- Key Laboratory of Zoonosis Research, Ministry of Education, College of Animal Science, Jilin University, Changchun, China
Alzheimer’s disease (AD) is the most common neurodegenerative disease, characterized by progressive cognitive impairment and memory loss. So far, the pathogenesis of AD has not been fully understood. Research have shown that endoplasmic reticulum (ER) stress and unfolded protein response (UPR) participate in the occurrence and development of AD. Furthermore, various studies, both in vivo and in vitro, have shown that targeting ER stress and ER stress-mediated apoptosis contribute to the recovery of AD. Thus, targeting ER stress and ER stress-mediated apoptosis may be effective for treating AD. In this review, the molecular mechanism of ER stress and ER stress-mediated apoptosis, as well as the therapeutic effects of some natural compounds and small molecule inhibitors targeting ER stress and ER stress-mediated apoptosis in AD will be introduced.
Introduction
The endoplasmic reticulum (ER) is a crucial organelle of eukaryotic cells, whose functions include protein synthesis and folding, lipid biogenesis and calcium metabolism (Schwarz and Blower, 2016). Numerous cellular stresses, such as disequilibrium of calcium homeostasis, redox imbalance, changes in protein glycosylation, or protein folding defects in the ER, can trigger ER stress response, causing accumulation of unfolded or misfolded proteins in the ER lumen (Senft and Ronai, 2015). In order to maintain the homeostasis of the ER, the unfolded protein response (UPR), an integrated signal transduction pathway, is activated (Walter and Ron, 2011). UPR can inhibit protein transcription and translation, promote the degradation of misfolded proteins and increase the ability of correct protein folding (Hetz, 2012). However, under chronic or excessive ER stress, UPR fails to maintain the homeostasis of ER, then the apoptosis signaling pathway is activated, leading to a variety of diseases, including Alzheimer’s disease (AD) (Uddin et al., 2020).
AD is the most common neurodegenerative disease, characterized by progressive cognitive impairment and memory loss. Besides, abnormal accumulation of misfolded proteins in the brain, such as β-amyloid (Aβ) peptide and hyperphosphorylated tau protein, is the main neuropathological hallmark of AD (Villain and Dubois, 2019; Ghemrawi and Khair, 2020). So far, the pathogenesis of AD has not been fully understood, and there is no effective treatment for this disease. The existing treatments can only relieve the symptoms or slow down the progression of the disease, but the patients can’t be cured (Morris et al., 2018). Therefore, it is necessary to find a novel and effective treatment for AD.
Recently, research have shown that ER stress and UPR participate in the occurrence and development of AD (Hoozemans et al., 2009; Salminen et al., 2009; Uddin et al., 2020). Furthermore, various studies, both in vivo and in vitro, have shown that targeting ER stress and ER stress-mediated apoptosis contribute to the recovery of AD (Xu et al., 2018; Zhu et al., 2019; Song et al., 2020; Oliveira et al., 2021). Thus, targeting ER stress and ER stress-mediated apoptosis may be effective for treating AD. In this review, the molecular mechanism of ER stress and ER stress-mediated apoptosis, as well as the therapeutic effects of some natural compounds and small molecule inhibitors targeting ER stress and ER stress-mediated apoptosis in AD will be introduced.
ER Stress, UPR, and Apoptosis
ER Stress-Mediated UPR Signaling Pathways
Early ER stress will induce UPR, which might be protective to cells (Walter and Ron, 2011). Three ER transmembrane proteins are involved in UPR signaling pathways: PERK (protein kinase RNA-like ER kinase), IRE1 (inositol-requiring enzyme 1) and ATF6 (activating transcription factor 6) (Chen and Brandizzi, 2013; Hillary and FitzGerald, 2018; Rozpedek et al., 2019). Under physiological conditions, these proteins are combined with ER chaperone BiP (immunoglobulin binding protein, also called glucose-regulated protein 78, GRP78), and located in the ER membrane in an inactive state (Carrara et al., 2015; Ghemrawi and Khair, 2020). However, under ER stress, when a misfolded or unfolded protein binds to BiP, BiP is dissociated from these transmembrane proteins, then downstream signaling pathways of UPR are initiated (Figure 1; Ron and Walter, 2007; Parmar and Schroder, 2012). These three transmembrane proteins play a key role in the three signaling pathways of UPR, promoting the correct folding of unfolded and misfolded proteins, thus maintaining ER homeostasis.
