- 1NHC Key Laboratory of Parasite and Vector Biology (National Institute of Parasitic Diseases, Chinese Center for Disease Control and Prevention), Shanghai, China
- 2State Key Laboratory of Genetic Engineering, Ministry of Education Key Laboratory of Contemporary Anthropology, Human Phenome Institute, Fudan University, Shanghai, China
- 3Ministry of Education Key Laboratory for Biodiversity Science and Ecological Engineering, Department of Microbiology and Microbial Engineering, School of Life Sciences, Fudan University, Shanghai, China
- 4Department of Infectious Diseases, Huashan Hospital, Fudan University, Shanghai, China
- 5Department of Immunology and Microbiology, Shanghai Jiao Tong University School of Medicine, Shanghai, China
- 6Department of Radiology, Shanghai Public Health Clinical Center, Fudan University, Shanghai, China
Schistosoma japonicum infection showed protective effects against allergic airway inflammation (AAI). However, controversial findings exist especially regarding the timing of the helminth infection and the underlying mechanisms. Most previous studies focused on understanding the preventive effect of S. japonicum infection on asthma (infection before allergen sensitization), whereas the protective effects of S. japonicum infection (allergen sensitization before infection) on asthma were rarely investigated. In this study, we investigated the protective effects of S. japonicum infection on AAI using a mouse model of OVA-induced asthma. To explore how the timing of S. japonicum infection influences its protective effect, the mice were percutaneously infected with cercaria of S. japonicum at either 1 day (infection at lung-stage during AAI) or 14 days before ovalbumin (OVA) challenge (infection at post–lung-stage during AAI). We found that lung-stage S. japonicum infection significantly ameliorated OVA-induced AAI, whereas post–lung-stage infection did not. Mechanistically, lung-stage S. japonicum infection significantly upregulated the frequency of regulatory T cells (Treg cells), especially OVA-specific Treg cells, in lung tissue, which negatively correlated with the level of OVA-specific immunoglobulin E (IgE). Depletion of Treg cells in vivo partially counteracted the protective effect of lung-stage S. japonicum infection on asthma. Furthermore, transcriptomic analysis of lung tissue showed that lung-stage S. japonicum infection during AAI shaped the microenvironment to favor Treg induction. In conclusion, our data showed that lung-stage S. japonicum infection could relieve OVA-induced asthma in a mouse model. The protective effect was mediated by the upregulated OVA-specific Treg cells, which suppressed IgE production. Our results may facilitate the discovery of a novel therapy for AAI.
Introduction
The prevalence of asthma has increased dramatically in the past three decades (Eder et al., 2006; Ali, 2011) and represents a great health burden, especially in developed countries (Barnes, 2004; Thomsen, 2015). Atopic asthma is the most common form of asthma. It is an immunological disorder characterized by inflammation of the airways and lungs triggered by allergen with marked TH2 responses, overactive immunoglobulin E (IgE) production, mucus hypersecretion, and large amount of eosinophil influx to the airways (Lambrecht and Hammad, 2015).
The exact social and environmental factors that lead to the hyperreactive immune disorder is not fully understood. A leading theory behind the rapid rise of allergy and asthma rates is the “hygiene hypothesis,” which suggests that the decreasing incidence of infections in Western countries may contribute to the rise of both autoimmune and allergic diseases (Okada et al., 2010). This hypothesis was supported by an observation showing that the Western lifestyle was linked with significantly higher prevalence of atopic diseases (Herbert et al., 2009). A putative explanation to this phenomenon is that the overall reduction in common TH1-inducing (bacterial, viral, and parasitical) infections results in a decreased ability to counterbalance TH2-polarized allergic diseases (Umetsu, 2012; Yang et al., 2016; Stiemsma and Turvey, 2017). Following this lead, a variety of experimental studies have shown that helminth infections can downregulate host immunity and immunopathology in allergy and other immune disorders (Sitcharungsi and Sirivichayakul, 2013; Maizels, 2016; Maizels and McSorley, 2016). Schistosome was one of the parasites that have been found to have protective effects against autoimmune diseases and allergies such as arthritis and asthma (Osada et al., 2009; Janssen et al., 2016; Qiu et al., 2017). These explorations hold great promise in identifying a new and more specific intervention measure for atopic asthma that does not result in certain side effects, such as increased susceptibility to infection and necrosis, which can be triggered by steroid hormone drugs such as dexamethasone (Kuprys-Lipinska and Kuna, 2014; Al-Ahmad et al., 2018; Falk, 2018).
Schistosome is an ancient parasite affecting more than 230 million people in 78 tropical and subtropical countries (LoVerde, 2019). During its life stages in definitive hosts, schistosome invades its mammalian hosts through the skin, migrates from skin to lung, and then develops and matures in the liver, finally residing in the mesenteric venules (McManus et al., 2018). Although it has been shown by multiple studies that schistosome could abate allergic airway inflammation (AAI), the understanding of its underlying mechanisms remains limited. Most previous studies focused on testing the preventive effect (infection before allergen sensitization) of the Schistosoma japonicum infection against allergic asthma. Under this setting, controversial results have been reported regarding both the timing of the infection (acute vs. chronic) (Smits et al., 2007; van der Vlugt et al., 2012; Layland et al., 2013) and the effector component (egg vs. worms) (Mangan et al., 2006; Pacifico et al., 2009; Obieglo et al., 2018), which reflects the complexities of the schistosome life cycle and its immune regulatory components. Moreover, contradictory results were also reported regarding the roles of regulatory T cells (Treg cells) in schistosome-mediated protection. Some studies showed that Treg cell was an important effector in schistosome-mediated protection against asthma (Medeiros et al., 2003; Smits et al., 2007; Pacifico et al., 2009; Layland et al., 2013; Zhang et al., 2019), whereas a more recent study showed that the protection was independent of Treg cells (Obieglo et al., 2018).
Unlike previous studies that were focused on testing the preventive effect (infection before allergen sensitization) of S. japonicum infection against allergic asthma, the primary goal of this study was to investigate the protective effect of S. japonicum infection on asthmatic inflammation (infection after allergen sensitization) and to clarify the underlying mechanism. To this aim, mice were percutaneously infected with cercaria of S. japonicum at either 1 day before OVA-induced asthma attack (infection at lung-stage during AAI) or 14 days before OVA-induced asthma attack (infection at post–lung-stage during AAI). We found that only lung-stage S. japonicum infection could upregulate the frequency of allergen-specific Treg cell, which significantly alleviated AAI by inhibiting IgE production and inflammatory cytokine secretion.
Materials and Methods
Ethics Statement
All experiments and methods were performed in accordance with relevant guidelines and regulations. Mice experiments were carried out at the National Institute of Parasitic Disease, Chinese Center for Disease Control and Prevention (NIPD, China CDC) in Shanghai, China. All animal experiment protocols used in this study were approved by the Laboratory Animal Welfare & Ethic Committee of the National Institute of Parasitic Diseases (permit no. IPD-2016-7).
OVA-Induced AAI and S. japonicum Infection
Female BALB/c mice (6–8 weeks old) were randomly divided into six groups in this experiment, which were OVA-induced AAI (OVA) group, OVA-induced AAI with lung-stage S. japonicum infection (OVA + INF, lung stage) group, OVA-induced AAI with post–lung-stage infection (OVA + INF, post–lung stage) group, and OVA-induced AAI with dexamethasone (DXM) treatment (OVA + DXM) group, as well as infection (INF) group and normal (NOR) group. For OVA-induced AAI, the mice were sensitized by injecting 10 μg of alum-adjuvanted ovalbumin (OVA; cat. # 77120 and 77161; Thermo Fisher, United States) intraperitoneally on days 0 and 14. Subsequently, the mice were challenged with aerosolized OVA [1% in phosphate-buffered solution (PBS)] for 30 min in the chamber of a Medical Compressor Nebulizer (DEDAKJ, Germany) on days 21 to 24 (Figures 1A,B). The mice of the normal control and S. japonicum infection control groups were challenged with PBS. To test the protective effect of infection on OVA-induced AAI, mice were infected with 15 cercaria of S. japonicum at either 1 day (infection within 6 days: lung-stage infection) or 14 days before OVA-induced asthma attack (20 days within infection: post–lung-stage infection).
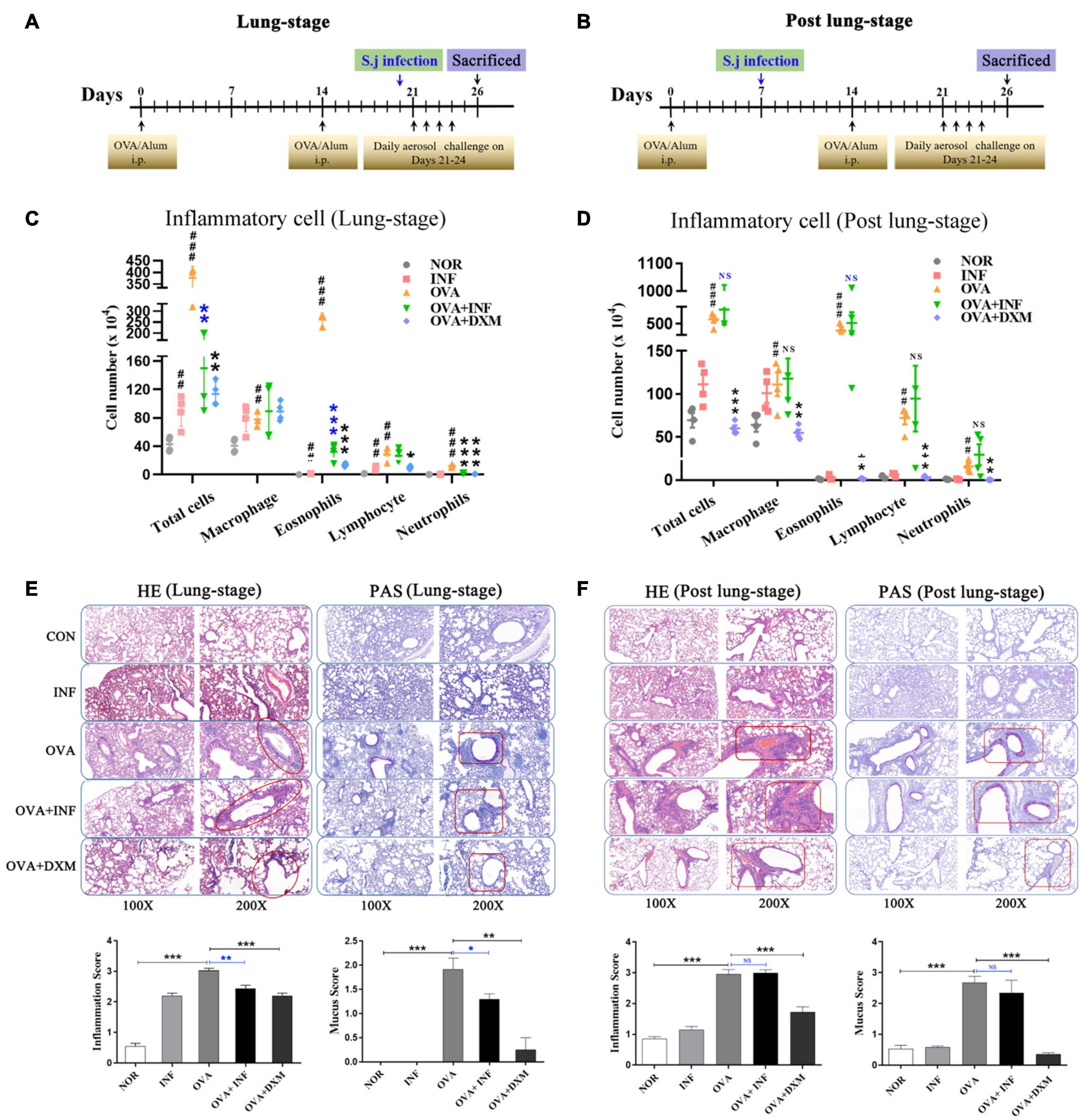
Figure 1. Lung-stage S. japonicum infection alleviated the attack of OVA-induced AAI, whereas post–lung-stage infection did not. Experimental design of OVA-induced AAI treated with either lung-stage (A) or post–lung-stage (B) S. japonicum infection in murine model. (C,D) Inflammatory cell (total cell, macrophage, eosnophils, lymphocytes, neutrophils) infiltration in BALF of mice after OVA challenge was compared in NOR, INF, OVA, OVA + INF, and OVA + DXM groups (n = 5 or 6 mice per group, experiment performed twice). (E,F) Representative images of H&E and PAS staining of lung tissue after OVA challenge. Statistical analysis of inflammation score and mucus secretion score are also shown in (E,F), respectively, among five groups (n = 5 or 6 mice per group, experiment performed twice). NOR, normal mice (without OVA sensitization and challenge); INF, mice without OVA sensitization and challenge but infected with schistosome; OVA, mice with OVA sensitization and challenge but without S. japonicum infection; OVA + INF, mice sensitized and challenged with OVA and treated with S. japonicum infection; OVA + DXM, mice sensitized and challenged with OVA and treated with dexamethasone. All data are shown as mean ± SEM. *P < 0.05, **P < 0.01; NS, not significant by one-way analysis of variance (ANOVA) with Tukey test. #, ##, and ### indicate P < 0.05, <0.01, and <0.001, respectively, OVA versus NOR (C,D). *, **, *** indicated P < 0.05, <0.01, and <0.001, respectively, OVA + INF or OVA + DXM versus OVA (C,D).
