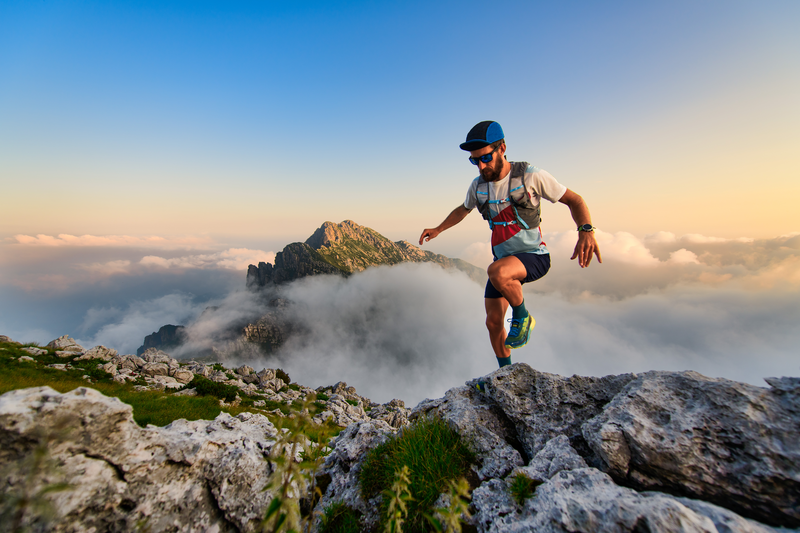
94% of researchers rate our articles as excellent or good
Learn more about the work of our research integrity team to safeguard the quality of each article we publish.
Find out more
MINI REVIEW article
Front. Cell Dev. Biol. , 03 November 2022
Sec. Cellular Biochemistry
Volume 10 - 2022 | https://doi.org/10.3389/fcell.2022.1005681
This article is part of the Research Topic Novel Ligands and Receptors in Endocrine Disorders View all 11 articles
Endocrine and metabolic diseases show increasing incidence and high treatment costs worldwide. Due to the complexity of their etiology and mechanism, therapeutic strategies are still lacking. Osteoprotegerin (OPG), a member of the tumor necrosis factor receptor superfamily, appears to be a potential candidate for the treatment of these diseases. Studies based on clinical analysis and rodent animal models reveal the roles of OPG in various endocrine and metabolic processes or disorders, such as bone remodeling, vascular calcification, and β-cell proliferation, through the receptor activator of nuclear factor kappa-B ligand (RANKL) and the receptor activator of NF-κB (RANK). Thus, in this review, we mainly focus on relevant diseases, including osteoporosis, cardiovascular disease (CVD), diabetes, and gestational diabetes mellitus (GDM), to summarize the effects of the RANKL/RANK/OPG system in endocrine and metabolic tissues and diseases, thereby providing a comprehensive insight into OPG as a potential drug for endocrine and metabolic diseases.
Endocrine and metabolic disorders have become global health challenges due to their complications in physiological and pathological processes in multiple endocrine and metabolic tissues, which are risk factors for many diseases, such as osteoporosis, cardiovascular disease, diabetes mellitus, and pregnancy complications (Khosla and Hofbauer, 2017; Schafer et al., 2017; Helfer and Wu, 2018). To date, some key signaling systems responsible for the regulation of endocrine and metabolic processes have been revealed. Among them, RANKL/RANK signaling is a critical participant in endocrine metabolism (Kiechl et al., 2013; Kondegowda et al., 2015).
RANKL, a transmembrane ligand belonging to the TNF superfamily, is a crucial regulator of bone resorption and is a type II transmembrane glycoprotein composed of 317 amino acid residues (Anderson et al., 1997). RANKL can bind to RANK on neighboring cells and activate NF-κB signaling to regulate biological processes, such as the cell cycle, proliferation, differentiation, and apoptosis (Blomberg Jensen et al., 2021). RANKL is involved in regulating many processes, such as immune surveillance, bone resorption, monocyte chemotaxis, fibrosis, β-cell proliferation, and glucose homeostasis (Bernardi et al., 2016; Huang et al., 2020). RANKL can be found in membrane-bound and soluble forms, in which RANKL binds to two other RANKL molecules to form a trimer that binds to the receptor RANK (Rinotas et al., 2020). RANKL/RANK acts as a major regulator of BRCA1/BRCA2 mutations and is involved in controlling the development of breast cancer by inhibiting NF-κB activation and EGFR signaling (Galluzzi et al., 2016; Sirinian et al., 2018). RANKL mRNA in the bone marrow and lymphoid tissues with high expression, especially through the specific receptor RANK on osteoclast cells, promotes osteoclast maturation and inhibits osteoclast apoptosis. One study using a RANKL monoclonal antibody to block the specific binding of RANKL and RANK verified that the inhibition of osteoclasts could significantly increase the bone density of each place with a low bone mass after menopause and effectively reduce the conversion rate of bones (Kostenuik et al., 2009).
