- 1Department of Gastroenterology, The First Affiliated Hospital of Nanchang University, Nanchang, China
- 2Department of Rheumatology, The First Affiliated Hospital of Nanchang University, Nanchang, China
Leukocyte invasion (neutrophils and monocytes/macrophages) is closely related to the severity of acute pancreatitis (AP) and plays an important role in the systemic inflammatory response and other organ injuries secondary to AP. Increased and sustained activation of neutrophils are major determinants of pancreatic injury and inflammation. After the onset of AP, the arrival of the first wave of neutrophils occurs due to a variety of triggers and is critical for the exacerbation of inflammation. In this review, we summarize the functional characteristics of neutrophils, elastase, and heparin-binding proteins in granules, the mechanisms of neutrophil recruitment and the role of neutrophil extracellular traps (NETs) in AP.
Introduction
Neutrophils are polynuclear white blood cells and one of the main responders to acute inflammation. White blood cells are usually the first cell type recruited to the site of inflammation and fight pathogens through a variety of mechanisms. In humans, neutrophils account for 50–70% of all circulating white blood cells, while in mice, neutrophils account for 10–25% of circulating white blood cells (Doeing et al., 2003; Mestas and Hughes, 2004). In circulation, the nuclei of mature neutrophils are segmented and approximately 7–10 μm in diameter, and the cytoplasm is rich in granules and secretory vesicles (Borregaard, 2010). Neutrophils are the first responders in host defense against a variety of pathogens, including bacteria, fungi, and protozoa. The migration of neutrophils to the site of inflammation is essential for clearing the infection, and a significant reduction in the number of neutrophils in the blood can lead to severe immune deficiency. In addition to clearing pathogens in acute infections, neutrophils play important and adverse roles in other inflammation-related diseases.
Acute pancreatitis (AP) is pancreatic inflammation caused by abnormal activation of trypsinogen, which can lead to systemic inflammatory response syndrome (SIRS) and organ failure (Lankisch et al., 2015). In particular, acute lung injury (ALI) can be observed at an early stage in patients with severe acute pancreatitis (SAP) (Zhou et al., 2010). Although there has been considerable progress in recent years in the treatment of AP, especially SAP, the mortality rate is still high, and the specific pathogenesis is still unclear. Typical features of pancreatic injury and a large number of inflammatory cells, including neutrophils and macrophages, have been observed in pancreatic tissue specimens from patients with AP and animal models. Neutrophils are primarily thought to be the first immune cells to be recruited to inflammatory tissue in the event of acute inflammation. Neutrophils are important in systemic injury and death associated with SAP that is induced by choline-deficient ethionine-supplemented (CDE) diet L-arginine or deoxycholic acid injection into the pancreatic duct. Neutrophil depletion can significantly reduce pancreatic injury and damage to organ systems and significantly improve the SAP survival rate (Inoue et al., 1995; Kyriakides et al., 2001; Chen et al., 2015). In addition, neutrophils mediate further activation of trypsinogen byproducts of NADPH oxidase, exacerbate pancreatic injury and even cause lung injury (Gukovskaya et al., 2002; Abdulla et al., 2011). Thus, the purpose of this review is to further discuss the function of neutrophils and the pathogenesis of AP.
Features and Functions of Neutrophils
Neutrophils are derived from myeloid progenitor cells in the bone marrow and are regulated by many transcription factors, proteins, and receptors. The main neutrophil regulator is granulocyte colony stimulating factor (G-CSF) (Lieschke et al., 1994). Neutrophils have been regarded as having a short lifespan of 6–8 h in humans and mice, but they have also been reported to live up to 5.4 days (Pillay et al., 2010; Tak et al., 2013). However, during the inflammatory response, neutrophils are stimulated and activated, and their lifespan is significantly increased several-fold, thus ensuring the persistence of neutrophils at the site of infection (Colotta et al., 1992; Summers et al., 2010). Neutrophils have many biological functions, including chemotaxis, antimicrobial functions, phagocytosis, degranulation, and neutrophil extracellular trap (NET) production. In vivo, neutrophils are the first line of defense against bacterial and fungal infections. These cells kill invading pathogens with a large number of antibacterial agents, including reactive oxygen species (ROS) and hydrolases. Neutrophils release NETs and antibacterial granules through NETosis to kill and prevent the spread of pathogens (Brinkmann et al., 2004). It is well-known that during acute inflammation, neutrophils are rapidly recruited to tissues affected by inflammation in a multistep recruitment cascade (Maas et al., 2018). Although neutrophil recruitment is traditionally thought to be unidirectional (from blood to tissue), some studies have also shown that neutrophils can migrate from tissue to blood and even other tissues, causing secondary tissue damage (Woodfin et al., 2011; Wang et al., 2017).
Neutrophil Recruitment in Acute Pancreatitis
Neutrophil Recruitment to Pancreas During AP
During an inflammatory response, the recruitment cascade of leukocytes occurs and involves tethering, rolling, adhesion, crawling, and eventually migration. Initial neutrophil recruitment cascades on the surface of endothelial cells result from stimulation by inflammatory mediators (including cytokines, leukotrienes, and histamines) that are released by tissue-resident leukocytes as a result of encounters with pathogens (Ley et al., 2007; Phillipson and Kubes, 2011; Sadik et al., 2011). Large doses of cerulein induce a significant increase in the expression of CXCL2 (also known as macrophage inflammatory protein-2), which is an effective neutrophil attractant (Pastor et al., 2003). Decreased levels of CXCL2 in the pancreas in AP reduce pancreatic neutrophil infiltration and tissue damage and reduce neutrophil invasion in lung tissue (Merza et al., 2013). Similarly, platelet-derived CXCL4 regulates neutrophil recruitment via secretion of CXCL2, and inhibition of CXCL4 markedly decreases pancreatic injury and plasma levels of CXCL2 in animals with AP (Wetterholm et al., 2016). When chemokines bind to polymorphonuclear leukocytes (PMNs), the intracellular store of MAC1 is also relocated to the cell surface (Jones et al., 1988). Deletion of CD18, a subunit of the MAC-1 complex, prevents transmigration of neutrophils into the pancreas during pancreatitis, greatly reducing disease severity and decreasing intrapancreatic protease activation (Sendler et al., 2013). The binding of lymphocyte function antigen-1 (LFA-1) to immunoglobulin-like cell adhesion molecule 1 (ICAM1) is essential for neutrophils to adhere firmly to endothelial cells (Phillipson et al., 2006). LFA-1 plays a key role in the regulation of pancreatic neutrophil recruitment, CXCL2 formation and tissue damage in taurocholate-induced AP (Awla et al., 2011). The interaction between PMNs and the endothelium is mediated by specific adhesion molecules in the selectin and integrin families, and adhesion also leads to enhanced activation of the neutrophil signaling pathway. Inhibition of P-selectin alleviated tissue damage by reducing neutrophil-endothelium interactions and improving pancreatic microcirculation in experimental AP (Hackert et al., 2009; Hartman et al., 2012). Neutrophils began to show adaptive changes in morphology, with actin cytoskeleton rearrangement (Leick et al., 2014). The neutrophils began to extend foot processes across the entire endothelial cell surface and then to move.
