- 1Biological Resources Laboratory, University of Illinois at Chicago, Chicago, IL, United States
- 2Department of Anatomy and Cell Biology, College of Medicine, University of Illinois at Chicago, Chicago, IL, United States
Seizures are emerging as a common symptom in Alzheimer’s disease (AD) patients, often attributed to high levels of amyloid β (Aβ). However, the extent that AD disease risk factors modulate seizure activity in aging and AD-relevant contexts is unclear. APOE4 is the greatest genetic risk factor for AD and has been linked to seizures independent of AD and Aβ. The goal of the present study was to evaluate the role of APOE genotype in modulating seizures in the absence and presence of high Aβ levels in vivo. To achieve this goal, we utilized EFAD mice, which express human APOE3 or APOE4 in the absence (EFAD−) or presence (EFAD+) of familial AD mutations that result in Aβ overproduction. When quantified during cage change day, we found that unlike APOE3, APOE4 is associated with tonic-clonic seizures. Interestingly, there were lower tonic-clonic seizures in E4FAD+ mice compared to E4FAD− mice. Restraint handing and auditory stimuli failed to recapitulate the tonic-clonic phenotype in EFAD mice that express APOE4. However, after chemical-induction with pentylenetetrazole, there was a higher incidence of tonic-clonic seizures with APOE4 compared to APOE3. Interestingly, the distribution of seizures to the tonic-clonic phenotype was higher with FAD mutations. These data support that APOE4 is associated with higher tonic-clonic seizures in vivo, and that FAD mutations impact tonic-clonic seizures in a paradigm dependent manner.
Introduction
Alzheimer disease (AD) is the most common form of dementia and is defined by cognitive decline, extracellular plaques containing amyloid-β (Aβ) and intraneuronal tangles of tau. In addition, accumulating evidence suggest that seizures are a common and important component of the AD phenotype (reviewed in Palop and Mucke, 2009; Friedman et al., 2012; Pandis and Scarmeas, 2012; Lam and Noebels, 2020). For example, the risk of clinical unprovoked seizures of unknown etiology is 6–10-fold higher in AD and between 1 and 60% of AD patients experience unprovoked seizures (Hauser et al., 1986; Risse et al., 1990; Romanelli et al., 1990; McAreavey et al., 1992; Mendez et al., 1994; Volicer et al., 1995; Hesdorffer et al., 1996; Amatniek et al., 2006; Lozsadi and Larner, 2006; Rao et al., 2009; Scarmeas et al., 2009; Bernardi et al., 2010; Irizarry et al., 2012; Imfeld et al., 2013; Vossel et al., 2013; Cheng et al., 2015; Sarkis et al., 2016). However, the incidence of seizures in AD is likely to be underestimated because AD patients also experience non-convulsive seizures that are often unrecognized due to their symptomatic overlap with other behavioral changes such as memory loss, hallucinations, anxiety, and confusion. In support of this concept, subclinical epileptiform activity has been observed in up to 40% of AD patients when assessed by electroencephalogram recordings (Vossel et al., 2013, 2016; Brunetti et al., 2020; Lam et al., 2020). Recent data also suggest that seizures occur in early, preclinical stages of dementia and accelerate disease progression (Cretin et al., 2016; DiFrancesco et al., 2017; Costa et al., 2019; Keret et al., 2020). Therefore, evaluating the role of AD risk factors in modulating seizure activity is a crucial step to establish whether there is link with disease progression.
APOE genotype is the greatest genetic risk factor for sporadic AD, with APOE4 increasing risk up to 12-fold compared to APOE3 (reviewed in Mahley et al., 2007; Liu et al., 2013; Flowers and Rebeck, 2020). The role of APOE in AD is extremely complex and includes modulation of functions both independent and dependent of AD pathology (particularly Aβ). For example, APOE4 is associated with learning and memory dysfunction during aging, independent of AD (reviewed in Tai et al., 2016), which is recapitulated in APOE targeted replacement mice (Grootendorst et al., 2005; Villasana et al., 2006; Bour et al., 2008; Rodriguez et al., 2013; Tai et al., 2016; Thomas et al., 2017; Zaldua et al., 2020). Critically, there is an association between APOE genotype and seizures (Hirsch, 2007). With APOE4 there is higher epilepsy risk (Liang et al., 2019), particularly post trauma (Diaz-Arrastia et al., 2003; Harden, 2004), an earlier age of onset for intractable seizures (Briellmann et al., 2000; Gambardella et al., 2005; Kauffman et al., 2010) and greater memory dysfunction in patients with chronic temporal lobe epilepsy (Gouras et al., 1997; Gambardella et al., 2005; Busch et al., 2007). Unfortunately, in vivo research on this topic is limited, with only one report of higher seizures with APOE4 in APOE-targeted replacement mice (Hunter et al., 2012). This study highlights the ability of APOE to modulate brain function independent of AD pathology, but there is a strong link between APOE4 and Aβ. In humans and mice that overproduce Aβ, with APOE4 levels of all different types of Aβ (soluble, soluble oligomeric, intraneuronal, extracellular) are higher compared to APOE3. Aβ itself is also linked to higher seizures as evidenced by the higher incidence of seizures found in familial AD (FAD) patients (reviewed in Palop and Mucke, 2009). FAD accounts for 5% of all cases and is caused by mutations in proteins (the amyloid precursor protein, or presenilins) that result in higher Aβ production; 40–80% of FAD patients experience seizures (Palop and Mucke, 2009). Mouse models of FAD mutations also develop epileptic spiking consistent with partial seizures (Palop et al., 2007; Minkeviciene et al., 2009; Siwek et al., 2015; Gureviciene et al., 2019), have a lower seizure threshold to pentylenetetrazole (Del Vecchio et al., 2004) and have higher audiogenic seizures (Westmark et al., 2010; Kazim et al., 2017). However, it is currently unknown whether APOE genotypes modulate seizures in the presence of human Aβ (i.e., APOE/FAD mice), and if the combination of APOE4 and Aβ results in higher seizures compared to APOE4 alone. Addressing these questions could provide novel insight on the contribution of APOE genotype to seizures in AD-relevant contexts, and therefore provide the framework for future research focused on identifying the underlying mechanisms.
The goal of the present study was to evaluate the role of APOE genotype in modulating seizure incidence in the absence and presence of high Aβ levels in vivo. To achieve this goal, we utilized EFAD mice that express APOE3 or APOE4 in the absence (EFAD−) or presence (EFAD+) of Aβ overproduction. We recorded total seizure incidence during cage changes, evaluated whether handling restraint or auditory cues precipitated seizures and assessed seizure threshold to pentylenetetrazole.
Methods
Animals
All experiments procedures were approved by the Institutional Animal Care and Use Committee at the University of Illinois at Chicago. We used EFAD mice (Youmans et al., 2012), which were produced by crossing mice that express 5 Familial Alzheimer’s disease mutations (5xFAD; APP K670N/M671L + I716V + V717I and PS1 M146L + L286V, C57BL6/B6xSJL) with APOE-targeted replacement mice (APOE-TR, C57BL6). EFAD non-carrier mice are APOE+/+ 5xFAD–/– (E4FAD−) and carriers are APOE+/+/5xFAD± (EFAD+). All EFAD mice were at least 8 months old at the start of the study. Mice were group-housed in a 12–12-h light-dark cycle (lights off at 11 a.m. and on at 11 p.m.).
Colony Observations of Seizures
Tonic-clonic seizures are relatively easy to identify in the mouse colony as the noise during an episode is unique and loud and were recorded in EFAD mice during routine once weekly cage change by trained members of the animal husbandry staff. Cages and the corresponding mouse (tail) were marked that displayed seizure behavior during cage change at the end of the light cycle. Tonic-clonic seizures were reported if they occurred when the cage was removed from the housing rack, during cage changing in the changing station, or once the cage was placed back on the housing rack.
Acute Handling Restraint Stress-Induced Seizures
The prevalence of acute handling restraint stress-induced seizure behavior (tonic-clonic) was assessed in the same mice as those evaluated for seizures during cage change day. Mice were manually restrained for a maximum of 30 s using the scruffing technique that involves grasping the loose skin located around the dorsal aspect of the mouse’s neck. If a seizure began while in restraint, the mouse was placed into an empty cage to assess the duration of tonic-clonic seizure and recovery. If a seizure did not begin while in restraint, the mouse was placed in a new cage and observed for 1 min for seizure occurrence, prior to returning to the home cage.
Audiogenic-Induced Seizures
A subset of EFAD mice were subjected to an audiogenic seizure protocol adapted from Yagi et al. (2005) to investigate seizure susceptibility triggered by sound. Briefly, mice were allowed to acclimate in the sound-attenuating cabinet (Ugo Basile) for 1 min prior to the presentation of four 11 kHz tone at an intensity of 105 dB for 20 s, with a 2 s interval. All behavioral changes were recorded using a video camera affixed to the chamber and evaluated off-line.
