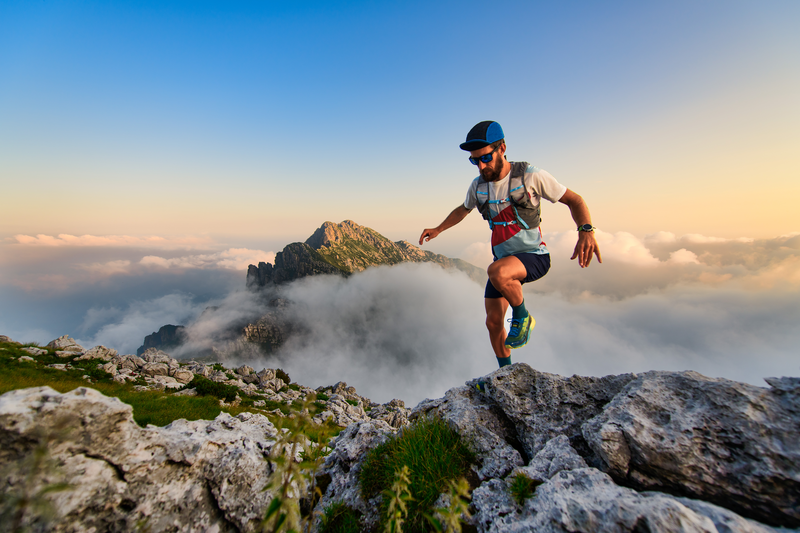
94% of researchers rate our articles as excellent or good
Learn more about the work of our research integrity team to safeguard the quality of each article we publish.
Find out more
REVIEW article
Front. Cell Dev. Biol. , 30 September 2020
Sec. Cell Growth and Division
Volume 8 - 2020 | https://doi.org/10.3389/fcell.2020.575706
Mammalian ovaries consist of follicles as basic functional units. Each follicle comprised an innermost oocyte and several surrounding flattened granulosa cells. Unlike males, according to the initial size of the primordial follicle pool and the rate of its activation and depletion, a female’s reproductive life has been determined early in life. Primordial follicles, once activated, will get into an irreversible process of development. Most follicles undergo atretic degeneration, and only a few of them could mature and ovulate. Although there are a lot of researches contributing to exploring the activation of primordial follicles, little is known about its underlying mechanisms. Thus, in this review, we collected the latest papers and summarized the signaling pathways as well as some factors involved in the activation of primordial follicles, hoping to lead to a more profound understanding of the cellular and molecular mechanisms of primordial follicle activation.
It is universally accepted that the total population of primordial follicles within the postnatal mammalian ovary will not increase and be used up gradually (McGee and Hsueh, 2000; Maidarti et al., 2020). Primordial follicles are the reproductive units of the mammalian ovary that are composed of diplotene oocytes surrounded by a layer of flattened granulosa cells (Adhikari and Liu, 2009; Hsueh et al., 2015). They remain in a dormant phase until being recruited into the growing pool via a process known as primordial follicular activation (Lee and Chang, 2019; Wright et al., 2020). Physiologically, only a limited number of primordial follicles are activated to enter the growing follicle pool each wave (Hsueh et al., 2015; Zhang and Liu, 2015). The pool of primordial follicles drops sharply with the increase of a woman’s age, especially after 35 years (Hansen et al., 2008; Lew, 2019). When about 1,000 primordial follicles remain, this can lead to fertility cessation and the onset of menopause (Hansen et al., 2008; Ford et al., 2020). Therefore, the number of primordial follicles in the ovary determines the female reproductive ability (Oktem and Urman, 2010; Findlay et al., 2015).
Primordial follicle activation is characterized by the robust growth of oocyte and morphological changes of primordial follicle granulosa cells (pfGCs), i.e., the flattened pfGCs differentiate into cuboidal granulosa cells and then begin to proliferate (Liu et al., 2006; Hsueh et al., 2015). However, activation is irreversible. If the activated follicles are not selected for completing the process (culminating in ovulation), those follicles will undergo atresia at some stage of their maturation (John et al., 2008), which is a blow for infertile women with a diminishing ovarian reserve. But a global activation of all primordial follicles inevitably causes early exhaustion of the follicle pool and premature ovarian failure (POF) (Kawamura et al., 2016; Kallen et al., 2018). Given that dormant primordial follicles are the most abundant fertility reserve in female mammals and could be found in women with various ovarian diseases, using them for clinical treatment of female infertility is an excellent idea (Song et al., 2015; Zhang and Liu, 2015; Park et al., 2020). A new infertility treatment, called in vitro activation (IVA), was developed, which enables POF patients to conceive using their own eggs by the activation of residual dormant follicles (Kawamura et al., 2013, 2016). The birth of a healthy baby with the use of phosphatase and tensin homolog deleted on chromosome 10 (PTEN) inhibitors is a compelling evidence (Kawamura et al., 2013).
However, research on the activation of human primordial follicles is hindered due to limited access to tissue samples. Therefore, studies on the activation of primordial follicles have been largely performed in mice to assess its factors and pathways. Here, we will introduce the intracellular signaling mechanisms important for primordial follicle activation from the dormant state in mammals.
Phosphoinositide 3-kinase (PI3K) signaling, a classic signaling pathway, is fundamental for regulating cell proliferation, survival, migration, as well as metabolism and consists of various signaling molecules including kinases, phosphatases, and transcription factors that establish cascades of intracellular signaling (Blume-Jensen and Hunter, 2001; Cantley, 2002; Zheng et al., 2012). In fact, an earlier study has reported that transient treatment of PI3K stimulators has been found to activate primordial follicles in neonatal mouse ovaries and in human ovarian cortical tissues (Li et al., 2010; Sun et al., 2015; Maidarti et al., 2020). In another experiment using PI3K stimulators as infertility treatment, one healthy baby was delivered out of 13 attempts (Kawamura et al., 2013). These results suggest that PI3K is a significant factor in the activation of primordial follicles.
In cells, the main function of PI3K is to phosphorylate phosphatidylinositol-4,5-bisphosphate (PIP2) to produce phosphatidylinositol-3,4,5-triphosphate (PIP3) at the intracellular membrane, whereas PTEN prevents the conversion of PIP2 to PIP3 (Cantley, 2002; Zhang et al., 2019). PIP3, if accumulated in the oocyte, could stimulate the phosphorylation of serine/threonine protein kinase (Akt) (Kim and Kurita, 2018) and increase the nuclear exclusion of forkhead box O3 (FOXO3), thereby triggering primordial follicle activation in humans and mice (John et al., 2008; Kawamura et al., 2013; Takeuchi et al., 2019). When unphosphorylated, FOXO3 will relocate to the nucleus and function as a transcription factor, leading to apoptosis and cell cycle arrest (Liu et al., 2006).
FOXO3, a downstream effector of PI3K signaling, will shuttle from the nucleus to the cytoplasm of mouse oocytes when PI3K signaling is activated in the oocytes (Ezzati et al., 2015; Lee and Chang, 2019). It is highly expressed in mouse primordial follicles, and its expression level would be declined in primary and later-growing follicles, indicating that FOXO3 relates to the activation of primordial follicles (Liu et al., 2007). To prove this, FOXO3 was deleted from the oocytes of mouse primordial follicles, causing the global activation of dormant primordial follicles and the mutant female mice becoming infertile in young adulthood because of follicle depletion (Castrillon et al., 2003; Hosaka et al., 2004; Gallardo et al., 2008). In contrast, when the oocyte-specific FOXO3 was overexpressed in mice, the mice showed infertility caused by the retardation of oocyte growth and follicular development (Liu et al., 2007), indicating that FOXO3 suppressed the activation of ovarian follicles (Cui et al., 2019). Furthermore, constitutively active FOXO3 in oocytes could preserve the ovarian reserve in mice (Pelosi et al., 2013).
PTEN, a lipid phosphatase, converts PIP3 back to PIP2 and thus acts as a negative regulator of PI3K signaling (Cantley, 2002). To explore the relationship between PTEN and follicular activation, PTEN was deleted from the oocytes of primordial follicles in mice, causing the entire primordial follicle pool to be activated prematurely and causing follicular depletion in young adulthood (Reddy et al., 2008). Meanwhile, the level of phospho-Akt was elevated in mutant mice (Reddy et al., 2008). Other studies using a PTEN inhibitor have also shown the activation of dormant primordial follicles due to enhancing PI3K signaling in mammals (Adhikari et al., 2012; Maidarti et al., 2019); thus, a healthy baby has been born from a primordial follicle of a woman with POF (Kawamura et al., 2013). These results suggest that PTEN is essential for maintaining the dormancy of the primordial follicle pool and most likely works through influencing PI3K signaling in mammals (Hu et al., 2018; Takeuchi et al., 2019).
