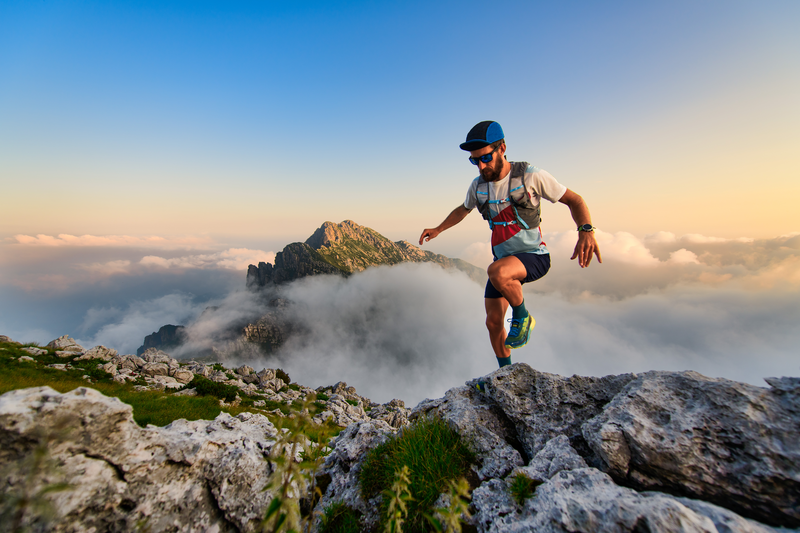
95% of researchers rate our articles as excellent or good
Learn more about the work of our research integrity team to safeguard the quality of each article we publish.
Find out more
REVIEW article
Front. Cell Dev. Biol. , 14 January 2022
Sec. Molecular and Cellular Pathology
Volume 9 - 2021 | https://doi.org/10.3389/fcell.2021.833840
This article is part of the Research Topic Decipher Cellular and Molecular Mechanism of the Development and Degeneration of Functional Spinal Unit with Potential Therapeutic Approaches View all 18 articles
Exosomes are extracellular vesicles formed by various donor cells that regulate gene expression and cellular function in recipient cells. Exosomes derived from mesenchymal stem cells (MSC-Exos) perform the regulatory function of stem cells by transporting proteins, nucleic acids, and lipids. Intervertebral disc degeneration (IDD) is one of the main causes of low back pain, and it is characterized by a decreased number of nucleus pulposus cells, extracellular matrix decomposition, aging of the annulus fibrosus, and cartilage endplate calcification. Besides, nutrient transport and structural repair of intervertebral discs depend on bone and cartilage and are closely related to the state of the bone. Trauma, disease and aging can all cause bone injury. However, there is a lack of effective drugs against IDD and bone injury. Recent MSC-Exos fine tuning has led to significant progress in the IDD treatment and bone repair and regeneration. In this review, we looked at the uniqueness of MSC-Exos, and the potential treatment mechanisms of MSC-Exos with respect to IDD, bone defects and injuries.
Exosomes are bilayered extracellular functional vesicles released by different cells with a diameter ranging between 40–120 nm (Simons and Raposo, 2009). In the early stages, endosomes containing intraluminal vesicles (ILVs) are formed preliminarily, and then large mature multivesicular bodies (MVBs) within the cell release ILVs into the extracellular space to form exosomes (Zhang et al., 2019) (Figure 1). Exosomes carry out their functions by fusing with cell membranes or binding membrane proteins of the recipient cells. They contain functional proteins, nucleic acids (mRNA, miRNA, lncRNA, etc.) and lipids, and are carriers of intercellular communication between donor and recipient cells (Wang et al., 2021b). MiRNA is a crucial communication medium contained in exosomes, which can regulate the expression of genes and proteins in recipient cells and inhibit the degradation of exosomes (Valadi et al., 2007; Jong et al., 2012). After entering target cells, exosomal miRNA binds to target gene mRNA through partial sequence complementation and participate in tissue repair, inflammation, apoptosis and other processes, thus playing an important role in the regulation of gene expression (Ti et al., 2016; Chen et al., 2019). Exosomes originate from a wide range of sources, and almost all cells can secrete exosomes. The exosomes secreted under normal and pathological conditions are different, even for the same cells (Zhang et al., 2015). If exosomes fail to bind to their target cells in time, they are rapidly metabolized. When applied topically or injected throughout the body, they can provide multiple therapeutic benefits, such as repairing the damaged intervertebral discs and bone tissues (Riau et al., 2019).
FIGURE 1. Typical process of exosome generation, secretion, and transfer from the donor cells to the recipient cells, and the exosome structure is shown. Early endosomes containing ILVs are formed preliminarily and then developed into mature MVBs to release and form exosomes into the extracellular. The exosomes have a bilayered membrane structure containing functional proteins, nucleic acids (mRNA, miRNA, lncRNA, etc.), and lipids, some of which are released into recipient cells to regulate gene expression and cell function.
MSCs with multidirectional differentiation and self-replication potential have long been considered as an effective method for repairing intervertebral disc disorders and bone injuries, but there are many safety problems (Uccelli et al., 2008; Guadix et al., 2017). Many studies have demonstrated that exosomes derived from mesenchymal stem cells (MSC-Exos) have similar biological effects to MSCs in terms of tissue regeneration and repair functions (Cosenza et al., 2017). The application of MSCs avoids the problems associated with intact cell transplantation, such as immune rejection, infection, and non-directed cell differentiation (Lou et al., 2017). Exosomes secreted by MSCs from bone marrow, adipose, umbilical cord can all promote tissue regeneration and repair (Zhou et al., 2019; Li et al., 2020a; Zhang et al., 2021c). MSCs of different ages can regulate each other through exosomes, while younger MSC-Exos can enhance the proliferation and osteogenic differentiation of older MSCs (Jia et al., 2020).
IDD is associated with various factors such as aging, abnormal biomechanical burden, reduced nutrient supply to the cartilage endplates (Dowdell et al., 2017). In IDD, the water content of the nucleus pulposus decreases, and the pressure load decreases (Adams and Roughley, 2006). At the same time, the annulus fibrosus carries more load and is, therefore, more prone to damage. The healing potential of intervertebral discs without vascular nourishment is low, and there is no effective treatment to inhibit or even repair IDD (Urban and Roberts, 2003). Besides, the health of the surrounding bone and cartilage is closely related to the overall condition of the intervertebral disc, since the disc receives its nutrients from the endplate’s blood supply (Geer, 2018). Although new materials can reduce bone defects, it is still necessary to explore bioactive substances that can promote bone regeneration and repair (Dimitriou et al., 2011; Amini et al., 2012). Therefore, we focused on acellular MSC-Exos over the past years to elucidate their potential effectiveness on IDD treatment and bone regeneration and repair. In this review, we looked at the relationships between MSC-Exos and IDD, MSC-Exos and bone repair and regeneration, and further discussed the mechanism of action of MSC-Exos on the treatment of IDD and promoting bone repair and regeneration.
Low back pain is a global health hazard (Manchikanti et al., 2014). Disc herniation and spinal stenosis caused by IDD are the main causes of low back pain, with a high incidence amongst the elderly (Croft et al., 2021). The pathogenesis of IDD is characterized by a decreased number of nucleus pulposus cells (NPCs), extracellular matrix (ECM) decomposition, annulus fibrosus aging, and cartilage endplate calcification (Grunhagen et al., 2011; Yang et al., 2020b). The nucleus pulposus is located at the center of the intervertebral disc and is composed of the proteoglycan elastin and ECM, which are important components responsible for pressure bearing in the intervertebral disc [2]. In IDD, the nucleus pulposus is the first to degenerate, mostly following an abnormal amount of stress on the vertebrae, aging, nutrition and other factors (Urban and Roberts, 2003). The exosomes derived from MSCs contain a variety of regulatory factors that inhibit the development of IDD. Studies have shown that MSC-Exos could prevent IDD by inhibiting apoptosis and promoting the proliferation of NPCs, inhibiting ECM degradation, alleviating inflammatory response and oxidative stress, promoting chondrogenic differentiation, and protecting endplate chondrocytes and annulus fibrosus. Therefore, MSC-Exos may be a promising option to delay or even reverse IDD (Hu et al., 2020; Krut et al., 2021). The related mechanisms are described in detail below and displayed in Figure 2.