OPG acts as a soluble secreted glycoprotein first discovered by Simon et al. in the intestine of rats in 1997. OPG is a new member of the tumor necrosis factor receptor superfamily that can inhibit differentiation and maturation in osteoclasts and increase the bone density (Simonet et al., 1997). OPG is expressed in the healthy and pathological statuses of various tissues, including the bone, heart, blood vessels, kidney, liver, spleen, thymus, lymph nodes, placenta, adipose tissue, and pancreas (Shen et al., 2012; Bernardi et al., 2016). Indeed, OPG is a fusion receptor that selectively binds to RANKL and inhibits bone resorption, acting as a decoy receptor (Kwon et al., 1998; Akinci et al., 2011).
The bone is a special endocrine tissue that can protect the body organs and the hematopoietic bone marrow, providing structural support for muscles and maintaining the balance of ions and factors in vertebrates (Siddiqui and Partridge, 2016). The most striking function of the bone is paracrine signaling originating from bone cells. Current research has confirmed that the bone can be used as an endocrine organ (Zhou et al., 2021). The main components of a bone include osteoblasts, osteocytes, marrow adipocyte tissue, and mineralized fibrous tissue (Zhang et al., 2015; Lecka-Czernik et al., 2017). The RANKL/RANK/OPG system participates in pathological and physiological processes by secreting bone-derived hormones (Ferron et al., 2008; DiGirolamo et al., 2012; Wei et al., 2015; Zhang et al., 2015; Bernardi et al., 2016). RANKL can activate RANK in osteoclast precursor cells by residing in bone-forming cells and inducing osteoclastogenesis to promote bone resorption (Ikebuchi et al., 2018). Moreover, OPG acts as a natural inhibitor of RANKL and protects against bone loss in a mouse model (Simonet et al., 1997). OPG binds to RANKL and blocks the activation of RANK, which prevents bone resorption by differentiation and activation in osteoclasts (Ono et al., 2020). In clinics, there is a drug targeting RANKL, denosumab (Dmab), a monoclonal antibody that has been broadly used to treat osteoporosis and decrease the risk of fractures (McCloskey et al., 2012). Collectively, both osteogenesis and bone resorption are two key processes for bone remodeling, which is regulated by RANKL in osteocytes, suggesting that it is possible to use OPG as a natural biological inhibitor of RANKL for the treatment of bone-related diseases.
Cardiovascular diseases (CVDs) remain the leading cause of death and premature disability. In 2019, there were more than 523 million patients with CVD worldwide, of which approximately 18.6 million died from CVD (Roth et al., 2020). By 2020, cardiovascular diseases, such as atherosclerosis, had become the main cause of the total disease burden (Mozaffarian, 2016). These findings reinforce the need for diagnostic and prognostic tools that are helpful for CVD interventions. OPG levels were strongly correlated with arterial stiffness and the number of atherosclerotic sites in dysmetabolic patients (Monseu et al., 2016). Plasma OPG levels were increased in hypertensive patients compared to normal blood pressure volunteers, and serum OPG levels were significantly correlated with inflammation and hypertension, which may be used as a predictive indicator of hypertensive vascular endothelial dysfunction (Stepien et al., 2011; Arnold et al., 2019). Moreover, hypertensive patients with high plasma OPG levels are significantly more likely to have three or more target organ (cardiac, vascular, and renal) lesions at 10 years of cardiovascular risk for hypertensive retinopathy (Blazquez-Medela et al., 2012). These studies indicate that an increase in OPG levels might play a potential role in cardiovascular diseases. Recent research studies have indicated that vascular calcification is likely to be traditionally associated with the OPG/RANKL signaling system, which regulates bone remodeling. RANKL promoted vascular calcification, a disorder that causes blood vessel hardening and dysfunction (Lee et al., 2019), acting as a significant risk factor for type 2 diabetes mellitus, which is strongly associated with cardiovascular complications. Vascular calcification can be blocked by OPG acting as a RANKL decoy receptor (Ndip et al., 2011; Harper et al., 2016). An OPG-knockout mouse model showed aortic calcification, suggesting that OPG could protect large blood vessels from medial calcification (Callegari et al., 2013). These studies indicate that OPG could prevent vascular calcification.