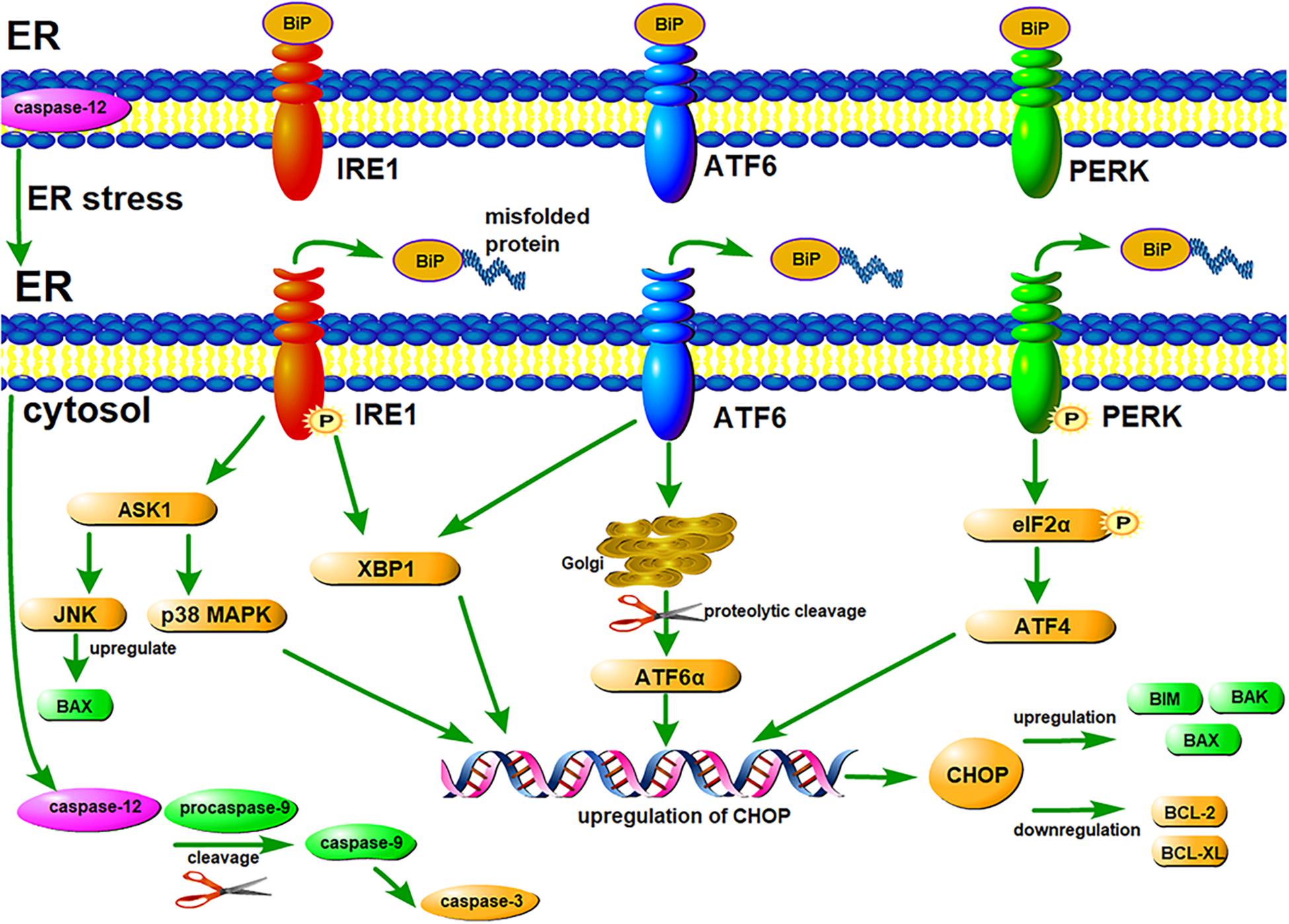
Figure 1. ER stress-mediated UPR signaling pathways and apoptosis signaling pathways. Three ER transmembrane proteins, IRE1, ATF6, and PERK, are involved in UPR signaling pathways. Under normal conditions, these proteins are combined with ER chaperone BiP, and located in the ER membrane in an inactive state. Under ER stress, when a misfolded protein binds to BiP, BiP dissociates from IRE1, ATF6, and PERK. Subsequently, PERK and IRE1 undergo autophosphorylation, and ATF6 undergoes proteolytic cleavage. Downstream signaling pathways of UPR are initiated, thereby maintaining ER homeostasis. However, under chronic or excessive ER stress, UPR fails to maintain the homeostasis of ER, then apoptosis signaling pathways are activated. All the three ER transmembrane proteins can up-regulate CHOP, leading to apoptosis by regulating the expression of some BCL-2 family members. Activated IRE1 can activate JNK, inducing apoptosis by up-regulating the expression of some pro-apoptotic proteins. Caspase-12 can induce apoptosis by activating the caspase cascade reaction.
PERK is a type I ER transmembrane protein with a serine/threonine protein kinase domain (Rozpedek et al., 2019). Under mild ER stress, PERK first undergoes autophosphorylation, and then eukaryotic initiation factor 2α (eIF2α) is activated by phosphorylation. Phosphorylated eIF2α can block protein synthesis and reduce the protein load of ER (Cnop et al., 2017).
IRE1 is also a type I ER transmembrane protein with endonuclease activities, which can be divided into two isforms, IRE1α and IRE1β (Chen and Brandizzi, 2013). When ER stress occurs, IRE1α is first activated by autophosphorylation and dimerization (Hetz et al., 2011). Then, through unconventional splicing of X-box binding protein 1 (XBP1) mRNA, an active transcription factor, XBP1s, is produced. XBP1s can promote the correct folding of unfolded and misfolded porteins, thereby restore ER homeostasis (Hollien et al., 2009).
ATF6 is a type II transmembrane protein, which encodes a basic leucine zipper (bZIP) transcription factor (Hillary and FitzGerald, 2018). In conditions of ER stress, ATF6 moves from the ER to the Golgi, where it undergoes proteolytic cleavage. Subsequently, the active transcription factor, ATF6α, is produced and transferred to the nucleus, where it regulates the expression of UPR related genes, such as CHOP, BiP, and XBP1 (Wu et al., 2007; Ghemrawi and Khair, 2020).
Chronic or Excessive ER Stress-Mediated Apoptosis Signaling Pathways
On the contrary, under chronic or excessive ER stress, UPR fails to maintain the homeostasis of ER, then the apoptosis signaling pathways are activated, even resulting in cell death (Senft and Ronai, 2015; Iurlaro and Munoz-Pinedo, 2016). ER stress can induce apoptosis through three major signaling pathways: CHOP pathway, JNK pathway and caspase-12 pathway (Figure 1; Hu et al., 2018).
PERK-CHOP Pathway
Phosphorylated eIF2α allows the translation of activating transcription factor 4 (ATF4), an activator of apoptosis-related genes (Hu et al., 2018). Subsequently, AFT4 up-regulates the expression of several apoptosis-related genes, for example, C/EBP homologous protein (CHOP) (Oyadomari and Mori, 2004). CHOP can regulate the expression of some BCL-2 family members, including the down-regulation of anti-apoptotic proteins like BCL-2 and BCL-XL, and the up-regulation of pro-apoptotic proteins like BIM, BAK, and BAX (Hata et al., 2015). Furthermore, the oligomerization of BAK-BAX will lead to the release of cytochrome c, which causes apoptosis through the mitochondrial pathway (Brenner and Mak, 2009).
IRE1-JNK Pathway
Activated IRE1 can activate apoptotic-signaling kinase-1 (ASK1) (Ron and Hubbard, 2008). Then downstream kinases, such as Jun-N-terminal kinase (JNK) and p38 mitogen-activated protein kinase (p38 MAPK), are activated (Urano et al., 2000). JNK can up-regulate the expression of pro-apoptotic proteins, such as BIM and PUMA, leading to the activation of BAX and subsequent apoptosis (Deng et al., 2001). Meanwhile, p38 MAPK can promote the transcription of CHOP, as well as regulate the expression of some BCL-2 family members (Oyadomari and Mori, 2004; Hata et al., 2015).
Caspase-12 Pathway
ER stress can activate caspase-12, and then the caspase cascade is activated (Iurlaro and Munoz-Pinedo, 2016). Activated caspase-12 moves from the ER to the cytosol, where it cleaves procaspase-9 to form caspase-9 (Szegezdi et al., 2003). Caspase-9 in turn activates caspase-3, the main executioner of apoptosis (Lossi et al., 2018).