Neutrophil Recruitment to Lung During AP
Neutrophils are the first cells of the immune system to collect at damaged or inflammatory sites and are believed to play a key role in the development of AP-associated ALI (Willemer et al., 1991). Lung injury is caused by local lung endothelial cell injury secondary to the production of oxygen free radicals by neutrophils (Guice et al., 1989). During AP, a large number of neutrophils are rapidly recruited from the peripheral circulation to the site of pancreatic inflammation. Toll-like receptor 4 (TLR4) mediates the immune response and plays a proinflammatory role in the progression of acute pancreatitis by promoting neutrophil recruitment (Sharif et al., 2009). The neutrophils that accumulate in the pancreas migrate in a retrograde manner to the circulatory system, causing systemic inflammation and damage to other organs. Circulating neutrophils isolated from patients during AP cause endothelial damage (Paulino et al., 2007). These cells are tissue-characteristic neutrophils, have distinct phenotypes and are known as reverse-migrating neutrophils. Reverse-migrating neutrophils have a more robust ability to produce ROS than other types of neutrophils, thereby exacerbating local tissue damage. Knockdown of junctional adhesion molecule-C (JAM-C) exacerbates lung injury and systemic inflammation in experimental pancreatitis. An increase in neutrophil reverse transendothelial migration (rTEM) was observed in JAM-C-deficient mice, and JAM-C played a key role in promoting rTEM (Wu et al., 2016). Neutrophils that reach the basement membrane pause before crossing the basement membrane. Matrix metalloproteinase 9 (MMP-9) is necessary for neutrophil migration by degrading some basement membrane components, including type IV collagens, fibronectins, and gelatin. In a model of severe necrotizing pancreatitis, MMP-9 is released from neutrophils stimulated by pancreatic enzymes and proinflammatory mediators. Inhibition of MMP-9 by batimastat (BB-94) reduces neutrophil transmigration and alveolar capillary permeability in pancreatitis-associated lung injury (Keck et al., 2002).
The Proteins in Neutrophil Granules in Acute Pancreatitis
Neutrophils also release granules that have antimicrobial properties in the progression of many inflammatory disorders via a variety of mechanisms. Granules include primary neutrophil granules, which are composed of elastase, cathepsin G, myeloperoxidase (MPO), and other proteins, and secondary and tertiary granule particles, which are composed of proteins such as lactoferrin and gelatinase (Papayannopoulos et al., 2010; Metzler et al., 2014).
Neutrophil Elastase
Analysis of serum samples of AP patients in the clinic showed that the activity of neutrophil elastase (NE) was associated with the predicted severity of AP and AP-associated respiratory failure (Novovic et al., 2013). During the early pathological process of pancreatitis, the dissolution of cell-cell contacts causes the formation of pancreatic edema and cell damage. While E-cadherin is the key protein mediating cell contact, neutrophil elastin cleaves E-cadherin to cause the dissociation of cell contacts, which then permits increased transmigration of leukocytes into the pancreas (John et al., 2019). Inhibition of NE in vivo significantly reduced the translocation of leukocytes and decreased disease severity (Mayerle et al., 2005; Jo et al., 2008). Neutrophils with increased NE activity exacerbate pulmonary edema and damage in ALI (Kinoshita et al., 2000). NE is not only involved in pancreatic tissue injury but also aggravates the systemic inflammatory response, thus causing the critical process of AP. Therefore, effective targeted inhibition of NE may be a promising strategy for the treatment of AP, thereby reducing the incidence of severe disease.
Cathepsin G
Cathepsin G (CTSG) is a serine protease of the chymotrypsin C family that is stored in primary granules of neutrophils. Its major biological functions include the degradation of extracellular matrix and plasma proteins. The broad serine proteinase inhibitor (serpin) Lex032, which can inhibit both CTSG and NE, reduces the capillary no-reflow phenomenon and pancreatic tissue damage in ischemia/reperfusion-induced pancreatitis (von Dobschuetz et al., 1999). However, Aghdassi et al. found that CTSG knockout affects neutrophil invasion in early AP but does not affect the severity of the disease because CTSG had no effect on intraacinar cell trypsinogen activation (Aghdassi et al., 2019). Compared with targeted inhibition of NE activity, inhibition of CTSG does not seem to have a promising effect in the experimental AP model.
Heparin-Binding Protein
Human neutrophils secrete cationic antimicrobial protein 37, which efficiently binds heparin and is also named heparin-binding protein (HBP). HBP plays an important role in regulating inflammation and promoting vascular leakage (Gautam et al., 2001; Soehnlein et al., 2008). Through network bioinformatics analysis, Nune et al. found that among normal healthy people and patients with pancreatic diseases, the extracellular HBP concentration coefficient was highest in AP patients (Nunes et al., 2013). Glycosaminoglycan, as an inhibitor of HBP, can reduce the occurrence of cerulein-induced AP by reducing the inflammatory response and even improve the survival rate of the sepsis mouse model (Juhas et al., 2018). In addition, many studies have shown that serum HBP levels are positively correlated with the severity of many critical inflammatory diseases and can be used as a promising serum marker to predict disease severity (Linder et al., 2009; Lin et al., 2013; Tyden et al., 2017). Based on the characteristics of HBP, it was hypothesized that HBP is involved in the process of severe disease and the functional injury of organs other than the pancreas in SAP.
