- 1Department of Gastrointestinal Nutrition and Hernia Surgery, The Second Hospital of Jilin University, Changchun, Jilin, China
- 2Department of Surgical Oncology and General Surgery, The First Affiliated Hospital of China Medical University, Shenyang, Liaoning, China
Focal adhesion kinase (FAK) is a non-receptor tyrosine kinase and an adaptor protein that primarily regulates adhesion signaling and cell migration. FAK promotes cell survival in response to stress. Increasing evidence has shown that at the pathological level, FAK is highly expressed in multiple tumors in several systems (including lung, liver, gastric, and colorectal cancers) and correlates with tumor aggressiveness and patient prognosis. At the molecular level, FAK promotes tumor progression mainly by altering survival signals, invasive capacity, epithelial-mesenchymal transition, the tumor microenvironment, the Warburg effect, and stemness of tumor cells. Many effective drugs have been developed based on the comprehensive role of FAK in tumor cells. In addition, its potential as a tumor marker cannot be ignored. Here, we discuss the pathological and pre-clinical evidence of the role of FAK in cancer development; we hope that these findings will assist in FAK-based clinical studies.
1 Introduction
Focal adhesion kinase (FAK) is a multifunctional tyrosine kinase protein encoded by PTK2 (or FAK) that is overexpressed in tumor cells associated with adverse clinical outcomes (Zhou et al., 2018). As a non-receptor cytoplasmic tyrosine kinase and scaffolding protein located in the adhesive plaque, FAK mediates and integrates signals initiated by growth factor, integrin, vascular endothelial growth factor receptor (VEGFR), and G protein-coupled receptor. This activates downstream signals (such as PI3K, Akt, and MAPK) and regulates intracellular functions (Devaud et al., 2019; Fan et al., 2019). Moreover, growing evidence has revealed that FAK is involved in the regulation of multiple tumorigenic processes, including growth factor signaling, cell cycle progression, cell survival, migration, metastasis, angiogenesis, and the establishment of an immunosuppressive tumor microenvironment (TME) through kinase-dependent and independent scaffolding functions in the cytosol and nucleus (Haskell et al., 2003; Kobayashi et al., 2009; Osipov et al., 2019).
2 Characteristics of the focal adhesion kinase molecule
The human gene encoding FAK (also known as PTK2) is located on chromosome 8q24.3, a region shown to have frequent aberrations in human oncology (Pylayeva et al., 2009; Schaller, 2010). The coding sequence of FAK, a highly conserved 125 kDa non-receptor tyrosine kinase, contains 34 exons (Corsi et al., 2006). FAK consists of an amino-terminal region containing a 4.1-Ezrin-Radixin-moesin (FERM) structural domain, a central kinase structural domain, and a carboxy-terminal focal adhesion targeting (FAT) structural domain (Alanko and Ivaska, 2016). Three proline-rich regions (PRRs) are anchored to the linkage region between these structural domains. Phosphorylation occurs at several important tyrosine residues, including the autophosphorylation site Tyr397, Tyr576/577 in the activation loop of the kinase structural domain, and Tyr861, Tyr925, and Tyr1007 in the C-terminal structural domain (Wu et al., 2022). It is well known that both the N- and C-terminal structural domains mediate the interaction of FAK with other proteins essential for activating its kinase structural domain and regulating different cellular functions. FAK is maintained in an inactive state through the binding of the FERM structural domain to the kinase structural domain, which prevents access to the critical autophosphorylation site tyrosine 397 (Tyr397) (Frame et al., 2010). After binding to the extracellular matrix or growth factors, integrins stimulate G protein-linked receptors, leading to a signaling substitution of the FERM structural domain. This results in Tyr397 autophosphorylation, conformational changes in FAK and/or its binding partners, and binding and/or regulation of downstream effector molecules (such as Src, MAPK, PI3K, paxlin, and Rac) (Frame et al., 2010). The C-terminal structural domain provides binding sites for proteins, such as p130Cas and VEGFR3 (Frame et al., 2010). It includes the FAT sequence, which is responsible for FAK localization to focal adhesions and facilitates its co-localization with integrins by interacting with integrin-related proteins. The lipid domain is also associated with several Rho GTPases, such as p190RhoGF (Aboubakar Nana et al., 2019a) (Figure 1).
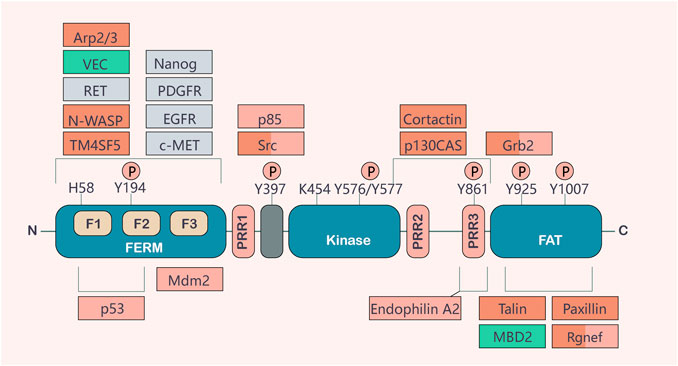
FIGURE 1. Basic structure and function of FAK. FAK consists of a central activation region and a protein band [4.1-Ezrin-Radioxin-moesin (FERM)] homologous structural domain located at the amino terminus and a carboxy-terminal focal adhesion targeting (FAT) structural domain. These two terminal structural domains are separated from the activation domain by a linker region containing proline-rich regions (PRRs). Important tyrosine phosphorylation (P) sites include Y397, K454, and H58, which play key roles in FAK activation. FAK binding partners are shown at their sites of interaction in FAK. The binding of different partners affects functions, such as cell motility (red), cell survival (orange), or both (red/orange). Actors involved in FAK activation are shown in gray, and important contributions to the tumor environment are shown in green (Sulzmaier et al., 2014).
3 Focal adhesion kinase regulates tumor development and progression
FAK expression and activation are regulated by several mechanisms: at the gene level by gene amplification (Agochiya et al., 1999; Okamoto et al., 2003); at the RNA level by selective splicing (Corsi et al., 2006; Devaud et al., 2019) or FAK mRNA upregulation (Tremblay et al., 1996; Fujii et al., 2004); at the translational and post-translational levels via phosphorylation (Imaizumi et al., 1997), dephosphorylation (Hauck et al., 2001); and non-coding RNA regulation (Egawa et al., 2016; Cheng et al., 2017; Qu et al., 2017; Wang et al., 2019; Yan et al., 2019; Pan and Xie, 2020; Tang et al., 2020; Zhang et al., 2021a). FAK plays an integral role in the development of various tumors through these mechanisms.
Multiple methods, including western blotting (WB), quantitative real-time polymerase chain reaction (qPCR), and immunohistochemistry (IHC), have shown increased FAK expression or enhanced activity in many human cancers, including lung (Zhou et al., 2018; Aboubakar Nana et al., 2019b), head and neck (Zhang and Sun, 2020), oral cavity (Kato et al., 2020), thyroid (Ignjatović et al., 2021), breast (Roy-Luzarraga et al., 2020), ovarian (Li et al., 2015), prostate (Goto et al., 2020), colon (Murata et al., 2008), liver (Francalanci et al., 2020), stomach (Luo et al., 2020), pancreatic (Furuyama et al., 2006), kidney (Béraud et al., 2015), skin (Najjar et al., 2020), and bone (Thanapprapasr et al., 2017; Gu and Zhou, 2018) cancer. In addition, an increased expression or activity of FAK has been reported in various cancer cell lines (Aboubakar Nana et al., 2019c). Here, we selected several representative cancers to investigate the tumor effects of FAK (Figure 2; Table 1).
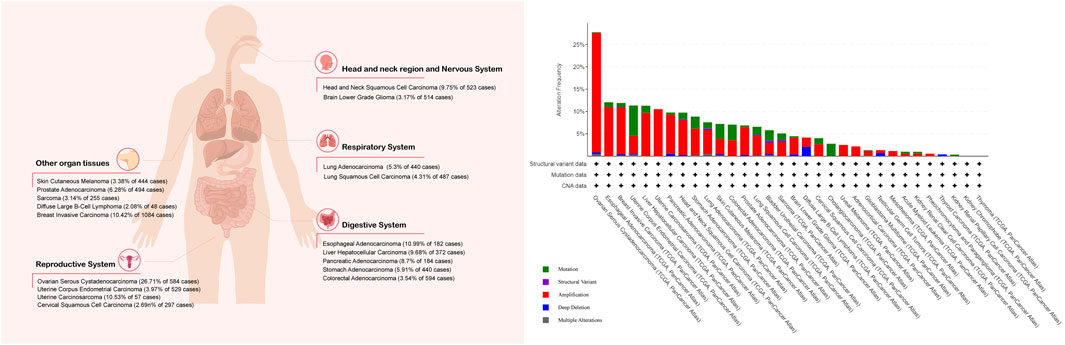
FIGURE 2. Percentage of tumor samples with increased FAK mRNA. The Cancer Genome Atlas was queried using the cBioPortal (cBioPortal for Cancer Genomics: PTK2 in Adrenocortical Carcinoma (TCGA, PanCancer Atlas) and 31 other studies). The search criteria included mRNA expression data (Z-scores for all genes) and tumor datasets with mRNA data.
3.1 The molecular mechanisms of focal adhesion kinase aberrant expression and activation in tumors
3.1.1 Control of focal adhesion kinase expression
Overexpression of FAK transcripts is crucial for FAK-mediated tumor cell function. The PTK2 promoter is also activated or made more active by the transcription factors NF-κB (Corsi et al., 2006), BACH1 (Xie et al., 2022), ETV1 (Zhang et al., 2022), ETS1 (Tomar et al., 2018), NANOG (Ho et al., 2012), AGO2 (Cheng et al., 2013), and ETV4 (Li et al., 2013), which similarly increases the expression of FAK mRNA. In contrast, P53 (Cance and Golubovskaya, 2008) and ATF3 (Tian et al., 2021) limit some of the tumor’s functions by lowering the activity of the PTK2 promoter and the number of transcripts. By directly increasing PTK2 and IGF1R in hepatocellular carcinoma cells, BACH1 speeds up the development and spread of Hepatocellular carcinoma (HCC) (Xie et al., 2022). Additionally, HCC patients with ETV1/PTK2 or ETV1/c-MET co-positive hepatocellular carcinoma in two different cohorts had a worse prognosis. ETV1 can enhance HCC metastasis in HCC by upregulating PTK2 and MET (Zhang et al., 2022). ETS1, a crucial transcription factor produced by the microenvironment in ovarian cancer cells, predicts a poor prognosis and targets PTK2 while promoting graft colonization by increasing FAK transcript levels (Tomar et al., 2018). In colon cancer cells, NANOG increases FAK expression, and FAK’s phosphorylation is a component of the signaling loop that increases NANOG activity (Ho et al., 2012). AGO2 is a component of the cellular RNA interference apparatus that is increased in hepatocellular carcinoma and stimulates FAK transcription (Cheng et al., 2013). In mice, inhibiting AGO2 lowers FAK levels while preventing tumor development and metastasis. ETV4 induced FAK expression in vitro, again considering its role as a transcription factor affecting PTK2 promoter activity (Li et al., 2013). ATF3 is a downstream transcription factor of ROS, and increased levels of ATF3 can reduce the transcriptional level of FAK, reducing prostate cancer cells’ invasiveness (Tian et al., 2021). In non-coding RNA studies, CircC16orf62 was found to act as a molecular sponge for miR-138-5p and a competitive endogenous RNA for PTK2, which promotes the activation of the downstream AKT/mTOR pathway (Zhang et al., 2021b). Hypomethylation of the FAK promoter region was also associated with the high expression of FAK in HCC (Fan et al., 2019).
Selective splicing of mRNA (Alternative Splicing, AS) enhances the fine-tuning of protein function. By generating from an initial unique pre-messenger RNA, different protein isoforms varying in expression, subcellular localization, interactions and activities, AS represents a critical player in protein function regulation in development, physiology and disease (Kelemen et al., 2013). It was found that FAK mRNA showed three different alternative splice variants in colorectal cancer, namely FAK0, FAK28, and FAK6, and was associated with the invasive ability of colorectal cancer (Devaud et al., 2019). In papillary thyroid carcinoma, the number of FAK33 variants was elevated and positively correlated with total FAK transcripts and pY397-FAK protein levels, as well as with the advanced features of papillary thyroid carcinoma (Ignjatović et al., 2022). After comparing breast cancer tissues with normal tissues, FAK26 was a spliceosome expressed only in breast cancer tissues and allowed FAK proteins to acquire resistance to caspase-mediated cleavage (Yao et al., 2014). For this AS, it has been demonstrated that circRPAP2 may attenuate the selective splicing of PTK2 by competing with PTK2 pre-mRNA for binding to the RRM1 structural domain of SRSF1 (Yu and Fang, 2022).
3.1.2 Regulation of focal adhesion kinase activity
FAK activation is mainly controlled by FAK dimerization, intramolecular inhibition of the FERM structural domain, FAK phosphorylation and other mechanisms. The most typical mechanism that promotes FAK activation involves the aggregation of integrin receptors upon cell binding to extracellular matrix (ECM) proteins, a process that involves FAK dimerization. The dimerization is formed by binding of the n-terminal FERM structural domain of FAK and is stabilized by the interaction of the FERM and c-terminal FAT structural domains. FAT binds to the basic motif on FERM that regulates coactivation and nuclear localization (Brami‐Cherrier et al., 2014). This leads to autophosphorylation of FAK at the Y397 site, binding of Src family kinases to the phosphorylation site, and mediates phosphorylation of the FAK kinase structural domain activation loop to form an activated FAK - Src complex (Lietha et al., 2007). In addition to Src, RET can also phosphorylate residues of Tyr576 and Tyr577 to activate FAK (Plaza-Menacho et al., 2011). Experiments using fluorescent biosensors have shown that when ECM binds or interacts with phosphatidylinositol lipids, the FERM structural domain undergoes conformational changes that unwind the self-inhibitory interactions (Goñi et al., 2014). Enhancing the stiffness or tension associated with cell- ECM interactions by strengthening integrin signaling has also been shown to promote FAK activation (Bauer et al., 2019), which is essential not only for mechanotransduction but also critical for tumor progression. In addition to binding partners to accelerate conformational changes in the FERM structural domain, growth factor receptors, such as MET, epidermal growth factor receptor (EGFR) and platelet-derived growth factor receptor (PDGFR), can also phosphorylate Tyr194 to relieve self-inhibition and induce FAK activation (Chen et al., 2011). In addition, Tyr397 phosphorylation is also associated with FAK activity. SHP2 is responsible for the dephosphorylation of pTyr397 and inhibits FAK activity (von Wichert et al., 2003). Phosphorylation-dependent isomerization of protein tyrosine phosphatase (PTP)-PEST promotes the interaction of PTP-PEST with FAK and the dephosphorylation of the Tyr397 site on FAK, leading to FAK inactivation (Zheng et al., 2011). SHP2 and PTP-PEST synergistically control FAK activity with Src and promote the kinetics of focal adhesion complexes, thereby facilitating cell motility (Wu et al., 2015; Chuang et al., 2021).
3.2 Effect of focal adhesion kinase on tumor progression
3.2.1 Lung cancer
Lung cancer is a malignant tumor with high morbidity and mortality rates. As early as 1996, phosphorylated FAK was shown to be a significant component of 100–130 kDa phosphorylated proteins in lung surgery specimens and was associated with poor patient prognosis (Nishimura et al., 1996). Increased FAK phosphorylation is strongly associated with lymph node metastasis and disease-free survival in tumors (Imaizumi et al., 1997). Smoking is an important environmental factor in lung cancer, and a recent study confirmed that smoking activates the c-Src/FAK pathway (Stading et al., 2021), subsequently promoting lung carcinogenesis and progression (Zhou et al., 2019), drug resistance (Kang et al., 2013), and maintenance of KRAS-driven lung adenocarcinoma (Zhou et al., 2018). This provides ample evidence that the role of FAK in lung cancer cannot be ignored.
Lung cancer is pathologically divided into small cell lung cancer (SCLC) and non-small cell lung cancer (NSCLC). SCLC accounts for approximately 10% and has a poorer prognosis than NSCLC. Recent studies on the differences in FAK and p-FAK expression in SCLC and NSCLC have shown that the staining scores of FAK and p-FAK were significantly higher in lung cancer and SCLC tissues than in normal lung and NSCLC tissues (Aboubakar Nana et al., 2019b). There are many subtypes of NSCLC, such as lung squamous carcinoma, lung adenocarcinoma, and large cell lung cancer. FAK overexpression in NSCLC was associated with the stage as well as the adenocarcinoma subtype and positively correlated with lymph node metastasis (Ji et al., 2013). Whether there is a link between FAK expression and NSCLC prognosis is unclear and may be ethnically relevant (Ji et al., 2013; Dy et al., 2014; Aboubakar Nana et al., 2019b).
Although FAK appears to be more relevant in SCLC, most in vitro experiments have been conducted on NSCLC. Consistent with these pathological features, Fu et al. (2015) found that in NSCLC cells, ENO1 could enhance the proliferation, migration, invasion, epithelial-mesenchymal transition (EMT), and glycolytic capacity of tumor cells by activating the FAK/PI3K/AKT pathway. Moreover, depletion of FAK using siRNA inhibited the phosphorylation of molecules such as Src, ERK1/2, PI3K, and Akt (Fu et al., 2015). Additionally, Wang et al. (2020a) found that secretory PKM2 directly binds to integrin β1 and activates the FAK/SRC/ERK axis to promote lung cancer metastasis. Fu et al. (2020) also found that secretory OPN leads to acquired epidermal growth factor receptor tyrosine kinase inhibitor (EGFR-TKI) resistance by activating the integrin αVβ3/FAK pathway, which provides novel insights for the application of FAK inhibitors in lung cancer treatment.
