- 1Guangdong Provincial Key Laboratory on Brain Function Repair and Regeneration, Department of Functional Neurosurgery, Zhujiang Hospital of Southern Medical University, Guangzhou, China
- 2Department of Neurosurgery, The First Hospital of China Medical University, Shenyang, China
- 3Chinese Glioma Genome Atlas Network, Beijing, China
- 4Department of Neurosurgery, Beijing Tiantan Hospital, Capital Medical University, Beijing, China
- 5Division of Neurosurgery, Department of Surgery, Li Ka Shing Faculty of Medicine, The University of Hong Kong, Hong Kong, China
- 6School of Life Sciences and Biopharmaceutics, Guangdong Pharmaceutical University, Guangzhou, China
Mutation of the telomerase reverse transcriptase (TERT) promoter has been demonstrated as an unfavorable prognostic marker in patients with isocitrate dehydrogenase wild-type (IDHwt) glioma. This study aimed to investigate the immune role of TERT promoter mutation status which could improve prognostic prediction in IDHwt. TERT mutation status, IDH mutation, and 1p-19q codeletion status data were obtained from 614 glioma cases from the Cancer Genome Atlas, and 325 cases from the Chinese Glioma Genome Atlas. The same information was obtained from 49 clinical glioma tissues. TERT mutation is preferentially present in glioblastoma and IDH-wt gliomas and is associated with poor prognosis. Moreover, TERT mutation was associated with infiltration of neutrophils and expression of neutrophil chemokines. which might partially contribute to the poor outcome in IDH-wt glioma. Furthermore, patients with IDH-wt glioma did not harbor increased peripheral neutrophils, implying that the infiltrated neutrophil in the tumor environment might due to cytokine chemotaxis. In this study, we hereby propose that TERT mutation might be a molecular driver of the dysfunctional immune microenvironment in IDH-wt glioma. TERT mutation may be a potential immune therapeutic target for optimizing treatment combinations and patient selection for glioma immunotherapy.
Introduction
Glioma is the most common and malignant primary brain tumor in adults (Weller et al., 2015). Telomerase re-activation is frequent in malignancies and confers replicative immortality. It has been associated with poor outcome in brain tumors (Lotsch et al., 2013; Gojo et al., 2017; Gabler et al., 2019). Telomerase, the key enzyme for telomere length maintenance, is crucial for the survival of cancer cells (Duan and Zhao, 2018). Through its activation, tumor cells circumvent cellular senescence caused by telomere shortening, thus obtaining immortality (Martinez and Blasco, 2011). In brain cancers, concurrent mutations of BRAF and TERT promoter have been identified to be associated with tumor aggressiveness (Mistry et al., 2015; Matsumura et al., 2017; Mackay et al., 2018; Phillips et al., 2019). Telomeres are regions of repetitive nucleotide sequences (TTAGGG). They are composed of a DNA and telomere-binding proteins that at the ends of chromosomes (Blackburn et al., 2015). Ohali et al. (2006) reported that telomere length is associated with neuroblastoma prognosis. It is a reverse transcriptase that is composed of a catalytic protein subunit called telomerase reverse transcriptase (TERT), coding by the TERT gene in humans, and a human telomerase RNA (hTR) element encoded by hTERC (Duan and Zhao, 2018). Previous research have shown that TERT downregulation obviously inhibits the proliferation and invasion of neuroblastoma cells, and induces apoptosis (Chakrabarti et al., 2013). The poor prognosis of glioma is result from the transformed cells combining the tumor microenvironment, including vascular and stromal cells and inflammatory infiltrates (Hewedi et al., 2013; Poon et al., 2017). Current glioma diagnosis and classification system scarcely interrogates the effect of TERT mutation on glioma immune microenvironment. Here, we aimed to identify biomarkers associated with TERT promoter mutation status to improve prognosis prediction in IDH-wt glioma.
Materials and Methods
Patients and Databases
Datasets of The Cancer Genome Atlas (TCGA)1 and the Chinese Glioma Genome Atlas (CGGA)2 datasets were downloaded online. This study collected transcriptome expression data of 614 TCGA gliomas and 325 CGGA gliomas, including age, gender, diagnosis, WHO classification, chemotherapy and radiotherapy regimens, molecular data and prognosis. Meanwhile, tumor tissues of 49 gliomas who received surgical treatment in the first hospital of China Medical University from 2017 to 2018 were collected, including 12 WHO grade II, 22 WHO grade III and 15 glioblastoma patients (Supplementary Table 1). This study was approved by the ethics committee of the First Hospital of China Medical University. Written informed consent was obtained from all patients.
TERT Mutation
The TERT mutation status and mRNA expression data were downloaded from TCGA. The TERT status of 49 glioma patients was determined by sequencing.
IDH Mutation and 1p-19q Codeletion Status
IDH mutation and 1p-19q codeletion status data were downloaded from the TCGA and CGGA datasets. The IDH1 and IDH2 status of 49 glioma patients was ascertained as reported earlier (Gao et al., 2019).
Immunohistochemistry and Immunofluorescence
Formalin fixed paraffin embedded tissues were used for immunohistochemistry and immunofluorescence. Four micron thick slices were cut, dewaxed in xylene, rinsed in graded ethanol, and rehydrated in distilled water. After the antigen was extracted with sodium citrate buffer (10 mM sodium citrate, pH 6.0), the endogenous peroxidase activity was blocked by 3% H2O2. The sections were incubated with primary antibodies and DAB staining solution was used for signal generating. Sections were stained with hematoxylin, dehydrated and sealed. For immunofluorescence, the antigen was extracted by EDTA buffer (1 mm Tris/EDTA, pH 9.0). The endogenous fluorescence was eliminated by AutoFluo Quencher (Servicebio, Wuhan, Cat# G1221). The details of the immunofluorescence and immunohistochemistry antibodies are showed in Supplementary Table 2. Image Pro Plus (v.6.0) was used to score the staining of each marker. The integral optical density area ratio of each marker was calculated to evaluate the staining intensity.
Statistical Analysis
R language (v. 3.6.2), SPSS software (v. 22.0), and GraphPad Prism (v. 8.3.0) for Windows were used for statistical analyses and generating figures. Two-tailed Student’s t-test was used to separate the genes with differential expression according to TERT mutation. Correction of p-values was performed to control the false discovery rate (FDR) using R-values of FDR < 0.01, which were considered statistically significant. The multivariate Cox proportional hazard model was used to evaluate independent prognostic variables, and Kaplan–Meier curves were employed to depict survival distributions. Immune cells that correlated with the TERT mutation status were explored by a two-tailed Student’s t-test using SPSS, considering the effect of variant grades or IDH status.