Cell division cycle 42 (CDC42) is a member of the Rho GTPase family and is crucial in controlling multicellular functions including actin cytoskeletal dynamics, membrane trafficking, transcription, and cell cycle control (Duquette and Lamarche-Vane, 2014; Pérez et al., 2020). Previous studies have revealed that CDC42 is essential for meiotic maturation and might activate the PI3K pathway in mouse oocytes by regulating PTEN and PIP3 (Li et al., 2003; Na and Zernicka-Goetz, 2006). Recently, Yan et al. (2018) suggested that oocyte CDC42 controls follicle activation by activating PI3K signaling directly and downregulating PTEN expression in the oocytes of mouse dormant primordial follicles. They reported that CDC42 expression increases in oocytes during the activation of primordial follicles and that silencing of CDC42 expression significantly suppressed primordial follicle activation. Most importantly, it revealed that PI3K signaling is activated through the binding of the CDC42 active form to the phosphatidylinositol-4,5-bisphosphate 3-kinase catalytic subunit beta, also called p110β, which is in accordance with previous idea (Fritsch et al., 2013).
In a word, these results suggest that the PI3K–Akt–FOXO3 signaling pathway plays an important role in maintaining the quiescence of primordial follicles in mammals (Figure 1). Loss of function of any of the inhibitory molecules for follicular activation, including PTEN and FOXO3, leads to a premature and irreversible activation of the primordial follicle pool (Adhikari and Liu, 2009).
Figure 1. Illustration of the PI3K signaling pathway in an oocyte. The main function of PI3K is to phosphorylate PIP2 to produce PIP3 at the intracellular membrane, whereas PTEN prevents the conversion of PIP2 to PIP3. PIP3, if accumulated in the oocyte, could stimulate the phosphorylation of Akt and increase the nuclear exclusion of FOXO3, thereby triggering oocyte awakening. When unphosphorylated, FOXO3 will relocate to the nucleus and function as a transcription factor, leading to oocyte arrest. In addition, CDC42 can activate the PI3K signaling pathway by activating PI3K directly and downregulating PTEN expression. PI3K, phosphoinositide 3-kinase; PIP2, phosphorylate phosphatidylinositol-4,5-bisphosphate; PIP3, phosphatidylinositol-3,4,5-triphosphate; PTEN, phosphatase and tensin homolog deleted on chromosome 10; Akt, serine/threonine protein kinase; FOXO3, forkhead box O3; CDC42, cell division cycle 42.
The mammalian target of rapamycin (mTOR) is a highly conserved serine/threonine kinase that regulates cell growth, metabolism, survival, and migration, which modulate processes such as protein synthesis, ribosome biogenesis, and autophagy (Laplante and Sabatini, 2012; Yurube et al., 2020). PI3K/Akt not only localizes FOXO3 in the cytoplasm but also could activate mTOR signal. The PI3K/Akt/mTOR pathway is an intracellular signaling pathway important in regulating the cell cycle (Tewari et al., 2019). mTOR exists in two forms: mTORC1, which is sensitive to rapamycin, and mTORC2, which is not. The mTOR1 inhibitor rapamycin could suppress primordial follicle development and maintain follicle pool size in mice (Yorino and Kawamura, 2020). mTORC1 in oocytes plays an indispensable part in the activation of primordial follicles in the mouse ovary (Tong et al., 2013).
It was also found that the driving force behind mTORC1 signaling is the activated S6 kinase beta-1 (S6K1)–ribosomal protein S6 (rpS6) signaling that promotes protein translation and ribosome biogenesis in mouse oocytes (Reddy et al., 2010; Lee et al., 2012). S6K1 is the main substrate of mTORC1 and is phosphorylated by mTORC1 (Ahmed et al., 2019). S6K1 can also be activated by phosphoinositide-dependent kinase-1 (PDK1) (Gao et al., 2019), which may explain why the inhibition of mTORC1 signaling in mouse oocytes by deleting the regulatory-associated protein of mTOR (RPTOR) has no impact on follicular development and female fertility (Gorre et al., 2014). After being phosphorylated, S6K1 activates rpS6, which is essential for protein translation. Whether it is an incompetence of rpS6 in oocytes by deleting PDK1 or deleting the rpS6 gene, this would result in an accelerated loss of primordial follicles due to atresia, indicating that the mTORC1–S6K1–rpS6 cascade is indispensable for maintaining the survival of mouse primordial follicles (Reddy et al., 2009; Adhikari et al., 2010).
In cells, the activity of mTORC1 is negatively regulated by a heterodimeric complex consisting of tuberous sclerosis complex 1 (TSC1) and tuberous sclerosis complex 2 (TSC2) (Hansmann et al., 2020). The TSC1–TSC2 complex inhibits mTORC1 through a GTPase-activating protein domain located in TSC2, and TSC2 must be stabilized by TSC1 to protect it from ubiquitination and degradation (Chong-Kopera et al., 2006). In mutant mice lacking the TSC1 in oocytes, the entire pool of primordial follicles is activated prematurely, and the disruption of TSC2 in oocytes has shown an identical outcome (Adhikari et al., 2009, 2010). Furthermore, liver kinase B1 (LKB1), a multifunctional serine/threonine kinase secreted from mouse oocytes, can inhibit mTORC1 by phosphorylating TSC2 (Jiang et al., 2016).
p27kip1 is a member of the Cip/Kip family of cyclin-dependent kinase (CDK) inhibitors. It is a general CDK inhibitor whose specific late G1 destruction allows the progression of the cell across the G1/S boundary (Gonçalves, 2018). Rajareddy et al. (2007) showed that p27kip1 was expressed in the nuclei of mouse dormant oocytes and that primordial follicles in p27kip1-deficient mice were overactivated, resulting in POF. Hirashima et al. (2011) demonstrated that p27kip1 negatively regulated mouse primordial oocyte growth and that the knockdown of p27kip1 led primordial oocytes to enter the growth phase in vitro. These results indicated that p27kip1 plays a key role in preventing primordial follicle activation prematurely (Adhikari et al., 2009). But it is unknown for the regulation of p27kip1 –CDK signaling in oocytes. Jang et al. (2017) identified three putative FOXO3 binding elements in the mouse p27kip1 gene and found that FOXO3 could bind strongly to the putative binding site P2. Furthermore, cisplatin suppressed the binding affinity of FOXO3 for the putative elements in the p27kip1 promoter, and co-treatment with melatonin and ghrelin rescued FOXO3 binding to the P2 site of the p27kip1 promoter (Jang et al., 2017). Therefore, the p27kip1–CDK signaling in oocytes may be regulated by the PI3K–Akt–FOXO3 signaling pathway.
The growth of oocyte follows the proliferation and differentiation of pfGCs, which implies that pfGCs might be predominant in regulating the activation of primordial follicles (Zhang and Liu, 2015). However, a different view holds that oocytes govern follicular activation (Eppig et al., 2002; Adhikari and Liu, 2009; Yan et al., 2019). It is still a mystery whether pfGCs develop first to activate oocytes or not. But, until recently, modern research using genetically modified mouse models discovered a relatively complete signal transduction pathway in pfGCs to drive the development of oocytes (Zhang et al., 2014).
Just as mTORC1 does in oocytes, mTORC1 in pfGCs also plays a key part in the activation of primordial follicles. RPTOR is the key component of the mTORC1 pathway in mouse pfGCs (Zhang et al., 2014). Zhang et al. (2014) discovered that the deletion of RPTOR in pfGCs caused the flattened pfGCs fail to differentiate into cuboidal granulosa cells, and compared to the control group, most of the oocytes in the mutant ovaries remained in a quiescent state in primordial follicles and eventually die out in adulthood. This result suggests that the deletion of RPTOR in pfGCs could suppress follicular activation and prevent the awakening of dormant oocytes (Zhang et al., 2014). In mouse ovaries with deletion of TSC1, all flattened pfGCs had differentiated into cuboidal granulosa cells and caused the premature awakening of all dormant oocytes and the activation of all primordial follicles (Adhikari et al., 2010). The same phenomenon also occurred in the ovaries that use the mTORC1 inhibitor rapamycin (Zhang et al., 2014). These results showed that elevated mTORC1 signaling in pfGCs could activate primordial follicles and that pfGCs are not only supporting the survival of dormant oocytes but are also determining their fates (Zhang et al., 2014).
The KIT ligand, KITL, also called stem cell factor (SCF), is a receptor protein tyrosine kinase (Martinez-Anton et al., 2019). KITL plays important roles in primordial follicle activation, oocyte growth and survival, and granulosa cell proliferation in mammals (Tan et al., 2017; Adib et al., 2019; Islam et al., 2020). In mammalian follicles, KITL is produced by pfGCs (Vanderhyden, 2002; Jin et al., 2005), whereas KIT is expressed on the surface of oocytes (Driancourt et al., 2000). A previous study has demonstrated that the communication between mouse oocytes and the surrounding granulosa cells mainly depends on KIT and KITL (Driancourt et al., 2000). Zhang et al. (2014) found that active mTORC1 signaling enhances the expression of KITL in mouse pfGCs and that KITL–KIT signaling serves as the bridge between the pfGCs and the dormant oocytes for activating primordial follicles. Besides, PI3K signaling is one of the important downstream pathways activated by KITL–KIT in mice (Driancourt et al., 2000). KITL secreted from pfGCs binds to the KIT receptor on the oocyte surface, resulting in the phosphorylation of KIT Y719 and the subsequent activation of mouse oocyte PI3K signaling (Zhang et al., 2014). However, the mTORC1–KITL/KIT cascade is just a tip of the iceberg in the whole complex signaling pathways that associate pfGCs with the oocyte. More research is needed to figure out whether there are other factors involved between mTORC1 signaling and KITL/KIT.
