Res. 124 (2), 208–210. doi:10.1161/CIRCRESAHA.118.314287
Ratajczak, M. Z., Ratajczak, J., Suszynska, M., Miller, D. M., Kucia, M., and Shin, D. M. (2017). A novel view of the adult stem cell compartment from the perspective of a quiescent population of very small embryonic-like stem cells. Circ. Res. 120 (1), 166–178. doi:10.1161/CIRCRESAHA.116.309362
Ratajczak, M. Z., Shin, D. M., and Kucia, M. (2009). Very small embryonic/epiblast-like stem cells: A missing link to support the germ line hypothesis of cancer development? Am. J. Pathol. 174 (6), 1985–1992. doi:10.2353/ajpath.2009.081143
Ratajczak, M. Z., Shin, D. M., Schneider, G., Ratajczak, J., and Kucia, M. (2013). Parental imprinting regulates insulin-like growth factor signaling: A rosetta stone for understanding the biology of pluripotent stem cells, aging and cancerogenesis. Leukemia 27 (4), 773–779. doi:10.1038/leu.2012.322
Rezaie, J., Feghhi, M., and Etemadi, T. (2022). A review on exosomes application in clinical trials: Perspective, questions, and challenges. Cell Commun. Signal. 20 (1), 145. doi:10.1186/s12964-022-00959-4
Shaikh, A., Anand, S., Kapoor, S., Ganguly, R., and Bhartiya, D. (2017). Mouse bone marrow VSELs exhibit differentiation into three embryonic germ lineages and germ & hematopoietic cells in culture. Stem Cell Rev. Rep. 13 (2), 202–216. doi:10.1007/s12015-016-9714-0
Sharma, D., and Bhartiya, D. (2022). Dysfunctional ovarian stem cells due to neonatal endocrine disruption result in PCOS and ovarian insufficiency in adult mice. Stem Cell Rev. Rep. 18, 2912–2927. doi:10.1007/s12015-022-10414-z
Sharma, D., and Bhartiya, D. (2021a). Stem cells in adult mice ovaries form germ cell nests, undergo meiosis, neo-oogenesis and follicle assembly on regular basis during estrus cycle. Stem Cell Rev. Rep. 17 (5), 1695–1711. doi:10.1007/s12015-021-10237-4
Sharma, D., and Bhartiya, D. (2021b). Aged mice ovaries harbor stem cells and germ cell nests but fail to form follicles. J. Ovarian Res. 15 (1), 37–13. doi:10.1186/s13048-022-00968-4
Shin, D. M., Kucia, M., and Ratajczak, M. Z. (2011). Nuclear and chromatin reorganization during cell senescence and aging - a mini-review. Gerontology 57 (1), 76–84. doi:10.1159/000281882
Shin, D. M., Liu, R., Klich, I., Ratajczak, J., Kucia, M., and Ratajczak, M. Z. (2010). Molecular characterization of isolated from murine adult tissues very small embryonic/epiblast like stem cells (VSELs). Mol. Cells 29 (6), 533–538. doi:10.1007/s10059-010-0081-4
Shin, D. M., Liu, R., Wu, W., Waigel, S. J., Zacharias, W., Ratajczak, M. Z., et al. (2012). Global gene expression analysis of very small embryonic-like stem cells reveals that the Ezh2-dependent bivalent domain mechanism contributes to their pluripotent state. Stem Cells Dev. 21 (10), 1639–1652. doi:10.1089/scd.2011.0389
Shin, D. M., Zuba-Surma, E. K., Wu, W., Ratajczak, J., Wysoczynski, M., Ratajczak, M. Z., et al. (2009). Novel epigenetic mechanisms that control pluripotency and quiescence of adult bone marrow-derived Oct4+ very small embryonic-like stem cells. Leukemia 23 (11), 2042–2051. doi:10.1038/leu.2009.153
Shivdasani, R. A., Clevers, H., and de Sauvage, F. J. (2021). Tissue regeneration: Reserve or reverse? Science 371 (6531), 784–786. doi:10.1126/science.abb6848
Sikder, S., Arunkumar, G., Melters, D. P., and Dalal, Y. (2022). Breaking the aging epigenetic barrier. Front. Cell Dev. Biol. 10, 943519. doi:10.3389/fcell.2022.943519
Singh, P., Metkari, S., and Bhartiya, D. (2022). Additional evidence to support OCT-4 positive VSELs and EnSCs as the elusive tissue-resident stem/progenitor cells in adult mice uterus. Stem Cell Res. Ther. 13 (1), 60. doi:10.1186/s13287-022-02703-8
Singh, P., and Bhartiya, D. (2022). Molecular insights into endometrial cancer in mice. Stem Cell Rev. Rep. 18, 1702–1717. doi:10.1007/s12015-022-10367-3