Neutrophil Extracellular Traps in Acute Pancreatitis
Neutrophils have a key role in combating bacterial infections and have evolved additional mechanisms that increase their effectiveness in eliminating pathogens. NETs are the second bactericidal mechanism of neutrophils (Brinkmann et al., 2004). NETs are composed of decondensed DNA complexed with histones and granulosa proteins and do not contain any other cytoskeletal proteins. Nucleic acid substances are the backbone of NETs and form a skeletal structure that holds various protein particles together. NETs are produced by a variety of inducing factors, such as ROS, IL-8, LPS, complement factor 5 a, and β-glucan (Delgado-Rizo et al., 2017). ROS production is the key to PMN outbreaks and NET release (Stoiber et al., 2015). NETs trap and kill microbes, amplify immune responses, and induce coagulation (Sollberger et al., 2018). There are three important enzymes in NET production: peptidyl arginine deiminase 4 (PAD4), NE and MPO. Calcium ions can activate PAD4, which catalyzes the transformation of histone arginine into citrulline, reducing the strong positive charge of histones and weakening the binding of histones to DNA to promote chromatin depolymerization, which is the basis for NET formation in vivo (Demaurex et al., 1994; Wang and Wang, 2013; Sorensen and Borregaard, 2016). After chromatin depolymerization, the nuclear membrane ruptures, and chromatin is released into the cytoplasm, which is then released into the extracellular space through modification by granular protein to form NETs (Metzler et al., 2014). Even when stimulated by pathogenic activators such as bacteria, PAD4 knockout mice were unable to form NETs (Li et al., 2010). Therefore, the PAD4-induced deimination of histones is a prerequisite for the formation of NETs in vivo. Chlor-amidine irreversibly inhibited PAD4 through covalent modification at the active site of the enzymes and prevented NET-mediated vascular damage, endothelial dysfunction, and kidney injury in a mouse model of lupus (Knight et al., 2015; Witalison et al., 2015). NETs are a physical barrier that limits the spread of pathogens and inflammatory transmitters, preventing damage to normal tissues. NETs seem to play a protective role in the necrotic areas of acute necrotizing pancreatitis and form a provisionary tissue barrier separating necrotic areas from the remaining viable tissue (Bilyy et al., 2016). NETs can kill pathogens and are related to nucleic acid substances, NE, antimicrobial peptides and other components. The DNA skeleton makes NETs highly bactericidal, and the phosphodiester backbone of DNA is critical for this bactericidal activity (Halverson et al., 2015). Although NETs play a role in killing microbes, they have some negative effects, such as stimulating autoimmunity and thrombosis (Sorensen and Borregaard, 2016).
NETs play an important role in the pathophysiological mechanism of AP by promoting pancreatic tissue damage in a variety of ways. In a mouse model of acute necrotizing pancreatitis (ANP) induced by sodium taurocholate, a large amount of NETs and histones were deposited in the pancreatic tissue (Merza et al., 2015). NETs induce the activation of trypsinogen through STAT3, MMP-9, and other pathways, thereby amplifying the degree of pancreatic injury (Awla et al., 2012; Zhang et al., 2013; Korhonen et al., 2015; Merza et al., 2015). The clearance of neutrophils significantly reduces NET deposition in pancreatic tissue and cell-free DNA levels in plasma. Deoxyribonuclease I (DNase I) can depolymerize the DNA skeleton in NETs and thus destroy the structure of NETs (Meng et al., 2012). After DNase I administration, the expression level of histones in the pancreatic tissue of SAP mice decreased, the degree of pancreatic edema significantly decreased, and the level of blood amylase was also reduced (Merza et al., 2015). Chloroquine, as an autophagy inhibitor, improves the prognosis of mice with AP by reducing NET formation and the levels of serum cell-free DNA and citrullinated histone H3 (Murthy et al., 2019). In addition, one study revealed another mechanism by which the accumulation of NETs in the pancreatic duct leads to catheter obstruction, which drives pancreatic inflammation, while PAD4 deficiency prevents disease progression by reducing the formation of NETs in the duct (Leppkes et al., 2016). Therefore, targeted inhibition of NETs is expected to be a clinical strategy for the treatment of AP.
Local and systemic early pathological events of AP are associated with vascular disorders, including endothelial activation and injury, increased vascular permeability, and coagulation activation (Dumnicka et al., 2017). NETs promote thrombosis and have been shown to trap large concentrations of platelets, activated thrombin, and fibrin clots in a mouse model of sepsis. Similarly, the removal of NETs by DNase I can significantly decrease thrombin activity, reduce platelet aggregation, and improve microvascular permeability (McDonald et al., 2017). NETs provide a physical scaffold for thrombosis (Fuchs et al., 2010). Furthermore, platelet inositol hexaphosphatase 1 (IP6K1) is a key NET formation gene. Thrombin stimulation of IP6K1-deficient platelets in the presence of neutrophils results in no NET formation. NET formation induced by taurocholate reduced pancreatic inflammation and tissue damage in IP6K1-deficient mice (Madhi et al., 2019). Therefore, NETs play a role in mediating inflammation and thrombosis, bridging the transition between hypercoagulability and severe inflammation in the early pathophysiology of pancreatitis. A recent study found that dabigatran, an anticoagulant and antitrypsin drug, has a promising synergistic effect in mice with severe pancreatitis (Gui et al., 2020).
The systemic inflammatory response is the main manifestation of SAP, and respiratory failure is the most common complication of SAP. In the serum of SAP patients, the levels of cell-free DNA and DNA-histone complexes were significantly elevated. Circulating NETs play an important role in the recruitment of neutrophils in the lung, resulting in lung injury (Merza et al., 2015). NET structures were detected in the blood of patients with transfusion-related acute lung injury (TRALI), and NETs were also observed in the TRALI mouse model; NET formation was alleviated by DNA enzyme I treatment (Caudrillier et al., 2012). This finding indicates that NETs cause lung epithelial cell injury, and the targeted formation of NETs may be a new strategy for the treatment of lung injury in SAP.
Perspectives of Anti-neutrophil Treatments in AP
In the early stage of acute pancreatitis, sterile inflammation is present, which induces systemic inflammatory response syndrome (SIRS), and the recruitment and activation of neutrophils play a major role (Sendler et al., 2020). The use of glucocorticoids and immunosuppressive agents in acute pancreatitis is still controversial, mainly because of the subsequent compensatory anti-inflammatory response syndrome (CARS) (Dong et al., 2015; Sendler et al., 2020). In the early stage of AP, neutrophils play an important proinflammatory role as the first recruit of acute inflammatory cells. Depletion of neutrophils by anti-Gr-1 antibody relieved plasma amylase and MPO activities, inflammation and tissue damage in the pancreas and lung in an L-arginine, choline-deficient ethionine (CDE) diet, or taurocholate-induced AP mouse model (Bhatia et al., 1998; Abdulla et al., 2011; Chen et al., 2015). Although one study showed that neutrophil depletion by injection of anti-Gr1 resulted in blockade of intracapillary leucocyte accumulation and led to the development of severe acute pancreatitis with fulminant intrapancreatic hemorrhage in taurocholate or enterokinase pancreatitis (Ryschich et al., 2009), intracapillary leucocyte accumulation was not observed in other models of acute pancreatitis, and decreasing leucocyte extravasation exhibited a protective effect. Platelet activating factor, an endogenous lipid mediator of inflammation, mediates the activation and infiltration of neutrophils into the tissue, and administration of its antagonist abolished apoptosis in experimental models of pancreatitis (Sandoval et al., 1996). In the early stages of AP, activated neutrophils release proteins in neutrophil granules, and NETs cause pancreatic inflammation and injury and even a systemic inflammatory response. Pharmacological inhibition of neutrophil biological behavior or the production of neutrophil products has become a treatment strategy for acute pancreatitis.
