- Department of Gastroenterology, Hannover Medical School, Hannover, Germany
During the maturation of intestinal epithelial cells along the crypt/surface axis, a multitude of acid/base transporters are differentially expressed in their apical and basolateral membranes, enabling processes of electrolyte, macromolecule, nutrient, acid/base and fluid secretion, and absorption. An intracellular pH (pHi)-gradient is generated along the epithelial crypt/surface axis, either as a consequence of the sum of the ion transport activities or as a distinctly regulated entity. While the role of pHi on proliferation, migration, and tumorigenesis has been explored in cancer cells for some time, emerging evidence suggests an important role of the pHi in the intestinal stem cells (ISCs) proliferative rate under physiological conditions. The present review highlights the current state of knowledge about the potential regulatory role of pHi on intestinal proliferation and differentiation.
Introduction
Mechanisms of acid/base control in the gastrointestinal tract came into focus a century ago, because during the decades of very high gastric ulcer prevalence, a relationship between peptic ulcers and gastric acidity had been recognized (Banic et al., 2011). The ability to assess pHi in mammalian gastrointestinal cells with the use of fluorescent dyes (Thomas, 1986) made the study of pHi-recovery after an acidic or alkaline insult possible, as well as the delineation of the involved ion transporters (Flemstrom and Isenberg, 2001; Kaunitz and Akiba, 2006; Seidler, 2013). pHi measurements have also been utilized to outline the transport proteins involved in intestinal absorptive and secretory processes (Zachos et al., 2005; Hug et al., 2011; Seidler and Nikolovska, 2019).
In the apical membranes, the anion channel cystic fibrosis transmembrane conductance regulator (CFTR) and the Cl–/HCO3– exchanger SLC26A3 (and possibly SLC26A6) export HCO3– into the lumen and are therefore “acid loaders.” Likewise, proton-coupled nutrient transporters load the enterocytes with acid moieties during digestion. The activity of acid loaders is counteracted by the apical acid extruders, the Na+/H+ exchangers NHE2, NHE3, and NHE8 (SLC9A2/3/8); a process that results in salt and water absorption. Apical proton ATPases are also expressed in various cell types along the GI tract. The basolateral membranes also express both acid loaders, such as the Cl–/HCO3– exchanger AE2 (SLC4A2), and acid extruders, such as the NHE1 and the Na+/HCO3– cotransporters NBCn1 (SLC4A7) and NBCe1 (SLC4A4). Immunostaining and in situ hybridization techniques localized these transporters in the respective membranes, often with an expression gradient along cryptal or crypt/villus axis, and along the proximal to distal gut axis (Strong et al., 1994; Ameen et al., 1995; Ameen N. et al., 2000; Ameen N.A. et al., 2000; Alper et al., 1999; Chu et al., 2002; Jacob et al., 2002; Wang et al., 2002; Boedtkjer et al., 2008; Jakab et al., 2011; Singh et al., 2013b). Figure 1 depicts a colonic crypt, with experimentally determined pHi-gradient along its axis, and the relevant acid/base transporters on the apical and basolateral membranes.
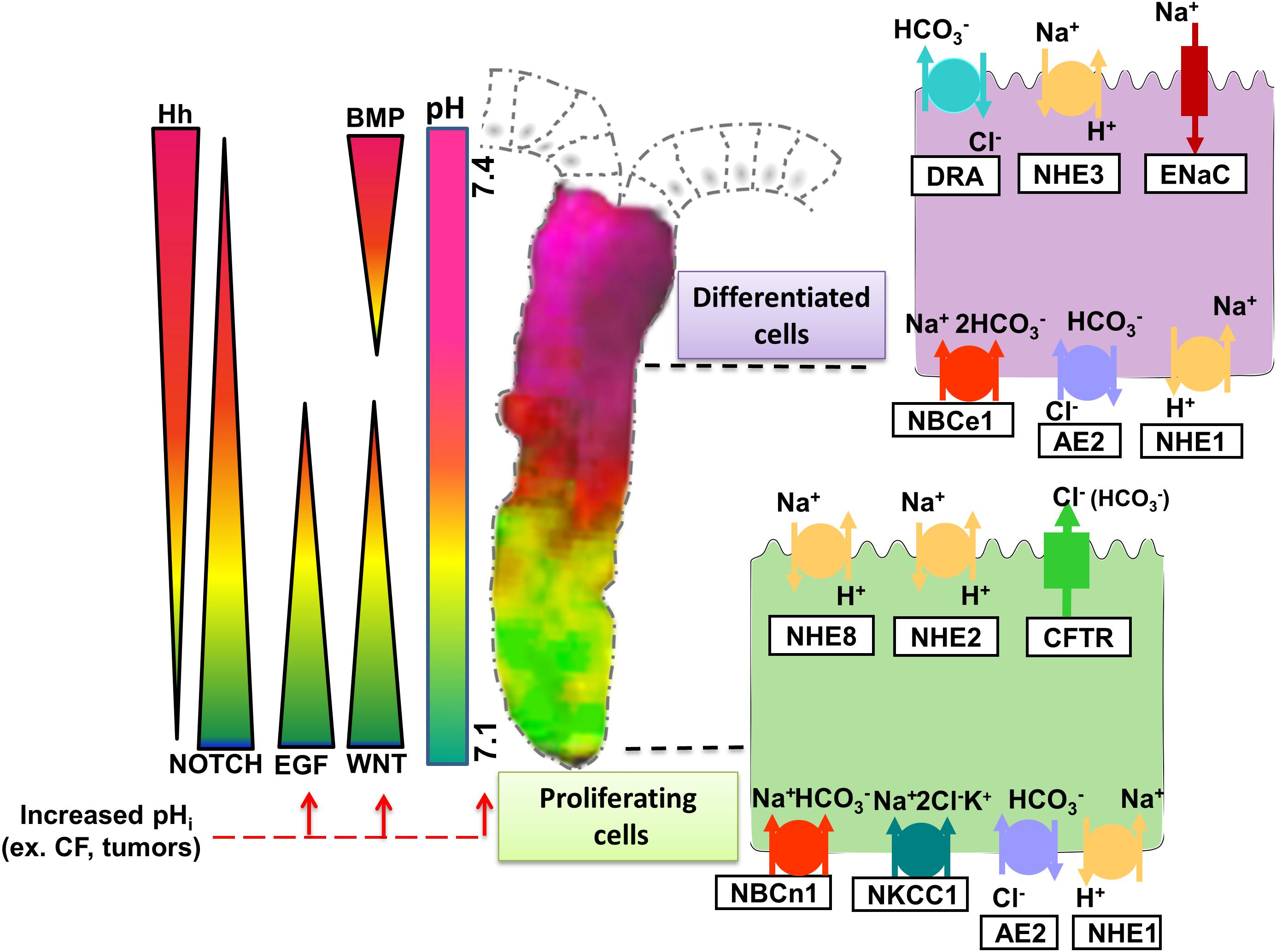
Figure 1. Representation of experimentally determined pHi gradient and the major signaling cascades in the colonic crypt that together control intestinal homeostasis. Wnt and EGF are essential for proliferation and maintenance of ISCs. Notch signaling targets the proliferating cells directing them to secretory or absorptive lineage differentiation. Hedgehog (Hh), expressed by epithelial cells in the upper part of the crypt maintains the myofibroblasts and prompts BMP ligand expression, which then promotes differentiation while restraining cell proliferation of the epithelial cells. Since they are involved in different steps of intestinal homeostasis, the signaling pathways form an opposing gradient along the crypt axis. The activity of the signaling pathways is influenced by a pHi gradient spread along the crypt, with more acidic pHi values present at the bottom and more alkaline values toward the cryptal surface. The pHi gradient is generated by the activity of the different ion transporters expressed on the intestinal epithelial