Bioinformatics Analyses
The Database for Annotation, Visualization, and Integrated Discovery (DAVID v6.8)3, STRING (v10.5)4, and Kyoto Encyclopedia of Genes and Genomes (KEGG)5 were performed to gene annotation and pathway analyses. Gene set enrichment analysis (GSEA) was used to explore the biological functions of TERT mutations. To further define the immune cell subpopulations affected by TERT mutation, we used gene set variation analysis (GSVA) to explore the relationship between TERT and the predefined, highly distinctive, transcriptional profile of each immune cell type (Chtanova et al., 2005; Wendt et al., 2006; Hyrcza et al., 2007; Bindea et al., 2013). Eighteen types of immune cells with corresponding gene signatures were selected (Supplementary Table 3).
Results
TERT Mutation Tended to Occur Frequently in Glioblastoma and IDHwt Glioma, With a Trend for Poor Survival
To identify the distribution of TERT status, we analyzed the TERT mutation ratio by hierarchical analysis. First, we found that glioblastomas are more prone to TERT mutations than grade II and grade III gliomas (Figure 1A) as well as gliomas with IDHwt or 1p19q codeletion (Figures 1B,C). Furthermore, TERT mutant (TERTmut) gliomas were associated with shorter patient survival across glioblastoma and IDHwt subgroups, despite no significant difference from IDHwt subgroups (Figures 1D–G and Supplementary Table 4).
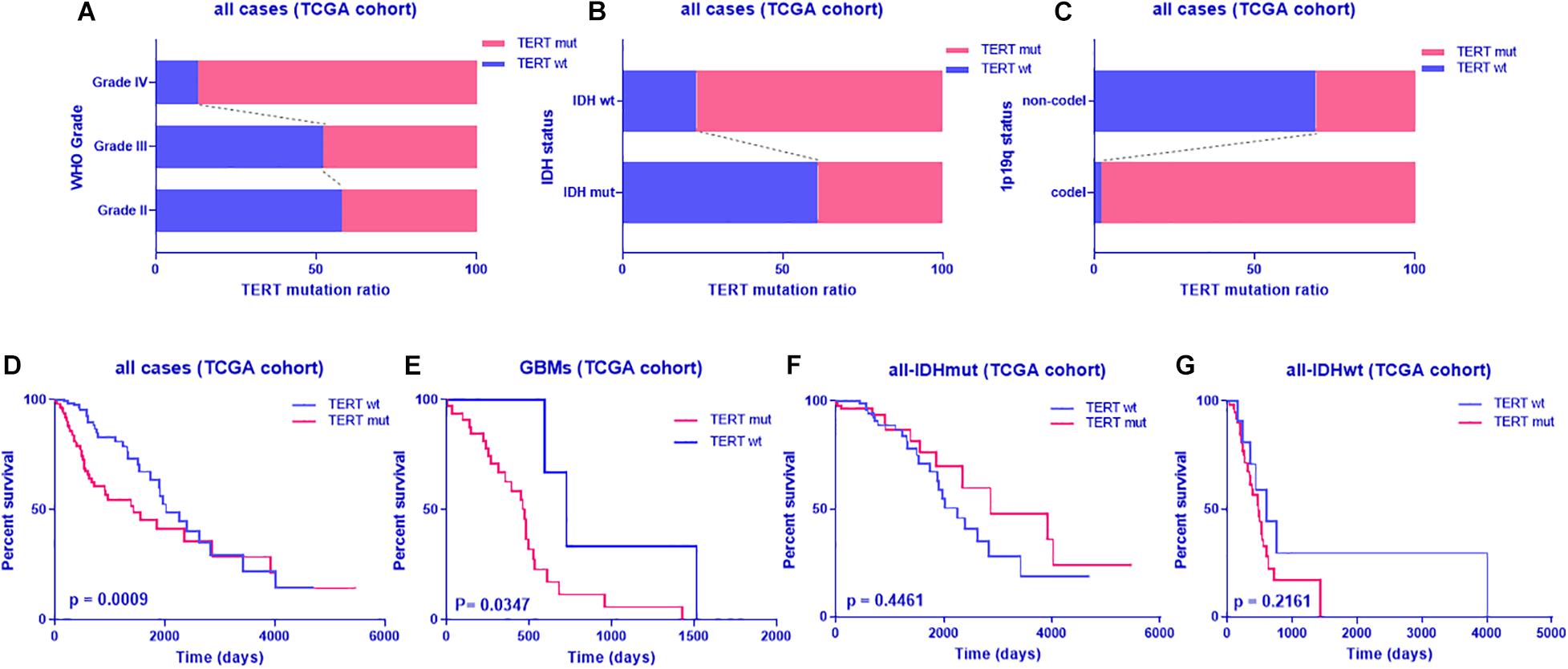
Figure 1. TERT mutation pattern and prognostic value in glioma from TCGA dataset. TERT mutation radio in glioma according to WHO grade (A), IDH status (B) or 1p-19q co-deletion (C); Kaplan-Meier analyses of overall survival according to TERT status (D, all cases; E, glioblastoma; F, all cases with IDH mutant; G, all cases with IDH wild type); discriminative power of TERT was assessed with Kaplan-Meier plotting method and the Log-rank test. Significant p < 0.05.
TERT Mutation Is Associated With Immune Response Promotion in IDH-wt Glioma
To better understand the mechanism of TERT mutation in IDHwt glioma, we determined the differentially expressed genes between TERT wild-type and mutation. Among the 20,497 genes analyzed, 3,265 genes were upregulated in TERTmut gliomas, and 1,682 genes showed increased expression in TERT wild-type ones (adjusted p < 0.01, Supplementary Table 5). Subsequent analyses showed that the upregulated genes in TERTmut gliomas were enriched in various immune-related pathways including cell division, interferon-gamma-mediated signaling pathway, NF-κB signaling pathway, positive regulation of NF-κB signaling, inflammatory response, innate immune response, immune response, and antigen processing and presentation (Figure 2A). Therefore, we considered that the TERT promoter status was associated with the immune response in glioma. Among the 822 immune genes, the expression levels of 361 genes were upregulated in TERTmut gliomas and 91 genes in TERT wild-type cells (t-test, p < 0.05, Supplementary Table 5). We then analyzed the TERT mutation-related immune genes using Gene Ontology (GO). GO analyses showed that multiple immune-related processes were enriched in TERTmut gliomas, including cytokine-cytokine receptor interaction, chemokine signaling pathway, graft-versus-host disease, intestinal immune network for IgA production, Jak-STAT signaling pathway, antigen processing and presentation, HTLV-I infection, NF-κB signaling pathway, natural killer cell-mediated cytotoxicity, and PI3K-Akt signaling pathway (Figure 2B), which verified our hypothesis that TERT promoter status is associated with immune response in glioma. We further validated this hypothesis using GSEA, which highlighted that TERTmut gliomas showed increased immune responses (Supplementary Figure 1).
