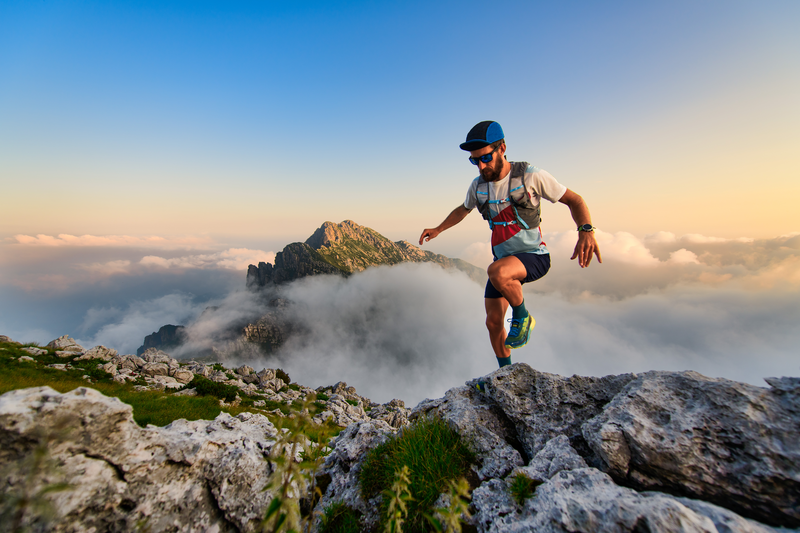
94% of researchers rate our articles as excellent or good
Learn more about the work of our research integrity team to safeguard the quality of each article we publish.
Find out more
MINI REVIEW article
Front. Cell Dev. Biol. , 21 January 2022
Sec. Molecular and Cellular Reproduction
Volume 9 - 2021 | https://doi.org/10.3389/fcell.2021.827214
This article is part of the Research Topic Fertilization in the Spotlight: Dynamics and Mechanisms of Sperm-Egg Interaction View all 16 articles
Fertilization is one of the most important events in living organisms to generate a new life with a mixed genetic background. To achieve successful fertilization, sperm and eggs must undergo complex processes in a sequential order. Fertilization of marine invertebrate Ciona intestinalis type A (Ciona robusta) has been studied for more than a hundred years. Ascidian sperm are attracted by chemoattractants from eggs and bind to the vitelline coat. Subsequently, sperm penetrate through the vitelline coat proteolytically and finally fuse with the egg plasma membrane. Here, we summarize the fertilization mechanisms of ascidians, particularly from sperm-egg interactions to sperm penetration of the egg coat. Since ascidians are hermaphrodites, inbreeding depression is a serious problem. To avoid self-fertilization, ascidians possess a self-incompatibility system. In this review, we also describe the molecular mechanisms of the self-incompatibility system in C. intestinalis type A governed by three allelic gene pairs of s-Themis and v-Themis.
Fertilization of marine invertebrates has been extensively studied in classical research. This is not only because a large quantity of gametes can be obtained but also because in vitro fertilization experiments are easier in marine invertebrates than in mammals. Studying marine invertebrates led to the discovery of fundamental biological processes, including the fertilization process. The marine invertebrate Ciona intestinalis type A [another name: Ciona robusta (Brunetti et al., 2015)] is one of the model animals used to study many fields of biology, including reproductive biology (Sawada et al., 2001). The availability of draft genome sequences, transcriptomic and proteomics data and gene knockdown and gene editing techniques has supported the progress of C. intestinalis (type A) studies (Satou et al., 2001; Dehal et al., 2002; Shoguchi et al., 2006; Endo et al., 2011; Sasaki et al., 2014; Sasakura et al., 2017; Pickett and Zeller, 2018). Since ascidians are hermaphrodites, self-fertilization can occur. To avoid self-fertilization, many ascidians acquire a self-incompatibility (SI) system. After self/nonself-recognition, only nonself sperm can penetrate through the proteinaceous egg coat called the vitelline coat (VC) or chorion.
Ascidian fertilization consists of five major steps: 1) sperm chemotaxis, 2) sperm binding to the VC, 3) self/nonself-recognition, 4) sperm penetration through the VC, 5) gamete fusion (Figure 1), similar to the fertilization processes as previously reviewed (Vacquier and Swanson, 2011; Gallo and Costantini, 2012). In C. intestinalis (type A), spermatozoa are attracted toward the eggs by sperm attractant SAAF, a sulfated steroid 3,4,7,26-tetrahydroxycholestane-3,26-disulfate (Yoshida et al., 2002). Transient intracellular Ca2+ increase is induced by SAAF gradient (Shiba et al., 2008), which is mediated by store-operated calcium channel (Yoshida et al., 2003) and sperm-membrane SAAF-binding protein, Ca-ATPase (Yoshida et al., 2018). After sperm chemotaxis, spermatozoa bind to the VC and undergo “sperm reaction”, which is characterized by vigorous movement on the VC and mitochondrial sliding and eventual shedding (Lambert and Epel, 1979; Lambert and Koch, 1988). Most animal spermatozoon besides teleosts has an acrosome at the tip of sperm head and undergoes acrosome reaction, an exocytosis of the acrosomal vesicle, upon sperm binding to the carbohydrate or proteinaceous egg coat. However, the acrosome of ascidian sperm is very tiny and it is still unclear whether the acrosome reaction takes place before (De Santis et al., 1980) or after (Fukumoto, 1988) sperm penetration of the VC. In this minireview, we describe our current understanding and future perspectives on ascidian fertilization, particularly in steps 2) to 4). After sperm penetration of the egg coat, gamete fusion takes place, which is mediated by sperm IZUMO1 and egg JUNO and CD9 in mice (see review: Okabe, 2018). However, the molecular basis of gamete fusion is poorly understood in ascidians. Recently, GCS1/Hap2 is reported to play a pivotal role in gamete fusion in plants and animals (see review: Mori et al., 2015), but the homologous genes and their functions are not known in ascidians or vertebrates.
FIGURE 1. Fertilization steps of C. intestinalis. Fertilization is initiated by the following steps. (1) Sperm chemotaxis. (2) Binding of spermatozoa to the VC. (3) Interaction of self/nonself-recognition proteins that induce the signal transduction cascade leading to the SI response. (4) Penetration of nonself-recognized sperm through the VC. (5) Fusion of the egg and sperm plasma membranes resulting in fertilization and subsequent activation of zygote development.