Conclusions and Future Directions
Total neutrophil deficiency or a significant reduction in the number of neutrophils can significantly reduce AP injury and inflammation. However, the reduction in neutrophils in mice is often associated with immunodeficiency. During the process of AP, neutrophils in pancreatic tissue appear to be overactivated or inappropriately recruited, resulting in pancreatic injury and even lung tissue damage. Prophylactic rectal non-selective non-steroidal anti-inflammatory drugs (NSAIDs) reduce the incidence or severity of post-ERCP (endoscopic retrograde cholangiopancreatography) pancreatitis, which reduces neutrophil infiltration into the pancreas (Geng et al., 2020). In summary, this review showed that neutrophil recruitment, granulocyte release, and NET formation are all involved in the exacerbation of AP (Figure 1). With the discovery of a new mechanism of neutrophils in AP, more precise or targeted therapy can be used to limit neutrophil-mediated destruction, minimize pancreatic injury and systemic inflammation, and minimize damage to patient immunity.
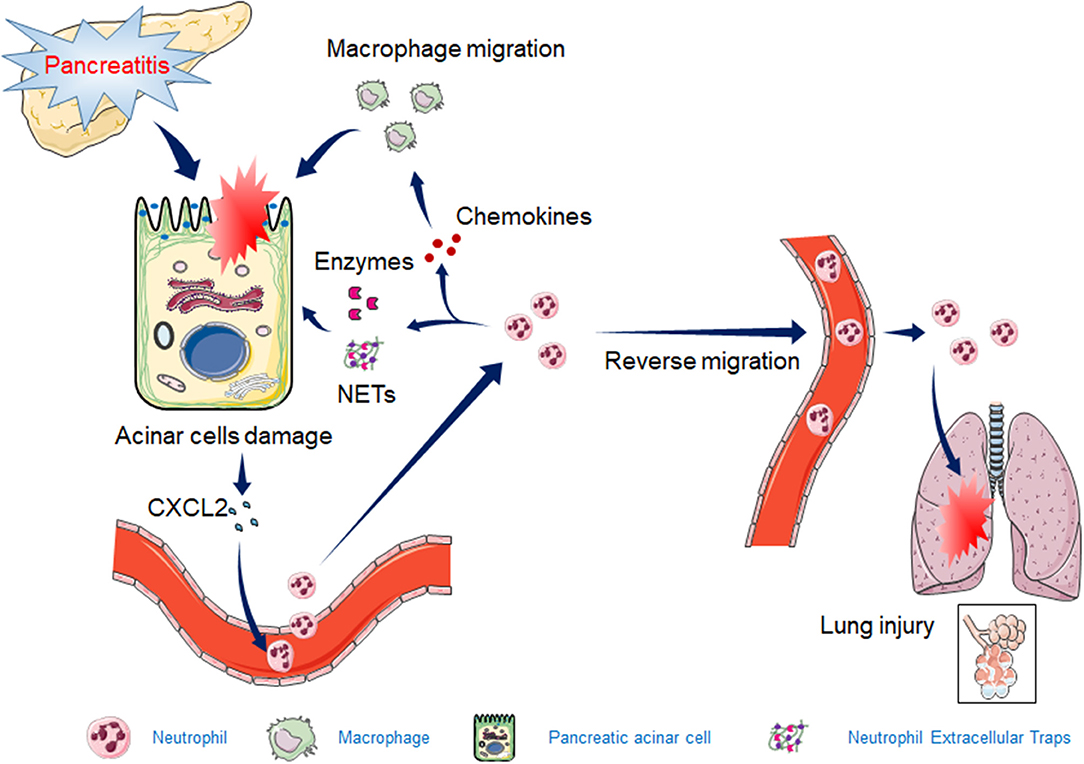
Figure 1. The mechanism by which neutrophils exacerbate acute pancreatitis (AP). The release of CXCL2 in early AP tissues induces the recruitment of neutrophils. Activated neutrophils induce the release of granulocytes, NET formation, and macrophage recruitment by releasing inflammatory chemokines, leading to increased pancreatic injury. Some of the activated neutrophils reverse migrate to the circulatory system, causing acute lung injury.
Author Contributions
JW and YR conceived the study, participated in the design, and drafted the manuscript. XY, XL, LX, and NL edited and checked the manuscript. All of the authors have read and approved the final manuscript.
Funding
This study was funded by the National Natural Science Foundation of China (Nos: 81760121 and 82060125), Funding Scheme for Outstanding Young Talents in Jiangxi Province (20192BCB23021), and Jiangxi Provincial Natural Science Foundation (20202BAB206010).
Conflict of Interest
The authors declare that the research was conducted in the absence of any commercial or financial relationships that could be construed as a potential conflict of interest.
Abbreviations
AP, acute pancreatitis; NETs, neutrophil extracellular traps; SIRS, systemic inflammatory response syndrome; ALI, acute lung injury; SAP, severe acute pancreatitis; CDE, choline-deficient ethionine-supplemented; G-CSF, granulocyte colony stimulating factor; ROS, reactive oxygen species; PMN, polymorphonuclear leukocyte; LFA-1, lymphocyte function antigen-1; ICAM1, immunoglobulin-like cell adhesion molecule 1; JAM-C, junctional adhesion molecule-C; rTEM, reverse transendothelial migration; MMP-9, matrix metalloproteinase 9; MPO, myeloperoxidase; NE, neutrophil elastase; CTSG, Cathepsin G; HBP, heparin-binding protein; PAD4, peptidyl arginine deiminase 4; ANP, acute necrotizing pancreatitis; DNase I, Deoxyribonuclease I; IP6K1, platelet inositol hexaphosphatase 1; TRALI, transfusion-related acute lung injury; CARS, compensatory anti-inflammatory response syndrome; CDE, choline-deficient ethionine; NSAIDs, non-steroidal anti-inflammatory drugs.
