- 1Department of Orthodontics, School of Dental Medicine, Tsurumi University, Yokohama, Japan
- 2vTv Therapeutics LLC, High Point, NC, United States
Bone destructive diseases such as periodontitis are common worldwide and are caused by excessive osteoclast formation and activation. Receptor activator of nuclear factor-κB ligand (RANKL) is essential factor for osteoclastogenesis. This triggers reactive oxygen species (ROS), which has a key role in intracellular signaling as well exerting cytotoxicity. Cells have protective mechanisms against ROS, such as nuclear factor E2-related factor 2 (Nrf2), which controls the expression of many antioxidant enzyme genes. Conversely, BTB and CNC homology 1 (Bach1), a competitor for Nrf2, transcriptionally represses the expression of anti-oxidant enzymes. Previously, we demonstrated that RANKL induces Bach1 nuclear import and attenuates the expression of Nrf2-mediated antioxidant enzymes, thereby augmenting intracellular ROS signaling and osteoclastogenesis. However, it remains unknown if Bach1 inhibitors attenuate osteoclastogenesis. In this study, we hypothesized that Bach1 inhibition would exert an anti-osteoclastogenic effects via diminishing of intracellular ROS signaling through augmented antioxidation. We used RAW 264.7 cells as osteoclast progenitor cells. Using flow cytometry, we found that Bach1 inhibitors attenuated RANKL-mediated ROS generation, which resulted in the inhibition of osteoclastogenesis. Local injection of a Bach1 inhibitor into the calvaria of male BALB/c mice blocked bone destruction induced by lipopolysaccharide. In conclusion, we demonstrate that Bach1 inhibitor attenuates RANKL-mediated osteoclastogenesis and bone destruction in mice by inducing the expression of Nrf2-regulated antioxidant enzymes that consequently decrease intracellular ROS levels. Bach1 inhibitors have potential in inhibiting bone destructive diseases such as periodontitis, rheumatoid arthritis and osteoporosis.
Introduction
Osteoclasts are multi-nucleated cells derived from macrophages/monocytes and play a central role in bone resorption (Harada and Rodan, 2003). In bone destructive diseases such as periodontitis, rheumatoid arthritis and osteoporosis, dysregulation of osteoclasts cause bone destruction (Taubman et al., 2005). Several pathways are known to regulate osteoclast differentiation, with macrophage colony-stimulating factor (M-CSF) and Receptor activator of nuclear factor-κB ligand (RANKL) particularly critical for osteoclastogenesis (Takayanagi, 2007). Reactive oxygen species (ROS) is an important signal for osteoclastogenesis to induce the change of the concentration of intracellular calcium ion (Bax et al., 1992). Nuclear factor E2-related factor 2 (Nrf2) is a transcriptional factor which binds to antioxidant response elements (ARE) and controls gene expression of many antioxidant enzymes against oxidative stress (Favreau and Pickett, 1991; Wild et al., 1998; Alam et al., 1999; Thimmulappa et al., 2002). Previously, we reported that overexpression of Nrf2 inhibited osteoclastogenesis, while knockdown of Nrf2 induced osteoclastogenesis (Kanzaki et al., 2013). Therefore, we consider Nrf2 a key regulatory molecule of osteoclastogenesis.
We previously demonstrated that RANKL induces nuclear import of BTB and CNC homology 1 (Bach1) and nuclear export of Nrf2 (Kanzaki et al., 2017). We additionally found that Bach1 is exported from the nucleus to the cytoplasm by 5-aminolevulinic acid (ALA) and sodium ferrous citrate (SFC) treatment, which is followed by an increase in nuclear Nrf2, and inhibition of osteoclastogenesis (Kanzaki et al., 2017). Bach1 is a transcriptional repressor that binds to AREs and suppresses Nrf2 activity (Dhakshinamoorthy et al., 2005). It has been also reported that gold nanoparticles and sodium arsenite elicit Bach1 nuclear export and Nrf2 nuclear import, and increase expression of anti-oxidative enzymes such as NQO1 and HMOX1 (Liu et al., 2013; Lai et al., 2015). While Bach1 nuclear import is considered important for osteoclastogenesis (Kanzaki et al., 2017), it remains unknown whether Bach1 inhibition, which accelerates Bach1 nuclear export, exerts an anti-osteoclastogenic effect.
In this study, we hypothesized that Bach1 inhibition would suppress osteoclastogenesis and bone destruction via attenuation of intracellular ROS signaling through antioxidant mechanisms. To test this hypothesis, we used Bach1 inhibitors for in vitro and in vivo experiments.
Materials and Methods
Chemicals
Recombinant RANKL was purchased from Wako Pure Chemical (Osaka, Japan). Compounds High Point Pharmaceuticals (HPP)-A, HPP-B, HPP-C, and HPP-E are provided by vTv Therapeutics LLC, and each compound is subject to H1 NMR analysis for structural and purity (>95%) verification. Compounds HPP-A, HPP-B, HPP-C, and HPP-E each have the same core structure but different sidechain structures (R1 to R3) shown Figure 1 and may be prepared according to the general procedures provided in PCT International Publication Number WO 2011/103018. HPPs are able to modulate the transcriptional repression of Bach1 of ARE genes (Attucks et al., 2014). Potential binding of HPPs to Bach1 were also confirmed. Furthermore, these HPPs are non-electrophilic molecule which have shown not to conjugate with glutathione.
Cells
Mouse monocyte cell line, RAW264.7, was obtained from the Riken Bioresource Center (Tsukuba, Japan).
Cell Culture
RAW264.7 cells were cultured in α-modified Eagle’s medium (Wako Pure Chemical) that contained 10% fetal bovine serum (FBS; Atlas Biologicals, Fort Collins, CO, United States), with antibiotics (100 U/mL of penicillin and 100 μg/mL of streptomycin). All cells were cultured at 37°C in a 5% CO2 incubator.
