- 1Department of Surgical Oncology, Sir Run Run Shaw Hospital, Zhejiang University, Hangzhou, China
- 2School of Medicine, Zhejiang University, Hangzhou, China
- 3Department of Thyroid and Breast Surgery, Taizhou Hospital of Zhejiang Province Affiliated to Wenzhou Medical University, Luqiao, China
Aberrant methylation has been regarded as a hallmark of cancer. 5-hydroxymethylcytosine (5hmC) is recently identified as the ten-eleven translocase (ten-eleven translocase)-mediated oxidized form of 5-methylcytosine, which plays a substantial role in DNA demethylation. Cell-free DNA has been introduced as a promising tool in the liquid biopsy of cancer. There are increasing evidence indicating that 5hmC in cell-free DNA play an active role during carcinogenesis. However, it remains unclear whether 5hmC could surpass classical markers in cancer detection, treatment, and prognosis. Here, we systematically reviewed the recent advances in the clinic and basic research of DNA 5-hydroxymethylation in cancer, especially in cell-free DNA. We further discuss the mechanisms underlying aberrant 5hmC patterns and carcinogenesis. Synergistically, 5-hydroxymethylation may act as a promising biomarker, unleashing great potential in early cancer detection, prognosis, and therapeutic strategies in precision oncology.
Introduction
Carcinogenesis is a multistep and complex process in which both genetic and epigenetic aberrations play substantial roles (Feinberg et al., 2016; Thomson and Meehan, 2017). 5-methylcytosine (5mC) is a major form of DNA modification observed in most mammals’ CpG (Cytosine-phosphate-Guanine) dinucleotides (60–80%) (Smith and Meissner, 2013). The alteration of 5mC in DNA methylation patterns has been considered as a hallmark of cancer. Global hypomethylation leads to genomic instability, whereas hypermethylation at promoter regions leads to transcriptional silencing of tumor suppressor genes (TSGs), both of the above contributing significantly to the carcinogenesis (Sandoval and Esteller, 2012; Berdasco and Esteller, 2019). Additionally, hypomethylation and hypermethylation can regulate target gene expression by activating or silencing enhancers, respectively (Clermont et al., 2016; Ehrlich, 2019). These processes are dysregulated in many human cancers, leading to cellular de-differentiation and malignant transformation (Taberlay et al., 2014; Clermont et al., 2016). Along with 5mC, 5-hydroxymethylcytosine (5hmC) has been recently recognized as a stable modification that has multiple roles from cell pluripotency to carcinogenesis rather than merely intermedium of DNA demethylation (Putiri et al., 2014; Moen et al., 2015; López et al., 2017).
5hmC is the most abundant oxidation product of 5mC during active DNA demethylation that is mediated by ten-eleven translocase (TET) (Kriaucionis and Heintz, 2009; Tahiliani et al., 2009). TET enzymes oxidize 5mC to 5hmC, and further to 5-formylcytosine (5fC) and 5-carboxylcytosine (5caC) (Ito et al., 2011; He et al., 2011) in a 2-oxoglutarate (2-OG) and Fe (II)-dependent manner (Kohli and Zhang, 2013; Koivunen and Laukka, 2018) (Figure 1). 5hmC is found enriched over gene bodies, promoters, and enhancers upstream of transcriptional start sites, suggesting that 5hmC plays a role in the regulation of gene expression (Thomson and Meehan, 2017). 5hmC displays a cell- or tissue-specific distribution in different malignancies, and reduced global 5hmC levels were identified in multiple cancers (Lian et al., 2012; Orr et al., 2012; Chen et al., 2016; Tong et al., 2019; Wang et al., 2020), implying that 5hmC may have a promising value in cancer screen, diagnosis, progression and survival (Lian et al., 2012; Zhang et al., 2016a; Zhong et al., 2018). Moreover, 5hmC is suggested as a potential target to synergize with conventional treatment of cancer (Thomson and Meehan, 2017). Thus, 5hmC could be considered as a robust biomarker in clinical practice and promote precision medicine, although awaiting further validation.
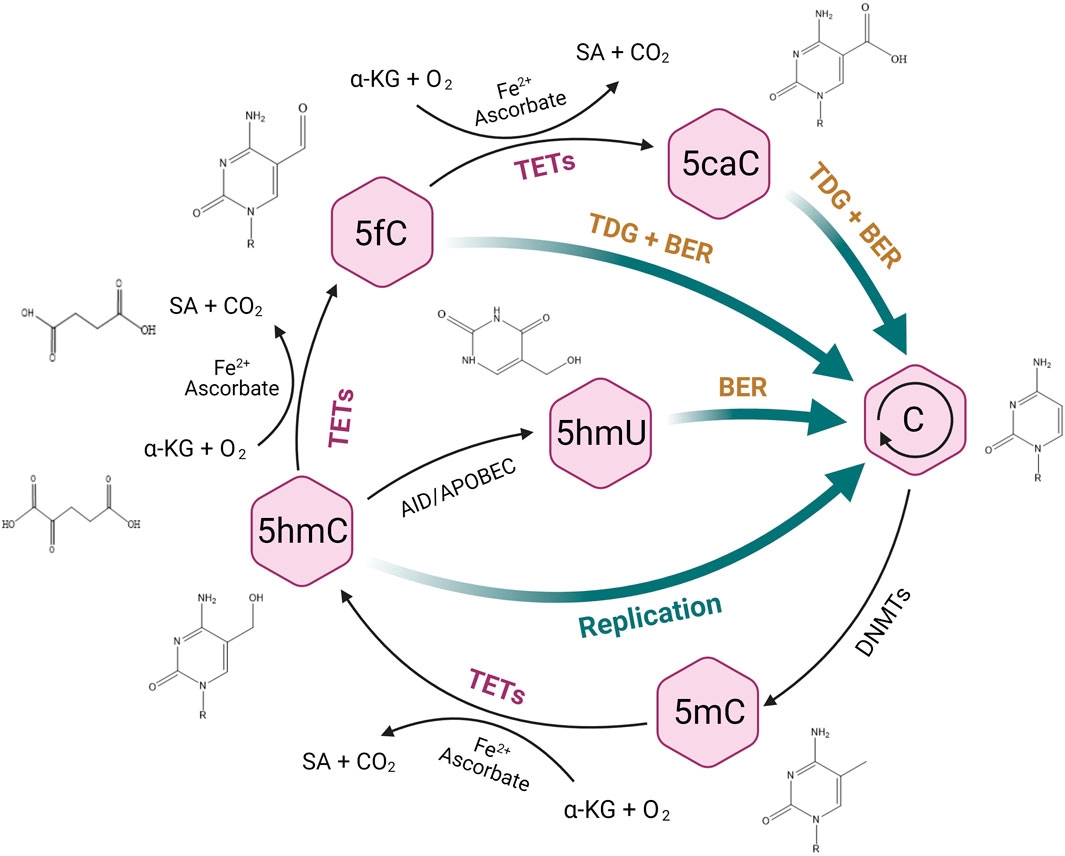
FIGURE 1. TET-mediated DNA demethylation process. TET enzymes can oxidize 5-methylcytosine (5mC) to 5-hydroxymethylcytosine (5hmC), and further to 5-formylcytosine (5fC) and 5-carboxylcytosine (5caC), followed by the excision of 5fC and 5caC via thymine DNA glycosylase (TDG) and the replacement of unmethylated cytosine through base-excision repair (BER). 5hmC can also be deaminated to 5-hydroxymethyluracil (5hmU) by AID/APOBEC enzymes and subsequently to an unnmethylated status by BER.
Liquid biopsy, along with the detection of circulating tumor DNA (ctDNA) has been revolutionizing the cancer screen and treatment (Tuaeva et al., 2019). ctDNA is tumor-derived fraction of circulating cell-free DNA (cfDNA) that can reflect both genetic and epigenetic profiles of cancer (Wan et al., 2017) (Figure 2). The ctDNA-based liquid biopsy offers substantial advantages over traditional biopsies (Chen and Zhao, 2019; De Rubis et al., 2019; Shohdy and West, 2020). First, it is minimally invasive and it avoids the risks of tissue biopsy such as tumor seeding (Diaz and Bardelli, 2014). Second, it could provide dynamic surveillance of genetic or epigenetic changes during cancer development (Diaz and Bardelli, 2014; Wan et al., 2017). Third, it could help capture the heterogeneity of the tumor, as ctDNA fragments are derived from all clones of the cancer (Diaz and Bardelli, 2014). The detection of 5hmC in cfDNA has been reported and it is increasingly fueled by the development of high-throughput sequencing methodology (Li et al., 2017). For instance, considerable researchers used 5hmC-Seal method to capture 5hmC patterns in colorectal, gastric, hepatocellular and lung cancers (Li et al., 2017; Zhang et al., 2018; Cai et al., 2019). However, 5hmC as a biomarker in liquid biopsy of cancer remains unclear, which warrants more exploration.
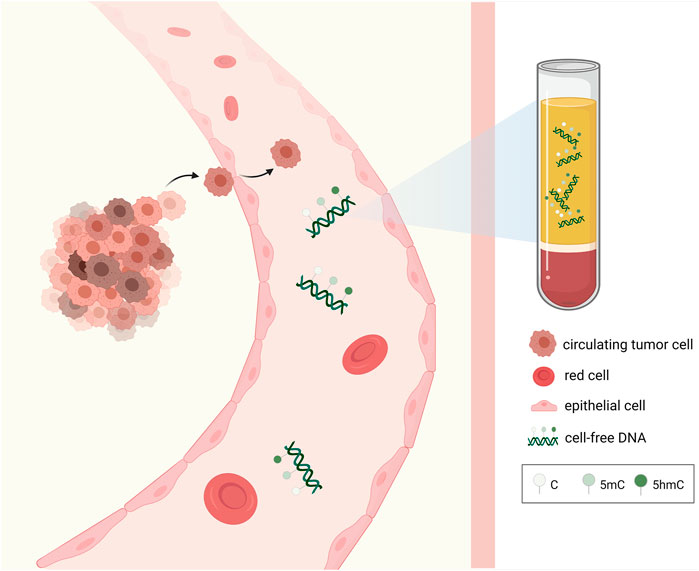
FIGURE 2. Epigenetic alterations of cell-free DNA extracted from the blood. Tumor-derived epigenetic alterations including 5mC, 5hmC of cfDNA obtained from the blood via liquid biopsy can be utilized as novel biomarkers for cancer diagnosis, prognosis, and treatment.