FIGURE 2. Potential mechanisms of MSC-Exos for treating IDD. (A) Inhibiting NPC apoptosis and promoting NPC proliferation. MSC-Exos alleviate NPC apoptosis induced by acidic pH through repressing caspase-3 expression and attenuating caspase-3 cleavage. BMSC-Exos can increase miR-155 expression to upregulate HO-1 expression and downregulate Bach1 expression, subsequently activating autophagy in NPCs to inhibit the cell apoptosis in the state of low blood supply. MSC-Exos can deliver miR-532-5p, which targets RASSF5, and eventually inhibit TNF-α-induced NPC apoptosis. By delivering miR-142-3p to target MLK3 in NPCs, MSC-Exos inhibit the activation of the MAPK pathway and alleviate IL-1β-induced apoptosis and inflammation in NPCs. MSC-Exos can transfer miR-21, which directly targets PTEN; the PTEN silencing actives PI3K/Akt pathway and suppresses activation of Bad, Bax, and caspase-3 and inhibits TNF-α-induced NPC apoptosis. (B) Inhibiting ECM degradation. BMSC-Exos suppress the levels of MMP-1, MMP-3, MMP-13 in degenerative NPCs to inhibit ECM decomposition metabolism and evaluate expression levels of aggrecan, collagen II, SOX9, further inhibiting the ECM degradation. (C) Inhibiting inflammation response. MSC-Exos can decrease inflammatory factor expression including IL-1α, IL-1β, IL-6, IL-17, NF-κB-p65 and TNF-α in NPCs. MSC-Exos can inactivate the NLRP3 and inhibit the expression of NT-GSDMD, IL-18 and IL-1β proteins in degenerative NPCs, therefore inhibiting the NLRP3-mediated inflammatory pyroptosis. (D) Inhibiting oxidative stress. MSC-Exos can reduce the ROS and MDA level and inhibit oxidative stress-induced NPC apoptosis. MSC-Exos can activate Akt/ERK pathway to decrease CHOP protein expression, therefore inhibiting the cleavage of caspase-3, caspase-12 to treat AGEs-related ER stress-induced IDD. (E) Promoting chondrogenic differentiation. MSC-Exo treatment can induce chondrogenesis in degenerative NPCs earlier by increased the aggrecan, collagen II and SOX9 expression. (F) Protective effect on endplate chondrocytes. MSC-Exos containing miR-31-5p could negatively regulate ER stress by targeting ATF6, and further reducing caspase-3, caspase-7 and caspase-9 expression to inhibit apoptosis and calcification of endplate chondrocytes. (G) Protective effect on annulus fibrosus of IDD. BMSC-Exos can suppress PI3K/Akt/mTOR signaling pathway-mediated autophagy and inhibit the IL-1β-induced inflammation and apoptosis in annulus fibrosus cells.
The main pathophysiologic mechanism of IDD involves a decline in the number of NPCs and ECM degradation, with multiple reactions such as inflammation and oxidative stress also being involved in this process. Therefore, inhibition of nucleus pulposus cell apoptosis and promotion of cell proliferation is the focus of IDD treatment. Exosomes derived from bone marrow mesenchymal stem cell (BMSC-Exos) have been reported to increase the proliferative ability of NPCs along with increasing the concentration of MSC-Exos (Li et al., 2020a; Hu et al., 2021). For exosomes derived from adipose-derived mesenchymal stem cells (ADMSC-Exos), the proliferation and migration rate of human NPCs were elevated through the ADMSC-Exo treatment (Zhang et al., 2021c). Furthermore, BMSC-Exos were able to prevent and mitigate NPC apoptosis induced by acidic pH by repressing caspase-3 expression and attenuating caspase-3 cleavage. When NPCs are placed in the pathological state of low blood supply, BMSC-Exos could increase miR-155 expression in NPCs, thereby downregulating Bach1 expression and upregulating heme oxygenase-1(HO-1) expression, activating autophagy in NPCs, inhibiting the level of apoptosis, thereby inhibiting IDD (Shi et al., 2021). Besides, BMSC-Exos could reduce the apoptosis rate of NPCs induced by tumor necrosis factor-α (TNF-α), while the miR-532-5p level was decreased in apoptotic NPCs. RASSF5 was demonstrated as a target of miR-532-5p; BMSC-Exos may inhibit apoptosis by targeting RASSF5 to deliver miR-532-5p to inhibit NPC apoptosis (Zhu et al., 2020a). BMSC-Exos also could alleviate interleukin-1β (IL-1β)-induced apoptosis and inflammation in NPCs, which may be mediated by delivering miR-142-3p to target mixed-lineage kinase-3 (MLK3) in NPCs and further inhibiting mitogen-activated protein kinase (MAPK) signaling (Zhu et al., 2020b). Using MSC-Exos enriched in miR-21 to transfer miR-21 to TNF-α-induced NPCs, the apoptosis level in the NPCs could be downregulated (Cheng et al., 2018). In this process, miR-21 directly targets phosphatase and tensin homolog (PTEN), which is negatively regulated by miR-21. The PTEN silencing actives phosphoinositide 3-kinases (PI3K)/ protein kinase B (Akt) pathway then decreases the activation level of downstream factors of Bad, Bax and caspase-3, and finally inhibit TNF-α-induced apoptosis.
Disc height is reduced due to the loss of matrix, which is mainly caused by matrix metalloproteinases (MMPs), which can hydrolyse ECM components such as proteoglycan collagen, thereby accelerating the pathological process of IDD (Kozaci et al., 2006). BMSC-Exo treatment can promote the expression levels of anabolic/matrix protective genes including aggrecan, collagen II, SRY-box transcription factor 9 (SOX9); suppress the levels of matrix-degrading genes such as MMP-1, MMP-3, MMP-13 in degenerative NPCs (Lu et al., 2017; Li et al., 2020a). Moreover, studies have shown that lactic acid accumulation can reduce the pH value in IDD (Malandrino et al., 2014). Acidic pH adversely affects the proliferation of NPCs, and destroys the metabolic balance of the ECM, which limits the therapeutic potential of MSCs and is a negative factor affecting intervertebral disc repair (Huang et al., 2013). Moreover, ADMSC-Exos have also been found to suppress the MMP-13 expression, inhibit ECM decomposition in degenerative NPCs, and increase collagen II expression to promote ECM formation (Xing et al., 2021).
Previous researches have confirmed inflammation and related signaling pathways as important factors in the onset and progression of IDD, an obvious etiologic factor of low back pain (Lyu et al., 2021). Studies have demonstrated that the secretion of inflammatory factors such as IL-1α, IL-1β, IL-6, IL-17, nuclear factor-κB p65 (NF-κB p65), TNF-α was increased in the NPCs from degenerative discs, and found that ADMSC-Exos could decrease the inflammation level (Zhang et al., 2021c). Additionally, ADMSC-Exos could inactivate the NLRP3 inflammasome, inhibit the expression of N-terminal gasdermin D (NT-GSDMD) and IL-1β proteins in degenerative NPCs, thereby more significantly reducing the inflammatory response (Xing et al., 2021). Besides, it was also shown that MSC-Exos significantly decrease NLRP3 expression and reduce caspase activation, hence downregulating the expression levels of downstream cytokines IL-18 and IL-1β, inhibiting NLRP3-mediated inflammatory pyroptosis in the degenerative NPCs (Zhang et al., 2020a). And the establishment of an ECM hydrogel system could sustainably release ADMSC-Exos, allowing exosomes to remain in the degenerative disc for up to 28 days to exert a more anti-inflammatory effect.