After sperm attraction to the egg, gamete interaction takes place between the egg coat and sperm head (Figure 1). The egg coat is an extracellular matrix called the zona pellucida (ZP) in mammals and the vitelline envelope (VE) or VC in other animals. Structurally and evolutionally conserved egg coat proteins and ZP domain proteins are the major components of ZP, VE and VC (Aagaard et al., 2006; Goudet et al., 2008; Yamada et al., 2009). In mouse, the highly glycosylated proteins ZP1, ZP2 and ZP3, all of which contain a ZP domain at the C termini, are the building blocks of a filamentous network generated by polymerization of ZP domains (Bork and Sander, 1992; Jovine et al., 2002; Stsiapanava et al., 2020). Current studies have revealed that the N-terminal region of mouse and human ZP2 and the marine mollusk abalone VE receptor for lysin (VERL) crucially regulate gamete interactions (Gahlay et al., 2010; Avella et al., 2014; Raj et al., 2017). Similarly, the VC of ascidian eggs is constructed by ZP domain proteins (Sawada et al., 2002a; Kürn et al., 2007a; Yamada et al., 2009). In C. intestinalis, 11 ZP domain proteins have been identified by proteomic analysis of VC, and CiVC57 constitutes the most abundant ZP domain protein in VC. CiVC57 consists of a von Willebrand factor domain, 24 EGF-like repeats, a ZP domain, and a C-terminal transmembrane domain (Yamada et al., 2009). CiUrabin is an abundant protein on the surface of sperm, and in the lipid-raft-membrane fraction, it can bind to CiVC57, suggesting that CiVC57 and CiUrabin play a key role in gamete interaction (Yamaguchi et al., 2011; Nakazawa et al., 2015). CiUrabin belongs to a cysteine-rich secretory protein (CRISP) family containing a pathogenesis-related (PR) domain and a glycosylphosphatidylinositol (GPI)-anchor attachment site (Yamaguchi et al., 2011). Sperm CRISP family proteins participate in several steps in mammalian fertilization (Gonzalez et al., 2021). In particular, the PR domain of CRISP-1 is evolutionarily conserved and involved in sperm-egg binding (Ellerman et al., 2006). In addition, sperm-egg binding in another ascidian Halocynthia roretzi is mediated by the CRISP family protein HrUrabin (Urayama et al., 2008). Most likely, the interaction between CiVC57 and CiUrabin participates in the primary binding between sperm and VC in C. intestinalis (Yamaguchi et al., 2011; Saito et al., 2012).
It is also worth noting that C. intestinalis sperm α-L-fucosidase and L-fucosyl residues of glycoproteins on the VC play a key role in sperm binding to the VC for the following reasons (Hoshi et al., 1983; Hoshi et al., 1985). Sperm binding to the VC of glycerin-treated (glycerinated) eggs, whose VC sperm can bind to but not penetrate, was inhibited by L-fucose but not by D-fucose (Rosati and De Santis, 1980). In addition, α-L-fucosidase substrates (aryl α-L-fucoside) and competitive inhibitors (aryl β-L-fucoside) but not stereoisomer (aryl α-D-fucoside) blocked sperm binding to VC (Hoshi et al., 1983; Hoshi, 1985; Hoshi, 1986). α-L-fucosidase is located at the tip and the surface of the sperm head, as revealed by immunocytochemistry using several monoclonal antibodies raised against the purified enzyme. Whereas C. intestinalis sperm α-L-fucosidase showed an optimum pH for activity of approximately 3.9, it showed 2% or less of maximum activity in normal seawater (Hoshi et al., 1985). Sperm bound to VC in normal seawater detached within 7 min at 20°C but not at 0°C after decreasing the pH near the optimum pH of the enzyme (Hoshi et al., 1985; Hoshi, 1986). These results led to the conclusion that α-L-fucosidase at the tip of the sperm head is responsible for the primary binding of sperm to the fucosyl glycoprotein(s) on the VC in C. intestinalis (De Santis et al., 1983).
Shortly after sperm binding to the VC, a self/nonself-recognition process takes place on the VC (Morgan, 1939; Rosati and De Santis, 1978; Kawamura et al., 1987; Figure 1, Figure 2A). Identification of self/nonself-recognition molecules was attempted by several groups, and candidate molecules have been proposed (Kawamura et al., 1991; Pinto et al., 1995; Marino et al., 1998; Kürn et al., 2007a; Kürn et al., 2007b; Harada et al., 2008). Marino et al. proposed that autologous peptide-associating hsp70 on the surface of folliele cells participates in self/nonself-recognition during oogenesis, by analogy to antigenic peptide-presenting MHC (Marino et al., 1998). However, the peptides trapped by hsp70 have not been identified. Kawamura et al. found several factors in the acid-extract involved in allorecogniton, i.e., a non-allorecognizing glucose-enriched inhibitor of the gamete interaction and Glu/Gln-enriched peptide modulators, which function as cofactors in allorecognition of sperm receptors (Kawamura et al., 1991; Figure 2A). Khalturin and his colleague identified several genes expressed in oocytes and follicle cells that are highly polymorphic among individuals. They suggested that these highly polymorphic ZP domain proteins and sushi domain-containing protein vCRL1 may be the molecular basis for fertilization and allorecognition in C. intestinalis type B (Khalturin et al., 2005; Kürn et al., 2007a; Kürn et al., 2007b). However, their segregation and gene-knockdown analyses of vCRL1 indicated that vCRL is important for the establishment and maintenance of the blood system, not in self/nonself-recognition (Sommer et al., 2012).
FIGURE 2. Current status of fertilization and self-incompatibility system. (A) Schematic representation of artificial self/nonself-fertilization in C. intestinalis. There are several ways to achieve artificial self-fertilization. Removing the VC and treating the VC with acidic seawater or proteases relieves the self-fertilization block. In addition, the inhibition of Ca2+ influx in spermatozoa, which is controlled by the external Ca2+ concentration, is sufficient to block the self-fertilization signal. (B) Gamete proteins involved in each step of fertilization are illustrated. Ub, ubiquitin; Tast, tunicate astacin-like metalloprotease with thrombospondin-type-1 repeat.