Applications of Natural Compounds and Small Molecule Inhibitors Targeting ER Stress and ER Stress-Mediated Apoptosis in Treating Alzheimer’s Disease
Researches have shown that ER stress and UPR participate in the occurrence and development of AD (Hoozemans et al., 2009; Salminen et al., 2009; Uddin et al., 2020). During AD, the continuous accumulation of Aβ or p-tau leads to unbalanced ER calcium homeostasis, abnormal protein folding and ER stress (Sobow et al., 2004; Mondragon-Rodriguez et al., 2012). Neuronal cells are especially sensitive to protein misfolding, therefore, the excessive accumulation of Aβ or p-tau will result in synaptic dysfunction and apoptosis, even neuronal death (Remondelli and Renna, 2017; Ghemrawi and Khair, 2020). In addition, some UPR-related proteins, such as phosphorylated PERK, eIF2α and IRE1 were found in AD brains (Devi and Ohno, 2014; Duran-Aniotz et al., 2017; Uddin et al., 2020). Meanwhile, ER stress markers, including GRP78, CHOP and caspase-12, are up-regulated in AD patients (Santos and Ferreira, 2018).
Recently, various studies, both in vivo and in vitro, have shown that targeting ER stress and ER stress-mediated apoptosis contribute to the recovery of AD (Xu et al., 2018; Zhu et al., 2019; Song et al., 2020; Oliveira et al., 2021). Several active components extracted from the plants and small molecule inhibitors of UPR signaling pathways and other signaling pathways have displayed good therapeutic effects on AD models. A brief summary of some natural compounds and small molecule inhibitors targeting ER stress in AD studies is shown in Table 1.
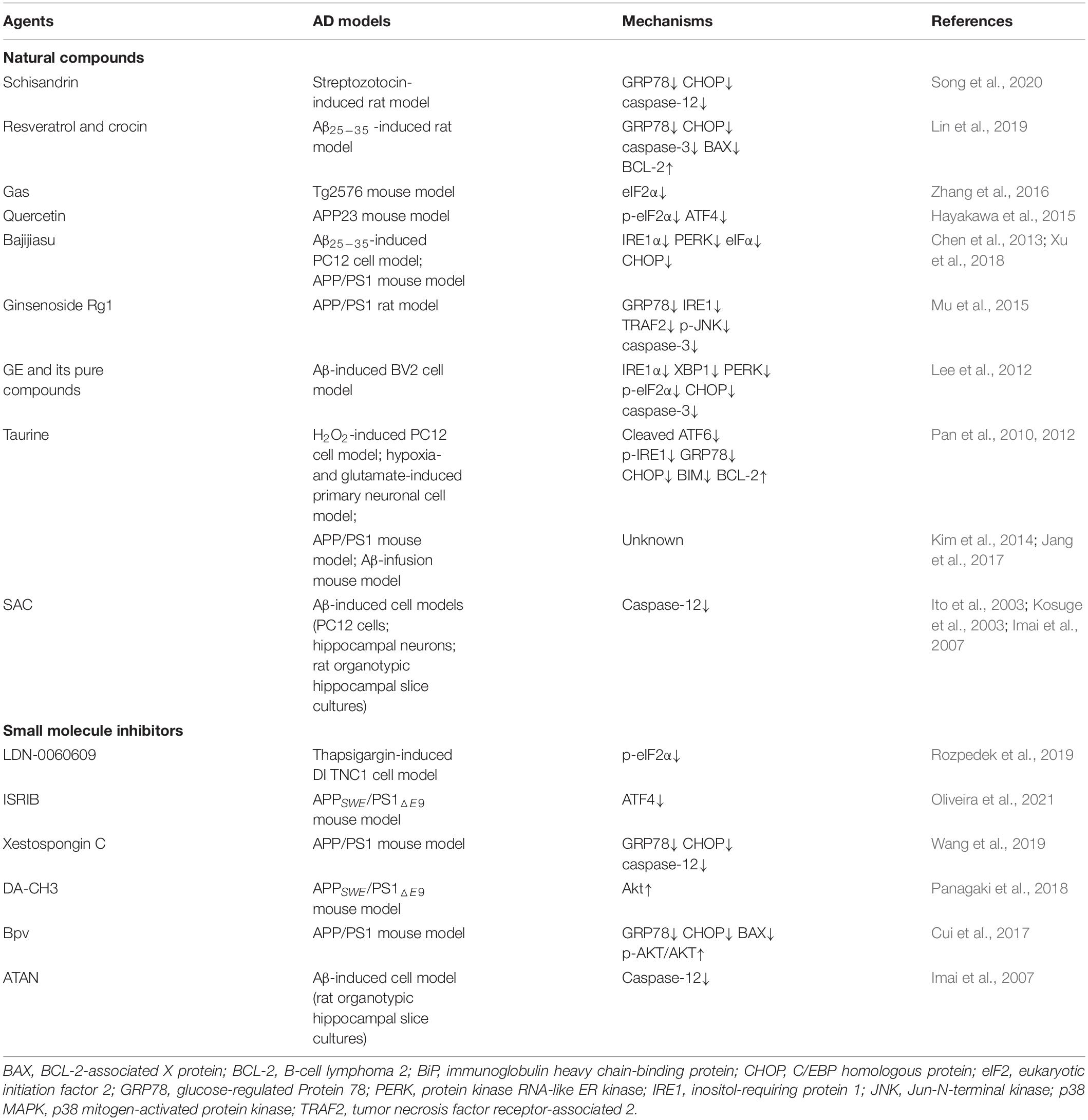
Table 1. Natural compounds and small molecule inhibitors targeting ER stress and ER stress-mediated apoptosis in treating Alzheimer’s disease.
Targeting PERK-CHOP Signaling Pathway
The PERK-CHOP signaling pathway plays an essential role in inducing cell apoptosis. In the early stage of ER stress, activated PERK can further activate eIF2α, which can block protein synthesis and exert a protective effect on cells (Rozpedek et al., 2019). However, under chronic or excessive ER stress, PERK-CHOP signaling pathway will be activated, leading to apoptosis (Hu et al., 2018).
Schisandrin (Sch)
Schisandrin (Sch) is an active component extracted from schisandra chinensis Baill (Zhu et al., 2019). In the Streptozotocin (STZ)-induced AD rats, after the treatment of Sch, the expression of ER stress markers, including GRP78, CHOP, and cleaved caspase-12, was obviously decreased (Song et al., 2020). Moreover, Sch also improved the learning and memory capacity of STZ-induced AD rats, by enhancing the activity of Sirtuin 1 (SIRT1, an enzyme contributes to the acquisition and maintenance of memory) (Song et al., 2020).
Resveratrol and Crocin
In the Aβ25–35-induced AD rats, both enhanced expression of GRP78, CHOP, caspase-3, BAX and attenuated expression of BCL-2 were observed in the hippocampal CA1 region (Hippo) and prefrontal cortical (PFC), while the situation was reversed by treatment with resveratrol (a polyphenolic stilbenoid, present in grapes, mulberries, peanuts and other plants; Malaguarnera, 2019) and crocin (a non-tetraquinone pigment extracted from Crocus sativus L.; Shafahi et al., 2018). Furthermore, improved learning and memory ability and decreased number of apoptotic neurons were also detected in AD rats (Lin et al., 2019).