Pentylenetetrazole (PTZ)-Induced Seizures
A single dose (60 mg/kg, s.c.) of pentylenetetrazole (PTZ, Sigma-AldrichTM, St. Louis, United States; purity ≥ 99%) was used to evaluate seizure thresholds in the same cohort of mice exposed to the audiogenic protocol. After injection, mice were placed in acrylic boxes, and behavioral changes induced by PTZ were recorded for 30 min using a video camera above the testing arena and analyzed off-line by investigators blinded to APOE genotype and sex. This dose of PTZ typically induces a range of seizure-like behaviors from freezing and myoclonic twitches to tonic-clonic seizures (Brault et al., 2011; Garcia-Cabrero et al., 2013; Bezzina et al., 2015; Van Erum et al., 2020). Tonic-clonic seizures begin with freezing behavior alternating with myoclonic twitching of the forelimbs that progress to violent jumping and running. Mice with tonic-clonic seizures lasting greater than 3 min were euthanized. We also assigned a score to the types of seizures; 3 = tonic-clonic, 2 = freezing and 1 = no seizure.
Statistics
In Figures 1 and 2 comparisons were made using Chi-squared test (GraphPad Prism version 8) to assess the incidence of seizure behaviors. Fisher’s exact test was utilized when one variable equaled zero. p < 0.05 was considered significant. We employed sequential statistical analysis, by first focusing on our primary research question of whether APOE genotype modulates seizure behaviors (APOE3 vs. APOE4). We then evaluated the impact of sex (male vs. female), and FAD mutations (EFAD + vs. EFAD-) on seizures within each APOE genotype. In Figure 3 seizure score was evaluated via two-way ANOVA.
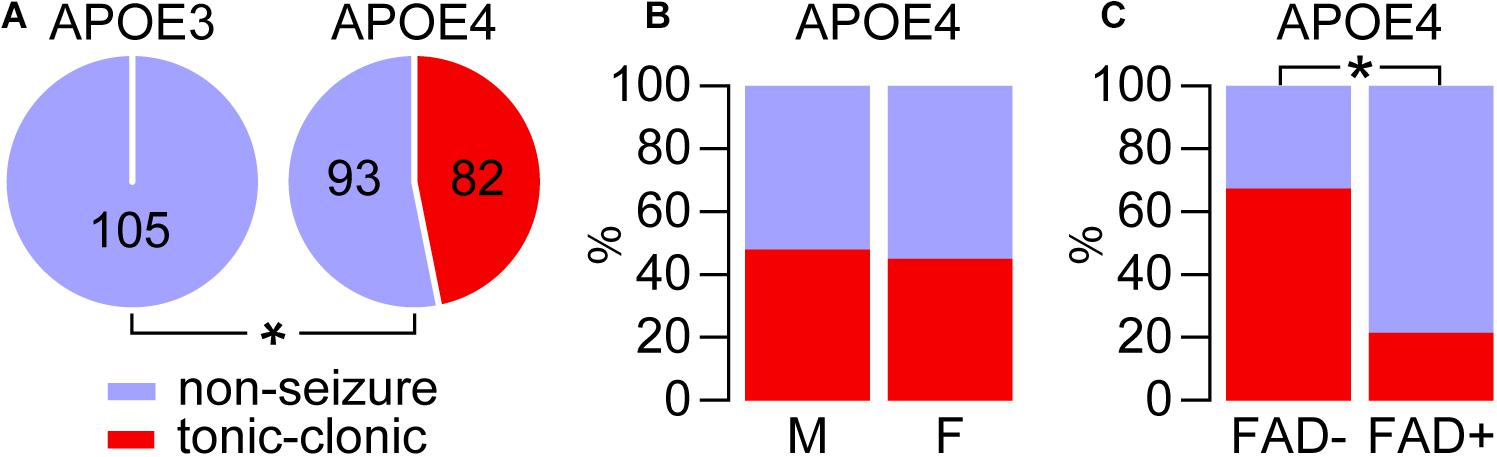
Figure 1. APOE4 but not APOE3 is associated with tonic-clonic seizures during cage change day. The incidence of tonic-clonic seizures was evaluated during cage change day over a 5-week period in mice that express APOE3 or APOE4 in the absence (E3FAD– and E4FAD–) and presence (E3FAD+ and E4FAD+) of five familial AD mutations. (A) Tonic-clonic seizures manifested in mice that express APOE4, but not APOE3 (*p < 0.00001, E4FAD– and E4FAD+ vs. E3FAD– and E3FAD+ mice, Fisher Exact test). (B) There were similar seizure frequencies in male and female APOE4 mice (p = 0.70, male E4FAD– and E4FAD+ vs. female E4FAD– and E4FAD+, Chi-square test). (C) Interestingly, there were higher seizures in the absence of FAD mutations in APOE4 mice (*p < 0.0001, E4FAD– vs. E4FAD+ mice, Chi-square test). n = 23 male E3FAD–, 25 female E3FAD–, 58 male E4FAD–, 34 female E4FAD–, 28 male E3FAD+, 29 female E3FAD+, 46 male E4FAD+, 37 female E4FAD+ mice.
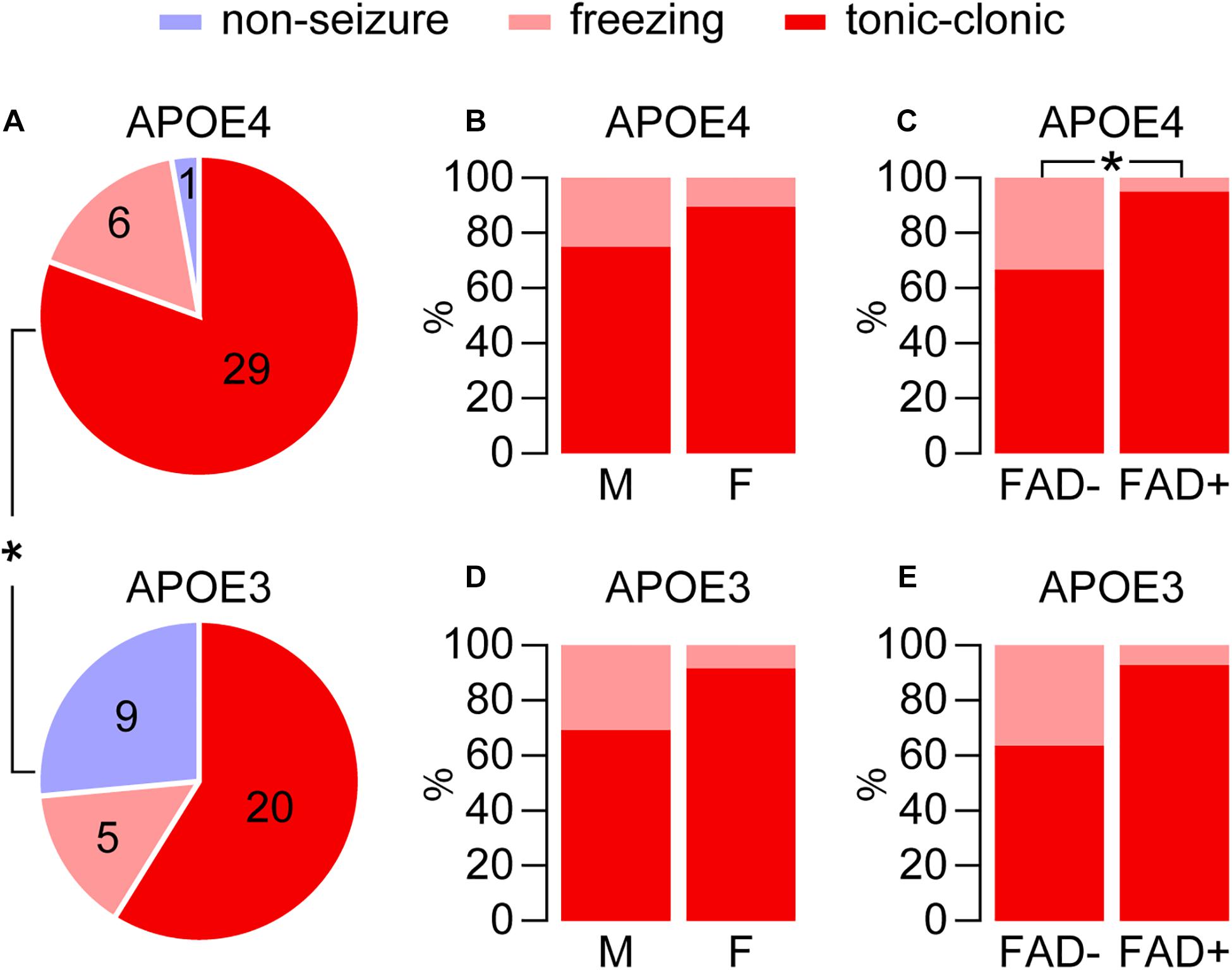
Figure 2. Higher seizures with APOE4 for pentylenetetrazole-induced seizures. Seizure frequency was evaluated in EFAD mice after treatment with pentylenetetrazole (PTZ, 60 mg/kg), which resulted in a range of seizure-like behaviors from freezing and myoclonic twitches to tonic-clonic seizures. (A) Seizure behaviors were modulated by APOE genotype and were higher with APOE4 in EFAD mice (*p < 0.028, E4FAD– and E4FAD+ vs. E3FAD– and E3FAD+ mice, Chi-square test). (B) With APOE4 expression, in male (12/16) and female (17/19) mice there was a similar distribution of seizures (tonic-clonic compared to total, p = 0.26, male E4FAD– and E4FAD + vs. female E4FAD– and E4FAD+, Chi-square test). (C) However, there was higher distribution of tonic-clonic seizures in E4FAD+ (19/20) compared to E4FAD– (10/15) mice (*p = 0.028, E4FAD– vs. E4FAD+ mice, Chi-square test). With APOE3 expression (D) tonic-clonic seizure frequency was also similar for each sex (p = 0.16, 9/13 male E3FAD– and E3FAD+ vs. 11/12 female E3FAD– and E3FAD+, Chi-square test). (E) Although not statistically significant, there was trend of higher tonic-clonic seizure incidence with FAD mutations (p = 0.07, 7/11 E3FAD– vs. 13/14 E3FAD+ mice, Chi-square test). n = 6 male E3FAD–, 11 female E3FAD–, 8 male E4FAD–, 8 female E4FAD–, 9 male E3FAD+, 8 female E3FAD+, 9 male E4FAD+, 11 female E4FAD+.