cells. Proliferating cells are characterized by high activity/expression of CFTR, NHE2, and NHE8 on their apical membrane, and NBCn1, NKCC1, AE2, and NHE1 on the basolateral membrane. As the cells transition from proliferating to differentiated state, the expression of NHE3, DRA (Slc26a3), and ENaC becomes more dominant at the apical membrane and NBCe1, NHE1, and AE2 on the basolateral membrane. Tightly regulated expression and activity of the ion transporters at different segments of the crypt allows maintenance of distinct pHi values that in turn control the activity of the signaling pathways essential for synchronized proliferation and differentiation to retain intestinal homeostasis. Increase of pHi caused by alterations in ion transporter expression/activity (e.g., Cystic Fibrosis-CF) or tumors leads to activation of certain signaling pathways and hyperproliferation.
The role of the steady-state pHi in the cellular physiology of the intestinal epithelium has not been addressed experimentally, partially because of experimental uncertainties in the calibration process that allows conversion of the fluorometric intensity into an actual pHi value (O’Connor and Silver, 2013), and because of the short lifetime of isolated intestinal mucosal preparations. Recent progress toward preservation of functional intestinal stem cells and therefore cultivation of native intestinal epithelium in so-called “organoid cultures” has enabled scientists to observe cellular functions, including the pHi, of the intestinal epithelium in “steady-state.” This minireview highlights recent novel findings regarding the role of pHi in intestinal proliferation and discusses the potential role of pHi in the signaling pathways that regulate the constant renewal of the intestinal mucosa.
pHi-Regulatory Studies in the Intestinal Epithelium
Temporal changes in the pHi of epithelial cells in the GI tract are imposed physiologically due to changes in luminal pH. For example, the consequences of short-term exposure of the mouse gastric and duodenal epithelium to acidic luminal perfusate (mimicking the stage of gastric emptying), or of the colonic epithelium by short chain fatty acids (mimicking bacterial metabolism), have been studied in detail. The epithelium counteracts this intracellular acidification by activation and/or rapid trafficking of a variety of ion transporters to the brush border and basolateral membrane, facilitating proton extrusion, and HCO3– import to re-establish the resting pHi (Chu and Montrose, 1995; Akiba et al., 2001; Singh et al., 2013a). These processes are coordinated by a large array of neural, paracrine, and direct epithelial regulatory mechanisms (Smith et al., 2006; Singh et al., 2012; Takeuchi et al., 2012; Akiba and Kaunitz, 2014).
In contrast to transient pHi alterations, the consequences of sustained deviations from the resting pHi as a result of impaired ion transport have hardly been studied in the native intestinal epithelium. For decades, the role of steady-state pHi alterations has been addressed primarily in tumor cells. In cancer cells numerous H+ extrusion and base loading mechanisms are upregulated, which generally leads to an inverted transmembrane pH gradient, characterized by alkalization of intracellular pH and extracellular acidosis, which is considered a hallmark of cancer metabolism (Webb et al., 2011; Swietach et al., 2014; Pedersen et al., 2017; Flinck et al., 2018; Becker and Deitmer, 2020; Liu et al., 2020). In this scenario, both the high intracellular and the low extracellular pH contribute to the malignant behavior (Pillai et al., 2019; Boedtkjer and Pedersen, 2020). Early studies supporting a role of mitogenic activation of Na+/H+ exchange and intracellular alkalinization in proliferation of non-transformed cells, such as fibroblasts (Grinstein et al., 1989) has been met with criticism because a concomitant activation of acid loaders abolished the rise in pHi in fibroblasts in the presence of CO2/HCO3– in the medium (Gillies and Martinezzaguilan, 1991). Recent technological advances and an expanded knowledge about the molecular nature of acid/base transporters as well as the mechanisms of epithelial growth and differentiation allow addressing the question about the influence of pHi in epithelial homeostasis.
