- Immuno-Oncology Program, Ares Pharmaceuticals, LLC, St. Paul, MN, United States
The repurposing of FDA-approved Bruton’s tyrosine kinase (BTK) inhibitors as therapeutic agents for solid tumors may offer renewed hope for chemotherapy-resistant cancer patients. Here we review the emerging evidence regarding the clinical potential of BTK inhibitors in solid tumor therapy. The use of BTK inhibitors may through lead optimization and translational research lead to the development of new and effective combination regimens for metastatic and/or therapy-refractory solid tumor patients.
Bruton’s Tyrosine Kinase as a Validated Molecular Target in Cancer Cells
Bruton’s tyrosine kinase (BTK) is linked to multiple signal-transduction pathways and networks, regulating survival, activation, proliferation, and differentiation of B-lineage lymphoid cells (Vassilev et al., 1999; Mahajan et al., 2001; Uckun and Sudbeck, 2001; Uckun et al., 2002, 2004, 2007b; Uckun and Malaviya, 2004; Uckun and Qazi, 2010; Burger, 2019). BTK is expressed in neoplastic cells from patients with B-lineage lymphoid malignancies (Vassilev et al., 1999; Mahajan et al., 2001; Uckun and Sudbeck, 2001; Uckun et al., 2002, 2004, 2007b; Uckun and Malaviya, 2004; Uckun and Qazi, 2010; Bond et al., 2019; Burger, 2019; Kim, 2019). The anti-apoptotic BTK-PI3K-AKT signaling pathway is critical for the survival of tumor cells (Figure 1). Multiple antiapoptotic signaling molecules and pathways linked to NF-κB, PI3-K/AKT, and STAT5 are regulated by BTK. Consequently, BTK has emerged as a new molecular target for treatment of B-lineage leukemias and lymphomas as well as —more recently—solid tumors. BTK inhibitors (BTKi) have replaced several chemotherapy-based regimens in standard of care for some of the B-lineage lymphoid malignancies, especially in patients with CLL and mantle cell lymphoma (MCL) (D’Cruz and Uckun, 2013; Thompson and Burger, 2018; Bond et al., 2019; Jurczak et al., 2019; Kim, 2019). The first-generation BTKi ibrutinib binds covalently to a cysteine residue (Cys481) in the active site of the ATP-binding domain of BTK. Second-generation BTKi were designed to have fewer off-target effects than ibrutinib (D’Cruz and Uckun, 2013). The second-generation BTKi acalabrutinib also binds Cys481 in the BTK active site, and it is FDA approved for the treatment of adults with CLL or SLL (Feng et al., 2019). Another second-generation BTKi, zanubrutinib, received an accelerated approval from the FDA for the treatment of adult patients with MCL (Feng et al., 2019). A novel oncogenic isoform of BTK with a survival-promoting function is abundantly expressed in breast cancer, ovarian cancer, prostate cancer, and colorectal cancer (Eifert et al., 2013; Guo et al., 2014; Kokabee et al., 2015; Wang et al., 2016; Conconi et al., 2017; Molina-Cerrillo et al., 2017; Campbell et al., 2018; Chen et al., 2018; Lavitrano et al., 2019). Overexpression of BTK in solid tumor cells was associated with elevated expression of genes with functions related to cell adhesion, cytoskeletal structure, and extracellular matrix as well as aggressiveness of the cancer (Guo et al., 2014). Knockdown of these isoforms by RNA interference using siRNA or treatment with BTKi like ibrutinib resulted in inhibition of growth as well as apoptosis and enhanced chemosensitivity of cancer cells (Eifert et al., 2013; Guo et al., 2014; Kokabee et al., 2015; Wang et al., 2016; Molina-Cerrillo et al., 2017; Campbell et al., 2018; Chen et al., 2018; Lavitrano et al., 2019). Grassilli et al. (2016) reported that this 65-kDa novel isoform of BTK is expressed in colorectal cancer cells in a mitogen-activated protein kinase (MAPK)-dependent manner. Furthermore, BTKi ibrutinib inhibited the proliferation of human colorectal cancer cell lines in vitro (Grassilli et al., 2016) and enhanced the chemosensitivity of drug-resistant colorectal cancer cells (Ianzano et al., 2016). Inhibition of BTK also reduced the clonogenicity of cancer stem cells and decreased their resistance to chemotherapy drugs (Metzler et al., 2020). BTKi were shown to synergize with the standard chemotherapy drug 5-fluorouracil against chemotherapy-resistant colorectal cancer cells (Lavitrano et al., 2019). First-generation BTKi LFM-A13 caused apoptosis in human colorectal cancer cells and exhibited potent anticancer activity against xenografted human colorectal cancer cells in mice both as a single agent and in combination with erythropoietin (Tankiewicz-Kwedlo et al.,2018a,b). p65BTK was also detected in non-small cell lung cancer (NSCLC) cell lines, including those with mutant KRAS, and treatment of these cell lines with BTKi resulted in loss of viability and inhibition of clonogenic growth (Giordano et al., 2019). Furthermore, BTKi enhanced the sensitivity of NSCLC cell lines to standard chemotherapy drugs (Giordano et al., 2019). Wei et al. (2016) reported that human glioblastoma (GBM) cells express p77BTK, and downregulation of BTK expression inhibits the antiapoptotic AKT/mTOR pathway, and BTKi ibrutinib exhibits in vivo antitumor activity in a mouse xenograft model of GBM. Recently, Sala et al. (2019) reported that p65BTK is expressed in patient-derived human glioma cells, and BTKi diminish their viability.