References
Abdulla, A., Awla, D., Thorlacius, H., and Regner, S. (2011). Role of neutrophils in the activation of trypsinogen in severe acute pancreatitis. J. Leukoc. Biol. 90, 975–982. doi: 10.1189/jlb.0411195
Aghdassi, A. A., John, D. S., Sendler, M., Storck, C., van den Brandt, C., Kruger, B., et al. (2019). Absence of the neutrophil serine protease cathepsin G decreases neutrophil granulocyte infiltration but does not change the severity of acute pancreatitis. Sci. Rep. 9:16774. doi: 10.1038/s41598-019-53293-0
Awla, D., Abdulla, A., Syk, I., Jeppsson, B., Regner, S., and Thorlacius, H. (2012). Neutrophil-derived matrix metalloproteinase-9 is a potent activator of trypsinogen in acinar cells in acute pancreatitis. J. Leukoc. Biol. 91, 711–719. doi: 10.1189/jlb.0811443
Awla, D., Abdulla, A., Zhang, S., Roller, J., Menger, M. D., Regner, S., et al. (2011). Lymphocyte function antigen-1 regulates neutrophil recruitment and tissue damage in acute pancreatitis. Br. J. Pharmacol. 163, 413–423. doi: 10.1111/j.1476-5381.2011.01225.x
Bhatia, M., Saluja, A. K., Hofbauer, B., Lee, H. S., Frossard, J. L., and Steer, M. L. (1998). The effects of neutrophil depletion on a completely noninvasive model of acute pancreatitis-associated lung injury. Int. J. Pancreatol. 24, 77–83.
Bilyy, R., Fedorov, V., Vovk, V., Leppkes, M., Dumych, T., Chopyak, V., et al. (2016). Neutrophil extracellular traps form a barrier between necrotic and viable areas in acute abdominal inflammation. Front. Immunol. 7:424. doi: 10.3389/fimmu.2016.00424
Borregaard, N. (2010). Neutrophils, from marrow to microbes. Immunity 33, 657–670. doi: 10.1016/j.immuni.2010.11.011
Brinkmann, V., Reichard, U., Goosmann, C., Fauler, B., Uhlemann, Y., Weiss, D. S., et al. (2004). Neutrophil extracellular traps kill bacteria. Science 303, 1532–1535. doi: 10.1126/science.1092385
Caudrillier, A., Kessenbrock, K., Gilliss, B. M., Nguyen, J. X., Marques, M. B., Monestier, M., et al. (2012). Platelets induce neutrophil extracellular traps in transfusion-related acute lung injury. J. Clin. Invest. 122, 2661–2671. doi: 10.1172/JCI61303
Chen, G., Xu, F., Li, J., and Lu, S. (2015). Depletion of neutrophils protects against L-arginine-induced acute pancreatitis in mice. Cell. Physiol. Biochem. 35, 2111–2120. doi: 10.1159/000374017
Colotta, F., Re, F., Polentarutti, N., Sozzani, S., and Mantovani, A. (1992). Modulation of granulocyte survival and programmed cell death by cytokines and bacterial products. Blood 80, 2012–2020. doi: 10.1182/blood.V80.8.2012.bloodjournal8082012
Delgado-Rizo, V., Martinez-Guzman, M. A., Iniguez-Gutierrez, L., Garcia-Orozco, A., Alvarado-Navarro, A., and Fafutis-Morris, M. (2017). Neutrophil extracellular traps and its implications in inflammation: an overview. Front. Immunol. 8:81. doi: 10.3389/fimmu.2017.00081
Demaurex, N., Monod, A., Lew, D. P., and Krause, K. H. (1994). Characterization of receptor-mediated and store-regulated Ca2+ influx in human neutrophils. Biochem. J. 297(Pt 3), 595–601. doi: 10.1042/bj2970595
Doeing, D. C., Borowicz, J. L., and Crockett, E. T. (2003). Gender dimorphism in differential peripheral blood leukocyte counts in mice using cardiac, tail, foot, and saphenous vein puncture methods. BMC Clin. Pathol. 3:3. doi: 10.1186/1472-6890-3-3
Dong, L.-H., Liu, Z.-M., Wang, S.-J., Zhao, S.-J., Zhang, D., Chen, Y., et al. (2015). Corticosteroid therapy for severe acute pancreatitis: a meta-analysis of randomized, controlled trials. Int. J. Clin. Exp. Pathol. 8, 7654–7660.
Dumnicka, P., Maduzia, D., Ceranowicz, P., Olszanecki, R., Drozdz, R., and Kusnierz-Cabala, B. (2017). The interplay between inflammation, coagulation and endothelial injury in the early phase of acute pancreatitis: clinical implications. Int. J. Mol. Sci. 18:354. doi: 10.3390/ijms18020354
Fuchs, T. A., Brill, A., Duerschmied, D., Schatzberg, D., Monestier, M., Myers, D. D. Jr., et al. (2010). Extracellular DNA traps promote thrombosis. Proc. Natl. Acad. Sci. U.S.A. 107, 15880–15885. doi: 10.1073/pnas.1005743107
Gautam, N., Olofsson, A. M., Herwald, H., Iversen, L. F., Lundgren-Akerlund, E., Hedqvist, P., et al. (2001). Heparin-binding protein (HBP/CAP37): a missing link in neutrophil-evoked alteration of vascular permeability. Nat. Med. 7, 1123–1127. doi: 10.1038/nm1001-1123
Geng, C., Li, X., Li, Y., Song, S., and Wang, C. (2020). Nonsteroidal anti-inflammatory drugs alleviate severity of post-endoscopic retrograde cholangiopancreatography pancreatitis by inhibiting inflammation and reducing apoptosis. J. Gastroenterol. Hepatol. 35, 896–904. doi: 10.1111/jgh.15012
Gui, F., Zhang, Y., Wan, J., Zhan, X., Yao, Y., Li, Y., et al. (2020). Trypsin activity governs increased susceptibility to pancreatitis in mice expressing human PRSS1R122H. J. Clin. Invest. 130, 189–202. doi: 10.1172/JCI130172
Guice, K. S., Oldham, K. T., Caty, M. G., Johnson, K. J., and Ward, P. A. (1989). Neutrophil-dependent, oxygen-radical mediated lung injury associated with acute pancreatitis. Ann. Surg. 210, 740–747. doi: 10.1097/00000658-198912000-00008
Gukovskaya, A. S., Vaquero, E., Zaninovic, V., Gorelick, F. S., Lusis, A. J., Brennan, M.-L., et al. (2002). Neutrophils and NADPH oxidase mediate intrapancreatic trypsin activation in murine experimental acute pancreatitis. Gastroenterology 122, 974–984. doi: 10.1053/gast.2002.32409