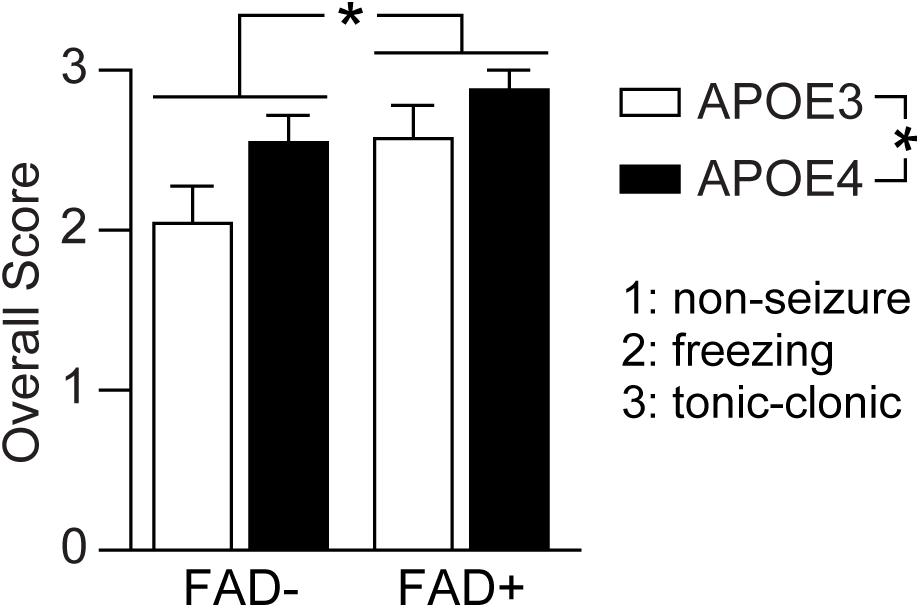
Figure 3. APOE genotype and FAD mutations independently modulate pentylenetetrazole-induced seizures. Seizure score was evaluated in EFAD mice after pentylenetetrazole (PTZ, 60 mg/kg) treatment using a scale of 3 (tonic-clonic), 2 (freezing) and 1 (non-seizure). When assessed by two-way ANOVA, APOE and FAD modulated seizure score, however there was no interaction. Data is expressed as mean ± S.E.M. Data evaluated by two-Way ANOVA. F(1, 65) = APOE genotype: 5.59, *p = 0.021; FAD: 6.32 *p = 0.014; APOE × FAD interaction: 0.331, p = 0.5670. n = 6 male E3FAD–, 11 female E3FAD–, 8 male E4FAD–, 8 female E4FAD–, 9 male E3FAD+, 8 female E3FAD+, 9 male E4FAD+, 11 female E4FAD.
Results
To identify how APOE genotype modulates behavior and brain function in aging and AD-relevant contexts, we utilize EFAD mice, which express human APOE3 or APOE4 in the absence (EFAD-) or presence (EFAD+) of FAD mutations that result in Aβ overproduction. Over the last few years, we started to observe seizures in mice expressing APOE4, especially after spending more time in the mouse colony during the dark/active cycles. Thus, the goal of this study was to systematically determine the effect of APOE on seizure frequency and thresholds in EFAD− and EFAD+ mice. Specifically, we utilized E3FAD−, E3FAD+, E4FAD−, and E4FAD+ mice, both male and female that were at least 8 months of age at the start of the study. The age cut-off was selected as anecdotally we had had not observed seizures in younger mice.
APOE4 but Not APOE3 Is Associated With Tonic-Clonic Seizures During Cage Change Day
The types of seizures we observe in E4FAD− and E4FAD+ mice are tonic-clonic, which typically begins with freezing behavior, progresses to severe myoclonic twitching of the forelimbs with violent movements (uncontrolled jumping, running), followed by recumbency. Tonic-clonic seizures are readily identifiable, and after discussions with the animal husbandry staff, it became apparent that some EFAD mice had been seizing in response to the stimuli produced during routine cage change. Therefore, we sequentially evaluated the effect of APOE genotype, sex and FAD/Aβ on seizure frequency during weekly cage change over a 5-week period. All available mice of at least 8 months of age in the colony were utilized for this study: 23 male E3FAD−, 25 female E3FAD−, 58 male E4FAD−, 34 female E4FAD−, 28 male E3FAD+, 29 female E3FAD+, 46 male E4FAD+, 37 female E4FAD+ mice.
We first assessed whether APOE genotype was associated with higher seizure frequency, though combining data for FAD- and FAD+ mice for each genotype (Figure 1A). There were tonic-clonic seizures in 46.9% of mice that express APOE4 (82 out of 175) compared to 0% that express APOE3 (0 out of 105). We next evaluated whether within the APOE4 genotype group (E4FAD− and E4FAD+), biological sex affected seizure frequency. We found that both male and female APOE4 mice showed similar frequency of seizure occurrence (Figure 1B): 48.1% in males (50/104) vs. 45.1% in females (32/71). Thus, unlike some other readouts found in this model (Aβ levels, neuroinflammation, cerebrovascular function) (reviewed in Tai et al., 2017; Balu et al., 2019), there is no effect of sex on seizures with APOE4 during cage changing. We next asked whether APOE4 alone or APOE4 and FAD mutations/Aβ levels contribute to tonic-clonic seizures (Figure 1C). Our data demonstrate that E4FAD− mice (67.4%, 62/92) are more likely to experience seizure behaviors than E4FAD+ mice (24.1%, 20/93 E4FAD+). This effect was found within each sex, as both male (60.3%, 35/58 E4FAD− vs. 32.6%, 15/46 E4FAD+, p < 0.01, Chi-square) and female (79.4% 27/34 E4FAD− vs. 13.5%, 5/37 E4FAD+, p < 0.0001, Chi-square) E4FAD− mice showed higher incidence of seizures when compared to E4FAD+ mice. Collectively, these data demonstrate that APOE4 is associated with tonic-clonic seizures compared to APOE3, and that FAD mutations/Aβ levels are associated with a lower number of seizures in APOE4 mice.
Acute Handling Restraint and Auditory Stimulus Do Not Trigger Seizures in EFAD Mice
We next explored whether the effect of APOE genotype on tonic-clonic seizures can be elicited by acute stress alone. To this end, we conducted a standardized handling restraint test in all the mice that were evaluated for seizures during cage change day. During restraint handling, only 1.1% of mice that express APOE4 (2 out of 175; 1 male E4FAD− and 1 female E4FAD−) showed seizures, whereas none of the APOE3 mice (0/105) did. These data indicate that acute stress associated with handling is insufficient to induce seizures to the extent that we observed during cage change day with APOE4 in EFAD mice.