In this review, we focus on the value of 5hmC detection in cfDNA as an emerging biomarker in cancer management and possible mechanisms. Specifically, we discuss how 5hmC exerts its role in the early detection, prognosis, and treatment of various malignancies. We further overview the mechanisms underlying aberrant 5hmC patterns, TETs, and carcinogenesis. Notably, we emphasize that cfDNA-based liquid biopsy combined with genome-wide sequencing of 5hmC patterns may serve as an efficient tool to implement 5hmC into clinical applications in the era of precision medicine.
Deoxyribonucleic Acid 5-Hydroxymethylation Promotes Early Detection of Cancer
Early detection of cancer leads to better outcome (Wan et al., 2017; Shohdy and West, 2020). The detection of epigenetic alterations including 5hmC could promote early detection of cancer (Jankowska et al., 2015). 5hmC modification is tissue-specific and disease-specific (Laird et al., 2013; Sproul and Meehan, 2013; Jankowska et al., 2015), and has been reported in the process of multiple precancerous pathogenesis. For instance, the increase and decrease of 5hmC level were respectively associated with the cervical precancerous lesions (Su et al., 2019) and the breast precancerous lesions (Zhang et al., 2020). 5hmC was also involved in hepatocellular carcinoma (HCC), melanoma and esophageal cancer (Lian et al., 2012; Tian et al., 2018; Liu et al., 2019a; Krais et al., 2019). Furthermore, as cfDNA can be identified in a minimal invasively and dynamically way in body fluids, recent studies demonstrated that 5hmC in cfDNA could act as a novel biomarker in cancer detection (Li et al., 2017; Song et al., 2017), taking a substantial step toward precision medicine.
5hmC in cfDNA has been detected in multiple malignancies, including HCC (Cai et al., 2019), colorectal cancer (CRC) (Gao et al., 2019), lung cancer (Zhang et al., 2018), gastric cancer (Li et al., 2017) and pancreatic ductal adenocarcinoma (PDAC) (Song et al., 2017; Guler et al., 2020). A reduced global 5hmC level in HCC was found to be positively correlated with tumor stage (Chen et al., 2013; Chen et al., 2017; Liu et al., 2019a) and it could be observed even in chronic hepatitis without significant methylation (Wang et al., 2019). Cai et al. reported the promising value of 5hmC in cfDNA in early detection of HCC (Cai et al., 2019). The research analyzed the genome-wide 5hmC patterns in cfDNA among 2,554 Chinese HCC patients, showing that the 5hmC profile in cfDNA could effectively distinguish early HCC patients from healthy controls and liver cirrhosis. Moreover, the research designed a weighted model comprised of a 32-gene-based 5hmC marker panel, which is proved to outperform alpha-fetoprotein (AFP) in early detection of HCC (Cai et al., 2019). Likewise, lung cancer also exhibits a global loss of 5hmC (Jin et al., 2011). Song et al. found that the global level of 5hmC in cfDNA progressively decreased from early-stage lung cancer to metastatic lung cancer, indicating that 5hmC in cfDNA could contribute to the early detection and surveillance of lung cancer (Song et al., 2017). Another study based on a Chinese cohort reported that 5hmC of specific genes in cfDNA have a higher sensitivity than traditional biomarkers, including CEA, CA125, NSE, and CYFRA21-1 in the early detection of non-small cell lung cancer (NSCLC) (Zhang et al., 2018). However, 5hmC pattern in cfDNA from this study was found to exhibit global gains in gene bodies as well as promoter regions in NSCLC patients (Zhang et al., 2018). Guler et al. revealed that 5hmC peaks of cfDNA in promoter region in PDAC patients were lower than those in non-cancer patients, whereas it was significantly higher over 3′UTR, transcription termination sites (TTS) and intron regions in PDAC patients, respectively. 5hmC profile in PDAC tissues was reported could classify PDAC cfDNA, which indicates the cfDNA could mirror the 5hmC enrichment observed in tumor (Guler et al., 2020). Another study reported that 5hmC peaks gained in cfDNA were present in the gene body of representative genes in colorectal cancer tissues, compared with patients of precancerous adenomas and healthy donors, such as BCL11A, KCNK9 and NTSR1 (Gao et al., 2019). Similarly, Xiao et al. determined the genome-wide distribution of 5hmC in cfDNA, indicating that the CRC patients have the widest distribution of 5hmC, whereas the healthy donors own the narrowest distribution, and the precancerous adenomas have the moderate distribution (Xiao et al., 2021). 5hmC in cfDNA was also reported associating with molecular subtypes in non-Hodgkin lymphoma (Chiu et al., 2021). Therefore, accumulating evidences indicate that 5hmC in cfDNA, both the distribution and global level, could be served as robust biomarkers in early detection of cancer, though further research is still warranted.
Despite the fact that cfDNA could be identified in the blood, only a small fraction is from the tumor and it remains difficult to identify aberrant epigenetic profiles precisely (Jankowska et al., 2015; Shen et al., 2018). Specifically, though 5hmC profiles are apparent in tumors at an early stage, it is still challenging to detect them by next-generation sequencing, as ctDNA itself is at an imperceptible level, especially in early stage cancer (Locke et al., 2019; Kilgour et al., 2020). It is reported that ctDNA in asymptomatic cancer is not detectable based on current methodology using typical 10 ml of blood, hindering its application in cancer early detection (Fiala and Diamandis, 2018). However, the emerging 5hmC-Seal technology has been utilized to capture genome-wide profiling of 5hmC and it proved to be a highly sensitive and robust approach for genome-wide profiling of 5hmC that requires only very limited input of DNA (< 10 ng) (Han et al., 2016; Applebaum et al., 2020; Guler et al., 2020). Also, other innovative methodologies permit the implementation of ctDNA in early-stage cancer detection (Liu et al., 2019b; Hu et al., 2019). Therefore, although 5hmC in ctDNA is difficult to detect, rapidly evolving methodology permit its application in early detection of cancer.
Deoxyribonucleic Acid 5-Hydroxymethylation Predicts Cancer Prognosis
In the era of precision medicine, increasing studies demonstrated the potential of 5hmC, TET protein family as potential clinical biomarkers to predict the prognosis of cancer, however, the evidence remains inadequate.
The decrease of 5hmC level is closely correlated with poor prognosis in multiple malignancies, including HCC (Liu et al., 2013; Liu et al., 2014; Liu et al., 2019a), CRC (Zhong et al., 2018), NSCLC (Liao et al., 2016), breast cancer (Tsai et al., 2015), gastric cancer (Yang et al., 2013; Frycz et al., 2014), papillary thyroid carcinoma (PTC) (Tong et al., 2019), glioblastomas (GBM) (Orr et al., 2012), esophageal carcinoma (EC) (Shi et al., 2016), cholangiocarcinoma (CCA) (Dong et al., 2015; Bai et al., 2021). In HCC, it has been reported that loss of 5hmC was positively correlated with tumor size, AFP level as well as poor overall survival (Liu et al., 2013). In patients with breast cancer, especially the ER/PR-negative subtype, the reduction of 5hmC was found strongly associated with poor disease-specific survival (DSS) and disease-free survival (DFS) (Tsai et al., 2015). In patients with PTC, one study revealed that the level of 5hmC was lower in those with lymph node metastasis than those without, which indicated that 5hmC may be a useful tool in predicting the lymph node involvement of PTC (Tong et al., 2019). Moreover, the reduction of 5hmC level showed its prognostic value in WHO grade II diffuse astrocytomas (DA) and WHO grade IV GBM (Kraus et al., 2015; Zhang et al., 2016a; Johnson et al., 2016). Low 5hmC was correlated with increased mortality and poor survival in patients with GBM (Orr et al., 2012), and it could act as a biomarker in predicting tumor infiltration instead of ki67 and H3S10p (Kraus et al., 2015). It has been revealed that the loss of 5hmC may affect normal gene transcription, which could in turn promote abnormal proliferation of tumor cells, and eventually affect the survival in cervical squamous cell carcinoma (CSCC) (Zhang et al., 2016b). On the other hand, high level of 5hmC was associated with less invasive tumor phenotype and better prognosis in colon cancer (Zhong et al., 2018), and it was also displayed as a predictor of post-operative recurrence in TMPRSS2-ERG negative prostate cancer (Strand et al., 2015). Therefore, the detection of 5hmC levels in various cancers serves as a promising approach in predicting cancer prognosis.
The detection of 5hmC signature in cfDNA may provide a novel tool in predicting cancer outcomes. For instance, Song et al. observed a gradual decrease of 5hmC in cfDNA in early stage and advanced metastatic lung cancer, suggesting that such stage-dependent 5hmC loss in cfDNA may be a powerful tool for outcome prediction (Song et al., 2017). Cai et al. reported a novel HCC score predicting the prognosis of HCC, which consisted of cfDNA, 5hmC, and protein biomarkers [des-gamma-carboxy prothrombin (DCP) and AFP]. The results indicated that a higher HCC score was positively associated with the risk of recurrence and metastasis (Cai et al., 2021). In addition to the global level of 5hmC, the distribution of 5hmC in cfDNA also process a prognostic value. For instance, Chiu et al. reported that in diffuse large B-cell lymphoma (DLBCL), the distinct 5hmC profile in cfDNA could reflect origin of cell, thereby distinguishing different subtypes of DLBCL, and it was associated with the events of recurrence, retreatment, or death in DLBCL (Chiu et al., 2019; Chiu et al., 2021). Collectively, these studies indicate that 5hmC has a great potential as a biomarker of cancer progression, while the detection of 5hmC in cfDNA could contribute to the development of liquid biopsy in cancer.
