- Department of Neurosurgery, Hôpital Erasme, Université Libre de Bruxelles, Brussels, Belgium
Among the most biologically, thus clinically, aggressive primary brain tumors are found malignant gliomas. Despite recent advances in adjuvant therapies, which include targeted and immunotherapies, after surgery and radio/chemotherapy, the tumor is recurrent and always lethal. Malignant gliomas also contain a pool of initiating stem cells that are highly invasive and resistant to conventional treatment. Ion channels and transporters are markedly involved in cancer cell biology, including glioma cell biology. Transient receptor potential (TRP) ion channels are calcium-permeable channels implicated in Ca2+ changes in multiple cellular compartments by modulating the driving force for Ca2+ entry. Recent scientific reports have shown that these channels contribute to the increase in glioblastoma aggressiveness, with glioblastoma representing the ultimate level of glioma malignancy. The current review focuses on each type of TRP ion channel potentially involved in malignant glioma cell death, with the ultimate goal of identifying new therapeutic targets to clinically combat malignant gliomas. It thus appears that cannabidiol targeting the TRPV2 type could be such a potential target.
Introduction
Malignant Glioma Generality
Among the most common malignant primary tumors are encountered malignant gliomas, which are associated with dismal prognosis. Precise statistics from the United States report for example 17,000 new diagnoses in 2017 (Ostrom et al., 2017). These tumors are characterized by extensive proliferation, invasion, migration, angiogenesis, immunosuppression, and resistance to conventional treatment (Lefranc et al., 2018; Locarno et al., 2020). Malignant gliomas include grade II (gliomas), III (anaplastic gliomas), and IV (glioblastoma) tumors. The median survival of glioblastoma is only 16 months because of the high rate of tumor recurrence (>95%) (Lefranc et al., 2018), even under aggressive treatment, including large surgical resection followed by combined radio- and temozolomide chemotherapy and adjuvant chemotherapy with the same compound (Stupp et al., 2009). This high rate of tumor recurrence is linked to the dramatic infiltrative properties of glioma cells into the brain parenchyma, rendering therefore elusive curative surgical resection as well as conventional treatments using genotoxic radiotherapy and cytotoxic chemotherapy, and even antiangiogenic therapies (Wick et al., 2017). Targeted therapies and immunotherapies also failed in efficaciously combating malignant gliomas (McGranahan et al., 2019).
Heterogeneous populations of tumor-differentiated cells coexisting with subpopulations displaying stem cell properties are present in glioblastomas. The marked biological, thus clinical, aggressiveness of glioblastoma stem cells (GSCs) relates among others to their dramatic invasive nature into the brain parenchyma, high level of mobility into the brain parenchyma, and high resistance to both radio- and chemotherapy. GSCs have also the capacity to self-renew and are now known to be directly responsible for the recurrence and clinical relapse of glioblastomas (Colwell et al., 2017; Matarredona and Pastor, 2019).
All grade II gliomas have the tendency to transform into more aggressive grade III (anaplastic) or even grade IV gliomas (secondary glioblastoma); likewise, grade III gliomas can similarly transform into grade IV (secondary glioblastoma).
A glioblastoma is a mosaic of various cell populations associated with distinct dynamic cell states as recently revealed by genome-wide sequencing, and this dramatic cell heterogeneity within a given glioblastoma renders any type of treatment very difficult (Sottoriva et al., 2013; Patel et al., 2014; Bernstock et al., 2019).
Ion Channels Involved in Malignant Glioma Progression
Ion channels are classified by ion selectivity (sodium channels, potassium channels, chloride channels, proton channels, unselective channels, etc.), gating mechanism (voltage-gated, ligand-gated, cyclic nucleotide-gated, light-gated, and mechanosensitive), or localization (plasma membrane or intracellular) (Alexander et al., 2019). These channels display marked roles in a plethora of cellular processes and in cancer progression (Becchetti et al., 2013; Litan and Langhans, 2015; Prevarskaya et al., 2018).
Several ion channels are implicated in malignant glioma proliferation, migration, invasion, and cell death. For example, genome-wide analyses of glioblastoma revealed that, of 555 genes involved in potassium, sodium, chloride, calcium channels, and other ion transport, 55 mutations were detected, affecting 90% of the glioblastoma samples studied (Parsons et al., 2008).
It has been experimentally demonstrated already two decades ago that glioma cells invading the brain parenchyma must modify their shape and/or volume to perform their invasive journey (Soroceanu et al., 1999). Shape-volume changes in glioma cells are mediated, at least partly, by chloride currents, which, while affecting net salt fluxes across glioma cell membranes, induce water efflux, resulting in turn in glioma cell shrinkage facilitating their migration through minute extracellular spaces of the brain (Ransom et al., 2001; Habela et al., 2009).
In gliomas, cell condensation is a hallmark of intrinsic and extrinsic apoptosis and requires the concerted activation of chloride- and calcium-activated potassium channels, leading to the loss of water (Ernst et al., 2008). We previously reviewed the implications of the roles of potassium channels in glioma progression and migration, e.g., Kv1.3 and Kv1.5, Kv10.1, Kv11.1, KCa1.1, and KCa3.1 (Lefranc et al., 2012, 2018).
Morrone et al. (2016) reviewed the role of calcium channels in malignant brain tumor therapy.
The present review focuses on transient receptor potential (TRP) calcium channels, which modulate the driving force for Ca2+ entry from extra- into intracellular compartments (Figure 1). For each type of TRP ion channel described below, we focused our attention in identifying specific TRP channels involved in glioma cell death, rendering them as potential new therapeutic targets to combat general malignant gliomas, with a particular focus on glioblastoma (Table 1).
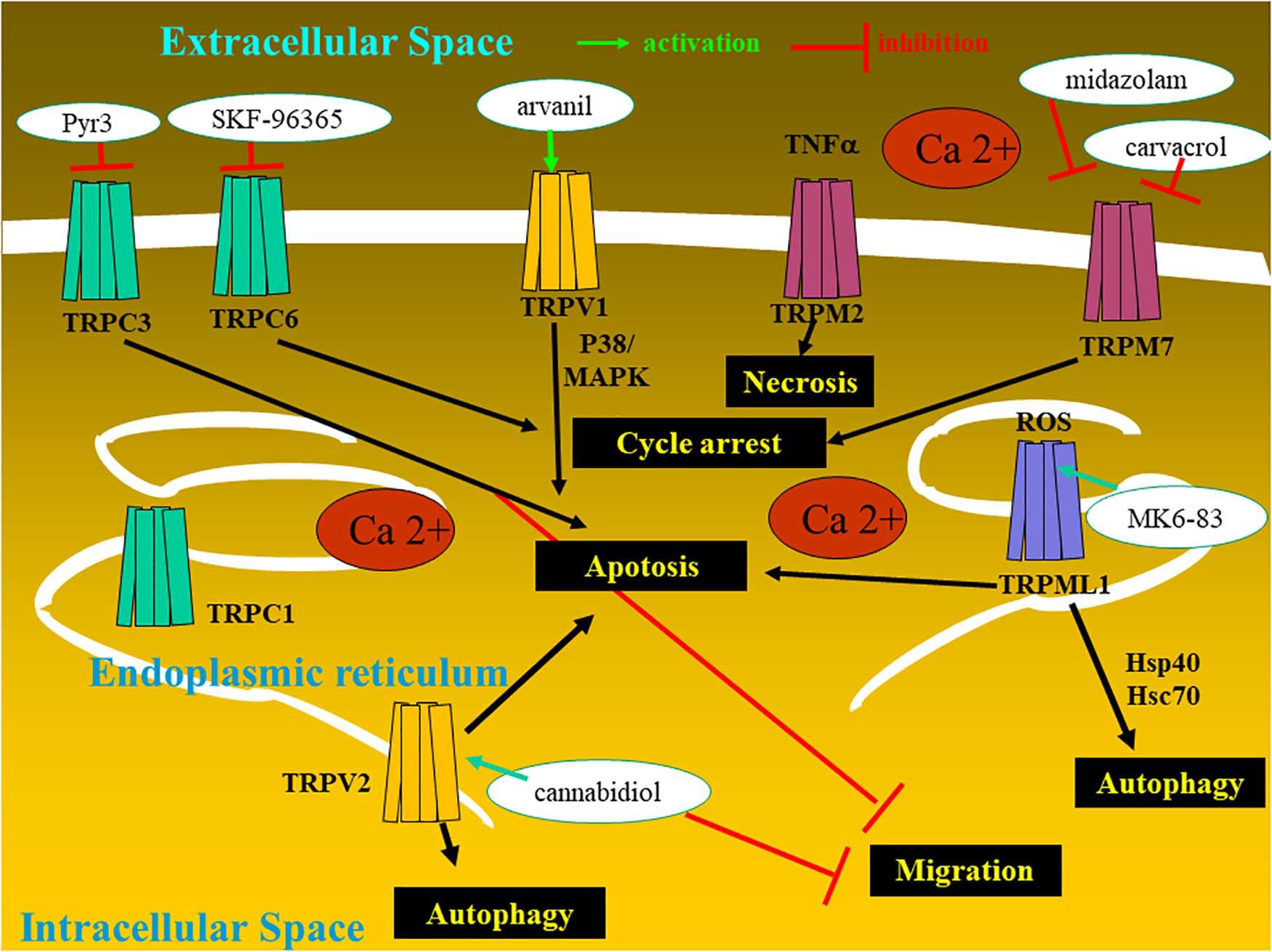
Figure 1. Glioma cell with the expression of transient receptor potential (TRP) ion channels and the therapeutic potentialities.
