- 1Shandong Provincial Key Laboratory of Animal Cell and Developmental Biology, School of Life Sciences, Shandong University, Qingdao, China
- 2State Key Laboratory of Cell Biology, Center for Excellence in Molecular Cell Science, Shanghai Institute of Biochemistry and Cell Biology, Chinese Academy of Sciences, Shanghai, China
- 3School of Life Sciences and Technology, ShanghaiTech University, Shanghai, China
- 4Shandong Provincial Collaborative Innovation Center of Cell Biology, Shandong Normal University, Jinan, China
Stereocilia are actin-based cell protrusions on the apical surface of inner ear hair cells, playing a pivotal role in hearing and balancing sensation. The development and maintenance of stereocilia is tightly regulated and deficits in this process usually lead to hearing or balancing disorders. The Rho GTPase cell division cycle 42 (CDC42) is a key regulator of the actin cytoskeleton. It has been reported to localize in the hair cell stereocilia and play important roles in stereocilia maintenance. In the present work, we utilized hair cell-specific Cdc42 knockout mice and CDC42 inhibitor ML141 to explore the role of CDC42 in stereocilia development. Our data show that stereocilia height and width as well as stereocilia resorption are affected in Cdc42-deficient cochlear hair cells when examined at postnatal day 8 (P8). Moreover, ML141 treatment leads to planar cell polarity (PCP) deficits in neonatal hair cells. We also show that overexpression of a constitutively active mutant CDC42 in cochlear hair cells leads to enhanced stereocilia developmental deficits. In conclusion, the present data suggest that CDC42 plays a pivotal role in regulating hair cell stereocilia development.
Introduction
As the mechanosensitive receptor cells in the inner ear, hair cells are characterized by their hairy-looking hair bundles, which consist of hundreds of actin-based stereocilia and one microtubule-based kinocilium on the apical surface of each cell (Flock and Cheung, 1977). The kinocilium is important for hair bundle development as well as planar cell polarity (PCP) establishment, while the stereocilia are essential for mechano-electrical transduction (MET), the process that converts mechanical signals into electrical signals (Lindeman et al., 1971; Hudspeth and Jacobs, 1979; Jones et al., 2008). In each hair cell, the stereocilia are organized into several rows of increasing heights, forming a staircase-like pattern (Tilney et al., 1980). Deflection of stereocilia toward the taller row direction opens the MET channels at the tips of shorter row stereocilia, eventually leading to the influx of cations into hair cells (Hudspeth and Jacobs, 1979; Beurg et al., 2009).
The actin core of stereocilia consists of a bundle of cross-linked actin filaments (F-actin), with their barbed (plus) ends pointing toward the distal tips (Flock and Cheung, 1977; Tilney et al., 1980). During development, the stereocilia start as short apical microvilli and develop into the final mature morphology by increasing the length and numbers of F-actin core (Tilney et al., 1992; Krey et al., 2020). In adults, the stereocilia are quite stable and actin polymerization/depolymerization is only detected at the distal tips (Zhang et al., 2012; Drummond et al., 2015; Narayanan et al., 2015). The development and maintenance of stereocilia is tightly regulated, and deficits in this process usually lead to hearing loss or balancing deficits (Ciuman, 2011; Barr-Gillespie, 2015). With the rapid progress of genetic, transcriptomic, and proteomic techniques, many proteins have been identified to participate in the development and/or maintenance of stereocilia (Barr-Gillespie, 2015; McGrath et al., 2017; Ellwanger et al., 2018; Krey and Barr-Gillespie, 2019; Velez-Ortega and Frolenkov, 2019).
The Rho GTPase cell division cycle 42 (CDC42) is a key regulator of the actin cytoskeleton (Sit and Manser, 2011). It has been long known that CDC42 stimulates Arp2/3-dependent actin polymerization and plays an important role in the formation of filopodia, another finger-like actin-based cell protrusion that is similar to stereocilia and microvilli (Rohatgi et al., 1999; Chen et al., 2000; Yang et al., 2006). Recently, Ueyama et al. (2014) reported that CDC42 localizes in the stereocilia of cochlear hair cells, and that Cdc42 gene disruption causes deficits in stereocilia maintenance. In the present work, we further explore the role of CDC42 in stereocilia development using Cdc42 conditional knockout mice and CDC42 inhibitor. Our present data suggest that CDC42 regulates hair cell stereocilia development both in a cell autonomous and non-autonomous manner.
Materials and Methods
Plasmids and Antibodies
Mouse cDNAs encoding wild-type or mutant CDC42 were subcloned into pEGFP-C2. Rabbit anti-CAPZB2 antibody (Cat. No. AB6017) was purchased from Merck, and its specificity has been validated previously (Avenarius et al., 2017). Rabbit anti-MYO15A antibody was described and validated previously (Zou et al., 2014). Mouse anti-EPS8 antibody (Cat. No. 610143) was purchased from BD Biosciences, and its specificity has been validated previously (Zampini et al., 2011). Other antibodies and additional reagents were as follows: mouse anti-GFP antibody (Abmart, Cat. No. M20004); Alexa Fluor 488-conjugated donkey anti-rabbit IgG (Thermo Fisher Scientific, Cat. No. A21206); Alexa Fluor 488-conjugated donkey anti-mouse IgG (Thermo Fisher Scientific, Cat. No. A21202); Alex Fluor 546-conjugated donkey anti-mouse IgG (Thermo Fisher Scientific, Cat. No. A10036); TRITC-conjugated phalloidin (Sigma-Aldrich, Cat. No. P1951); iFluor 405-conjugated phalloidin (Abcam, Cat. No. ab176752).
Mice
All animal experiments were approved by the Animal Ethics Committee of Shandong University School of Life Sciences (Permit Number: SYDWLL-2020-31) and performed accordingly. Cdc42loxP/+ mice were developed as previously reported (van Hengel et al., 2008). Atoh1Cre/ + knock-in mice that express Cre recombinase under the control of Atoh1 promoter were developed as previously reported (Yang et al., 2010).
Scanning Electron Microscopy and Stereocilia Length/Width Quantification
SEM was performed as previously described (Wang et al., 2017). Dissected mouse temporal bone was fixed with 2.5% glutaraldehyde in 0.1 M phosphate buffer overnight at 4°C. The cochleae were then taken out and post-fixed with 1% osmium tetroxide in 0.1 M phosphate buffer at 4°C for 2 h. After dehydration in ethanol and critically point drying using a Leica EM CPD300 (Leica, Germany), samples were mounted and sputter coated with platinum (15 nm) using a Cressington 108 sputter coater (Cressington, United Kingdom). Images were taken using a Quanta250 field-emission scanning electron microscope (FEI, Netherlands) with a beam strength of 5 kV.
Stereocilia length quantification were performed as previously described (Li et al., 2020). Briefly, SEM images were taken in two different imaging planes with a known angle between them, and the relative stereocilia projection length and its angles in the reconstructed three-dimensional coordinates were measured using Photoshop. The stereocilia length was then calculated using the equations mentioned in the above report. Stereocilia width quantification were performed as previously described (McGrath et al., 2021). Briefly, stereocilia with approximately perpendicular orientation in SEM images were used for calculations. A line was drawn perpendicularly across the stereocilium at approximately the same distance above the taper region of each measured stereocilium. The length of this line was then measured using Image J to give rise to the width of the stereocilium.