3.2.2 Liver cancer
HCC is a prevalent disease with high morbidity and mortality rates. A study of FAK overexpression in 64 HCC tissues undergoing hepatectomy without pre-operative treatment showed that FAK expression was correlated with the clinicopathological features of HCC and was strongly upregulated in HCC compared with that in primary lesions and portal vein invasion (Itoh et al., 2004). Chen et al. (2010) reached the same conclusion and found that overexpression of FAK and its phosphorylated form in HCC tissues was associated with tumor stage, vascular invasion, and intrahepatic metastasis. The same phenomenon has been observed in human hepatoblastoma tissues (Gillory et al., 2013). In addition, several studies have demonstrated that FAK mRNA and protein expression levels are independent prognostic factors that affect disease-free survival and overall survival of patients with HCC (Fujii et al., 2004; Fan et al., 2019).
SiRNA-mediated inhibition of FAK expression in HCC cell lines revealed that the growth and apoptosis of HCC cell lines were not affected, but their adhesion and invasion abilities were reduced to different degrees (Chen et al., 2010). The FAK-ERK1/2 signaling pathway in HCC may play a vital role in reducing the stiffness of HCC stem cells and enhancing the invasive ability of HCC. These effects can be inhibited by FAK inhibitors (Sun et al., 2017; Sun et al., 2018a). Collagen is an essential component of the TME. The collagen type IV alpha1 chain (COL4A1) is known to be highly expressed in HCC and promotes the growth and metastasis of HCC by activating the FAK/Src pathway (Wang et al., 2020b). FAK is also a driver of cholangiocarcinogenesis, and in vivo experiments have shown that ablation of FAK significantly delayed the initiation of Akt/YAP-driven intrahepatic cholangiocarcinoma (iCCA) in mice. Additionally, growth was reduced considerably when FAK inhibitors and palbociclib (a CDK4/6 inhibitor) were administered simultaneously to mice (Song et al., 2021).
3.2.3 Gastric cancer
Gastric cancer is the third leading cause of cancer-related deaths worldwide (Ferlay et al., 2015). FAK is overexpressed in half of gastric cancer cases (Tani et al., 1996; Su et al., 2002; Luo et al., 2020). The same applies to the level of FAK expression in pathological specimens of patients with gastric cancer, which is positively correlated with the size, pathological stage (Luo et al., 2020), depth of infiltration, lymph node metastasis, and venous invasion of the patient’s tumor (Park et al., 2010).
Integrins also play a role in the cancer-promoting effects of FAK in gastric cancer. Annexin A6, transported in the extracellular vesicles of cancer-associated fibroblasts (CAFs), promotes drug resistance in a mouse metastatic tumor model by mediating the activation of FAK/YAP pathway in cancer cells via integrin β1 (Uchihara et al., 2020). In addition to drug resistance, integrin β1/FAK/YAP can mediate gastric cancer metastasis (Xiang et al., 2018). Extracellular matrix protein 1 (ECM1) mediates the activation of the FAK/SOX/HIF-1α axis by directly interacting with integrin β4 to increase metastasis and aerobic glycolysis in gastric cancer cells (Gan et al., 2018). Similarly, integrin α5β1 promotes the migration of gastric cancer cells through the FAK/ERK1 pathway (Yao et al., 2020). FAK/Akt/mTOR also seems to be the focus of research in gastric cancer, and many molecules are involved in this pathway, promoting gastric cancer growth and migration (Wu et al., 2019; Wu et al., 2021a; Qiao et al., 2021).
3.2.4 Endometrial carcinomas
Estrogen-dependent endometrial carcinomas express only low levels of FAK, whereas non-dependent endometrial carcinomas show FAK overexpression; p-FAK has the same expression pattern (Zhou et al., 2013a). A synergistic study on the overexpression of EZH2, FAK, and p-FAK found that all of them were positively associated with a high histological grade, type II tumors, vascular lymphatic invasion, lymph node metastasis, myometrial invasion, and cervical involvement. Contrariwise, only p-FAK overexpression was associated with omental metastasis (Zhou et al., 2013a). A study of FAK and PTEN at the pathological level also showed a positive correlation between their expression (Zhou et al., 2013b). Similarly, the expression of various molecules (such as AFP and EZH2) was found to show a synergistic increase with FAK expression in different tumor tissues (Fujii et al., 2004). This indicates, to some extent, the role of FAK in tumor development and its potential as a tumor marker. Studies on a variety of tumor tissues have revealed that the expression of many molecules such as cholecystokinin-2 receptor (Yu et al., 2006), adrenomedullin (Li et al., 2015), HER-2/neu (Lark et al., 2005), p-Src (Schmitz et al., 2005), p-Akt (Schmitz et al., 2005), PYK2 (Gutenberg et al., 2004), p120RasGAP (Hecker et al., 2004), adenosine diphosphate ribosylation factor guanylate kinase 1 (Luo et al., 2020), AFP (Fujii et al., 2004), EZH2 (Fujii et al., 2004), H3K27me3 (Francalanci et al., 2020), EGFR (Šelemetjev et al., 2018), PTEN (Zhou et al., 2013b), and pyk2 (Gutenberg et al., 2004) shows a synergistic increase with FAK expression. These molecules affect tumor development to varying degrees by interacting upstream and downstream of FAK.
3.2.5 Breast cancer
As one of the most common malignant diseases among women, breast cancer also displays a high degree of diversity in terms of pathological characteristics, disease progression, and response to treatment. Numerous studies have shown that FAK is downregulated in benign breast epithelium and moderately or strongly expressed in most malignant breast tissue (Weiner et al., 1993; Cance et al., 2000; Watermann et al., 2005; Almstedt et al., 2017). In particular, the high expression of FAK in early metastatic tissues suggests that it plays an important role in breast cancer metastasis (Lightfoot et al., 2004). FAK expression in breast cancer is associated with sex hormone levels. This may be related to the estrogen-related G protein-coupled receptors (Rigiracciolo et al., 2019a). High FAK expression is associated with a high histological grade, high T-stage, estrogen receptor-negative expression, progesterone receptor-negative expression, and triple-negative phenotype (Schmitz et al., 2005; Yom et al., 2011; Rigiracciolo et al., 2019a). Additionally, high FAK expression in primary foci correlates with younger patient age and lymphovascular invasion (Golubovskaya et al., 2014). Furthermore, high FAK expression is significantly and positively correlated with shorter overall survival and progression-free survival in patients with metastatic tumors (Golubovskaya et al., 2014). However, in a study of 162 lymph node-negative breast cancer tissues, FAK expression showed no prognostic significance (Schmitz et al., 2005). FAK has been suggested to play a significant role in breast cancer metastasis and affects the survival of patients with metastatic tumors.
The heterogeneity of triple-negative breast cancer with FAK-related mechanisms is possibly mediated by GPER, CTGF, and Gpx1 (Rigiracciolo et al., 2019b; Lee et al., 2020; Kim et al., 2021). Extracellular CTGF directly binds integrin αvβ3 and activates the FAK/Src/NF-κB p65 signaling axis, leading to the upregulation of Glut3 transcription, through which the glycolytic and migratory capacities of breast cancer cells are enhanced (Kim et al., 2021). Gpx1, a redox protective factor for FAK kinase, prevents kinase inactivation via H2O2, whereas Gpx1 deletion downregulates FAK/c-Src activation, thus preventing the spread and metastasis of tumor cells (Lee et al., 2020). Likewise, the role of FAK in the TME of breast cancer is an important research direction (Wu et al., 2020; Wang et al., 2021). Analysis of CAFs from knockout mice revealed that miR-16 and miR-148a help mediate FAK activity to enhance tumor cell activity and metastasis (Wu et al., 2020). In co-cultures of breast cancer cells and monocytes, breast cancer cells secrete CSF1 and induce monocytes to express and release CXCL7, which in turn acts on cancer cells to promote FAK activation, MMP13 expression, migration, and invasion. In a xenograft mouse model, administration of the CXCL7 antibody significantly reduced the abundance of M2 macrophages in the TME and reduced tumor growth and distant metastasis (Wang et al., 2021).
4 Mechanism by which focal adhesion kinase regulates tumor progression
Tumor development and metastasis are complex processes that involve tumor cell shedding, invasion, migration, vascularity, and proliferation in distal parts of the body. Signaling pathways promote tumor progression and growth by regulating cell adhesion, invasion, and migration. Numerous studies on the signaling pathways between FAK and several types of cancers have revealed the biological mechanisms by which FAK promotes cancer development. This also corroborates the link between FAK overexpression and its molecular role at the pathological level (Figure 3).
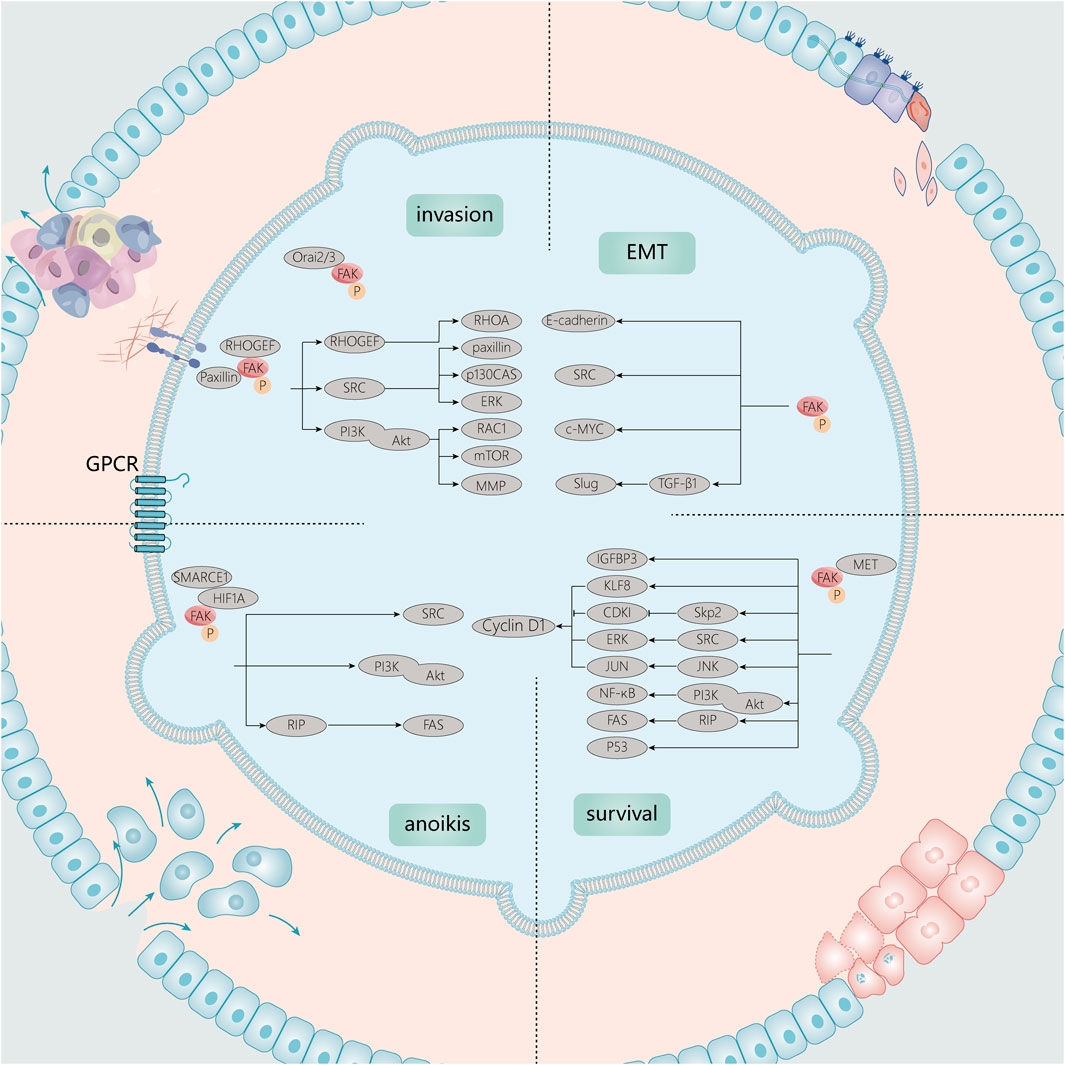
FIGURE 3. Signaling molecules associated with FAK in tumor growth and invasion. Tumor development and metastasis are complex processes. In growth signaling, FAK is closely linked to anoikis, cell cycle, and apoptotic processes. In EMT and invasion, FAK can also function through associated Src, PI3K/Akt, and other signaling pathways.
4.1 Focal adhesion kinase and tumor survival signaling
The disorderly proliferation of malignant tumors is the major pathway of tumor progression. It is influenced by a variety of factors both inside and outside the cell. In addition, the ability of tumors to resist death, including apoptosis and anoikis, is a key aspect of tumor survival and progression.
4.1.1 Cell cycle
The role of FAK in cell cycle progression has been reported previously. The gene encoding cyclin D1, a key regulator of G1/S phase progression, is the major target of FAK action in cell cycle control. Zhao et al. (1998) and Zhao et al. (2001) found that FAK could regulate cyclin D1 gene expression mediated by the ERK1/2 pathway at the EtsB-binding site (Njei et al., 2015). Expression of the autophosphorylation site-mutated FAK molecule (FAK-397F) in glioblastoma cells leads to exit from the G1 phase by decreasing the expression of cyclinD1 and E and enhancing the expression of p27 (Kip1) and p21 (Waf1) (Ding et al., 2005). In particular, in a mouse model, Marta et al. found that intranuclear FAK regulation, which is dependent on IGFBP3 transcription, regulates squamous cell carcinoma cell cycle progression and tumor growth in vivo and that FAK interacts with many RUNX1 regulatory proteins (Canel et al., 2017). Moreover, Zhang et al. (2019) found that MET/FAK signaling enables CDK4/6 non-dependent CDK2 activation, which leads to cell cycle delivery. Furthermore, they found that the inhibition of CDK4/6 and MET/FAK can synergistically alter the fate of tumor cells.
4.1.2 Apoptosis
FAK is associated with apoptosis in cancer cells. First, FAK inhibition can lead to the loss of adhesion and apoptosis of tumor cells, which has been confirmed at an early stage (Xu et al., 1996; Xu et al., 2000). Sonoda et al. (2000) demonstrated that FAK induces IAPs by activating the PI3K/Akt pathway along with NF-κB. This ultimately inhibits apoptosis by inhibiting the caspase-3 cascade. RIP, a major component of the death receptor complex, mediates apoptosis by interacting with Fas and tumor necrosis factor receptor 1 by binding to junctional proteins. The pro-apoptotic signal provided by RIP is inhibited by its binding to FAK (Kurenova et al., 2004). In addition, the FERM structural domain of nuclear FAK interacts with the N-terminal structural domain of wild-type p53 and MDM-2 to promote the degradation of p53, thereby preventing apoptosis (Lim et al., 2008; Golubovskaya and Cance, 2011).
4.1.3 Anti-anoikis
One reason for the crucial role of FAK in promoting tumor proliferation is that FAK can promote cell survival in suspension, also known as anoikis apoptosis resistance, first identified by Frisch et al. (1996). In the death receptor-mediated mechanism of anoikis, the dissociation of FAK and receptor-interacting protein (RIP) leads to the binding of RIP to FAS. This forms a death-inducing signaling complex (DISC) that activates caspase-3. Indeed, activation of the FAK/Src complex is focused on the upregulation of signaling cascades (including PI3K-Akt, ERK1/2, and other mitogen-activated protein kinases) which maintain cell survival by promoting the resistance of isolated cells to “anoikis”. In addition, the combination of FAK and RIP enhances anoikis resistance by inhibiting the binding of RIP to Fas and the formation of the death signaling complex, which allows cells to escape anoikis.
4.2 Focal adhesion kinase, epithelial-mesenchymal transition and invasion
Tumor cell invasion into the surrounding microenvironment is a critical step in cancer progression, allowing cancer cells to metastasize to secondary locations. This requires a shift to a motor phenotype by altering focal adhesion and cytoskeletal dynamics as well as altering matrix metalloproteinase (MMP) expression or activating to promote extracellular matrix (ECM) invasion (Weiss et al., 2022).
FAK mediates cell invasion and metastasis by promoting EMT (Canel et al., 2013; Frisch et al., 2013), in which E-cadherin plays a pivotal role as FAK mediates changes in E-cadherin expression (Avizienyte et al., 2002a; Canel et al., 2010; Serrels et al., 2011; Gayrard et al., 2018). Furthermore, SRC-FAK-dependent actomyosin remodeling relaxes E-cadherin without causing β-linked protein dissociation (Gayrard et al., 2018). FAK phosphorylation is required for Src-induced E-cadherin downregulation in colon cancer cells (Avizienyte et al., 2002b), and inhibition of FAK activity reduces Src-mediated cell invasion and blocks metastasis of FAK drug-targeted invasion and metastasis (Hauck et al., 2002). In addition, the knockdown of KIF26A increases the binding of c-MYC to the FAK promoter region and decreases the expression of E-cadherin, consequently promoting EMT (Ma et al., 2021). In parallel to E-cadherin-mediated EMT, TGF-β1-induced Slug expression also modulates EMT and promotes cell migration in human squamous cell carcinoma cells; this effect can be inhibited by FAK inhibitors (Saito et al., 2013). Accordingly, FAK plays a significant role in EMT, invasion, and metastasis. In contrast, the downstream molecular mechanisms of FAK-regulated EMT with E-cadherin-mediated cell-cell adhesion or integrin-ECM-mediated adhesion and their interactions and roles in metastasis remain to be investigated.