Cell Viability Assay
Cytotoxicity of Bach1 inhibitors was examined using the AlamarBlueTM Cell Viability Reagent (Thermo Fisher Scientific, San Jose, CA, United States). In brief, RAW264.7 cells were seeded on 24-well plates and cultured with various concentrations of Bach1 inhibitors for 1 day. AlamarBlueTM Cell Viability Reagent was added to culture media and fluorescence intensity (excitation: 530 nm, emission: 590 nm) measured using the Synergy HTX Multi-Mode plate Reader (BioTek Japan, Tokyo, Japan) after 1 h.
Osteoclastogenesis Assay
Cells plated in 96-well plates (RAW264.7 cells: 103 cells/well) in triplicate were stimulated by recombinant RANKL (100 ng/ml) with or without Bach1 inhibitors. Cells were stained for tartrate-resistant acid phosphatase (TRAP) using an acid phosphatase kit (Sigma-Aldrich, St. Louis, MO, United States) after 4 days of culture. Dark red multinucleated cells (≥3 nuclei) were counted as TRAP-positive multinucleated cells.
Real-Time RT-PCR Analysis
RNA was extracted from RAW264.7 cells using the GenElute mammalian total RNA Miniprep kit (Sigma-Aldrich, St. Louis, MO, United States) with on-column genomic DNA digestion in accordance with the manufacturer’s instructions. For gene expression analysis of antioxidant enzymes, RNA was extracted at 1 day after Bach1 inhibitors treatment, and the gene expressions of Hmox1 and Nqo1 was analyzed because these anti-oxidant enzymes were reported to relate osteoclastogenesis (Zwerina et al., 2005; Sakamoto et al., 2012; Hyeon et al., 2013). For gene expression analysis of osteoclast markers, RNA was extracted at 4 days after RANKL stimulation, and the gene expressions of Atp6v0d2, Cathepsin K, Matrix metalloproteinase 9, Trap, Dcstamp, and Oscar were analyzed. RNA (500 ng) was reverse transcribed with iScript cDNA-Supermix (Bio-Rad Laboratories, Hercules, CA, United States).
Real-time RT-PCR was performed with SsoFast EvaGreen-Supermix (Bio-Rad Laboratories). PCR primers used in the experiments were from PrimerBank and are previously described (Kanzaki et al., 2013). Fold changes of gene of interest were calculated by using the Δ-Δ Ct method with Ribosomal protein S18 as reference gene. Data shown are representative of three independent experiments performed in triplicate.
Resorption Assay
RAW264.7 cells were plated on synthesized calcium phosphate substrate (bone resorption assay plate; PG Research, Tokyo, Japan) and stimulated with RANKL in the presence or absence of Bach1 inhibitors. After 7 days of cultivation, cells were removed with bleach, and the calcium phosphate substrate was washed with distilled water and then dried. Photographs were taken, and the average of the resorbed area per field was calculated from 12 images of each sample, using the software ImageJ (National Institutes of Health, Bethesda, MD, United States).
Preparation of Nuclear Protein Lysate
Nuclear protein lysate was prepared from RAW 264.7 cells using the LysoPureTM Nuclear and Cytoplasmic Extractor Kit (Wako, Osaka, Japan) according to the manufacturer’s instructions. Nuclear protein samples were extracted after 6 h of HPP-E treatment. Briefly, cultured cells were washed with PBS and treated with cell lysis buffer. After centrifugation, the nuclear pellet was washed twice and lysed with nuclear lysis reagent. The supernatant was used as the nuclear protein extract after centrifugation. The protein concentrations of the nuclear lysates were measured with the Quick StartTM protein assay kit (Bio-Rad), and concentrations adjusted to be similar.
Western Blot Analysis
Prepared protein lysates were then electrophoresed on TGX Precast gels (Bio-Rad Laboratories), and proteins were transferred to PVDF membranes using a Trans-Blot® TurboTM (Bio-Rad Laboratories). After washing, membranes were treated by PDVF Blocking Reagent® (Toyobo Co., Ltd., Osaka, Japan), then incubated with a primary antibody diluted in Can Get Signal® Solution-1 (Toyobo Co., Ltd.). After thorough washing with PBS containing 0.5% of Tween-20 (PBS-T), membranes were incubated with horse-radish peroxidase-conjugated secondary antibody in Can Get Signal Solution-2 (Toyobo Co., Ltd.), and washed with PBS-T. Chemiluminescence was produced using Luminata Forte (EMD Millipore Corporation, Billerica, MA, United States) and detected with LumiCube (Liponics, Tokyo, Japan). Primary antibodies used were anti-Nrf2 (1/1000 dilution; Santa Cruz Biotechnology Inc., Santa Cruz, CA, United States), and anti-histone H3 (1/4000; Cell Signaling Technology Japan, Tokyo, Japan).
Intracellular ROS Detection
Cells were pretreated with or without HPP-E for 1 h, stimulated with soluble RANKL for 6 h, washed with PBS, and collected. Cells were then stained with fluorescent superoxide probe (BES-So-AM; Wako Pure Chemical) on ice. After washing, intracellular ROS was detected using a flow cytometer (AccuriC6; BD Biosciences, San Jose, CA, United States). The viable monocyte/macrophage fraction was gated on a forward scatter/side scatter plot, and intracellular ROS levels were monitored in the FL-1 channel. Data shown are representative of three independent experiments performed in triplicate.
