- 1Department of Physiology, College of Medicine and Health Sciences, United Arab Emirates University, Al Ain, United Arab Emirates
- 2Biomedical Sciences, Warwick Medical School, University of Warwick, Coventry, United Kingdom
- 3Tommy’s National Miscarriage Research Centre, University Hospitals Coventry and Warwickshire NHS Trust, Coventry, United Kingdom
Embryo implantation is a complex and tightly regulated process. In humans, uterine luminal epithelium functions as a biosensor gauging the embryo quality and transmitting this information to the underlying endometrial stromal cells. This quality control ensures that only high quality embryos are implanted, while aberrant ones are rejected. The mechanisms of the embryo-uterine mucosa crosstalk remain incompletely understood. Trypsin, a serine protease secreted by the blastocyst, has been implicated in the cross-signaling. Here we address the mechanisms by which trypsin triggers the intracellular calcium signaling in uterine epithelium. We found that protease-activated G-protein coupled receptors are the main mechanism mediating the effects of trypsin in human uterine epithelium. In addition, trypsin activates the epithelial sodium channels thus increasing the intracellular Na+ concentration and promoting Ca2+ entry on the reverse mode of the sodium/calcium exchanger.
Introduction
Pregnancy is a complex and highly coordinated physiological process that includes implantation, decidualization, placentation and birth of offspring through the process of parturition (Cha et al., 2012). The fertilized egg undergoes multiple mitotic divisions and enters the uterine cavity as a blastocyst. At the blastocyst stage, embryos mature, hatch from their outer shells (zona pellucidae) and then gain implantation competency. Further embryonic development critically depends on implantation of the hatched blastocyst into the maternal endometrium. The main purpose of implantation is to ensure that the blastocyst firmly anchors into the endometrial stroma, which will support the developing embryo and allow the process of placentation to begin (Wang and Dey, 2006). Implantation involves intimate interaction between an implantation-competent blastocyst and a receptive uterus, which occurs in a limited time period referred to as the window of implantation. Based on animal models, the uterine luminal epithelium has been considered solely responsible for the window of implantation. In polytocous species like mice or rats, transient expression of receptivity genes allow simultaneous implantation and correct spacing of multiple embryos at identical stages of development in an optimal endometrial environment (Kaneko et al., 2011). In contrast, humans, as a monotocous species, have to deal with highly invasive embryos that often display genomic instability and chromosomal aberrations (Mertzanidou et al., 2013). Under these conditions, the luminal epithelium functions as a biosensor gauging the embryo quality and transmitting this information to the underlying endometrial stromal cells to induce an environment that either supports further implantation or discards the aberrant embryo by menstruation-like shedding of the endometrium. Although a multitude of cellular signaling pathways involved in embryo-uterine crosstalk have been identified through gene expression studies and transgenic mouse models, a comprehensive understanding of the mechanisms of embryo-uterine interaction is still missing (Aplin, 2006; Zhang et al., 2013). Our previous work has shown that many metabolic and pro-inflammatory genes in human and murine endometrium are up-regulated in response to embryo-conditioned culture medium, suggesting that developing embryos secrete humoral factor(s) capable of inducing profound transcriptional responses in the endometrium (Brosens et al., 2014). Experiments in mice have shown that embryo-released serine protease, trypsin, triggers intracellular Ca2+ ([Ca2+]i) transients that lead to prostaglandin E2 (PGE2) release, phosphorylation of the transcription factor CREB and up-regulation of cyclooxygenase 2 (COX-2) required for prostaglandin synthesis from arachidonic acid (Ruan et al., 2012). This study has demonstrated the involvement of the epithelial Na+ channel (ENaC) in trypsin-induced [Ca2+]i transients. Similarly to other epithelia (Rossier and Stutts, 2009), trypsin activates the uterine ENaC via proteolytic cleavage of the gamma subunit leading to inward current and membrane depolarization. The authors proposed that this depolarization triggers Ca2+ entry into epithelial cells through voltage-gated L-type Ca2+ channels (Ruan et al., 2012). However, evidence for the involvement of voltage-gated Ca2+ channels in uterine epithelial responses is not very convincing since trypsin-induced [Ca2+]i transients were only partially inhibited by high doses (above 10 μM) of the L-type Ca2+ channel blocker nifedipine. At these concentrations, nifedipine may affect other ion channels/transporters in addition to the voltage-gated Ca2+ channel blockade. Indeed, a direct inhibition of alveolar ENaC by high doses of voltage-gated Ca2+ channel blockers has been described in the literature (Marunaka and Niisato, 2002). Most likely, mechanisms underlying the trypsin-induced Ca2+ signaling in uterine epithelium are more complex than a simple Ca2+ entry through voltage-gated Ca2+ channels. Nonetheless, the findings presented by Ruan et al. (2012) are important as they draw attention to a novel role for the embryo-derived serine proteases, namely their contribution to the embryo-uterine signaling dialogue, and highlight the importance of epithelial [Ca2+]i signaling for embryo implantation. Previous studies have demonstrated that trophoblast spheroids can elevate [Ca2+]i in RL95-2 cells, a human uterine epithelial cell line, by activating Ca2+ entry via mechano-sensitive Ca2+ permeable channels leading to the induction of epithelial adhesiveness (Tinel et al., 2000). However, the mechanism(s) mediating the protease-induced [Ca2+]i signaling in human uterine epithelium remains incompletely understood. In this report, we present evidence for the protease-activated G-protein coupled receptors (GPCR) involvement in trypsin-induced Ca2+ signaling in the primary human uterine epithelium and in the Ishikawa cell line.
