- 1Cancer Center, The First Hospital of Jilin University, Changchun, China
- 2VA Palo Alto Health Care System and Stanford University Medical School, Palo Alto, CA, United States
Studies have reported the vital role of nerves in tumorigenesis and cancer progression. Nerves infiltrate the tumor microenvironment thereby enhancing cancer growth and metastasis. Perineural invasion, a process by which cancer cells invade the surrounding nerves, provides an alternative route for metastasis and generation of tumor-related pain. Moreover, central and sympathetic nervous system dysfunctions and psychological stress-induced hormone network disorders may influence the malignant progression of cancer through multiple mechanisms. This reciprocal interaction between nerves and cancer cells provides novel insights into the cellular and molecular bases of tumorigenesis. In addition, they point to the potential utility of anti-neurogenic therapies. This review describes the evolving cross-talk between nerves and cancer cells, thus uncovers potential therapeutic targets for cancer.
Introduction
Globally, cancer is among the leading causes of mortality. Solid tumors are known to sculpt their microenvironment (e.g., molding angiogenesis pathways and forming pre-metastatic niches) in a bid to maximize their growth and metastatic potential (Magnon et al., 2013; Peinado et al., 2017). With regard to neurogenesis and angiogenesis processes, the process of cancer progression exhibits similarities with embryonic development, tissue repair, and regeneration (Boilly et al., 2017; Mauffrey et al., 2019). Neurons and nerve fibers have recently been identified as vital components of the tumor microenvironment that favor the initiation and progression of a variety of solid tumors (Stopczynski et al., 2014; March et al., 2020; Voronina et al., 2020). Accumulating evidence indicates that the nervous system participates in all stages of cancer, even in those that precede cancer development, such as pancreatic intraepithelial neoplasia (PanIN) or prostate intraepithelial neoplasia (PIN) (Magnon et al., 2013; Cole et al., 2015; Magnon, 2015; Saloman et al., 2016b; Kuol et al., 2018; Faulkner et al., 2019).
The nervous system regulates the functions of most organs. The peripheral nervous system (PNS), largely derived from the neural crest, connects the central nervous system (CNS) and the organs/limbs. The PNS consists of the motor and autonomic efferent fibers and sensory afferent fibers. The PNS and CNS mediate adaptive-reactive alterations in chronic diseases such as cancer. These alterations are referred to as “neuroplasticity” (Demir et al., 2015). “Neural plasticity” refers to the morphological and/or functional alterations of nerves, including changes in nerve fiber or trunk morphology, density, fiber qualities, and Schwann cell alterations (Demir et al., 2015). Studies have reported that the autonomic nervous system (ANS), composed of the sympathetic and parasympathetic divisions, plays important roles in tumorigenesis and cancer progression (Zhao et al., 2014; Rutledge et al., 2017). For example, gastric lesser curvature has higher vagal innervation, larger ganglia, and a higher incidence of gastric cancer when compared to the greater curvature (Zhao et al., 2014). Patients with spinal cord injuries have lower incidences of prostate cancer (PCa) due to functional denervation of the prostate gland indicating nerve dependence in PCa (Rutledge et al., 2017). The nervous system also indirectly influences cancer progression by regulating hormone secretion, such as epinephrine [E] and cortisol, through the hypothalamic-pituitary-adrenal (HPA) axis. The involvement of sensory neurons in tumorigenesis and cancer progression has also been reported (Saloman et al., 2016a). Besides modulating cancer proliferation and metastasis, the nervous system also regulates different aspects of cancer such as inflammation and angiogenesis (Stopczynski et al., 2014). In turn, immune cells or stromal cells can also be involved in nerve dependence in cancer.
Generally, cancer neurobiology has important implications in cancer pathogenesis and therapy. In this review, we elucidate on the crosstalk between nerves and cancer cells as well as the role of stress in cancer. Therefore, we present potential avenues for exploiting the emerging roles of the aforementioned processes in cancer prognosis and treatment.
Nerve Emergence in the Tumor Microenvironment
Apart from blood and lymphatic vessels, evidence indicates that neurogenesis (increased number of neurons) and axonogenesis (tumor-induced neural sprouting toward the tumor microenvironment) also play a vital role in tumorigenesis and cancer progression. Studies have reported that neurogenesis and axonogenesis are present in pre-neoplastic lesions and probably contribute to the initiation of cancer as an early event of the pre-malignant phase (Ayala et al., 2008; Mauffrey et al., 2019; Figure 1).
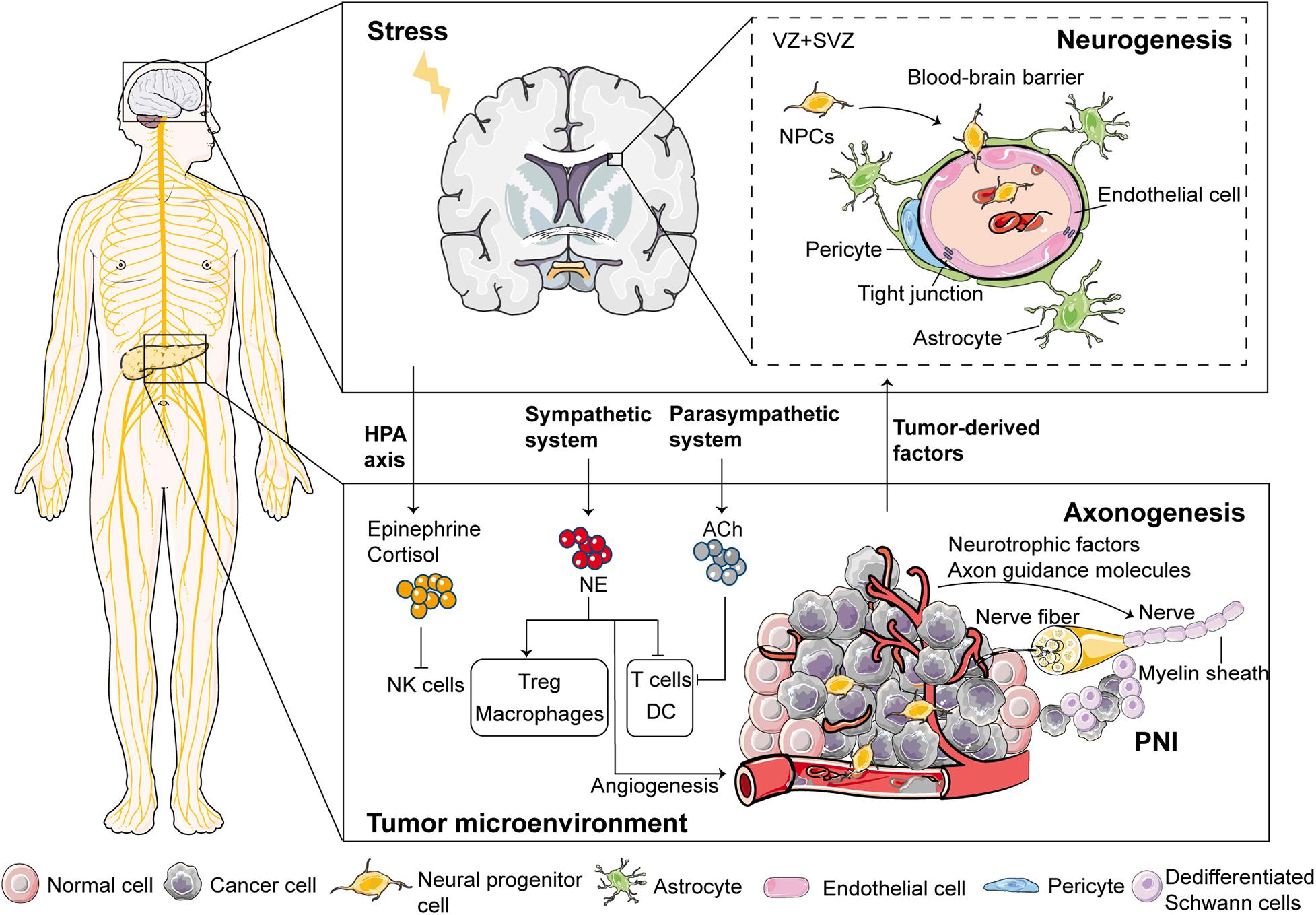
Figure 1. Proposed mechanisms involved in the emergence of nerves in the tumor microenvironment. Regarding neurogenesis (i.e., increased number of neurons), the neural progenitor cells (NPCs) leave the subventricular zone (SVZ) and egress into circulation through the disrupted blood-brain barrier during cancer development. After reaching and infiltrating the primary tumor or metastatic tissues, these NPCs differentiate into new adrenergic neurons, which regulate tumor growth and metastasis. For axonogenesis (i.e., tumor-induced neural sprouting toward the tumor microenvironment), cancer cells and some stromal cells can enhance neuronal outgrowth and initiate tumor innervation through multiple mechanisms. Sympathetic nerves release NE while parasympathetic nerves release ACh in the tumor microenvironment, and are, therefore, involved in modulating immune responses. Moreover, stress regulates immune responses through two major neural pathways; the HPA axis and the sympathetic nervous system. Schwann cells can act as leading cells that actively dissociate, recruit, and guide cancer cells to neurites through heterocellular contact, thereby, leading to PNI. VZ, ventricular zones.
Stem Cell Neurogenesis
Neurogenesis involves the proliferation and differentiation of neural stem cells (NSCs) (Lois and Alvarez-Buylla, 1994). It generates neural progenitor cells (NPCs) with high migration abilities, followed by erminal differentiation of the NPCs into mature neural cells (Lois and Alvarez-Buylla, 1994). Neurogenesis majorly occurs during embryonic and early postnatal brain development (Lois and Alvarez-Buylla, 1994). It has been identified in several human brain areas after birth, with the generated neurons often migrating long distances (up to several centimeters) from the ventricular zones (VZ)/sub-ventricular zone (SVZ) before reaching their final destination (García-González et al., 2017). NSCs and NPCs persist throughout life in SVZ of the lateral ventricles and the subgranule zone of the dentate gyrus in the hippocampus (Venkatesh and Monje, 2017). Studies have reported that NSCs and NPCs can generate new neurons and glia throughout life (Lois and Alvarez-Buylla, 1994).
A retrospective case-controlled and age-matched study found that the number of neurons was higher in prostatic ganglia in patients with PCa than in controls (Ayala et al., 2008). This result supports the existence of neurogenesis in PCa (Ayala et al., 2008). A possible hypothesis for the origin of cancer-related neo-neurons could be that; stem cells give rise to cells that differentiate into neurons (Mauffrey et al., 2019). Mechanisms that drive neurogenesis in tumors can be compared to those that underlie neural degeneration-regeneration (Mauffrey et al., 2019). Neural progenitors proliferate in the CNS after brain injury or during tissue regeneration to generate newborn neurons which migrate to injured sites to ensure a functional wound-healing response. The Na-K-Cl cotransporter 1 (NKCC1) has been shown to regulate cell volume (Mejia-Gervacio et al., 2011). It also helps maintain the migratory speed of neuroblasts along the rostral migratory stream (Mejia-Gervacio et al., 2011). Recent studies have suggested that NPCs [doublecortin (DCX) +] are transported from the CNS (SVZ) into circulation after blood-brain barrier (BBB) disruption during cancer development (Mauffrey et al., 2019). DCX is a microtubule-associated protein that is principally expressed in immature migrating neurons (Brown et al., 2003). It is one of the most important markers of migrating neuroblasts (Brown et al., 2003). After reaching and infiltrating primary tumors or metastatic tissues, these NPCs can further differentiate into new adrenergic neurons that are involved in PCa growth and metastasis (Mauffrey et al., 2019).
However, the upstream signaling pathways that contribute to the occurrence of neurogenesis in cancer have not been elucidated (García-González et al., 2017). Besides, questions on how neural progenitors penetrate the BBB, and thus migrate from the SVZ and what regulates different stages of neural progenitor differentiation in tumors have not been answered. The BBB comprises a complex array of tight junctions formed by endothelial cells, pericytes, astroglia, and perivascular mast cells (Rozniecki et al., 2010). Acute stress activates perivascular brain mast cells through the release of corticotropin-releasing-hormone which then disrupt the BBB (Rozniecki et al., 2010). Postnatal SVZ neurogenesis is modulated by multiple neurotransmitters (small molecules released by synapse or synapse-like structures) such as serotonin, acetylcholine (ACh), and dopamine (Venkatesh and Monje, 2017). The migratory process of NPCs in response to brain damage is regulated by multiple mechanisms in the tumor microenvironment, including: cellular pathway activation (e.g., DCX and PI3K/Akt); receptor-mediated interactions and adhesion molecules (e.g., chemokines); and cellular junction as well as extracellular matrix (ECM)-related proteins [e.g., matrix metalloproteinases (MMPs)] (Zarco et al., 2019). For example, postnatal neuroblasts express serotonin receptor 3A (5HT3A) and serotonergic modulation plays a role in postnatal migratory streams that originate from the SVZ (García-González et al., 2017). Notably, serotonin and the serotonin receptor are dysregulated during metastasis and angiogenesis in several cancers (Alpini et al., 2008; Sarrouilhe and Mesnil, 2019). Cholangiocarcinoma patients show high levels of serotonin due to elevated expression of tryptophan hydroxylase 1 (the rate-limiting enzyme in the biosynthesis of serotonin), and the suppressed expression of monoamine oxidase A (an enzyme accounting for degradation of serotonin) (Alpini et al., 2008). Future studies should aim at investigating the underlying mechanisms of stem cell neurogenesis in cancer patients, and whether the mechanism of neurogenesis is the same across different cancers.