Malignant Glioma Cell Death
Glioma, melanoma, non-small cell lung cancer, and esophageal cancer, among others, are resistant to proapoptotic stimuli and are typically associated with dismal prognoses (Hanahan and Weinberg, 2011; Kornienko et al., 2013) and display therefore resistance to conventional cytotoxic pro-apoptotic drugs. Cytotoxic compounds that induce non-apoptotic cellular mechanisms, such as necrosis, senescence, autophagy, and mitotic catastrophe, are of great hopes to combat these cancer types displaying various levels of resistance to pro-apoptotic stimuli (Tang et al., 2019). The readers interested by precise definitions about the various cell death types should refer to the nomenclature established by Kroemer and colleagues (Galluzzi et al., 2012). The authors distinguished 13 distinct cell death types (Galluzzi et al., 2012), to which we have added methuosis, paraptosis, oncosis, and lysosomal membrane permeabilization (LMP) cell death types (Kornienko et al., 2013).
In the current review, the subchapter entitled therapeutic perspective aims to analyze the types of TRP-targeting drugs that could be of help in overcoming the resistance of glioma cancer cells and glioma stem cells to conventional therapies (Figure 2 and Table 1).
Modulating Transient Receptor Potential Channels for Inducing Cell Death in Malignant Glioma
Transient Receptor Potential Ion Channels
The mammalian TRP channel superfamily encompasses 28 identified members of Ca2+-permeable channels, with diverse physiological functions and cellular distributions (Ramsey et al., 2006; Venkatachalam and Montell, 2007; Nilius and Owsianik, 2011). TRP channels can be localized on the plasma membrane or in intracellular membranes and are involved in numerous fundamental cell functions (Nilius and Owsianik, 2011). Based on their structural homology and function, TRP channels are grouped into seven subfamilies in mammals: TRPC (canonical), TRPM (melastatin), TRPV (vanilloid), TRPP (polycystin), TRPA (ankyrin-like), TRPML (mucolipin), and TRPN (Drosophila NOMPC) (Montell et al., 2002; Li, 2017; Table 1). All TRP channels contain six transmembrane segments and a pore-forming loop between the 5th and 6th segments (Nilius and Owsianik, 2011). As TRPs are non-selective cation channels, their effects can be attributed to K+ and Na+ flux, but the role of Ca2+ is the most studied. TRP channels are non-selective Ca2+-permeable cation channels, with the exception of TRPM4 and TRPM5, which are Ca2+-impermeable. Some hallmarks of cancer pathophysiology are associated with the dysregulation of multiple downstream Ca2+-homeostasis-related effectors, a fact that explains why TRP channels are actually involved in the regulation versus dysregulation of growth, proliferation, migration, and invasion of cancer cells, including melanoma; prostate, breast, kidney, and bladder carcinomas; and gliomas (Bodding, 2007; Prevarskaya et al., 2007; Gkika and Prevarskaya, 2009; Chen et al., 2014; Bernardini et al., 2015; Santoni et al., 2020a,b; Yang and Kim, 2020).
Transient Receptor Potential Ion Channels in Malignant Glioma Cell Death
Among the TRP channels already identified in glioma cells with demonstrated regulatory effects on migration, proliferation, and apoptosis, let us cite TRPC1, TRPC3, TRPC6, TRPV1, TRPV2, TRPML 1 and 2, TRPM2, TRPM7, and TRPM8 (Alptekin et al., 2015; Morelli et al., 2016, 2019; Chang et al., 2018; Figure 1 and Table 1).
TRPC Channels
TRPCs relate to the canonical family, which includes seven members that assemble as homo- or heterotetramers (Putney, 2005; Schaefer, 2005). TRPC channels may be activated directly by diacylglycerol (Kress et al., 2008) or indirectly through calcium release from the endoplasmic reticulum following stimulation of the inositol triphosphate receptor (Sours-Brothers et al., 2009). We report below only those TRPC channels for which roles have been evidenced in glioma cell biology.
TRPC1
TRPC1 (subfamily C member 1) appears to be important for cytokinesis in cell proliferation and migration (Nesin and Tsiokas, 2014) and angiogenesis. TRPC1–lipid raft complexes are essential for certain stimulus-induced chemotaxis as it is for example the case with epidermal growth factors (Bomben et al., 2011).
Human malignant gliomas contain Ca2+-permeable TRPC1 channels as evidenced biophysically by Bomben and Sontheimer (2008), who also showed that multinucleated glioma cells resulting from incomplete cell division during their extensive proliferation result in the functional loss of TRPC1 channels regulating calcium signaling during cytokinesis (Bomben and Sontheimer, 2010). These authors also provided in vivo evidence that loss of TRPC1 function impairs tumor growth in immunocompromised mice, suggesting that pharmacological inhibition of these channels may slow tumor growth (Bomben and Sontheimer, 2010).
TRPC3
TRPC3 levels are associated with both diagnostic and prognostic values: high-grade gliomas have higher TRPC3 expression levels than normal brain tissues, and glioma patients with high TRPC3 expression have a shorter survival time than patients with a lower TRPC3 expression (Chang et al., 2018). Reduced proliferation was demonstrated in vitro in U87MG glioma cells with a reduced expression of TRPC3 (Chang et al., 2018). Chang et al. (2018) showed, accordingly, that glioblastoma cell proliferation was decreased by ethyl-1-(4-2,3,3-trichloroacrylamide)phenyl)-5-(trifluoromethyl)-1H-pyrazole-4-carboxylate (Pyr3), a selective TRPC3 channel blocker (Figure 2). This compound induced caspase-dependent apoptosis and mitochondrial membrane potential imbalance in two glioblastoma cell lines as well as inhibition of migration and invasion in vitro; in a xenograft animal model in vivo, this compound in combination with temozolomide inhibited glioblastoma tumor growth (Chang et al., 2018).
TRPC6
Not only is the TRPC6 channel overexpressed in human glioma cells at both the protein and mRNA levels, as compared to normal glial cells (Chigurupati et al., 2010), but also TRPC6 expression relates to the grade of glioma (Ding et al., 2010). Hypoxia increases Notch 1 activation, which in turn induces the expression of TRPC6 in primary samples and cell lines derived from glioblastoma (Chigurupati et al., 2010). Under hypoxia, TRPC6 channels also control in vitro hydroxylation and stability of hypoxia inducible factor-1 alpha in human glioma cells (Li et al., 2015). Impairing TRPC6 activity in vitro in human glioma cells induced cell cycle arrest at the G2/M phase and, in vivo, reduced human xenograft growth in immunocompromised mice, while increasing the survival of the xenografted mice (Ding et al., 2010).
TRPV Channels
TRPV1 is the prototype of the TRPV channel family, which includes six members; it is activated by heat and synthetic or endogenous vanilloids (Caterina et al., 1997). TRPV2 shares approximately 50% sequence identity with TRPV1, while showing distinct cellular functions from those mediated by TRPV1 (Cohen et al., 2015). TRPV1 expression is mainly localized to the plasma membrane, while TRPV2 is localized to the intracellular membranes under unstimulated conditions (Cohen et al., 2013). TRPV2 is not stimulated by heat or by vanilloid exposure (Cohen et al., 2013 and 2015). TRPV2 activity is induced by 2-aminoethoxydiphenyl borate, probenecid, and cannabidiol and inhibited by ruthenium red, gadolinium, and tranilast (Caterina et al., 1997; Bang et al., 2007; Perálvarez-Marín et al., 2013; Nabissi et al., 2015). The translocation of TRPV2 from the endosome to the plasma membrane, a feature that influences both cell proliferation and cell death, is stimulated for example by growth factors, cytokines, hormones, and endocannabinoids (Liberati et al., 2014a,b). Uncontrolled cell proliferation and apoptotic resistance occur with loss or changes in TRPV2-mediated signals, whereas TRPV2 activation stimulates both the migration and the invasiveness of cancer cells (Liberati et al., 2014a,b).
The scientific literature reports the implications of TRPV1 and TRPV2 in glioma cell biology, as described below.
TRPV1
TRPV1 is mainly expressed by primary sensory neurons involved in nociception and neurogenic inflammation (Caterina et al., 2000).
TRPV1 gene and protein expressions are progressively lost, while the level of malignancy increases in gliomas, with a marked loss of TRPV1 expression in almost all (93%) of the glioblastomas analyzed by Amantini et al. (2007). Moreover, TRPV1 mRNA expression was correlated with patients’ overall survival (OS). TRPV1 variant 3 mRNA expression reached significance (p = 0.0009) for survival with short OS glioblastoma patients, showing a lower TRPV1 variant 3 mRNA expression compared with long OS patients (Nabissi et al., 2016). TRPV1 is implicated in the capsaicin-induced p38 mitogen-activated protein kinase-dependent apoptosis of glioma cells in vitro (Amantini et al., 2007). TRPV1 stimulation also triggers tumor cell death via the activating transcription factor-3 (ATF3)-controlled branch of the endoplasmic reticulum stress pathway.