The Hippo signaling pathway is conserved in metazoan animals and is essential for organ size control (Halder and Johnson, 2011; Kwon et al., 2013; Gao et al., 2020). Hippo signaling consists of several negative growth regulators acting in a serine/threonine kinase cascade that eventually phosphorylates and inactivates key Hippo signaling effectors, Yes-associated protein (YAP)/transcriptional coactivator with PDZ binding motif (TAZ). When Hippo signaling is disrupted, decreases in the phosphorylated YAP (pYAP) increase the nuclear YAP levels, leading to the increased expression of downstream immediate early gene (CCN) growth factors and baculoviral inhibitors of apoptosis repeat containing (BIRC) apoptosis inhibitors (Kawashima and Kawamura, 2018). These CCN proteins, in turn, stimulate cell growth, survival, and proliferation (Kwon et al., 2013).
At present, there are a lot of ovarian diseases causing infertility, such as primary ovarian insufficiency (POI) and polycystic ovarian syndrome (PCOS). In the clinical setting, therapies for those patients who want to be pregnant include ovarian wedge resection (Andrews, 1952) and “drilling” by diathermy or laser due to their function of promoting follicle growth (Farquhar et al., 2012). Furthermore, extensive primordial follicle activation was observed after transplantation in marmoset, bovine, and human tissues (Gavish et al., 2018). Recently, a research found that the underlying mechanisms of these disruptive procedures, which have confused scientists for decades, were linked to the increased actin polymerization and, thus, the disruption of ovarian Hippo signaling (Kawamura et al., 2013). Fragmentation of the mammalian ovarian cortex into small cubes changed the cytoskeletal actin dynamics and induced the disruption of the Hippo signaling pathway, leading to the production of CCN growth factors and anti-apoptotic BIRC (Kawashima and Kawamura, 2018).
The expression of the core components of the Hippo pathway changes during mouse follicular development, especially before and after primordial follicle activation in vitro, which may be related to the significant decrease of the ratio of pYAP1/YAP1 (Sun et al., 2015). Kawamura et al. (2016) fragmented ovaries from juvenile (day 10) mice and grafted under kidney capsules for 5 days. The results showed that the percentages of the late secondary and antral/preovulatory follicles also increased, accompanied by decreases in primordial follicles. These results are in accordance with clinical therapeutic outcomes wherein human ovarian fragmentation could induce follicle growth, including the activation of primordial follicles (Kawamura et al., 2013). They discovered transient increases in the ratios of F-actin to G-actin after ovarian fragmentation and decreases in the pYAP levels as well as in the pYAP-to-total YAP ratios, suggesting Hippo signaling disruption (Kawamura et al., 2013). Masciangelo et al. (2020) confirmed that the Hippo pathway was involved in human follicle activation and suggested a link between the PI3K and Hippo pathways, with both contributing to the phenomenon of follicle burnout. Recently, Kawamura et al. (2020) used the drug-free in vitro activation approach to treat patients with poor ovarian response and retrieved more oocytes. Although it has been known that Hippo signaling exerts some effect on the initiation of primordial follicles, further research is needed to elucidate its specific mechanism.
There are multiple factors involved in the control of primordial follicle activation, and their functions are varied. Some factors promote primordial follicle activation while others inhibit it. There is a balance between the activation and maintenance of the primordial follicle pool. In short, these factors together maintain a normal and continuous follicular development process.
Spermatogenesis- and oogenesis-specific basic helix-loop-helix-containing protein 1 (Sohlh1) is a germ cell-specific gene that encodes the basic helix-loop-helix transcriptional regulator that is essential in oogenesis and spermatogenesis (Suzuki et al., 2013). Sohlh1 is specifically expressed in germ cell cysts and oocytes of primordial follicles in female mice, and Sohlh1-null mice are sterile due to a defect of follicle development during the primordial-to-primary follicle transition (Pangas et al., 2006). In Sohlh1–/– mice, only primordial follicles and empty follicles can be observed in ovaries from 0.5 to 23.5 days post-parturition, and primordial follicle activation was not observed at 7.5 days post-parturition (Liu et al., 2019). The possible mechanism is that the absence of Sohlh1 may affect the activation of primordial follicles via the downregulation of KIT and the inhibition of the KIT/PI3K/Akt pathway (Liu et al., 2019). Compared with its expression in mice, Sohlh1 exhibits a much more extensive expression pattern in human tissues and is mainly located in the oocytes of primordial and primary follicles, granular cells, and theca cells of secondary follicles (Zhang et al., 2015).
Newborn ovary homeobox gene (NOBOX) is an oocyte-specific homeobox gene that plays a key role in controlling the process of primordial follicle activation (Bayne et al., 2015). NOBOX is expressed in primordial and growing oocytes in the ovaries of mice (Suzumori et al., 2002) and humans (Huntriss et al., 2006). The lack of NOBOX could accelerate postnatal oocyte loss and abolish the transition from primordial to growing follicles in mice (Rajkovic et al., 2004; Belli et al., 2013). The expression of FOXO3 mRNA is significantly downregulated in the NOBOX-null mouse ovary (Choi et al., 2007), which indicates that NOBOX could regulate the activation of primordial follicles through the modulation of FOXO3. Furthermore, several NOBOX mutations have been reported to be associated with POI (Ferrari et al., 2016; Li et al., 2017).
LIM homeobox 8 (Lhx8) is a member of the LIM homeobox transcription factor family and is highly conserved (Zhou et al., 2015). Choi et al. (2008) showed that Lhx8 is expressed preferentially in the oocytes and germ cells within the mouse ovary and that Lhx8–/– ovaries fail to maintain the primordial follicles, and the transition from primordial to growing follicles does not occur. Ren et al. (2015) found that the conditional deficiency of Lhx8 in the oocytes of mouse primordial follicles leads to a massive primordial oocyte activation and partly functions by interacting with the PI3K signaling pathway indirectly. These results indicated that Lhx8 is essential for the maintenance of primordial follicles.
Forkhead box L2 (FOXL2) is a member of the forkhead box superfamily of transcriptional factors that possess a DNA binding domain (Jin et al., 2020). Zheng et al. (2014) showed that FOXL2 is expressed in the pfGCs in mouse primordial follicles and in the granulosa cells in growing follicles. In FOXL2-null mice, ovarian granulosa cells do not complete the squamous-to-cuboidal transition, leading to the absence of secondary follicles and to oocyte atresia (Schmidt et al., 2004). Furthermore, nearly all primordial follicles have already initiated folliculogenesis 2 weeks after birth (Schmidt et al., 2004). In humans, FOXL2 transcripts were less abundant in the granulosa cells from primary follicles compared to the granulosa cells from primordial follicles, providing preliminary evidence that FOXL2 downregulation between these stages may coincide with a role in primordial follicle activation (Ernst et al., 2018). FOXL2 is highly expressed in the ovaries and the eyelids, and autosomal-dominant germline mutations in FOXL2 cause blepharophimosis, ptosis, and epicanthus inversus syndrome (BPES) manifested by an eyelid malformation and POI (Crisponi et al., 2001). As can be seen above, FOXL2 is a key factor in regulating the activation of primordial follicles through initiating pfGCs.
Bone morphogenetic proteins (BMPs) constitute the largest subdivision of the transforming growth factor-β (TGF-β) family of ligands (Lowery and Rosen, 2018). There is accumulating evidence indicating that BMP4 and BMP7 are essential for the primordial-to-primary follicle transition (Lee et al., 2001; da Cunha et al., 2017). BMP4 and BMP7 are expressed in ovarian stromal cells and/or theca cells and have recently been implicated as positive regulators of the primordial-to-primary follicle transition (Knight and Glister, 2006).
BMP4-treated rat ovaries had a higher number of developing primary follicles and fewer arrested primordial follicles than the untreated controls (Nilsson and Skinner, 2003). BMP4 also promotes an increase in the diameters of the follicles and oocytes of primary and secondary follicles in an in vitro bovine ovary culture (da Cunha et al., 2017). Furthermore, the numbers of primary follicles were lower and those of primordial follicles were higher in anti-BMP-4-treated mouse ovaries compared to control ovaries (Tanwar et al., 2008). Gremlin1 and Gremlin2, as inhibitors of BMP4, have been demonstrated to reverse the effect of BMP4 to stimulate rat primordial follicle transition (Nilsson et al., 2014). When Gremlin1 was knocked out, the mice died within 48 h after birth, and researchers discovered reduced oocyte numbers and a delayed primordial follicle development (Myers et al., 2011). These results indicated that BMP4 promotes primordial follicle development. However, it is interesting to note that, in sheep, BMP4 did not influence primordial follicle activation or promote follicle growth (Bertoldo et al., 2014). Different species may account for the differences, but further studies will be needed to explore the truth.
As for BMP7, Lee et al. (2001) showed that rat ovaries treated with BMP7 in vivo had decreased numbers of primordial follicles and increased numbers of growth follicles, suggesting that BMP7 may facilitate the transition of follicles from the primordial stage to the pool of growth follicles.