In summary, DNA 5-hydroxymethylation in the genome is a novel prognostic biomarker in multiple cancers. The detection of 5hmC in cfDNA could help predict the outcomes of patients with cancer. However, the research on the detection of 5hmC still needs to be explored unremittingly by researchers in the future.
Targeting Deoxyribonucleic Acid 5-Hydroxymethylation in Cancer
Targeting DNA demethylation has great potential in cancer treatment. Lian et al. suggested that feasible manipulation of overexpressing TET2 could restore the 5hmC level and further suppress tumor growth and invasion in melanoma, unlatching a novel therapeutic avenue for melanoma by targeting 5hmC generating pathway to reestablish 5hmC levels or landscape in melanoma cells (Lian et al., 2012). Another study showed that TET2 plays a key role in controlling the numbers and survival of slow-cycling cancer cells (SCCCs) through controlling the expression of cell death-related genes that regulate TNF-α signaling and restraining its proapoptotic signaling (Puig et al., 2018). Slow-cycling is a temporary state preserving potential cancer initiation and increased chemo-resistance. High TET2 activity and 5hmC level were associated with resistance to chemotherapy, thereby increasing the risk of recurrence of patients. Thus, 5hmC could be served as a biomarker for identifying the increased chemo-resistant SCCCs and TET2 could be potentially targeted for treatment of SCCCs (Puig et al., 2018). Interestingly, high expression of TET1 leads to global hydroxymethylation in T-cell acute lymphoblastic leukemia (T-ALL), which protects cells from DNA damage and thereby promoting leukemic growth. Bamezai et al. outlined that PARP could induce TET1 expression, thus TET1 could be pharmacologically targeted via application of PARP inhibitor Olaparib, which showed great therapeutic potential in T-ALL treatment (Bamezai et al., 2021). Fisetin, a natural flavonol, was reported to inhibit the activity of TET1, reduce 5hmC levels in CCNY/CDK16 promoter, and further restrain the invasion and progression of renal cancer stem cells. It indicated that TET1 could be targeted and 5hmC could be a potential indicator for the treatment of fisetin in renal cancer (Si et al., 2019). Besides, Vitamin C, a cofactor for TETs to enhance 5hmC conversion, is noticeably involved in the process of DNA demethylation (Dickson et al., 2013; Minor et al., 2013; Sant et al., 2018). Sant et al. reported that the reduced expression of Na+-dependent Vitamin C transporter 2 (SVCT2) was a primary cause of 5hmC loss in breast cancer (Sant et al., 2018). Treatment of vitamin C might make up for the deficiency of SVCT2, thus restoring 5hmC levels via activating TET activity, and inducing apoptosis in breast cancer cells (Sant et al., 2018). Moreover, it has been suggested that 5hmC or 5hmC/5mC ratio might be a potential biomarker of differentiation and proliferation in pituitary neuroendocrine tumor, which could also be used as a biomarker of the effect of decitabine (Szabó et al., 2020). Therefore, we propose that 5hmC in cancer treatment could be a promising target, although more research is warranted.
5hmC could be a biomarker in cancer immunotherapy, especially in predicting immune cell exhaustion and immune evasion. Johnson et al. found a significant age-related differentially hydroxymethylated region occurred in the intronic region of TOX2, which is associated with CD8+ T cell exhaustion. It belongs to a family of transcription factors that modify chromatin structure during T cell development (Johnson et al., 2020). Researchers reported that circTRIM33-12 could inhibit immune evasion of HCC by sponging oncogenic miR-191 and upregulating TET1 expression, which lead to a significant increase of 5hmC levels (Wang et al., 2019). Similarly, Taylor et al. found that the immune evasion subtype of lung squamous cell carcinoma has significant enhanced EGFR signaling, which further boosted the expression of the transcription factor ETS1. ETS1 could upregulate miR-29b, which result in the downregulation of TET1 and subsequent reduction of 5hmC level (Taylor et al., 2016). In another study, TET2 was reported promoting tumor progression in melanoma via ARG1, an immunosuppressive gene in tumor-associated macrophages. ARG1 embraced a low level of 5hmC in Tet2-null bone marrow derived macrophages (BMDM) (Pan et al., 2017). Targeting TET2 and its interactors (e.g., Fe2+, 2-OG, Vitamin C) could regulate the activity of B cells, T cells, and macrophages, which could contribute to immunotherapy of cancer (Jiang, 2020). Luchtel et al. reported that ascorbic acid treatment could increase the level of 5hmC in CD8+ T cells and reinforce their cytotoxicity against lymphoma cells (Luchtel et al., 2020). Therefore, the 5-hydroxymethylation of DNA could be a promising biomarker and a target of cancer immunotherapy, although further studies are required.
Targeting different oncogenes in the pathways of DNA demethylation, including TETs and its cofactors, may be potential strategies in cancer treatment in the future. 5hmC in these processes can be taken as a valuable biomarker for the response to multiple therapies. In terms of immunotherapy of cancer, 5hmC provides a new approach for immunotherapy-sensitive tumors, especially in overcoming immune cell exhaustion and cancer immune evasion. Although TET or 5hmC plays a critical role in cancer treatment, the involvement of TET and 5hmC in inhibiting cancer development is still far from clarified. Some attempts have been undertaken to develop pharmaceutical drugs targeting TET or its correlated signaling pathways in cancer development. For instance, TET1 has been considered as a critical oncogenic epigenetic regulator in acute myeloid leukemia (AML), which plays its role by promoting the expression of oncogenic targets, including HOXA9, MEIS1 and PBX3, and suppressing the expression of tumor suppressor miR-22 (Huang et al., 2013; Huang et al., 2016; Jiang et al., 2016). Jiang et al. found that application of selective inhibitors (NSC-370284 and UC-514321) could directly target STAT/TET1 signaling, which was an effective and promising therapeutic strategy to treat TET1-high AML, especially when combined with standard chemotherapy (Jiang et al., 2017). Therefore, targeting TET1 signaling might be a considerable strategy in AML treatment. Moreover, TET1 and 5hmC were reported associating with an obesity-linked pathway driving cancer stem cells in triple-negative breast cancer, which providing new understanding on the cancer prevention and treatment (Bao et al., 2020). With the development of liquid biopsy, the detection of cfDNA provides new pathways for cancer treatment (Goodall et al., 2017; Siravegna et al., 2017). For instance, a recent study investigated the alteration of 5hmC profiles during the treatment of neuroblastoma. The study showed that 5hmC profiles in cfDNA changed over time, paralleled with changes in metastatic disease burden as well as patients’ response to therapy (Applebaum et al., 2020). Thus, the detection of 5hmC profiles in cfDNA may be a promising biomarker that can be widely applied in monitoring the response to cancer treatment in future.
Mechanisms Underlying Deoxyribonucleic Acid 5-Hydroxymethylation in Cancer
Although the alteration of DNA 5-hydroxymethylation can be utilized as potential biomarkers in cancer screen, prognosis, and treatment, the mechanisms underlying these epigenetic alterations remain obscure. Exploring how the alteration of TETs and 5hmC play roles in tumorigenesis and tumor progression could accelerate the development of cancer therapy.
The abnormal expression of TETs, or inhibition of TETs cofactors lead to the alteration in the level and localization of 5hmC, impacting the cancer development and anti-tumor immunity (Koivunen and Laukka, 2018) (Figure 3). Compared with TET2 which is frequently mutated in hematopoietic malignancies (Huang and Rao, 2014; Jeschke et al., 2016), TET1 mutation is a rare occurrence in cancer (Thomson and Meehan, 2017). Instead it is found both methylated and transcriptionally suppressed in vitro in multiple malignancies, though whether it is the cause of hypermethylation is still unclear (Wu and Brenner, 2014). In addition to the genetic or transcriptomic disruption, the activity of TETs can be regulated by its substrates, cofactors and post-translational modifications (Thomson and Meehan, 2017). It has been found that patients with hematological malignancies and gliomas often harbor mutations in IDH1 an IDH2, which can convert 2-OG into 2-hydroxyglutaraye (2-HG) and thereby inhibit the catalytic activity of TET (Figueroa et al., 2010; Xu et al., 2011). The loss of 5hmC in cancer can be further explained by the post-transcriptional regulation of TET. For instance, the IDAX protein, an interacting partner of TET2 that was often proved to be mutated in tumors, leads to TET2 degradation via caspase activation (Ko et al., 2013). However, decrease in hydroxymethylation in promoters of tumor suppressor genes is scarcely reported during carcinogenesis, which may require further investigations.
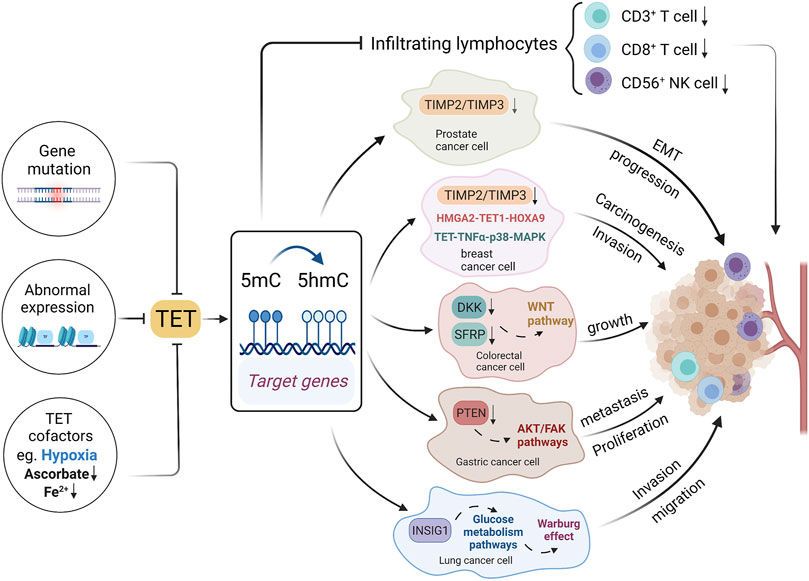
FIGURE 3. Mechanisms underlying 5hmC and TET alterations in cancer. Gene mutations, abnormal expression and cofactors could affect TET expressions, thereby affecting DNA hydroxymethylation. The TET gene family could affect the tumor infiltrating lymphocyte, and it could also affect tumor development through multiple signal pathways.
