- 1Faculty of Biosciences and Technology for Food, Agriculture and Environment, University of Teramo, Teramo, Italy
- 2Istituto Zooprofilattico Sperimentale Dell’Abruzzo e Del Molise “G. Caporale”, Teramo, Italy
- 3Institute of Biochemistry and Cell Biology (CNR-IBBC/EMMA/Infrafrontier/IMPC), National Research Council, Rome, Italy
MicroRNAs are small non-coding molecules that control several cellular functions and act as negative post-transcriptional regulators of the mRNA. While their implication in several biological functions is already known, an important role as regulators of different physiological and pathological processes in fertilization and embryo development is currently emerging. Indeed, miRNAs have been found in the oviductal fluid packaged within the extracellular vesicles, which might act as natural nanoshuttles by transporting lipids, proteins, RNA molecules and miRNAs from the oviduct to the gametes or embryos. Here, an exhaustive bibliography search was carried out, followed by the construction of a computational model based on the networks theory in an attempt to recreate and elucidate the pathways potentially activated by the oviductal miRNA. The omics data published to date were gathered to create the Oviductal MiRNome, in which the miRNA target genes and their interactions are represented by using stringApp and the Network analyzer from Cytoscape 3.7.2. Then, the hyperlinked nodes were identified to investigate the pathways in which they are involved using the gene ontology enrichment analysis. To study the phenotypical effects after the removal of key genes on the reproductive system and embryo, knockout mouse lines for every protein-coding gene were investigated by using the International Mouse Phenotyping Consortium database. The creation of the Oviductal MiRNome revealed the presence of important genes and their interactions within the network. The functional enrichment analysis revealed that the hyperlinked nodes are involved in fundamental cellular functions, both structural and regulatory/signaling, suggesting their implication in fertilization and early embryo development. This fact was as well evidenced by the effects of the gene deletion in KO mice on the reproductive system and embryo development. The present study highlights the importance of studying the miRNA profiles and their enormous potential as tools to improve the assisted reproductive techniques currently used in human and animal reproduction.
Introduction
In mammals, the oviduct provides a favourable microenvironment for several events related to fertilization, as the sperm acquisition of fertilizing ability (the capacitation) (Gadella and Boerke, 2016), the sperm-egg recognition and binding (Coy et al., 2012) and the first phases of the embryo development (Li and Winuthayanon, 2017). It exerts its function either by direct or indirect mechanisms: while the oviductal epithelial cells (OECs) directly interact with the spermatozoa storing them in the so-called “functional sperm reservoir” until ovulation (Suarez, 2016), the key molecules contained in the oviductal fluid (OF) contribute to sustain and drive the biochemical machinery of spermatozoa and early embryo development (Avilés et al., 2010; Coy and Yanagimachi, 2015). In this regard, the OF is a complex mixture of molecules that are either passively or actively transported over the epithelial barrier from the circulating blood or the interstitial tissue, or de novo secreted by the OECs (Saint-Dizier et al., 2020). It is mainly composed by aminoacids, energy sources , inorganic salts, glycosaminoglycans and numerous proteins (Ballester et al., 2014; Coy and Yanagimachi, 2015; Canha-Gouveia et al., 2019). In particular, the two most abundant proteins identified are the oviduct-specific glycoprotein (OVGP1) and albumin (Mondéjar et al., 2012; Canha-Gouveia et al., 2019), with important roles mainly for sperm capacitation and embryo development. Growth factors are also key proteins of the OF, which exert an activity of cell division control and contribute to the efficient development of early embryos (Pillai et al., 2017). Other components of the OF include low molecular weight hormones such as steroids (progesterone and estradiol) and prostaglandins, which are secreted by the ovarian follicles and the corpus luteus and can reach the OF via a local countercurrent transfer (Einer-Jensen and Hunter, 2005). Among these lipid-based molecules stands up progesterone, which is known to play a key role in multiple sperm capacitation events as hyperactivation (Fujinoki et al., 2016), chemotaxis (Teves et al., 2006; Guidobaldi et al., 2008; Oren-Benaroya et al., 2008), induction of acrosome reaction (Therien and Manjunath, 2003; Baldi et al., 2009) and in vitro capacitation (Foresta et al., 2009; López-Torres and Chirinos, 2017). Furthermore, steroid hormones and prostaglandins may participate in the transport of gametes and embryos by modulating both muscular contractility and ciliary beat frequency in the oviduct (Ezzati et al., 2014).
During the last years, an additional and more complex component has been identified within the OF. Extracellular vesicles (EVs), also known as oviductosomes (OVS) (Gross et al., 2017; Fereshteh et al., 2018), are released by epithelial cells to the oviductal lumen, being thus classified as exosomes (30–100 nm) or microvesicles (>100 nm) based on their size (Almiñana et al., 2018). They carry bioactive molecules such as lipids, proteins, mRNA and microRNAs (miRNAs) (Asaadi et al., 2021) that can be delivered and fuse with gametes or embryo regulating their functions and interactions and participating in the maternal-embryo communication (Almiñana and Bauersachs, 2019; Bauersachs et al., 2020; Bridi et al., 2020; Harris et al., 2020).
Since the information available on this regard is still poor, here we followed a computational strategy using the networks theory in an attempt to reconstruct the pathways potentially activated by the oviductal miRNA. To this aim, we gathered the omics data published to date to create the Oviductal MiRNome, in which are represented the miRNA target genes and their interactions. The network obtained and the subsequent analysis allowed us to infer important information, useful to improve our knowledge on the potential role of miRNAs in the reproductive events.
Materials and methods
Data collection, network construction and analysis
To create the network representing the murine oviductal miRNome (MiRNome and MiRnome_MC), we collected recent data regarding the mirRNome from published peer-reviewed international manuscripts included in Scopus (https://www.scopus.com; accessed on 14/12/2021). To date, only one study systematically analysing the oviductal miRNA profile in the mouse model has been published (Fereshteh and coll, 2018, PMID: 30382141 (Fereshteh et al., 2018)). For each differentially expressed miRNA, we identified the target genes using miRDB (http://mirdb.org/, last accessed on 10/06/2022), an online database for miRNA target prediction and functional annotations (Chen and Wang, 2020). The identified target genes were selected for a target score >90 and submitted to network creation in stringApp for Cytoscape 3.7.2 (Doncheva et al., 2019). The interaction provided by the stringApp were filtered for the Mus musculus species, adopting a confidence score of 0.700. The main topological parameters of the network (Table 1) were analysed using Network Analyzer, a plugin of Cytoscape 3.7.2.
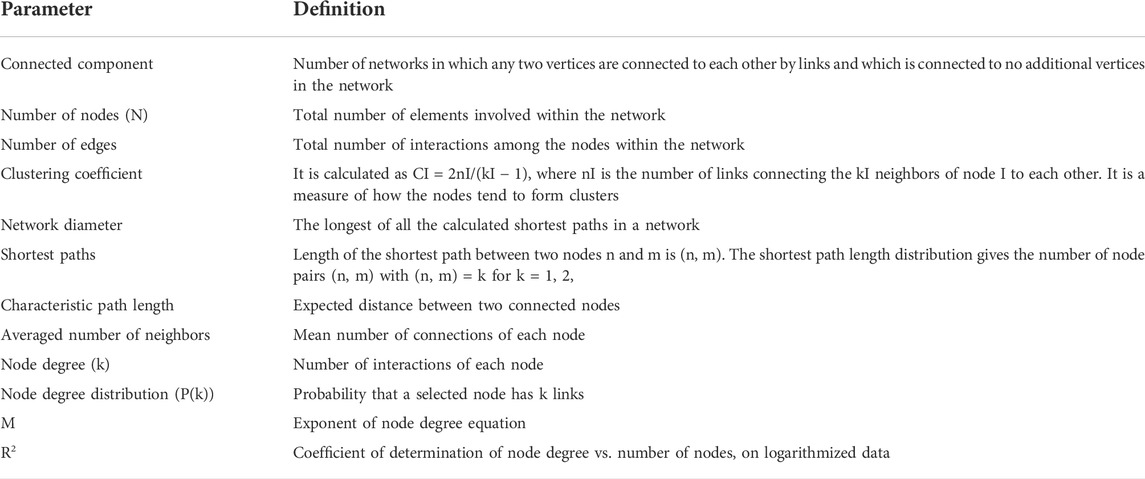
TABLE 1. Topological parameters of the network. The table shows the definitions of the main topological parameters assessed in this study.
In keeping with Bernabò et al. (Ordinelli et al., 2018; Bernabò et al., 2019), we identified the hubs within Murine Oviductal MiRNome as the nodes with a degree at least one standard deviation above the network mean. In details, the hubs were identified based on the following formula:
where:
ND = node degree
μ = averaged node degree
σ = standard deviation of node degree.
Enrichment analysis
We carried out an enrichment Gene ontology (GO) characterizes the relationship between genes by specifically annotating and categorizing the molecular function of a gene product, the associated biological process and the cellular component, referring to the place in the cell where a gene product performs a function (Ashburner et al., 2000). We used The Gene Ontology Consortium’s online tool (http://www.geneontology.org/, last accessed on 10/06/2021) for the enrichment analysis of our hubs list, selecting as species Mus musculus.
Identification of phenotypical effects of the deletion of genes relative to murine oviductal MiRNome hubs in KO mice in the reproductive system and embryos
To study the phenotypical effects after the removal of key genes (i.e., genes codifying for the murine oviductal MiRNome hubs) on the reproductive system and embryo, we used the International Mouse Phenotyping Consortium (IMPC) (https://www.mousephenotype.org/), a portal that provides a freely available comprehensive catalogue of mammalian genes function, by producing a knockout mouse line for every protein-coding gene.