In addition, experimental studies have shown that OPG can promote the adhesion of immune cells on endothelial cells, which is an important barrier to maintain the integrity of the inner surface of blood vessels, and its damage is the beginning of atherosclerosis, while the lack of OPG can cause endothelial cell damage (Rochette et al., 2018). With high OPG concentrations, endothelial cells stimulated by the vascular endothelial growth factor are more likely to survive. However, the mechanisms of OPG and RANKL remain to be determined and remain controversial in cardiovascular diseases. Hence, there might be an underlying mechanism involved in cardiovascular functions along with RANKL/RANK/OPG signaling.
Diabetes results in insufficient absolute insulin secretion, resulting in higher blood glucose and causing metabolic disorders and even damage to multiple systems. Diabetes kills more than 3 million people worldwide each year, making it the third leading cause of death from cardiovascular diseases and tumors (Corona et al., 2011). Diabetes can be divided into type 1 and 2 diabetes, according to the etiology of diabetes. Moderate-type diabetes accounts for more than 90% of the total incidence. Insulin deficiency and insulin resistance are two indispensable causes of diabetes. In insulin-dependent diabetes mellitus, islet cells can secrete a certain amount of insulin, but the sensitivity of insulin is reduced, resulting in relative insulin deficiency in the body and causing metabolic disorders in the body and hyperglycemia. The main pathogenesis of diabetes is the inability of β-cells to secrete insulin required for metabolism, resulting in an imbalance in blood glucose homeostasis (Arnes and Sussel, 2015). Bone metabolism has received increasing attention regarding the regulation of blood glucose homeostasis. In a clinical study, an increase in serum OPG levels was reported in both patients with type 1 and type 2 diabetes mellitus compared to normal controls by different groups of investigators (Browner et al., 2001; Knudsen et al., 2003; Galluzzi et al., 2005; Giovannini et al., 2017). There is a strong potential association between OPG and diabetes. Rodent animal models reveal that OPG is a common gene upregulated in islets involved in β-cell replication from the models of β-cell expansion that include pregnancy, obesity, insulin resistance, and β-cell regeneration (Rieck et al., 2009). OPG regulated the proliferation of β-cells in young and old rodent models of diabetes mellitus through prolactin (Kondegowda et al., 2015). OPG-knockout mice and the inflammation induced by lipopolysaccharides (LPS) in a mouse model show an obvious decline in insulin secretion and impaired glucose tolerance. After the artificial addition of exogenous OPG, the symptoms are evidently improved, suggesting that OPG can increase the secretion of insulin, which regulates blood glucose homeostasis (Kuroda et al., 2016). In addition, in animal experiments, a high-fat diet can induce the upregulation of OPG levels in mice. In contrast, after exogenous injection of human OPG molecular protein, the blood glucose of mice is disturbed and their glucose tolerance is significantly impaired, resulting in impaired insulin secretion in mice (Toffoli et al., 2011; Bernardi et al., 2014). Human OPG induces inflammation in mice, leading to the death of cells, which has the opposite effect, with the consequence of disordered regulation of glucose metabolism (Kondegowda et al., 2015). Whether Dmab, as a targeted drug for osteoporosis, can control glucose remains to be deeply investigated in the future.
Previously, different studies have elucidated the specific role of the RANKL/RANK/OPG system in mammary tissues, including gland physiology and hormone-driven epithelial proliferation during pregnancy (Infante et al., 2019). In addition, progesterone induces RANKL expression levels in both mice and humans (Liu et al., 2019), suggesting that RANKL might play roles in physiological processes during pregnancy. Regarding pregnancy complications, we mainly referred to hypertension and hyperglycemia during pregnancy. There was a significant increase in OPG during pregnancy, and the serum OPG rapidly decreased to the prepregnancy level after delivery, which is consistent with a previous experiment on mice (Yano et al., 2001; Naylor et al., 2003).