In vivo Bone Destruction Model
All animals were treated ethically, and animal experiments were performed in compliance with the Regulations for Animal Experiments and Related Activities at Tsurumi University. The calvarial bone destruction mouse model, induced by repeated LPS injections, has been described previously (Kanzaki et al., 2014). Twenty 7-week-old male BALB/c mice (Clea Japan, Tokyo, Japan) were used. Mice were randomly assigned to four groups (n = 5 each): a Dimethyl sulfoxide (DMSO)-injected group (control group; 10 μl PBS + 2 μl DMSO), a HPP-E-injected group (HPP-E group; 10 μl PBS + 2 μl of HPP-E 2 μM), an LPS-induced bone resorption group (LPS group; 10 μl of 1 μg/μl LPS + 2 μl DMSO), and an LPS-induced bone resorption and HPP-E-injected group (LPS + HPP-E group; 10 μl of 1 μg/μl LPS + 2 μl of HPP-E 2 μM). To reduce the number of experimental animals, we did not set the group of no-injection or PBS-injection because of the previous experiments revealed no difference of these groups to DMSO-injected group (data not shown).
Under anesthesia, injections were performed with a 30-gauge needle at a point on the midline of the skull located between the eyes on days 1, 3, 5, 7, and 9. On day 11, mice were sacrificed by cervical dislocation and cranial tissue samples were fixed overnight with 4% paraformaldehyde in PBS.
Micro-Computed Tomography Analysis for Bone Destruction
Samples of cranial tissue were scanned with an X-ray micro-computed tomography (μCT) system (inspeXio SMX-225CT; Shimadzu Corp., Kyoto, Japan). After reconstitution, the DICOM files were rendered into three-dimensional images using Pluto software (Kanzaki et al., 2014). Percentage of resorbed area, calculated from the ratio of the number of pixels in the resorbed area in the cranial bone to the number of pixels in the total analyzed image of the cranial bone, was calculated with ImageJ software. The region of interest was set between the fronto-parietal (coronal) suture and parieto-occipital (lambdoidal) suture.
Statistical Analysis
All data are presented as the mean ± standard deviation from three independent experiments. ANOVA and Tukey’s HSD test were used for evaluating the statistical significance (SPSS® 11.0J; IBM, Chicago, IL, United States). P < 0.05 was considered to be statistically significant.
Results
Assessment of Bach1 Inhibitor Cytotoxicity
First, we examined whether Bach1 inhibitors, HPP-A, HPP-B, HPP-C, and HPP-E, exhibit cytotoxicity against RAW264.7 cells. There was no cell cytotoxicity at 80 nM, but the highest tested concentration of 2000 nM exhibited cytotoxicity compared with the control (Figure 2). Therefore, we used 80 and 400 nM of HPPs for subsequent experiments due to no cytotoxicity of all HPPs at 80 nM, and relatively low level of cytotoxicity at 400 nM.
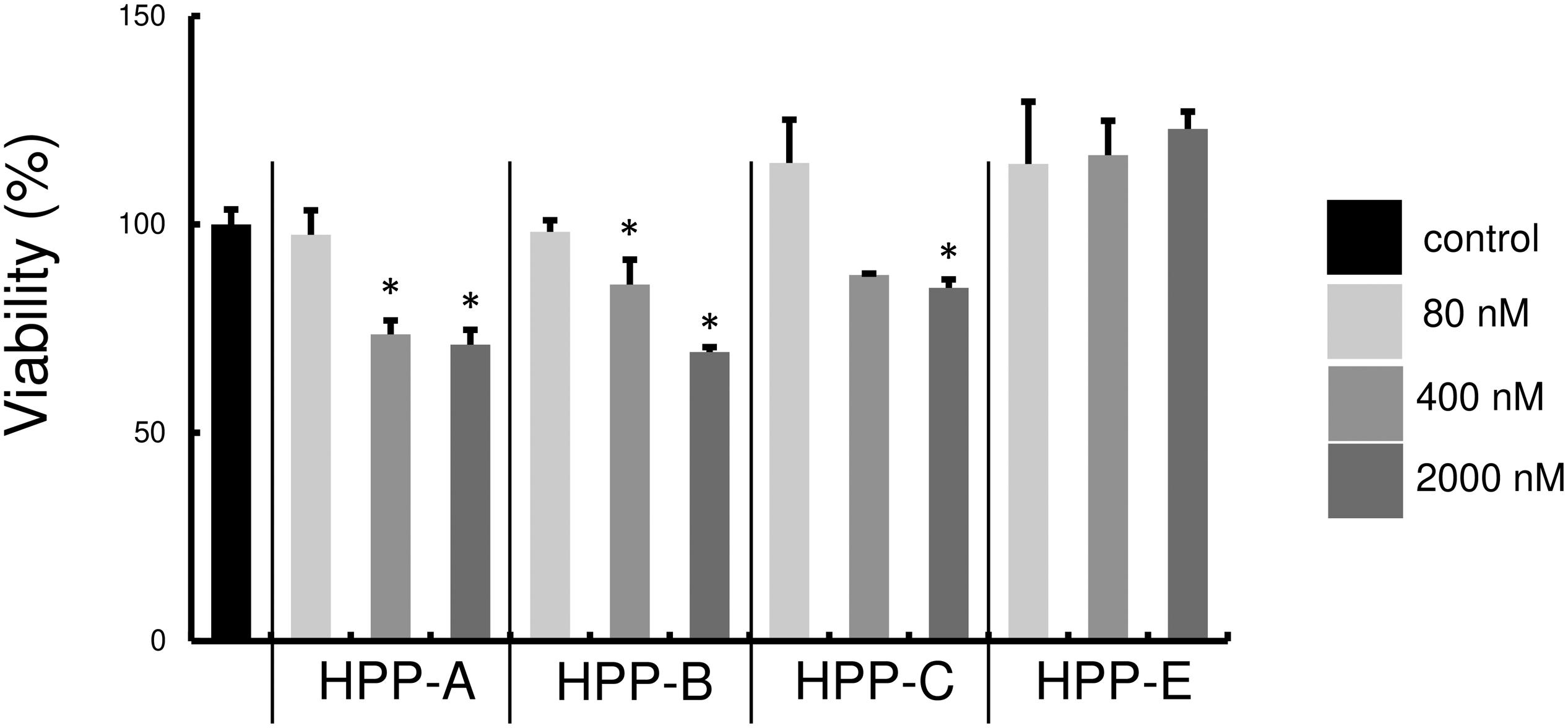
Figure 2. Cytotoxicity of Bach1 inhibitors against RAW 264.7 cells. Cytotoxicity at various concentrations of HPPs was assessed using AlamarBlue reagent. Percentage of control is shown. The data shown are representative of three independent experiments performed in triplicate. ∗P < 0.05 vs. control.