Materials and Methods
Cell Culture
Initial experiments were performed on primary cultures of human uterine epithelial cells. Endometrial biopsies were obtained from women attending the Implantation Research Clinic, University Hospitals Coventry and Warwickshire National Health Service Trust. Written informed consent was obtained in accordance with the Declaration of Helsinki 2000. The study was approved by the NHS National Research Ethics Committee of Hammersmith and Queen Charlotte’s Hospital NHS Trust (1997/5065). Endometrial biopsies were obtained from four women attending the Implantation Clinic at University Hospitals Coventry and Warwickshire NHS Trust. All research was undertaken with full ethical approval and with written informed consent obtained from all participants in accordance with The Declaration of Helsinki 2000. Biopsies were taken during the secretory phase of the menstrual cycle using an EndocellTM cannula, starting from the uterine fundus and moving downward to the internal cervical ostium. The biopsy was collected in a labeled Bijoux tube containing 5 ml of cell culture medium and transferred to the lab for processing as quickly as possible. The endometrial epithelial cells were isolated from the endometrial biopsies using collagenase and DNase digestion as described in detail elsewhere (Barros et al., 2016). After separation of uterine glands from the stromal and immune cells, the former were further digested with trypsin to obtain a single-cell suspension. Dissociated epithelial cells were plated into glass-bottomed 35 mm Petri dishes (Mattek) and incubated at 37°C in a humidified incubator in 5% CO2 for up to a week before using them in Ca2+ imaging experiments. Three to six Petri dishes per biopsy were used in the Ca2+ imaging experiments.
In the initial experiments, we found that primary uterine epithelial cells generated [Ca2+]i responses to trypsin application similar to those observed in Ishikawa cells, an uterine epithelial cancer cell line. Given this similarity between the primary human endometrial epithelial cells and the Ishikawa cell line, subsequent mechanistic experiments were performed on Ishikawa cells. The Ishikawa cell line was obtained from Sigma-Aldrich and cultured in glass-bottomed 35 mm Petri dishes using a cell culture method similar to that described by Schmidt and colleagues (Schmidt et al., 2014). Ishikawa cells were used in Ca2+ imaging experiments within a week after passaging. Before the experiment, the cells were cultured overnight in serum-free DMEM.
Ca2+ Imaging
For imaging intracellular calcium ([Ca2+]i) responses to trypsin, a ratiometric Ca2+-sensitive indicator Fura-2 was employed. The cells were washed with physiological saline solution (PSS) of the following composition (in mmol/l): 135 NaCl, 4 KCl, 1.2 MgCl2, 2 CaCl2, 5 HEPES and 10 Glucose. The pH of this solution was adjusted to 7.4 with NaOH. The Fura-2 indicator was loaded into cells as acetoxymethyl ester dissolved in DMSO and diluted with PSS to a final concentration of 2.5 μM. The loading solution also contained 0.05% of non-ionic detergent Pluronic F127 to aid the dye dispersal and 2 mM probenecid to facilitate dye retention in the cytoplasm. After 60 min loading at room temperature the cells were washed with PSS and incubated on the bench while protected from ambient light for another 30 min to ensure complete de-esterification of the dye. After that, the Petri dish with Fura-2 loaded cells was mounted on the stage of an inverted microscope (Olympus IX71, United Kingdom) and superfused with pre-warmed to 35°C PSS. Trypsin and other drugs were applied to cells within the field of view using a gravity-fed multi-tube preheater (MPRE8, Cell MicroControls, United States). A fast monochromator (Polychrome V, TILL Photonics, Germany) provided Fura-2 excitation alternating between 360 ± 12 and 380 ± 12 nm. The resulting Fura-2 fluorescence was separated from the excitation light by a 495 nm dichromatic mirror (Chroma, United States) and recorded at a wavelength above 510 nm. Time series of fluorescence images were collected at 1 ratio-pair per second using a front-illuminated EMCCD camera (Luca, Andor, United Kingdom) controlled by the WinFluor software package (J. Dempster, Strathclyde University, United Kingdom). The camera exposure was set to 50 ms and the cells were not illuminated for the rest of the cycle time. This substantially reduced the photo-bleaching of Fura-2 during long-lasting experiments. The same software was used for the extraction of fluorescence intensities and calculation of Fura-2 ratio representing the [Ca2+]i dynamics.
All solutions were prepared using analytical grade salts and deionized water. Trypsin was supplied by Worthington Biochemical Corporation, United States. DMSO, Pluronic F127 and Fura-2/AM were purchased from the Molecular Probes, United States. PAR agonist and antagonist peptides, amiloride, SN-6 and the store operated channel inhibitor GSK7975A were supplied by Tocris Bioscience, United Kingdom. All other reagents were purchased from Sigma, United Kingdom.
Data Quantification and Analysis
Background fluorescence was calculated from areas devoid of cells and subtracted from each time series. To quantify the cellular [Ca2+]i responses to trypsin and other agents, regions of interest (ROIs) were drawn around each cell within the field of view, the mean intensity values were extracted from the ROIs and the ratio between fluorescence intensities exited at 360 and 380 nm were calculated in WinFluor. The technical graphing and data analysis software package IgorPro 7.0.8.1 (Wavemetrics, United States) was used for statistical analyses and preparation of figures. The data were obtained from at least three different cultures. Where appropriate, statistical comparisons were made using unpaired t-test. When multiple comparisons were made, a 1-way ANOVA followed by Dunnet’s post hoc test was used.