Alkaline Steady-State pHi Caused by Loss of the CFTR Channel in Intestinal Stem Cells (ISCs) Is a Causative Factor in ISC Hyperproliferation
Simpson et al. (2005) had identified an alkaline pHi in the intact epithelium of Cftr–/– compared to identically treated wild type (wt) mouse duodenal mucosa. When techniques became available for intestinal stem cell maintenance and thus long term culture of native intestinal organoids, the same group used the technique to demonstrate a sustained alkalized resting pHi in the epithelial cells of small intestinal organoids grown from Cftr–/– crypts (Walker et al., 2016). Interestingly, the group demonstrated that the alkaline pHi was not primarily due to the defective HCO3– conductance via CFTR, but to its defective Cl– conductance, resulting in intracellular Cl– retention and an inability of the basolateral acid loader AE2 to export HCO3–i in exchange for Cl–o. Accordingly, pHi could be normalized by normalizing [Cl–]i in Cftr–/– enterocytes (Walker et al., 2016). Employing an array of sophisticated methods, the group demonstrated an expression and functional activity of CFTR in murine ISCs, an alkaline intracellular pHi in Cftr–/– ISCs, accompanied by hyperproliferation in Cftr–/– organoids. These findings suggest that the Cftr–/–-associated crypt and villus elongations, which are also observed in the absence of inflammatory markers (Tan et al., 2020) and the hyperproliferation described in murine Cftr–/– intestinal epithelium (Gallagher and Gottlieb, 2001) may be partially a consequence of the lack of CFTR in ISCs. Crossbreeding of Cftr–/– and wt mice with transgenic mice which express a fluorophore (EGFP)-labeled WNT transducer Disheveled (Dsv) and the cell membrane-targeted, two-color fluorescent Cre-reporter RosaTmT/mG enabled the group to study the proximity of Dsv to the membrane receptor Frizzled 7, which has been recognized as a key event in WNT signaling (Axelrod, 2001) in Cftr–/– and wt ISCs with live cell imaging. Subjecting the organoids to manipulations that reduced inner membrane negative charge, [Cl]i or pHi, the authors established the causative role of pHi alkalinity for increased WNT signaling in Cftr–/– ISCs.
Loss of CFTR function in CF patients is associated with a significantly increased risk of developing digestive tract cancers, but not of lung cancers (Neglia et al., 1995; Maisonneuve et al., 2003, 2013; Scott et al., 2020). CFTR is expressed in ISCs, but not detectable in the progenitor basal cells of the respiratory mucosa (Plasschaert et al., 2018). Since both organs are subjected to the typical CF epithelial manifestations of dysbiosis, inflammation, and remodeling, the findings by Strubberg et al. (2018) may have identified an intrinsic factor favoring malignant growth in the CF intestinal epithelium.
Associations Between pHi and/or Acid/Base Transporters and Epithelial Morphogenesis in Native Intestinal Epithelium
From the existing literature pool, only the study done by Strubberg et al. (2018) provides a molecular mechanism linking steady-state pHi and proliferation in native epithelium. In a number of cellular systems, however, it is found that slightly alkaline pH (∼0.3 pH units above the steady-state pHi) is important for initiating DNA synthesis and proliferation [reviewed in Flinck et al. (2018)]. Here we report studies in native intestinal epithelium in which pHi and/or proliferation was measured, but the molecular mechanism linking the two has yet to be explored.
SLC4A4 (NBCe1) is expressed predominantly in small intestinal villous (Jakab et al., 2011) and colonic surface cells and NBCe1 KO proximal surface colonocytes have significantly reduced steady-state pHi compared to WT (Yu et al., 2016). Due to the short life span and the tiny intestine of these mice, the proliferation rate in the colon was not addressed, but a study with LS174 cells (human colonic adenocarcinoma cells) showed that SLC4A4 knockdown impaired cell proliferation (Parks and Pouyssegur, 2015). The Cl–/HCO3– exchanger Slc26a3, which mutation is the molecular cause of congenital chloride diarrhea, is mainly expressed in the colonic absorptive epithelial cells lining the luminal surface, not in the ISCs (Hoglund et al., 1996; Xiao et al., 2012). Loss of Slc26a3 function, in the colon leads to increased steady state pHi in the surface epithelium (Xiao et al., 2014). Colonic epithelial hyperplasia has been described in the original publication of the Slc26a3–/– mouse (Schweinfest et al., 2006). Possibly the alkaline pH interferes with intracellular acidification required to trigger programmed cell death at the colonic surface epithelium (Park et al., 1999; Kniep et al., 2006). Increased crypt length, seen in Cftr–/– epithelium, is not a feature of Slc26a3–/– colonic epithelium (Kini et al., 2020).
What about the effect of an acidic pHi on proliferation and differentiation? While many cell lines are viable in acidic medium, the pharmacological or genetic inhibition of acid extruders or base loaders generally curbs proliferation and has been repeatedly suggested as an antiproliferative treatment in tumors (Chambard and Pouyssegur, 1986). It was recently reported that a genetic deletion of an acid extruder, namely NHE8, in colonic ISC displays hyperproliferative phenotype, but the pHi in the affected cells was not measured (Xu et al., 2019).
Cellular Signaling Pathways That May Link pHi to Proliferation and Differentiation
Regeneration, expansion and lineage differentiation of the intestinal epithelium is modulated by various signaling pathways, namely Wnt/β-catenin, EGF (epidermal growth factor), BMP (bone morphogenetic protein), Notch, Hedgehog, and Eph–ephrin which mainly occur in gradients along the crypt/villus axis as depicted in Figure 1 (Spit et al., 2018). These signaling cascades are derived from the epithelial or the mesenchymal niche (Spit et al., 2018). A potential cross-interaction between the gradients of signaling pathways and the intracellular pH gradient along the crypt/villus axis might exist, but is understudied. In this paragraph we point out important signaling pathways for proliferation and differentiation in which a relationship to pHi has been delineated in other cellular system, and which are worth studying in the native intestinal epithelium.
Wnt signaling is the main driving force of ISC proliferation. Increased Wnt/β-catenin signaling leads to hyperproliferation observed in Cftr–/– ISCs as described above. The molecular mechanism behind the increased Wnt activity involves the alkaline pHi-facilitated association of the Wnt transducer Disheveled (Dvl) to the plasma membrane and binding to the Frizzled-7 receptor (Fz) (Walker et al., 2016; Strubberg et al., 2018). Similarly, a study in Drosophila melanogaster cells shows that the activity of dNhe2 (a Drosophila analog of the mammalian NHE1), which allows maintenance of alkaline pHi, is necessary for the binding and surface recruitment of Dvl by Fz (Simons et al., 2009). Another component of the Wnt signaling pathway, β-catenin is also influenced by the pHi. Increasing the pHi by glycolysis stimulates β-catenin acetylation leading to Wnt signaling activation in embryos and human tail bud-like cells differentiated in vitro from iPS cells (Oginuma et al., 2020). Intracellular acidification induces the transcriptional repressor DDIT3 that suppresses the activity of Wnt, as shown in vitro and in a mouse xenograft tumor model (Melnik et al., 2018). However, another group has shown that cell alkalization with NH4Cl in MDCK epithelial cells and Drosophila melanogaster led to decreased β-catenin abundance at cell–cell junctions and in the nucleus (White et al., 2018), while lower pHi in NHE1-deficient PS120 fibroblasts significantly increased β-catenin at membrane protrusions (White et al., 2018). Recently the involvement of potassium channels, namely KCNQ1, in the Wnt/β-catenin signaling pathway has been shown. A colocalization of KCNQ1and β-catenin at the adherence junctions was detected in rat colonic crypts and HT29 cells (Rapetti-Mauss et al., 2017) and KCNQ1 inhibition leads to re-localization of β-catenin in the cytosol, Wnt/β-catenin signaling pathway stimulation with increased expression of Cyclin D1 and C-Jun as Wnt target genes (Rapetti-Mauss et al., 2017, 2020). Although, pHi is not directly implied, K+ channels allow hyperpolarization of the membrane voltage, thus contributing indirectly to pH regulation (Spitzner et al., 2007).