Reactive oxygen species (ROS) is a crucial factor for intervertebral disc signal transduction, and the excessive production of ROS can accelerate IDD (Suzuki et al., 2015; Feng et al., 2017). Finding therapeutic targets to reduce excessive ROS is a valuable research orientation, which could work mainly by inhibiting oxidative stress. Hu et al. (Hu et al., 2021) found that BMSC-Exos could reduce ROS and malondialdehyde (MDA) level and inhibit oxidative stress-induced NPC apoptosis. Besides, suppressing the Akt/extracellular signal-regulated kinase (ERK) pathways was demonstrated to aggravate endoplasmic reticulum (ER) stress-induced apoptosis (Xu et al., 2017). MSC-Exos could protect the NPCs against advanced glycation end products (AGEs)-related ER apoptosis by activating Akt/ERK signaling, which could reduce C/EBP homologous protein (CHOP) expression, and attenuate the cleavage of caspase-3, caspase-12 (Liao et al., 2019). Therefore, BMSC-Exos may be the effective therapeutic method to treat AGEs-related ER stress-induced IDD.
The reduction of chondrogenic NPCs and the lower expression of chondrogenic genes were the critical manifestations of IDD (Maldonado and Oegema, 1992; Choi et al., 2015). An important function of chondrocyte-like NPCs was to produce ECM, and more chondrocyte-like NPCs can produce more ECM (Adams and Roughley, 2006). SOX9 is one of the early markers of chondrogenesis for NPCs (Chimal-Monroy et al., 2003). It was reported that MSC-Exos could promote SOX9 expression in NPCs from degenerative NP tissue more quickly, meaning that MSC-Exo treatment can induce earlier chondrogenesis in degenerative NPCs (Hingert et al., 2020). A study (Zhang et al., 2021c) reported that after treatment of NPCs with ADMSC-Exos for 7, 14 and 21 days, the levels of chondrocytic genes (collagen II, aggrecan and SOX9) were significantly increased, suggesting that ADMSC-Exos had restored the chondrogenic differentiation properties of degenerative NPCs.
Degenerative changes of the cartilage endplate can hinder nutrient transfer to the intervertebral disc and aggravate IDD (Zhu et al., 2016; Wong et al., 2019). It was reported that MSC-Exos containing miR-31-5p could negatively regulate activating endoplasmic reticulum (ER) stress by targeting transcription factor 6 (ATF6), and further inhibit expression of caspase-3, caspase-7, and caspase-9, thereby inhibiting tert-butyl hydroperoxide-induced apoptosis and calcification in endplate chondrocytes (Xie et al., 2020). By injecting MSC-Exos to the sub-endplate of the IDD model in rat tails, the MSC-Exos displays an inhibiting effect on IDD. Conversely, the protective effects were reduced when the miR-31-5p levels were downregulated in MSC-Exos.
Due to complicated biomechanics, both the number of cells in the annulus fibrosus and nucleus pulposus are found to be considerably decreased during the IDD (Vergroesen et al., 2015). Gene analyses have delineated that autophagy-related gene expression is significantly increased in degenerative annulus fibrosus tissues. The number of autophagic vesicles and autophagosomes was enhanced, suggesting that autophagy may play an essential role in the pathogenesis of IDD (Gruber et al., 2015). Research has equally revealed that, BMSC-Exos could inhibit IL-1β-induced inflammation and apoptosis and promote the proliferation of annulus fibrosus cells, thus exerting a protective effect on the annulus fibrosus, and this may be by suppressing PI3K/Akt/mTOR signaling pathway-mediated autophagy (Li et al., 2020b).
Various bone defects caused by trauma, tumor, infection, congenital deformity and osteoporosis, seriously reduce the life quality of patients and are commonly seen in the clinic (Benjamin, 2010). A small quantity of bone defects or injuries can usually repair themselves, but large and complex bone defects usually need to be filled with artificial or autologous bone, but issues such as bone insufficiency and immune rejection are still encountered. Additionally, osteoarthritis is a common disease of progressive destruction of articular cartilage, accompanied by increased pain, currently lacking effective drugs targeting cartilage repair and regeneration (Li et al., 2013). MSC-Exos are effective at promoting bone repair and regeneration independently, and play an immunomodulatory role by binding with receptors to promote osteogenesis (Zhang et al., 2020b; Fan et al., 2021). Current research suggests that MSC-Exos can promote osteogenic differentiation and angiogenesis, regulate immune function, induce chondrogenesis and improve osteoporosis (Lu et al., 2019; Yang et al., 2021). The related mechanisms are described in detail in the following contents and shown in Figure 3.
FIGURE 3. Potential mechanisms of MSC-Exos for promoting bone repair and regeneration. (A) Promoting osteogenic differentiation. MSC-Exos can activate the PI3K/Akt signaling pathway to promote osteogenic differentiation and proliferation of BMSCs. The Wnt/β-catenin signaling pathway activated by MSC-Exos with increased expression of β-catenin and Wnt3a can promote osteoblast proliferation and differentiation. Exosomes enriched with miR-375 inhibit the IGFBP3 expression to promote osteogenic differentiation of hBMSCs. (B) Promoting angiogenesis. BMSC-Exos activates the Akt/mTOR pathway to stimulate angiogenesis and further promotes bone regeneration. Exosomal miR-21 can upregulate the NOTCH1/DLL4 pathway and promote angiogenesis. (C) Immunoregulation. MSC-Exos can decrease the gene expression of IL-1β, IL-6, TNF-α, and iNOS in the inflammatory macrophages. (D) Inducing chondrogenesis. MSC-Exos increase the protein and mRNA expression of collagen II and SOX9 to improve cartilage regeneration. MSC-Exos can promote the macrophage’s polarization toward the M2 phenotype and further inhibit the inflammatory response to benefit chondrogenesis. BMSC-Exo treatment can decrease the c-MYC expression level, which indicates the chondrocyte’s maturation. (E) Improving osteoporosis. MALAT1 contained in BMC-Exos promotes alkaline phosphatase activity of osteoblasts and mineralizes nodules by increasing SATB2 expression. Activating MAPK signaling and increasing the P-p38 and P-JNK expression by MSC-Exos can promote osteoblast differentiation. BMSC-Exos enriched with miR-196a can inhibit Dkk1 expression to activate the Wnt/β-catenin pathway, thereby improving osteoporosis. Exosomes derived from human umbilical cord MSCs can inhibit BMSC apoptosis through the miR-1263/Mob1/Hippo signaling pathway to improve osteoporosis.
MSCs have outstanding osteogenic differentiation capacity, and they have been widely used in promoting bone repair and regeneration (Pittenger et al., 1999; Jiang et al., 2002). The miRNAs, growth factors, cytokines contained in MSC-Exos could promote the osteogenic differentiation abilities of MSCs (Wang et al., 2015). Studies have shown that MSC-Exos can profoundly improve the osteogenic differentiation and proliferation of BMSCs, and reinforce the osteogenic response of BMSCs by activating the PI3K/Akt signaling pathway (Zhang et al., 2016). Besides, the exosomes derived from younger BMSCs depicted a stronger ability to promote osteogenic differentiation. It was reported that younger BMSC-Exos (2 weeks) could enhance the proliferation and osteogenic differentiation of older BMSCs (15 months) (Jia et al., 2020). The in vivo experiments also verified that bone regeneration was significantly accelerated in rats treated with MSC-Exos. Moreover, activation of the Wnt/β-catenin signaling pathway can stimulate osteoblast proliferation and differentiation, and promote bone fracture repair and regeneration (Gaur et al., 2005). The human umbilical cord MSC-Exos treatment could promote the expression levels of β-catenin and Wnt3a protein in the Wnt signaling pathway in fracture site cells, indicating that MSC-Exos probably promotes osteoblast proliferation and differentiation as well as bone fracture repair through the Wnt signaling pathway (Zhou et al., 2019). Furthermore, exosomes enriched with miR-375 could promote the osteogenic differentiation of BMSCs by inhibiting insulin-like growth factor binding protein 3 (IGFBP3) expression as a negative regulator of osteogenic differentiation (Chen et al., 2019).