Axonogenesis
Internal organs and glands are regulated by PNS, especially ANS. For instance, the stomach is predominantly innervated by the parasympathetic nervous system while the pancreas is regulated by both sympathetic and parasympathetic nerves. Most solid tumors (except the brain and spinal cord tumors) are innervated by nerve fibers that arise from the PNS, which form part of the tumor microenvironment. It is postulated that alterations in neurotrophic factor signaling before tumorigenesis can impact the progression from precancerous lesions to cancer by influencing tumor precursor cells and/or tissue innervation (Ayala et al., 2008). In a study involving genetically engineered pancreatic ductal adenocarcinoma (PDAC) mice models, alterations in neurotrophic factor expression and their receptors (Ngf, Gfrα2, and Nrtn) in the pancreas were shown to occur during the premalignant phase (Stopczynski et al., 2014). Moreover, PanIN lesions were caused by hyper-innervation in neurotrophic factor-rich microenvironments (Stopczynski et al., 2014). It is worth noting that axonogenesis in cancer may overlap with axonogenesis that occurs during embryonic development, where neurotrophic growth factors released from organs drive axonogenesis.
Nerve fibers that innervate normal tissues can be chemoattracted to tumor environments and outgrow as a result of neurotrophic factors that are released by the cancer cells. Cancer cells can promote neuronal outgrowth and initiate their own innervation through paracrine secretion, a phenomenon referred to as the neurotrophic effect (Figure 2). Neurotrophins composed of nerve growth factor (NGF), brain-derived neurotrophic factor (BDNF), neurotrophin-3 (NT-3), and NT-4/5 have been shown to drive axonogenesis by stimulating tyrosine kinase receptors expressed in nerve terminals. To exert biological effects, neurotrophic factors bind the tyrosine receptor kinase (TRK) family [also called neurotrophic tyrosine kinase receptors (NTRKs)], including TRKA, TRKB, and TRKC and the p75 neurotrophin receptor (p75NTR) (Chao, 2003; Arévalo and Wu, 2006; Lagadec et al., 2009). The two families are categorized as cell membrane receptors where the TRK family represents a specific high-affinity receptor whereas p75NTR is considered the low-affinity nerve growth receptor (NGFR) (Arévalo and Wu, 2006).
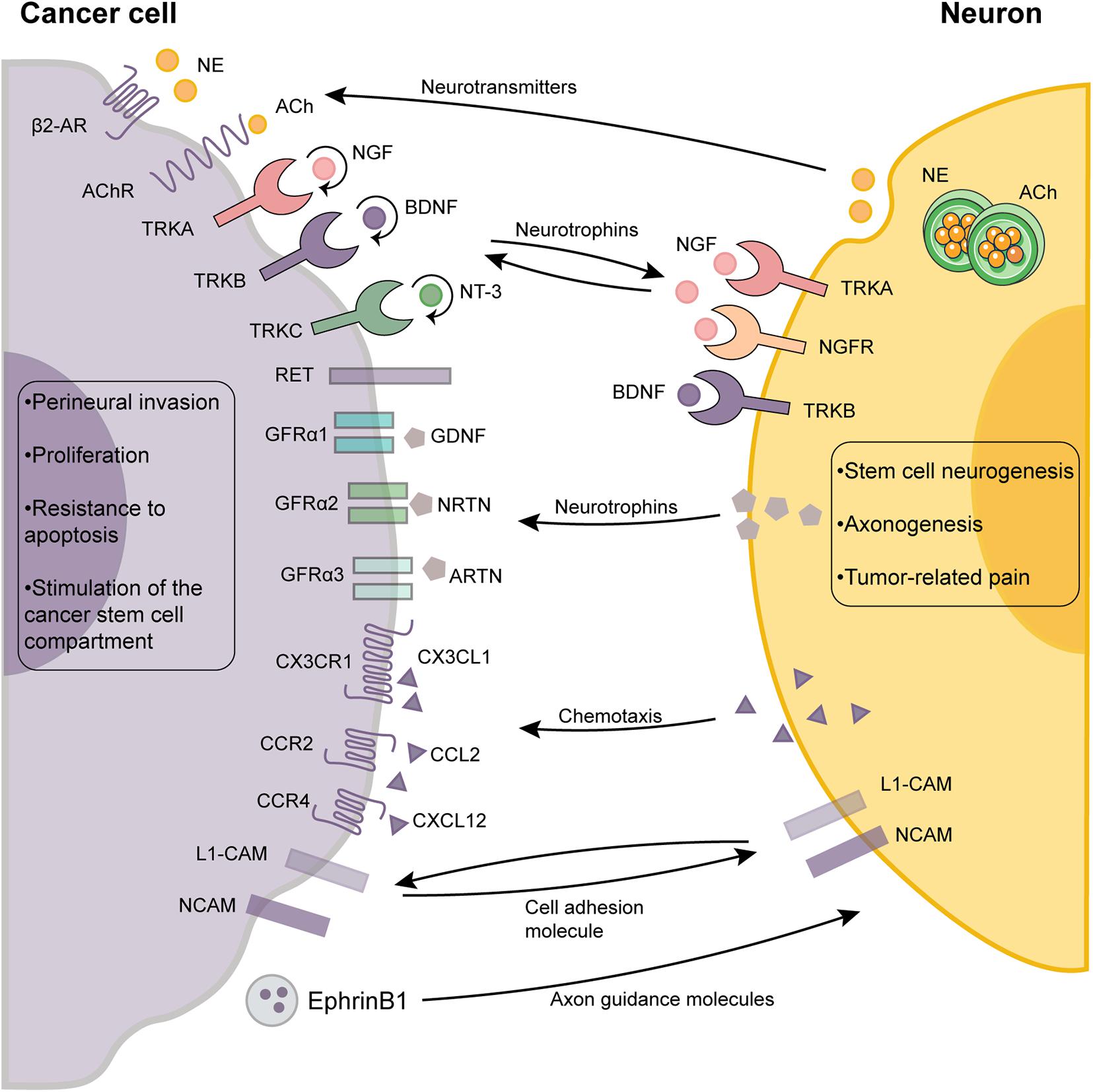
Figure 2. The cross-talk between cancer cells and neurons in the tumor microenvironment. The reciprocal autocrine and paracrine signaling between the cancer cells and neurons plays a pivotal role in neurogenesis, axonogenesis and perineural invasion. Autocrine signaling (round arrows) of cancer cells also promotes tumor growth and dissemination by activating multiple signaling pathways. The outcome of the interactions between neurons and cancer cells are shown as boxes. ACh, acetylcholine; β2-AR, β2-adrenergic receptors; BDNF, brain-derived neurotrophic factor; GDNF, glial cell line-derived neurotrophic factor; GFRα, glial cell line-derived family receptor alpha; L1-CAM, L1 cell adhesion molecule; NCAM, neural cell adhesion molecule; NGF, neurotrophins composing of nerve growth factor; NT-3, neurotrophin-3; NE, norepinephrine; TRK, tyrosine receptor kinase.
The roles of NGF and its receptors (TRKA and p75NTR) in cancer are well established (Laurent et al., 2004; Voronina et al., 2020). Breast cancer cells can stimulate neuronal growth using multiple neurotrophins such as NGF which can be released by Ca2+-mediated exocytosis of NGF-containing vesicles (Laurent et al., 2004; Voronina et al., 2020). NGF can also be secreted by tumor-associated immune cells and fibroblasts. Similarly, neuronal differentiation and axonogenesis can be induced by the proNGF (the precursor of NGF), which was shown to be secreted by PCa cells in vitro (Pundavela et al., 2014). In ovarian cancer, there is a feedforward loop through which adrenergic signaling can induce BDNF secretion from tumor cells via a β-adrenergic receptor 3 (ADRβ3)/cAMP/Epac/JNK-dependent manner. The BDNF/TRKB signaling axis further upregulates tumoral innervation (Allen et al., 2018). Immunohistochemical analysis revealed significantly elevated expression levels of BDNF and TRKB in hepatocellular carcinoma (HCC) (Guo et al., 2011).
Additionally, axon guidance molecules such as netrins, secreted by cancer cells act in synergy with neurotrophic growth factors to enhance axonogenesis (Biankin et al., 2012; Madeo et al., 2018). During the early pancreatic cancer stages, some axon guidance genes, especially those encoding for proteins of the SLIT/ROBO signaling pathway, exhibited recurrent mutations and copy-number variations (Biankin et al., 2012). Dysregulated axon guidance genes were also identified in murine models of early pancreatic carcinogenesis, suggesting that they are involved in its pathogenesis (Biankin et al., 2012). Similarly, evidence from other cancers including lung, breast, kidney, and cervical cancer has also implicated aberrant SLIT/ROBO signaling in carcinogenesis. Cancer cells secrete an exosome that packages the axonal guidance molecule, EphrinB1, which was shown to induce axonogenesis in vitro and tumor innervation in vivo (Madeo et al., 2018). Therefore, the crosstalk between cancer cells and nerve cells largely contributes to axonogenesis. However, more studies are required to characterize other mediators.
The Effects of Nerves on Tumors
The nervous system has been reported to be a regulator of cancer development and progression in multiple tissues (Magnon et al., 2013; Cole et al., 2015; Magnon, 2015; Saloman et al., 2016b; Kuol et al., 2018; Faulkner et al., 2019). In addition to being direct stimulators of cancer cells, nerves also exert a broad and extended impact on the tumor microenvironment by establishing interactions with other cells such as stromal, endothelial, and immune cells in the tumor microenvironment (Zahalka et al., 2017).
Nerves Regulate the Local Inflammation and Immune Response
The perineural inflammatory response is attributed to various inflammatory mediators that are locally released by afferent neurons (Stopczynski et al., 2014; Saloman et al., 2016a). Chronic inflammation increases the risk of malignancy and cancer progression (Stopczynski et al., 2014). Even a few sensitized pancreatic afferents can induce neurogenic inflammation in early pre-tumor stages, thereby contributing to, or accelerating the progression from PanIN to PDAC (Stopczynski et al., 2014). Neonatal capsaicin treatment can be used to ablate the sensory neuron that innervates the pancreas (Saloman et al., 2016a). This procedure can prevent neurogenic inflammation and prolong the survival of PDAC mice in a dose-dependent manner (Saloman et al., 2016a).
Neural regulation of immune responses has been identified as an emerging field in cancer biology (Dantzer, 2018). Vagal immunomodulatory effects, also known as the cholinergic anti-inflammatory pathway, have been linked to the action of acetylcholine, which is the predominant neurotransmitter of the parasympathetic system. Vagotomy elevates the risk of, or mortality from gastric, colorectal, and lung cancer (Åhsberg et al., 2009). This elevation has been attributed to the inhibition of anti-inflammation (Åhsberg et al., 2009). The nicotinic acetylcholine receptor-α7 (α7nAChR) is widely expressed in various immune cells, such as lymphocytes, suggesting that the vagus nerve may be involved in regulating immune responses in the tumor microenvironment (Antonica et al., 1994). Sectioning the right vagus was shown to reduce the number of lymphocytes released from the thymus into venous circulation (Antonica et al., 1994). The vagus nerve can also upregulate the expression of Tff2, which encodes a secreted anti-inflammatory peptide in memory T cells, thereby, suppressing the expansion of myeloid-derived suppressor cells in the spleen as well as cancer development (Dubeykovskaya et al., 2016). Nicotine, a selective cholinergic agonist, inhibits the transcriptional activity of nuclear factor-κB and the production of proinflammatory mediators in human peripheral monocytes using α7nAChR (Yoshikawa et al., 2006).