EGF signaling is another important modulator of ISC proliferation. EGF is produced by the adjacent fibroblasts and Paneth cells (Sato et al., 2011; Farin et al., 2014), and activates the signaling cascade by binding to the EGF receptor (EGFR) on ISCs. Extracellular pH influences the binding of EGF to its receptor, with maximized binding at pH8 and reduced interaction at pH6.5 (Nunez et al., 1993). Early research showed that EGF can increase pHi in A431 human epidermoid carcinoma cell line (Rothenberg et al., 1983). Later investigation in chicken granulosa cells (Li et al., 1991), Hep G2 hepatoma cells (Strazzabosco et al., 1995), and in primary cultured rabbit surface epithelial cells (Nylander-Koski et al., 2006) showed that EGF induced intracellular alkalization occurs via activation of NHE1. These data point that an alkaline pHi shift, caused by activation of NHE1 on the basolateral membrane, is an important event in EGF signaling pathway that stimulates cell proliferation. Indeed, EGFR forms a complex with NHE1 via NHERF1 (Cardone et al., 2015). Apical EGF can also activate EGFR signaling and promote proliferation similarly to basolateraly induced EGFR activation (Kuwada et al., 1998). EGF impacts the apical NHEs, it stimulates NHE2 mRNA expression and activity in rat intestinal epithelial (RIE) cell (Xu et al., 2001), but it has a negative effect on NHE8 expression (Xu et al., 2010). The interplay between apical or basolateral EGFR activation, different NHEs, and the pHi is not completely understood. It seems plausible that a constant slightly acidic pHi in the ISC zone may prevent hyperactivation of the signaling pathways that could result in hyperproliferation and possible tumor formation (Liu et al., 2020).
BMP, Notch, Hh, and Eph are more dominant in the upper sections of the crypt and involved in cell fate decision and terminal differentiation. Knowledge about the impact of the pHi on the later signaling cascades is scarce. The activation of the Notch signaling involves binding of the ligands to the receptor, and subsequent activation of the endocytosis machinery and this later step is influenced by the vacuolar (H+)-ATPase (V-ATPase), a proton transporter involved in the acidification of endosomal compartments (Yan et al., 2009). Notch signaling plays an important role in the determination of cell fate by regulating the balance between cell proliferation and differentiation (Baron, 2003), thus impacting the transit amplifying cells. Therefore, there is a high possibility that the Notch signaling can be affected by the activity of ion transporters present in the transit amplifying cells, such as NHE2 (Guan et al., 2006) via fine-tuning the pHi value. A shift in the pHi toward more alkaline values (from 7.4 to a 7.65) has been observed in mouse embryonic stem cells during their differentiation in vitro (Ulmschneider et al., 2016). Hh signaling, important for the follicle stem cells differentiation in Drosophila, is also strongly influenced by pHi alterations.
The reported studies are only examples, which, taken together, suggest that the pHi may trigger stimulation or inhibition of different signaling pathways active in proliferation and differentiation of the intestinal epithelium. However, the exact molecular mechanism correlating the pHi and signaling pathways gradient in the intestinal epithelium is yet to be determined.
Conclusion and Outlook
Addressing the role of the steady-state pHi in intestinal epithelial homeostasis has been hampered by the absence of models that accurately assess the pHi in different epithelial compartments along the crypt-villus axis, and to induce long-term and selective pHi-alterations. The ability to generate intestinal organoids and monitor their growth over days in culture has enabled scientists to intensely study the process of intestinal cell renewal and differentiation. Some of the molecular events that link the elevated pHi secondary to loss of functional CFTR to intestinal epithelial hyperproliferation were elegantly explored by generating intestinal organoids from Cftr–/– and wt mice crossed onto a variety of transgenic reporter mouse lines (Strubberg et al., 2018). These seminal studies may provide clarification of the increased incidence of colorectal cancer in CF patients and contribute toward their prevention. Recent progress in the drug development for CFTR corrector and modifiers with the potential to rescue CFTR function in CF patients may correct the pHi-regulatory dysfunction and reduce cancer risk (Phuan et al., 2019; Egan, 2020). The potential to combine direct pHi assessment with genetic, molecular biological and pharmacological tools, as already established for tumor cells (Liu et al., 2020; Stock, 2020), in intestinal organoids may provide insight into protonation/deprotonation events of key regulatory proteins in enterocyte proliferation and differentiation.
Author Contributions
MA, US, and KN designed and wrote the review. All the authors contributed to the article and approved the submitted version.
Funding
The work on this review was supported by the grant DFG Se460/21-1 and FOR5046/P7 to US.
Conflict of Interest
The authors declare that the research was conducted in the absence of any commercial or financial relationships that could be construed as a potential conflict of interest.
Abbreviations
ISCs, intestinal stem cells; CFTR, cystic fibrosis transmembrane conductance regulator; SLC26A3, Solute Carrier Family 26 Member 3 (Cl–/HCO3– exchangers); NHE1/2/3/8 (SLC9A), sodium/hydrogen exchangers 1/2/3/8; AE2 (SLC4A2), Anion exchange protein 2; NBCn1 (SLC4A7), Electroneutral sodium/bicarbonate-dependent cotransporter; NBCn1 (SLC4A4), Electrogenic sodium/bicarbonate cotransporter; GI, gastrointestinal; WT, wild type; KO, knock out; CF, cystic fibrosis; EGF, epidermal growth factor; BMP, bone morphogenetic protein; Dvl, Disheveled; Fz, Frizzled receptor; Hh, Hedgehog signaling.