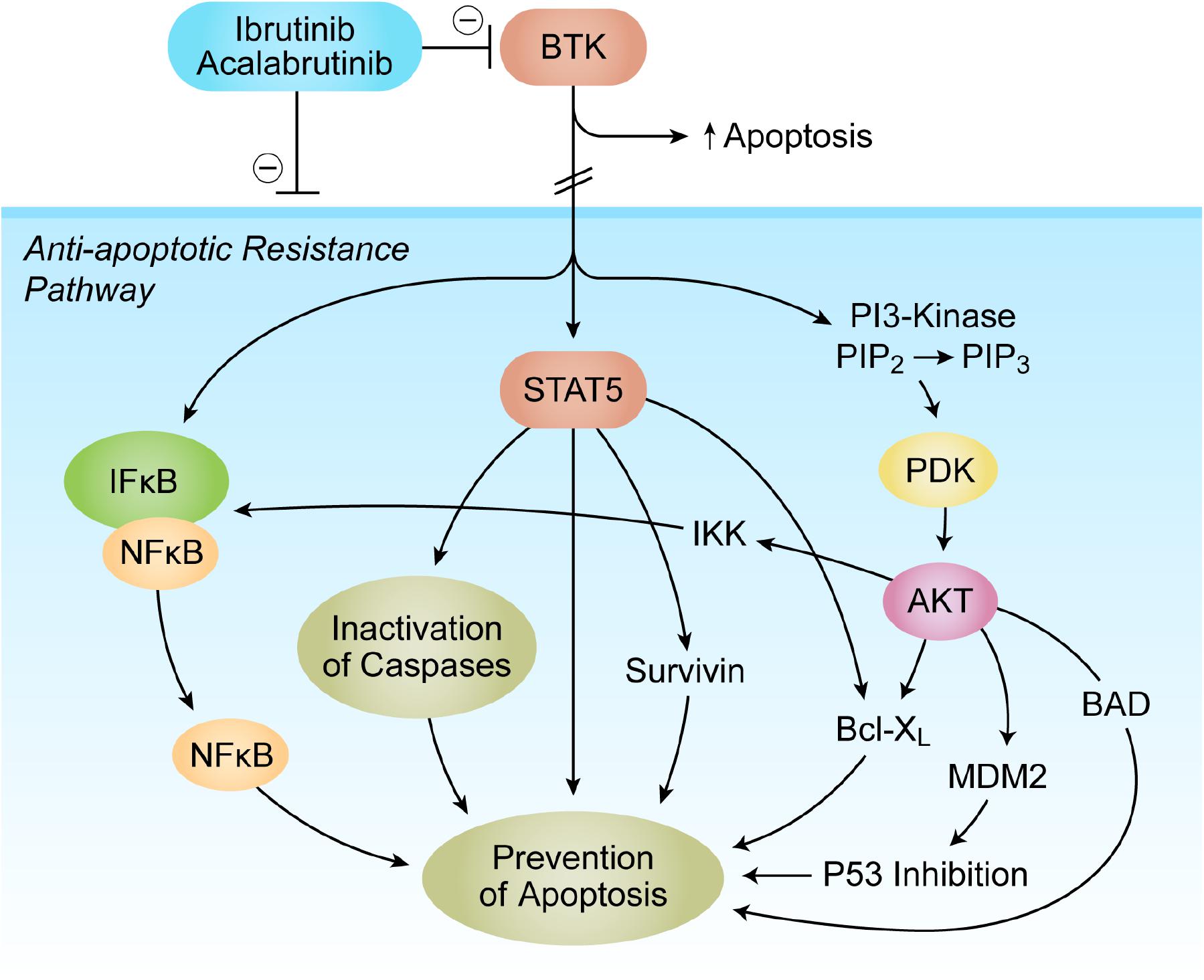
Figure 1. Bruton’s tyrosine kinase (BTK) as a Master Regulator of Apoptosis in tumor microenvironment (TME). The anti-apoptotic BTK-PI3K-AKT signaling pathway is critical for the survival of tumor cells. Multiple antiapoptotic signaling molecules and pathways linked to NF-κB, PI3-K/AKT, and STAT5 are regulated by BTK. See text for a detailed discussion.
Both BTK and the related TEC kinases ETK and BMX are abundantly expressed in prostate cancer cells, and knockdown of BTK expression in prostate cancer cells results in reduced proliferative activity (Guo et al., 2014; Kokabee et al., 2015; Chen et al., 2018). Inhibition of BTK and ETK with a small molecule inhibitor caused inhibition of proliferation, clonogenic growth, invasiveness of human prostate cancer cell lines both in in vitro and an in vivo SCID mouse xenograft model (Guo et al., 2014). BTK inhibition was also associated with substantial downregulation of oncogenic genes, such as MYC, in prostate cancer cell lines and enhances their chemosensitivity to standard drugs such as docetaxel (Guo et al., 2014). Likewise, ovarian cancer cells express BTK, and high expression levels are correlated with aggressiveness of disease, progression to Stage IV metastatic cancer, and poor survival (Zucha et al., 2015).
Similarly, numerous studies have shown that BTK inhibition causes substantial cytotoxicity to HER2+ breast cancer cells, inhibits their proliferation and clonogenicity, and diminishes their resistance to chemotherapy both in vitro and in vivo (Eifert et al., 2013; Chen et al., 2016; Wang et al., 2016; Metzler et al., 2020; Wen et al., 2020). The results obtained with non-specific BTKi like ibrutinib should be interpreted with due caution because several other kinases, including ERBB2/HER-2 that have ibrutinib-binding cysteine residues in their kinase domains are inhibited by ibrutinib (Berglof et al., 2015). Nonetheless, LFM-A13, a first-generation BTKi with no HER-2 or EGF-R inhibitory activity, also exhibited antitumor activity in the MMTV/neu transgenic mouse model of HER2-positive breast cancer. It was at least as effective as the standard breast cancer drugs paclitaxel and gemcitabine, and it improved the efficacy of paclitaxel (Uckun, 2007; Uckun et al., 2007a). In the DMBA breast cancer model, the BTKi LFM-A13 significantly delayed spontaneous tumor appearance as well as tumor progression, and it substantially improved tumor-free survival (Güven et al., 2020). The tumors developing despite chemoprevention with LFM-A13 were small and grew slowly. Hence, BTK inhibition prevented the development of aggressive and rapidly progressive mammary gland tumors.