In contrast, the sympathetic nervous system (SNS) modulates β-adrenergic receptors (β-AR) signaling of immune cells in tumors through the circulating epinephrine/NE or local NE secretion by SNS nerve fibers (Nissen et al., 2018). Innate immune cells express ADRα1, ADRα2, and ADRβ2 while adaptive immune cells mainly express ADRβ2 (Dantzer, 2018). Studies have reported that the activity of innate immune cells is modulated by activation of ARs (Huan et al., 2017). The SNS activates ADRα1 to enhance the activity of Kupffer cells (i.e., macrophages in the liver) in regulating inflammatory responses, thereby accelerating HCC occurrence (Huan et al., 2017). Through ADRβ2, epinephrine induces macrophage polarization toward the M2 type that accelerates breast cancer progression (Qin et al., 2015). AR signaling can also inhibit the maturation, migration, and antigen presentation of dendritic cells (DC) (Ueshima et al., 2013). Glucocorticoids enhance the expression of ADRβ2 in natural killer (NK) cells and suppress the number and cytotoxic activity of NK and NKT cells, thereby inhibiting tumor immunosurveillance (Inbar et al., 2011; De Lorenzo et al., 2015). With regards to adaptive immunity, activation of the β-AR signaling pathway significantly suppresses CD8+ T cells, including their production, proliferation, and cytolytic killing capacity of IFN-γ. It also activates regulatory T cells (Tregs) (Nissen et al., 2018). Activation of the β2-AR signaling pathway in lymphocytes modulates the responsiveness of retention-promoting chemokine receptors CCR7 and CXCR4, thereby blocking the egress of lymphocytes from lymph nodes (Nakai et al., 2014). On the contrary, a recent study demonstrated that the ablation of SNS contributes to the immature myeloid-derived suppressor cells (MDSCs) accumulation, which facilitates the expansion of Tregs and suppresses tumor immunity (Nevin et al., 2020). In all, targeting the communication between neurons and leukocytes may be a potential approach for alleviating immunosuppression in the tumor microenvironment.
Perineural Invasion (PNI) Is a Vital Route of Solid Tumor Dissemination
Perineural invasion (PNI) refers to the invasion of the perineurium of large nerves that surround the tumor by cancer cells, thus causing them to metastasize. Generally, PNI is a frequently disregarded route of solid tumor dissemination, except for the direct invasion of surrounding tissues, lymphatics, and haematogenic spread (Marchesi et al., 2010b). For example, some head and neck neoplasm are in anatomical proximity with some cranial nerves, which can serve as channels with low resistance for migration (Liu et al., 2020). However, recent studies have considered PNI pathogenesis to be an active invasion of cancer cells into the perineurium space rather than a simple passive diffusion (Marchesi et al., 2010b). PNI is common among tumors of highly innervated organs such as pancreatic cancer and PCa. PDAC cells have been demonstrated to exist inside celiac ganglion and its surrounding, suggesting a fashion of tumor spread along the sensory afferents or the sympathetic nerves that synapse in the celiac ganglion (Stopczynski et al., 2014).
The peripheral nerves are made up of three layers (the endoneurium, the perineurium, and the epineurium) (Amit et al., 2015). The endoneurium encloses several nerve fibers, which are composed of an axonal process wrapped with a myelin sheath supported by Schwann cells (Amit et al., 2015). Despite the varying incidences of PNI in different cancers, it is associated with poor prognosis and reduced survival (Bapat et al., 2011). Results from a retrospective study that enrolled 133 extrahepatic cholangiocarcinoma patients revealed that 73.7% of the patients had PNI (Murakami et al., 2013). Those patients exhibited a significantly low overall 5-year survival rate (Murakami et al., 2013). Intraneural invasion (i.e., the invasion of cancer cells in the endoneurium) exerts a worse impact on clinical prognosis than PNI (Amit et al., 2015). However, the mechanisms by which cancer cells destroy the peripheral nerve barrier have not been established.
PNI is mediated by various signaling molecules secreted by cancer cells and nerves (Bapat et al., 2011). Nerve fibers can secrete multiple active neuropeptides and neurotransmitters within the vicinity of cancer or stromal cells to activate corresponding membrane receptors involved in tumor growth and metastasis (Li et al., 2014; Table 1).
Neurotransmitters and Their Receptors in PNI
Several neurotransmitters have been reported to be highly correlated with PNI (Ma et al., 2019; March et al., 2020). Catecholamines are neurochemicals that have catechins and amines converted from tyrosine as their precursor. They include norepinephrine (NE), epinephrine, and dopamine. NE/β2-AR signaling promotes proliferation, migration, and PNI by inducing epithelial-mesenchymal transition (EMT) and upregulating MMPs (MMP-2 and MMP-9) in salivary adenoid cystic carcinoma (Ma et al., 2019). NE has also been shown to accelerate pancreatic cancer PNI and progression through the β-AR/PKA/STAT3 signaling pathway in vivo, where the activation of STAT3 upregulates the downstream expression levels of NGF and MMPs (Guo et al., 2013). Parasympathetic nerves provoke the proliferation and migration of PCa cells by secreting Ach, which stimulates cholinergic receptor muscarinic 1 (March et al., 2020). In addition, monoamine oxidase A (MAOA), a catecholamine neurotransmitter degrading enzyme, was found to be remarkably decreased by epigenetic alterations in clinical HCC samples (Li et al., 2014). Therefore, MAOA downregulation is closely associated with metastasis and poor prognoses among HCC patients (Li et al., 2014).
Neurotrophins and Their Receptors in PNI
Several studies have supported the involvement of neurotrophic factors in PNI (Sclabas et al., 2005; Ma et al., 2008; Wang et al., 2009). A positive correlation was observed among the expression levels of NGF, p75NTR, TRKA, TRKB, glial cell line-derived neurotrophic factor (GDNF), and PNI in pancreatic cancer tissues or cell lines (Sclabas et al., 2005; Ma et al., 2008; Wang et al., 2009). However, NGF was found to have no correlation with PNI, recurrence-free, and OS in extrahepatic cholangiocarcinoma (Urabe et al., 2016). Dorsal root ganglion (DRG) and pancreatic cancer cell co-culture models suggested that a mutual tropism exists between the nerves/DRGs and pancreatic cancer cells through multiple molecular factors (e.g., NGF-TRKA pathway) (Ceyhan et al., 2008; Ma et al., 2008). Besides, TRKB signaling induces EMT, resulting in elevated cancer cell migration and metastasis (Fujikawa et al., 2012). However, the involvement of NT-3/4 in PNI has not yet been established.
The GDNF family of ligands (GFL) comprises four neurotrophic factors, including GDNF, neurturin (NRTN), artemin (ARTN), and persephin (PSPN) (Airaksinen and Saarma, 2002). GFLs bind co-receptors of the glial cell line-derived family receptor alpha (GFRα) family (GDNF to GFRα1, NRTN to GFRα2, ARTN to GFRα3, and PSPN to GFRα), followed by the recruitment and activation of transmembrane RET receptor tyrosine kinase (Airaksinen and Saarma, 2002). GDNF can be secreted by nerves, Schwann cells, and endoneurial macrophages. It was shown to recruit pancreatic cancer cells expressing RET and GFRα1 in both co-culture and mice models (Gil et al., 2010; Cavel et al., 2012). It also facilitates the migration and PNI of PDAC cells through the PI3k/Akt and Ras-Raf-MEK-ERK pathways (Veit et al., 2004; Ben et al., 2010). The NRTN/GFRα-2 axis also elevates neural density and an aggressive phenotype in pancreatic cancer (Wang et al., 2014).
Chemokines and Their Receptors in PNI
Chemokines are a class of small soluble proteins that modulate cell migration during wound healing, embryonic development, and tumor metastasis (Marchesi et al., 2010a; He et al., 2015). CCL2, a chemokine released by DRG/nerve, contributes to migration and PNI of PCa cells that express its receptor (CCR2) (He et al., 2015). Apart from being a chemokine, CCL2 can also act as a repair factor that is secreted after neural injury caused by cancer infiltration during a nerve repair inflammatory response (He et al., 2015). In addition, the CX3CL1 chemokine was found to be expressed by neurons while its receptor, CX3CR1, was overexpressed in both PDAC cell lines and surgical samples (Marchesi et al., 2010a). Therefore, the CX3CR1/CX3CL1 axis plays a role in PNI and dissemination of pancreatic cancer cells along intra- and extra-pancreatic nerves (Marchesi et al., 2010a). CXCL12/CXCR4 has been shown to stimulate the invasiveness of PCa cells in vitro, and increase the number of nerves in vivo (Zhang et al., 2008).
Other Cell-Surface Molecules or Receptors in PNI
The L1 cell adhesion molecule (L1-CAM, also known as CD171) and neural cell adhesion molecule (NCAM, also known as CD56) are members of the neuronal immunoglobulin superfamily that regulates neuronal adhesion and migration (Ben et al., 2010). Peripheral nerve injury induces the upregulation of L1-CAM in the contact surface of injured peripheral axons and Schwann cells, suggesting its possible involvement in nerve regeneration (Yamanaka et al., 2007). A recent study reported that Schwann cells secret L1-CAM, which acts as a chemo-attractant, thus aiding in the recruitment of cancer cells by activating the MAPK signaling pathway (Na’ara et al., 2019). L1-CAM also elevates the expression levels of MMPs, including MMP-2 and MMP-9, by activating STAT3 in PDAC cells (Na’ara et al., 2019). The main role of MMP-2 and MMP-9 in PNI is degrading the ECM around the tumor and nerve tissue (Klein et al., 2004). Therefore, overexpression of L1-CAM and NCAM has significant correlations with PNI, vascular invasion, and poor overall survival outcomes among cancer patients (Kameda et al., 1999; Shang et al., 2007; Ben et al., 2010).
Semaphorins are a family of proteins that act as axon guidance factors during cancer development (Müller et al., 2007; Ding et al., 2013). Plexins are their main functional receptors. Semaphorin 3A and its receptors, plexins A1-A4 and neuropilin-1 (NRP1), are overexpressed in pancreatic cancer and are correlated with negative clinicopathological manifestations (Müller et al., 2007). Cytoplasmic expression of semaphorin 4F in PCa cells is positively correlated with nerve density, PNI diameter, and PCa recurrence risk (Ding et al., 2013).
The Roles of Schwann Cells in PNI
Schwann cells are peripheral glial cells that are involved in neural homeostasis, repair, and regeneration. Their emergence has been detected in murine and human PanIN lesions (Demir et al., 2014). They are chemoattracted to cancer cells and their precursor lesions by NFG-p75NTR/TRKA, suggesting that they migrate first in the premalignant phase (Bentley and Lee, 2000; Demir et al., 2014). P75NTR–/– mice embryos exhibited a deficit in Schwann cell migration (Bentley and Lee, 2000).
Cancer cells take advantage of the canonical functions of Schwann cells to promote PNI (Deborde et al., 2016). For example, invagination of Schwann cells between individual axons during human fetal development separates them from each other, thereby isolating individual axons from large bundles. Schwann cells induce protrusions in cancer cells and intercalate between cancer cells to facilitate their dispersal from the tumor (Deborde et al., 2016). In addition, during nerve repair, Schwann cells dedifferentiate/activate, lose their myelination ability, induce and guide axonal extensions. This is followed by the re-expression of some proteins, such as glial fibrillary acidic protein (GFAP) (reviewed by Jessen and Mirsky, 2016). At the same time, there is a significant increase in the number of GFAP-positive Schwann cells when cancer cells injure/invade nerves (Deborde et al., 2016). Schwann cells can also guide cancer cells toward neurites by inducing the extension of directed protrusions in cancer cells (Deborde et al., 2016). Therefore, they can act as leading cells that actively dissociate, recruit, and guide cancer cells to neurites through heterocellular contact that ultimately leads to PNI (Deborde et al., 2016).
Nerves Sustain Tumor Proliferation and Induce Resistance to Apoptosis
Autocrine secretion of neurotrophins modulates the growth and dissemination of cancer cells by activating different signaling pathways. Pro-survival signals can be triggered by NGF binding to TRKA; BDNF and NT-4 binding to TRKB; and NT-3 binding to TRKC. Studies have reported that breast and pancreatic cancer cells express receptors such as TRKA and phospho-TRKA for several neuropeptides and neurotransmitters (Lagadec et al., 2009). Sclabas et al. (2005) reported that 50% of the human pancreatic cancer specimens in their study had overexpressed TRKB levels (Sclabas et al., 2005). TRKA can be activated in an autocrine manner since the majority of breast cancers express NGF (Adriaenssens et al., 2008; Lagadec et al., 2009). Expression of NGF stimulates tumor cell proliferation and survival through the constitutive activation of the PI3K-Akt and ERK/p38 MAP kinase (MAPK) pathways (Adriaenssens et al., 2008; Lagadec et al., 2009). In addition, neuronal endings have been shown to secrete a soluble synaptic protein, neuroligin-3 (NLGN3), which stimulates high-grade glioma proliferation and further NLGN3 expression through the PI3K-mTOR signaling pathway (Venkatesh et al., 2015). The proliferation of gastric cancer cells can be accelerated by Netrin-1 through the ERK/MAPK signaling pathway and focal adhesion kinase (FAK) activation (Yin et al., 2018).
