Expression of Concern: Resveratrol improves Bnip3-related mitophagy and attenuates high-fat-induced endothelial dysfunction
- 1Department of Cardiology, Foshan Hospital Affiliated with Southern Medical University (The Second People’s Hospital of Foshan), Foshan, China
- 2Department of Critical Care Medicine, Nanfang Hospital, Southern Medical University, Guangzhou, China
- 3Department of Oncology Hematology, Foshan Hospital Affiliated with Southern Medical University (The Second People’s Hospital of Foshan), Foshan, China
Statin treatment reduces cardiovascular risk. However, individuals with well-controlled low-density lipoprotein (LDL) levels may remain at increased risk owing to persistent high triglycerides and low high-density lipoprotein cholesterol. Because resveratrol promotes glucose metabolism and mitigates cardiovascular disorders, we explored its mechanism of protective action on high-fat-induced endothelial dysfunction. Human umbilical venous endothelial cells were treated with oxidized LDL (ox-LDL) in vitro. Endothelial function, cell survival, proliferation, migration, and oxidative stress were analyzed through western blots, quantitative polymerase chain reaction, ELISA, and immunofluorescence. ox-LDL induced endothelial cell apoptosis, proliferation arrest, and mobilization inhibition, all of which resveratrol reduced. ox-LDL suppressed the activities of mitochondrial respiration complex I and III and reduced levels of intracellular antioxidative enzymes, resulting in reactive oxygen species overproduction and mitochondrial dysfunction. Resveratrol treatment upregulated Bnip3-related mitophagy and prevented ox-LDL-mediated mitochondrial respiration complexes inactivation, sustaining mitochondrial membrane potential and favoring endothelial cell survival. We found that resveratrol enhanced Bnip3 transcription through hypoxia-inducible factor 1 (HIF1) and 5′ AMP-activated protein kinase (AMPK). Inhibition of AMPK and HIF1 abolished resveratrol-mediated protection of mitochondrial redox balance and endothelial viability. Together, these data demonstrate resveratrol reduces hyperlipemia-related endothelial damage by preserving mitochondrial homeostasis.
Introduction
Hyperlipemia has been established as an independent risk factor for the development of atherosclerotic cardiovascular disease (Zeron and Albuquerque, 2019). Although statins have been used in clinical studies to reduce hyperlipemia, therapeutic resistance occurs in patients (Luirink et al., 2019), leading to additional studies on lipid-suppressing approaches for intervention. Although statins lower low-density lipoprotein (LDL) levels, they have little influence on high-density lipoprotein cholesterol (HDL-C) and triglycerides (Trieb et al., 2019). A remaining challenge, therefore, is to develop new drugs or compounds for controlling blood HDL-C levels. Resveratrol is a stilbenoid, which is produced by several plants in response to injury or when plants are under pathogen attack (Chen et al., 2019a; Wang et al., 2019). Many foods also contain resveratrol, including grapes, blueberries, raspberries, mulberries, and peanuts (Kim et al., 2019), and its protective actions include antioxidative and anti-inflammatory properties (Luo et al., 2019). At nutritionally relevant concentrations, resveratrol increases antioxidative enzyme gene expression and inhibits transcription of proinflammatory cytokines (Lejri et al., 2019; Rao et al., 2019). Previous studies have shown resveratrol promotes glucose metabolism as an adjunctive therapy for the management of diabetes-associated complications (Dludla et al., 2020). Additionally, resveratrol accelerates white adipocyte tissue browning (Li et al., 2020b) and fatty acid oxidation (Zhang et al., 2019d). However, few studies have explored the protective roles of resveratrol in hyperlipemia.
For the past few decades, most studies have focused on the roles of hyperlipemia in the heart, kidneys, and pancreas. Compared with these organs, endothelium is more vulnerable to hyperlipemia-triggered pathological injuries, including oxidative stress, metabolic disorder, cell senescence, and fibrosis (Wang et al., 2020a; Zhou and Toan, 2020). Additionally, owing to direct contact with blood, endothelium responds to blood composition alterations (Heusch, 2019; Wang et al., 2020b). Accordingly, hyperlipemia-mediated damage is likely to be seen in endothelium (Korbel et al., 2018). In addition, LDL is primarily degraded by endothelium through the LDL receptor, which is expressed on the surface of endothelium. Impaired endothelial function is associated with an increase in the blood LDL (Su et al., 2019). Based on this information, endothelium is an ideal barrier to regulate hyperlipemia. Several drugs targeting endothelium have been developed or investigated to attenuate hyperlipemia-related endothelial damage under metabolic disorder. Sitagliptin, a glucagon-like peptide analog, promotes upregulated vascular endothelial growth factor (VEGF) and enhances angiogenesis in diabetic rats (Khodeer et al., 2019). In a type-2 diabetes model, Empagliflozin inhibits oxidative stress and promotes endothelial cell migration and regeneration (Zhou et al., 2018c). Interestingly, resveratrol has been reported to inhibit hyperglycemia-mediated inflammatory “metabolic memory” in human retinal vascular endothelial cells (Zhang et al., 2015). However, no data are available to confirm whether resveratrol protects endothelial cells against high-fat-induced injuries.
At the sub-cellular level, hyperlipemia-induced injuries are usually caused by oxidative stress through free fatty acid (FFA) metabolism (Cesar et al., 2018). Compared with glucose, FFA metabolism consumes more oxygen, which is correlated with elevated reactive oxygen species (ROS) (Lee et al., 2019). In addition, hyperlipemia is always followed by metabolic reprogramming, which primarily uses FFAs rather than glucose as the energy substrates (Kowaltowski, 2019). Decreased glucose metabolism is accompanied with a decline in the production of antioxidative factors (Hysi et al., 2019). These two effects work together to augment intracellular oxidative stress, leading to endothelial cell dysfunction, including proliferation arrest, angiogenesis delay, mobilization inhibition, and apoptosis activation (Huang et al., 2018). A total of 85% intracellular ROS are generated at dysfunctional mitochondria through the tricarboxylic acid cycle and oxidative phosphorylation because of decreased expression or activity of mitochondrial respiration complexes (Silverblatt et al., 2019). Two strategies ameliorate mitochondrial ROS production: one is mediated through upregulation of endothelial antioxidative capacity, and the other is achieved through acceleration of dysfunction mitochondria clearance (Daiber and Chlopicki, 2020). Mitophagy, a selective form of autophagy, selectively targets damaged mitochondria and sustains mitochondrial homeostasis (Zhou et al., 2018d). The antioxidative property of mitophagy has been reported to play a role in the setting of diabetes, fatty liver disease, hypertension, and cardiac ischemia-reperfusion injury (Shi et al., 2018; Zhou et al., 2018a, 2019). Therefore, in this study, we investigated whether the ROS-reducing effect of resveratrol on high-fat-treated endothelial cells is mediated by mitophagy.
Materials and Methods
Cell Treatment and Transfection
HUVECs were cultured at 37°C in a humidified 5% CO2 environment (Zhang et al., 2019a). Culture medium was refreshed every 2–3 days. Cells were passed using trypsin-EDTA (Sigma, Steinheim, Germany) at 90–100%. HUVECs were used up to passage five. HUVEC stock solutions up to passage two were stored at 180°C in Dulbecco’s modified Eagle medium (DMEM) GlutaMAX containing 20% FBS and 10% DMSO (Sigma) (Zhao et al., 2019).
HUVECs were transiently transfected in triplicate with siRNA using Lipofectamine 2000 (Life Technologies, Frederick, MD, United States) according to the manufacturer’s instructions. Briefly, 8 mol/L siRNA and Lipofectamine transfection reagent (twice the amount of the total DNA quantity) were prepared separately in serum-free medium (Opti-MEM; Thermo Fisher Scientific, Waltham, MA, United States). Lipofectamine solution was added dropwise on DNA solution, and the mixture was incubated for 30 min at 37°C before gently added in each cell culture dish (Zhu et al., 2019). After 4 h, transfection medium was replaced with serum-free medium. After overnight recovery, cells were incubated with ox-LDL for the indicated time (Zarfati et al., 2019). Transient transfections were performed in triplicate wells in HUVECs and repeated n times.
Real-Time Quantitative Polymerase Chain Reaction (RT-qPCR)
Total RNAs were extracted with TRIzol reagent (Strasbourg, France) according to the manufacturer’s instructions, and integrity was assayed by gel agarose electrophoresis. First-strand cDNA was synthesized with 1 μg of total RNA using iScriptTM cDNA Synthesis Kit (Bio-Rad, Hercules, CA, United States) according to the manufacturer’s instructions in a total volume of 20 μL (Zhang et al., 2019c). Transcript cDNA levels were analyzed in duplicate by RT-PCR performed with the CFX96 RT-PCR system (Bio-Rad) using SsoAdvancedTM Universal SYBR® Green Supermix (Bio-Rad), of which three contained 500 10–9 mol/L specific primers (Eurofins MWG Operon, Online Table I). Serial dilutions of pooled cDNA were used in each experiment to assess PCR efficiency. Gene expression was quantified relative to the geometric means of the housekeeping gene expression amplified in the same tube of investigated genes, and the Δ Δ Ct method was used to determine gene expression (Zhang et al., 2019b).
ROS Measurement
HUVECs were suspended in DMEM and exposed to ox-LDL after transfection with Bnip3 siRNA. Total ROS production was measured by immunofluorescence with a 2′,7′-dichlorofluorescin diacetate Cellular ROS Detection Assay Kit (ab113851; Abcam, Cambridge, MA, United States) according to the manufacturer’s instructions (Quispe et al., 2019). Mitochondria-derived ROS levels in cardiomyocytes were measured using a mitochondrial superoxide indicator (MitoSOXTM Red, M36008; Thermo Fisher Scientific) through immunofluorescence as previously described (Knani et al., 2019).