Bruton’s tyrosine kinase inhibition is also associated with inhibition of tumor growth in pancreas cancer (Massó-Vallés et al., 2015; Gunderson et al., 2016). In view of the broad-spectrum anti-cancer activity exerted by BTKi in various non-clinical cancer models, BTK inhibition with ibrutinib and acalabrutinib has been evaluated in several proof-of-concept solid tumor trials (e.g., NCT02403271, NCT03525925, NCT03379428, NCT02599824, and NCT02562898) aimed at assessing its potential clinical benefit in patients with solid tumors, including ovarian cancer, breast cancer, lung cancer, prostate cancer, and pancreas cancer (Massó-Vallés et al., 2016; Hong et al., 2019; Overman et al., 2020). The maturation of data from these trials will provide valuable insights regarding the clinical impact potential of BTK inhibition as part of multimodality treatment regimens for difficult-to-treat forms of cancer. The reported suppression of cancer stemness in non-clinical models awaits confirmation from clinical proof-of-concept studies (Pan et al., 2020).
Bruton’s Tyrosine Kinase and Tumor Microenvironment
Several cellular elements of the tumor microenvironment (TME) of solid tumor patients contribute to the immune evasion, proliferation, and drug resistance of tumor cells, including myeloid-derived suppressor cells (MDSCs), tumor-associated M2-like, “alternatively activated,” macrophages, and regulatory T cells (Tregs) (Figure 2). Notably, some solid tumors abundantly express IL-2 inducible T-cell kinase (ITK), a TEC kinase related to BTK (Figure 2). It has been reported that ITK inhibition by the existing BTKi can result in improved T-cell responses via reduced production of IL-10 and TGFβ that have immunosuppressive effects (Dubovsky et al., 2013; Sagiv-Barfi et al., 2015; Chen et al., 2016; Stiff et al., 2016). Furthermore, in a breast cancer mouse model, BTKi ibrutinib improved the efficacy of anti-PD-L1 treatment (Sagiv-Barfi et al., 2015). On the other hand, BTK inhibition may potentially reduce the potency of immune checkpoint inhibitors. That is because BTK expressing tumor infiltrating cells within the TME include memory B cells that cooperate with memory T-cells to ensure a robust immune response to cancer cells. A recent study in which >500 lung adenocarcinoma cases were analyzed for possible contribution of BTK to an immune-dominant profile of the TME revealed that BTK expression in the TME was associated with a less aggressive disease and an improved survival outcome (Bi et al., 2020). MDSCs in the TME have been shown to express BTK (Figure 2), and it has been proposed that BTK inhibition may therefore lift the MDSC-mediated suppression of the antitumor immunity within the TME (Stiff et al., 2016). The potential effects of BTK inhibition on the tumor microenvironment and the potency of immune-checkpoint inhibitors will be clarified in part by the ongoing clinical trials that combine BTK inhibition with immune checkpoint blockade.
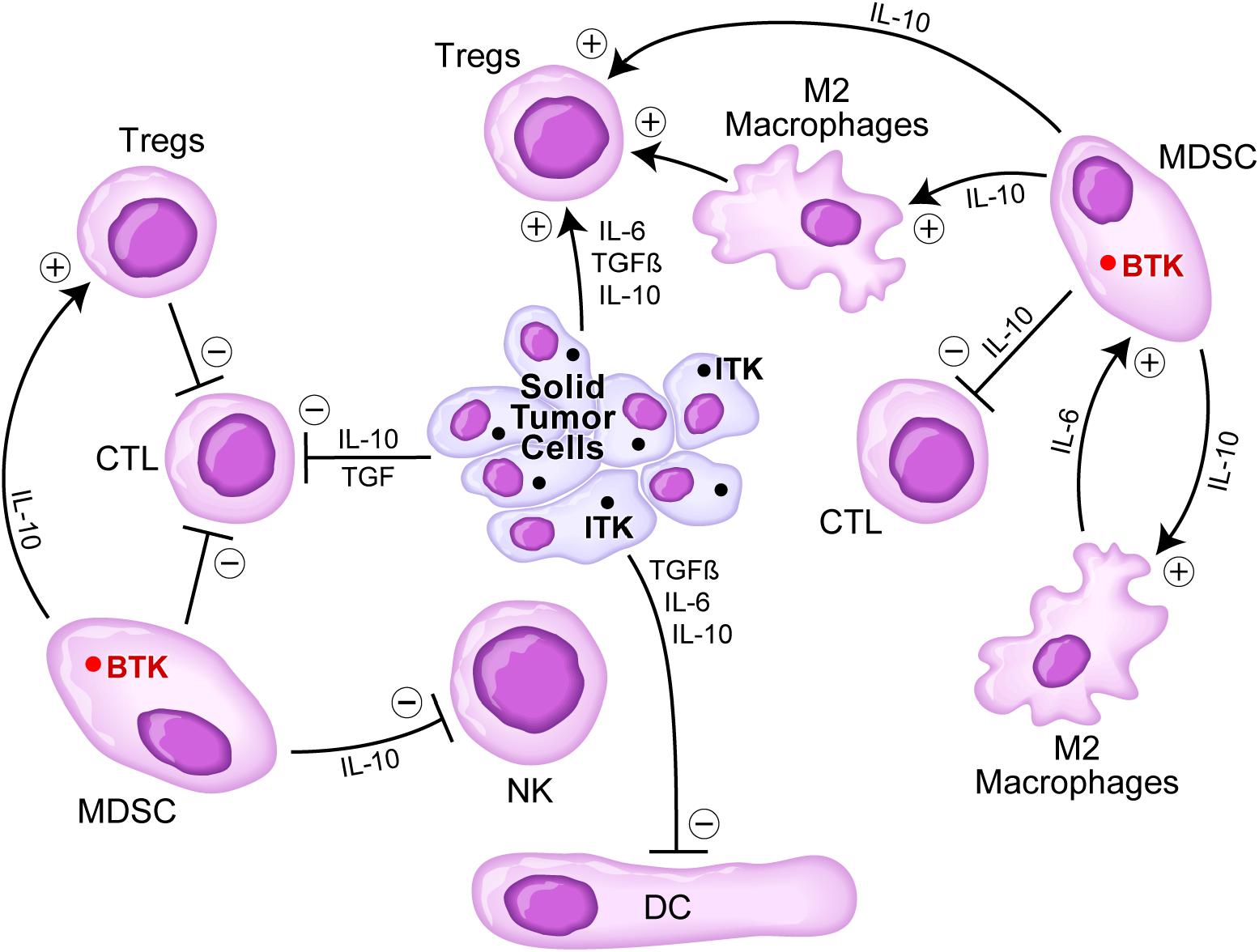
Figure 2. Immunosuppressive TME in solid tumors. Tumor cells secrete several cytokines including IL-6, TGFβ, and IL-10 that inhibit DCs, CTLs, but stimulate regulatory T cells (Tregs). Myeloid-derived suppressor cells (MDSCs) are stimulated via IL-6 by M2 macrophages and stimulate M2 macrophages as well as Tregs via IL-10, but they inhibit via IL10 CTLs and NK cells. Since solid tumor cells express the TEC kinase inducible T-cell kinase (ITK) related to BTK and MDSCs express BTK, BTK inhibition might offer an effective strategy to overcome an immunosuppressive TME. See text for a detailed discussion.