Somatic mutant neural stem and precursor cells (NPCs) are thought to be the source of high-grade astrocytomas which are much more frequent in adults than in children. Stock et al. (2012) have shown that high-grade astrocytoma-associated NPCs induce tumor cell death via the release of endovanilloids, which induce Ca2+ responses. Endovanilloids directly stimulate the vanilloid receptor TRPV1 (Toth et al., 2009). However, the anti-tumorigenic response of NPCs is lost with age. Stock et al. (2012) report that NPC-mediated tumor suppression can be mimicked in the adult brain by systemic administration of the synthetic brain barrier-permeable vanilloid Arvanil (Figure 2), suggesting that TRPV1 agonists hold potential as new high-grade glioma therapeutics.
TRPV2
TRPV2 expression decreases during glioma progression to higher clinical stages; TRPV2 negatively controls glioma cell survival and proliferation and protects cells from Fas-induced apoptosis in an ERK-dependent manner (Nabissi et al., 2010). This receptor also negatively controls resistance to carmustine (BCNU)-induced apoptosis (Nabissi et al., 2010). High glioblastoma resistance to standard chemotherapy is one of the major hallmarks of glioblastoma biological aggressiveness. The TRPV2 agonist cannabidiol, by increasing TRPV2 expression and activity by triggering TRPV2-dependent Ca2+ influx, increases chemotherapeutic drug uptake and synergizes with cytotoxic agents (doxorubicin, temozolomide, and BCNU) to induce in vitro apoptosis in glioma cells but not in normal human astrocytes (Nabissi et al., 2013). TRPV2 activation promotes differentiation and inhibits the proliferation of glioblastoma stem cells (GSCs) in vitro and in vivo (Morelli et al., 2012). Redifferentiating cancer stem cell subpopulations could be achieved while using drug-induced differentiation, knowing that cancer stem cells are radio- and chemoresistant. Stimulating TRPV2 cannabidiol triggers GSC differentiation by inducing autophagy and inhibiting GSC proliferation and clonogenicity. Moreover, the cannabidiol and BCNU combination overcame GCS resistance to BCNU treatment by inducing apoptosis (Nabissi et al., 2015). In glioblastoma, TRPV2 is part of an interactome-based signature complex (Doñate-Macián et al., 2018), which is negatively associated with patient survival, and it is expressed in high risk of recurrence and temozolomide-resistant patients (Santoni et al., 2020a).
TRPML Channels
Endosome/lysosome Ca2+ channel proteins are characteristic of the TRPML channel family. In mammals, there are three TRPML proteins (TRPML-1, TRPML-2, and TRPML-3). A link between TRPML channel physiology and tumor biology has been suggested, and we focus here on glioma biology.
TRPML1
TRPML1, which is primarily localized in the late endosome/lysosome, is ubiquitously expressed in mammalian cells. It plays roles in the control of cell viability and in chaperone-mediated autophagy (Venkatachalam et al., 2006). A mutated TRPML1 gene in humans causes a neurodegenerative disease in children, i.e., mucolipidosis type IV (Bargal et al., 2000). TRPML1 is a proton-impermeable, cation-selective channel with permeability to both Ca2+ and Fe2+. Chaperone-mediated autophagy-related proteins [for example the heat shock cognate protein Hsc70 and the 40-kDa heat shock protein (Hsp40)] interact with the large TRPML1 intraluminal loop (Venkatachalam et al., 2006).
Morelli et al. (2019) showed that the loss/reduction of TRPML1 mRNA expression strongly correlates with short survival in glioblastoma patients. This feature could be explained, at least partly, by the fact that TRPML1 targets the apoptosis-like gene 2 (ALG-2) gene whose protein promotes caspase-3-independent cell death associated with glioblastoma progression and poor prognosis (Vergarajauregui et al., 2009; Zhang et al., 2017). Morelli et al. (2019) have also conducted elegant experiments with various glioma cell types to demonstrate that TRPML1 is an oxidative stress sensor that activates irreversible autophagy leading to cell death.
TRPML2
While TRPML2 is found in normal astrocytes and neural stem/progenitor cells, its expression at both mRNA and protein levels dramatically augment (Morelli et al., 2016) in high-grade glioblastoma cell lines of astrocytic origin and glioblastoma tissues (Morelli et al., 2016). Morelli et al. (2016) experimentally demonstrated that cell viability and proliferation are inhibited in TRPML2 knockdown glioblastoma cells, while caspase-3-dependent apoptosis is increased.
TRPM Channels
The TRPM subfamily is composed of eight members consisting of four six-transmembrane domain subunits, resulting in homomeric or heteromeric channels. TRPM subfamily members have been involved in several physiological functions and pathophysiological human processes.
TRPM2
Oxidative stress and tumor necrosis factor alpha are two extracellular signals known to activate TRPM2 channels, with consequently the activation of necrotic cell death (Zhang et al., 2006). Ishii et al. (2007) showed that the insertion of TRPM2 channels by means of transfection into the malignant glioma cell line A172 enhanced cell death induced by H2O2. In a recent study, Bao et al. (2020) observed a significant increase in TRPM2-AS, a long non-coding RNA with a length greater than 200 base pairs), which is transcribed from the antisense chain of TRPM2, in 111 glioma patients with glioma as compared to the normal control group. Overexpressing TRPM2-AS in human glioblastoma cells increases their proliferation, migration, and invasion, while downregulation of TRPM2-AS inhibits these three processes (Bao et al., 2020). TRPM2-AS signaling in glioma cells involves c-Jun N-terminal kinase (JNK), c-Jun protein, and regulator of G-protein signaling 4 (RGS4) (Bao et al., 2020).
TRPM7
A large number of breast, lung, pancreatic, prostate, gastric, and head and neck cancers and malignant gliomas express high TRPM7 levels (Jiang et al., 2007; Kim et al., 2008; Guilbert et al., 2009; Rybarczyk et al., 2012; Sun et al., 2014; Alptekin et al., 2015; Chen et al., 2016). For example, malignant glioma tissues express higher TRPM7 mRNA than normal brain tissues (Chen et al., 2016; Wan et al., 2020). TRPM7 silencing reduced glioma cell growth by inhibiting cell entry into S and G2/M phases and promoting cell apoptosis (Wan et al., 2020). TRPM7 expression in glioblastoma cells was found to be positively correlated with Notch1 signaling activity and CD133 and ALDH1 expression; briefly, downregulation of TRPM7 by siTRPM7 decreased Notch1 signaling whereas upregulation of TRPM7 increased Notch1 signaling (Wan et al., 2020).
Carvacrol (Figure 2) is one of the several inhibitors of TRPM7 already identified (Parnas et al., 2009). This compound is a secondary metabolite (a monoterpenoid phenol) found in oregano essential oils from numerous genera (Baser, 2008). Suppression of TRPM7 activity through the use of carvacrol and the use of TRPM7-siRNA dramatically reduced the proliferation, migration, and invasion levels of the U87MG malignant glioma cell line, which expresses higher levels of TRPM7 mRNA and protein than normal human astrocytes (Chen et al., 2015; Leng et al., 2015). MGR2 glioma cells also express TRPM7 and display TRPM7 currents (Chen et al., 2016). Chen et al. (2016) identified a widely used anesthetic compound in clinics since the 1970s, i.e., midazolam (Figure 2), as a TRPM7 inhibitor. The use of midazolam for in vitro treatment periods as short as seconds on glioma cells suppressed TRPM7 currents and calcium influx, while treatment for 48 h vanished TRPM7 expression (Chen et al., 2016). The inhibitory effect of midazolam on TRPM7 currents results in a decrease in proliferation and G0/G1 phase cell cycle arrest in two human glioblastoma cell lines (Chen et al., 2016). Of note, midazolam is a short-acting benzodiazepine that crosses the blood–brain barrier with a favorable pharmacological profile, and it could be used at first glance to treat patients with malignant glioma if one considers TRPM7 as a valuable target. However, the concentration (100 μM) of midazolam used in vitro in the study reported by Chen et al. (2016) is much higher than the clinical concentration ranges reported for this compound. It must indeed be emphasized that midazolam used at high doses induces sedative and hypnotic effects and therefore precludes its use as a chronic treatment for malignant glioma patients (Olkkola and Ahonen, 2008). Novel derivatives of midazolam or medical devices for its local delivery should be developed if it is to be used for glioma chemotherapy.
TRPM8
TRPM8 was first identified in prostate carcinoma (Tsavaler et al., 2001) and then in a number of other cancer types (Liu et al., 2014; Yee et al., 2014; Yu et al., 2014); it has been more recently shown to be upregulated in glioblastoma compared to normal brain tissue (Alptekin et al., 2015; Zeng et al., 2019), while TRPM8 expression is highly heterogeneous in human glioblastoma specimens as well as in established cell lines (Klumpp et al., 2017). Zeng et al. (2019) showed that high expression of TRPM8 mRNA was associated with a shorter OS time in patients with glioblastoma. TRPM8 channels facilitate Ca2+ entry in glioblastoma cells, and their activation has been shown to stimulate large-conductance K+ channel activity and, consequently, glioblastoma cell migration (Wondergem et al., 2008; Wondergem and Bartley, 2009; Klumpp et al., 2017). In vitro, using the U251 human glioblastoma cell line, Zeng et al. (2019) showed that TRPM8 enhances the sensitivity of glioblastoma cells to apoptosis and regulates the proliferation and invasion abilities. Klumpp et al. (2017) showed in vitro using human glioblastoma cells that (i) TRPM8 signaling is involved in cell cycle regulation and represses apoptotic cell death; (ii) clinically compatible ionizing radiation doses for treating glioblastoma patients induce upregulation of TRPM8 function; and (iii) elevated TRPM8 function, in turn, confers radioresistance (Klumpp et al., 2017). A combination of TRPM8 targeting and radiotherapy could be an interesting approach for future glioblastoma therapy. As developed in the next section, some strategies to target TRPM8 have already been developed and/or are ongoing.