Bronchoalveolar Lavage Collection and Cell Counting
Mice were euthanized 48 h after the last aerosolized OVA challenge (day 26), and bronchoalveolar lavage fluids (BALFs) were collected as previously reported method (Li et al., 2012). Briefly, after euthanasia, tracheotomy was carried out, and an arteriovenous indwelling needle (20-gauge; BRAUN, Germany) was inserted into the trachea. Lavages were collected by washing the lung twice with 0.3 mL PBS. Cells in BALFs were harvested after centrifugation, and the supernatants were stored at –80°C for cytokine detection. Cell pellet was fixed with paraformaldehyde (4%) and stained with a hematoxylin–eosin (H&E). A total of 1,000 cells from multiple fields were examined for each slide. Counts of total cells, eosinophils, macrophage, neutrophils, and lymphocytes were performed on blinded samples, as described previously (Chang and Yen, 2004).
Lung Histopathology
Lung tissues were fixed in 4% phosphate-buffered formaldehyde overnight and then embedded in paraffin and cut for H&E and periodic acid–Schiff (PAS) staining. Images of the stained sections were captured with a NIKON DS-U3 microscope (NIKON, Japan). Lung inflammation and the intensity of goblet cell metaplasia were assessed and scored 0 to 4 by two blinded, independent investigators, as described previously (Hopfenspirger and Agrawal, 2002).
Determination of Total and OVA-Specific IgE in Serum
The levels of total and OVA-specific IgE in serum were measured using enzyme-linked immunosorbent assay. Briefly, Maxisorp 96-well microtiter plates (Thermo Fisher Scientific, United States) were coated with rat monoclonal anti-mouse IgE antibody for total IgE detection (1:1,000; cat. # ab99571, Abcam, United Kingdom) or 10 μg/mL OVA for OVA-specific IgE (cat. # A5503, Sigma, United States) 100 μL/well, respectively, in carbonate–bicarbonate buffer, pH 9.6, for 12–16 h at 4°C. Then, the plates were blocked for at least 2 h at 37°C with 100 μL/well of PBS plus bovine serum albumin (BSA) (1%). After wash, 100 μL serum diluted with PBS containing 0.05% Tween 20 (PBST) (1:40 for total IgE; 1:5 for OVA-specific IgE) was added to each well and incubated at 37°C for 2 h. Next, horseradish peroxidase–labeled goat anti-mouse IgE antibody was diluted with PBST (1:2,000; cat. # ab99574, Abcam, United Kingdom) and added to each well at 100 μL/well. After 2 h of incubation at 37°C, the plates were washed with PBST five times. Finally, color was developed by addition of 100 μL/well of TMB (cat. # PA107, TIANGEN, China), and after incubation at room temperature for maximal 30 min, the reaction was stopped with 5% sulfuric acid (50 μL/well). Optical density values were determined at 450 nm using the multimode microplate readers (BioTek, United States). The concentration of total IgE was then calculated according to the standard curve.
Cytokine Detection in BALFs
Levels of interleukin 4 (IL-4), IL-5, IL-13, IL-10, eotaxin, and interferon γ (IFN-γ) in BALFs were measured using a custom-made Bio-Plex Pro Reagent Kit V (6-plex customization) (cat. # MHSTCMAG-70K, Wayen Biotechnologies, China) according to the manufacturer’s instructions. The fluorescence-labeled beads were detected using a corrected Bio-Plex MAGPIX system (Bio-Rad, Luminex Corporation, Austin, TX, United States), and the cytokine concentrations were calculated using Bio-Plex manager 6.1 (Bio-Rad).
Lymphocyte Isolation From Lung Tissues
After collection, lung tissues were washed three to four times with RPMI (Roswell Park Memorial Institute) medium, minced to tiny pieces, and then digested in 0.1% type IV collagenase (cat. # C8160, Solarbio, China) solution at 37°C for 30 min. Digested lung tissues were filtered through a 70-μm cell strainer, and erythrocytes were lysed with a red blood cell lysis buffer (cat. # R1010, Solarbio, China).
Flow Cytometry Assay
Single-cell suspensions were stained with a panel of surface monoclonal antibodies (mAbs) in FACS buffer (PBS containing 2 mM EDTA and 0.5% BSA) for 30 min on ice, including fluorescein isothiocyanate (FITC)–conjugated anti-CD4 (clone # 88-8111-40, eBioscience, United States), APC-conjugated anti-CD25 mAb (clone # 88-8111-40, eBioscience, United States), SuperBright645-conjugated anti-CD45.1 (clone # 64-0453-82, eBioscience, United States), and Pe-cyanine7–conjugated anti-CD45.2 (clone # 25-0453-82, eBioscience, United States). Subsequently, cells were fixed with fix/perm buffer (clone # 88-8111-40, eBioscience, United States) on ice for 20 min, and then stained with mAbs targeting intracellular markers in a Perm/wash buffer for 30 min on ice. For the detection of Treg cell, PE-labeled anti-Foxp3 mAb (clone # 88-8111-40, eBioscience, United States) was used. For detecting OVA-specific IL-4 and IFN-γ secretion, isolated lymphocytes were initially stimulated for 6 h with 5 μg/mL OVA peptide (323-339) (China peptides, China); leukocyte activation cocktail, with BD GolgiPlug (BD, United States) was added for another 4 h and then stained with mAbs Perp-cy5.5–conjugated anti-CD3 (clone # 145-2C11, eBioscience, United States) and FITC-conjugated anti-CD4 (clone # 88-8111-40, eBioscience, United States) for 30 min on ice. Subsequently, cells were fixed with fix/perm buffer (clone # 88-8111-40, eBioscience, United States) on ice for 20 min. Then PE-conjugated anti–IL-4 (clone # 12-7041-81, eBioscience, United States) or APC-conjugated anti-IFN-γ (clone # 17-7311-81, eBioscience, United States) for 30 min on ice was used. Finally, after two washes, all cells were resuspended in PBS containing 1% paraformaldehyde and subjected to flow cytometry analysis (Cytometer LX, Beckman).
Adoptive Transfer of Naive CD4+ T Cells
Naive CD4+ T cells of CD45.1+ OT II mice were purified using EasySep Mouse Naive CD4+ T Cell Isolation Kit (cat. # 19765, StemCell, United States) according to the manufacturer’s protocol. The purity of isolated cells was checked by flow cytometry and was confirmed to be >85%. Freshly purified naive CD4+ T cells were suspended in PBS and injected intravenously into CD45.2+ congenic C57BL/6 recipient mice, 1 × 106 cells/mouse. The induction of AAI and S. japonicum infection was performed as described above.
In vivo Depletion of Treg Cells
Anti-CD25 antibody clone PC61 has been widely used to deplete Treg cells for characterizing Treg cell function in vivo (Setiady et al., 2010); 100 μg/mouse anti-CD25 antibody (cat. # 16-0251-85, clone # PC61.5, eBioscience, United States) or isotype IgG (cat. # 16-4301-85, clone # eBRG1, eBioscience, United States) was dissolved with 150 μL sterile PBS and injected intravenously into the mice 21 days after OVA sensitization. A second shot of 50 μg/mouse antibodies was given on day 23 after OVA sensitization (Figure 7A). After depletion, the mice were randomly divided into two groups: OVA + INF + αCD25 and OVA + INF + IgG. OVA sensitization, aerosol challenge, and S. japonicum infection were performed as described above.
RNA Sequencing
Total RNA was extracted from lung tissues by using Trizol reagent (cat. # 15596026, Invitrogen). RNA purity was checked using the Nano Photometer spectrophotometer (IMPLEN, CA, United States). RNA integrity was assessed using the RNA Nano 6000 Assay Kit of the Bioanalyzer 2100 system (Agilent Technologies, CA, United States); 1 μg total RNA from each sample was used to construct the sequencing library using Poly(A) mRNA Capture Module (cat. # RK20340, Abclonal, United States) and Fast RNA-seq Lib Prep Module for Illumina (cat. # RK20304, Abclonal, United States). Index codes were added to attribute sequences of each sample. Then, the libraries were sequenced on Illumina Novaseq platform [2 × 150 base pairs (bp)]. A total of seven samples, three from the OVA group and four from the OVA + INF group, were sequenced in one lane, producing more than 30 million reads per library.
Differential Expression Genes Analysis and Functional Enrichment Analysis
Sequencing quality was evaluated by FastQC software1. Poor-quality reads and adaptors were trimmed by Trimmomatic software (released version 0.222), and only reads longer than 50 bp were used for further analysis. The high-quality reads were mapped to mouse genome (mouse BALB/cJ) downloaded in Ensembl database. The HTseq (Anders et al., 2015) was used to quantify gene expression, and R DEseq2 package (Anders and Huber, 2010) was employed for differential expression analysis. Only genes with false discovery rate (FDR) adjusted P < 0.05 and absolute value of fold change > 2 were considered as differential expression genes (DEGs). Functional enrichment of Gene Ontology (GO) terms and Kyoto Encyclopedia of Genes and Genomes analyses of DEGs were conducted by R Cluster Profiler package (Yu et al., 2012) with FDR correction. Significantly enriched GO terms and KEGG pathways were identified with corrected P < 0.05. DEG-related pathways enrichment terms were performed with the Panther Classification System3.
Data and materials availability: RNA sequencing data are deposited in the SRA database SRA (SRA accession no. PRJNA609083).
Statistical Analysis
All statistical analyses were performed using GraphPad Prism 8.0 (GraphPad Software, Inc., San Diego, CA, United States). The data of quantitative variables were presented as mean ± standard error of mean (SEM). P < 0.05 was considered statistically significant.
Results
Lung-Stage S. japonicum Infection Ameliorated OVA-Induced AAI in a Murine Model
A mouse model of OVA-induced AAI was adopted to test the protective effect of S. japonicum infection on allergic asthma (Figures 1A,B). Compared to the control group, mice in the OVA group showed significant infiltration of inflammatory cells in BALFs (Figures 1C,D), which resembled the main clinical feature of AAI (Persson, 2019). Moreover, after S. japonicum infection, the results showed that the lung-stage infection significantly reduced the infiltration of inflammatory cells, especially eosinophils (Figure 1C), whereas post–lung-stage infection did not (Figure 1D). Histopathologic examination further confirmed the above findings by showing that lung-stage infection significantly suppressed the OVA-induced eosinophil-rich leukocyte infiltration and mucus hypersecretion (Figure 1E), whereas post–lung-stage infection showed no obvious protective effect (Figure 1F).