Pregnant women are first tested for impaired glucose tolerance and are diagnosed with GDM. Complications associated with gestational diabetes include overweight newborn infants or excessive babies, increased perinatal mortality, and risk of type 2 diabetes in offspring (Canouil et al., 2021). According to the International Diabetes Federation (IDF), the incidence of GDM has reached 16.8% in recent years. Retrospective clinical studies showed that the circulating levels of OPG in women with a history of GDM were significantly increased compared to normal women later in life, and they were positively correlated with various metabolic diseases with a high incidence later in life (Akinci et al., 2011; Telejko et al., 2015). We also have proven that placenta-derived OPG could regulate β-cell proliferation and glucose metabolism in placental glucose involved in glucose homeostasis in pregnant mice (Huang et al., 2020). OPG could promote glucose homeostasis during pregnancy, while there is no direct evidence to prove that OPG regulates hypertension during pregnancy. Taken together, these findings indicate that OPG seems to be a potential natural biology inhibitor for the treatment of pregnancy complications.
In summary, the RANKL/RANK/OPG system is important for homeostasis, CVD, bone diseases, and diabetes and has been one of the most important advances in physiology and biology in the past decade, as shown in Figure 1. The discovery of RANKL, RANK, and OPG has led to the development of specific inhibitors of RANKL, such as OPG, and a monoclonal antibody to RANKL has been tested in humans in clinical trials. Whether there are adverse effects on these tissues, especially on immune response, still needs to be clarified for the application of the RANKL/RANK/OPG system as a drug target.
FIGURE 1. Representation of RANKL/RANK/OPG. RANKL binds to its receptor RANK, which can be found in membrane-bound molecules involved in bone resorption in osteoclasts, decreasing GLUT and PRL expression levels in the placenta, vascular calcification in blood vessels, and inhibiting β-cell proliferation and insulin secretion in islets. Osteoprotegerin (OPG), as a decoy receptor for RANKL, is secreted by the bone marrow and as a secreted glycoprotein. OPG competitively binds to RANKL to inhibit some functions of RANKL/RANK signaling in the bone, placenta, blood vessels, and pancreas. In addition, OPG binds to glycosaminoglycans such as heparin sulfate proteoglycans (HSPGs). OPG, osteoprotegerin; RANK, receptor activator of nuclear factor kappa-B; RANKL, receptor activator of nuclear factor kappa-B ligand; GLUTs, glucose transport proteins; PRL, prolactin. The figure was created with BioRender.com.
The original contributions presented in the study are included in the article/supplementary material. Further inquiries can be directed to the corresponding authors.
LZ and BH wrote this manuscript. BH, MH, FZ, MJ, and LZ revised and edited this manuscript. All authors contributed to the article and approved the submitted version.
This study was supported by the National Natural Science Foundation of China (82103857 and 82003419), the Natural Science Foundation in Higher Education of Anhui (KJ2020A0152 and KJ2021A0244), the Anhui Provincial Natural Science Foundation (2208085QH231), the Grants for Scientific Research of BSKY (XJ2020012), and research on the clinical prevention and control model of birth defects based on accurate information management (2022061).
The authors declare that the research was conducted in the absence of any commercial or financial relationships that could be construed as a potential conflict of interest.
All claims expressed in this article are solely those of the authors and do not necessarily represent those of their affiliated organizations, or those of the publisher, the editors, and the reviewers. Any product that may be evaluated in this article, or claim that may be made by its manufacturer, is not guaranteed or endorsed by the publisher.