Hackert, T., Sperber, R., Hartwig, W., Fritz, S., Schneider, L., Gebhard, M. M., et al. (2009). P-selectin inhibition reduces severity of acute experimental pancreatitis. Pancreatology 9, 369–374. doi: 10.1159/000212098
Halverson, T. W., Wilton, M., Poon, K. K., Petri, B., and Lewenza, S. (2015). DNA is an antimicrobial component of neutrophil extracellular traps. PLoS Pathog. 11:e1004593. doi: 10.1371/journal.ppat.1004593
Hartman, H., Abdulla, A., Awla, D., Lindkvist, B., Jeppsson, B., Thorlacius, H., et al. (2012). P-selectin mediates neutrophil rolling and recruitment in acute pancreatitis. Br. J. Surg. 99, 246–255. doi: 10.1002/bjs.7775
Inoue, S., Nakao, A., Kishimoto, W., Murakami, H., Itoh, K., Itoh, T., et al. (1995). Anti-neutrophil antibody attenuates the severity of acute lung injury in rats with experimental acute pancreatitis. Arch. Surg. 130, 93–98. doi: 10.1001/archsurg.1995.01430010095020
Jo, Y. J., Choi, H. S., Jun, D. W., Lee, O. Y., Kang, J. S., Park, I. G., et al. (2008). The effects of a new human leukocyte elastase inhibitor (recombinant guamerin) on cerulein-induced pancreatitis in rats. Int. Immunopharmacol. 8, 959–966. doi: 10.1016/j.intimp.2008.02.014
John, D. S., Aschenbach, J., Kruger, B., Sendler, M., Weiss, F. U., Mayerle, J., et al. (2019). Deficiency of cathepsin C ameliorates severity of acute pancreatitis by reduction of neutrophil elastase activation and cleavage of E-cadherin. J. Biol. Chem. 294, 697–707. doi: 10.1074/jbc.RA118.004376
Jones, D. H., Anderson, D. C., Burr, B. L., Rudloff, H. E., Smith, C. W., Krater, S. S., et al. (1988). Quantitation of intracellular Mac-1 (CD11b/CD18) pools in human neutrophils. J. Leukoc. Biol. 44, 535–544. doi: 10.1002/jlb.44.6.535
Juhas, S., Harris, N., Il'kova, G., Rehak, P., Zsila, F., Yurgenzon Kogan, F., et al. (2018). RX-207, a small molecule inhibitor of protein interaction with glycosaminoglycans (SMIGs), reduces experimentally induced inflammation and increases survival rate in cecal ligation and puncture (CLP)-induced sepsis. Inflammation 41, 307–314. doi: 10.1007/s10753-017-0688-0
Keck, T., Balcom JHT, Fernandez-del Castillo, C., Antoniu, B. A., and Warshaw, A. L. (2002). Matrix metalloproteinase-9 promotes neutrophil migration and alveolar capillary leakage in pancreatitis-associated lung injury in the rat. Gastroenterology 122, 188–201. doi: 10.1053/gast.2002.30348
Kinoshita, M., Ono, S., and Mochizuki, H. (2000). Neutrophils mediate acute lung injury in rabbits: role of neutrophil elastase. Eur. Surg. Res. 32, 337–346. doi: 10.1159/000052215
Knight, J. S., Subramanian, V., O'Dell, A. A., Yalavarthi, S., Zhao, W., Smith, C. K., et al. (2015). Peptidylarginine deiminase inhibition disrupts NET formation and protects against kidney, skin and vascular disease in lupus-prone MRL/lpr mice. Ann. Rheum. Dis. 74, 2199–2206. doi: 10.1136/annrheumdis-2014-205365
Korhonen, J. T., Dudeja, V., Dawra, R., Kubes, P., and Saluja, A. (2015). Neutrophil extracellular traps provide a grip on the enigmatic pathogenesis of acute pancreatitis. Gastroenterology 149, 1682–1685. doi: 10.1053/j.gastro.2015.10.027
Kyriakides, C., Jasleen, J., Wang, Y., Moore, F. D. Jr., Ashley, S. W., and Hechtman, H. B. (2001). Neutrophils, not complement, mediate the mortality of experimental hemorrhagic pancreatitis. Pancreas 22, 40–46. doi: 10.1097/00006676-200101000-00007
Lankisch, P. G., Apte, M., and Banks, P. A. (2015). Acute pancreatitis. Lancet 386, 85–96. doi: 10.1016/S0140-6736(14)60649-8
Leick, M., Azcutia, V., Newton, G., and Luscinskas, F. W. (2014). Leukocyte recruitment in inflammation: basic concepts and new mechanistic insights based on new models and microscopic imaging technologies. Cell Tissue Res. 355, 647–656. doi: 10.1007/s00441-014-1809-9
Leppkes, M., Maueroder, C., Hirth, S., Nowecki, S., Gunther, C., Billmeier, U., et al. (2016). Externalized decondensed neutrophil chromatin occludes pancreatic ducts and drives pancreatitis. Nat. Commun. 7:10973. doi: 10.1038/ncomms10973
Ley, K., Laudanna, C., Cybulsky, M. I., and Nourshargh, S. (2007). Getting to the site of inflammation: the leukocyte adhesion cascade updated. Nat. Rev. Immunol. 7, 678–689. doi: 10.1038/nri2156
Li, P., Li, M., Lindberg, M. R., Kennett, M. J., Xiong, N., and Wang, Y. (2010). PAD4 is essential for antibacterial innate immunity mediated by neutrophil extracellular traps. J. Exp. Med. 207, 1853–1862. doi: 10.1084/jem.20100239
Lieschke, G. J., Grail, D., Hodgson, G., Metcalf, D., Stanley, E., Cheers, C., et al. (1994). Mice lacking granulocyte colony-stimulating factor have chronic neutropenia, granulocyte and macrophage progenitor cell deficiency, and impaired neutrophil mobilization. Blood 84, 1737–1746. doi: 10.1182/blood.V84.6.1737.bloodjournal8461737
Lin, Q., Shen, J., Shen, L., Zhang, Z., and Fu, F. (2013). Increased plasma levels of heparin-binding protein in patients with acute respiratory distress syndrome. Crit. Care 17:R155. doi: 10.1186/cc12834
Linder, A., Christensson, B., Herwald, H., Bjorck, L., and Akesson, P. (2009). Heparin-binding protein: an early marker of circulatory failure in sepsis. Clin. Infect. Dis. 49, 1044–1050. doi: 10.1086/605563
Maas, S. L., Soehnlein, O., and Viola, J. R. (2018). Organ-specific mechanisms of transendothelial neutrophil migration in the lung, liver, kidney, and aorta. Front. Immunol. 9:2739. doi: 10.3389/fimmu.2018.02739