Invasion-associated cellular activities depend on branching networks of signal transduction pathways, including the activation of trimeric G proteins, phosphoinositide 3-kinase (PI3K), Src, signal transducer and activator of transcription, and the Rab, Rac, and Rho family of small GTPases. The heterotrimeric G protein, Gαq, activates FAK. This subsequently regulates YAP through tyrosine phosphorylation of MOB1 and inhibits core Hippo signaling (Feng et al., 2019). G-protein-coupled estrogen receptor (GPER) signaling triggers phosphorylation of Y397-FAK and an increase in adherent patches (FAs) in TNBC cells, and FAK inhibition prevents the invasion of TNBC cells upon GPER activation (Rigiracciolo et al., 2019a). Numerous reports show that FAK enhances tumor invasion through PI3K/AKT (Fu et al., 2015; Wu et al., 2019) and Src (Dong et al., 2021) signaling. In studies of melanoma invasion, STK11 was found to inhibit the invasion process of cutaneous melanoma through signal transducer and activator of transcription 3/5 and FAK repression (Dzung et al., 2022). In addition, the Rab (Choe et al., 2018; Xu et al., 2021), Rac (Acevedo-Díaz et al., 2019), and Rho (Tornin et al., 2018) families of small GTPases with FAK have been reported to affect tumor invasion in a number of ways. Some metastasis-related enzymes also play a role through the FAK signaling pathway, such as euchromatic histone methyltransferase 2 (G9a) (Sun et al., 2021), MMP-2 (Kwon et al., 2021), and PKCθ (Chadelle et al., 2022). Thus, FAK plays a vital role in the process of tumor invasion through its interaction with a range of invasion-associated molecules.
Recent studies have shown a strong relationship between calcium levels and FAK, which may also contribute to the upregulation of FAK expression and affect tumor aggressiveness. Calcium release-activated calcium modulator 2 (Orai2) is primarily upregulated during lymph node metastasis in gastric cancer. It enhances gastric cancer cell metastasis by inducing FAK-mediated MAPK/ERK activation and promotes the dissociation of focal adhesions at the posterior margin of cells (Wu et al., 2021b). Studies on Orai3 calcium channels have revealed that Orai3 alters cell adhesion capacity in two ways: 1) by reducing calpain activity, cell adhesion, and migration in a calcium-dependent manner and 2) via interaction with FAK to regulate the actin cytoskeleton (Chamlali et al., 2021), which is the main driver of cell adhesion and mechanosensing in a Ca2+-independent manner.
4.3 Focal adhesion kinase and tumor microenvironment
The TME is composed of cellular components (endothelial cells, immune cells, stromal cells, and fibroblasts) and non-cellular components (ECM, cytokines/chemokines, growth factors, and hormones) surrounding the tumor. FAK is known to play a vital role in promoting TME remodeling, which includes several processes such as angiogenesis, immune cell recruitment, and ECM (Figure 4).
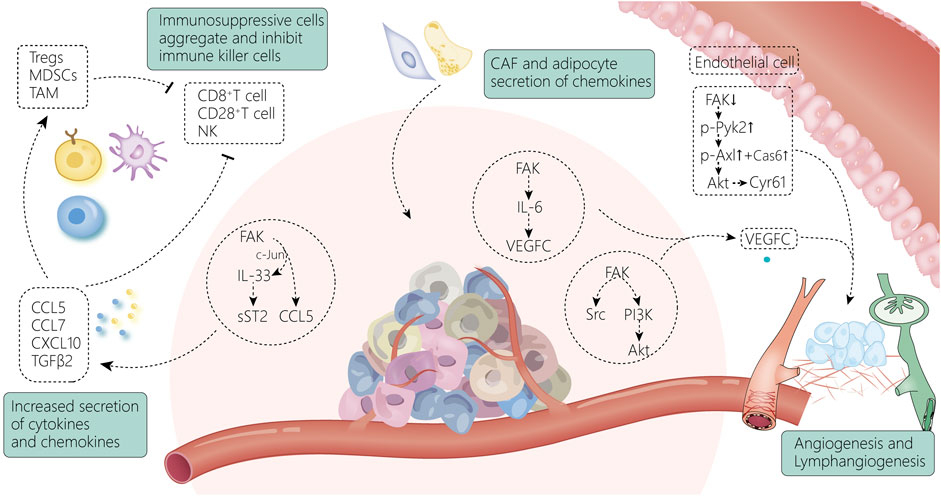
FIGURE 4. FAK and the tumor microenvironment are intricately linked. The increase in chemokines secreted by tumor cells through FAK-related pathways induces the accumulation of immunosuppressive cells and suppresses immune killer cells, allowing tumor cells to gradually escape from the immune system (Huang et al., 2016a); meanwhile, FAK activates the VEGF-C-secreted signaling pathway within the tumor tissue, leading to enhanced vascular regeneration and lymphatic vessel regeneration around the tumor. Endothelial cells (Pedrosa et al., 2019), fibroblasts (Demircioglu et al., 2020), and adipocytes (Blücher et al., 2020) surrounding the tumor can also contribute to the shaping of the TME by activating FAK-related pathways.
4.3.1 Cytokines and immune cells
FAK expression drives the establishment of an immunosuppressive TME by increasing the expression of various chemokines. It has been found that nuclear FAK increases the expression of homing signals (Huehn and Hamann, 2005; Ondondo et al., 2013), such as CCL5, CCL7, CXCL10, and TGFβ2, which are chemokines and cytokines associated with the recruitment of regulatory T cells (Tregs) (Serrels et al., 2015). This suggests that FAK activation in cancer cells plays a critical role in regulating the tumor immune landscape. FAK also enhances the expression of IL-33 (Griffith et al., 2021), and the FAK-IL-33 complex can increase the transcription of chemokine genes by interacting with CCL5 transcriptional regulators. It can also enhance the suppressive activity of Treg cells by interacting with ST2L on the surface of immune cells, thereby promoting tumor growth (Schiering et al., 2014). Alternatively, it activates the cytotoxic function of CD8+ T cells, resulting in improved antitumor immunity (Yang et al., 2011). FAK depletion results in the regression of CD80-expressing tumors by increasing the number of CD28+ T cells within the TME (Canel et al., 2020). LysM-Cre was used to knock out FAK in mononuclear phagocytes in an MMTV-polyoma middle T murine model of breast cancer, and knockout myeloid cells were found to show faster tumor growth. Increased tumor size was associated with a decrease in the number of natural killer cells, suggesting that FAK expression in myeloid cells correlates with the recruitment or survival of natural killer cells in the TME (Llewellyn et al., 2018).
4.3.2 Angiogenesis
FAK has been shown to play a key role in tumor angiogenesis in multiple in vivo mouse models (Tavora et al., 2010; Kostourou et al., 2013). FAK in endothelial cells initiates angiogenesis, and FAK deletion reduces VEGF- and bFGF-induced angiogenesis (Tavora et al., 2010), which may be achieved through the FAK/Src/PI3K(P55)/Akt axis (Pedrosa et al., 2019). FAK affects angiogenesis and is mainly associated with Tyr397 and Tyr861 (Kostourou et al., 2013). Endothelial cell-specific expression of the FAK Y397F mutant reduces tumor angiogenesis (Pedrosa et al., 2019), where FAK affects VEGFR2 transcription through its kinase activity (Sun et al., 2018b; Shiau et al., 2021). This has also been demonstrated in recent studies, where phosphorylated Try397-FAK was found to be an important part of angiogenesis promotion in experiments in which protrudin (Arora et al., 2022) and HAX1 (You et al., 2022) affected angiogenesis. Try397-FAK can affect angiogenesis via ERG (D'Amico et al., 2022). In a subcutaneous Lewis lung cancer tumor model, only mice with pericyte-specific FAK-Y861F mutation showed reduced angiogenesis and tumor growth. This is associated with a notable increase in vascular degeneration (Lees et al., 2021). In addition, the detection of secretion and protein expression of FAK-Y861F pericytes revealed that cytokines and proteins promote tumor cell apoptosis and increased secretion (Lees et al., 2021). Therefore, pericyte FAK-Y861F plays a role in controlling tumor growth (Lees et al., 2021), and pericyte FAK deficiency increases tumor growth and angiogenesis (Lechertier et al., 2020a). Interestingly, when studying the specific mechanism by which FAK phosphorylation at Tyr397 and Tyr861 regulates tumor angiogenesis, it was found that FAKY397F/Y397F and FAKY861F/Y861F mice had different end-stage tumor vascular responses. This may be due to the enhanced p190Rhogef/p130Cas dependent signal of FAK-Y861F rather than FAK-Y397F (Pedrosa et al., 2019). Furthermore, pericyte FAK deletion enhances Gas6-stimulated phosphorylation of the receptor tyrosine kinase Axl and upregulates Cyr61, while pericyte-derived Cyr61 indicates that tumor cells upregulate the expression of the pro-angiogenic/tumorigenic transmembrane receptor tissue factor (Lechertier et al., 2020b). In addition to being a vascular signal, endothelial FAK is a regulatory site for tumor chemoradiotherapy sensitivity (Roy-Luzarraga and Hodivala-Dilke, 2016). FAK also affects ECM by promoting vascular permeability (Lee et al., 2010; Chen et al., 2012), thereby increasing the probability of tumor metastasis (Jean et al., 2014).
4.3.3 Lymphangiogenesis
Lymphopenia and immunocytotoxicity are also associated with metastasis (Mlecnik et al., 2016). FAK reduces lymphocyte toxicity and affects lymphatic vessel formation (Morita et al., 2015). Among the known lymphangiogenic factors, vascular endothelial growth factor-C (VEGF-C) is the best characterized and recognized as a major regulator of lymphangiogenesis. It reshapes the lymphatic microenvironment by regulating the production of chemokines in lymphatic endothelial cells (Chen et al., 2019). FAK affects VEGF-C production via various signaling pathways. For example, FAK inhibition can reduce IL-6-induced VEGF-C expression and VEGF-C promoter luciferase activity (Huang et al., 2016a). Leptin-induced VEGF-C is mediated by the FAK/PI3K/Akt signaling pathway and negatively regulates the expression of microRNA-27b (Yang et al., 2016). The expression level of Nrp2 in tumor-associated lymphatic endothelial cells in colorectal cancer is significantly correlated with tumor lymphatic density. Nrp2 promotes tumor lymphangiogenesis through the integrin α9β1/FAK/Erk pathway rather than the VEGF-C/VEGFR3 signaling pathway (Ou et al., 2015).
4.3.4 Extracellular matrix remodeling
Based on FAK signaling, the metabolic relationship between the ECM and the tumor is mutual. The absence of FAK in CAFs leads to enhanced glycolysis in malignant cells because FAK deletion in CAFs increases the production of chemokines CCL6 and CCL12. This in turn activates protein kinase A through CCR1/CCR2 in cancer cells (Demircioglu et al., 2020). At the same time, adipose tissue in obesity can also induce the activation of tumor FAK signaling by secreting chemokines or fatty acids and change tumor invasiveness and lipid metabolism (Blücher et al., 2020). Desmosplasia is a characteristic of most solid tumors in which PI3K plays a vital role, affecting tumor development. PI3K activation occurs when increased matrix stiffness is triggered through integrin-mediated FAK and its downstream pathway (Kallergi et al., 2007; Provenzano et al., 2008; Tung et al., 2015). The regulation of PIP3 by PI3K and the subsequent activation of Akt and mTOR are the means of remodeling the tumor environment. Through this medium, desmosplasia and increased ECM deposition affect cell metabolism, promoting cell proliferation and survival (Wozniak et al., 2003) as well as carcinogenic transformation and tumor metastasis (Levental et al., 2009). It is also the main cause of acquired chemoresistance (Darvishi et al., 2022). Therefore, FAK plays a significant role in physical construction of the TME.
4.4 Focal adhesion kinase and metabolic reprogramming
It has become apparent that high levels of FAK can orchestrate tumor progression by promoting metabolic reprogramming (Zhang et al., 2016). However, the specific mechanisms remain unclear.
4.4.1 Glucose metabolism
After blocking FAK with siRNA and inhibitors, glucose uptake and glycolysis in glioblastoma multiforme cells were inhibited, but mitochondrial function was significantly enhanced (Che et al., 2021). In addition, fat-selective loss of FAK leads to impaired glucose tolerance and insulin sensitivity (Luk et al., 2017). Growth factors, such as insulin/IGF-1 and anchorage, are the primary extracellular cues that stimulate cell proliferation. FAK interactions with IGF1R (Kasprzak, 2021) and integrins (Che et al., 2021) transmit these growth signals by activating effectors, such as PI3K/Akt, promoting glucose consumption to fuel rapid growth of tumor cells. The N-terminal FERM structural domain of FAK binds directly to the IGF1R (Stanicka et al., 2018), leading to the activation of PI3K/Akt (Godoy-Parejo et al., 2019) and YAP (Rigiracciolo et al., 2020) signaling. Inhibition of the FAK-IGF1R interaction by small molecules induces apoptosis and inhibits tumor growth (Lehman et al., 2021). Impaired non-dependent biological functions of IGF1R kinase lead to a decrease in intracellular glucose levels, resulting in decreased cancer cell viability (Wang et al., 2022). Likewise, integrins are among the reinforcing factors in the Warburg effect of tumors (Yousefi et al., 2021). Studies have shown that FAK is a downstream effector of integrin αV/β3 and regulates the metabolic changes in glioblastoma cells to glycolysis (Che et al., 2021). CD81 can interact with integrins αV/β1 and αV/β5 to form a complex that mediates irisin-induced FAK signal transduction, and subsequently regulates the growth and energy balance of beige fat progenitor cells (Oguri et al., 2020). Twist, a key regulator of EMT, enhances aerobic glycolysis by activating β1-integrin/FAK/PI3K/AKT/mTOR and inhibiting P53 signaling (Yang et al., 2015). ECM1 significantly increased the uptake of 18F-deoxyglucose by xenografts, and further studies have found that ECM1 interacts with integrin β4 and induces the expression of the transcription factor SOX2 through the integrin β4/FAK/glycogen synthase kinase 3β/HIF-1α pathway. This changes the gene expression of EMT factors and glucose metabolism-related enzymes (Gan et al., 2018).
In addition, CTGF promotes aerobic glycolysis via the FAK/Src/NF-κB p65/Glut3 pathway (Kim et al., 2021). Hexokinase 2 (HK2) is highly expressed in ascites and metastases in patients with ovarian cancer. It is the first key enzyme to be involved in glucose metabolism. HK2 overexpression can regulate lactate production through the expression of MMP9/Nanog/Sox9 mediated by the FAK/ERK1/2 signaling pathway and participates in ovarian cancer metastasis and stem cell regulation (Siu et al., 2019).
4.4.2 Lipid metabolism
As the key regulator of de novo lipid synthesis, fatty acid synthase (FASN) is highly expressed in many tumors. Inhibition of FASN reduces the activity of p-FAK, indicating that FAK may contribute to changes in the invasive phenotype of tumor cells caused by metabolic reprogramming (Jafari et al., 2019). Additionally, inhibition of critical lipogenic enzymes ACLY and FAS results in the reduction of FAK, Akt, and paxillin activity and cell viability (Zaytseva et al., 2012).
4.4.3 Amino acid metabolism
FAK expression is related to glutamine metabolism, which may mediate changes in glutamine metabolism through the PI3K/Akt pathway, thus playing a role in cell autophagy, stress, and growth (Zhang and Hochwald, 2014). FAK stimulates PI3K/Akt signaling, whereas PI3K/Akt activation increases the levels of glutamine and its synthetase (Van Der Vos et al., 2012). The YAP/TAZ pathway plays an important role in amino acid metabolic reprogramming (Jeon et al., 2022), and FAK/Src signaling has been shown to mediate the activation of YAP/TAZ signaling in tumor cells (Totaro et al., 2018; Ma et al., 2020). However, there are still questions regarding the specific mechanisms of FAK in amino acid metabolic reprogramming, such as whether it affects the expression of key enzymes and whether there are key signaling pathways.
Whether FAK has a core pathway and function through the metabolism of the three nutrients remains to be investigated, which is an important part of future research on the Warburg effect (Figure 5).
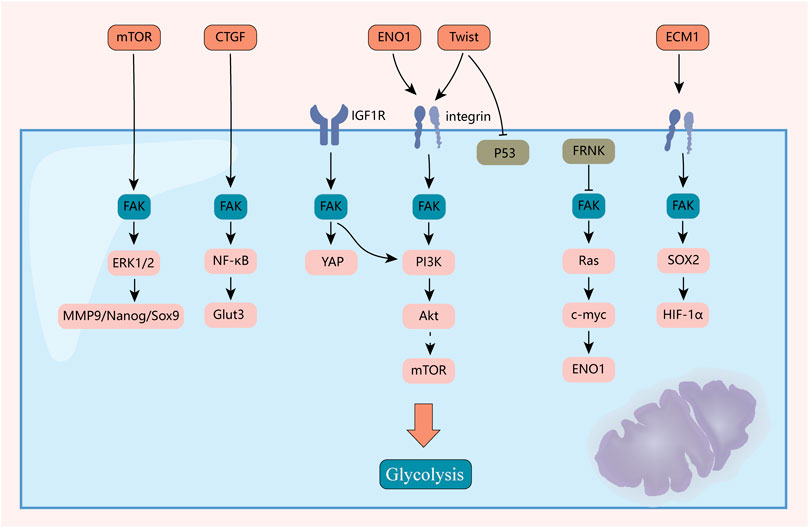
FIGURE 5. Mechanisms of FAK-mediated reprogramming of tumor glycolytic metabolism. FAK plays an important role in reprogramming the metabolism of the three major nutrients. This figure highlights the mechanisms by which it plays a role in reprogramming sugar metabolism.
4.5 Focal adhesion kinase and tumor stemness
Cancer stem cells (CSCs) are important for clonal growth and metastasis of solid tumors. FAK may contribute to CSC activity in diverse types of tumors (Sun et al., 2018a; Diaz Osterman et al., 2019). In a histological study of liver cancer, we found that FAK expression in liver cancer patients was positively correlated with the expression of liver cancer stem cell markers (Fan et al., 2019).
