- 1Regenerative Medicine Group, Research Institute Hospital 12 de Octubre (imas12), Madrid, Spain
- 2Pathology Department, Hospital Universitario 12 de Octubre, Madrid, Spain
- 3Obstetrics and Gynecology Department, Hospital Universitario 12 de Octubre, Madrid, Spain
- 4Male’s Integral Health Group, Urology Department, Research Institute Hospital 12 de Octubre (imas12), Hospital Universitario 12 de Octubre, Madrid, Spain
Stress urinary incontinence (SUI) is a condition that causes the involuntary loss of urine when making small efforts, which seriously affects daily life of people who suffer from it. Women are more affected by this form of incontinence than men, since parity is the main risk factor. Weakening of the pelvic floor tissues is the cause of SUI, although a complete understanding of the cellular and molecular mechanisms of the pathology is still lacking. Reconstructive surgery to strengthen tissue in SUI patients is often associated with complications and/or is ineffective. Mesenchymal stromal cells from the maternal side of the placenta, i.e. the decidua, are proposed here as a therapeutic alternative based on the regenerative potential of mesenchymal cells. The animal model of SUI due to vaginal distention simulating labor has been used, and decidual mesenchymal stromal cell (DMSC) transplantation was effective in preventing a drop in pressure at the leak point in treated animals. Histological analysis of the urethras from DMSC-treated animals after VD showed recovery of the muscle fiber integrity, low or no extracellular matrix (ECM) infiltration and larger elastic fibers near the external urethral sphincter, compared to control animals. Cells isolated from the suburethral connective tissue of SUI patients were characterized as myofibroblasts, based on the expression of several specific genes and proteins, and were shown to achieve premature replicative senescence. Co-culture of SUI myofibroblasts with DMSC via transwell revealed a paracrine interaction between the cells through signals that mediated DMSC migration, SUI myofibroblast proliferation, and modulation of the proinflammatory and ECM-degrading milieu that is characteristic of senescence. In conclusion, DMSC could be an alternative therapeutic option for SUI by counteracting the effects of senescence in damaged pelvic tissue.
1 Introduction
Stress urinary incontinence (SUI) is a pathological condition characterized by the involuntary loss of urine due to simple efforts such as sneezing, laughing or coughing (Norton and Brubaker, 2006). SUI is the most common form of incontinence worldwide and affects more women than men, with an estimated 35-fold higher prevalence in women of the same age groups (Maral et al., 2001). SUI is not only a medical problem but also a socio-economic problem, given the high percentage of the female population affected and the serious deterioration in their quality of life with global repercussions on personal and work life. The prevalence of SUI in the US is estimated to be 26% (Lee et al., 2021) while in European countries it ranges from 23% in Spain to 42% in the UK (Hunskaar et al., 2004). Annual SUI costs, including direct and indirect costs, are estimated to be close to €10 billion in Europe (Milsom et al., 2014), although the costs of diagnosis, treatment, and follow-up of patients with SUI vary depending on the countries (Zwolsman et al., 2019). The main risk factors for the appearance of SUI are pregnancy and vaginal delivery, although aging, decreased estrogen in menopause and obesity also predispose to SUI. Urinary continence is possible by virtue of a complex mechanism by which urethral resistance counteracts intravesical pressure. In SUI, urethral resistance is insufficient to remain closed and prevent urine leakage when intra-abdominal pressure increases, and this is thought to be primarily due to direct damage to the urethral sphincter and/or weakening of the supporting structures causing hypermobility of the urethra (Gill et al., 2010).
The connective tissue that houses the posterior wall of the urethra and the anterior wall of the vagina is part of the endopelvic fascia and consists of a dense extracellular (ECM) regulated by the resident fibroblasts (Herschorn, 2004). This connective tissue is described as functioning like a sling or a hammock where the urethra would be compressed as intra-abdominal pressure increases. A deficiency in this structure would prevent the complete closure of the urethra and could contribute to SUI (Ashton-Miller and DeLancey, 2007), and several studies have shown alterations in the metabolism of collagen and elastin in periurethral connective tissues of patients affected by SUI (Chen et al., 2006; Chen and Yeh, 2011). Aging, as well as the hormonal decay of menopause are known causes that lead to changes in the composition and structure of the ECM in the anterior wall of the vagina, resulting in mechanical alterations (Falconer et al., 1996; Lin et al., 2007) strongly related to pelvic floor disorders (Gardella et al., 2022). In pregnancy there is an intensive remodeling of the pelvic support structures to allow childbirth, and degenerative processes have been described that affect these structures as a consequence of mechanical stretching and ischemia in vaginal delivery (Ashton-Miller and Delancey, 2009). Placement of a sling made of synthetic or autologous materials to reinforce weakened native tissues is considered the gold standard for SUI surgery. However, this intervention is frequently associated with post-operative complications such as pain, voiding dysfunction, vaginal or bladder perforation, and failure in long-term subjective cure rates ranging from 43% to 92% (Ford et al., 2015).
Regenerative medicine, whose objective is the restoration or rejuvenation of tissues using endogenous or exogenous replacement stem cells, appears as a therapeutic alternative in SUI. Mesenchymal stem cells (MSC) are self-renewing adult stem cells that retain multilineage differentiation capacity. Their long-term in vitro expansion, homing capacity, and immunomodulatory and immunosuppressive effects have made them one of the most exciting sources for stem cell research and therapy (Torre et al., 2018; Merimi et al., 2021). MSC are able to replace cells in damaged tissue, although it is thought that they mainly exert their therapeutic action by a paracrine effect, that is, through secreted factors that will influence the response mechanisms of cells in the tissue (Jimenez-Puerta et al., 2020). There are many sources of MSC such as bone marrow, adipose tissue, or perinatal derivatives, among others (Ledesma-Martinez et al., 2016; Torre and Flores, 2020; Gao et al., 2021; Krawczenko and Klimczak, 2022). MSC transplantation has been tested in preclinical SUI models and has been shown to be safe, feasible, and effective in repairing tissue deficiencies (Sima and Chen, 2020; Maiborodin et al., 2022). MSC from different tissues have been tested in these preclinical models such as from bone marrow (Janssen et al., 2019), adipose tissue (Cui et al., 2018; Ni et al., 2018), dental pulp (Zordani et al., 2019), urine (Wu et al., 2019), and muscle (Bilhar et al., 2018; Cui et al., 2018). The safety of MSC transplantation has been sufficiently demonstrated in many phase I and II clinical trials (Rodriguez-Fuentes et al., 2021), both in autologous and in allogenic use, and there are several off-the-shelf MSC-based drug products approved by regulatory agencies in different countries (Levy et al., 2020). Periurethral injection of autologous adipose-derived MSC has been used for treatment of incontinence (Gotoh et al., 2020) and women (Kuismanen et al., 2014; Garcia-Arranz et al., 2020), proving to be a safe and effective treatment option. Among the perinatal derivatives that are source of MSC are the placenta, the umbilical cord and the amniotic membrane. The cells obtained from these perinatal tissues that are discarded after delivery, are unlimited, easy to obtain, and without ethical concerns (Silini et al., 2020). Perinatal cells obtained from the maternal layer of the term human placenta, i.e. the decidua, were phenotypically characterized as mesenchymal cells and named as decidua mesenchymal stromal cells (DMSC) (Macias et al., 2010). DMSC are a homogeneous cell population endowed with a remarkable capacity for proliferation and differentiation in culture (Macias et al., 2010; Cerrada et al., 2014; Serrano et al., 2021). These cells have been used in various animal models of disease where it has been shown that their therapeutic action is due to their migration capacity and inhibitory action on mammary tumor cells (Vegh et al., 2013), or to their immunomodulation capacity in the experimental model of autoimmune encephalomyelitis (EAE) (Bravo et al., 2016). Furthermore, DMSC are considered a safe product for allogenic uses given their hypoimmunogenic profile (Macias et al., 2010). DMSC have important advantages over adipose-derived MSC, which are the most commonly used MSC for UI. These advantages include availability, accessibility, easier to obtain, higher yield, naivety, and unique immune-modulatory properties (Macias et al., 2010; Hass et al., 2011; Silini et al., 2020; Flores et al., 2022). The aim of this study was to use DMSC to study their suitability as a treatment for SUI using in vivo and in vitro models. For in vivo studies, we have transplanted DMSC in the periurethral region of female rats that have been subjected to vaginal distention as a model of SUI caused by childbirth and we studied their effect on incontinence. For in vitro studies, we isolated suburethral connective tissue cells from patients with SUI and used them as an in vitro model to study the interaction between DMSC and these cells with the aim of identifying the mechanisms involved in DMSC-induced tissue repair.
2 Materials and methods
2.1 Animal model of stress UI caused by childbirth
Vaginal distention in female Sprague-Dawley rats was used to simulate the maternal injuries of childbirth. Animal experiments were carried out in compliance with the animal protection requirements and the approval of the Animal Welfare Ethics Committee at our institution. Twelve virgin female Sprague Dawley rats (Janvier Labs), 17 weeks old and weighing 300–350 g were used for this study. They were acclimatized for 14 days before starting the procedures in conditions of 12-h light-dark cycles. The rats were anesthetized using a combination of inhaled isoflurane and intraperitoneal ketamine-xylazine (37.5 and 5 mg/kg, respectively) and supplemented as needed. Vaginal distention (VD) was performed as previously described (Pan et al., 2007). Briefly, vagina was first accommodated using lubricated bouge dilators (24 F–32 F, Uterine Dilator Hegar, MEDICON). VD was performed via vaginal insertion of a modified lubricated 10-Fr Foley catheter and subsequent filling of the balloon with 2 mL of phosphate-buffered saline (PBS) for half an hour to let the vagina accommodate and then complete up to 3 ml. The rats were placed in anti-Trendelenburg position and the balloon was kept inside the vagina for 6 h to simulate childbirth injuries (Phull et al., 2011). Animals were monitored during the procedure using a pulse oximeter (UT100V Veterinary Pulse Oximeter; Utech Co., Ltd.). Before the end of the VD procedure and for two additional days, subcutaneous buprenorphine (.05 mg/Kg) was administered every 12 h for pain control. One week after VD, the animals were randomly distributed into two groups, control and DMSC-treated animals (n = 6 in each group). The treated group received two doses of DMSC resuspended in Hank´s balanced salt solution (HBSS) (2 × 106 DMSC per dose and 1 week apart) while control group received two injections of the same volume of HBSS without cells. DMSC/HBSS were administered into the periurethral region by bilateral injections at the 3 and 9 o’clock positions. UI was assessed by measurement of the leak point pressure (LPP). To evaluate DMSC´s engrafment, the cells were labeled with VivoTrack™ fluorescent labeling dye (PerkinElmer, Tres Cantos, Madrid, Spain) before injection. VivoTrack 680 is a near-infrared (NIR) fluorescent lipophilic dye that intercalates in the cell membrane. Viability and percentage of stained DMSC was tested before injecting the cells using a BD FACSCalibur instrument (Becton Dickinson, San José, CA, United States). VivoTrack-labeled DMSC were injected into the periurethral area and NIR fluorescent imaging was performed by Bruker In Vivo Xtreme animal imaging system (Bruker, bioNova científica, Madrid, Spain) once a week during 3 weeks.
2.2 Measurement of leak point pressure (LPP)
Under anesthesia with inhaled isoflurane (5% for induction; 2% for maintenance), the LPP measurement was conducted as previously described (Damaser et al., 2003). Briefly, a polyethylene catheter (PE-50 Instech Laboratories, Inc.) was inserted transurethrally into the bladder that was forced to emptying. Next, to determine its capacity, the bladder was filled with PBS mixed with methylene blue until urine leakage was observed at the urethra meatus. Three filling cycles were performed to calculate the average capacity. For the Leak Point Pressure (LPP) measurement, the bladder was filled to 50% of capacity with PBS plus methylene blue. The PE-50 catheter was connected to a data acquisition device (MP36R Research System, Biopac Systems Inc.) which was used in combination with an acquisition and analysis software (AcqKnowledge Software) to record the intravesical pressure. LPP was determined by manually applying abdominal pressure to record the minimum pressure exerted to cause leakage through the urethra (Damaser et al., 2003). LPP was measured three times for animal and the average pressure was considered.