Results
Murine oviductal MiRNome creation, analysis and visualization
The experimental design, including all the steps for the creation and analysis of the network, is illustrated in Figure 1. We collected the experimentally validated targets for the gathered oviductal miRNAs from miRDB. A total of 2,689 miRNA-target were collected from mouse and were used to build the Murine Oviductal MiRNome. Specifically, the network obtained is undirected and composed by 850 connected components, 2,665 nodes and 6,599 links. Since the largest connected component has 1746 nodes, representing 65.5% of Murine Oviductal MiRNome, all the further analysis were carried out on this network, called MiRNome Main Component, MiRNome_MC (Figure 2).
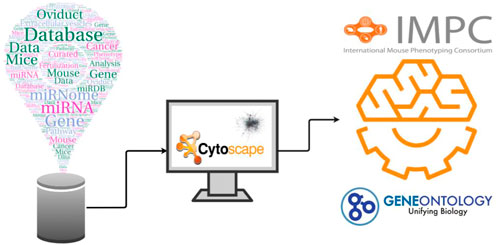
FIGURE 1. Experimental design. The figure illustrates the steps followed during the development of the work. 1) we collected the oviductal miRNA from the literature and we obtained from the miRDB their experimentally verified targets. 2)The identified target genes were submitted to network creation in stringApp for Cytoscape 3.7.2.3) We performed an enrichment Gene Ontology and used the International Mouse Phenotyping Consortium to investigate the phenotypic impact on the reproductive system and embryo after the removal of important genes.
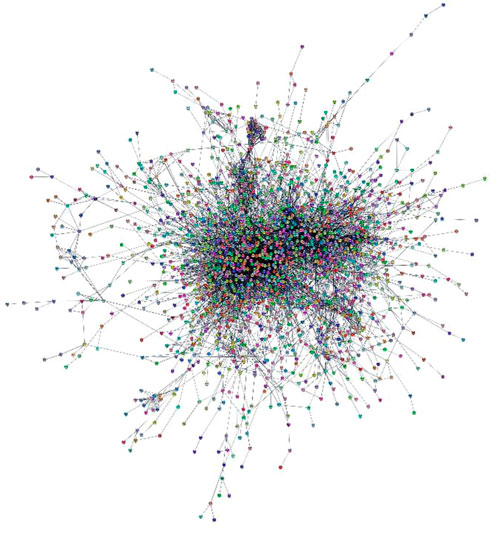
FIGURE 2. MiRNome_MC network. The figure shows the MiRNome Main Component (MiRNome_MC). The network was created with Cytoscape 3.7.2.
The values of its main topological parameters are listed in Table 2 and in Supplementary Material 1.
The node degree distribution follows a power law, characterized by a negative exponent (–1.702) and it is with the node degree (R2 = 0.1989). Thus, the MiRNome_MC does not possess an evident hierarchical pattern and it can be considered as a Barabasi—Albert network (Table 3).
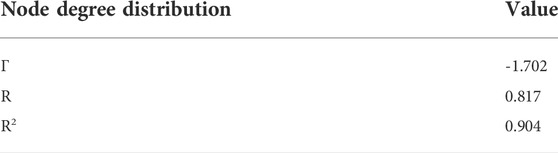
TABLE 3. Node degree distribution in MiRNome_MC. The node degree distribution represents the probability that a selected nodes has k links (γ = exponent of node degree equation; R2 = coefficient of determination of node degree vs. number of nodes, on logarithmized data).
Identification of hubs and gene ontology enrichment analysis
We found a total of 179 hubs in MiRNome_MC (the complete list of hubs and the topological parameters are available in the supplementary material 1), representing almost the 10% of the nodes (179 out of 1746). Then, we recorded the biological processes in which the hubs are engaged using gene ontology (GO) annotation, such as metabolic processes, gene expression, cell signaling, cell cycle and death. Most importantly, several miRNA targets were associated with GO categories “reproduction (GO:0000003)”, “developmental process involved in reproduction (GO:0003006)”, “embryo development (GO:0009790)” and “embryonic morphogenesis (GO:0048598)” (supplementary material 2).
And 13 genes were found respectively on reproductive system and embryo phenotype of mice KO models studies among the 179 hubs
By evaluating the effects derived from the depletion of genes among the 179 hubs of the Murine Oviductal MiRNome_MC, we found that 11 genes have been studied on mice KO models due to their relationship with the reproductive system (Table 4 and supplementary material 3), while 13 genes were the focus of works related to the embryo phenotype (Table 5 and supplementary material 3). Among these miRNAs target genes, three of them, namely Afdn, Itpr1 and Med1, were found to be related to both analyzed functions in knock-out mice (i.e., reproductive system and embryo phenotype), while only Rac1 showed a much greater number of links with respect to the other genes (93 versus 30-17).
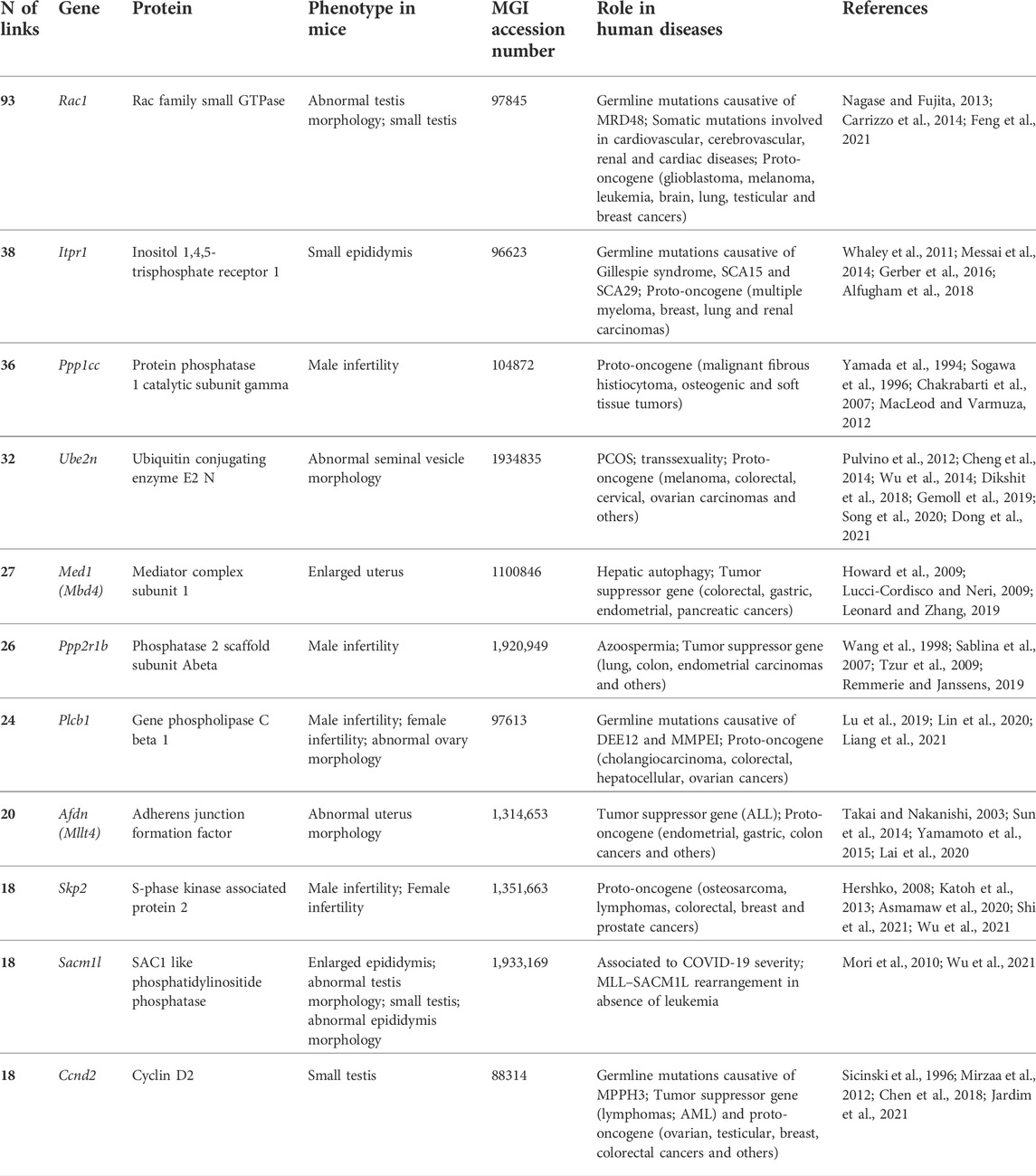
TABLE 4. Genes studied in mice KO models in terms of their relationship with the reproductive system. The table shows the number of links for each gene, the genes and the protein for which codifies, the phenotype in mice with the corresponding MGI (mouse genome informatic database accession number), the role in human diseases and their corresponding references. PCOS: Polycystic ovary syndrome; ALL: Acute lymphocytic leukemia; MLL: mixed lineage leukemia; AML: acute myeloid leukemia; MPPH: Megalencephaly-polymicrogyria-polydactyly-hydrocephalus.