An additional stressor that can induce seizures in mice, inducing FAD mice, is auditory stimuli, which we next evaluated in a subset of EFAD mice; 6 male E3FAD−, 11 female E3FAD−, 8 male E4FAD−, 8 female E4FAD−, 9 male E3FAD+, 8 female E3FAD+, 9 male E4FAD+, 11 female E4FAD+. We found that auditory stimulus alone is insufficient to induce seizures in any of the groups of EFAD mice tested. Together, these results suggest that acute stress due to handling and auditory stimuli do not mimic the number of seizures we observed with cage change day in EFAD mice.
Higher Seizures With APOE4 for Pentylenetetrazole (PTZ)-Induced Seizures
As neither E3FAD− or E3FAD+ had seizures during cage change day, our next goal was to characterize the effect of APOE genotype on seizure susceptibility after chemical induction using PTZ (60 mg/kg). For these experiments we used the same cohort of mice subjected to auditory stimulus testing.
We paralleled our analysis of cage change day seizures, through first evaluating the role of APOE genotype in modulating seizure frequency after PTZ injection (i.e., E4FAD− and E4FAD+ vs. E3FAD− and E3FAD+). As found in other studies (Brault et al., 2011; Garcia-Cabrero et al., 2013; Bezzina et al., 2015; Van Erum et al., 2020) PTZ administration resulted in a range of seizure-like behaviors from freezing and myoclonic twitches to tonic-clonic seizures. Overall, the incidence of the combined seizure behaviors was higher with APOE4 compared to APOE3 (97.2%, 35/36 E4FAD− and E4FAD+ mice vs. 73.5%, 25/34 E3FAD− and E3FAD+ mice, Figure 2A). In addition to exhibiting a higher frequency of tonic-clonic seizures (80.5%, 29/36 APOE4 vs. 58.8%, 20/34 APOE3, p < 0.05, Chi-square test), only 1 out of the remaining 7 APOE4 mice showed no seizures compared to 9 out of the 14 APOE3 mice.
We next evaluated if sex or FAD genotype within the APOE4 group (E4FAD− and E4FAD+) modulated PTZ-induced seizures. There was similar frequency of total seizure occurrence in both male (94.1%, 16/17) and female (100%, 19/19) APOE4 mice (p = 0.47, male E4FAD− and E4FAD+ vs. female E4FAD− and E4FAD+, Fisher’s exact test). In addition, sex did not alter the distribution of seizures (tonic-clonic seizures compared to total seizures, 12/16 male, 17/19 female, Figure 2B). E4FAD− (93.8%, 15/16) and E4FAD+ (100%, 20/20) mice also exhibited comparable levels of total seizure occurrences (p = 0.44, E4FAD− vs. E4FAD+ mice, Fisher’s exact test). However, when evaluated as a distribution of total seizures, a higher proportion of E4FAD+ mice (95%, 19/20) developed tonic-clonic seizures when compared to the E4FAD− group (66.6%, 10/15) (Figure 2C).
As with APOE4, a similar frequency of total seizures was observed between male (86.7%, 13/15 male) and female APOE3 mice (63.2%, 12/19 female) (p = 0.12, male E3FAD- and E3FAD+ vs. female E3FAD− and E3FAD+, Chi-square test) with similar seizure distribution (9/13 male, 11/12 female, Figure 2D). E3FAD− (64.7%, 11/17) and E3FAD+ (82.3% 14/17) mice also exhibited comparable levels of total seizures (p = 0.24, E3FAD− vs. E3FAD+ mice, Chi-square test). Although there was a higher frequency of tonic-clonic seizures in E3FAD + mice (92.8%, 13/14) compared to the E3FAD− group (63.6%, 7/11), this was not statistically significant (p = 0.07, Figure 2E). Our study may have lacked power to detect differences in PTZ-induced tonic-clonic seizures between E3FAD- and E3FAD + mice. Therefore, we performed additional analysis by assigning a score (3 = tonic-clonic, 2 = freezing and 1 = no seizure) to each mouse after PTZ injection (Figure 3). When assessed by two-way ANOVA, a significant main effect of APOE and FAD were detected, indicating that both variables modulated the behavioral effects of PTZ. However, there was no APOE x FAD interaction, supporting our initial analysis that after PTZ treatment, the incidence of seizures is higher with APOE4 and with FAD mutations.
Collectively, these results demonstrate that compared to APOE3, APOE4 is associated with higher incidence of PTZ-induced seizures. Further, in contrast to cage change day, our data indicate that FAD mutations/Aβ levels are associated with a higher distribution of tonic-clonic seizures in APOE4, and potentially APOE3 mice.
Discussion
Compared to APOE3, APOE4 is associated with tonic-clonic seizures when assessed during cage change day and greater seizure incidence after PTZ injection. Although sex did not modulate seizure incidence in EFAD mice, there was an important impact of FAD genotype. For tonic-clonic seizures in APOE4 mice, FAD mutations were associated with lower incidence when measured during cage change day, but a higher distribution after PTZ injections. Collectively, our data support that APOE4-associated seizures are an important component of the behavioral phenotype in aging-and AD-relevant mice. Thus, research focused on evaluating the cellular basis of these seizures could provide mechanistic insight onto how APOE and FAD mutations modulate neural circuit function and connectivity.
Higher Seizure Incidence With APOE4: AD Relevance
Seizures have emerged as an important component of the AD phenotype, with multiple groups reporting higher seizure incidence in patients with dementia and AD (reviewed in Palop and Mucke, 2009; Friedman et al., 2012; Pandis and Scarmeas, 2012; Lam and Noebels, 2020). Rather than a cause of AD, seizures represent a manifestation of altered neuronal function, which may exacerbate brain dysfunction and disease progression caused by the complex repertoire of AD pathologies. Although tonic-clonic seizures could be managed with anti-epileptic medications, sub-clinical seizures may be overlooked in AD patients and the underlying causes of the neuronal hyperexcitability will remain. Thus, understanding the extent AD risk factors modulate seizures could enable mechanistic research on their underlying causes that in turn can be translated to effective biomarker and therapeutic applications for AD patients in the clinic. In a disease as complex as AD, the threshold for neuronal dysfunction to produce seizures may be modified by genetic and lifestyle risk factors. In this study, we report that seizures are higher with one of the greatest genetic risk factors for AD, APOE4, in both the absence and presence of FAD mutations (see section “Seizure Incidence Is Modified by FAD Mutations but Not Sex” for discussion on sex and FAD). These data are consistent with reports suggesting that epilepsy risk is higher with APOE4 (Liang et al., 2019), including after trauma (Diaz-Arrastia et al., 2003; Harden, 2004). Further, APOE4 is associated with an earlier age of onset for intractable seizures (Briellmann et al., 2000; Gambardella et al., 2005; Kauffman et al., 2010), greater memory dysfunction with chronic temporal lobe epilepsy (Gouras et al., 1997; Gambardella et al., 2005; Busch et al., 2007) and higher epileptiform activity after hyperventilation (Ponomareva et al., 2008). Accordingly, targeted replacement mice with APOE4, which are similar to the E4FAD− mice, also showed higher seizures and a faster progression through PTZ-induced seizures (Hunter et al., 2012). It is therefore conceivable that compared to APOE3, APOE4 would increase the incidence of seizures and the onset of epileptiform activity in AD patients, however, there are currently no reports of such an association. Thus, further pre-clinical and clinical studies are warranted to clarify whether the neuronal changes that increase seizure phenotypes with APOE4 is independent of AD or interact with AD pathology to manifest as a different behavior in patients.
Seizure Incidence Is Modified by FAD Mutations but Not Sex
Previous studies in APOE knock-in mice have demonstrated that the effects of APOE4 on a number of functions are particularly prominent in female mice. For example, detrimental effects of APOE4 on learning and memory behaviors, Aβ levels, cerebrovascular function and neuroinflammation are typically higher in female mice (Balu et al., 2019). Interestingly, we did not observe an effect of sex for tonic-clonic seizures. Therefore, the changes in neuronal circuits that result in the manifestation of tonic-clonic seizures may be more proximal to the biological effects of apoE4, rather than an interaction with sex hormones.