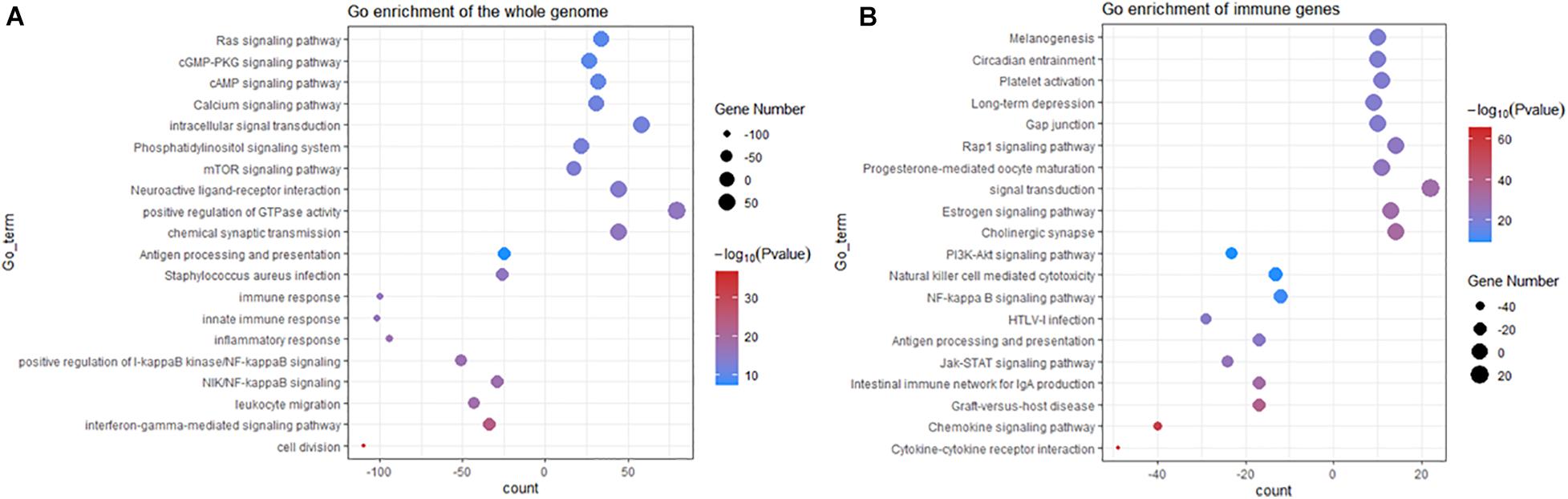
Figure 2. GO analyses of upregulated gene set enrichment according to TERT mutation in IDHwt gliomas (including KEGG). (A) GO analyses enrichment pathways for differentially expressed genes from the whole RNA-Seq genes among IDHwt gliomas. (B) GO pathway analyses for differentially expressed immune genes among IDHwt gliomas; Plot size shows gene counts on enrichment of pathway; Color depth shows the –log10p value from low level (blue) to high (red); significant p < 0.05.
TERT Mutation Is Accompanied by Increased Neutrophils in the Local IDH-wt Glioma Microenvironment
To further stratify the immune variation of TERT mutated tumors in glioma microenvironment, we analyzed the enrichment scores of 26 immune cell-characterized gene sets across TERT mutation status (Table S6). We found that TERTmut glioma contained infiltration of neutrophils (rTERTmut = 0.054 ± 0.361, rTERTwt = −0.203 ± 0.286, p < 0.01, TCGA cohort, t-test) in IDHwt glioma cases, whereas the numbers of microglia and central memory T-cells (Tcm) were low (Figure 3A). The same trend of neutrophils was observed in the CGGA cohort (Figure 3B, rTERTmut = 0.091 ± 0.364, rTERTwt = −0.044 ± 0.332, p < 0.05, CGGA cohort, t-test). However, macrophages were not correlated with TERT status.
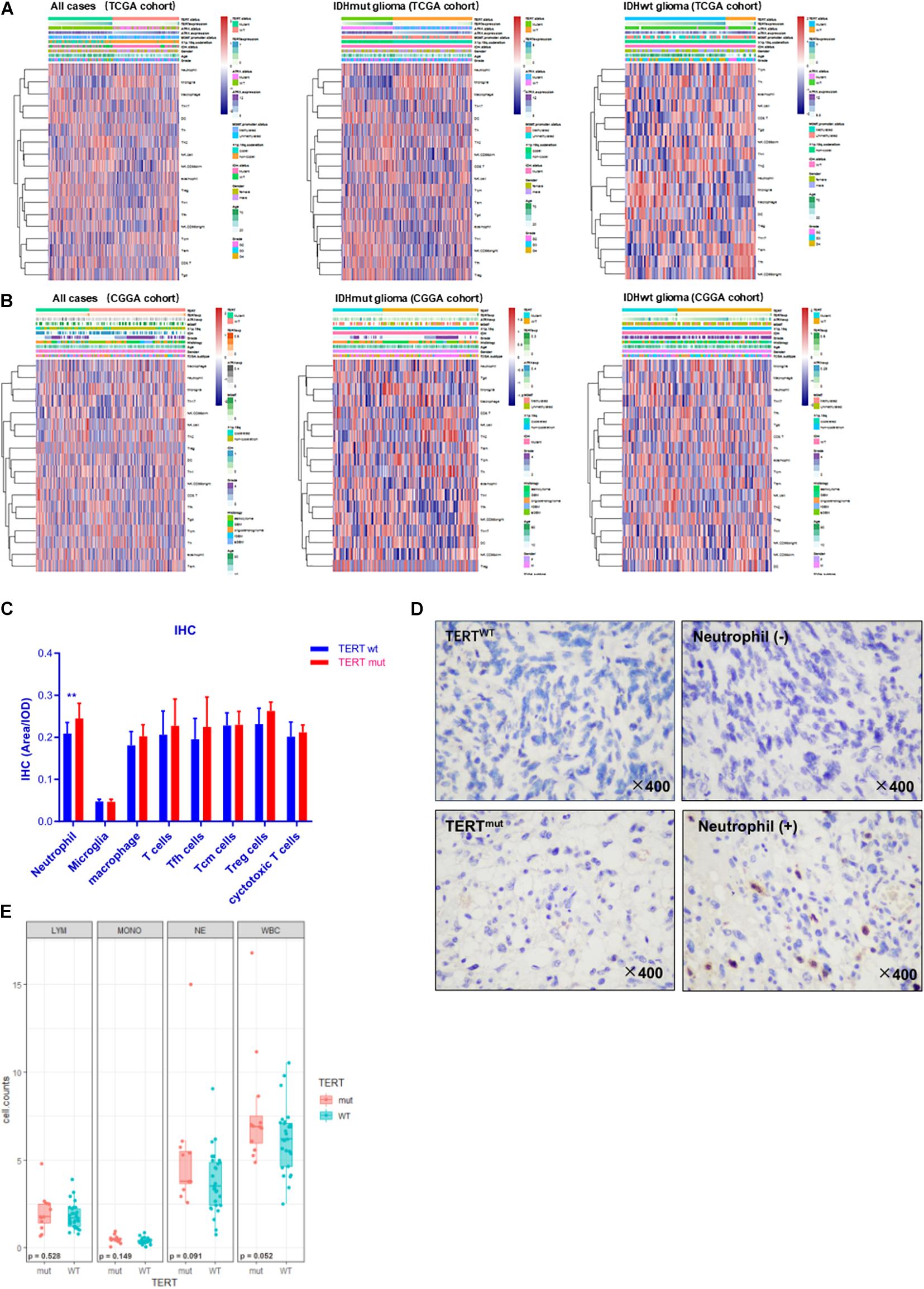
Figure 3. Immune cell enrichment in gliomas according to TERT status. Samples were divided into a TERT mutant (TERTmut) group and TERT wild-type (TERTwt) group. All cases, IDH mutant and IDH wild-type cases from TCGA cohort (A). The same subgroup gliomas from the Chinese Glioma Genome Atlas (CGGA) cohort (B). Immunostaining results of multiple immune cells according to TERT mutation. (C) Immunohistochemistry result among 49 IDHwt