Type I collagen increases the initiation potential, self-renewal ability, and frequency of CSCs in pancreatic ductal adenocarcinoma by activating FAK (Begum et al., 2017). In colon cancer cells, knockdown of transmembrane heparan sulfate proteoglycan syndecan-1 significantly enhances the stem cell phenotype of SDC-1-deficient cells by enhancing the FAK-Wnt signaling axis (Kumar Katakam et al., 2021). In malignant pleural mesothelioma, significant decreases in stem cell markers can be caused by inhibition of PFKFB3, and, thus, the disruption of the FAK-Stat3-SOX2 nexus (Sarkar Bhattacharya et al., 2022). In studies related to the transformation of normal stem cells into tumor stem cells (CSCs) without genetic manipulation, fibroblast growth factor 2 (FGF2) was found to induce normal stem cells to acquire stemness expression of tumor stem cells and initiate cancer; this process was found to be associated with integrin/FAK/PI3K/AKT signaling pathway activation (Sheta et al., 2021). In oral squamous cell carcinoma (OSCC), KRT17 regulates stemness marker levels via the integrin/FAK/Src/ERK/β-catenin pathway (Jang et al., 2022). In addition to its contribution to the maintenance of tumor stemness, the effect of FAK on the stemness of embryonic stem cells has been identified by a wider range of researchers (Baumann, 2021; Hur et al., 2021).
5 Potential for focal adhesion kinase applications in tumor biomarker and therapy
FAK small-molecule inhibitors can be divided into two major groups: 1) inhibitors that target the enzymatic or kinase-dependent functions of FAK, such as inhibitors that target the structural domain of the ATP-binding site and variant inhibitors that target other sites of FAK but still block kinase activity, and 2) inhibitors that target the scaffold function of FAK (Golubovskaya, 2014). The application of FAK inhibitors can directly and synergistically enhance the therapeutic and killing effects on tumor cells and restore the sensitivity of a few drug-resistant tumor cells.
As FAK mediates resistance to treatment, the application of FAK inhibitors can restore the sensitivity of some tumor cells after chemoresistance. In high-grade serous ovarian cancer models in vivo, Y397-FAK phosphorylation increased upon sublethal cisplatin treatment of platinum-resistant tumors (Diaz Osterman et al., 2019). Since platinum-induced cell stress can activate FAK, it has been suggested that FAK activation may function to permit acquired platinum tumor resistance (Diaz Osterman et al., 2019). In addition, FAK inhibition allows resistant tumors to regain cisplatin sensitivity (Mohanty et al., 2020). Cancer patients treated with EGFR inhibitors often develop resistance to treatment. Some evidence suggests that EGFR-TKI resistance works through an integrin-mediated pathway (Seguin et al., 2014), and FAK is involved in the increase in resistance of cancer cells to EGFR-TKI (Solanki et al., 2018). The combination of erlotinib and FAK inhibitors has been shown to be effective in reducing the survival of EGFR-TKI-resistant NSCLC cells (Murakami et al., 2017). In addition, according to Grace et al., during epithelial cell migration, the complex formed by EGFR and FAK has a common downstream Ezrin, and FAK and/or Ezrin could be targeted and/or used in combination with EGFR to overcome the resistance of cancer cells to EGFR-TKI in the future. Reversal of EMT and repolarization of tumor-associated macrophages (TAMs) using simvastatin targeting the role of FAK in lipid metabolism can treat drug-resistant cancers (Jin et al., 2019). The YAP pathway leading to tumor drug resistance is now a comparatively clear mechanism (Nguyen and Yi, 2019). FAK is required for Y357-FAK phosphorylation, and both play a vital role in intrahepatic cholangiocarcinoma (ICCA) development through the FAK/Akt/YAP pathway. ICCA growth was significantly reduced when treated with both FAK inhibitor and CDK4/6 inhibitor palboclib in both in vivo and in vitro experiments (Song et al., 2021). FAK inhibitors synergize with KRAS G12C inhibitors to treat different cancers; this process is also accomplished through the FAK-YAP signaling pathway (Zhang et al., 2021c).
FAK inhibitors can synergistically increase the sensitivity of various cancers to chemotherapeutic agents because they not only reduce FAK expression (Le Large et al., 2021; Tong et al., 2022) but also inhibit numerous signaling pathways associated with FAK. In a study on the effect of tyroservatide (YSV) on lung cancer cell metastasis, YSV was found to inhibit the adhesion and invasion of human lung cancer cells and had a therapeutic effect on lung cancer metastasis. YSV significantly inhibited the phosphorylation of FAK Tyr397 and FAK Tyr576/577 in highly metastatic human lung cancer cells (Huang et al., 2016b). In vivo experiments have shown that endothelial cell-specific FAK deletion sensitizes tumor cells to DNA damage treatment, thereby reducing tumor growth in mice (Newport et al., 2022). In addition, treatment with adriamycin may alter vascular, secretory signaling associated with improved chemosensitivity of acute tumor cells in FAK−/− mice compared with that in wild-type mice (Newport et al., 2022). FAK inhibitors can inhibit tumor progression by altering epigenetic forms. TAE226, in combination with SOR, effectively reduced HCC growth, both in vitro and in vivo. TAE226-mediated FAK deletion and SOR-promoted MAPK downregulation led to a decrease in HDAC1/2 expression in the nucleus, which in turn increased histone H3 lysine 27 acetylation (H3K27ac). This inhibited histone H3 lysine 27 trimethylation (H3K27me3) and suppressed tumor progression through altered epigenetic forms (Romito et al., 2022).
Inhibition of FAK renders tumors more sensitive to radiotherapy (RT) (Eke et al., 2012; Zhang et al., 2021d). In 2002, Kasahara et al. first reported that FAK overexpression significantly enhanced radiation resistance in human leukemia cells. The results of this study showed that FAK overexpression inhibited the caspase-8 expression and caspase-3 activation, thereby exerting resistance to ionizing radiation (IR)-induced apoptosis. This process has since been found to be mediated through various signaling pathways, such as paxillin, Akt1, JNK, and ERK1/2 (Hehlgans et al., 2012; Ou et al., 2012). This is not only related to DNA damage repair, EMT-related protein expression, and cell cycle arrest but may also be related to the immune microenvironment (Skinner et al., 2016a; Skinner et al., 2016b; Hou et al., 2016; Tang et al., 2016). CD8+ T-cell infiltration was significantly enhanced after treatment with FAK inhibitor combined with RT. Additionally, granulocyte infiltration was significantly reduced, and macrophage and T-cell infiltration was significantly increased in the FAK inhibitor combined with radiotherapy group compared with that in the radiotherapy alone group (Osipov et al., 2021) (Figure 6; Tables 2, 3).
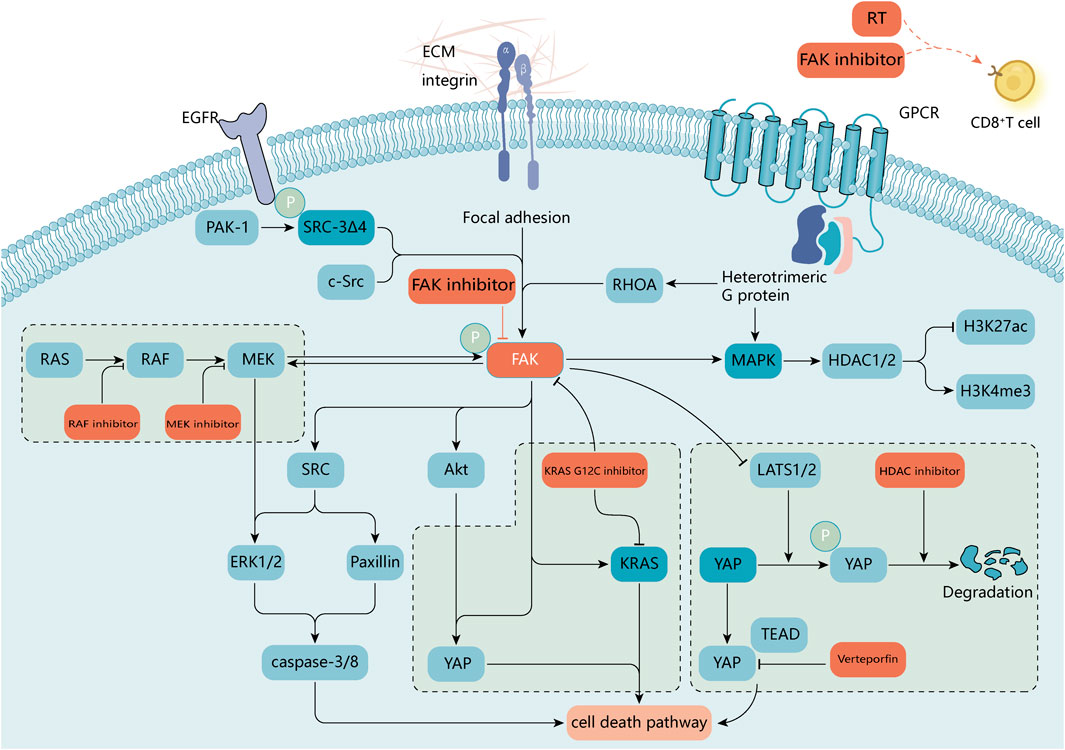
FIGURE 6. A molecular target for combination therapy with FAK inhibitors. FAK supports a variety of oncogenic processes and is beneficial in combination with a variety of available antitumor agents. In RAS-mutated or RAF-mutated cancer cells, blocking the RAS-RAF-MEK pathway with RAF or MEK inhibitors activates FAK and promotes cell survival by reactivating ERK signaling. Activated FAK in diffuse gastric cancer and uveal melanoma attenuates the negative regulation of the transcriptional activator YAP by large tumor suppressor 1 and 2 (LATS1/2). FAK activity can promote nuclear translocation of YAP, and combinations of FAK inhibitors with inhibitors of YAP expression [e.g., histone deacetylase (HDAC) inhibitors] or transcriptional activity may be required to enhance inhibition of oncogenic YAP signaling (Hicks‐Berthet and Varelas, 2017; Song et al., 2021). Inhibition of RHOA or FAK selectively induces mutant KRAS cell death in non-small cell lung cancer studies (Konstantinidou et al., 2013). In breast cancer sRc-3Δ4, a splice isoform of the oncogene was found to be a signaling adapter linking EGFR and FAK and promoting EGF-induced phosphorylation of FAK and c-Src (Long et al., 2010). FAK inhibitors also play a role in epigenetics (Romito et al., 2022), and radiation treatment in combination with FAK inhibitors affects the immune microenvironment surrounding the tumor (Osipov et al., 2021). The small GTPase, RAS homolog family member A (RHOA), regulates the actin cytoskeleton. ECM, extracellular matrix; GPCR, G protein-coupled receptor.
The high expression of FAK in a wide range of tumors, as illustrated in Part II of this paper, suggests its potential as a diagnostic marker. When combined with clinical data, FAK expression levels are found to correlate with prognostic levels in tumors such as liver cancer, gastric cancer, colorectal cancer, bladder cancer, OSCC, breast cancer, thyroid cancer, AML, and melanoma; therefore, FAK has essential qualities as a prognostic marker.
6 Conclusion
In this study, we first analyzed the molecular pathology of FAK expression in various tumor types. We found that it was not only overexpressed in tumors but also correlated with clinical features, such as tumor stage and prognosis of cancer patients. We then described how FAK overexpression exerts regulatory effects at the molecular level in tumor cells and their surroundings. This process participates in many cancer-related processes, such as tumor invasion, EMT, construction of the TME, metabolic reprogramming, and maintenance of tumor stemness. The role of FAK in clinical applications is also summarized. FAK inhibitors combined with other established chemotherapeutic agents can reduce the rate of treatment resistance and further enhance the tumor-killing capacity.
As mentioned earlier, future research on FAK could be combined with the clinical characteristics of patients with tumors to specifically explain how the function of FAK hair differs in pre-, mid-, and late-stage tumor patients. Research on FAK inhibitors is important as it could potentially lead to treating patients with tumors in the future. The scientific justification for the clinical application of FAK needs to be refined. In addition, the potential of FAK for therapeutic and diagnostic purposes is promising and can further oncology research.
Author contributions
ZZ, LJ, SJ, GH, JZ, and TL substantially contributed to the conception, drafting, editing, and final approval of this manuscript.
Funding
This project was supported in part by the Science and Technology Research Project of the Education Department of Jilin Province, JJKH20211123KJ.
Conflict of interest
The authors declare that the research was conducted in the absence of any commercial or financial relationships that could be construed as a potential conflict of interest.
Publisher’s note
All claims expressed in this article are solely those of the authors and do not necessarily represent those of their affiliated organizations, or those of the publisher, the editors and the reviewers. Any product that may be evaluated in this article, or claim that may be made by its manufacturer, is not guaranteed or endorsed by the publisher.
References
Aboubakar Nana, F., Hoton, D., Ambroise, J., Lecocq, M., Vanderputten, M., Sibille, Y., et al. (2019). Increased expression and activation of fak in small-cell lung cancer compared to non-small-cell lung cancer. Cancers (Basel) 11 (10), E1526. Epub 2019/10/30. doi:10.3390/cancers11101526
Aboubakar Nana, F., Lecocq, M., Ladjemi, M. Z., Detry, B., Dupasquier, S., Feron, O., et al. (2019). Therapeutic potential of focal adhesion kinase inhibition in small cell lung cancer. Mol. Cancer Ther. 18 (1), 17–27. Epub 2018/10/26. doi:10.1158/1535-7163.MCT-18-0328
Aboubakar Nana, F., Vanderputten, M., and Ocak, S. (2019). Role of focal adhesion kinase in small-cell lung cancer and its potential as a therapeutic target. Cancers (Basel) 11 (11), E1683. Epub 2019/11/02. doi:10.3390/cancers11111683
Acevedo-Díaz, A., Ortiz-Soto, G., Suárez-Arroyo, I. J., Zayas-Santiago, A., and Martínez Montemayor, M. M. (2019). Ganoderma lucidum extract reduces the motility of breast cancer cells mediated by the rac–lamellipodin Axis. Nutrients 11 (5), 1116. doi:10.3390/nu11051116
Agochiya, M., Brunton, V. G., Owens, D. W., Parkinson, E. K., Paraskeva, C., Keith, W. N., et al. (1999). Increased dosage and amplification of the focal adhesion kinase gene in human cancer cells. Oncogene 18 (41), 5646–5653. doi:10.1038/sj.onc.1202957
Alanko, J., and Ivaska, J. (2016). Endosomes: Emerging platforms for integrin-mediated fak signalling. Trends Cell Biol. 26 (6), 391–398. Epub 20160302. doi:10.1016/j.tcb.2016.02.001
Almstedt, K., Sicking, I., Battista, M. J., Huangfu, S., Heimes, A. S., Weyer-Elberich, V., et al. (2017). Prognostic significance of focal adhesion kinase in node-negative breast cancer. Breast Care (Basel) 12 (5), 329–333. Epub 20171030. doi:10.1159/000477895
Arora, A., Kivelä, A. M., Wang, L., Minkeviciene, R., Taskinen, J. H., Zhang, B., et al. (2022). Protrudin regulates fak activation, endothelial cell migration and angiogenesis. Cell. Mol. Life Sci. 79 (4), 220–20. doi:10.1007/s00018-022-04251-z
Avizienyte, E., Wyke, A. W., Jones, R. J., McLean, G. W., Westhoff, M. A., Brunton, V. G., et al. (2002). Src-induced de-regulation of E-cadherin in colon cancer cells requires integrin signalling. Nat. Cell Biol. 4 (8), 632–638. doi:10.1038/ncb829
Avizienyte, E., Wyke, A. W., Jones, R. J., McLean, G. W., Westhoff, M. A., Brunton, V. G., et al. (2002). Src-induced de-regulation of E-cadherin in colon cancer cells requires integrin signalling. Nat. Cell Biol. 4 (8), 632–638. doi:10.1038/ncb829
Bauer, M. S., Baumann, F., Daday, C., Redondo, P., Durner, E., Jobst, M. A., et al. (2019). Structural and mechanistic insights into mechanoactivation of focal adhesion kinase. Proc. Natl. Acad. Sci. U. S. A. 116 (14), 6766–6774. Epub 20190315. doi:10.1073/pnas.1820567116
Baumann, K. (2021). Evs promote stemness. Nat. Rev. Mol. Cell Biol. 22 (2), 72–73. doi:10.1038/s41580-020-00327-5
Begum, A., Ewachiw, T., Jung, C., Huang, A., Norberg, K. J., Marchionni, L., et al. (2017). The extracellular matrix and focal adhesion kinase signaling regulate cancer stem cell function in pancreatic ductal adenocarcinoma. Plos One 12 (7), e0180181. doi:10.1371/journal.pone.0180181
Béraud, C., Dormoy, V., Danilin, S., Lindner, V., Béthry, A., Hochane, M., et al. (2015). Targeting fak scaffold functions inhibits human renal cell carcinoma growth. Int. J. Cancer 137 (7), 1549–1559. Epub 20150409. doi:10.1002/ijc.29522
Blücher, C., Iberl, S., Schwagarus, N., Müller, S., Liebisch, G., Höring, M., et al. (2020). Secreted factors from adipose tissue reprogram tumor lipid metabolism and induce motility by modulating pparα/angptl4 and fak. Mol. Cancer Res. 18 (12), 1849–1862. doi:10.1158/1541-7786.MCR-19-1223
Brami‐Cherrier, K., Gervasi, N., Arsenieva, D., Walkiewicz, K., Boutterin, M. C., Ortega, A., et al. (2014). Fak dimerization controls its kinase‐dependent functions at focal adhesions. EMBO J. 33 (4), 356–370. doi:10.1002/embj.201386399
Cance, W. G., and Golubovskaya, V. M. (2008). Focal adhesion kinase versus P53: Apoptosis or survival? Sci. Signal. 1 (20), pe22. Epub 20080520. doi:10.1126/stke.120pe22
Cance, W. G., Harris, J. E., Iacocca, M. V., Roche, E., Yang, X., Chang, J., et al. (2000). Immunohistochemical analyses of focal adhesion kinase expression in benign and malignant human breast and colon tissues: Correlation with preinvasive and invasive phenotypes. Clin. Cancer Res. 6 (6), 2417–2423.