Therapeutic Perspectives
As summarized above, TRP channels exert various roles in cancer cell biology, including glioma ones (Gaunt et al., 2016; He and Ma, 2016; Jardin and Rosado, 2016; Li and Ding, 2017; Zhan and Shi, 2017). A number of more or less specific compounds from synthetic versus natural origin that selectively target different subtypes of TRP channels have been discovered, including some preclinical candidates (Wang et al., 2020). We recall these promising compounds below.
Some reasonably specific pharmacological TRPM8 inhibitors are already available (Ohmi et al., 2014; Lehto et al., 2015), including antibodies binding the extracellular TRPM8 protein and inhibiting TRPM8 function (Miller et al., 2014). However, the available studies have been performed in vitro only, and preclinical studies in orthotopic glioblastoma animal models are still missing. There is still a long road ahead until these types of compounds will enter clinics for treating malignant glioma patients.
Riluzole (Figure 2) is a TRPC5 agonist; however, it can also act on other ion channels so this limits its use. Riluzole is an approved drug for the treatment of amyotrophic lateral sclerosis, and it entered clinical trials for melanoma therapy. The precise mechanism(s) of action of riluzole is not yet fully deciphered. The riluzole-induced activation of TRPC5 channels, while expressed heterologously (as in HEK293 Human Embryonic Kidney cells) or endogenously (as in U87MG glioblastoma cells), seems to be independent of various cytosolic components, such as phospholipase C activity or intracellular calcium stores, suggesting therefore that riluzole could have a rather direct effect on TRPC5 (Richter et al., 2014). Furthermore, prednisolone (Figure 2), largely used in the context of glioma treatment to decrease glioblastoma-associated edema, also acts as a weak activator of TRPC5 (Beckmann et al., 2017). As emphasized above for TRPM8, there is still a long road before these types of compounds targeting TRPC5 will enter clinics to treat malignant glioma patients.
SKF-96365 (Figure 2), a non-specific TRPC6 and TRPC7 antagonist, displays cytotoxic effects in several cancer cell types (Song et al., 2014). In glioblastoma cells, SKF-96365 exerts anti-growth effects through the promotion of the reverse mode of Na+/Ca2+ exchangers, thereby increasing Ca2+ (Song et al., 2014). This compound does not seem very appropriate, at least in our current knowledge of its mode of action, to be an actual candidate to combat glioblastoma in clinical situations.
Cannabinoids: New Application for Old Agents
In contrast to the compounds we refer to above, certain cannabinoids could be of major importance to combat glioblastoma in clinical situations and a TRP context as explained hereafter. The term cannabinoids originally described bioactive constituents of the plant Cannabis sativa, used traditionally for their medicinal purpose as well as their recreational properties. Cannabinoids can reduce glioma growth both in vitro and in vivo (Velasco et al., 2007; Sarfaraz et al., 2008). Among the cannabinoid compounds, we emphasize the potential of cannabidiol (Figure 2) to combat glioblastoma in clinical situations for the reasons we explained here below. Cannabidiol is a cannabinoid that lacks unwanted psychotropic liability and has no significant agonist activity on cannabinoid receptors (Howlett et al., 2002; Pertwee et al., 2005). Cannabidiol has been investigated as an antitumoral agent in a number of studies (Dumitru et al., 2018 for review).
In vitro, cannabidiol inhibits migration (Vaccani et al., 2005) and induces apoptosis in human glioma cells (Massi et al., 2004, 2006; Solinas et al., 2013), while it increases chemotherapeutic drug uptake and parallelly potentiates the cytotoxic activity of chemotherapeutic agents in a TRPV2-dependent manner in human glioma cells (Nabissi et al., 2013). In vitro, cannabidiol enhances the inhibitory effects of cannabinoid 1 and cannabinoid 2 receptor agonist delta(9)-tetrahydrocannabinol (Δ9-THC) on human glioblastoma cell survival and proliferation (Marcu et al., 2010). The combination of cannabidiol with Δ9-THC and temozolomide reduces the growth of U87MG glioma xenografts (Torres et al., 2011). Cannabidiol may also be effective at reducing the proliferation of GSC chemoresistant subpopulations present in glioblastomas (Singh et al., 2004). In glioma xenografts, including those derived from glioma stem cells, López-Valero et al. (2018) showed that a combined therapy of oral cannabinoids and temozolomide synergistically reduced the growth and enhanced the survival of xenografted animals. Cannabidiol, by activating TRPV2, (i) triggers GSC differentiation, (ii) activates their autophagic processes, (iii) inhibits glioma stem cell proliferation, (iv) inhibits their clonogenic capability, and (v) abrogates their resistance to carmustine (BCNU) (Nabissi et al., 2015).
A pilot phase I clinical trial for the treatment of glioblastoma patients indicated a good safety profile for Δ9-THC, which is a psychoactive cannabinoid (Velasco et al., 2007). The intratumoral administration of this compound was first tested in a small series of nine patients (Guzmán et al., 2006). Preclinical studies have also investigated the antitumor effects of the cannabinoid combination (Δ9-THC and cannabidiol) and found an enhanced antineoplastic effect (Ladin et al., 2016) in combination with temozolomide or radiotherapy (Torres et al., 2011; Scott et al., 2014; Ladin et al., 2016).
A placebo-controlled phase II clinical trial investigated a tetrahydrocannabidiol–cannabidiol mixture in combination with dose-intense temozolomide in glioblastoma (NCT01812603) (Schultz and Beyer, 2017). This study included 21 adult glioblastoma patients receiving a maximum of 12 sprays orally per day, delivering 100 μl of a solution containing 27 mg/ml Δ9-THC and 25 mg/ml cannabidiol. The control group received temozolomide and only reached a 44% 1-year survival rate. In sharp contrast, the tetrahydrocannabidiol–cannabidiol mixture plus temozolomide group showed an 83% 1-year survival rate, with a median survival of over 662 days compared with 369 days in the control group (Schultz and Beyer, 2017; Schultz, 2018). In another study, nine consecutive patients with brain tumors received cannabidiol at a daily dose of 400 mg concomitantly to the standard therapeutic procedure of maximal resection followed by combined radio- and chemotherapy and adjuvant chemotherapy (Likar et al., 2019). The authors reported that, by the time of the submission of their article, all but one patient were still alive, with a mean survival time of 22.3 months (range from 7 to 47). Importantly, the well-acknowledged median survival for glioblastoma patients is 16 months, as reported in the reference study by Stupp et al. (2009). A recent case report study demonstrated satisfactory clinical and imaging responses for two patients with a confirmed diagnosis of high-grade glioma (grades III or IV), benefiting after surgery from chemoradiation followed by the combination of procarbazine, lomustine, and vincristine associated with cannabidiol (Dall’Stella et al., 2019).
Conclusion
Multiple ion channels rely on intracellular Ca2+, and it makes sense to target a common Ca2+ source, such as specific TRP channels, which are heavily involved in glioma cell biology.
We are only at the beginning of our understanding of the precise roles of various TRP channels in glioma cell biology, and further studies are required to truly understand the physiopathological roles of TRP channels in glioma progression. However, some promising data from the literature, even if still scarce, already point to the very high promise of targeting TRPV2 by means of cannabidiol, a cannabinoid that lacks unwanted psychotropic liability.
Author Contributions
The author confirms being the sole contributor of this work and has approved it for publication.
Conflict of Interest
The author declares that the research was conducted in the absence of any commercial or financial relationships that could be construed as a potential conflict of interest.
Publisher’s Note
All claims expressed in this article are solely those of the authors and do not necessarily represent those of their affiliated organizations, or those of the publisher, the editors and the reviewers. Any product that may be evaluated in this article, or claim that may be made by its manufacturer, is not guaranteed or endorsed by the publisher.