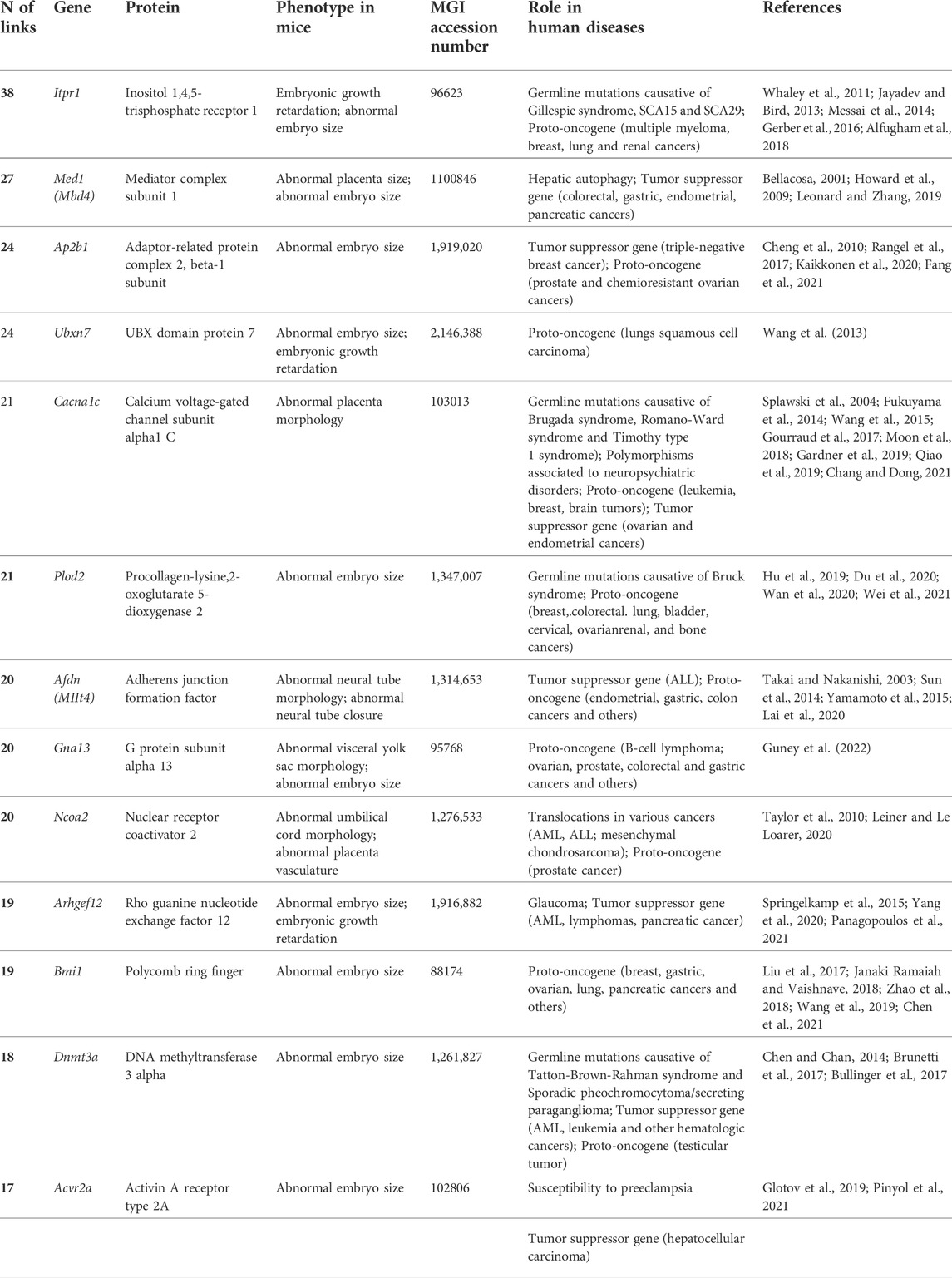
TABLE 5. Genes studied in mice KO models in terms of their relationship with the embryo phenotype. The table shows the number of links for each gene, the genes and the protein for which codifies, the phenotype in mice with the corresponding MGI (mouse genome informatic database accession number), the role in human diseases and their corresponding references. ALL: Acute lymphocytic leukemia; AML: acute myeloid leukemia.
Discussion
The potential role of miRNAs in the regulation of genes during fertilization and early embryo development has recently attracted the attention of several researchers (Gross et al., 2017; Reza et al., 2019; Salilew-Wondim et al., 2020). MicroRNAs are small (22 nucleotides) non-coding molecules involved in the control of cellular functions and that act generally as negative post-transcriptional regulators of mRNA (Lau et al., 2001). They have been shown to participate in numerous biological processes, including gametogenesis and embryo development (Salilew-Wondim et al., 2020), and are present in several tissues of the reproductive system such us testis, epididymis, spermatozoa and seminal plasma (Salas-Huetos et al., 2020), evincing their strong involvement in the reproductive field. Moreover, microRNAs are emerging as regulators of different physiological and pathological processes in fertilization and embryo development (Hossain et al., 2012; Gross et al., 2017; Tesfaye et al., 2018; Salilew-Wondim et al., 2020). Interestingly, they have been discovered also in the oviductal fluid (Almiñana et al., 2018; Fereshteh et al., 2018; Gonella-Diaza et al., 2021; Mazzarella et al., 2021), packaged within the extracellular vesicles (EVs, also called oviductosomes, OVS) (Gross et al., 2017; Fereshteh et al., 2018) that might act as natural nanoshuttles, bringing key components (lipids, proteins, RNA molecules and miRNAs) from the oviduct to the gametes or the embryos (Almiñana et al., 2018). As a result, the OVS may encapsulate miRNAs, preventing degradation and increasing their stability in OF. The oviductal EVs may then release their contents into the embryo via mechanisms such as membrane fusion or endocytosis. However, it is still unknown whether these vesicles can use more than one route or if vesicular uptake is cell type specific (Pavani et al., 2017). Little is known regarding their biogenesis but that are transcribed as primary miRNAs (pri-miRNAs) (Lee et al., 2002) and processed to precursor miRNAs (pre-miRNA) by the nuclear RNase III, DROSHA (Ha and Kim, 2014; Leitão and Enguita, 2022). Pre-miRNAs are then transferred to the cytoplasm, where an RNase III endonuclease DICER cleaves the pre-miRNA to the mature form of the miRNA (Ha and Kim, 2014). The mature miRNA generated by DICER is chained with Argonaute (AGO) protein to form an effector complex called RNA-induced silencing complex (RISC) able to exert its regulatory action (Ha and Kim, 2014; Leitão and Enguita, 2022). However, little is still known about the biological functions of miRNAs expressed in the EVs.
Here, we adopted a computational biology approach gathering the available data to reconstruct the pathway that may be activated by the oviductal miRNA in a mouse model. Mouse (Mus musculus) is the most commonly used animal model in biomedical research, including reproductive biology (Jamsai and O’Bryan, 2011; Ramal-Sanchez et al., 2021). Furthermore, the mouse model allows the assessment of IVF rates and early stages of embryo development, as well as the ascertainment of the resulting offspring in terms of health status and potential epigenetic modifications. Most importantly, because of their high genetic similarity to humans and ease of genetic manipulation, mice models are largely used to study the vast majority of human diseases (Rosenthal and Brown, 2007; Perlman, 2016). Nowadays, many types of mouse models, such as knockout/knockin, transgenic and chemical-mutagenized mutant mouse models have been made available not only for biomedical research but also to reveal disease-associated genes, including causes of male infertility (Jamsai and O’Bryan, 2011). Specifically, we realized the network representing the murine oviductal miRNome (MiRNome and MiRnome_MC), with the available published omic data. Once the network model was obtained, we assessed its topology to infer important biological information. As evident for the parameters listed in Table 3, the miRNome is a scale-free network that follows the Barabasi-Albert (BA) model. It means that the node degree distribution follows a power-law with a negative exponent and is not correlated with the clustering coefficient (Albert and Barabasi, 2002; Ravasz and Barabási, 2003). Thus, it is possible to identify a low number of highly connected nodes (the “hubs”) coexisting with a higher number of scarcely connected nodes (Barabási and Oltvai, 2004). These networks are characterized by a high robustness against the random damages and by an efficient information transfer. In particular, the hubs exert a significant control over the whole network, assuring that the information is spread within the network in a very robust, fast and efficient way and that the network is able to quickly response to internal and external stimuli (Albert and Barabasi, 2002; Ravasz and Barabási, 2003; Barabási and Oltvai, 2004).
In this regard, first we carried out the identification of the hyperlinked nodes and then investigated the pathway in which they are involved using the gene ontology enrichment analysis. In general, the functional enrichment analysis revealed that these genes codify for proteins that are involved in fundamental cellular functions, both structural (membrane, cell junction and cytoskeleton organization, collagen synthesis) and regulatory/signaling (cell cycle regulation, signal transduction, transcriptional activation, endocytosis, calcium channel activity, ubiquitination, dephosphorylation, methylation, chromatin repression), by referring to 4 and 18 genes, respectively. All these roles suggest their implication in fertilization and early embryo development, as evidenced by the effects of the gene deletion in KO mice on the reproductive system and embryo development (Tables 4 and 5). Our findings are consistent with previous research, since Fereshteh and coll. (2018) have demonstrated that murine EVs can deliver miRNAs to the sperm cells, such as miR-34c-5p, which is located in the sperm centromere and promotes the first zygote cleavage (Fereshteh et al., 2018). At the same time, murine EVs contain other miRNAs that may target several embryonic development-related genes (Fereshteh et al., 2018). In vitro, EVs supplementation altered the bovine transcriptome, implying that oviductal EVs miRNA cargo may have a potential role in controlling embryonic development (Bauersachs et al., 2020). Furthermore, it has been recently showed that the expressed miRNA in bovine EVs modulate different pathways, including PI3K/AKT, mTOR and MAPK, which are related to transcription, translation, proliferation, growth, control of the cytoskeletal organization and metabolism, and that may influence the early embryo development within the oviduct (Mazzarella et al., 2021). PI3K/AKT/mTOR signaling pathways also regulates angiogenesis (Karar and Maity, 2011), a crucial process for the proper functioning of the female reproductive system and for pregnancy establishment (Rizov et al., 2017). Additionally, several reports have suggested that the deletion of miRNA processing genes may have a serious effect on reproductive functions or embryo development, mostly due to altered miRNA levels (Kaczmarek et al., 2020). Notably, the loss of Dicer1 and Ago2 in mice are embryonically lethal (Bernstein et al., 2003; Yang et al., 2005; Alisch et al., 2007). Among others, the deficiency of Dicer in the mouse reproductive tract hampered uterine development, resulting in pregnancy loss after wild-type embryo transfer (Gonzalez and Behringer, 2009). Thus, microRNAs are involved in several processes affecting the function of the reproductive system, as well as the conception, the implantation process, and the embryonic development.