Angiogenesis is a prerequisite for bone regeneration and provides the necessary growth factors and nutrients for the repair of bone injuries and defects (Yu et al., 2009). Besides, new blood vessels serve as a route for transferring the inflammatory cells, and the precursor cells of cartilage and bone, allowing them to reach the site of bone injury. Angiogenesis is regulated by various growth factors, such as various miRNAs, vascular endothelial growth factors (Hankenson et al., 2011). BMSC-Exos stimulates angiogenesis by activating the Akt/mammalian target of rapamycin (mTOR) pathway, which further promotes bone regeneration (Liang et al., 2019). Besides, MSC-Exos can enhance the proliferation, migration, and angiogenic differentiation of endothelial progenitor cells, further driving the process of angiogenesis (Zhang et al., 2021b). Mechanistic studies revealed that exosomal miR-21 promote angiogenesis by upregulating the NOTCH1/DLL4 pathway (Zhang et al., 2021b). It was also found that miR-214-3p was significantly increased in the BMSC-Exos of bone-losing mice. Moreover, knee loading was found to promote angiogenesis and bone regeneration by enhancing the formation of type H vessels and downregulating miR-214-3p levels in BMSC-Exos (Wang et al., 2021a).
Bone regeneration and healing is a complicated process, and the levels of cytokines produced in bone injury are first elevated and then gradually decline (Marsell and Einhorn, 2011; Einhorn and Gerstenfeld, 2015). However, continuous or abnormal activation of immune cells or secretion of proinflammatory molecules is detrimental to bone regeneration (Gibon et al., 2017). Macrophages as immune cells play a crucial role in bone regeneration, secreting inflammatory and chemotactic mediators, and initiating the recruitment of MSCs (Loi et al., 2016). MSC-Exos possess a sustained inflammation-regulatory ability, which could decrease the gene expression of IL-1β, IL-6, TNF-α, and suppress the expression of an M1 phenotypic marker (iNOS) mRNA in the inflammatory macrophages (Wei et al., 2019; Zhang et al., 2020b). The scanning electronic microscopy results depicted that the morphology of macrophages was significantly elongated after treatment with BMSC-Exos.
Cartilage damage and defect regeneration remain challenges due to its limited healing capacity. (Sun et al., 2010; Chen et al., 2018). Osteoarthritis is one of the most common joint diseases associated with progressive damage and loss of articular cartilage, thus exploring drugs that promote cartilage regeneration could be promising for the treatment of osteoarthritis (Hunter and Bierma-Zeinstra, 2019). MSC-Exos could increase chondrocyte proliferation and improve cartilage regeneration by increasing the protein translation and mRNA expression of hyaline cartilage-specific genes aggrecan, collagen II, and SOX9 (Li et al., 2021; Liao et al., 2021). Similarly, MSC-Exos could promote macrophage polarization toward the M2 phenotype and further inhibit the inflammatory response, creating favorable conditions for osteochondral regeneration (Jiang et al., 2021). In the process of cartilage formation treated with BMSC-Exos, the expression level of c-MYC was reduced, indicating that the exosomes could promote cartilage maturation (Iwamoto et al., 1993).
Osteoporosis is caused by complex metabolic factors and is characterized by an obvious decline in bone mineral density and bone microstructure damage (Saito and Marumo, 2010; Hamann et al., 2012). The disease is related to an imbalance between the number and function of osteoblasts and osteoclasts. Moreover, angiogenesis, inflammation, oxidative stress and miRNAs have been involved in the process of osteoporosis (Li et al., 2018; Lu et al., 2021). It was reported that MSC-Exos could promote osteogenesis of BMSCs and promote the proliferation of osteoblasts to alleviate osteoporosis (Qi et al., 2016; Zhao et al., 2018). It was demonstrated that BMSC-exosomal metastasis-associated lung adenocarcinoma transcript 1 (MALAT1) promoted osteoblast activity in osteoporotic mice by the miR-34c/SATB2 signaling pathway (Yang et al., 2019). MALAT1 contained in exosomes derived from BMSCs promoted alkaline phosphatase activity of osteoblasts and mineralized nodules by increasing the expression level of SATB2 (Yang et al., 2019). Previous researches have demonstrated that activating MAPK signaling plays a crucial role in inducing osteoblasts differentiation to reduce and prevent osteoporosis, which may be mediated by increasing the expression levels of P-p38 and P-Jun N-terminal kinase (P-JNK) (Gallea et al., 2001; Zhao et al., 2018). Besides, MSC-Exos could suppress the activation of the NLRP3 inflammasome, inhibit the IL-1β and IL-18 secretion, and alleviate the inflammatory response to improve osteoporosis (Zhang et al., 2021a). Moreover, BMSC-Exos enriched with miR-196a could promote osteogenic differentiation (Peng et al., 2021). Mechanistic studies showed that miR-196a delivered by BMSC-Exos plays an essential role in enhancing osteoblastic differentiation by inhibiting Dkk1 expression to activate the Wnt/β-catenin pathway. Exosomes from human umbilical cord MSCs are also able to inhibit BMSC apoptosis and improve the degree of osteoporosis in rats, which was mediated via the miR-1263/Mob1/Hippo signaling pathway (Yang et al., 2020a).
Currently, exosomes are widely viewed as effective therapeutic components derived from MSCs, and the secretion of exosomes is an important way for MSCs to promote the repair of surrounding tissue injuries. There are ongoing researches on the benefits of therapy with MSC-Exos for IDD, as well as bone defects and injuries. The core underlying pathophysiologic mechanism of IDD are abnormalities and a reduced number of NPCs. The functional substance in MSC-Exos can regulate the cell metabolism and function by transferring to NPCs, endplate chondrocytes and annulus fibrosus cells, thus inhibiting IDD. Additionally, MSC-Exos also showed great therapeutic potential in terms of repair in bone defects and injuries via promoting osteogenic differentiation and angiogenesis and regulating the immune response, and similar results have been illustrated with respect to its therapeutic and preventive effects against cartilage injuries and osteoporosis. Furthermore, the application of novel biomaterials such as hydrogels could prolong the duration of exosomes at the bone injury site and maintain the function and stability of intracapsular proteins and miRNA. In order to enable MSCs to play a better role in repairing tissue injury, studies should continue the exploration of new methods to promote the delivery of bioactive substances in exosomes more efficient and novel biomaterials that can maintain the physiological state of MSC-Exos.
All authors contributed to the review conception and design. The first draft of the manuscript was written by WL. Material preparation, literature collection and analysis were performed by WL, BH, and DS. The work was critically revised by YH and PY. All authors commented on previous versions of the manuscript, as well as, read and approved the final manuscript.
This work was supported by the Youth Science Fund of the Beijing Municipal Natural Science Foundation (No. 7204264), National Natural Science Foundation of China (No. 81772421), Innovation Grant of National Clinical Research Center for Orthopedics, Sports Medicine and Rehabilitation (No. 2021-NCRC-CXJJ-PY-34), and National Key Research and Development Program of China (No. 2019YFC0120604).
The authors declare that the research was conducted in the absence of any commercial or financial relationships that could be construed as a potential conflict of interest.
All claims expressed in this article are solely those of the authors and do not necessarily represent those of their affiliated organizations, or those of the publisher, the editors and the reviewers. Any product that may be evaluated in this article, or claim that may be made by its manufacturer, is not guaranteed or endorsed by the publisher.
We appreciate the guidance and support from YH and PY on this study.