Bach1 Inhibitors Suppress RANKL-Mediated Osteoclastogenesis
We next examined whether Bach1 inhibitors exhibited an inhibitory effect on RANKL-dependent osteoclastogenesis. RANKL (100 ng/ml) stimulation of RAW264.7 cells gave a high number of TRAP-positive multinucleated cells, compared with the control (Figures 3A,B). Even at 400 nM, HPP-A and HPP-B had no effect on the number of TRAP-positive multinucleated cells (Figures 3C,D), but they decreased following treatment with HPP-C, and HPP-E (Figures 3E,F). The size and the number of nuclear in the multi-nucleated cells seemed to be reduced by the treatment of HPPs. The comparison at lower concentration (80 nM) clearly demonstrated the stronger inhibition of osteoclastogenesis of HPP-E as compared to HPP-C. Among them, HPP-E resulted in a decrease compared to controls (Figure 3G). These results indicated that HPP-E strongly inhibits osteoclast differentiation in RAW264.7 cells in vitro.
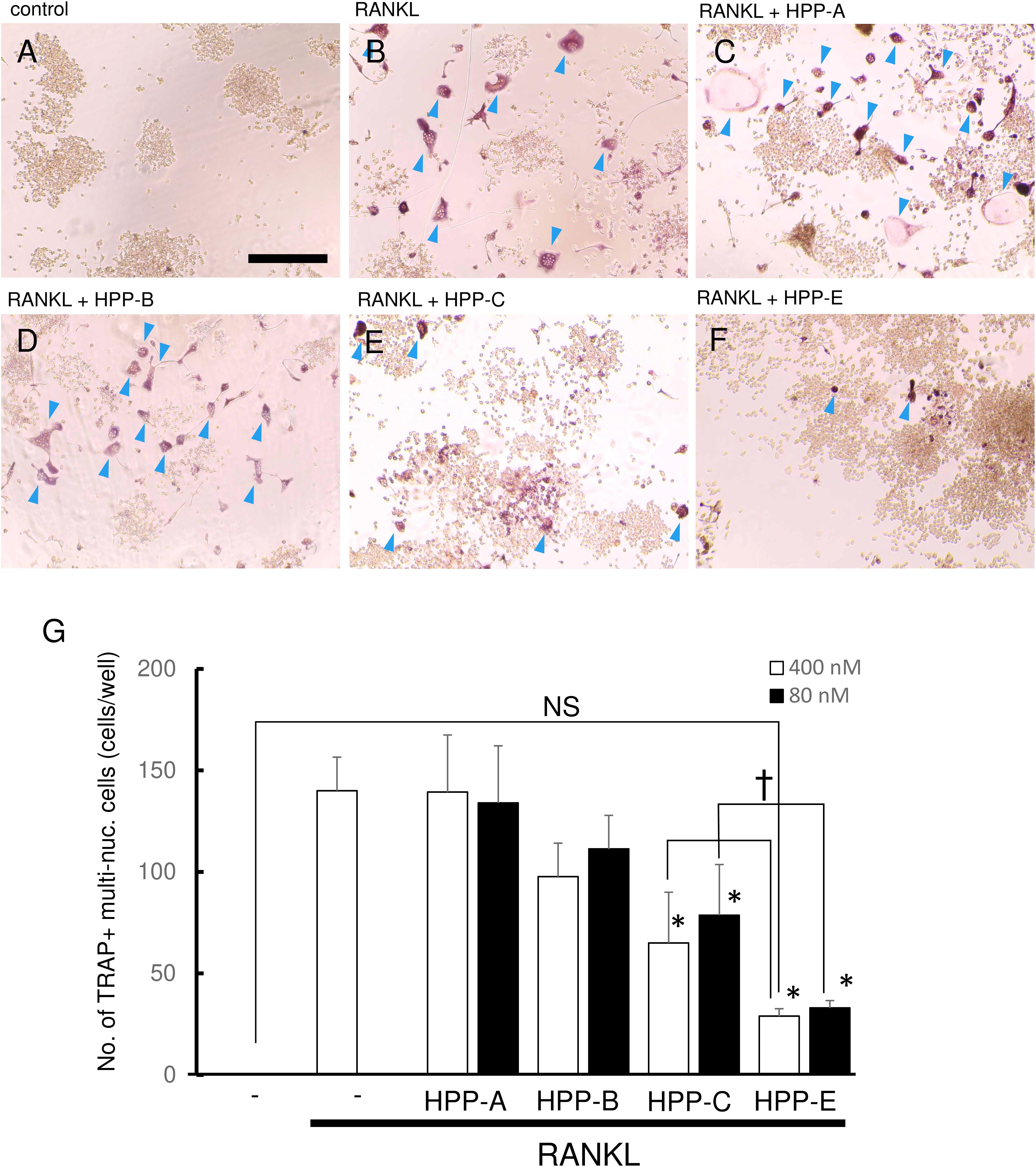
Figure 3. Bach1 inhibitors attenuate RANKL-mediated osteoclastogenesis. Representative photographs of TRAP staining are shown of randomly selected field: (A) control, (B) RANKL (100 ng/ml), (C) RANKL (100 ng/ml) + HPP-A (400 nM), (D) RANKL (100 ng/ml) + HPP-B (400 nM), (E) RANKL (100 ng/ml) + HPP-C (400 nM), and (F) RANKL (100 ng/ml) + HPP-E (400 nM). Arrowhead indicates TRAP-positive multinucleated cells. Scale bars; 100 μm. (G) Mean number of TRAP-positive multi-nucleated cells differentiated from RAW 264.7 cells at 400 nM (open bar) and 80 nM (close bar). The data shown are representative of three independent experiments performed in triplicate. ∗P < 0.05 vs. RANKL alone. †P < 0.05 between the groups. NS: no significant difference compared with the control.