Cell Survival Assay
A total of 50,000 cells/well were plated onto a 12-well plate. After 22 h, cells were replenished with fresh growth medium (Wolint et al., 2019), then 2 h later, cells were transfected with siRNA. Twenty-four hours after transfection, cell viability was measured through MTT assay as previously described (Trindade et al., 2019).
Mitochondrial Isolation
Mitochondria were isolated from cells, digested with trypsin, homogenized with a glass/Teflon Potter Elvejhem homogenizer (Thomas Scientific, Swedesboro, NJ, United States), and then centrifuged at 800 × g for 10 min at 4°C. The supernatant was centrifuged at 8,000 × g for 10 min at 4°C, and the remaining supernatant was discarded. The pellet containing mitochondria was washed and centrifuged at 8,000 × g for 10 min at 4°C before resuspension (Zakeri et al., 2019). Mitochondrial protein concentration was determined by colorimetry using Bio-Rad protein assay dye reagent (500-0006; Bio-Rad).
Cellular Respiration Assays
XPF extracellular flux analyzer (Seahorse Biosciences) was used for real = time analysis of the oxygen consumption rate (OCR) of intact cells according to the manufacturer’s instructions (van Duinen et al., 2019). Briefly, macrophages were seeded at 5 × 105 cells/well in. After incubation with siRNA, mitochondrial respiration was detected according to the manufacturer’s instructions (Vijayan et al., 2019). Results were normalized to the actual cell count immediately after OCR recordings (Zhong et al., 2019).
Immunostaining and Fluorescence Microscopy
Cells were quickly rinsed with PBS and fixed with 4% paraformaldehyde at room temperature for 15 min. Paraformaldehyde was then neutralized with NH4Cl for 15 min before cells were permeabilized with 0.5% Triton X-100 for 5 min, washed three times with PBS, rinsed with PBS 5% BSA for 40 min, then incubated overnight at 4°C with primary antibodies, followed by washes and incubation with Alexa Fluor® 633 goat anti-rabbit IgG (H + L) (Molecular Probes, A21071; 1:1,000) for 1 h at room temperature (Xiao et al., 2019). Next, cells were rinsed with PBS, incubated with 5 μg/mL Hoechst 33342 (Sigma) for 5 min, washed again with PBS, and mounted with 15 μL Mowiol® 4–88 (Calbiochem, San Diego, CA, United States). Cells were examined with a confocal microscope (Leica TCS-SP8 gated STED) (Yan et al., 2018). Alexa Fluor 633 was excited at 633 nm with a white light laser, and emission measured at 640–800 nm with a hybrid detector. Hoechst 33342 was excited by a 405-nm diode, and emission measured at 420–460 nm.
Statistical Analysis
All data are shown as the mean ± standard error of the mean (SEM). A 2-sided, unpaired Student’s T-test between the groups for normal distributed variables and Mann-Whitney test for non-normal distributed variables were used for statistical testing. Differences across three or more groups were tested with ANOVA using Turkey’s multiple-comparison test or multiple Student’s T-test with Holm-Sidak corrections for multiple comparison. A p–value less than 0.05 was considered significant.
Results
Resveratrol Ameliorates Oxidized Low-Density Lipoprotein (ox-LDL)-Mediated Endothelial Dysfunction
To understand the role of resveratrol in the dysfunction of high-fat-mediated endothelial cells, human umbilical venous endothelial cells (HUVECs) were cultured with ox-LDL in the presence or absence of resveratrol. Then, endothelial cell viability, proliferation, and mobilization were determined. Figure 1A shows that compared with the control group, MTT assay demonstrated ox-LDL reduced endothelial viability, whereas this alteration was corrected by resveratrol. Immunofluorescence assay for caspase-3 also showed that the expression of caspase-3 was rapidly increased or drastically inhibited by ox-LDL or resveratrol, respectively (Figures 1B,C). Therefore, these results indicate that resveratrol sustains endothelial viability in the setting of hyperlipemic stress. RNA analysis of cyclin D1 and cyclin E also illustrated that endothelial cell proliferation rate was impaired by ox-LDL (Figures 1D,E). Interestingly, resveratrol treatment upregulated the transcription of cyclin D1 and cyclin E (Figures 1D,E), suggesting that resveratrol attenuates high-fat-induced endothelial growth arrest. With respect to endothelial mobilization, Transwell assay demonstrated that the number of migrated endothelial cells was downregulated after exposure to ox-LDL (Figure 1F). Resveratrol pretreatment maintained endothelial cell mobilization through increasing the number of migrated cells. Thus, these data indicate that endothelial mobility is preserved by resveratrol in the setting of hyperlipemia.
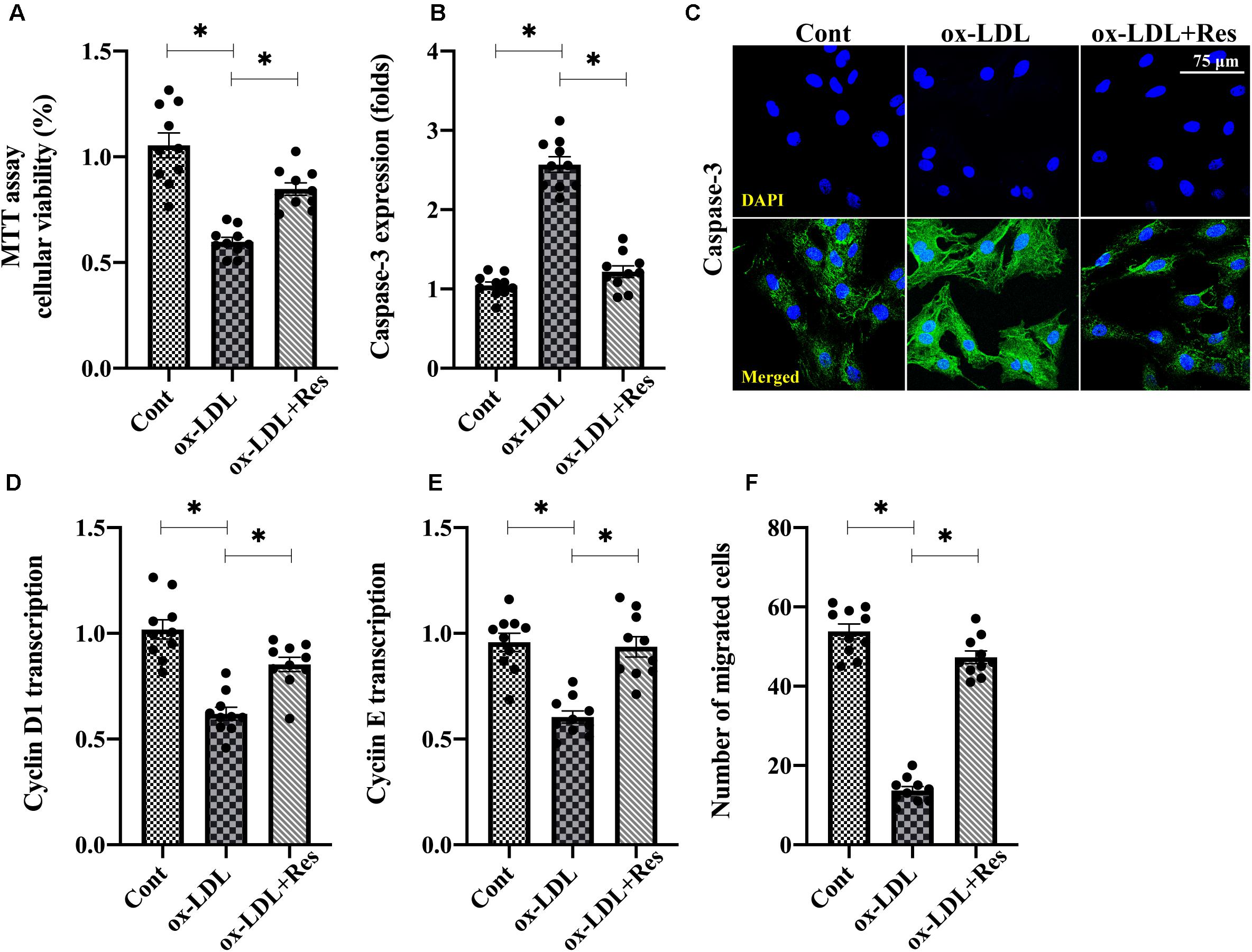
Figure 1. Resveratrol ameliorates ox-LDL-mediated endothelial dysfunction. (A) HUVECs were incubated with ox-LDL in the presence or absence of resveratrol. Cell viability was determined through MTT assay. (B,C) Immunofluorescence staining was used to observe the alterations of caspase-3 in endothelial cells under ox-LDL treatment. (D,E) RNA was collected from HUVECs after treatment with ox-LDL in the presence or absence of resveratrol. Then, transcription of cyclin D1 and cyclin (E) were measured to reflect endothelial cell proliferation. (F) Transwell assay was used to detect endothelial cell migratory response. The number of migrated endothelial cells was recorded. *p < 0.05.
Oxidative Stress Is Inhibited by Resveratrol in ox-LDL-Treated Endothelial Cells
To explain the protective effects underlying resveratrol-sustained endothelial viability, proliferation and mobilization, endothelial redox status, mitochondrial oxidative stress was analyzed. First, the levels of mitochondrial ROS were elevated in response to ox-LDL treatment (Figures 2A,B); resveratrol ameliorated this effect, confirming its antioxidative property. Antioxidative factors such as GSH, SOD, and GPX neutralized upregulated ROS. However, GSH, SOD, and GPX transcriptions were drastically downregulated in ox-LDL-treated endothelial cells (Figures 2C–E), although resveratrol could restore their expressions.