Gastrodin (Gas)
Tg2576 transgenic mouse is an AD model with high expression of β-site APP-cleaving enzyme 1 (BACE1), which is a rate-limiting enzyme for Aβ generation and could aggravate the process of AD (O’Connor et al., 2008). Gastrodin (Gas), an active component of Gastrodia elata Blume, was found to improve learning and memory abilities and attenuate intracellular oxidative stress of Tg2576 mice (Zhang et al., 2016). Moreover, Gas down-regulated BACE1 expression via inhibiting activation of PKR and eIF2α (Zhang et al., 2016).
Quercetin
In the APP23 AD model mice, after long-term feeding with quercetin, a polyhydroxylated flavonoid, the expression of growth arrest and DNA damaged-inducible gene 34 (GADD34) was induced, leading to the down-regulation of phosphorylated-eIF2α and ATF4 (Ohta et al., 2011; Hayakawa et al., 2015). This resulted in improvement of memory in aged AD mice, and delayed deterioration in memory of the mice at the early stage of AD (Hayakawa et al., 2015).
LDN-0060609
Compound LDN-0060609 is a small molecule PERK inhibitor (Rozpedek et al., 2019). In rat astrocytic DI TNC1 cell line, LDN-0060609 pretreatment attenuated the pro-apoptotic, PERK-dependent signaling pathway induced by thapsigargin (Th) treatment. It significantly inhibited eIF2α phosphorylation and increased cell survival in vitro. Notably, LDN-0060609 has no cytotoxicity to DI TNC1 cells and also has no influence on cell cycle progression (Rozpedek et al., 2019; Rozpedek-Kaminska et al., 2020). Therefore, it may contribute to prevention against apoptosis and neurodegeneration in AD without the cytotoxic effect.
ISRIB
ISRIB is a small molecule integrated stress response (ISR) inhibitor, which can reverse the phosphorylation of eIF2α and inhibit the downstream targets of eIF2α, such as ATF4, CHOP, and GADD34 (Sidrauski et al., 2015). In an AD mouse model induced by intracerebroventricular injection of amyloid-β oligomers (AβOs), ISRIB treatment counteracted the increase in ATF4 protein level, protecting mice from long-term memory impairment (Oliveira et al., 2021). Furthermore, ISRIB was shown to attenuate translational repression, restore synaptic plasticity and memory in transgenic APPSWE/PS1△E9 AD mice (Oliveira et al., 2021).
Xestospongin C (XeC)
Xestospongin C (XeC), a compound isolated from the Xestospongia species, is a reversible IP3 receptor antagonist (Gafni et al., 1997). It was found that XeC could improve the cognitive behavior of APP/PS1 AD mice. XeC also reduced the number of Aβ plaques and down-regulated the expression of GRP78, CHOP and caspase-12 in APP/PS1 mice (Wang et al., 2019). In addition, XeC significantly ameliorated Aβ1–42-induced neuronal apoptosis and intracellular Ca2+ overload in primary cultured hippocampal neurons (Wang et al., 2019).
Targeting IRE1-JNK Signaling Pathway
Activated IRE1 can activate JNK, leading to the up-regulation of pro-apoptotic proteins and subsequent apoptosis (Urano et al., 2000; Deng et al., 2001). Activation of IRE1-JNK signaling pathway plays a key role in ER stress-mediated apoptosis.
Bajijiasu
Bajijiasu (also known as bajisu) is a natural active ingredient isolated from Morinda officinalis (Chen et al., 2014). It was shown to play a protective role against Aβ25–35-induced neurotoxicity in PC12 cells (Chen et al., 2013). In a double transgenic APP/PS1 mouse model of AD, oral administration of bajijiasu improved learning and memory abilities of APP/PS1 mice. Also, Bajijiasu protected neurons from apoptosis by down-regulating the expression of IRE1α, PERK, eIF2α, and CHOP. Moreover, reduced ROS and MDA levels in both the hippocampus and cortex were detected (Xu et al., 2018).
Ginsenoside Rg1
Ginsenoside Rg1 is a steroidal saponin highly abundant in ginseng (Wu et al., 2013). In a APP/PS1 rat model, AD rats were fed with 0.5% Rg1-enriched food to investigate the neuroprotective effects of Rg1. After Rg1 treatment, the accumulation of Aβ plaque and neurofbrillary tangles (NFTs) was significantly decreased in the AD rats, as well as the reduced expression of caspase-3 and the number of apoptotic cells. Furthermore, down-regulation of GRP78, IRE1, and p-JNK was observed in the AD rats, indicating that Rg1 exhibited neuroprotective effects by inhibiting the ER stress-mediated JNK apoptotic pathway (Mu et al., 2015).
Gastrodia Elata (GE) and Its Pure Compounds
In the BV2 mouse microglial cells, Aβ not only induced cytotoxicity and apoptosis but also promoted the expression of IRE1α, XBP1, PERK, phosphorylated eIF2α, CHOP, caspase-3 (Lee et al., 2012). However, after treatment with Gastrodia elata (GE) and its pure compounds, gastrodin (Gas) and 4-hydroxybenzyl alcohol (4HBA), these ER stress-relevant proteins were down-regulated. Moreover, Gas and 4HBA inhibited the neurotoxicity induced by Aβ and increased cells viability (Lee et al., 2012).
Targeting ATF6-AKT Signaling Pathway
Under ER stress, the ATF6 pathway can mediate cell survival by regulating the activation of Akt and the expression of GRP78 and CHOP (Cui et al., 2017).
Taurine
Taurine, a free amino acid, is abundant in the brain and plays an important role in the central nervous system (Seidel et al., 2019). It was found that taurine can protect PC12 cells against H2O2-induced ER stress, leading to the increase in cell viability, as well as the down-regulation of GRP78, CHOP, and BIM (Pan et al., 2010). Meanwhile, in primary neuronal cultures, taurine can also modulate hypoxia- and glutamate-induced ER stress by down-regulating the expression of caspase-12, CHOP, cleaved ATF6 and p-IRE1 (Pan et al., 2012). Later, in APP/PS1 transgenic AD mouse model, oral administration of taurine significantly ameliorated cognitive deficits of the adult AD mice (Kim et al., 2014). Further study conducted in the oligomeric Aβ-infusion AD mouse model showed that taurine can ameliorate cognitive impairment by directly binding to oligomeric Aβ (Jang et al., 2017). Nevertheless, mechanisms underlying taurine mediated cognitive improvement still need further elucidation.