TET1 is usually known as a tumor suppressor, which could inhibit cancer progression via various pathways (Hsu et al., 2012; Fu et al., 2014; Neri et al., 2015; Yan et al., 2020). TET1 directly binds to the promoter of the target genes, catalyzing 5mC to 5hmC and ultimately activating gene transcription (Pei et al., 2016). In accordance with this mechanism, one study showed that the downregulation of TET1 could reduce the hydroxymethylation at the promoter of tumor suppressor gene AJAP1, subsequently activating WNT/β-catenin pathway and promoting the development of bladder cancer (Yan et al., 2020). TET1 loss in prostate cancer and breast cancer was found contributing to the hypermethylation of tissue inhibitors of metalloproteinases genes (TIMP2 and TIMP3), which resulted in the epithelial-mesenchymal transition (EMT) and tumor progression (Hsu et al., 2012). Another study reported the HMGA2-TET1-HOXA9 axis could regulate breast cancer cell invasion, and it holds a prognostic signature in predicting patient survival (Sun et al., 2013). TET1 is negatively regulated by HMGA2, a chromatin remodeling factor that is highly expressed in most epithelial cancers. TET1 positively modulated HOXA9 by directly binding to or inducing the histone binding of H3K4Me3 to the HOXA gene promoter regions, leading to local demethylation and gene transcription in breast cancer. Additionally, the downregulation of TET1 was reported to occur at the early stage and its expression could inhibit the proliferation of cancer cells in CRC (Neri et al., 2015). TET1-mediated demethylation induces a 5hmC increase at the promoter regions of DKK and SFRP genes, which were upstream inhibitors of WNT signaling pathway, hence TET downregulation can lead to cancer growth via repressing these inhibitors of WNT pathway (Neri et al., 2015). Similar mechanism was also reported in pancreatic cancer (Wu et al., 2019). Likewise, TET1 inhibits proliferation and metastasis of gastric cancer cells through demethylating PTEN promoter regions, which subsequently suppress the PTEN-inhibited AKT and FAK pathways (Pei et al., 2016). Besides DNA 5-hydroxymethylation mediated by TETs in tumor-suppressor genes, several oncogenes which encompass an acquisition of 5hmC were also reported. One study demonstrates that the 5hmC landscape in pancreatic ductal adenocarcinoma (PDAC) peaks in the gene that encodes the bromodomain-containing transcription factor BRD4, overlapping with active enhancer marks such as H3K4me1, and 5hmC acquisition by BRD4 enhancer is found to be a potential mechanism for regulating oncogenic activation (Bhattacharyya et al., 2017). In one word, 5hmC under the regulation of TETs is involved in the initiation and progression of tumors via various pathways, which is of great significance for its status as a promising biomarker in the field of precise oncology.
Besides, it is exciting to find that TETs and 5hmC changes are involved in immune signaling pathways, which may boost antitumor immunity. One study reported that in basal-like breast cancer (BLBC), the activation of nuclear factor кB (NF-кB) could suppress TET1 by binding to its promoter, highlighting a novel epigenetic-immunity connection (Collignon et al., 2018). NF-кB is a transcription factor involved in the activation of immune cells and regulation of immune signaling pathways (Gerondakis and Siebenlist, 2010; Hoesel and Schmid, 2013). Compared to TET1-high patients, low TET1 expression and subsequent 5hmC loss were associated with high infiltration of immune cells and better survival in BLBC (Collignon et al., 2018). Thus, the level of 5hmC could act as a marker of the immune response targeting tumor cells. TET2 also plays a substantial role in antitumor immunity. One study demonstrated that TET2 depletion could reduce Th1-type chemokines and PD-L1 expression by mediating the IFN-γ/JAK/STAT pathway, thereby decreasing tumor-infiltrating lymphocytes such as CD3+ and CD8+ T cell, and eventually it could compromise the efficacy of anti-PD-1/PD-L1 immunotherapy (Xu et al., 2019). Moreover, the 5hmC level in this study was positively correlated with the expression of chemokines and PD-L1 in tumor cells, infiltration of antitumor immune cells including CD3+ T cells, CD8+ T cells, and CD56+ NK cells, and the response towards anti-PD-1/PD-L1 therapy. Thus, TETs and 5hmC could potentially be predictors of antitumor immunity, which may help boost the immunotherapy of cancer.
Oxygen is an essential element of TETs catalysis during demethylation (Schito and Semenza, 2016). Several studies have reported the association between TETs activity and hypoxia in different cancer (Mariani et al., 2014; Tsai et al., 2014; Wu et al., 2015). One study reported that hypoxia could reduce 5hmC levels in tumor-suppressor gene promoters by directly impairing TET oxidative activity in oncogenic process (Thienpont et al., 2016). However, in another study, hypoxia could conversely increase the expression of TET1, TET3 as well as 5hmC level in promoters, leading to carcinogenesis and poor prognosis of breast cancer patients via a TET-TNFα-p38-MAPK signaling (Wu et al., 2015). Interestingly, it was also reported that hypoxia-induced TET1 was associated with lipid metabolism in inducing EMT and cancer metastasis. ‘Hypoxia-induced TET1 could increase the 5hmC level in the promoter of insulin induced gene 1 (INSIG1), the main regulator of cholesterol biosynthesis (Tsai et al., 2014). The knockdown of INSIG1 could abolish the activation of mesenchymal genes, mitigating the activity of migration and invasion of lung cancer cells, and it could even decrease gene expression in the glucose metabolism pathway that contribute to the hypoxia-induced Warburg effect in tumor cells (Tsai et al., 2014). Thus, TET1 may play a crucial role in regulating cholesterol and glucose metabolism in tumor cells, although the mechanisms need to be further explored. In summary, the relationship between aberrant 5hmC patterns and carcinogenesis relies on both transcriptional state of TETs and the hypoxic environment in cancer cells (Thomson and Meehan, 2017). With further exploration of the mechanisms underlying 5hmC and carcinogenesis, the identification of new therapeutic targets and medications could be a promising field in the precise medicine of cancer.
Conclusion and Perspective
In this review, we overviewed the current research on use of DNA hydroxymethylation as a novel biomarker in the era of precision medicine. 5hmC has been highly prevailing in recent years in cancer diagnosis and prognosis. Different from genetic mutations encoded in DNA sequence, DNA hydroxymethylation is potentially reversible, which holds great promise in cancer treatment by using pharmaceutical intervention to recover normal epigenetic patterns (Thomson and Meehan, 2017). It is worth considering to effectively translate cancer-associated 5hmC patterns into clinical practice in the field of oncology.
With the development of genome-wide sequencing assays in the last few years, 5hmC profiling can be detected via ctDNA or cfDNA-based liquid biopsies, which are effective, minimally invasive tools for cancer early detection and progression surveillance. Although several studies demonstrated the detection methods of 5hmC signatures in cfDNA remain crude (Zeng et al., 2019). Another challenge is that circulating DNA from scarce tumor sub-populations can only be shown at an extremely low level in cfDNA, restraining its detection (Chen et al., 2013). This should be considered when designing novel assays and evaluating the role of 5hmC patterns in cancer by liquid biopsy. Overall, 5hmC could be a robust and pivotal biomarker in the era of liquid biopsy and precision medicine.
Author Contributions
LW, FC, QW designed the review. LX researched the literature and drafted the manuscript. AB, JC, YZ, LC, MM, SJ, LC, CC, ZL, XZ, FC edited the manuscript. All authors read and approved the final manuscript.
Funding
The work was supported by the National Natural Science Foundation of China (No. 81972453, No. 81972597). Zhejiang Provincial Medical and Health Science and Technology (Youth Talent Program) Project No. 2020393822.The work was sponsored by Zheng Shu Medical Elite Scholarship Fund.
Conflict of Interest
The authors declare that the research was conducted in the absence of any commercial or financial relationships that could be construed as a potential conflict of interest.
Publisher’s Note
All claims expressed in this article are solely those of the authors and do not necessarily represent those of their affiliated organizations, or those of the publisher, the editors and the reviewers. Any product that may be evaluated in this article, or claim that may be made by its manufacturer, is not guaranteed or endorsed by the publisher.
Abbreviations
5mC, 5-methylcytosine; TSGs, Tumor suppressor genes; 5hmC, 5-hydroxymethylcytosine; TET, ten-eleven translocase; 5fC, 5-formylcytosine; 5caC, 5-carboxylcytosine; 2-OG, 2-oxoglutarate; ctDNA, circulating tumor DNA; cfDNA, cell-free DNA; HCC, hepatocellular carcinoma; CRC, colorectal cancer; AFP, alpha-fetoprotein; NSCLC, non-small cell lung cancer; PDAC, pancreatic ductal adenocarcinoma; TTS, transcription termination sites; PTC, papillary thyroid carcinoma; GBM, glioblastomas; EC, esophageal carcinoma; CCA, cholangiocarcinoma; DSS, disease-specific survival; DFS, disease-free survival; DA, diffuse astrocytomas; CSCC, cervical squamous cell carcinoma; DCP, des-gamma-carboxy prothrombin; DLBCL, diffuse large B-cell lymphoma; SCCCs, slow-cycling cancer cells; AML, acute myeloid leukemia; SVCT2 Na+-dependent Vitamin C transporter 2; BMDM, bone marrow derived macrophages; TIMP, tissue inhibitors of metalloproteinases; EMT, epithelial-mesenchymal transition; PDAC, pancreatic ductal adenocarcinoma; BLBC, basal-like breast cancer; NF-кB, nuclear factor кB; TDG, Thymine DNA glycosylase; BER, base-excision repair; 5hmU, 5-hydroxymethyluracil.