Self-sterility in C. intestinalis was first reported by Thomas Hunt Morgan, the founder of modern genetics, more than a hundred years ago (Morgan, 1910). Murabe and Hoshi (2002) and Harada et al. (2008) repeated Morgan’s experiments to determine whether crossing between sperm and eggs from selfed F1 siblings resulted in fertile or sterile offspring (Morgan, 1942; 1942), and they confirmed Morgan’s observation that one-way cross-sterility occurs between selfed F1 siblings, and this scarcely occurs under natural conditions (Murabe and Hoshi, 2002; Harada et al., 2008). Morgan concluded that the SI system is genetically controlled and hypothesized that this explains one-way cross sterility (Morgan, 1942). Morgan assumed that the “male” SI genes are expressed in haploids, while the “female” SI genes are expressed in diploids. This is called the “haploid sperm hypothesis”. Heterozygous individuals (A/a) release A-sperm and a-sperm, either of which can fertilize a homozygous individual’s eggs (a/a-eggs or A/A-eggs). On the other hand, homozygous individuals (A/A or a/a) release A-sperm or a-sperm alone, respectively, which cannot fertilize a heterozygous individual’s eggs (A/a-eggs), because both the A-sperm receptor and a-sperm receptor reside in the VC. Based on these criteria, Harada et al. carried out fertilization experiments between selfed F1 siblings and searched for a one-way cross-sterile combination. Seventy marker genes on 14 chromosomes were examined by PCR to determine whether they were homozygous or heterozygous (Harada et al., 2008). According to these strategies, two loci (locus A on chromosome 2q and locus B on chromosome 7q) were identified as loci responsible for SI. Among the approximately 20 genes in locus A, only one gene product (fibrinogen-like protein) was identified in the VC by proteome analysis (Yamada et al., 2009), and a polymorphic gene expressed in the testis was identified as a candidate sperm-side SI factor. These gene pairs were designated v-Themis-A and s-Themis-A, and two similar gene pairs were identified in locus B and named s/v-Themis-B and s/v-Themis-B2 (Harada et al., 2008; Sawada et al., 2020). Taken together, there are three multiallelic pairs of SI candidate genes: egg-side genes (v-Themis-A, v-Themis-B and v-Themis-B2) and sperm-side genes (s-Themis-A, s-Themis-B and s-Themis-B2) (Harada et al., 2008; Yamada et al., 2009; Sawada et al., 2020). Interestingly, these are highly polymorphic genes and are tightly linked: v-Themis genes are encoded in the first intron of s-Themis genes in the opposite direction (Harada et al., 2008; Sawada et al., 2020). The sperm-side SI genes s-Themis-A, B, and B2 show homology to mammalian PKD1 or PKDREJ, which both contain a hypervariable region (HVR), receptor for egg jelly (REJ), G protein-coupled receptor proteolysis site (GPS), lipoxygenase homology 2 (LH2) domain, and 5 (in case of s-Themis-A) or 11 (in case of s-Themis-B and s-Themis-B2) pass transmembrane (TM) domain. Notably, s-Themis-B and s-Themis-B2 possess a cation channel [polycystic kidney disease (PKD) channel] domain in their C-terminal regions (Harada et al., 2008; Sawada et al., 2020). When three allelic gene pairs were matched (in the case of the same haplotypes), even nonself-fertilization was strongly blocked (Sawada et al., 2020). In addition, by gene editing experiments, s/v-Themis-A genes and s/v-Themis-B/B2 genes were found to be indispensable for self-sterility (Sawada et al., 2020). After sperm recognize the VC as self, drastic and acute Ca2+ influx occurs in spermatozoa, referred to as the SI response (Saito et al., 2012). This dramatic SI response causes sperm detachment from the VC and loss of sperm motility, because intracellular Ca2+ concentration regulates sperm behavior and flagellar beating. Although the SI response abolishes the fertility of self-recognized sperm, low-Ca2+ seawater enables self-fertilization (Hashimoto et al., submitted; Figures 2A,B). These results indicate that the increase in intracellular Ca2+ concentration triggers the SI response in spermatozoa.
Since the SI system is abolished by acid treatment of the VC, putative SI proteins might be released (Kawamura et al., 1991). Therefore, acid extracts of the VC were investigated by LC/MS, and Ci-v-Themis-like protein was identified as a new SI candidate protein (Otsuka et al., 2013). As the name indicates, Ci-v-Themis-like protein has a fibrinogen-like domain, similar to v-Themis-A, -B and -B2. However, Ci-v-Themis-like protein is not polymorphic among individuals. Although this protein is unlikely to participate in the SI system, it is probably involved in gamete binding or the assembly of v-Themis proteins on the VC (Otsuka et al., 2013).
Taken together, spermatozoa are equally attracted to self and nonself eggs and are capable of binding to the VC before recognizing the eggs as self or nonself. The interaction between sperm fucosidase and the VC fucose moiety must be maintained even after recognition as nonself, since nonself sperm binding to the VC is inhibited by fucose and fucose glycosides (Hoshi et al., 1983; Hoshi et al., 1985). Sperm fucosidase-fucose interactions may be involved in the interaction between s-Themis and v-Themis. On the other hand, the CiUrabin-CiVC57 interaction may be broken after sperm recognize the VC as self, since most self-sperm detach from the VC (Kawamura et al., 1987; Saito et al., 2012). In this process, GPI-specific phospholipase might be activated after sperm recognize the VC as self. Shortly after sperm binding to the VC, gametes undergo a judgment of self- or nonself-gametes using three allelic protein pairs of s-Themis and v-Themis, and only self-recognized sperm are rejected because self-recognition triggers the SI response (Figure 2B). s/v-Themis homologous genes were also identified in self-sterile species, such as H. roretzi and C. savignyi. However, participation of these proteins in SI is not known, since crossing experiments and genetic analysis have not been done. Since human sperm PKDREJ shows significant polymorphism (Hamm et al., 2007), it is an intriguing issue to clarify whether these variations are related to fertilization efficiency.
Sperm utilize the lytic agent “lysin”, which enables sperm to penetrate through the proteinaceous egg coat (Figure 1). In ascidians, nonself-sperm lysin must be activated after self/nonself-recognition on the VC, which allows sperm to penetrate the VC. Generally, deuterostome spermatozoa appear to utilize enzymatic lysins, since protease inhibitors can inhibit fertilization, particularly sperm penetration of the ZP, VE or VC. In mammals, sperm acrosin, an acrosomal trypsin-like protease, is thought to be a zona-lysin (Hoshi, 1985). However, acrosin was found to be a nonessential gene for sperm penetration of the ZP and fertilization in mouse, as revealed by studies of acrosin knockout (KO) mice (Baba et al., 1994). In fact, acrosomal protease(s) other than acrosin are responsible for the sperm penetration process in mice (Yamagata et al., 1998). On the other hand, it was recently reported that acrosin is essential for hamster fertilization, more precisely sperm penetration of the ZP, by studying an acrosin-KO hamster model (Hirose et al., 2020). Therefore, whether acrosin is a zona-lysin depends on the species.