Whole-Mount Immunostaining
All steps were performed at room temperature unless otherwise indicated. The auditory sensory epithelia were dissected out of the temporal bone and fixed with 4% paraformaldehyde (PFA) in PBS for 20 min, followed by permeabilization and blocking with PBT1 (0.1% Triton X-100, 1% BSA, and 5% heat-inactivated goat serum in PBS, pH 7.3) for 40 min. After that, the samples were incubated with primary antibody in PBT1 at 4°C overnight, followed by sequential incubation with secondary antibody in PBT2 (0.1% Triton X-100 and 0.1% BSA in PBS) for 2 h and phalloidin in PBS for 30 min. The samples were mounted in PBS/glycerol (1:1), and images were taken using a confocal microscope with a 1.4 NA/100 × Kort M27 objective lens (LSM 700, Zeiss, Germany).
Injectoporation
Injectoporation was performed as previously described (Du et al., 2020). Briefly, the cochlear sensory epithelia were isolated from P2 mice and cultured in DMEM/F12 with 1.5 μg/ml ampicillin. Expression plasmids (0.2 μg/μl in Hanks’ balanced salt solution) were delivered to hair cells using a glass pipette of 2 μm tip diameter. A series of three pulses at 60 V lasting 15 ms at 1-s intervals were applied by an electroporator (ECM Gemini X2, BTX, CA). The tissues were cultured for 24 h in vitro and then incubated with phalloidin in PBS for 30 min. The samples were mounted in PBS/glycerol (1:1), and images were taken using a confocal microscope with a 1.4 NA/63 × Kort M27 objective lens (LSM 900, Zeiss, Germany).
Protein Purification and Localization in Permeabilized Hair Cells
Protein expression and purification was performed as previously described (Cao et al., 2013). Briefly, the coding sequence encoding wild-type or mutant CDC42 fused to EGFP was inserted into expression vector pET-28a, which was then transformed into Escherichia coli BL21(DE3) cells. His-tagged EGFP-CDC42 protein was induced in the presence of 0.4 mM isopropyl-β-d-thiogalactopyranoside (IPTG) at 16°C, then purified using Ni-NTA agarose (TransGen) according to manufacturer’s instructions.
The subcellular localization of purified protein in permeabilized hair cells was examined as described before (McGrath et al., 2021). All steps were performed at room temperature unless otherwise indicated. Briefly, the cochlear epithelia were dissected out of temporal bone in Hank’s balanced salt solution, followed by incubation with 40 μg purified EGFP-CDC42 protein in cytoskeletal buffer (20 mM HEPES, pH 7.5, 138 mM KCl, 4 mM MgCl2, 3 mM EGTA, 1% bovine serum albumin, 0.05% saponin, and 2 mM fresh ATP) for 5 min. Samples were then fixed with 4% PFA for 30 min, followed by blocking with 0.1 M PBS containing 5% (v/v) donkey serum for an hour. Afterward, samples were incubated with mouse anti-GFP antibody in 0.1 M PBS containing 5% (v/v) donkey serum for an hour, then Alex Fluor 546-conjugated donkey anti-mouse IgG in PBST buffer (0.01% Triton X-100 and 0.1% BSA in PBS) for an hour, followed by incubation with iFluor 405-conjugaetd phalloidin in PBS for 30 min. After mounting in PBS/glycerol (1:1), samples were imaged with a confocal microscope with a 1.4 NA/63× Kort M27 objective lens (LSM 900, Zeiss, Germany).
Statistical Analysis
All experiments were performed at least three times independently. The numbers of analyzed animals or cells are indicated in the figures or figure legends. Data were shown as means ± standard error of mean (SEM). Student’s two-tailed unpaired t-test was used to determine statistical significance, and P < 0.05 was considered statistically significant.
Results
Hair Cell-Specific Cdc42 Inactivation Leads to Stereocilia Development Deficits
To investigate the potential role of CDC42 in stereocilia development, we first crossed Cdc42loxp/loxp mice with Atoh1Cre/+ knock-in mice that express Cre recombinase in developing cochlear hair cells from embryonic day 14.5 (E14.5) (Yang et al., 2010). The resultant Atoh1Cre/+;Cdc42loxp/loxp mice are referred to as Cdc42 cKO mice thereafter in this work. Atoh1Cre/+;Cdc42loxp/+ and Cdc42loxp/loxp mice were used as controls. Scanning electron microscopy (SEM) was then performed to examine the morphology of hair bundles in Cdc42 cKO mice and control mice. At postnatal day 0.5 (P0.5), the morphology of hair bundle is largely indistinguishable between Cdc42 cKO and control cochlear hair cells, with multiple rows of immature stereocilia protruding from the apical surface of hair cells (Figures 1A–C). By P8, most of the short-row immature stereocilia are resorbed and only 3 or 4 rows are remained in the control OHCs and IHCs, respectively (Figures 1B,C). However, in P8 Cdc42 cKO OHCs, there are more fourth-row stereocilia remained, suggesting that immature stereocilia resorption is affected by Cdc42 inactivation (Figures 1B,E). The extra fourth-row stereocilia in the Cdc42 cKO OHCs persist when examined at P30, suggesting that it is a result of reduced stereocilia resorption instead of delayed stereocilia resorption (Figure 1B). Meanwhile, the stereocilia number in the regular rows is slightly decreased in the Cdc42 cKO OHCs (Supplementary Figures 1A–C). By P30, stereocilia fusion, fragmentation, or even complete stereocilia loss could be observed in the Cdc42 cKO OHCs and IHCs (Figures 1A,D,H).