Lung-Stage S. japonicum Infection Inhibited IgE Production and Suppressed TH2 Cytokine Secretions
IgE is the key factor mediating the pathological immune responses that lead to allergic asthma (Galli and Tsai, 2012). To further characterize the protective effects of S. japonicum infection, we measured the total and OVA-specific IgE in serum of mice. The results showed that lung-stage infection significantly downregulated both the total and OVA-specific IgE to levels comparable with DXM treated mice (Figures 2A,B). In contrast, post–lung-stage infection tended to elevate the total and OVA-specific IgE levels despite no significant difference was reached (Figures 2C,D). Moreover, we also measured a panel of cytokines and chemokines in BALFs and found that lung-stage infection altered the cytokine/chemokine secretion pattern induced by aerosolized OVA challenge (Figure 3A and Supplementary Figure 1). More specifically, IL-5 and eotaxin were reduced to levels that are similarly attained with DXM treatment (Figure 3B). On the contrary, post–lung-stage infection increased IL-4 and IL-5 secretion (Figure 3B).
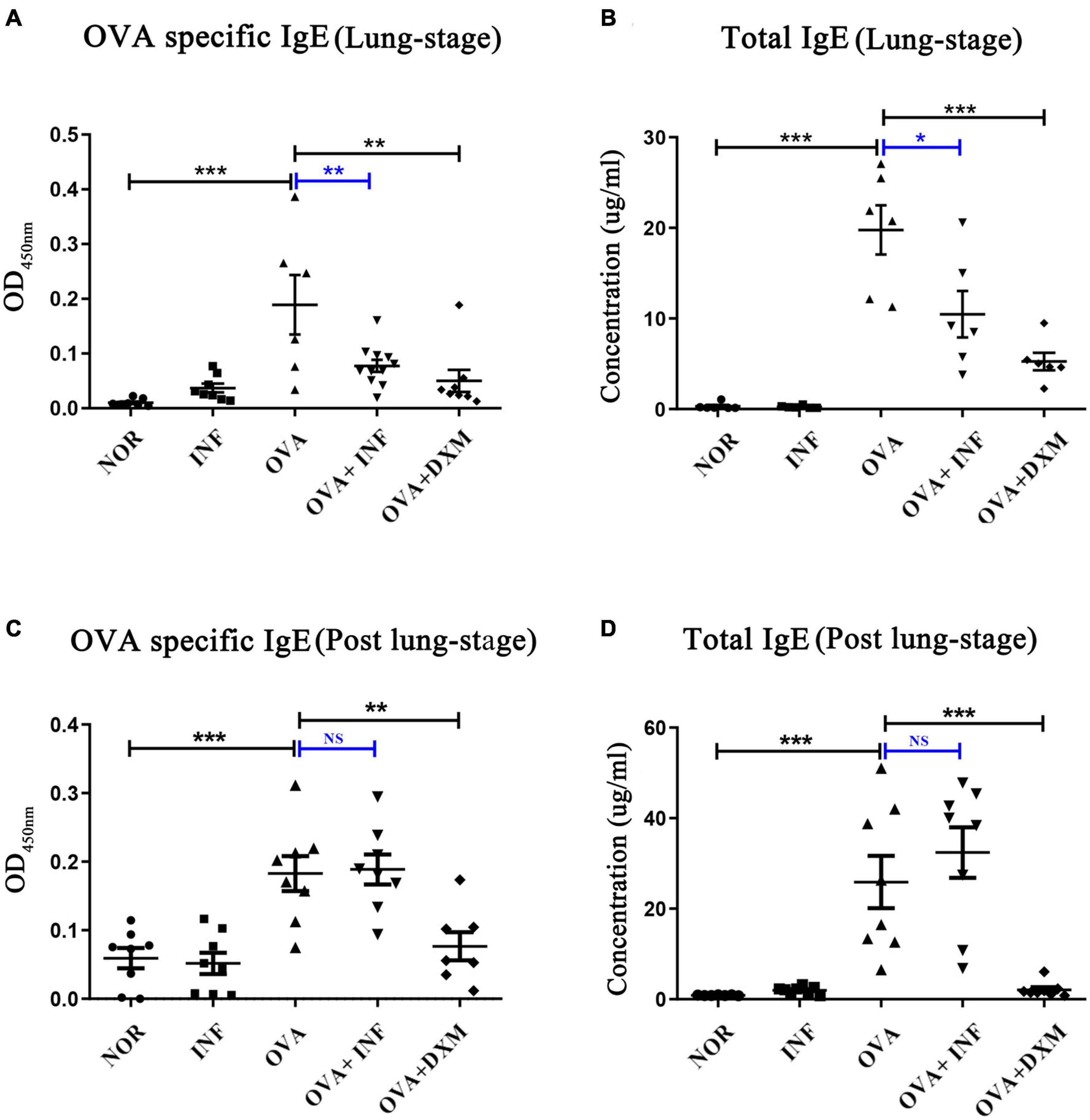
Figure 2. Lung-stage S. japonicum infection suppressed both the total and OVA-specific IgE after OVA challenge, whereas post–lung-stage infection did not. (A,C) OVA-specific IgE in sera were measured by enzyme-linked immunosorbent assay from NOR, INF, OVA, OVA + INF, and OVA + DXM groups (n = 5 or 6 mice per group, experiment performed twice). (B,D) The concentrations of total IgE in mouse serum were compared among all groups after OVA challenge (n = 5 or 6 mice per group, experiment performed twice). All data are shown as mean ± SEM. *P < 0.05, **P < 0.01, ***P < 0.001; NS, not significant by one-way analysis of variance (ANOVA) with Tukey test.
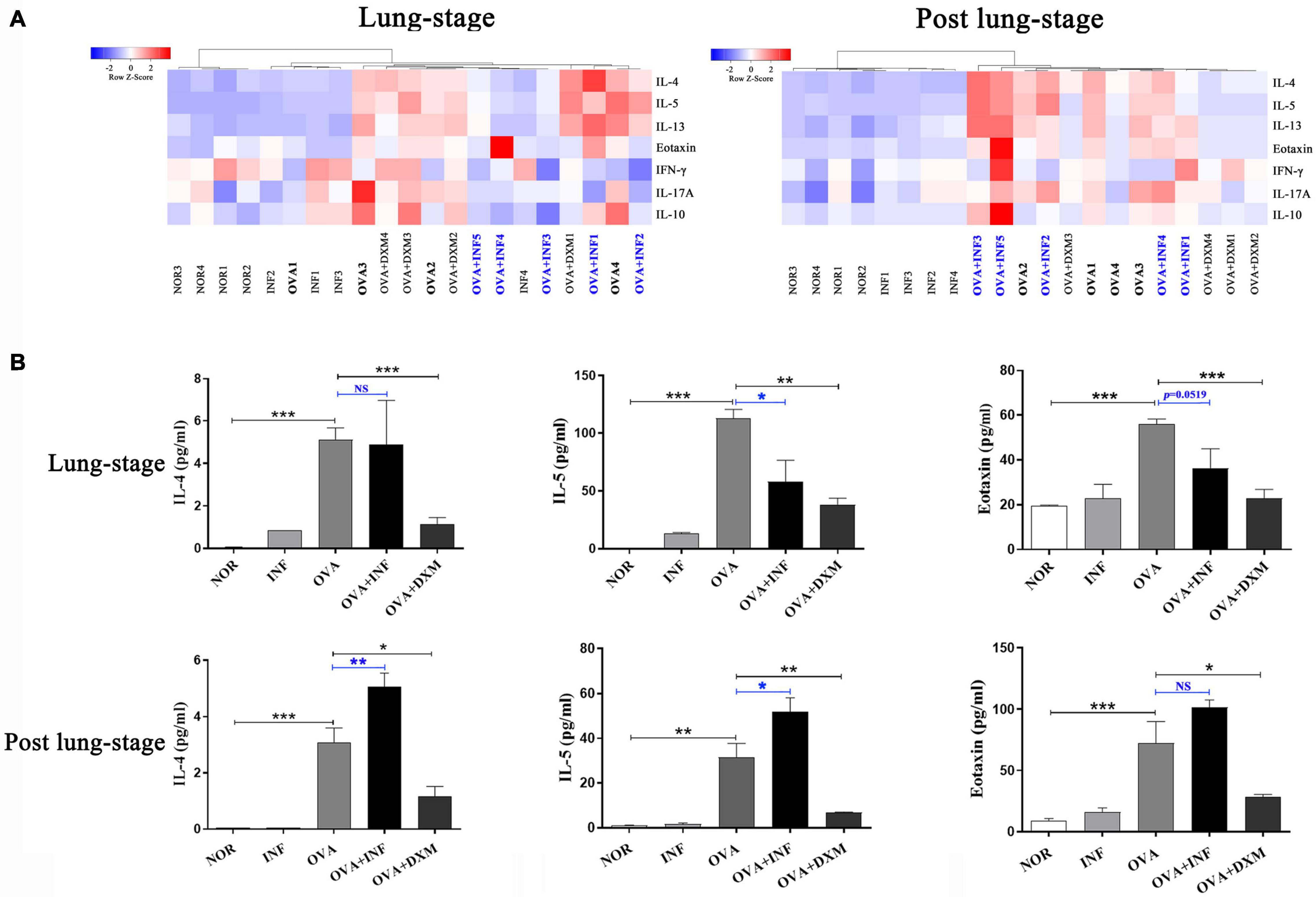
Figure 3. Lung-stage S. japonicum infection inhibited TH2 cytokine secretion after OVA challenge, while post–lung-stage infection did not. (A) Heatmaps of multiple cytokines in BALF of mice treated with lung-stage S. japonicum infection (left) and post–lung-stage S. japonicum infection (right) after OVA challenge by Luminex (n = 5 or 6 mice per group, experiment performed twice). (B) Concentrations of IL-4, IL-5 and eotaxin in BALFs were compared among all groups (n = 5 or 6 mice per group, experiment performed twice). Data are shown as mean ± SEM. *P < 0.05, **P < 0.01, ***P < 0.001; NS, not significant by one-way analysis of variance (ANOVA) with Tukey test.
Lung-Stage S. japonicum Infection Upregulated the Frequencies of Treg Cells Especially OVA-Specific Treg Cells in Lung
Treg cell was suggested to be a key factor of S. mansoni–mediated protection against AAI (Layland et al., 2013). Here, we first assessed the frequencies of total Treg cells (CD4+CD25+Foxp3+ Treg cell) in spleen and lung. As shown in Figure 4A, lung-stage infection upregulated the frequency of total Treg cells both in lung and spleen (Figure 4A), whereas post–lung-stage infection only slightly improved the proportion of Treg cells in the spleen (Figure 4B). Then, by adoptive transfer of OVA-specific naive CD4+ T cells (CD45.1+) into wide-type CD45.2+ mice (Figure 5A), we found that the frequency of OVA-specific Treg cells (CD45.1+ Treg) in lung increased by more than threefold after S. japonicum infection (P < 0.001), whereas the frequency of endogenous Treg cells (CD45.2+ Treg cell) in lung was not significantly improved (Figures 5B,C). The proportion of total Treg cells was increased in lung and LDLNs after S. japonicum infection (Figures 5B,C).