2.3 Histological analysis
At the end of the study, urethras were harvested and placed in a formalin fixative solution for 48 h before embedding in paraffin. 4 μm slices were used for Hematoxylin and Eosin (H&E), Masson’s trichrome and elastin van Gieson (EVG) staining. Masson’s trichrome-stained slides were evaluated by two blinded investigators for semi-quantitative analysis of tissue integrity using a 1–4 grading scale ranging from significant tissue disruption to normal tissue, as previously described (Dissaranan et al., 2014). Two blinded investigators also used Masson’s trichrome-stained slides to determine the total number of muscle fibers in the external urethral sphincter.
2.4 Isolation and culture of cells
SUI patients, non-SUI patients, and pregnant women were recruited at the Department of Obstetrics and Gynecology under informed consent approved by the Ethics Committee. All steps of tissue processing were performed in a biosafety cabinet using appropriate aseptic techniques.
2.4.1 Isolation of cells from suburethral tissue from SUI and non-SUI patients
A total of 24 women with a median age of 56 (age range 43–69 years) with a diagnosis of mild to severe SUI were included in the study. Three gynecology patients without urinary incontinence were used as controls. Suburethral tissues were obtained by biopsy and cell cultures were established by overnight enzymatic digestion with 100 units/µL of collagenase type II (GIBCO). The cells were collected by centrifugation at 250xg for 10 min and washed twice with HBSS. The cellular pellet was resuspended in complete culture medium consisting of DMEM with 10% fetal bovine serum (Biowest, LabClinics), 1% non-essential aminoacids and 1% penicillin/streptomycin, and seeded in a 24-well plate. Cells were maintained at 37°C in 5% CO2 until passage 11–13.
2.4.2 Isolation and culture of decidua mesenchymal stromal cells (DMSC)
Human placentas were obtained during natural or cesarean births and decidua-derived mesenchymal stromal cells (DMSC) were isolated from placental membranes by trypsin/EDTA enzymatic digestion as we described previously (Macias et al., 2010). The phenotype of DMSC was characterized by flow cytometry as we described previously (data not shown) (Macias et al., 2010; Serrano et al., 2021). Isolated cells were cultured in DMEM supplemented with 10% fetal bovine serum, 2 mM glutamine, .1 mM sodium pyruvate, 55 μM β-mercaptoethanol, 1% non-essential amino acids, 1% penicillin/streptomycin, and 10 ng/ml epidermal growth factor (EGF). For the experiments, cells from different donors have been used individually in passages between 2 and 7.
2.5 Quantitative real-time PCR
RNA extraction was performed using NZY Total RNA Isolation kit (NZYTech, Lda., Lisboa, Portugal) according to the manufacturer’s protocol. RNA concentration and purity were determined by spectrophotometry in Nanodrop One (Thermo Fisher Scientific, Madrid, Spain) and .5 μg of the total RNA were retro-transcribed using the High Capacity cDNA Reverse Transcription kit (Applied Biosystem, Thermo Fisher Scientific, Madrid, Spain). Real-time PCR was performed using the ABI 7500 Fast Sequence Detection System (Applied Biosystems, Thermo Fisher Scientific, Madrid, Spain) and SYBR green qPCR Master mix (Promega Biotech Ibérica SL). The PCR primers and the size of the amplified products are in Table 1. TATA-binding protein (TBP) was used as an endogenous reference for normalization of the target gene quantity by mean of the differences of Ct or threshold cycle (dCt). The 2^-dCt indicates the relative amount of the target gene respect to the housekeeping, in each sample. The 2^-ddCt indicates fold-change of the gene expression respect to a basal condition.
2.6 Cell contraction assay
To study the ability of suburethral isolated fibroblasts to in vitro reorganize and contract collagen matrices we used the collagen-based Contraction Assay Kit (Cell Biolabs, bioNova, Madrid, Spain) following the manufacturer´s instructions but with some modifications. Briefly, 1 × 105 suburethral cells were suspended in 15 μl of DMEM and mixed with 80 μl of the collagen solution (1 × 106 cells/mL). The collagen/cell mixture was distributed into 96-well plates and incubated for 1 h at 37°C. Immediately after collagen polymerization, 180 μL of complete culture medium was added on top of each collagen gel lattice. Cultures were incubated for 16 h, allowing stress to develop. To initiate contraction, collagen gels were carefully detached from the edges of the culture wells with the help of a needle, releasing the accumulated mechanical load. The bottom of the culture plates containing the gels were scanned at different times after the release (Canon image RUNNER ADVANCE 4245i). The diameter of each gel surface was measured with the ImageJ program (National Institutes of Health, Bethesda, MA, United States). The contraction index is expressed as percentage of the total area of the well that is occupied by the collagen gel.
2.7 Senescence-associated beta galactosidase assay (SA-β-gal)
SA-β-gal assay was performed as we have described previously with some modifications (Torre et al., 2021). Briefly, cells were fixed with 2% formaldehyde (vol/vol) and 0.2% glutaraldehyde (vol/vol) for 5 min, washed twice with PBS, and stained with SA-β-gal solution overnight at 37°C. Next, cells were washed twice with PBS and visualized and photographed using a Leica DMIL microscope (Leica Microsistemas S.L.U., L’Hospitalet de Llobregat, Spain).
2.8 Immunofluorescence staining
Cells were seeded at density of 7 × 103 cells/well in 8-well Lab-Tek chamber slides (Nunc, Thermo Fisher Scientific, Madrid, Spain). After 48 h of culture, cells were fixed with 10% buffered-formalin for 15 min at room temperature, permeabilized with .5% Tween-20 in PBS for 10 min, and incubated with 5% horse serum to block non-specific binding. Primary antibodies (1:50 dilution) were incubated overnight at 4°C, washed with PBS twice and incubated with 1:200 dilution of fluorescein isothiocyanate (FITC)-conjugated or tetramethylrhodamine (TRITC)-conjugated secondary antibodies (Jackson Immunoresearch Laboratories, Vitro SA, Madrid, Spain). Nuclei were counterstained with .2 mg/ml 4′,6′diamidino-2-phenylindole (DAPI) (Sigma-Aldrich Quimica SA, Madrid, Spain) for 1 min. Cells were visualized by a Zeiss LSM 510 Meta Inverted Confocal Microscope (Carl Zeiss Meditec Iberia SAU, Madrid, Spain). The primary antibodies used were rabbit polyclonal IgG anti-αSMA (NBP1-35269, Novus Biologicals, bioNova científica, Madrid, Spain), rabbit monoclonal IgG anti-vimentin (NBP2-12472, Novus Biologicals, bioNova científica, Madrid, Spain), mouse monoclonal IgG1 anti-desmin (NB110-60508, Novus Biologicals, bioNova científica, Madrid, Spain) and mouse monoclonal IgG2b κ anti- Smoothelin (sc-376902, Santa Cruz Biotechnology, Quimigen S.L., Madrid, Spain).
2.9 Transwell migration assay
In vitro DMSC migration was determined using Millicell 24-well transwell Boyden chambers with 8 μm pore polycarbonate membranes (Merck Millipore, Spain) as we previously described (Vegh et al., 2013; Paris et al., 2016; Paris et al., 2017). Briefly, 2 × 105 DMSC in 100 μl of migration media (DMEM supplemented with 55 μM b-mercaptoethanol, 1% non-essential amino acids and without FBS) were seeded in the upper chamber of the transwell system and 5 × 104 suburethral tissue cells were seeded on the well below. Migration medium without cells was used as a negative control. The migration was assessed at 24 h by the CytoSelect 24-Well Cell Migration Assay (8 μm, Colorimetric, Cell Biolabs, bioNova, Madrid, Spain) following the manufacturer´s instructions. Migratory cells were stained with the Cell Stain Solution and the color was subsequently extracted with the Extraction Solution, and quantified by absorbance at 560 nm using an Enspire 2300 plate reader (PerkinElmer, Tres Cantos, Madrid, Spain). All experiments were done in triplicate.
2.10 Co-culture effect of DMSC on suburethral cell proliferation
To determine the in vitro effect of DMSC on the proliferation of suburethral cells, transwell culture inserts with .4 μm pore polycarbonate membranes and tissue cultured treatment (Merck Millipore, Spain) were used. Suburethral cells were cultured in 24 well plates at 3 × 104 cells per well and DMSC were seeded onto transwell inserts at 1.5 × 104 cells per insert. After 24 h, DMSC seeded onto transwell inserts were transferred to the 24-well plate of suburethral cells for co-culturing. As a control, suburethral cells without DMSC in inserts were prepared. Twenty 4 hours later, the inserts and the culture medium were removed and the viability of suburethral cells was evaluated by PrestoBlue cell viability assay (Invitrogen, Fisher Scientific, Madrid, Spain) following the manufacturer’s instructions. Briefly, 200 μl of fresh medium containing .1 mg/mL of PrestoBlue was added to the wells that were incubated for 1 h. Then, 100 μl of this medium was transferred to a black flat-bottom plate for fluorescence readings at 570 nm and 600 nm using a plate reader (Enspire 2300; PerkinElmer, Tres Cantos, Madrid, Spain). Assays were performed in triplicate, and at least twice with cells isolated from five different patients. Fluorescence of cocultured suburethral cells was expressed as a percentage relative to the fluorescence of suburethral cells cultured without DMSC which was set as a value of 100.
2.11 Multiplex assay to measure the co-culture effect of DMSC on suburethral cell secretion
To determine the in vitro effect of DMSC on the secretome of suburethral cells, Transwell culture inserts with .4 μm pore polycarbonate membranes and tissue cultured treated (Merck Millipore, Spain) were used. Suburethral cells were cultured in 24 well plates at 3 × 104 cells per well and DMSC were seeded onto Transwell inserts at 1.5 × 104 cells per insert. After 24 h, DMSC seeded onto Transwell inserts were transferred to the 24-well plate for co-culturing with suburethral cells in DMEM supplemented with 2% FBS. As controls of the secretion, 3 × 104 suburethral cells and 1.5 × 104 DMSC were grown as monocultures and fresh medium was added as described above twenty four hours later, the inserts were removed and the cell supernatant containing secreted proteins was collected and centrifuged at 10.000xg for 15 min to remove cell debris. The secretome of suburethral cells co-cultured with DMSC, suburethral cells alone and DMSC alone was evaluated by a bead-based immunoassay according to manufacturer’s protocol (ProcartaPlexTM Multiplex Immunoassay, InvitrogenTM Life Technologies, Labclinics, Madrid, Spain) for the following soluble factors: interleukin (IL)-6, IL-8, hepatocyte growth factor (HGF), monocyte chemoattractant protein (MCP)-1, MCP-3, vascular endothelial growth factor (VEGF)-A, stromal cell-derived factor (SDF)-1, matrix metalloproteinase (MMP)-2, MMP-3, and MMP-9.
2.12 Statistical analysis
Data were analyzed using GraphPad Prism software v6.0 (GraphPad Software, San Diego, CA, United States). The data were expressed as the mean ± SD. Inter-group statistical significance was performed with the unpaired Student’s t-test and mean was compared by Student’s t-test, Mann-Whitney U-test, and Wilcoxon tests as appropriate. In all experiments a p < .05 was considered statistically significant.
3 Results
3.1 Effects of DMSC on urodynamic parameters and urethral histology in a rat model of SUI
We have evaluated the regenerative role of DMSC in rats after VD. In order to maximize the damage inflicted by the distention as well as the duration of the effects, a time extended procedure has been performed as previously described (Pan et al., 2007). Baseline LPP values were determined during the 2 weeks prior to VD and the evolution of the damage was analyzed by measuring the LPP during the 6 weeks after VD (Figure 1A). To avoid bias, weekly measurements were carried out without knowing which group each individual belonged to, simulating the “double blind” technique of clinical trials. The consequence of VD was a progressive decrease in the LPP value that was evident 2 weeks after injury in control animals, i.e., those injected with HBSS (1st PI, Figure 1B, white circles), which became significant from the third week after VD or second week post-injection until the end of the study (2nd PI, Figure 1B, white circles). However, the two DMSC injections after injury prevented the decrease in LPP respect to baseline values in the treated group of animals, as shown in Figure 1B (black squares). LPP values were significantly different between DMSC-treated and control animals from the second week after the first injection (1st PI, Figure 1B) suggesting that DMSC treatment prevents loss of continence or induces recovery of continence in rats with SUI.