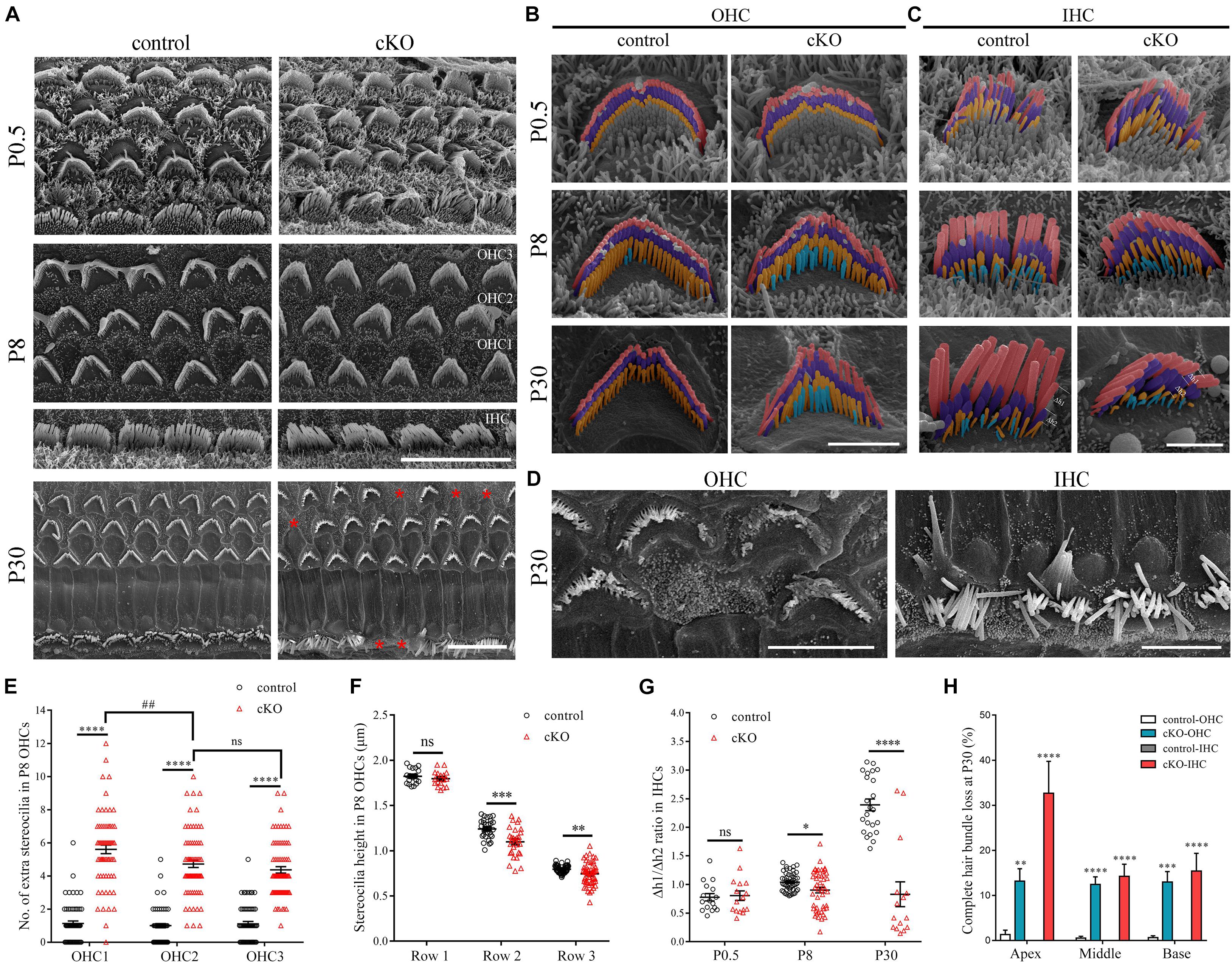
Figure 1. Hair cell-specific Cdc42 inactivation leads to stereocilia development deficits. (A) Low magnification SEM images of the hair bundles from control or cKO mice at different ages as indicated. Shown are images taken from the middle turns. Asterisks indicate complete loss of hair bundles. Scale bar: 10 μm. (B,C) High magnification pseudo-colored SEM images of hair bundles from control or cKO OHCs (B) and IHCs (C) at different ages as indicated. The first, second, and third row stereocilia are indicated by pink, purple, and yellow, respectively. The extra fourth-row stereocilia are indicated by cyan. Δh1 and Δh2 represent the relative distance from 1st row stereocilium tip to its parallel connecting 2nd row stereocilium tip and the 2nd row stereocilium tip to its parallel connecting 3rd row stereocilium tip, respectively. Shown are images taken from the middle turns. Scale bars: 2 μm. (D) High magnification SEM images of severely disorganized hair bundles from control or cKO mice at P30. Shown are images taken from the middle turns. Scale bar: 5 μm. (E) Number of the extra fourth-row stereocilia per P8 OHC was analyzed according to the results from (B). (F) Average stereocilia height in different rows of P8 OHCs were analyzed according to the results from (B). (G) The Δh1/Δh2 ratio in IHCs at different ages was analyzed according to the results from (C). (H) Percentage of P30 OHCs and IHCs with complete hair bundle loss was calculated according to the results from (A). For statistical analyses in (E–H), images were randomly taken from at least three animals for each group. The bars indicate mean ± SEM values. ∗P < 0.05; ∗∗P < 0.01; ∗∗∗P < 0.001; ****P < 0.0001; ##P < 0.01; ns, no significant difference.
The stereocilia height is also affected by Cdc42 inactivation. By P8, there is significant variability of stereocilia height in the second and third rows in Cdc42 cKO OHCs and IHCs, which becomes more apparent at P30 (Figures 1B,C,F). Moreover, in the Cdc42 cKO OHCs, the average height of second- and third-row stereocilia is slightly decreased compared with control OHCs (Figures 1B,F). The height of IHC stereocilia is difficult to measure because of the imaging angle in most SEM images (Figure 1C). Nevertheless, the ratio of height difference between row 1 and row 2 stereocilia to that between row 2 and row 3 stereocilia is significantly decreased in the Cdc42 cKO IHCs especially at P30 (Figures 1C,G).
Albeit the height of the tallest row stereocilia is largely unaffected in the Cdc42 cKO hair cells, it seems that their width is decreased compared to control mice (Figures 1B,C). To examine the width of the tallest stereocilia more precisely, we took SEM images from the side of the tallest row stereocilia and quantified the stereocilia width. The results show that the width of the tallest-row stereocilia of Cdc42 cKO OHCs is significantly decreased from P8 (Figures 2A,C). Similarly, the width of the tallest-row stereocilia of Cdc42 cKO IHCs is significantly decreased from P0.5 (Figures 2B,D). Taken together, our present data suggest that CDC42 plays an important role in stereocilia development.
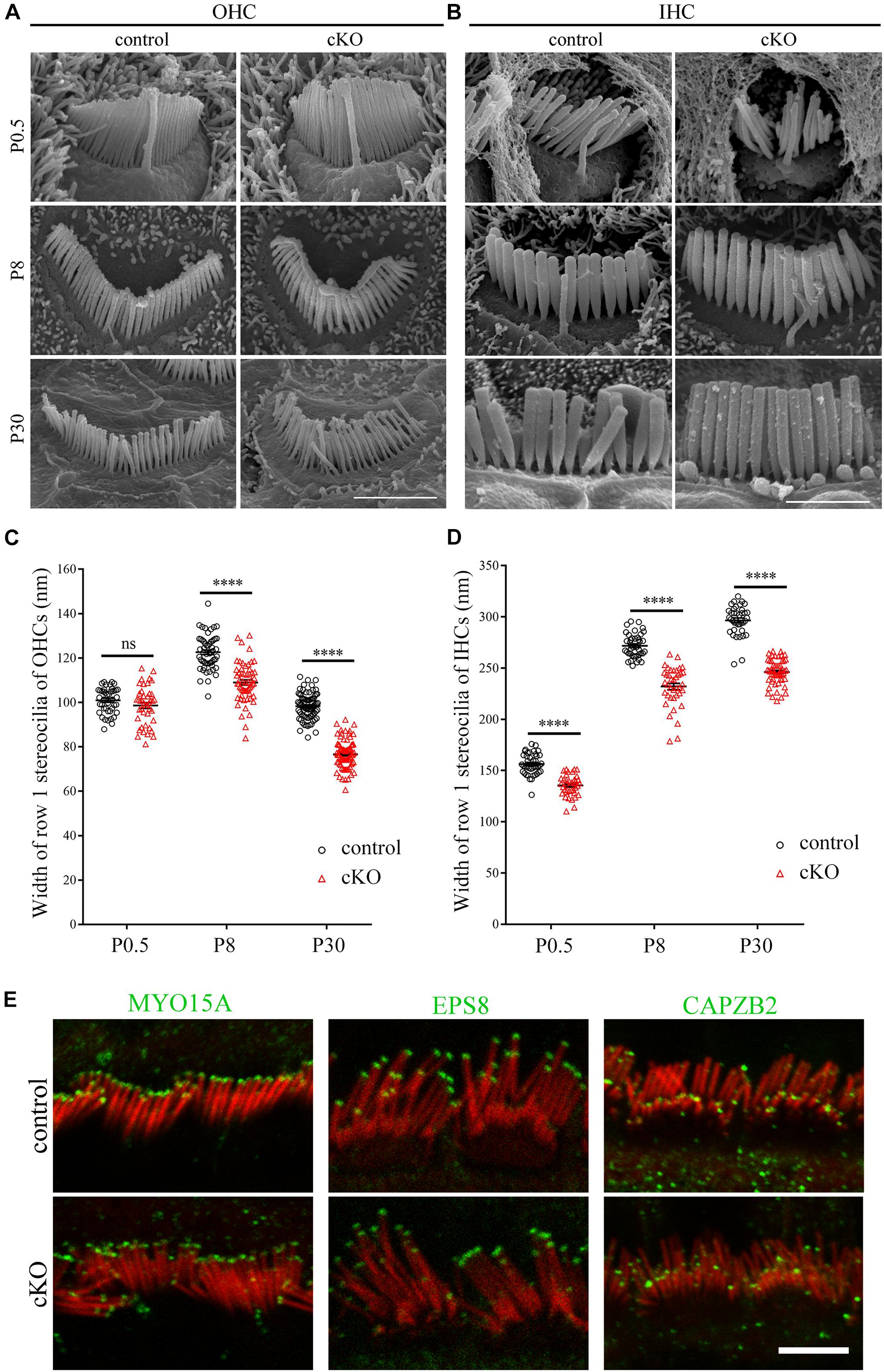
Figure 2. Hair cell-specific Cdc42 inactivation leads to decreased stereocilia width. (A,B) High magnification SEM images of hair bundles (dorsal view) from OHCs (A) and IHCs (B) at different ages as indicated. Shown are images taken from the middle turns. Scale bars: 2 μm. (C,D) Width of the tallest-row stereocilia in OHCs (C) and IHCs (D) at different ages as indicated were analyzed according to the results from (A,B), respectively. For statistical analyses in (C,D), images were randomly taken from at least 3 animals for each group. The bars indicate mean ± SEM values. ****P < 0.0001; ns, no significant difference. (E) Whole-mount immunostaining showing localization of MYO15A, EPS8 and CAPZB2 in IHCs of P9 control or cKO mice. TRITC-phalloidin (red) was used to visualize the stereociliary F-actin core. All images were taken from the apical turns of cochlea using a confocal microscope. Scale bar: 5 μm.