Adams, M. A., and Roughley, P. J. (2006). What Is Intervertebral Disc Degeneration, and what Causes it? Spine 31 (18), 2151–2161. doi:10.1097/01.brs.0000231761.73859.2c
Amini, A. R., Laurencin, C. T., and Nukavarapu, S. P. (2012). Bone Tissue Engineering: Recent Advances and Challenges. Crit. Rev. Biomed. Eng. 40 (5), 363–408. doi:10.1615/critrevbiomedeng.v40.i5.10
Benjamin, R. M. (2010). Bone Health: Preventing Osteoporosis. Public Health Rep. 125 (3), 368–370. doi:10.1177/003335491012500302
Chen, S., Tang, Y., Liu, Y., Zhang, P., Lv, L., Zhang, X., et al. (2019). Exosomes Derived from miR-375-Overexpressing Human Adipose Mesenchymal Stem Cells Promote Bone Regeneration. Cell Prolif 52 (5), e12669. doi:10.1111/cpr.12669
Chen, Y., Xue, K., Zhang, X., Zheng, Z., and Liu, K. (2018). Exosomes Derived from Mature Chondrocytes Facilitate Subcutaneous Stable Ectopic Chondrogenesis of Cartilage Progenitor Cells. Stem Cel Res Ther 9 (1), 318. doi:10.1186/s13287-018-1047-2
Cheng, X., Zhang, G., Zhang, L., Hu, Y., Zhang, K., Sun, X., et al. (2018). Mesenchymal Stem Cells Deliver Exogenous miR-21viaexosomes to Inhibit Nucleus Pulposus Cell Apoptosis and Reduce Intervertebral Disc Degeneration. J. Cel. Mol. Med. 22 (1), 261–276. doi:10.1111/jcmm.13316
Chimal-Monroy, J., Rodriguez-Leon, J., Montero, J. A., Gañan, Y., Macias, D., Merino, R., et al. (2003). Analysis of the Molecular cascade Responsible for Mesodermal Limb Chondrogenesis: Sox Genes and BMP Signaling. Develop. Biol. 257 (2), 292–301. doi:10.1016/s0012-1606(03)00066-6
Choi, H., Johnson, Z., and Risbud, M. (2015). Understanding Nucleus Pulposus Cell Phenotype: a Prerequisite for Stem Cell Based Therapies to Treat Intervertebral Disc Degeneration. Curr. Stem Cel Res. Ther. 10 (4), 307–316. doi:10.2174/1574888x10666150113112149
Cosenza, S., Ruiz, M., Toupet, K., Jorgensen, C., and Noël, D. (2017). Mesenchymal Stem Cells Derived Exosomes and Microparticles Protect Cartilage and Bone from Degradation in Osteoarthritis. Sci. Rep. 7 (1), 16214. doi:10.1038/s41598-017-15376-8
Croft, A. S., Illien-Jünger, S., Grad, S., Guerrero, J., Wangler, S., and Gantenbein, B. (2021). The Application of Mesenchymal Stromal Cells and Their Homing Capabilities to Regenerate the Intervertebral Disc. Int. J. Mol. Sci. 22 (7), 3519. doi:10.3390/ijms22073519
De Geer, C. M. (2018). Intervertebral Disk Nutrients and Transport Mechanisms in Relation to Disk Degeneration: A Narrative Literature Review. J. Chiropractic Med. 17 (2), 97–105. doi:10.1016/j.jcm.2017.11.006
de Jong, O. G., Verhaar, M. C., Chen, Y., Vader, P., Gremmels, H., Posthuma, G., et al. (2012). Cellular Stress Conditions Are Reflected in the Protein and RNA Content of Endothelial Cell-Derived Exosomes. J. Extracellular Vesicles 1, 18396. doi:10.3402/jev.v1i0.18396
Dimitriou, R., Jones, E., McGonagle, D., and Giannoudis, P. V. (2011). Bone Regeneration: Current Concepts and Future Directions. BMC Med. 9, 66. doi:10.1186/1741-7015-9-66
Dowdell, J., Erwin, M., Choma, T., Vaccaro, A., Iatridis, J., and Cho, S. K. (2017). Intervertebral Disk Degeneration and Repair. Neurosurgery 80 (3S), S46–S54. doi:10.1093/neuros/nyw078
Einhorn, T. A., and Gerstenfeld, L. C. (2015). Fracture Healing: Mechanisms and Interventions. Nat. Rev. Rheumatol. 11 (1), 45–54. doi:10.1038/nrrheum.2014.164
Fan, L., Guan, P., Xiao, C., Wen, H., Wang, Q., Liu, C., et al. (2021). Exosome-functionalized Polyetheretherketone-Based Implant with Immunomodulatory Property for Enhancing Osseointegration. Bioactive Mater. 6 (9), 2754–2766. doi:10.1016/j.bioactmat.2021.02.005
Feng, C., Yang, M., Lan, M., Liu, C., Zhang, Y., Huang, B., et al. (2017). ROS: Crucial Intermediators in the Pathogenesis of Intervertebral Disc Degeneration. Oxidative Med. Cell Longevity 2017, 1–12. doi:10.1155/2017/5601593
Gallea, S., Lallemand, F., Atfi, A., Rawadi, G., Ramez, V., Spinella-Jaegle, S., et al. (2001). Activation of Mitogen-Activated Protein Kinase Cascades Is Involved in Regulation of Bone Morphogenetic Protein-2-Induced Osteoblast Differentiation in Pluripotent C2C12 Cells. Bone 28 (5), 491–498. doi:10.1016/s8756-3282(01)00415-x
Gaur, T., Lengner, C. J., Hovhannisyan, H., Bhat, R. A., Bodine, P. V. N., Komm, B. S., et al. (2005). Canonical WNT Signaling Promotes Osteogenesis by Directly Stimulating Runx2 Gene Expression. J. Biol. Chem. 280 (39), 33132–33140. doi:10.1074/jbc.M500608200
Gibon, E., Lu, L. Y., Nathan, K., and Goodman, S. B. (2017). Inflammation, Ageing, and Bone Regeneration. J. Orthopaedic Translation 10, 28–35. doi:10.1016/j.jot.2017.04.002
Gruber, H. E., Hoelscher, G. L., Ingram, J. A., Bethea, S., and Hanley, E. N. (2015). Autophagy in the Degenerating Human Intervertebral Disc. Spine 40 (11), 773–782. doi:10.1097/brs.0000000000000865
Grunhagen, T., Shirazi-Adl, A., Fairbank, J. C. T., and Urban, J. P. G. (2011). Intervertebral Disk Nutrition: a Review of Factors Influencing Concentrations of Nutrients and Metabolites. Orthop. Clin. North America 42 (4), 465–477. doi:10.1016/j.ocl.2011.07.010
Guadix, J. A., Zugaza, J. L., and Gálvez-Martín, P. (2017). Characteristics, Applications and Prospects of Mesenchymal Stem Cells in Cell Therapy. Medicina Clínica (English Edition) 148 (9), 408–414. doi:10.1016/j.medcli.2016.11.03310.1016/j.medcle.2017.04.018
Hamann, C., Kirschner, S., Günther, K.-P., and Hofbauer, L. C. (2012). Bone, Sweet Bone-Osteoporotic Fractures in Diabetes Mellitus. Nat. Rev. Endocrinol. 8 (5), 297–305. doi:10.1038/nrendo.2011.233
Hankenson, K. D., Dishowitz, M., Gray, C., and Schenker, M. (2011). Angiogenesis in Bone Regeneration. Injury 42 (6), 556–561. doi:10.1016/j.injury.2011.03.035
Hingert, D., Ekström, K., Aldridge, J., Crescitelli, R., and Brisby, H. (2020). Extracellular Vesicles from Human Mesenchymal Stem Cells Expedite Chondrogenesis in 3D Human Degenerative Disc Cell Cultures. Stem Cel Res Ther 11 (1), 323. doi:10.1186/s13287-020-01832-2