The anti-Mullerian hormone (AMH) is a glycoprotein of the TGF-β superfamily and is produced by the granulosa cells of pre-antral and early antral ovarian follicles in several species (Umer et al., 2019). It is generally accepted that AMH inhibits the activation of mammalian primordial follicles (Shahrokhi et al., 2018). An earlier study using AMH-knockout mice has already shown that there were more growing follicles and fewer primordial follicles in AMH–/– mice compared with the control group (Durlinger et al., 1999). Subsequently, other scientists who cultured mouse ovaries in the absence or presence of AMH noted that cultured ovaries with AMH contained 40% fewer growing follicles compared with control ovaries (Durlinger et al., 2002), and AMH treatment decreased the primordial-to-primary follicle transition (Nilsson et al., 2007). Not just in mice, the role of AMH in the suppression of follicle activation was also verified in bovines (Yang et al., 2017) and humans (Carlsson et al., 2006). Administration of a recombinant human AMH reduced the follicle activation induced by cyclophosphamide in a mouse model, thereby protecting the primordial follicle reserve and improving long-term fertility and reproductive outcomes (Roness et al., 2019).
Growth differentiation factor-9 (GDF-9), a member of the TGF-β superfamily, is secreted by mammalian oocytes (Emori and Sugiura, 2014). Nilsson et al. (2002) reported that GDF-9 promoted the growth of early primary follicles, but not primordial follicles, in cultured rat ovaries. In GDF-9-deficient female mice, the primordial and primary one-layer follicles can be formed, but there is a blockage in follicular development beyond the primary one-layer follicle stage, which leads to complete infertility (Dong et al., 1996). These results indicated that GDF-9 mainly activated the growth of primary follicles and might induce the activation of primordial follicles indirectly through the exhaustion of primary follicles. Moreover, oocyte growth was not affected in GDF-9-deficient female mice, indicating that GDF-9 functions by activating the granulosa cells while limiting the growth of oocytes in primordial follicles (Dong et al., 1996).
Basic fibroblast growth factor (bFGF), also called FGF-2, is mainly situated in the oocytes of early stage rat follicles (Nilsson et al., 2001). In rat ovaries, bFGF was found to decrease the number of primordial follicles and concomitantly increase the amount of developing follicles (Nilsson et al., 2001). Interestingly, the ability of bFGF to induce primordial follicle development needs the presence of KITL (Nilsson and Skinner, 2004). Except for rat ovaries, experiments using the ovaries of goats and humans also suggest that bFGF could activate primordial follicles (Garor et al., 2009; Wang et al., 2014).
A significant body of work has shown that epidermal growth factor (EGF) is an important regulator of primordial follicle activation in mammals (McNatty et al., 1999; Silva et al., 2004; Adhikari and Liu, 2009; Lu et al., 2015). EGF initiates rat primordial follicle growth by activating proto-oncogene c-erB2 (Li-Ping et al., 2010) and c-src (Du et al., 2012). c-erB2, encoding a transmembrane EGF receptor (Coussens et al., 1985; Rajadurai et al., 2018), induces rat primordial follicle activation via the downstream PKC and MAPK pathway (Li-Ping et al., 2010). EGF, as the upstream activator of the Src protein, regulates rat primordial follicle growth through the Src→PI3K→PKC→MAPK pathway (Du et al., 2012). But Maruo et al. (1993) demonstrated that the EGF and EGF receptor were observed only in oocytes in the pre-antral follicle stage in humans, but not in primordial follicles. Therefore, more comprehensive studies are needed.
Leukemia inhibitory factor (LIF) is a pleiotropic cytokine from the interleukin (IL)-6 family regulating various cellular functions (Mathieu et al., 2012). There are several reports on the function of LIF in follicular growth. For example, LIF has been reported to accelerate the primordial-to-primary follicle transition in rat ovaries (Nilsson et al., 2002) and goat ovarian tissues (da Nóbrega et al., 2012) in vitro. Yet, Komatsu et al. (2015) discovered that LIF inhibited the growth of mouse primary, secondary, and antral follicles and proved this using the neutralizing antibody in vitro. This result is the opposite of previous views and suggests that LIF is likely to coordinate follicular growth in the ovary (Komatsu et al., 2015).
The activation of primordial follicles is a complex but orchestrated process, which is regulated by multiple factors and pathways (Figure 2). The PI3K–Akt–FOXO3 signaling pathway plays a key role in the maintenance of primordial follicles, while the mTOR signal regulates the activation of primordial follicles. Factors such as FOXL2, Sohlh1, NOBOX, GDF-9, BMP4, BMP7, bFGF, and EGF initiate the activation of primordial follicles, whereas Lhx8 and AMH are suppressive factors in this process. In addition, there have been successes in triggering the activation of primordial follicles in vitro to obtain fertilizable oocytes with PI3K stimulators or CDC42 activators in mice.
Figure 2. The factors and pathways regulating the activation of primordial follicles. The PI3K–Akt signal is the main transduction pathway in oocytes and plays a key role in the activation of primordial follicles. On the one hand, the PI3K–Akt signal can upregulate mTOR to activate primordial follicles; on the other hand, it can inhibit FOXO3 to locate to the nucleus and prevent it from maintaining the quiescence of primordial follicles. The activation of primordial follicles can also be activated by the mTOR signal in pfGCs and the Hippo signal after ovary resection. Factors such as FOXL2, Sohlh1, NOBOX, GDF-9, BMP4, BMP7, bFGF, and EGF initiate the activation of primordial follicles, whereas Lhx8 and AMH are suppressive factors in this process. PI3K, phosphoinositide 3-kinase; Akt, serine/threonine protein kinase; mTOR, mammalian target of rapamycin; FOXO3, forkhead box O3; FOXL2, forkhead box L2; Sohlh1, spermatogenesis- and oogenesis-specific basic helix-loop-helix-containing protein 1; NOBOX, newborn ovary homeobox gene; GDF-9, growth differentiation factor-9: BMP, bone morphogenetic protein; bFGF, basic fibroblast growth factor; EGF, epidermal growth factor; Lhx8, LIM homeobox 8; AMH, anti-Mullerian hormone.
Although many drugs have not been used in humans due to ethical issues, our growing knowledge of the molecules that control the activation of primordial follicles will provide new but promising methods for obtaining mature eggs, helping women who are unable to have a child because of various ovarian diseases.
YC and WY wrote the manuscript and performed all of the necessary literature searches and data compilation. XS and CZ performed the necessary literature searches and data compilation. GS revised the manuscript and gave valuable suggestions. DH designed the review, reviewed it, and approved the submitted manuscript. All the authors contributed to the article and approved the submitted version.
This work was supported by the National Natural Science Foundation of China (no. 81771575) and the open project of the NHC Key Laboratory of Male Reproduction and Genetics (no. KF201906).
The authors declare that the research was conducted in the absence of any commercial or financial relationships that could be construed as a potential conflict of interest.
Adhikari, D., Flohr, G., Gorre, N., Shen, Y., Yang, H., Lundin, E., et al. (2009). Disruption of Tsc2 in oocytes leads to overactivation of the entire pool of primordial follicles. Mol. Hum. Reprod. 15, 765–770. doi: 10.1093/molehr/gap092
Adhikari, D., Gorre, N., Risal, S., Zhao, Z., Zhang, H., Shen, Y., et al. (2012). The safe use of a PTEN inhibitor for the activation of dormant mouse primordial follicles and generation of fertilizable eggs. PLoS One 7:e39034. doi: 10.1371/journal.pone.0039034
Adhikari, D., and Liu, K. (2009). Molecular mechanisms underlying the activation of mammalian primordial follicles. Endocr. Rev. 30, 438–464. doi: 10.1210/er.2008-0048
Adhikari, D., Zheng, W., Shen, Y., Gorre, N., Hämäläinen, T., Cooney, A. J., et al. (2010). Tsc/mTORC1 signaling in oocytes governs the quiescence and activation of primordial follicles. Hum. Mol. Genet. 19, 397–410. doi: 10.1093/hmg/ddp483
Adib, S., Valojerdi, M. R., and Alikhani, M. (2019). Dose optimisation of PTEN inhibitor, bpV (HOpic), and SCF for the in-vitro activation of sheep primordial follicles. Growth Fact. 37, 178–189. doi: 10.1080/08977194.2019.1680661
Ahmed, A. R., Owens, R. J., Stubbs, C. D., Parker, A. W., Hitchman, R., Yadav, R. B., et al. (2019). Direct imaging of the recruitment and phosphorylation of S6K1 in the mTORC1 pathway in living cells. Sci. Rep. 9:3408.
Andrews, M. C. (1952). Bilateral polycystic ovaries associated with sterility, amenorrhea and hirsutism. Va. Med. Mon. 79, 544–548.