FAD mice are known to exhibit epileptiform activity and lower threshold to seizure induction with auditory or PTZ stimuli; however, reports of tonic-clonic seizures are lacking (Palop and Mucke, 2009). 5xFAD mice were used to generate EFAD mice and are therefore similar to EFAD + mice but express mouse APOE and have an earlier onset of Aβ deposition. As for other FAD mice, to our knowledge there are no reports of tonic-clonic seizures in 5xFAD mice. However, when defined by electroencephalography recordings there are seizures in 5xFAD mice, possibly as early as 4 months (Abe et al., 2020), but that become prevalent at older ages (>10 months) (Paesler et al., 2015; Abe et al., 2020; Angel et al., 2020). In addition, one proposal is that the abnormal epileptiform activity predisposes 5xFAD mice to convulsive seizures with further stress, as has been demonstrated with genetic approaches (Paesler et al., 2015; Angel et al., 2020). Our data that 0% of E3FAD+ mice experience seizures are consistent with these findings, however, E4FAD+ mice did undergo a tonic-clonic seizures. These results indicate that in the presence of FAD mutations, compared to APOE3 APOE4 may have lowered the threshold for the onset of tonic-clonic seizures, as proposed for additional stressors in 5xFAD mice.
Although our data support that compared to APOE3, APOE4 is associated with higher tonic-clonic seizures, the precise interaction between APOE4 and FAD mutations remained poorly understood. During cage change day, there were higher tonic-clonic seizures in E4FAD− mice compared to E4FAD+ mice. This result is somewhat surprising, since typically the assumption is that the combination of APOE4 and FAD mutations would result in higher dysfunction than APOE4 alone. One potential explanation for lower seizures in E4FAD+ mice compared to E4FAD− mice is that the FAD mutations have changed the types of seizures that are occurring with APOE4 (e.g., to higher epileptiform activity and partial seizures as in FAD mice). On the other hand, it is possible that we have missed the detection of seizures in FAD + mice during, before or after cage changes. Alternatively, disruption of neuronal circuits involved in tonic-clonic seizures (e.g., brain stem, amygdala) with FAD and APOE4 could also blunt or alter the response to stimuli produced during routine cage change. Indeed, our data obtained following PTZ injection suggest that there is higher neuronal dysfunction with FAD mutations as revealed by E4FAD+ mice exhibiting higher distribution of tonic-clonic seizures than E4FAD− mice, an effect that was also trending in APOE3 mice.
It is also conceivable that distinct neural circuits are recruited between seizures induced during cage changes and elicited by PTZ. Stimuli produced during cage changes (sounds, new environment, handling) results in stress and anxiety in rodents, as evident from changes in behavior, hormone levels and heart rates (Duke et al., 2001; Meller et al., 2011; Rasmussen et al., 2011). In fact, placing a mouse in a new environment in ways that are similar, if not identical to the cage change procedure in our study is used as an assay of tonic-clonic seizures susceptibility (Todorova et al., 1999; Leussis and Heinrichs, 2006; Hunter et al., 2012; Qi et al., 2018). In this regard, tonic-clonic seizures during cage changes are the result of stress/anxiety signals inducing neuronal hyperexcitability. On the other hand, PTZ induces seizures by directly impacting neuronal activity, and although the precise mechanism of action is unknown (Hansen et al., 2004), it is thought to involve antagonism of GABA-A receptors. Therefore, PTZ-induced tonic-clonic seizures are a more direct maker of alterations in neuronal functional connectivity (i.e., balance of GABAergic and glutamatergic inputs). Due to the different ways that they induce seizures, there are several potential explanations for the seemingly opposite effect of FAD mutations on APOE4 associated cage change and PTZ-induced tonic-clonic seizures. For example, the combined effects of APOE4 and FAD may have disrupted neuronal circuits to an extent that stress cannot induce seizures, yet the remaining neurons are more sensitive to chemical-induced seizures. Alternatively, there are greater memory impairments in E4FAD+ mice than E4FAD− mice, and so EFAD− mice may anticipate what the stimuli of cage change represents. Finally, E4FAD− and E4FAD+ mice may exhibit different levels of susceptibility to stress-induced effects through aging as a result of distinct mechanisms of adaptation occurring at the neural circuit level.
Potential Mechanisms Underlying APOE4-Associated Seizures
Our data also point to potential cellular mechanisms underlying the impact of APOE4 on tonic-clonic seizure incidence in vivo. There are general and specific considerations for discussing this concept, all of which continue to be the focus of several research groups (reviewed in Mahley et al., 2007; Liu et al., 2013; Flowers and Rebeck, 2020). The question of how a single amino acid difference between apolipoprotein E3 (apoE3, cysteine at 112) and apolipoprotein E4 (apoE4, arginine at 112) results in modulation of such a wide range of functions in the brain is proving extremely complicated to answer and is likely context dependent. Apolipoprotein E is produced by cells in the periphery and in the brain. Within the central nervous system apoE is produced primarily by glia (astrocytes and microglia), but also by pericytes and neurons and all apoE is found on lipoprotein particles in the interstitial fluid. Therefore, one initial question surrounds the levels and lipidation state of apoE-containing lipoproteins. One suggestion is that apoE4-containing lipoproteins are lower in levels, less lipidated and/or smaller than apoE3-containing lipoproteins, which could have a profound impact on neural circuit connectivity and function. For example, changes in apoE levels and lipidation could disrupt lipoprotein functions in the interstitial fluid such as homeostasis of cholesterol and lipids, binding to debris and other substrates, and as an adaptor molecule. In addition, the structural properties of apoE4 are thought to result in altered activation and recycling of the apoE receptors in all cell types, and/or the generation of intracellular toxic apoE4 fragments in neurons. Thus, through these fundamental processes, apoE can alter neuronal network excitability directly, or indirectly through effects on inflammation, cerebrovascular function, and general homeostatic functions. Intertwined are an equally complex set of research questions that include but not limited to whether APOE4 is a toxic gain or loss of function and whether APOE4 imparts advantages on brain function during specific developmental windows that are detrimental in the context of aging and in response to stressors (Mahley et al., 2007; Liu et al., 2013; Flowers and Rebeck, 2020). That apoE impacts such a myriad of cell types and functions in normal and stress conditions is at the heart of why dissecting role of apoE in brain function is extremely complex. Specifically, in the context of seizures, all these changes during aging between APOE3 and APOE4 will likely converge to cause hyperexcitable neuronal networks in different brain regions that are important for tonic-clonic seizure manifestation.
APOE4 has been linked to changes in neuron structure and activity in multiple brain regions including the amydagala, cortex, and the hippocampus. Of particular interest is the emerging concept that apoE4 disrupts inhibitory network function (reviewed in Najm et al., 2019). For example, in APOE-targeted replacement mice, compared to APOE3, with APOE4 there are lower levels of GABAergic somatostatin-positive interneurons in the hippocampus, an effect that appears driven by apoE production by neurons (Najm et al., 2019). Thus, the loss of GABAergic interneurons could contribute to network hyperexcitability and higher pyramidal cell firing (Nuriel et al., 2017). In humans, there is reduced deactivation of the default mode network with APOE4 in task-based assays (Pihlajamaki and Sperling, 2009) in association with higher hippocampal (Dickerson et al., 2005) and entorhinal activation (Bondi et al., 2005). Collectively, these observations suggest that lower GABAergic activity with APOE4 could reduce the threshold for seizures even though APOE4 is known to disrupt excitatory neuronal activity as well (Mahley et al., 2007; Liu et al., 2013; Flowers and Rebeck, 2020). Overall, future studies are needed to establish the extent by which APOE4 associated seizures are directly or indirectly dependent on neuronal excitability in different cortical and subcortical brain regions.
Limitations and Future Directions
Although we provided data on seizure incidence, an important question that remains unclear is the underlying neuronal mechanisms that are disrupted with APOE4 to contribute to the development of seizures. Addressing this question is ultimately critical from mechanistic standpoint as well for testing in human patients. Future detailed experiments could focus on tracking epileptiform activity and seizure incidence in EFAD mice across the lifespan. We focused on older mice as we had not observed seizures during cage change day in younger mice, however there are some complications with utilizing older FAD mice (discussed in Tai et al., 2021), particularly for E4FAD+ mice. In E4FAD+ mice, Aβ pathology (soluble and extracellular) initiates around 4 months and so there is advanced pathology (e.g., Aβ, neuroinflammation, blood-brain barrier deficits) by 8 months of age. The high pathology may have altered brain function in ways that are too advanced to detect changes that are more relevant for early stages of Aβ deposition and evaluate the impact of APOE4 and FAD on PTZ-induced seizure induction, which require younger mice. Ultimately, however, to provide more detailed mechanistic insight, pharmacological or genetic manipulations targeting either apoE, functions modulated by apoE or defined cell types and circuits could be conducted to reveal a connection with seizure activity. A discussion on the limitations of mouse models is beyond the scope of this manuscript (reviewed in Tai et al., 2021), however, it will be important to evaluate whether a seizure phenotype also manifests in APP-knock in APOE4 mice, to validate that findings are not due to the FAD mutations.