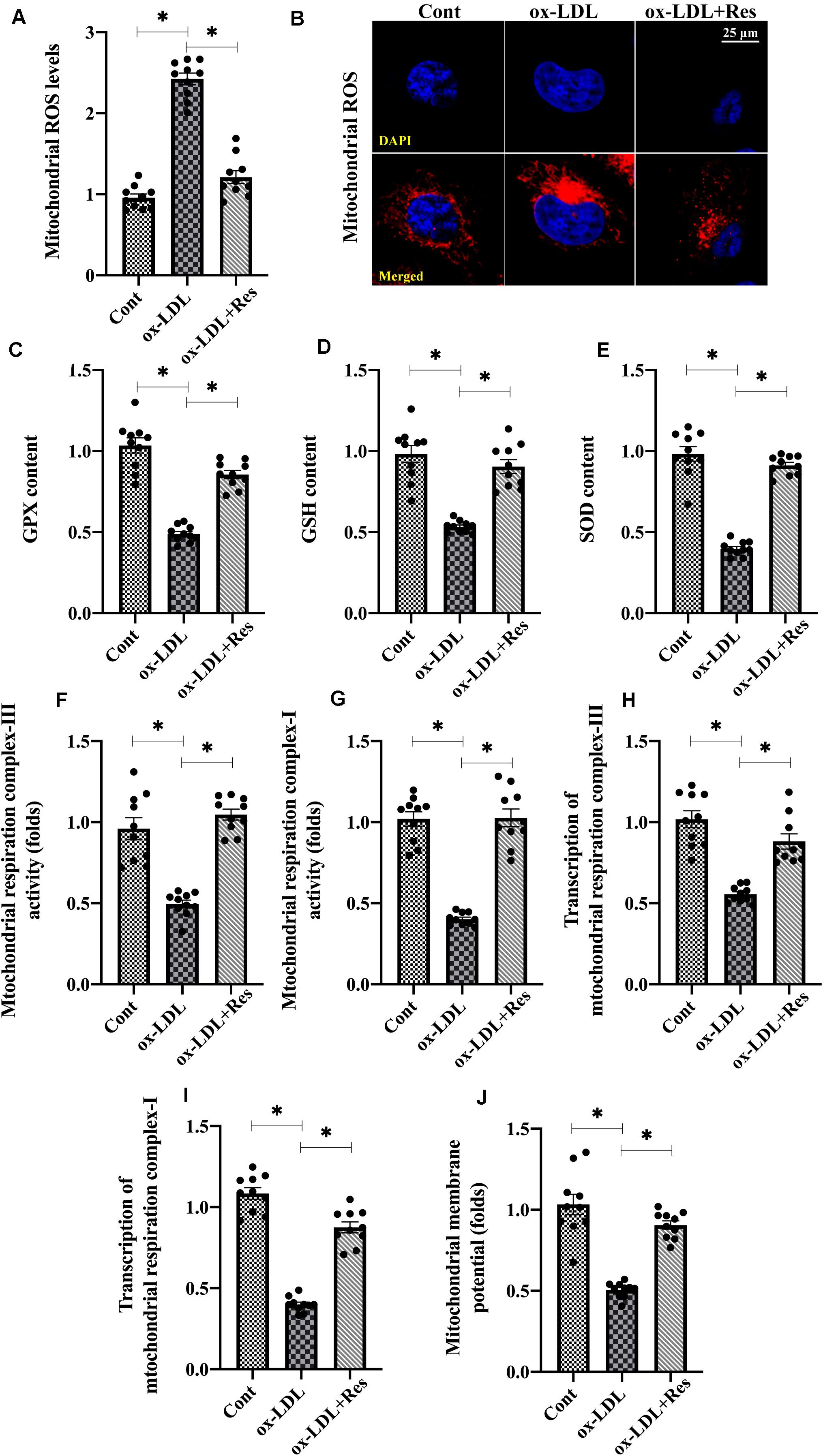
Figure 2. Oxidative stress is inhibited by resveratrol in ox-LDL-treated endothelial cells. (A,B) Immunofluorescence assay for mitochondrial ROS in endothelial cells treated with ox-LDL in the presence or absence of resveratrol. (C–E). ELISA was used to evaluate the activities of GSH, SOD, and GPX in endothelial cells. (F,G). The activities of mitochondrial respiration complex I and III were measured through ELISA. (H,I) qPCR was used to analyze the transcription of mitochondrial respiration complex I and III. (J) JC-1 probe was used to stain mitochondrial membrane potential. The red-to-green fluorescence intensity was used to quality mitochondrial membrane potential. *p < 0.05.
Mitochondrial ROS are primarily generated by mitochondrial respiration complexes, especially mitochondrial respiration complex I and III (DeLeon-Pennell et al., 2018). Using ELISA, we found that the mitochondrial respiration complex I and III activities decreased with ox-LDL treatment, whereas this phenotypic alteration could be normalized with resveratrol (Figures 2F,G). Mitochondrial respiration complex I and III transcriptions were also downregulated or upregulated by ox-LDL or resveratrol, respectively (Figures 2H,I). Owing to mitochondrial ROS overload, mitochondrial function, which is evaluated by the mitochondrial membrane potential, was also blunted in ox-LDL-treated endothelial cells (Figure 2J). However, resveratrol pretreatment stabilized mitochondrial membrane potential in the presence of ox-LDL (Figure 2J). Together, our results indicate that mitochondrial oxidative stress, which is triggered by ox-LDL, could be repressed by resveratrol in endothelial cells.
Resveratrol Activates Bnip3-Related Mitophagy in Endothelial Cells in the Presence of ox-LDL
As a compensatory repairing system, mitophagy selectively guides dysfunction to be degraded by lysosome contributing to intracellular redox balance. Therefore, we investigated whether resveratrol regulated endothelial mitochondrial oxidative stress through mitophagy. Mitophagy is activated by several adaptors, including Parkin and BCL2/adenovirus E1B 19-kDa protein-interacting protein 3 (Bnip3) (Zhou et al., 2018b). Interestingly, ox-LDL treatment repressed Parkin and Bnip3 expression in endothelial cells (Figures 3A,B). Resveratrol treatment reversed Bnip3 expression but had a slight effect on Parkin expression in ox-LDL-treated endothelial cells (Figures 3A,B), suggesting that endothelial mitophagy could be activated by resveratrol in a manner dependent on Bnip3. To demonstrate the promotive action by resveratrol on mitophagy, mt-Kemia probe, an acid mitochondria indicator, was added into endothelial cell medium. Under normal conditions, mitophagy is moderate and, thus, parts of acid mitochondria could be detected (Figures 3C,D). After exposure to ox-LDL, the acid mitochondrial number was reduced, and this trend could be corrected by resveratrol (Figures 3C,D). In addition, RNA analysis demonstrated that ATG5 and Beclin1, the mitophagy markers, were transcriptionally inhibited by ox-LDL (Figures 3E,F). However, resveratrol treatment upregulated the ATG5 and Beclin1 RNA expression (Figures 3E,F), reconfirming a contributory action underlying resveratrol on endothelial mitophagy in the presence of ox-LDL.
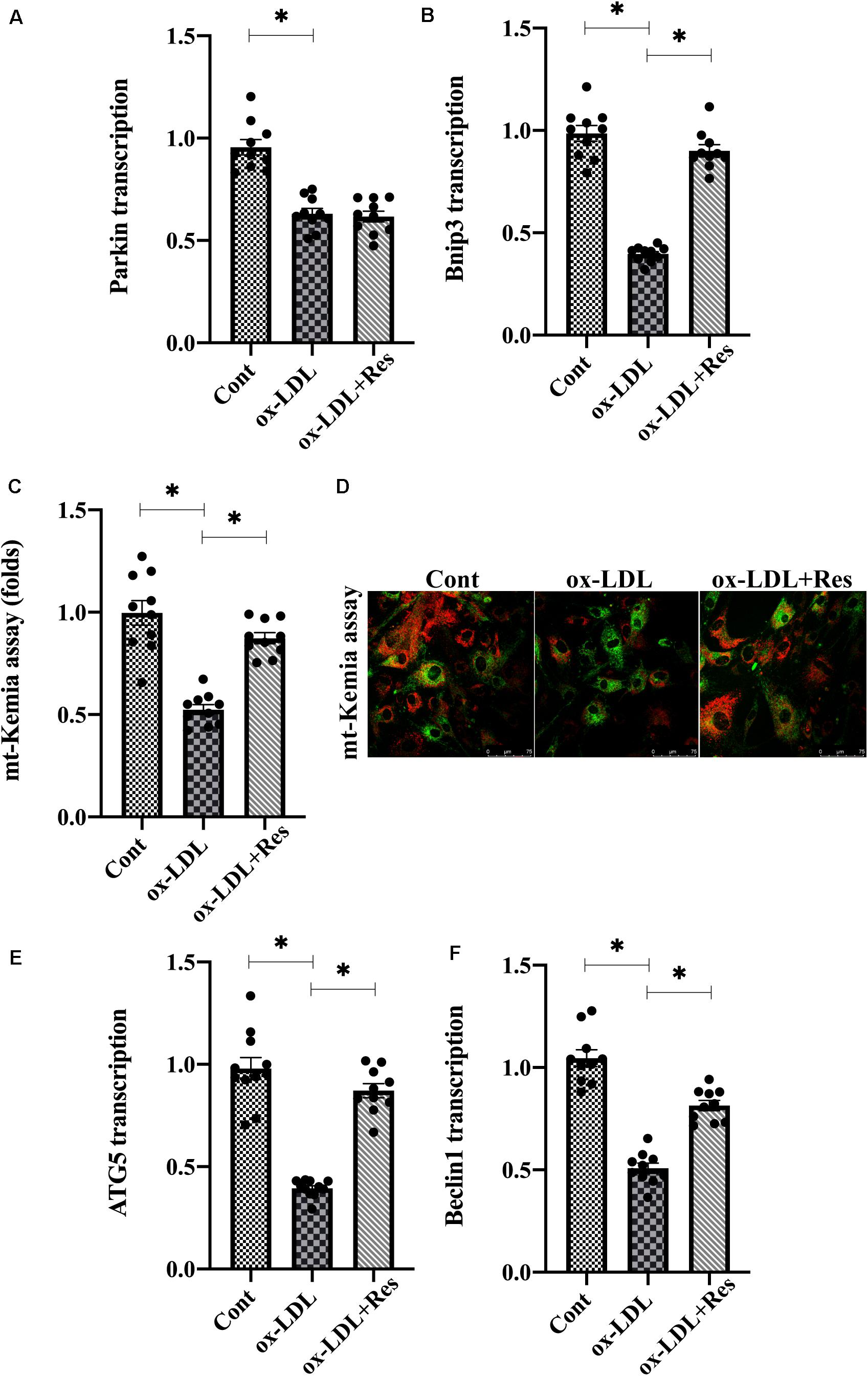
Figure 3. Resveratrol activates Bnip3-related mitophagy in endothelial cells in the presence of ox-LDL. (A,B) After treatment with ox-LDL, RNA in endothelial cells was collected and Parkin and Bnip3 transcription was measured. (C,D) Endothelial mitophagy was detected through mt-Kemia. The acid mitochondria number was recorded to reflect the activity of mitophagy. (E,F) ATG5 and Beclin1 transcription was determined through qPCR. Endothelial cells were treated with ox-LDL in the presence or absence of resveratrol. *p < 0.05.