Stereociliary Tip Localization of Row 1 and 2 Complex Components Is Not Affected in the Cdc42 cKO Hair Cells
It has been known that stereocilia height is tightly regulated by the so-called row 1 and row 2 complex, which are localized at the tips of the highest-row stereocilia or shorter-row stereocilia, respectively (Krey et al., 2020; McGrath and Perrin, 2020). We then performed whole-mount immunostaining to examine whether Cdc42 inactivation affects the stereociliary tip localization of known row 1 components MYO15A and EPS8 as well as row 2 component CAPZB2 (Belyantseva et al., 2003; Manor et al., 2011; Zampini et al., 2011; Avenarius et al., 2017). The results show that stereociliary tip localization of these proteins is not affected in the Cdc42 cKO IHCs (Figure 2E) and OHCs (Supplementary Figure 2). Therefore, it seems that although Cdc42 inactivation leads to stereocilia development deficits, it does not affect the general stereocilia row identity.
CDC42 Inhibitor ML141 Treatment Leads to Stereocilia Development Deficits
We next examined the role of CDC42 in stereocilia development using a known CDC42 inhibitor ML141 (Surviladze et al., 2010). The cochlear sensory epithelia from P0.5 wild-type mice were cultured in presence of ML141 at 0, 1, 2.5, or 5 μM for 96 h, followed by fixation and SEM imaging (Figure 3A). The results show that 1 μM ML141 treatment leads to significant stereocilia disorganization, which is exaggerated at higher concentration of ML141 (Figures 3B–E). Noticeably, the staircase-like pattern of hair bundle is compromised, and more rows of stereocilia are observed in the ML141-treated hair cells (Figures 3B–E). Moreover, the PCP of hair cells is also significantly altered. We classified hair cells into three groups according to their PCP phenotypes, namely type I (roughly normal PCP), type II (circle-shaped stereocilia), and type III (reversed PCP) (Figure 3F). In the untreated controls, almost all hair cells are type I and only a small fraction of hair cells are type II (Figure 3G). However, in the presence of ML141, most hair cells show altered PCP in a dosage-dependent manner (Figure 3G). Taken together, our data show that stereocilia development is significantly affected by CDC42 inactivation either genetically or chemically.
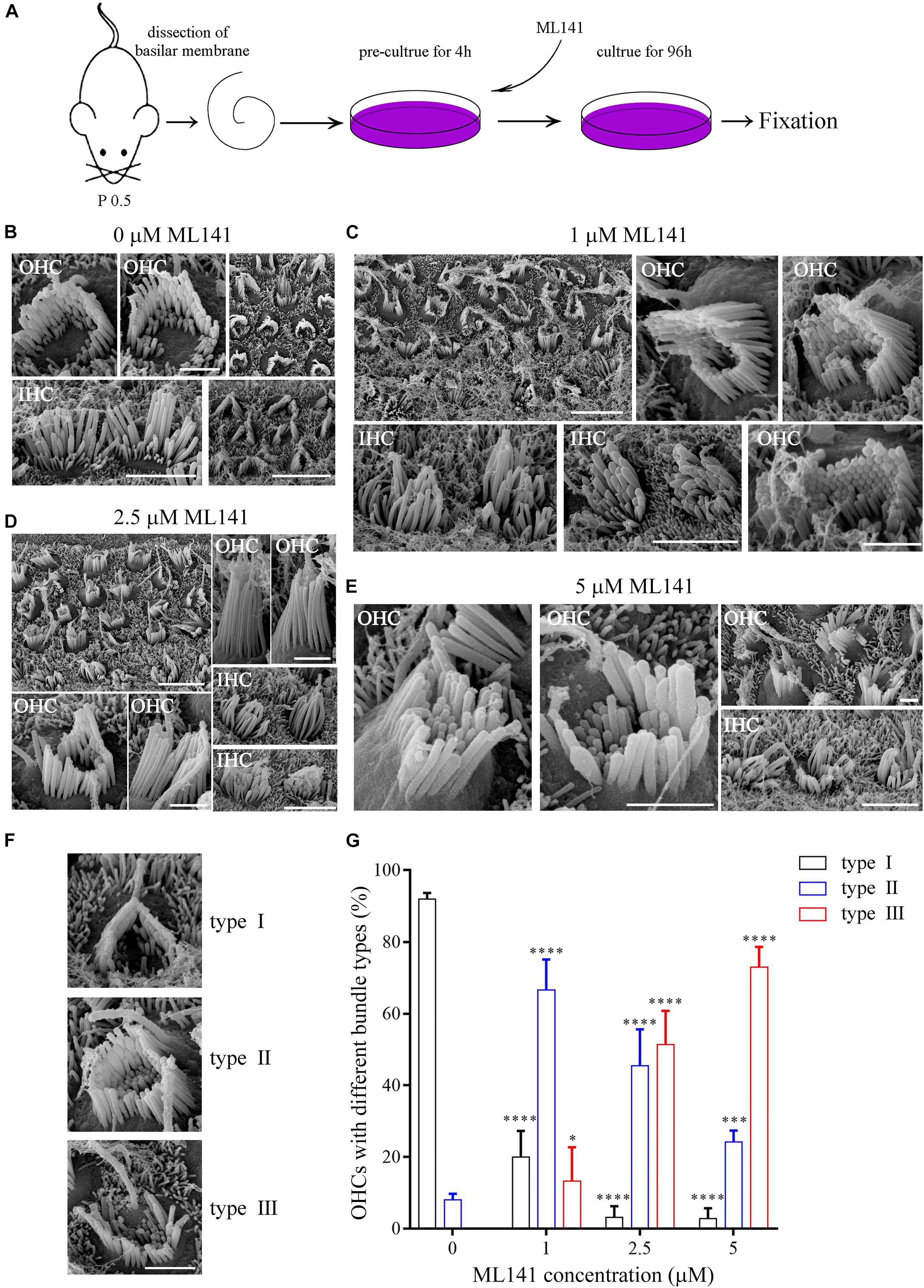
Figure 3. CDC42 inhibitor ML141 treatment leads to stereocilia development deficits. (A) Schematic drawing of the strategy of ML141 treatment and SEM imaging. (B–E) SEM images of hair bundles after treatment of ML141 at different concentrations as indicated. Scale bars: 5 μm in low magnification images; 1 μm in high magnification OHC images; 3 μm in high magnification IHC images. (F) Representative images showing three types of hair bundles with different PCP phenotypes. Scale bar: 1 μm. (G) Percentages of hair cells of different types were analyzed from three independent experiments. The bars indicate the mean ± SEM values. ∗P < 0.05; ∗∗∗P < 0.001; ****P < 0.0001.