Canel, M., Byron, A., Sims, A. H., Cartier, J., Patel, H., Frame, M. C., et al. (2017). Nuclear fak and Runx1 cooperate to regulate Igfbp3, cell-cycle progression, and tumor growth. Cancer Res. 77 (19), 5301–5312. Epub 20170814. doi:10.1158/0008-5472.Can-17-0418
Canel, M., Serrels, A., Frame, M. C., and Brunton, V. G. (2013). E-Cadherin-Integrin crosstalk in cancer invasion and metastasis. J. Cell Sci. 126 (2), 393–401. Epub 20130322. doi:10.1242/jcs.100115
Canel, M., Serrels, A., Miller, D., Timpson, P., Serrels, B., Frame, M. C., et al. (2010). Quantitative in vivo imaging of the effects of inhibiting integrin signaling via Src and fak on cancer cell movement: Effects on E-cadherin dynamics. Cancer Res. 70 (22), 9413–9422. doi:10.1158/0008-5472.CAN-10-1454
Canel, M., Taggart, D., Sims, A. H., Lonergan, D. W., Waizenegger, I. C., and Serrels, A. (2020). T-cell Co-stimulation in combination with targeting fak drives enhanced anti-tumor immunity. Elife 9, e48092. doi:10.7554/eLife.48092
Chadelle, L., Liu, J., Choesmel-Cadamuro, V., Karginov, A. V., Froment, C., Burlet-Schiltz, O., et al. (2022). Pkcθ-mediated serine/threonine phosphorylations of fak govern adhesion and protrusion dynamics within the lamellipodia of migrating breast cancer cells. Cancer Lett. 526, 112–130. doi:10.1016/j.canlet.2021.11.026
Chamlali, M., Kouba, S., Rodat-Despoix, L., Todesca, L. M., Pethö, Z., Schwab, A., et al. (2021). Orai3 calcium channel regulates breast cancer cell migration through calcium-dependent and-independent mechanisms. Cells 10 (12), 3487. doi:10.3390/cells10123487
Che, P., Yu, L., Friedman, G. K., Wang, M., Ke, X., Wang, H., et al. (2021). Integrin Αvβ3 engagement regulates glucose metabolism and migration through focal adhesion kinase (fak) and protein arginine methyltransferase 5 (Prmt5) in glioblastoma cells. Cancers 13 (5), 1111. doi:10.3390/cancers13051111
Chen, J-Y., Lai, Y-S., Chu, P-Y., Chan, S-H., Wang, L-H., and Hung, W-C. (2019). Cancer-derived vegf-C increases chemokine production in lymphatic endothelial cells to promote cxcr2-dependent cancer invasion and mdsc recruitment. Cancers 11 (8), 1120. doi:10.3390/cancers11081120
Chen, J. S., Huang, X. H., Wang, Q., Chen, X. L., Fu, X. H., Tan, H. X., et al. (2010). Fak is involved in invasion and metastasis of hepatocellular carcinoma. Clin. Exp. Metastasis 27, 71–82. doi:10.1007/s10585-010-9306-3
Chen, T. H., Chan, P. C., Chen, C. L., and Chen, H. C. (2011). Phosphorylation of focal adhesion kinase on tyrosine 194 by met leads to its activation through relief of autoinhibition. Oncogene 30 (2), 153–166. Epub 20100830. doi:10.1038/onc.2010.398
Chen, X. L., Nam, J-O., Jean, C., Lawson, C., Walsh, C. T., Goka, E., et al. (2012). Vegf-induced vascular permeability is mediated by fak. Dev. Cell 22 (1), 146–157. doi:10.1016/j.devcel.2011.11.002
Cheng, N., Li, Y., and Han, Z. G. (2013). Argonaute2 promotes tumor metastasis by way of up-regulating focal adhesion kinase expression in hepatocellular carcinoma. Hepatology 57 (5), 1906–1918. doi:10.1002/hep.26202
Cheng, Z., Liu, F., Zhang, H., Li, X., Li, Y., Li, J., et al. (2017). Mir-135a inhibits tumor metastasis and angiogenesis by targeting fak pathway. Oncotarget 8 (19), 31153–31168. Epub 2017/04/19. doi:10.18632/oncotarget.16098
Choe, S. R., Kim, Y. N., Park, C. G., Cho, K. H., Cho, D. Y., and Lee, H. Y. (2018). Rcp induces fak phosphorylation and ovarian cancer cell invasion with inhibition by curcumin. Exp. Mol. Med. 50 (4), 52–10. doi:10.1038/s12276-018-0078-1
Chuang, H. H., Zhen, Y. Y., Tsai, Y. C., Chuang, C. H., Huang, M. S., Hsiao, M., et al. (2021). Targeting Pin1 for modulation of cell motility and cancer therapy. Biomedicines 9 (4), 359. Epub 20210331. doi:10.3390/biomedicines9040359
Corsi, J. M., Rouer, E., Girault, J. A., and Enslen, H. (2006). Organization and post-transcriptional processing of focal adhesion kinase gene. BMC Genomics 7, 198. Epub 20060804. doi:10.1186/1471-2164-7-198
D'Amico, G., Fernandez, I., Gómez-Escudero, J., Kim, H., Maniati, E., Azman, M. S., et al. (2022). Erg activity is regulated by endothelial fak coupling with trim25/usp9x in vascular patterning. Development 149 (13), dev200528. doi:10.1242/dev.200528
Darvishi, B., Eisavand, M. R., Majidzadeh, -A. K., and Farahmand, L. (2022). Matrix stiffening and acquired resistance to chemotherapy: Concepts and clinical significance. Br. J. Cancer 126, 1253–1263. doi:10.1038/s41416-021-01680-8
Demircioglu, F., Wang, J., Candido, J., Costa, A. S. H., Casado, P., de Luxan Delgado, B., et al. (2020). Cancer associated fibroblast fak regulates malignant cell metabolism. Nat. Commun. 11 (1), 1290. Epub 20200310. doi:10.1038/s41467-020-15104-3
Devaud, C., Tilkin-Mariamé, A. F., Vignolle-Vidoni, A., Souleres, P., Denadai-Souza, A., Rolland, C., et al. (2019). Fak alternative splice mrna variants expression pattern in colorectal cancer. Int. J. Cancer 145 (2), 494–502. Epub 20190214. doi:10.1002/ijc.32120
Diaz Osterman, C. J., Ozmadenci, D., Kleinschmidt, E. G., Taylor, K. N., Barrie, A. M., Jiang, S., et al. (2019). Fak activity sustains intrinsic and acquired ovarian cancer resistance to platinum chemotherapy. Elife 8, 4. doi:10.7554/eLife.47327
Ding, Q., Grammer, J. R., Nelson, M. A., Guan, J. L., Stewart, J. E., and Gladson, C. L. (2005). P27kip1 and cyclin D1 are necessary for focal adhesion kinase regulation of cell cycle progression in glioblastoma cells propagated in vitro and in vivo in the scid mouse brain. J. Biol. Chem. 280 (8), 6802–6815. Epub 20041119. doi:10.1074/jbc.M409180200
Dong, C., Li, X., Yang, J., Yuan, D., Zhou, Y., Zhang, Y., et al. (2021). Ppfibp1 induces glioma cell migration and invasion through fak/src/jnk signaling pathway. Cell Death Dis. 12 (9), 827–911. doi:10.1038/s41419-021-04107-7
Dy, G., Ylagan, L., Pokharel, S., Miller, A., Brese, E., Bshara, W., et al. (2014). The prognostic significance of focal adhesion kinase expression in stage I non-small-cell lung cancer. J. Thorac. Oncol. 9 (9), 1278–1284. doi:10.1097/jto.0000000000000248
Dzung, A., Saltari, A., Tiso, N., Lyck, R., Dummer, R., and Levesque, M. P. (2022). Stk11 prevents invasion through signal transducer and activator of transcription 3/5 and fak repression in cutaneous melanoma. J. Invest. Dermatol. 142 (4), 1171–1182.e10. e10. doi:10.1016/j.jid.2021.09.035
Egawa, H., Jingushi, K., Hirono, T., Ueda, Y., Kitae, K., Nakata, W., et al. (2016). The mir-130 family promotes cell migration and invasion in bladder cancer through fak and Akt phosphorylation by regulating pten. Sci. Rep. 6, 20574. Epub 2016/02/04. doi:10.1038/srep20574
Eke, I., Deuse, Y., Hehlgans, S., Gurtner, K., Krause, M., Baumann, M., et al. (2012). β₁Integrin/FAK/cortactin signaling is essential for human head and neck cancer resistance to radiotherapy. J. Clin. Invest. 122 (4), 1529–1540. doi:10.1172/JCI61350
Fan, Z., Duan, J., Wang, L., Xiao, S., Li, L., Yan, X., et al. (2019). Ptk2 promotes cancer stem cell traits in hepatocellular carcinoma by activating wnt/Β-catenin signaling. Cancer Lett. 450, 132–143. Epub 20190305. doi:10.1016/j.canlet.2019.02.040
Feng, X., Arang, N., Rigiracciolo, D. C., Lee, J. S., Yeerna, H., Wang, Z., et al. (2019). A platform of synthetic lethal gene interaction networks reveals that the gnaq uveal melanoma oncogene controls the Hippo pathway through fak. Cancer Cell 35 (3), 457–472. e5. doi:10.1016/j.ccell.2019.01.009
Ferlay, J., Soerjomataram, I., Dikshit, R., Eser, S., Mathers, C., Rebelo, M., et al. (2015). Cancer incidence and mortality worldwide: Sources, methods and major patterns in globocan 2012. Int. J. Cancer 136 (5), E359–E386. Epub 20141009. doi:10.1002/ijc.29210
Frame, M. C., Patel, H., Serrels, B., Lietha, D., and Eck, M. J. (2010). The ferm domain: Organizing the structure and function of fak. Nat. Rev. Mol. Cell Biol. 11 (11), 802–814. doi:10.1038/nrm2996
Francalanci, P., Giovannoni, I., De Stefanis, C., Romito, I., Grimaldi, C., Castellano, A., et al. (2020). Focal adhesion kinase (fak) over-expression and prognostic implication in pediatric hepatocellular carcinoma. Int. J. Mol. Sci. 21 (16), E5795. Epub 2020/08/19. doi:10.3390/ijms21165795
Frisch, S. M., Schaller, M., and Cieply, B. (2013). Mechanisms that link the oncogenic epithelial-mesenchymal transition to suppression of anoikis. J. Cell Sci. 126 (1), 21–29. doi:10.1242/jcs.120907
Frisch, S. M., Vuori, K., Ruoslahti, E., and Chan-Hui, P. Y. (1996). Control of adhesion-dependent cell survival by focal adhesion kinase. J. Cell Biol. 134 (3), 793–799. doi:10.1083/jcb.134.3.793
Fu, Q-F., Liu, Y., Fan, Y., Hua, S-N., Qu, H-Y., Dong, S-W., et al. (2015). Alpha-enolase promotes cell glycolysis, growth, migration, and invasion in non-small cell lung cancer through fak-mediated pi3k/akt pathway. J. Hematol. Oncol. 8 (1), 22–13. doi:10.1186/s13045-015-0117-5
Fu, Y., Zhang, Y., Lei, Z., Liu, T., Cai, T., Wang, A., et al. (2020). Cryo-EM studies of virus-antibody immune complexes. Virol. Sin. 13 (1), 1–13. doi:10.1007/s12250-019-00190-5
Fujii, T., Koshikawa, K., Nomoto, S., Okochi, O., Kaneko, T., Inoue, S., et al. (2004). Focal adhesion kinase is overexpressed in hepatocellular carcinoma and can Be served as an independent prognostic factor. J. Hepatol. 41 (1), 104–111. Epub 2004/07/13. doi:10.1016/j.jhep.2004.03.029
Furuyama, K., Doi, R., Mori, T., Toyoda, E., Ito, D., Kami, K., et al. (2006). Clinical significance of focal adhesion kinase in resectable pancreatic cancer. World J. Surg. 30 (2), 219–226. Epub 2006/01/21. doi:10.1007/s00268-005-0165-z
Gan, L., Meng, J., Xu, M., Liu, M., Qi, Y., Tan, C., et al. (2018). Extracellular matrix protein 1 promotes cell metastasis and glucose metabolism by inducing integrin β4/fak/sox2/hif-1α signaling pathway in gastric cancer. Oncogene 37 (6), 744–755. doi:10.1038/onc.2017.363
Gayrard, C., Bernaudin, C., Déjardin, T., Seiler, C., and Borghi, N. (2018). Src-and confinement-dependent fak activation causes E-cadherin relaxation and Β-catenin activity. J. Cell Biol. 217 (3), 1063–1077. doi:10.1083/jcb.201706013
Gillory, L. A., Stewart, J. E., Megison, M. L., Nabers, H. C., Mroczek-Musulman, E., and Beierle, E. A. (2013). Fak inhibition decreases hepatoblastoma survival both in vitro and in vivo. Transl. Oncol. 6 (2), 206–215. Epub 2013/04/02. doi:10.1593/tlo.12505
Godoy-Parejo, C., Deng, C., Liu, W., and Chen, G. (2019). Insulin stimulates pi3k/akt and cell adhesion to promote the survival of individualized human embryonic stem cells. Stem cells 37 (8), 1030–1041. doi:10.1002/stem.3026
Golubovskaya, M. V., and Cance, G. W. (2011). Fak and P53 protein interactions. Anticancer. Agents Med. Chem. 11 (7), 617–619. doi:10.2174/187152011796817619
Golubovskaya, V. M. (2014). Targeting fak in human cancer: From finding to first clinical trials. Front. Biosci. 19, 687–706. Epub 2014/01/07. doi:10.2741/4236
Golubovskaya, V. M., Ylagan, L., Miller, A., Hughes, M., Wilson, J., Wang, D., et al. (2014). High focal adhesion kinase expression in breast carcinoma is associated with lymphovascular invasion and triple-negative phenotype. Bmc Cancer 14, 769. Epub 20141017. doi:10.1186/1471-2407-14-769
Goñi, G. M., Epifano, C., Boskovic, J., Camacho-Artacho, M., Zhou, J., Bronowska, A., et al. (2014). Phosphatidylinositol 4, 5-bisphosphate triggers activation of focal adhesion kinase by inducing clustering and conformational changes. Proc. Natl. Acad. Sci. U. S. A. 111 (31), E3177–E3186. doi:10.1073/pnas.1317022111
Goto, Y., Ando, T., Izumi, H., Feng, X., Arang, N., Gilardi, M., et al. (2020). Muscarinic receptors promote castration-resistant growth of prostate cancer through a fak-yap signaling Axis. Oncogene 39 (20), 4014–4027. Epub 20200323. doi:10.1038/s41388-020-1272-x
Griffith, B. G., Upstill-Goddard, R., Brunton, H., Grimes, G. R., Biankin, A. V., Serrels, B., et al. (2021). Fak regulates il-33 expression by controlling chromatin accessibility at C-jun motifs. Sci. Rep. 11 (1), 229–314. doi:10.1038/s41598-020-80111-9
Gu, H. J., and Zhou, B. (2018). Focal adhesion kinase promotes progression and predicts poor clinical outcomes in patients with osteosarcoma. Oncol. Lett. 15 (5), 6225–6232. Epub 20180301. doi:10.3892/ol.2018.8152
Gutenberg, A., Brück, W., Buchfelder, M., and Ludwig, H. C. (2004). Expression of tyrosine kinases fak and Pyk2 in 331 human astrocytomas. Acta Neuropathol. 108 (3), 224–230. Epub 20040617. doi:10.1007/s00401-004-0886-3
Haskell, H., Natarajan, M., Hecker, T. P., Ding, Q., Stewart, J., Grammer, J. R., et al. (2003). Focal adhesion kinase is expressed in the angiogenic blood vessels of malignant astrocytic tumors in vivo and promotes capillary tube formation of brain microvascular endothelial cells. Clin. Cancer Res. 9 (6), 2157–2165.
Hauck, C. R., Hsia, D. A., and Schlaepfer, D. D. (2002). The focal adhesion kinase--a regulator of cell migration and invasion. IUBMB Life 53 (2), 115–119. doi:10.1080/15216540211470
Hauck, C. R., Sieg, D. J., Hsia, D. A., Loftus, J. C., Gaarde, W. A., Monia, B. P., et al. (2001). Inhibition of focal adhesion kinase expression or activity disrupts epidermal growth factor-stimulated signaling promoting the migration of invasive human carcinoma cells. Cancer Res. 61 (19), 7079–7090.