Hoshi et al. explored sperm proteases functioning as lysins using Halocynthia roretzi. Trypsin inhibitor (leupeptin) and chymotrypsin inhibitor (chymostatin) blocked the fertilization of intact eggs but not of VC-free eggs. Therefore, sperm trypsin-like and chymotrypsin-like proteases appear to participate in the sperm penetration of the VC, most likely as lysins (Hoshi et al., 1981). Sawada and his colleagues purified two trypsin-like proteases, ascidian acrosin and spermosin, from H. roretzi sperm extract (Sawada et al., 1984a). The involvement of both enzymes in fertilization was confirmed by comparing the abilities of various leupeptin analogs in inhibition of fertilization with their purified enzymatic activities (Sawada et al., 1984b). However, these enzymes showed no appreciable VC-degrading activity. Then, chymotrypsin-like protease was purified using Suc-Leu-Leu-Val-Tyr-MCA, the strongest inhibitor of fertilization among the chymotrypsin substrates tested, and the purified enzyme was identified as the proteasome (Sawada et al., 2002a). The purified proteasome from sperm can degrade the main component of the VC, HrVC70, after ubiquitination. Furthermore, anti-proteasome and anti-multiubiquitin antibodies and proteasome inhibitors inhibited fertilization. Lys-234 of HrVC70 appears to be ubiquitinated during fertilization, followed by degradation by the proteasome secreted upon sperm activation (sperm reaction) (Saitoh et al., 1993; Sawada et al., 2002a; Sakai et al., 2003). These results indicate that the sperm ubiquitin–proteasome system plays a pivotal role in fertilization, functioning as a VC lysin (Sawada et al., 2002b; Sawada, 2002). Notably, spermatozoa of mammals (Sutovsky et al., 2004), quail (Sasanami et al., 2012) and sea urchins (Yokota and Sawada, 2007) also utilize the sperm proteasome as egg-coat lysin.
In C. intestinalis, the effects of leupeptin and chymostatin on fertilization were examined (Hoshi, 1985). In this species, fertilization was inhibited by chymostatin but not by leupeptin, suggesting that C. intestinalis (Phlebobranch) and H. roretzi (Stolidobranch) utilize a different lysin system. A 24-kDa chymotrypsin-like protease was purified from C. intestinalis sperm, and the purified enzyme affected the outer layer of the VC, as revealed by electron microscopy (Marino et al., 1992). Analogous to H. roretzi, the participation of the sperm proteasome in fertilization was investigated in C. intestinalis. The proteasome inhibitors MG115 and MG132 inhibited the fertilization of intact eggs but not VC-free eggs (Sawada et al., 1998). In addition, these inhibitors showed no appreciable inhibition of sperm binding to the VC of glycerinated eggs. These results suggest that the proteasome plays a key role in sperm penetration through the VC but not in sperm binding to the VC (Figure 2B). Further studies are needed to clarify the target VC protein(s) of these proteases.
To investigate whether these proteases are exposed to the plasma membrane of the sperm head, proteomic analysis of sperm surface proteins from C. intestinalis was performed (Nakazawa et al., 2015). Unexpectedly, chymotrypsin-like protease or proteasome subunits were not identified under the conditions tested. Instead, several metalloproteases, which are referred to as “Tast (tunicate astacin-like metalloprotease with thrombospondin type 1 repeat)”, were identified as the major proteases (Nakazawa et al., 2019). The involvement of metalloproteases in fertilization was tested by the metalloprotease inhibitor GM6001. GM6001 strongly inhibited the fertilization of intact eggs but not VC-free eggs, and GM6001 did not inhibit sperm binding to the VC of glycerinated eggs. Furthermore, when isolated VC was incubated with intact sperm, several VC proteins, including CiVC57, were degraded and inhibited by GM6001. Therefore, Tasts are promising candidates for VC lysin, although we cannot exclude the possibility that the 24-kDa chymotrypsin-like protease and the proteasome are also involved in sperm penetration of the VC. Generally, metalloproteases, such as matrix metalloproteases, or the proteasome are very powerful tools to degrade insoluble proteins. It is interesting to note that several egg coat-targeting proteases, such as mouse egg ovastacin (Burkart et al., 2012) and medaka hatching enzymes (Yasumasu et al., 1992), belong to astacin-like metalloproteases. Further detailed studies on the molecular mechanisms of degradation of VC proteins by sperm proteases functioning as lysins remain to be elucidated.
We summarized the current working hypothesis of the mechanisms of fertilization in C. intestinalis, particularly from the sperm binding to the VC to the sperm penetration through the VC (Figure 2B). Shortly after sperm binding to the VC, which is mediated by interactions between CiUrabin and CiVC57, self/nonself-recognition molecules may be recruited to the binding site. When three allelic pairs (haplotypes), i.e., s/v-Themis-A, s/v-Themis-B, and s/v-Themis-B2, are matched (self-recognition), drastic Ca2+ influx occurs in spermatozoa, and the self-recognized sperm detaches from the VC or quits moving on the VC. Recognizing autologous proteins rather than an enormous number of allogeneic proteins is much easier and more reasonable. Genome editing experiments clearly demonstrated that s/v-Themis-A and s/v-Themis-B/B2 are indispensable for self/nonself-recognition. In addition, s-Themis-B and -B2 were more crucial than s-Themis-A in the SI system (Sawada et al., 2020). This is not at variance with the fact that both s-Themis-B and s-Themis-B2 contain a cation channel at their C-termini that is probably involved in Ca2+ influx. Similar to the SI system in Papaveraceae, Ca2+ influx in spermatozoa may induce sperm cell apoptosis. Further studies are necessary to demonstrate the protein–protein interaction between s-Themis and v-Themis. Intracellular signal transduction after the SI response is also an intriguing issue to be investigated. In the lysin system, we revealed the importance of a novel astacin-like metalloprotease (Tast) in sperm passage of the VC. Elucidating the physiological substrates of sperm Tasts and proteasomes are also important issues that remain to be clarified.