Coumarins as a New Class of Bruton’s Tyrosine Kinase Inhibitors
Coumarins are derivatives of 2H-1-benzopyran-2-one, which naturally occurs in plants as free coumarins or their glycoside derivatives (Kashman et al., 1992; Currens et al., 1996; McKee et al., 1998; Creagh et al., 2001; Shokoohinia et al., 2018; Bhatia and Rawal, 2019; Kawai et al., 2019; Li et al., 2019; Lin et al., 2019; Makowska et al., 2019; Ramdani et al., 2019; Selvaraj et al., 2019; Wang et al., 2019; Zhang and Xu, 2019). Coumarins have been known for their proapoptotic anticancer activity with inhibitory effects on tumor-promoting signal transduction pathways as well as antiviral activity (Kashman et al., 1992; Currens et al., 1996; McKee et al., 1998; Creagh et al., 2001; Shokoohinia et al., 2018; Bhatia and Rawal, 2019; Kawai et al., 2019; Li et al., 2019; Lin et al., 2019; Makowska et al., 2019; Ramdani et al., 2019; Selvaraj et al., 2019; Wang et al., 2019; Zhang and Xu, 2019). The naturally occurring coumarin derivatives, (+)-calanolide A and (–)-calanolide B, have been identified as inhibitors of non-nucleoside HIV-1-specific reverse-transcriptase inhibitory activity (Kashman et al., 1992; Currens et al., 1996; Creagh et al., 2001). In recent years, the coumarin scaffold has also been used in developing anticancer drugs. Several semi-synthetic calanolide derivatives have been developed as antiviral drug candidates (Creagh et al., 2001; Sagiv-Barfi et al., 2015; Chen et al., 2016). Researchers have hybridized coumarin moieties with other anticancer pharmacophores as a strategy of developing novel anticancer drugs (Flavin et al., 1996; Bhatia and Rawal, 2019; Kawai et al., 2019; Lin et al., 2019; Makowska et al., 2019; Ramdani et al., 2019; Selvaraj et al., 2019; Wang et al., 2019; Zhang and Xu, 2019). In addition, some natural coumarins such as Psoralidin (Li et al., 2019) and Osthol (Shokoohinia et al., 2018) have been reported to exhibit potent in vitro and in vivo anticancer activity. Coumarin–fatty acid conjugates as well as coumarin hybrids generated via coupling with isoxazole, thiazole, monastrol, chalcone, triazole, sulfonamide, triphenylethylene, benzimidazole, pyran, imidazole, stilbene, estrogen, or phenylsulfonylfuroxan exhibited promising pro-apoptotic anticancer activity (Bhatia and Rawal, 2019; Kawai et al., 2019; Makowska et al., 2019; Selvaraj et al., 2019; Zhang and Xu, 2019).
We discovered that the crystal structure of the BTK kinase domain reveals a distinct 7 Å × 7 Å rectangular binding pocket near the hinge region of the BTK kinase domain with Leu-460, Tyr-476, Arg-525, and Asp-539 residues occupying the corners of the rectangle (Uckun and Sudbeck, 2001; Uckun et al., 2002, 2004; Uckun and Malaviya, 2004). The overall geometry inside the active site near the hinge region was estimated to be sufficient to accommodate the rationally designed BTK-inhibitory calanolide derivatives (Uckun and Sudbeck, 2001; Uckun et al., 2002, 2004; Uckun and Malaviya, 2004).
Conclusion
The repurposing of FDA-approved BTKi as therapeutic agents for solid tumors may offer renewed hope for chemotherapy-resistant cancer patients. Advanced prostate cancer has a dismal outcome, and patients with metastatic disease are in urgent need for therapeutic innovations (Litwin and Tan, 2017; Siegel et al., 2019). Androgen deprivation by both chemical and surgical castration is initially useful in the treatment of metastatic prostate cancer, but patients ultimately enter the castration-resistant stage (CRPC) where there is no effective treatment (Litwin and Tan, 2017; Siegel et al., 2019). Likewise, advanced and metastatic breast cancer patients, especially those with triple-negative breast cancer (TNBC) are in urgent need for therapeutic innovations (Bergin and Loi, 2019; Pandy et al., 2019; Thill et al., 2019). The discovery of effective treatment strategies using chemotherapy drugs, precision medicines, biologics, and natural compounds is a major area of translational research emphasis in contemporary oncology, especially for breast cancer and prostate cancer. Re-purposed BTKi currently approved for B-lineage lymphoid malignancies as well as new BTKi with enhanced potency against solid tumors may provide the basis for more effective combination regimens.