References
Applebaum, M. A., Barr, E. K., Karpus, J., West-Szymanski, D. C., Oliva, M., Sokol, E. A., et al. (2020). 5-Hydroxymethylcytosine Profiles in Circulating Cell-free DNA Associate with Disease Burden in Children with Neuroblastoma. Clin. Cancer Res. 26 (6), 1309–1317. doi:10.1158/1078-0432.CCR-19-2829
Bai, X., Zhang, H., Zhou, Y., Nagaoka, K., Meng, J., Ji, C., et al. (2021). Ten‐Eleven Translocation 1 Promotes Malignant Progression of Cholangiocarcinoma with Wild‐Type Isocitrate Dehydrogenase 1. Hepatology 73 (5), 1747–1763. doi:10.1002/hep.31486
Bamezai, S., Demir, D., Pulikkottil, A. J., Ciccarone, F., Fischbein, E., Sinha, A., et al. (2021). TET1 Promotes Growth of T-Cell Acute Lymphoblastic Leukemia and Can Be Antagonized via PARP Inhibition. Leukemia 35 (2), 389–403. doi:10.1038/s41375-020-0864-3
Bao, B., Teslow, E. A., Mitrea, C., Boerner, J. L., Dyson, G., and Bollig-Fischer, A. (2020). Role of TET1 and 5hmC in an Obesity-Linked Pathway Driving Cancer Stem Cells in Triple-Negative Breast Cancer. Mol. Cancer Res. 18 (12), 1803–1814. doi:10.1158/1541-7786.mcr-20-0359
Berdasco, M., and Esteller, M. (2019). Clinical Epigenetics: Seizing Opportunities for Translation. Nat. Rev. Genet. 20 (2), 109–127. doi:10.1038/s41576-018-0074-2
Bhattacharyya, S., Pradhan, K., Campbell, N., Mazdo, J., Vasantkumar, A., Maqbool, S., et al. (2017). Altered Hydroxymethylation Is Seen at Regulatory Regions in Pancreatic Cancer and Regulates Oncogenic Pathways. Genome Res. 27 (11), 1830–1842. doi:10.1101/gr.222794.117
Cai, J., Chen, L., Zhang, Z., Zhang, X., Lu, X., Liu, W., et al. (2019). Genome-wide Mapping of 5-hydroxymethylcytosines in Circulating Cell-free DNA as a Non-invasive Approach for Early Detection of Hepatocellular Carcinoma. Gut 68 (12), 2195–2205. doi:10.1136/gutjnl-2019-318882
Cai, Z., Zhang, J., He, Y., Xia, L., Dong, X., Chen, G., et al. (2021). Liquid Biopsy by Combining 5-hydroxymethylcytosine Signatures of Plasma Cell-free DNA and Protein Biomarkers for Diagnosis and Prognosis of Hepatocellular Carcinoma. ESMO Open 6 (1), 100021. doi:10.1016/j.esmoop.2020.100021
Chen, K., Zhang, J., Guo, Z., Ma, Q., Xu, Z., Zhou, Y., et al. (2016). Loss of 5-hydroxymethylcytosine Is Linked to Gene Body Hypermethylation in Kidney Cancer. Cell Res 26 (1), 103–118. doi:10.1038/cr.2015.150
Chen, M.-L., Shen, F., Huang, W., Qi, J.-H., Wang, Y., Feng, Y.-Q., et al. (2013). Quantification of 5-methylcytosine and 5-hydroxymethylcytosine in Genomic DNA from Hepatocellular Carcinoma Tissues by Capillary Hydrophilic-Interaction Liquid Chromatography/quadrupole TOF Mass Spectrometry. Clin. Chem. 59 (5), 824–832. doi:10.1373/clinchem.2012.193938
Chen, M., and Zhao, H. (2019). Next-generation Sequencing in Liquid Biopsy: Cancer Screening and Early Detection. Hum. Genomics 13 (1), 34. doi:10.1186/s40246-019-0220-8
Chen, Q., Yin, D., Zhang, Y., Yu, L., Li, X.-D., Zhou, Z.-J., et al. (2017). MicroRNA-29a Induces Loss of 5-hydroxymethylcytosine and Promotes Metastasis of Hepatocellular Carcinoma through a TET-SOCS1-MMP9 Signaling axis. Cell Death Dis 8 (6), e2906. doi:10.1038/cddis.2017.142
Chiu, B. C.-H., Chen, C., You, Q., Chiu, R., Venkataraman, G., Zeng, C., et al. (2021). Alterations of 5-hydroxymethylation in Circulating Cell-free DNA Reflect Molecular Distinctions of Subtypes of Non-hodgkin Lymphoma. Npj Genom. Med. 6 (1), 11. doi:10.1038/s41525-021-00179-8
Chiu, B. C.-H., Zhang, Z., You, Q., Zeng, C., Stepniak, E., Bracci, P. M., et al. (2019). Prognostic Implications of 5-hydroxymethylcytosines from Circulating Cell-free DNA in Diffuse Large B-Cell Lymphoma. Blood Adv. 3 (19), 2790–2799. doi:10.1182/bloodadvances.2019000175
Clermont, P. L., Parolia, A., Liu, H. H., and Helgason, C. D. (2016). DNA Methylation at Enhancer Regions: Novel Avenues for Epigenetic Biomarker Development. Front. Biosci. (Landmark Ed. 21, 430–446. doi:10.2741/4399
Collignon, E., Canale, A., Al Wardi, C., Bizet, M., Calonne, E., Dedeurwaerder, S., et al. (2018). Immunity Drives TET1 Regulation in Cancer through NF-Κb. Sci. Adv. 4 (6), eaap7309. doi:10.1126/sciadv.aap7309
De Rubis, G., Rajeev Krishnan, S., and Bebawy, M. (2019). Liquid Biopsies in Cancer Diagnosis, Monitoring, and Prognosis. Trends Pharmacol. Sci. 40 (3), 172–186. doi:10.1016/j.tips.2019.01.006
Diaz, L. A., and Bardelli, A. (2014). Liquid Biopsies: Genotyping Circulating Tumor DNA. Jco 32 (6), 579–586. doi:10.1200/jco.2012.45.2011
Dickson, K. M., Gustafson, C. B., Young, J. I., Züchner, S., and Wang, G. (2013). Ascorbate-induced generation of 5-hydroxymethylcytosine is unaffected by varying levels of iron and 2-oxoglutarate. Biochemical and Biophysical Research Communications 439 (4), 522–527. doi:10.1016/j.bbrc.2013.09.010
Dong, Z.-R., Zhang, C., Cai, J.-b., Zhang, P.-F., Shi, G.-M., Gao, D.-m., Sun, H.-C., Qiu, S.-J., Zhou, J., Ke, A.-W., and Fan, J. (2015). Role of 5-hydroxymethylcytosine level in diagnosis and prognosis prediction of intrahepatic cholangiocarcinoma. Tumor Biol. 36 (4), 2763–2771. doi:10.1007/s13277-014-2900-2
Ehrlich, M. (2019). DNA hypermethylation in disease: mechanisms and clinical relevance. Epigenetics 14 (12), 1141–1163. doi:10.1080/15592294.2019.1638701
Feinberg, A. P., Koldobskiy, M. A., and Göndör, A. (2016). Epigenetic modulators, modifiers and mediators in cancer aetiology and progression. Nat Rev Genet 17 (5), 284–299. doi:10.1038/nrg.2016.13
Fiala, C., and Diamandis, E. P. (2018). Utility of circulating tumor DNA in cancer diagnostics with emphasis on early detection. BMC Med 16 (1), 166. doi:10.1186/s12916-018-1157-9
Figueroa, M. E., Abdel-Wahab, O., Lu, C., Ward, P. S., Patel, J., Shih, A., Li, Y., Bhagwat, N., Vasanthakumar, A., Fernandez, H. F., Tallman, M. S., Sun, Z., Wolniak, K., Peeters, J. K., Liu, W., Choe, S. E., Fantin, V. R., Paietta, E., Löwenberg, B., Licht, J. D., Godley, L. A., Delwel, R., Valk, P. J. M., Thompson, C. B., Levine, R. L., and Melnick, A. (2010). Leukemic IDH1 and IDH2 mutations result in a hypermethylation phenotype, disrupt TET2 function, and impair hematopoietic differentiation. Cancer cell 18 (6), 553–567. doi:10.1016/j.ccr.2010.11.015
Frycz, B. A., Murawa, D., Borejsza-Wysocki, M., Marciniak, R., Murawa, P., Drews, M., Kołodziejczak, A., Tomela, K., and Jagodziński, P. P. (2014). Decreased expression of ten-eleven translocation 1 protein is associated with some clinicopathological features in gastric cancer. Biomedicine & Pharmacotherapy 68 (2), 209–212. doi:10.1016/j.biopha.2013.12.011
Fu, H.-L., Ma, Y., Lu, L.-G., Hou, P., Li, B.-J., Jin, W.-L., et al. (2014). TET1 Exerts its Tumor Suppressor Function by Interacting with P53-EZH2 Pathway in Gastric Cancer. J. Biomed. Nanotechnology 10 (7), 1217–1230. doi:10.1166/jbn.2014.1861
Gao, P., Lin, S., Cai, M., Zhu, Y., Song, Y., Sui, Y., et al. (2019). 5‐Hydroxymethylcytosine Profiling from Genomic and Cell‐free DNA for Colorectal Cancers Patients. J. Cel Mol Med 23 (5), 3530–3537. doi:10.1111/jcmm.14252
Gerondakis, S., and Siebenlist, U. (2010). Roles of the NF- B Pathway in Lymphocyte Development and Function. Cold Spring Harbor Perspect. Biol. 2 (5), a000182. doi:10.1101/cshperspect.a000182