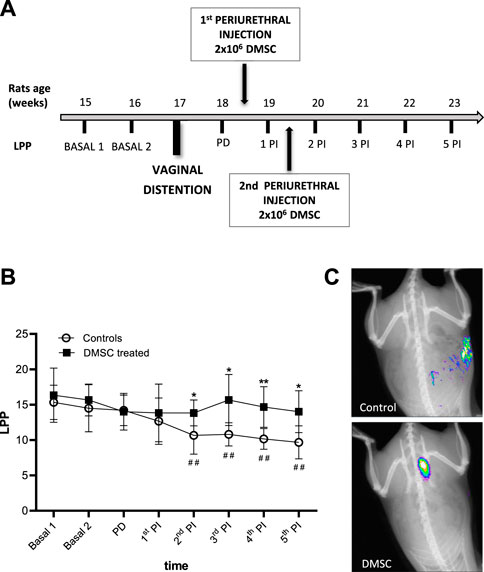
FIGURE 1. Periurethral injection of DMSC markedly prevented fall of abdominal leak point pressure (LPP) or enhanced LPP in rats after vaginal distention (VD). (A) Flowchart of the design of measurement of LPP, vaginal distention and treatment with DMSC. Basal 1 and Basal 2 are LPP measurements from the 2 weeks prior to VD. Post-damage (PD) is the LPP measurement after VD and before injection. Post-injection (PI) are LPP measurements after the first injection of DMSC or HBSS (controls). (B) Leak point pressure (LPP) measurement in DMSC-treated and control animals. DMSC prevents the drop of leak point pressures in VD rats while in control rats there is a significant drop in LPP from the third week after VD or second week post-injection. Data are shown as mean ± standard deviation (SD). *p < .05; **p < .01; ##p < .01. Asterisks correspond to the comparison of DMSC-treated vs. control animals at each time point. The hashtag symbol indicates the comparison of control animals with respect to their baseline. (C) Examples of in vivo fluorescence images of rats transplanted with NIR-labelled DMSC or injected with HBSS (controls) 14 days after VD.
To assess the homing and engrafment of DMSC in the injected area, cells were stained with a near-infrared (NIR) fluorescent dye prior to injection, which allowed their visualization by means of an in vivo imaging system. The images showed that the DMSC remain in the ventral area for at least 14 days after the second injection, this is around 10 days before the end of the study (Figure 1C). At the end of the study, immunofluorescence analysis of urethral cross-sections using an anti-human mitochondria antibody failed to detect the presence of DMSC (data not shown). By H&E-staining of urethral sections, we did not identify any sign of inflammation due to DMSC injection suggesting that the cells are well tolerated and no adverse reactions occur, although the animals used in this study are immunocompetent (Figure 2A). To explore the mechanisms that might contribute to DMSC-mediated recovery of continence, we carried out further histological analysis of urethras using Masson’s trichrome and EVG staining. As is evident in Figure 2A, the VD involved extensive rupture of the muscle fibers of the external urethral sphincter (EUS) with marked infiltrates of ECM, as well as atrophy of elastic fibers near the EUS. Interestingly, it is shown that DMSC treatment resulted in a significant increase in the integrity (Figures 2A,B) and the number of muscle fibers in the EUS (Figures 2A,C). Furthermore, EUS showed less infiltration of ECM and the presence of larger elastic fibers in DMSC-treated animals (Figure 2A).
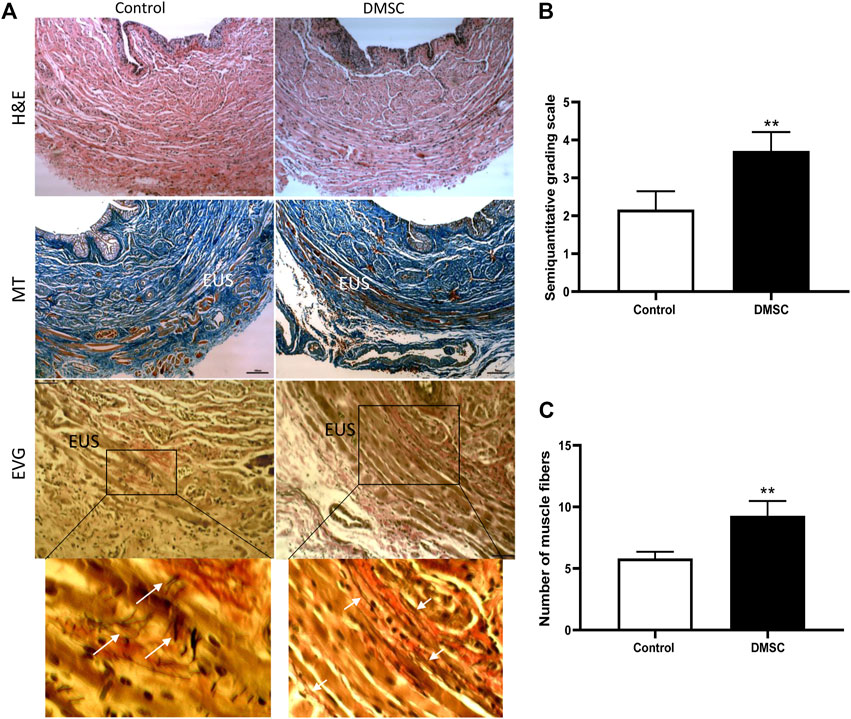
FIGURE 2. Periurethral injection of DMSC reverses VD-induced muscle and connective tissue disruption in the urethra. (A) Histological staining of urethral sections after VD and DMSC or HBSS (Control) treatment. Examples of urethral cross-sections stained with haematoxylin/eosin (H&E; upper panels), Masson´s trichrome (MT; middle panels) or elastin von Giesson (EVG; lower panels) 6 weeks after VD. EUS: external urethral sphincter. Inset in EVG panels is shown in higher magnification in the panels placed just below. Scale bar: 100 μm. White arrows indicate disorganized elastin fibers in Control-EVG examples while elastin fibers in DMSC-EGV examples are more organized. (B) Semi-quantitative analysis of Masson’s-trichrome-staining using a grading scale showing that the urethra of rats in the DMSC-treated group appeared to have more complete muscle fibers in the EUS than those in the control group. (C) Analysis of total number of muscle fibers in EUS in DMSC-treated and in control animals showed significant differences between the two groups. Data of B and C are shown as mean ± standard deviation (SD) and correspond to the comparison of DMSC-treated vs. control animals. **p < .01.
3.2 Characterization of fibroblast cells isolated from SUI patients
To deepen into the cellular and molecular mechanisms by which DMSC exert their effect on the recovery of continence, an in vitro model was carried out using cells obtained from SUI patients. The cells were isolated from the suburethral tissue and expanded in culture. They grow on plastic as adherent fibroblast-like cells (Figure 3A) with an estimated doubling time of 72 h (data not shown). Gene and protein expression analysis, showed that SUI cells expressed alpha smooth muscle actin (α-SMA), vimentin, desmin and smoothelin, suggesting that they are myofibroblasts (Figures 3A,B). Interestingly, immunofluorescence staining showed that cells positive for α-SMA were also positive for smoothelin (results not shown). The expression of other myofibroblast markers such as podoplanin, tenascin, cadherins and collagen I, further confirmed the presence of myofibroblasts in suburethral cells isolated from SUI patients. To analyze whether myofibroblast differentiation could be associated to pelvic floor pathology, we carried out the characterization of cells from the suburethral tissue of patients without SUI (non-SUI) or other pelvic floor disorder. We found that these non-SUI cells expressed most of the myofibroblast markers previously detected in SUI cells, except for desmin (Supplementary Figure S1). Desmin expression was extremely low or almost undetectable (appearing above cycle 36 in qPCR) in non-SUI cells. When the expression of the other myofibroblast genes was corrected to the housekeeping gene (2^dCt), we found that it was higher in SUI cells, except for collagen that was higher in non-SUI cells, although the differences were not statistically significant (data not shown).
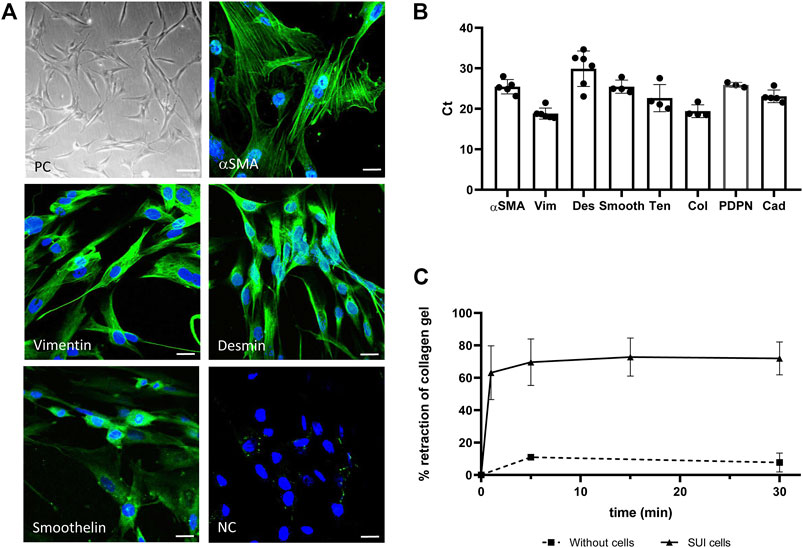
FIGURE 3. Characterization of primary suburethral myofibroblasts cultures. (A) Representative photomicrograph of suburethral myofibroblasts isolated from SUI patients. Cells are fibroblast-shaped as shown in phase contrast (PC) panel and express several markers of myofibroblast, such us α-SMA, vimentin, desmin and smoothelin (in green) as shown in confocal microscopy images. NC is the negative control of immunofluorescence. Bar = 100 μm in PC panel and 22 μm in immunofluorescence panels. (B) Gene expression of myofibroblast markers was determined by real-time qPCR. The analyzed cells correspond to passage 3. Data are presented as mean ± standard deviation (SD). Gene abbreviations: α-SMA, alpha smooth muscle actin; Vim, vimentin; Des, desmin; Smooth, smoothelin; Ten, tenascin; Col, collagen I, PDPN, podoplanin; Cad, Cadherin. (C) Retraction of a collagen lattice by suburethral myofibroblasts. Evolution of the diameter of collagen gel surface incubated with suburethral myofibroblasts (triangles) or without cells (squares) once the gel is detached from the edges of the culture wells. Data is calculated as the area of the well that is not occupied by the collagen gel after detachment respect to the total area of the well, and expressed as percentage.
Contractility is a property of myofibroblasts and we determined by a collagen-based contraction assay whether SUI myofibroblasts, coming from a damaged tissue, maintained their functionality. We calculated the area of the well that is occupied by the collagen gel A) respect to the total area (A0) at different time points and expressed the contraction index as [1-(A/A0)]*100 (Figure 3C). Using this approach, we observed that SUI cells contracted the matrices by 60% within the first minute of release and reaching a maximum of 80% retraction within 5 minutes of release (Figure 3C). While contraction was significantly increased in the presence of SUI fibroblasts, control matrices without cells had no contraction. These results confirmed that SUI cells had the contractile phenotype of myofibroblast.