gliomas. (D) Immunohisto- chemical staining showed that neutrophils increased with TERT mutation in IDHwt gliomas, –, Lower than the mean value; +, higher than the mean value. (E) Immune cell counts of peripheral blood according to TERT mutation in IDHwt glioma; LYM, lymphocytes; MONO, monocytes; NE, neutrophils; WBC, total leukocytes; significant p < 0.05.
To confirm the above findings, we investigated tumoral infiltrated immune cell in 49 IDH-wt glioma tissue and interrogated their association with TERT mutation status (Figures 3C,D). In line with the bioinformatics findings, the profiles of infiltrated immune cells differed between TERTmut and TERT wild-type gliomas. More neutrophils were identified in TERTmut gliomas (p < 0.01).
We then measured the level of peripheral neutrophils in preoperative patients. We found insignificant difference in neutrophil counts between TERTmut and wild-type gliomas (Figure 3E). Therefore, we speculated that the neutrophil enrichment in the TERTmut glioma local microenvironment was due to cytokine-induced chemotaxis.
TERT-Mut Is Accompanied by Increased Neutrophils Relative to Chemokines in the Tumor
To investigate the relationship between TERT mutation and neutrophil enrichment in glioma, chemokine levels were compared between TERTmut glioma and TERT wild-type glioma (Supplementary Table 7). We found that the following neutrophil chemokines—MMP9, CCL2, CCL5, CXCR4, CXCR2, CCL23, and IL8 (p = 0.07)—were enriched in TERTmut gliomas (t-test, p < 0.05, Figure 4).
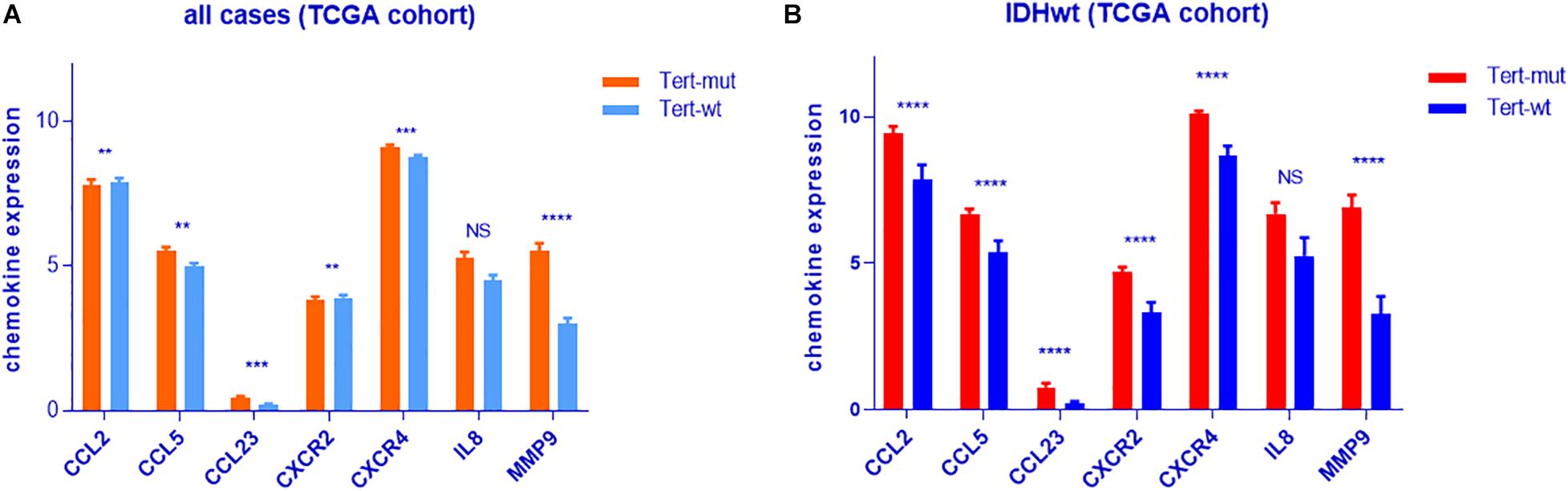
Figure 4. Analyses of neutrophil chemokine expression in IDHwt glioma according to TERT mutation. (A) All samples from TCGA cohort. (B) IDHwt samples from TCGA cohort. **p < 0.01; ***p < 0.001; ****p < 0.0001; NS, not significant.