Most of the cytotoxic anti-cancer drugs can trigger the p53-dependent apoptotic program of cancer cells. Studies have shown that catecholamines mediate the chemotherapeutic resistance of cervical cancer cells which overexpress ADRβ2 both in vitro and in vivo (Chen et al., 2017). Catecholamines elevate the expression levels of the silent information regulator 1 (Sirt1), a type III histone deacetylase, by activating β2-adrenergic receptor signaling (Chen et al., 2017). Therefore, they can inhibit doxorubicin-induced p53-dependent cytotoxicity in cervical cancer cells since Sirt1 impairs p53 functions by mediating its deacetylation (Chen et al., 2017).
Anoikis refers to programmed cell death that is associated with losses in cell-matrix interactions. Metastatic success of tumor cells is dependent on their ability to resist anoikis (Douma et al., 2004; Fujikawa et al., 2012; Okugawa et al., 2013). Overexpressed TRKB enhances tumor metastasis by suppressing caspase-associated anoikis (Douma et al., 2004; Fujikawa et al., 2012; Okugawa et al., 2013). In contrast, TRKC is considered to be a tumor suppressor and a good-prognostic factor since it induces apoptosis in the absence of ligands such as NT-3 (Bouzas-Rodriguez et al., 2010). Upregulated autocrine NT-3 secretion has been reported in most of the aggressive human neuroblastomas (NBs). It blocks TRKC-induced apoptosis of NB cell lines (Bouzas-Rodriguez et al., 2010).
Tumor Innervation Drives Tumor Angiogenesis
Generally, nerves bundle along blood vessels. They can provide a critical set of signals that assist tumors to redevelop a vascular network, which ensures nutrition and communication during cancer proliferation and progression (Zahalka et al., 2017). Studies have documented the roles of neurotransmitters and neuropeptides in regulating angiogenesis (Kermani et al., 2005; Zahalka et al., 2017).
A study using mice models reported that adrenergic nerves stimulate angiogenesis in early PCa growth by the release of NE (Zahalka et al., 2017). The study indicated that angiogenesis requires an alteration of endothelial cell metabolism from oxidative phosphorylation to aerobic glycolysis (Zahalka et al., 2017). In addition, adrenergic signals from autonomic nerves fuel angiogenesis and cancer progression by inhibiting oxidative phosphorylation in endothelial cells (Zahalka et al., 2017). In breast cancer cell lines, direct activation of β-AR signaling was shown to enhance the expression of proangiogenic factors, including vascular endothelial growth factor (VEGF) and interleukin (IL)-6 (Madden et al., 2011). Neuropeptide Y, a sympathetic neurotransmitter, stimulates VEGF expression, thereby promoting angiogenesis and breast cancer progression in a paracrine manner in vitro (Medeiros and Jackson, 2013). Nicotine can also accelerate the proliferation of endothelial cells as well as angiogenesis through nAChR especially α7nAChR (Pillai and Chellappan, 2012). BDNF recruits bone-marrow derived Sca-1 + hematopoietic cell subsets expressing TRKB to enhance capillary formation (Kermani et al., 2005). By binding to the plexin B1 receptor on endothelial cells, semaphorin 4D, secreted by the tumor-associated macrophages (TAMs), accelerates tumor angiogenesis and vessel maturation (Sierra et al., 2008).
Nerves Stimulate the Cancer Stem Cell (CSC) Compartment
Accumulating evidence indicates that innervation impacts the development of stem cell compartments and tissue homeostasis (Zhao et al., 2014; Yin et al., 2015; Hayakawa et al., 2017). Recent studies have indicated a similar crosstalk between nerves and CSC. The intrinsic regulation of CSCs occurs through vital proliferative and survival pathways such as the Wnt, Notch, and Hedgehog pathways (Yin et al., 2015). Cholinergic nerves can activate Wnt signaling in gastric stem cells through muscarinic receptor-3 (M3R), which activates yes-associated protein (YAP). This activation contributes to stem cell expansion (Zhao et al., 2014; Hayakawa et al., 2017). However, vagal nerve signaling exhibits an opposite effect that inhibits KRAS-mutated PDAC (Renz et al., 2018). Cholinergic signaling, directly and indirectly, suppresses CSC compartment and PDAC cell growth partly through the cholinergic receptor muscarinic 1 (CHRM1). This leads to the inhibition of the downstream MAPK/EGFR and PI3K/AKT pathways (Renz et al., 2018). Sensory nerves activate Hedgehog signaling in touch dome epithelia, an innervated subset of interfollicular epidermis cells, thereby conferring high susceptibilities for the occurrence of basal cell carcinomas (Peterson et al., 2015).
Paracrine BDNF of differentiated recurrent triple-negative breast cancer (TNBC) cells promote the self-renewal potential of TRKB+ CSC through transcriptional activation of KLF4 (Yin et al., 2015). These TRKB+ cells represent a distinct CSC subpopulation, accounting for recurrent TNBC growth (Yin et al., 2015). In head and neck squamous cell carcinoma (HNSCC), p75NTR is considered to be a more specific marker of CSCs than CD44 (Murillo-Sauca et al., 2014). However, the CSC-regulating potential for nerves does not rule out their indirect roles, such as interactions with the immune system.
Nerves Are Involved in the Formation of Tumor-Related Pain
Pain, a typical cancer sign, impacts on the quality of life and functional status of cancer patients (Jimenez-Andrade et al., 2011). Several mechanisms, including inflammation, tumor-induced acidosis, and tumor-induced injury to peripheral nerve fibers are associated with the generation of tumor-related pain (Peters et al., 2005; Jimenez-Andrade et al., 2011; Cohnen et al., 2020).
Inflammatory cells such as proinflammatory macrophages and dendritic cells are recruited by cancer cells to the outside of the sciatic nerve. They initiate a series of events, including epineurium damage and depletion of triglycerides from epineural adipocytes (Cohnen et al., 2020). Jimenez-Andrade et al. suggested that normal nerve fibers that innervate tissues can be sensitized or injured by factors that are released by cancer and tumor-associated stromal/inflammatory/immune cells (Jimenez-Andrade et al., 2011). Sensitized nerve fibers are vulnerable to noxious stimuli and tumor-induced and/or released factors such as NGF, endothelins, prostaglandins, protons, bradykinin, and cytokines (Jimenez-Andrade et al., 2011). Emerging evidence has revealed that the NGF signaling pathway involves inflammatory hyperalgesia and cancer-associated pain (Mizumura and Murase, 2015). Besides, NGF interacts with TRKA and/or p75NTR expressed on the perineurium, thereby, directly activating and sensitizing sensory nerves that are close to pancreatic cancer. NGF also regulates the expression and function of transient receptor potential vanilloid 1 (TRPV1), a non-selective cation channel, thereby, stimulating and activating sensory neurons (Zhu et al., 2011).
PNI causes prominent cancer pain, such as severe back pain in PDAC patients. Many of the PNI signaling molecules overlap with those in pain signaling (Sclabas et al., 2005; Bapat et al., 2011). Moreover, invading cancer cells can damage the neuronal sheath (Sclabas et al., 2005; Bapat et al., 2011). PNI of the head and neck cancer can result in cranial neuropathy, such as trigeminal neuropathy, which is characterized as facial or paresthesias pain (Boerman et al., 1999). In PDAC, pancreatic nerve hypertrophy and PNI are associated with PDAC-related pain. This pain is attributed to nerve damage by the invading tumor cells, and sensitized pancreatic afferents (Stopczynski et al., 2014). L1-CAM might also be involved in the occurrence of neuropathic pain by activating intracellular signaling cascades, such as p38 MAPK in nociceptive pathways (Yamanaka et al., 2007).
Stress Promotes Cancer Progression
The nervous system can perceive signals from the outside environment, process them, and transmit them to the CNS, where they are then integrated. Studies have shown that cancer patients frequently suffer from chronic emotional stress due to the social, emotional, and physical effects caused by cancer (Chida et al., 2008). Epidemiological studies have established an association between chronic stress conditions and high risk or poor survival outcomes among cancer patients (Lillberg et al., 2003; Chida et al., 2008). Some viable explanations account for the link between psychological stress and tumorigenesis. For example, people under stress are more likely to develop some unhealthy behaviors (e.g., excessive drinking, smoking, etc.) or a long-term inflammatory response, which raise the risk for tumorigenesis (Dai et al., 2020). Stress affects various immunological and neurochemical aspects involved in tumorigenesis and cancer progression (Thaker et al., 2006; Feng et al., 2012; Le et al., 2016; Zhou et al., 2016). It regulates immune responses through two major neural pathways; the HPA axis and the SNS pathways (Reiche et al., 2004).
Under stress conditions, activation of the ANS or the HPA axis leads to the secretion of diverse mediators (e.g., glucocorticoids and catecholamines) that provoke tumorigenesis and cancer progression (Thaker et al., 2006; Zhou et al., 2016). Chronic stress was shown to promote tumorigenesis in mice partially by attenuating p53 function (Feng et al., 2012). During chronic stress, elevated glucocorticoid levels induce a negative regulator of p53, the serum- and glucocorticoid-induced protein kinase (SGK1), to suppress p53 function (Feng et al., 2012). In a stressed mouse model, elevated levels of tissue catecholamines were shown to activate ADRβ2, which upregulated VEGF gene expression in ovarian carcinoma cells, resulting in enhanced angiogenesis and malignant cell growth (Thaker et al., 2006). The TAMs in ovarian cancer patients express elevated VEGF and MMP-9 because of the high psychological stress levels (e.g., chronic life stress, depression, and perceived stress) (Lutgendorf et al., 2008). Since macrophages express β-adrenergic and α-adrenergic receptors, the mechanisms underlying these associations are attributed to elevated stress hormones, including NE and cortisol (Lutgendorf et al., 2008). Similarly, elevated NE levels enhance the invasive potential of ovarian cancer cells most likely due to higher expression levels of MMP-2 and MMP-9 (Sood et al., 2006). Chronic stress can induce the proliferation of hematopoietic stem cells as well as increased levels of inflammatory leukocytes including neutrophils and inflammatory monocytes (Heidt et al., 2014). Mechanically, elevated NE levels decrease CXCL12 through ADRβ3 on bone marrow niche cells (Heidt et al., 2014). CXCL12 is known to restrain the proliferation and migration of the hematopoietic stem and progenitor cell (Heidt et al., 2014). This is reflected from the side, the immunosuppressive activity induced by corticosteroid hormones in the HPA axis seem to not play a major role in stress. On the positive side, social support can alleviate such a phenomenon (Lutgendorf et al., 2008).
Surgical stress upregulates epinephrine and NE secretion, which bind βAR in immune cells (Zhou et al., 2016). Elevated epinephrine markedly promotes Treg proliferation, which suppresses antitumor immunity in breast cancer patients (Zhou et al., 2016). However, during the perioperative period, propranolol, a non-selective β-adrenergic antagonist, has been reported to restore cell-mediated immunity in breast cancer patients with surgery-induced immunosuppression (Zhou et al., 2016). In addition, cold stress was shown to suppress antitumor immune responses and accelerated tumor growth and metastasis in mice (Kokolus et al., 2013). However, Walker et al. reported that in the mice received denervation of the splanchnic nerves, stress-enhanced cancer proliferation and metastasis were not affected by elimination of circulating epinephrine (Walker et al., 2019). In all, the activities of catecholamines may vary from cancer to cancer by binding different target cells.
A previous study indicated that chronic stress in mice elevated lymphatic vessel density, a stronger flow in lymphatic vessels, and dilation to promote tumor cell dissemination (Le et al., 2016). Activation of TAMs by the β-adrenoceptor signaling pathway enhances their ability to secrete enhanced inflammatory molecules (e.g., PGE2), which stimulate the tumor cells to secrete VEGFC that is necessary for lymphatic remodeling (Le et al., 2016). Targeting SNS can inhibit the effect of chronic stress on lymphatic remodeling to prevent tumor cell dissemination through the lymphatic routes (Le et al., 2016). Therefore, psycho-behavioral intervention or therapies for stress and anxiety disorders might synergistically improve the efficacy of cancer treatment.
Clinical Value and Application
A better understanding of cancer neurobiology is necessary as it provides new insights into the prognosis and therapeutic targets for tumors.
Nerves Are an Emerging Hallmark of Cancer
Neurogenesis and axonogenesis are emerging as a feature of aggressive tumors, such as colorectal cancer (Albo et al., 2011). Assessment of nerve density in the tumor microenvironment might serve as a potential predictive marker of cancer aggressiveness. A retrospective study involving radical prostatectomy tissues from 43 prostate adenocarcinoma patients revealed that the densities of sympathetic and parasympathetic nerve fibers in the tumor and surrounding normal tissues are correlated with poor clinical outcomes (Magnon et al., 2013).