Hu, Y., Tao, R., Wang, L., Chen, L., Lin, Z., Panayi, A. C., et al. (2021). Exosomes Derived from Bone Mesenchymal Stem Cells Alleviate Compression-Induced Nucleus Pulposus Cell Apoptosis by Inhibiting Oxidative Stress. Oxidative Med. Cell Longevity 2021, 1–12. doi:10.1155/2021/2310025
Hu, Z.-L., Li, H.-Y., Chang, X., Li, Y.-Y., Liu, C.-H., Gao, X.-X., et al. (2020). Exosomes Derived from Stem Cells as an Emerging Therapeutic Strategy for Intervertebral Disc Degeneration. World J. Stem Cell 12 (8), 803–813. doi:10.4252/wjsc.v12.i8.803
Huang, Y.-C., Leung, V. Y. L., Lu, W. W., and Luk, K. D. K. (2013). The Effects of Microenvironment in Mesenchymal Stem Cell-Based Regeneration of Intervertebral Disc. Spine J. 13 (3), 352–362. doi:10.1016/j.spinee.2012.12.005
Hunter, D. J., and Bierma-Zeinstra, S. (2019). Osteoarthritis. The Lancet 393 (10182), 1745–1759. doi:10.1016/s0140-6736(19)30417-9
Iwamoto, M., Yagami, K., Lu Valle, P., Olsen, B. R., Petropoulos, C. J., Ewert, D. L., et al. (1993). Expression and Role of C-Myc in Chondrocytes Undergoing Endochondral Ossification. J. Biol. Chem. 268 (13), 9645–9652. doi:10.1016/s0021-9258(18)98398-5
Jia, Y., Qiu, S., Xu, J., Kang, Q., and Chai, Y. (2020). Exosomes Secreted by Young Mesenchymal Stem Cells Promote New Bone Formation during Distraction Osteogenesis in Older Rats. Calcif Tissue Int. 106 (5), 509–517. doi:10.1007/s00223-019-00656-4
Jiang, S., Tian, G., Yang, Z., Gao, X., Wang, F., Li, J., et al. (2021). Enhancement of Acellular Cartilage Matrix Scaffold by Wharton's Jelly Mesenchymal Stem Cell-Derived Exosomes to Promote Osteochondral Regeneration. Bioactive Mater. 6 (9), 2711–2728. doi:10.1016/j.bioactmat.2021.01.031
Jiang, Y., Jahagirdar, B. N., Reinhardt, R. L., Schwartz, R. E., Keene, C. D., Ortiz-Gonzalez, X. R., et al. (2002). Pluripotency of Mesenchymal Stem Cells Derived from Adult Marrow. Nature 418 (6893), 41–49. doi:10.1038/nature00870
Kaplan, D., Reagan, M. R., and Kaplan, D. L. (2010). Role of Cartilage-Forming Cells in Regenerative Medicine for Cartilage Repair. Orthop. Res. Rev. 2 (2), 85–94. doi:10.2147/ORR.S7194
Kozacı, L. D., Guner, A., Oktay, G., and Guner, G. (2006). Alterations in Biochemical Components of Extracellular Matrix in Intervertebral Disc Herniation: Role of MMP-2 and TIMP-2 in Type II Collagen Loss. Cell Biochem. Funct. 24 (5), 431–436. doi:10.1002/cbf.1250
Krut, Z., Pelled, G., Gazit, D., and Gazit, Z. (2021). Stem Cells and Exosomes: New Therapies for Intervertebral Disc Degeneration. Cells 10 (9), 2241. doi:10.3390/cells10092241
Li, G., Yin, J., Gao, J., Cheng, T. S., Pavlos, N. J., Zhang, C., et al. (2013). Subchondral Bone in Osteoarthritis: Insight into Risk Factors and Microstructural Changes. Arthritis Res. Ther. 15 (6), 223. doi:10.1186/ar4405
Li, M., Li, R., Yang, S., Yang, D., Gao, X., Sun, J., et al. (2020a). Exosomes Derived from Bone Marrow Mesenchymal Stem Cells Prevent Acidic pH-Induced Damage in Human Nucleus Pulposus Cells. Med. Sci. Monit. 26, e922928. doi:10.12659/msm.922928
Li, Q., Yu, H., Sun, M., Yang, P., Hu, X., Ao, Y., et al. (2021). The Tissue Origin Effect of Extracellular Vesicles on Cartilage and Bone Regeneration. Acta Biomater. 125, 253–266. doi:10.1016/j.actbio.2021.02.039
Li, Y., Jin, D., Xie, W., Wen, L., Chen, W., Xu, J., et al. (2018). Mesenchymal Stem Cells-Derived Exosomes: A Possible Therapeutic Strategy for Osteoporosis. Curr. Stem Cel Res. 13 (5), 362–368. doi:10.2174/1574888x13666180403163456
Li, Z.-q., Kong, L., Liu, C., and Xu, H.-G. (2020b). Human Bone Marrow Mesenchymal Stem Cell-Derived Exosomes Attenuate IL-1β-induced Annulus Fibrosus Cell Damage. Am. J. Med. Sci. 360 (6), 693–700. doi:10.1016/j.amjms.2020.07.025
Liang, B., Liang, J.-M., Ding, J.-N., Xu, J., Xu, J.-G., and Chai, Y.-M. (2019). Dimethyloxaloylglycine-stimulated Human Bone Marrow Mesenchymal Stem Cell-Derived Exosomes Enhance Bone Regeneration through Angiogenesis by Targeting the AKT/mTOR Pathway. Stem Cel Res Ther 10 (1), 335. doi:10.1186/s13287-019-1410-y
Liao, Q., Li, B. J., Li, Y., Xiao, Y., Zeng, H., Liu, J. M., et al. (2021). Low-intensity Pulsed Ultrasound Promotes Osteoarthritic Cartilage Regeneration by BMSC-Derived Exosomes via Modulating the NF-Κb Signaling Pathway. Int. Immunopharmacology 97, 107824. doi:10.1016/j.intimp.2021.107824
Liao, Z., Luo, R., Li, G., Song, Y., Zhan, S., Zhao, K., et al. (2019). Exosomes from Mesenchymal Stem Cells Modulate Endoplasmic Reticulum Stress to Protect against Nucleus Pulposus Cell Death and Ameliorate Intervertebral Disc Degeneration In Vivo. Theranostics 9 (14), 4084–4100. doi:10.7150/thno.33638
Loi, F., Córdova, L. A., Pajarinen, J., Lin, T.-h., Yao, Z., and Goodman, S. B. (2016). Inflammation, Fracture and Bone Repair. Bone 86, 119–130. doi:10.1016/j.bone.2016.02.020
Lou, G., Chen, Z., Zheng, M., and Liu, Y. (2017). Mesenchymal Stem Cell-Derived Exosomes as a New Therapeutic Strategy for Liver Diseases. Exp. Mol. Med. 49 (6), e346. doi:10.1038/emm.2017.63
Lu, J., Wang, Q.-Y., and Sheng, J.-G. (2019). Exosomes in the Repair of Bone Defects: Next-Generation Therapeutic Tools for the Treatment of Nonunion. Biomed. Res. Int. 2019, 1–11. doi:10.1155/2019/1983131
Lu, J., Zhang, Y., Liang, J., Diao, J., Liu, P., and Zhao, H. (2021). Role of Exosomal MicroRNAs and Their Crosstalk with Oxidative Stress in the Pathogenesis of Osteoporosis. Oxidative Med. Cell Longevity 2021, 1–8. doi:10.1155/2021/6301433
Lu, K., Li, H.-y., Yang, K., Wu, J.-l., Cai, X.-w., Zhou, Y., et al. (2017). Exosomes as Potential Alternatives to Stem Cell Therapy for Intervertebral Disc Degeneration: In-Vitro Study on Exosomes in Interaction of Nucleus Pulposus Cells and Bone Marrow Mesenchymal Stem Cells. Stem Cel Res Ther 8 (1), 108. doi:10.1186/s13287-017-0563-9
Lyu, F.-J., Cui, H., Pan, H., Mc Cheung, K., Cao, X., Iatridis, J. C., et al. (2021). Painful Intervertebral Disc Degeneration and Inflammation: from Laboratory Evidence to Clinical Interventions. Bone Res. 9 (1), 7. doi:10.1038/s41413-020-00125-x