MDSCs in the TME have been shown to express BTK, and it has been proposed that BTK inhibition may therefore lift the MDSC-mediated suppression of the antitumor immunity within the TME (Stiff et al., 2016). However, BTK inhibition may potentially reduce the potency of immune checkpoint inhibitors because of disruption of cognate interactions between BTK expressing memory B-cells and memory T-cells. Whether or not BTK inhibition will result in a clinically meaningful inhibition of MDSC and/or help overcome resistance to ICI awaits clinical proof of concept. A recent randomized study in metastatic urothelial cancer patients that evaluated a combination of the ICI pembrolizumab with acalabrutinib failed to show any benefit from this combination vs. pembrolizumab alone (Zhang et al., 2020). On the other hand, a promising efficacy signal was obtained during the interim analysis of a randomized study (Clinicaltrials.gov identifier: NCT02599324) employing ibrutinib plus paclitaxel in patients with metastatic urothelial carcinoma (Castellano et al., 2019). Likewise, a combination of ibrutinib with the anti-EGF receptor antibody cetuximab showed moderate activity in patients with metastatic colorectal cancer (Oh et al., 2020). The identification of the most effective and best-tolerated combination regimens will likely require rationally designed clinical studies with multiple treatment cohorts enrolling in parallel and adaptive trial designs.
Author Contributions
FU and TV have made significant and substantive contributions to the study, reviewed and revised the manuscript, and provided final approval for submission of the final version. FU conceived the study, designed the evaluations reported in this manuscript, directed the data compilation and analysis, analyzed relevant data, and prepared the initial draft of the manuscript. Both authors contributed to the article and approved the submitted version.
Funding
This study received funding from the Ares Pharmaceuticals, LLC. The funder had the following involvement with the study: It provided the salaries of the authors. The funder was not involved in the study design, collection, analysis, interpretation of data, and writing of this article or decision to submit it for publication.
Conflict of Interest
FU was an employee of Ares Pharmaceuticals, LLC. TV was a consultant of Ares Pharmaceuticals, LLC.
References
Bergin, A. R. T., and Loi, S. (2019). Triple-negative breast cancer: recent treatment advances. F1000Res 8:F1000.FacultyRev–1342.
Berglof, A., Hamasy, A., Meinke, S., Palma, M., Krstic’, M., Månsson, A., et al. (2015). Targets for Ibrutinib Beyond B Cell Malignancies. Scand. J. Immunol. 82, 208–217. doi: 10.1111/sji.12333
Bhatia, R., and Rawal, R. K. (2019). Coumarin Hybrids: promising scaffolds in the treatment of breast cancer. Mini Rev. Med. Chem. 19, 1443–1458. doi: 10.2174/1389557519666190308122509
Bi, K. W., Wei, X. G., Qin, X. X., and Li, B. (2020). BTK Has potential to be a prognostic factor for lung adenocarcinoma and an indicator for tumor microenvironment remodeling: a study based on TCGA Data Mining. Front. Oncol. 10:424. doi: 10.3389/fonc.2020.00424
Bond, D. A., Alinari, L., and Maddocks, K. (2019). Bruton tyrosine kinase inhibitors for the treatment of mantle cell lymphoma: review of current evidence and future directions. Clin. Adv. Hematol. Oncol. 17, 223–233.
Burger, J. A. (2019). Bruton tyrosine kinase inhibitors: present and future. Cancer J. 25, 386–393. doi: 10.1097/PPO.0000000000000412
Campbell, R., Chong, G., and Hawkes, E. A. (2018). Novel Indications for Bruton’s tyrosine kinase inhibitors, beyond hematological malignancies. J. Clin. Med. 7:62. doi: 10.3390/jcm7040062
Castellano, D. E., Morales-Barrera, R., IDuran, I., Keam, B., Joo Chung, I., Arkenau, T., et al. (2019). Interim analysis of ibrutinib plus paclitaxel for patients with metastatic urothelial carcinoma previously treated with platinum-based chemotherapy. J. Clin. Oncol. 37:(7_suppl), 365–365. doi: 10.1200/JCO.2019.37.7_suppl.365
Chen, J., Kinoshita, T., Sukbuntherng, J., Chang, B. Y., and Elias, L. (2016). Ibrutinib inhibits ERBB receptor tyrosine kinases and HER2-amplified breast cancer cell growth. Mol. Cancer Ther. 15, 2835–2844. doi: 10.1158/1535-7163.mct-15-0923
Chen, S., Cai, C., Sowalsky, A. G., Ye, H., Ma, F., Yuan, X., et al. (2018). Mediated regulation of multiple tyrosine kinases contributes to castration resistance in prostate cancer. Cancer Res. 78, 5203–5215. doi: 10.1158/0008-5472.CAN-17-3615
Conconi, D., Fruscio, R., Cialdella, A., Romano, G., Decio, A., Damia, G., et al. (2017). “p65BTK is a novel therapeutic target in ovarian cancer,” in Proceedings of the 2nd Special Conference EACR AACR SIC, Florence, doi: 10.13140/RG.2.2.19764.96644
Creagh, T., Ruckle, J. L., Tolbert, D. T., Giltner, J., Eiznhamer, D. A., Dutta, B., et al. (2001). Safty and pharmacokinetics of single doses of (+)-calanolides a, a novel, naturally occurring nonnucleoside reverse transcriptase inhibitor, in healthy, human immunodeficiency virus-negative human subjects. Antimicrob Agents Chemother 45, 1379–1386. doi: 10.1128/aac.45.5.1379-1386.2001
Currens, M. J., Mariner, J. M., McMahon, J. B., and Boyd, M. R. (1996). Kinetic analysis of inhibition of human immunodeficiency virus type-1 reverse transcriptase by calanolide A. J. Pharmacol.. Exp. Ther. 279, 652–661.