Goodall, J., Mateo, J., Yuan, W., Mossop, H., Porta, N., Miranda, S., et al. (2017). Circulating Cell-free DNA to Guide Prostate Cancer Treatment with PARP Inhibition. Cancer Discov. 7 (9), 1006–1017. doi:10.1158/2159-8290.cd-17-0261
Guler, G. D., Ning, Y., Ku, C.-J., Phillips, T., McCarthy, E., Ellison, C. K., et al. (2020). Detection of Early Stage Pancreatic Cancer Using 5-hydroxymethylcytosine Signatures in Circulating Cell Free DNA. Nat. Commun. 11 (1), 5270. doi:10.1038/s41467-020-18965-w
Han, D., Lu, X., Shih, A. H., Nie, J., You, Q., Xu, M. M., et al. (2016). A Highly Sensitive and Robust Method for Genome-wide 5hmC Profiling of Rare Cell Populations. Mol. Cel. 63 (4), 711–719. doi:10.1016/j.molcel.2016.06.028
He, Y.-F., Li, B.-Z., Li, Z., Liu, P., Wang, Y., Tang, Q., et al. (2011). Tet-mediated Formation of 5-carboxylcytosine and its Excision by TDG in Mammalian DNA. Science 333 (6047), 1303–1307. doi:10.1126/science.1210944
Hoesel, B., and Schmid, J. A. (2013). The Complexity of NF-Κb Signaling in Inflammation and Cancer. Mol. Cancer 12, 86. doi:10.1186/1476-4598-12-86
Hsu, C.-H., Peng, K.-L., Kang, M.-L., Chen, Y.-R., Yang, Y.-C., Tsai, C.-H., et al. (2012). TET1 Suppresses Cancer Invasion by Activating the Tissue Inhibitors of Metalloproteinases. Cel Rep. 2 (3), 568–579. doi:10.1016/j.celrep.2012.08.030
Hu, L., Liu, Y., Han, S., Yang, L., Cui, X., Gao, Y., et al. (2019). Jump-seq: Genome-wide Capture and Amplification of 5-Hydroxymethylcytosine Sites. J. Am. Chem. Soc. 141 (22), 8694–8697. doi:10.1021/jacs.9b02512
Huang, H., Jiang, X., Li, Z., Li, Y., Song, C.-X., He, C., et al. (2013). TET1 Plays an Essential Oncogenic Role in MLL-Rearranged Leukemia. Proc. Natl. Acad. Sci. 110 (29), 11994–11999. doi:10.1073/pnas.1310656110
Huang, H., Jiang, X., Wang, J., Li, Y., Song, C.-X., Chen, P., et al. (2016). Identification of MLL-fusion/MYC⊣miR-26⊣TET1 Signaling Circuit in MLL-Rearranged Leukemia. Cancer Lett. 372 (2), 157–165. doi:10.1016/j.canlet.2015.12.032
Huang, Y., and Rao, A. (2014). Connections between TET Proteins and Aberrant DNA Modification in Cancer. Trends Genet. 30 (10), 464–474. doi:10.1016/j.tig.2014.07.005
Ito, S., Shen, L., Dai, Q., Wu, S. C., Collins, L. B., Swenberg, J. A., et al. (2011). Tet Proteins Can Convert 5-methylcytosine to 5-formylcytosine and 5-carboxylcytosine. Science 333 (6047), 1300–1303. doi:10.1126/science.1210597
Jankowska, A. M., Millward, C. L., and Caldwell, C. W. (2015). The Potential of DNA Modifications as Biomarkers and Therapeutic Targets in Oncology. Expert Rev. Mol. Diagn. 15 (10), 1325–1337. doi:10.1586/14737159.2015.1084229
Jeschke, J., Collignon, E., and Fuks, F. (2016). Portraits of TET-Mediated DNA Hydroxymethylation in Cancer. Curr. Opin. Genet. Development 36, 16–26. doi:10.1016/j.gde.2016.01.004
Jiang, S. (2020). Tet2 at the Interface between Cancer and Immunity. Commun. Biol. 3 (1), 667. doi:10.1038/s42003-020-01391-5
Jiang, X., Hu, C., Arnovitz, S., Bugno, J., Yu, M., Zuo, Z., et al. (2016). miR-22 Has a Potent Anti-tumour Role with Therapeutic Potential in Acute Myeloid Leukaemia. Nat. Commun. 7, 11452. doi:10.1038/ncomms11452
Jiang, X., Hu, C., Ferchen, K., Nie, J., Cui, X., Chen, C.-H., et al. (2017). Targeted Inhibition of STAT/TET1 axis as a Therapeutic Strategy for Acute Myeloid Leukemia. Nat. Commun. 8 (1), 2099. doi:10.1038/s41467-017-02290-w
Jin, S.-G., Jiang, Y., Qiu, R., Rauch, T. A., Wang, Y., Schackert, G., et al. (2011). 5-Hydroxymethylcytosine Is Strongly Depleted in Human Cancers but its Levels Do Not Correlate with IDH1 Mutations. Cancer Res. 71 (24), 7360–7365. doi:10.1158/0008-5472.can-11-2023
Johnson, K. C., Houseman, E. A., King, J. E., von Herrmann, K. M., Fadul, C. E., and Christensen, B. C. (2016). 5-Hydroxymethylcytosine Localizes to Enhancer Elements and Is Associated with Survival in Glioblastoma Patients. Nat. Commun. 7, 13177. doi:10.1038/ncomms13177
Johnson, N. D., Huang, L., Li, R., Li, Y., Yang, Y., Kim, H. R., et al. (2020). Age-related DNA Hydroxymethylation Is Enriched for Gene Expression and Immune System Processes in Human Peripheral Blood. Epigenetics 15 (3), 294–306. doi:10.1080/15592294.2019.1666651
Kilgour, E., Rothwell, D. G., Brady, G., and Dive, C. (2020). Liquid Biopsy-Based Biomarkers of Treatment Response and Resistance. Cancer Cell 37 (4), 485–495. doi:10.1016/j.ccell.2020.03.012
Ko, M., An, J., Bandukwala, H. S., Chavez, L., Äijö, T., Pastor, W. A., et al. (2013). Modulation of TET2 Expression and 5-methylcytosine Oxidation by the CXXC Domain Protein IDAX. Nature 497 (7447), 122–126. doi:10.1038/nature12052
Kohli, R. M., and Zhang, Y. (2013). TET Enzymes, TDG and the Dynamics of DNA Demethylation. Nature 502 (7472), 472–479. doi:10.1038/nature12750
Koivunen, P., and Laukka, T. (2018). The TET Enzymes. Cell. Mol. Life Sci. 75 (8), 1339–1348. doi:10.1007/s00018-017-2721-8
Krais, A. M., Park, Y. J., Reifenberger, G., Meister, M., Plass, C., and Schmeiser, H. H. (2019). Sensitive Detection of Hydroxymethylcytosine Levels in normal and Neoplastic Cells and Tissues. Electrophoresis 40 (9), 1293–1297. doi:10.1002/elps.201800523
Kraus, T. F. J., Kolck, G., Greiner, A., Schierl, K., Guibourt, V., and Kretzschmar, H. A. (2015). Loss of 5-hydroxymethylcytosine and Intratumoral Heterogeneity as an Epigenomic Hallmark of Glioblastoma. Tumor Biol. 36 (11), 8439–8446. doi:10.1007/s13277-015-3606-9
Kriaucionis, S., and Heintz, N. (2009). The Nuclear DNA Base 5-hydroxymethylcytosine Is Present in Purkinje Neurons and the Brain. Science 324 (5929), 929–930. doi:10.1126/science.1169786
Laird, A., Thomson, J. P., Harrison, D. J., and Meehan, R. R. (2013). 5-hydroxymethylcytosine Profiling as an Indicator of Cellular State. Epigenomics 5 (6), 655–669. doi:10.2217/epi.13.69
Li, W., Zhang, X., Lu, X., You, L., Song, Y., Luo, Z., et al. (2017). 5-Hydroxymethylcytosine Signatures in Circulating Cell-free DNA as Diagnostic Biomarkers for Human Cancers. Cel Res 27 (10), 1243–1257. doi:10.1038/cr.2017.121
Lian, C. G., Xu, Y., Ceol, C., Wu, F., Larson, A., Dresser, K., et al. (2012). Loss of 5-hydroxymethylcytosine Is an Epigenetic Hallmark of Melanoma. Cell 150 (6), 1135–1146. doi:10.1016/j.cell.2012.07.033
Liao, Y., Gu, J., Wu, Y., Long, X., Ge, D., Xu, J., et al. (2016). Low Level of 5-Hydroxymethylcytosine Predicts Poor Prognosis in Non-small Cell Lung Cancer. Oncol. Lett. 11 (6), 3753–3760. doi:10.3892/ol.2016.4474
Liu, C., Liu, L., Chen, X., Shen, J., Shan, J., Xu, Y., et al. (2013). Decrease of 5-hydroxymethylcytosine Is Associated with Progression of Hepatocellular Carcinoma through Downregulation of TET1. PLoS One 8 (5), e62828. doi:10.1371/journal.pone.0062828
Liu, J., Jiang, J., Mo, J., Liu, D., Cao, D., Wang, H., et al. (2019). Global DNA 5-Hydroxymethylcytosine and 5-Formylcytosine Contents Are Decreased in the Early Stage of Hepatocellular Carcinoma. Hepatology 69 (1), 196–208. doi:10.1002/hep.30146
Liu, W.-R., Tian, M.-X., Jin, L., Yang, L.-X., Ding, Z.-B., Shen, Y.-H., et al. (2014). High Expression of 5-hydroxymethylcytosine and Isocitrate Dehydrogenase 2 Is Associated with Favorable Prognosis after Curative Resection of Hepatocellular Carcinoma. J. Exp. Clin. Cancer Res. 33, 32. doi:10.1186/1756-9966-33-32