Noteworthy, all events in the oviduct are orchestrated by the neuroendocrine axis through the dynamic changes induced by steroid hormones. In a recent study, Almiñana and coll. (2018) showed interesting differences in the miRNAs content and the protein composition of oviductal extracellular vesicles isolated from cows at various stages of the estrous cycle, suggesting that ovarian steroid hormones may regulate the EVs production and secretion (Almiñana et al., 2018). These changes, directed by the neuroendocrine axis, are reflected on the early embryonic genome reprogramming. In fact, the delicate process known as the maternal-zygotic transition (MZT) occurs in the oviduct (Baroux et al., 2008). In mammalian pre-implantation embryos, the maternal gene products regulate the initial events of embryogenesis, while the zygotic genome remains transcriptionally silent (Baroux et al., 2008; Hamm and Harrison, 2018). Developmental control is then passed from the mother to the zygote (Hamm and Harrison, 2018). In addition, a recent comprehensive characterization of the bovine OF proteome revealed a spatiotemporal regulation of the OF according to the anatomical region of the oviduct, the proximity to the ovulating ovary and the stage of the cycle (Mahé et al., 2022). Future approaches should investigate the potential involvement of miRNA in the fine-tune regulation of the oviductal microenvironment.
Importantly, miRNAs play a significant role in reproduction and may represent excellent research candidates with the potential to improve the understanding of the complex landscape in which fertilization occurs, as well as the underlying molecular mechanisms that prevent implantation and embryo progression. This knowledge may be useful in developing new in vitro fertilization (IVF) systems that mimic the physiological condition as closely as possible. Indeed, the current IVF systems lack the interaction of gametes with several components naturally present in the reproductive tract during fertilization and the early stages of development, that may be responsible for the impaired in vitro development and viability, but also for some epigenetic changes in in vitro-produced embryos, resulting in imprinting disorders such as Beckwith-Wiedemann Syndrome (BWS) and Angelman syndrome (AS) (Harris et al., 2020). Therefore, detailed knowledge on oviductal transcriptome and secretome are needed to develop better embryo culture medium and conditions in order to reduce the adverse periconceptional environment in in vitro derived embryos.
Interestingly, 7 out of the 21 protein-coding genes studied here (RAC1, ITPR1, PLCB1, CACNA1C, PLOD2, CCND2, DNMT3A) are causative of inherited monogenic diseases when germinally mutated in humans, and all of them (with the possible exclusion of SACM1L), result to be deregulated in several types of human cancers when somatically mutated. As such, they can be classified as proto-oncogenes (RAC1, ITPR1, PPP1CC, UBE2N, PLCB1, UBXN7, PLOD2, GNA13, NCOA2, BML1, SKP2), tumor suppressor genes (MED1, PPP2R1B, ARHGEF12, ACVR2A) or both (AP2B1, CCANA1C, AFDN, CCND2, DNMT3A) (Tables 4 and 5). This last observation highlights the importance of oviductal miRNAs in acting themselves as tumor suppressor genes or oncogenes, depending on the cancer type and cellular context (Barbato et al., 2017; Ghafouri-Fard et al., 2020).
The most prominent role as proto-oncogenes and the fact that at least 9 of these oviductal miRNA-target genes (PPP1CC, PPP2R1B, CCND2, ACVR2A, MED1, NCOA2, PLCB1, ARHGEF12, GNA13) are involved in proliferation regulatory pathways (i.e. cell cycle regulation, transcriptional activation, signal transduction) may explain the observed effect of their deletion in KO mice on embryo development, suggesting as the embryo could be the mainly sensitive target of their action.
Regarding the reproductive system, three of these genes (UBE2N, PPP2R1B and ACVR2A) have been shown to be involved in reproductive disfunctions in humans (polycystic ovary syndrome and transsexuality, azoospermia, and susceptibility to preeclampsia, respectively (Fitzpatrick et al., 2009; Dong et al., 2021; Du et al., 2021). So far, a total of 15 genes out of 21 (UBE2N, PPP2R1B, CACNA1C, AP2B1, NCOA2, RAC1, MED1, PLOD2, GNA13, AFDN, CCND2, BMI1, PLCB1, DNMT3A and SKP2) have been associated to tumors of the female or male reproductive tracts (cervical carcinoma, ovarian, endometrial, prostate, and testicular cancers (Tables 4 and 5), in addition to other types of cancers or diseases. All the human orthologs genes have been better described in Supplementary material 4, including their functional role and implications in human diseases, both inherited and acquired.
These results support the idea that understanding the role of oviductal miRNAs in human cancer pathogenesis could be fundamental for their application as biomarkers and as potential therapeutic options for malignancies treatments. More specifically, a recent in vitro study has shown the involvement of 20 specific miRNAs in promoting the development and progression of ovarian carcinoma, the most aggressive and hard-to-detect gynecological cancer worldwide (Loginov et al., 2022). More recently, the protective role exerted by two miRNAs, miR-145 and miR-93-5p, in suppressing ovarian cancer cell proliferation and migration through a negative transcriptional regulation of the proto-oncogene CCND2 have been described in two different studies (Hua et al., 2019; Chen et al., 2022).
In conclusion, the adoption of a biological network-based approach allowed us to infer new and interesting processes involved in fertilization and in the early embryo development, demonstrating the utility of computational modelling strategies in the reproductive field. Our data clearly revealed that the targets of the miRNAs are involved in processes critical for fertilization and early embryo development. Interestingly, data from the KO mice model seem to support the biological relevance of our findings; nevertheless, more functional experiments are required to further our understanding of the role of oviductal miRNA in fertilization or early embryo development. However, we highlight here the importance of studying the miRNA profiles and their enormous potential as tools to improve the assisted reproduction techniques (ARTs) currently used in human and animal reproduction and as biomarkers or potential therapeutic options for malignancies treatments in humans.
Data availability statement
The original contributions presented in the study are included in the article/Supplementary material, further inquiries can be directed to the corresponding author.
Author contributions
AT, CC, and NB, conceptualized the work and performed the networks; all Authors curated the data and performed the formal analysis; AT, CC, AC, and NB. prepared the original draft; all Authors reviewed and edited the original draft and contributed to the writing; AT, CC, LV. and MR-S. prepared the figures; NB and BB. supervised and funded the research. All Authors reviewed and approved the publication of this manuscript in its current form.
Conflict of interest
The authors declare that the research was conducted in the absence of any commercial or financial relationships that could be construed as a potential conflict of interest.
Publisher’s note
All claims expressed in this article are solely those of the authors and do not necessarily represent those of their affiliated organizations, or those of the publisher, the editors and the reviewers. Any product that may be evaluated in this article, or claim that may be made by its manufacturer, is not guaranteed or endorsed by the publisher.
Supplementary material
The Supplementary Material for this article can be found online at: https://www.frontiersin.org/articles/10.3389/fcell.2022.1015360/full#supplementary-material
References
Albert, R., and Barabasi, A. L. (2002). Statistical mechanics of complex networks. Rev. Mod. Phys. 74, 47–97. doi:10.1088/1478-3967/1/3/006
Alfugham, N., Gadoth, A., Lennon, V. A., Komorowski, L., Scharf, M., Hinson, S., et al. (2018). ITPR1 autoimmunity: Frequency, neurologic phenotype, and cancer association. Neurol. Neuroimmunol. NeuroInflammation 5, 418. doi:10.1212/NXI.0000000000000418
Alisch, R. S., Jin, P., Epstein, M., Caspary, T., and Warren, S. T. (2007). Argonaute2 is essential for mammalian gastrulation and proper mesoderm formation. PLoS Genet. 3, e227–e2571. doi:10.1371/JOURNAL.PGEN.0030227