Results
We reasoned that the concentrations of the embryo-secreted protease are unlikely to reach a micro-molar level around the uterine epithelium during human embryo implantation. We therefore tested a low-nanomolar concentration range of trypsin for its effectiveness at triggering the [Ca2+]i responses in primary uterine epithelial cells and in the Ishikawa cell line. Figure 1 illustrates typical [Ca2+]i responses to the application of 5 nM trypsin dissolved in PSS. In the majority of cells, trypsin at this concentration triggered a rapid transient rise in [Ca2+]i followed by a long-lasting oscillation (Figures 1A,C). In about a quarter of all cells tested (31 out of 132), a steady elevation of [Ca2+]i was observed after the initial [Ca2+]i transient instead of oscillations. The responses to 1 and 0.5 nM trypsin were similar to that triggered by 5–10 nM but the number of cells responding to trypsin declined in proportion to the decrease in trypsin concentration (not illustrated). We elected to use 5–10 nM trypsin in all the subsequent experiments.
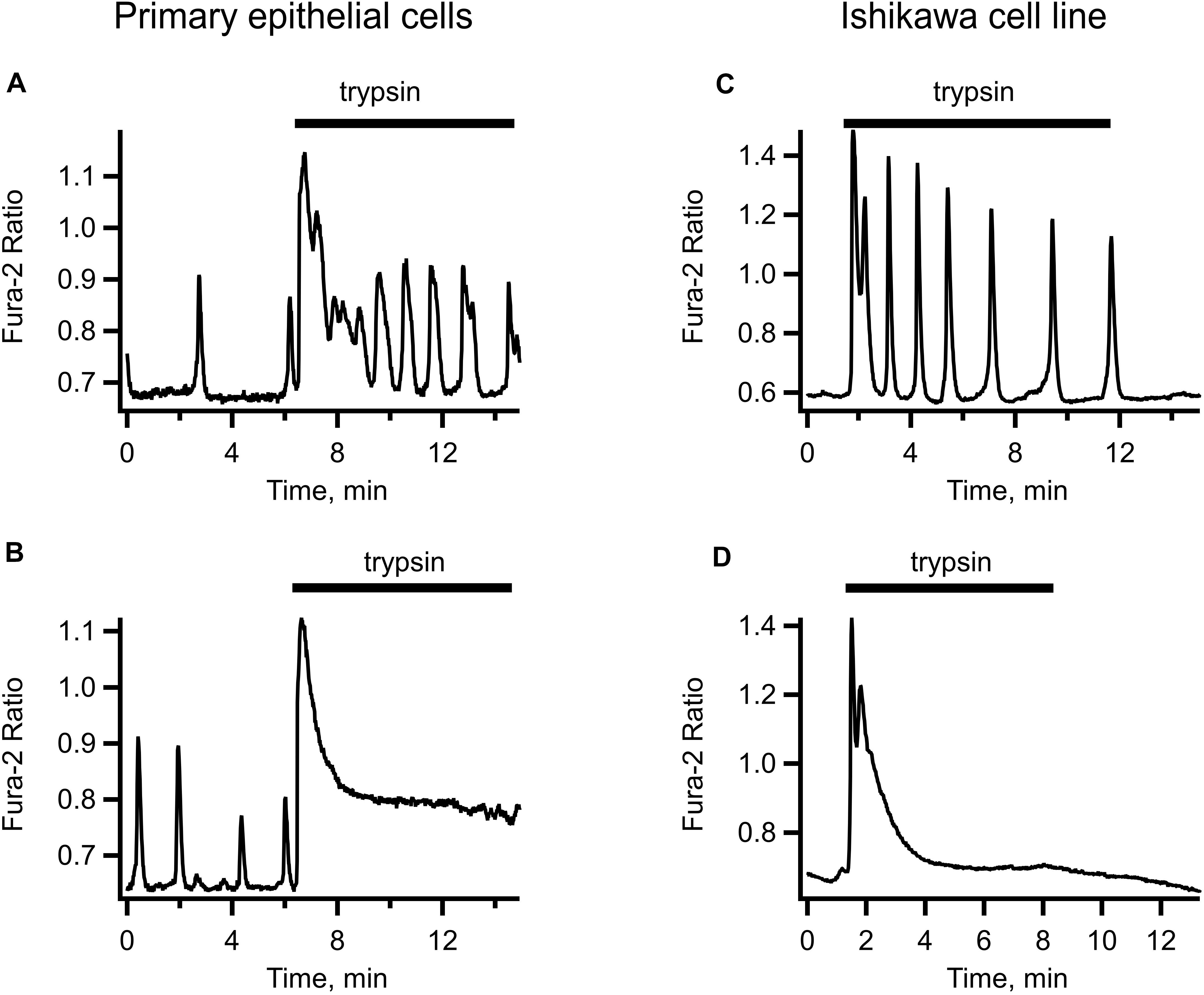
Figure 1. Examples of trypsin-induced [Ca2+]i signals in human primary uterine epithelial cells (left-hand panel) and in the Ishikawa cell line (right-hand panel). Application of 5 nM trypsin (indicated by a solid bar above each trace) triggered an initial transient elevation of [Ca2+]i followed by [Ca2+]i oscillations of variable frequency and amplitude (A,C) or by a non-oscillatory plateau of variable height (B,D). Records are typical of 87 cells from 4 separate cultures.
The [Ca2+]i dynamics were very similar in the primary epithelium and in Ishikawa cells, bar for the occasional irregular spontaneous activity sometimes observed in primary epithelium before the application of trypsin but never in the cell line (compare left-hand and right-hand panels of Figure 1). Given the similarity of trypsin-induced [Ca2+]i signaling in Ishikawa cells to that in primary uterine epithelium and the amenity of the cell line to different experimental maneuvers, we used Ishikawa cells to conduct in subsequent experiments.