Constitutively Active Mutant CDC42 Leads to Enhanced Stereocilia Deficits
As a small Rho GTPase, CDC42 is reversibly cycling between an active (GTP-bound) and inactive (GDP-bound) state (Hart et al., 1991). We next wanted to examine the effect of CDC42 in different state on stereocilia. We made use of two point mutations that mimic CDC42 in different state, namely inactive CDC42 mutant (T17N) and constitutively active CDC42 mutant (Q61L) (Kozma et al., 1995). We first examined whether exogenous CDC42 mutants could target to the stereocilia following permeabilization of unfixed cochlear sensory epithelia with 0.05% saponin (McGrath et al., 2021). The results show that wild-type CDC42 and two CDC42 mutants all localize in the stereocilia of P0.5 mice, with enrichment at the stereociliary tips (Figure 4A). However, all three forms of CDC42 are mainly detected in the cell body of saponin-permeabilized P6 cochlear hair cells, suggesting that CDC42 may show dynamic subcellular localization in the hair cells during development (Supplementary Figures 3A–C).
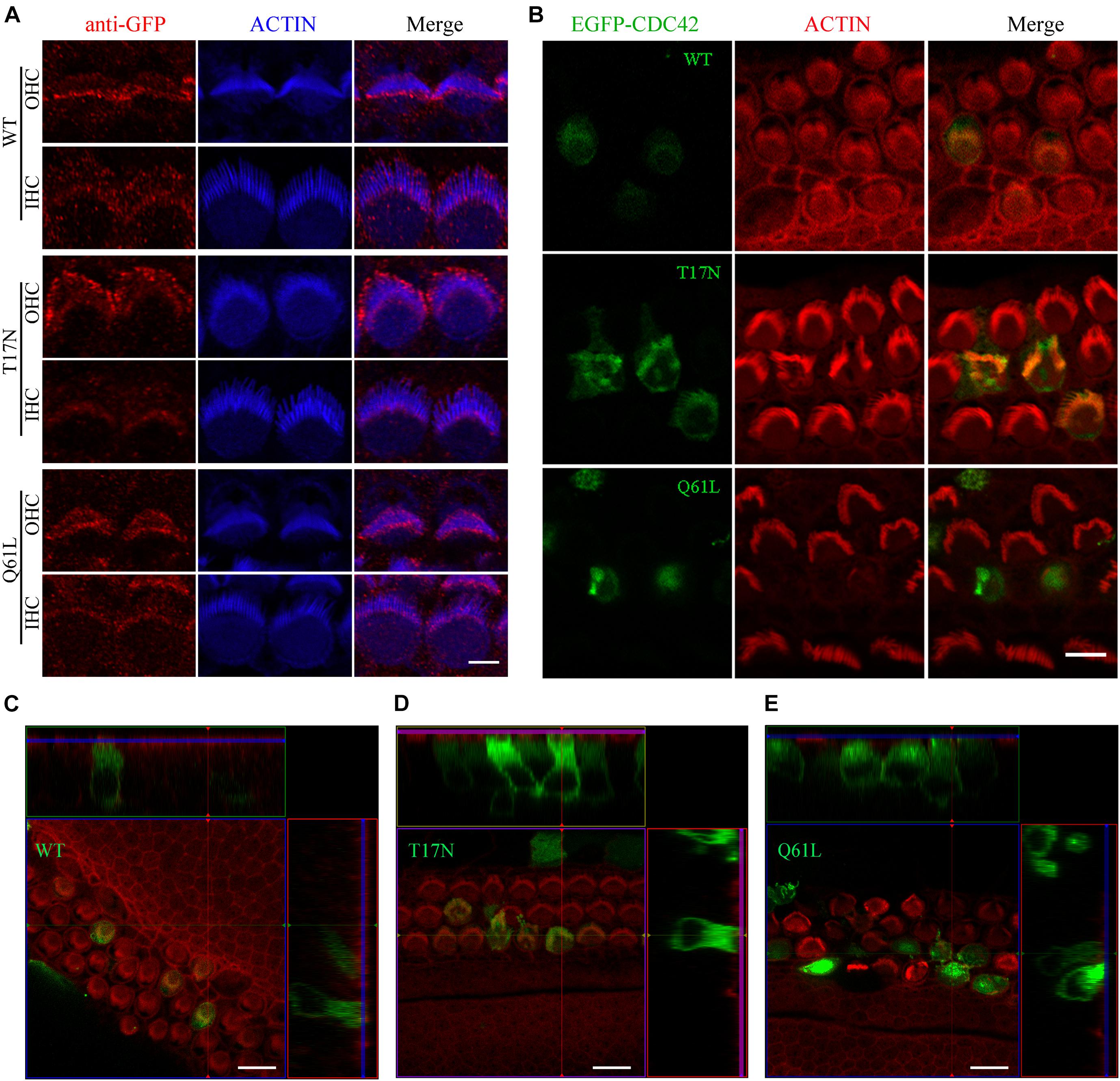
Figure 4. Constitutively active CDC42 mutant leads to enhanced stereocilia deficits. (A) Whole-mount staining showing localization of purified exogenous wild-type or mutant CDC42 proteins in saponin-permeabilized P0.5 OHCs and IHCs. iFluor 405-conjugated phalloidin was used to visualize stereociliary F-actin core. GFP antibody was used to amplify the signals of EGFP fused with CDC42 proteins. Scale bar: 2 μm. (B) Cochlear explants from P2 wild-type mice were injectoporated with expression vectors to express EGFP-tagged wild-type or mutant CDC42 in hair cells. TRITC-conjugated phalloidin was used to visualize stereociliary F-actin core. Scale bar: 5 μm. (C–E) 3D reconstruction showing the side view of hair cells injectoporated with different CDC42 constructs as indicated. Scale bar: 10 μm.
We then examined the effect of different CDC42 mutants on stereocilia development by employing the injectoporation assay and confocal microscopy. CDC42-expression vectors were injectoporated into P2 wild-type cochlear sensory epithelia, followed by in vitro culture for 24 h and confocal imaging. Stereociliary F-actin core was visualized using phalloidin. The results show that wild-type and CDC42 T17N mutant localize in the stereocilia as well as cell body of injectoporated hair cells, with largely normal stereocilia morphology (Figures 4B–D). In contrast, CDC42 Q61L mutant injectoporation results in complete stereocilia loss in most hair cells (21 out of 24 analyzed cells), suggesting that this constitutively active mutant CDC42 leads to enhanced stereocilia deficits (Figures 4B,E).
Discussion
It was recently reported that the Rho GTPase CDC42 is localized in the stereocilia of cochlear hair cells, and that cochlear hair cell stereocilia of Atoh1-Cre; Cdc42flox/flox mice develop normally but progressively degenerate after maturation, suggesting that CDC42 plays an important role in stereocilia maintenance but not development (Ueyama et al., 2014). In the present work, however, we show that CDC42 is indispensable for normal stereocilia development in cochlear hair cells. One possible reason for this discrepancy might be the different Atoh1-Cre mouse lines used in these two works. Ueyama and colleagues used transgenic Atoh1-Cre mice (Matei et al., 2005), whereas we used Atoh1-Cre knock-in mice (Yang et al., 2010). Therefore, different Cre recombinase activity in these two mouse lines might account for the slightly different stereocilia phenotypes. Another possibility is that the subtle stereocilia development deficits revealed in the present work might have simply been overlooked in the earlier study.