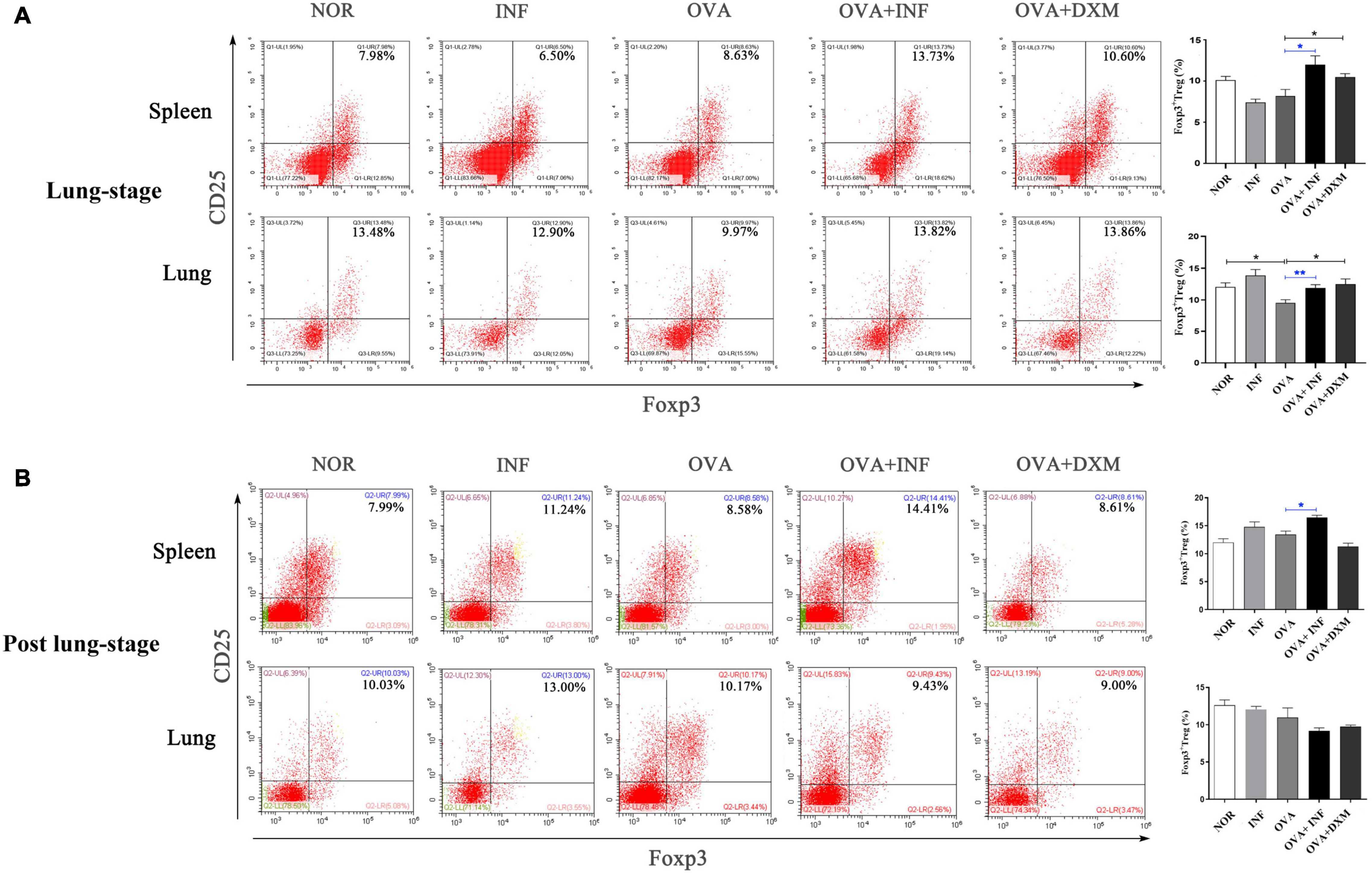
Figure 4. Lung-stage S. japonicum infection upregulated Treg cell frequency in lung and spleen after OVA challenge. (A,B) Comparisons of Treg cell populations (CD4+CD25+Foxp3+ Treg cell) in lungs and spleens among all groups. Representative data of flow cytometry analysis for each group are shown together with statistical comparisons. n = 5 or 6 mice per group, experiment performed twice. *P < 0.05, **P < 0.01 by one-way analysis of variance (ANOVA) with Tukey test.
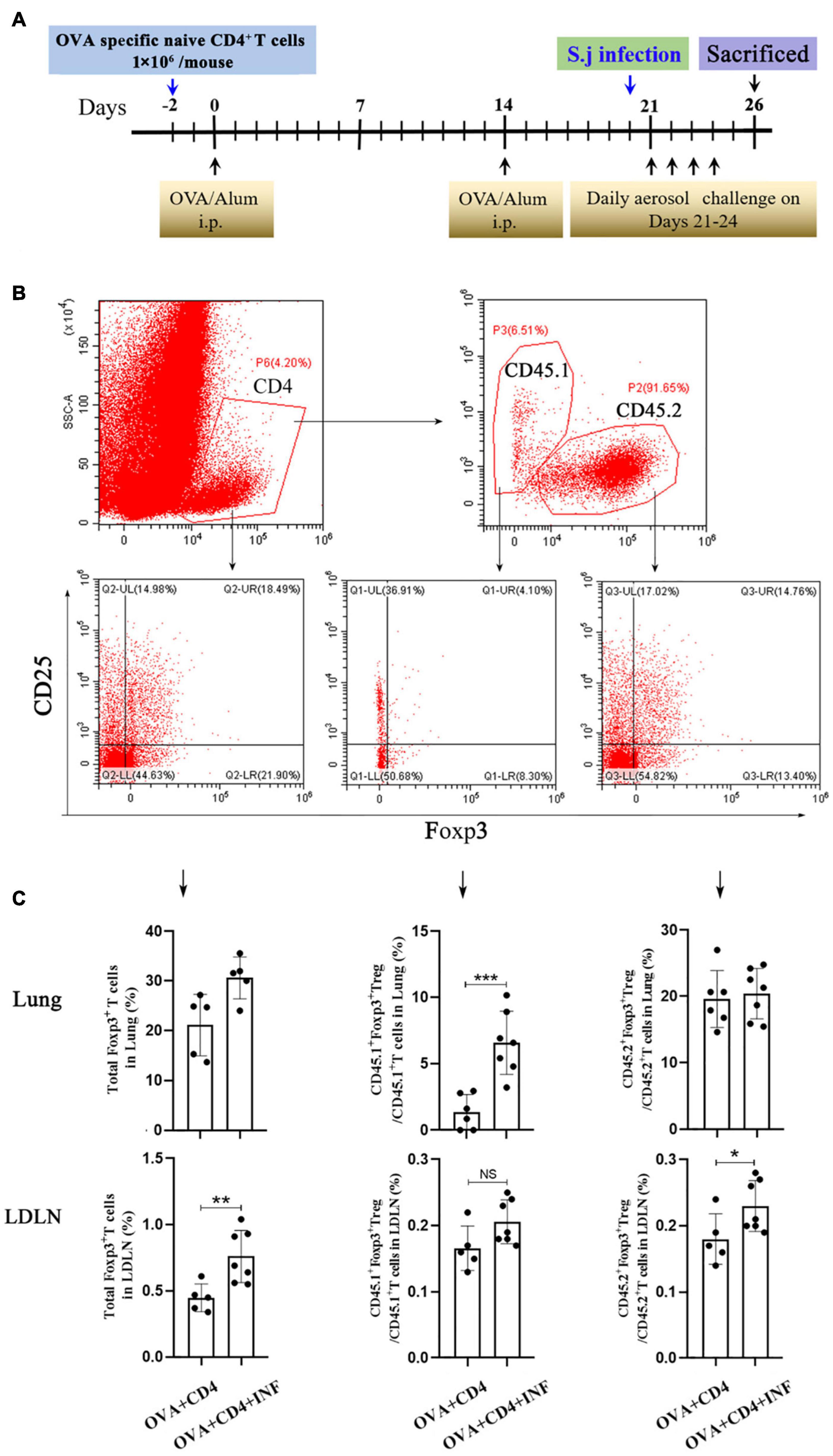
Figure 5. Lung-stage S. japonicum infection upregulated OVA-specific Treg cells after OVA challenge. (A) Design of experiment for testing the protective effect of lung-stage S. japonicum infection on OVA-induced AAI after adoptive transfer of OVA-specific naive CD4+ T cells. (B,C) Gate strategy and statistical comparisons of flow cytometry analysis for total Treg, CD45.1+ Treg cells (OVA-specific) and CD45.2+ Treg cells in lung and lung draining lymph nodes (LDLN). n = 5 or 6 mice per group, experiment performed twice. *P < 0.05, **P < 0.01, ***P < 0.001; NS, not significant by the one-way analysis of variance (ANOVA) with Tukey test.
We also found that the ratio of OVA-specific IL-4+ versus IFN-γ+ CD4+ T cells significantly decreased after lung-stage S. japonicum infection (Supplementary Figure 2), suggesting that specific CD4+ T cell responses shifted from TH2 toward TH1 responses.
The Protective Effect of Lung-Stage S. japonicum Infection Was Treg Cell–Dependent
Significant negative correlations between the frequency of Treg cells and OVA-specific IgE or IgG (Figure 6) were observed, indicating that the protective effect of S. japonicum infection on AAI might be mediated by Treg cell. To elucidate the role of Treg cell, we performed in vivo depletion of Treg cells using anti-mouse CD25 antibody (Figure 7A). The efficiency of Treg cell deletion is demonstrated by the significantly reduced percentage of CD25+ Treg cells as shown in Supplementary Figure 3. Our data showed that Treg depletion (OVA + INF + αCD25 group) aggravated OVA-induced AAI compared to isotype control group. Inflammatory cell infiltration, mucus secretion (shown by PAS staining), OVA-specific IgE production, and eotaxin secretion significantly increased after Treg depletion (Figure 7).
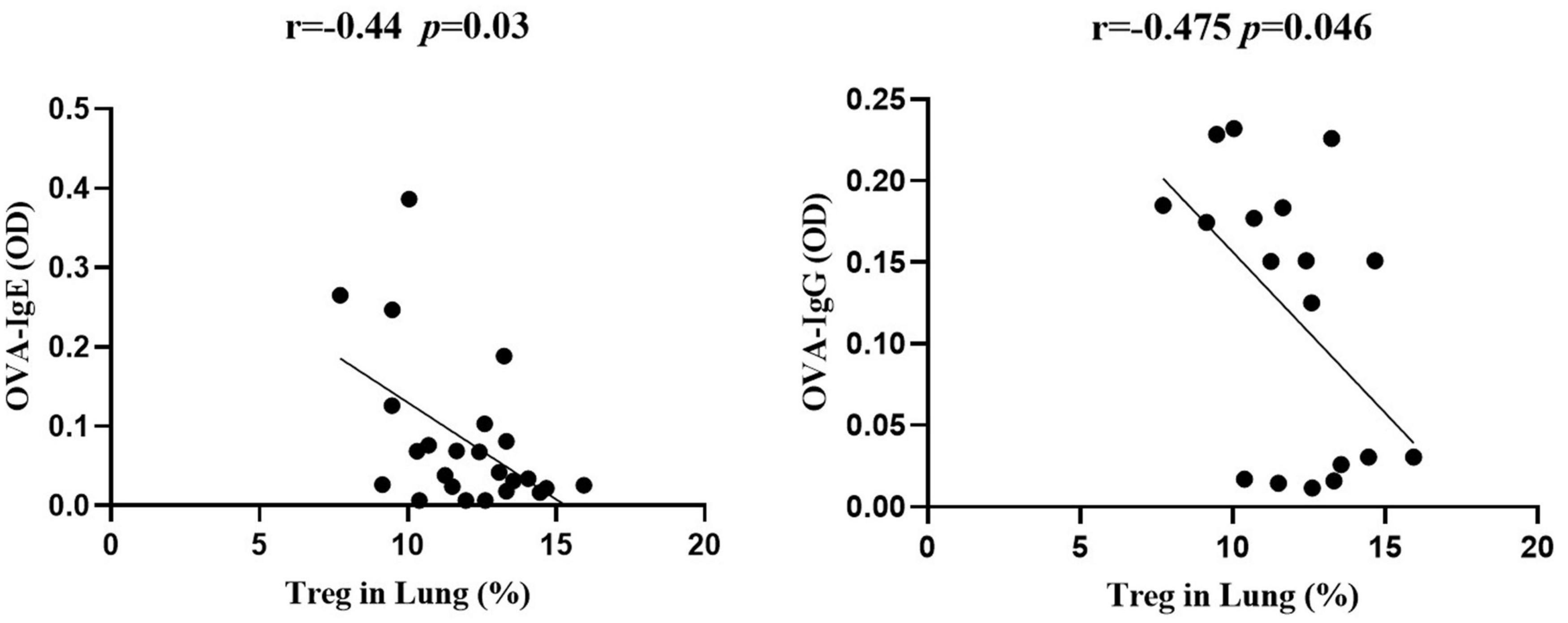
Figure 6. The frequency of Treg cells in lung negatively correlated with OVA-specific IgE and IgG. Correlation analysis between Treg cell frequency in lung and the optical density values of OVA-specific IgE (left) and IgG (right) in serum (n = 5 or 6 mice per group, experiment performed twice).