References
Alexander, S. P. H., Mathie, A., Peters, J. A., Veale, E. L., Striessnig, J., Kelly, E., et al. (2019). The concise guide to pharmacology 2019/20: ion channels. Br. J. Pharmacol. 176(Suppl. 1) S142–S228. doi: 10.1111/bph.14749
Alptekin, M., Eroglu, S., Tutar, E., Sencan, S., Geyik, M. A., Ulasli, M., et al. (2015). Gene expressions of TRP channels in glioblastoma multiforme and relation with survival. Tumour. Biol. 36, 9209–9213. doi: 10.1007/s13277-015-3577-x
Amantini, C., Mosca, M., Nabissi, M., Lucciarini, R., Caprodossi, S., Arcella, A., et al. (2007). Capsaicin-induced apoptosis of glioma cells is mediated by TRPV1 vanilloid receptor and requires p38 MAPK activation. J. Neurochem. 102, 977–990. doi: 10.1111/j.1471-4159.2007.04582.x
Bang, S., Kim, K. Y., Yoo, S., Lee, S. H., and Hwang, S. W. (2007). Transient receptor potential V2 expressed in sensory neurons is activated by probenecid. Neurosci. Lett. 425, 120–125. doi: 10.1016/j.neulet.2007.08.035
Bao, M. H., Lv, Q. L., Szeto, V., Wong, R., Zhu, S. Z., Zhang, Y. Y., et al. (2020). TRPM2-AS inhibits the growth, migration, and invasion of gliomas through JNK, c-Jun, and RGS4. J. Cell Physiol. 235, 4594–4604. doi: 10.1002/jcp.29336
Bargal, R., Avidan, N., Ben-Asher, E., Olender, Z., Zeigler, M., Frumkin, A., et al. (2000). Identification of the gene causing mucolipidosis type IV. Nat. Genet. 26, 118–123. doi: 10.1038/79095
Baser, K. H. (2008). Biological and pharmacological activities of carvacrol and carvacrol bearing essential oils. Curr. Pharm. Des. 14, 3106–3119. doi: 10.2174/138161208786404227
Becchetti, A., Munaron, L., and Arcangeli, A. (2013). The role of ion channels and transporters in cell proliferation and cancer. Front. Physiol. 4:312. doi: 10.3389/fphys.2013.00312
Beckmann, H., Richter, J., Hill, K., Urban, N., Lemoine, H., and Schaefer, M. (2017). A benzothiadiazine derivative and methylprednisolone are novel and selective activators of transient receptor potential canonical 5 (TRPC5) channels. Cell Calcium 66, 10–18. doi: 10.1016/j.ceca.2017.05.012
Bernardini, M., Fiorio Pla, A., Prevarskaya, N., and Gkika, D. (2015). Human transient receptor potential (TRP) channel expression profiling in carcinogenesis. Int. J. Dev. Biol. 59, 399–406. doi: 10.1387/ijdb.150232dg
Bernstock, J. D., Mooney, J. H., Ilyas, A., Chagoya, G., Estevez-Ordonez, D., Ibrahim, A., et al. (2019). Molecular and cellular intratumoral heterogeneity in primary glioblastoma: clinical and translational implications. J. Neurosurg. 23, 1–9. doi: 10.3171/2019.5.JNS19364
Bodding, M. (2007). TRP proteins and cancer. Cell Signal 19, 617–624. doi: 10.1016/j.cellsig.2006.08.012
Bomben, V. C., and Sontheimer, H. (2010). Disruption of transient receptor potential canonical channel 1 causes incomplete cytokinesis and slows the growth of human malignant gliomas. Glia 58, 1145–1156. doi: 10.1002/glia.20994
Bomben, V. C., and Sontheimer, H. W. (2008). Inhibition of transient receptor potential canonical channels impairs cytokinesis in human malignant gliomas. Cell Prolif. 41, 98–121. doi: 10.1111/j.1365-2184.2007.00504.x
Bomben, V. C., Turner, K. L., Barclay, T. T. C., and Sontheimer, H. (2011). Transient receptor potential canonical channels are essential for chemotactic migration of human malignant gliomas. J. Cell Physiol. 226, 1879–1888. doi: 10.1002/jcp.22518
Caterina, M. J., Leffler, A., Malmberg, A. B., Martin, W. J., Trafton, J., Petersen-Zeitz, K. R., et al. (2000). Impaired nociception and pain sensation in mice lacking the capsaicin receptor. Science 288, 306–313. doi: 10.1126/science.288.5464.306
Caterina, M. J., Schumacher, M. A., Tominaga, M., Rosen, T. A., Levine, J. D., and Julius, D. (1997). The capsaicin receptor: a heat-activated ion channel in the pain pathway. Nature 389, 816–824. doi: 10.1038/39807
Chang, H. H., Cheng, Y. C., Tsai, W. C., Tsa, M. J., and Chen, Y. (2018). Pyr3 induces apoptosis and inhibits migration in human glioblastoma cells. Cell Physiol. Biochem. 48, 1694–1702. doi: 10.1159/000492293
Chen, J., Dou, Y., Zheng, X., Leng, T., Lu, X., Ouyang, Y., et al. (2016). TRPM7 channel inhibition mediates midazolam-induced proliferation loss in human malignant glioma. Tumour. Biol. 37, 14721–14731. doi: 10.1007/s13277-016-5317-2
Chen, J., Luan, Y., Yu, R., Zhang, Z., Zhang, J., and Wang, W. (2014). Transient receptor potential (TRP) channels, promising potential diagnostic and therapeutic tools for cancer. Biosci. Trends 8, 1–10. doi: 10.5582/bst.8.1
Chen, W. L., Barszczyk, A., Turlova, E., Deurloo, M., Liu, B., Yang, B. B., et al. (2015). Inhibition of TRPM7 by carvacrol suppresses glioblastoma cell proliferation, migration and invasion. Oncotarget 6, 16321–16340. doi: 10.18632/oncotarget.3872
Chigurupati, S., Venkataraman, R., Barrera, D., Naganathan, A., Madan, M., Paul, L., et al. (2010). Receptor channel TRPC6 is a key mediator of Notch-driven glioblastoma growth and invasiveness. Cancer Res. 70, 418–427. doi: 10.1158/0008-5472.CAN-09-2654
Cohen, M. R., Huynh, K. W., Cawley, D., and Moiseenkova-Bell, V. Y. (2013). Understanding the cellular function of TRPV2 channel through generation of specific monoclonal antibodies. PLoS One 8:e85392. doi: 10.1371/journal.pone.0085392
Cohen, M. R., Johnson, W. M., Pilat, J. M., Kiselar, J., DeFrancesco-Lisowitz, A., Zigmond, R. E., et al. (2015). Nerve Growth factor regulates transient receptor potential vanilloid 2 via extracellular signal-regulated kinase signaling to enhance neurite outgrowth in developing neurons. Mol. Cell Biol. 35, 4238–4252. doi: 10.1128/MCB.00549-15
Colwell, N., Larion, M., Giles, A. J., Seldomridge, A. N., Sizdahkhani, S., Gilbert, M. R., et al. (2017). Hypoxia in the glioblastoma microenvironment: shaping the phenotype of cancer stem-like cells. Neuro. Oncol. 19, 887–896. doi: 10.1093/neuonc/now258
Dall’Stella, P. B., Docema, M. F. L., Maldaun, M. V. C., Feher, O., and Lancellotti, C. L. P. (2019). Case report: clinical outcome and image response of two patients with secondary high-grade glioma treated with chemoradiation, PCV, and cannabidiol. Front. Oncol. 8:643. doi: 10.3389/fonc.2018.00643
Ding, X., He, Z., Zhou, K., Cheng, J., Yao, H., Lu, D., et al. (2010). Essential role of trpc6 channels in G2/M phase transition and development of human glioma. J. Natl. Cancer Inst. 102, 1052–1068. doi: 10.1093/jnci/djq217
Doñate-Macián, P., Gómez, A., Dégano, I. R., and Perálvarez-Marín, A. A. (2018). TRPV2 interactome-based signature for prognosis in glioblastoma patients. Oncotarget 9, 18400–18409. doi: 10.18632/oncotarget.24843
Dumitru, C. A., Sandalcioglu, I. E., and Karsak, M. (2018). Cannabinoids in glioblastoma therapy: new applications for old drugs. Front. Mol. Neurosci. 11:159. doi: 10.3389/fnmol.2018.00159
Ernst, N. J., Habela, C. W., and Sontheimer, H. (2008). Cytopkasmic condensation is both necessary and sufficient to induce apoptotic cell death. J. Cell Sci. 121(Pt 3), 290–297. doi: 10.1242/jcs.017343
Galluzzi, L., Vitale, I., Abrams, J. M., Alnemri, E. S., Baehrecke, E. H., Blagosklonny, M. V., et al. (2012). Molecular definitions of cell death subroutines: recommendations of the Nomenclature Committee on Cell Death 2012. Cell Death Differ 19, 107–120. doi: 10.1038/cdd.2011.96
Gaunt, H. J., Vasudev, N. S., and Beech, D. J. (2016). Transient receptor potential canonical 4 and 5 proteins as targets in cancer therapeutics. Eur. Biophys. J. 45, 611–620. doi: 10.1007/s00249-016-1142-1
Gkika, D., and Prevarskaya, N. (2009). Molecular mechanisms of TRP regulation in tumor growth and metastasis. Biochim. Biophys. Acta 1793, 953–958. doi: 10.1016/j.bbamcr.2008.11.010
Guilbert, A., Gautier, M., Dhennin-Duthille, I., Haren, N., Sevestre, H., and Ouadid-Ahidouch, H. (2009). Evidence that TRPM7 is required for breast cancer cell proliferation. Am. J. Physiol. Cell Physiol. 297, C493–C502. doi: 10.1152/ajpcell.00624.2008
Guzmán, M., Duarte, M. J., Blázquez, C., Ravina, J., Rosa, M. C., Galve-Roperh, I., et al. (2006). A pilot clinical study of Delta9-tetrahydrocannabinol in patients with recurrent glioblastoma multiforme. Br. J. Cancer 95, 197–203. doi: 10.1038/sj.bjc.6603236
Habela, C. W., Ernest, N. J., Swindall, A. F., and Sontheimer, H. (2009). Chloride accumulation drives volume dynamics underlying cell proliferation and migration. J. Neurophysiol. 101, 750–757. doi: 10.1152/jn.90840
Hanahan, D., and Weinberg, R. A. (2011). Hallmarks of cancer: the next generation. Cell 144, 646–674. doi: 10.1016/j.cell.2011.02.013
He, D. X., and Ma, X. (2016). Transient receptor potential channel C5 in cancer chemoresistance. Acta Pharmacol. Sin. 37, 19–24. doi: 10.1038/aps.2015.109
Howlett, A. C., Barth, F., Bonner, T. I., Cabral, G., Casellas, P., Devane, W. A., et al. (2002). International union of pharmacology. XXVII. Classification of cannabinoid receptors. Pharmacol. Rev. 54, 161–202. doi: 10.1124/pr.54.2.161
Ishii, M., Oyama, A., Hagiwara, T., Miyazaki, A., Mori, Y., Kiuchi, Y., et al. (2007). Facilitation of H2O2-induced A172 human glioblastoma cell death by insertion of oxidative stress-sensitive TRPM2 channels. Anticancer Res. 27, 3987–3992.