Growth of SUI myofibroblast cultures was analyzed (Figure 4A). Cells at different passages were counted and the number of accumulated cells was calculated. An exponential growth was observed until passage 10 when SUI cells almost stopped dividing. Interestingly, cells isolated from suburethral tissue of non-SUI patients continued their exponential growth beyond passage 10, and the number of cells accumulated was significantly higher from passage 12 onwards (Figure 4A). Morphological changes associated with a senescent phenotype, such as flattened and enlarged cells, were visible in high-passage SUI myofibroblast cultures compared to cells from no incontinent women (non-SUI cells) (Figure 4B). SUI cells exhibited significantly more cells positive for the marker of senescence SA-β-gal. (Figure 4B). It is remarkable the presence of binucleated cells and other abnormalities associated with aging affecting the morphology of the nuclei in SUI SA-β-gal positive cells such as enlargement, irregular shape, invaginations, blebbing in nuclear margin, and even total loss of structure (Figure 4C). Furthermore, another feature of senescence in late passage SUI cells was the significant upregulation of the genes encoding p16 and p21 cell cycle regulators, CDKN2A and CDKN1A, compared to early passage (Figure 4D).
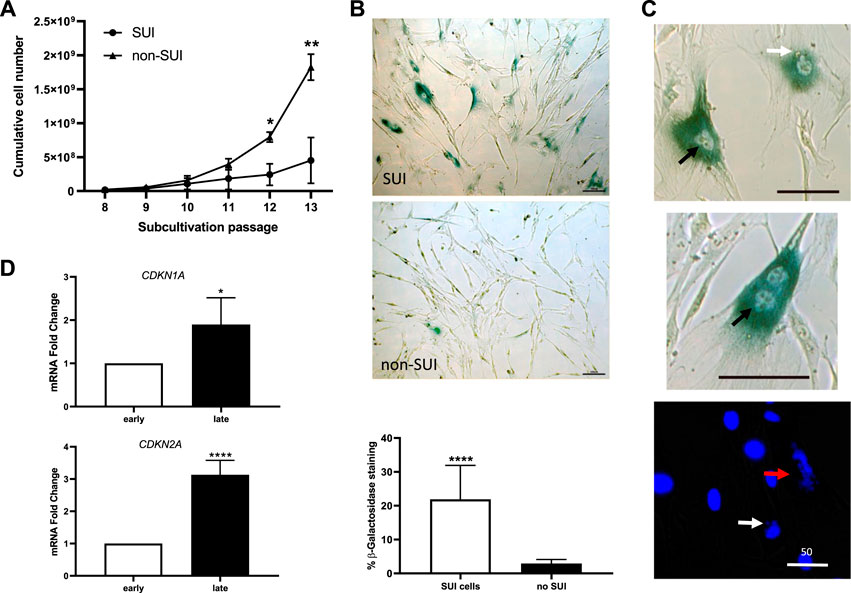
FIGURE 4. Suburethral myofibroblasts presented a limited cell proliferation and premature senescence. (A) Cells from SUI myofibroblast cultures (n = 5) or non-SUI (n = 3) fibroblast cultures were counted at each passage and the cumulative cell number was determined. Error bars are SD of mean; (*p < .05, **p < .01). (B) Representative photomicrograph of blue-colored staining for SA-β-gal activity in cells from SUI myofibroblast cultures or non-SUI cultures. The images correspond to cell passage 9. The graphic represents the percentage of SA-β-gal positive cells (blue) cells calculated respect to the number of total cells found in 10 and 15 fields of SUI (n = 2) and non-SUI (n = 3) cell cultures, respectively. (C) Analysis of senescence markers in SUI myofibroblast in early and late passages, 3 and 11, respectively. Change in CDKN1A and CDKN2A mRNA expression (n = 5). Error bars are SD of mean; (*p < .05, ****p < .0001). (D) High magnification of senescent cells found in SUI cultures, showing irregular nuclei (black arrow, upper panel), blebbing nuclei (white arrow, upper and lower panel), broken cell nucleus (red arrow), or binucleate cells (middle panel). Bar = 100 micras, except where specified to be 50 micras.
3.3 Effect of co-culture on the proliferation, migration and paracrine response of DMSC and suburethral myofibroblast cells from SUI patients
To identify the mechanisms that could be involved in the regenerative action of DMSC in SUI pathology, we performed several in vitro assays to study the interaction between SUI myofibroblast and DMSC. First, we tried to find out whether SUI myofibroblasts exert in vitro chemoattraction on DMSC, given that, as we previously reported using in vitro and in vivo studies, DMSC migrate and home at sites of injury (Vegh et al., 2013; Paris et al., 2016). As it is shown in Figure 5A, our results indicate that DMSC migrated significantly towards SUI myofibroblasts compared to their migration towards complete culture media used as a negative control.
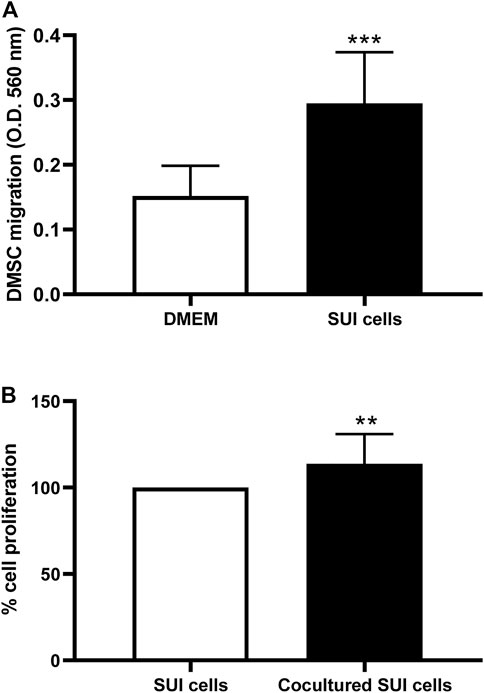
FIGURE 5. Co-culture of DMSC and SUI myofibroblasts has effects on the behavior of both cell types. (A) In vitro transwell migration assay of DMSC toward suburethral myofibroblasts or culture medium. Suburethral myofibroblasts enhanced the migration ability of DMSC in vitro. Data is expressed as mean ± SD, n = 5 in duplicate, ***p < .001. (B) Proliferation of suburethral myofibroblasts was determined by Presto blue assay after co-culture with DMSC seeded on Transwell® culture inserts. DMSC enhanced the proliferation of suburethral myofibroblast in vitro. The cells analyzed correspond to passages 5–6. Data is expressed as Mean ± SD, n = 5, **p < .01.
To study the soluble factors secreted by SUI myofibroblasts that might be involved in the DMSC migration, we used a multiplex immunoassay. The results showed that the secretome of SUI myofibroblasts contained several chemokines known to regulate the recruitment of immune cells during the inflammatory phase of wound healing, such as SDF-1, MCP-1, and MCP-3 (Table 2). These results would suggest that suburethral myofibroblast obtained from patients with SUI probably present some type of damage that would give rise to a pro-inflammatory environment capable of promoting the migration and homing of DMSC.
Next, to assess whether DMSC exert their effect through a paracrine action, that is, through the secretion of soluble factors that could alter the behavior of SUI myofibroblasts, transwell co-cultures between SUI myofibroblast and DMSC were established. We first analyzed whether DMSC have an effect on the proliferation of SUI myofibroblasts (Figure 5B). It was evident that co-culture with DMSC significantly induced SUI myofibroblast proliferation compared to the cells cultured alone (Figure 5B). Cytokines and growth factors in the secretome of the SUI and DMSC cocultures, as well as of both separate cell cultures, were analyzed by multiplex protein assay (Figure 6). This assay showed that co-culture of SUI myofibroblasts and DMSC resulted in a significant decrease in the levels of the pro-inflammatory cytokines IL-6 and IL-8 when expressed as a ratio respect to their secretion by both cell types cultured separately (Figure 6A). Likewise, a significant decrease was observed in the levels of two angiogenesis-related growth factors, such as HGF and VEGF-A, and of the chemoattractant proteins MCP-1 and MCP-3 (Figure 6A). MMP-2, MMP-3, and MMP-9 were also found to be significantly decreased in DMSC and SUI myofibroblast co-culture when analyzed relative to the levels found in both cell types when cultured separately (Figure 6B). MMPs are enzymes that degrade extracellular matrix proteins and are also involved in various cell functions, such as cell proliferation, migration, differentiation, apoptosis, angiogenesis, and host defense. All factors analyzed have been associated with the cellular senescence phenotype and linked to the development of diseases. Our results suggested that DMSC would ameliorated SUI through a paracrine action acting on the proliferation and the secretion of senescence-related factors by SUI myofibroblasts.
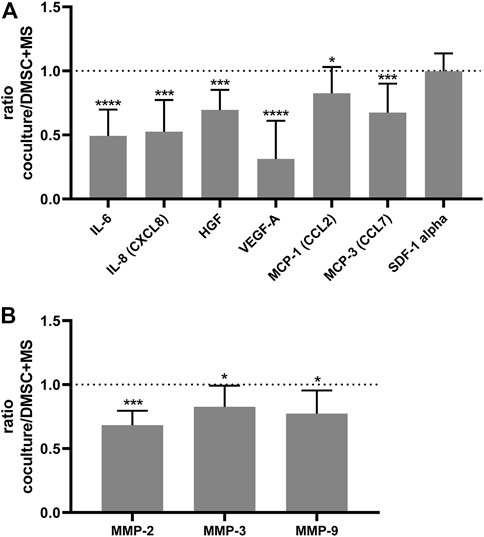
FIGURE 6. DMSC exert a paracrine action that modulates the secretion of soluble factors by SUI myofibroblasts. (A) Ratio of the amount measured by Luminex of IL-6, IL-8, HGF, MCP-1, MCP-3, and VEGF in the coculture of DMSC and suburethral myofibroblast cells relative to the amounts in the monocultures (n = 6). (B) Ratio of the amount measured by Luminex of MMP-2, MMP-3, and MMP-9 in the co-culture of DMSC and suburethral myofibroblast cells relative to the amounts in the monocultures (n = 5). The cells analyzed correspond to passages 5–6. These factors are involved in inflammation, angiogenesis, ECM-remodeling, and chemoattraction.
4 Discussion
Several mechanisms have been described by which childbirth predisposes women to develop pelvic floor pathologies, including mechanical damage that affects the pelvic floor support systems, denervation, ischemia and reperfusion injury, and defective soft tissues remodeling, among others (Memon and Handa, 2013). VD in rats is a validated animal model of birth-related trauma that approximates the consequences of vaginal delivery on pelvic support tissues and loss of continence in women (Cannon et al., 2002). In these animal model, it is described that the inflation of a balloon to distend the vagina causes damage to nearby organs and tissues over a prolonged period of time (Hijaz et al., 2008). VD produces a decrease in blood flow and hypoxia in the urogenital organs, that is bladder, urethra and vagina (Damaser et al., 2005), as well as important histological abnormalities such as a reduction in the content of collagen, disorganization and fragmentation of elastic fibers, and a shortening of striated muscle fibers (Wang et al., 2011; Dissaranan et al., 2014). Functionally, VD produces a decrease in urethral resistance and development of SUI (Cannon et al., 2002). Although VD is a good model to recapitulate the birth injury, this animal model has the limitation that the functional, structural and biomechanical defects have a short durability, and some kind of recovery occurs between 10 days and 6 weeks, depending on the time of VD (Herrera-Imbroda et al., 2015). For that reason, we have used a 6-h VD procedure to provide maximum damage (Pan et al., 2007) and placed the animals in a reverse Trendelenburg position so that the pressure of the inflated balloon within the vagina simulates delivery (Phull et al., 2011). Our results show that 6 weeks after VD, LPP has not yet recovered in control animals. It would be very interesting to carry out additional studies in the future to see the long-term effects of both the damage and the therapeutic effect of DMSC.