References
Akiba, Y., Furukawa, O., Guth, P. H., Engel, E., Nastaskin, I., Sassani, P., et al. (2001). Cellular bicarbonate protects rat duodenal mucosa from acid-induced injury. J. Clin. Invest. 108, 1807–1816. doi: 10.1172/jci200112218
Akiba, Y., and Kaunitz, J. D. (2014). Prostaglandin pathways in duodenal chemosensing. J. Gastroenterol. Hepatol. 29, 93–98. doi: 10.1111/jgh.12731
Alper, S. L., Rossmann, H., Wilhelm, S., Stuart-Tilley, A. K., Shmukler, B. E., and Seidler, U. (1999). Expression of AE2 anion exchanger in mouse intestine. Am. J. Physiol. 277, G321–G332.
Ameen, N., Alexis, J., and Salas, P. (2000). Cellular localization of the cystic fibrosis transmembrane conductance regulator in mouse intestinal tract. Histochem. Cell Biol. 114, 69–75. doi: 10.1007/s004180000164
Ameen, N. A., van Donselaar, E., Posthuma, G., de Jonge, H., McLaughlin, G., Geuze, H. J., et al. (2000). Subcellular distribution of CFTR in rat intestine supports a physiologic role for CFTR regulation by vesicle traffic. Histochem. Cell Biol. 114, 219–228. doi: 10.1007/s004180000167
Ameen, N. A., Ardito, T., Kashgarian, M., and Marino, C. R. (1995). A unique subset of rat and human intestinal villus cells express the cystic fibrosis transmembrane conductance regulator. Gastroenterology 108, 1016–1023. doi: 10.1016/0016-5085(95)90198-1
Axelrod, J. D. (2001). Unipolar membrane association of dishevelled mediates frizzled planar cell polarity signaling. Genes Dev. 15, 1182–1187.
Banic, M., Malfertheiner, P., Babic, Z., Ostojic, R., Kujundzic, M., Fatovic-Ferencic, S., et al. (2011). Historical impact to drive research in peptic ulcer disease. Dig. Dis. 29, 444–453. doi: 10.1159/000331512
Baron, M. (2003). An overview of the Notch signalling pathway. Semin Cell Dev. Biol. 14, 113–119. doi: 10.1016/s1084-9521(02)00179-9
Becker, H. M., and Deitmer, J. W. (2020). Transport metabolons and acid/base balance in tumor cells. Cancers 12:899. doi: 10.3390/cancers12040899
Boedtkjer, E., and Pedersen, S. F. (2020). The acidic tumor microenvironment as a driver of cancer. in: annual review of physiology. Palo Alto Annu. Rev. 82, 103–126. doi: 10.1146/annurev-physiol-021119-034627
Boedtkjer, E., Praetorius, J., Fuchtbauer, E. M., and Aalkjaer, C. (2008). Antibody-independent localization of the electroneutral Na+-HCO3- cotransporter NBCn1 (slc4a7) in mice. Am. J. Physiol. Cell Physiol. 294, C591–C603.
Cardone, R. A., Greco, M. R., Zeeberg, K., Zaccagnino, A., Saccomano, M., Bellizzi, A., et al. (2015). A novel NHE1-Centered signaling cassette drives epidermal growth factor receptor-dependent pancreatic tumor metastasis and is a target for combination therapy. Neoplasia 17, 155–166. doi: 10.1016/j.neo.2014.12.003
Chambard, J. C., and Pouyssegur, J. (1986). Intracellular pH controls growth factor-induced ribosomal-protein S6-phosphorylation and protein-synthesis in the GO- G1-transition of fibroblasts. Exp. Cell Res. 164, 282–294. doi: 10.1016/0014-4827(86)90029-7
Chu, J. S., Chu, S. Y., and Montrose, M. H. (2002). Apical Na+/H+ exchange near the base of mouse colonic crypts. Am. J. Physiol. Cell Physiol. 283, C358–C372.
Chu, S. C., and Montrose, M. H. (1995). An Na+-independent short-chain fatty-acid transporter contributes to intracellular pH regulation in murine colonocytes. J. Gen. Physiol. 105, 589–615. doi: 10.1085/jgp.105.5.589
Egan, M. E. (2020). Cystic fibrosis transmembrane conductance receptor modulator therapy in cystic fibrosis, an update. Curr. Opin. Pediatr. 32, 384–388. doi: 10.1097/mop.0000000000000892
Farin, H. F., Karthaus, W. R., Kujala, P., Rakhshandehroo, M., Schwank, G., Vries, R. G. J., et al. (2014). Paneth cell extrusion and release of antimicrobial products is directly controlled by immune cell–derived IFN-γ. J. Exp. Med. 211, 1393–1405. doi: 10.1084/jem.20130753
Flemstrom, G., and Isenberg, J. I. (2001). Gastroduodenal mucosal alkaline secretion and mucosal protection. News Physiol. Sci. 16, 23–28. doi: 10.1152/physiologyonline.2001.16.1.23
Flinck, M., Kramer, S. H., and Pedersen, S. F. (2018). Roles of pH in control of cell proliferation. Acta Physiol. 223:e13068. doi: 10.1111/apha.13068
Gallagher, A. M., and Gottlieb, R. A. (2001). Proliferation, not apoptosis, alters epithelial cell migration in small intestine of CFTR null mice. Am. J. Physiol. Gastrointest. Liver Physiol. 281, G681–G687.
Gillies, R. J., and Martinezzaguilan, R. (1991). Regulation of intracellular pH in BALB/C 3T3 cells - bicarbonate raises pH via NAHCO3/HCL exchange and attenuates the activation of NA+/H+ exchange by serum. J. Biol. Chem. 266, 1551–1556. doi: 10.1016/s0021-9258(18)52329-2
Grinstein, S., Rotin, D., and Mason, M. J. (1989). NA+/H+ exchange and growth factor-induced cytosolic pH changes - role in cellular proliferation. Biochim. Biophys. Acta 988, 73–97. doi: 10.1016/0304-4157(89)90004-x
Guan, Y., Dong, J., Tackett, L., Meyer, J. W., Shull, G. E., and Montrose, M. H. (2006). NHE2 is the main apical NHE in mouse colonic crypts but an alternative Na+-dependent acid extrusion mechanism is upregulated in NHE2-null mice. Am. J. Physiol. Gastrointest. Liver Physiol. 291, G689–G699.