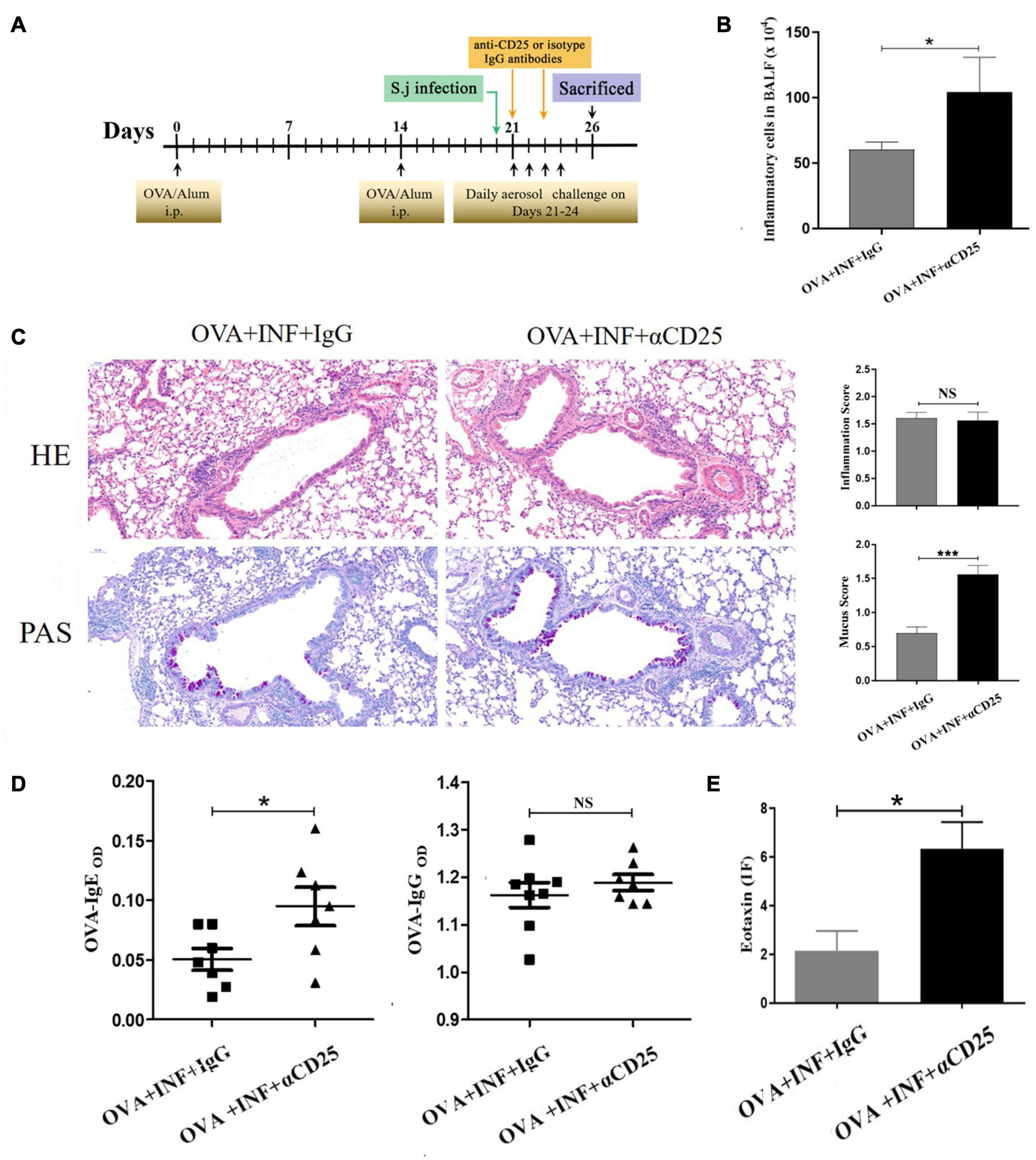
Figure 7. In vivo depletion of Treg cells counteracted the protective effect of lung-stage S. japonicum infection on OVA-induced AAI. (A) Design of experiment for testing the role of Treg cells in the protective effect mediated by lung-stage S. japonicum infection. (B) Comparisons of total inflammatory cell counts in BALF between lung-stage schistosome-infected mice treated with either anti-CD25 antibody or isotype control IgG (n = 8 mice per group, experiment performed twice). (C) Lung histopathology analysis of lung-stage schistosome–infected mice treated with either anti-CD25 antibody or isotype control IgG. Upper, H&E staining; lower, PAS staining (n = 8 mice per group, experiment performed twice). (D) Comparisons of OVA-specific IgE and IgG in sera between Treg cell–depleted and control mice (n = 8 mice per group, experiment performed twice). (E) Comparisons of eotaxin levels in BALF between lung stage–infected mice treated with either anti-CD25 antibody or isotype control (n = 8 mice per group, experiment performed twice). Data are shown as mean ± SEM. *P < 0.05, ***P < 0.001 by one-way analysis of variance (ANOVA) with Tukey test.
Lung-Stage S. japonicum Infection Molded the Microenvironment to Facilitate the Generation of Treg Cells
To reveal factors that contributed to the induction of Treg cells upon lung-stage S. japonicum infection, we created transcriptomic profiles of the lung tissues from the schistosome infected and non-infected mice after OVA challenge. The results showed that 203 genes were upregulated, and 279 genes were downregulated after lung-stage S. japonicum infection (Figure 8A and Supplementary Data File 1). GO analysis of DEGs showed that the top three terms of significantly enriched genes (P < 0.05) are mainly distributed in the T cell activation, the leukocyte proliferation, and the regulation of leukocyte proliferation (Figure 8B) pathways. Panther analysis showed that 84 DEGs are related to immune system processes (Supplementary Figure 4), and 70 of them were downregulated (Supplementary Data File 1). Further analysis showed that three genes (CD46, Epor, and Klra17) reported to promote Treg cell response were upregulated (Gehrie et al., 2011; Tsai et al., 2012; Purroy et al., 2017), and eight genes (Clec7a, CCR6, Spi-B, ABCG1, ADA, Ctsk, Ctss, and Ptgir) reported to inhibit Treg cell response were downregulated (Liu et al., 2013; Tang et al., 2015; Cheng et al., 2016; Naval-Macabuhay et al., 2016; Rauch et al., 2016; Yan et al., 2017; Zhou et al., 2017; Kulkarni et al., 2018; Figure 8C and Table 1) in schistosome-infected mice. We postulated that lung-stage S. japonicum infection generated a microenvironment facilitating Treg cell development in lung (Figure 8C).
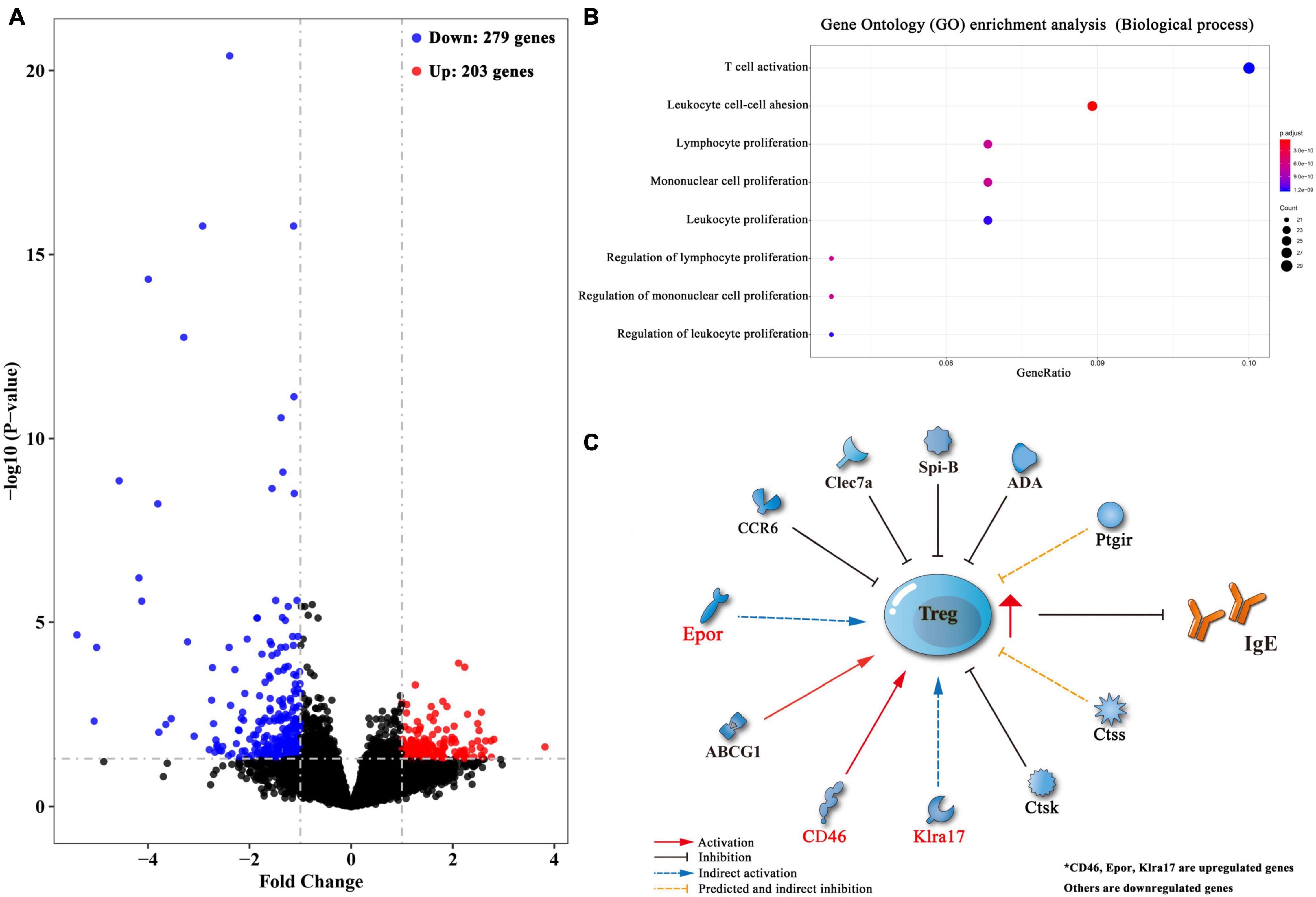
Figure 8. Transcriptomic analysis of differentially expressed genes (DEGs) between lung tissues of OVA-induced asthmatic mice treated with and without lung-stage S. japonicum infection. (A) Volcano plot of detected gene transcription profile in lung tissues of OVA-induced asthmatic mice treated with lung-stage schistosome infection compared with no-treatment control mice after OVA challenge. (B) The top eight functional enrichment pathways of Gene Ontology (GO) analysis for biological process in DEGs (P < 0.05). (C) Predicted gene network that might promote the generation of Treg cells in DEGs. Data were from at least three individuals per group per experiment, experiment performed twice.