Store Operated Ca2+ Entry Is Vital for the Oscillations
When trypsin was applied in the absence of extracellular Ca2+, there was only a single [Ca2+]i transient observed, which was followed by an exponential decline below the pre-stimulation level. Readmission of extracellular Ca2+ in a continuous presence of trypsin triggered immediate rise in [Ca2+]i and reappearance of the oscillations (illustrated in Figure 2A). To test the route for the extracellular Ca2+ entry, we employed GSK7975A (Derler et al., 2013), a specific inhibitor of the store operated Ca2+ channels. In the presence of this inhibitor, trypsin-induced [Ca2+]i oscillations were abolished, either immediately (Figure 2B) or after several declining oscillations (Figure 2C). The initial [Ca2+]i transient was unaffected by the GSK7975A application, but it was not followed by the oscillations, only a slightly elevated [Ca2+]i level was maintained. Sometimes this was accompanied by slow and irregular fluctuations of [Ca2+]i (Figure 2D). These results suggest that the main mechanism that sustains the trypsin-induced [Ca2+]i oscillations was Ca2+ entry through the STIM/Orai store operated Ca2+ channels, a hallmark feature of the GPCR-mediated Ca2+ signaling. We explored this in the next series of experiments.
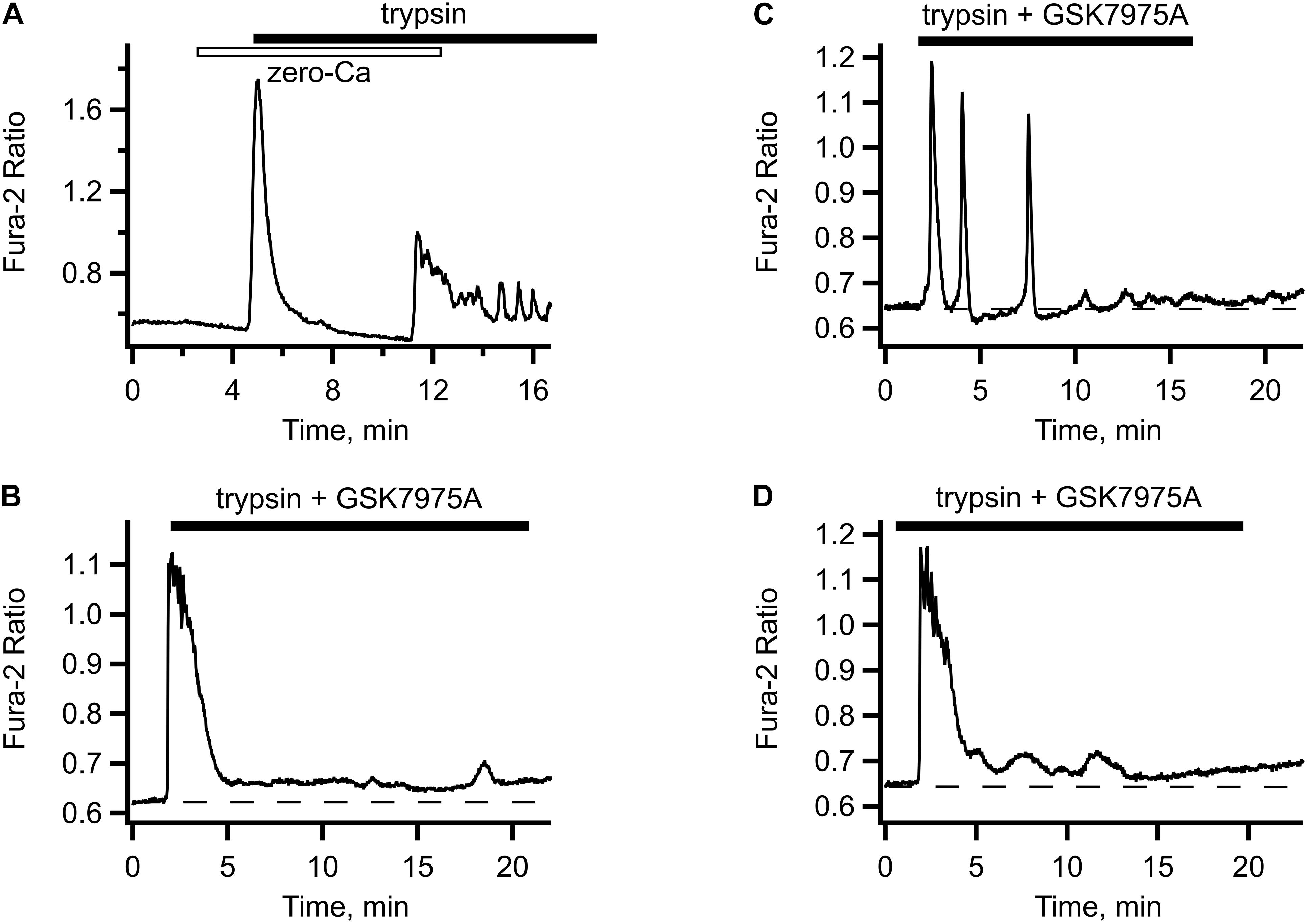
Figure 2. Store-operated Ca2+ entry contributes to trypsin- induced [Ca2+]i. (A) In Ca2+-free solution, trypsin application produced only the initial [Ca2+]i transient but no oscillations (typical of n = 7). Upon re-addition of extracellular Ca2+, [Ca2+]i oscillations reappeared. (B–D) Typical responses to trypsin (5 nM) in the presence of store operated Ca2+ channel inhibitor GSK7975A. Addition of GSK7975A (5 μM) to trypsin containing solution abolished the oscillations, either immediately (B) or after several oscillations of diminishing amplitude (C) leaving behind a slightly elevated level of [Ca2+]i sometimes accompanied by slow irregular fluctuations (D) (n = 34 cells from two different cultures).