In this study we have evaluated the regenerative role of perinatal mesenchymal stromal cells obtained from the decidua (DMSC) in the described rat VD model. Our results showed that periurethral transplantation of DMSC 1 week after prolonged VD preserved urethral functionality by preventing LPP drop, which was however significant in untreated animals from the second week after injury. Labeled DMSC were detected in the lesion for up to 14 days, but there was no signal thereafter, probably due to limited survival of xenotransplanted cells in immunocompetent animals (Dai et al., 2015). Histological analysis of the urethras at the end of the study, i.e. six weeks after VD, showed that DMSC treatment increased the integrity of the muscle fibers of the EUS, in which little ECM infiltration and larger elastic fibers were found, compared with the extensive disruption of the muscle fibers found in the EUS of untreated animals. MSC isolated from muscle (Cui et al., 2018; Bortolini et al., 2019), urine (Wu et al., 2019), and adipose tissue (Cui et al., 2018; Ni et al., 2018) have also been used experimentally in the VD animal model of SUI, showing that MSC induced morphological and histological regeneration and improved urinary function in rats through a paracrine process (Sima and Chen, 2020). This paracrine effect could be stimulated, at least in part, by the hypoxic microenvironment generated by the VD. It has been demonstrated that hypoxia stimulates the paracrine action of MSC, their proliferation and migration (Yang et al., 2022). To our knowledge, this is the first demonstration of the use of perinatal MSC in the treatment of experimental SUI.
To understand the mechanisms of action underlying the therapeutic effects and in vivo behavior of DMSC, we have used an in vitro model based on cells isolated from suburethral tissue of SUI patients. First, we have characterized these SUI-isolated cells to better understand the physiopathology of SUI and, second, we have studied their interaction with DMSC when cocultured. Cells isolated from SUI patients are adherent fibroblast-like cells with an estimated doubling time of 72 h. According to expression analysis we conclude that suburethral SUI cells are myofibroblasts expressing several characteristic markers such as the α-SMA, and the cytoskeleton proteins, vimentin, and desmin. While α-SMA expression is considered the most reliable marker of myofibroblasts (Serini and Gabbiani, 1999) and vimentin is an intermediate filament of stromal cells, desmin expression has been associated with the presence of lesional myofibroblasts (Skalli et al., 1989). The colocalization of vimentin and desmin has been previously described in the immunohistochemical analysis of vaginal wall tissue from patients with SUI (Wen et al., 2012). Here we have also found that SUI myofibroblast express other myofibroblast markers such as smoothelin, podoplanin, tenascin, cadherin and collagen I. This is the first study to show the expression of these myofibroblast markers in SUI cells although their role in the physiopathology of SUI needs to be addressed. All these genes are expressed in myofibroblasts of several chronic diseases associated with fibrosis and inflammation (Aneiros-Fernandez et al., 2011; Darby et al., 2014; Kasprzycka et al., 2015; Quintanilla et al., 2019; Chen et al., 2021). Here we have shown co-localization of α-SMA and smoothelin in SUI myofibroblast. Myofibroblasts that co-express α-SMA and smoothelin (Lepreux et al., 2013), podoplanin (Braun et al., 2011; Nazari et al., 2016; Enomoto et al., 2018; Hirayama et al., 2018), cadherin (Hinz et al., 2004; Bowler et al., 2018), tenascin (Katoh et al., 2020) or collagen I (Zhang et al., 1994) have been found during the fibroblast-to-myofibroblast transition and are associated with advanced stages of fibrosis in several diseases. All these myofibroblast markers have also been found in the control group of women, as described by others for women operated on for other gynecological indications (Ruiz-Zapata et al., 2020). A limitation of the present work is the low number of not incontinence patients, so these results need to be confirmed in the future with a higher number of samples. Furthermore, it is noteworthy that the women in the control group were not incontinent but were not very different to the SUI group in terms of parity, and vaginal deliveries. The endopelvic fascia contains fibroblasts, myofibroblasts, and other cell types, and the structural features of this tissue along with the molecular composition of the ECM are responsive to local mechanical forces (Siccardi and Valle, 2022). It has been described the presence of myofibroblasts with contraction capacity in different fascial tissues under normal conditions. These myofibroblasts regulate fascial tension and probably influence the dynamics of the supported muscle tissues (Schleip et al., 2019). Furthermore, myofibroblasts derive from the tissue fibroblasts in response to injury. The fibroblast-to-myofibroblast transition is a well-known hallmark of the pathological state of tissues and is closely related to ECM remodeling processes (D'Urso and Kurniawan, 2020). Changes in the molecular composition and/or in the mechanical properties of the ECM induce alterations in various cellular functions such as migration, proliferation, differentiation and apoptosis (Jaalouk and Lammerding, 2009). We suggest that the myofibroblast differentiation in the suburethral tissue of patients with incontinence may be part of response mechanisms to tissue damage and ECM remodeling processes after childbirth, menopause and/or tissue aging. In other pathology of the pelvic floor, pelvic organ prolapse (POP), which is etiologically related to incontinence, it has been seen that the stiffness and composition of the ECM regulate the differentiation of vaginal myofibroblasts (Ruiz-Zapata et al., 2020). Monitoring the growth of SUI myofibroblasts in culture we observed that they almost stopped dividing around the passage 10, whereas suburethral cells isolated from non-incontinent women continued to grow exponentially until at least passage 13. SUI myofibroblasts around passage 10 showed positivity for senescence-associated beta-galactosidase staining as well as increased expression of genes encoding p16 and p21, confirming the aging of SUI cells (Del Rey et al., 2019). Additionaly, the aging of the SUI culture was evident by nuclear morphological anomalies previously described as senescence hallmarks (Alicka et al., 2020; Pathak et al., 2021). Premature cellular senescence has been described as a cause of POP (Huang et al., 2020), and has also been implicated in other health issues such as miscarriage (Torre et al., 2021) or rheumatoid arthritis (Del Rey et al., 2019). Based on our observations regarding SUI cells behavior in culture we agree with other authors that cellular senescence may be one of the main pathological mechanisms underlying SUI (Alperin et al., 2022). Cellular senescence is a process that can be caused by mechanical stress (Liu et al., 2022), oxidative stress (Del Rey et al., 2019) or estrogen depletion (Wei et al., 2021), among others, and contributes to tissue aging since senescent cells negatively affect nearby cells and surrounding tissues through paracrine mechanisms. To our knowledge, this is the first demonstration of cellular senescence mechanisms associated with SUI.
SUI myofibroblasts were co-cultured with DMSC using the transwell assay to understand the molecular mechanisms of this interaction. Our results suggest that there is a paracrine interaction between DMSC and SUI myofibroblasts through soluble mediators, causing changes in their cellular behavior and secretome. DMSC induced SUI myofibroblast proliferation, as observed by the significant increase in metabolic activity of these cells cocultured with DMSC compared to SUI myofibroblasts cultured alone. This effect may be one of the therapeutic mechanisms by which DMSC enhance tissue repair of pelvic floor support tissues in vivo. In other published study, the culture medium of MSC isolated from bone marrow induced the proliferation of fibroblasts isolated from the anterior vaginal wall of patients with SUI (Jiang et al., 2021). Similarly, the curative effect of low-intensity extracorporeal shock wave therapy in patients with SUI (Lin et al., 2021) is attributed, among others, to a cell proliferation effect as observed in the SUI animal model induced by VD (Wu et al., 2018). Furthermore, in this cell-to-cell communication, DMSC were found to be strongly attracted to SUI cells when co-cultured. Indeed, we determine that the secretome of SUI cells contains several chemokines, such as SDF-1, MCP-1, and MCP-3 which act as chemoattractants towards immune cells during tissue repair process. Similar to leucocytes, MSC express many receptors and cell adhesion molecules that respond to chemokines released from tissues and are involved in their homing. SDF-1 and MCP-1 are some of the main chemokines that exert chemoattraction on MSC in general (Ghaffari-Nazari, 2018), and have also been identified as chemoattractants on decidua MSC (Abumaree et al., 2016). Furthermore, MCP-3 has been recognized in vitro and in vivo as a homing factor of bone marrow MSC to injured myocardium (Schenk et al., 2007). We have already shown that the therapeutic action of DMSC strongly relies on their ability to migrate into injured tissues (Vegh et al., 2013; Paris et al., 2016), and these chemokines may be responsible for the migration effect induced by SUI myofibroblast. Secretome analysis of SUI and DMSC myofibroblast co-culture by multiplex showed a significant reduction in the secretion of MCP-1 and MCP-3, and no change in SDF-1 respect to the amount secreted by these cells when grown in monoculture, suggesting a paracrine interaction between both cell types. Other authors have published in vivo data reporting the relevance of these chemokines in incontinence. MCP-3 has been found significantly over-expressed in urethral and vaginal tissues immediately following VD (Woo et al., 2007), and MCP-1 has been determined to be a predictive biomarker of urinary incontinence in males undergoing prostatectomy (Liss et al., 2016). In contrast, SDF-1 was significantly underexpressed in urethral and vaginal tissues after VD (Woo et al., 2007), but it has been successfully evaluated as an experimental therapy for SUI in rats after VD based on its role in neovascularization and chemotaxis of stem cells (Khalifa et al., 2020).
The coculture of DMSC and SUI myofibroblasts also showed downregulation in the secretion of IL-6, IL-8, HGF, and VEGF. Upregulation of IL-8, VEGF, MCP-1, MCP-2, or MCP-3 has been found in the female genitourinary tract immediately after VD (Wood et al., 2008). These cytokines and chemokines are secreted in inflammatory processes, but they are also part of what is known as SASP or senescence-associated secretory phenotype (Coppe et al., 2010; Lopes-Paciencia et al., 2019). SUI, as other pelvic floor disorders, is not considered an inflammatory disease but rather a multifactorial chronic disorder related to tissue deterioration. The secretion of SASP components, due to their proinflammatory effect, can be detrimental to the tissue giving rise to a degenerative phenotype and favoring the progression of the disease. For this reason, other authors have stated that both, UI and POP, are the result of degenerative changes in the tissues of the pelvic floor (Radziszewski et al., 2005; Sima and Chen, 2020), which strongly limits their ability to regenerate.
Likewise, we observed a significant downregulation of secreted MMP-2, MMP-3, and MMP-9 in our coculture study. Closely associated with cellular senescence is ECM remodeling, a process with consequences on tissue homeostasis. These phenomena feed off each other since some of the molecules that make up the SASP are MMPs or enzymes that degrade the ECM (Coppe et al., 2010; Lopes-Paciencia et al., 2019), and abnormal ECM remodeling is thought to lead to cellular senescence (Blokland et al., 2020). There is also in vivo data on the implication of ECM-degrading enzymes in incontinence, as increased MMP-2 and MMP-9 activity was reported in the vaginal wall after VD (Rahn et al., 2008). These MMPs degrade collagen, collagen fragments and elastin and if their expression is altered, an abnormal degradation of the ECM occurs (Cabral-Pacheco et al., 2020). A decrease in collagen and elastin content has been described in the pelvic tissue of SUI patients, suggesting that alterations in connective tissue metabolism are involved in the onset of SUI (Pantatosakis et al., 2016).
5 Conclusion
Periurethral injection of two doses of DMSC prevented loss of continence in rats after VD, which is an animal model that reproduces SUI caused by childbirth. Despite of the absence of prolonged DMSC engraftment, they were able to reverse the VD-induced disruption of muscle and connective tissues in the urethra, probably by paracrine mechanisms. Characterization of cells isolated from suburethral tissue of patients with SUI has reported the presence of myofibroblasts, a cell related to tissue injury that reached premature senescence in the culture. In coculture, SUI myofibroblasts significantly increase DMSC migration, and DMSC induced SUI myofibroblasts to proliferate. Currently, the molecular mechanisms underlying SUI remain unknown, but it is very likely that alterations in ECM are at the origin, since parity and aging, the main risk factors for SUI, are characterized by intense ECM remodeling. Changes in the molecular composition and/or mechanical properties of the ECM affect cellular functions and can lead to cellular senescence. Senescent cells are characterized by a permanent cell growth arrest and a senescence-associated secretory phenotype or SASP, which includes the secretion of proinflammatory and ECM-degrading factors that can be harmful to tissues. Coculture of DMSC and SUI myofibroblasts resulted in downregulation of several SASP factors. Based on these results, we hypothesized that DMSC could be a therapeutic option for SUI by counteracting the effects of senescence in damaged pelvic tissue through migration to the injured tissue, stimulation of cell proliferation, and modulation of the secretion of SASP components, which would allow the recovery of tissue homeostasis.