Almiñana, C., and Bauersachs, S. (2019). Extracellular vesicles in the oviduct: Progress, challenges and implications for the reproductive success. Bioengineering 6, 32. doi:10.3390/bioengineering6020032
Almiñana, C., Tsikis, G., Labas, V., Uzbekov, R., da Silveira, J. C., Bauersachs, S., et al. (2018). Deciphering the oviductal extracellular vesicles content across the estrous cycle: Implications for the gametes-oviduct interactions and the environment of the potential embryo. BMC Genomics 19, 622. doi:10.1186/s12864-018-4982-5
Asaadi, A., Dolatabad, N. A., Atashi, H., Raes, A., Van Damme, P., Hoelker, M., et al. (2021). Extracellular vesicles from follicular and ampullary fluid isolated by density gradient ultracentrifugation improve bovine embryo development and quality. Int. J. Mol. Sci. 22, 5788–E616. doi:10.3390/IJMS22020578
Ashburner, M., Ball, C. A., Blake, J. A., Botstein, D., Butler, H., Cherry, J. M., et al. (2000). Gene ontology: Tool for the unification of biology. The gene ontology Consortium. Nat. Genet. 25, 25–29. doi:10.1038/75556
Asmamaw, M. D., Liu, Y., Zheng, Y. C., Shi, X. J., and Liu, H. M. (2020). Skp2 in the ubiquitin-proteasome system: A comprehensive review. Med. Res. Rev. 40, 1920–1949. doi:10.1002/MED.21675
Avilés, M., Gutiérrez-Adán, A., and Coy, P. (2010). Oviductal secretions: Will they be key factors for the future ARTs? Mol. Hum. Reprod. 16, 896–906. doi:10.1093/molehr/gaq056
Baldi, E., Luconi, M., Muratori, M., Marchiani, S., Tamburrino, L., and Forti, G. (2009). Nongenomic activation of spermatozoa by steroid hormones: Facts and fictions. Mol. Cell. Endocrinol. 308, 39–46. doi:10.1016/j.mce.2009.02.006
Ballester, L., Romero-Aguirregomezcorta, J., Soriano-Úbeda, C., Matás, C., Romar, R., and Coy, P. (2014). Timing of oviductal fluid collection, steroid concentrations, and sperm preservation method affect porcine in vitro fertilization efficiency. Fertil. Steril. 102, 1762–1768. doi:10.1016/j.fertnstert.2014.08.009
Barabási, A. L., and Oltvai, Z. N. (2004). Network biology: Understanding the cell’s functional organization. Nat. Rev. Genet. 5, 101–113. doi:10.1038/nrg1272
Barbato, S., Solaini, G., and Fabbri, M. (2017). MicroRNAs in oncogenesis and tumor suppression. Int. Rev. Cell Mol. Biol. 333, 229–268. doi:10.1016/BS.IRCMB.2017.05.001
Baroux, C., Autran, D., Gillmor, C. S., Grimanelli, D., and Grossniklaus, U. (2008). The maternal to zygotic transition in animals and plants. Cold Spring Harb. Symp. Quant. Biol. 73, 89–100. doi:10.1101/sqb.2008.73.053
Bauersachs, S., Mermillod, P., and Almiñana, C. (2020). The oviductal extracellular vesicles’ RNA cargo regulates the bovine embryonic transcriptome. Int. J. Mol. Sci. 21, 1303. doi:10.3390/ijms21041303
Bellacosa, A. (2001). Role of MED1 (MBD4) Gene in DNA repair and human cancer. J. Cell. Physiol. 187, 137–144. doi:10.1002/JCP.1064
Bernabò, N., Ramal-Sanchez, M., Valbonetti, L., Machado-Simoes, J., Ordinelli, A., Capacchietti, G., et al. (2019). Cyclin–cdk complexes are key controllers of capacitation-dependent actin dynamics in mammalian spermatozoa. Int. J. Mol. Sci. 20, 42366–E4316. doi:10.3390/ijms20174236
Bernstein, E., Kim, S. Y., Carmell, M. A., Murchison, E. P., Alcorn, H., Li, M. Z., et al. (2003). Dicer is essential for mouse development. Nat. Genet. 35, 215–217. doi:10.1038/NG1253
Bridi, A., Perecin, F., and da Silveira, J. C. (2020). Extracellular vesicles mediated early embryo–maternal interactions. Int. J. Mol. Sci. 21, 1163. doi:10.3390/ijms21031163
Brunetti, L., Gundry, M. C., and Goodell, M. A. (2017). DNMT3A in leukemia. Cold Spring Harb. Perspect. Med. 7, a030320. doi:10.1101/CSHPERSPECT.A030320
Bullinger, L., Döhner, K., and Dohner, H. (2017). Genomics of acute myeloid leukemia diagnosis and pathways. J. Clin. Oncol. 35, 934–946. doi:10.1200/JCO.2016.71.2208
Canha-Gouveia, A., Paradela, A., Ramos-Fernández, A., Prieto-Sánchez, M. T., Sánchez-Ferrer, M. L., Corrales, F., et al. (2019). Which low-abundance proteins are present in the human milieu of gamete/embryo maternal interaction? Int. J. Mol. Sci. 20, 5305. doi:10.3390/ijms20215305
Carrizzo, A., Forte, M., Lembo, M., Formisano, L., Puca, A., and Vecchione, C. (2014). Rac-1 as a new therapeutic target in cerebro- and cardio-vascular diseases. Curr. Drug Targets 15, 1231–1246. doi:10.2174/1389450115666141027110156
Chakrabarti, R., Cheng, L., Puri, P., Soler, D., and Vijayaraghavan, S. (2007). Protein phosphatase PP1γ2 in sperm morphogenesis and epididymal initiation of sperm motility. Asian J. Androl. 9, 445–452. doi:10.1111/j.1745-7262.2007.00307.x
Chang, X., and Dong, Y. (2021). CACNA1C is a prognostic predictor for patients with ovarian cancer. J. Ovarian Res. 14, 88. doi:10.1186/S13048-021-00830-Z
Chen, B. F., and Chan, W. Y. (2014). The de novo DNA methyltransferase DNMT3A in development and cancer. Epigenetics 9, 669–677. doi:10.4161/EPI.28324
Chen, C., Xia, E., Bhandari, A., Wang, Y., Shen, Y., Sindan, N., et al. (2018). LncRNA CCND2-AS1 is up-regulated and regulates proliferation, migration, and invasion in breast cancer. Int. J. Clin. Exp. Pathol. 11, 1453–1459.
Chen, G., Yan, Y., Qiu, X., Ye, C., Jiang, X., Song, S., et al. (2022). miR-93-5p suppresses ovarian cancer malignancy and negatively regulate CCND2 by binding to its 3’UTR region. Discov. Oncol. 13, 15. doi:10.1007/S12672-022-00478-1
Chen, M. H., Fu, L. S., Zhang, F., Yang, Y., and Wu, X. Z. (2021). LncAY controls BMI1 expression and activates BMI1/Wnt/β-catenin signaling axis in hepatocellular carcinoma. Life Sci. 280, 119748. doi:10.1016/J.LFS.2021.119748
Chen, Y., and Wang, X. (2020). miRDB: an online database for prediction of functional microRNA targets. Nucleic Acids Res. 48, D127–D131. doi:10.1093/NAR/GKZ757
Cheng, J., Fan, Y. H., Xu, X., Zhang, H., Dou, J., Tang, Y., et al. (2014). A small-molecule inhibitor of UBE2N induces neuroblastoma cell death via activation of p53 and JNK pathways. Cell Death Dis. 5, e1079. doi:10.1038/CDDIS.2014.54
Cheng, L., Lu, W., Kulkarni, B., Pejovic, T., Yan, X., Chiang, J. H., et al. (2010). Analysis of chemotherapy response programs in ovarian cancers by the next generation sequencing technologies. Gynecol. Oncol. 117, 159–169. doi:10.1016/J.YGYNO.2010.01.041
Coy, P., García-Vázquez, F. A., Visconti, P. E., and Avilés, M. (2012). Roles of the oviduct in mammalian fertilization. Reproduction 144, 649–660. doi:10.1530/REP-12-0279
Coy, P., and Yanagimachi, R. (2015). The common and species-specific roles of oviductal proteins in mammalian fertilization and embryo development. Bioscience 65, 973–984. doi:10.1093/biosci/biv119
Dikshit, A., Zhang, J. Y., Dikshit, A., and Zhang, J. Y. (2018). UBE2N plays a pivotal role in maintaining melanoma malignancy. Oncotarget 9, 37347–37348. doi:10.18632/ONCOTARGET.26482
Doncheva, N. T., Morris, J. H., Gorodkin, J., and Jensen, L. J. (2019). Cytoscape StringApp: Network analysis and visualization of proteomics data. J. Proteome Res. 18, 623–632. doi:10.1021/acs.jproteome.8b00702
Dong, R., Gao, S., and Shan, M. J. (2021). Identification of the similarly expressed genes in patients with polycystic ovary syndrome and transsexuals. Med. Baltim. 100, e26990. doi:10.1097/MD.0000000000026990
Du, M., Yuan, L., Zhang, Z., Zhang, C., Zhu, M., Zhang, Z., et al. (2021). PPP2R1B is modulated by ubiquitination and is essential for spermatogenesis. FASEB J. 35, e21564. doi:10.1096/FJ.202002810R
Du, W., Liu, N., Zhang, Y., Liu, X., Yang, Y., Chen, W., et al. (2020). PLOD2 promotes aerobic glycolysis and cell progression in colorectal cancer by upregulating HK2. Biochem. Cell Biol. 98, 386–395. doi:10.1139/BCB-2019-0256
Einer-Jensen, N., and Hunter, R. H. F. (2005). Counter-current transfer in reproductive biology. Reproduction 129, 9–18. doi:10.1530/REP.1.00278
Ezzati, M., Djahanbakhch, O., Arian, S., and Carr, B. R. (2014). Tubal transport of gametes and embryos: A review of physiology and pathophysiology. J. Assist. Reprod. Genet. 31, 1337–1347. doi:10.1007/S10815-014-0309-X
Fang, W., Liao, C., Shi, R., Simon, J. M., Ptacek, T. S., Zurlo, G., et al. (2021). ZHX2 promotes HIF1α oncogenic signaling in triple-negative breast cancer. Elife 10, e70412. doi:10.7554/ELIFE.70412
Feng, X., Zhang, H., Meng, L., Song, H., Zhou, Q., Qu, C., et al. (2021). Hypoxia-induced acetylation of PAK1 enhances autophagy and promotes brain tumorigenesis via phosphorylating ATG5. Autophagy 17, 723–742. doi:10.1080/15548627.2020.1731266
Fereshteh, Z., Schmidt, S. A., Al-Dossary, A. A., Accerbi, M., Arighi, C., Cowart, J., et al. (2018). Murine Oviductosomes (OVS) microRNA profiling during the estrous cycle: Delivery of OVS-borne microRNAs to sperm where miR-34c-5p localizes at the centrosome. Sci. Rep. 8, 16094–16118. doi:10.1038/s41598-018-34409-4