D’Cruz, O. J., and Uckun, F. M. (2013). Novel Bruton’s tyrosine kinase inhibitors currently in development. Onco Targets Ther. 6, 161–176. doi: 10.2147/OTT.S33732
Dubovsky, J. A., Beckwith, K. A., Natarajan, G., Woyach, J. A., Jaglowski, S., Zhong, Y., et al. (2013). Ibru- tinib is an irreversible molecular inhibitor of ITK driving a Th1-selective pressure in T lymphocytes. Blood 122, 2539–2549. doi: 10.1182/blood-2013-06-507947
Eifert, C., Wang, X., Kokabee, L., Kourtidis, A., Jain, R., Gerdes, M. J., et al. (2013). A novel isoform of the B cell tyrosine kinase BTK protects breast cancer cells from apoptosis. Genes Chromosomes Cancer. 52, 961–975. doi: 10.1002/gcc.22091
Feng, Y., Duan, W., Cu, X., Liang, C., and Xin, M. (2019). Bruton’s tyrosine kinase (BTK) inhibitors in treating cancer: a patent review (2010-2018). Expert Opin. Ther. Pat. 29, 217–241. doi: 10.1080/13543776.2019.1594777
Flavin, M. T., Rizzo, J. D., Khilevich, A., Kucherenko, A., Sheinkman, A. K., Vilaychack, V., et al. (1996). Synthesis,chromatographic resolution, and anti-human immunodeficiency virus activity of (±)-Calanolide A and its enantiomers. J. Med. Chem. 39, 1303–1313. doi: 10.1021/jm950797i
Giordano, F., Vaira, V., Cortinovis, D., Bonomo, S., Goedmakers, J., Brena, F., et al. (2019). p65BTK is a novel potential actionable target in KRAS-mutated/EGFR-wild type lung adenocarcinoma. J. Exp. Clin. Cancer Res. 38:260. doi: 10.1186/s13046-019-1199-7
Grassilli, E., Pisano, F., Cialdella, A., Bonomo, S., Missaglia, C., Cerrito, M. G., et al. (2016). A novel oncogenic BTK isoform is overexpressed in colon cancers and required for RAS-mediated transformation. Oncogene 35, 4368–4378. doi: 10.1038/onc.2015.504
Gunderson, A. J., Kaneda, M. M., Tsujikawa, T., Nguyen, A. V., Affara, N. I., Ruffell, B., et al. (2016). Bruton tyrosine kinase-dependent immune cell cross-talk drives pancreas cancer. Cancer Dis- cov. 6, 270–285. doi: 10.1158/2159-8290.cd-15-0827
Guo, W., Liu, R., Bhardwaj, G., Yang, J. C., Changou, C., Ma, A. H., et al. (2014). Targeting Btk/Etk of prostate cancer cells by a novel dual inhibitor. Cell Death Dis. 5, e1409. doi: 10.1038/cddis.2014.343
Güven, D. C., Orhan, C., Şahin, K., and Uçkun, F. M. (2020). Data-Driven identification of chemopreventive agents for breast cancer. Turk. J. Med. Sci. 50, 1691–1696. doi: 10.3906/sag-2003-138
Hong, D., Rasco, D., Veeder, M., Luke, J. J., Chandler, J., Balmanoukian, A., et al. (2019). Phase 1b/2 study of the bruton tyrosine kinase inhibitor ibrutinib and the PD-L1 inhibitor durvalumab in patients with pretreated solid tumors. Oncology 97, 102–111. doi: 10.1159/000500571
Ianzano, L., Bonomo, S., Cialdella, A., Pisano, F., Cerrito, M. G., Missaglia, C., et al. (2016). p65BTK targeting restores the apoptotic response to chemotherapy of p53-null drug-resistant colon cancer cells. Eur. J. Cancer 69, S140. doi: 10.1016/s0959-8049(16)33016-7
Jurczak, W., Długosz-Danecka, M., and Wang, M. (2019). Acalabrutinib for adults with mantle cell lymphoma. Expert Rev. Clin. Pharmacol. 12, 179–187. doi: 10.1080/17512433.2019.1568868
Kashman, Y., Gustafson, K. R., Fuller, R. W., Cardellina, J. H. II, McMahon, J. B., Currens, M. J., et al. (1992). The calanolides, a novel HIV-inhibitory class of coumarin derivatives from the tropical rainforest tree. Calophyllum Lanigerum. J. Med. Chem. 35, 2735–2743. doi: 10.1021/jm00093a004
Kawai, J., Toki, T., Ota, M., Inoue, H., Takata, Y., Asahi, T., et al. (2019). Discovery of a potent. selective, and orally available MTHFD2 inhibitor (DS18561882) with in vivo antitumor activity. J. Med. Chem. 62, 10204–10220. doi: 10.1021/acs.jmedchem.9b01113
Kim, H. O. (2019). Development of BTK inhibitors for the treatment of B-cell malignancies. Arch. Pharm. Res. 42, 171–181. doi: 10.1007/s12272-019-01124-1
Kokabee, L., Wang, X., Sevinsky, C. J., Wang, W. L., Cheu, L., Chittur, S. V., et al. (2015). Bruton’s tyrosine kinase is a potential therapeutic target in prostate cancer. Cancer Biol. Ther. 16, 1604–1615. doi: 10.1080/15384047.2015.1078023
Lavitrano, M., Ianzano, L., Bonomo, S., Cialdella, A., Cerrito, M. G., Pisano, F., et al. (2019). BTK inhibitors synergize with 5-FU to treat drug-resistant TP53-null colon cancers. J. Pathol. 250, 134–147. doi: 10.1002/path.5347
Li, J., Fu, Y., Hu, X., and Xiong, Y. (2019). Psoralidin inhibits the proliferation of human liver cancer cells by triggering cell cycle arrest, apoptosis and autophagy and inhibits tumor growth in vivo. J. Buon. 24, 1950–1955.
Lin, M. H., Wang, J. S., Hsieh, Y. C., Zheng, J. H., and Cho, E. C. (2019). NO2 functionalized coumarin derivatives suppress cancer progression and facilitate apoptotic cell death in KRAS mutant colon cancer. Chem. Biol. Interact. 309:108708. doi: 10.1016/j.cbi.2019.06.021
Litwin, M. S., and Tan, H. J. (2017). The diagnosis and treatment of prostate cancer: A review. JAMA 317, 2532–2542.