Bayne, R. A., Kinnell, H. L., Coutts, S. M., He, J., Childs, A. J., and Anderson, R. A. (2015). GDF9 is transiently expressed in oocytes before follicle formation in the human fetal ovary and is regulated by a novel NOBOX transcript. PLoS One 10:e0119819. doi: 10.1371/journal.pone.0119819
Belli, M., Cimadomo, D., Merico, V., Redi, C. A., Garagna, S., and Zuccotti, M. (2013). The NOBOX protein becomes undetectable in developmentally competent antral and ovulated oocytes. Int. J. Dev. Biol. 57, 35–39. doi: 10.1387/ijdb.120125mz
Bertoldo, M. J., Duffard, N., Bernard, J., Frapsauce, C., Calais, L., Rico, C., et al. (2014). Effects of bone morphogenetic protein 4 (BMP4) supplementation during culture of the sheep ovarian cortex. Anim. Reprod. Sci. 149, 124–134. doi: 10.1016/j.anireprosci.2014.07.010
Blume-Jensen, P., and Hunter, T. (2001). Oncogenic kinase signalling. Nature 411, 355–365. doi: 10.1038/35077225
Cantley, L. C. (2002). The phosphoinositide 3-kinase pathway. Science 296, 1655–1657. doi: 10.1126/science.296.5573.1655
Carlsson, I. B., Scott, J. E., Visser, J. A., Ritvos, O., Themmen, A. P., and Hovatta, O. (2006). Anti-Müllerian hormone inhibits initiation of growth of human primordial ovarian follicles in vitro. Hum. Reprod. 21, 2223–2227. doi: 10.1093/humrep/del165
Castrillon, D. H., Miao, L., Kollipara, R., Horner, J. W., and DePinho, R. A. (2003). Suppression of ovarian follicle activation in mice by the transcription factor Foxo3a. Science 301, 215–218. doi: 10.1126/science.1086336
Choi, Y., Ballow, D. J., Xin, Y., and Rajkovic, A. (2008). Lim homeobox gene, lhx8, is essential for mouse oocyte differentiation and survival. Biol. Reprod. 79, 442–449. doi: 10.1095/biolreprod.108.069393
Choi, Y., Qin, Y., Berger, M. F., Ballow, D. J., Bulyk, M. L., and Rajkovic, A. (2007). Microarray analyses of newborn mouse ovaries lacking Nobox. Biol. Reprod. 77, 312–319. doi: 10.1095/biolreprod.107.060459
Chong-Kopera, H., Inoki, K., Li, Y., Zhu, T., Garcia-Gonzalo, F. R., Rosa, J. L., et al. (2006). TSC1 stabilizes TSC2 by inhibiting the interaction between TSC2 and the HERC1 ubiquitin ligase. J. Biol. Chem. 281, 8313–8316. doi: 10.1074/jbc.c500451200
Coussens, L., Yang-Feng, T. L., Liao, Y. C., Chen, E., Gray, A., McGrath, J., et al. (1985). Tyrosine kinase receptor with extensive homology to EGF receptor shares chromosomal location with neu oncogene. Science 230, 1132–1139. doi: 10.1126/science.2999974
Crisponi, L., Deiana, M., Loi, A., Chiappe, F., Uda, M., Amati, P., et al. (2001). The putative forkhead transcription factor FOXL2 is mutated in blepharophimosis/ptosis/epicanthus inversus syndrome. Nat. Genet. 27, 159–166. doi: 10.1038/84781
Cui, C., Han, S., Yin, H., Luo, B., Shen, X., Yang, F., et al. (2019). FOXO3 is expressed in ovarian tissues and acts as an apoptosis initiator in granulosa cells of chickens. Biomed Res. Int. 2019:6902906. doi: 10.1155/2019/6902906
da Cunha, E. V., de Souza, G. B., Passos, J. R. S., Silva, A. W. B., Dau, A. M., Saraiva, M. V. A., et al. (2017). Effects of bone morphogenetic protein 4 (BMP4) on in vitro development and survival of bovine preantral follicles enclosed in fragments ovarian tissue. Zygote 25, 256–264. doi: 10.1017/s0967199417000089
da Nóbrega, J. E. Jr., Gonçalves, P. B., Chaves, R. N., Magalhães Dde, M., Rossetto, R., Lima-Verde, I. B., et al. (2012). Leukemia inhibitory factor stimulates the transition of primordial to primary follicle and supports the goat primordial follicle viability in vitro. Zygote 20, 73–78. doi: 10.1017/s0967199411000074
Dong, J., Albertini, D. F., Nishimori, K., Kumar, T. R., Lu, N., and Matzuk, M. M. (1996). Growth differentiation factor-9 is required during early ovarian folliculogenesis. Nature 383, 531–535. doi: 10.1038/383531a0
Driancourt, M. A., Reynaud, K., Cortvrindt, R., and Smitz, J. (2000). Roles of KIT and KIT LIGAND in ovarian function. Rev. Reprod. 5, 143–152. doi: 10.1530/revreprod/5.3.143
Du, X. Y., Huang, J., Xu, L. Q., Tang, D. F., Wu, L., Zhang, L. X., et al. (2012). The proto-oncogene c-src is involved in primordial follicle activation through the PI3K, PKC and MAPK signaling pathways. Reprod. Biol. Endocrinol. 10:58. doi: 10.1186/1477-7827-10-58
Duquette, P. M., and Lamarche-Vane, N. (2014). Rho GTPases in embryonic development. Small GTPases 5:8.
Durlinger, A. L., Gruijters, M. J., Kramer, P., Karels, B., Ingraham, H. A., and Nachtigal, M. W. (2002). Anti-Müllerian hormone inhibits initiation of primordial follicle growth in the mouse ovary. Endocrinology 143, 1076–1084. doi: 10.1210/endo.143.3.8691
Durlinger, A. L., Kramer, P., Karels, B., de Jong, F. H., Uilenbroek, J. T., and Grootegoed, J. A. (1999). Control of primordial follicle recruitment by anti-Müllerian hormone in the mouse ovary. Endocrinology 140, 5789–5796. doi: 10.1210/endo.140.12.7204
Emori, C., and Sugiura, K. (2014). Role of oocyte-derived paracrine factors in follicular development. Anim. Sci. J. 85, 627–633. doi: 10.1111/asj.12200
Eppig, J. J., Wigglesworth, K., and Pendola, F. L. (2002). The mammalian oocyte orchestrates the rate of ovarian follicular development. Proc. Natl. Acad. Sci. U.S.A. 99, 2890–2894. doi: 10.1073/pnas.052658699
Ernst, E. H., Franks, S., Hardy, K., Villesen, P., and Lykke-Hartmann, K. (2018). Granulosa cells from human primordial and primary follicles show differential global gene expression profiles. Hum. Reprod. 33, 666–679. doi: 10.1093/humrep/dey011
Ezzati, M. M., Baker, M. D., Saatcioglu, H. D., Aloisio, G. M., Pena, C. G., Nakada, Y., et al. (2015). Regulation of FOXO3 subcellular localization by Kit ligand in the neonatal mouse ovary. J. Assist. Reprod. Genet. 32, 1741–1747. doi: 10.1007/s10815-015-0589-9
Farquhar, C., Brown, J., and Marjoribanks, J. (2012). Laparoscopic drilling by diathermy or laser for ovulation induction in anovulatory polycystic ovary syndrome. Cochrane Database Syst. Rev. 6:CD001122.
Ferrari, I., Bouilly, J., Beau, I., Guizzardi, F., Ferlin, A., Pollazzon, M., et al. (2016). Impaired protein stability and nuclear localization of NOBOX variants associated with premature ovarian insufficiency. Hum. Mol. Genet. 25, 5223–5233.
Findlay, J. K., Hutt, K. J., Hickey, M., and Anderson, R. A. (2015). How is the number of primordial follicles in the ovarian reserve established? Biol. Reprod. 93:111.
Ford, E. A., Beckett, E. L., Roman, S. D., McLaughlin, E. A., and Sutherland, J. M. (2020). Advances in human primordial follicle activation and premature ovarian insufficiency. Reproduction 159, R15–R29.