Neutrophil Enrichment in Tumor Microenvironment Is Related With the Survival of Glioma Patients
To identify whether neutrophil infiltration accounts for poor survival of patients with TERTmut glioma, we analyzed the prognosis in cases based on neutrophil enrichment scores from the GSVA results. As expected, high neutrophil levels indicated poor survival in patients with glioma (Figure 5). This observation was in accordance with the findings in TERT mutation.
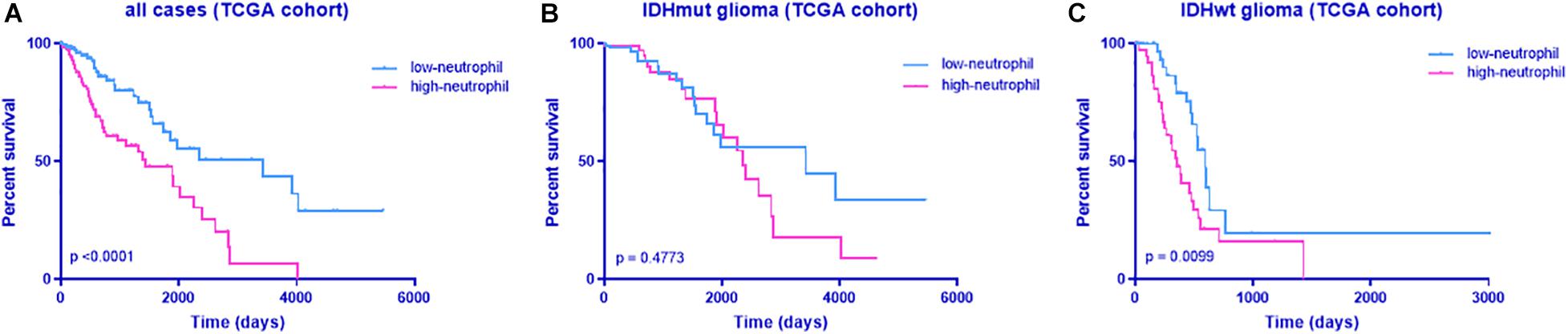
Figure 5. Kaplan–Meier analyses of overall survival according to the neutrophil enrichment score from GSVA. (A) All cases, (B) all cases with IDH mutant, and (C) all cases with IDH wild-type. The discriminative power of TERT was assessed with the Kaplan–Meier plotting method and the log-rank test. Significant p < 0.05.
Discussion
Inflammation promotes the proliferation, survival and metastasis of tumor cells, which is helpful to overturn adaptive immunity and enhance response to chemotherapy (Mantovani et al., 2008). The microenvironment of glioma is dominated by macrophages, which are thought to be transformed by glioma cells to promote tumor growth (Morantz et al., 1979; Poon et al., 2017). The poor survival of IDHwt gliomas may, at least partially, result from high levels of immune components infiltration (Yu et al., 2010). However, the underlying molecular mechanisms is still unclear. Therefore, more relevant studies are needed to provide deeper insights for improving precisive diagnosis and therapy for glioma (Roth et al., 1998; Rohn et al., 2001; Brown et al., 2017; Yang et al., 2017).
To this end, we proposed that the TERT mutation may play essential roles in mediating IDH-related immune response in tumor microenvironment (TME). We found that TERT mutation is associated with neutrophil enrichment, indicating that this mutation may closely associated with tumor immunity. Moreover, we identified a variety of specific chemokines infiltrated in TERTmut tumors, which might attribute to the chemotaxis of neutrophils in the microenvironment.
Tumor-associated neutrophils (TANs) release neutrophil elastase (ELA2), collagenase (MMP8) and gelatinase B (MMP9), which contain in their granules. These enzymes can promote the invasion of tumor cells by remodeling the extracellular matrix or directly acting on tumor cells (Dumitru et al., 2013; Bonavita et al., 2016). Neutrophils can even enhance the production of VEGF and the invasion of tumor cells by producing oncostatin M (Nozawa et al., 2006) and MMP9. On the other hand, neutrophils can inhibit tumor growth through antibody-dependent cytotoxicity (Stoppacciaro et al., 1993), which determines its important role in anti-cancer monoclonal antibody therapy (Brandsma et al., 2015). Interferon (IFN)-activated neutrophils can release TRAIL/APO2 ligand (tumor necrosis factor-related apoptosis-inducing ligand), which selectively induces apoptosis in tumor cells (Kemp et al., 2005; Cassatella et al., 2006). TANs may be classified into IFN-β-induced anti-tumoral neutrophils (N1), and TGF-β- induced tumor-promoting neutrophils (N2). N1 and N2 can be distinguished by various biological functions, such as expression of adhesion molecules, inflammatory mediators, chemokines, and chemokine receptors (Jablonska et al., 2010; Eruslanov et al., 2014).
These studies show that tumor-infiltrating neutrophils can be polarized. However, it is still unclear whether the functional differences of different neutrophils states is due to the regulation of cytokines in tumor microenvironment or the infiltration of different neutrophil subsets. CCRL2/CXCR2 is the main chemokine of neutrophils in vitro (Del Prete et al., 2017), which regulate both adaptive and innate immune responses (Del Prete et al., 2013). Blocking CXCR2 receptor can inhibit neutrophil infiltration into tumor, suppress tumor growth and reduce angiogenesis (Jablonska et al., 2014). In breast cancer, inhibition of CXCR2 increases the efficacy of chemotherapy (Acharyya et al., 2012). These data suggest that CXCR2 expressed by neutrophils is essential for their homing to tumors tissue with high expression of CXCR2 ligand. In tumors, CXCR4 is overexpressed in circulating neutrophil subsets, which promotes angiogenesis and tumor progression (Massena et al., 2015). Furthermore, CXCR4 may elevated in N2 neutrophils since it is inhibited by IFN-β (Jablonska et al., 2010). The expression of the CC chemokine receptors CCR1, CCR2, CCR3, and CCR5 is upregulated by neutrophils (Hartl et al., 2008). There have been emerging studies on the role of CCL2-CCR2 axis by neutrophil-monocyte cooperation biology (Hagerling et al., 2019; Hou et al., 2020). In tumor, neutrophils can be mobilized and recruited through CCL2–CCR2 axis (Pahler et al., 2008). However, whether CCL2 has positive or negative effects on tumor growth depends on it recruits pro-tumor or anti-tumor neutrophils/monocytes to the tumor (Mitchem and DeNardo, 2012; Mitchem et al., 2013; Lavender et al., 2017).