In addition, we found that eight genes (DOCK2, IRF4, Rac2, Lgals3, H2-Oa, Pdcd1lg2, Sash3, and Mzb1) related to B cell function or differentiation (Croker et al., 2002; Scheikl et al., 2009; Flach et al., 2010; Gu et al., 2013; Peng and Eckhardt, 2013; de Oliveira et al., 2018; Jing et al., 2019; Low et al., 2019) were also downregulated after S. japonicum infection (Table 2), which might potentially contribute to the inhibition of IgE response. Genes related to lung development (FOXF1, ANO9, TRIM6, MMP27, Epor, Gata1, and Serpina) (Nuttall et al., 2004; Rock et al., 2008; Sato et al., 2012) and cell integrity (Villin and CRB1) (Mehalow et al., 2003; Khurana and George, 2008) were also found to be upregulated too, which indirectly supported the observed protective effect of S. japonicum infection (Table 2).
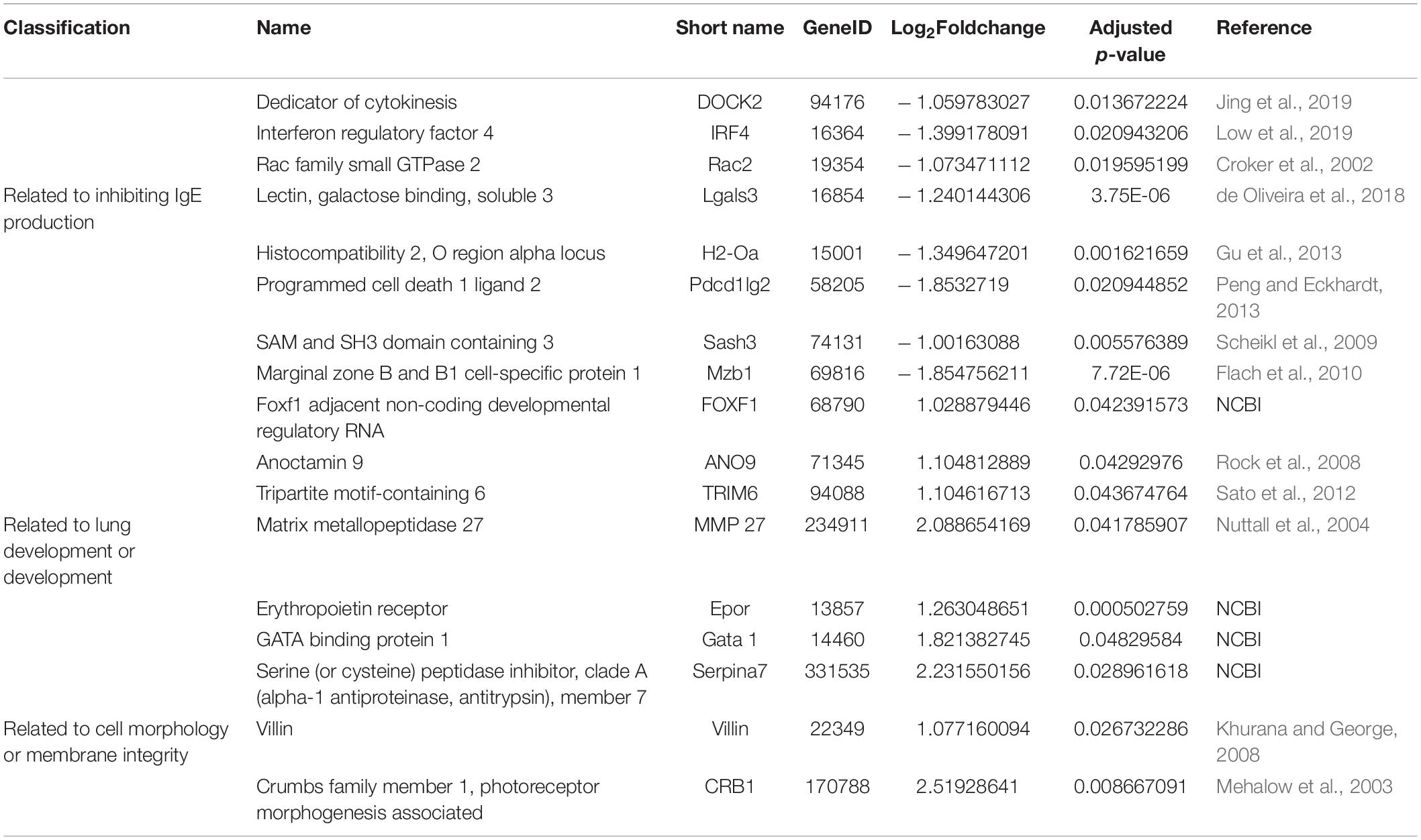
Table 2. Differential expression genes reported to facilitate B cell or plasma cell, lung development, and cellular morphology.
Discussion
The eradication of helminths (and other pathogens) is suggested to have resulted in decreased immune-regulatory ability, which might be the cause of the increasing prevalence of allergic and autoimmune disorders especially in developed and urbanized countries (de Ruiter et al., 2017; Harnett and Harnett, 2017; Bach, 2018). The protective effect of parasitic infection against allergies and autoimmune disease has been extensively explored especially after the hygiene hypothesis was introduced into this field (Maizels et al., 2014), among which the immunoregulation of schistosome is best illustrated (Capron, 2011; Layland et al., 2013; Qiu et al., 2017).
In this study, to investigate how the timing of S. japonicum infection influenced the development of allergic asthma, we compared the protective effect of two phases of S. japonicum infection: lung stage and post–lung stage. We found that lung-stage S. japonicum infection significantly relieved OVA-induced AAI, but post–lung-stage infection showed no protective effect. Within lung-stage infection (3–7 days postinfection), schistosomula transformed from cercaria were completely located in lung tissue of the host (Rheinberg et al., 1998), which might modulate the local immune response to abate OVA-induced AAI. We postulated that this might be the reason that the protective effect of lung-stage infection was superior to post–lung-stage infection. And indeed, we found that lung-stage infection significantly upregulated Treg cell response in lung tissues.
Multiple factors such as worm species, timing, intensity and chronicity of infection, and host genetics have been investigated to illustrate the mechanisms of helminth-mediated regulation of host immunity (Cooper, 2009). Nonetheless, the relationship between helminths and asthma still remains not well understood. Mechanistic studies reported contradictory results; for example, one study showed that S. mansoni–mediated suppression of AAI was patency dependent and mediated by infection-induced Treg cells (Layland et al., 2013), whereas another study showed that protection mediated by S. mansoni egg was independent of either Treg cells or Breg cells (Obieglo et al., 2018). In the current study, we found that lung-stage S. japonicum infection that occurred during OVA challenge could upregulate the frequency of Treg cells and suppress OVA-specific IL-4 response. Upregulation of Treg cells by S. japonicum infection has been reported by few previous studies (Baru et al., 2010; Layland et al., 2013); however, to our knowledge, this is the first proof showing that the lung-stage S. japonicum infection can upregulate allergen OVA-specific Treg cell.
To elucidate the role of Treg cells in S. japonicum infection–mediated alleviation of AAI, we first analyzed the relationship between Treg cells and OVA-specific IgE and found that the frequency of Treg cells in lung negatively correlated with OVA-specific IgE. The suppression of IgE secretion by Treg cells has been observed and described by multiple previous studies (Meiler et al., 2008; Wing et al., 2008; Khan, 2020). IL-10 and CTLA-4 pathways were suggested to be associated with the suppression of IgE; however, the detailed molecular mechanism is still elusive. Furthermore, by in vivo depletion of Treg cell, we found that the decrease in IgE secretion was Treg cell–dependent. IgE acts as the major mediator contributing to AAI (Gabet et al., 2019). Our results demonstrated that the protective effect of S. japonicum infection on AAI was mediated by Treg cell–dependent inhibition of IgE, which was consistent with a previous report showing that the preventive effect of chronic S. mansoni infection against later AAI was also Treg cells dependent (Layland et al., 2013).
Mechanisms underlying the induction of Treg or Breg cells by helminth-related antigens have been reported (Zaccone et al., 2009; Haeberlein et al., 2017). However, we did not find out the exact active molecules of schistosome that led to the upregulation of Treg cells in this study. Nonetheless, we think that it is very likely that the observed protective effect was a collective result of multiple components of the schistosome, as previous studies showed that multiple enzymes released by schistosomula could regulate host immunity (Hansell et al., 2008; Liu et al., 2015). We plan to acutely define these components in the future.
Instead of identifying effector antigens, in this study, we tried to understand how the lung-stage S. japonicum infection influences local immune responses in lung. To do so, we performed a transcriptomic comparison between lung tissues of schistosome-infected and non-infected mice. The results showed that, after lung-stage S. japonicum infection, most genes related to immune response were downregulated (70/84), which implied that the general immune state in lung tended to be downregulated by S. japonicum infection. Among these genes, we found that three genes (CD46, Epor, and Klra17) reported to promote Treg cell response were upregulated, and eight genes (Clec7a, CCR6, Spi-B, ABCG1, ADA, Ctsk, Ctss, and Ptgir) reported to inhibit Treg cell response were downregulated in schistosome-infected mice, suggesting that S. japonicum infection generated a milieu facilitating Treg cell induction in the lung. In the meantime, we also observed that some molecules reported to facilitate the function of B cells or plasma cells were downregulated, which was consistent with our finding that IgE response was suppressed.
Collectively, our study showed that lung-stage S. japonicum infection established a regulatory environment in the lungs, which can help to relieve OVA-induced AAI in a mouse model. Although the exact mechanism of Treg cell upregulation remains elusive, our data showed that lung-stage S. japonicum infection can improve the population of allergen-specific Treg cells that suppress IgE production. These results highlight the value of lung-stage S. japonicum infection as a potential therapy for allergic asthma.
Data Availability Statement
RNA sequencing data are deposited in the SRA database SRA, SRA accession number: PRJNA609083.
Ethics Statement
The animal study was reviewed and approved by Laboratory Animal Welfare & Ethic Committee (LAWEC) of National Institute of Parasitic Diseases (Permit Number: IPD-2016-7).
Author Contributions
ZL, WZ, WY, BZ, YX, XW, YW, QW, CS, and JL conducted the experiment. ZL, YW, and WH designed the experiment and analyzed the data. FL analyzed the data of RNA-sequence. ZW, FQ, JC, and BX provided intellectual input and aided the experimental design. ZL wrote the manuscript. YW and WH revised the manuscript. All the authors contributed to the article and approved the submitted version.
Funding
This work was funded by the National Key Research and Development Project (2018YFA0507300) and the National Natural Science Foundation of China (31725025, 31572513, and 81271867).
Conflict of Interest
The authors declare that the research was conducted in the absence of any commercial or financial relationships that could be construed as a potential conflict of interest.
Supplementary Material
The Supplementary Material for this article can be found online at: https://www.frontiersin.org/articles/10.3389/fcell.2021.678377/full#supplementary-material
Supplementary Figure 1 | Comparisons of concentrations of IL-13, IL-10, IL-17A, and IFN-γ in BALF. FI indicated fluorescence intensity. Data are shown as mean ± SEM. n = 5 or 6 mice per group per experiment, experiment performed twice. *P < 0.05, **P < 0.01, ***P < 0.001 by one-way analysis of variance (ANOVA) with Tukey test.
Supplementary Figure 2 | The influence of lung-stage S. japonicum infection on OVA-specific IFN-γ and IL-4 response after OVA challenge. (A) Gating strategy of flow cytometry. (B) Frequencies of OVA-specific CD3+CD4+IL-4+ T cells, CD3+CD4+IFN-γ+ T cells, and their ratios in lung and LDLN. Data are shown as mean ± SEM, n = 8 mice per group per experiment, experiment performed twice. *P < 0.05, **P < 0.01 and NS, not significant by one-way analysis of variance (ANOVA) with Tukey test.
Supplementary Figure 3 | The efficiency of Treg cell deletion. The representative flow cytometry of CD25+Foxp3+ Treg cells in peripheral blood treated with anti-CD25 neutralized antibody.