Effects of PAR Peptides
Figure 3A illustrates the effect of the PAR2 activating peptide SLIGRL-NH2 application to Ishikawa cell. The response was similar to that induced by trypsin and comprised an initial transient rise in [Ca2+]i due to the release from the intracellular store followed by [Ca2+]i oscillations. After the washout of PAR2 agonist peptide, the cells were capable of responding to trypsin (Figure 3A). Application of TFLLR-NH2, the PAR1 activating peptide, also induced [Ca2+]i responses in Ishikawa cells (not illustrated). On the other hand, pretreatment of the cells with FSLLRY-NH2, a PAR-2 inhibiting peptide, abolished both components of the [Ca2+]i response to trypsin. That is, not only the [Ca2+]i oscillations were abolished but the initial [Ca2+]i transient was eliminated as well (see Figure 3B). Interestingly, a small and slow rise in [Ca2+]i, similar to that observed in the experiments with GSK7975A remained in the presence of FSLLRY-NH2. This may indicate the presence of an additional trypsin-activated Ca2+ entry pathway unrelated to the GPCRs or store-operated Ca2+ channels. An obvious candidate for such a mechanism could be ENaC that has already been shown to contribute to embryo implantation in mice (Ruan et al., 2012). We explored this in Ishikawa cells using amiloride, a potent inhibitor of ENaC.
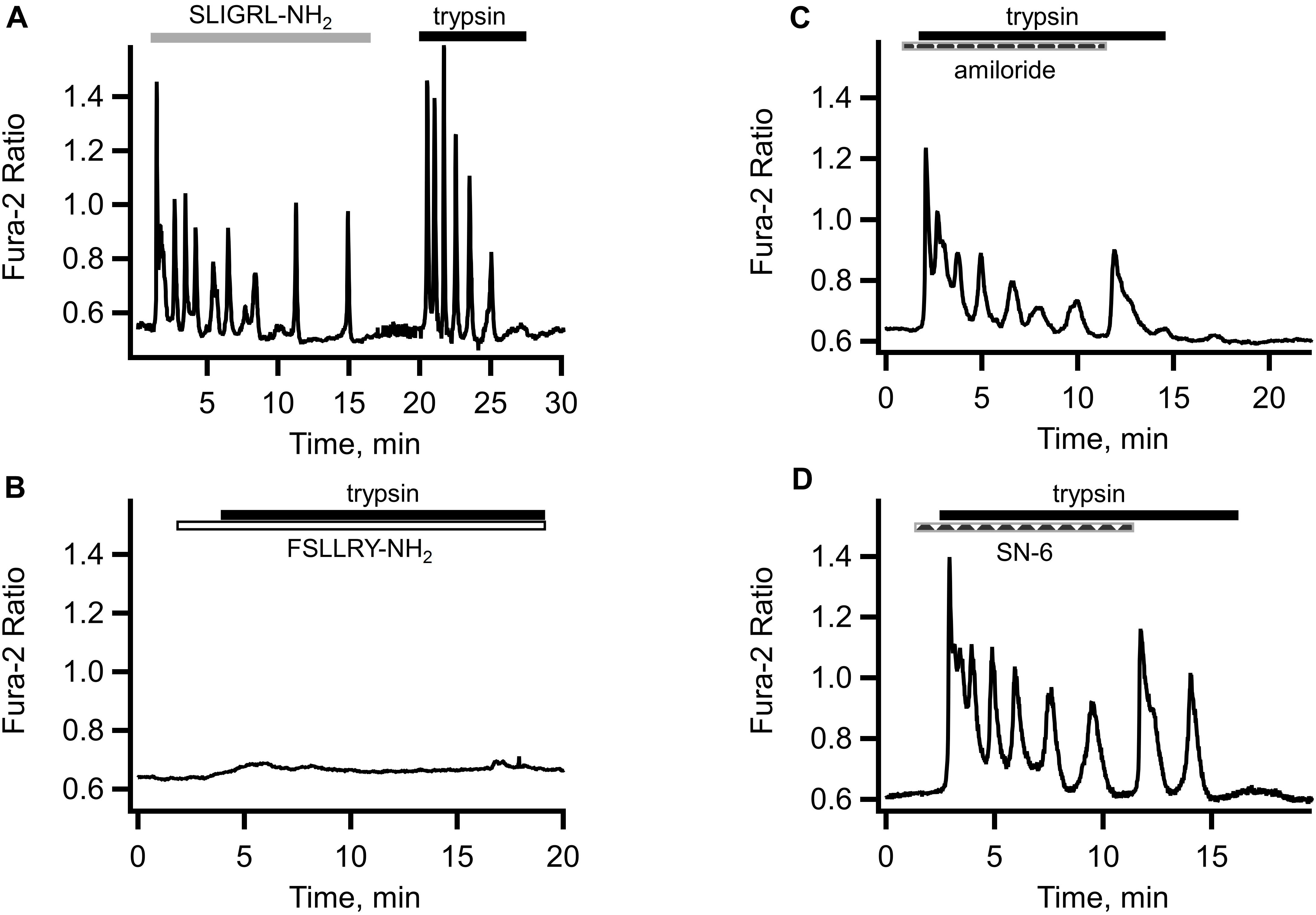
Figure 3. Role of proteinase activated receptors, ENaC and sodium/calcium exchanger in the trypsin-induced [Ca2+]i responses. (A) Application of PAR2 agonist SLIGRL-NH2 (10 μM, empty bar) produced a [Ca2+]i response similar to that elicited by subsequent trypsin (5 nM, solid bar) application to the same cell. (B) Both the initial transient and [Ca2+]i oscillations in response to trypsin (5 nM, solid bar) were abolished by the PAR2 inhibiting peptide FSLLRY-NH2 (10 μM, empty bar). Traces shown are typical of 46 cells from three separate cultures. (C) Application of ENaC inhibitor amiloride (10 μM, dashed bar) partially inhibited [Ca2+]i oscillations but not the initial [Ca2+]i transient in response to trypsin (5 nM, solid bar) (n = 113 cells from four separate cultures). (D) Inhibitor of the reverse mode of sodium/calcium exchanger SN-6 (10 μM, dashed bar) had similar to amiloride effect (n = 24 cell from three separate cultures).