Jardin, I., and Rosado, J. A. (2016). STIM and calcium channel complexes in cancer. Biochim. Biophys. Acta 1863(6 Pt B), 1418–1426. doi: 10.1016/j.bbamcr.2015.10.003
Jiang, J., Li, M. H., Inoue, K., Chu, X. P., Seeds, J., and Xiong, Z. G. (2007). Transient receptor potential melastatin 7-like current in human head and neck carcinoma cells: role in cell proliferation. Cancer Res. 67, 10929–10938. doi: 10.1158/0008-5472.CAN-07-1121
Kim, B. J., Park, E. J., Lee, J. H., Jeon, J.-H., Kim, S. J., and So, I. (2008). Suppression of transient receptor potential melastatin 7 channel induces cell death in gastric cancer. Cancer Sci. 99, 2502–2509. doi: 10.1111/j.1349-7006.2008.00982.x
Klumpp, D., Frank, S. C., Klumpp, L., Sezgin, E. C., Eckert, M., Edalat, L., et al. (2017). TRPM8 is required for survival and radioresistance of glioblastoma cells. Oncotarget 8, 95896–95913. doi: 10.18632/oncotarget.21436
Kornienko, A., Mathieu, V., Rastogi, S. K., Lefranc, F., and Kiss, R. (2013). Therapeutic agents triggering nonapoptotic cancer cell death. J. Med. Chem. 56, 4823–4839. doi: 10.1021/jm400136m
Kress, M., Karasek, J., Ferrer-Monteil, A. V., Scherbakov, N., and Haberberger, R. V. (2008). TRPC channels and diacylglycerol dependent calcium signaling in rat sensory neurons. Histochem. Cell Biol. 130, 655–667. doi: 10.1007/s00418-008-0477-9
Ladin, D. A., Soliman, E., Griffin, L., and Van Dross, R. (2016). Preclinical and clinical assessment of cannabinoids as anti-cancer agents. Front. Pharmacol. 7:361. doi: 10.3389/fphar.2016.00361
Lefranc, F., Le Rhun, E., Kiss, R., and Weller, M. (2018). Glioblastoma quo vadis: will migration and invasiveness reemerge as therapeutic targets? Cancer Treat. Rev. 68, 145–154. doi: 10.1016/j.ctrv.2018.06.017
Lefranc, F., Pouleau, H. B., Rynkowski, M., and De Witte, O. (2012). Voltage-dependent K+ channels as oncotargets in malignant gliomas. Oncotarget 3, 516–517. doi: 10.18632/oncotarget.514
Lehto, S. G., Weyer, A. D., Zhang, M., Youngblood, B. D., Wang, J., Wang, W., et al. (2015). AMG2850, a potent and selective TRPM8 antagonist, is not effective in rat models of inflammatory mechanical hypersensitivity and neuropathic tactile allodynia. Naunyn. Schmiedeb. Arch Pharmacol. 388, 465–476. doi: 10.1007/s00210-015-1090-9
Leng, T. D., Li, M. H., Shen, J. F., Liu, M. L., Li, X. B., Sun, H. W., et al. (2015). Suppression of TRPM7 inhibits proliferation, migration, and invasion of malignant human glioma cells. CNS Neurosci. Ther. 21, 252–261. doi: 10.1111/cns.12354
Li, H. (2017). TRP channel classification. Adv. Exp. Med. Biol. 976, 1–8. doi: 10.1007/978-94-024-1088-4_1
Li, S., and Ding, X. (2017). TRPC channels and glioma. Adv. Exp. Med. Biol. 976, 157–165. doi: 10.1007/978-94-024-1088-4_14
Li, S., Wang, J., Wei, Y., Liu, Y., Ding, X., Dong, B., et al. (2015). Crucial role of TRPC6 in maintaining the stability of HIF-1alpha in glioma cells under hypoxia. J. Cell Sci. 128, 3317–3329. doi: 10.1242/jcs.173161
Liberati, S., Morelli, M. B., Amantini, C., Farfariello, V., Santoni, M., Conti, A., et al. (2014a). Loss of TRPV2 homeostatic control of cell proliferation drives tumor progression. Cells 3, 112–128. doi: 10.3390/cells3010112
Liberati, S., Morelli, M. B., Amantini, C., Santoni, M., Nabissi, M., Cardinali, C., et al. (2014b). Advances in transient receptor potential vanilloid-2 channel expression and function in tumor growth and progression. Curr. Protein Pept. Sci. 15, 732–737. doi: 10.2174/1389203715666140704115913
Likar, R., Koestenberger, M., Stultschnig, M., and Nahler, G. (2019). Concomitant treatment of malignant brain tumors with CBD – A case series and review of the literature. Anticancer Res. 39, 5797–5801. doi: 10.21873/anticanres.13783
Litan, A., and Langhans, S. A. (2015). Cancer as a channelopathy: ion channels and pumps in tumor development and progression. Front. Cell Neurosci. 9:86. doi: 10.3389/fncel.2015.00086
Liu, J., Chen, Y., Shuai, S., Ding, D., Li, R., and Luo, R. (2014). TRPM8 promotes aggressiveness of breast cancer cells by regulating EMT via activating AKT/GSK-3β pathway. Tumour. Biol. 35, 8969–8977. doi: 10.1007/s13277-014-2077-8
Locarno, C. V., Simonelli, M., Carenza, C., Capucetti, A., Stanzani, E., Lorenzi, E., et al. (2020). Role of myeloid cells in the immunosuppressive microenvironment in gliomas. Immunobiology 225:151853. doi: 10.1016/j.imbio.2019.10.002
López-Valero, I., Saiz-Ladera, C., Torres, S., Hernández-Tiedra, S., García-Taboada, E., Rodríguez-Fornés, F., et al. (2018). Targeting glioma initiating cells with a combined therapy of cannabinoids and temozolomide. Biochem. Pharmacol. 157, 266–274. doi: 10.1016/j.bcp.2018.09.007
Marcu, J. P., Christian, R. T., Lau, D., Zielinski, A. J., Horowitz, M. P., Lee, J., et al. (2010). Cannabidiol enhances the inhibitory effects of delta9-tetrahydrocannabinol on human glioblastoma cell proliferation and survival. Mol. Cancer Ther. 9, 180–189. doi: 10.1158/1535-7163.MCT-09-0407
Massi, P., Vaccani, A., Bianchessi, S., Costa, B., Macchi, P., and Parolaro, D. (2006). The non-psychoactive cannabidiol triggers caspase activation and oxidative stress in human glioma cells. Cell Mol. Life Sci. 63, 2057–2066. doi: 10.1007/s00018-006-6156-x
Massi, P., Vaccani, A., Ceruti, S., Colombo, A., Abbracchio, M. P., and Parolaro, D. (2004). Antitumor effects of cannabidiol, a nonpsychoactive cannabinoid, on human glioma cell lines. J. Pharmacol. Exp. Ther. 308, 838–845. doi: 10.1124/jpet.103.061002
Matarredona, E. R., and Pastor, A. M. (2019). Neural stem cells of the subventricular zone as the origin of human glioblastoma stem cells, therapeutic implications. Front Oncol. 9:779. doi: 10.3389/fonc.2019.00779
McGranahan, T., Therkelsen, K. E., Ahmad, S., and Nagpal, S. (2019). Current state of immunotherapy for treatment of glioblastoma. Curr. Treat. Options Oncol. 20:24. doi: 10.1007/s11864-019-0619-4
Miller, S., Rao, S., Wang, W., Liu, H., Wang, J., and Gavva, N. R. (2014). Antibodies to the extracellular pore loop of TRPM8 act as antagonists of channel activation. PLoS One 9:e107151. doi: 10.1371/journal.pone.0107151
Montell, C., Birnbaumer, L., and Flockerzi, V. (2002). The TRP channels, a remarkably functional family. Cell 108, 595–598. doi: 10.1016/s0092-8674(02)00670-0
Morelli, M. B., Amantini, C., Tomassoni, D., Nabissi, M., Arcella, A., and Santoni, G. (2019). Transient receptor potential mucolipin-1 channels in glioblastoma: role in patient’s survival. Cancers (Basel) 11:525. doi: 10.3390/cancers11040525