Malandrino, A., Lacroix, D., Hellmich, C., Ito, K., Ferguson, S. J., and Noailly, J. (2014). The Role of Endplate Poromechanical Properties on the Nutrient Availability in the Intervertebral Disc. Osteoarthritis and Cartilage 22 (7), 1053–1060. doi:10.1016/j.joca.2014.05.005
Maldonado, B. A., and Oegema, T. R. (1992). Initial Characterization of the Metabolism of Intervertebral Disc Cells Encapsulated in Microspheres. J. Orthop. Res. 10 (5), 677–690. doi:10.1002/jor.1100100510
Manchikanti, L., Singh, V., Falco, F. J. E., Benyamin, R. M., and Hirsch, J. A. (2014). Epidemiology of Low Back Pain in Adults. Neuromodulation: Tech. Neural Interf. 17 (2), 3–10. doi:10.1111/ner.12018
Marsell, R., and Einhorn, T. A. (2011). The Biology of Fracture Healing. Injury 42 (6), 551–555. doi:10.1016/j.injury.2011.03.031
Peng, Z., Lu, S., Lou, Z., Li, Z., Li, S., Yang, K., et al. (2021). Exosomes from Bone Marrow Mesenchymal Stem Cells Promoted Osteogenic Differentiation by Delivering miR-196a that Targeted Dickkopf-1 to Activate Wnt/β-Catenin Pathway. Bioengineered. doi:10.1080/21655979.2021.1996015
Pittenger, M. F., Mackay, A. M., Beck, S. C., Jaiswal, R. K., Douglas, R., Mosca, J. D., et al. (1999). Multilineage Potential of Adult Human Mesenchymal Stem Cells. Science 284 (5411), 143–147. doi:10.1126/science.284.5411.143
Qi, X., Zhang, J., Yuan, H., Xu, Z., Li, Q., Niu, X., et al. (2016). Exosomes Secreted by Human-Induced Pluripotent Stem Cell-Derived Mesenchymal Stem Cells Repair Critical-Sized Bone Defects through Enhanced Angiogenesis and Osteogenesis in Osteoporotic Rats. Int. J. Biol. Sci. 12 (7), 836–849. doi:10.7150/ijbs.14809
Riau, A. K., Ong, H. S., Yam, G. H. F., and Mehta, J. S. (2019). Sustained Delivery System for Stem Cell-Derived Exosomes. Front. Pharmacol. 10, 1368. doi:10.3389/fphar.2019.01368
Saito, M., and Marumo, K. (2010). Collagen Cross-Links as a Determinant of Bone Quality: a Possible Explanation for Bone Fragility in Aging, Osteoporosis, and Diabetes Mellitus. Osteoporos. Int. 21 (2), 195–214. doi:10.1007/s00198-009-1066-z
Shi, M., Zhao, Y., Sun, Y., Xin, D., Xu, W., and Zhou, B. (2021). Therapeutic Effect of Co-culture of Rat Bone Marrow Mesenchymal Stem Cells and Degenerated Nucleus Pulposus Cells on Intervertebral Disc Degeneration. Spine J. 21 (9), 1567–1579. doi:10.1016/j.spinee.2021.05.007
Simons, M., and Raposo, G. (2009). Exosomes - Vesicular Carriers for Intercellular Communication. Curr. Opin. Cel Biol. 21 (4), 575–581. doi:10.1016/j.ceb.2009.03.007
Suzuki, S., Fujita, N., Hosogane, N., Watanabe, K., Ishii, K., Toyama, Y., et al. (2015). Excessive Reactive Oxygen Species Are Therapeutic Targets for Intervertebral Disc Degeneration. Arthritis Res. Ther. 17, 316. doi:10.1186/s13075-015-0834-8
Ti, D., Hao, H., Fu, X., and Han, W. (2016). Mesenchymal Stem Cells-Derived Exosomal microRNAs Contribute to Wound Inflammation. Sci. China Life Sci. 59 (12), 1305–1312. doi:10.1007/s11427-016-0240-4
Uccelli, A., Moretta, L., and Pistoia, V. (2008). Mesenchymal Stem Cells in Health and Disease. Nat. Rev. Immunol. 8 (9), 726–736. doi:10.1038/nri2395
Urban, J. P., and Roberts, S. (2003). Degeneration of the Intervertebral Disc. Arthritis Res. Ther. 5 (3), 120–130. doi:10.1186/ar629
Valadi, H., Ekström, K., Bossios, A., Sjöstrand, M., Lee, J. J., and Lötvall, J. O. (2007). Exosome-mediated Transfer of mRNAs and microRNAs Is a Novel Mechanism of Genetic Exchange between Cells. Nat. Cel Biol 9 (6), 654–659. doi:10.1038/ncb1596
Vergroesen, P.-P. A., Kingma, I., Emanuel, K. S., Hoogendoorn, R. J. W., Welting, T. J., van Royen, B. J., et al. (2015). Mechanics and Biology in Intervertebral Disc Degeneration: a Vicious circle. Osteoarthritis and Cartilage 23 (7), 1057–1070. doi:10.1016/j.joca.2015.03.028
Wang, K.-X., Xu, L.-L., Rui, Y.-F., Huang, S., Lin, S.-E., Xiong, J.-H., et al. (2015). The Effects of Secretion Factors from Umbilical Cord Derived Mesenchymal Stem Cells on Osteogenic Differentiation of Mesenchymal Stem Cells. PLoS One 10 (3), e0120593. doi:10.1371/journal.pone.0120593
Wang, X., Li, X., Li, J., Zhai, L., Liu, D., Abdurahman, A., et al. (2021a). Mechanical Loading Stimulates Bone Angiogenesis through Enhancing Type H Vessel Formation and Downregulating Exosomal miR-214-3p from Bone Marrow-Derived Mesenchymal Stem Cells. FASEB J. 35 (1), e21150. doi:10.1096/fj.202001080RR
Wang, Z., Wu, Y., Zhao, Z., Liu, C., and Zhang, L. (2021b). Study on Transorgan Regulation of Intervertebral Disc and Extra-skeletal Organs through Exosomes Derived from Bone Marrow Mesenchymal Stem Cells. Front. Cel Dev. Biol. 9, 741183. doi:10.3389/fcell.2021.741183
Wei, F., Li, Z., Crawford, R., Xiao, Y., and Zhou, Y. (2019). Immunoregulatory Role of Exosomes Derived from Differentiating Mesenchymal Stromal Cells on Inflammation and Osteogenesis. J. Tissue Eng. Regen. Med. 13 (11), 1978–1991. doi:10.1002/term.2947
Wong, J., Sampson, S. L., Bell-Briones, H., Ouyang, A., Lazar, A. A., Lotz, J. C., et al. (2019). Nutrient Supply and Nucleus Pulposus Cell Function: Effects of the Transport Properties of the Cartilage Endplate and Potential Implications for Intradiscal Biologic Therapy. Osteoarthritis and Cartilage 27 (6), 956–964. doi:10.1016/j.joca.2019.01.013
Xie, L., Chen, Z., Liu, M., Huang, W., Zou, F., Ma, X., et al. (2020). MSC-derived Exosomes Protect Vertebral Endplate Chondrocytes against Apoptosis and Calcification via the miR-31-5p/ATF6 Axis. Mol. Ther. - Nucleic Acids 22, 601–614. doi:10.1016/j.omtn.2020.09.026
Xing, H., Zhang, Z., Mao, Q., Wang, C., Zhou, Y., Zhou, X., et al. (2021). Injectable Exosome-Functionalized Extracellular Matrix Hydrogel for Metabolism Balance and Pyroptosis Regulation in Intervertebral Disc Degeneration. J. Nanobiotechnol 19 (1), 264. doi:10.1186/s12951-021-00991-5
Xu, D., Jin, H., Wen, J., Chen, J., Chen, D., Cai, N., et al. (2017). Hydrogen Sulfide Protects against Endoplasmic Reticulum Stress and Mitochondrial Injury in Nucleus Pulposus Cells and Ameliorates Intervertebral Disc Degeneration. Pharmacol. Res. 117, 357–369. doi:10.1016/j.phrs.2017.01.005