Our SEM results reveal that the morphology of cochlear hair cell stereocilia is largely indistinguishable between P0.5 Cdc42 cKO mice and control mice. Stereocilia deficits are apparent in P8 Cdc42 cKO cochlear hair cells, which include increased stereocilia height variation and decreased stereocilia width. Moreover, the height difference between rows is significantly altered in IHCs, and extra shortest row stereocilia exist in OHCs of Cdc42 cKO mice. Despite of the stereocilia deficits, the stereocilia row identity largely remains normal, given that the stereociliary tip localization of row 1 complex components MYO15A and EPS8 as well as row 2 complex component CAPZB2 is unchanged at this age. Moreover, CDC42 inhibitor ML141 causes similar stereocilia development deficits plus PCP phenotypes, further supporting an important role of CDC42 in stereocilia development.
Stereocilia development is a multi-step, tightly controlled process (Tilney et al., 1992; Kaltenbach et al., 1994). In immature cochlear hair cells, there are multiple rows of elongated stereocilia on the apical surface, among which the shorter rows are eventually resorbed, leaving 3–4 rows of stereocilia grow to their final height in the mature mammalian cochlear hair cells (Kaltenbach et al., 1994). The underlying mechanism of shorter row stereocilia resorption largely remains unknown. Our present data suggest that CDC42 might play a role in this process. The extra fourth-row stereocilia in Cdc42 cKO OHCs is possibly a result of reduced stereocilia resorption, which might be explained by altered actin polymerization and stabilization of stereocilia due to Cdc42 inactivation. Similar reason might also account for the observed deficits in stereocilia length and width of Cdc42 cKO cochlear hair cells.
Noticeably, our present data suggest that appropriate CDC42 activity is important for stereocilia development. It has been reported that in cultured cells, overexpression of constitutively active CDC42 mutant (Q61L) promotes the formation of filopodia (Kozma et al., 1995). In line with this, gain of CDC42 activity by disrupting ARHGAP1 (previously known as CDC42GAP) also promotes filopodia formation (Yang et al., 2006). However, our present data show that overexpression of CDC42 Q61L mutant in cochlear hair cells leads to complete loss of stereocilia. This is in sharp contrast to the reports in filopodia formation. It is possible that stereocilia development and/or maintenance is regulated so tightly that imbalance of this process would inevitably cause stereocilia deficits. Similar scenario is also observed in DIA1, whose dysfunction is associated with autosomal dominant sensorineural hearing loss DFNA1 (Lynch et al., 1997). Constitutive activation of DIA1 causes stereocilia disorganization as well as hearing loss in mice (Ueyama et al., 2016; Ninoyu et al., 2020).
Our present data show that treatment with CDC42 inhibitor results in more severe stereocilia phenotypes than hair cell-specific Cdc42 inactivation. Noticeably, apparent PCP deficits are observed in cochlear culture treated with CDC42 inhibitor, but not in the hair cell-specific Cdc42 cKO mice, suggesting that other cells such as supporting cells are involved in CDC42-mediated PCP regulation. This is consistent with previous reports that dysregulated PCP is observed in mice deleting Cdc42 in both hair cells and supporting cells, but not in mice deleting Cdc42 only in hair cells (Ueyama et al., 2014; Kirjavainen et al., 2015). In line with this, in situ hybridization reveals that Cdc42 transcripts are expressed ubiquitously in the cochlea (Anttonen et al., 2012). The ubiquitous expression of Cdc42 in the inner ear is also supported by RNAseq results of embryonic and adult mice (Cai et al., 2015; Scheffer et al., 2015; Liu et al., 2018). Taken together, the present data suggest that CDC42 regulates stereocilia development both in a cell autonomous and non-autonomous manner.
Loss of another Rho GTPase RAC1 has also been shown to cause stereocilia development deficits, such as stereocilia flattening, fragmentation, as well as altered PCP (Grimsley-Myers et al., 2009). Different stereocilia phenotypes observed in Cdc42 and Rac1 knockout mice suggest that these two Rho GTPases play important and non-complementary roles in stereocilia development. Guanine nucleotide exchange factor (GEF) ARHGEF6 specifically activates CDC42 and RAC1, and loss of ARHGEF6 causes stereocilia deficits (Zhu et al., 2018). Moreover, p21-activated kinase 1 (PAK1), a downstream effector of CDC42 and RAC1, is required for stereocilia development (Cheng et al., 2021). These studies highlight the essential role of Rho GTPases in stereocilia development. At present, the precise localization of these proteins in the stereocilia remains unknown, which prevents us from fully understanding the underlying mechanism. New imaging methods such as super-resolution microscopy definitely will help to address this question (Liu et al., 2019; Qi et al., 2019, 2020).
Data Availability Statement
The original contributions presented in the study are included in the article/Supplementary Material, further inquiries can be directed to the corresponding author/s.
Ethics Statement
The animal study was reviewed and approved by the Animal Ethics Committee of Shandong University School of Life Sciences (Permit Number: SYDWLL-2020-31).
Author Contributions
ZX: study concept and design. HD, HZ, YS, and XZ: acquisition of data. HD, ZC, YW, and ZX: analysis and interpretation of data. YW and ZX: supervision. HD, YW, and ZX: drafting the manuscript. All authors contributed to the article and approved the submitted version.
Funding
This work was supported by grants from the National Key Basic Research Program of China (2018YFC1003600), the National Natural Science Foundation of China (82071051 and 81771001), and the Shandong Provincial Natural Science Foundation (ZR2020ZD39 and ZR2020QC098).
Conflict of Interest
The authors declare that the research was conducted in the absence of any commercial or financial relationships that could be construed as a potential conflict of interest.
Publisher’s Note
All claims expressed in this article are solely those of the authors and do not necessarily represent those of their affiliated organizations, or those of the publisher, the editors and the reviewers. Any product that may be evaluated in this article, or claim that may be made by its manufacturer, is not guaranteed or endorsed by the publisher.
Acknowledgments
We thank Sen Wang, Xiaomin Zhao, and Haiyan Yu from the core facilities for life and environmental sciences, Shandong University for the technical support in SEM and confocal microscopy.