Sonnenschein, C., and Soto, A. M. (2020). Over a century of cancer research: Inconvenient truths and promising leads. PLoS Biol. 18 (4), e3000670. doi:10.1371/journal.pbio.3000670
Sonoda, Y. (2021). Human CD34-negative hematopoietic stem cells: The current understanding of their biological nature. Exp. Hematol. 96, 13–26. doi:10.1016/j.exphem.2021.02.004
Squillaro, T., Peluso, G., and Galderisi, U. (2016). Clinical trials with mesenchymal stem cells: An update. Cell Transpl. 25 (5), 829–848. doi:10.3727/096368915X689622
Taichman, R. S., Wang, Z., Shiozawa, Y., Jung, Y., Song, J., Balduino, A., et al. (2010). Prospective identification and skeletal localization of cells capable of multilineage differentiation in vivo. Stem Cells Dev. 19 (10), 1557–1570. doi:10.1089/scd.2009.0445
Tian, C., He, J., An, Y., Yang, Z., Yan, D., Pan, H., et al. (2021). Bone marrow mesenchymal stem cells derived from juvenile macaques reversed ovarian ageing in elderly macaques. Stem Cell Res. Ther. 12 (1), 460. doi:10.1186/s13287-021-02486-4
Virant-Klun, I., Skutella, T., Kubista, M., Vogler, A., Sinkovec, J., and Meden-Vrtovec, H. (2013). Expression of pluripotency and oocyte-related genes in single putative stem cells from human adult ovarian surface epithelium cultured in vitro in the presence of follicular fluid. Biomed. Res. Int. 2013, 861460. doi:10.1155/2013/861460
Virant-Klun, I. (2018). Functional testing of primitive oocyte-like cells developed in ovarian surface epithelium cell culture from small VSEL-like stem cells: Can they be fertilized one day? Stem Cell Rev. Rep. 14 (5), 715–721. doi:10.1007/s12015-018-9832-y
Wakao, S., Kitada, M., Kuroda, Y., Shigemoto, T., Matsuse, D., Akashi, H., et al. (2011). Multilineage-differentiating stress-enduring (MUSE) cells are a primary source of induced pluripotent stem cells in human fibroblasts. Proc. Natl. Acad. Sci. U. S. A. 108 (24), 9875–9880. doi:10.1073/pnas.1100816108
Wu, J., Liu, Y., Song, Y., Wang, L., Ai, J., and Li, K. (2022). Aging conundrum: A perspective for ovarian aging. Front. Endocrinol. 13, 952471. doi:10.3389/fendo.2022.952471
Xu, M., Shaw, G., Murphy, M., and Barry, F. (2019). Induced pluripotent stem cell-derived mesenchymal stromal cells are functionally and genetically different from bone marrow-derived mesenchymal stromal cells. Stem cells 37 (6), 754–765. doi:10.1002/stem.2993
Yamanaka, S. (2020). Pluripotent stem cell-based cell therapy—Promise and challenges. Cell stem Cell 27 (4), 523–531. doi:10.1016/j.stem.2020.09.014
Zhang, B., Tian, X., Hao, J., Xu, G., and Zhang, W. (2020). Mesenchymal stem cell-derived extracellular vesicles in tissue regeneration. Cell Transpl. 29, 0963689720908500. doi:10.1177/0963689720908500
Keywords: stem cells, regeneration, cancer, iPS cells, VSELs
Citation: Bhartiya D, Jha N, Tripathi A and Tripathi A (2023) Very small embryonic-like stem cells have the potential to win the three-front war on tissue damage, cancer, and aging. Front. Cell Dev. Biol. 10:1061022. doi: 10.3389/fcell.2022.1061022
Received: 04 October 2022; Accepted: 14 November 2022;
Published: 04 January 2023.
Edited by:
Rachid Lahlil, Institut de Recherche en Hématologie et Transplantation, FranceReviewed by:
Manuela Monti, University of Pavia, ItalyDong-Myung Shin, University of Ulsan, South Korea
Copyright © 2023 Bhartiya, Jha, Tripathi and Tripathi. This is an open-access article distributed under the terms of the Creative Commons Attribution License (CC BY). The use, distribution or reproduction in other forums is permitted, provided the original author(s) and the copyright owner(s) are credited and that the original publication in this journal is cited, in accordance with accepted academic practice. No use, distribution or reproduction is permitted which does not comply with these terms.
*Correspondence: Deepa Bhartiya, deepa.bhartiya@epigeneres.com