Dipotassium Bisperoxo-(5-Hydroxypyridine-2-Carboxyl)-Oxovanadate (bpv)
Phosphatase and tensin homolog deleted on chromosome ten (PTEN) is a tumor suppressor, which plays an essential role in regulating neuronal survival or apoptosis (Kitagishi and Matsuda, 2013). Dipotassium bisperoxo-(5-hydroxypyridine-2-carboxyl)-oxovanadate (bpv), a PTEN inhibitor, could decrease apoptosis and suppress the expression of ER stress related protein GRP78, CHOP, and Bax in APP/PS1 transgenic AD mice. Further study showed that the neuroprotective role of bpv in APP/PS1 mice was mediated by activation of PI3K/AKT signaling pathways (Cui et al., 2017).
DA-CH3
Chronic treatment with DA-CH3, a novel dual GLP-1/GIP receptor agonist (Holscher, 2018), could rescue the spatial acquisition and memory impairments of APPSWE/PS1△E9 mice. Additionally, excessive plaque deposition, gliosis and synaptic damage was ameliorated in the APPSWE/PS1△E9 brain. Further research found that the alleviated ER stress and autophagy impairments in the APPSWE/PS1△E9 mouse brain might be attributed to the up-regulation of the Akt activation (Panagaki et al., 2018).
Targeting Caspase-12 Signaling Pathway
ER stress can induce mitochondrial apoptosis by regulating Bcl-2 family members, such as Bcl-2 and Bcl-XL. However, persistent ER stress will activate caspase-12, and further activate caspase-3, finally triggering cell apoptosis (Iurlaro and Munoz-Pinedo, 2016).
S-allyl-L-cysteine (SAC) is an organosulfur compound extracted from aged garlic (Moriguchi et al., 1996). It was shown that SAC could prevent neuronal death induced by Aβ in PC12 cells (Ito et al., 2003), cultured hippocampal neurons (Kosuge et al., 2003), and rat organotypic hippocampal slice cultures (OHCs) (Imai et al., 2007). Besides, Aβ-induced increase in caspase-12 protein expression was suppressed by SAC in cultured hippocampal neurons and OHCs. Furthermore, SAC also decreased the Aβ-induced intracellular reactive oxygen species (ROS) levels in hippocampal neurons (Kosuge et al., 2003). In addition, Z-ATAN-fmk (ATAN), a specific caspase-12 inhibitor, could markedly suppress neurotoxicity induced by Aβ in OHCs (Imai et al., 2007).
Discussion
It is proved that ER stress and UPR play an essential role in the occurrence and development of AD (Devi and Ohno, 2014; Duran-Aniotz et al., 2017; Uddin et al., 2020). The related findings, both in vivo and in vitro, have shown that many natural compounds and small molecular inhibitors targeting ER stress and ER stress-mediated neuronal apoptosis contribute to the recovery of AD (Xu et al., 2018; Zhu et al., 2019; Song et al., 2020; Oliveira et al., 2021). However, there are still a lot of questions to be considered if we want to convert these agents into a safe and reliable drug for clinical application, such as the safety, efficacy, and applicability.
Nevertheless, due to the species differences between humans and mice, the existing AD mouse models cannot fully simulate the pathologic and clinical features of human AD, which limits their application in preclinical studies of AD (de Bem et al., 2020). Therefore, it is necessary to develop animal models more similar to human AD to test the safety and efficacy of these agents (Medina and Avila, 2014). Recently, with the development of efficient genome editing technology, larger animals, such as rabbits, pigs and non-human primates, can be used to construct models of AD (Bosze et al., 2003; Chan, 2013; Yang and Wu, 2018). By using the CRISPR/Cas9 system and base editing system, these animal models can accurately simulate the gene mutation sites of human AD, and provide an ideal platform for AD pathogenesis research and preclinical evaluation (Tu et al., 2015; Gaudelli et al., 2017). In the near future, more unknown mechanisms between ER stress and AD will be clarified, which will offer more safe and effective therapeutic strategies to AD.
Author Contributions
XG and YX initiated the project, wrote, revised, and finalized the manuscript. XG searched the database. Both authors contributed to the article and approved the submitted version.
Funding
This work was financially supported by the National Key Research and Development Program of China Stem Cell and Translational Research (2017YFA0105101). The Program for Changjiang Scholars and Innovative Research Team in University (No. IRT_16R32). The Strategic Priority Research Program of the Chinese Academy of Sciences (XDA16030501 and XDA16030503), Key Research and Development Program of Guangzhou Regenerative Medicine and Health Guangdong Laboratory (2018GZR110104004).
Conflict of Interest
The authors declare that the research was conducted in the absence of any commercial or financial relationships that could be construed as a potential conflict of interest.
Publisher’s Note
All claims expressed in this article are solely those of the authors and do not necessarily represent those of their affiliated organizations, or those of the publisher, the editors and the reviewers. Any product that may be evaluated in this article, or claim that may be made by its manufacturer, is not guaranteed or endorsed by the publisher.
References
Bosze, Z., Hiripi, L., Carnwath, J. W., and Niemann, H. (2003). The transgenic rabbit as model for human diseases and as a source of biologically active recombinant proteins. Transgenic Res. 12, 541–553.
Brenner, D., and Mak, T. W. (2009). Mitochondrial cell death effectors. Curr. Opin. Cell Biol. 21, 871–877. doi: 10.1016/j.ceb.2009.09.004
Carrara, M., Prischi, F., Nowak, P. R., Kopp, M. C., and Ali, M. M. (2015). Noncanonical binding of BiP ATPase domain to Ire1 and Perk is dissociated by unfolded protein CH1 to initiate ER stress signaling. Elife 4:e03522.
Chan, A. W. (2013). Progress and prospects for genetic modification of nonhuman primate models in biomedical research. ILAR J. 54, 211–223. doi: 10.1093/ilar/ilt035
Chen, D. L., Zhang, P., Lin, L., Shuai, O., Zhang, H. M., Liu, S. H., et al. (2013). Protective effect of Bajijiasu against beta-amyloid-induced neurotoxicity in PC12 cells. Cell. Mol. Neurobiol. 33, 837–850. doi: 10.1007/s10571-013-9950-7
Chen, D. L., Zhang, P., Lin, L., Zhang, H. M., Deng, S. D., Wu, Z. Q., et al. (2014). Protective effects of bajijiasu in a rat model of Abeta(2)(5)(-)(3)(5)-induced neurotoxicity. J. Ethnopharmacol. 154, 206–217. doi: 10.1016/j.jep.2014.04.004
Chen, Y., and Brandizzi, F. (2013). IRE1: ER stress sensor and cell fate executor. Trends Cell Biol. 23, 547–555.