In this study, CCL2, CCL5, CXCR4, MMP9, and CXCR2 expression was found to be high in TERTmut glioma from all samples and IDHwt subgroups with high levels of neutrophil infiltration, which indicated that N2 phenotype neutrophils were associated with TERT mutation (Bonavita et al., 2016). In summary, overexpression of these chemokines may recruit specific neutrophils into the local tumor microenvironment and exhibiting anti-glioma effects.
The relationship between TERTmut and patient survival did not reach statistical significance in IDH subgroups. It could be result from the limited number of cases in each subgroup. Further studies are needed to determine the prognostic value of TERT mutations in these subgroups of patients. Further studies are needed to validate the relationship between Tert mutation and tumor associated neutrophil. Ex vivo profiling of the cell components in IDHwt glioma tissues would provide valuable information of tumor microenvironment. The correlation analysis can only provide preliminary evidence of the relationship rather than determine the causal relationship between Tert mutation and tumor associated neutrophil infiltration.
To conclude, this study suggest that TERT correlates with immune response and the infiltration of neutrophils in the IDH wild-type glioma microenvironment. Accordingly, TERT may serve as a potential therapeutic target. Further studies are warranted to confirm our findings and unveil the underlying mechanism.
Data Availability Statement
The original contributions presented in the study are included in the article/Supplementary Material, further inquiries can be directed to the corresponding author/s.
Ethics Statement
This study was approved by the Ethics Committee of The First Hospital of China Medical University. Written informed consent was obtained from all patients.
Author Contributions
MG performed the study and drafted the manuscript. YL performed the study and revised the manuscript. YL and SZ supervised the study and revised the manuscript. All authors have read and approved the final manuscript.
Funding
This work was supported by the National Natural Science Foundation of China (No. 81671240 to SZ) and the Liaoning Science and Technology Plan Project (No. 2014021068 to YL).
Conflict of Interest
The authors declare that the research was conducted in the absence of any commercial or financial relationships that could be construed as a potential conflict of interest.
Acknowledgments
Publicly available data from TCGA and CGGA were utilized in this study, and we would like to thank the authors for making their data available.
Supplementary Material
The Supplementary Material for this article can be found online at: https://www.frontiersin.org/articles/10.3389/fcell.2021.654407/full#supplementary-material
Supplementary Figure 1 | GSEA analyses of upregulated gene set enrichment in IDHwt glioma according to TERT mutation. (A) GO gene set enrichment in TERTmut glioma. (B) GO gene set enrichment in TERTwt glioma. (C) Immunologic gene set enrichment in TERTmut glioma. (D) Immunologic gene set enrichment in TERTwt glioma. Blue column with upper scale, NES; gray plot with lower scale, −log10 p-value; p < 0.05.
Footnotes
- ^ http://cancergenome.nih.gov
- ^ http://www.cgga.org.cn
- ^ http://david.abcc.n-cifcrf.gov
- ^ https://string-db.org/
- ^ http://www.kegg.jp/kegg/pathway.html
References
Acharyya, S., Oskarsson, T., Vanharanta, S., Malladi, S., Kim, J., Morris, P. G., et al. (2012). A CXCL1 paracrine network links cancer chemoresistance and metastasis. Cell 150, 165–178. doi: 10.1016/j.cell.2012.04.042
Bindea, G., Mlecnik, B., Tosolini, M., Kirilovsky, A., Waldner, M., Obenauf, A. C., et al. (2013). Spatiotemporal dynamics of intratumoral immune cells reveal the immune landscape in human cancer. Immunity 39, 782–795. doi: 10.1016/j.immuni.2013.10.003
Blackburn, E. H., Epel, E. S., and Lin, J. (2015). Human telomere biology: a contributory and interactive factor in aging, disease risks, and protection. Science 350, 1193–1198. doi: 10.1126/science.aab3389
Bonavita, O., Massara, M., and Bonecchi, R. (2016). Chemokine regulation of neutrophil function in tumors. Cytokine Growth Factor Rev. 30, 81–86. doi: 10.1016/j.cytogfr.2016.03.012
Brandsma, A. M., Ten Broeke, T., Nederend, M., Meulenbroek, L. A., van Tetering, G., Meyer, S., et al. (2015). Simultaneous targeting of FcgammaRs and FcalphaRI enhances tumor cell killing. Cancer Immunol. Res. 3, 1316–1324. doi: 10.1158/2326-6066.CIR-15-0099-T
Brown, M. C., Holl, E. K., Boczkowski, D., Dobrikova, E., Mosaheb, M., Chandramohan, V., et al. (2017). Cancer immunotherapy with recombinant poliovirus induces IFN-dominant activation of dendritic cells and tumor antigen-specific CTLs. Sci. Transl. Med. 9:408. doi: 10.1126/scitranslmed.aan4220
Cassatella, M. A., Huber, V., Calzetti, F., Margotto, D., Tamassia, N., Peri, G., et al. (2006). Interferon-activated neutrophils store a TNF-related apoptosis-inducing ligand (TRAIL/Apo-2 ligand) intracellular pool that is readily mobilizable following exposure to proinflammatory mediators. J. Leukoc Biol. 79, 123–132. doi: 10.1189/jlb.0805431
Chakrabarti, M., Banik, N. L., and Ray, S. K. (2013). Sequential hTERT knockdown and apigenin treatment inhibited invasion and proliferation and induced apoptosis in human malignant neuroblastoma SK-N-DZ and SK-N-BE2 cells. J. Mol. Neurosci. 51, 187–198. doi: 10.1007/s12031-013-9975-x
Chtanova, T., Newton, R., Liu, S. M., Weininger, L., Young, T. R., Silva, D. G., et al. (2005). Identification of T cell-restricted genes, and signatures for different T cell responses, using a comprehensive collection of microarray datasets. J. Immunol. 175, 7837–7847.