Hoglund, P., Haila, S., Socha, J., Tomaszewski, L., Saarialho-Kere, U., Karjalainen-Lindsberg, M. L., et al. (1996). Mutations of the Down-regulated in adenoma (DRA) gene cause congenital chloride diarrhoea. Nat. Genet. 14, 316–319. doi: 10.1038/ng1196-316
Hug, M. J., Clarke, L. L., and Gray, M. A. (2011). How to measure CFTR-dependent bicarbonate transport: from single channels to the intact epithelium. Methods Mol. Biol. 741, 489–509. doi: 10.1007/978-1-61779-117-8_30
Jacob, P., Rossmann, H., Lamprecht, G., Kretz, A., Neff, C., Lin-Wu, E., et al. (2002). Down-regulated in adenoma mediates apical Cl-/HCO3- exchange in rabbit, rat, and human duodenum. Gastroenterology 122, 709–724. doi: 10.1053/gast.2002.31875
Jakab, R. L., Collaco, A. M., and Ameen, N. A. (2011). Physiological relevance of cell-specific distribution patterns of CFTR, NKCC1, NBCe1, and NHE3 along the crypt-villus axis in the intestine. Am. J. Physiol. Gastroint. Liver Physiol. 300, G82–G98.
Kaunitz, J. D., and Akiba, Y. (2006). Review article: duodenal bicarbonate - mucosal protection, luminal chemosensing and acid-base balance. Alimen. Pharmacol. Ther. 24, 169–176. doi: 10.1111/j.1365-2036.2006.00041.x
Kini, A., Singh, A. K., Riederer, B., Yang, I., Tan, X. J., di Stefano, G., et al. (2020). Slc26a3 deletion alters pH-microclimate, mucin biosynthesis, microbiome composition and increases theTNF alpha expression in murine colon. Acta Physiol. 230:e13498. doi: 10.1111/apha.13498
Kniep, E. M., Roehlecke, C., Ozkucur, N., Steinberg, A., Reber, F., Knels, L., et al. (2006). Inhibition of apoptosis and reduction of intracellular pH decrease in retinal neural cell cultures by a blocker of carbonic anhydrase. Invest. Ophthalmol. Vis. Sci. 47, 1185–1192. doi: 10.1167/iovs.05-0555
Kuwada, S. K., Lund, K. A., Li, X. F., Cliften, P., Amsler, K., Opresko, L. K., et al. (1998). Differential signaling and regulation of apical vs. basolateral EGFR in polarized epithelial cells. Am. J. Physiol. Cell Physiol. 275, C1419–C1428.
Li, M., Morley, P., Asem, E. K., and Tsang, B. K. (1991). Epidermal growth factor elevates intracellular pH in chicken granulosa cells. Endocrinology 129, 656–662. doi: 10.1210/endo-129-2-656
Liu, Y., White, K. A., and Barber, D. L. (2020). Intracellular pH regulates cancer and stem cell behaviors: a protein dynamics perspective. Front. Oncol. 10:401. doi: 10.3389/fonc.2020.01401
Maisonneuve, P., FitzSimmons, S. C., Neglia, J. P., Campbell, P. W., and Lowenfels, A. B. (2003). Cancer risk in nontransplanted and transplanted cystic fibrosis patients: a 10-year study. JNCI Natl. Cancer Inst. 95, 381–387. doi: 10.1093/jnci/95.5.381
Maisonneuve, P., Marshall, B. C., Knapp, E. A., and Lowenfels, A. B. (2013). Cancer risk in cystic fibrosis: a 20-year nationwide study from the United States. JNCI Natl. Cancer Inst. 105, 122–129. doi: 10.1093/jnci/djs481
Melnik, S., Dvornikov, D., Muller-Decker, K., Depner, S., Stannek, P., Meister, M., et al. (2018). Cancer cell specific inhibition of Wnt/beta-catenin signaling by forced intracellular acidification. Cell Discov. 4:37.
Neglia, J. P., Fitzsimmons, S. C., Maisonneuve, P., Schoni, M. H., Schoniaffolter, F., Corey, M., et al. (1995). The risk of cancer among patients with cystic-fibrosis. N. Engl. J. Med. 332, 494–499.
Nunez, M., Mayo, K. H., Starbuck, C., and Lauffenburger, D. (1993). pH sensitivity of epidermal growth factor receptor complexes. J. Cell Biochem. 51, 312–321. doi: 10.1002/jcb.240510310
Nylander-Koski, O., Mustonen, H., Puolakkainen, P., Kiviluoto, T., and Kivilaakso, E. (2006). Epidermal growth factor enhances intracellular pH regulation via calcium signaling in acid-exposed primary cultured rabbit gastric epithelial cells. Digest. Dis. Sci. 51, 1322–1330. doi: 10.1007/s10620-006-9075-7
O’Connor, N., and Silver, R. B. (2013). Ratio Imaging: Practical Considerations for Measuring Intracellular Ca2+ and pH in Living Cells. In: Digital Microscopy, 4th Edn. San Diego, CA: Elsevier Academic Press Inc, 387–406.
Oginuma, M., Harima, Y., Tarazona, O. A., Diaz-Cuadros, M., Michaut, A., Ishitani, T., et al. (2020). Intracellular pH controls WNT downstream of glycolysis in amniote embryos. Nature 584, 98–101. doi: 10.1038/s41586-020-2428-0
Park, H. J., Lyons, J. C., Ohtsubo, T., and Song, C. W. (1999). Acidic environment causes apoptosis by increasing caspase activity. Br. J. Cancer 80, 1892–1897. doi: 10.1038/sj.bjc.6690617
Parks, S. K., and Pouyssegur, J. (2015). The Na+/HCO3- Co-Transporter SLC4A4 plays a role in growth and migration of colon and breast cancer cells. J. Cell. Physiol. 230, 1954–1963. doi: 10.1002/jcp.24930
Pedersen, S. F., Novak, I., Alves, F., Schwab, A., and Pardo, L. A. (2017). Alternating pH landscapes shape epithelial cancer initiation and progression: focus on pancreatic cancer. Bioessays 39:1600253. doi: 10.1002/bies.201600253
Phuan, P. W., Tan, J. A., Rivera, A. A., Zlock, L., Nielson, D. W., Finkbeiner, W. E., et al. (2019). Nanomolar-potency ‘co-potentiator’ therapy for cystic fibrosis caused by a defined subset of minimal function CFTR mutants. Sci. Rep. 9:17640.