Madhi, R., Rahman, M., Taha, D., Linders, J., Merza, M., Wang, Y., et al. (2019). Platelet IP6K1 regulates neutrophil extracellular trap-microparticle complex formation in acute pancreatitis. JCI Insight e129270. doi: 10.1172/jci.insight.129270
Mayerle, J., Schnekenburger, J., Kruger, B., Kellermann, J., Ruthenburger, M., Weiss, F. U., et al. (2005). Extracellular cleavage of E-cadherin by leukocyte elastase during acute experimental pancreatitis in rats. Gastroenterology 129, 1251–1267. doi: 10.1053/j.gastro.2005.08.002
McDonald, B., Davis, R. P., Kim, S. J., Tse, M., Esmon, C. T., Kolaczkowska, E., et al. (2017). Platelets and neutrophil extracellular traps collaborate to promote intravascular coagulation during sepsis in mice. Blood 129, 1357–1367. doi: 10.1182/blood-2016-09-741298
Meng, W., Paunel-Gorgulu, A., Flohe, S., Hoffmann, A., Witte, I., MacKenzie, C., et al. (2012). Depletion of neutrophil extracellular traps in vivo results in hypersusceptibility to polymicrobial sepsis in mice. Crit. Care 16:R137. doi: 10.1186/cc11442
Merza, M., Hartman, H., Rahman, M., Hwaiz, R., Zhang, E., Renstrom, E., et al. (2015). Neutrophil extracellular traps induce trypsin activation, inflammation, and tissue damage in mice with severe acute pancreatitis. Gastroenterology 149, 1920–1931.e8. doi: 10.1053/j.gastro.2015.08.026
Merza, M., Wetterholm, E., Zhang, S., Regner, S., and Thorlacius, H. (2013). Inhibition of geranylgeranyltransferase attenuates neutrophil accumulation and tissue injury in severe acute pancreatitis. J. Leukoc. Biol. 94, 493–502. doi: 10.1189/jlb.1112546
Mestas, J., and Hughes, C. C. (2004). Of mice and not men: differences between mouse and human immunology. J. Immunol. 172, 2731–2738. doi: 10.4049/jimmunol.172.5.2731
Metzler, K. D., Goosmann, C., Lubojemska, A., Zychlinsky, A., and Papayannopoulos, V. (2014). A myeloperoxidase-containing complex regulates neutrophil elastase release and actin dynamics during NETosis. Cell Rep. 8, 883–896. doi: 10.1016/j.celrep.2014.06.044
Murthy, P., Singhi, A. D., Ross, M. A., Loughran, P., Paragomi, P., Papachristou, G. I., et al. (2019). Enhanced neutrophil extracellular trap formation in acute pancreatitis contributes to disease severity and is reduced by chloroquine. Front. Immunol. 10:28. doi: 10.3389/fimmu.2019.00028
Novovic, S., Andersen, A. M., Nord, M., Astrand, M., Ottosson, T., Jorgensen, L. N., et al. (2013). Activity of neutrophil elastase reflects the progression of acute pancreatitis. Scand. J. Clin. Lab. Invest. 73, 485–493. doi: 10.3109/00365513.2013.807935
Nunes, Q. M., Mournetas, V., Lane, B., Sutton, R., Fernig, D. G., and Vasieva, O. (2013). The heparin-binding protein interactome in pancreatic diseases. Pancreatology 13, 598–604. doi: 10.1016/j.pan.2013.08.004
Papayannopoulos, V., Metzler, K. D., Hakkim, A., and Zychlinsky, A. (2010). Neutrophil elastase and myeloperoxidase regulate the formation of neutrophil extracellular traps. J. Cell Biol. 191, 677–691. doi: 10.1083/jcb.201006052
Pastor, C. M., Rubbia-Brandt, L., Hadengue, A., Jordan, M., Morel, P., and Frossard, J. L. (2003). Role of macrophage inflammatory peptide-2 in cerulein-induced acute pancreatitis and pancreatitis-associated lung injury. Lab. Invest. 83, 471–478. doi: 10.1097/01.LAB.0000063928.91314.9F
Paulino, E. C., de Souza, L. J., Molan, N. A., Machado, M. C., and Jancar, S. (2007). Neutrophils from acute pancreatitis patients cause more severe in vitro endothelial damage compared with neutrophils from healthy donors and are differently regulated by endothelins. Pancreas 35, 37–41. doi: 10.1097/MPA.0b013e31805c177b
Phillipson, M., Heit, B., Colarusso, P., Liu, L., Ballantyne, C. M., and Kubes, P. (2006). Intraluminal crawling of neutrophils to emigration sites: a molecularly distinct process from adhesion in the recruitment cascade. J. Exp. Med. 203, 2569–75. doi: 10.1084/jem.20060925
Phillipson, M., and Kubes, P. (2011). The neutrophil in vascular inflammation. Nat. Med. 17, 1381–1390. doi: 10.1038/nm.2514
Pillay, J., den Braber, I., Vrisekoop, N., Kwast, L. M., de Boer, R. J., Borghans, J. A., et al. (2010). In vivo labeling with 2H2O reveals a human neutrophil lifespan of 5.4 days. Blood 116, 625–627. doi: 10.1182/blood-2010-01-259028
Ryschich, E., Kerkadze, V., Deduchovas, O., Salnikova, O., Parseliunas, A., Märten, A., et al. (2009). Intracapillary leucocyte accumulation as a novel antihaemorrhagic mechanism in acute pancreatitis in mice. Gut 58, 1508–1516. doi: 10.1136/gut.2008.170001
Sadik, C. D., Kim, N. D., and Luster, A. D. (2011). Neutrophils cascading their way to inflammation. Trends Immunol. 32, 452–460. doi: 10.1016/j.it.2011.06.008
Sandoval, D., Gukovskaya, A., Reavey, P., Gukovsky, S., Sisk, A., Braquet, P., et al. (1996). The role of neutrophils and platelet-activating factor in mediating experimental pancreatitis. Gastroenterology 111, 1081–1091. doi: 10.1016/S0016-5085(96)70077-X
Sendler, M., Dummer, A., Weiss, F. U., Kruger, B., Wartmann, T., Scharffetter-Kochanek, K., et al. (2013). Tumour necrosis factor alpha secretion induces protease activation and acinar cell necrosis in acute experimental pancreatitis in mice. Gut 62, 430–439. doi: 10.1136/gutjnl-2011-300771