Hecker, T. P., Ding, Q., Rege, T. A., Hanks, S. K., and Gladson, C. L. (2004). Overexpression of fak promotes ras activity through the formation of a fak/P120rasgap complex in malignant astrocytoma cells. Oncogene 23 (22), 3962–3971. Epub 2004/04/13. doi:10.1038/sj.onc.1207541
Hehlgans, S., Eke, I., and Cordes, N. (2012). Targeting fak radiosensitizes 3-dimensional grown human hnscc cells through reduced Akt1 and mek1/2 signaling. Int. J. Radiat. Oncol. Biol. Phys. 83 (5), e669–e676. doi:10.1016/j.ijrobp.2012.01.065
Hicks‐Berthet, J., and Varelas, X. (2017). Integrin‐Fak‐Cdc42‐Pp1a signaling gnaws at yap/taz activity to control incisor stem cells. BioEssays 39 (10), 1700116. doi:10.1002/bies.201700116
Ho, B., Olson, G., Figel, S., Gelman, I., Cance, W. G., and Golubovskaya, V. M. (2012). Nanog increases focal adhesion kinase (fak) promoter activity and expression and directly binds to fak protein to Be phosphorylated. J. Biol. Chem. 287 (22), 18656–18673. Epub 20120405. doi:10.1074/jbc.M111.322883
Hou, J., Zhou, Z., Chen, X., Zhao, R., Yang, Z., Wei, N., et al. (2016). Her2 reduces breast cancer radiosensitivity by activating focal adhesion kinase in vitro and in vivo. Oncotarget 7 (29), 45186–45198. doi:10.18632/oncotarget.9870
Huang, Y., Zhao, L., Fu, Z., Zhao, M., Song, X., Jia, J., et al. (2016). Therapeutic effects of tyroservatide on metastasis of lung cancer and its mechanism affecting integrin-focal adhesion kinase signal transduction. Drug Des. devel. Ther. 10, 649–663. doi:10.2147/dddt.S86284
Huang, Y-H., Yang, H-Y., Huang, S-W., Ou, G., Hsu, Y-F., and Hsu, M-J. (2016). Interleukin-6 induces vascular endothelial growth factor-C expression via src-fak-stat3 signaling in lymphatic endothelial cells. Plos One 11 (7), e0158839. doi:10.1371/journal.pone.0158839
Huehn, J., and Hamann, A. (2005). Homing to suppress: Address codes for Treg migration. Trends Immunol. 26 (12), 632–636. Epub 20051021. doi:10.1016/j.it.2005.10.001
Hur, Y. H., Feng, S., Wilson, K. F., Cerione, R. A., and Antonyak, M. A. (2021). Embryonic stem cell-derived extracellular vesicles maintain esc stemness by activating fak. Dev. Cell 56 (3), 277–291.e6. e6. doi:10.1016/j.devcel.2020.11.017
Ignjatović, V. B., Janković Miljuš, J. R., Rončević, J. V., Tatić, S. B., Išić Denčić, T. M., Đorić, I., et al. (2021). Focal adhesion kinase splicing and protein activation in papillary thyroid carcinoma progression. Histochem. Cell Biol. 157, 183–194. Epub 20211124. doi:10.1007/s00418-021-02056-y
Ignjatović, V. B., Janković Miljuš, J. R., Rončević, J. V., Tatić, S. B., Išić Denčić, T. M., Đorić, I., et al. (2022). Focal adhesion kinase splicing and protein activation in papillary thyroid carcinoma progression. Histochem. Cell Biol. 157 (2), 183–194. Epub 20211124. doi:10.1007/s00418-021-02056-y
Imaizumi, M., Nishimura, M., Takeuchi, S., Murase, M., and Hamaguchi, M. (1997). Role of tyrosine specific phosphorylation of cellular proteins, especially egf receptor and P125fak in human lung cancer cells. Lung Cancer 17 (1), 69–84. Epub 1997/05/01. doi:10.1016/s0169-5002(97)00650-8
Itoh, S., Maeda, T., Shimada, M., Aishima, S., Shirabe, K., Tanaka, S., et al. (2004). Role of expression of focal adhesion kinase in progression of hepatocellular carcinoma. Clin. Cancer Res. 10 (8), 2812–2817. Epub 2004/04/23. doi:10.1158/1078-0432.ccr-1046-03
Jafari, N., Drury, J., Morris, A. J., Onono, F. O., Stevens, P. D., Gao, T., et al. (2019). De novo fatty acid synthesis-driven sphingolipid metabolism promotes metastatic potential of colorectal cancer. Mol. Cancer Res. 17 (1), 140–152. doi:10.1158/1541-7786.MCR-18-0199
Jang, T-H., Huang, W-C., Tung, S-L., Lin, S-C., Chen, P-M., Cho, C-Y., et al. (2022). Microrna-485-5p targets keratin 17 to regulate oral cancer stemness and chemoresistance via the integrin/fak/src/erk/Β-catenin pathway. J. Biomed. Sci. 29 (1), 42–20. doi:10.1186/s12929-022-00824-z
Jean, C., Chen, X. L., Nam, J-O., Tancioni, I., Uryu, S., Lawson, C., et al. (2014). Inhibition of endothelial fak activity prevents tumor metastasis by enhancing barrier function. J. Cell Biol. 204 (2), 247–263. doi:10.1083/jcb.201307067
Jeon, H. Y., Choi, J., Kraaier, L., Kim, Y. H., Eisenbarth, D., Yi, K., et al. (2022). Airway secretory cell fate conversion via yap‐mtorc1‐dependent essential amino acid metabolism. EMBO J. 41 (8), e109365. doi:10.15252/embj.2021109365
Ji, H., Pang, D., Fu, S., Jin, Y., Yao, L., Qi, J., et al. (2013). Overexpression of focal adhesion kinase correlates with increased lymph node metastasis and poor prognosis in non-small-cell lung cancer. J. Cancer Res. Clin. Oncol. 139 (3), 429–435. doi:10.1007/s00432-012-1342-8
Jin, H., He, Y., Zhao, P., Hu, Y., Tao, J., Chen, J., et al. (2019). Targeting lipid metabolism to overcome emt-associated drug resistance via integrin β3/fak pathway and tumor-associated macrophage repolarization using legumain-activatable delivery. Theranostics 9 (1), 265–278. doi:10.7150/thno.27246
Kallergi, G., Agelaki, S., Markomanolaki, H., Georgoulias, V., and Stournaras, C. (2007). Activation of fak/pi3k/rac1 signaling controls actin reorganization and inhibits cell motility in human cancer cells. Cell. Physiol. biochem. 20 (6), 977–986. doi:10.1159/000110458
Kang, Y., Hu, W., Ivan, C., Dalton, H. J., Miyake, T., Pecot, C. V., et al. (2013). Role of focal adhesion kinase in regulating Yb-1-Mediated paclitaxel resistance in ovarian cancer. J. Natl. Cancer Inst. 105 (19), 1485–1495. Epub 2013/09/26. doi:10.1093/jnci/djt210
Kasprzak, A. (2021). Insulin-like growth factor 1 (Igf-1) signaling in glucose metabolism in colorectal cancer. Int. J. Mol. Sci. 22 (12), 6434. doi:10.3390/ijms22126434
Kato, A., Kato, K., Miyazawa, H., Kobayashi, H., Noguchi, N., and Kawashiri, S. (2020). Focal adhesion kinase (fak) overexpression and phosphorylation in oral squamous cell carcinoma and their clinicopathological significance. Pathol. Oncol. Res. 26 (3), 1659–1667. doi:10.1007/s12253-019-00732-y
Kelemen, O., Convertini, P., Zhang, Z., Wen, Y., Shen, M., Falaleeva, M., et al. (2013). Function of alternative splicing. Gene 514 (1), 1–30. Epub 20120815. doi:10.1016/j.gene.2012.07.083
Kim, H., Son, S., Ko, Y., and Shin, I. (2021). Ctgf regulates cell proliferation, migration, and glucose metabolism through activation of fak signaling in triple-negative breast cancer. Oncogene 40 (15), 2667–2681. Epub 20210310. doi:10.1038/s41388-021-01731-7
Kobayashi, H., Azumi, M., Kimura, Y., Sato, K., Aoki, N., Kimura, S., et al. (2009). Focal adhesion kinase as an immunotherapeutic target. Cancer Immunol. Immunother. 58 (6), 931–940. Epub 20081022. doi:10.1007/s00262-008-0608-0
Konstantinidou, G., Ramadori, G., Torti, F., Kangasniemi, K., Ramirez, R. E., Cai, Y., et al. (2013). Rhoa-Fak is a required signaling Axis for the maintenance of kras-driven lung adenocarcinomas. Cancer Discov. 3 (4), 444–457. doi:10.1158/2159-8290.CD-12-0388
Kostourou, V., Lechertier, T., Reynolds, L. E., Lees, D. M., Baker, M., Jones, D. T., et al. (2013). Fak-heterozygous mice display enhanced tumour angiogenesis. Nat. Commun. 4 (1), 2020–2111. doi:10.1038/ncomms3020
Kumar Katakam, S., Tria, V., Sim, W. C., Yip, G. W., Molgora, S., Karnavas, T., et al. (2021). The heparan sulfate proteoglycan syndecan‐1 regulates colon cancer stem cell function via a focal adhesion kinase—wnt signaling Axis. FEBS J. 288 (2), 486–506. doi:10.1111/febs.15356
Kurenova, E., Xu, L-H., Yang, X., Baldwin, A. S., Craven, R. J., Hanks, S. K., et al. (2004). Focal adhesion kinase suppresses apoptosis by binding to the death domain of receptor-interacting protein. Mol. Cell. Biol. 24 (10), 4361–4371. doi:10.1128/mcb.24.10.4361-4371.2004
Kwon, Y., Park, S-J., Nguyen, B. T., Kim, M. J., Oh, S., Lee, H., et al. (2021). Multi-layered proteogenomic analysis unravels cancer metastasis directed by mmp-2 and focal adhesion kinase signaling. Sci. Rep. 11 (1), 17130–17216. doi:10.1038/s41598-021-96635-7
Lark, A. L., Livasy, C. A., Dressler, L., Moore, D. T., Millikan, R. C., Geradts, J., et al. (2005). High focal adhesion kinase expression in invasive breast carcinomas is associated with an aggressive phenotype. Mod. Pathol. 18 (10), 1289–1294. doi:10.1038/modpathol.3800424
Le Large, T., Bijlsma, M., El Hassouni, B., Mantini, G., Lagerweij, T., Henneman, A., et al. (2021). Focal adhesion kinase inhibition synergizes with nab-paclitaxel to target pancreatic ductal adenocarcinoma. J. Exp. Clin. Cancer Res. 40 (1), 91–12. doi:10.1186/s13046-021-01892-z
Lechertier, T., Reynolds, L. E., Kim, H., Pedrosa, A. R., Gómez-Escudero, J., Muñoz-Félix, J. M., et al. (2020). Pericyte fak negatively regulates gas6/axl signalling to suppress tumour angiogenesis and tumour growth. Nat. Commun. 11 (1), 2810. Epub 20200604. doi:10.1038/s41467-020-16618-6
Lechertier, T., Reynolds, L. E., Kim, H., Pedrosa, A. R., Gómez-Escudero, J., Muñoz-Félix, J. M., et al. (2020). Pericyte fak negatively regulates gas6/axl signalling to suppress tumour angiogenesis and tumour growth. Nat. Commun. 11 (1), 2810–2814. doi:10.1038/s41467-020-16618-6
Lee, E., Choi, A., Jun, Y., Kim, N., Yook, J. I., Kim, S. Y., et al. (2020). Glutathione peroxidase-1 regulates adhesion and metastasis of triple-negative breast cancer cells via fak signaling. Redox Biol. 29, 101391. doi:10.1016/j.redox.2019.101391
Lee, J., Borboa, A. K., Chun, H. B., Baird, A., and Eliceiri, B. P. (2010). Conditional deletion of the focal adhesion kinase fak alters remodeling of the blood–brain barrier in glioma. Cancer Res. 70 (24), 10131–10140. doi:10.1158/0008-5472.CAN-10-2740
Lees, D. M., Reynolds, L. E., Pedrosa, A. R., Roy-Luzarraga, M., and Hodivala-Dilke, K. M. (2021). Phosphorylation of pericyte fak-Y861 affects tumour cell apoptosis and tumour blood vessel regression. Angiogenesis 24 (3), 471–482. Epub 20210317. doi:10.1007/s10456-021-09776-8
Lehman, C. E., Spencer, A., Hall, S., Shaw, J. J., Wulfkuhle, J., Petricoin, E. F., et al. (2021). Igf1r and Src inhibition induce synergistic cytotoxicity in hnscc through inhibition of fak. Sci. Rep. 11 (1), 10826–10914. doi:10.1038/s41598-021-90289-1
Levental, K. R., Yu, H., Kass, L., Lakins, J. N., Egeblad, M., Erler, J. T., et al. (2009). Matrix crosslinking forces tumor progression by enhancing integrin signaling. Cell 139 (5), 891–906. doi:10.1016/j.cell.2009.10.027
Li, M., Hong, L. I., Liao, M., and Guo, G. (2015). Expression and clinical significance of focal adhesion kinase and adrenomedullin in epithelial ovarian cancer. Oncol. Lett. 10 (2), 1003–1007. Epub 2015/12/02. doi:10.3892/ol.2015.3278
Li, S., Huang, X., Zhang, D., Huang, Q., Pei, G., Wang, L., et al. (2013). Requirement of Pea3 for transcriptional activation of fak gene in tumor metastasis. Plos One 8 (11), e79336. Epub 20131118. doi:10.1371/journal.pone.0079336
Lietha, D., Cai, X., Ceccarelli, D. F., Li, Y., Schaller, M. D., and Eck, M. J. (2007). Structural basis for the autoinhibition of focal adhesion kinase. Cell 129 (6), 1177–1187. doi:10.1016/j.cell.2007.05.041
Lightfoot, H. M., Lark, A., Livasy, C. A., Moore, D. T., Cowan, D., Dressler, L., et al. (2004). Upregulation of focal adhesion kinase (fak) expression in ductal carcinoma in situ (dcis) is an early event in breast tumorigenesis. Breast Cancer Res. Treat. 88 (2), 109–116. Epub 2004/11/27. doi:10.1007/s10549-004-1022-8
Lim, S-T., Chen, X. L., Lim, Y., Hanson, D. A., Vo, T-T., Howerton, K., et al. (2008). Nuclear fak promotes cell proliferation and survival through ferm-enhanced P53 degradation. Mol. Cell 29 (1), 9–22. doi:10.1016/j.molcel.2007.11.031
Llewellyn, R. A., Gutknecht, M. F., Thomas, K. S., Conaway, M. R., and Bouton, A. H. (2018). Focal adhesion kinase (fak) deficiency in mononuclear phagocytes alters murine breast tumor progression. Am. J. Cancer Res. 8 (4), 675–687.
Long, W., Yi, P., Amazit, L., LaMarca, H. L., Ashcroft, F., Kumar, R., et al. (2010). SRC-3Delta4 mediates the interaction of EGFR with FAK to promote cell migration. Mol. Cell 37 (3), 321–332. doi:10.1016/j.molcel.2010.01.004
Luk, C. T., Shi, S. Y., Cai, E. P., Sivasubramaniyam, T., Krishnamurthy, M., Brunt, J. J., et al. (2017). Fak signalling controls insulin sensitivity through regulation of adipocyte survival. Nat. Commun. 8 (1), 14360–14413. doi:10.1038/ncomms14360
Luo, Q., Zhang, S., Zhang, D., Yuan, F., Chen, X., and Yang, S. (2020). Expression of Asap1 and fak in gastric cancer and its clinicopathological significance. Oncol. Lett. 20 (1), 974–980. Epub 2020/06/23. doi:10.3892/ol.2020.11612
Ma, H., Wang, J., Zhao, X., Wu, T., Huang, Z., Chen, D., et al. (2020). Periostin promotes colorectal tumorigenesis through integrin-fak-src pathway-mediated yap/taz activation. Cell Rep. 30 (3), 793–806. e6. doi:10.1016/j.celrep.2019.12.075
Ma, R-R., Zhang, H., Chen, H-F., Zhang, G-H., Tian, Y-R., and Gao, P. (2021). Mir-19a/Mir-96-Mediated low expression of Kif26a suppresses metastasis by regulating fak pathway in gastric cancer. Oncogene 40 (14), 2524–2538. doi:10.1038/s41388-020-01610-7
Mlecnik, B., Bindea, G., Kirilovsky, A., Angell, H. K., Obenauf, A. C., Tosolini, M., et al. (2016). The tumor microenvironment and immunoscore are critical determinants of dissemination to distant metastasis. Sci. Transl. Med. 8 (327), 327ra26. doi:10.1126/scitranslmed.aad6352
Mohanty, A., Pharaon, R., Nam, A., Yin, H., Chang, S., Guo, L., et al. (2020). Abstract 6397: Focal adhesion kinase (FAK) inhibition overcomes cisplatin resistance in head and neck squamous cell carcinoma (HNSCC). Cancer Res. 80 (16), 6397. doi:10.1158/1538-7445.am2020-6397
Morita, Y., Hata, K., Nakanishi, M., Omata, T., Morita, N., Yura, Y., et al. (2015). Cellular fibronectin 1 promotes vegf-C expression, lymphangiogenesis and lymph node metastasis associated with human oral squamous cell carcinoma. Clin. Exp. Metastasis 32 (7), 739–753. doi:10.1007/s10585-015-9741-2
Murakami, Y., Sonoda, K., Abe, H., Watari, K., Kusakabe, D., Azuma, K., et al. (2017). The activation of Src family kinases and focal adhesion kinase with the loss of the amplified, mutated egfr gene contributes to the resistance to afatinib, erlotinib and osimertinib in human lung cancer cells. Oncotarget 8 (41), 70736–70751. Epub 20170807. doi:10.18632/oncotarget.19982
Murata, T., Naomoto, Y., Yamatsuji, T., Okawa, T., Shirakawa, Y., Gunduz, M., et al. (2008). Localization of fak is related with colorectal carcinogenesis. Int. J. Oncol. 32 (4), 791–796. Epub 2008/03/25.