Pillai, S. R., Damaghi, M., Marunaka, Y., Spugnini, E. P., Fais, S., and Gillies, R. J. (2019). Causes, consequences, and therapy of tumors acidosis. Cancer Metastasis Rev. 38, 205–222. doi: 10.1007/s10555-019-09792-7
Plasschaert, L. W., Zilionis, R., Choo-Wing, R., Savova, V., Knehr, J., Roma, G., et al. (2018). A single-cell atlas of the airway epithelium reveals the CFTR-rich pulmonary ionocyte. Nature 560, 377–381. doi: 10.1038/s41586-018-0394-6
Rapetti-Mauss, R., Berenguier, C., Allegrini, B., and Soriani, O. (2020). Interplay between ion channels and the wnt/beta-catenin signaling pathway in cancers. Front. Pharmacol. 11:525020. doi: 10.3389/fphar.2020.525020
Rapetti-Mauss, R., Bustos, V., Thomas, W., McBryan, J., Harvey, H., Lajczak, N., et al. (2017). Bidirectional KCNQ1:beta-catenin interaction drives colorectal cancer cell differentiation. Proc. Natl. Acad. Sci. U.S.A. 114, 4159–4164. doi: 10.1073/pnas.1702913114
Rothenberg, P., Glaser, L., Schlesinger, P., and Cassel, D. (1983). Activation of Na+/H+ exchange by epidermal growth factor elevates intracellular pH in A431 cells. J. Biol. Chem. 258, 12644–12653. doi: 10.1016/s0021-9258(17)44225-6
Sato, T., van Es, J. H., Snippert, H. J., Stange, D. E., Vries, R. G., van den Born, M., et al. (2011). Paneth cells constitute the niche for Lgr5 stem cells in intestinal crypts. Nature 469, 415–418. doi: 10.1038/nature09637
Schweinfest, C. W., Spyropoulos, D. D., Henderson, K. W., Kim, J. H., Chapman, J. M., Barone, S., et al. (2006). slc26a3 (dra)-deficient mice display chloride-losing diarrhea, enhanced colonic proliferation, and distinct up-regulation of ion transporters in the colon. J. Biol. Chem. 281, 37962–37971. doi: 10.1074/jbc.m607527200
Scott, P., Anderson, K., Singhania, M., and Cormier, R. (2020). Cystic Fibrosis. CFTR, and colorectal cancer. Int. J. Mol. Sci. 21:2891. doi: 10.3390/ijms21082891
Seidler, U., and Nikolovska, K. (2019). Slc26 family of anion transporters in the gastrointestinal tract: expression, function, regulation, and role in disease. Compr. Physiol. 9, 839–872. doi: 10.1002/cphy.c180027
Seidler, U. E. (2013). Gastrointestinal HCO3- transport and epithelial protection in the gut: new techniques, transport pathways and regulatory pathways. Curr. Opin. Pharmacol. 13, 900–908. doi: 10.1016/j.coph.2013.10.001
Simons, M., Gault, W. J., Gotthardt, D., Rohatgi, R., Klein, T. J., Shao, Y. M., et al. (2009). Electrochemical cues regulate assembly of the Frizzled/Dishevelled complex at the plasma membrane during planar epithelial polarization. Nat. Cell Biol. 11, 286–U142.
Simpson, J. E., Gawenis, L. R., Walker, N. M., Boyle, K. T., and Clarke, L. L. (2005). Chloride conductance of CFTR facilitates basal Cl-/HCO3- exchange in the villous epithelium of intact murine duodenum. Am. J. Physiol. Gastroint. Liver Physiol. 288, G1241–G1251.
Singh, A. K., Liu, Y. J., Riederer, B., Engelhardt, R., Thakur, B. K., Soleimani, M., et al. (2013a). Molecular transport machinery involved in orchestrating luminal acid-induced duodenal bicarbonate secretion in vivo. J. Physiol. Lond. 591, 5377–5391. doi: 10.1113/jphysiol.2013.254854
Singh, A. K., Xia, W. L., Riederer, B., Juric, M., Li, J. H., Zheng, W., et al. (2013b). Essential role of the electroneutral Na+HCO3 cotransporter NBCn1 in murine duodenal acidbase balance and colonic mucus layer build-up in vivo. J. Physiol. Lond. 591, 2189–2204. doi: 10.1113/jphysiol.2012.247874
Singh, A. K., Spiessberger, B., Zheng, W., Xiao, F., Lukowski, R., Wegener, J. W., et al. (2012). Neuronal cGMP kinase I is essential for stimulation of duodenal bicarbonate secretion by luminal acid. FASEB J. 26, 1745–1754. doi: 10.1096/fj.11-200394
Smith, A. J., Chappell, A. E., Buret, A. G., Barrett, K. E., and Dong, H. (2006). 5-hydroxytryptamine contributes significantly to a reflex pathway by which the duodenal mucosa protects itself from gastric acid injury. FASEB J. 20, 2486–2495. doi: 10.1096/fj.06-6391com
Spit, M., Koo, B.-K., and Maurice, M. M. (2018). Tales from the crypt: intestinal niche signals in tissue renewal, plasticity and cancer. Open Biol. 8:180120. doi: 10.1098/rsob.180120
Spitzner, M., Ousingsawat, J., Scheidt, K., Kunzelmann, K., and Schreiber, R. (2007). Voltage-gated K+ channels support proliferation of colonic carcinoma cells. FASEB J. 21, 35–44. doi: 10.1096/fj.06-6200com
Stock, C. (2020). How dysregulated ion channels and transporters take a hand in esophageal, liver, and colorectal cancer. Rev. Physiol. Biochem. Pharmacol. [Epub ahead of print].