Fritsch, R., de Krijger, I., Fritsch, K., George, R., Reason, B., Kumar, M. S., et al. (2013). RAS and RHO families of GTPases directly regulate distinct phosphoinositide 3-kinase isoforms. Cell 153, 1050–1063. doi: 10.1016/j.cell.2013.04.031
Gallardo, T. D., John, G. B., Bradshaw, K., Welt, C., Reijo-Pera, R., Vogt, P. H., et al. (2008). Sequence variation at the human FOXO3 locus: a study of premature ovarian failure and primary amenorrhea. Hum. Reprod. 23, 216–221. doi: 10.1093/humrep/dem255
Gao, P., Mu, M., Chen, Y., He, J., Tao, X., and Song, C. (2020). Yes-associated protein upregulates filopodia formation to promote alveolar epithelial-cell phagocytosis. Immunol. Lett. 225, 44–49. doi: 10.1016/j.imlet.2020.06.009
Gao, W., Wang, K., Zhang, L., Li, J., Liu, J., Chen, X., et al. (2019). Pharmacological inhibition of S6K1 facilitates platelet activation by enhancing Akt phosphorylation. Platelets 30, 241–250. doi: 10.1080/09537104.2017.1416075
Garor, R., Abir, R., Erman, A., Felz, C., Nitke, S., and Fisch, B. (2009). Effects of basic fibroblast growth factor on in vitro development of human ovarian primordial follicles. Fertil. Steril. 91, 1967–1975. doi: 10.1016/j.fertnstert.2008.04.075
Gavish, Z., Spector, I., Peer, G., Schlatt, S., Wistuba, J., Roness, H., et al. (2018). Follicle activation is a significant and immediate cause of follicle loss after ovarian tissue transplantation. J. Assist. Reprod. Genet. 35, 61–69. doi: 10.1007/s10815-017-1079-z
Gonçalves, G. A. (2018). p27kip1 as a key regulator of endometriosis. Eur. J. Obstet. Gynecol. Reprod. Biol. 221, 1–4. doi: 10.1016/j.ejogrb.2017.11.026
Gorre, N., Adhikari, D., Lindkvist, R., Brännström, M., Liu, K., and Shen, Y. (2014). mTORC1 Signaling in oocytes is dispensable for the survival of primordial follicles and for female fertility. PLoS One 9:e110491. doi: 10.1371/journal.pone.0110491
Halder, G., and Johnson, R. L. (2011). Hippo signaling: growth control and beyond. Development 138, 9–22. doi: 10.1242/dev.045500
Hansen, K. R., Knowlton, N. S., Thyer, A. C., Charleston, J. S., Soules, M. R., and Klein, N. A. (2008). A new model of reproductive aging: the decline in ovarian non-growing follicle number from birth to menopause. Hum. Reprod. 23, 699–708. doi: 10.1093/humrep/dem408
Hansmann, P., Brückner, A., Kiontke, S., Berkenfeld, B., Seebohm, G., Brouillard, P., et al. (2020). Structure of the TSC2 GAP domain: mechanistic insight into catalysis and pathogenic mutations. Structure 28, 933–942.e4. doi: 10.1016/j.str.2020.05.008
Hirashima, Y., Moniruzzaman, M., and Miyano, T. (2011). p27(Kip1) negatively regulates the activation of murine primordial oocytes. J. Reprod. Dev. 57, 217–222. doi: 10.1262/jrd.10-119H
Hosaka, T., Biggs, W. H., Tieu, D., Boyer, A. D., Varki, N. M., and Cavenee, W. K. (2004). Disruption of forkhead transcription factor (FOXO) family members in mice reveals their functional diversification. Proc. Natl. Acad. Sci. U.S.A. 101, 2975–2980. doi: 10.1073/pnas.0400093101
Hsueh, A. J., Kawamura, K., Cheng, Y., and Fauser, B. C. (2015). Intraovarian control of early folliculogenesis. Endocr. Rev. 36, 1–24. doi: 10.1210/er.2014-1020
Hu, Y., Yuan, D. Z., Wu, Y., Yu, L. L., Xu, L. Z., Yue, L. M., et al. (2018). Bisphenol A initiates excessive premature activation of primordial follicles in mouse ovaries via the PTEN signaling pathway. Reprod. Sci. 25, 609–620. doi: 10.1177/1933719117734700
Huntriss, J., Hinkins, M., and Picton, H. M. (2006). cDNA cloning and expression of the human NOBOX gene in oocytes and ovarian follicles. Mol. Hum. Reprod. 12, 283–289. doi: 10.1093/molehr/gal035
Islam, M. N., Alam, M. H., Khatun, A., Akter, I., Modak, A. K., Hashem, M. A., et al. (2020). Effects of stem cell factor on in vitro growth of buffalo oocytes. Theriogenology 142, 114–119. doi: 10.1016/j.theriogenology.2019.09.044
Jang, H., Na, Y., Hong, K., Lee, S., Moon, S., Cho, M., et al. (2017). Synergistic effect of melatonin and ghrelin in preventing cisplatin-induced ovarian damage via regulation of FOXO3a phosphorylation and binding to the p27(Kip1) promoter in primordial follicles. J. Pineal Res. 63:e12432. doi: 10.1111/jpi.12432
Jiang, Z. Z., Hu, M. W., Ma, X. S., Schatten, H., Fan, H. Y., Wang, Z. B., et al. (2016). LKB1 acts as a critical gatekeeper of ovarian primordial follicle pool. Oncotarget 7, 5738–5753. doi: 10.18632/oncotarget.6792
Jin, H., Lee, B., Luo, Y., Choi, Y., Choi, E. H., Jin, H., et al. (2020). FOXL2 directs DNA double-strand break repair pathways by differentially interacting with Ku. Nat. Commun. 11:2010. doi: 10.1038/s41467-020-15748-1
Jin, X., Han, C. S., Yu, F. Q., Wei, P., Hu, Z. Y., and Liu, Y. X. (2005). Anti-apoptotic action of stem cell factor on oocytes in primordial follicles and its signal transduction. Mol. Reprod. Dev. 70, 82–90. doi: 10.1002/mrd.20142
John, G. B., Gallardo, T. D., Shirley, L. J., and Castrillon, D. H. (2008). Foxo3 is a PI3K-dependent molecular switch controlling the initiation of oocyte growth. Dev. Biol. 321, 197–204. doi: 10.1016/j.ydbio.2008.06.017
Kallen, A., Polotsky, A. J., and Johnson, J. (2018). Untapped reserves: controlling primordial follicle growth activation. Trends Mol. Med. 24, 319–331. doi: 10.1016/j.molmed.2018.01.008
Kawamura, K., Cheng, Y., Suzuki, N., Deguchi, M., Sato, Y., Takae, S., et al. (2013). Hippo signaling disruption and Akt stimulation of ovarian follicles for infertility treatment. Proc. Natl. Acad. Sci. U.S.A. 110, 17474–17479. doi: 10.1073/pnas.1312830110
Kawamura, K., Ishizuka, B., and Hsueh, A. J. W. (2020). Drug-free in-vitro activation of follicles for infertility treatment in poor ovarian response patients with decreased ovarian reserve. Reprod. Biomed. 40, 245–253. doi: 10.1016/j.rbmo.2019.09.007
Kawamura, K., Kawamura, N., and Hsueh, A. J. (2016). Activation of dormant follicles: a new treatment for premature ovarian failure. Curr. Opin. Obstet. Gynecol. 28, 217–222. doi: 10.1097/GCO.0000000000000268
Kawashima, I., and Kawamura, K. (2018). Regulation of follicle growth through hormonal factors and mechanical cues mediated by Hippo signaling pathway. Syst. Biol. Reprod. Med. 64, 3–11. doi: 10.1080/19396368.2017.1411990
Kim, S. Y., and Kurita, T. (2018). New insights into the role of phosphoinositide 3-kinase activity in the physiology of immature oocytes: lessons from recent mouse model studies. Eur. Med. J. Reprod. Health 3, 119–125.