Liu, Y., Siejka-Zielińska, P., Velikova, G., Bi, Y., Yuan, F., Tomkova, M., et al. (2019). Bisulfite-free Direct Detection of 5-methylcytosine and 5-hydroxymethylcytosine at Base Resolution. Nat. Biotechnol. 37 (4), 424–429. doi:10.1038/s41587-019-0041-2
Locke, W. J., Guanzon, D., Ma, C., Liew, Y. J., Duesing, K. R., Fung, K. Y. C., et al. (2019). DNA Methylation Cancer Biomarkers: Translation to the Clinic. Front. Genet. 10, 1150. doi:10.3389/fgene.2019.01150
López, V., Fernández, A. F., and Fraga, M. F. (2017). The Role of 5-hydroxymethylcytosine in Development, Aging and Age-Related Diseases. Ageing Res. Rev. 37, 28–38. doi:10.1016/j.arr.2017.05.002
Luchtel, R. A., Bhagat, T., Pradhan, K., Jacobs, W. R., Levine, M., Verma, A., et al. (2020). High-dose Ascorbic Acid Synergizes with Anti-PD1 in a Lymphoma Mouse Model. Proc. Natl. Acad. Sci. USA 117 (3), 1666–1677. doi:10.1073/pnas.1908158117
Mariani, C. J., Vasanthakumar, A., Madzo, J., Yesilkanal, A., Bhagat, T., Yu, Y., et al. (2014). TET1-mediated Hydroxymethylation Facilitates Hypoxic Gene Induction in Neuroblastoma. Cel Rep. 7 (5), 1343–1352. doi:10.1016/j.celrep.2014.04.040
Minor, E. A., Court, B. L., Young, J. I., and Wang, G. (2013). Ascorbate Induces Ten-Eleven Translocation (Tet) Methylcytosine Dioxygenase-Mediated Generation of 5-hydroxymethylcytosine. J. Biol. Chem. 288 (19), 13669–13674. doi:10.1074/jbc.c113.464800
Moen, E. L., Mariani, C. J., Zullow, H., Jeff-Eke, M., Litwin, E., Nikitas, J. N., et al. (2015). New Themes in the Biological Functions of 5-methylcytosine and 5-hydroxymethylcytosine. Immunol. Rev. 263 (1), 36–49. doi:10.1111/imr.12242
Neri, F., Dettori, D., Incarnato, D., Krepelova, A., Rapelli, S., Maldotti, M., et al. (2015). TET1 Is a Tumour Suppressor that Inhibits colon Cancer Growth by Derepressing Inhibitors of the WNT Pathway. Oncogene 34 (32), 4168–4176. doi:10.1038/onc.2014.356
Orr, B. A., Haffner, M. C., Nelson, W. G., Yegnasubramanian, S., and Eberhart, C. G. (2012). Decreased 5-hydroxymethylcytosine Is Associated with Neural Progenitor Phenotype in normal Brain and Shorter Survival in Malignant Glioma. PLoS One 7 (7), e41036. doi:10.1371/journal.pone.0041036
Pan, W., Zhu, S., Qu, K., Meeth, K., Cheng, J., He, K., et al. (2017). The DNA Methylcytosine Dioxygenase Tet2 Sustains Immunosuppressive Function of Tumor-Infiltrating Myeloid Cells to Promote Melanoma Progression. Immunity 47 (2), 284–297. doi:10.1016/j.immuni.2017.07.020
Pei, Y.-F., Tao, R., Li, J.-F., Su, L.-P., Yu, B.-Q., Wu, X.-Y., et al. (2016). TET1 Inhibits Gastric Cancer Growth and Metastasis by PTEN Demethylation and Re-expression. Oncotarget 7 (21), 31322–31335. doi:10.18632/oncotarget.8900
Puig, I., Tenbaum, S. P., Chicote, I., Arqués, O., Martínez-Quintanilla, J., Cuesta-Borrás, E., et al. (2018). TET2 Controls Chemoresistant Slow-Cycling Cancer Cell Survival and Tumor Recurrence. J. Clin. Invest. 128 (9), 3887–3905. doi:10.1172/jci96393
Putiri, E. L., Tiedemann, R. L., Thompson, J. J., Liu, C., Ho, T., Choi, J.-H., et al. (2014). Distinct and Overlapping Control of 5-methylcytosine and 5-hydroxymethylcytosine by the TET Proteins in Human Cancer Cells. Genome Biol. 15 (6), R81. doi:10.1186/gb-2014-15-6-r81
Sandoval, J., and Esteller, M. (2012). Cancer Epigenomics: beyond Genomics. Curr. Opin. Genet. Development 22 (1), 50–55. doi:10.1016/j.gde.2012.02.008
Sant, D. W., Mustafi, S., Gustafson, C. B., Chen, J., Slingerland, J. M., and Wang, G. (2018). Vitamin C Promotes Apoptosis in Breast Cancer Cells by Increasing TRAIL Expression. Sci. Rep. 8 (1), 5306. doi:10.1038/s41598-018-23714-7
Schito, L., and Semenza, G. L. (2016). Hypoxia-Inducible Factors: Master Regulators of Cancer Progression. Trends Cancer 2 (12), 758–770. doi:10.1016/j.trecan.2016.10.016
Shen, S. Y., Singhania, R., Fehringer, G., Chakravarthy, A., Roehrl, M. H. A., Chadwick, D., et al. (2018). Sensitive Tumour Detection and Classification Using Plasma Cell-free DNA Methylomes. Nature 563 (7732), 579–583. doi:10.1038/s41586-018-0703-0
Shi, X., Yu, Y., Luo, M., Zhang, Z., Shi, S., Feng, X., et al. (2016). Loss of 5-Hydroxymethylcytosine Is an Independent Unfavorable Prognostic Factor for Esophageal Squamous Cell Carcinoma. PLoS One 11 (4), e0153100. doi:10.1371/journal.pone.0153100
Shohdy, K. S., and West, H. (2020). Circulating Tumor DNA Testing-Liquid Biopsy of a Cancer. JAMA Oncol. 6 (5), 792. doi:10.1001/jamaoncol.2020.0346
Si, Y., Liu, J., Shen, H., Zhang, C., Wu, Y., Huang, Y., et al. (2019). Fisetin Decreases TET1 Activity and CCNY/CDK16 Promoter 5hmC Levels to Inhibit the Proliferation and Invasion of Renal Cancer Stem Cell. J. Cel Mol Med 23 (2), 1095–1105. doi:10.1111/jcmm.14010
Siravegna, G., Marsoni, S., Siena, S., and Bardelli, A. (2017). Integrating Liquid Biopsies into the Management of Cancer. Nat. Rev. Clin. Oncol. 14 (9), 531–548. doi:10.1038/nrclinonc.2017.14
Smith, Z. D., and Meissner, A. (2013). DNA Methylation: Roles in Mammalian Development. Nat. Rev. Genet. 14 (3), 204–220. doi:10.1038/nrg3354
Song, C.-X., Yin, S., Ma, L., Wheeler, A., Chen, Y., Zhang, Y., et al. (2017). 5-Hydroxymethylcytosine Signatures in Cell-free DNA Provide Information about Tumor Types and Stages. Cel Res 27 (10), 1231–1242. doi:10.1038/cr.2017.106
Sproul, D., and Meehan, R. R. (2013). Genomic Insights into Cancer-Associated Aberrant CpG Island Hypermethylation. Brief. Funct. Genomics 12 (3), 174–190. doi:10.1093/bfgp/els063
Strand, S. H., Hoyer, S., Lynnerup, A.-S., Haldrup, C., Storebjerg, T. M., Borre, M., et al. (2015). High Levels of 5-hydroxymethylcytosine (5hmC) Is an Adverse Predictor of Biochemical Recurrence after Prostatectomy in ERG-Negative Prostate Cancer. Clin. Epigenet 7, 111. doi:10.1186/s13148-015-0146-5
Su, P.-H., Hsu, Y.-W., Huang, R.-L., Chen, L.-Y., Chao, T.-K., Liao, C.-C., et al. (2019). TET1 Promotes 5hmC-dependent Stemness, and Inhibits a 5hmC-independent Epithelial-Mesenchymal Transition, in Cervical Precancerous Lesions. Cancer Lett. 450, 53–62. doi:10.1016/j.canlet.2019.01.033
Sun, M., Song, C.-X., Huang, H., Frankenberger, C. A., Sankarasharma, D., Gomes, S., et al. (2013). HMGA2/TET1/HOXA9 Signaling Pathway Regulates Breast Cancer Growth and Metastasis. Proc. Natl. Acad. Sci. 110 (24), 9920–9925. doi:10.1073/pnas.1305172110
Szabó, B., Németh, K., Mészáros, K., Szücs, N., Czirják, S., Reiniger, L., et al. (2020). Demethylation Status of Somatic DNA Extracted from Pituitary Neuroendocrine Tumors Indicates Proliferative Behavior. J. Clin. Endocrinol. Metab. 105 (6), dgaa156. doi:10.1210/clinem/dgaa156
Taberlay, P. C., Statham, A. L., Kelly, T. K., Clark, S. J., and Jones, P. A. (2014). Reconfiguration of Nucleosome-Depleted Regions at Distal Regulatory Elements Accompanies DNA Methylation of Enhancers and Insulators in Cancer. Genome Res. 24 (9), 1421–1432. doi:10.1101/gr.163485.113
Tahiliani, M., Koh, K. P., Shen, Y., Pastor, W. A., Bandukwala, H., Brudno, Y., et al. (2009). Conversion of 5-methylcytosine to 5-hydroxymethylcytosine in Mammalian DNA by MLL Partner TET1. Science 324 (5929), 930–935. doi:10.1126/science.1170116