Data availability statement
The raw data supporting the conclusion of this article will be made available by the authors, without undue reservation.
Ethics statement
The studies involving human participants were reviewed and approved by Comité de Ética de la Investigación con medicamentos (CEIm) from the Instituto de Investigación Hospital 12 de Octubre (imas12). The patients/participants provided their written informed consent to participate in this study. The animal study was reviewed and approved by Dirección General de Agricultura, Ganadería y Alimentación. Consejería de Medio Ambiente y Ordenación del Territorio. Comunidad de Madrid.
Author contributions
PD, MJP-L, AA-G, and JC: data collection and data analysis. MM-L, LF, AM-C, AG, MCG-V, JM-P, and EM: sample collection, interpretation of data, and intellectual contribution. PD and AF designed and interpreted the work, wrote the manuscript. AF obtained funding and supervised the study. All the authors have approved the final version of the manuscript.
Funding
This work was supported by the Instituto de Salud Carlos III (ISCIII), Ministry of Economy, Industry, and Competitiveness, co-funded by the European Regional Development Fund, grant numbers PI15/01803 and PI18/01278 (to AF). Moreover, the project has received two grants for the hiring of Laboratory Technicians from the Comunidad de Madrid in 2016 and 2020 (Youth Employment Operational Program (YEI): Contracts for Research assistants and laboratory technicians of the Community of Madrid and the European Social Fund, PEJ16/MED/TL-1388 and PEJ-2020-TL/BMD-19230).
Acknowledgments
The authors gratefully acknowledge to Manuel José del Rey for technical assistance and access to materials. The authors would like to thank the Instituto de Investigación Hospital 12 de Octubre (imas12) for the funding allocated to the incorporation of support staff in the 2016–2020 annual calls. Further, the authors would like to thank to Mercedes Avilés Escudero and María López Berlanga from the Innovation Support Unit and to Carmen Romero from the Scientific Support Unit, both from the Instituto de Investigación Hospital 12 de Octubre (imas12), for their support and advice.
Conflict of interest
The authors declare that the research was conducted in the absence of any commercial or financial relationships that could be construed as a potential conflict of interest.
Publisher’s note
All claims expressed in this article are solely those of the authors and do not necessarily represent those of their affiliated organizations, or those of the publisher, the editors and the reviewers. Any product that may be evaluated in this article, or claim that may be made by its manufacturer, is not guaranteed or endorsed by the publisher.
Supplementary material
The Supplementary Material for this article can be found online at: https://www.frontiersin.org/articles/10.3389/fcell.2022.1033080/full#supplementary-material
Supplementary Figure S1 | Gene expression of myofibroblast markers in suburethral fibroblasts cultures of gynecological patients without incontinence (non-SUI). Gene expression of myofibroblast markers was determined by real-time qPCR in suburethral fibroblasts isolated from patients without incontinence. The analyzed cells correspond to passage 3 (n=2 or 4). Data are presented as mean ± standard deviation (SD). Gene abbreviations are as follow: α-SMA, alpha smooth muscle actin; Vim, vimentin; Des, desmin; Smooth, smoothelin; Ten, tenascin; Col, collagen I, PDPN, podoplanin; Cad, Cadherin. Desmin does not appear in this graph because non-SUI cells do not express it
References
Abumaree, M. H., Abomaray, F. M., Alshehri, N. A., Almutairi, A., Alaskar, A. S., Kalionis, B., et al. (2016). Phenotypic and functional characterization of mesenchymal stem/multipotent stromal cells from decidua parietalis of human term placenta. Reprod. Sci. 23, 1193–1207. doi:10.1177/1933719116632924
Alicka, M., Kornicka-Garbowska, K., Kucharczyk, K., Kepska, M., Rcken, M., and Marycz, K. (2020). Age-dependent impairment of adipose-derived stem cells isolated from horses. Stem Cell Res. Ther. 11, 4. doi:10.1186/s13287-019-1512-6
Alperin, M., Abramowitch, S., Alarab, M., Bortolini, M., Brown, B., Burnett, L. A., et al. (2022). Foundational science and mechanistic insights for a shared disease model: An expert consensus. Female Pelvic Med. Reconstr. Surg. 28, 347–350. doi:10.1097/SPV.0000000000001216
Aneiros-Fernandez, J., Husein-Elahmed, H., Arias-Santiago, S., Campos, A., Carriel, V., Sanchez-Montesinos, I., et al. (2011). Expression of smoothelin and smooth muscle actin in the skin. Histol. Histopathol. 26, 673–678. doi:10.14670/HH-26.673
Ashton-Miller, J. A., and Delancey, J. O. (2007). Functional anatomy of the female pelvic floor. Ann. N. Y. Acad. Sci. 1101, 266–296. doi:10.1196/annals.1389.034
Ashton-Miller, J. A., and Delancey, J. O. (2009). On the biomechanics of vaginal birth and common sequelae. Annu. Rev. Biomed. Eng. 11, 163–176. doi:10.1146/annurev-bioeng-061008-124823
Bilhar, A. P. M., Bortolini, M. A. T., Se, A. B., Feitosa, S. M., Salerno, G. R. F., Zanoteli, E., et al. (2018). Molecular and immunohistochemical analysis of the urethra of female rats after induced trauma and intravenous therapy with muscle derived stem cells. Neurourol. Urodyn. 37, 2151–2159. doi:10.1002/nau.23567
Blokland, K. E. C., Pouwels, S. D., Schuliga, M., Knight, D. A., and Burgess, J. K. (2020). Regulation of cellular senescence by extracellular matrix during chronic fibrotic diseases. Clin. Sci. (Lond) 134, 2681–2706. doi:10.1042/CS20190893
Bortolini, M. A. T., Feitosa, S. M., Bilhar, A. P. M., Salerno, G. G. R., Zanoteli, E., Simoes, M. J., et al. (2019). Molecular and histomorphological evaluation of female rats' urethral tissues after an innovative trauma model of prolonged vaginal distention: Immediate, short-term and long-term effects. Int. Urogynecol J. 30, 465–476. doi:10.1007/s00192-018-3634-2
Bowler, M. A., Bersi, M. R., Ryzhova, L. M., Jerrell, R. J., Parekh, A., and Merryman, W. D. (2018). Cadherin-11 as a regulator of valve myofibroblast mechanobiology. Am. J. Physiol. Heart Circ. Physiol. 315, H1614–H1626. doi:10.1152/ajpheart.00277.2018
Braun, N., Alscher, D. M., Fritz, P., Edenhofer, I., Kimmel, M., Gaspert, A., et al. (2011). Podoplanin-positive cells are a hallmark of encapsulating peritoneal sclerosis. Nephrol. Dial. Transpl. 26, 1033–1041. doi:10.1093/ndt/gfq488
Bravo, B., Gallego, M. I., Flores, A. I., Bornstein, R., Puente-Bedia, A., Hernandez, J., et al. (2016). Restrained Th17 response and myeloid cell infiltration into the central nervous system by human decidua-derived mesenchymal stem cells during experimental autoimmune encephalomyelitis. Stem Cell Res. Ther. 7, 43. doi:10.1186/s13287-016-0304-5
Cabral-Pacheco, G. A., Garza-Veloz, I., Castruita-De, R C., Ramirez-Acuna, J. M., Perez-Romero, B. A., Guerrero-Rodriguez, J. F., et al. (2020). The roles of matrix metalloproteinases and their inhibitors in human diseases. Int. J. Mol. Sci. 21, 9739. doi:10.3390/ijms21249739
Cannon, T. W., Wojcik, E. M., Ferguson, C. L., Saraga, S., Thomas, C., and Damaser, M. S. (2002). Effects of vaginal distension on urethral anatomy and function. BJU Int. 90, 403–407. doi:10.1046/j.1464-410x.2002.02918.x
Cerrada, A., Torre, D L P., Grande, J., Haller, T., Flores, A. I., and Perez-Gil, J. (2014). Human decidua-derived mesenchymal stem cells differentiate into functional alveolar type II-like cells that synthesize and secrete pulmonary surfactant complexes. PLoS One 9, e110195. doi:10.1371/journal.pone.0110195
Chen, B., Wen, Y., Zhang, Z., Guo, Y., Warrington, J. A., and Polan, M. L. (2006). Microarray analysis of differentially expressed genes in vaginal tissues from women with stress urinary incontinence compared with asymptomatic women. Hum. Reprod. 21, 22–29. doi:10.1093/humrep/dei276
Chen, B., and Yeh, J. (2011). Alterations in connective tissue metabolism in stress incontinence and prolapse. J. Urol. 186, 1768–1772. doi:10.1016/j.juro.2011.06.054
Chen, X., Xiang, H., Yu, S., Lu, Y., and Wu, T. (2021). Research progress in the role and mechanism of Cadherin-11 in different diseases. J. Cancer 12, 1190–1199. doi:10.7150/jca.52720
Coppe, J. P., Desprez, P. Y., Krtolica, A., and Campisi, J. (2010). The senescence-associated secretory phenotype: The dark side of tumor suppression. Annu. Rev. Pathol. 5, 99–118. doi:10.1146/annurev-pathol-121808-102144
Cui, L., Meng, Q., Wen, J., Gao, Z., Yan, Z., Tian, Y., et al. (2018). A functional comparison of treatment of intrinsic sphincter deficiency with muscle-derived and adipose tissue-derived stem cells. IUBMB Life 70, 976–984. doi:10.1002/iub.1896
D'Urso, M., and Kurniawan, N. A. (2020). Mechanical and physical regulation of fibroblast-myofibroblast transition: From cellular mechanoresponse to tissue pathology. Front. Bioeng. Biotechnol. 8, 609653. doi:10.3389/fbioe.2020.609653
Dai, M., Xu, P., Hou, M., Teng, Y., and Wu, Q. (2015). In vivo imaging of adipose-derived mesenchymal stem cells in female nude mice after simulated childbirth injury. Exp. Ther. Med. 9, 372–376. doi:10.3892/etm.2014.2092
Damaser, M. S., Kim, F. J., and Minetti, G. M. (2003). Methods of testing urethral resistance in the female rat. Adv. Exp. Med. Biol. 539, 831–839. doi:10.1007/978-1-4419-8889-8_51