Fitzpatrick, E., Johnson, M. P., Dyer, T. D., Forrest, S., Elliott, K., Blangero, J., et al. (2009). Genetic association of the activin A receptor gene (ACVR2A) and pre-eclampsia. Mol. Hum. Reprod. 15, 195–204. doi:10.1093/MOLEHR/GAP001
Foresta, C., Rossato, M., Mioni, R., and Zorzi, M. (2009). Progesterone induces capacitation in human spermatozoa. Andrologia 24, 33–35. doi:10.1111/j.1439-0272.1992.tb02605.x
Fujinoki, M., Takei, G. L., and Kon, H. (2016). Non-genomic regulation and disruption of spermatozoal in vitro hyperactivation by oviductal hormones. J. Physiol. Sci. 66, 207–212. doi:10.1007/s12576-015-0419-y
Fukuyama, M., Wang, Q., Kato, K., Ohno, S., Ding, W. G., Toyoda, F., et al. (2014). Long QT syndrome type 8: Novel CACNA1C mutations causing QT prolongation and variant phenotypes. Europace 16, 1828–1837. doi:10.1093/EUROPACE/EUU063
Gadella, B. M., and Boerke, A. (2016). An update on post-ejaculatory remodeling of the sperm surface before mammalian fertilization. Theriogenology 85, 113–124. doi:10.1016/J.THERIOGENOLOGY.2015.07.018
Gardner, R. J. M. K., Crozier, I. G., Binfield, A. L., Love, D. R., Lehnert, K., Gibson, K., et al. (2019). Penetrance and expressivity of the R858H CACNA1C variant in a five-generation pedigree segregating an arrhythmogenic channelopathy. Mol. Genet. genomic Med. 7, e00476. doi:10.1002/MGG3.476
Gemoll, T., Miroll, E., Klein, O., Lischka, A., Eravci, M., Thorns, C., et al. (2019). Spatial UBE2N protein expression indicates genomic instability in colorectal cancers. BMC Cancer 19, 710. doi:10.1186/s12885-019-5856-1
Gerber, S., Alzayady, K. J., Burglen, L., Brémond-Gignac, D., Marchesin, V., Roche, O., et al. (2016). Recessive and dominant de novo ITPR1 mutations cause gillespie syndrome. Am. J. Hum. Genet. 98, 971–980. doi:10.1016/J.AJHG.2016.03.004
Ghafouri-Fard, S., Shoorei, H., and Taheri, M. (2020). miRNA profile in ovarian cancer. Exp. Mol. Pathol. 113, 104381. doi:10.1016/J.YEXMP.2020.104381
Glotov, A. S., Kazakov, S. V., Vashukova, E. S., Pakin, V. S., Danilova, M. M., Nasykhova, Y. A., et al. (2019). Targeted sequencing analysis of ACVR2A gene identifies novel risk variants associated with preeclampsia. J. Matern. Fetal. Neonatal Med. 32, 2790–2796. doi:10.1080/14767058.2018.1449204
Gonella-Diaza, A. M., Lopes, E., da Silva, K. R., Nociti, R. P., Andrade, G. M., Atuesta-Bustos, J. E., et al. (2021). Steroidal regulation of oviductal micrornas is associated with microrna-processing in beef cows. Int. J. Mol. Sci. 22, 953–1025. doi:10.3390/ijms22020953
Gonzalez, G., and Behringer, R. R. (2009). Dicer is required for female reproductive tract development and fertility in the mouse. Mol. Reprod. Dev. 76, 678–688. doi:10.1002/MRD.21010
Gourraud, J. B., Barc, J., Thollet, A., Le Marec, H., and Probst, V. (2017). Brugada syndrome: Diagnosis, risk stratification and management. Arch. Cardiovasc. Dis. 110, 188–195. doi:10.1016/J.ACVD.2016.09.009
Gross, N., Kropp, J., and Khatib, H. (2017). MicroRNA signaling in embryo development. Biol. (Basel) 6, 34. doi:10.3390/biology6030034
Guidobaldi, H. A., Teves, M. E., Uñates, D. R., Anastasía, A., and Giojalas, L. C. (2008). Progesterone from the cumulus cells is the sperm chemoattractant secreted by the rabbit oocyte cumulus complex. PLoS One 3, e3040. doi:10.1371/journal.pone.0003040
Guney, E., Lucas, C.-H. G., Qi, Z., Yu, J., Zhang, R., Ohgami, R. S., et al. (2022). A genetically distinct pediatric subtype of primary CNS large B-cell lymphoma is associated with favorable clinical outcome. Blood Adv. 6, 3189–3193. doi:10.1182/BLOODADVANCES.2021006018
Ha, M., and Kim, V. N. (2014). Regulation of microRNA biogenesis. Nat. Rev. Mol. Cell Biol. 15, 509–524. doi:10.1038/nrm3838
Hamm, D. C., and Harrison, M. M. (2018). Regulatory principles governing the maternal-to-zygotic transition: Insights from Drosophila melanogaster. Open Biol. 8, 180183. doi:10.1098/rsob.180183
Harris, E. A., Stephens, K. K., and Winuthayanon, W. (2020). Extracellular vesicles and the oviduct function. Int. J. Mol. Sci. 21, 82800–E8320. doi:10.3390/ijms21218280
Hershko, D. D. (2008). Oncogenic properties and prognostic implications of the ubiquitin ligase Skp2 in cancer. Cancer 112, 1415–1424. doi:10.1002/CNCR.23317
Hossain, M. M., Salilew-Wondim, D., Schellander, K., and Tesfaye, D. (2012). The role of microRNAs in mammalian oocytes and embryos. Anim. Reprod. Sci. 134, 36–44. doi:10.1016/j.anireprosci.2012.08.009
Howard, J. H., Frolov, A., Tzeng, C. W. D., Stewart, A., Midzak, A., Majmundar, A., et al. (2009). Epigenetic downregulation of the DNA repair gene MED1/MBD4 in colorectal and ovarian cancer. Cancer Biol. Ther. 8, 94–100. doi:10.4161/CBT.8.1.7469
Hu, H.-L., Wang, C.-F., Wei, X.-H., Lv, J.-X., Cao, X.-H., Shi, Y.-Y., et al. (2019). Correlation between procollagen-lysine, 2-oxoglutarate 5-dioxygenase 2 and breast cancer. Int. J. Clin. Exp. Pathol. 12, 1015–1021.
Hua, M., Qin, Y., Sheng, M., Cui, X., Chen, W., Zhong, J., et al. (2019). MiR-145 suppresses ovarian cancer progression via modulation of cell growth and invasion by targeting CCND2 and E2F3. Mol. Med. Rep. 49, 3575–3583. doi:10.3892/mmr.2019.10004
Jamsai, D., and O’Bryan, M. K. (2011). Mouse models in male fertility research. Asian J. Androl. 13, 139–151. doi:10.1038/AJA.2010.101
Janaki Ramaiah, M., and Vaishnave, S. (2018). BMI1 and pten are key determinants of breast cancer therapy: A plausible therapeutic target in breast cancer. Gene 678, 302–311. doi:10.1016/J.GENE.2018.08.022
Jardim, D. L., Millis, S. Z., Ross, J. S., Woo, M. S.-A., Ali, S. M., and Kurzrock, R. (2021). Cyclin pathway genomic alterations across 190, 247 solid tumors: Leveraging large-scale data to inform therapeutic directions. Oncologist 26, e78–e89. doi:10.1634/THEONCOLOGIST.2020-0509
Jayadev, S., and Bird, T. D. (2013). Hereditary ataxias: Overview. Genet. Med. 15, 673–683. doi:10.1038/GIM.2013.28
Kaczmarek, M. M., Najmula, J., Guzewska, M. M., and Przygrodzka, E. (2020). MiRNAs in the peri-implantation period: Contribution to embryo-maternal communication in pigs. Int. J. Mol. Sci. 21, 22299–E2316. doi:10.3390/ijms21062229
Kaikkonen, E., Takala, A., Pursiheimo, J. P., Wahlström, G., and Schleutker, J. (2020). The interactome of the prostate-specific protein Anoctamin 7. Cancer Biomark. 28, 91–100. doi:10.3233/CBM-190993
Karar, J., and Maity, A. (2011). PI3K/AKT/mTOR pathway in angiogenesis. Front. Mol. Neurosci. 4, 51. doi:10.3389/FNMOL.2011.00051
Katoh, M., Igarashi, M., Fukuda, H., Nakagama, H., and Katoh, M. (2013). Cancer genetics and genomics of human FOX family genes. Cancer Lett. 328, 198–206. doi:10.1016/J.CANLET.2012.09.017
Lai, Y., Xu, P., Wang, J., Xu, K., Wang, L., and Meng, Y. (2020). Tumour suppressive long non-coding RNA AFDN-DT inhibits gastric cancer invasion via transcriptional regulation. J. Cell. Mol. Med. 24, 3157–3166. doi:10.1111/JCMM.14988
Lau, N. C., Lim, L. P., Weinstein, E. G., and Bartel, D. P. (2001). An abundant class of tiny RNAs with probable regulatory roles in Caenorhabditis elegans. Science 294, 858–862. doi:10.1126/SCIENCE.1065062
Lee, Y., Jeon, K., Lee, J. T., Kim, S., and Kim, V. N. (2002). MicroRNA maturation: Stepwise processing and subcellular localization. EMBO J. 21, 4663–4670. doi:10.1093/EMBOJ/CDF476
Leiner, J., and Le Loarer, F. (2020). The current landscape of rhabdomyosarcomas: An update. Virchows Arch. 476, 97–108. doi:10.1007/S00428-019-02676-9
Leitão, A. L., and Enguita, F. J. (2022). A structural view of miRNA biogenesis and function. Noncoding. RNA 8, 10. doi:10.3390/NCRNA8010010
Leonard, M., and Zhang, X. (2019). Estrogen receptor coactivator Mediator Subunit 1 (MED1) as a tissue-specific therapeutic target in breast cancer. J. Zhejiang Univ. Sci. B 20, 381–390. doi:10.1631/JZUS.B1900163
Li, S., and Winuthayanon, W. (2017). Oviduct: Roles in fertilization and early embryo development. J. Endocrinol. 232, R1–R26. doi:10.1530/JOE-16-0302
Liang, S., Guo, H., Ma, K., Li, X., Wu, D., Wang, Y., et al. (2021). A PLCB1-PI3K-AKT signaling Axis Activates EMT to promote cholangiocarcinoma progression. Cancer Res. 81, 5889–5903. doi:10.1158/0008-5472.CAN-21-1538/674082/AM/A-PLCB1-PI3K-AKT-SIGNALING-AXIS-ACTIVATES-EMT-TO
Lin, D., Fu, Z., Yang, G., Gao, D., Wang, T., Liu, Z., et al. (2020). Exportin-5 SUMOylation promotes hepatocellular carcinoma progression. Exp. Cell Res. 395, 112219. doi:10.1016/J.YEXCR.2020.112219