Taylor, M. A., Wappett, M., Delpuech, O., Brown, H., and Chresta, C. M. (2016). Enhanced MAPK Signaling Drives ETS1-Mediated Induction of miR-29b Leading to Downregulation of TET1 and Changes in Epigenetic Modifications in a Subset of Lung SCC. Oncogene 35 (33), 4345–4357. doi:10.1038/onc.2015.499
Thienpont, B., Steinbacher, J., Zhao, H., D’Anna, F., Kuchnio, A., Ploumakis, A., et al. (2016). Tumour Hypoxia Causes DNA Hypermethylation by Reducing TET Activity. Nature 537 (7618), 63–68. doi:10.1038/nature19081
Thomson, J. P., and Meehan, R. R. (2017). The Application of Genome-wide 5-hydroxymethylcytosine Studies in Cancer Research. Epigenomics 9 (1), 77–91. doi:10.2217/epi-2016-0122
Tian, X., Sun, B., Chen, C., Gao, C., Zhang, J., Lu, X., et al. (2018). Circulating Tumor DNA 5-hydroxymethylcytosine as a Novel Diagnostic Biomarker for Esophageal Cancer. Cel Res 28 (5), 597–600. doi:10.1038/s41422-018-0014-x
Tong, M., Gao, S., Qi, W., Shi, C., Qiu, M., Yang, F., et al. (2019). 5-Hydroxymethylcytosine as a Potential Epigenetic Biomarker in Papillary Thyroid Carcinoma. Oncol. Lett. 18 (3), 2304–2309. doi:10.3892/ol.2019.10531
Tsai, K.-W., Li, G.-C., Chen, C.-H., Yeh, M.-H., Huang, J.-S., Tseng, H.-H., et al. (2015). Reduction of Global 5-hydroxymethylcytosine Is a Poor Prognostic Factor in Breast Cancer Patients, Especially for an ER/PR-negative Subtype. Breast Cancer Res. Treat. 153 (1), 219–234. doi:10.1007/s10549-015-3525-x
Tsai, Y.-P., Chen, H.-F., Chen, S.-Y., Cheng, W.-C., Wang, H.-W., Shen, Z.-J., et al. (2014). TET1 Regulates Hypoxia-Induced Epithelial-Mesenchymal Transition by Acting as a Co-activator. Genome Biol. 15 (12), 513. doi:10.1186/s13059-014-0513-0
Tuaeva, N. O., Falzone, L., Porozov, Y. B., Nosyrev, A. E., Trukhan, V. M., Kovatsi, L., et al. (2019). Translational Application of Circulating DNA in Oncology: Review of the Last Decades Achievements. Cells 8 (10), 1251. doi:10.3390/cells8101251
Wan, J. C. M., Massie, C., Garcia-Corbacho, J., Mouliere, F., Brenton, J. D., Caldas, C., et al. (2017). Liquid Biopsies Come of Age: towards Implementation of Circulating Tumour DNA. Nat. Rev. Cancer 17 (4), 223–238. doi:10.1038/nrc.2017.7
Wang, P., Yan, Y., Yu, W., and Zhang, H. (2019). Role of Ten-Eleven Translocation Proteins and 5-hydroxymethylcytosine in Hepatocellular Carcinoma. Cell Prolif 52 (4), e12626. doi:10.1111/cpr.12626
Wang, Z., Du, M., Yuan, Q., Guo, Y., Hutchinson, J. N., Su, L., et al. (2020). Epigenomic Analysis of 5-hydroxymethylcytosine (5hmC) Reveals Novel DNA Methylation Markers for Lung Cancers. Neoplasia 22 (3), 154–161. doi:10.1016/j.neo.2020.01.001
Wu, B.-K., and Brenner, C. (2014). Suppression of TET1-dependent DNA Demethylation Is Essential for KRAS-Mediated Transformation. Cel Rep. 9 (5), 1827–1840. doi:10.1016/j.celrep.2014.10.063
Wu, J., Li, H., Shi, M., Zhu, Y., Ma, Y., Zhong, Y., et al. (2019). TET1-mediated DNA Hydroxymethylation Activates Inhibitors of the Wnt/β-Catenin Signaling Pathway to Suppress EMT in Pancreatic Tumor Cells. J. Exp. Clin. Cancer Res. 38 (1), 348. doi:10.1186/s13046-019-1334-5
Wu, M.-Z., Chen, S.-F., Nieh, S., Benner, C., Ger, L.-P., Jan, C.-I., et al. (2015). Hypoxia Drives Breast Tumor Malignancy through a TET-Tnfα-P38-MAPK Signaling Axis. Cancer Res. 75 (18), 3912–3924. doi:10.1158/0008-5472.can-14-3208
Xiao, Z., Wu, W., Wu, C., Li, M., Sun, F., Zheng, L., et al. (2021). 5‐Hydroxymethylcytosine Signature in Circulating Cell‐free DNA as a Potential Diagnostic Factor for Early‐stage Colorectal Cancer and Precancerous Adenoma. Mol. Oncol. 15 (1), 138–150. doi:10.1002/1878-0261.12833
Xu, W., Yang, H., Liu, Y., Yang, Y., Wang, P., Kim, S.-H., et al. (2011). Oncometabolite 2-Hydroxyglutarate Is a Competitive Inhibitor of α-Ketoglutarate-Dependent Dioxygenases. Cancer cell 19 (1), 17–30. doi:10.1016/j.ccr.2010.12.014
Xu, Y.-p., Lv, L., Liu, Y., Smith, M. D., Li, W.-C., Tan, X.-m., et al. (2019). Tumor Suppressor TET2 Promotes Cancer Immunity and Immunotherapy Efficacy. J. Clin. Invest. 129 (10), 4316–4331. doi:10.1172/jci129317
Yan, Y.-L., Huang, Z.-N., Zhu, Z., Cui, Y.-Y., Li, M.-Q., Huang, R.-M., et al. (2020). Downregulation of TET1 Promotes Bladder Cancer Cell Proliferation and Invasion by Reducing DNA Hydroxymethylation of AJAP1. Front. Oncol. 10, 667. doi:10.3389/fonc.2020.00667
Yang, Q., Wu, K., Ji, M., Jin, W., He, N., Shi, B., et al. (2013). Decreased 5-hydroxymethylcytosine (5-hmC) Is an Independent Poor Prognostic Factor in Gastric Cancer Patients. J. Biomed. Nanotechnology 9 (9), 1607–1616. doi:10.1166/jbn.2013.1713
Zeng, C., Stroup, E. K., Zhang, Z., Chiu, B. C.-H., and Zhang, W. (2019). Towards Precision Medicine: Advances in 5-hydroxymethylcytosine Cancer Biomarker Discovery in Liquid Biopsy. Cancer Commun. 39 (1), 12. doi:10.1186/s40880-019-0356-x
Zhang, F., Liu, Y., Zhang, Z., Li, J., Wan, Y., Zhang, L., et al. (2016). 5-hydroxymethylcytosine Loss Is Associated with Poor Prognosis for Patients with WHO Grade II Diffuse Astrocytomas. Sci. Rep. 6, 20882. doi:10.1038/srep20882
Zhang, J., Han, X., Gao, C., Xing, Y., Qi, Z., Liu, R., et al. (2018). 5-Hydroxymethylome in Circulating Cell-free DNA as A Potential Biomarker for Non-small-cell Lung Cancer. Genomics, Proteomics & Bioinformatics 16 (3), 187–199. doi:10.1016/j.gpb.2018.06.002
Zhang, L.-Y., Han, C.-S., Li, P.-L., and Zhang, X.-C. (2016). 5-Hydroxymethylcytosine Expression Is Associated with Poor Survival in Cervical Squamous Cell Carcinoma. Jpn. J. Clin. Oncol. 46 (5), 427–434. doi:10.1093/jjco/hyw002
Zhang, Z., Jin, Y., Zhang, W., Chu, C., Zhang, K., Gao, X., et al. (2020). Values of 5mC, 5hmC, and TET2 for Identifying the Presence and Progression of Breast Precancerous Lesion. J. Clin. Lab. Anal. 34 (5), e23162. doi:10.1002/jcla.23162
Keywords: 5-hydroxymethylcytosine, cell-free DNA, ten-eleven translocase, liquid biopsy, cancer biomarker
Citation: Xu L, Zhou Y, Chen L, Bissessur AS, Chen J, Mao M, Ju S, Chen L, Chen C, Li Z, Zhang X, Chen F, Cao F, Wang L and Wang Q (2021) Deoxyribonucleic Acid 5-Hydroxymethylation in Cell-Free Deoxyribonucleic Acid, a Novel Cancer Biomarker in the Era of Precision Medicine. Front. Cell Dev. Biol. 9:744990. doi: 10.3389/fcell.2021.744990
Received: 21 July 2021; Accepted: 29 November 2021;
Published: 10 December 2021.
Edited by:
Catherine Alix-Panabieres, Centre Hospitalier Universitaire De Montpellier, FranceReviewed by:
Daniel Hernández Sotelo, Autonomous University of Guerrero, MexicoFabio Ciccarone, Università Telematica San Raffaele, Italy
Copyright © 2021 Xu, Zhou, Chen, Bissessur, Chen, Mao, Ju, Chen, Chen, Li, Zhang, Chen, Cao, Wang and Wang. This is an open-access article distributed under the terms of the Creative Commons Attribution License (CC BY). The use, distribution or reproduction in other forums is permitted, provided the original author(s) and the copyright owner(s) are credited and that the original publication in this journal is cited, in accordance with accepted academic practice. No use, distribution or reproduction is permitted which does not comply with these terms.
*Correspondence: Feilin Cao, drcfl@126.com; Linbo Wang, linbowang@zju.edu.cn; Qinchuan Wang, wangqinchuan@zju.edu.cn