Strazzabosco, M., Poci, C., Spirlì, C., Zsembery, A., Granato, A., Massimino, M. L., et al. (1995). Intracellular pH regulation in Hep G2 cells: effects of epidermal growth factor, transforming growth factor-alpha, and insulinlike growth factor-II on Na+/H+ exchange activity. Hepatology 22, 588–597. doi: 10.1016/0270-9139(95)90584-7
Strong, T. V., Boehm, K., and Collins, F. S. (1994). Localization of cystic fibrosis transmembrane conductance regulator mRNA in the human gastrointestinal tract by in situ hybridization. J. Clin. Invest. 93, 347–354. doi: 10.1172/jci116966
Strubberg, A. M., Liu, J. H., Walker, N. M., Stefanski, C. D., MacLeod, R. J., Magness, S. T., et al. (2018). Cftr Modulates Wnt/beta-catenin signaling and stem cell proliferation in murine intestine. Cell. Mol. Gastroenterol. Hepatol. 5, 253–271. doi: 10.1016/j.jcmgh.2017.11.013
Swietach, P., Vaughan-Jones, R. D., Harris, A. L., and Hulikova, A. (2014). The chemistry, physiology and pathology of pH in cancer. Philos. Trans. R. Soc. B Biol. Sci. 369:20130099. doi: 10.1098/rstb.2013.0099
Takeuchi, K., Aihara, E., Kimura, M., Dogishi, K., Hara, T., and Hayashi, S. (2012). Gas mediators involved in modulating duodenal HCO3- secretion. Curr. Med. Chem. 19, 43–54.
Tan, Q., di Stefano, G., Tan, X., Renjie, X., Römermann, D., Talbot, S. R., et al. (2020). Linaclotide, lubiprostone and tenapanor improve gut fluidity and alkalinity in CFTR-deficient and F508del mutant mice via NHE3 inhibition. Br. J. Pharmacol. [Epub ahead of print].
Ulmschneider, B., Grillo-Hill, B. K., Benitez, M., Azimova, D. R., Barber, D. L., and Nystul, T. G. (2016). Increased intracellular pH is necessary for adult epithelial and embryonic stem cell differentiation. J. Cell Biol. 215, 345–355. doi: 10.1083/jcb.201606042
Walker, N. M., Liu, J. H., Stein, S. R., Stefanski, C. D., Strubberg, A. M., and Clarke, L. L. (2016). Cellular chloride and bicarbonate retention alters intracellular pH regulation in Cftr KO crypt epithelium. Am. J. Physiol. Gastroint. Liver Physiol. 310, G70–G80.
Wang, Z. H., Petrovic, S., Mann, E., and Soleimani, M. (2002). Identification of an apical Cl-/HCO3- exchanger in the small intestine. Am. J. Physiol. Gastroint. Liver Physiol. 282, G573–G579.
Webb, B. A., Chimenti, M., Jacobson, M. P., and Barber, D. L. (2011). Dysregulated pH: a perfect storm for cancer progression. Nat. Rev. Cancer 11, 671–677. doi: 10.1038/nrc3110
White, K. A., Grillo-Hill, B. K., Esquivel, M., Peralta, J., Bui, V. N., Chire, I., et al. (2018). beta-Catenin is a pH sensor with decreased stability at higher intracellular pH. J. Cell Biol. 217, 3965–3976. doi: 10.1083/jcb.201712041
Xiao, F., Juric, M., Li, J. H., Riederer, B., Yeruva, S., Singh, A. K., et al. (2012). Loss of downregulated in adenoma (DRA) impairs mucosal HCO3-secretion in murine ileocolonic inflammation. Inflammatory Bowel Dis. 18, 101–111. doi: 10.1002/ibd.21744
Xiao, F., Yu, Q., Li, J., Johansson, M. E. V., Singh, A. K., Xia, W., et al. (2014). Slc26a3 deficiency is associated with loss of colonic HCO3- secretion, absence of a firm mucus layer and barrier impairment in mice. Acta Physiol. 211, 161–175. doi: 10.1111/apha.12220
Xu, H., Collins, J. F., Bai, L. Q., Kiela, P. R., Lynch, R. M., and Ghishan, F. K. (2001). Epidermal growth factor regulation of rat NHE2 gene expression. Am. J. Physiol. Cell Physiol. 281, C504–C513.
Xu, H., Li, J., Chen, H., and Ghishan, F. K. (2019). NHE8 deficiency promotes colitis-associated cancer in mice via expansion of Lgr5-Expressing cells. Cell. Mol. Gastroenterol. Hepatol. 7, 19–31. doi: 10.1016/j.jcmgh.2018.08.005
Xu, H., Zhang, B., Li, J., Chen, H. C., Tooley, J., and Ghishan, F. K. (2010). Epidermal growth factor inhibits intestinal NHE8 expression via reducing its basal transcription. Am. J. Physiol. -Cell Physiol. 299, C51–C57.
Yan, Y., Denef, N., and Schupbach, T. (2009). The vacuolar proton pump, V-ATPase, is required for notch signaling and endosomal trafficking in Drosophila. Dev. Cell. 17, 387–402. doi: 10.1016/j.devcel.2009.07.001
Yu, Q., Liu, X. M., Liu, Y. J., Riederer, B., Li, T. L., Tian, D. A., et al. (2016). Defective small intestinal anion secretion, dipeptide absorption, and intestinal failure in suckling NBCe1-deficient mice. Pflugers Arch. Eur. J. Physiol. 468, 1419–1432. doi: 10.1007/s00424-016-1836-3
Keywords: intracellular pH, epithelial ion transport, proliferation, signaling pathways, intestinal epithelial cells
Citation: Amiri M, Seidler UE and Nikolovska K (2021) The Role of pHi in Intestinal Epithelial Proliferation–Transport Mechanisms, Regulatory Pathways, and Consequences. Front. Cell Dev. Biol. 9:618135. doi: 10.3389/fcell.2021.618135
Received: 16 October 2020; Accepted: 04 January 2021;
Published: 22 January 2021.
Edited by:
Markus Ritter, Paracelsus Medical University, AustriaReviewed by:
John Geibel, School of Medicine Yale University, United StatesLaurent Counillon, University of Nice Sophia Antipolis, France
Copyright © 2021 Amiri, Seidler and Nikolovska. This is an open-access article distributed under the terms of the Creative Commons Attribution License (CC BY). The use, distribution or reproduction in other forums is permitted, provided the original author(s) and the copyright owner(s) are credited and that the original publication in this journal is cited, in accordance with accepted academic practice. No use, distribution or reproduction is permitted which does not comply with these terms.
*Correspondence: Katerina Nikolovska, Tmlrb2xvdnNrYS5LYXRlcmluYUBtaC1oYW5ub3Zlci5kZQ==