Supplementary Material
The Supplementary Material for this article can be found online at: https://www.frontiersin.org/articles/10.3389/fcell.2021.765559/full#supplementary-material
References
Anttonen, T., Kirjavainen, A., Belevich, I., Laos, M., Richardson, W. D., Jokitalo, E., et al. (2012). Cdc42-dependent structural development of auditory supporting cells is required for wound healing at adulthood. Sci. Rep. 2:978. doi: 10.1038/srep00978
Avenarius, M. R., Krey, J. F., Dumont, R. A., Morgan, C. P., Benson, C. B., Vijayakumar, S., et al. (2017). Heterodimeric capping protein is required for stereocilia length and width regulation. J. Cell Biol. 216, 3861–3881. doi: 10.1083/jcb.201704171
Barr-Gillespie, P. G. (2015). Assembly of hair bundles, an amazing problem for cell biology. Mol. Biol. Cell 26, 2727–2732. doi: 10.1091/mbc.E14-04-0940
Belyantseva, I. A., Boger, E. T., and Friedman, T. B. (2003). Myosin XVa localizes to the tips of inner ear sensory cell stereocilia and is essential for staircase formation of the hair bundle. Proc. Natl. Acad. Sci. U.S.A. 100, 13958–13963. doi: 10.1073/pnas.2334417100
Beurg, M., Fettiplace, R., Nam, J. H., and Ricci, A. J. (2009). Localization of inner hair cell mechanotransducer channels using high-speed calcium imaging. Nat. Neurosci. 12, 553–558. doi: 10.1038/nn.2295
Cai, T., Jen, H. I., Kang, H., Klisch, T. J., Zoghbi, H. Y., and Groves, A. K. (2015). Characterization of the transcriptome of nascent hair cells and identification of direct targets of the Atoh1 transcription factor. J. Neurosci. 35, 5870–5883. doi: 10.1523/JNEUROSCI.5083-14.2015
Cao, H., Yin, X., Cao, Y., Jin, Y., Wang, S., Kong, Y., et al. (2013). FCHSD1 and FCHSD2 are expressed in hair cell stereocilia and cuticular plate and regulate actin polymerization in vitro. PLoS One 8:e56516. doi: 10.1371/journal.pone.0056516
Chen, F., Ma, L., Parrini, M. C., Mao, X., Lopez, M., Wu, C., et al. (2000). Cdc42 is required for PIP(2)-induced actin polymerization and early development but not for cell viability. Curr. Biol. 10, 758–765. doi: 10.1016/s0960-9822(00)00571-6
Cheng, C., Hou, Y., Zhang, Z., Wang, Y., Lu, L., Zhang, L., et al. (2021). Disruption of the autism-related gene Pak1 causes stereocilia disorganization, hair cell loss, and deafness in mice. J. Genet. Genomics 48, 324–332. doi: 10.1016/j.jgg.2021.03.010
Ciuman, R. R. (2011). Auditory and vestibular hair cell stereocilia: relationship between functionality and inner ear disease. J. Laryngol. Otol. 125, 991–1003. doi: 10.1017/S0022215111001459
Drummond, M. C., Barzik, M., Bird, J. E., Zhang, D. S., Lechene, C. P., Corey, D. P., et al. (2015). Live-cell imaging of actin dynamics reveals mechanisms of stereocilia length regulation in the inner ear. Nat. Commun. 6:6873. doi: 10.1038/ncomms7873
Du, H., Zou, L., Ren, R., Li, N., Li, J., Wang, Y., et al. (2020). Lack of PDZD7 long isoform disrupts ankle-link complex and causes hearing loss in mice. FASEB J. 34, 1136–1149. doi: 10.1096/fj.201901657RR
Ellwanger, D. C., Scheibinger, M., Dumont, R. A., Barr-Gillespie, P. G., and Heller, S. (2018). Transcriptional dynamics of hair-bundle morphogenesis revealed with Cell Trails. Cell Rep. 23, 2901–2914. doi: 10.1016/j.celrep.2018.05.002
Flock, A., and Cheung, H. C. (1977). Actin filaments in sensory hairs of inner ear receptor cells. J. Cell Biol. 75(2 Pt 1), 339–343.
Grimsley-Myers, C. M., Sipe, C. W., Geleoc, G. S., and Lu, X. (2009). The small GTPase Rac1 regulates auditory hair cell morphogenesis. J. Neurosci. 29, 15859–15869. doi: 10.1523/JNEUROSCI.3998-09.2009
Hart, M. J., Eva, A., Evans, T., Aaronson, S. A., and Cerione, R. A. (1991). Catalysis of guanine-nucleotide exchange on the Cdc42hs protein by the Dbl oncogene product. Nature 354, 311–314. doi: 10.1038/354311a0
Hudspeth, A. J., and Jacobs, R. (1979). Stereocilia mediate transduction in vertebrate hair cells. Proc. Natl. Acad. Sci. U.S.A. 76, 1506–1509.
Jones, C., Roper, V. C., Foucher, I., Qian, D., Banizs, B., Petit, C., et al. (2008). Ciliary proteins link basal body polarization to planar cell polarity regulation. Nat. Genet. 40, 69–77. doi: 10.1038/ng.2007.54
Kaltenbach, J. A., Falzarano, P. R., and Simpson, T. H. (1994). Postnatal development of the hamster cochlea. II. growth and differentiation of stereocilia bundles. J. Comp. Neurol. 350, 187–198. doi: 10.1002/cne.903500204
Kirjavainen, A., Laos, M., Anttonen, T., and Pirvola, U. (2015). The Rho GTPase Cdc42 regulates hair cell planar polarity and cellular patterning in the developing cochlea. Biol. Open 4, 516–526. doi: 10.1242/bio.20149753
Kozma, R., Ahmed, S., Best, A., and Lim, L. (1995). The Ras-related protein Cdc42hs and Bradykinin promote formation of peripheral actin microspikes and filopodia in Swiss 3T3 fibroblasts. Mol. Cell. Biol. 15, 1942–1952. doi: 10.1128/MCB.15.4.1942
Krey, J. F., and Barr-Gillespie, P. G. (2019). Molecular composition of vestibular hair bundles. Cold Spring Harb. Perspect. Med. 9:a033209. doi: 10.1101/cshperspect.a033209
Krey, J. F., Chatterjee, P., Dumont, R. A., O’Sullivan, M., Choi, D., Bird, J. E., et al. (2020). Mechanotransduction-dependent control of stereocilia dimensions and row identity in inner hair cells. Curr. Biol. 30, 442–454. doi: 10.1016/j.cub.2019.11.076
Li, S. H., Mecca, A., Kim, J., Caprara, G. A., Wagner, E. L., Du, T. T., et al. (2020). Myosin-VIIa is expressed in multiple isoforms and essential for tensioning the hair cell mechanotransduction complex. Nat. Commun. 11:2066. doi: 10.1038/s41467-020-15936-z
Lindeman, H. H., Ades, H. W., Bredberg, G., and Engstrom, H. (1971). The sensory hairs and the tectorial membrane in the development of the cat’s organ of Corti. A scanning electron microscopic study. Acta Otolaryngol. 72, 229–242.
Liu, H. Z., Chen, L., Giffen, K. P., Stringham, S. T., Li, Y., Judge, P. D., et al. (2018). Cell-specific transcriptome analysis shows that adult Pillar and Deiters’ cells express genes encoding machinery for specializations of cochlear hair cells. Front. Mol. Neurosci. 11:356. doi: 10.3389/fnmol.2018.00356
Liu, Y., Qi, J. Y., Chen, X., Tang, M. L., Chu, C. F., Zhu, W. J., et al. (2019). Critical role of spectrin in hearing development and deafness. Sci. Adv. 5:eaav7803. doi: 10.1126/sciadv.aav7803
Lynch, E. D., Lee, M. K., Morrow, J. E., Welcsh, P. L., Leon, P. E., and King, M. C. (1997). Nonsyndromic deafness DFNA1 associated with mutation of a human homolog of the Drosophila gene diaphanous. Science 278, 1315–1318.