Akinci, B., Celtik, A., Yuksel, F., Genc, S., Yener, S., Secil, M., et al. (2011). Increased osteoprotegerin levels in women with previous gestational diabetes developing metabolic syndrome. Diabetes Res. Clin. Pract. 91 (1), 26–31. doi:10.1016/j.diabres.2010.09.028
Anderson, D. M., Maraskovsky, E., Billingsley, W. L., Dougall, W. C., Tometsko, M. E., Roux, E. R., et al. (1997). A homologue of the TNF receptor and its ligand enhance T-cell growth and dendritic-cell function. Nature 390 (6656), 175–179. doi:10.1038/36593
Arnes, L., and Sussel, L. (2015). Epigenetic modifications and long noncoding RNAs influence pancreas development and function. Trends Genet. 31 (6), 290–299. doi:10.1016/j.tig.2015.02.008
Arnold, N. D., Pickworth, J. A., West, L. E., Dawson, S., Carvalho, J. A., Casbolt, H., et al. (2019). A therapeutic antibody targeting osteoprotegerin attenuates severe experimental pulmonary arterial hypertension. Nat. Commun. 10 (1), 5183. doi:10.1038/s41467-019-13139-9
Bernardi, S., Bossi, F., Toffoli, B., and Fabris, B. (2016). Roles and clinical applications of OPG and TRAIL as biomarkers in cardiovascular disease. Biomed. Res. Int. 2016, 1752854. doi:10.1155/2016/1752854
Bernardi, S., Fabris, B., Thomas, M., Toffoli, B., Tikellis, C., Candido, R., et al. (2014). Osteoprotegerin increases in metabolic syndrome and promotes adipose tissue proinflammatory changes. Mol. Cell. Endocrinol. 394 (1-2), 13–20. doi:10.1016/j.mce.2014.06.004
Blazquez-Medela, A. M., Garcia-Ortiz, L., Gomez-Marcos, M. A., Recio-Rodriguez, J. I., Sanchez-Rodriguez, A., Lopez-Novoa, J. M., et al. (2012). Osteoprotegerin is associated with cardiovascular risk in hypertension and/or diabetes. Eur. J. Clin. Invest. 42 (5), 548–556. doi:10.1111/j.1365-2362.2011.02619.x
Blomberg Jensen, M., Andreassen, C. H., Jorgensen, A., Nielsen, J. E., Juel Mortensen, L., Boisen, I. M., et al. (2021). RANKL regulates male reproductive function. Nat. Commun. 12 (1), 2450. doi:10.1038/s41467-021-22734-8
Browner, W. S., Lui, L. Y., and Cummings, S. R. (2001). Associations of serum osteoprotegerin levels with diabetes, stroke, bone density, fractures, and mortality in elderly women. J. Clin. Endocrinol. Metab. 86 (2), 631–637. doi:10.1210/jcem.86.2.7192
Callegari, A., Coons, M. L., Ricks, J. L., Yang, H. L., Gross, T. S., Huber, P., et al. (2013). Bone marrow- or vessel wall-derived osteoprotegerin is sufficient to reduce atherosclerotic lesion size and vascular calcification. Arterioscler. Thromb. Vasc. Biol. 33 (11), 2491–2500. doi:10.1161/ATVBAHA.113.301755
Canouil, M., Khamis, A., Keikkala, E., Hummel, S., Lobbens, S., Bonnefond, A., et al. (2021). Epigenome-wide association study reveals methylation loci associated with offspring gestational diabetes mellitus exposure and maternal methylome. Diabetes Care 44 (9), 1992–1999. doi:10.2337/dc20-2960
Corona, G., Monami, M., Rastrelli, G., Aversa, A., Sforza, A., Lenzi, A., et al. (2011). Type 2 diabetes mellitus and testosterone: A meta-analysis study. Int. J. Androl. 34, 528–540. doi:10.1111/j.1365-2605.2010.01117.x
DiGirolamo, D. J., Clemens, T. L., and Kousteni, S. (2012). The skeleton as an endocrine organ. Nat. Rev. Rheumatol. 8 (11), 674–683. doi:10.1038/nrrheum.2012.157
Dorota, D. K., Bogdan, K. G., Mieczyslaw, G., Bozena, L. G., and Jan, O. (2012). The concentrations of markers of bone turnover in normal pregnancy and preeclampsia. Hypertens. Pregnancy 31 (1), 166–176. doi:10.3109/10641955.2010.484084
Ferron, M., Hinoi, E., Karsenty, G., and Ducy, P. (2008). Osteocalcin differentially regulates beta cell and adipocyte gene expression and affects the development of metabolic diseases in wild-type mice. Proc. Natl. Acad. Sci. U. S. A. 105 (13), 5266–5270. doi:10.1073/pnas.0711119105