Conclusion
Our data demonstrate that unlike APOE3, APOE4 is associated with tonic seizures, when evaluated during cage changes. There is also a higher incidence of tonic-clonic seizures with APOE4 compared to APOE3 following PTZ injection. However, in contrast to cage changes, the distribution of seizures to the tonic-clonic phenotype is higher with FAD mutations. These data support that APOE4 is associated with higher tonic-clonic seizures, and that FAD mutations impact tonic-clonic seizures in a paradigm dependent manner.
Data Availability Statement
All data supporting the conclusions of this manuscript will be made available to any qualified researcher without undue reservation.
Ethics Statement
The animal study was reviewed and approved by the Institutional Animal Care and Use Committee at the University of Illinois at Chicago.
Author Contributions
LL, FM, KT, and LT conceived the study, performed the experiments, and wrote the manuscript. All authors approved the submitted version.
Funding
This work was supported by the National Institutes of Health Grants R01AG061114, R01AG061114-01S1 (LT) and the University of Illinois at Chicago – College of Medicine Institutional funds (LT and KT).
Conflict of Interest
The authors declare that the research was conducted in the absence of any commercial or financial relationships that could be construed as a potential conflict of interest.
Acknowledgments
We would like to acknowledge the following animal husbandry staff for their efforts in identifying tonic-clonic seizure activity in our mouse colony during cage changes; Jose Arroyo, Jose Serrano and Leonardo Gonzalez.
References
Abe, Y., Ikegawa, N., Yoshida, K., Muramatsu, K., Hattori, S., Kawai, K., et al. (2020). Behavioral and electrophysiological evidence for a neuroprotective role of aquaporin-4 in the 5xFAD transgenic mice model. Acta Neuropathol. Commun. 8:67. doi: 10.1186/s40478-020-00936-3
Amatniek, J. C., Hauser, W. A., DelCastillo-Castaneda, C., Jacobs, D. M., Marder, K., Bell, K., et al. (2006). Incidence and predictors of seizures in patients with Alzheimer’s disease. Epilepsia 47, 867–872. doi: 10.1111/j.1528-1167.2006.00554.x
Angel, A., Volkman, R., Royal, T. G., and Offen, D. (2020). Caspase-6 knockout in the 5xFAD model of Alzheimer’s disease reveals favorable outcome on memory and neurological hallmarks. Int. J. Mol. Sci. 21:1144. doi: 10.3390/ijms21031144
Balu, D., Karstens, A. J., Loukenas, E., Maldonado Weng, J., York, J. M., Valencia-Olvera, A. C., et al. (2019). The role of APOE in transgenic mouse models of AD. Neurosci. Lett. 707:134285. doi: 10.1016/j.neulet.2019.134285
Bernardi, S., Scaldaferri, N., Vanacore, N., Trebbastoni, A., Francia, A., D’Amico, A., et al. (2010). Seizures in Alzheimer’s disease: a retrospective study of a cohort of outpatients. Epileptic Disord. 12, 16–21. doi: 10.1684/epd.2010.0290
Bezzina, C., Verret, L., Juan, C., Remaud, J., Halley, H., Rampon, C., et al. (2015). Early onset of hypersynchronous network activity and expression of a marker of chronic seizures in the Tg2576 mouse model of Alzheimer’s disease. PLoS One 10:e0119910. doi: 10.1371/journal.pone.0119910
Bondi, M. W., Houston, W. S., Eyler, L. T., and Brown, G. G. (2005). fMRI evidence of compensatory mechanisms in older adults at genetic risk for Alzheimer disease. Neurology 64, 501–508. doi: 10.1212/01.WNL.0000150885.00929.7E
Bour, A., Grootendorst, J., Vogel, E., Kelche, C., Dodart, J. C., Bales, K., et al. (2008). Middle-aged human apoE4 targeted-replacement mice show retention deficits on a wide range of spatial memory tasks. Behav. Brain Res. 193, 174–182. doi: 10.1016/j.bbr.2008.05.008
Brault, V., Martin, B., Costet, N., Bizot, J. C., and Herault, Y. (2011). Characterization of PTZ-induced seizure susceptibility in a down syndrome mouse model that overexpresses CSTB. PLoS One 6:e27845. doi: 10.1371/journal.pone.0027845
Briellmann, R. S., Torn-Broers, Y., Busuttil, B. E., Major, B. J., Kalnins, R. M., Olsen, M., et al. (2000). APOE epsilon4 genotype is associated with an earlier onset of chronic temporal lobe epilepsy. Neurology 55, 435–437. doi: 10.1212/wnl.55.3.435
Brunetti, V., D’Atri, A., Della Marca, G., Vollono, C., Marra, C., Vita, M. G., et al. (2020). Subclinical epileptiform activity during sleep in Alzheimer’s disease and mild cognitive impairment. Clin. Neurophysiol. 131, 1011–1018. doi: 10.1016/j.clinph.2020.02.015
Busch, R. M., Lineweaver, T. T., Naugle, R. I., Kim, K. H., Gong, Y., Tilelli, C. Q., et al. (2007). ApoE-epsilon4 is associated with reduced memory in long-standing intractable temporal lobe epilepsy. Neurology 68, 409–414. doi: 10.1212/01.wnl.0000253021.60887.db
Cheng, C. H., Liu, C. J., Ou, S. M., Yeh, C. M., Chen, T. J., Lin, Y. Y., et al. (2015). Incidence and risk of seizures in Alzheimer’s disease: a nationwide population-based cohort study. Epilepsy Res. 115, 63–66. doi: 10.1016/j.eplepsyres.2015.05.009
Costa, C., Romoli, M., Liguori, C., Farotti, L., Eusebi, P., Bedetti, C., et al. (2019). Alzheimer’s disease and late-onset epilepsy of unknown origin: two faces of beta amyloid pathology. Neurobiol. Aging 73, 61–67. doi: 10.1016/j.neurobiolaging.2018.09.006
Cretin, B., Sellal, F., Philippi, N., Bousiges, O., Di Bitonto, L., Martin-Hunyadi, C., et al. (2016). Epileptic prodromal Alzheimer’s disease, a retrospective study of 13 new cases: expanding the spectrum of Alzheimer’s disease to an epileptic variant? J. Alzheimers Dis. 52, 1125–1133. doi: 10.3233/JAD-150096
Del Vecchio, R. A., Gold, L. H., Novick, S. J., Wong, G., and Hyde, L. A. (2004). Increased seizure threshold and severity in young transgenic CRND8 mice. Neurosci. Lett. 367, 164–167. doi: 10.1016/j.neulet.2004.05.107
Diaz-Arrastia, R., Gong, Y., Fair, S., Scott, K. D., Garcia, M. C., Carlile, M. C., et al. (2003). Increased risk of late posttraumatic seizures associated with inheritance of APOE epsilon4 allele. Arch. Neurol. 60, 818–822. doi: 10.1001/archneur.60.6.818
Dickerson, B. C., Salat, D. H., Greve, D. N., Chua, E. F., Rand-Giovannetti, E., Rentz, D. M., et al. (2005). Increased hippocampal activation in mild cognitive impairment compared to normal aging and AD. Neurology 65, 404–411. doi: 10.1212/01.wnl.0000171450.97464.49
DiFrancesco, J. C., Tremolizzo, L., Polonia, V., Giussani, G., Bianchi, E., Franchi, C., et al. (2017). Adult-onset epilepsy in presymptomatic Alzheimer’s disease: a retrospective study. J. Alzheimers Dis. 60, 1267–1274. doi: 10.3233/JAD-170392
Duke, J. L., Zammit, T. G., and Lawson, D. M. (2001). The effects of routine cage-changing on cardiovascular and behavioral parameters in male sprague-dawley rats. Contemp. Top. Lab. Anim. Sci. 40, 17–20.