Liu, X., Wei, W., Li, X., Shen, P., Ju, D., Wang, Z., et al. (2017). BMI1 and MEL18 promote colitis-associated cancer in mice via REG3B and STAT3. Gastroenterology 153, 1607–1620. doi:10.1053/J.GASTRO.2017.07.044
Loginov, V. I., Pronina, I. V., Filippova, E. A., Burdennyy, A. M., Lukina, S. S., Kazubskaya, T. P., et al. (2022). Aberrant methylation of 20 miRNA genes specifically involved in various steps of ovarian carcinoma spread: From primary tumors to peritoneal macroscopic metastases. Int. J. Mol. Sci. 23, 1300. doi:10.3390/IJMS23031300
López-Torres, A. S., and Chirinos, M. (2017). Modulation of human sperm capacitation by progesterone, estradiol, and luteinizing hormone. Reprod. Sci. 24, 193–201. doi:10.1177/1933719116641766
Lu, M. L., Zhang, Y., Li, J., Fu, Y., Li, W. H., Zhao, G. F., et al. (2019). MicroRNA-124 inhibits colorectal cancer cell proliferation and suppresses tumor growth by interacting with PLCB1 and regulating Wnt/β-catenin signaling pathway. Eur. Rev. Med. Pharmacol. Sci. 23, 121–136. doi:10.26355/EURREV_201901_16756
Lucci-Cordisco, E., and Neri, G. (2009). Silent beginning: Early silencing of the MED1/MBD4 gene in colorectal tumorigenesis. Cancer Biol. Ther. 8, 192–193. doi:10.4161/CBT.8.2.7647
MacLeod, G., and Varmuza, S. (2012). Tandem affinity purification in transgenic mouse embryonic stem cells identifies DDOST as a novel PPP1CC2 interacting protein. Biochemistry 51, 9678–9688. doi:10.1021/BI3010158/SUPPL_FILE/BI3010158_SI_003.PDF
Mahé, C., Lavigne, R., Com, E., Pineau, C., Locatelli, Y., Zlotkowska, A. M., et al. (2022). Spatiotemporal profiling of the bovine oviduct fluid proteome around the time of ovulation. Sci. Rep. 12, 4135–4216. doi:10.1038/s41598-022-07929-3
Mazzarella, R., Bastos, N. M., Bridi, A., del Collado, M., Andrade, G. M., Pinzon, J., et al. (2021). Changes in oviductal cells and small extracellular vesicles miRNAs in pregnant cows. Front. Vet. Sci. 8, 639752–639814. doi:10.3389/fvets.2021.639752
Messai, Y., Noman, M. Z., Hasmim, M., Janji, B., Tittarelli, A., Boutet, M., et al. (2014). ITPR1 protects renal cancer cells against natural killer cells by inducing autophagy. Cancer Res. 74, 6820–6832. doi:10.1158/0008-5472.CAN-14-0303
Mirzaa, G. M., Conway, R. L., Gripp, K. W., Lerman-Sagie, T., Siegel, D. H., DeVries, L. S., et al. (2012). Megalencephaly-capillary malformation (MCAP) and megalencephaly-polydactyly-polymicrogyria-hydrocephalus (MPPH) syndromes: Two closely related disorders of brain overgrowth and abnormal brain and body morphogenesis. Am. J. Med. Genet. A 158A, 269–291. doi:10.1002/AJMG.A.34402
Mondéjar, I., Acuña, O. S., Izquierdo-Rico, M. J., Coy, P., and Avilés, M. (2012). The oviduct: Functional genomic and proteomic approach. Reprod. Domest. Anim. 47, 22–29. doi:10.1111/j.1439-0531.2012.02027.x
Moon, A. L., Haan, N., Wilkinson, L. S., Thomas, K. L., and Hall, J. (2018). CACNA1C: Association with psychiatric disorders, behavior, and neurogenesis. Schizophr. Bull. 44, 958–965. doi:10.1093/SCHBUL/SBY096
Mori, T., Nishimura, N., Hasegawa, D., Kawasaki, K., Kosaka, Y., Uchide, K., et al. (2010). Persistent detection of a novel MLL-SACM1L rearrangement in the absence of leukemia. Leuk. Res. 34, 1398–1401. doi:10.1016/J.LEUKRES.2010.05.001
Nagase, M., and Fujita, T. (2013). Role of Rac1-mineralocorticoid-receptor signalling in renal and cardiac disease. Nat. Rev. Nephrol. 9, 86–98. doi:10.1038/nrneph.2012.282
Ordinelli, A., Bernabò, N., Orsini, M., Mattioli, M., and Barboni, B. (2018). Putative human sperm interactome: A networks study. BMC Syst. Biol. 12, 52–10. doi:10.1186/s12918-018-0578-6
Oren-Benaroya, R., Orvieto, R., Gakamsky, A., Pinchasov, M., and Eisenbach, M. (2008). The sperm chemoattractant secreted from human cumulus cells is progesterone. Hum. Reprod. 23, 2339–2345. doi:10.1093/humrep/den265
Panagopoulos, I., Andersen, K., Eilert-Olsen, M., Zeller, B., Munthe-Kaas, M. C., Buechner, J., et al. (2021). Therapy-induced deletion in 11q23 leading to fusion of KMT2A with ARHGEF12 and development of B lineage acute lymphoplastic leukemia in a child treated for acute myeloid leukemia caused by t(9;11)(p21;q23)/kmt2a-MLLT3. Cancer Genomics Proteomics 18, 67–81. doi:10.21873/CGP.20242
Pavani, K. C., Alminana, C., Wydooghe, E., Catteeuw, M., Ramírez, M. A., Mermillod, P., et al. (2017). Emerging role of extracellular vesicles in communication of preimplantation embryos in vitro. Reprod. Fertil. Dev. 29, 66–83. doi:10.1071/RD16318
Perlman, R. L. (2016). Mouse models of human disease: An evolutionary perspective. Evol. Med. Public Health 2016, 170–176. doi:10.1093/EMPH/EOW014
Pillai, V. V., Weber, D. M., Phinney, B. S., and Selvaraj, V. (2017). Profiling of proteins secreted in the bovine oviduct reveals diverse functions of this luminal microenvironment. PLoS One 12, e0188105–e0188122. doi:10.1371/journal.pone.0188105
Pinyol, R., Torrecilla, S., Wang, H., Montironi, C., Piqué-Gili, M., Torres-Martin, M., et al. (2021). Molecular characterisation of hepatocellular carcinoma in patients with non-alcoholic steatohepatitis. J. Hepatol. 75, 865–878. doi:10.1016/J.JHEP.2021.04.049
Pulvino, M., Liang, Y., Oleksyn, D., DeRan, M., Van Pelt, E., Shapiro, J., et al. (2012). Inhibition of proliferation and survival of diffuse large B-cell lymphoma cells by a small-molecule inhibitor of the ubiquitin-conjugating enzyme Ubc13-Uev1A. Blood 120, 1668–1677. doi:10.1182/BLOOD-2012-02-406074
Qiao, Z., Jiang, Y., Wang, L., Wang, L., Jiang, J., and Zhang, J. (2019). Mutations in KIAA1109, CACNA1C, BSN, AKAP13, CELSR2, and HELZ2 are associated with the prognosis in endometrial cancer. Front. Genet. 10, 909. doi:10.3389/fgene.2019.00909
Ramal-Sanchez, M., Fontana, A., Valbonetti, L., Ordinelli, A., Bernabò, N., and Barboni, B. (2021). Graphene and reproduction: A love-hate relationship. Nanomaterials 11, 547–616. doi:10.3390/nano11020547
Rangel, R., Guzman-Rojas, L., Kodama, T., Kodama, M., Newberg, J. Y., Copeland, N. G., et al. (2017). Identification of new tumor suppressor genes in triple-negative breast cancer. Cancer Res. 77, 4089–4101. doi:10.1158/0008-5472.CAN-17-0785
Ravasz, E., and Barabási, A.-L. (2003). Hierarchical organization in complex networks. Phys. Rev. E Stat. Nonlin. Soft Matter Phys. 67, 026112. doi:10.1103/PhysRevE.67.026112
Remmerie, M., and Janssens, V. (2019). PP2A: A promising biomarker and therapeutic target in endometrial cancer. Front. Oncol. 9, 462. doi:10.3389/FONC.2019.00462
Reza, A. M. M. T., Choi, Y. J., Han, S. G., Song, H., Park, C., Hong, K., et al. (2019). Roles of microRNAs in mammalian reproduction: From the commitment of germ cells to peri-implantation embryos. Biol. Rev. Camb. Philos. Soc. 94, 415–438. doi:10.1111/brv.12459
Rizov, M., Andreeva, P., and Dimova, I. (2017). Molecular regulation and role of angiogenesis in reproduction. Taiwan. J. Obstet. Gynecol. 56, 127–132. doi:10.1016/J.TJOG.2016.06.019
Rosenthal, N., and Brown, S. (2007). The mouse ascending: Perspectives for human-disease models. Nat. Cell Biol. 9, 993–999. doi:10.1038/ncb437
Sablina, A. A., Chen, W., Arroyo, J. D., Corral, L., Hector, M., Bulmer, S. E., et al. (2007). The tumor suppressor PP2A Abeta regulates the RalA GTPase. Cell 129, 969–982. doi:10.1016/J.CELL.2007.03.047
Saint-Dizier, M., Schoen, J., Chen, S., Banliat, C., and Mermillod, P. (2020). Composing the early embryonic microenvironment: Physiology and regulation of oviductal secretions. Int. J. Mol. Sci. 21, 2233–E321. doi:10.3390/ijms21010223
Salas-Huetos, A., James, E. R., Aston, K. I., Carrell, D. T., Jenkins, T. G., and Yeste, M. (2020). The role of miRNAs in male human reproduction: A systematic review. Andrology 8, 7–26. doi:10.1111/ANDR.12714
Salilew-Wondim, D., Gebremedhn, S., Hoelker, M., Tholen, E., Hailay, T., and Tesfaye, D. (2020). The role of micrornas in mammalian fertility: From gametogenesis to embryo implantation. Int. J. Mol. Sci. 21, 585. doi:10.3390/ijms21020585
Shi, L., Yan, Y., He, Y., Yan, B., Pan, Y., Orme, J. J., et al. (2021). Mutated SPOP E3 ligase promotes 17βHSD4 protein degradation to drive androgenesis and prostate cancer progression. Cancer Res. 81, 3593–3606. doi:10.1158/0008-5472.CAN-20-3258/673692/AM/MUTATED-SPOP-E3-LIGASE-PROMOTES-17-HSD4-PROTEIN
Sicinski, P., Donaher, J. L., Geng, Y., Parker, S. B., Gardner, H., Park, M. Y., et al. (1996). Cyclin D2 is an FSH-responsive gene involved in gonadal cell proliferation and oncogenesis. Nature 384, 470–474. doi:10.1038/384470A0
Sogawa, K., Yamada, T., Sugita, A., Kito, K., Tachibana, M., Nezu, K., et al. (1996). Role of protein phosphatase in malignant osteogenic and soft tissue tumors. Res. Commun. Mol. Pathol. Pharmacol. 93, 33–42.