Mahajan, S., Vassilev, A., Sun, N., Ozer, Z., Mao, C., and Uckun, F. M. (2001). Transcription factor STAT5A is a substrate of Bruton’s tyrosine kinase in B cells. J. Biol. Chem. 276, 31216–31228. doi: 10.1074/jbc.m104874200
Makowska, A., Wolff, L., Sączewski, F., Bednarski, P. J., and Kornicka, A. (2019). Synthesis and cytotoxic evaluation of benzoxazole/benzothiazole-2-imino-coumarin hybrids and their coumarin analogues as potential anticancer agents. Pharmazie 74, 648–657. doi: 10.1691/ph.2019.9664
Massó-Vallés, D., Jauset, T., Serrano, E., Sodir, N. M., Pedersen, K., Affara, N. I., et al. (2015). Ibrutinib exerts potent antifibrotic and antitumor ac- tivities in mouse models of pancreatic adeno- carcinoma. Cancer Res. 75, 1675–1681. doi: 10.1158/0008-5472.can-14-2852
Massó-Vallés, D., Jauset, T., and Soucek, L. (2016). Ibrutinib repurposing: from b-cell malignancies to solid tumors. Oncoscience 3, 147–148. doi: 10.18632/oncoscience.310
McKee, T. C., Covington, C. D., Fuller, R. W., Bokesch, H. R., Young, S., Cardellina, I. I. J. H., et al. (1998). Pyranocoumarins from tropical species of the genus calophyllum: a chemotaxonomic study of extracts in the national cancer institute collection. J. Nat. Prod. 61, 1252–1256. doi: 10.1021/np980140a
Metzler, J. M., Burla, L., Fink, D., and Imesch, P. (2020). Ibrutinib in gynecological malignancies and breast cancer: a systematic review. Int. J. Mol. Sci. 21:4154. doi: 10.3390/ijms21114154
Molina-Cerrillo, J., Alonso-Gordoa, T., Gajate, P., and Grande, E. (2017). Bruton’s tyrosine kinase (BTK) as a promising target in solid tumors. Cancer Treat Rev. 58, 41–50. doi: 10.1016/j.ctrv.2017.06.001
Oh, D.-Y., Arkenau, T., Lee, K.-W., Hilger-Rolfe, J., Cole, G., Kim, S. Y., et al. (2020). Phase Ib/II study of ibrutinib (ibr) in combination with cetuximab (cetux) in patients (pts) with previously treated metastatic colorectal cancer (mCRC). Ann. Oncol. 31, S428. doi: 10.1016/j.annonc.2020.08.550
Overman, M., Javle, M., Davis, R. E., Vats, P., Kumar-Sinha, C., Xiao, L., et al. (2020). Randomized phase II study of the Bruton tyrosine kinase inhibitor \acalabrutinib, alone or with pembrolizumab in patients with advanced pancreatic cancer. J Immunother Cancer. 8, e000587. doi: 10.1136/jitc-2020-000587
Pan, Y., Chiu, Y. H., Chiu, S. C., Cho, D. Y., Lee, L. M., Wen, Y. C., et al. (2020). Inhibition of Bruton’s tyrosine kinase suppresses cancer stemness and promotes carboplatin-induced cytotoxicity against bladder cancer cells. Anticancer Res. 40, 6093–6099. doi: 10.21873/anticanres.14630
Pandy, J. G. P., Balolong-Garcia, J. C., Cruz-Ordinario, M. V. B., and Que, F. V. F. (2019). Triple negative breast cancer and platinum-based systemic treatment: a meta-analysis and systematic review. BMC Cancer. 19:1065. doi: 10.1186/s12885-019-6253-5
Ramdani, L. H., Talhi, O., Decombat, C., Vermerie, M., Berry, A., Silva, A., et al. (2019). Bis(4-hydroxy-2H-chromen-2-one) Coumarin Induces Apoptosis in MCF-7 human breast cancer cells through aromatase inhibition. Anticancer Res. 39, 6107–6114. doi: 10.21873/anticanres.13818
Sagiv-Barfi, I., Kohrt, H. E. K., Czerwinski, D. K., Ng, P. P., Chang, B. Y., and Levy, R. (2015). Therapeutic antitumor immunity by checkpoint blockade is enhanced by ibrutinib, an inhibitor of both BTK and ITK. Proc. Natl. Acad. Sci. U.S.A. 112, E966–E972.
Sala, L., Cirillo, G., Riva, G., Romano, G., Giussani, C., Cialdella, A., et al. (2019). Specific expression of a new bruton tyrosine kinase isoform (p65BTK) in the glioblastoma gemistocytic histotype. Front. Mol. Neurosci. 12:2. doi: 10.3389/fnmol.2019.00002
Selvaraj, J., John, J. B. A., Joghee, N. M., Antony, J., Wadhwani, A., and Natarajan, J. (2019). Coumarin- Fatty acid conjugates as potential ERα/AKT-1 Antagonists for ER positive breast cancer. Anticancer Agents Med. Chem. 20, 437–449. doi: 10.2174/1871520619666191028104339
Shokoohinia, Y., Jafari, F., Mohammadi, Z., Bazvandi, L., Hosseinzadeh, L., Chow, N., et al. (2018). Potential anticancer properties of osthol: a comprehensive mechanistic review. Nutrients 10:36. doi: 10.3390/nu10010036
Siegel, R. L., Miller, K. D., and Jemal, A. (2019). Cancer statistics, 2019. CA Cancer J. Clin. 69, 7–34.