Flowers, S. A., and Rebeck, G. W. (2020). APOE in the normal brain. Neurobiol. Dis. 136:104724. doi: 10.1016/j.nbd.2019.104724
Friedman, D., Honig, L. S., and Scarmeas, N. (2012). Seizures and epilepsy in Alzheimer’s disease. CNS Neurosci. Ther. 18, 285–294. doi: 10.1111/j.1755-5949.2011.00251.x
Gambardella, A., Aguglia, U., Chifari, R., Labate, A., Manna, I., Serra, P., et al. (2005). ApoE epsilon4 allele and disease duration affect verbal learning in mild temporal lobe epilepsy. Epilepsia 46, 110–117. doi: 10.1111/j.0013-9580.2005.15804.x
Garcia-Cabrero, A. M., Guerrero-Lopez, R., Giraldez, B. G., Llorens-Martin, M., Avila, J., Serratosa, J. M., et al. (2013). Hyperexcitability and epileptic seizures in a model of frontotemporal dementia. Neurobiol. Dis. 58, 200–208. doi: 10.1016/j.nbd.2013.06.005
Gouras, G. K., Relkin, N. R., Sweeney, D., Munoz, D. G., Mackenzie, I. R., and Gandy, S. (1997). Increased apolipoprotein E epsilon 4 in epilepsy with senile plaques. Ann. Neurol. 41, 402–404. doi: 10.1002/ana.410410317
Grootendorst, J., Bour, A., Vogel, E., Kelche, C., Sullivan, P. M., Dodart, J. C., et al. (2005). Human apoE targeted replacement mouse lines: h-apoE4 and h-apoE3 mice differ on spatial memory performance and avoidance behavior. Behav. Brain Res. 159, 1–14. doi: 10.1016/j.bbr.2004.09.019
Gureviciene, I., Ishchenko, I., Ziyatdinova, S., Jin, N., Lipponen, A., Gurevicius, K., et al. (2019). Characterization of epileptic spiking associated with brain amyloidosis in APP/PS1 mice. Front. Neurol. 10:1151. doi: 10.3389/fneur.2019.01151
Hansen, S. L., Sperling, B. B., and Sanchez, C. (2004). Anticonvulsant and antiepileptogenic effects of GABAA receptor ligands in pentylenetetrazole-kindled mice. Prog. Neuropsychopharmacol. Biol. Psychiatry 28, 105–113. doi: 10.1016/j.pnpbp.2003.09.026
Harden, C. L. (2004). The apolipoprotein E epsilon (epsilon) 4 allele is important for trauma-related epilepsy. Epilepsy Curr. 4, 29–30. doi: 10.1111/j.1535-7597.2004.04112.x
Hauser, W. A., Morris, M. L., Heston, L. L., and Anderson, V. E. (1986). Seizures and myoclonus in patients with Alzheimer’s disease. Neurology 36, 1226–1230. doi: 10.1212/wnl.36.9.1226
Hesdorffer, D. C., Hauser, W. A., Annegers, J. F., Kokmen, E., and Rocca, W. A. (1996). Dementia and adult-onset unprovoked seizures. Neurology 46, 727–730. doi: 10.1212/wnl.46.3.727
Hirsch, L. J. (2007). ApoE, MemorE, and EpilepsE. Epilepsy Curr. 7, 149–150. doi: 10.1111/j.1535-7511.2007.00208.x
Hunter, J. M., Cirrito, J. R., Restivo, J. L., Kinley, R. D., Sullivan, P. M., Holtzman, D. M., et al. (2012). Emergence of a seizure phenotype in aged apolipoprotein epsilon 4 targeted replacement mice. Brain Res. 1467, 120–132. doi: 10.1016/j.brainres.2012.05.048
Imfeld, P., Bodmer, M., Schuerch, M., Jick, S. S., and Meier, C. R. (2013). Seizures in patients with Alzheimer’s disease or vascular dementia: a population-based nested case-control analysis. Epilepsia 54, 700–707. doi: 10.1111/epi.12045
Irizarry, M. C., Jin, S., He, F., Emond, J. A., Raman, R., Thomas, R. G., et al. (2012). Incidence of new-onset seizures in mild to moderate Alzheimer disease. Arch. Neurol. 69, 368–372. doi: 10.1001/archneurol.2011.830
Kauffman, M. A., Consalvo, D., Moron, D. G., Lereis, V. P., and Kochen, S. (2010). ApoE epsilon4 genotype and the age at onset of temporal lobe epilepsy: a case-control study and meta-analysis. Epilepsy Res. 90, 234–239. doi: 10.1016/j.eplepsyres.2010.05.007
Kazim, S. F., Chuang, S. C., Zhao, W., Wong, R. K., Bianchi, R., and Iqbal, K. (2017). Early-onset network hyperexcitability in presymptomatic Alzheimer’s disease transgenic mice is suppressed by passive immunization with anti-human APP/Abeta antibody and by mGluR5 blockade. Front. Aging Neurosci. 9:71. doi: 10.3389/fnagi.2017.00071
Keret, O., Hoang, T. D., Xia, F., Rosen, H. J., and Yaffe, K. (2020). Association of late-onset unprovoked seizures of unknown etiology with the risk of developing dementia in older veterans. JAMA Neurol. 77, 710–715. doi: 10.1001/jamaneurol.2020.0187
Lam, A. D., and Noebels, J. (2020). Night watch on the titanic: detecting early signs of Epileptogenesis in Alzheimer disease. Epilepsy Curr. 20, 369–374. doi: 10.1177/1535759720964775
Lam, A. D., Sarkis, R. A., Pellerin, K. R., Jing, J., Dworetzky, B. A., Hoch, D. B., et al. (2020). Association of epileptiform abnormalities and seizures in Alzheimer disease. Neurology 95, e2259–e2270. doi: 10.1212/WNL.0000000000010612
Leussis, M. P., and Heinrichs, S. C. (2006). Routine tail suspension husbandry facilitates onset of seizure susceptibility in EL mice. Epilepsia 47, 801–804. doi: 10.1111/j.1528-1167.2006.00525.x
Liang, Y., Zhou, Z., Wang, H., Cheng, X., Zhong, S., and Zhao, C. (2019). Association of apolipoprotein E genotypes with epilepsy risk: a systematic review and meta-analysis. Epilepsy Behav. 98(Pt A), 27–35. doi: 10.1016/j.yebeh.2019.06.015
Liu, C. C., Liu, C. C., Kanekiyo, T., Xu, H., and Bu, G. (2013). Apolipoprotein E and Alzheimer disease: risk, mechanisms and therapy. Nat. Rev. Neurol. 9, 106–118. doi: 10.1038/nrneurol.2012.263
Lozsadi, D. A., and Larner, A. J. (2006). Prevalence and causes of seizures at the time of diagnosis of probable Alzheimer’s disease. Dement. Geriatr. Cogn. Disord. 22, 121–124. doi: 10.1159/000093664
Mahley, R. W., Huang, Y., and Weisgraber, K. H. (2007). Detrimental effects of apolipoprotein E4: potential therapeutic targets in Alzheimer’s disease. Curr. Alzheimer Res. 4, 537–540. doi: 10.2174/156720507783018334
McAreavey, M. J., Ballinger, B. R., and Fenton, G. W. (1992). Epileptic seizures in elderly patients with dementia. Epilepsia 33, 657–660. doi: 10.1111/j.1528-1157.1992.tb02343.x
Meller, A., Kasanen, I., Ruksenas, O., Apanaviciene, N., Baturaite, Z., Voipio, H. M., et al. (2011). Refining cage change routines: comparison of cardiovascular responses to three different ways of cage change in rats. Lab. Anim. 45, 167–173. doi: 10.1258/la.2011.010134
Mendez, M. F., Catanzaro, P., Doss, R. C., Raul, A., and Frey, W. H. II (1994). Seizures in Alzheimer’s disease: clinicopathologic study. J. Geriatr. Psychiatry Neurol. 7, 230–233. doi: 10.1177/089198879400700407
Minkeviciene, R., Rheims, S., Dobszay, M. B., Zilberter, M., Hartikainen, J., Fulop, L., et al. (2009). Amyloid beta-induced neuronal hyperexcitability triggers progressive epilepsy. J. Neurosci. 29, 3453–3462. doi: 10.1523/JNEUROSCI.5215-08.2009
Najm, R., Jones, E. A., and Huang, Y. (2019). Apolipoprotein E4, inhibitory network dysfunction, and Alzheimer’s disease. Mol. Neurodegener. 14:24. doi: 10.1186/s13024-019-0324-6
Nuriel, T., Angulo, S. L., Khan, U., Ashok, A., Chen, Q., Figueroa, H. Y., et al. (2017). Neuronal hyperactivity due to loss of inhibitory tone in APOE4 mice lacking Alzheimer’s disease-like pathology. Nat. Commun. 8:1464. doi: 10.1038/s41467-017-01444-0
Paesler, K., Xie, K., Hettich, M. M., Siwek, M. E., Ryan, D. P., Schroder, S., et al. (2015). Limited effects of an eIF2alphaS51A allele on neurological impairments in the 5xFAD mouse model of Alzheimer’s disease. Neural Plast. 2015:825157. doi: 10.1155/2015/825157
Palop, J. J., Chin, J., Roberson, E. D., Wang, J., Thwin, M. T., Bien-Ly, N., et al. (2007). Aberrant excitatory neuronal activity and compensatory remodeling of inhibitory hippocampal circuits in mouse models of Alzheimer’s disease. Neuron 55, 697–711. doi: 10.1016/j.neuron.2007.07.025
Palop, J. J., and Mucke, L. (2009). Epilepsy and cognitive impairments in Alzheimer disease. Arch. Neurol. 66, 435–440. doi: 10.1001/archneurol.2009.15
Pandis, D., and Scarmeas, N. (2012). Seizures in Alzheimer disease: clinical and epidemiological data. Epilepsy Curr. 12, 184–187. doi: 10.5698/1535-7511-12.5.184
Pihlajamaki, M., and Sperling, R. A. (2009). Functional MRI assessment of task-induced deactivation of the default mode network in Alzheimer’s disease and at-risk older individuals. Behav. Neurol. 21, 77–91. doi: 10.3233/BEN-2009-0231
Ponomareva, N. V., Korovaitseva, G. I., and Rogaev, E. I. (2008). EEG alterations in non-demented individuals related to apolipoprotein E genotype and to risk of Alzheimer disease. Neurobiol. Aging 29, 819–827. doi: 10.1016/j.neurobiolaging.2006.12.019
Qi, J., Kim, M., Sanchez, R., Ziaee, S. M., Kohtz, J. D., and Koh, S. (2018). Enhanced susceptibility to stress and seizures in GAD65 deficient mice. PLoS One 13:e0191794. doi: 10.1371/journal.pone.0191794
Rao, S. C., Dove, G., Cascino, G. D., and Petersen, R. C. (2009). Recurrent seizures in patients with dementia: frequency, seizure types, and treatment outcome. Epilepsy Behav. 14, 118–120. doi: 10.1016/j.yebeh.2008.08.012
Rasmussen, S., Miller, M. M., Filipski, S. B., and Tolwani, R. J. (2011). Cage change influences serum corticosterone and anxiety-like behaviors in the mouse. J. Am. Assoc. Lab. Anim. Sci. 50, 479–483.