Bach1 Inhibitors Suppress Osteoclast Function
To further examine the inhibitory effect of Bach1 inhibitors on osteoclastogenesis, the expression of several osteoclast differentiation marker genes in RAW264.7 cells was examined using real-time RT-PCR. RANKL induced the expression of osteoclast marker genes, which, in turn, was suppressed by HPP-B, HPP-C, and HPP-E (Figure 4). HPP-A showed weak suppression of these marker genes, with no significant differences in Atp6v0d2, Mmp9, and Oscar.
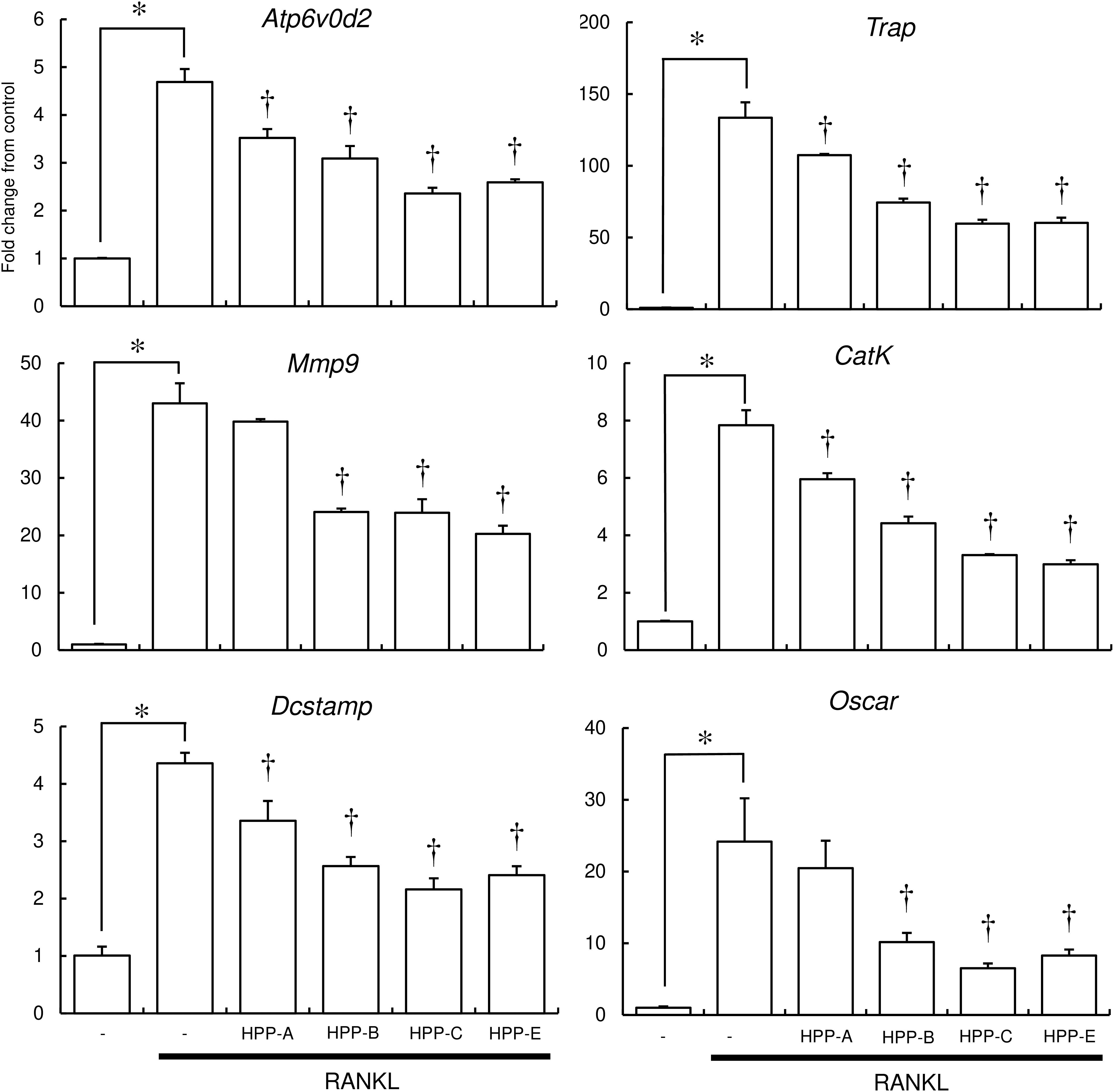
Figure 4. Real-time PCR analysis of osteoclast marker gene expression. The expression of osteoclast marker genes (Atp6v0d2, Trap, Mmp9, Cathepsin K, Dcstamp, and Oscar) in RANKL-stimulated RAW 264.7 cells were examined by real-time PCR. Gene expression was calibrated using the Rps18 house-keeping gene, and values indicate the fold-change compared with control. Data shown are representative of three independent experiments performed in triplicate. ∗P < 0.05 vs. control. †P < 0.05 vs. RANKL alone.
Next, resorption activity was examined using the bone resorption assay plate (Figure 5). RANKL stimulation of RAW264.7 cells induced numerous resorption areas on the substrate (Figure 5B), and HPP-C and HPP-E reduced the resorption areas (Figures 5E–G). These results suggested that HPP-E inhibited not only osteoclastogenesis, but also osteoclast activity.