Galluzzi, F., Stagi, S., Salti, R., Toni, S., Piscitelli, E., Simonini, G., et al. (2005). Osteoprotegerin serum levels in children with type 1 diabetes: A potential modulating role in bone status. Eur. J. Endocrinol. 153 (6), 879–885. doi:10.1530/eje.1.02052
Galluzzi, L., Buque, A., and Kroemer, G. (2016). Prevention of breast cancer by RANKL/RANK blockade. Cell Res. 26 (7), 751–752. doi:10.1038/cr.2016.79
Giovannini, S., Tinelli, G., Biscetti, F., Straface, G., Angelini, F., Pitocco, D., et al. (2017). Serum high mobility group box-1 and osteoprotegerin levels are associated with peripheral arterial disease and critical limb ischemia in type 2 diabetic subjects. Cardiovasc. Diabetol. 16 (1), 99. doi:10.1186/s12933-017-0581-z
Harper, E., Forde, H., Davenport, C., Rochfort, K. D., Smith, D., and Cummins, P. M. (2016). Vascular calcification in type-2 diabetes and cardiovascular disease: Integrative roles for OPG, RANKL and TRAIL. Vasc. Pharmacol. 82, 30–40. doi:10.1016/j.vph.2016.02.003
Helfer, G., and Wu, Q. F. (2018). Chemerin: A multifaceted adipokine involved in metabolic disorders. J. Endocrinol. 238 (2), R79–R94. doi:10.1530/JOE-18-0174
Huang, B., Zhu, W., Zhao, H., Zeng, F., Wang, E., Wang, H., et al. (2020). Placenta-derived osteoprotegerin is required for glucose homeostasis in gestational diabetes mellitus. Front. Cell Dev. Biol. 8, 563509. doi:10.3389/fcell.2020.563509
Ikebuchi, Y., Aoki, S., Honma, M., Hayashi, M., Sugamori, Y., Khan, M., et al. (2018). Coupling of bone resorption and formation by RANKL reverse signalling. Nature 561 (7722), 195–200. doi:10.1038/s41586-018-0482-7
Infante, M., Fabi, A., Cognetti, F., Gorini, S., Caprio, M., and Fabbri, A. (2019). RANKL/RANK/OPG system beyond bone remodeling: Involvement in breast cancer and clinical perspectives. J. Exp. Clin. Cancer Res. 38 (1), 12. doi:10.1186/s13046-018-1001-2
Khosla, S., and Hofbauer, L. C. (2017). Osteoporosis treatment: Recent developments and ongoing challenges. Lancet. Diabetes Endocrinol. 5 (11), 898–907. doi:10.1016/S2213-8587(17)30188-2
Kiechl, S., Wittmann, J., Giaccari, A., Knoflach, M., Willeit, P., Bozec, A., et al. (2013). Blockade of receptor activator of nuclear factor-κB (RANKL) signaling improves hepatic insulin resistance and prevents development of diabetes mellitus. Nat. Med. 19 (3), 358–363. doi:10.1038/nm.3084
Knudsen, S., Foss, C., Poulsen, P., Andersen, N., Mogensen, C., and Rasmussen, L. (2003). Increased plasma concentrations of osteoprotegerin in type 2 diabetic patients with microvascular complications. Diabetes 52, A21.
Kondegowda, N. G., Fenutria, R., Pollack, I. R., Orthofer, M., Garcia-Ocana, A., Penninger, J. M., et al. (2015). Osteoprotegerin and denosumab stimulate human beta cell proliferation through inhibition of the receptor activator of NF-kappa B ligand pathway. Cell Metab. 22 (1), 77–85. doi:10.1016/j.cmet.2015.05.021
Kostenuik, P. J., Nguyen, H. Q., McCabe, J., Warmington, K. S., Kurahara, C., Sun, N., et al. (2009). Denosumab, a fully human monoclonal antibody to RANKL, inhibits bone resorption and increases BMD in knock-in mice that express chimeric (Murine/Human) RANKL. J. Bone Min. Res. 24 (2), 182–195. doi:10.1359/Jbmr.081112
Kuroda, Y., Maruyama, K., Fujii, H., Sugawara, I., Ko, S. B. H., Yasuda, H., et al. (2016). Osteoprotegerin regulates pancreatic beta-cell homeostasis upon microbial invasion. Plos One 11 (1). e0146544 doi:10.1371/journal.pone.0146544
Kwon, B. S., Wang, S., Udagawa, N., Haridas, V., Lee, Z. H., Kim, K. K., et al. (1998). TR1, a new member of the tumor necrosis factor receptor superfamily, induces fibroblast proliferation and inhibits osteoclastogenesis and bone resorption. FASEB J. 12 (10), 845–854. doi:10.1096/fasebj.12.10.845
Lecka-Czernik, B., Stechschulte, L. A., Czernik, P. J., Sherman, S. B., Huang, S., and Krings, A. (2017). Marrow adipose tissue: Skeletal location, sexual dimorphism, and response to sex steroid deficiency. Front. Endocrinol. 8, 188. doi:10.3389/fendo.2017.00188