Najjar, S., Homan, S., Sheehan, C., and Carlson, J. A. (2020). Serine-910 phosphorylated focal adhesion kinase expression predicts better overall and disease-free survival in melanoma. Appl. Immunohistochem. Mol. Morphol. 28 (2), 130–138. doi:10.1097/pai.0000000000000744
Newport, E., Pedrosa, A. R., Lees, D., Dukinfield, M., Carter, E., Gomez‐Escudero, J., et al. (2022). Elucidating the role of the kinase activity of endothelial cell focal adhesion kinase in angiocrine signalling and tumour growth. J. Pathol. 256, 235–247. doi:10.1002/path.5833
Nguyen, C. D. K., and Yi, C. (2019). Yap/taz signaling and resistance to cancer therapy. Trends Cancer 5 (5), 283–296. Epub 20190327. doi:10.1016/j.trecan.2019.02.010
Nishimura, M., Machida, K., Imaizumi, M., Abe, T., Umeda, T., Takeshima, E., et al. (1996). Tyrosine phosphorylation of 100-130 kda proteins in lung cancer correlates with poor prognosis. Br. J. Cancer 74 (5), 780–787. Epub 1996/09/01. doi:10.1038/bjc.1996.436
Njei, B., Rotman, Y., Ditah, I., and Lim, J. K. (2015). Emerging trends in hepatocellular carcinoma incidence and mortality. Hepatology 61 (1), 191–199. Epub 2014/08/22. doi:10.1002/hep.27388
Oguri, Y., Shinoda, K., Kim, H., Alba, D. L., Bolus, W. R., Wang, Q., et al. (2020). Cd81 controls beige fat progenitor cell growth and energy balance via fak signaling. Cell 182 (3), 563–577. e20. Epub 2020/07/03. doi:10.1016/j.cell.2020.06.021
Okamoto, H., Yasui, K., Zhao, C., Arii, S., and Inazawa, J. (2003). Ptk2 and Eif3s3 genes may Be amplification targets at 8q23-Q24 and are associated with large hepatocellular carcinomas. Hepatology 38 (5), 1242–1249. doi:10.1053/jhep.2003.50457
Ondondo, B. O., Gallimore, A., Jones, E. G., and Godkin, A. (2013). Home sweet home: The tumor microenvironment as a haven for regulatory T cells. Front. Immunol. 4, 197. doi:10.3389/fimmu.2013.00197
Osipov, A., Blair, A. B., Liberto, J., Wang, J., Li, K., Herbst, B., et al. (2021). Inhibition of focal adhesion kinase enhances antitumor response of radiation therapy in pancreatic cancer through Cd8+ T cells. Cancer Biol. Med. 18 (1), 206–214. doi:10.20892/j.issn.2095-3941.2020.0273
Osipov, A., Saung, M. T., Zheng, L., and Murphy, A. G. (2019). Small molecule immunomodulation: The tumor microenvironment and overcoming immune escape. J. Immunother. Cancer 7 (1), 224. Epub 20190822. doi:10.1186/s40425-019-0667-0
Ou, J., Pan, F., Geng, P., Wei, X., Xie, G., Deng, J., et al. (2012). Silencing fibronectin extra domain a enhances radiosensitivity in nasopharyngeal carcinomas involving an fak/akt/jnk pathway. Int. J. Radiat. Oncol. Biol. Phys. 82 (4), e685–e691. doi:10.1016/j.ijrobp.2011.09.040
Ou, J-J., Wei, X., Peng, Y., Zha, L., Zhou, R-B., Shi, H., et al. (2015). Neuropilin-2 mediates lymphangiogenesis of colorectal carcinoma via a vegfc/vegfr3 independent signaling. Cancer Lett. 358 (2), 200–209. doi:10.1016/j.canlet.2014.12.046
Pan, K., and Xie, Y. (2020). Lncrna foxc2-as1 enhances Foxc2 mrna stability to promote colorectal cancer progression via activation of Ca(2+)-fak signal pathway. Cell Death Dis. 11 (6), 434. Epub 2020/06/10. doi:10.1038/s41419-020-2633-7
Park, J. H., Lee, B. L., Yoon, J., Kim, J., Kim, M. A., Yang, H. K., et al. (2010). Focal adhesion kinase (fak) gene amplification and its clinical implications in gastric cancer. Hum. Pathol. 41 (12), 1664–1673. Epub 2010/09/28. doi:10.1016/j.humpath.2010.06.004
Pedrosa, A-R., Bodrug, N., Gomez-Escudero, J., Carter, E. P., Reynolds, L. E., Georgiou, P. N., et al. (2019). Tumor angiogenesis is differentially regulated by phosphorylation of endothelial cell focal adhesion kinase tyrosines-397 and-861. Cancer Res. 79 (17), 4371–4386. doi:10.1158/0008-5472.CAN-18-3934
Plaza-Menacho, I., Morandi, A., Mologni, L., Boender, P., Gambacorti-Passerini, C., Magee, A. I., et al. (2011). Focal adhesion kinase (fak) binds ret kinase via its ferm domain, priming a direct and reciprocal ret-fak transactivation mechanism. J. Biol. Chem. 286 (19), 17292–17302. Epub 20110322. doi:10.1074/jbc.M110.168500
Provenzano, P. P., Inman, D. R., Eliceiri, K. W., Knittel, J. G., Yan, L., Rueden, C. T., et al. (2008). Collagen density promotes mammary tumor initiation and progression. BMC Med. 6 (1), 11–15. doi:10.1186/1741-7015-6-11
Pylayeva, Y., Gillen, K. M., Gerald, W., Beggs, H. E., Reichardt, L. F., and Giancotti, F. G. (2009). Ras- and pi3k-dependent breast tumorigenesis in mice and humans requires focal adhesion kinase signaling. J. Clin. Invest. 119 (2), 252–266. Epub 20090119. doi:10.1172/jci37160
Qiao, D., Jin, J., Xing, J., Zhang, Y., Jia, N., Ren, X., et al. (2021). Baicalein inhibits gastric cancer cell proliferation and migration through a fak interaction via akt/mtor signaling. Am. J. Chin. Med. 49 (02), 525–541. doi:10.1142/S0192415X21500245
Qu, X., Sheng, J., Shen, L., Su, J., Xu, Y., Xie, Q., et al. (2017). Autophagy inhibitor chloroquine increases sensitivity to cisplatin in Qbc939 cholangiocarcinoma cells by mitochondrial ros. PLoS One 12 (3), e0173712. Epub 2017/03/17. doi:10.1371/journal.pone.0173712
Rigiracciolo, D. C., Nohata, N., Lappano, R., Cirillo, F., Talia, M., Scordamaglia, D., et al. (2020). Igf-1/Igf-1r/Fak/Yap transduction signaling prompts growth effects in triple-negative breast cancer (tnbc) cells. Cells 9 (4), 1010. doi:10.3390/cells9041010
Rigiracciolo, D. C., Santolla, M. F., Lappano, R., Vivacqua, A., Cirillo, F., Galli, G. R., et al. (2019). Focal adhesion kinase (fak) activation by estrogens involves gper in triple-negative breast cancer cells. J. Exp. Clin. Cancer Res. 38 (1), 58. Epub 20190206. doi:10.1186/s13046-019-1056-8
Rigiracciolo, D. C., Santolla, M. F., Lappano, R., Vivacqua, A., Cirillo, F., Galli, G. R., et al. (2019). Focal adhesion kinase (fak) activation by estrogens involves gper in triple-negative breast cancer cells. J. Exp. Clin. Cancer Res. 38 (1), 58–16. doi:10.1186/s13046-019-1056-8
Romito, I., Porru, M., Braghini, M. R., Pompili, L., Panera, N., Crudele, A., et al. (2022). Correction to: Focal adhesion kinase inhibitor Tae226 combined with sorafenib slows down hepatocellular carcinoma by multiple epigenetic effects. J. Exp. Clin. Cancer Res. 41 (1), 40–46. doi:10.1186/s13046-022-02247-y
Roy-Luzarraga, M., Abdel-Fatah, T., Reynolds, L. E., Clear, A., Taylor, J. G., Gribben, J. G., et al. (2020). Association of low tumor endothelial cell py397-focal adhesion kinase expression with survival in patients with neoadjuvant-treated locally advanced breast cancer. JAMA Netw. Open 3 (10), e2019304. Epub 20201001. doi:10.1001/jamanetworkopen.2020.19304
Roy-Luzarraga, M., and Hodivala-Dilke, K. (2016). Molecular pathways: Endothelial cell fak—A target for cancer treatment. Clin. Cancer Res. 22 (15), 3718–3724. doi:10.1158/1078-0432.CCR-14-2021
Saito, D., Kyakumoto, S., Chosa, N., Ibi, M., Takahashi, N., Okubo, N., et al. (2013). Transforming growth factor-β1 induces epithelial-mesenchymal transition and integrin a3β1-mediated cell migration of hsc-4 human squamous cell carcinoma cells through Slug. J. Biochem. 153 (3), 303–315. Epub 20121217. doi:10.1093/jb/mvs144
Sarkar Bhattacharya, S., Thirusangu, P., Jin, L., Staub, J., Shridhar, V., and Molina, J. R. (2022). Pfkfb3 works on the fak-stat3-sox2 Axis to regulate the stemness in mpm. Br. J. Cancer 127, 1352–1364. doi:10.1038/s41416-022-01867-7
Schaller, M. D. (2010). Cellular functions of fak kinases: Insight into molecular mechanisms and novel functions. J. Cell Sci. 123 (7), 1007–1013. doi:10.1242/jcs.045112
Schiering, C., Krausgruber, T., Chomka, A., Fröhlich, A., Adelmann, K., Wohlfert, E. A., et al. (2014). The alarmin il-33 promotes regulatory T-cell function in the intestine. Nature 513 (7519), 564–568. Epub 20140716. doi:10.1038/nature13577
Schmitz, K. J., Grabellus, F., Callies, R., Otterbach, F., Wohlschlaeger, J., Levkau, B., et al. (2005). High expression of focal adhesion kinase (P125fak) in node-negative breast cancer is related to overexpression of her-2/neu and activated Akt kinase but does not predict outcome. Breast Cancer Res. 7 (2), R194–R203. Epub 20050107. doi:10.1186/bcr977
Seguin, L., Kato, S., Franovic, A., Camargo, M. F., Lesperance, J., Elliott, K. C., et al. (2014). An integrin ß₃-kras-ralb complex drives tumour stemness and resistance to egfr inhibition. Nat. Cell Biol. 16 (5), 457–468. Epub 20140420. doi:10.1038/ncb2953
Šelemetjev, S., Bartolome, A., Išić Denčić, T., Đorić, I., Paunović, I., Tatić, S., et al. (2018). Overexpression of epidermal growth factor receptor and its downstream effector, focal adhesion kinase, correlates with papillary thyroid carcinoma progression. Int. J. Exp. Pathol. 99 (2), 87–94. Epub 20180417. doi:10.1111/iep.12268
Serrels, A., Canel, M., Brunton, V. G., and Frame, M. C. (2011). Src/Fak-mediated regulation of E-cadherin as a mechanism for controlling collective cell movement: Insights from in vivo imaging. Cell adh. Migr. 5 (4), 360–365. doi:10.4161/cam.5.4.17290
Serrels, A., Lund, T., Serrels, B., Byron, A., McPherson, R. C., von Kriegsheim, A., et al. (2015). Nuclear fak controls chemokine transcription, tregs, and evasion of anti-tumor immunity. Cell 163 (1), 160–173. doi:10.1016/j.cell.2015.09.001
Sheta, M., Hassan, G., Afify, S. M., Monzur, S., Kumon, K., Quora, H. A. A., et al. (2021). Chronic exposure to Fgf2 converts ipscs into cancer stem cells with an enhanced integrin/focal adhesion/pi3k/akt Axis. Cancer Lett. 521, 142–154. doi:10.1016/j.canlet.2021.08.026
Shiau, J-P., Wu, C-C., Chang, S-J., Pan, M-R., Liu, W., Ou-Yang, F., et al. (2021). Fak regulates Vegfr2 expression and promotes angiogenesis in triple-negative breast cancer. Biomedicines 9 (12), 1789. doi:10.3390/biomedicines9121789
Siu, M. K., Jiang, Y-X., Wang, J-J., Leung, T. H., Han, C. Y., Tsang, B. K., et al. (2019). Hexokinase 2 regulates ovarian cancer cell migration, invasion and stemness via fak/erk1/2/mmp9/nanog/sox9 signaling cascades. Cancers 11 (6), 813. doi:10.3390/cancers11060813
Skinner, H. D., Giri, U., Yang, L., Woo, S. H., Story, M. D., Pickering, C. R., et al. (2016). Proteomic profiling identifies ptk2/fak as a driver of radioresistance in hpv-negative head and neck cancer. Clin. Cancer Res. 22 (18), 4643–4650. doi:10.1158/1078-0432.CCR-15-2785
Skinner, H. D., Giri, U., Yang, L., Woo, S. H., Story, M. D., Pickering, C. R., et al. (2016). Proteomic profiling identifies ptk2/fak as a driver of radioresistance in hpv-negative head and neck cancer. Clin. Cancer Res. 22 (18), 4643–4650. Epub 20160401. doi:10.1158/1078-0432.Ccr-15-2785
Solanki, H. S., Raja, R., Zhavoronkov, A., Ozerov, I. V., Artemov, A. V., Advani, J., et al. (2018). Targeting focal adhesion kinase overcomes erlotinib resistance in smoke induced lung cancer by altering phosphorylation of epidermal growth factor receptor. Oncoscience 5 (1-2), 21–38. Epub 20180223. doi:10.18632/oncoscience.395
Song, X., Xu, H., Wang, P., Wang, J., Affo, S., Wang, H., et al. (2021). Focal adhesion kinase (fak) promotes cholangiocarcinoma development and progression via yap activation. J. Hepatol. 75 (4), 888–899. doi:10.1016/j.jhep.2021.05.018
Sonoda, Y., Matsumoto, Y., Funakoshi, M., Yamamoto, D., Hanks, S. K., and Kasahara, T. (2000). Anti-apoptotic role of focal adhesion kinase (fak): Induction of inhibitor-of-apoptosis proteins and apoptosis suppression by the overexpression of fak in a human leukemic cell line, hl-60. J. Biol. Chem. 275 (21), 16309–16315. doi:10.1074/jbc.275.21.16309
Stading, R., Gastelum, G., Chu, C., Jiang, W., and Moorthy, B. (2021). Molecular mechanisms of pulmonary carcinogenesis by polycyclic aromatic hydrocarbons (pahs): Implications for human lung cancer. Seminars cancer Biol. doi:10.1016/j.semcancer.2021.07.001
Stanicka, J., Rieger, L., O’Shea, S., Cox, O., Coleman, M., O’Flanagan, C., et al. (2018). Fes-related tyrosine kinase activates the insulin-like growth factor-1 receptor at sites of cell adhesion. Oncogene 37 (23), 3131–3150. doi:10.1038/s41388-017-0113-z
Su, J. M., Gui, L., Zhou, Y. P., and Zha, X. L. (2002). Expression of focal adhesion kinase and Alpha5 and Beta1 integrins in carcinomas and its clinical significance. World J. Gastroenterol. 8 (4), 613–618. Epub 2002/08/14. doi:10.3748/wjg.v8.i4.613
Sulzmaier, F. J., Jean, C., and Schlaepfer, D. D. (2014). Fak in cancer: Mechanistic findings and clinical applications. Nat. Rev. Cancer 14 (9), 598–610. Epub 20140807. doi:10.1038/nrc3792
Sun, J., Luo, Q., Liu, L., and Song, G. (2018). Low-level shear stress promotes migration of liver cancer stem cells via the fak-erk1/2 signalling pathway. Cancer Lett. 427, 1–8. doi:10.1016/j.canlet.2018.04.015
Sun, J., Luo, Q., Liu, L., Yang, X., Zhu, S., and Song, G. (2017). Salinomycin attenuates liver cancer stem cell motility by enhancing cell stiffness and increasing F-actin formation via the fak-erk1/2 signalling pathway. Toxicology 384, 1–10. doi:10.1016/j.tox.2017.04.006
Sun, S., Wu, H-J., and Guan, J-L. (2018). Nuclear fak and its kinase activity regulate Vegfr2 transcription in angiogenesis of adult mice. Sci. Rep. 8 (1), 2550–2611. doi:10.1038/s41598-018-20930-z
Sun, T., Zhang, K., Pangeni, R. P., Wu, J., Li, W., Du, Y., et al. (2021). G9a promotes invasion and metastasis of non–small cell lung cancer through enhancing focal adhesion kinase activation via nf-?b signaling pathway. Mol. Cancer Res. 19 (3), 429–440. doi:10.1158/1541-7786.MCR-20-0557
Tang, H., Long, Q., Zhuang, K., Yan, Y., Han, K., Guo, H., et al. (2020). Mir-665 promotes the progression of gastric adenocarcinoma via elevating fak activation through targeting Socs3 and is negatively regulated by lncrna Meg3. J. Cell. Physiol. 235 (5), 4709–4719. Epub 2019/10/28. doi:10.1002/jcp.29349
Tang, K. J., Constanzo, J. D., Venkateswaran, N., Melegari, M., Ilcheva, M., Morales, J. C., et al. (2016). Focal adhesion kinase regulates the DNA damage response and its inhibition radiosensitizes mutant kras lung cancer. Clin. Cancer Res. 22 (23), 5851–5863. Epub 20160524. doi:10.1158/1078-0432.Ccr-15-2603
Tani, T., von Koskull, H., and Virtanen, I. (1996). Focal adhesion kinase Pp125fak is associated with both intercellular junctions and matrix adhesion sites in vivo. Histochem. Cell Biol. 105 (1), 17–25. Epub 1996/01/01. doi:10.1007/bf01450874
Tavora, B., Batista, S., Reynolds, L. E., Jadeja, S., Robinson, S., Kostourou, V., et al. (2010). Endothelial fak is required for tumour angiogenesis. EMBO Mol. Med. 2 (12), 516–528. doi:10.1002/emmm.201000106
Thanapprapasr, K., Nartthanarung, A., Thanapprapasr, D., and Jinawath, A. (2017). Pfak-Y397 overexpression as both a prognostic and a predictive biomarker for patients with metastatic osteosarcoma. Plos One 12 (8), e0182989. Epub 20170828. doi:10.1371/journal.pone.0182989
Tian, H., Chou, F-j., Tian, J., Zhang, Y., You, B., Huang, C-P., et al. (2021). Asc-J9® suppresses prostate cancer cell proliferation and invasion via altering the atf3-ptk2 signaling. J. Exp. Clin. Cancer Res. 40 (1), 3–12. doi:10.1186/s13046-020-01760-2
Tomar, S., Plotnik, J. P., Haley, J., Scantland, J., Dasari, S., Sheikh, Z., et al. (2018). Ets1 induction by the microenvironment promotes ovarian cancer metastasis through focal adhesion kinase. Cancer Lett. 414, 190–204. doi:10.1016/j.canlet.2017.11.012
Tong, S., Yin, H., Fu, J., and Li, Y. (2022). Niban apoptosis regulator 1 promotes gemcitabine resistance by activating the focal adhesion kinase signaling pathway in bladder cancer. J. Cancer 13 (4), 1103–1118. doi:10.7150/jca.66248
Tornin, J., Hermida-Prado, F., Padda, R. S., Gonzalez, M. V., Alvarez-Fernandez, C., Rey, V., et al. (2018). Fus-chop promotes invasion in myxoid liposarcoma through a src/fak/rho/rock-dependent pathway. Neoplasia 20 (1), 44–56. doi:10.1016/j.neo.2017.11.004
Totaro, A., Panciera, T., and Piccolo, S. (2018). Yap/taz upstream signals and downstream responses. Nat. Cell Biol. 20 (8), 888–899. doi:10.1038/s41556-018-0142-z
Tremblay, L., Hauck, W., Aprikian, A. G., Begin, L. R., Chapdelaine, A., and Chevalier, S. (1996). Focal adhesion kinase (Pp125fak) expression, activation and association with paxillin and P50csk in human metastatic prostate carcinoma. Int. J. Cancer 68 (2), 164–171. Epub 1996/10/09. doi:10.1002/(sici)1097-0215(19961009)68:2<169::aid-ijc4>3.0.co;2-w
Tung, J. C., Barnes, J. M., Desai, S. R., Sistrunk, C., Conklin, M. W., Schedin, P., et al. (2015). Tumor mechanics and metabolic dysfunction. Free Radic. Biol. Med. 79, 269–280. doi:10.1016/j.freeradbiomed.2014.11.020
Uchihara, T., Miyake, K., Yonemura, A., Komohara, Y., Itoyama, R., Koiwa, M., et al. (2020). Extracellular vesicles from cancer-associated fibroblasts containing annexin A6 induces fak-yap activation by stabilizing Β1 integrin, enhancing drug resistance. Cancer Res. 80 (16), 3222–3235. doi:10.1158/0008-5472.CAN-19-3803
Van Der Vos, K. E., Eliasson, P., Proikas-Cezanne, T., Vervoort, S. J., Van Boxtel, R., Putker, M., et al. (2012). Modulation of glutamine metabolism by the PI(3)K-PKB-FOXO network regulates autophagy. Nat. Cell Biol. 14 (8), 829–837. doi:10.1038/ncb2536
von Wichert, G., Haimovich, B., Feng, G. S., and Sheetz, M. P. (2003). Force-dependent integrin-cytoskeleton linkage formation requires downregulation of focal complex dynamics by Shp2. Embo J. 22 (19), 5023–5035. doi:10.1093/emboj/cdg492
Wang, C., Zhang, S., Liu, J., Tian, Y., Ma, B., Xu, S., et al. (2020). Secreted pyruvate kinase M2 promotes lung cancer metastasis through activating the integrin beta1/fak signaling pathway. Cell Rep. 30 (6), 1780–1797. e6. doi:10.1016/j.celrep.2020.01.037
Wang, J., Wen, T., Li, Z., Che, X., Gong, L., Yang, X., et al. (2019). Microrna-1224 inhibits tumor metastasis in intestinal-type gastric cancer by directly targeting fak. Front. Oncol. 9, 222. Epub 2019/04/26. doi:10.3389/fonc.2019.00222
Wang, P., Mak, V. C., and Cheung, L. W. (2022). Drugging igf-1r in cancer: New insights and emerging opportunities. Genes & Diseases.