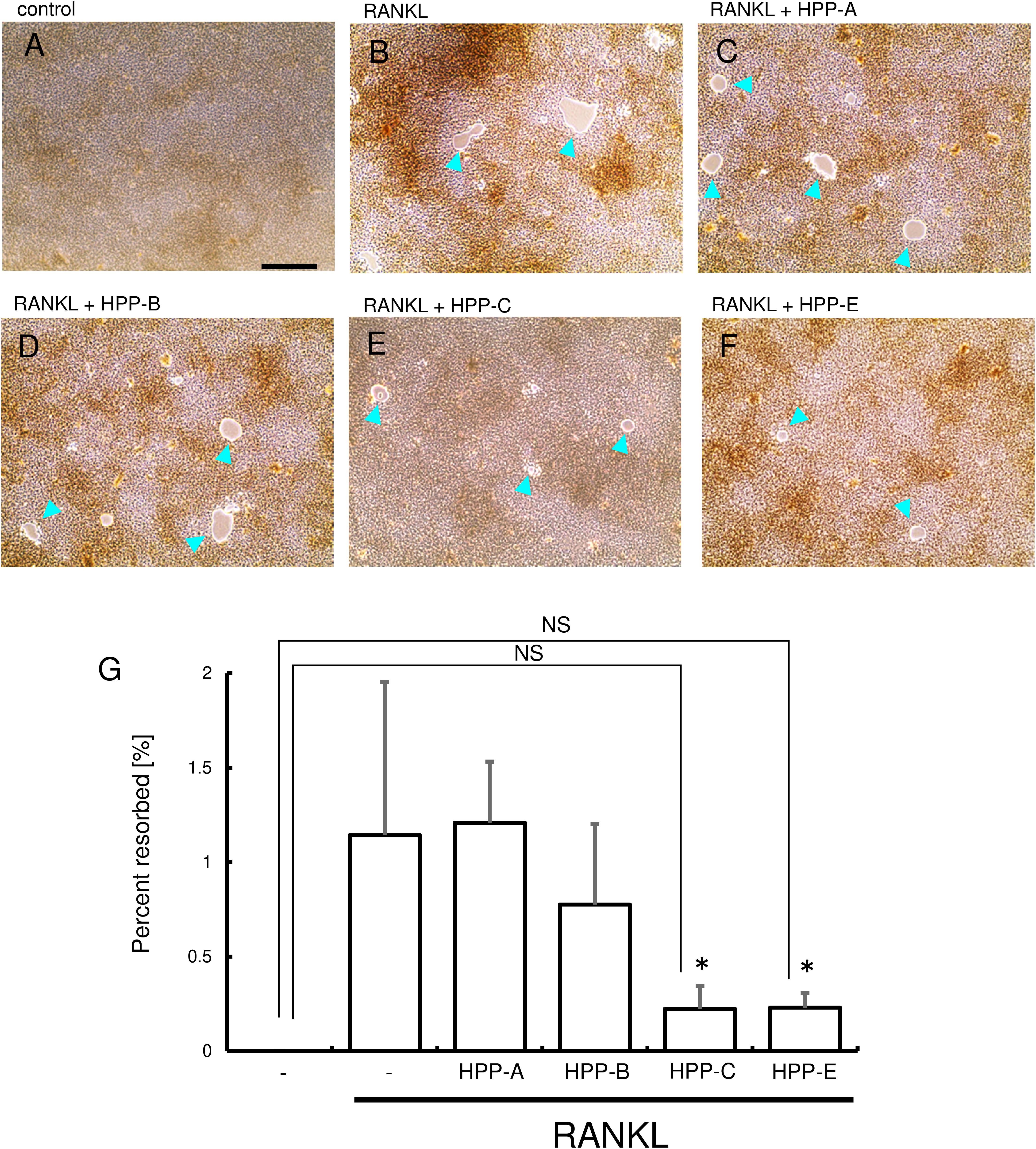
Figure 5. Resorption assay. RAW 264.7 cells were treated with Bach1 inhibitors following stimulation with RANKL (100 ng/mL). Representative photographs are shown of randomly selected fields: (A) control, (B) RANKL (100 ng/mL), (C) RANKL (100 ng/mL) + HPP-A (400 nM), (D) RANKL (100 ng/mL) + HPP-B (400 nM), (E) RANKL (100 ng/mL) + HPP-C (400 nM), and (F) RANKL (100 ng/mL) + HPP-E (400 nM). Arrowhead indicates resorbed area. Scale bars; 100 μm. (G) Percentage resorbed area is shown. The data shown are representative of three independent experiments performed in triplicate. ∗P < 0.05 vs. RANKL alone. NS: no significant difference against the control.
Bach1 Inhibitor Increases Nuclear Nrf2
Based on the results of TRAP staining, real-time RT-PCR, and the bone resorption assay, HPP-E exhibited the most potent inhibition of osteoclastogenesis. Therefore, we examined the molecular mechanism of HPP-E inhibition on osteoclastic signaling. Western blotting using nuclear extracts of RAW264.7 cells clearly demonstrated that treatment of RAW264.7 cells with HPP-E led to nuclear translocation of Nrf2 (Figure 6A). Relative band intensity of Nrf2 calibrated by Histone H3 was almost twice by HPP-E treatment.