Lee, G. L., Yeh, C. C., Wu, J. Y., Lin, H. C., Wang, Y. F., Kuo, Y. Y., et al. (2019). TLR2 promotes vascular smooth muscle cell chondrogenic differentiation and consequent calcification via the concerted actions of osteoprotegerin suppression and IL-6-mediated RANKL induction. Arterioscler. Thromb. Vasc. Biol. 39 (3), 432–445. doi:10.1161/ATVBAHA.118.311874
Liu, S., Yin, P., Kujawa, S. A., Coon, J. S. T., Okeigwe, I., and Bulun, S. E. (2019). Progesterone receptor integrates the effects of mutated MED12 and altered DNA methylation to stimulate RANKL expression and stem cell proliferation in uterine leiomyoma. Oncogene 38 (15), 2722–2735. doi:10.1038/s41388-018-0612-6
McCloskey, E. V., Johansson, H., Oden, A., Austin, M., Siris, E., Wang, A., et al. (2012). Denosumab reduces the risk of osteoporotic fractures in postmenopausal women, particularly in those with moderate to high fracture risk as assessed with FRAX. J. Bone Min. Res. 27 (7), 1480–1486. doi:10.1002/jbmr.1606
Monseu, M., Dubois, S., Boursier, J., Aube, C., Gagnadoux, F., Leftheriotis, G., et al. (2016). Osteoprotegerin levels are associated with liver fat and liver markers in dysmetabolic adults. Diabetes Metab. 42 (5), 364–367. doi:10.1016/j.diabet.2016.02.004
Mozaffarian, M. (2016). Heart disease and stroke statistics-2016 update: A report from the American heart association. Circulation 133 (15), E599. doi:10.1161/Cir.0000000000000409
Naylor, K. E., Rogers, A., Fraser, R. B., Hall, V., Eastell, R., Blumsohn, A., et al. (2003). Serum osteoprotegerin as a determinant of bone metabolism in a longitudinal study of human pregnancy and lactation. J. Clin. Endocrinol. Metab. 88 (11), 5361–5365. doi:10.1210/jc.2003-030486
Ndip, A., Williams, A., Jude, E. B., Serracino-Inglott, F., Richardson, S., Smyth, J. V., et al. (2011). The RANKL/RANK/OPG signaling pathway mediates medial arterial calcification in diabetic charcot neuroarthropathy. Diabetes 60 (8), 2187–2196. doi:10.2337/db10-1220
Ono, T., Hayashi, M., Sasaki, F., and Nakashima, T. (2020). RANKL biology: Bone metabolism, the immune system, and beyond. Inflamm. Regen. 40, 2. doi:10.1186/s41232-019-0111-3
Rieck, S., White, P., Schug, J., Fox, A. J., Smirnova, O., Gao, N., et al. (2009). The transcriptional response of the islet to pregnancy in mice. Mol. Endocrinol. 23 (10), 1702–1712. doi:10.1210/me.2009-0144
Rinotas, V., Papakyriakou, A., Violitzi, F., Papaneophytou, C., Ouzouni, M. D., Alexiou, P., et al. (2020). Discovery of small-molecule inhibitors of receptor activator of nuclear factor-κb ligand with a superior therapeutic index. J. Med. Chem. 63 (20), 12043–12059. doi:10.1021/acs.jmedchem.0c01316
Rochette, L., Meloux, A., Rigal, E., Zeller, M., Cottin, Y., and Vergely, C. (2018). The role of osteoprotegerin in the crosstalk between vessels and bone: Its potential utility as a marker of cardiometabolic diseases. Pharmacol. Ther. 182, 115–132. doi:10.1016/j.pharmthera.2017.08.015
Roth, G. A., Mensah, G. A., Johnson, C. O., Addolorato, G., Ammirati, E., Baddour, L. M., et al. (2020). Global burden of cardiovascular diseases and risk factors, 1990-2019: Update from the GBD 2019 study. J. Am. Coll. Cardiol. 76 (25), 2982–3021. doi:10.1016/j.jacc.2020.11.010
Schafer, M. J., Miller, J. D., and LeBrasseur, N. K. (2017). Cellular senescence: Implications for metabolic disease. Mol. Cell. Endocrinol. 455, 93–102. doi:10.1016/j.mce.2016.08.047
Shen, P., Gong, Y., Wang, T., Chen, Y., Jia, J., Ni, S., et al. (2012). Expression of osteoprotegerin in placenta and its association with preeclampsia. PLoS One 7 (8), e44340. doi:10.1371/journal.pone.0044340
Siddiqui, J. A., and Partridge, N. C. (2016). Physiological bone remodeling: Systemic regulation and growth factor involvement. Physiol. (Bethesda) 31 (3), 233–245. doi:10.1152/physiol.00061.2014