Damaser, M. S., Whitbeck, C., Chichester, P., and Levin, R. M. (2005). Effect of vaginal distension on blood flow and hypoxia of urogenital organs of the female rat. J. Appl. Physiol. 98, 1884–1890. doi:10.1152/japplphysiol.01071.2004
Darby, I. A., Laverdet, B., Bonte, F., and Desmouliere, A. (2014). Fibroblasts and myofibroblasts in wound healing. Clin. Cosmet. Investig. Dermatol 7, 301–311. doi:10.2147/CCID.S50046
Torre, D L P., Torre, F-D L M., and Flores, A. I. (2021). Premature senescence of placental decidua cells as a possible cause of miscarriage produced by mycophenolic acid. J. Biomed. Sci. 28, 3. doi:10.1186/s12929-020-00704-4
Torre, D L P., Pérez-Lorenzo, M. J., and Flores, A. I. (2018). “Human placenta-derived mesenchymal stromal cells: A review from basic research to clinical applications. Stromal cells-structure, function, and therapeutic implications,” in Stromal Cells - Structure, Function, and Therapeutic Implications [Internet]. Editors M. T. Valarmathi(London: Intechopen). doi:10.5772/intechopen.76718
Del Rey, M. J., Valin, A., Usategui, A., Ergueta, S., Martin, E., Municio, C., et al. (2019). Senescent synovial fibroblasts accumulate prematurely in rheumatoid arthritis tissues and display an enhanced inflammatory phenotype. Immun. Ageing 16, 29. doi:10.1186/s12979-019-0169-4
Dissaranan, C., Cruz, M. A., Kiedrowski, M. J., Balog, B. M., Gill, B. C., Penn, M. S., et al. (2014). Rat mesenchymal stem cell secretome promotes elastogenesis and facilitates recovery from simulated childbirth injury. Cell Transpl. 23, 1395–1406. doi:10.3727/096368913X670921
Enomoto, Y., Matsushima, S., Meguro, S., Kawasaki, H., Kosugi, I., Fujisawa, T., et al. (2018). Podoplanin-positive myofibroblasts: A pathological hallmark of pleuroparenchymal fibroelastosis. Histopathology 72, 1209–1215. doi:10.1111/his.13494
Falconer, C., Ekman-Ordeberg, G., Ulmsten, U., Westergren-Thorsson, G., Barchan, K., and Malmstrom, A. (1996). Changes in paraurethral connective tissue at menopause are counteracted by estrogen. Maturitas 24, 197–204. doi:10.1016/s0378-5122(96)82010-x
Flores, A. I., Pipino, C., Jerman, U. D., Liarte, S., Gindraux, F., Kreft, M. E., et al. (2022). Perinatal derivatives: How to best characterize their multimodal functions in vitro. Part C: Inflammation, angiogenesis, and wound healing. Front. Bioeng. Biotechnol. 10, 965006. doi:10.3389/fbioe.2022.965006
Ford, A. A., Rogerson, L., Cody, J. D., and Ogah, J. (2015). Mid-urethral sling operations for stress urinary incontinence in women. New Jersey, NJ: Cochrane Database Syst Rev, CD006375. doi:10.1002/14651858.CD006375
Gao, Q., Wang, L., Wang, S., Huang, B., Jing, Y., and Su, J. (2021). Bone marrow mesenchymal stromal cells: Identification, classification, and differentiation. Front. Cell Dev. Biol. 9, 787118. doi:10.3389/fcell.2021.787118
Garcia-Arranz, M., Alonso-Gregorio, S., Fontana-Portella, P., Bravo, E., Diez Sebastian, J., Fernandez-Santos, M. E., et al. (2020). Two phase I/II clinical trials for the treatment of urinary incontinence with autologous mesenchymal stem cells. Stem Cells Transl. Med. 9, 1500–1508. doi:10.1002/sctm.19-0431
Gardella, B., Scatigno, A. L., Belli, G., Gritti, A., Visona, S. D., and Dominoni, M. (2022). Aging of pelvic floor in animal models: A sistematic review of literature on the role of the extracellular matrix in the development of pelvic floor prolapse. Front. Med. (Lausanne) 9, 863945. doi:10.3389/fmed.2022.863945
Ghaffari-Nazari, H. (2018). The known molecules involved in MSC homing and migration. J. Stem Cell Res. Med. 3, 1–4. doi:10.15761/jscrm.1000127
Gill, B. C., Moore, C., and Damaser, M. S. (2010). Postpartum stress urinary incontinence: Lessons from animal models. Expert Rev. Obstet. Gynecol. 5, 567–580. doi:10.1586/eog.10.48
Gotoh, M., Shimizu, S., Yamamoto, T., Ishizuka, O., Yamanishi, T., Mizokami, A., et al. (2020). Regenerative treatment for male stress urinary incontinence by periurethral injection of adipose-derived regenerative cells: Outcome of the ADRESU study. Int. J. Urol. 27, 859–865. doi:10.1111/iju.14311
Hass, R., Kasper, C., Bohm, S., and Jacobs, R. (2011). Different populations and sources of human mesenchymal stem cells (MSC): A comparison of adult and neonatal tissue-derived MSC. Cell Commun. Signal 9, 12. doi:10.1186/1478-811X-9-12
Herrera-Imbroda, B., Lara, M. F., Izeta, A., Sievert, K. D., and Hart, M. L. (2015). Stress urinary incontinence animal models as a tool to study cell-based regenerative therapies targeting the urethral sphincter. Adv. Drug Deliv. Rev. 82-83, 106–116. doi:10.1016/j.addr.2014.10.018
Herschorn, S. (2004). Female pelvic floor anatomy: The pelvic floor, supporting structures, and pelvic organs. Rev. Urol. 6, S2–S10.
Hijaz, A., Daneshgari, F., Sievert, K. D., and Damaser, M. S. (2008). Animal models of female stress urinary incontinence. J. Urol. 179, 2103–2110. doi:10.1016/j.juro.2008.01.096
Hinz, B., Pittet, P., Smith-Clerc, J., Chaponnier, C., and Meister, J. J. (2004). Myofibroblast development is characterized by specific cell-cell adherens junctions. Mol. Biol. Cell 15, 4310–4320. doi:10.1091/mbc.e04-05-0386
Hirayama, K., Kono, H., Nakata, Y., Akazawa, Y., Wakana, H., Fukushima, H., et al. (2018). Expression of podoplanin in stromal fibroblasts plays a pivotal role in the prognosis of patients with pancreatic cancer. Surg. Today 48, 110–118. doi:10.1007/s00595-017-1559-x
Huang, L., Zhao, Z., Wen, J., Ling, W., Miao, Y., and Wu, J. (2020). Cellular senescence: A pathogenic mechanism of pelvic organ prolapse (review). Mol. Med. Rep. 22, 2155–2162. doi:10.3892/mmr.2020.11339
Hunskaar, S., Lose, G., Sykes, D., and Voss, S. (2004). The prevalence of urinary incontinence in women in four European countries. BJU Int. 93, 324–330. doi:10.1111/j.1464-410x.2003.04609.x
Jaalouk, D. E., and Lammerding, J. (2009). Mechanotransduction gone awry. Nat. Rev. Mol. Cell Biol. 10, 63–73. doi:10.1038/nrm2597
Janssen, K., Lin, D. L., Hanzlicek, B., Deng, K., Balog, B. M., Vaart, C. H V D., et al. (2019). Multiple doses of stem cells maintain urethral function in a model of neuromuscular injury resulting in stress urinary incontinence. Am. J. Physiol. Ren. Physiol. 317, F1047–F1057. doi:10.1152/ajprenal.00173.2019
Jiang, M., Liu, J., Liu, W., Zhu, X., Bano, Y., Liao, H., et al. (2021). Bone marrow stem cells secretome accelerates simulated birth trauma-induced stress urinary incontinence recovery in rats. Aging (Albany NY) 13, 10517–10534. doi:10.18632/aging.202812
Jimenez-Puerta, G. J., Marchal, J. A., Lopez-Ruiz, E., and Galvez-Martin, P. (2020). Role of mesenchymal stromal cells as therapeutic agents: Potential mechanisms of action and implications in their clinical use. J. Clin. Med. 9, 445. doi:10.3390/jcm9020445
Kasprzycka, M., Hammarstrom, C., and Haraldsen, G. (2015). Tenascins in fibrotic disorders-from bench to bedside. Cell Adh Migr. 9, 83–89. doi:10.4161/19336918.2014.994901
Katoh, D., Kozuka, Y., Noro, A., Ogawa, T., Imanaka-Yoshida, K., and Yoshida, T. (2020). Tenascin-C induces phenotypic changes in fibroblasts to myofibroblasts with high contractility through the integrin αvβ1/transforming growth factor β/SMAD signaling Axis in human breast cancer. Am. J. Pathol. 190, 2123–2135. doi:10.1016/j.ajpath.2020.06.008
Khalifa, A. O., Kavran, M., Mahran, A., Isali, I., Woda, J., Flask, C. A., et al. (2020). Stromal derived factor-1 plasmid as a novel injection for treatment of stress urinary incontinence in a rat model. Int. Urogynecol J. 31, 107–115. doi:10.1007/s00192-019-03867-3
Krawczenko, A., and Klimczak, A. (2022). Adipose tissue-derived mesenchymal stem/stromal cells and their contribution to angiogenic processes in tissue regeneration. Int. J. Mol. Sci. 23, 2425. doi:10.3390/ijms23052425
Kuismanen, K., Sartoneva, R., Haimi, S., Mannerstrom, B., Tomas, E., Miettinen, S., et al. (2014). Autologous adipose stem cells in treatment of female stress urinary incontinence: Results of a pilot study. Stem Cells Transl. Med. 3, 936–941. doi:10.5966/sctm.2013-0197
Ledesma-Martinez, E., Mendoza-Nunez, V. M., and Santiago-Osorio, E. (2016). Mesenchymal stem cells derived from dental pulp: A review. Stem Cells Int. 2016, 4709572. doi:10.1155/2016/4709572
Lee, U. J., Feinstein, L., Ward, J. B., Kirkali, Z., Martinez-Miller, E. E., Matlaga, B. R., et al. (2021). Prevalence of urinary incontinence among a nationally representative sample of women, 2005-2016: Findings from the urologic diseases in America project. J. Urol. 205, 1718–1724. doi:10.1097/JU.0000000000001634
Lepreux, S., Guyot, C., Billet, F., Combe, C., Balabaud, C., Bioulac-Sage, P., et al. (2013). Smoothelin, a new marker to determine the origin of liver fibrogenic cells. World J. Gastroenterol. 19, 9343–9350. doi:10.3748/wjg.v19.i48.9343
Levy, O., Kuai, R., Siren, E. M. J., Bhere, D., Milton, Y., Nissar, N., et al. (2020). Shattering barriers toward clinically meaningful MSC therapies. Sci. Adv. 6, eaba6884. doi:10.1126/sciadv.aba6884
Lin, K. L., Chueh, K. S., Lu, J. H., Chuang, S. M., Wu, B. N., Lee, Y. C., et al. (2021). Low intensity extracorporeal shock wave therapy as a novel treatment for stress urinary incontinence: A randomized-controlled clinical study, 57. Medicina (Kaunas).