Knight, P. G., and Glister, C. (2006). TGF-beta superfamily members and ovarian follicle development. Reproduction 132, 191–206. doi: 10.1530/rep.1.01074
Komatsu, K., Koya, T., Wang, J., Yamashita, M., Kikkawa, F., and Iwase, A. (2015). Analysis of the effect of leukemia inhibitory factor on follicular growth in cultured murine ovarian tissue. Biol. Reprod. 93:18. doi: 10.1095/biolreprod.115.128421
Kwon, Y., Vinayagam, A., Sun, X., Dephoure, N., Gygi, S. P., Hong, P., et al. (2013). The Hippo signaling pathway interactome. Science 342, 737–740. doi: 10.1126/science.1243971
Laplante, M., and Sabatini, D. M. (2012). mTOR signaling in growth control and disease. Cell 149, 274–293. doi: 10.1016/j.cell.2012.03.017
Lee, H. N., and Chang, E. M. (2019). Primordial follicle activation as new treatment for primary ovarian insufficiency. Clin. Exp. Reprod. Med. 46, 43–49. doi: 10.5653/cerm.2019.46.2.43
Lee, S. E., Sun, S. C., Choi, H. Y., Uhm, S. J., and Kim, N. H. (2012). mTOR is required for asymmetric division through small GTPases in mouse oocytes. Mol. Reprod. Dev. 79, 356–366. doi: 10.1002/mrd.22035
Lee, W. S., Otsuka, F., Moore, R. K., and Shimasaki, S. (2001). Effect of bone morphogenetic protein-7 on folliculogenesis and ovulation in the rat. Biol. Reprod. 65, 994–999. doi: 10.1095/biolreprod65.4.994
Lew, R. (2019). Natural history of ovarian function including assessment of ovarian reserve and premature ovarian failure. Best Pract. Res. Clin. Obstet. Gynaecol. 55, 2–13. doi: 10.1016/j.bpobgyn.2018.05.005
Li, J., Kawamura, K., Cheng, Y., Liu, S., Klein, C., and Liu, S. (2010). Activation of dormant ovarian follicles to generate mature eggs. Proc. Natl. Acad. Sci. U.S.A. 107, 10280–10284. doi: 10.1073/pnas.1001198107
Li, L., Wang, B., Zhang, W., Chen, B., Luo, M., Wang, J., et al. (2017). A homozygous NOBOX truncating variant causes defective transcriptional activation and leads to primary ovarian insufficiency. Hum. Reprod. 32, 248–255. doi: 10.1093/humrep/dew271
Li, Z., Hannigan, M., Mo, Z., Liu, B., Lu, W., Wu, Y., et al. (2003). Directional sensing requires G beta gamma-mediated PAK1 and PIX alpha-dependent activation of Cdc42. Cell 114, 215–227. doi: 10.1016/S0092-8674(03)00559-2
Li-Ping, Z., Da-Lei, Z., Jian, H., Liang-Quan, X., Ai-Xia, X., Xiao-Yu, D., et al. (2010). Proto-oncogene c-erbB2 initiates rat primordial follicle growth via PKC and MAPK pathways. Reprod. Biol. Endocrinol. 8:66. doi: 10.1186/1477-7827-8-66
Liu, G., Li, Y., Du, B., Sun, Q., Qi, W., Liu, Y., et al. (2019). Primordial follicle activation is affected by the absence of Sohlh1 in mice. Mol. Reprod. Dev. 86, 20–31. doi: 10.1002/mrd.23078
Liu, K., Rajareddy, S., Liu, L., Jagarlamudi, K., Boman, K., Selstam, G., et al. (2006). Control of mammalian oocyte growth and early follicular development by the oocyte PI3 kinase pathway: new roles for an old timer. Dev. Biol. 299, 1–11. doi: 10.1016/j.ydbio.2006.07.038
Liu, L., Rajareddy, S., Reddy, P., Du, C., Jagarlamudi, K., Shen, Y., et al. (2007). Infertility caused by retardation of follicular development in mice with oocyte-specific expression of Foxo3a. Development 134, 199–209. doi: 10.1242/dev.02667
Lowery, J. W., and Rosen, V. (2018). The BMP pathway and its inhibitors in the skeleton. Physiol. Rev. 98, 2431–2452. doi: 10.1152/physrev.00028.2017
Lu, C. L., Yan, J., Zhi, X., Xia, X., Wang, T. R., Yan, L. Y., et al. (2015). Basic fibroblast growth factor promotes macaque follicle development in vitro. Reproduction 149, 425–433. doi: 10.1530/REP-14-0557
Maidarti, M., Anderson, R. A., and Telfer, E. E. (2020). Crosstalk between PTEN/PI3K/Akt signalling and DNA damage in the oocyte: implications for primordial follicle activation, oocyte quality and ageing. Cells 9:200. doi: 10.3390/cells9010200
Maidarti, M., Clarkson, Y. L., McLaughlin, M., Anderson, R. A., and Telfer, E. E. (2019). Inhibition of PTEN activates bovine non-growing follicles in vitro but increases DNA damage and reduces DNA repair response. Hum. Reprod. 34, 297–307. doi: 10.1093/humrep/dey354
Martinez-Anton, A., Gras, D., Bourdin, A., Dubreuil, P., and Chanez, P. (2019). KIT as a therapeutic target for non-oncological diseases. Pharmacol. Ther. 197, 11–37. doi: 10.1016/j.pharmthera.2018.12.008
Maruo, T., Ladines-Llave, C. A., Samoto, T., Matsuo, H., Manalo, A. S., Ito, H., et al. (1993). Expression of epidermal growth factor and its receptor in the human ovary during follicular growth and regression. Endocrinology 132, 924–931. doi: 10.1210/endo.132.2.8425504
Masciangelo, R., Hossay, C., Chiti, M. C., Manavella, D. D., Amorim, C. A., Donnez, J., et al. (2020). Role of the PI3K and Hippo pathways in follicle activation after grafting of human ovarian tissue. J. Assist. Reprod. Genet. 37, 101–108. doi: 10.1007/s10815-019-01628-1
Mathieu, M. E., Saucourt, C., Mournetas, V., Gauthereau, X., Thézé, N., Praloran, V., et al. (2012). LIF-dependent signaling: new pieces in the Lego. Stem Cell Rev. 8, 1–15. doi: 10.1007/s12015-011-9261-7
McGee, E. A., and Hsueh, A. J. (2000). Initial and cyclic recruitment of ovarian follicles. Endocr. Rev. 21, 200–214. doi: 10.1210/edrv.21.2.0394
McNatty, K. P., Heath, D. A., Lundy, T., Fidler, A. E., Quirke, L., O’Connell, A., et al. (1999). Control of early ovarian follicular development. J. Reprod. Fertil. 54, 3–16.
Myers, M., Tripurani, S. K., Middlebrook, B., Economides, A. N., Canalis, E., and Pangas, S. A. (2011). Loss of gremlin delays primordial follicle assembly but does not affect female fertility in mice. Biol. Reprod. 85, 1175–1182. doi: 10.1095/biolreprod.111.091728
Na, J., and Zernicka-Goetz, M. (2006). Asymmetric positioning and organization of the meiotic spindle of mouse oocytes requires CDC42 function. Curr. Biol. 16, 1249–1254. doi: 10.1016/j.cub.2006.05.023
Nilsson, E., Parrott, J. A., and Skinner, M. K. (2001). Basic fibroblast growth factor induces primordial follicle development and initiates folliculogenesis. Mol. Cell. Endocrinol. 175, 123–130. doi: 10.1016/S0303-7207(01)00391-4
Nilsson, E., Rogers, N., and Skinner, M. K. (2007). Actions of anti-Mullerian hormone on the ovarian transcriptome to inhibit primordial to primary follicle transition. Reproduction 134, 209–221. doi: 10.1530/REP-07-0119
Nilsson, E. E., Kezele, P., and Skinner, M. K. (2002). Leukemia inhibitory factor (LIF) promotes the primordial to primary follicle transition in rat ovaries. Mol. Cell. Endocrinol. 188, 65–73. doi: 10.1016/S0303-7207(01)00746-8
Nilsson, E. E., Larsen, G., and Skinner, M. K. (2014). Roles of Gremlin 1 and Gremlin 2 in regulating ovarian primordial to primary follicle transition. Reproduction 147, 865–874. doi: 10.1530/REP-14-0005
Nilsson, E. E., and Skinner, M. K. (2003). Bone morphogenetic protein-4 acts as an ovarian follicle survival factor and promotes primordial follicle development. Biol. Reprod. 69, 1265–1272.
Nilsson, E. E., and Skinner, M. K. (2004). Kit ligand and basic fibroblast growth factor interactions in the induction of ovarian primordial to primary follicle transition. Mol. Cell. Endocrinol. 214, 19–25.
Pangas, S. A., Choi, Y., Ballow, D. J., Zhao, Y., Westphal, H., Matzuk, M. M., et al. (2006). Oogenesis requires germ cell-specific transcriptional regulators Sohlh1 and Lhx8. Proc. Natl. Acad. Sci. U.S.A. 103, 8090–8095.
Park, B. K., Park, M. J., Kim, H. G., Han, S. E., Kim, C. W., Joo, B. S., et al. (2020). Role of visfatin in restoration of ovarian aging and fertility in the mouse aged 18 months. Reprod. Sci. 27, 681–689.
Pelosi, E., Omari, S., Michel, M., Ding, J., Amano, T., Forabosco, A., et al. (2013). Constitutively active Foxo3 in oocytes preserves ovarian reserve in mice. Nat. Commun. 4:1843.
Pérez, P., Soto, T., Gómez-Gil, E., and Cansado, J. (2020). Functional interaction between Cdc42 and the stress MAPK signaling pathway during the regulation of fission yeast polarized growth. Int. Microbiol. 23, 31–41.
Rajadurai, P., Fatt, H. K., and Ching, F. Y. (2018). Prevalence of HER2 positivity and its clinicopathological correlation in locally Advanced/Metastatic Gastric Cancer Patients in Malaysia. J. Gastrointest. Cancer 49, 150–157.
Rajareddy, S., Reddy, P., Du, C., Liu, L., Jagarlamudi, K., Tang, W., et al. (2007). p27kip1 (cyclin-dependent kinase inhibitor 1B) controls ovarian development by suppressing follicle endowment and activation and promoting follicle atresia in mice. Mol. Endocrinol. 21, 2189–2202.
Rajkovic, A., Pangas, S. A., Ballow, D., Suzumori, N., and Matzuk, M. M. (2004). NOBOX deficiency disrupts early folliculogenesis and oocyte-specific gene expression. Science 305, 1157–1159.
Reddy, P., Adhikari, D., Zheng, W., Liang, S., Hämäläinen, T., Tohonen, V., et al. (2009). PDK1 signaling in oocytes controls reproductive aging and lifespan by manipulating the survival of primordial follicles. Hum. Mol. Genet. 18, 2813–2824.
Reddy, P., Liu, L., Adhikari, D., Jagarlamudi, K., Rajareddy, S., Shen, Y., et al. (2008). Oocyte-specific deletion of Pten causes premature activation of the primordial follicle pool. Science 319, 611–613.
Reddy, P., Zheng, W., and Liu, K. (2010). Mechanisms maintaining the dormancy and survival of mammalian primordial follicles. Trends Endocrinol. Metab. 21, 96–103.
Ren, Y., Suzuki, H., Jagarlamudi, K., Golnoski, K., McGuire, M., Lopes, R., et al. (2015). Lhx8 regulates primordial follicle activation and postnatal folliculogenesis. BMC Biol. 13:39.