Inhibition of Bnip3-Related Mitophagy Suppresses Resveratrol-Induced Protection on Mitochondrial Homeostasis
To understand whether Bnip3-related mitophagy is required for resveratrol-induced mitochondrial protection, we silenced Bnip3 in resveratrol-treated endothelial cells. Then, mitochondrial function and redox biology were analyzed. Figures 4A,B shows that compared with the control group, mitochondrial ROS production was elevated by ox-LDL. Although resveratrol suppressed mitochondrial ROS generation, this effect was abolished by Bnip3 siRNA (Figures 4A,B). The levels of antioxidative factors, such as GSH, SOD, and GPX, were downregulated by ox-LDL and reversed to near-normal levels after resveratrol pretreatment (Figures 4A–E). Interestingly, with the loss of Bnip3-related mitophagy, resveratrol failed to upregulate intracellular antioxidative factors in ox-LDL-treated endothelial cells (Figures 4C–E). In addition, ox-LDL repressed mitochondrial respiration complex I and III activities. Resveratrol corrected this alteration in a manner dependent on Bnip3 (Figures 4F,G), suggesting that Bnip3-mediated mitophagy may promote mitochondrial respiration. Last, TUNEL staining was used to confirm whether Bnip3-related mitophagy was necessary for endothelial cell survival. Figures 4H,I shows that compared with the control group, ox-LDL increased the ratio of TUNEL-positive endothelial cells. Although resveratrol could inhibit ox-LDL-mediated endothelial cell death, this protective action was undetectable in endothelial cells transfected with Bnip3 siRNA (Figures 4H,I). Together, our results indicate that Bnip3-related mitophagy is required for resveratrol-mediated mitochondrial protection in ox-LDL-treated endothelial cells.
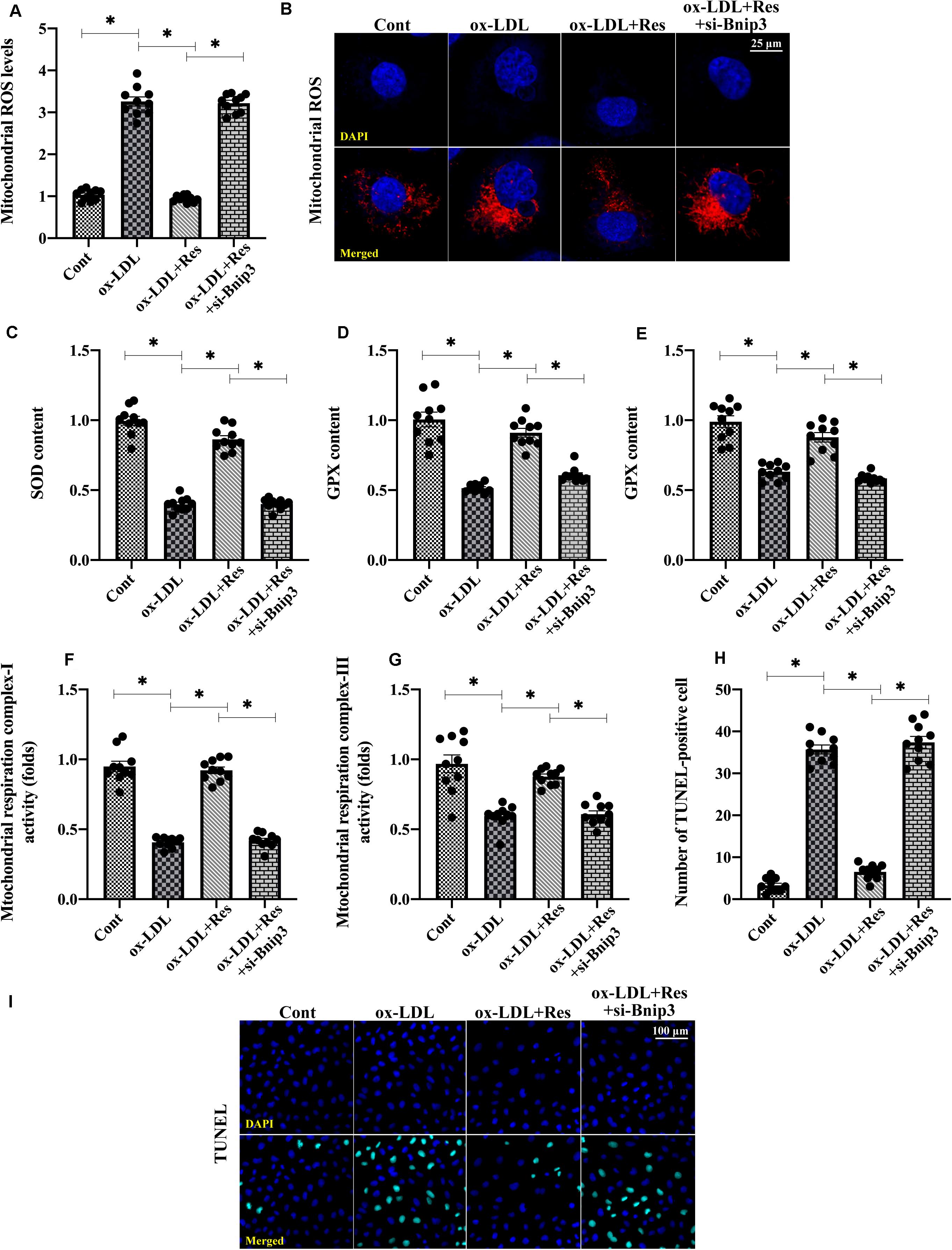
Figure 4. Inhibition of Bnip3-related mitophagy suppresses resveratrol-induced protection on mitochondrial homeostasis. (A,B) Bnip3 siRNA was transfected into endothelial cells before treated with resveratrol. Then, mitochondrial ROS levels were measured through immunofluorescence. (C–E) ELISA was used to evaluate GSH, SOD, and GPX activity in endothelial cells. (F,G) Mitochondrial respiration complex I and III activities were measured through ELISA. (H,I) TUNEL staining was used to quantify apoptotic endothelial cells in response to Bnip3 knockdown. *p < 0.05.
Resveratrol Regulates Bnip3 Through Hypoxia-Induced Factor 1 (HIF1) and 5′ AMP-Activated Protein Kinase (AMPK)
Previous studies have reported that Bnip3 is primarily regulated by two pathways: one is HIF1 (Guo et al., 2001) and the other is AMPK (Park et al., 2013). Experiments were conducted to understand whether these two pathways were activated by resveratrol, promoting Bnip3-related mitophagy. Immunofluorescence assay demonstrated that both HIF1 and AMPK were downregulated in response to ox-LDL treatment (Figures 5A–C). Interestingly, resveratrol treatment was associated with an increase in HIF1 and AMPK levels (Figures 5A–C), confirming our hypothesis that both HIF1 and AMPK could be positively regulated by resveratrol. To investigate whether increased HIF1 and AMPK were implicated in resveratrol-induced Bnip3 upregulation, acriflavine (Acr) and compound c (CC), the antagonists of HIF1 and AMPK, respectively, were incubated with endothelial cells before resveratrol treatment. Then, Bnip3 transcription and mitophagy activity were remeasured. Figure 5D shows that resveratrol treatment sustained the transcription of Bnip3 in ox-LDL-treated endothelial cells. However, once supplemented with either Acr or CC, the transcription of Bnip3 was downregulated (Figure 5D), suggesting that inhibition of HIF1 or AMPK could abolish the regulatory effects by resveratrol on Bnip3. mt-Kemia assays showed resveratrol upregulated the number of acid mitochondria in the presence of ox-LDL (Figures 5E,F), indicative of mitophagy activation in response to resveratrol. However, treatment with Acr or CC reduced acid mitochondrial content in resveratrol-treated endothelial cells, suggesting that HIF1 blockade of AMPK is followed by mitophagy inactivation. Taken together, our results indicate that resveratrol upregulates Bnip3-related mitophagy through HIF1 and AMPK.