Cnop, M., Toivonen, S., Igoillo-Esteve, M., and Salpea, P. (2017). Endoplasmic reticulum stress and eIF2alpha phosphorylation: the Achilles heel of pancreatic beta cells. Mol. Metab. 6, 1024–1039. doi: 10.1016/j.molmet.2017.06.001
Cui, W., Wang, S., Wang, Z., Wang, Z., Sun, C., and Zhang, Y. (2017). Inhibition of PTEN Attenuates Endoplasmic Reticulum Stress and Apoptosis via Activation of PI3K/AKT Pathway in Alzheimer’s Disease. Neurochem. Res. 42, 3052–3060. doi: 10.1007/s11064-017-2338-1
de Bem, A. F., Krolow, R., Farias, H. R., De Rezende, V. L., Gelain, D. P., Moreira, J. C. F., et al. (2020). Animal Models of Metabolic Disorders in the Study of Neurodegenerative Diseases: an Overview. Front. Neurosci. 14:604150. doi: 10.3389/fnins.2020.604150
Deng, X., Xiao, L., Lang, W., Gao, F., Ruvolo, P., and May, W. S. Jr. (2001). Novel role for JNK as a stress-activated Bcl2 kinase. J. Biol. Chem. 276, 23681–23688. doi: 10.1074/jbc.m100279200
Devi, L., and Ohno, M. (2014). PERK mediates eIF2alpha phosphorylation responsible for BACE1 elevation, CREB dysfunction and neurodegeneration in a mouse model of Alzheimer’s disease. Neurobiol. Aging 35, 2272–2281. doi: 10.1016/j.neurobiolaging.2014.04.031
Duran-Aniotz, C., Cornejo, V. H., Espinoza, S., Ardiles, A. O., Medinas, D. B., Salazar, C., et al. (2017). IRE1 signaling exacerbates Alzheimer’s disease pathogenesis. Acta Neuropathol. 134, 489–506. doi: 10.1007/s00401-017-1694-x
Gafni, J., Munsch, J. A., Lam, T. H., Catlin, M. C., Costa, L. G., Molinski, T. F., et al. (1997). Xestospongins: potent membrane permeable blockers of the inositol 1,4,5-trisphosphate receptor. Neuron 19, 723–733. doi: 10.1016/s0896-6273(00)80384-0
Gaudelli, N. M., Komor, A. C., Rees, H. A., Packer, M. S., Badran, A. H., Bryson, D. I., et al. (2017). Programmable base editing of AT to GC in genomic DNA without DNA cleavage. Nature 551, 464–471. doi: 10.1038/nature24644
Ghemrawi, R., and Khair, M. (2020). Endoplasmic Reticulum Stress and Unfolded Protein Response in Neurodegenerative Diseases. Int. J. Mol. Sci. 21:6127. doi: 10.3390/ijms21176127
Hata, A. N., Engelman, J. A., and Faber, A. C. (2015). The BCL2 Family: key Mediators of the Apoptotic Response to Targeted Anticancer Therapeutics. Cancer Discov. 5, 475–487. doi: 10.1158/2159-8290.cd-15-0011
Hayakawa, M., Itoh, M., Ohta, K., Li, S., Ueda, M., Wang, M. X., et al. (2015). Quercetin reduces eIF2alpha phosphorylation by GADD34 induction. Neurobiol. Aging 36, 2509–2518. doi: 10.1016/j.neurobiolaging.2015.05.006
Hetz, C. (2012). The unfolded protein response: controlling cell fate decisions under ER stress and beyond. Nat. Rev. Mol. Cell Biol. 13, 89–102. doi: 10.1038/nrm3270
Hetz, C., Martinon, F., Rodriguez, D., and Glimcher, L. H. (2011). The unfolded protein response: integrating stress signals through the stress sensor IRE1alpha. Physiol. Rev. 91, 1219–1243. doi: 10.1152/physrev.00001.2011
Hillary, R. F., and FitzGerald, U. (2018). A lifetime of stress: ATF6 in development and homeostasis. J. Biomed. Sci. 25:48.
Hollien, J., Lin, J. H., Li, H., Stevens, N., Walter, P., and Weissman, J. S. (2009). Regulated Ire1-dependent decay of messenger RNAs in mammalian cells. J. Cell Biol. 186, 323–331. doi: 10.1083/jcb.200903014
Holscher, C. (2018). Novel dual GLP-1/GIP receptor agonists show neuroprotective effects in Alzheimer’s and Parkinson’s disease models. Neuropharmacology 136, 251–259. doi: 10.1016/j.neuropharm.2018.01.040
Hoozemans, J. J., Van Haastert, E. S., Nijholt, D. A., Rozemuller, A. J., Eikelenboom, P., and Scheper, W. (2009). The unfolded protein response is activated in pretangle neurons in Alzheimer’s disease hippocampus. Am. J. Pathol. 174, 1241–1251. doi: 10.2353/ajpath.2009.080814
Hu, H., Tian, M., Ding, C., and Yu, S. (2018). The C/EBP Homologous Protein (CHOP) Transcription Factor Functions in Endoplasmic Reticulum Stress-Induced Apoptosis and Microbial Infection. Front. Immunol. 9:3083. doi: 10.3389/fimmu.2018.03083
Imai, T., Kosuge, Y., Ishige, K., and Ito, Y. (2007). Amyloid beta-protein potentiates tunicamycin-induced neuronal death in organotypic hippocampal slice cultures. Neuroscience 147, 639–651. doi: 10.1016/j.neuroscience.2007.04.057
Ito, Y., Kosuge, Y., Sakikubo, T., Horie, K., Ishikawa, N., Obokata, N., et al. (2003). Protective effect of S-allyl-L-cysteine, a garlic compound, on amyloid beta-protein-induced cell death in nerve growth factor-differentiated PC12 cells. Neurosci. Res. 46, 119–125. doi: 10.1016/s0168-0102(03)00037-3
Iurlaro, R., and Munoz-Pinedo, C. (2016). Cell death induced by endoplasmic reticulum stress. FEBS J. 283, 2640–2652.
Jang, H., Lee, S., Choi, S. L., Kim, H. Y., Baek, S., and Kim, Y. (2017). Taurine Directly Binds to Oligomeric Amyloid-beta and Recovers Cognitive Deficits in Alzheimer Model Mice. Adv. Exp. Med. Biol. 975, 233–241. doi: 10.1007/978-94-024-1079-2_21
Kim, H. Y., Kim, H. V., Yoon, J. H., Kang, B. R., Cho, S. M., Lee, S., et al. (2014). Taurine in drinking water recovers learning and memory in the adult APP/PS1 mouse model of Alzheimer’s disease. Sci. Rep. 4:7467.