Role of ENaC
As illustrated in Figure 3C, ENaC inhibition failed to eliminate the initial component of the trypsin-induced [Ca2+]i response but reduced the amplitude and, to a lesser extent, the frequency of subsequent [Ca2+]i oscillations. De-inhibition of ENaC by rapid removal of amiloride led to a transient resurgence of the trypsin-induced [Ca2+]i oscillations amplitude (Figure 3C). This was consistently observed in all 78 cells from five different cultures. A similar effect was observed in the experiments with SN-6, a preferential inhibitor of the reverse mode of the sodium/calcium exchanger (Niu et al., 2007; Gandhi et al., 2013). Pre-incubation of cells with 10 μM of SN-6 reduced the amplitude and frequency of [Ca2+]i oscillations without affecting the initial [Ca2+]i transient in response to trypsin (Figure 3D). There was also a re-bounce effect upon a rapid washout of SN-6. In fact, the resurgence of the [Ca2+]i oscillation was more pronounced compared to that after the amiloride withdrawal. Taken together, these results suggest that the additional pathway for the extracellular Ca2+ entry is the reverse mode of the sodium/calcium exchanger (NCX).
Discussion
In this report, we examined the mechanism of the trypsin-induced [Ca2+]i signaling in human uterine epithelial cells. Our main findings are: (i) the effects of trypsin application are similar in primary human uterine epithelium and in the Ishikawa cell line; and (ii) the peptide agonists of PAR1 and PAR2 can mimic the effects of low concentrations of trypsin on uterine epithelial [Ca2+]i dynamics while the PAR inhibitor peptide eliminates this effect. These results are compatible with the idea that the proteinase activated receptors play a major role in the trypsin-induced endometrial [Ca2+]i signaling. This is in contrast to the mechanism proposed for implantation in mice, where ENaC and the L-type Ca2+ channels are thought to be pivotal players (Ruan et al., 2012). Nevertheless, our results with ENaC inhibitor amiloride and the NCX inhibitor SN-6 suggest that ENaC-NCX system can provide an additional route for extracellular Ca2+ entry in parallel to that afforded by the PAR activation of store-operated Ca2+ channels. The schematic drawing in Figure 4 summarizes our understanding of the result obtained in this study. We suggest that trypsin released from the trophectoderm of implanting embryo binds to PAR2, thereby triggering a classical GPCR-mediated intracellular Ca2+ release followed by store-operated Ca2 entry via the STIM/orai voltage-independent Ca2+ channels. In parallel, trypsin cleaves the gamma-subunit of the epithelial sodium channel thereby activating the Na+ entry via ENaC. The role of epithelial sodium channels and the cystic fibrosis transmembrane conductance regulator (CFTR) chloride channels in uterine fluid secretion and reabsorption has long been recognized as an important determinant of uterine closure, a mechanism by which embryos are held in contact with the uterine epithelium before initiation of implantation (Chan et al., 2002; Naftalin et al., 2002). Similar to other ion transporting epithelia, uterine ENaC and CFTR co-localize to microvilli on the apical membrane and functionally interact with each other to provide electrically coupled ionic fluxes (Horisberger, 2003; Konstas et al., 2003). Inward currents through ENaC and CFTR are counterbalanced by outward current through the large conductance Ca2+-activated potassium channels (Zhang et al., 2012). We propose that Na+ entry into the intravillous space via trypsin-activated ENaC will depolarize the cellular membrane and increase the intravillous Na2+ concentration sufficiently high to reverse the NCX thereby providing means for Ca2+ entry into the intravillous space. Diffusion of Ca2+ from the microvilli into the bulk cytoplasm will increase [Ca2+]i and, in parallel with store-operated calcium entry, act as a source for re-filling of the endoplasmic reticulum. Increased [Ca2+]i will also activate the BK channels leading to repolarization and termination of Ca2+ entry via the reverse mode of NCX. Thus, the proposed two mechanisms will act in coherence to sustain the [Ca2+]i oscillations. Misbalance between the rates of Ca2+ entry and Ca2+ accumulation into the endoplasmic reticulum may manifest itself as a steady, non-oscillatory Ca plateau of variable height, which we observed in a proportion of cells. The coexistence of the two parallel routes for Ca2+ entry will ensure a robust activation of prostaglandin E production vital for decidualization of endometrial stromal cells.