TS and HS summarized the current investigation of fertilization in ascidian C. intestinalis. TS and HS wrote the manuscript, which was edited by HS.
This work was supported by JSPS KAKENHI Grant Number JP17K15128 (to TS) and JP17H03672, JP20K06621 (to HS), as well as by a research grant from the Fujiwara Natural History Foundation (to TS).
The authors declare that the research was conducted in the absence of any commercial or financial relationships that could be construed as a potential conflict of interest.
All claims expressed in this article are solely those of the authors and do not necessarily represent those of their affiliated organizations, or those of the publisher, the editors and the reviewers. Any product that may be evaluated in this article, or claim that may be made by its manufacturer, is not guaranteed or endorsed by the publisher.
Aagaard, J. E., Yi, X., Maccoss, M. J., and Swanson, W. J. (2006). Rapidly Evolving Zona Pellucida Domain Proteins Are a Major Component of the Vitelline Envelope of Abalone Eggs. Proc. Natl. Acad. Sci. 103, 17302–17307. doi:10.1073/pnas.0603125103
Avella, M. A., Baibakov, B., and Dean, J. (2014). A Single Domain of the ZP2 Zona Pellucida Protein Mediates Gamete Recognition in Mice and Humans. J. Cell Biol 205, 801–809. doi:10.1083/jcb.201404025
Baba, T., Azuma, S., Kashiwabara, S., and Toyoda, Y. (1994). Sperm from Mice Carrying a Targeted Mutation of the Acrosin Gene Can Penetrate the Oocyte Zona Pellucida and Effect Fertilization. J. Biol. Chem. 269, 31845–31849. doi:10.1016/s0021-9258(18)31772-1
Bork, P., and Sander, C. (1992). A Large Domain Common to Sperm Receptors (Zp2 and Zp3) and TGF-β Type III Receptor. FEBS Lett. 300, 237–240. doi:10.1016/0014-5793(92)80853-9
Brunetti, R., Gissi, C., Pennati, R., Caicci, F., Gasparini, F., and Manni, L. (2015). Morphological Evidence that the Molecularly Determined Ciona intestinalis Type A and Type B Are Different Species: Ciona Robusta and Ciona intestinalis. J. Zoolog. Syst. Evol. Res. 53, 186–193. doi:10.1111/jzs.12101
Burkart, A. D., Xiong, B., Baibakov, B., Jiménez-Movilla, M., and Dean, J. (2012). Ovastacin, a Cortical Granule Protease, Cleaves ZP2 in the Zona Pellucida to Prevent Polyspermy. J. Cell Biol 197, 37–44. doi:10.1083/jcb.201112094
De Santis, R., Jamunno, G., and Rosati, F. (1980). A Study of the Chorion and the Follicle Cells in Relation to the Sperm-Egg Interaction in the Ascidian, Ciona intestinalis. Dev. Biol. 74, 490–499. doi:10.1016/0012-1606(80)90449-2
De Santis, R., Pinto, M. R., Cotelli, F., Rosati, F., Monroy, A., and D'alessio, G. (1983). A Fucosyl Glycoprotein Component with Sperm Receptor and Sperm-Activating Activities from the Vitelline Coat of Ciona intestinalis Eggs. Exp. Cell Res. 148, 508–513. doi:10.1016/0014-4827(83)90172-6
Dehal, P., Satou, Y., Campbell, R. K., Chapman, J., Degnan, B., De Tomaso, A., et al. (2002). The Draft Genome of Ciona intestinalis : Insights into Chordate and Vertebrate Origins. Science 298, 2157–2167. doi:10.1126/science.1080049
Ellerman, D. A., Cohen, D. J., Da Ros, V. G., Morgenfeld, M. M., Busso, D., and Cuasnicú, P. S. (2006). Sperm Protein "DE" Mediates Gamete Fusion through an Evolutionarily Conserved Site of the CRISP Family. Dev. Biol. 297, 228–237. doi:10.1016/j.ydbio.2006.05.013
Endo, T., Ueno, K., Yonezawa, K., Mineta, K., Hotta, K., Satou, Y., et al. (2011). CIPRO 2.5: Ciona intestinalis Protein Database, a Unique Integrated Repository of Large-Scale Omics Data, Bioinformatic Analyses and Curated Annotation, with User Rating and Reviewing Functionality. Nucleic Acids Res. 39, D807–D814. doi:10.1093/nar/gkq1144
Fukumoto, M. (1988). Fertilization in Ascidians: Apical Processes and Gamete Fusion in Ciona intestinalis Spermatozoa. J. Cell Sci. 89, 189–196. doi:10.1242/jcs.89.2.189
Gahlay, G., Gauthier, L., Baibakov, B., Epifano, O., and Dean, J. (2010). Gamete Recognition in Mice Depends on the Cleavage Status of an Egg's Zona Pellucida Protein. Science 329, 216–219. doi:10.1126/science.1188178
Gallo, A., and Costantini, M. (2012). Glycobiology of Reproductive Processes in Marine Animals: the State of the Art. Mar. Drugs 10, 2861–2892. doi:10.3390/md10122861
Gonzalez, S. N., Sulzyk, V., Weigel Muñoz, M., and Cuasnicu, P. S. (2021). Cysteine-Rich Secretory Proteins (CRISP) Are Key Players in Mammalian Fertilization and Fertility. Front. Cell Dev. Biol. 9. doi:10.3389/fcell.2021.800351
Goudet, G., Mugnier, S., Callebaut, I., and Monget, P. (2008). Phylogenetic Analysis and Identification of Pseudogenes Reveal a Progressive Loss of Zona Pellucida Genes during Evolution of Vertebrates. Biol. Reprod. 78, 796–806. doi:10.1095/biolreprod.107.064568
Hamm, D., Mautz, B. S., Wolfner, M. F., Aquadro, C. F., and Swanson, W. J. (2007). Evidence of Amino Acid Diversity-Enhancing Selection within Humans and Among Primates at the Candidate Sperm-Receptor Gene PKDREJ. Am. J. Hum. Genet. 81, 44–52. doi:10.1086/518695
Harada, Y., Takagaki, Y., Sunagawa, M., Saito, T., Yamada, L., Taniguchi, H., et al. (2008). Mechanism of Self-Sterility in a Hermaphroditic Chordate. Science 320, 548–550. doi:10.1126/science.1152488
Hirose, M., Honda, A., Fulka, H., Tamura-Nakano, M., Matoba, S., Tomishima, T., et al. (2020). Acrosin Is Essential for Sperm Penetration through the Zona Pellucida in Hamsters. Proc. Natl. Acad. Sci. USA 117, 2513–2518. doi:10.1073/pnas.1917595117
Hoshi, M., De Santis, R., Pinto, M. R., Cotelli, F., and Rosati, F. (1985). Sperm Glycosidases as Mediators of Sperm-Egg Bindig in the Ascidians. Zoolog. Sci. 2, 65–69.