Supplementary Figure 4 | Panther pathway analysis of DEGs between lung-stage S. japonicum–infected mice and no-treatment control mice after OVA challenge.
Footnotes
- ^ http://www.bioinformatics.babraham.ac.uk/projects/fastqc/
- ^ http://www.usadellab.org/cms/index.php?page=trimmomatic
- ^ http://pantherdb.org/
References
Al-Ahmad, M., Arifhodzic, N., Nurkic, J., Maher, A., Rodriguez-Bouza, T., Al-Ahmed, N., et al. (2018). Real-life” efficacy and safety aspects of 4-year omalizumab treatment for asthma. Med. Princ. Pract. 27, 260–266. doi: 10.1159/000487482
Ali, F. R. (2011). Does this patient have atopic asthma? Clin. Med. 11, 376–380. doi: 10.7861/clinmedicine.11-4-376
Anders, S., and Huber, W. (2010). Differential expression analysis for sequence count data. Genome Biol. 11:R106.
Anders, S., Pyl, P. T., and Huber, W. (2015). HTSeq–a python framework to work with high-throughput sequencing data. Bioinformatics 31, 166–169.
Bach, J. F. (2018). The hygiene hypothesis in autoimmunity: the role of pathogens and commensals. Nat. Rev. Immunol. 18, 105–120. doi: 10.1038/nri.2017.111
Barnes, P. J. (2004). The size of the problem of managing asthma. Respir. Med. 98, (Suppl. B), S4–S8.
Baru, A. M., Hartl, A., Lahl, K., Krishnaswamy, J. K., Fehrenbach, H., Yildirim, A. O., et al. (2010). Selective depletion of Foxp3+ Treg during sensitization phase aggravates experimental allergic airway inflammation. Eur. J. Immunol. 40, 2259–2266. doi: 10.1002/eji.200939972
Capron, M. (2011). Effect of parasite infection on allergic disease. Allergy 66, (Suppl. 95), 16–18. doi: 10.1111/j.1398-9995.2011.02624.x
Chang, E. E., and Yen, C. M. (2004). Eosinophil chemoattracted by eotaxin from cerebrospinal fluid of mice infected with Angiostrongylus cantonensis assayed in a microchamber. Kaohsiung J. Med. Sci. 20, 209–215. doi: 10.1016/s1607-551x(09)70108-1
Cheng, H. Y., Gaddis, D. E., Wu, R., McSkimming, C., Haynes, L. D., Taylor, A. M., et al. (2016). Loss of ABCG1 influences regulatory T cell differentiation and atherosclerosis. J. Clin. Invest. 126, 3236–3246. doi: 10.1172/jci83136
Cooper, P. J. (2009). Interactions between helminth parasites and allergy. Curr. Opin. Allergy Clin. Immunol. 9, 29–37. doi: 10.1097/aci.0b013e32831f44a6
Croker, B. A., Tarlinton, D. M., Cluse, L. A., Tuxen, A. J., Light, A., Yang, F. C., et al. (2002). The Rac2 guanosine triphosphatase regulates B lymphocyte antigen receptor responses and chemotaxis and is required for establishment of B-1a and marginal zone B lymphocytes. J. Immunol. 168, 3376–3386. doi: 10.4049/jimmunol.168.7.3376
de Oliveira, F. L., Dos Santos, S. N., Ricon, L., da Costa, T. P., Pereira, J. X., Brand, C., et al. (2018). Lack of galectin-3 modifies differentially Notch ligands in bone marrow and spleen stromal cells interfering with B cell differentiation. Sci. Rep. 8:3495.
de Ruiter, K., Tahapary, D. L., Sartono, E., Soewondo, P., Supali, T., Smit, J. W. A., et al. (2017). Helminths, hygiene hypothesis and type 2 diabetes. Parasite Immunol. 39:e12404. doi: 10.1111/pim.12404
Eder, W., Ege, M. J., and von Mutius, E. (2006). The asthma epidemic. N. Engl. J. Med. 355, 2226–2235.
Falk, N. (2018). Allergy and asthma: asthma management. FP Essent. 472, 25–29. doi: 10.1089/pai.1997.11.25
Flach, H., Rosenbaum, M., Duchniewicz, M., Kim, S., Zhang, S. L., Cahalan, M. D., et al. (2010). Mzb1 protein regulates calcium homeostasis, antibody secretion, and integrin activation in innate-like B cells. Immunity 33, 723–735. doi: 10.1016/j.immuni.2010.11.013
Gabet, S., Ranciere, F., Just, J., de Blic, J., Lezmi, G., Amat, F., et al. (2019). Asthma and allergic rhinitis risk depends on house dust mite specific IgE levels in PARIS birth cohort children. World Allergy Organ. J. 12:100057. doi: 10.1016/j.waojou.2019.100057
Galli, S. J., and Tsai, M. (2012). IgE and mast cells in allergic disease. Nat. Med. 18, 693–704. doi: 10.1038/nm.2755
Gehrie, E., Van der Touw, W., Bromberg, J. S., and Ochando, J. C. (2011). Plasmacytoid dendritic cells in tolerance. Methods Mol. Biol. 677, 127–147.
Gu, Y., Jensen, P. E., and Chen, X. (2013). Immunodeficiency and autoimmunity in H2-O-deficient mice. J. Immunol. 190, 126–137. doi: 10.4049/jimmunol.1200993
Haeberlein, S., Obieglo, K., Ozir-Fazalalikhan, A., Chaye, M. A. M., Veninga, H., van der Vlugt, L., et al. (2017). Schistosome egg antigens, including the glycoprotein IPSE/alpha-1, trigger the development of regulatory B cells. PLoS Pathog. 13:e1006539. doi: 10.1371/journal.ppat.1006539
Hansell, E., Braschi, S., Medzihradszky, K. F., Sajid, M., Debnath, M., Ingram, J., et al. (2008). Proteomic analysis of skin invasion by blood fluke larvae. PLoS Negl. Trop. Dis. 2:e262. doi: 10.1371/journal.pntd.0000262
Harnett, M. M., and Harnett, W. (2017). Can parasitic worms cure the modern world’s ills? Trends Parasitol. 33, 694–705. doi: 10.1016/j.pt.2017.05.007
Henderson, J. G., and Hawiger, D. (2015). Regulation of extrathymic Treg cell conversion by CD5. Oncotarget 6, 26554–26555. doi: 10.18632/oncotarget.5809
Herbert, O., Barnetson, R. S., Weninger, W., Kramer, U., Behrendt, H., and Ring, J. (2009). Western lifestyle and increased prevalence of atopic diseases: an example from a small papua new guinean island. World Allergy Organ. J. 2, 130–137. doi: 10.1097/wox.0b013e3181accf27
Hopfenspirger, M. T., and Agrawal, D. K. (2002). Airway hyperresponsiveness, late allergic response, and eosinophilia are reversed with mycobacterial antigens in ovalbumin-presensitized mice.. Immunol. 168, 2516–2522. doi: 10.4049/jimmunol.168.5.2516
Janssen, L., Silva Santos, G. L., Muller, H. S., Vieira, A. R., de Campos, T. A., and de Paulo Martins, V. (2016). Schistosome-derived molecules as modulating actors of the immune system and promising candidates to treat autoimmune and inflammatory diseases. J. Immunol. Res. 2016:5267485.
Jing, Y., Kang, D., Liu, L., Huang, H., Chen, A., Yang, L., et al. (2019). Dedicator of cytokinesis protein 2 couples with lymphoid enhancer-binding factor 1 to regulate expression of CD21 and B-cell differentiation. J. Allergy Clin. Immunol. 144, 1377.e4–1390.e4.
Keir, M. E., Butte, M. J., Freeman, G. J., and Sharpe, A. H. P. D. - (2008). 1 and its ligands in tolerance and immunity. Annu. Rev. Immunol. 26, 677–704. doi: 10.1146/annurev.immunol.26.021607.090331
Khan, M. A. (2020). Regulatory T cells mediated immunomodulation during asthma: a therapeutic standpoint. J. Transl. Med. 18:456.
Khurana, S., and George, S. P. (2008). Regulation of cell structure and function by actin-binding proteins: villin’s perspective. FEBS Lett. 582, 2128–2139. doi: 10.1016/j.febslet.2008.02.040
Kudo-Saito, C., Shirako, H., Ohike, M., Tsukamoto, N., and Kawakami, Y. (2013). CCL2 is critical for immunosuppression to promote cancer metastasis. Clin. Exp. Metast. 30, 393–405. doi: 10.1007/s10585-012-9545-6
Kulkarni, N., Meitei, H. T., Sonar, S. A., Sharma, P. K., Mujeeb, V. R., Srivastava, S., et al. (2018). CCR6 signaling inhibits suppressor function of induced-Treg during gut inflammation. J. Autoimmun. 88, 121–130. doi: 10.1016/j.jaut.2017.10.013
Kuprys-Lipinska, I., and Kuna, P. (2014). [Changes in the newest recommendations on asthma management and prevention - GINA report 2014. What should we pay attention to?]. Pneumonol. Alergol. Pol. 82, 393–401.
Layland, L. E., Straubinger, K., Ritter, M., Loffredo-Verde, E., Garn, H., Sparwasser, T., et al. (2013). Schistosoma mansoni-mediated suppression of allergic airway inflammation requires patency and Foxp3+ Treg cells. PLoS Negl. Trop. Dis. 7:e2379. doi: 10.1371/journal.pntd.0002379
Li, R., Cheng, C., Chong, S. Z., Lim, A. R., Goh, Y. F., Locht, C., et al. (2012). Attenuated Bordetella pertussis BPZE1 protects against allergic airway inflammation and contact dermatitis in mouse models. Allergy 67, 1250–1258. doi: 10.1111/j.1398-9995.2012.02884.x
Liu, M., Ju, C., Du, X. F., Shen, H. M., Wang, J. P., Li, J., et al. (2015). Proteomic analysis on cercariae and schistosomula in reference to potential proteases involved in host invasion of Schistosoma japonicum Larvae. J. Proteome Res. 14, 4623–4634. doi: 10.1021/acs.jproteome.5b00465
Liu, W., Li, H., Zhang, X., Wen, D., Yu, F., Yang, S., et al. (2013). Prostaglandin I2-IP signalling regulates human Th17 and Treg cell differentiation. Prostaglandins Leukot. Essent. Fatty Acids 89, 335–344. doi: 10.1016/j.plefa.2013.08.006
Low, M. S. Y., Brodie, E. J., Fedele, P. L., Liao, Y., Grigoriadis, G., Strasser, A., et al. (2019). IRF4 activity is required in established plasma cells to regulate gene transcription and mitochondrial homeostasis. Cell Rep. 29, 2634.e5–2645.e5.
Maizels, R. M. (2016). Parasitic helminth infections and the control of human allergic and autoimmune disorders. Clin. Microbiol. Infect. 22, 481–486. doi: 10.1016/j.cmi.2016.04.024
Maizels, R. M., and McSorley, H. J. (2016). Regulation of the host immune system by helminth parasites. J. Allergy Clin. Immunol. 138, 666–675. doi: 10.1016/j.jaci.2016.07.007
Maizels, R. M., McSorley, H. J., and Smyth, D. J. (2014). Helminths in the hygiene hypothesis: sooner or later? Clin. Exp. Immunol. 177, 38–46. doi: 10.1111/cei.12353
Mangan, N. E., van Rooijen, N., McKenzie, A. N., and Fallon, P. G. (2006). Helminth-modified pulmonary immune response protects mice from allergen-induced airway hyperresponsiveness. J. Immunol. 176, 138–147. doi: 10.4049/jimmunol.176.1.138
Massoud, A. H., Yona, M., Xue, D., Chouiali, F., Alturaihi, H., Ablona, A., et al. (2014). Dendritic cell immunoreceptor: a novel receptor for intravenous immunoglobulin mediates induction of regulatory T cells. J. Allergy Clin. Immunol. 133, 853.e5–863.e5.