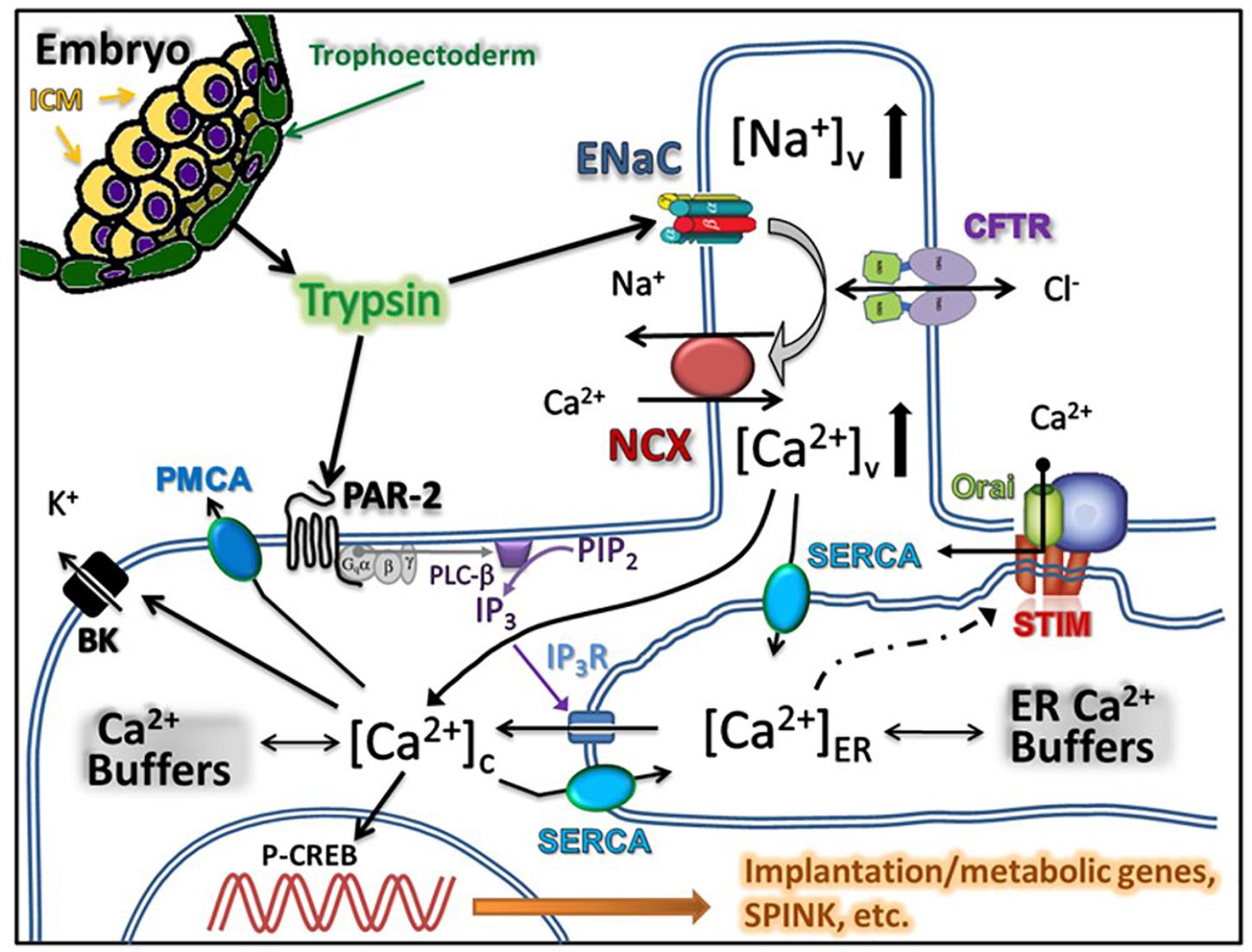
Figure 4. Summary of the proposed model for trypsin induced Ca2+ signaling in uterine epithelium. Trypsin is released by the trophectoderm of the implanting embryo and acts on proteinase activated receptors thereby triggering the release of Ca2+ from the endoplasmic reticulum. Emptying the reticulum triggers store-operated Ca2+ entry. In parallel, trypsin activates ENaC located in the microvilli of the uterine epithelial cells leading to the increase in the intravillous Na2+ level, reversal of the Na+/Ca2+ exchanger and additional Ca2+ entry into cytoplasm.
Data Availability Statement
The raw data supporting the conclusions of this article will be made available by the authors, without undue reservation.
Ethics Statement
The studies involving human participants were reviewed and approved by the NHS National Research Ethics Committee of Hammersmith and Queen Charlotte’s Hospital NHS Trust (1997/5065). The patients/participants provided their written informed consent to participate in this study.
Author Contributions
AS and JB conceived and planned the study. JB collected endometrial biopsies and provided reagents and other materials. AS performed the experiments, analyzed the data, and wrote the manuscript. Both authors reviewed and approved the final version of the manuscript.
Funding
AS was funded by grants from the UAEU College of Medicine and Zayed bin Sultan Charitable Foundation. JB was supported by the Welcome Trust.
Conflict of Interest
The authors declare that the research was conducted in the absence of any commercial or financial relationships that could be construed as a potential conflict of interest.
Publisher’s Note
All claims expressed in this article are solely those of the authors and do not necessarily represent those of their affiliated organizations, or those of the publisher, the editors and the reviewers. Any product that may be evaluated in this article, or claim that may be made by its manufacturer, is not guaranteed or endorsed by the publisher.
References
Aplin, J. D. (2006). Embryo implantation: the molecular mechanism remains elusive. Reprod. Biomed. Online 13, 833–839. doi: 10.1016/s1472-6483(10)61032-2
Barros, F. S. V., Brosens, J. J., and Brighton, P. J. (2016). Isolation and Primary Culture of Various Cell Types from Whole Human Endometrial Biopsies. Bio Protoc. 6:e2028.