Hoshi, M., De Santis, R., Pinto, M. R., Cotelli, F., and Rosata, F. (1983). Is Sperm α-L-Fucosidase Responsible for Sperm-Egg Binding in Ciona Intestinalis. The Sperm Cell., Editor J. André (The Hauge: Martinus Nijhoff Publishers), 107–110. doi:10.1007/978-94-009-7675-7_20
Hoshi, M. (1985). “Lysins,” in Biology of Fertilization. Editors C. B. Metz, and A. Monroy (New York: Academic Press), 431–462. doi:10.1016/b978-0-12-492602-8.50020-6
Hoshi, M., Numakunai, T., and Sawada, H. (1981). Evidence for Participation of Sperm Proteinases in Fertilization of the Solitary Ascidian, Halocynthia Roretzi: Effects of Protease Inhibitors. Dev. Biol. 86, 117–121. doi:10.1016/0012-1606(81)90322-5
Hoshi, M. (1986). Sperm Glycosidase as a Plausible Mediator of Sperm Binding to the Vitelline Envelope in Ascidians. Adv. Exp. Med. Biol. 207, 251–260. doi:10.1007/978-1-4613-2255-9_15
Jovine, L., Qi, H., Williams, Z., Litscher, E., and Wassarman, P. M. (2002). The ZP Domain Is a Conserved Module for Polymerization of Extracellular Proteins. Nat. Cell Biol 4, 457–461. doi:10.1038/ncb802
Kawamura, K., Fujita, H., and Nakauchi, M. (1987). Cytological Characterization of Self Incompatibility in Gametes of the Ascidian, Ciona intestinalis. Dev. Growth Differ. 29, 627–642. doi:10.1111/j.1440-169X.1987.00627.x
Kawamura, K., Nomura, M., Kameda, T., Shimamoto, H., and Nakauchi, M. (1991). Self-Nonself Recognition Activity Extracted from Self-Sterile Eggs of the Ascidian, Ciona intestinalis. Dev. Growth Differ. 33, 139–148. doi:10.1111/j.1440-169X.1991.00139.x
Khalturin, K., Kurn, U., Pinnow, N., and Bosch, T. C. (2005). Towards a Molecular Code for Individuality in the Absence of MHC: Screening for Individually Variable Genes in the Urochordate. Dev. Comp. Immunol. 29, 759–773. doi:10.1016/j.dci.2005.01.006
Kürn, U., Sommer, F., Bosch, T. C. G., and Khalturin, K. (2007a). In the Urochordate Ciona intestinalis Zona Pellucida Domain Proteins Vary Among Individuals. Dev. Comp. Immunol. 31, 1242–1254. doi:10.1016/j.dci.2007.03.011
Kürn, U., Sommer, F., Hemmrich, G., Bosch, T. C. G., and Khalturin, K. (2007b). Allorecognition in Urochordates: Identification of a Highly Variable Complement Receptor-like Protein Expressed in Follicle Cells of Ciona. Dev. Comp. Immunol. 31, 360–371. doi:10.1016/j.dci.2006.06.008
Lambert, C. C., and Epel, D. (1979). Calcium-mediated Mitochondrial Movement in Ascidian Sperm during Fertilization. Dev. Biol. 69, 296–304. doi:10.1016/0012-1606(79)90293-8
Lambert, C. C., and Koch, R. A. (1988). Sperm Binding and Penetration during Ascidian Fertilization. Dev. Growth Differ. 30, 325–336. doi:10.1111/j.1440-169x.1988.00325.x
Marino, R., De Santis, R., Hirohashi, N., Hoshi, M., Pinto, M. R., and Usui, N. (1992). Purification and Characterization of a Vitelline Coat Lysin from Ciona Intestinalis Spermatozoa. Mol. Reprod. Dev. 32, 383–388. doi:10.1002/mrd.1080320412
Marino, R., Pinto, M. R., Cotelli, F., Lamia, C. L., and De Santis, R. (1998). The Hsp70 Protein Is Involved in the Acquisition of Gamete Self-Sterility in the Ascidian Ciona intestinalis. Development 125, 899–907. doi:10.1242/dev.125.5.899
Morgan, T. H. (1910). Cross- and Self-Fertilization in Ciona Intestinalis. Archiv für Entwicklungsmechanik der Organismen 30, 206–235. doi:10.1007/BF02263809
Morgan, T. H. (1939). The Genetic and the Physiological Problems of Self-Sterility in Ciona. III. Induced Self-Fertilization. J. Exp. Zool. 80, 19–54. doi:10.1002/jez.1400800103
Morgan, T. H. (1942). The Genetic and the Physiological Problems of Self-Sterility in Ciona. VI. Theoretical Discussion of Genetic Data. J. Exp. Zool. 95, 37–59. doi:10.1002/jez.1400950105
Morgan, T. H. (1942). The Genetic and the Physiological Problems of Self-Sterility in Ciona. V. The Genetic Problem. J. Exp. Zool. 90, 199–228. doi:10.1002/jez.1400900205
Mori, T., Kawai-Toyooka, H., Igawa, T., and Nozaki, H. (2015). Gamete Dialogs in Green Lineages. Mol. Plant 8, 1442–1454. doi:10.1016/j.molp.2015.06.008
Murabe, N., and Hoshi, M. (2002). Re-examination of Sibling Cross-Sterility in the Ascidian, Ciona intestinalis: Genetic Background of the Self-Sterility. Zoolog. Sci. 19, 527–538. doi:10.2108/zsj.19.527
Nakazawa, S., Shirae-Kurabayashi, M., Otsuka, K., and Sawada, H. (2015). Proteomics of Ionomycin-Induced Ascidian Sperm Reaction: Released and Exposed Sperm Proteins in the Ascidian Ciona intestinalis. Proteomics 15, 4064–4079. doi:10.1002/pmic.201500162
Nakazawa, S., Shirae-Kurabayashi, M., and Sawada, H. (2019). The Role of Metalloproteases in Fertilisation in the Ascidian Ciona Robusta. Sci. Rep. 9, 1009. doi:10.1038/s41598-018-37721-1
Okabe, M. (2018). Sperm-egg Interaction and Fertilization: Past, Present, and Future. Biol. Reprod. 99, 134–146. doi:10.1093/biolre/ioy028