Manor, U., Disanza, A., Grati, M., Andrade, L., Lin, H., Di Fiore, P. P., et al. (2011). Regulation of stereocilia length by myosin XVa and whirlin depends on the actin-regulatory protein Eps8. Curr. Biol. 21, 167–172. doi: 10.1016/j.cub.2010.12.046
Matei, V., Pauley, S., Kaing, S., Rowitch, D., Beisel, K. W., Morris, K., et al. (2005). Smaller inner ear sensory epithelia in Neurog 1 null mice are related to earlier hair cell cycle exit. Dev. Dyn. 234, 633–650. doi: 10.1002/dvdy.20551
McGrath, J., and Perrin, B. J. (2020). Cell biology: function guides form of auditory sensory cells. Curr. Biol. 30, R128–R130. doi: 10.1016/j.cub.2019.12.039
McGrath, J., Roy, P., and Perrin, B. J. (2017). Stereocilia morphogenesis and maintenance through regulation of actin stability. Semin. Cell Dev. Biol. 65, 88–95. doi: 10.1016/j.semcdb.2016.08.017
McGrath, J., Tung, C. Y., Liao, X., Belyantseva, I. A., Roy, P., Chakraborty, O., et al. (2021). Actin at stereocilia tips is regulated by mechanotransduction and ADF/cofilin. Curr. Biol. 31, 1141–1153. doi: 10.1016/j.cub.2020.12.006
Narayanan, P., Chatterton, P., Ikeda, A., Ikeda, S., Corey, D. P., Ervasti, J. M., et al. (2015). Length regulation of mechanosensitive stereocilia depends on very slow actin dynamics and filament-severing proteins. Nat. Commun. 6:6855. doi: 10.1038/Ncomms7855
Ninoyu, Y., Sakaguchi, H., Lin, C., Suzuki, T., Hirano, S., Hisa, Y., et al. (2020). The integrity of cochlear hair cells is established and maintained through the localization of Dia1 at apical junctional complexes and stereocilia. Cell Death Dis. 11:536. doi: 10.1038/s41419-020-02743-z
Qi, J. Y., Liu, Y., Chu, C. F., Chen, X., Zhu, W. J., Shu, Y. L., et al. (2019). A cytoskeleton structure revealed by super-resolution fluorescence imaging in inner ear hair cells. Cell Discov. 5:12. doi: 10.1038/s41421-018-0076-4
Qi, J. Y., Zhang, L. Y., Tan, F. Z., Liu, Y., Chu, C. F., Zhu, W. J., et al. (2020). Espin distribution as revealed by super-resolution microscopy of stereocilia. Am. J. Transl. Res. 12, 130–141.
Rohatgi, R., Ma, L., Miki, H., Lopez, M., Kirchhausen, T., Takenawa, T., et al. (1999). The interaction between N-WASP and the Arp2/3 complex links Cdc42-dependent signals to actin assembly. Cell 97, 221–231. doi: 10.1016/s0092-8674(00)80732-1
Scheffer, D. I., Shen, J., Corey, D. P., and Chen, Z. Y. (2015). Gene expression by mouse inner ear hair cells during development. J. Neurosci. 35, 6366–6380. doi: 10.1523/JNEUROSCI.5126-14.2015
Sit, S. T., and Manser, E. (2011). Rho GTPases and their role in organizing the actin cytoskeleton. J. Cell Sci. 124, 679–683. doi: 10.1242/jcs.064964
Surviladze, Z., Waller, A., Strouse, J. J., Bologa, C., Ursu, O., Salas, V., et al. (2010). “A potent and selective inhibitor of Cdc42 GTPase,” in Probe Reports from the NIH Molecular Libraries Program, (Bethesda, MD: National Center for Biotechnology Information).
Tilney, L. G., Derosier, D. J., and Mulroy, M. J. (1980). The organization of actin filaments in the stereocilia of cochlear hair cells. J. Cell Biol. 86, 244–259.
Tilney, L. G., Tilney, M. S., and DeRosier, D. J. (1992). Actin filaments, stereocilia, and hair cells: how cells count and measure. Annu. Rev. Cell Biol. 8, 257–274. doi: 10.1146/annurev.cb.08.110192.001353
Ueyama, T., Ninoyu, Y., Nishio, S. Y., Miyoshi, T., Torii, H., Nishimura, K., et al. (2016). Constitutive activation of DIA1 (DIAPH1) via C-terminal truncation causes human sensorineural hearing loss. EMBO Mol. Med. 8, 1310–1324. doi: 10.15252/emmm.201606609
Ueyama, T., Sakaguchi, H., Nakamura, T., Goto, A., Morioka, S., Shimizu, A., et al. (2014). Maintenance of stereocilia and apical junctional complexes by Cdc42 in cochlear hair cells. J. Cell Sci. 127(Pt 9), 2040–2052. doi: 10.1242/jcs.143602
van Hengel, J., D’Hooge, P., Hooghe, B., Wu, X., Libbrecht, L., De Vos, R., et al. (2008). Continuous cell injury promotes hepatic tumorigenesis in cdc42-deficient mouse liver. Gastroenterology 134, 781–792. doi: 10.1053/j.gastro.2008.01.002
Velez-Ortega, A. C., and Frolenkov, G. I. (2019). Building and repairing the stereocilia cytoskeleton in mammalian auditory hair cells. Hear. Res. 376, 47–57. doi: 10.1016/j.heares.2018.12.012
Wang, Y., Li, J., Yao, X., Li, W., Du, H., Tang, M., et al. (2017). Loss of CIB2 causes profound hearing loss and abolishes mechanoelectrical transduction in mice. Front. Mol. Neurosci. 10:401. doi: 10.3389/fnmol.2017.00401
Yang, H., Xie, X., Deng, M., Chen, X., and Gan, L. (2010). Generation and characterization of Atoh1-Cre knock-in mouse line. Genesis 48, 407–413. doi: 10.1002/dvg.20633
Yang, L., Wang, L., and Zheng, Y. (2006). Gene targeting of Cdc42 and Cdc42GAP affirms the critical involvement of Cdc42 in filopodia induction, directed migration, and proliferation in primary mouse embryonic fibroblasts. Mol. Biol. Cell 17, 4675–4685. doi: 10.1091/mbc.e06-05-0466
Zampini, V., Ruttiger, L., Johnson, S. L., Franz, C., Furness, D. N., Waldhaus, J., et al. (2011). Eps8 regulates hair bundle length and functional maturation of mammalian auditory hair cells. PLoS Biol. 9:e1001048. doi: 10.1371/journal.pbio.1001048
Zhang, D. S., Piazza, V., Perrin, B. J., Rzadzinska, A. K., Poczatek, J. C., Wang, M., et al. (2012). Multi-isotope imaging mass spectrometry reveals slow protein turnover in hair-cell stereocilia. Nature 481, 520–524. doi: 10.1038/nature10745
Zhu, C., Cheng, C., Wang, Y., Muhammad, W., Liu, S., Zhu, W., et al. (2018). Loss of ARHGEF6 causes hair cell stereocilia deficits and hearing loss in mice. Front. Mol. Neurosci. 11:362. doi: 10.3389/fnmol.2018.00362
Keywords: inner ear, hair cells, stereocilia, CDC42, knockout mice
Citation: Du H, Zhou H, Sun Y, Zhai X, Chen Z, Wang Y and Xu Z (2021) The Rho GTPase Cell Division Cycle 42 Regulates Stereocilia Development in Cochlear Hair Cells. Front. Cell Dev. Biol. 9:765559. doi: 10.3389/fcell.2021.765559
Received: 27 August 2021; Accepted: 06 October 2021;
Published: 22 October 2021.
Edited by:
Hongzhe Li, VA Loma Linda Healthcare System, United StatesReviewed by:
Ke Liu, Capital Medical University, ChinaXue Gao, PLA Rocket Force Characteristic Medical Center, China
Lingchao Ji, Peking University Shenzhen Hospital, China
Weiqiang Yang, Peking University Shenzhen Hospital Shenzhen, China, in collaboration with reviewer LJ
Copyright © 2021 Du, Zhou, Sun, Zhai, Chen, Wang and Xu. This is an open-access article distributed under the terms of the Creative Commons Attribution License (CC BY). The use, distribution or reproduction in other forums is permitted, provided the original author(s) and the copyright owner(s) are credited and that the original publication in this journal is cited, in accordance with accepted academic practice. No use, distribution or reproduction is permitted which does not comply with these terms.
*Correspondence: Yanfei Wang, d2FuZ195ZkBzZHUuZWR1LmNu; Zhigang Xu, eHV6Z0BzZHUuZWR1LmNu