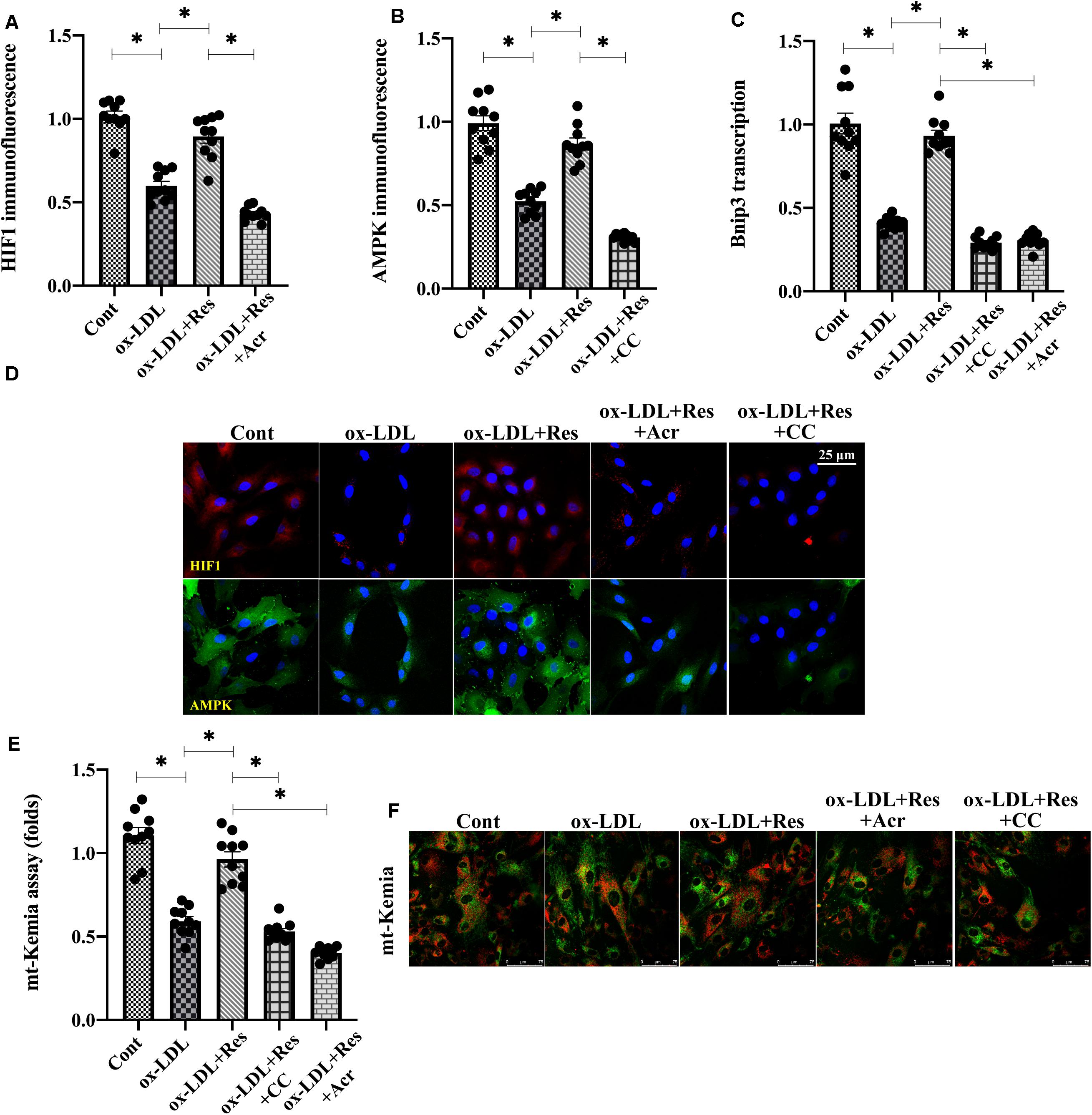
Figure 5. Resveratrol regulates Bnip3 through the HIF1 and AMPK. (A–C) Immunofluorescence assay for HIF1 and AMPK in endothelial cells treated with ox-LDL in the presence or absence of resveratrol. Acriflavine (Acr) and compound c (CC), the antagonists of HIF1 and AMPK, respectively, were incubated with endothelial cells before resveratrol treatment. (D) qPCR was used to determine the Bnip3 alteration in endothelial cells treated with ox-LDL, resveratrol, Acr, or CC. (E,F) Endothelial mitophagy was detected through mt-Kemia. The acid mitochondria number was recorded to reflect mitophagy activity. *p < 0.05.
Discussion
Oxidized LDL contributes to endothelial dysfunction, which is followed by vascular inflammation, smooth muscle proliferation, plaque formation, luminal stenosis, and other pathological alterations involved in the development of atherosclerosis. Statin treatment results in significant reductions in cardiovascular risk; however, individuals with well-controlled LDL levels may remain at increased risk owing to persistent high triglycerides and low HDL (Seidel et al., 2019). Therefore, an urgent need exists for hyperlipemia management. In the present study, we found that resveratrol improved endothelial function through sustaining endothelial cell viability, proliferation, and mobilization (Rusnati et al., 2019). Resveratrol treatment activated HIF1 and AMPK pathways, contributing to Bnip3 upregulation and mitophagy activation. Subsequently, Bnip3-related mitophagy attenuated oxidative stress and sustained mitochondrial function in the setting of hyperlipemia. To our knowledge, these data provide the first evidence for the use of resveratrol in preventing high-fat-mediated endothelial dysfunction. Our results identify Bnip3-related mitophagy as a primary protective mechanism responsible for resveratrol-mediated endothelial protection.
Most previous in-depth studies have explored the roles of resveratrol in cardiovascular disorders. For example, resveratrol enhances the expression of Nrf2 in myocardium and, thus, alleviates myocardial ischemia-reperfusion injury (Schreiber et al., 2019; Xu et al., 2019). Administration of resveratrol is shown to retard the progression of pulmonary arterial hypertension (Ferreira et al., 2019). Treatment with resveratrol attenuates isoprenaline-related cardiotoxicity in Wistar rats (Sammeturi et al., 2019). Resveratrol prevents ventricular hypertrophy (Chelladurai et al., 2019) and cardiac remodeling following chronic kidney disease (Li et al., 2020a). Resveratrol also helps to protect aortic valve stenosis (Samiei et al., 2019), septic cardiomyopathy (Liang et al., 2019), diabetic cardiomyopathy (Hoseini et al., 2019), and heart failure (Algieri et al., 2019). Most associated studies have focused on the influence of resveratrol on hyperlipemia-related cardiomyocyte damage or metabolic reprogramming, but not on high-fat-related endothelial dysfunction. Our data support that resveratrol, leading to improved endothelial function in the setting of hyperlipemia, may open a therapeutic window for the treatment of atherosclerosis.
At the molecular level, two mechanisms involved in how resveratrol attenuates hyperlipemia-related cardiomyocyte injury have been reported: one is driven by upregulation of antioxidative factors and the other involves downregulation of pro-inflammation cytokines. For example, resveratrol attenuates lipid peroxidation (Jalili et al., 2019) through modulation of several antioxidative signaling pathways such as Nrf2 (Zhuang et al., 2019), Sirt1 (Liu et al., 2019a), Akt/mTOR (Radwan and Karam, 2020), and ERK1/2 (Fathalipour et al., 2019). In addition, the mRNA expression of inflammatory cytokines in diabetic mice are largely inhibited by resveratrol (Xing et al., 2020). Inflammation-related signaling pathways, such as NF-κB (Ma et al., 2020), Smad2/3 (Zou et al., 2019), and HSP70 (Khafaga et al., 2019), are also blocked by resveratrol. In the present study, we found that resveratrol modulated mitochondrial ROS production through affecting mitochondrial respiration complex I and III activities. This provides novel insight into the regulatory actions of resveratrol on redox biology. Similar to our results, previous studies have also reported the involvement of resveratrol in mitochondrial homeostasis (Nwadozi et al., 2019). For example, resveratrol improves mitochondrial ATP generation through the AMPK pathway in the ischemic brain (Pineda-Ramirez et al., 2020). Mitochondrial biogenesis is partly enhanced by resveratrol through the miR-22/Sirt1 signaling pathway (Mao et al., 2019). Mitochondrial calcium homeostasis and mitochondrial potential stabilization are also under the control of resveratrol (Algieri et al., 2019). Mitochondrial morphological alterations, such as mitochondrial fission and fusion, are also balanced by resveratrol in different types of cells, such as hepatocytes (Chen et al., 2019b), cardiomyocytes (Lu et al., 2019), and endothelium (Yu et al., 2019).
Our data identified Bnip3-related mitophagy was activated by resveratrol and attenuated ox-LDL-induced mitochondrial damage as well as oxidative stress. Interestingly, after exposure to ox-LDL, both Parkin and Bnip3 were downregulated, whereas resveratrol upregulated Bnip3 transcription. This finding is consistent with a previous study that Parkin-dependent mitophagy usually works in neurodegenerative disease, whereas Bnip3-related mitophagy affects metabolic disorders such as fatty liver disease and diabetes (Li et al., 2018). Additionally, we demonstrated resveratrol upregulated Bnip3 transcription through HIF1 and AMPK. AMPK is a sensor of cellular energy status. Increased AMPK is able to upregulate gene transcription, including Bnip3. This phenomenon has been observed in diabetic nephropathy (Liu et al., 2019b) and muscle atrophy (Bak et al., 2019). HIF1 is a hypoxia-activated transcriptional factor (Martinez-Outschoorn et al., 2010), whereas Bnip3 is a hypoxia-related gene (Guo et al., 2001). Additional studies have reported the causal relationship between HIF1 activation and Bnip3 upregulation (Park et al., 2013). In the present study, we found that both AMPK and HIF1 were employed by resveratrol to enhance Bnip3-related mitophagy. The interlinked signaling pathways of Bnip3-related mitophagy in response to resveratrol treatment would offer new targets for therapeutic approaches of endothelial protection in the setting of hyperlipemia.