Stiff, A., Trikha, P., Wesolowski, R., Kendra, K., Hsu, V., Uppati, S., et al. (2016). Myeloid-derived suppressor cells express bruton’s tyrosine kinase and can be depleted in tumor-bearing hosts by ibrutinib treatment. Cancer Res. 76, 2125–2136. doi: 10.1158/0008-5472.can-15-1490
Tankiewicz-Kwedlo, A., Hermanowicz, J. M., Domaniewski, T., Pawlak, K., Rusak, M., Pryczynicz, A., et al. (2018a). Simultaneous use of erythropoietin and LFM-A13 as a new therapeutic approach for colorectal cancer. Br. J. Pharmacol. 175, 743–762. doi: 10.1111/bph.14099
Tankiewicz-Kwedlo, A., Hermanowicz, J. M., Pawlak, K., Czarnomysy, R., Bielawski, K., Prokop, I., et al. (2018b). Erythropoietin intensifies the proapoptotic activity of LFM-A13 in cells and in a mouse model of colorectal cancer. Int. J Mol. Sci. 19:1262. doi: 10.3390/ijms19041262
Thill, M., Jackisch, C., Janni, W., Müller, V., Albert, U. S., Bauerfeind, I., et al. (2019). AGO recommendations for the diagnosis and treatment of patients with locally advanced and metastatic breast cancer: update 2019. Breast Care (Basel) 14, 247–255. doi: 10.1159/000500999
Thompson, P. A., and Burger, J. A. (2018). Bruton’s tyrosine kinase inhibitors: first and second generation agents for patients with Chronic Lymphocytic Leukemia (CLL). Expert Opin. Investig. Drugs. 27, 31–42. doi: 10.1080/13543784.2018.1404027
Uckun, F. M. (2007). Chemosensitizing anti-cancer activity of LFM-A13, a leflunomide metabolite analog targeting polo-like kinases. Cell Cycle 6, 3021–3026. doi: 10.4161/cc.6.24.5096
Uckun, F. M., Dibirdik, I., Qazi, S., Vassilev, A., Ma, H., Mao, C., et al. (2007a). Anti-breast cancer activity of LFM-A13, a potent inhibitor of Polo-like kinase (PLK). Bioorg Med Chem. 15, 800–814. doi: 10.1016/j.bmc.2006.10.050
Uckun, F. M., and Malaviya, R. (2004). BTK Inhibitors and Methods for Their Identification and use. US Patent 6,753,348. Jun 22. Alexandria VA: US Patent.
Uckun, F. M., and Qazi, S. (2010). Bruton’s tyrosine kinase as a molecular target in treatment of leukemias and lymphomas as well as inflammatory disorders and autoimmunity. Expert Opin. Ther. Pat. 20, 1457–1470. doi: 10.1517/13543776.2010.517750
Uckun, F. M., and Sudbeck, E. (2001). Calanolides for Inhibiting BTK. US Patent No. 6,306,897 B1. Date of Patent: October 23. Mountain View, CA: Google.
Uckun, F. M., Tibbles, H. E., and Vassilev, A. O. (2007b). Bruton’s tyrosine kinase as a new therapeutic target. Anticancer Agents Med Chem. 7, 624–632. doi: 10.2174/187152007784111331
Uckun, F. M., Zheng, Y., and Ghosh, S. (2002). BTK Inhibitors and Methods for Their Identification and use. US Patent 6,365,626. Apr 2. Alexandria VA: US Patent.
Uckun, F. M., Zheng, Y., and Ghosh, S. (2004). BTK Inhibitors and Methods for Their Identification and use European Patent 1 071 658 B1. Jun 16. Munich: European Patent.
Vassilev, A., Ozer, Z., Navara, C., Mahajan, S., and Uckun, F. M. (1999). Bruton’s tyrosine kinase as an inhibitor of the Fas/CD95 death-inducing signaling complex. J. Biol. Chem. 274, 1646–1656. doi: 10.1074/jbc.274.3.1646
Wang, T., Peng, T., Wen, X., Wang, G., Sun, Y., Liu, S., et al. (2019). Design. synthesis and preliminary biological evaluation of benzylsulfone coumarin derivatives as anti-cancer agents. Molecules 24:4034. doi: 10.3390/molecules24224034
Wang, X., Wong, J., Sevinsky, C. J., Kokabee, L., Khan, F., Sun, Y., et al. (2016). Bruton’s tyrosine kinase inhibitors prevent therapeutic escape in breast cancer cells. Mol. Cancer Ther. 15, 2198–2208. doi: 10.1158/1535-7163.MCT-15-0813
Wei, L., Su, Y.-K., Lin, C.-M., Chao, T.-Y., Huang, S.-P., Huynh, T.-T., et al. (2016). Preclinical investigation of ibrutinib, a Bruton’s kinase tyrosine (Btk) inhibitor, in suppressing glioma tumorigenesis and stem cell phenotypes. Oncotarget 7, 69961–69975. doi: 10.18632/oncotarget.11572
Wen, T., Wang, J., Shi, Y., Qian, H., and Liu, P. (2020). Inhibitors targeting Bruton’s tyrosine kinase in cancers: drug development advances. Leukemia. 35, 312–332. doi: 10.1038/s41375-020-01072-6
Zhang, L., and Xu, Z. (2019). Coumarin-containing hybrids and their anticancer activities. Eur. J. Med. Chem. 181, 111587. doi: 10.1016/j.ejmech.2019.111587
Zhang, T., Harrison, M. R., O’Donnell, P. H., Alva, A. S., Hahn, N. M., Appleman, L. J., et al. (2020). A randomized phase 2 trial of pembrolizumab versus pembrolizumab and acalabrutinib in patients with platinum-resistant metastatic urothelial cancer. Cancer 126, 4485–4497. doi: 10.1002/cncr.33067
Keywords: BTK-Bruton’s tyrosine kinase, solid tumors, breast cancer, prostate cancer, metastasis
Citation: Uckun FM and Venkatachalam T (2021) Targeting Solid Tumors With BTK Inhibitors. Front. Cell Dev. Biol. 9:650414. doi: 10.3389/fcell.2021.650414
Received: 07 January 2021; Accepted: 08 March 2021;
Published: 14 April 2021.
Edited by:
Emanuela Grassilli, University of Milano-Bicocca, ItalyReviewed by:
Douglas Conklin, State University of New York, University at Albany, United StatesLuca Mologni, University of Milano-Bicocca, Italy
Copyright © 2021 Uckun and Venkatachalam. This is an open-access article distributed under the terms of the Creative Commons Attribution License (CC BY). The use, distribution or reproduction in other forums is permitted, provided the original author(s) and the copyright owner(s) are credited and that the original publication in this journal is cited, in accordance with accepted academic practice. No use, distribution or reproduction is permitted which does not comply with these terms.
*Correspondence: Fatih M. Uckun, ZmF0aWgudWNrdW5AYXJlc21pdC5jb20=