Morelli, M. B., Nabissi, M., Amantini, C., Farfariello, V., Ricci-Vitiani, L., di Martino, S., et al. (2012). The transient receptor potential vanilloid-2 cation channel impairs glioblastoma stem-like cell proliferation and promotes differentiation. Int. J. Cancer 131, E1067–E1077. doi: 10.1002/ijc.27588
Morelli, M. B., Nabissi, M., Amantini, C., Tomassoni, D., Rossi, F., Cardinali, C., et al. (2016). Overexpression of transient receptor potential mucolipin-2 ion channels in gliomas: role in tumor growth and progression. Oncotarget 7, 43654–43668. doi: 10.18632/oncotarget.9661
Morrone, F. B., Gehring, M. P., and Nicoletti, N. F. (2016). Calcium channels and associated receptors in malignant brain tumor therapy. Mol. Pharmacol. 90, 403–409. doi: 10.1124/mol.116.103770
Nabissi, M., Morelli, M. B., Amantini, C., Farfariello, V., Ricci-Vitiani, L., Caprodossi, S., et al. (2010). TRPV2 channel negatively controls glioma cell proliferation and resistance to Fas-induced apoptosis in ERK-dependent manner. Carcinogenesis 31, 794–803. doi: 10.1093/carcin/bgq019
Nabissi, M., Morelli, M. B., Amantini, C., Liberati, S., Santoni, M., Ricci-Vitiani, L., et al. (2015). Cannabidiol stimulates Aml-1a-dependent glial differentiation and inhibits glioma stem-like cells proliferation by inducing autophagy in a TRPV2-dependent manner. Int. J. Cancer 137, 1855–1869. doi: 10.1002/ijc.29573
Nabissi, M., Morelli, M. B., Arcella, A., Cardinali, C., Santoni, M., Bernardini, G., et al. (2016). Post-transcriptional regulation of 5’-untranslated regions of human transient receptor potential vanilloid type-1 (TRPV-1) channels: role in the survival of glioma patients. Oncotarget 7, 81541–81554. doi: 10.18632/oncotarget.13132
Nabissi, M., Morelli, M. B., Santoni, M., and Santoni, G. (2013). Triggering of the TRPV2 channel by cannabidiol sensitizes glioblastoma cells to cytotoxic chemotherapeutic agents. Carcinogenesis 34, 48–57. doi: 10.1093/carcin/bgs328
Nesin, V., and Tsiokas, L. (2014). TRPC1. Handb. Exp. Pharmacol. 222, 15–51. doi: 10.1007/978-3-642-54215-2_2
Nilius, B., and Owsianik, G. (2011). The transient receptor potential family of ion channels. Genome Biol. 12:218. doi: 10.1186/gb-2011-12-3-218
Ohmi, M., Shishido, Y., Inoue, T., Ando, K., Fujiuchi, A., Yamada, A., et al. (2014). Identification of a novel 2-pyridyl-benzensulfonamide derivative, RQ-00203078, as a selective and orally active TRPM8 antagonist. Bioorg. Med. Chem. Lett. 24, 5364–5368. doi: 10.1016/j.bmcl.2014.10.074
Olkkola, K. T., and Ahonen, J. (2008). Midazolam and other benzodiazepines. Handb. Exp. Pharmacol. 182, 335–360. doi: 10.1007/978-3-540-74806-9_16
Ostrom, Q. T., Gittleman, H., Liao, P., Vecchione-Koval, T., Wolinsky, Y., Kruchko, C., et al. (2017). CBTRUS Statistical Report: Primary brain and other central nervous system tumors diagnosed in the United States in 2010–2014. Neuro Oncol. 19(Suppl. 5) v1–v88. doi: 10.1093/neuonc/nox158
Parnas, M., Peters, M., Dadon, D., Lev, S., Vertkin, I., Slutsky, I., et al. (2009). Carvacrol is a novel inhibitor of Drosophila TRPL and mammalian TRPM7 channels. Cell Calcium 45, 300–309. doi: 10.1016/j.ceca.2008.11.009
Parsons, D. W., Jones, S., Zhang, X., Lin, J. C., Leary, R. J., Angenendt, P., et al. (2008). An integrated genomic analysis of human glioblastoma multiforme. Science 321, 1807–1812. doi: 10.1126/science.1164382
Patel, A. P., Tirosh, I., Trombetta, J. J., Shalek, A. K., Gillespie, S. M., Wakimoto, H., et al. (2014). Single-cell RNA-seq highlights intratumoral heterogeneity in primary glioblastoma. Science 344, 1396–1401. doi: 10.1126/science.1254257
Perálvarez-Marín, A., Doñate-Macian, P., and Gaudet, R. (2013). What do we know about the transient receptor potential vanilloid 2 (TRPV2) ion channel? FEBS J. 280, 5471–5487. doi: 10.1111/febs.12302
Pertwee, R. G., Thomas, A., Stevenson, L. A., Maor, Y., and Mechoulam, R. (2005). Evidence that (-)-7-hydroxy-4’-dimethylheptyl-cannabidiol activates a non-CB(1), non-CB(2), non-TRPV1 target in the mouse vas deferens. Neuropharmacology 48, 1139–1146. doi: 10.1016/j.neuropharm.2005.01.010
Prevarskaya, N., Skryma, R., and Shuba, Y. (2018). Ion channels in cancer: are cancer hallmarks oncochannelopathies? Physiol. Rev. 98, 559–621. doi: 10.1152/physrev.00044.2016
Prevarskaya, N., Zhang, L., and Barritt, G. (2007). TRP channels in cancer. Biochim. Biophys. Acta 1772, 937–946. doi: 10.1016/j.bbadis.2007.05.006
Putney, J. W. (2005). Physiological mechanisms of TRPC activation. Pflugers Arch. 451, 29–34. doi: 10.1007/s00424-005-1416-4
Ramsey, I. S., Delling, M., and Clapham, D. E. (2006). An introduction to TRP channels. Annu. Rev. Physiol. 68, 619–647. doi: 10.1146/annurev.physiol.68.040204.100431
Ransom, C. B., O’Neal, J. T., and Sontheimer, H. (2001). Volume-activated chloride currents contribute to the resting conductance and invasive migration of human glioma cells. J. Neurosci. 21, 7674–7683. doi: 10.1523/JNEUROSCI.21-19-07674
Richter, J. M., Schaefer, M., and Hill, K. (2014). Riluzole activates TRPC5 channels independently of PLC activity. Br. J. Pharmacol. 171, 158–170. doi: 10.1111/bph.12436
Rybarczyk, P., Gautier, M., Hague, F., Dhennin-Duthille, I., Chatelain, D., Kerr-Conte, J., et al. (2012). Transient receptor potential melastatin-related 7 channel is overexpressed in human pancreatic ductal adenocarcinomas and regulates human pancreatic cancer cell migration. Int. J. Cancer 131, E851–E861. doi: 10.1002/ijc.27487
Santoni, G., Amantini, C., Maggi, F., Marinelli, O., Santoni, M., Nabissi, M., et al. (2020a). The TRPV2 cation channels: from urothelial cancer invasiveness to glioblastoma multiforme interactome signature. Lab. Invest. 100, 186–198. doi: 10.1038/s41374-019-0333-7
Santoni, G., Morelli, M. B., Santoni, M., Nabissi, M., Marinelli, O., and Amantini, C. (2020b). Targeting transient receptor potential channels by MicroRNAs drives tumor development and progression. Adv. Exp. Med. Biol. 1131, 605–623. doi: 10.1007/978-3-030-12457-1_24
Sarfaraz, S., Adhami, V. M., Syed, D. N., Afaq, F., and Mukhtar, H. (2008). Cannabinoids for cancer treatment: progress and promise. Cancer Res. 68, 339–342. doi: 10.1158/0008-5472.CAN-07-2785
Schaefer, M. (2005). Homo- and heteromeric assembly of TRP channel subunits. Pflugers Arch. 451, 35–42. doi: 10.1007/s00424-005-1467-6
Schultz, S. (2018). GW Pharmaceuticals Plc Investor Presentation—February 2018. Available online at: http://ir.gwpharm.com/static-files/e7afbad8-ab2c4c8a-8e21-b9d3a7d36c70 (accessed May, 2018).
Schultz, S., and Beyer, M. (2017). GW Pharmaceuticals Achieves Positive Results in Phase 2 Proof of Concept Study in Glioma. Available online at: http://ir.gwpharm.com/static-files/cde942fe-555c-4b2f-9cc9-f34d24c7ad27 (accessed May, 2018).