Brosens, J. J., Salker, M. S., Teklenburg, G., Nautiyal, J., Salter, S., Lucas, E. S., et al. (2014). Uterine Selection of Human Embryos at Implantation. Sci. Rep. 4:3894. doi: 10.1038/srep03894
Cha, J., Sun, X., and Dey, S. K. (2012). Mechanisms of implantation: strategies for successful pregnancy. Nat. Med. 18, 1754–1767. doi: 10.1038/nm.3012
Chan, L. N., Tsang, L. L., Rowlands, D. K., Rochelle, L. G., Boucher, R. C., Liu, C. Q., et al. (2002). Distribution and regulation of ENaC subunit and CFTR mRNA expression in murine female reproductive tract. J. Membr. Biol. 185, 165–176. doi: 10.1007/s00232-001-0117-y
Derler, I., Schindl, R., Fritsch, R., Heftberger, P., Riedl, M. C., Begg, M., et al. (2013). The action of selective CRAC channel blockers is affected by the Orai pore geometry. Cell Calcium 53, 139–151. doi: 10.1016/j.ceca.2012.11.005
Gandhi, A., Siedlecka, U., Shah, A. P., Navaratnarajah, M., Yacoub, M. H., and Terracciano, C. M. (2013). The effect of SN-6, a novel sodium-calcium exchange inhibitor, on contractility and calcium handling in isolated failing rat ventricular myocytes. Cardiovasc. Ther. 31, e115–e124. doi: 10.1111/1755-5922.12045
Horisberger, J.-D. (2003). ENaC-CFTR interactions: the role of electrical coupling of ion fluxes explored in an epithelial cell model. Pflugers Arch. 445, 522–528. doi: 10.1007/s00424-002-0956-0
Kaneko, Y., Day, M. L., and Murphy, C. R. (2011). Integrin β3 in rat blastocysts and epithelial cells is essential for implantation in vitro: studies with Ishikawa cells and small interfering RNA transfection. Hum. Reprod. 26, 1665–1674. doi: 10.1093/humrep/der128
Konstas, A.-A., Koch, J.-P., and Korbmacher, C. (2003). cAMP-dependent activation of CFTR inhibits the epithelial sodium channel (ENaC) without affecting its surface expression. Pflugers Arch. 445, 513–521. doi: 10.1007/s00424-002-0957-z
Marunaka, Y., and Niisato, N. (2002). Effects of Ca(2+) channel blockers on amiloride-sensitive Na(+) permeable channels and Na(+) transport in fetal rat alveolar type II epithelium. Biochem. Pharmacol. 63, 1547–1552. doi: 10.1016/s0006-2952(02)00880-8
Mertzanidou, A., Wilton, L., Cheng, J., Spits, C., Vanneste, E., Moreau, Y., et al. (2013). Microarray analysis reveals abnormal chromosomal complements in over 70% of 14 normally developing human embryos. Hum. Reprod. 28, 256–264. doi: 10.1093/humrep/des362
Naftalin, R. J., Thiagarajah, J. R., Pedley, K. C., Pocock, V. J., and Milligan, S. R. (2002). Progesterone stimulation of fluid absorption by the rat uterine gland. Reproduction 123, 633–638. doi: 10.1530/rep.0.1230633
Niu, C.-F., Watanabe, Y., Ono, K., Iwamoto, T., Yamashita, K., Satoh, H., et al. (2007). Characterization of SN-6, a novel Na+/Ca2+ exchange inhibitor in guinea pig cardiac ventricular myocytes. Eur. J. Pharmacol. 573, 161–169. doi: 10.1016/j.ejphar.2007.06.033
Rossier, B. C., and Stutts, M. J. (2009). Activation of the epithelial sodium channel (ENaC) by serine proteases. Annu. Rev. Physiol. 71, 361–379. doi: 10.1146/annurev.physiol.010908.163108
Ruan, Y. C., Guo, J. H., Liu, X., Zhang, R., Tsang, L. L., Dong, J. D., et al. (2012). Activation of the epithelial Na+ channel triggers prostaglandin E2 release and production required for embryo implantation. Nat. Med. 18, 1112–1117. doi: 10.1038/nm.2771
Schmidt, S., Schneider, S., Yang, W., Liu, G., Schmidt, E.-M., Schmid, E., et al. (2014). TGFβ1 and SGK1-sensitive store-operated Ca2+ entry and Orai1 expression in endometrial Ishikawa cells. Mol. Hum. Reprod. 20, 139–147. doi: 10.1093/molehr/gat066
Tinel, H., Denker, H. W., and Thie, M. (2000). Calcium influx in human uterine epithelial RL95-2 cells triggers adhesiveness for trophoblast-like cells. Model studies on signalling events during embryo implantation. Mol. Hum. Reprod. 6, 1119–1130. doi: 10.1093/molehr/6.12.1119
Wang, H., and Dey, S. K. (2006). Roadmap to embryo implantation: clues from mouse models. Nat. Rev. Genet. 7, 185–199. doi: 10.1038/nrg1808
Zhang, R.-J., Zou, L.-B., Zhang, D., Tan, Y.-J., Wang, T.-T., Liu, A.-X., et al. (2012). Functional expression of large-conductance calcium-activated potassium channels in human endometrium: a novel mechanism involved in endometrial receptivity and embryo implantation. J. Clin. Endocrinol. Metab. 97, 543–553. doi: 10.1210/jc.2011-2108
Keywords: proteinase-activated receptors, calcium signaling, endoplasmic reticulum, trypsin, endometrium, ENaC, Ishikawa cells
Citation: Shmygol A and Brosens JJ (2021) Proteinase Activated Receptors Mediate the Trypsin-Induced Ca2 + Signaling in Human Uterine Epithelial Cells. Front. Cell Dev. Biol. 9:709902. doi: 10.3389/fcell.2021.709902
Received: 14 May 2021; Accepted: 21 July 2021;
Published: 09 August 2021.
Edited by:
Madhuri S. Salker, University Hospital Tübingen, GermanyReviewed by:
Ramakrishna Kommagani, Washington University School of Medicine in St. Louis, United StatesChandrasekar Raman, Joslin Diabetes Center and Harvard Medical School, United States
Copyright © 2021 Shmygol and Brosens. This is an open-access article distributed under the terms of the Creative Commons Attribution License (CC BY). The use, distribution or reproduction in other forums is permitted, provided the original author(s) and the copyright owner(s) are credited and that the original publication in this journal is cited, in accordance with accepted academic practice. No use, distribution or reproduction is permitted which does not comply with these terms.
*Correspondence: Anatoliy Shmygol, YS5zaG15Z29sQHVhZXUuYWMuYWU=