Otsuka, K., Yamada, L., and Sawada, H. (2013). cDNA Cloning, Localization, and Candidate Binding Partners of Acid-Extractable Vitelline-Coat Protein Ci-v-themis-like in the Ascidian Ciona intestinalis. Mol. Reprod. Dev. 80, 840–848. doi:10.1002/mrd.22213
Pickett, C. J., and Zeller, R. W. (2018). Efficient Genome Editing Using CRISPR-Cas-Mediated Homology Directed Repair in the Ascidian Ciona Robusta. Genesis 56, e23260. doi:10.1002/dvg.23260
Pinto, M. R., De Santis, R., and Usui, N. (1995). Specific Induction of Self-Discrimination by Follicle Cells in Ciona intestinalis Oocytes. Dev. Growth Differ. 37, 287–291. doi:10.1046/j.1440-169X.1995.t01-2-00006.x
Raj, I., Sadat Al Hosseini, H., Dioguardi, E., Nishimura, K., Han, L., Villa, A., et al. (2017). Structural Basis of Egg Coat-Sperm Recognition at Fertilization. Cell 169, 1315–1326. doi:10.1016/j.cell.2017.05.033
Rosati, F., and De Santis, R. (1978). Studies on Fertilization in the Ascidans. Exp. Cell Res. 112, 111–119. doi:10.1016/0014-4827(78)90531-1
Rosati, F., and Santis, R. D. (1980). Role of the Surface Carbohydrates in Sperm-Egg Interaction in Ciona intestinalis. Nature 283, 762–764. doi:10.1038/283762a0
Saito, T., Shiba, K., Inaba, K., Yamada, L., and Sawada, H. (2012). Self-incompatibility Response Induced by Calcium Increase in Sperm of the Ascidian Ciona intestinalis. Proc. Natl. Acad. Sci. 109, 4158–4162. doi:10.1073/pnas.1115086109
Saitoh, Y., Sawada, H., and Yokosawa, H. (1993). High-molecular-weight Protease Complexes (Proteasomes) of Sperm of the Ascidian, Halocynthia Roretzi: Isolation, Characterization, and Physiological Roles in Fertilization. Dev. Biol. 158, 238–244. doi:10.1006/dbio.1993.1182
Sakai, N., Sawada, H., and Yokosawa, H. (2003). Extracellular Ubiquitin System Implicated in Fertilization of the Ascidian, Halocynthia Roretzi: Isolation and Characterization. Dev. Biol. 264, 299–307. doi:10.1016/j.ydbio.2003.07.016
Sasaki, H., Yoshida, K., Hozumi, A., and Sasakura, Y. (2014). CRISPR/Cas9‐mediated Gene Knockout in the Ascidian Ciona Intestinalis. Dev. Growth Differ. 56, 499–510. doi:10.1111/dgd.12149
Sasakura, Y., Yoshida, K., and Treen, N. (2017). Genome Editing of the Ascidian Ciona intestinalis with TALE Nuclease. Methods Mol. Biol. 1630, 235–245. doi:10.1007/978-1-4939-7128-2_19
Sasanami, T., Sugiura, K., Tokumoto, T., Yoshizaki, N., Dohra, H., Nishio, S., et al. (2012). Sperm Proteasome Degrades Egg Envelope Glycoprotein ZP1 during Fertilization of Japanese Quail (Coturnix japonica). Reproduction 144, 423–431. doi:10.1530/rep-12-0165
Satou, Y., Imai, K. S., and Satoh, N. (2001). Action of Morpholinos in Ciona Embryos. Genesis 30, 103–106. doi:10.1002/gene.1040
Sawada, H., Pinto, M. R., and De Santis, R. (1998). Participation of Sperm Proteasome in Fertilization of the Phlebobranch ascidian Ciona Intestinalis. Mol. Reprod. Dev. 50, 493–498. doi:10.1002/(sici)1098-2795(199808)50:4<493:aid-mrd13>3.0.co;2-3
Sawada, H., Sakai, N., Abe, Y., Tanaka, E., Takahashi, Y., Fujino, J., et al. (2002a). Extracellular Ubiquitination and Proteasome-Mediated Degradation of the Ascidian Sperm Receptor. Proc. Natl. Acad. Sci. 99, 1223–1228. doi:10.1073/pnas.032389499
Sawada, H., Takahashi, Y., Fujino, J., Flores, S. Y., and Yokosawa, H. (2002b). Localization and Roles in Fertilization of Sperm Proteasomes in the ascidianHalocynthia Roretzi. Mol. Reprod. Dev. 62, 271–276. doi:10.1002/mrd.10089
Sawada, H., Yamamoto, K., Yamaguchi, A., Yamada, L., Higuchi, A., Nukaya, H., et al. (2020). Three Multi-Allelic Gene Pairs Are Responsible for Self-Sterility in the Ascidian Ciona intestinalis. Sci. Rep. 10, 2514. doi:10.1038/s41598-020-59147-4
Sawada, H., Yokosawa, H., and Ishii, S. (1984a). Purification and Characterization of Two Types of Trypsin-like Enzymes from Sperm of the Ascidian (Prochordata) Halocynthia Roretzi. Evidence for the Presence of Spermosin, a Novel Acrosin-like Enzyme. J. Biol. Chem. 259, 2900–2904. doi:10.1016/S0021-9258(17)43233-9
Sawada, H., Yokosawa, H., and Lambert, C. C. (2001). The Biology of Ascidians. Tokyo: Springer. doi:10.1007/978-4-431-66982-1
Sawada, H., Yokosawa, H., Someno, T., Saino, T., and Ishii, S. (1984b). Evidence for the Participation of Two Sperm Proteases, Spermosin and Acrosin, in Fertilization of the Ascidian, Halocynthia Roretzi: Inhibitory Effects of Leupeptin Analogs on Enzyme Activities and Fertilization. Dev. Biol. 105, 246–249. doi:10.1016/0012-1606(84)90281-1
Shiba, K., Baba, S. A., Inoue, T., and Yoshida, M. (2008). Ca2+ Bursts Occur Around a Local Minimal Concentration of Attractant and Trigger Sperm Chemotactic Response. Proc. Natl. Acad. Sci. 105, 19312–19317. doi:10.1073/pnas.0808580105
Shoguchi, E., Kawashima, T., Satou, Y., Hamaguchi, M., Sin-I, T., Kohara, Y., et al. (2006). Chromosomal Mapping of 170 BAC Clones in the Ascidian Ciona intestinalis. Genome Res. 16, 297–303. doi:10.1101/gr.4156606