McManus, D. P., Dunne, D. W., Sacko, M., Utzinger, J., Vennervald, B. J., and Zhou, X. N. (2018). Schistosomiasis. Nat. Rev. Dis. Primers 4:13.
Medeiros, M. Jr., Figueiredo, J. P., Almeida, M. C., Matos, M. A., Araujo, M. I., Cruz, A. A., et al. (2003). Schistosoma mansoni infection is associated with a reduced course of asthma. J. Allergy Clin. Immunol. 111, 947–951. doi: 10.1067/mai.2003.1381
Mehalow, A. K., Kameya, S., Smith, R. S., Hawes, N. L., Denegre, J. M., Young, J. A., et al. (2003). CRB1 is essential for external limiting membrane integrity and photoreceptor morphogenesis in the mammalian retina. Hum. Mol. Genet. 12, 2179–2189. doi: 10.1093/hmg/ddg232
Meiler, F., Klunker, S., Zimmermann, M., Akdis, C. A., and Akdis, M. (2008). Distinct regulation of IgE, IgG4 and IgA by T regulatory cells and toll-like receptors. Allergy 63, 1455–1463. doi: 10.1111/j.1398-9995.2008.01774.x
Naval-Macabuhay, I., Casanova, V., Navarro, G., Garcia, F., Leon, A., Miralles, L., et al. (2016). Adenosine deaminase regulates Treg expression in autologous T cell-dendritic cell cocultures from patients infected with HIV-1. J. Leukoc. Biol. 99, 349–359. doi: 10.1189/jlb.3a1214-580rr
Nuttall, R. K., Sampieri, C. L., Pennington, C. J., Gill, S. E., Schultz, G. A., and Edwards, D. R. (2004). Expression analysis of the entire MMP and TIMP gene families during mouse tissue development. FEBS Lett. 563, 129–134. doi: 10.1016/s0014-5793(04)00281-9
Obieglo, K., Schuijs, M. J., Ozir-Fazalalikhan, A., Otto, F., van Wijck, Y., Boon, L., et al. (2018). Isolated Schistosoma mansoni eggs prevent allergic airway inflammation. Parasite Immunol. 40:e12579. doi: 10.1111/pim.12579
Okada, H., Kuhn, C., Feillet, H., and Bach, J. F. (2010). The ‘hygiene hypothesis’ for autoimmune and allergic diseases: an update. Clin. Exp. Immunol. 160, 1–9. doi: 10.1111/j.1365-2249.2010.04139.x
Osada, Y., Shimizu, S., Kumagai, T., Yamada, S., and Kanazawa, T. (2009). Schistosoma mansoni infection reduces severity of collagen-induced arthritis via down-regulation of pro-inflammatory mediators. Int. J. Parasitol. 39, 457–464. doi: 10.1016/j.ijpara.2008.08.007
Pacifico, L. G., Marinho, F. A., Fonseca, C. T., Barsante, M. M., Pinho, V., Sales-Junior, P. A., et al. (2009). Schistosoma mansoni antigens modulate experimental allergic asthma in a murine model: a major role for CD4+ CD25+ Foxp3+ T cells independent of interleukin-10. Infect. Immun. 77, 98–107. doi: 10.1128/iai.00783-07
Peng, C., and Eckhardt, L. A. (2013). Role of the Igh intronic enhancer Emu in clonal selection at the pre-B to immature B cell transition. J. Immunol. 191, 4399–4411. doi: 10.4049/jimmunol.1301858
Persson, C. (2019). In vivo observations provide insight into roles of eosinophils and epithelial cells in asthma. Eur. Respir. J. 54:1900470.
Purroy, C., Fairchild, R. L., Tanaka, T., Baldwin, W. M. III, Manrique, J., Madsen, J. C., et al. (2017). Erythropoietin receptor-mediated molecular crosstalk promotes T cell immunoregulation and transplant survival. J. Am. Soc. Nephrol. 28, 2377–2392. doi: 10.1681/asn.2016101100
Qiu, S., Fan, X., Yang, Y., Dong, P., Zhou, W., Xu, Y., et al. (2017). Schistosoma japonicum infection downregulates house dust mite-induced allergic airway inflammation in mice. PLoS One 12:e0179565. doi: 10.1371/journal.pone.0179565
Rauch, K. S., Hils, M., Lupar, E., Minguet, S., Sigvardsson, M., Rottenberg, M. E., et al. (2016). Id3 maintains Foxp3 expression in regulatory T Cells by controlling a transcriptional network of E47. Spi-B, and SOCS3. Cell Rep. 17, 2827–2836. doi: 10.1016/j.celrep.2016.11.045
Rheinberg, C. E., Mone, H., Caffrey, C. R., Imbert-Establet, D., Jourdane, J., and Ruppel, A. (1998). Schistosoma haematobium, S. intercalatum, S. japonicum, S. mansoni, and S. rodhaini in mice: relationship between patterns of lung migration by schistosomula and perfusion recovery of adult worms. Parasitol. Res. 84, 338–342. doi: 10.1007/s004360050407
Rock, J. R., Futtner, C. R., and Harfe, B. D. (2008). The transmembrane protein TMEM16A is required for normal development of the murine trachea. Dev. Biol. 321, 141–149. doi: 10.1016/j.ydbio.2008.06.009
Sato, T., Okumura, F., Ariga, T., and Hatakeyama, S. (2012). TRIM6 interacts with Myc and maintains the pluripotency of mouse embryonic stem cells. J. Cell Sci. 125(Pt 6), 1544–1555.
Scheikl, T., Reis, B., Pfeffer, K., Holzmann, B., and Beer, S. (2009). Reduced notch activity is associated with an impaired marginal zone B cell development and function in Sly1 mutant mice. Mol. Immunol. 46, 969–977. doi: 10.1016/j.molimm.2008.09.023
Setiady, Y. Y., Coccia, J. A., and Park, P. U. (2010). In vivo depletion of CD4+FOXP3+ Treg cells by the PC61 anti-CD25 monoclonal antibody is mediated by FcgammaRIII+ phagocytes. Eur. J. Immunol. 40, 780–786. doi: 10.1002/eji.200939613
Sitcharungsi, R., and Sirivichayakul, C. (2013). Allergic diseases and helminth infections. Pathog. Glob. Health 107, 110–115. doi: 10.1179/2047773213y.0000000080
Smits, H. H., Hammad, H., van Nimwegen, M., Soullie, T., Willart, M. A., Lievers, E., et al. (2007). Protective effect of Schistosoma mansoni infection on allergic airway inflammation depends on the intensity and chronicity of infection. J. Allergy Clin. Immunol. 120, 932–940. doi: 10.1016/j.jaci.2007.06.009
Stiemsma, L. T., and Turvey, S. E. (2017). Asthma and the microbiome: defining the critical window in early life. Allergy Asthma Clin. Immunol. 13:3.
Tang, C., Kamiya, T., Liu, Y., Kadoki, M., Kakuta, S., Oshima, K., et al. (2015). Inhibition of Dectin-1 signaling ameliorates colitis by inducing lactobacillus-mediated regulatory T cell expansion in the intestine. Cell Host Microbe 18, 183–197. doi: 10.1016/j.chom.2015.07.003
Thomsen, S. F. (2015). Epidemiology and natural history of atopic diseases. Eur. Clin. Respir. J. 2. doi: 10.3402/ecrj.v2.24642
Tsai, Y. G., Niu, D. M., Yang, K. D., Hung, C. H., Yeh, Y. J., Lee, C. Y., et al. (2012). Functional defects of CD46-induced regulatory T cells to suppress airway inflammation in mite allergic asthma. Lab. Invest. 92, 1260–1269. doi: 10.1038/labinvest.2012.86
Umetsu, D. T. (2012). Early exposure to germs and the hygiene hypothesis. Cell Res. 22, 1210–1211. doi: 10.1038/cr.2012.65
van der Vlugt, L. E., Labuda, L. A., Ozir-Fazalalikhan, A., Lievers, E., Gloudemans, A. K., Liu, K. Y., et al. (2012). Schistosomes induce regulatory features in human and mouse CD1d(hi) B cells: inhibition of allergic inflammation by IL-10 and regulatory T cells. PLoS One 7:e30883. doi: 10.1371/journal.pone.0030883
Watanabe, T., Masuyama, J., Sohma, Y., Inazawa, H., Horie, K., Kojima, K., et al. (2006). CD52 is a novel costimulatory molecule for induction of CD4+ regulatory T cells. Clin. Immunol. 120, 247–259. doi: 10.1016/j.clim.2006.05.006
Wing, K., Onishi, Y., Prieto-Martin, P., Yamaguchi, T., Miyara, M., Fehervari, Z., et al. (2008). 4 control over Foxp3+ regulatory T cell function. Science 322, 271–275. doi: 10.1126/science.1160062
Yan, X., Wu, C., Chen, T., Santos, M. M., Liu, C. L., Yang, C., et al. (2017). Cathepsin S inhibition changes regulatory T-cell activity in regulating bladder cancer and immune cell proliferation and apoptosis. Mol. Immunol. 82, 66–74. doi: 10.1016/j.molimm.2016.12.018
Yang, J. Q., Zhou, Y., and Singh, R. R. (2016). Effects of invariant NKT cells on parasite infections and hygiene hypothesis. J. Immunol. Res. 2016:2395645.
Yu, A., Zhu, L., Altman, N. H., and Malek, T. R. A. (2009). low interleukin-2 receptor signaling threshold supports the development and homeostasis of T regulatory cells. Immunity 30, 204–217. doi: 10.1016/j.immuni.2008.11.014
Yu, G., Wang, L. G., Han, Y., and He, Q. Y. (2012). clusterProfiler: an R package for comparing biological themes among gene clusters. OMICS 16, 284–287. doi: 10.1089/omi.2011.0118
Zaccone, P., Burton, O., Miller, N., Jones, F. M., Dunne, D. W., and Cooke, A. (2009). Schistosoma mansoni egg antigens induce Treg that participate in diabetes prevention in NOD mice. Eur. J. Immunol. 39, 1098–1107. doi: 10.1002/eji.200838871
Zhang, W., Li, L., Zheng, Y., Xue, F., Yu, M., Ma, Y., et al. (2019). Schistosoma japonicum peptide SJMHE1 suppresses airway inflammation of allergic asthma in mice. J. Cell Mol. Med. 23, 7819–7829. doi: 10.1111/jcmm.14661
Keywords: allergic airway inflammation, asthma, helminth therapy, Treg, Schistosoma infection, parasite–host interaction
Citation: Li Z, Zhang W, Luo F, Li J, Yang W, Zhu B, Wu Q, Wang X, Sun C, Xie Y, Xu B, Wang Z, Qian F, Chen J, Wan Y and Hu W (2021) Allergen-Specific Treg Cells Upregulated by Lung-Stage S. japonicum Infection Alleviates Allergic Airway Inflammation. Front. Cell Dev. Biol. 9:678377. doi: 10.3389/fcell.2021.678377
Received: 09 March 2021; Accepted: 22 April 2021;
Published: 08 June 2021.
Edited by:
Qingfeng Zhang, Tongji University, ChinaReviewed by:
Chuan Su, Nanjing Medical University, ChinaXin Ping Zhu, Capital Medical University, China
Copyright © 2021 Li, Zhang, Luo, Li, Yang, Zhu, Wu, Wang, Sun, Xie, Xu, Wang, Qian, Chen, Wan and Hu. This is an open-access article distributed under the terms of the Creative Commons Attribution License (CC BY). The use, distribution or reproduction in other forums is permitted, provided the original author(s) and the copyright owner(s) are credited and that the original publication in this journal is cited, in accordance with accepted academic practice. No use, distribution or reproduction is permitted which does not comply with these terms.
*Correspondence: Wei Hu, huw@fudan.edu.cn; Yanmin Wan, yanmin_wan@fudan.edu.cn