Del Prete, A., Bonecchi, R., Vecchi, A., Mantovani, A., and Sozzani, S. (2013). CCRL2, a fringe member of the atypical chemoattractant receptor family. Eur. J. Immunol. 43, 1418–1422. doi: 10.1002/eji.201243179
Del Prete, A., Martinez-Munoz, L., Mazzon, C., Toffali, L., Sozio, F., Za, L., et al. (2017). The atypical receptor CCRL2 is required for CXCR2-dependent neutrophil recruitment and tissue damage. Blood 130, 1223–1234. doi: 10.1182/blood-2017-04-777680
Duan, X. F., and Zhao, Q. (2018). TERT-mediated and ATRX-mediated Telomere Maintenance and Neuroblastoma. J. Pediatr. Hematol. Oncol. 40, 1–6. doi: 10.1097/MPH.0000000000000840
Dumitru, C. A., Lang, S., and Brandau, S. (2013). Modulation of neutrophil granulocytes in the tumor microenvironment: mechanisms and consequences for tumor progression. Semin. Cancer Biol. 23, 141–148. doi: 10.1016/j.semcancer.2013.02.005
Eruslanov, E. B., Bhojnagarwala, P. S., Quatromoni, J. G., Stephen, T. L., Ranganathan, A., Deshpande, C., et al. (2014). Tumor-associated neutrophils stimulate T cell responses in early-stage human lung cancer. J. Clin. Invest. 124, 5466–5480. doi: 10.1172/JCI77053
Gabler, L., Lotsch, D., Kirchhofer, D., van Schoonhoven, S., Schmidt, H. M., Mayr, L., et al. (2019). TERT expression is susceptible to BRAF and ETS-factor inhibition in BRAF(V600E)/TERT promoter double-mutated glioma. Acta Neuropathol. Commun. 7:128. doi: 10.1186/s40478-019-0775-6
Gao, M., Lin, Y., Liu, X., Li, Y., Zhang, C., Wang, Z., et al. (2019). ISG20 promotes local tumor immunity and contributes to poor survival in human glioma. Oncoimmunology 8:e1534038. doi: 10.1080/2162402X.2018.1534038
Gojo, J., Lotsch, D., Spiegl-Kreinecker, S., Pajtler, K. W., Neumayer, K., Korbel, P., et al. (2017). Telomerase activation in posterior fossa group A ependymomas is associated with dismal prognosis and chromosome 1q gain. Neuro. Oncol. 19, 1183–1194. doi: 10.1093/neuonc/nox027
Hagerling, C., Gonzalez, H., Salari, K., Wang, C. Y., Lin, C., Robles, I., et al. (2019). Immune effector monocyte-neutrophil cooperation induced by the primary tumor prevents metastatic progression of breast cancer. Proc. Natl. Acad. Sci. U.S.A. 116, 21704–21714. doi: 10.1073/pnas.1907660116
Hartl, D., Krauss-Etschmann, S., Koller, B., Hordijk, P. L., Kuijpers, T. W., Hoffmann, F., et al. (2008). Infiltrated neutrophils acquire novel chemokine receptor expression and chemokine responsiveness in chronic inflammatory lung diseases. J. Immunol. 181, 8053–8067.
Hewedi, I. H., Radwan, N. A., Shash, L. S., and Elserry, T. H. (2013). Perspectives on the immunologic microenvironment of astrocytomas. Cancer Manag. Res. 5, 293–299. doi: 10.2147/CMAR.S48942
Hou, P., Kapoor, A., Zhang, Q., Li, J., Wu, C. J., Li, J., et al. (2020). Tumor microenvironment remodeling enables bypass of oncogenic KRAS dependency in pancreatic cancer. Cancer Discov. 10, 1058–1077. doi: 10.1158/2159-8290.CD-19-0597
Hyrcza, M. D., Kovacs, C., Loutfy, M., Halpenny, R., Heisler, L., Yang, S., et al. (2007). Distinct transcriptional profiles in ex vivo CD4+ and CD8+ T cells are established early in human immunodeficiency virus type 1 infection and are characterized by a chronic interferon response as well as extensive transcriptional changes in CD8+ T cells. J. Virol. 81, 3477–3486. doi: 10.1128/JVI.01552-06
Jablonska, J., Leschner, S., Westphal, K., Lienenklaus, S., and Weiss, S. (2010). Neutrophils responsive to endogenous IFN-beta regulate tumor angiogenesis and growth in a mouse tumor model. J. Clin. Invest. 120, 1151–1164. doi: 10.1172/JCI37223
Jablonska, J., Wu, C. F., Andzinski, L., Leschner, S., and Weiss, S. (2014). CXCR2-mediated tumor-associated neutrophil recruitment is regulated by IFN-beta. Int. J. Cancer 134, 1346–1358. doi: 10.1002/ijc.28551
Kemp, T. J., Ludwig, A. T., Earel, J. K., Moore, J. M., Vanoosten, R. L., Moses, B., et al. (2005). Neutrophil stimulation with Mycobacterium bovis bacillus Calmette-Guerin (BCG) results in the release of functional soluble TRAIL/Apo-2L. Blood 106, 3474–3482. doi: 10.1182/blood-2005-03-1327
Lavender, N., Yang, J., Chen, S. C., Sai, J., Johnson, C. A., Owens, P., et al. (2017). The Yin/Yan of CCL2: a minor role in neutrophil anti-tumor activity in vitro but a major role on the outgrowth of metastatic breast cancer lesions in the lung in vivo. BMC Cancer 17:88. doi: 10.1186/s12885-017-3074-2
Lotsch, D., Ghanim, B., Laaber, M., Wurm, G., Weis, S., Lenz, S., et al. (2013). Prognostic significance of telomerase-associated parameters in glioblastoma: effect of patient age. Neuro. Oncol. 15, 423–432. doi: 10.1093/neuonc/nos329
Mackay, A., Burford, A., Molinari, V., Jones, D. T. W., Izquierdo, E., Brouwer-Visser, J., et al. (2018). Pathological, radiological, and immune profiling of non-brainstem pediatric high-grade glioma from the HERBY Phase II randomized trial. Cancer Cell 33, 829–842.e5. doi: 10.1016/j.ccell.2018.04.004
Mantovani, A., Allavena, P., Sica, A., and Balkwill, F. (2008). Cancer-related inflammation. Nature 454, 436–444. doi: 10.1038/nature07205
Martinez, P., and Blasco, M. A. (2011). Telomeric and extra-telomeric roles for telomerase and the telomere-binding proteins. Nat. Rev. Cancer 11, 161–176. doi: 10.1038/nrc3025