Kitagishi, Y., and Matsuda, S. (2013). Diets involved in PPAR and PI3K/AKT/PTEN pathway may contribute to neuroprotection in a traumatic brain injury. Alzheimers Res. Ther. 5:42. doi: 10.1186/alzrt208
Kosuge, Y., Koen, Y., Ishige, K., Minami, K., Urasawa, H., Saito, H., et al. (2003). S-allyl-L-cysteine selectively protects cultured rat hippocampal neurons from amyloid beta-protein- and tunicamycin-induced neuronal death. Neuroscience 122, 885–895. doi: 10.1016/j.neuroscience.2003.08.026
Lee, G. H., Kim, H. R., Han, S. Y., Bhandary, B., Kim, D. S., Kim, M. G., et al. (2012). Gastrodia elata Blume and its pure compounds protect BV-2 microglial-derived cell lines against beta-amyloid: the involvement of GRP78 and CHOP. Biol. Res. 45, 403–410. doi: 10.4067/s0716-97602012000400013
Lin, L., Liu, G., and Yang, L. (2019). Crocin Improves Cognitive Behavior in Rats with Alzheimer’s Disease by Regulating Endoplasmic Reticulum Stress and Apoptosis. Biomed. Res. Int. 2019:9454913.
Lossi, L., Castagna, C., and Merighi, A. (2018). Caspase-3 Mediated Cell Death in the Normal Development of the Mammalian Cerebellum. Int. J. Mol. Sci. 19:3999. doi: 10.3390/ijms19123999
Malaguarnera, L. (2019). Influence of Resveratrol on the Immune Response. Nutrients 11:946. doi: 10.3390/nu11050946
Medina, M., and Avila, J. (2014). The need for better AD animal models. Front. Pharmacol. 5:227. doi: 10.3389/fphar.2014.00227
Mondragon-Rodriguez, S., Perry, G., Zhu, X., and Boehm, J. (2012). Amyloid Beta and tau proteins as therapeutic targets for Alzheimer’s disease treatment: rethinking the current strategy. Int. J. Alzheimers Dis. 2012:630182.
Moriguchi, T., Saito, H., and Nishiyama, N. (1996). Aged garlic extract prolongs longevity and improves spatial memory deficit in senescence-accelerated mouse. Biol. Pharm. Bull. 19, 305–307. doi: 10.1248/bpb.19.305
Morris, G., Puri, B. K., Walder, K., Berk, M., Stubbs, B., Maes, M., et al. (2018). The Endoplasmic Reticulum Stress Response in Neuroprogressive Diseases: emerging Pathophysiological Role and Translational Implications. Mol. Neurobiol. 55, 8765–8787. doi: 10.1007/s12035-018-1028-6
Mu, J. S., Lin, H., Ye, J. X., Lin, M., and Cui, X. P. (2015). Rg1 exhibits neuroprotective effects by inhibiting the endoplasmic reticulum stress-mediated c-Jun N-terminal protein kinase apoptotic pathway in a rat model of Alzheimer’s disease. Mol. Med. Rep. 12, 3862–3868. doi: 10.3892/mmr.2015.3853
O’Connor, T., Sadleir, K. R., Maus, E., Velliquette, R. A., Zhao, J., Cole, S. L., et al. (2008). Phosphorylation of the translation initiation factor eIF2alpha increases BACE1 levels and promotes amyloidogenesis. Neuron 60, 988–1009. doi: 10.1016/j.neuron.2008.10.047
Ohta, K., Mizuno, A., Li, S., Itoh, M., Ueda, M., Ohta, E., et al. (2011). Endoplasmic reticulum stress enhances gamma-secretase activity. Biochem. Biophys. Res. Commun. 416, 362–366. doi: 10.1016/j.bbrc.2011.11.042
Oliveira, M. M., Lourenco, M. V., Longo, F., Kasica, N. P., Yang, W., Ureta, G., et al. (2021). Correction of eIF2-dependent defects in brain protein synthesis, synaptic plasticity, and memory in mouse models of Alzheimer’s disease. Sci. Signal 14:eabc5429. doi: 10.1126/scisignal.abc5429
Oyadomari, S., and Mori, M. (2004). Roles of CHOP/GADD153 in endoplasmic reticulum stress. Cell Death Differ. 11, 381–389. doi: 10.1038/sj.cdd.4401373
Pan, C., Giraldo, G. S., Prentice, H., and Wu, J. Y. (2010). Taurine protection of PC12 cells against endoplasmic reticulum stress induced by oxidative stress. J. Biomed. Sci. 17:S17.
Pan, C., Prentice, H., Price, A. L., and Wu, J. Y. (2012). Beneficial effect of taurine on hypoxia- and glutamate-induced endoplasmic reticulum stress pathways in primary neuronal culture. Amino Acids 43, 845–855. doi: 10.1007/s00726-011-1141-6
Panagaki, T., Gengler, S., and Holscher, C. (2018). The Novel DA-CH3 Dual Incretin Restores Endoplasmic Reticulum Stress and Autophagy Impairments to Attenuate Alzheimer-Like Pathology and Cognitive Decrements in the APPSWE/PS1DeltaE9 Mouse Model. J. Alzheimers Dis. 66, 195–218. doi: 10.3233/jad-180584
Parmar, V. M., and Schroder, M. (2012). Sensing endoplasmic reticulum stress. Adv. Exp. Med. Biol. 738, 153–168.
Remondelli, P., and Renna, M. (2017). The Endoplasmic Reticulum Unfolded Protein Response in Neurodegenerative Disorders and Its Potential Therapeutic Significance. Front. Mol. Neurosci. 10:187. doi: 10.3389/fnmol.2017.00187
Ron, D., and Hubbard, S. R. (2008). How IRE1 reacts to ER stress. Cell 132, 24–26. doi: 10.1016/j.cell.2007.12.017
Ron, D., and Walter, P. (2007). Signal integration in the endoplasmic reticulum unfolded protein response. Nat. Rev. Mol. Cell Biol. 8, 519–529. doi: 10.1038/nrm2199
Rozpedek, W., Pytel, D., Poplawski, T., Walczak, A., Gradzik, K., Wawrzynkiewicz, A., et al. (2019). Inhibition of the PERK-Dependent Unfolded Protein Response Signaling Pathway Involved in the Pathogenesis of Alzheimer’s Disease. Curr. Alzheimer Res. 16, 209–218. doi: 10.2174/1567205016666190228121157
Rozpedek-Kaminska, W., Siwecka, N., Wawrzynkiewicz, A., Wojtczak, R., Pytel, D., Diehl, J. A., et al. (2020). The PERK-Dependent Molecular Mechanisms as a Novel Therapeutic Target for Neurodegenerative Diseases. Int. J. Mol. Sci. 21:2108. doi: 10.3390/ijms21062108
Salminen, A., Kauppinen, A., Suuronen, T., Kaarniranta, K., and Ojala, J. (2009). ER stress in Alzheimer’s disease: a novel neuronal trigger for inflammation and Alzheimer’s pathology. J. Neuroinflammation 6:41. doi: 10.1186/1742-2094-6-41
Santos, L. E., and Ferreira, S. T. (2018). Crosstalk between endoplasmic reticulum stress and brain inflammation in Alzheimer’s disease. Neuropharmacology 136, 350–360. doi: 10.1016/j.neuropharm.2017.11.016
Schwarz, D. S., and Blower, M. D. (2016). The endoplasmic reticulum: structure, function and response to cellular signaling. Cell Mol. Life Sci. 73, 79–94. doi: 10.1007/s00018-015-2052-6
Seidel, U., Huebbe, P., and Rimbach, G. (2019). Taurine: a Regulator of Cellular Redox Homeostasis and Skeletal Muscle Function. Mol. Nutr. Food Res. 63:e1800569.