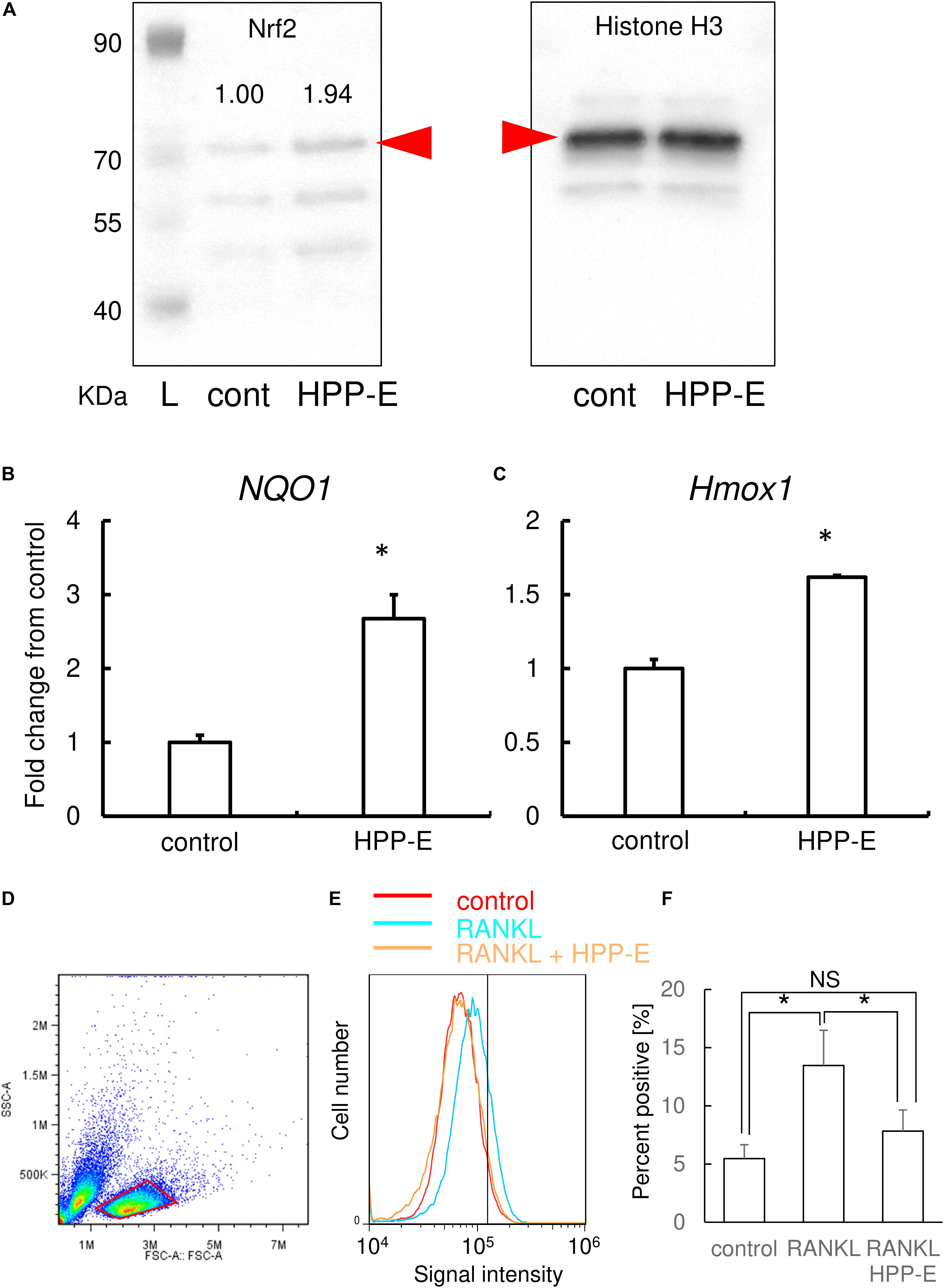
Figure 6. HPP-E induces Nrf2 nuclear translocation, anti-oxidation, and thereby ROS elimination. (A) Representative images of western blot analysis of nuclear protein for Nrf2 (the left side panel) and histone H3 (the right side panel) from three independent experiments are shown. Relative band density of Nrf2 normalized by Histone H3 are shown above the each band. Red arrowhead indicates the predicted molecular weight. L: molecular weight markers. Gene expression for NQO1 (B) and HMOX1 (C) are shown. Gene expression was calibrated using the Rps18 house-keeping gene, and values indicate the fold-change compared with control. The data shown are representative of three independent experiments performed in triplicate. ∗P < 0.05 vs. control. (D) The viable cellular fraction of monocytes/macrophage was gated on a forward scatter/side scatter plot (red area). (E) Representative results of intracellular ROS levels in control (red), RANKL-treated (blue), and RANKL- and HPP-E-treated (orange) RAW 264.7 cells from three independent experiments are shown. The vertical line indicates a conventional threshold for ROS-negative and -positive populations. (F) Mean percent of ROS-positive RAW 264.7 cells in each groups. ∗ P < 0.05 versus control.
Bach1 Inhibition Augments the Expression of Antioxidant Enzymes
To further examine the effect of HPP-E on the antioxidant response, we examined the expression of antioxidant enzyme genes, such as Nqo1 and Hmox1. The expression of these genes were increased by HPP-E in RAW264.7 cells (Figures 6B,C). This result indicated that HPP-E substantially induces an antioxidant response in RAW264.7 cells.
Bach1 Inhibition Attenuates RANKL-Mediated Intracellular ROS
Next, we investigated whether HPP-E could interfere with RANKL-triggered intracellular ROS production in RAW264.7 cells. Scatter plot of RAW 264.7 cells gave two populations (Figure 6D). Previously, we compared two population from the point of RANKL-dependent intracellular ROS signaling, and found that the right side population which we set the gate in the present study exhibited responsive ROS signaling, though the left side population exhibited less response (data not shown). Therefore, we set the gate on the right side population in the present study. Stimulation of RAW264.7 cells with RANKL increased intracellular production of superoxide, as detected using BES-So-AM. HPP-E treatment inhibited RANKL-mediated intracellular ROS augmentation (Figures 6E,F), indicating that HPP-E attenuated RANKL signaling by decreasing superoxide production.