Together, using biochemical approaches and genetic deletion in vitro, we identify a potentially novel pathway by which resveratrol attenuates high-fat-induced endothelia dysfunction dependently of the Bnip3-related mitophagy. However, clinical data and animal studies are necessary to support our findings.
Data Availability Statement
The raw data supporting the conclusions of this article will be made available by the authors, without undue reservation.
Author Contributions
CL and YT contributed to the study concepts, experiment performance, and the data acquisition. JW, QM, and SB contributed to manuscript preparation and the data analysis. ZX and XW contributed to statistical analysis and manuscript review. JL was involved in manuscript editing. All authors contributed to the article and approved the submitted version.
Funding
This study was supported by Medical Research Project of Foshan Municipal Commission of Health and Family Planning (No: 20180099), Key Specialist Department Training Project of Foshan City, Guangdong Province of China (No: Fspy3-2015020), Key Medical Specialist Construction Project of Foshan during the 13th 5-Year Plan Period (No: FSZDZK135027).
Conflict of Interest
The authors declare that the research was conducted in the absence of any commercial or financial relationships that could be construed as a potential conflict of interest.
References
Algieri, C., Trombetti, F., Pagliarani, A., Ventrella, V., Bernardini, C., Fabbri, M., et al. (2019). Mitochondrial Ca(2+) -activated F1 FO -ATPase hydrolyzes ATP and promotes the permeability transition pore. Ann. N. Y. Acad. Sci. 1457, 142–157. doi: 10.1111/nyas.14218
Bak, D. H., Na, J., Im, S. I., Oh, C. T., Kim, J. Y., Park, S. K., et al. (2019). Antioxidant effect of human placenta hydrolysate against oxidative stress on muscle atrophy. J. Cell Physiol. 234, 1643–1658. doi: 10.1002/jcp.27034
Cesar, V., Jozic, I., Begovic, L., Vukovic, T., Mlinaric, S., Lepedus, H., et al. (2018). Cell-type-specific modulation of hydrogen peroxide cytotoxicity and 4-Hydroxynonenal binding to human cellular proteins in vitro by antioxidant aloe vera extract. Antioxidants (Basel) 7:125. doi: 10.3390/antiox7100125
Chelladurai, P., Boucherat, O., Stenmark, K., Kracht, M., Seeger, W., Bauer, U. M., et al. (2019). Targeting histone acetylation in pulmonary hypertension and right ventricular hypertrophy. Br. J. Pharmacol. doi: 10.1111/bph.14932 Online ahead of print.
Chen, J. Y., Zhu, Q., Zhang, S., OuYang, D., and Lu, J. H. (2019a). Resveratrol in experimental Alzheimer’s disease models: a systematic review of preclinical studies. Pharmacol. Res. 150:104476. doi: 10.1016/j.phrs.2019.104476
Chen, T. S., Ju, D. T., Day, C. H., Yeh, Y. L., Chen, R. J., Viswanadha, V. P., et al. (2019b). Protective effect of autologous transplantation of resveratrol preconditioned adipose-derived stem cells in the treatment of diabetic liver dysfunction in rat model. J. Tissue Eng. Regen. Med. 13, 1629–1640. doi: 10.1002/term.2917
Daiber, A., and Chlopicki, S. (2020). Revisiting pharmacology of oxidative stress and endothelial dysfunction in cardiovascular disease: evidence for redox-based therapies. Free Radic Biol. Med. doi: 10.1016/j.freeradbiomed.2020.02.026 Online ahead of print.
DeLeon-Pennell, K. Y., Mouton, A. J., Ero, O. K., Ma, Y., Padmanabhan Iyer, R., Flynn, E. R., et al. (2018). LXR/RXR signaling and neutrophil phenotype following myocardial infarction classify sex differences in remodeling. Basic Res. Cardiol. 113:40. doi: 10.1007/s00395-018-0699-695
Dludla, P. V., Silvestri, S., Orlando, P., Gabuza, K. B., Mazibuko-Mbeje, S. E., Nyambuya, T. M., et al. (2020). Exploring the comparative efficacy of metformin and resveratrol in the management of diabetes-associated complications: a systematic review of preclinical studies. Nutrients 12:739. doi: 10.3390/nu12030739
Fathalipour, M., Eghtedari, M., Borges, F., Silva, T., Moosavi, F., Firuzi, O., et al. (2019). Caffeic Acid Alkyl amide derivatives ameliorate oxidative stress and modulate ERK1/2 and AKT signaling pathways in a rat model of diabetic retinopathy. Chem. Biodivers. 16:e1900405. doi: 10.1002/cbdv.201900405
Ferreira, A. C., Serejo, J. S., Durans, R., Pereira Costa, J. M., Santos Maciel, A. W., Mendes Vieira, A. S., et al. (2019). Dose-related effects of resveratrol in different models of pulmonary arterial hypertension: systematic review. Curr. Cardiol. Rev. doi: 10.2174/1573403X15666191203110554 Online ahead of print.
Guo, K., Searfoss, G., Krolikowski, D., Pagnoni, M., Franks, C., Clark, K., et al. (2001). Hypoxia induces the expression of the pro-apoptotic gene BNIP3. Cell Death Differ. 8, 367–376. doi: 10.1038/sj.cdd.4400810
Heusch, G. (2019). Coronary microvascular obstruction: the new frontier in cardioprotection. Basic Res. Cardiol. 114:45. doi: 10.1007/s00395-019-0756-758
Hoseini, A., Namazi, G., Farrokhian, A., Reiner, Z., Aghadavod, E., Bahmani, F., et al. (2019). The effects of resveratrol on metabolic status in patients with type 2 diabetes mellitus and coronary heart disease. Food Funct. 10, 6042–6051. doi: 10.1039/c9fo01075k
Huang, C. C., Kuo, H. M., Wu, P. C., Cheng, S. H., Chang, T. T., Chang, Y. C., et al. (2018). Soluble delta-like 1 homolog (DLK1) stimulates angiogenesis through Notch1/Akt/eNOS signaling in endothelial cells. Angiogenesis 21, 299–312. doi: 10.1007/s10456-018-9596-9597
Hysi, P. G., Khawaja, A. P., Menni, C., Tamraz, B., Wareham, N., Khaw, K. T., et al. (2019). Ascorbic acid metabolites are involved in intraocular pressure control in the general population. Redox Biol. 20, 349–353. doi: 10.1016/j.redox.2018.10.004
Jalili, C., Roshankhah, S., Salahshoor, M. R., and Mohammadi, M. M. (2019). Resveratrol attenuates malathion induced damage in some reproductive parameters by decreasing oxidative stress and lipid peroxidation in male rats. J. Family Reprod. Health 13, 70–79.