PNI, as an underestimated route of metastasis, has an association with poor prognosis or recurrence (Yamaguchi et al., 2002). The neoplastic cells disseminating along the nerve fascicles probably escape from resection (Chatterjee et al., 2012; Murakami et al., 2013; Zhou et al., 2015). In PNI-positive gastric cancer patients, the largest involved nerves (with a diameter ≥ 65 μm) were correlated with low 5-year disease-free survival outcomes (Zhou et al., 2015). However, a retrospective study involving 186 patients with parotid gland malignancies concluded that PNI positivity does not have a statistically significant correlation with worse OS and disease-free survival (Huyett et al., 2018). Moreover, facial nerve paresis and anesthesia were both significant symptoms for predicting PNI of parotid gland malignancies (Huyett et al., 2018). PNI assessment is usually based on the histological evaluation of tissue sections or full-body imaging, such as MRI (Boerman et al., 1999). Advanced microscopy techniques and imaging techniques can reveal fine structural analysis of neuron-cancer cell interactions in multiple tissue types of clinical samples (Chung et al., 2013).
Neurotransmitters (including epinephrine and NE), neurotrophins, as well as their receptors have been identified as potential diagnostic and prognostic cancer biomarkers (Abdel-Hamid et al., 2016). Overexpressed proNGF has been documented to be a clinical biomarker that predicts the prognosis of human PCa, since it correlates with the Gleason score (Pundavela et al., 2014). Upregulated TRKB expression level has been proposed to be an independent prognostic marker for gastric cancer (Okugawa et al., 2013).
Potential Anti-neurogenic Therapies Are Promising in Cancer Treatment
Multiple effects of nerves in the tumor microenvironment have been documented. Therefore, tumor innervation is emerging as an optional therapeutic target (Table 2). Since PNI has dual effects in tumor metastasis (regulate relapse after surgical resection and cancer pain generation) it is a promising therapeutic target.
Denervation
From a therapeutic perspective, based on the critical roles of nerves in cancer development, surgical or pharmacological denervation (the injection of neurotoxic drugs) constitutes a potential and innovative strategy for preventing cancer dissemination, improving survival, or inhibiting pain (Zhao et al., 2014). The unilateral vagotomy and injection of botulinum toxin A have been reported to attenuate gastric tumorigenesis and progression (Zhao et al., 2014). A phase 2 clinical trial evaluated the effect of botulinum toxin injection by gastroscopy in both the tumor and the adjacent stomach wall tissues of gastric cancer patients (NCT01822210). Such denervation, combined with other therapies is a viable approach for cancer patients who do not accept surgery as a treatment option. However, determining the timing of this treatment and coping with the corresponding side effects is very critical. For instance, denervation has been shown to promote the development of cancer-related lesions in the gastric remnant (Kaminishi et al., 1997).
Antagonists of Receptors
In the clinic, β-blockers have been extensively used to treat patients with hypertension, angina, or anxiety (Dézsi and Szentes, 2017). β-blockades have been shown to increase cancer patient survival, though the specific mechanism has not been established (Hayakawa et al., 2017; Kokolus et al., 2018). A combination of inhibiting angiogenesis through both neural signals and/or endothelial cell metabolism is a novel approach for overcoming antiangiogenic resistance (Thaker et al., 2006; Zahalka et al., 2017). A retrospective study involving 3561 PCa patients with high-risk or metastatic disease indicated that β-blockers reduce PCa-specific mortality (Grytli et al., 2014). Targeting β-AR signaling has been suggested to work in tandem with cancer immunotherapy to enhance therapeutic responses (Kokolus et al., 2018; Nissen et al., 2018). Various clinical trials are assessing the efficacy of β-blockers (e.g., propranolol, propranolol hydrochloride) for the treatment of prostate (NCT02944201 and NCT03152786), colorectal (NCT03919461), breast (NCT02596867), gastrointestinal (NCT03245554), pancreatic (NCT03838029) cancers, and melanoma (NCT01988831) as well as locally recurrent or metastatic solid tumors (NCT02013492). Propranolol has been tested in combination with neoadjuvant chemotherapy in breast (NCT01847001) and gastric (NCT04005365) cancer patients. Studies encompassing diverse tumor types should be done to evaluate the efficacy of β-blockades as adjuvant therapies in clinical oncology. Adrenergic signaling-induced immunosuppression may necessitate a combination of immunotherapies with β-blockers to improve efficiency.
Inhibitors of Neuroendocrine Factors
Targeting neurotrophins and neurotrophic signaling has been shown to suppress tumor progression and cancer-associated pain (Adriaenssens et al., 2008; McCaffrey et al., 2014; Hayakawa et al., 2017). Anti-NGF antibodies or small interfering RNAs against NGF inhibited breast tumor growth and metastasis in xenograft models (Adriaenssens et al., 2008). Given the impact of NGF in cancer pain, NGF blockade inhibits the sprouting of sensory nerve fibers and alleviates cancer-associated pain, especially bone cancer pain, through anti-hyperalgesia (i.e., normalizing a downregulated nociceptive threshold) (Bloom et al., 2011; Jimenez-Andrade et al., 2011; Mizumura and Murase, 2015). The efficacy of blocking antibodies against NGF (e.g., tanezumab, fasinumab) in patients with pain or cancer-induced pain have been investigated in some clinical trials (NCT00830180) (Mizumura and Murase, 2015). NGF blockade at early bone sarcoma stages has been shown to relieve tumor-induced bone destruction as well as reduce pain by 40–70% (McCaffrey et al., 2014). Therefore, inhibiting NGF could play a dual effect against both cancer growth and cancer-associated pain.
Furthermore, given the role of TRKs in tumor cell proliferation and PNI, inhibiting RTKs has therapeutic significance (Yilmaz et al., 2010). In HNSCC, a potent and selective TRK kinase inhibitor, AZ-64 that targets TRKB, has been shown to inhibit tumor proliferation and migration, as well as overcome chemotherapy resistance in vitro (Yilmaz et al., 2010). The TRK antagonist, K252a, was reported to inhibit the growth and invasion of gastric cancer as well as choriocarcinoma in vivo and in vitro (Kawamura et al., 2010; Okugawa et al., 2013). Several clinical trials are investigating the therapeutic potential of entrectinib, which is an orally bioavailable TRKA/B/C, ROS1, and ALK inhibitor in multiple tumors (NCT02097810, NCT02568267, and NCT02650401). In addition, several TRK inhibitors, including NOV1601 (NCT04014257), VMD-928 (NCT03556228), TSR-011 (NCT02048488), and DS-6051b (NCT02279433), are being evaluated in the on-going clinical trials. However, the results of these studies have not yet been reported. Combined with radiotherapy, AstraZeneca 1332, an inhibitor for TRKA, B, and C was reported to have an inhibitory effect on pancreatic cancer growth in vitro, while it exhibited inefficient effects in xenograft growth (Johnson et al., 2017).
Future Directions
In conclusion, the reciprocal signaling between cancer cells and nerves provides novel insights into the mechanisms of tumorigenesis and cancer progression. It has not been established whether neurogenesis and axonogenesis are coincidental or prerequisites in cancer development and progression. Moreover, it is unknown if these processes are specific to different cancer types. Studies have described the phenomenon of autonomic or sensory nerves in cancer. However, the potential involvement of motor nerves in cancer cannot be ignored and further investigations are recommended. Different cancer types utilizing various molecules or targeting to varied nerve types results in either the promotion or suppression of cancer development. More studies should explore the precise cellular and molecular mechanisms that underlie tumor innervation and PNI. However, PNI can only be evaluated after surgery, thus necessitating the need to establish predictive biomarkers for the existence or severity of PNI. It makes sense for surgeons to preoperatively assess the existence of PNI, carefully, and cope with the resection border during surgery. The development and implementation of single-cell sequencing and multi-omics assays may provide new insights into rare cells such as NPCs in the tumor microenvironment. Manipulation of the nervous system presents a potential avenue for the establishment of prognostic markers, risk stratification, and therapeutic strategies (including adjuvant treatment of cancer-induced pain) for cancer. However, many questions have yet to be answered. Different nerves function at different times and in different ways. For example, sympathetic nerves facilitate the early stages of carcinogenesis, while parasympathetic nerves accelerate cancer dissemination (Magnon et al., 2013). Denervation is considered to be most effective during the early cancer stages, even before the precancerous stage (Saloman et al., 2016b). Given these factors, what determines the intervention (e.g., denervation) time and who a suitable patient is? Further experimental or clinical studies should be performed to investigate the translational value or clinical applications of cancer neurobiology.
Author Contributions
WW and LL carried out the primary literature search, drafted and revised the manuscript, and participated in discussions. NC, CN, and ZL helped to modify the manuscript. JC and JH carried out the design of the research and literature analysis, drafted and revised the manuscript, and participated in discussions. All authors read and approved the final manuscript.
Funding
This work was supported by the Chronic Non-communicable Diseases Prevention and Control of National Ministry of Science and Technology (Grant No. 2016YFC1303804); Jilin Provincial Key Laboratory of Biological Therapy (Grant No. 20170622011JC); Jilin Provincial Science and Technology Department (Grant Nos. 20180101009JC and 20190303146SF); Scientific and Technological Research of Jilin Provincial Education Department (Grant No. JJKH20190023KJ); and Jilin Province Finance Department (Grant No. 2018SCZWSZX-010).
Conflict of Interest
The authors declare that the research was conducted in the absence of any commercial or financial relationships that could be construed as a potential conflict of interest.
Abbreviations
Ab, antibody; ALK, anaplastic lymphoma kinase; β-AR, β-adrenergic receptors; mAb, monoclonal antibody; NGF, nerve growth factor; HNSCC, head and neck squamous cell carcinoma; PCa, prostate cancer; PDAC, pancreatic ductal adenocarcinoma; ROS1, ROS proto-oncogene 1; TRK, tyrosine receptor kinase.