Yang, B.-c., Kuang, M.-j., Kang, J.-y., Zhao, J., Ma, J.-x., and Ma, X.-l. (2020a). Human Umbilical Cord Mesenchymal Stem Cell-Derived Exosomes Act via the miR-1263/Mob1/Hippo Signaling Pathway to Prevent Apoptosis in Disuse Osteoporosis. Biochem. Biophysical Res. Commun. 524 (4), 883–889. doi:10.1016/j.bbrc.2020.02.001
Yang, S., Zhang, F., Ma, J., and Ding, W. (2020b). Intervertebral Disc Ageing and Degeneration: The Antiapoptotic Effect of Oestrogen. Ageing Res. Rev. 57, 100978. doi:10.1016/j.arr.2019.100978
Yang, X., Yang, J., Lei, P., and Wen, T. (2019). LncRNA MALAT1 Shuttled by Bone Marrow-Derived Mesenchymal Stem Cells-Secreted Exosomes Alleviates Osteoporosis through Mediating microRNA-34c/SATB2 axis. Aging 11 (20), 8777–8791. doi:10.18632/aging.102264
Yang, Z., Zhang, W., Ren, X., Tu, C., and Li, Z. (2021). Exosomes: A Friend or Foe for Osteoporotic Fracture? Front. Endocrinol. 12, 679914. doi:10.3389/fendo.2021.679914
Yu, H., VandeVord, P. J., Mao, L., Matthew, H. W., Wooley, P. H., and Yang, S.-Y. (2009). Improved Tissue-Engineered Bone Regeneration by Endothelial Cell Mediated Vascularization. Biomaterials 30 (4), 508–517. doi:10.1016/j.biomaterials.2008.09.047
Zhang, J., Liu, X., Li, H., Chen, C., Hu, B., Niu, X., et al. (2016). Exosomes/tricalcium Phosphate Combination Scaffolds Can Enhance Bone Regeneration by Activating the PI3K/Akt Signaling Pathway. Stem Cel Res Ther 7 (1), 136. doi:10.1186/s13287-016-0391-3
Zhang, J., Zhang, J., Zhang, Y., Liu, W., Ni, W., Huang, X., et al. (2020a). Mesenchymal Stem Cells-Derived Exosomes Ameliorate Intervertebral Disc Degeneration through Inhibiting Pyroptosis. J. Cel. Mol. Med. 24 (20), 11742–11754. doi:10.1111/jcmm.15784
Zhang, L., Wang, Q., Su, H., and Cheng, J. (2021a). Exosomes from Adipose Derived Mesenchymal Stem Cells Alleviate Diabetic Osteoporosis in Rats through Suppressing NLRP3 Inflammasome Activation in Osteoclasts. J. Biosci. Bioeng. 131 (6), 671–678. doi:10.1016/j.jbiosc.2021.02.007
Zhang, T., Yin, M., Wang, L., Cao, W., and Zhong, S. (2020b). Diagnostic Performance of Blood-Based Liquid Biopsies in Hepatocellular Carcinoma. Med 99 (41), e22594. doi:10.1097/md.0000000000022594
Zhang, X., Yuan, X., Shi, H., Wu, L., Qian, H., and Xu, W. (2015). Exosomes in Cancer: Small Particle, Big Player. J. Hematol. Oncol. 8, 83. doi:10.1186/s13045-015-0181-x
Zhang, Y., Liu, Y., Liu, H., and Tang, W. H. (2019). Exosomes: Biogenesis, Biologic Function and Clinical Potential. Cell Biosci 9, 19. doi:10.1186/s13578-019-0282-2
Zhang, Y., Xie, Y., Hao, Z., Zhou, P., Wang, P., Fang, S., et al. (2021b). Umbilical Mesenchymal Stem Cell-Derived Exosome-Encapsulated Hydrogels Accelerate Bone Repair by Enhancing Angiogenesis. ACS Appl. Mater. Inter. 13 (16), 18472–18487. doi:10.1021/acsami.0c22671
Zhang, Z., Zhang, L., Yang, J., Huang, J., Cai, J., Zhang, S., et al. (2021c). Influence of Extracellular Nanovesicles Derived from Adipose-Derived Stem Cells on Nucleus Pulposus Cell from Patients with Intervertebral Disc Degeneration. Exp. Ther. Med. 22 (6), 1431. doi:10.3892/etm.2021.10866
Zhao, P., Xiao, L., Peng, J., Qian, Y. Q., and Huang, C. C. (2018). Exosomes Derived from Bone Marrow Mesenchymal Stem Cells Improve Osteoporosis through Promoting Osteoblast Proliferation via MAPK Pathway. Eur. Rev. Med. Pharmacol. Sci. 22 (12), 3962–3970. doi:10.26355/eurrev_201806_15280
Zhou, J., Liu, H. X., Li, S. H., Gong, Y. S., Zhou, M. W., Zhang, J. H., et al. (2019). Effects of Human Umbilical Cord Mesenchymal Stem Cells-Derived Exosomes on Fracture Healing in Rats through the Wnt Signaling Pathway. Eur. Rev. Med. Pharmacol. Sci. 23 (11), 4954–4960. doi:10.26355/eurrev_201906_18086
Zhu, G., Yang, X., Peng, C., Yu, L., and Hao, Y. (2020a). Exosomal miR-532-5p from Bone Marrow Mesenchymal Stem Cells Reduce Intervertebral Disc Degeneration by Targeting RASSF5. Exp. Cel Res. 393 (2), 112109. doi:10.1016/j.yexcr.2020.112109
Zhu, L., Shi, Y., Liu, L., Wang, H., Shen, P., and Yang, H. (2020b). Mesenchymal Stem Cells-Derived Exosomes Ameliorate Nucleus Pulposus Cells Apoptosis via Delivering miR-142-3p: Therapeutic Potential for Intervertebral Disc Degenerative Diseases. Cell Cycle 19 (14), 1727–1739. doi:10.1080/15384101.2020.1769301
Keywords: exosomes, mesenchymal stem cells (MeSH ID D059630), intervertebral disc degeneration (IDD), bone repair and regeneration, mechanism of action
Citation: Liang W, Han B, Hai Y, Sun D and Yin P (2022) Mechanism of Action of Mesenchymal Stem Cell-Derived Exosomes in the Intervertebral Disc Degeneration Treatment and Bone Repair and Regeneration. Front. Cell Dev. Biol. 9:833840. doi: 10.3389/fcell.2021.833840
Received: 12 December 2021; Accepted: 27 December 2021;
Published: 14 January 2022.
Edited by:
Bo Gao, Air Force Military Medical University, ChinaReviewed by:
Zhongyang Liu, Chinese PLA General Hospital, ChinaCopyright © 2022 Liang, Han, Hai, Sun and Yin. This is an open-access article distributed under the terms of the Creative Commons Attribution License (CC BY). The use, distribution or reproduction in other forums is permitted, provided the original author(s) and the copyright owner(s) are credited and that the original publication in this journal is cited, in accordance with accepted academic practice. No use, distribution or reproduction is permitted which does not comply with these terms.
*Correspondence: Yong Hai, eW9uZy5oYWlAY2NtdS5lZHUuY24=; Peng Yin, eWlucGVuZzM5MDRAMTI2LmNvbQ==
†These authors have contributed equally to this work and share first authorship
Disclaimer: All claims expressed in this article are solely those of the authors and do not necessarily represent those of their affiliated organizations, or those of the publisher, the editors and the reviewers. Any product that may be evaluated in this article or claim that may be made by its manufacturer is not guaranteed or endorsed by the publisher.
Research integrity at Frontiers
Learn more about the work of our research integrity team to safeguard the quality of each article we publish.