Scott, K. A., Dalgleish, A. G., and Liu, W. M. (2014). The combination of cannabidiol and Δ9-tetrahydrocannabinol enhances the anticancer effects of radiation in an orthotopic murine glioma model. Mol. Cancer Ther. 13, 2955–2967. doi: 10.1158/1535-7163.MCT-14-0402
Singh, S. K., Hawkins, C., Clarke, I. D., Squire, J. A., Bayani, J., Hide, T., et al. (2004). Identification of human brain tumour initiating cells. Nature 432, 396–401. doi: 10.1038/nature03128
Solinas, M., Massi, P., Cinquina, V., Valenti, M., Bolognini, D., Gariboldi, M., et al. (2013). Cannabidiol, a non-psychoactive cannabinoid compound, inhibits proliferation and invasion in U87-MG and T98G glioma cells through a multitarget effect. PLoS One 8:e76918. doi: 10.1371/journal.pone.0076918
Song, M., Chen, D., and Yu, S. P. (2014). The TRPC channel blocker SKF 96365 inhibits glioblastoma cell growth by enhancing reverse mode of the Na(+) /Ca(2+) exchanger and increasing intracellular Ca(2+). Br. J. Pharmacol. 171, 3432–3447. doi: 10.1111/bph.12691
Soroceanu, L., Manning, T. J. Jr., and Sontheimer, H. (1999). Modulation of glioma cell migration and invasion using Cl(-) and K(+) ion channel blockers. J. Neurosci. 19, 5942–5954. doi: 10.1523/JNEUROSCI.19-14-05942
Sottoriva, A., Spiteri, I., Piccirillo, S. G., Touloumis, A., Collins, V. P., Marioni, J. C., et al. (2013). Intratumor heterogeneity in human glioblastoma reflects cancer evolutionary dynamics. Proc. Natl. Acad. Sci. U.S.A. 110, 4009–4014. doi: 10.1073/pnas.1219747110
Sours-Brothers, S., Ding, M., Graham, S., and Ma, R. (2009). Interaction between TRPC1/TRPC4 assembly and STIM1 contributes to store-operated Ca2+ entry in mesangial cells. Exp. Biol. Med. (Maywood) 234, 673–682. doi: 10.3181/0809-RM-279
Stock, K., Kumar, J., Synowitz, M., Petrosino, S., Imperatore, R., Smith, E. S., et al. (2012). Neural precursor cells induce cell death of high-grade astrocytomas through stimulation of TRPV1. Nat. Med. 18, 1232–1238. doi: 10.1038/nm.2827
Stupp, R., Hegi, M. E., Mason, W. P., van den Bent, M. J., Taphoorn, M. J., Janzer, R. C., et al. (2009). european organisation for research and treatment of cancer brain tumour and radiation oncology groups; national cancer institute of canada clinical trials group. Effects of radiotherapy with concomitant and adjuvant temozolomide versus radiotherapy alone on survival in glioblastoma in a randomised phase III study: 5-year analysis of the EORTC-NCIC trial. Lancet Oncol. 10, 459–466. doi: 10.1016/S1470-2045(09)70025-7
Sun, Y., Sukumaran, P., Varma, A., Derry, S., Sahmoun, A. E., and Singh, B. B. (2014). Cholesterol-induced activation of TRPM7 regulates cell proliferation, migration, and viability of human prostate cells. Biochim. Biophys. Acta 1843, 1839–1850. doi: 10.1016/j.bbamcr.2014.04.019
Tang, D., Kang, R., Berghe, T. V., Vandenabeele, P., and Kroemer, G. (2019). The molecular machinery of regulated cell death. Cell Res. 29, 347–364. doi: 10.1038/s41422-019-0164-5
Torres, S., Lorente, M., Rodríguez-Fornés, F., Hernández-Tiedra, S., Salazar, M., García-Taboada, E., et al. (2011). A combined preclinical therapy of cannabinoids and temozolomide against glioma. Mol. Cancer Ther. 10, 90–103. doi: 10.1158/1535-7163.MCT-10-0688
Toth, A., Blumberg, P. M., and Boczan, J. (2009). Anandamide and the vanilloid receptor (TRPV1). Vitam. Horm. 81, 389–419. doi: 10.1016/S0083-6729(09)81015-7
Tsavaler, L., Shapero, M. H., Morkowski, S., and Laus, R. (2001). Trp-p8, a novel prostate-specific gene, is up-regulated in prostate cancer and other malignancies and shares high homology with transient receptor potential calcium channel proteins. Cancer Res. 61, 3760–3769.
Vaccani, A., Massi, P., Colombo, A., Rubino, T., and Parolaro, D. (2005). Cannabidiol inhibits human glioma cell migration through a cannabinoid receptor-independent mechanism. Br. J. Pharmacol. 144, 1032–1036. doi: 10.1038/sj.bjp.0706134
Velasco, G., Carracedo, A., Blázquez, C., Lorente, M., Aguado, T., Haro, A., et al. (2007). Cannabinoids and gliomas. Mol. Neurobiol. 36, 60–67. doi: 10.1007/s12035-007-0002-5
Venkatachalam, K., and Montell, C. (2007). TRP channels. Annu. Rev. Biochem. 76, 387–417. doi: 10.1146/annurev.biochem.75.103004.142819
Venkatachalam, K., Hofmann, T., and Montell, C. (2006). Lysosomal localization of TRPML3 depends on TRPML2 and the mucolipidosis-associated protein TRPML1. J. Biol. Chem. 281, 17517–17527. doi: 10.1074/jbc.M600807200
Vergarajauregui, S., Martina, J. A., and Puertollano, R. (2009). Identification of the penta-EF-hand protein ALG-2 as a Ca2+-dependent interactor of mucolipin-1. J. Biol. Chem. 284, 36357–36366. doi: 10.1074/jbc.M109.047241
Wan, J., Guo, A. A., King, P., Guo, S., Saafir, T., Jiang, Y., et al. (2020). TRPM7 induces tumorigenesis and stemness through notch activation in glioma. Front. Pharmacol. 11:590723. doi: 10.3389/fphar.2020.590723
Wang, H., Cheng, X., Tian, J., Xiao, Y., Tian, T., Xu, F., et al. (2020). TRPC channels: Structure, function, regulation and recent advances in small molecular probes. Pharmacol. Ther. 209:107497. doi: 10.1016/j.pharmthera.2020.107497
Wick, W., Gorlia, T., Bendszus, M., Taphoorn, M., Sahm, F., Harting, I., et al. (2017). Lomustine and bevacizumab in progressive glioblastoma. N. Engl. J. Med. 377, 1954–1963. doi: 10.1056/NEJMoa1707358
Wondergem, R., and Bartley, J. (2009). Menthol increases human glioblastoma intracellular Ca2+, BK channel activity and cell migration. J. Biomed. Sci. 16:90. doi: 10.1186/1423-0127-16-90
Wondergem, R., Ecay, T. W., Mahieu, F., Owsianik, G., and Nilius, B. H. G. F. (2008). /SF and menthol increase human glioblastoma cell calcium and migration. Biochem. Biophys Res. Commun. 372, 210–215. doi: 10.1016/j.bbrc.2008.05.032
Yang, D., and Kim, J. (2020). Emerging role of transient receptor potential (TRP) channels in cancer progression. BMB Rep. 53, 125–132. doi: 10.5483/BMBRep.2020.53.3.016
Yee, N. S., Li, Q., Kazi, A. A., Yang, Z., Berg, A., and Yee, R. K. (2014). Aberrantly over-expressed TRPM8 channels in pancreatic adenocarcinoma: correlation with tumor size/stage and requirement for cancer cells invasion. Cells 3, 500–516. doi: 10.3390/cells3020500
Yu, S., Xu, Z., Zou, C., Wu, D., Wang, Y., Yao, X., et al. (2014). Ion channel TRPM8 promotes hypoxic growth of prostate cancer cells via an O2 -independent and RACK1-mediated mechanism of HIF-1α stabilization. J. Pathol. 234, 514–525. doi: 10.1002/path.4413
Zeng, J., Wu, Y., Zhuang, S., Qin, L., Hua, S., Mungur, R., et al. (2019). Identification of the role of TRPM8 in glioblastoma and its effect on proliferation, apoptosis and invasion of the U251 human glioblastoma cell line. Oncol. Rep. 42, 1517–1526. doi: 10.3892/or.2019.7260
Zhan, C., and Shi, Y. (2017). TRPC channels and cell proliferation. Adv. Exp. Med. Biol. 976, 149–155. doi: 10.1007/978-94-024-1088-4_13
Zhang, D., Wang, F., Pang, Y., Zhao, E., Zhu, S., Chen, F., et al. (2017). ALG2 regulates glioblastoma cell proliferation, migration and tumorigenicity. Biochem. Biophys. Res. Commun. 486, 300–306. doi: 10.1016/j.bbrc.2017.03.032
Keywords: malignant glioma, cell death, TRP ion channels, treatment, cannabidiol
Citation: Lefranc F (2021) Transient Receptor Potential (TRP) Ion Channels Involved in Malignant Glioma Cell Death and Therapeutic Perspectives. Front. Cell Dev. Biol. 9:618961. doi: 10.3389/fcell.2021.618961
Received: 19 October 2020; Accepted: 29 April 2021;
Published: 12 August 2021.
Edited by:
Giovanna Valenti, University of Bari Aldo Moro, ItalyReviewed by:
Ella L. Kim, Johannes Gutenberg University Mainz, GermanyFederica Barbieri, University of Genoa, Italy
Maria Beatrice Morelli, University of Camerino, Italy
Copyright © 2021 Lefranc. This is an open-access article distributed under the terms of the Creative Commons Attribution License (CC BY). The use, distribution or reproduction in other forums is permitted, provided the original author(s) and the copyright owner(s) are credited and that the original publication in this journal is cited, in accordance with accepted academic practice. No use, distribution or reproduction is permitted which does not comply with these terms.
*Correspondence: Florence Lefranc, RmxvcmVuY2UubGVmcmFuY0BlcmFzbWUudWxiLmFjLmJl