Massena, S., Christoffersson, G., Vagesjo, E., Seignez, C., Gustafsson, K., Binet, F., et al. (2015). Identification and characterization of VEGF-A-responsive neutrophils expressing CD49d, VEGFR1, and CXCR4 in mice and humans. Blood 126, 2016–2026. doi: 10.1182/blood-2015-03-631572
Matsumura, N., Nakajima, N., Yamazaki, T., Nagano, T., Kagoshima, K., Nobusawa, S., et al. (2017). Concurrent TERT promoter and BRAF V600E mutation in epithelioid glioblastoma and concomitant low-grade astrocytoma. Neuropathology 37, 58–63. doi: 10.1111/neup.12318
Mistry, M., Zhukova, N., Merico, D., Rakopoulos, P., Krishnatry, R., Shago, M., et al. (2015). BRAF mutation and CDKN2A deletion define a clinically distinct subgroup of childhood secondary high-grade glioma. J. Clin. Oncol. 33, 1015–1022. doi: 10.1200/JCO.2014.58.3922
Mitchem, J. B., and DeNardo, D. G. (2012). Battle over CCL2 for control of the metastatic niche: neutrophils versus monocytes. Breast Cancer Res. 14:315. doi: 10.1186/bcr3149
Mitchem, J. B., Brennan, D. J., Knolhoff, B. L., Belt, B. A., Zhu, Y., Sanford, D. E., et al. (2013). Targeting tumor-infiltrating macrophages decreases tumor-initiating cells, relieves immunosuppression, and improves chemotherapeutic responses. Cancer Res. 73, 1128–1141. doi: 10.1158/0008-5472.CAN-12-2731
Morantz, R. A., Wood, G. W., Foster, M., Clark, M., and Gollahon, K. (1979). Macrophages in experimental and human brain tumors. Part 2: studies of the macrophage content of human brain tumors. J. Neurosurg. 50, 305–311. doi: 10.3171/jns.1979.50.3.0305
Nozawa, H., Chiu, C., and Hanahan, D. (2006). Infiltrating neutrophils mediate the initial angiogenic switch in a mouse model of multistage carcinogenesis. Proc. Natl. Acad. Sci. U.S.A. 103, 12493–12498. doi: 10.1073/pnas.0601807103
Ohali, A., Avigad, S., Ash, S., Goshen, Y., Luria, D., Feinmesser, M., et al. (2006). Telomere length is a prognostic factor in neuroblastoma. Cancer 107, 1391–1399. doi: 10.1002/cncr.22132
Pahler, J. C., Tazzyman, S., Erez, N., Chen, Y. Y., Murdoch, C., Nozawa, H., et al. (2008). Plasticity in tumor-promoting inflammation: impairment of macrophage recruitment evokes a compensatory neutrophil response. Neoplasia 10, 329–340. doi: 10.1593/neo.07871
Phillips, J. J., Gong, H., Chen, K., Joseph, N. M., van Ziffle, J., Bastian, B. C., et al. (2019). The genetic landscape of anaplastic pleomorphic xanthoastrocytoma. Brain Pathol. 29, 85–96. doi: 10.1111/bpa.12639
Poon, C. C., Sarkar, S., Yong, V. W., and Kelly, J. J. P. (2017). Glioblastoma-associated microglia and macrophages: targets for therapies to improve prognosis. Brain 140, 1548–1560. doi: 10.1093/brain/aww355
Rohn, T. A., Wagenknecht, B., Roth, W., Naumann, U., Gulbins, E., Krammer, P. H., et al. (2001). CCNU-dependent potentiation of TRAIL/Apo2L-induced apoptosis in human glioma cells is p53-independent but may involve enhanced cytochrome c release. Oncogene 20, 4128–4137. doi: 10.1038/sj.onc.1204534
Roth, W., Wagenknecht, B., Dichgans, J., and Weller, M. (1998). Interferon-alpha enhances CD95L-induced apoptosis of human malignant glioma cells. J. Neuroimmunol. 87, 121–129.
Stoppacciaro, A., Melani, C., Parenza, M., Mastracchio, A., Bassi, C., Baroni, C., et al. (1993). Regression of an established tumor genetically modified to release granulocyte colony-stimulating factor requires granulocyte-T cell cooperation and T cell-produced interferon gamma. J. Exp. Med. 178, 151–161.
Weller, M., Wick, W., Aldape, K., Brada, M., Berger, M., Pfister, S. M., et al. (2015). Glioma. Nat. Rev. Dis. Primers 1:15017. doi: 10.1038/nrdp.2015.17
Wendt, K., Wilk, E., Buyny, S., Buer, J., Schmidt, R. E., and Jacobs, R. (2006). Gene and protein characteristics reflect functional diversity of CD56dim and CD56bright NK cells. J. Leukoc Biol. 80, 1529–1541. doi: 10.1189/jlb.0306191
Yang, J., Liu, R., Deng, Y., Qian, J., Lu, Z., Wang, Y., et al. (2017). MiR-15a/16 deficiency enhances anti-tumor immunity of glioma-infiltrating CD8+ T cells through targeting mTOR. Int. J. Cancer 141, 2082–2092. doi: 10.1002/ijc.30912
Keywords: TERT mutation, glioma, IDH mutation, neutrophil, chemokines, RNA-seq
Citation: Gao M, Lin Y, Liu X, Zhao Z, Zhu Z, Zhang H, Ban Y, Bie Y, He X, Sun X and Zhang S (2021) TERT Mutation Is Accompanied by Neutrophil Infiltration and Contributes to Poor Survival in Isocitrate Dehydrogenase Wild-Type Glioma. Front. Cell Dev. Biol. 9:654407. doi: 10.3389/fcell.2021.654407
Received: 16 January 2021; Accepted: 22 March 2021;
Published: 30 April 2021.
Edited by:
Guohui Lu, The First Affiliated Hospital of Nanchang University, ChinaReviewed by:
Dipongkor Saha, Texas Tech University Health Sciences Center, Abilene, United StatesLihua Xu, Massachusetts General Hospital and Harvard Medical School, United States
Copyright © 2021 Gao, Lin, Liu, Zhao, Zhu, Zhang, Ban, Bie, He, Sun and Zhang. This is an open-access article distributed under the terms of the Creative Commons Attribution License (CC BY). The use, distribution or reproduction in other forums is permitted, provided the original author(s) and the copyright owner(s) are credited and that the original publication in this journal is cited, in accordance with accepted academic practice. No use, distribution or reproduction is permitted which does not comply with these terms.
*Correspondence: Yi Lin, linyi@cmu.edu.cn; Shizhong Zhang, zhangshizhong@smu.edu.cn