Khafaga, A. F., Noreldin, A. E., and Taha, A. E. (2019). The adaptogenic anti-ageing potential of resveratrol against heat stress-mediated liver injury in aged rats: role of HSP70 and NF-kB signalling. J. Therm. Biol. 83, 8–21. doi: 10.1016/j.jtherbio.2019.04.012
Khodeer, D. M., Bilasy, S. E., Farag, N. E., Mehana, A. E., and Elbaz, A. A. (2019). Sitagliptin protects diabetic rats with acute myocardial infarction through induction of angiogenesis: role of IGF-1 and VEGF. Can. J. Physiol. Pharmacol. 97, 1053–1063. doi: 10.1139/cjpp-2018-2670
Kim, O. Y., Chung, J. Y., and Song, J. (2019). Effect of resveratrol on adipokines and myokines involved in fat browning: perspectives in healthy weight against obesity. Pharmacol. Res. 148:104411. doi: 10.1016/j.phrs.2019.104411
Knani, L., Bartolini, D., Kechiche, S., Tortoioli, C., Murdolo, G., Moretti, M., et al. (2019). Melatonin prevents cadmium-induced bone damage: first evidence on an improved osteogenic/adipogenic differentiation balance of mesenchymal stem cells as underlying mechanism. J. Pineal. Res. 67:e12597. doi: 10.1111/jpi.12597
Korbel, C., Gerstner, M. D., Menger, M. D., and Laschke, M. W. (2018). Notch signaling controls sprouting angiogenesis of endometriotic lesions. Angiogenesis 21, 37–46. doi: 10.1007/s10456-017-9580-9587
Kowaltowski, A. J. (2019). Strategies to detect mitochondrial oxidants. Redox Biol. 21:101065. doi: 10.1016/j.redox.2018.101065
Lee, B. W. L., Ghode, P., and Ong, D. S. T. (2019). Redox regulation of cell state and fate. Redox Biol. 25:101056. doi: 10.1016/j.redox.2018.11.014
Lejri, I., Agapouda, A., Grimm, A., and Eckert, A. (2019). Mitochondria- and oxidative stress-targeting substances in cognitive decline-related disorders: from molecular mechanisms to clinical evidence. Oxid. Med. Cell Longev. 2019:9695412. doi: 10.1155/2019/9695412
Li, P., Song, X., Zhang, D., Guo, N., Wu, C., Chen, K., et al. (2020a). Resveratrol improves left ventricular remodeling in chronic kidney disease via Sirt1-mediated regulation of FoxO1 activity and MnSOD expression. Biofactors 46, 168–179. doi: 10.1002/biof.1584
Li, Z., Zhang, Z., Ke, L., Sun, Y., Li, W., Feng, X., et al. (2020b). Resveratrol promotes white adipocytes browning and improves metabolic disorders in Sirt1-dependent manner in mice. FASEB J. 34, 4527–4539. doi: 10.1096/fj.201902222R
Li, R., Xin, T., Li, D., Wang, C., Zhu, H., and Zhou, H. (2018). Therapeutic effect of Sirtuin 3 on ameliorating nonalcoholic fatty liver disease: the role of the ERK-CREB pathway and Bnip3-mediated mitophagy. Redox Biol. 18, 229–243. doi: 10.1016/j.redox.2018.07.011
Liang, Y., Zhou, J., Ji, K., Liu, H., Degen, A., Zhai, M., et al. (2019). Protective effect of resveratrol improves systemic inflammation responses in LPS-Injected lambs. Animals (Basel) 9:872. doi: 10.3390/ani9110872
Liu, D., Ma, Z., Xu, L., Zhang, X., Qiao, S., and Yuan, J. (2019a). PGC1alpha activation by pterostilbene ameliorates acute doxorubicin cardiotoxicity by reducing oxidative stress via enhancing AMPK and SIRT1 cascades. Aging (Albany NY) 11, 10061–10073. doi: 10.18632/aging.102418
Liu, Z., Liu, H., Xiao, L., Liu, G., Sun, L., and He, L. (2019b). STC-1 ameliorates renal injury in diabetic nephropathy by inhibiting the expression of BNIP3 through the AMPK/SIRT3 pathway. Lab Invest. 99, 684–697. doi: 10.1038/s41374-018-0176-177
Lu, J., Zheng, Y., Yang, J., Zhang, J., Cao, W., Chen, X., et al. (2019). Resveratrol alleviates inflammatory injury and enhances the apoptosis of fibroblastlike synoviocytes via mitochondrial dysfunction and ER stress in rats with adjuvant arthritis. Mol. Med. Rep. 20, 463–472. doi: 10.3892/mmr.2019.10273
Luirink, I. K., Wiegman, A., Kusters, D. M., Hof, M. H., Groothoff, J. W., de Groot, E., et al. (2019). 20-Year follow-up of statins in children with familial hypercholesterolemia. N. Engl. J. Med. 381, 1547–1556. doi: 10.1056/NEJMoa1816454
Luo, C., Xu, X., Wei, X., Feng, W., Huang, H., Liu, H., et al. (2019). Natural medicines for the treatment of fatigue: bioactive components, pharmacology, and mechanisms. Pharmacol. Res. 148:104409. doi: 10.1016/j.phrs.2019.104409
Ma, P., Yue, L., Yang, H., Fan, Y., Bai, J., Li, S., et al. (2020). Chondroprotective and anti-inflammatory effects of amurensin H by regulating TLR4/Syk/NF-kappaB signals. J. Cell Mol. Med. 24, 1958–1968. doi: 10.1111/jcmm.14893
Mao, G. X., Xu, X. G., Wang, S. Y., Li, H. F., Zhang, J., Zhang, Z. S., et al. (2019). Salidroside delays cellular senescence by stimulating mitochondrial biogenesis partly through a miR-22/SIRT-1 Pathway. Oxid Med. Cell Longev. 2019:5276096. doi: 10.1155/2019/5276096
Martinez-Outschoorn, U. E., Trimmer, C., Lin, Z., Whitaker-Menezes, D., Chiavarina, B., Zhou, J., et al. (2010). Autophagy in cancer associated fibroblasts promotes tumor cell survival: role of hypoxia, HIF1 induction and NFkappaB activation in the tumor stromal microenvironment. Cell Cycle 9, 3515–3533. doi: 10.4161/cc.9.17.12928
Nwadozi, E., Ng, A., Stromberg, A., Liu, H. Y., Olsson, K., Gustafsson, T., et al. (2019). Leptin is a physiological regulator of skeletal muscle angiogenesis and is locally produced by PDGFRalpha and PDGFRbeta expressing perivascular cells. Angiogenesis 22, 103–115. doi: 10.1007/s10456-018-9641-9646
Park, C. W., Hong, S. M., Kim, E. S., Kwon, J. H., Kim, K. T., Nam, H. G., et al. (2013). BNIP3 is degraded by ULK1-dependent autophagy via MTORC1 and AMPK. Autophagy 9, 345–360. doi: 10.4161/auto.23072
Pineda-Ramirez, N., Alquisiras-Burgos, I., Ortiz-Plata, A., Ruiz-Tachiquin, M. E., Espinoza-Rojo, M., and Aguilera, P. (2020). Resveratrol activates neuronal autophagy through AMPK in the ischemic brain. Mol. Neurobiol. 57, 1055–1069. doi: 10.1007/s12035-019-01803-1806
Quispe, R. L., Jaramillo, M. L., Galant, L. S., Engel, D., Dafre, A. L., Teixeira, et al. (2019). Diphenyl diselenide protects neuronal cells against oxidative stress and mitochondrial dysfunction: involvement of the glutathione-dependent antioxidant system. Redox Biol. 20, 118–129. doi: 10.1016/j.redox.2018.09.014
Radwan, R. R., and Karam, H. M. (2020). Resveratrol attenuates intestinal injury in irradiated rats via PI3K/Akt/mTOR signaling pathway. Environ. Toxicol. 35, 223–230. doi: 10.1002/tox.22859
Rao, T., Tan, Z., Peng, J., Guo, Y., Chen, Y., Zhou, H., et al. (2019). The pharmacogenetics of natural products: a pharmacokinetic and pharmacodynamic perspective. Pharmacol. Res. 146:104283. doi: 10.1016/j.phrs.2019.104283
Rusnati, M., Borsotti, P., Moroni, E., Foglieni, C., Chiodelli, P., Carminati, L., et al. (2019). The calcium-binding type III repeats domain of thrombospondin-2 binds to fibroblast growth factor 2 (FGF2). Angiogenesis 22, 133–144. doi: 10.1007/s10456-018-9644-9643
Samiei, N., Hosseini, S., Maleki, M., Moradi, L., Joghataei, M. T., and Arabian, M. (2019). Modulatory role of SIRT1 and resistin as therapeutic targets in patients with aortic valve stenosis. Arch. Med. Res. 50, 333–341. doi: 10.1016/j.arcmed.2019.10.001
Sammeturi, M., Shaik, A. H., Bongu, S. B. R., Cheemanapalli, S., Mohammad, A., and Kodidhela, L. D. (2019). Protective effects of syringic acid, resveratrol and their combination against isoprenaline administered cardiotoxicity in wistar rats. Saudi J. Biol. Sci. 26, 1429–1435. doi: 10.1016/j.sjbs.2019.09.027
Schreiber, T., Salhofer, L., Quinting, T., and Fandrey, J. (2019). Things get broken: the hypoxia-inducible factor prolyl hydroxylases in ischemic heart disease. Basic Res. Cardiol. 114:16. doi: 10.1007/s00395-019-0725-722
Seidel, T., Fiegle, D. J., Baur, T. J., Ritzer, A., Nay, S., Heim, C., et al. (2019). Glucocorticoids preserve the t-tubular system in ventricular cardiomyocytes by upregulation of autophagic flux. Basic. Res. Cardiol. 114:47. doi: 10.1007/s00395-019-0758-756
Shi, C., Cai, Y., Li, Y., Li, Y., Hu, N., Ma, S., et al. (2018). Yap promotes hepatocellular carcinoma metastasis and mobilization via governing cofilin/F-actin/lamellipodium axis by regulation of JNK/Bnip3/SERCA/CaMKII pathways. Redox Biol. 14, 59–71. doi: 10.1016/j.redox.2017.08.013
Silverblatt, J. A., Ziff, O. J., Dancy, L., Daniel, A., Carter, B., Scott, P., et al. (2019). Therapies to limit myocardial injury in animal models of myocarditis: a systematic review and meta-analysis. Basic Res. Cardiol. 114:48. doi: 10.1007/s00395-019-0754-x
Su, H. H., Liao, J. M., Wang, Y. H., Chen, K. M., Lin, C. W., Lee, I. H., et al. (2019). Exogenous GDF11 attenuates non-canonical TGF-beta signaling to protect the heart from acute myocardial ischemia-reperfusion injury. Basic Res. Cardiol. 114:20. doi: 10.1007/s00395-019-0728-z