References
Abdel-Hamid, N. M., Shehata, D. E., Abdel-ghany, A. A., Ragaa, A., and Wahid, A. (2016). Serum serotonin as unexpected potential marker for staging of experimental hepatocellular carcinoma. Biomed. Pharmacother. 83, 407–411. doi: 10.1016/j.biopha.2016.07.005
Adriaenssens, E., Vanhecke, E., Saule, P., Mougel, A., Page, A., Romon, R., et al. (2008). Nerve growth factor is a potential therapeutic target in breast cancer. Cancer Res. 68, 346–351. doi: 10.1158/0008-5472.CAN-07-1183
Åhsberg, K., Olsson, H., and Staël von Holstein, C. (2009). Increased mortality in prostate carcinoma and smoking-related disease after parietal cell vagotomy: a long-term follow-up study. Scand. J. Gastroenterol. 44, 947–951. doi: 10.1080/00365520903039945
Airaksinen, M. S., and Saarma, M. (2002). The GDNF family: signalling, biological functions and therapeutic value. Na. Rev. Neurosci. 3, 383–394. doi: 10.1038/nrn812
Albo, D., Akay, C. L., Marshall, C. L., Wilks, J. A., Verstovsek, G., Liu, H., et al. (2011). Neurogenesis in colorectal cancer is a marker of aggressive tumor behavior and poor outcomes. Cancer 117, 4834–4845. doi: 10.1002/cncr.26117
Allen, J. K., Armaiz-Pena, G. N., Nagaraja, A. S., Sadaoui, N. C., Ortiz, T., Dood, R., et al. (2018). Sustained adrenergic signaling promotes intratumoral innervation through BDNF induction. Cancer Res. 78, 3233–3242. doi: 10.1158/0008-5472.can-16-1701
Alpini, G., Invernizzi, P., Gaudio, E., Venter, J., Kopriva, S., Bernuzzi, F., et al. (2008). Serotonin metabolism is dysregulated in cholangiocarcinoma, which has implications for tumor growth. Cancer Res. 68, 9184–9193. doi: 10.1158/0008-5472.CAN-08-2133
Amit, M., Binenbaum, Y., Trejo-Leider, L., Sharma, K., Ramer, N., Ramer, I., et al. (2015). International collaborative validation of intraneural invasion as a prognostic marker in adenoid cystic carcinoma of the head and neck. Head Neck 37, 1038–1045. doi: 10.1002/hed.23710
Antonica, A., Magni, F., Mearini, L., and Paolocci, N. (1994). Vagal control of lymphocyte release from rat thymus. J. Auton. Nervous Sys. 48, 187–197. doi: 10.1016/0165-1838(94)90047-7
Arévalo, J. C., and Wu, S. H. (2006). Neurotrophin signaling: many exciting surprises! Cell Mol. Life Sci. 63, 1523–1537. doi: 10.1007/s00018-006-6010-1
Ayala, G. E., Dai, H., Powell, M., Li, R., Ding, Y., Wheeler, T. M., et al. (2008). Cancer-related axonogenesis and neurogenesis in prostate cancer. Clin. Cancer Res. 14, 7593–7603. doi: 10.1158/1078-0432.ccr-08-1164
Bapat, A. A., Hostetter, G., Von Hoff, D. D., and Han, H. (2011). Perineural invasion and associated pain in pancreatic cancer. Nat. Rev. Cancer 11, 695–707. doi: 10.1038/nrc3131
Ben, Q. W., Wang, J. C., Liu, J., Zhu, Y., Yuan, F., Yao, W. Y., et al. (2010). Positive expression of L1-CAM is associated with perineural invasion and poor outcome in pancreatic ductal adenocarcinoma. Ann. Surg. Oncol. 17, 2213–2221. doi: 10.1245/s10434-010-0955-x
Bentley, C. A., and Lee, K. F. (2000). p75 is important for axon growth and schwann cell migration during development. J. Neurosci. 20, 7706–7715. doi: 10.1523/jneurosci.20-20-07706.2000
Biankin, A. V., Waddell, N., Kassahn, K. S., Gingras, M. C., Muthuswamy, L. B., Johns, A. L., et al. (2012). Pancreatic cancer genomes reveal aberrations in axon guidance pathway genes. Nature 491, 399–405. doi: 10.1038/nature11547
Bloom, A. P., Jimenez-Andrade, J. M., Taylor, R. N., Castañeda-Corral, G., Kaczmarska, M. J., Freeman, K. T., et al. (2011). Breast cancer-induced bone remodeling, skeletal pain, and sprouting of sensory nerve fibers. J. Pain 12, 698–711. doi: 10.1016/j.jpain.2010.12.016
Boerman, R. H., Maassen, E. M., Joosten, J., Kaanders, H. A., Marres, H. A., van Overbeeke, J., et al. (1999). Trigeminal neuropathy secondary to perineural invasion of head and neck carcinomas. Neurology 53, 213–216. doi: 10.1212/wnl.53.1.213
Boilly, B., Faulkner, S., Jobling, P., and Hondermarck, H. (2017). Nerve dependence: from regeneration to cancer. Cancer Cell 31, 342–354. doi: 10.1016/j.ccell.2017.02.005
Bouzas-Rodriguez, J., Cabrera, J. R., Delloye-Bourgeois, C., Ichim, G., Delcros, J. G., Raquin, M. A., et al. (2010). Neurotrophin-3 production promotes human neuroblastoma cell survival by inhibiting TrkC-induced apoptosis. J. Clin. Invest. 120, 850–858. doi: 10.1172/jci41013
Brown, J. P., Couillard-Després, S., Cooper-Kuhn, C. M., Winkler, J., Aigner, L., and Kuhn, H. G. (2003). Transient expression of doublecortin during adult neurogenesis. J. Comp. Neurol. 467, 1–10. doi: 10.1002/cne.10874
Cavel, O., Shomron, O., Shabtay, A., Vital, J., Trejo-Leider, L., Weizman, N., et al. (2012). Endoneurial macrophages induce perineural invasion of pancreatic cancer cells by secretion of GDNF and activation of RET tyrosine kinase receptor. Cancer Res. 72, 5733–5743. doi: 10.1158/0008-5472.can-12-0764
Ceyhan, G. O., Demir, I. E., Altintas, B., Rauch, U., Thiel, G., Müller, M. W., et al. (2008). Neural invasion in pancreatic cancer: a mutual tropism between neurons and cancer cells. Biochem. Biophys. Res. Commun. 374, 442–447. doi: 10.1016/j.bbrc.2008.07.035
Chao, M. V. (2003). Neurotrophins and their receptors: a convergence point for many signalling pathways. Nat. Rev. Neurosci. 4, 299–309. doi: 10.1038/nrn1078
Chatterjee, D., Katz, M. H., Rashid, A., Wang, H., Iuga, A. C., Varadhachary, G. R., et al. (2012). Perineural and intraneural invasion in posttherapy pancreaticoduodenectomy specimens predicts poor prognosis in patients with pancreatic ductal adenocarcinoma. Am. J. Surg. Pathol. 36, 409–417. doi: 10.1097/PAS.0b013e31824104c5
Chen, H., Zhang, W., Cheng, X., Guo, L., Xie, S., Ma, Y., et al. (2017). β2-AR activation induces chemoresistance by modulating p53 acetylation through upregulating Sirt1 in cervical cancer cells. Cancer Sci. 108, 1310–1317. doi: 10.1111/cas.13275
Chida, Y., Hamer, M., Wardle, J., and Steptoe, A. (2008). Do stress-related psychosocial factors contribute to cancer incidence and survival? Nat. Clin. Pract. Oncol. 5, 466–475. doi: 10.1038/ncponc1134
Chung, K., Wallace, J., Kim, S. Y., Kalyanasundaram, S., Andalman, A. S., Davidson, T. J., et al. (2013). Structural and molecular interrogation of intact biological systems. Nature 497, 332–337. doi: 10.1038/nature12107
Cohnen, J., Kornstädt, L., Hahnefeld, L., Ferreiros, N., Pierre, S., Koehl, U., et al. (2020). Tumors provoke inflammation and perineural microlesions at adjacent peripheral nerves. Cells 9:320. doi: 10.3390/cells9020320
Cole, S. W., Nagaraja, A. S., Lutgendorf, S. K., Green, P. A., and Sood, A. K. (2015). Sympathetic nervous system regulation of the tumour microenvironment. Nat. Rev. Cancer 15, 563–572. doi: 10.1038/nrc3978
Dai, S., Mo, Y., Wang, Y., Xiang, B., Liao, Q., Zhou, M., et al. (2020). Chronic stress promotes cancer development. Front. Oncol. 10:1492. doi: 10.3389/fonc.2020.01492
Dantzer, R. (2018). Neuroimmune interactions: from the brain to the immune system and vice versa. Physiol. Rev. 98, 477–504. doi: 10.1152/physrev.00039.2016
De Lorenzo, B. H., de Oliveira Marchioro, L., Greco, C. R., and Suchecki, D. (2015). Sleep-deprivation reduces NK cell number and function mediated by β-adrenergic signalling. Psychoneuroendocrinology 57, 134–143. doi: 10.1016/j.psyneuen.2015.04.006
Deborde, S., Omelchenko, T., Lyubchik, A., Zhou, Y., He, S., McNamara, W. F., et al. (2016). Schwann cells induce cancer cell dispersion and invasion. J. Clin. Invest. 126, 1538–1554. doi: 10.1172/JCI82658
Demir, I. E., Boldis, A., Pfitzinger, P. L., Teller, S., Brunner, E., Klose, N., et al. (2014). Investigation of Schwann cells at neoplastic cell sites before the onset of cancer invasion. J. Natl. Cancer Inst. 106:dju18. doi: 10.1093/jnci/dju184
Demir, I. E., Friess, H., and Ceyhan, G. O. (2015). Neural plasticity in pancreatitis and pancreatic cancer. Nat. Rev. Gastroenterol. Hepatol. 12, 649–659. doi: 10.1038/nrgastro.2015.166
Dézsi, C. A., and Szentes, V. (2017). The real role of β-Blockers in daily cardiovascular therapy. Am. J. Cardiovasc. Drugs 17, 361–373. doi: 10.1007/s40256-017-0221-8
Ding, Y., He, D., Florentin, D., Frolov, A., Hilsenbeck, S., Ittmann, M., et al. (2013). Semaphorin 4F as a critical regulator of neuroepithelial interactions and a biomarker of aggressive prostate cancer. Clin. Cancer Res. 19, 6101–6111. doi: 10.1158/1078-0432.ccr-12-3669
Douma, S., Van Laar, T., Zevenhoven, J., Meuwissen, R., Van Garderen, E., and Peeper, D. S. (2004). Suppression of anoikis and induction of metastasis by the neurotrophic receptor TrkB. Nature 430, 1034–1039. doi: 10.1038/nature02765
Dubeykovskaya, Z., Si, Y., Chen, X., Worthley, D. L., Renz, B. W., Urbanska, A. M., et al. (2016). Neural innervation stimulates splenic TFF2 to arrest myeloid cell expansion and cancer. Nat. Commun. 7:10517. doi: 10.1038/ncomms10517
Faulkner, S., Jobling, P., March, B., Jiang, C. C., and Hondermarck, H. (2019). Tumor neurobiology and the war of nerves in cancer. Cancer Discov. 9, 702–710. doi: 10.1158/2159-8290.cd-18-1398
Feng, Z., Liu, L., Zhang, C., Zheng, T., Wang, J., Lin, M., et al. (2012). Chronic restraint stress attenuates p53 function and promotes tumorigenesis. Proc. Natl. Acad. Sci. U.S.A. 109, 7013–7018. doi: 10.1073/pnas.1203930109
Fujikawa, H., Tanaka, K., Toiyama, Y., Saigusa, S., Inoue, Y., Uchida, K., et al. (2012). High TrkB expression levels are associated with poor prognosis and EMT induction in colorectal cancer cells. J. Gastroenterol. 47, 775–784. doi: 10.1007/s00535-012-0532-0
García-González, D., Khodosevich, K., Watanabe, Y., Rollenhagen, A., Lübke, J. H. R., and Monyer, H. (2017). Serotonergic projections govern postnatal neuroblast migration. Neuron 94, 534.e9–549.e9. doi: 10.1016/j.neuron.2017.04.013
Gil, Z., Cavel, O., Kelly, K., Brader, P., Rein, A., Gao, S. P., et al. (2010). Paracrine regulation of pancreatic cancer cell invasion by peripheral nerves. J. Natl. Cancer Inst. 102, 107–118. doi: 10.1093/jnci/djp456
Grytli, H. H., Fagerland, M. W., Fosså, S. D., and Taskén, K. A. (2014). Association between use of β-blockers and prostate cancer–specific survival: a cohort study of 3561 prostate cancer patients with high-risk or metastatic disease. Eur. Urol. 65, 635–641. doi: 10.1016/j.eururo.2013.01.007
Guo, D., Hou, X., Zhang, H., Sun, W., Zhu, L., Liang, J., et al. (2011). More expressions of BDNF and TrkB in multiple hepatocellular carcinoma and anti-BDNF or K252a induced apoptosis, supressed invasion of HepG2 and HCCLM3 cells. J. Exp. Clin. Cancer Res. 30:97. doi: 10.1186/1756-9966-30-97
Guo, K., Ma, Q., Li, J., Wang, Z., Shan, T., Li, W., et al. (2013). Interaction of the sympathetic nerve with pancreatic cancer cells promotes perineural invasion through the activation of STAT3 signaling. Mol. Cancer Ther, 12, 264–273. doi: 10.1158/1535-7163.mct-12-0809
Hayakawa, Y., Sakitani, K., Konishi, M., Asfaha, S., Niikura, R., Tomita, H., et al. (2017). Nerve growth factor promotes gastric tumorigenesis through aberrant cholinergic signaling. Cancer Cell 31, 21–34. doi: 10.1016/j.ccell.2016.11.005
He, S., He, S., Chen, C. H., Deborde, S., Bakst, R. L., Chernichenko, N., et al. (2015). The chemokine (CCL2-CCR2) signaling axis mediates perineural invasion. Mol. Cancer Res. 13, 380–390. doi: 10.1158/1541-7786.mcr-14-0303
Heidt, T., Sager, H. B., Courties, G., Dutta, P., Iwamoto, Y., and Zaltsman, A. (2014). Chronic variable stress activates hematopoietic stem cells. Nat. Med. 20, 754–758. doi: 10.1038/nm.3589
Hondermarck, H. (2012). Neurotrophins and their receptors in breast cancer. Cytokine Growth Factor Rev. 23, 357–365. doi: 10.1016/j.cytogfr.2012.06.004
Huan, H. B., Wen, X. D., Chen, X. J., Wu, L., Wu, L. L., Zhang, L., et al. (2017). Sympathetic nervous system promotes hepatocarcinogenesis by modulating inflammation through activation of alpha1-adrenergic receptors of Kupffer cells. Brain Behav. Immun. 59, 118–134. doi: 10.1016/j.bbi.2016.08.016
Huyett, P., Duvvuri, U., Ferris, R. L., Johnson, J. T., Schaitkin, B. M., and Kim, S. (2018). Perineural invasion in parotid gland malignancies. Otolaryngol. Head Neck Surg 158, 1035–1041. doi: 10.1177/0194599817751888
Inbar, S., Neeman, E., Avraham, R., Benish, M., Rosenne, E., and Ben-Eliyahu, S. (2011). Do stress responses promote leukemia progression? An animal study suggesting a role for epinephrine and prostaglandin-E2 through reduced NK activity. PLoS One 6:e19246. doi: 10.1371/journal.pone.0019246
Jessen, K. R., and Mirsky, R. (2016). The repair schwann cell and its function in regenerating nerves. J. Physiol. 594, 3521–3531. doi: 10.1113/jp270874
Jimenez-Andrade, J. M., Ghilardi, J. R., Castañeda-Corral, G., Kuskowski, M. A., and Mantyh, P. W. (2011). Preventive or late administration of anti-NGF therapy attenuates tumor-induced nerve sprouting, neuroma formation, and cancer pain. Pain 152, 2564–2574. doi: 10.1016/j.pain.2011.07.020