Song, T. T., Xu, F., and Wang, W. (2020). Inhibiting ubiquitin conjugating enzyme E2 N by microRNA-590-3p reduced cell growth of cervical carcinoma. Kaohsiung J. Med. Sci. 36, 501–507. doi:10.1002/KJM2.12204
Splawski, I., Timothy, K. W., Sharpe, L. M., Decher, N., Kumar, P., Bloise, R., et al. (2004). Ca(V)1.2 calcium channel dysfunction causes a multisystem disorder including arrhythmia and autism. Cell 119, 19–31. doi:10.1016/J.CELL.2004.09.011
Springelkamp, H., Iglesias, A. I., Cuellar-Partida, G., Amin, N., Burdon, K. P., van Leeuwen, E. M., et al. (2015). ARHGEF12 influences the risk of glaucoma by increasing intraocular pressure. Hum. Mol. Genet. 24, 2689–2699. doi:10.1093/HMG/DDV027
Suarez, S. S. (2016). Mammalian sperm interactions with the female reproductive tract. Cell Tissue Res. 363, 185–194. doi:10.1007/S00441-015-2244-2
Sun, T. T., Wang, Y., Cheng, H., Zhang, X. H., Xiang, J. J., Zhang, J. T., et al. (2014). Disrupted interaction between CFTR and AF-6/afadin aggravates malignant phenotypes of colon cancer. Biochim. Biophys. Acta 1843, 618–628. doi:10.1016/J.BBAMCR.2013.12.013
Takai, Y., and Nakanishi, H. (2003). Nectin and afadin: Novel organizers of intercellular junctions. J. Cell Sci. 116, 17–27. doi:10.1242/JCS.00167
Taylor, B. S., Schultz, N., Hieronymus, H., Gopalan, A., Xiao, Y., Carver, B. S., et al. (2010). Integrative genomic profiling of human prostate cancer. Cancer Cell 18, 11–22. doi:10.1016/J.CCR.2010.05.026
Tesfaye, D., Gebremedhn, S., Salilew-Wondim, D., Hailay, T., Hoelker, M., Grosse-Brinkhaus, C., et al. (2018). MicroRNAs: Tiny molecules with a significant role in mammalian follicular and oocyte development. Reproduction 155, R121–R135. doi:10.1530/REP-17-0428
Teves, M. E., Barbano, F., Guidobaldi, H. A., Sanchez, R., Miska, W., and Giojalas, L. C. (2006). Progesterone at the picomolar range is a chemoattractant for mammalian spermatozoa. Fertil. Steril. 86, 745–749. doi:10.1016/j.fertnstert.2006.02.080
Therien, I., and Manjunath, P. (2003). Effect of progesterone on bovine sperm capacitation and acrosome reaction. Biol. Reprod. 69, 1408–1415. doi:10.1095/biolreprod.103.017855
Tzur, A., Kafri, R., LeBleu, V. S., Lahav, G., and Kirschner, M. W. (2009). Cell growth and size homeostasis in proliferating animal cells. Science 325, 167–171. doi:10.1126/SCIENCE.1174294
Wan, J., Qin, J., Cao, Q., Hu, P., Zhong, C., and Tu, C. (2020). Hypoxia-induced PLOD2 regulates invasion and epithelial-mesenchymal transition in endometrial carcinoma cells. Genes Genomics 42, 317–324. doi:10.1007/S13258-019-00901-Y
Wang, C. Y., Lai, M. D., Phan, N. N., Sun, Z., and Lin, Y. C. (2015). Meta-analysis of public microarray datasets reveals voltage-gated calcium gene signatures in clinical cancer patients. PLoS One 10, e0125766. doi:10.1371/JOURNAL.PONE.0125766
Wang, J., Qian, J., Hoeksema, M. D., Zou, Y., Espinosa, A. V., Rahman, S. M. J., et al. (2013). Integrative genomics analysis identifies candidate drivers at 3q26-29 amplicon in squamous cell carcinoma of the lung. Clin. Cancer Res. 19, 5580–5590. doi:10.1158/1078-0432.CCR-13-0594
Wang, R., Xue, X., Wang, Y., Zhao, H., Zhang, Y., Wang, H., et al. (2019). BMI1 deficiency results in female infertility by activating p16/p19 signaling and increasing oxidative stress. Int. J. Biol. Sci. 15, 870–881. doi:10.7150/IJBS.30488
Wang, S. S., Esplin, E. D., Li, J. L., Huang, L., Gazdar, A., Minna, J., et al. (1998). Alterations of the PPP2R1B gene in human lung and colon cancer. Science 282, 284–287. doi:10.1126/SCIENCE.282.5387.284
Wei, X., Lv, H., Yang, S., and Yang, X. (2021). CircRNA PLOD2 enhances ovarian cancer propagation by controlling miR-378. Saudi J. Biol. Sci. 28, 6260–6265. doi:10.1016/J.SJBS.2021.06.088
Whaley, N. R., Fujioka, S., and Wszolek, Z. K. (2011). Autosomal dominant cerebellar ataxia type I: A review of the phenotypic and genotypic characteristics. Orphanet J. Rare Dis. 6, 33. doi:10.1186/1750-1172-6-33
Wu, L., Zhu, J., Liu, D., Sun, Y., and Wu, C. (2021). An integrative multiomics analysis identifies putative causal genes for COVID-19 severity. Genet. Med. 23, 2076–2086. doi:10.1038/s41436-021-01243-5
Wu, X., Zhang, W., Font-Burgada, J., Palmer, T., Hamil, A. S., Biswas, S. K., et al. (2014). Ubiquitin-conjugating enzyme Ubc13 controls breast cancer metastasis through a TAK1-p38 MAP kinase cascade. Proc. Natl. Acad. Sci. U. S. A. 111, 13870–13875. doi:10.1073/PNAS.1414358111
Yamada, T., Sogawa, K., Masaki, T., Funamoto, Y., Kohno, K., Oka, S., et al. (1994). Enhanced expression of catalytic subunit isoform PP1γ1 of protein phosphatase type 1 in malignant fibrous histiocytoma. Res. Commun. Mol. Pathol. Pharmacol. 86, 125–128.
Yamamoto, T., Mori, T., Sawada, M., Matsushima, H., Ito, F., Akiyama, M., et al. (2015). Loss of AF-6/afadin induces cell invasion, suppresses the formation of glandular structures and might be a predictive marker of resistance to chemotherapy in endometrial cancer. BMC Cancer 15, 275. doi:10.1186/S12885-015-1286-X
Yang, Q., Li, K., Li, X., and Liu, J. (2020). Identification of key genes and pathways in myeloma side population cells by bioinformatics analysis. Int. J. Med. Sci. 17, 2063–2076. doi:10.7150/IJMS.48244
Yang, W. J., Yang, D. D., Na, S., Sandusky, G. E., Zhang, Q., and Zhao, G. (2005). Dicer is required for embryonic angiogenesis during mouse development. J. Biol. Chem. 280, 9330–9335. doi:10.1074/JBC.M413394200
Keywords: miRNA, gene, oviduct, fertilization, embryo development, extracellular vesicles, reproduction, miRnome
Citation: Taraschi A, Cimini C, Colosimo A, Ramal-Sanchez M, Valbonetti L, Bernabò N and Barboni B (2022) An interactive analysis of the mouse oviductal miRNA profiles. Front. Cell Dev. Biol. 10:1015360. doi: 10.3389/fcell.2022.1015360
Received: 09 August 2022; Accepted: 06 October 2022;
Published: 19 October 2022.
Edited by:
Sara Stigliani, San Martino Hospital (IRCCS), ItalyReviewed by:
Fernando Silveira Mesquita, Universidade Federal do Pampa, BrazilHirofumi Nishizono, Kanazawa Medical University, Japan
Copyright © 2022 Taraschi, Cimini, Colosimo, Ramal-Sanchez, Valbonetti, Bernabò and Barboni. This is an open-access article distributed under the terms of the Creative Commons Attribution License (CC BY). The use, distribution or reproduction in other forums is permitted, provided the original author(s) and the copyright owner(s) are credited and that the original publication in this journal is cited, in accordance with accepted academic practice. No use, distribution or reproduction is permitted which does not comply with these terms.
*Correspondence: Nicola Bernabò, bmJlcm5hYm9AdW5pdGUuaXQ=