Sendler, M., van den Brandt, C., Glaubitz, J., Wilden, A., Golchert, J., Weiss, F. U., et al. (2020). NLRP3 inflammasome regulates development of systemic inflammatory response and compensatory anti-inflammatory response syndromes in mice with acute pancreatitis. Gastroenterology 158, 253–269.e14. doi: 10.1053/j.gastro.2019.09.040
Sharif, R., Dawra, R., Wasiluk, K., Phillips, P., Dudeja, V., Kurt-Jones, E., et al. (2009). Impact of toll-like receptor 4 on the severity of acute pancreatitis and pancreatitis-associated lung injury in mice. Gut 58, 813–819. doi: 10.1136/gut.2008.170423
Soehnlein, O., Zernecke, A., Eriksson, E. E., Rothfuchs, A. G., Pham, C. T., Herwald, H., et al. (2008). Neutrophil secretion products pave the way for inflammatory monocytes. Blood 112, 1461–1471. doi: 10.1182/blood-2008-02-139634
Sollberger, G., Tilley, D. O., and Zychlinsky, A. (2018). Neutrophil extracellular traps: the biology of chromatin externalization. Dev. Cell. 44, 542–553. doi: 10.1016/j.devcel.2018.01.019
Sorensen, O. E., and Borregaard, N. (2016). Neutrophil extracellular traps - the dark side of neutrophils. J. Clin. Invest. 126, 1612–1620. doi: 10.1172/JCI84538
Stoiber, W., Obermayer, A., Steinbacher, P., and Krautgartner, W. D. (2015). The role of reactive oxygen species (ROS) in the formation of extracellular traps (ETs) in humans. Biomolecules 5, 702–723. doi: 10.3390/biom5020702
Summers, C., Rankin, S. M., Condliffe, A. M., Singh, N., Peters, A. M., and Chilvers, E. R. (2010). Neutrophil kinetics in health and disease. Trends Immunol. 31, 318–324. doi: 10.1016/j.it.2010.05.006
Tak, T., Tesselaar, K., Pillay, J., Borghans, J. A., and Koenderman, L. (2013). What's your age again? Determination of human neutrophil half-lives revisited. J. Leukoc. Biol. 94, 595–601. doi: 10.1189/jlb.1112571
Tyden, J., Herwald, H., Hultin, M., Wallden, J., and Johansson, J. (2017). Heparin-binding protein as a biomarker of acute kidney injury in critical illness. Acta Anaesthesiol. Scand. 61, 797–803. doi: 10.1111/aas.12913
von Dobschuetz, E., Hoffmann, T., and Messmer, K. (1999). Inhibition of neutrophil proteinases by recombinant serpin Lex032 reduces capillary no-reflow in ischemia/reperfusion-induced acute pancreatitis. J. Pharmacol. Exp. Ther. 290, 782–788.
Wang, J., Hossain, M., Thanabalasuriar, A., Gunzer, M., Meininger, C., and Kubes, P. (2017). Visualizing the function and fate of neutrophils in sterile injury and repair. Science 358, 111–116. doi: 10.1126/science.aam9690
Wang, S., and Wang, Y. (2013). Peptidylarginine deiminases in citrullination, gene regulation, health and pathogenesis. Biochim. Biophys. Acta 1829, 1126–1135. doi: 10.1016/j.bbagrm.2013.07.003
Wetterholm, E., Linders, J., Merza, M., Regner, S., and Thorlacius, H. (2016). Platelet-derived CXCL4 regulates neutrophil infiltration and tissue damage in severe acute pancreatitis. Transl. Res. 176, 105–118. doi: 10.1016/j.trsl.2016.04.006
Willemer, S., Feddersen, C. O., Karges, W., and Adler, G. (1991). Lung injury in acute experimental pancreatitis in rats. I. Morphological studies. Int. J. Pancreatol. 8, 305–321.
Witalison, E. E., Cui, X., Causey, C. P., Thompson, P. R., and Hofseth, L. J. (2015). Molecular targeting of protein arginine deiminases to suppress colitis and prevent colon cancer. Oncotarget 6, 36053–36062. doi: 10.18632/oncotarget.5937
Woodfin, A., Voisin, M. B., Beyrau, M., Colom, B., Caille, D., Diapouli, F. M., et al. (2011). The junctional adhesion molecule JAM-C regulates polarized transendothelial migration of neutrophils in vivo. Nat. Immunol. 12, 761–769. doi: 10.1038/ni.2062
Wu, D., Zeng, Y., Fan, Y., Wu, J., Mulatibieke, T., Ni, J., et al. (2016). Reverse-migrated neutrophils regulated by JAM-C are involved in acute pancreatitis-associated lung injury. Sci. Rep. 6:20545. doi: 10.1038/srep20545
Zhang, H., Neuhofer, P., Song, L., Rabe, B., Lesina, M., Kurkowski, M. U., et al. (2013). IL-6 trans-signaling promotes pancreatitis-associated lung injury and lethality. J. Clin. Invest. 123, 1019–1031. doi: 10.1172/JCI64931
Keywords: neutrophil, pancreatitis, neutrophil granules, NETs, inflammation
Citation: Wan J, Ren Y, Yang X, Li X, Xia L and Lu N (2021) The Role of Neutrophils and Neutrophil Extracellular Traps in Acute Pancreatitis. Front. Cell Dev. Biol. 8:565758. doi: 10.3389/fcell.2020.565758
Received: 29 June 2020; Accepted: 22 December 2020;
Published: 21 January 2021.
Edited by:
Roland Wohlgemuth, Lodz University of Technology, PolandReviewed by:
Julia Mayerle, LMU Munich University Hospital, GermanyHalesha Dhurvigere Basavarajappa, Beckman Research Institute, City of Hope, United States
Copyright © 2021 Wan, Ren, Yang, Li, Xia and Lu. This is an open-access article distributed under the terms of the Creative Commons Attribution License (CC BY). The use, distribution or reproduction in other forums is permitted, provided the original author(s) and the copyright owner(s) are credited and that the original publication in this journal is cited, in accordance with accepted academic practice. No use, distribution or reproduction is permitted which does not comply with these terms.
*Correspondence: Liang Xia, eGlhbGlhbmc3OSYjeDAwMDQwOzE2My5jb20=
†These authors have contributed equally to this work and share first authorship
‡These authors have contributed equally to this work