Simonet, W. S., Lacey, D. L., Dunstan, C. R., Kelley, M., Chang, M. S., Luthy, R., et al. (1997). Osteoprotegerin: A novel secreted protein involved in the regulation of bone density. Cell 89 (2), 309–319. doi:10.1016/S0092-8674(00)80209-3
Sirinian, C., Papanastasiou, A. D., Schizas, M., Spella, M., Stathopoulos, G. T., Repanti, M., et al. (2018). RANK-c attenuates aggressive properties of ER-negative breast cancer by inhibiting NF-κB activation and EGFR signaling. Oncogene 37 (37), 5101–5114. doi:10.1038/s41388-018-0324-y
Stepien, E., Wypasek, E., Stopyra, K., Konieczynska, M., Przybylo, M., and Pasowicz, M. (2011). Increased levels of bone remodeling biomarkers (osteoprotegerin and osteopontin) in hypertensive individuals. Clin. Biochem. 44 (10-11), 826–831. doi:10.1016/j.clinbiochem.2011.04.016
Telejko, B., Kalejta, K., Kuzmicki, M., Wawrusiewicz-Kurylonek, N., Lipinska, D., Pliszka, J., et al. (2015). The association of bone turnover markers with pro- and anti-inflammatory adipokines in patients with gestational diabetes. Ann. Agric. Environ. Med. 22 (2), 307–312. doi:10.5604/12321966.1152085
Toffoli, B., Bernardi, S., Candido, R., Sabato, N., Carretta, R., Corallini, F., et al. (2011). Osteoprotegerin induces morphological and functional alterations in mouse pancreatic islets. Mol. Cell. Endocrinol. 331 (1), 136–142. doi:10.1016/j.mce.2010.08.019
Wei, J., Flaherty, S., and Karsenty, G. (2015). Searching for additional endocrine functions of the skeleton: Genetic approaches and implications for therapeutics. Expert Rev. Endocrinol. Metab. 10 (4), 413–424. doi:10.1586/17446651.2015.1058152
Yano, K., Shibata, O., Mizuno, A., Kobayashi, F., Higashio, K., Morinaga, T., et al. (2001). Immunological study on circulating murine osteoprotegerin/osteoclastogenesis inhibitory factor (OPG/OCIF): Possible role of OPG/OCIF in the prevention of osteoporosis in pregnancy. Biochem. Biophys. Res. Commun. 288 (1), 217–224. doi:10.1006/bbrc.2001.5745
Zhang, Q., Riddle, R. C., and Clemens, T. L. (2015). Bone and the regulation of global energy balance. J. Intern. Med. 277 (6), 681–689. doi:10.1111/joim.12348
Keywords: nuclear factor kappa-B ligand, receptor activator of NF-κB, osteoprotegerin, osteoporosis, cardiovascular disease, gestational diabetes mellitus
Citation: Zhang L, Zeng F, Jiang M, Han M and Huang B (2022) Roles of osteoprotegerin in endocrine and metabolic disorders through receptor activator of nuclear factor kappa-B ligand/receptor activator of nuclear factor kappa-B signaling. Front. Cell Dev. Biol. 10:1005681. doi: 10.3389/fcell.2022.1005681
Received: 28 July 2022; Accepted: 17 October 2022;
Published: 03 November 2022.
Edited by:
Rujuan Zuo, Oslo University Hospital, NorwayReviewed by:
Muhammad Khan, University of the Punjab, PakistanCopyright © 2022 Zhang, Zeng, Jiang, Han and Huang. This is an open-access article distributed under the terms of the Creative Commons Attribution License (CC BY). The use, distribution or reproduction in other forums is permitted, provided the original author(s) and the copyright owner(s) are credited and that the original publication in this journal is cited, in accordance with accepted academic practice. No use, distribution or reproduction is permitted which does not comply with these terms.
*Correspondence: Maozhen Han, aGFubXpAYWhtdS5lZHUuY24=; Binbin Huang, aHVhbmdiYjkxQDEyNi5jb20=
†These authors have contributed equally to this work
Disclaimer: All claims expressed in this article are solely those of the authors and do not necessarily represent those of their affiliated organizations, or those of the publisher, the editors and the reviewers. Any product that may be evaluated in this article or claim that may be made by its manufacturer is not guaranteed or endorsed by the publisher.
Research integrity at Frontiers
Learn more about the work of our research integrity team to safeguard the quality of each article we publish.