Lin, S. Y., Tee, Y. T., Ng, S. C., Chang, H., Lin, P., and Chen, G. D. (2007). Changes in the extracellular matrix in the anterior vagina of women with or without prolapse. Int. Urogynecol J. Pelvic Floor Dysfunct. 18, 43–48. doi:10.1007/s00192-006-0090-1
Liss, M. A., Ahlering, T. E., Morales, B., Gordon, A., Osann, K., Skarecky, D., et al. (2016). Monocyte chemotactic protein-1 (MCP-1) as a predictor of prolonged urinary incontinence after radical prostatectomy. Open Urology Nephrol. J. 9, 44–50. doi:10.2174/1874303x01609010044
Liu, Y., Zhang, Z., Li, T., Xu, H., and Zhang, H. (2022). Senescence in osteoarthritis: From mechanism to potential treatment. Arthritis Res. Ther. 24, 174. doi:10.1186/s13075-022-02859-x
Lopes-Paciencia, S., Saint-Germain, E., Rowell, M. C., Ruiz, A. F., Kalegari, P., and Ferbeyre, G. (2019). The senescence-associated secretory phenotype and its regulation. Cytokine 117, 15–22. doi:10.1016/j.cyto.2019.01.013
Macias, M. I., Grande, J., Moreno, A., Dominguez, I., Bornstein, R., and Flores, A. I. (2010). Isolation and characterization of true mesenchymal stem cells derived from human term decidua capable of multilineage differentiation into all 3 embryonic layers. Am. J. Obstet. Gynecol. 203, e9–e495. doi:10.1016/j.ajog.2010.06.045
Maiborodin, I., Yarin, G., Marchukov, S., Pichigina, A., Lapii, G., Krasil'Nikov, S., et al. (2022). Cell Technologies in the stress urinary incontinence correction. Biomedicines 10, 309. doi:10.3390/biomedicines10020309
Maral, I., Ozkardes, H., Peskircioglu, L., and Bumin, M. A. (2001). Prevalence of stress urinary incontinence in both sexes at or after age 15 years: A cross-sectional study. J. Urol. 165, 408–412. doi:10.1097/00005392-200102000-00013
Memon, H. U., and Handa, V. L. (2013). Vaginal childbirth and pelvic floor disorders. Womens Health (Lond) 9, 265–277. doi:10.2217/whe.13.17
Merimi, M., El-Majzoub, R., Lagneaux, L., Moussa Agha, D., Bouhtit, F., Meuleman, N., et al. (2021). The therapeutic potential of mesenchymal stromal cells for regenerative medicine: Current knowledge and future understandings. Front. Cell Dev. Biol. 9, 661532. doi:10.3389/fcell.2021.661532
Milsom, I., Coyne, K. S., Nicholson, S., Kvasz, M., Chen, C. I., and Wein, A. J. (2014). Global prevalence and economic burden of urgency urinary incontinence: A systematic review. Eur. Urol. 65, 79–95. doi:10.1016/j.eururo.2013.08.031
Nazari, B., Rice, L. M., Stifano, G., Barron, A. M., Wang, Y. M., Korndorf, T., et al. (2016). Altered dermal fibroblasts in systemic sclerosis display podoplanin and CD90. Am. J. Pathol. 186, 2650–2664. doi:10.1016/j.ajpath.2016.06.020
Ni, J., Li, H., Zhou, Y., Gu, B., Xu, Y., Fu, Q., et al. (2018). Therapeutic potential of human adipose-derived stem cell exosomes in stress urinary incontinence - an in vitro and in vivo study. Cell Physiol. Biochem. 48, 1710–1722. doi:10.1159/000492298
Norton, P., and Brubaker, L. (2006). Urinary incontinence in women. Lancet 367, 57–67. doi:10.1016/S0140-6736(06)67925-7
Pan, H. Q., Kerns, J. M., Lin, D. L., Liu, S., Esparza, N., and Damaser, M. S. (2007). Increased duration of simulated childbirth injuries results in increased time to recovery. Am. J. Physiol. Regul. Integr. Comp. Physiol. 292, R1738–R1744. doi:10.1152/ajpregu.00784.2006
Pantatosakis, E., Karandrea, D., Liapis, E., Kondi-Pafiti, A., and Liapis, A. (2016). Immunohistochemical expression of hormonal receptors, collagen, elastin, and proteoglycans in genuine urinary incontinence. Clin. Exp. Obstet. Gynecol. 43, 849–852. doi:10.12891/ceog3390.2016
Paris, J. L., Torre, D L P., Manzano, M., Cabanas, M. V., Flores, A. I., and Vallet-Regi, M. (2016). Decidua-derived mesenchymal stem cells as carriers of mesoporous silica nanoparticles. in vitro and in vivo evaluation on mammary tumors. Acta Biomater. 33, 275–282. doi:10.1016/j.actbio.2016.01.017
Paris, J. L., Torre, P D L., Victoria Cabanas, M., Manzano, M., Grau, M., Flores, A. I., et al. (2017). Vectorization of ultrasound-responsive nanoparticles in placental mesenchymal stem cells for cancer therapy. Nanoscale 9, 5528–5537. doi:10.1039/c7nr01070b
Pathak, R. U., Soujanya, M., and Mishra, R. K. (2021). Deterioration of nuclear morphology and architecture: A hallmark of senescence and aging. Ageing Res. Rev. 67, 101264. doi:10.1016/j.arr.2021.101264
Phull, H. S., Pan, H. Q., Butler, R. S., Hansel, D. E., and Damaser, M. S. (2011). Vulnerability of continence structures to injury by simulated childbirth. Am. J. Physiol. Ren. Physiol. 301, F641–F649. doi:10.1152/ajprenal.00120.2011
Quintanilla, M., Montero-Montero, L., Renart, J., and Martin-Villar, E. (2019). Podoplanin in inflammation and cancer. Int. J. Mol. Sci. 20, 707. doi:10.3390/ijms20030707
Radziszewski, P., Borkowski, A., Torz, C., Bossowska, A., Gonkowski, S., and Majewski, M. (2005). Distribution of collagen type VII in connective tissues of postmenopausal stress-incontinent women. Gynecol. Endocrinol. 20, 121–126. doi:10.1080/09513590400021078
Rahn, D. D., Acevedo, J. F., and Word, R. A. (2008). Effect of vaginal distention on elastic fiber synthesis and matrix degradation in the vaginal wall: Potential role in the pathogenesis of pelvic organ prolapse. Am. J. Physiol. Regul. Integr. Comp. Physiol. 295, R1351–R1358. doi:10.1152/ajpregu.90447.2008
Rodriguez-Fuentes, D. E., Fernandez-Garza, L. E., Samia-Meza, J. A., Barrera-Barrera, S. A., Caplan, A. I., and Barrera-Saldana, H. A. (2021). Mesenchymal stem cells current clinical applications: A systematic review. Arch. Med. Res. 52, 93–101. doi:10.1016/j.arcmed.2020.08.006
Ruiz-Zapata, A. M., Heinz, A., Kerkhof, M. H., Rijt, C V D W-V., Schmelzer, C. E. H., Stoop, R., et al. (2020). Extracellular matrix stiffness and composition regulate the myofibroblast differentiation of vaginal fibroblasts. Int. J. Mol. Sci. 21, 4762. doi:10.3390/ijms21134762
Schenk, S., Mal, N., Finan, A., Zhang, M., Kiedrowski, M., Popovic, Z., et al. (2007). Monocyte chemotactic protein-3 is a myocardial mesenchymal stem cell homing factor. Stem Cells 25, 245–251. doi:10.1634/stemcells.2006-0293
Schleip, R., Gabbiani, G., Wilke, J., Naylor, I., Hinz, B., Zorn, A., et al. (2019). Fascia is able to actively contract and may thereby influence musculoskeletal dynamics: A histochemical and mechanographic investigation. Front. Physiol. 10, 336. doi:10.3389/fphys.2019.00336
Serini, G., and Gabbiani, G. (1999). Mechanisms of myofibroblast activity and phenotypic modulation. Exp. Cell Res. 250, 273–283. doi:10.1006/excr.1999.4543
Serrano, L. J., Torre, P D L., Liras, A., and Flores, A. I. (2021). Cell therapy for factor V deficiency: An approach based on human decidua mesenchymal stem cells. Biomed. Pharmacother. 142, 112059. doi:10.1016/j.biopha.2021.112059
Siccardi, M. A., and Valle, C. (2022). Anatomy, bony pelvis and lower limb, pelvic fascia. Treasure Island (FL: StatPearls.
Silini, A. R., Di Pietro, R., Lang-Olip, I., Alviano, F., Banerjee, A., Basile, M., et al. (2020). Perinatal derivatives: Where do we stand? A roadmap of the human placenta and consensus for tissue and cell nomenclature. Front. Bioeng. Biotechnol. 8, 610544. doi:10.3389/fbioe.2020.610544
Sima, Y., and Chen, Y. (2020). MSC-based therapy in female pelvic floor disorders. Cell Biosci. 10, 104. doi:10.1186/s13578-020-00466-4
Skalli, O., Schurch, W., Seemayer, T., Lagace, R., Montandon, D., Pittet, B., et al. (1989). Myofibroblasts from diverse pathologic settings are heterogeneous in their content of actin isoforms and intermediate filament proteins. Lab. Invest. 60, 275–285.
Torre, P., and Flores, A. I. (2020). Current Status and Future Prospects of Perinatal Stem Cells, 12. Basel): Genes.
Vegh, I., Grau, M., Gracia, M., Grande, J., Torre, P D L., and Flores, A. I. (2013). Decidua mesenchymal stem cells migrated toward mammary tumors in vitro and in vivo affecting tumor growth and tumor development. Cancer Gene Ther. 20, 8–16. doi:10.1038/cgt.2012.71
Wang, G., Lin, G., Zhang, H., Qiu, X., Ning, H., Banie, L., et al. (2011). Effects of prolonged vaginal distension and beta-aminopropionitrile on urinary continence and urethral structure. Urology 78, 968 e13–e19. doi:10.1016/j.urology.2011.07.1381
Wei, Y., Fu, J., Wu, W., Ma, P., Ren, L., and Wu, J. (2021). Estrogen prevents cellular senescence and bone loss through usp10-dependent p53 degradation in osteocytes and osteoblasts: The role of estrogen in bone cell senescence. Cell Tissue Res. 386, 297–308. doi:10.1007/s00441-021-03496-7
Wen, Y., Whitin, J., Yu, T., Cohen, H., Polan, M. L., and Chen, B. (2012). Identification of protein marker in vaginal wall tissues of women with stress urinary incontinence by protein chip array. J. Obstet. Gynaecol. Res. 38, 89–96. doi:10.1111/j.1447-0756.2011.01690.x
Woo, L. L., Hijaz, A., Kuang, M., Penn, M. S., Damaser, M. S., and Rackley, R. R. (2007). Over expression of stem cell homing cytokines in urogenital organs following vaginal distention. J. Urol. 177, 1568–1572. doi:10.1016/j.juro.2006.11.047
Wood, H. M., Kuang, M., Woo, L., Hijaz, A., Butler, R. S., Penn, M., et al. (2008). Cytokine expression after vaginal distention of different durations in virgin Sprague-Dawley rats. J. Urol. 180, 753–759. doi:10.1016/j.juro.2008.03.182
Wu, A. K., Zhang, X., Wang, J., Ning, H., Zaid, U., Villalta, J. D., et al. (2018). Treatment of stress urinary incontinence with low-intensity extracorporeal shock wave therapy in a vaginal balloon dilation induced rat model. Transl. Androl. Urology 7, S7–S16. doi:10.21037/tau.2017.12.36
Wu, R., Huang, C., Wu, Q., Jia, X., Liu, M., Xue, Z., et al. (2019). Exosomes secreted by urine-derived stem cells improve stress urinary incontinence by promoting repair of pubococcygeus muscle injury in rats. Stem Cell Res. Ther. 10, 80. doi:10.1186/s13287-019-1182-4
Yang, Y., Lee, E. H., and Yang, Z. (2022). Hypoxia-conditioned mesenchymal stem cells in tissue regeneration application. Tissue Eng. Part B Rev. 28, 966–977. doi:10.1089/ten.TEB.2021.0145
Zhang, K., Rekhter, M. D., Gordon, D., and Phan, S. H. (1994). Myofibroblasts and their role in lung collagen gene expression during pulmonary fibrosis. A combined immunohistochemical and in situ hybridization study. Am. J. Pathol. 145, 114–125.
Zordani, A., Pisciotta, A., Bertoni, L., Bertani, G., Vallarola, A., Giuliani, D., et al. (2019). Regenerative potential of human dental pulp stem cells in the treatment of stress urinary incontinence: In vitro and in vivo study. Cell Prolif. 52, e12675. doi:10.1111/cpr.12675
Keywords: stress urinary incontinence, vaginal distention, perinatal mesenchymal stromal cells, myofibroblast, senescence, extracellular matrix remodeling, regeneration, secretome
Citation: De La Torre P, Pérez-Lorenzo MJ, Alcázar-Garrido Á, Collado J, Martínez-López M, Forcén L, Masero-Casasola AR, García A, Gutiérrez-Vélez MC, Medina-Polo J, Muñoz E and Flores AI (2023) Perinatal mesenchymal stromal cells of the human decidua restore continence in rats with stress urinary incontinence induced by simulated birth trauma and regulate senescence of fibroblasts from women with stress urinary incontinence. Front. Cell Dev. Biol. 10:1033080. doi: 10.3389/fcell.2022.1033080
Received: 31 August 2022; Accepted: 29 December 2022;
Published: 18 January 2023.
Edited by:
Anuradha Vaidya, Symbiosis International University, IndiaReviewed by:
Tomasz Kloskowski, Nicolaus Copernicus University in Toruń, PolandMonika Sharma, Cellcolabs, Sweden
Copyright © 2023 De La Torre, Pérez-Lorenzo, Alcázar-Garrido, Collado, Martínez-López, Forcén, Masero-Casasola, García, Gutiérrez-Vélez, Medina-Polo, Muñoz and Flores. This is an open-access article distributed under the terms of the Creative Commons Attribution License (CC BY). The use, distribution or reproduction in other forums is permitted, provided the original author(s) and the copyright owner(s) are credited and that the original publication in this journal is cited, in accordance with accepted academic practice. No use, distribution or reproduction is permitted which does not comply with these terms.
*Correspondence: Ana I. Flores, aflores@h12o.es
†These authors contributed equally to this work and share first authorship