Johnson, M. D., Stone, B., Thibodeau, B. J., Baschnagel, A. M., Galoforo, S., Fortier, L. E., et al. (2017). The significance of Trk receptors in pancreatic cancer. Tumour. Biol. 39:1010428317692256. doi: 10.1177/1010428317692256
Kameda, K., Shimada, H., Ishikawa, T., Takimoto, A., Momiyama, N., Hasegawa, S., et al. (1999). Expression of highly polysialylated neural cell adhesion molecule in pancreatic cancer neural invasive lesion. Cancer Lett. 137, 201–207. doi: 10.1016/s0304-3835(98)00359-0
Kaminishi, M., Shimizu, N., Shimoyama, S., Yamaguchi, H., Tsuji, E., Aoki, F., et al. (1997). Denervation promotes the development of cancer-related lesions in the gastric remnant. J. Clin. Gastroenterol. 25(Suppl. 1), S129–S134. doi: 10.1097/00004836-199700001-00022
Kawamura, N., Kawamura, K., Manabe, M., and Tanaka, T. (2010). Inhibition of brain-derived neurotrophic factor/tyrosine kinase B signaling suppresses choriocarcinoma cell growth. Endocrinology 151, 3006–3014. doi: 10.1210/en.2009-1378
Kermani, P., Rafii, D., Jin, D. K., Whitlock, P., Schaffer, W., Chiang, A., et al. (2005). Neurotrophins promote revascularization by local recruitment of TrkB+ endothelial cells and systemic mobilization of hematopoietic progenitors. J. Clin. Invest. 115, 653–663. doi: 10.1172/jci22655
Klein, G., Vellenga, E., Fraaije, M. W., Kamps, W. A., and de Bont, E. S. (2004). The possible role of matrix metalloproteinase (MMP)-2 and MMP-9 in cancer, e.g. acute leukemia. Crit. Rev. Oncol. Hematol. 50, 87–100. doi: 10.1016/j.critrevonc.2003.09.001
Kokolus, K. M., Capitano, M. L., Lee, C.-T., Eng, J. W. L., Waight, J. D., Hylander, B. L., et al. (2013). Baseline tumor growth and immune control in laboratory mice are significantly influenced by subthermoneutral housing temperature. Proc. Natl. Acad. Sci. U.S.A. 110:20176. doi: 10.1073/pnas.1304291110
Kokolus, K. M., Zhang, Y., Sivik, J. M., Schmeck, C., Zhu, J., Repasky, E. A., et al. (2018). Beta blocker use correlates with better overall survival in metastatic melanoma patients and improves the efficacy of immunotherapies in mice. OncoImmunology 7:e1405205. doi: 10.1080/2162402X.2017.1405205
Kuol, N., Stojanovska, L., Apostolopoulos, V., and Nurgali, K. (2018). Role of the nervous system in cancer metastasis. J. Exp. Clin. Cancer Res. 37:5. doi: 10.1186/s13046-018-0674-x
Lagadec, C., Meignan, S., Adriaenssens, E., Foveau, B., Vanhecke, E., Romon, R., et al. (2009). TrkA overexpression enhances growth and metastasis of breast cancer cells. Oncogene 28, 1960–1970. doi: 10.1038/onc.2009.61
Laurent, D., Eric, A., Ikram El, Y.-B., Xuefen Le, B., Victor, N., and Hubert, H. (2004). Nerve growth factor receptors and signaling in breast cancer. Curr. Cancer Drug Targets 4, 463–470. doi: 10.2174/1568009043332853
Le, C. P., Nowell, C. J., Kim-Fuchs, C., Botteri, E., Hiller, J. G., Ismail, H., et al. (2016). Chronic stress in mice remodels lymph vasculature to promote tumour cell dissemination. Nat. Commun. 7:10634. doi: 10.1038/ncomms10634
Li, J., Yang, X. M., Wang, Y. H., Feng, M. X., Liu, X. J., Zhang, Y. L., et al. (2014). Monoamine oxidase A suppresses hepatocellular carcinoma metastasis by inhibiting the adrenergic system and its transactivation of EGFR signaling. J. Hepatol. 60, 1225–1234. doi: 10.1016/j.jhep.2014.02.025
Lillberg, K., Verkasalo, P. K., Kaprio, J., Teppo, L., Helenius, H., and Koskenvuo, M. (2003). Stressful life events and risk of breast cancer in 10,808 women: a cohort study. Am. J. Epidemiol. 157, 415–423. doi: 10.1093/aje/kwg002
Liu, X., Yang, X., Zhan, C., Zhang, Y., Hou, J., and Yin, X. (2020). Perineural invasion in adenoid cystic carcinoma of the salivary glands: where we are and where we need to go. Front. Oncol. 10:1493. doi: 10.3389/fonc.2020.01493
Lois, C., and Alvarez-Buylla, A. (1994). Long-distance neuronal migration in the adult mammalian brain. Science 264, 1145–1148. doi: 10.1126/science.8178174
Lutgendorf, S. K., Lamkin, D. M., Jennings, N. B., Arevalo, J. M., Penedo, F., DeGeest, K., et al. (2008). Biobehavioral influences on matrix metalloproteinase expression in ovarian carcinoma. Clin. Cancer Res. 14, 6839–6846. doi: 10.1158/1078-0432.ccr-08-0230
Ma, C., Gao, T., Ju, J., Zhang, Y., Ni, Q., Li, Y., et al. (2019). Sympathetic innervation contributes to perineural invasion of salivary adenoid cystic carcinoma via the β2-adrenergic receptor. Onco Targets Ther. 12, 1475–1495. doi: 10.2147/ott.s190847
Ma, J., Jiang, Y., Jiang, Y., Sun, Y., and Zhao, X. (2008). Expression of nerve growth factor and tyrosine kinase receptor A and correlation with perineural invasion in pancreatic cancer. J. Gastroenterol. Hepatol. 23, 1852–1859. doi: 10.1111/j.1440-1746.2008.05579.x
Madden, K. S., Szpunar, M. J., and Brown, E. B. (2011). β-Adrenergic receptors (β-AR) regulate VEGF and IL-6 production by divergent pathways in high β-AR-expressing breast cancer cell lines. Breast Cancer Res. Treat. 130, 747–758. doi: 10.1007/s10549-011-1348-y
Madeo, M., Colbert, P. L., Vermeer, D. W., Lucido, C. T., Cain, J. T., Vichaya, E. G., et al. (2018). Cancer exosomes induce tumor innervation. Nat. Commun. 9:4284. doi: 10.1038/s41467-018-06640-0
Magnon, C. (2015). Role of the autonomic nervous system in tumorigenesis and metastasis. Mol. Cell Oncol. 2, e975643. doi: 10.4161/23723556.2014.975643
Magnon, C., Hall, S. J., Lin, J., Xue, X., Gerber, L., Freedland, S. J., et al. (2013). Autonomic nerve development contributes to prostate cancer progression. Science 341:1236361. doi: 10.1126/science.1236361
March, B., Faulkner, S., Jobling, P., Steigler, A., Blatt, A., Denham, J., et al. (2020). Tumour innervation and neurosignalling in prostate cancer. Nat. Rev. Urol. 17, 119–130. doi: 10.1038/s41585-019-0274-3
Marchesi, F., Locatelli, M., Solinas, G., Erreni, M., Allavena, P., and Mantovani, A. (2010a). Role of CX3CR1/CX3CL1 axis in primary and secondary involvement of the nervous system by cancer. J. Neuroimmunol. 224, 39–44. doi: 10.1016/j.jneuroim.2010.05.007
Marchesi, F., Piemonti, L., Mantovani, A., and Allavena, P. (2010b). Molecular mechanisms of perineural invasion, a forgotten pathway of dissemination and metastasis. Cytokine Growth Factor Rev. 21, 77–82. doi: 10.1016/j.cytogfr.2009.11.001
Marshall, J. L., Kindler, H., Deeken, J., Bhargava, P., Vogelzang, N. J., Rizvi, N., et al. (2005). Phase I trial of orally administered CEP-701, a novel neurotrophin receptor-linked tyrosine kinase inhibitor. Invest. New Drugs 23, 31–37. doi: 10.1023/B:DRUG.0000047103.64335.b0
Mauffrey, P., Tchitchek, N., Barroca, V., Bemelmans, A.-P., Firlej, V., Allory, Y., et al. (2019). Progenitors from the central nervous system drive neurogenesis in cancer. Nature 569, 672–678. doi: 10.1038/s41586-019-1219-y
McCaffrey, G., Thompson, M. L., Majuta, L., Fealk, M. N., Chartier, S., Longo, G., et al. (2014). NGF blockade at early times during bone cancer development attenuates bone destruction and increases limb use. Cancer Res. 74, 7014–7023. doi: 10.1158/0008-5472.CAN-14-1220
Medeiros, P. J., and Jackson, D. N. (2013). Neuropeptide Y Y5-receptor activation on breast cancer cells acts as a paracrine system that stimulates VEGF expression and secretion to promote angiogenesis. Peptides 48, 106–113. doi: 10.1016/j.peptides.2013.07.029
Mejia-Gervacio, S., Murray, K., and Lledo, P. M. (2011). NKCC1 controls GABAergic signaling and neuroblast migration in the postnatal forebrain. Neural Dev. 6:4. doi: 10.1186/1749-8104-6-4
Mizumura, K., and Murase, S. (2015). Role of nerve growth factor in pain. Handb. Exp. Pharmacol. 227, 57–77. doi: 10.1007/978-3-662-46450-2_4
Müller, M. W., Giese, N. A., Swiercz, J. M., Ceyhan, G. O., Esposito, I., Hinz, U., et al. (2007). Association of axon guidance factor semaphorin 3A with poor outcome in pancreatic cancer. Int. J. Cancer 121, 2421–2433. doi: 10.1002/ijc.22949
Murakami, Y., Uemura, K., Sudo, T., Hashimoto, Y., Kondo, N., Nakagawa, N., et al. (2013). Perineural invasion in extrahepatic cholangiocarcinoma: prognostic impact and treatment strategies. J. Gastrointest. Surg. 17, 1429–1439. doi: 10.1007/s11605-013-2251-0
Murillo-Sauca, O., Chung, M. K., Shin, J. H., Karamboulas, C., Kwok, S., Jung, Y. H., et al. (2014). CD271 is a functional and targetable marker of tumor-initiating cells in head and neck squamous cell carcinoma. Oncotarget 5, 6854–6866. doi: 10.18632/oncotarget.2269
Na’ara, S., Amit, M., and Gil, Z. (2019). L1CAM induces perineural invasion of pancreas cancer cells by upregulation of metalloproteinase expression. Oncogene 38, 596–608. doi: 10.1038/s41388-018-0458-y
Nakai, A., Hayano, Y., Furuta, F., Noda, M., and Suzuki, K. (2014). Control of lymphocyte egress from lymph nodes through β2-adrenergic receptors. J. Exp. Med. 211, 2583–2598. doi: 10.1084/jem.20141132
Nevin, J. T., Moussa, M., Corwin, W. L., Mandoiu, I. I., and Srivastava, P. K. (2020). Sympathetic nervous tone limits the development of myeloid-derived suppressor cells. Sci. Immunol. 5:eaay9368. doi: 10.1126/sciimmunol.aay9368
Nissen, M. D., Sloan, E. K., and Mattarollo, S. R. (2018). β-Adrenergic signaling impairs antitumor CD8+ T-cell responses to B-cell Lymphoma immunotherapy. Cancer Immunol. Res. 6, 98–109. doi: 10.1158/2326-6066.CIR-17-0401
Okugawa, Y., Tanaka, K., Inoue, Y., Kawamura, M., Kawamoto, A., Hiro, J., et al. (2013). Brain-derived neurotrophic factor/tropomyosin-related kinase B pathway in gastric cancer. Br. J. Cancer 108, 121–130. doi: 10.1038/bjc.2012.499
Peinado, H., Zhang, H., Matei, I. R., Costa-Silva, B., Hoshino, A., Rodrigues, G., et al. (2017). Pre-metastatic niches: organ-specific homes for metastases. Nat. Rev. Cancer 17, 302–317. doi: 10.1038/nrc.2017.6
Peters, C. M., Ghilardi, J. R., Keyser, C. P., Kubota, K., Lindsay, T. H., Luger, N. M., et al. (2005). Tumor-induced injury of primary afferent sensory nerve fibers in bone cancer pain. Exp. Neurol. 193, 85–100. doi: 10.1016/j.expneurol.2004.11.028
Peterson, S. C., Eberl, M., Vagnozzi, A. N., Belkadi, A., Veniaminova, N. A., Verhaegen, M. E., et al. (2015). Basal cell carcinoma preferentially arises from stem cells within hair follicle and mechanosensory niches. Cell Stem Cell 16, 400–412. doi: 10.1016/j.stem.2015.02.006
Pillai, S., and Chellappan, S. (2012). α7 nicotinic acetylcholine receptor subunit in angiogenesis and epithelial to mesenchymal transition. Curr. Drug Targets 13, 671–679. doi: 10.2174/138945012800398847
Pundavela, J., Demont, Y., Jobling, P., Lincz, L. F., Roselli, S., Thorne, R. F., et al. (2014). ProNGF correlates with Gleason score and is a potential driver of nerve infiltration in prostate cancer. Am. J. Pathol. 184, 3156–3162. doi: 10.1016/j.ajpath.2014.08.009
Qin, J. F., Jin, F. J., Li, N., Guan, H. T., Lan, L., Ni, H., et al. (2015). Adrenergic receptor β2 activation by stress promotes breast cancer progression through macrophages M2 polarization in tumor microenvironment. BMB Rep. 48, 295–300. doi: 10.5483/bmbrep.2015.48.5.008
Reiche, E. M., Nunes, S. O., and Morimoto, H. K. (2004). Stress, depression, the immune system, and cancer. Lancet Oncol. 5, 617–625. doi: 10.1016/s1470-2045(04)01597-9
Renz, B. W., Tanaka, T., Sunagawa, M., Takahashi, R., Jiang, Z., Macchini, M., et al. (2018). Cholinergic signaling via muscarinic receptors directly and indirectly suppresses pancreatic tumorigenesis and cancer stemness. Cancer Discov. 8, 1458–1473. doi: 10.1158/2159-8290.cd-18-0046
Rozniecki, J. J., Sahagian, G. G., Kempuraj, D., Tao, K., Jocobson, S., Zhang, B., et al. (2010). Brain metastases of mouse mammary adenocarcinoma is increased by acute stress. Brain Res. 1366, 204–210. doi: 10.1016/j.brainres.2010.09.085
Rutledge, A., Jobling, P., Walker, M. M., Denham, J. W., and Hondermarck, H. (2017). Spinal cord injuries and nerve dependence in prostate cancer. Trends Cancer 3, 812–815. doi: 10.1016/j.trecan.2017.10.001
Saloman, J. L., Albers, K. M., Li, D., Hartman, D. J., Crawford, H. C., Muha, E. A., et al. (2016a). Ablation of sensory neurons in a genetic model of pancreatic ductal adenocarcinoma slows initiation and progression of cancer. Proc. Natl. Acad. Sci. U.S.A. 113, 3078–3083. doi: 10.1073/pnas.1512603113
Saloman, J. L., Albers, K. M., Rhim, A. D., and Davis, B. M. (2016b). Can stopping nerves. Stop Cancer? Trends Neurosci 39, 880–889. doi: 10.1016/j.tins.2016.10.002
Sarrouilhe, D., and Mesnil, M. (2019). Serotonin and human cancer: a critical view. Biochimie 161, 46–50. doi: 10.1016/j.biochi.2018.06.016
Sclabas, G. M., Fujioka, S., Schmidt, C., Li, Z., Frederick, W. A., Yang, W., et al. (2005). Overexpression of tropomysin-related kinase B in metastatic human pancreatic cancer cells. Clin. Cancer Res. 11(2 Pt 1), 440–449.