Risse, S. C., Lampe, T. H., Bird, T. D., Nochlin, D., Sumi, S. M., Keenan, T., et al. (1990). Myoclonus, seizures, and paratonia in Alzheimer disease. Alzheimer Dis. Assoc. Disord. 4, 217–225. doi: 10.1097/00002093-199040400-00003
Rodriguez, G. A., Burns, M. P., Weeber, E. J., and Rebeck, G. W. (2013). Young APOE4 targeted replacement mice exhibit poor spatial learning and memory, with reduced dendritic spine density in the medial entorhinal cortex. Learn. Mem. 20, 256–266. doi: 10.1101/lm.030031.112
Romanelli, M. F., Morris, J. C., Ashkin, K., and Coben, L. A. (1990). Advanced Alzheimer’s disease is a risk factor for late-onset seizures. Arch. Neurol. 47, 847–850. doi: 10.1001/archneur.1990.00530080029006
Sarkis, R. A., Dickerson, B. C., Cole, A. J., and Chemali, Z. N. (2016). Clinical and neurophysiologic characteristics of unprovoked seizures in patients diagnosed with dementia. J. Neuropsychiatry Clin. Neurosci. 28, 56–61. doi: 10.1176/appi.neuropsych.15060143
Scarmeas, N., Honig, L. S., Choi, H., Cantero, J., Brandt, J., Blacker, D., et al. (2009). Seizures in Alzheimer disease: who, when, and how common? Arch. Neurol. 66, 992–997. doi: 10.1001/archneurol.2009.130
Siwek, M. E., Muller, R., Henseler, C., Trog, A., Lundt, A., Wormuth, C., et al. (2015). Altered theta oscillations and aberrant cortical excitatory activity in the 5XFAD model of Alzheimer’s disease. Neural Plast. 2015:781731. doi: 10.1155/2015/781731
Tai, L. M., Balu, D., Avila-Munoz, E., Abdullah, L., Thomas, R., Collins, N., et al. (2017). EFAD transgenic mice as a human APOE relevant preclinical model of Alzheimer’s disease. J. Lipid Res. 58, 1733–1755. doi: 10.1194/jlr.R076315
Tai, L. M., Maldonado Weng, J., LaDu, M. J., and Brady, S. T. (2021). Relevance of transgenic mouse models for Alzheimer’s disease. Prog. Mol. Biol. Transl. Sci. 177, 1–48. doi: 10.1016/bs.pmbts.2020.07.007
Tai, L. M., Thomas, R., Marottoli, F. M., Koster, K. P., Kanekiyo, T., Morris, A. W., et al. (2016). The role of APOE in cerebrovascular dysfunction. Acta Neuropathol. 131, 709–723. doi: 10.1007/s00401-016-1547-z
Thomas, R., Morris, A. W. J., and Tai, L. M. (2017). Epidermal growth factor prevents APOE4-induced cognitive and cerebrovascular deficits in female mice. Heliyon 3:e00319. doi: 10.1016/j.heliyon.2017.e00319
Todorova, M. T., Burwell, T. J., and Seyfried, T. N. (1999). Environmental risk factors for multifactorial epilepsy in EL mice. Epilepsia 40, 1697–1707. doi: 10.1111/j.1528-1157.1999.tb01586.x
Van Erum, J., Valkenburg, F., Van Dam, D., and De Deyn, P. P. (2020). Pentylenetetrazole-induced seizure susceptibility in the Tau58/4 transgenic mouse model of tauopathy. Neuroscience 425, 112–122. doi: 10.1016/j.neuroscience.2019.11.007
Villasana, L., Acevedo, S., Poage, C., and Raber, J. (2006). Sex- and APOE isoform-dependent effects of radiation on cognitive function. Radiat. Res. 166, 883–891. doi: 10.1667/RR0642.1
Volicer, L., Smith, S., and Volicer, B. J. (1995). Effect of seizures on progression of dementia of the Alzheimer type. Dementia 6, 258–263. doi: 10.1159/000106956
Vossel, K. A., Beagle, A. J., Rabinovici, G. D., Shu, H., Lee, S. E., Naasan, G., et al. (2013). Seizures and epileptiform activity in the early stages of Alzheimer disease. JAMA Neurol. 70, 1158–1166. doi: 10.1001/jamaneurol.2013.136
Vossel, K. A., Ranasinghe, K. G., Beagle, A. J., Mizuiri, D., Honma, S. M., Dowling, A. F., et al. (2016). Incidence and impact of subclinical epileptiform activity in Alzheimer’s disease. Ann. Neurol. 80, 858–870. doi: 10.1002/ana.24794
Westmark, C. J., Westmark, P. R., and Malter, J. S. (2010). Alzheimer’s disease and down syndrome rodent models exhibit audiogenic seizures. J. Alzheimers Dis. 20, 1009–1013. doi: 10.3233/JAD-2010-100087
Yagi, H., Takamura, Y., Yoneda, T., Konno, D., Akagi, Y., Yoshida, K., et al. (2005). Vlgr1 knockout mice show audiogenic seizure susceptibility. J. Neurochem. 92, 191–202. doi: 10.1111/j.1471-4159.2004.02875.x
Youmans, K. L., Tai, L. M., Nwabuisi-Heath, E., Jungbauer, L., Kanekiyo, T., Gan, M., et al. (2012). APOE4-specific changes in Abeta accumulation in a new transgenic mouse model of Alzheimer disease. J. Biol. Chem. 287, 41774–41786. doi: 10.1074/jbc.M112.407957
Zaldua, S., Damen, F. C., Pisharody, R., Thomas, R., Fan, K. D., Ekkurthi, G. K., et al. (2020). Epidermal growth factor treatment of female mice that express APOE4 at an age of advanced pathology mitigates behavioral and cerebrovascular dysfunction. Heliyon 6:e03919. doi: 10.1016/j.heliyon.2020.e03919
Keywords: Alzheimer’s disease, apolipoprotein E, seizure, amyloid beta, sex
Citation: Lamoureux L, Marottoli FM, Tseng KY and Tai LM (2021) APOE4 Promotes Tonic-Clonic Seizures, an Effect Modified by Familial Alzheimer’s Disease Mutations. Front. Cell Dev. Biol. 9:656521. doi: 10.3389/fcell.2021.656521
Received: 20 January 2021; Accepted: 23 February 2021;
Published: 16 March 2021.
Edited by:
Friederike Klempin, Charité – Universitätsmedizin Berlin, GermanyReviewed by:
Robert Marr, Rosalind Franklin University of Medicine and Science, United StatesFrancesco Tamagnini, University of Reading, United Kingdom
Copyright © 2021 Lamoureux, Marottoli, Tseng and Tai. This is an open-access article distributed under the terms of the Creative Commons Attribution License (CC BY). The use, distribution or reproduction in other forums is permitted, provided the original author(s) and the copyright owner(s) are credited and that the original publication in this journal is cited, in accordance with accepted academic practice. No use, distribution or reproduction is permitted which does not comply with these terms.
*Correspondence: Leon M. Tai, leontai@uic.edu