Sommer, F., Awazu, S., Anton-Erxleben, F., Jiang, D., Klimovich, A. V., Klimovich, B. V., et al. (2012). Blood System Formation in the Urochordate Ciona intestinalis Requires the Variable Receptor vCRL1. Mol. Biol. Evol. 29, 3081–3093. doi:10.1093/molbev/mss120
Stsiapanava, A., Xu, C., Brunati, M., Zamora‐Caballero, S., Schaeffer, C., Bokhove, M., et al. (2020). Cryo‐EM Structure of Native Human Uromodulin, a Zona Pellucida Module Polymer. EMBO J. 39, e106807. doi:10.15252/embj.2020106807
Sutovsky, P., Manandhar, G., Mccauley, T. C., Caamaño, J. N., Sutovsky, M., Thompson, W. E., et al. (2004). Proteasomal Interference Prevents Zona Pellucida Penetration and Fertilization in Mammals. Biol. Reprod. 71, 1625–1637. doi:10.1095/biolreprod.104.032532
Urayama, S., Harada, Y., Nakagawa, Y., Ban, S., Akasaka, M., Kawasaki, N., et al. (2008). Ascidian Sperm Glycosylphosphatidylinositol-Anchored CRISP-like Protein as a Binding Partner for an Allorecognizable Sperm Receptor on the Vitelline Coat. J. Biol. Chem. 283, 21725–21733. doi:10.1074/jbc.M802631200
Vacquier, V. D., and Swanson, W. J. (2011). Selection in the Rapid Evolution of Gamete Recognition Proteins in Marine Invertebrates. Cold Spring Harbor Perspect. Biol. 3, a002931. doi:10.1101/cshperspect.a002931
Yamada, L., Saito, T., Taniguchi, H., Sawada, H., and Harada, Y. (2009). Comprehensive Egg Coat Proteome of the Ascidian Ciona intestinalis Reveals Gamete Recognition Molecules Involved in Self-Sterility. J. Biol. Chem. 284, 9402–9410. doi:10.1074/jbc.M809672200
Yamagata, K., Murayama, K., Kohno, N., Kashiwabara, S.-i., and Baba, T. (1998). p-Aminobenzamidine-sensitive Acrosomal Protease(s) Other Than Acrosin Serve the Sperm Penetration of the Egg Zona Pellucida in Mouse. Zygote 6, 311–319. doi:10.1017/S0967199498000264
Yamaguchi, A., Saito, T., Yamada, L., Taniguchi, H., Harada, Y., and Sawada, H. (2011). Identification and Localization of the Sperm CRISP Family Protein CiUrabin Involved in Gamete Interaction in the Ascidian Ciona intestinalis. Mol. Reprod. Dev. 78, 488–497. doi:10.1002/mrd.21329
Yasumasu, S., Yamada, K., Akasaka, K., Mitsunaga, K., Iuchi, I., Shimada, H., et al. (1992). Isolation of cDNAs for LCE and HCE, Two Constituent Proteases of the Hatching Enzyme of Oryzias latipes, and Concurrent Expression of Their mRNAs during Development. Dev. Biol. 153, 250–258. doi:10.1016/0012-1606(92)90110-3
Yokota, N., and Sawada, H. (2007). Sperm Proteasomes are Responsible for the Acrosome Reaction and Sperm Penetration of the Vitelline Envelope During Fertilization of the Sea Urchin Pseudocentrotus Depressus.. Dev. Biol. 308, 222–231. doi:10.1016/j.ydbio.2007.05.025
Yoshida, K., Shiba, K., Sakamoto, A., Ikenaga, J., Matsunaga, S., Inaba, K., et al. (2018). Ca2+ Efflux via Plasma Membrane Ca2+-ATPase Mediates Chemotaxis in Ascidian Sperm. Sci. Rep. 8, 16622. doi:10.1038/s41598-018-35013-2
Yoshida, M., Ishikawa, M., Izumi, H., De Santis, R., and Morisawa, M. (2003). Store-operated Calcium Channel Regulates the Chemotactic Behavior of Ascidian Sperm. Proc. Natl. Acad. Sci. 100, 149–154. doi:10.1073/pnas.0135565100
Keywords: ascidian, sperm, egg, self-sterility, self-incompatibility, lysin, proteasome
Citation: Saito T and Sawada H (2022) Fertilization of Ascidians: Gamete Interaction, Self/Nonself Recognition and Sperm Penetration of Egg Coat. Front. Cell Dev. Biol. 9:827214. doi: 10.3389/fcell.2021.827214
Received: 01 December 2021; Accepted: 30 December 2021;
Published: 21 January 2022.
Edited by:
Enrica Bianchi, University of York, United KingdomReviewed by:
Alessandra Gallo, Stazione Zoologica Anton Dohrn Napoli, ItalyCopyright © 2022 Saito and Sawada. This is an open-access article distributed under the terms of the Creative Commons Attribution License (CC BY). The use, distribution or reproduction in other forums is permitted, provided the original author(s) and the copyright owner(s) are credited and that the original publication in this journal is cited, in accordance with accepted academic practice. No use, distribution or reproduction is permitted which does not comply with these terms.
*Correspondence: Hitoshi Sawada, c2F3YWRhQGtpbmpvLXUuYWMuanA=
Disclaimer: All claims expressed in this article are solely those of the authors and do not necessarily represent those of their affiliated organizations, or those of the publisher, the editors and the reviewers. Any product that may be evaluated in this article or claim that may be made by its manufacturer is not guaranteed or endorsed by the publisher.
Research integrity at Frontiers
Learn more about the work of our research integrity team to safeguard the quality of each article we publish.