Shang, J., Sheng, L., Wang, K., Shui, Y., and Wei, Q. (2007). Expression of neural cell adhesion molecule in salivary adenoid cystic carcinoma and its correlation with perineural invasion. Oncol. Rep. 18, 1413–1416.
Sierra, J. R., Corso, S., Caione, L., Cepero, V., Conrotto, P., Cignetti, A., et al. (2008). Tumor angiogenesis and progression are enhanced by Sema4D produced by tumor-associated macrophages. J. Exp. Med. 205, 1673–1685. doi: 10.1084/jem.20072602
Sood, A. K., Bhatty, R., Kamat, A. A., Landen, C. N., Han, L., Thaker, P. H., et al. (2006). Stress hormone-mediated invasion of ovarian cancer cells. Clin. Cancer Res. 12, 369–375. doi: 10.1158/1078-0432.CCR-05-1698
Stopczynski, R. E., Normolle, D. P., Hartman, D. J., Ying, H., DeBerry, J. J., Bielefeldt, K., et al. (2014). Neuroplastic changes occur early in the development of pancreatic ductal adenocarcinoma. Cancer Res. 74, 1718–1727. doi: 10.1158/0008-5472.CAN-13-2050
Thaker, P. H., Han, L. Y., Kamat, A. A., Arevalo, J. M., Takahashi, R., Lu, C., et al. (2006). Chronic stress promotes tumor growth and angiogenesis in a mouse model of ovarian carcinoma. Nat. Med. 12, 939–944. doi: 10.1038/nm1447
Ueshima, H., Inada, T., and Shingu, K. (2013). Suppression of phagosome proteolysis and Matrigel migration with the α2-adrenergic receptor agonist dexmedetomidine in murine dendritic cells. Immunopharmacol. Immunotoxicol. 35, 558–566. doi: 10.3109/08923973.2013.822509
Urabe, K., Murakami, Y., Kondo, N., Uemura, K., Hashimoto, Y., Nakagawa, N., et al. (2016). Nerve growth factor expression is not associated with perineural invasion in extrahepatic cholangiocarcinoma. Dig. Dis. Sci. 61, 774–784. doi: 10.1007/s10620-015-3953-9
Veit, C., Genze, F., Menke, A., Hoeffert, S., Gress, T. M., Gierschik, P., et al. (2004). Activation of phosphatidylinositol 3-kinase and extracellular signal-regulated kinase is required for glial cell line-derived neurotrophic factor-induced migration and invasion of pancreatic carcinoma cells. Cancer Res. 64, 5291–5300. doi: 10.1158/0008-5472.can-04-1112
Venkatesh, H., and Monje, M. (2017). Neuronal activity in ontogeny and oncology. Trends Cancer 3, 89–112. doi: 10.1016/j.trecan.2016.12.008
Venkatesh, H. S., Johung, T. B., Caretti, V., Noll, A., Tang, Y., Nagaraja, S., et al. (2015). Neuronal activity promotes glioma growth through neuroligin-3 secretion. Cell 161, 803–816. doi: 10.1016/j.cell.2015.04.012
Voronina, N., Wong, J. K. L., Hubschmann, D., Hlevnjak, M., Uhrig, S., Heilig, C. E., et al. (2020). The landscape of chromothripsis across adult cancer types. Nat. Commun. 11:2320. doi: 10.1038/s41467-020-16134-7
Walker, A. K., Martelli, D., Ziegler, A. I., Lambert, G. W., Phillips, S. E., Hill, S. J., et al. (2019). Circulating epinephrine is not required for chronic stress to enhance metastasis. Psychoneuroendocrinology 99, 191–195. doi: 10.1016/j.psyneuen.2018.09.012
Wang, K., Demir, I. E., D’Haese, J. G., Tieftrunk, E., Kujundzic, K., Schorn, S., et al. (2014). The neurotrophic factor neurturin contributes toward an aggressive cancer cell phenotype, neuropathic pain and neuronal plasticity in pancreatic cancer. Carcinogenesis 35, 103–113. doi: 10.1093/carcin/bgt312
Wang, W., Zhao, H., Zhang, S., Kang, E., Chen, Y., Ni, C., et al. (2009). Patterns of expression and function of the p75(NGFR) protein in pancreatic cancer cells and tumours. Eur. J. Surg. Oncol. 35, 826–832. doi: 10.1016/j.ejso.2008.10.013
Yamaguchi, R., Nagino, M., Oda, K., Kamiya, J., Uesaka, K., and Nimura, Y. (2002). Perineural invasion has a negative impact on survival of patients with gallbladder carcinoma. Br. J. Surg. 89, 1130–1136. doi: 10.1046/j.1365-2168.2002.02184.x
Yamanaka, H., Obata, K., Kobayashi, K., Dai, Y., Fukuoka, T., and Noguchi, K. (2007). Alteration of the cell adhesion molecule L1 expression in a specific subset of primary afferent neurons contributes to neuropathic pain. Eur. J. Neurosci. 25, 1097–1111. doi: 10.1111/j.1460-9568.2007.05344.x
Yilmaz, T., Jiffar, T., de la Garza, G., Lin, H., Milas, Z., Takahashi, Y., et al. (2010). Theraputic targeting of Trk supresses tumor proliferation and enhances cisplatin activity in HNSCC. Cancer Biol. Ther. 10, 644–653. doi: 10.4161/cbt.10.6.12782
Yin, B., Ma, Z. Y., Zhou, Z. W., Gao, W. C., Du, Z. G., Zhao, Z. H., et al. (2015). The TrkB+ cancer stem cells contribute to post-chemotherapy recurrence of triple-negative breast cancers in an orthotopic mouse model. Oncogene 34, 761–770. doi: 10.1038/onc.2014.8
Yin, K., Shang, M., Dang, S., Wang, L., Xia, Y., Cui, L., et al. (2018). Netrin-1 induces the proliferation of gastric cancer cells via the ERK/MAPK signaling pathway and FAK activation. Oncol. Rep. 40, 2325–2333. doi: 10.3892/or.2018.6614
Yoshikawa, H., Kurokawa, M., Ozaki, N., Nara, K., Atou, K., Takada, E., et al. (2006). Nicotine inhibits the production of proinflammatory mediators in human monocytes by suppression of I-κB phosphorylation and nuclear factor-κB transcriptional activity through nicotinic acetylcholine receptor α7. Clin. Exp. Immunol. 146, 116–123. doi: 10.1111/j.1365-2249.2006.03169.x
Zahalka, A. H., Arnal-Estapé, A., Maryanovich, M., Nakahara, F., Cruz, C. D., Finley, L. W. S., et al. (2017). Adrenergic nerves activate an angio-metabolic switch in prostate cancer. Science 358, 321–326. doi: 10.1126/science.aah5072
Zarco, N., Norton, E., Quiñones-Hinojosa, A., and Guerrero-Cázares, H. (2019). Overlapping migratory mechanisms between neural progenitor cells and brain tumor stem cells. Cell Mol. Life Sci. 76, 3553–3570. doi: 10.1007/s00018-019-03149-7
Zhang, S., Qi, L., Li, M., Zhang, D., Xu, S., Wang, N., et al. (2008). Chemokine CXCL12 and its receptor CXCR4 expression are associated with perineural invasion of prostate cancer. J. Exp. Clin. Cancer Res. 27:62. doi: 10.1186/1756-9966-27-62
Zhao, C.-M., Hayakawa, Y., Kodama, Y., Muthupalani, S., Westphalen, C. B., Andersen, G. T., et al. (2014). Denervation suppresses gastric tumorigenesis. Sci. Transl. Med. 6:250ra115. doi: 10.1126/scitranslmed.3009569
Zhou, L., Li, Y., Li, X., Chen, G., Liang, H., Wu, Y., et al. (2016). Propranolol attenuates surgical stress–induced elevation of the regulatory T Cell response in patients undergoing radical mastectomy. J. Immunol. 196, 3460–3469. doi: 10.4049/jimmunol.1501677
Zhou, Z. H., Zhang, J. D., Zhao, H. B., and Wu, Y. Y. (2015). Diameter of involved nerves is a valuable prognostic factor for gastric cancer. Histol. Histopathol. 30, 1121–1127. doi: 10.14670/hh-11-609
Keywords: nerve, neurogenesis, cancer, perineural invasion, neurotrophins
Citation: Wang W, Li L, Chen N, Niu C, Li Z, Hu J and Cui J (2020) Nerves in the Tumor Microenvironment: Origin and Effects. Front. Cell Dev. Biol. 8:601738. doi: 10.3389/fcell.2020.601738
Received: 01 September 2020; Accepted: 30 November 2020;
Published: 17 December 2020.
Edited by:
Antonella Zannetti, Italian National Research Council, ItalyReviewed by:
Tang-Long Shen, National Taiwan University, TaiwanChristian Martin Stock, Hannover Medical School, Germany
Copyright © 2020 Wang, Li, Chen, Niu, Li, Hu and Cui. This is an open-access article distributed under the terms of the Creative Commons Attribution License (CC BY). The use, distribution or reproduction in other forums is permitted, provided the original author(s) and the copyright owner(s) are credited and that the original publication in this journal is cited, in accordance with accepted academic practice. No use, distribution or reproduction is permitted which does not comply with these terms.
*Correspondence: Jifan Hu, amlmYW5Ac3RhbmZvcmQuZWR1; Jiuwei Cui, Y3VpandAamx1LmVkdS5jbg==