Senft, D., and Ronai, Z. A. (2015). UPR, autophagy, and mitochondria crosstalk underlies the ER stress response. Trends Biochem. Sci. 40, 141–148. doi: 10.1016/j.tibs.2015.01.002
Shafahi, M., Vaezi, G., Shajiee, H., Sharafi, S., and Khaksari, M. (2018). Crocin Inhibits Apoptosis and Astrogliosis of Hippocampus Neurons Against Methamphetamine Neurotoxicity via Antioxidant and Anti-inflammatory Mechanisms. Neurochem. Res. 43, 2252–2259. doi: 10.1007/s11064-018-2644-2
Sidrauski, C., Tsai, J. C., Kampmann, M., Hearn, B. R., Vedantham, P., Jaishankar, P., et al. (2015). Pharmacological dimerization and activation of the exchange factor eIF2B antagonizes the integrated stress response. Elife 4:e07314.
Sobow, T., Flirski, M., and Liberski, P. P. (2004). Amyloid-beta and tau proteins as biochemical markers of Alzheimer’s disease. Acta Neurobiol. Exp. (Wars) 64, 53–70.
Song, L., Piao, Z., Yao, L., Zhang, L., and Lu, Y. (2020). Schisandrin ameliorates cognitive deficits, endoplasmic reticulum stress and neuroinflammation in streptozotocin (STZ)-induced Alzheimer’s disease rats. Exp. Anim. 69, 363–373. doi: 10.1538/expanim.19-0146
Szegezdi, E., Fitzgerald, U., and Samali, A. (2003). Caspase-12 and ER-stress-mediated apoptosis: the story so far. Ann. N. Y. Acad. Sci. 1010, 186–194. doi: 10.1196/annals.1299.032
Tu, Z., Yang, W., Yan, S., Guo, X., and Li, X. J. (2015). CRISPR/Cas9: a powerful genetic engineering tool for establishing large animal models of neurodegenerative diseases. Mol. Neurodegener. 10:35.
Uddin, M. S., Tewari, D., Sharma, G., Kabir, M. T., Barreto, G. E., Bin-Jumah, M. N., et al. (2020). Molecular Mechanisms of ER Stress and UPR in the Pathogenesis of Alzheimer’s Disease. Mol. Neurobiol. 57, 2902–2919. doi: 10.1007/s12035-020-01929-y
Urano, F., Wang, X., Bertolotti, A., Zhang, Y., Chung, P., Harding, H. P., et al. (2000). Coupling of stress in the ER to activation of JNK protein kinases by transmembrane protein kinase IRE1. Science 287, 664–666. doi: 10.1126/science.287.5453.664
Villain, N., and Dubois, B. (2019). Alzheimer’s Disease Including Focal Presentations. Semin. Neurol. 39, 213–226. doi: 10.1055/s-0039-1681041
Walter, P., and Ron, D. (2011). The unfolded protein response: from stress pathway to homeostatic regulation. Science 334, 1081–1086. doi: 10.1126/science.1209038
Wang, Z. J., Zhao, F., Wang, C. F., Zhang, X. M., Xiao, Y., Zhou, F., et al. (2019). Xestospongin C, a Reversible IP3 Receptor Antagonist, Alleviates the Cognitive and Pathological Impairments in APP/PS1 Mice of Alzheimer’s Disease. J. Alzheimers Dis. 72, 1217–1231. doi: 10.3233/jad-190796
Wu, J., Pan, Z., Cheng, M., Shen, Y., Yu, H., Wang, Q., et al. (2013). Ginsenoside Rg1 facilitates neural differentiation of mouse embryonic stem cells via GR-dependent signaling pathway. Neurochem. Int. 62, 92–102. doi: 10.1016/j.neuint.2012.09.016
Wu, J., Rutkowski, D. T., Dubois, M., Swathirajan, J., Saunders, T., Wang, J., et al. (2007). ATF6alpha optimizes long-term endoplasmic reticulum function to protect cells from chronic stress. Dev. Cell 13, 351–364. doi: 10.1016/j.devcel.2007.07.005
Xu, T. T., Zhang, Y., He, J. Y., Luo, D., Luo, Y., Wang, Y. J., et al. (2018). Bajijiasu Ameliorates beta-Amyloid-Triggered Endoplasmic Reticulum Stress and Related Pathologies in an Alzheimer’s Disease Model. Cell Physiol. Biochem. 46, 107–117. doi: 10.1159/000488414
Yang, H., and Wu, Z. (2018). Genome Editing of Pigs for Agriculture and Biomedicine. Front. Genet. 9:360. doi: 10.3389/fgene.2018.00360
Zhang, J. S., Zhou, S. F., Wang, Q., Guo, J. N., Liang, H. M., Deng, J. B., et al. (2016). Gastrodin suppresses BACE1 expression under oxidative stress condition via inhibition of the PKR/eIF2alpha pathway in Alzheimer’s disease. Neuroscience 325, 1–9. doi: 10.1016/j.neuroscience.2016.03.024
Keywords: Alzheimer’s disease, apoptosis, endoplasmic reticulum stress, neuroprotection, unfolded protein response
Citation: Gao X and Xu Y (2021) Therapeutic Effects of Natural Compounds and Small Molecule Inhibitors Targeting Endoplasmic Reticulum Stress in Alzheimer’s Disease. Front. Cell Dev. Biol. 9:745011. doi: 10.3389/fcell.2021.745011
Received: 21 July 2021; Accepted: 13 August 2021;
Published: 01 September 2021.
Edited by:
Yongye Huang, Northeastern University, ChinaCopyright © 2021 Gao and Xu. This is an open-access article distributed under the terms of the Creative Commons Attribution License (CC BY). The use, distribution or reproduction in other forums is permitted, provided the original author(s) and the copyright owner(s) are credited and that the original publication in this journal is cited, in accordance with accepted academic practice. No use, distribution or reproduction is permitted which does not comply with these terms.
*Correspondence: Yuanyuan Xu, eHV5dWFuQGpsdS5lZHUuY24=