Roness, H., Spector, I., Leichtmann-Bardoogo, Y., Savino, A. M., Dereh-Haim, S., and Meirow, D. (2019). Pharmacological administration of recombinant human AMH rescues ovarian reserve and preserves fertility in a mouse model of chemotherapy, without interfering with anti-tumoural effects. J. Assist. Reprod. Genet. 36, 1793–1803.
Schmidt, D., Ovitt, C. E., Anlag, K., Fehsenfeld, S., Gredsted, L., Treier, A. C., et al. (2004). The murine winged-helix transcription factor Foxl2 is required for granulosa cell differentiation and ovary maintenance. Development 131, 933–942.
Shahrokhi, S. Z., Kazerouni, F., and Ghaffari, F. (2018). Anti-Müllerian Hormone: genetic and environmental effects. Clin. Chim. Acta 476, 123–129.
Silva, J. R., van den Hurk, R., de Matos, M. H., dos Santos, R. R., Pessoa, C., de Moraes, M. O., et al. (2004). Influences of FSH and EGF on primordial follicles during in vitro culture of caprine ovarian cortical tissue. Theriogenology 61, 1691–1704.
Song, Z. H., Yu, H. Y., Wang, P., Mao, G. K., Liu, W. X., Li, M. N., et al. (2015). Germ cell-specific Atg7 knockout results in primary ovarian insufficiency in female mice. Cell Death Dis. 6:e1589.
Sun, X., Su, Y., He, Y., Zhang, J., Liu, W., Zhang, H., et al. (2015). New strategy for in vitro activation of primordial follicles with mTOR and PI3K stimulators. Cell Cycle 14, 721–731.
Suzuki, H., Dann, C. T., and Rajkovic, A. (2013). Generation of a germ cell-specific mouse transgenic CHERRY reporter, Sohlh1-mCherryFlag. Genesis 51, 50–58.
Suzumori, N., Yan, C., Matzuk, M. M., and Rajkovic, A. (2002). Nobox is a homeobox-encoding gene preferentially expressed in primordial and growing oocytes. Mech. Dev. 111, 137–141.
Takeuchi, A., Koga, K., Satake, E., Makabe, T., Taguchi, A., Miyashita, M., et al. (2019). Endometriosis triggers excessive activation of primordial follicles via PI3K-PTEN-Akt-Foxo3 pathway. J. Clin. Endocrinol. Metab. 104, 5547–5554. doi: 10.1210/jc.2019-00281
Tan, J., Zou, Y., Wu, X. W., Tian, L. F., Su, Q., He, J. X., et al. (2017). Increased SCF in follicular fluid and granulosa cells positively correlates with oocyte maturation, fertilization, and embryo quality in humans. Reprod. Sci. 24, 1544–1550. doi: 10.1177/1933719117697125
Tanwar, P. S., O’Shea, T., and McFarlane, J. R. (2008). In vivo evidence of role of bone morphogenetic protein-4 in the mouse ovary. Anim. Reprod. Sci. 106, 232–240. doi: 10.1016/j.anireprosci.2007.04.015
Tewari, D., Patni, P., Bishayee, A., Sah, A. N., and Bishayee, A. (2019). Natural products targeting the PI3K-Akt-mTOR signaling pathway in cancer: a novel therapeutic strategy. Semin. Cancer Biol. doi: 10.1016/j.semcancer.2019.12.008 [Epub ahead of print].
Tong, Y., Li, F., Lu, Y., Cao, Y., Gao, J., and Liu, J. (2013). Rapamycin-sensitive mTORC1 signaling is involved in physiological primordial follicle activation in mouse ovary. Mol. Reprod. Dev. 80, 1018–1034. doi: 10.1002/mrd.22267
Umer, S., Sammad, A., Zou, H., Khan, A., Weldegebriall Sahlu, B., Hao, H., et al. (2019). Regulation of AMH, AMHR-II, and BMPs (2,6) genes of bovine granulosa cells treated with exogenous FSH and their association with protein hormones. Genes 10:1038. doi: 10.3390/genes10121038
Vanderhyden, B. (2002). Molecular basis of ovarian development and function. Front. Biosci. 7, d2006–d2022. doi: 10.2741/A895
Wang, T. R., Yan, L. Y., Yan, J., Lu, C. L., Xia, X., Yin, T. L., et al. (2014). Basic fibroblast growth factor promotes the development of human ovarian early follicles during growth in vitro. Hum. Reprod. 29, 568–576. doi: 10.1093/humrep/det465
Wright, C. J., Cari, E. L., Sandoval, J., Bales, E., Sam, P. K., Zarate, M. A., et al. (2020). Control of murine primordial follicle growth activation by IκB/NFκB signaling. Reprod. Sci. doi: 10.1007/s43032-020-00225-3
Yan, H., Wen, J., Zhang, T., Zheng, W., He, M., Huang, K., et al. (2019). Oocyte-derived E-cadherin acts as a multiple functional factor maintaining the primordial follicle pool in mice. Cell Death Dis. 10:160. doi: 10.1038/s41419-018-1208-3
Yan, H., Zhang, J., Wen, J., Wang, Y., Niu, W., Teng, Z., et al. (2018). CDC42 controls the activation of primordial follicles by regulating PI3K signaling in mouse oocytes. BMC Biol. 16:73. doi: 10.1186/s12915-018-0541-4
Yang, M. Y., Cushman, R. A., and Fortune, J. E. (2017). Anti-Müllerian hormone inhibits activation and growth of bovine ovarian follicles in vitro and is localized to growing follicles. Mol. Hum. Reprod. 23, 282–291. doi: 10.1093/molehr/gax010
Yorino Sato Kawamura, K. (2020). Rapamycin treatment maintains developmental potential of oocytes in mice and follicle reserve in human cortical fragments grafted into immune-deficient mice. Mol. Cell. Endocrinol. 504:110694. doi: 10.1016/j.mce.2019.110694
Yurube, T., Ito, M., Kakiuchi, Y., Kuroda, R., and Kakutani, K. (2020). Autophagy and mTOR signaling during intervertebral disc aging and degeneration. JOR Spine 3:e1082. doi: 10.1002/jsp2.1082
Zhang, H., and Liu, K. (2015). Cellular and molecular regulation of the activation of mammalian primordial follicles: somatic cells initiate follicle activation in adulthood. Hum. Reprod. 21, 779–786. doi: 10.1093/humupd/dmv037
Zhang, H., Risal, S., Gorre, N., Busayavalasa, K., Li, X., Shen, Y., et al. (2014). Somatic cells initiate primordial follicle activation and govern the development of dormant oocytes in mice. Curr. Biol. 24, 2501–2508. doi: 10.1016/j.cub.2014.09.023
Zhang, M., Jang, H., and Nussinov, R. (2019). The mechanism of PI3Kα activation at the atomic level. Chem. Sci. 10, 3671–3680. doi: 10.1039/C8SC04498H
Zhang, X., Liu, R., Su, Z., Zhang, Y., Zhang, W., Liu, X., et al. (2015). Immunohistochemical study of expression of Sohlh1 and Sohlh2 in Normal Adult Human Tissues. PLoS One 10:e0137431. doi: 10.1371/journal.pone.0137431
Zheng, W., Nagaraju, G., Liu, Z., and Liu, K. (2012). Functional roles of the phosphatidylinositol 3-kinases (PI3Ks) signaling in the mammalian ovary. Mol. Cell. Endocrinol. 356, 24–30. doi: 10.1016/j.mce.2011.05.027
Zheng, W., Zhang, H., Gorre, N., Risal, S., Shen, Y., and Liu, K. (2014). Two classes of ovarian primordial follicles exhibit distinct developmental dynamics and physiological functions. Hum. Mol. Genet. 23, 920–928. doi: 10.1093/hmg/ddt486
Keywords: primordial follicle, activation, signaling pathways, factors, mechanism
Citation: Chen Y, Yang W, Shi X, Zhang C, Song G and Huang D (2020) The Factors and Pathways Regulating the Activation of Mammalian Primordial Follicles in vivo. Front. Cell Dev. Biol. 8:575706. doi: 10.3389/fcell.2020.575706
Received: 24 June 2020; Accepted: 07 September 2020;
Published: 30 September 2020.
Edited by:
Lisa Porter, University of Windsor, CanadaReviewed by:
Kouji Komatsu, Aichi Medical University, JapanCopyright © 2020 Chen, Yang, Shi, Zhang, Song and Huang. This is an open-access article distributed under the terms of the Creative Commons Attribution License (CC BY). The use, distribution or reproduction in other forums is permitted, provided the original author(s) and the copyright owner(s) are credited and that the original publication in this journal is cited, in accordance with accepted academic practice. No use, distribution or reproduction is permitted which does not comply with these terms.
*Correspondence: Donghui Huang, amhzeXlqc0AxMjYuY29t; Ge Song, c29uZ2dlcHBAMTI2LmNvbQ==
†These authors have contributed equally to this work
Disclaimer: All claims expressed in this article are solely those of the authors and do not necessarily represent those of their affiliated organizations, or those of the publisher, the editors and the reviewers. Any product that may be evaluated in this article or claim that may be made by its manufacturer is not guaranteed or endorsed by the publisher.
Research integrity at Frontiers
Learn more about the work of our research integrity team to safeguard the quality of each article we publish.