Trieb, M., Kornej, J., Knuplez, E., Hindricks, G., Thiele, H., Sommer, P., et al. (2019). Atrial fibrillation is associated with alterations in HDL function, metabolism, and particle number. Basic Res. Cardiol. 114:27. doi: 10.1007/s00395-019-0735-730
Trindade, F., Vitorino, R., Leite-Moreira, A., and Falcao-Pires, I. (2019). Pericardial fluid: an underrated molecular library of heart conditions and a potential vehicle for cardiac therapy. Basic Res. Cardiol. 114:10. doi: 10.1007/s00395-019-0716-713
van Duinen, V., Zhu, D., Ramakers, C., van Zonneveld, A. J., Vulto, P., and Hankemeier, T. (2019). Perfused 3D angiogenic sprouting in a high-throughput in vitro platform. Angiogenesis 22, 157–165. doi: 10.1007/s10456-018-9647-9640
Vijayan, B., Raj, V., Nandakumar, S., Kishore, A., and Thekkuveettil, A. (2019). Spermine protects alpha-synuclein expressing dopaminergic neurons from manganese-induced degeneration. Cell Biol. Toxicol. 35, 147–159. doi: 10.1007/s10565-018-09449-9441
Wang, J., Toan, S., and Zhou, H. (2020a). Mitochondrial quality control in cardiac microvascular ischemia-reperfusion injury: new insights into the mechanisms and therapeutic potentials. Pharmacol. Res. 156:104771. doi: 10.1016/j.phrs.2020.104771
Wang, J., Toan, S., and Zhou, H. (2020b). New insights into the role of mitochondria in cardiac microvascular ischemia/reperfusion injury. Angiogenesis 23, 299–314. doi: 10.1007/s10456-020-09720-9722
Wang, Y. S., Shen, C. Y., and Jiang, J. G. (2019). Antidepressant active ingredients from herbs and nutraceuticals used in TCM: pharmacological mechanisms and prospects for drug discovery. Pharmacol. Res. 150:104520. doi: 10.1016/j.phrs.2019.104520
Wolint, P., Bopp, A., Woloszyk, A., Tian, Y., Evrova, O., Hilbe, M., et al. (2019). Cellular self-assembly into 3D microtissues enhances the angiogenic activity and functional neovascularization capacity of human cardiopoietic stem cells. Angiogenesis 22, 37–52. doi: 10.1007/s10456-018-9635-9634
Xiao, X., Tang, J., Mao, Y., Li, X., Wang, J., Liu, C., et al. (2019). Guidance for the clinical evaluation of traditional Chinese medicine-induced liver injuryIssued by China Food and Drug Administration. Acta Pharm. Sin. B 9, 648–658. doi: 10.1016/j.apsb.2018.12.003
Xing, C., Wang, Y., Dai, X., Yang, F., Luo, J., Liu, P., et al. (2020). The protective effects of resveratrol on antioxidant function and the mRNA expression of inflammatory cytokines in the ovaries of hens with fatty liver hemorrhagic syndrome. Poult. Sci. 99, 1019–1027. doi: 10.1016/j.psj.2019.10.009
Xu, G., Zhao, X., Fu, J., and Wang, X. (2019). Resveratrol increase myocardial Nrf2 expression in type 2 diabetic rats and alleviate myocardial ischemia/reperfusion injury (MIRI). Ann. Palliat. Med. 8, 565–575. doi: 10.21037/apm.2019.11.25
Yan, B., Xie, S., Liu, Y., Liu, W., Li, D., Liu, M., et al. (2018). Histone deacetylase 6 modulates macrophage infiltration during inflammation. Theranostics 8, 2927–2938. doi: 10.7150/thno.25317
Yu, H., Pan, W., Huang, H., Chen, J., Sun, B., Yang, L., et al. (2019). Screening analysis of sirtuins family expression on anti-inflammation of resveratrol in endothelial cells. Med. Sci. Monit. 25, 4137–4148. doi: 10.12659/MSM.913240
Zakeri, Z., Lockshin, R. A., and Diederich, M. (2019). About canonical, non-canonical and immunogenic cell death: basic mechanisms and translational applications: a meeting report of the International Cell Death Society. Biochem. Pharmacol. 162, 1–2. doi: 10.1016/j.bcp.2018.09.011
Zarfati, M., Avivi, I., Brenner, B., Katz, T., and Aharon, A. (2019). Extracellular vesicles of multiple myeloma cells utilize the proteasome inhibitor mechanism to moderate endothelial angiogenesis. Angiogenesis 22, 185–196. doi: 10.1007/s10456-018-9649-y
Zeron, R. M. C., and Albuquerque, V. C. (2019). Hypertension and cholesterol. Rev Assoc Med Bras (1992) 65, 1421–1422. doi: 10.1590/1806-9282.65.12.1421
Zhang, E., Guo, Q., Gao, H., Xu, R., Teng, S., and Wu, Y. (2015). Metformin and resveratrol inhibited high glucose-induced metabolic memory of endothelial senescence through SIRT1/p300/p53/p21 pathway. PLoS One 10:e0143814. doi: 10.1371/journal.pone.0143814
Zhang, H., Jin, B., and Faber, J. E. (2019a). Mouse models of Alzheimer’s disease cause rarefaction of pial collaterals and increased severity of ischemic stroke. Angiogenesis 22, 263–279. doi: 10.1007/s10456-018-9655-9650
Zhang, H. F., Wang, Y. L., Tan, Y. Z., Wang, H. J., Tao, P., and Zhou, P. (2019b). Enhancement of cardiac lymphangiogenesis by transplantation of CD34(+)VEGFR-3(+) endothelial progenitor cells and sustained release of VEGF-C. Basic Res. Cardiol. 114:43. doi: 10.1007/s00395-019-0752-z
Zhang, W., Guo, T., Wang, C., He, Y., Zhang, X., Li, G., et al. (2019c). MOF capacitates cyclodextrin to mega-load mode for high-efficient delivery of valsartan. Pharm. Res. 36:117. doi: 10.1007/s11095-019-2650-2653
Zhang, Y. J., Zhao, H., Dong, L., Zhen, Y. F., Xing, H. Y., Ma, H. J., et al. (2019d). Resveratrol ameliorates high-fat diet-induced insulin resistance and fatty acid oxidation via ATM-AMPK axis in skeletal muscle. Eur. Rev. Med. Pharmacol. Sci. 23, 9117–9125. doi: 10.26355/eurrev_201910_19315
Zhao, J., Gao, J. L., Zhu, J. X., Zhu, H. B., Peng, X., Jiang, M., et al. (2019). The different response of cardiomyocytes and cardiac fibroblasts to mitochondria inhibition and the underlying role of STAT3. Basic Res. Cardiol. 114:12. doi: 10.1007/s00395-019-0721-726
Zhong, L., Xu, L., Liu, Y., Li, Q., Zhao, D., Li, Z., et al. (2019). Transformative hyaluronic acid-based active targeting supramolecular nanoplatform improves long circulation and enhances cellular uptake in cancer therapy. Acta Pharm. Sin B 9, 397–409. doi: 10.1016/j.apsb.2018.11.006
Zhou, H., Li, D., Zhu, P., Ma, Q., Toan, S., Wang, J., et al. (2018a). Inhibitory effect of melatonin on necroptosis via repressing the Ripk3-PGAM5-CypD-mPTP pathway attenuates cardiac microvascular ischemia-reperfusion injury. J. Pineal. Res. 65:e12503. doi: 10.1111/jpi.12503
Zhou, H., Wang, S., Hu, S., Chen, Y., and Ren, J. (2018b). ER-Mitochondria microdomains in cardiac ischemia-reperfusion injury: a fresh perspective. Front. Physiol. 9:755. doi: 10.3389/fphys.2018.00755
Zhou, H., Wang, S., Zhu, P., Hu, S., Chen, Y., and Ren, J. (2018c). Empagliflozin rescues diabetic myocardial microvascular injury via AMPK-mediated inhibition of mitochondrial fission. Redox Biol. 15, 335–346. doi: 10.1016/j.redox.2017.12.019
Zhou, H., Zhu, P., Wang, J., Zhu, H., Ren, J., and Chen, Y. (2018d). Pathogenesis of cardiac ischemia reperfusion injury is associated with CK2alpha-disturbed mitochondrial homeostasis via suppression of FUNDC1-related mitophagy. Cell Death Differ. 25, 1080–1093. doi: 10.1038/s41418-018-0086-87
Zhou, H., and Toan, S. (2020). Pathological roles of mitochondrial oxidative stress and mitochondrial dynamics in cardiac microvascular ischemia/reperfusion injury. Biomolecules 10:85. doi: 10.3390/biom10010085
Zhou, H., Zhu, P., Wang, J., Toan, S., and Ren, J. (2019). DNA-PKcs promotes alcohol-related liver disease by activating Drp1-related mitochondrial fission and repressing FUNDC1-required mitophagy. Signal Transduct. Target Ther. 4:56. doi: 10.1038/s41392-019-0094-91
Zhu, Y., Wang, P., Zhang, L., Bai, G., Yang, C., Wang, Y., et al. (2019). Superhero Rictor promotes cellular differentiation of mouse embryonic stem cells. Cell Death Differ. 26, 958–968. doi: 10.1038/s41418-018-0177-175
Zhuang, Y., Wu, H., Wang, X., He, J., He, S., and Yin, Y. (2019). Resveratrol attenuates oxidative stress-induced intestinal barrier injury through PI3K/Akt-Mediated Nrf2 signaling pathway. Oxid Med. Cell Longev. 2019:7591840. doi: 10.1155/2019/7591840
Keywords: resveratrol, mitochondria, oxidative stress, Bnip3, mitophagy
Citation: Li C, Tan Y, Wu J, Ma Q, Bai S, Xia Z, Wan X and Liang J (2020) Resveratrol Improves Bnip3-Related Mitophagy and Attenuates High-Fat-Induced Endothelial Dysfunction. Front. Cell Dev. Biol. 8:796. doi: 10.3389/fcell.2020.00796
Received: 17 June 2020; Accepted: 28 July 2020;
Published: 14 August 2020.
Edited and Reviewed by:
Hao Zhou, People’s Liberation Army General Hospital, ChinaCopyright © 2020 Li, Tan, Wu, Ma, Bai, Xia, Wan and Liang. This is an open-access article distributed under the terms of the Creative Commons Attribution License (CC BY). The use, distribution or reproduction in other forums is permitted, provided the original author(s) and the copyright owner(s) are credited and that the original publication in this journal is cited, in accordance with accepted academic practice. No use, distribution or reproduction is permitted which does not comply with these terms.
*Correspondence: Jianqiu Liang, ljqwqq@126.com
†These authors have contributed equally to this work