Wang, T., Jin, H., Hu, J., Li, X., Ruan, H., Xu, H., et al. (2020). Col4a1 promotes the growth and metastasis of hepatocellular carcinoma cells by activating fak-src signaling. J. Exp. Clin. Cancer Res. 39 (1), 148–216. doi:10.1186/s13046-020-01650-7
Wang, Y-H., Shen, C-Y., Lin, S-C., Kuo, W-H., Kuo, Y-T., Hsu, Y-L., et al. (2021). Monocytes secrete Cxcl7 to promote breast cancer progression. Cell Death Dis. 12 (12), 1090–1098. doi:10.1038/s41419-021-04231-4
Watermann, D. O., Gabriel, B., Jäger, M., Orlowska-Volk, M., Hasenburg, A., zur Hausen, A., et al. (2005). Specific induction of Pp125 focal adhesion kinase in human breast cancer. Br. J. Cancer 93 (6), 694–698. doi:10.1038/sj.bjc.6602744
Weiner, T. M., Liu, E. T., Craven, R. J., and Cance, W. G. (1993). Expression of focal adhesion kinase gene and invasive cancer. Lancet 342 (8878), 1024–1025. Epub 1993/10/23. doi:10.1016/0140-6736(93)92881-s
Weiss, F., Lauffenburger, D., and Friedl, P. (2022). Towards targeting of shared mechanisms of cancer metastasis and therapy resistance. Nat. Rev. Cancer 22 (3), 157–173. doi:10.1038/s41568-021-00427-0
Wozniak, M. A., Desai, R., Solski, P. A., Der, C. J., and Keely, P. J. (2003). Rock-generated contractility regulates breast epithelial cell differentiation in response to the physical properties of a three-dimensional collagen matrix. J. Cell Biol. 163 (3), 583–595. doi:10.1083/jcb.200305010
Wu, H-J., Hao, M., Yeo, S. K., and Guan, J-L. (2020). Fak signaling in cancer-associated fibroblasts promotes breast cancer cell migration and metastasis by exosomal mirnas-mediated intercellular communication. Oncogene 39 (12), 2539–2549. doi:10.1038/s41388-020-1162-2
Wu, J. C., Chen, Y. C., Kuo, C. T., Wenshin Yu, H., Chen, Y. Q., Chiou, A., et al. (2015). Focal adhesion kinase-dependent focal adhesion recruitment of Sh2 domains directs Src into focal adhesions to regulate cell adhesion and migration. Sci. Rep. 5, 18476. Epub 20151218. doi:10.1038/srep18476
Wu, S., Chen, M., Huang, J., Zhang, F., Lv, Z., Jia, Y., et al. (2021). Orai2 promotes gastric cancer tumorigenicity and metastasis through pi3k/akt signaling and mapk-dependent focal adhesion disassembly. Cancer Res. 81 (4), 986–1000. doi:10.1158/0008-5472.CAN-20-0049
Wu, S., Chen, M., Huang, J., Zhang, F., Lv, Z., Jia, Y., et al. (2021). ORAI2 promotes gastric cancer tumorigenicity and metastasis through PI3K/Akt signaling and MAPK-dependent focal adhesion disassembly. Cancer Res. 81 (4), 986–1000. doi:10.1158/0008-5472.CAN-20-0049
Wu, X., Wang, J., Liang, Q., Tong, R., Huang, J., Yang, X., et al. (2022). Recent progress on fak inhibitors with dual targeting capabilities for cancer treatment. Biomed. Pharmacother. 151, 113116. Epub 20220519. doi:10.1016/j.biopha.2022.113116
Wu, Y-J., Lin, S-H., Din, Z-H., Su, J-H., and Liu, C-I. (2019). Sinulariolide inhibits gastric cancer cell migration and invasion through downregulation of the emt process and suppression of fak/pi3k/akt/mtor and mapks signaling pathways. Mar. Drugs 17 (12), 668. doi:10.3390/md17120668
Xiang, X., Wang, Y., Zhang, H., Piao, J., Muthusamy, S., Wang, L., et al. (2018). Vasodilator-stimulated phosphoprotein promotes liver metastasis of gastrointestinal cancer by activating a β1-integrin-fak-yap1/taz signaling pathway. NPJ Precis. Oncol. 2 (1), 2–10. doi:10.1038/s41698-017-0045-7
Xie, M., Sun, M., Ji, X., Li, D., Chen, X., Zhang, B., et al. (2022). Overexpression of Bach1 mediated by Igf2 facilitates hepatocellular carcinoma growth and metastasis via Igf1r and Ptk2. Theranostics 12 (3), 1097–1116. doi:10.7150/thno.65775
Xu, L. H., Owens, L. V., Sturge, G. C., Yang, X., Liu, E. T., Craven, R. J., et al. (1996). Attenuation of the expression of the focal adhesion kinase induces apoptosis in tumor cells. Cell Growth Differ. 7 (4), 413–418.
Xu, L. H., Yang, X., Bradham, C. A., Brenner, D. A., Baldwin, A. S., Craven, R. J., et al. (2000). The focal adhesion kinase suppresses transformation-associated, anchorage-independent apoptosis in human breast cancer cells. Involvement of death receptor-related signaling pathways. J. Biol. Chem. 275 (39), 30597–30604. doi:10.1074/jbc.M910027199
Xu, Z., Li, H., Lin, C., Zeng, B., Chen, Y., and Luo, Y. (2021). Knockdown of Rabl3 suppresses the proliferation and invasion of oral squamous cell carcinoma through inactivating the fak/akt pathway. J. Bioenerg. Biomembr. 53 (2), 203–211. doi:10.1007/s10863-021-09871-x
Yan, D., Dong, W., He, Q., Yang, M., Huang, L., Kong, J., et al. (2019). Circular rna circpicalm sponges mir-1265 to inhibit bladder cancer metastasis and influence fak phosphorylation. EBioMedicine 48, 316–331. Epub 2019/10/28. doi:10.1016/j.ebiom.2019.08.074
Yang, L., Hou, Y., Yuan, J., Tang, S., Zhang, H., Zhu, Q., et al. (2015). Twist promotes reprogramming of glucose metabolism in breast cancer cells through pi3k/akt and P53 signaling pathways. Oncotarget 6 (28), 25755–25769. doi:10.18632/oncotarget.4697
Yang, Q., Li, G., Zhu, Y., Liu, L., Chen, E., Turnquist, H., et al. (2011). Il-33 synergizes with tcr and il-12 signaling to promote the effector function of Cd8+ T cells. Eur. J. Immunol. 41 (11), 3351–3360. Epub 20111013. doi:10.1002/eji.201141629
Yang, W-H., Chang, A-C., Wang, S-W., Wang, S-J., Chang, Y-S., Chang, T-M., et al. (2016). Leptin promotes vegf-C production and induces lymphangiogenesis by suppressing mir-27b in human chondrosarcoma cells. Sci. Rep. 6 (1), 28647–28710. doi:10.1038/srep28647
Yao, L., Li, K., Peng, W., Lin, Q., Li, S., Hu, X., et al. (2014). An aberrant spliced transcript of focal adhesion kinase is exclusively expressed in human breast cancer. J. Transl. Med. 12, 136. Epub 20140521. doi:10.1186/1479-5876-12-136
Yao, L-w., Wu, L-l., Zhang, L-h., Zhou, W., Wu, L., He, K., et al. (2020). Mfap2 is overexpressed in gastric cancer and promotes motility via the mfap2/integrin α5β1/fak/erk pathway. Oncogenesis 9 (2), 17. doi:10.1038/s41389-020-0198-z
Yom, C. K., Noh, D. Y., Kim, W. H., and Kim, H. S. (2011). Clinical significance of high focal adhesion kinase gene copy number and overexpression in invasive breast cancer. Breast Cancer Res. Treat. 128 (3), 647–655. Epub 20100903. doi:10.1007/s10549-010-1150-2
You, B., Pan, S., Gu, M., Zhang, K., Xia, T., Zhang, S., et al. (2022). Extracellular vesicles rich in Hax1 promote angiogenesis by modulating Itgb6 translation. J. Extracell. Vesicles 11 (5), e12221. doi:10.1002/jev2.12221
Yousefi, H., Vatanmakanian, M., Mahdiannasser, M., Mashouri, L., Alahari, N. V., Monjezi, M. R., et al. (2021). Understanding the role of integrins in breast cancer invasion, metastasis, angiogenesis, and drug resistance. Oncogene 40 (6), 1043–1063. doi:10.1038/s41388-020-01588-2
Yu, H. G., Tong, S. L., Ding, Y. M., Ding, J., Fang, X. M., Zhang, X. F., et al. (2006). Enhanced expression of cholecystokinin-2 receptor promotes the progression of colon cancer through activation of focal adhesion kinase. Int. J. Cancer 119 (12), 2724–2732. Epub 2006/09/26. doi:10.1002/ijc.22207
Yu, Y., and Fang, L. (2022). Circrpap2 regulates the alternative splicing of ptk2 by binding to Srsf1 in breast cancer. Cell Death Discov. 8 (1), 152. Epub 20220402. doi:10.1038/s41420-022-00965-y
Zaytseva, Y. Y., Rychahou, P. G., Gulhati, P., Elliott, V. A., Mustain, W. C., O'Connor, K., et al. (2012). Inhibition of fatty acid synthase attenuates CD44-associated signaling and reduces metastasis in colorectal cancer. Cancer Res. 72 (6), 1504–1517. doi:10.1158/0008-5472.CAN-11-4057
Zhang, B., Zhang, Y., Zhang, J., Liu, P., Jiao, B., Wang, Z., et al. (2021). Focal adhesion kinase (fak) inhibition synergizes with kras G12c inhibitors in treating cancer through the regulation of the fak–yap signaling. Adv. Sci. 8 (16), 2100250. doi:10.1002/advs.202100250
Zhang, C., Stockwell, S. R., Elbanna, M., Ketteler, R., Freeman, J., Al-Lazikani, B., et al. (2019). Signalling involving met and fak supports cell division independent of the activity of the cell cycle-regulating cdk4/6 kinases. Oncogene 38 (30), 5905–5920. Epub 20190712. doi:10.1038/s41388-019-0850-2
Zhang, J., Gao, Q., Zhou, Y., Dier, U., Hempel, N., and Hochwald, S. N. (2016). Focal adhesion kinase-promoted tumor glucose metabolism is associated with a shift of mitochondrial respiration to glycolysis. Oncogene 35 (15), 1926–1942. doi:10.1038/onc.2015.256
Zhang, J., and Hochwald, S. N. (2014). The role of fak in tumor metabolism and therapy. Pharmacol. Ther. 142 (2), 154–163. doi:10.1016/j.pharmthera.2013.12.003
Zhang, L., Yao, L., Zhou, W., Tian, J., Ruan, B., Lu, Z., et al. (2021). Mir-497 defect contributes to gastric cancer tumorigenesis and progression via regulating cdc42/itgb1/fak/pxn/akt signaling. Mol. Ther. Nucleic Acids 25, 567–577. Epub 2021/10/01. doi:10.1016/j.omtn.2021.07.025
Zhang, S., Lu, Y., Jiang, H-Y., Cheng, Z-M., Wei, Z-J., Wei, Y-H., et al. (2021). Circc16orf62 promotes hepatocellular carcinoma progression through the mir-138-5p/ptk2/akt Axis. Cell Death Dis. 12 (6), 597–613. doi:10.1038/s41419-021-03866-7
Zhang, T., Wang, Y., Xie, M., Ji, X., Luo, X., Chen, X., et al. (2022). Hgf-mediated elevation of Etv1 facilitates hepatocellular carcinoma metastasis through upregulating Ptk2 and C-met. J. Exp. Clin. Cancer Res. 41 (1), 275–320. doi:10.1186/s13046-022-02475-2
Zhang, Y., Liu, S., Zhou, S., Yu, D., Gu, J., Qin, Q., et al. (2021). Focal adhesion kinase: Insight into its roles and therapeutic potential in oesophageal cancer. Cancer Lett. 496, 93–103. doi:10.1016/j.canlet.2020.10.005
Zhang, Y., and Sun, X. (2020). Role of focal adhesion kinase in head and neck squamous cell carcinoma and its therapeutic prospect. Onco. Targets. Ther. 13, 10207–10220. Epub 2020/10/30. doi:10.2147/OTT.S270342
Zhao, J., Pestell, R., and Guan, J-L. (2001). Transcriptional activation of cyclin D1 promoter by fak contributes to cell cycle progression. Mol. Biol. Cell 12 (12), 4066–4077. doi:10.1091/mbc.12.12.4066
Zhao, J-H., Reiske, H., and Guan, J-L. (1998). Regulation of the cell cycle by focal adhesion kinase. J. Cell Biol. 143 (7), 1997–2008. doi:10.1083/jcb.143.7.1997
Zheng, Y., Yang, W., Xia, Y., Hawke, D., Liu, D. X., and Lu, Z. (2011). Ras-induced and extracellular signal-regulated kinase 1 and 2 phosphorylation-dependent isomerization of protein tyrosine phosphatase (Ptp)-Pest by Pin1 promotes fak dephosphorylation by ptp-pest. Mol. Cell. Biol. 31 (21), 4258–4269. Epub 20110829. doi:10.1128/mcb.05547-11
Zhou, B., Wang, G., Wen, Z., Zhou, Y., Huang, Y., Chen, Y., et al. (2018). Somatic mutations and splicing variants of focal adhesion kinase in non-small cell lung cancer. J. Natl. Cancer Inst. 110 (2), 195–204. doi:10.1093/jnci/djx157
Zhou, J., Roh, J-W., Bandyopadhyay, S., Chen, Z., Munkarah, A. R., Hussein, Y., et al. (2013). Overexpression of enhancer of zeste homolog 2 (Ezh2) and focal adhesion kinase (fak) in high grade endometrial carcinoma. Gynecol. Oncol. 128 (2), 344–348. doi:10.1016/j.ygyno.2012.07.128
Zhou, J., Roh, J. W., Bandyopadhyay, S., Chen, Z., Munkarah, A. R., Hussein, Y., et al. (2013). Overexpression of enhancer of zeste homolog 2 (Ezh2) and focal adhesion kinase (fak) in high grade endometrial carcinoma. Gynecol. Oncol. 128 (2), 344–348. Epub 2012/08/09. doi:10.1016/j.ygyno.2012.07.128
Keywords: FAK, cancer progression, clinical significance, molecular mechanisms, prognosis
Citation: Zhang Z, Li J, Jiao S, Han G, Zhu J and Liu T (2022) Functional and clinical characteristics of focal adhesion kinases in cancer progression. Front. Cell Dev. Biol. 10:1040311. doi: 10.3389/fcell.2022.1040311
Received: 09 September 2022; Accepted: 18 October 2022;
Published: 02 November 2022.
Edited by:
Wassim Abou-Kheir, American University of Beirut, LebanonReviewed by:
Pranshu Sahgal, Dana–Farber Cancer Institute, United StatesKeefe T. Chan, Peter MacCallum Cancer Centre, Australia
Copyright © 2022 Zhang, Li, Jiao, Han, Zhu and Liu. This is an open-access article distributed under the terms of the Creative Commons Attribution License (CC BY). The use, distribution or reproduction in other forums is permitted, provided the original author(s) and the copyright owner(s) are credited and that the original publication in this journal is cited, in accordance with accepted academic practice. No use, distribution or reproduction is permitted which does not comply with these terms.
*Correspondence: Tianzhou Liu, bGl1dGlhbnpob3VAamx1LmVkdS5jbg==
†These author share first authorship