Local Injection of Bach1 Inhibitor Ameliorates RANKL-Dependent Bone Destruction in Mice
Finally, we tested whether local HPP-E injection can ameliorate LPS-mediated bone destruction in mice calvaria. Repeated LPS injection resulted in bone destruction, as compared with the control group (Figures 7A,C). Furthermore, injection of HPP-E had no effect on bone destruction (Figure 7B). μCT imaging of resorbed areas in calvaria clearly demonstrated that the local HPP-E injection ameliorated LPS-mediated bone destruction (Figures 7C,D). We measured resorbed areas and found that HPP-E almost completely inhibited LPS-mediated bone destruction (Figure 7E). These results suggested that HPP-E is a potential inhibitor against bone destruction.
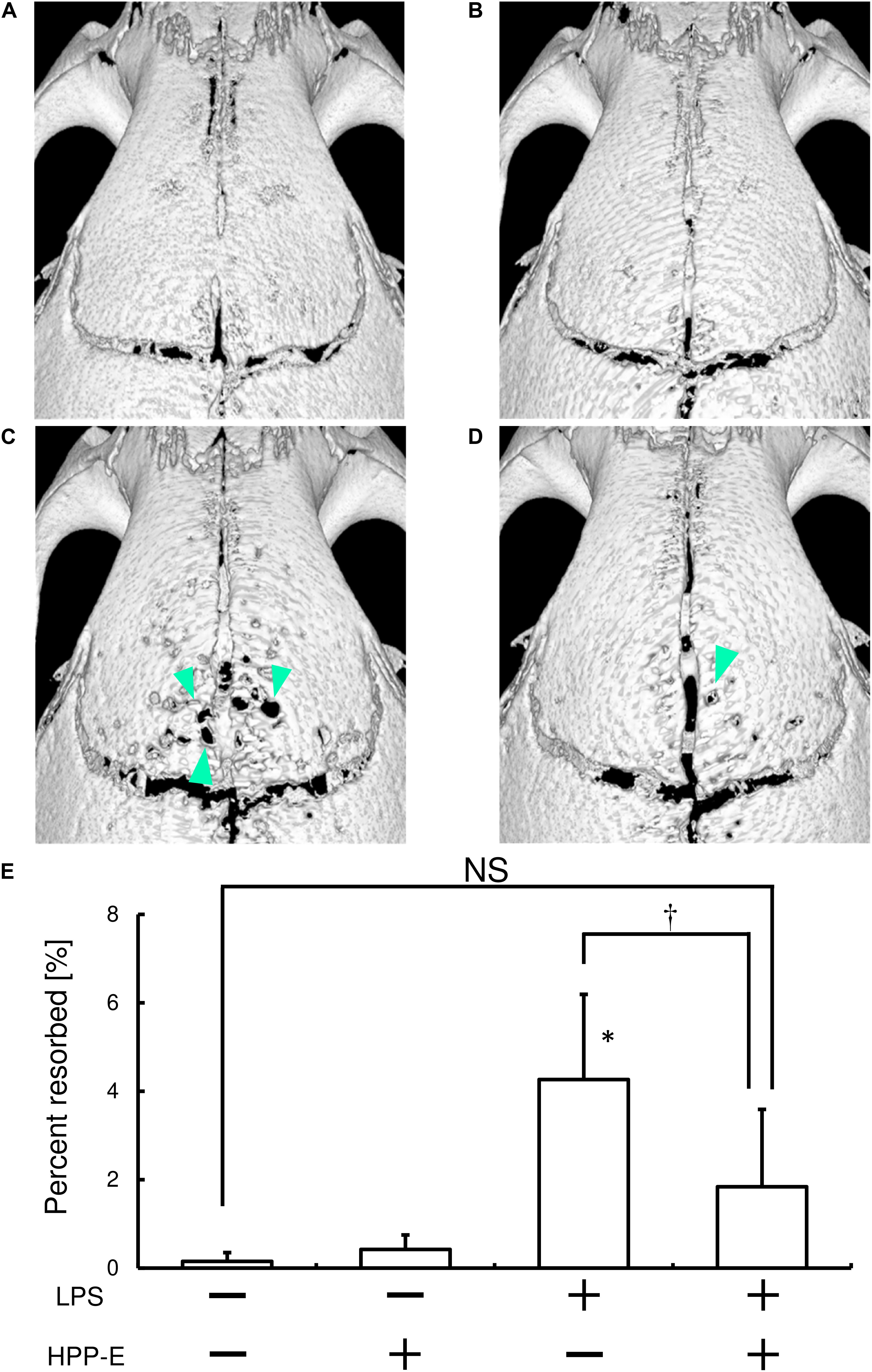
Figure 7. Local HPP-E injection ameliorates LPS-induced bone destruction in mice. Representative μCT images of control (A), HPP-E (B), LPS (C), and LPS+HPP-E treated mice (D) are shown. (E) Percentage of resorbed area in the cranial bone. NS: no significant difference between the groups. ∗P < 0.05 vs. control. †P < 0.05 between the groups.
Discussion
In the present study, we demonstrate for the first time that Bach1 inhibition augmented Nrf2 activation, which resulted in the inhibition of osteoclastogenesis. Bach1 inhibitors increased expression of cytoprotective enzymes, such as HMOX1 and NQO1, and reduced RANKL-mediated ROS generation in RAW 264.7 cells. Furthermore, an in vivo mouse bone destruction model demonstrated that a Bach1 inhibitor suppressed LPS-mediated bone destruction. These results indicated that Bach1 inhibitors would be useful for bone destruction diseases like osteoporosis, periodontitis, and rheumatoid arthritis. As to the experimental animal model, we utilized LPS-mediated bone destruction, which induces upregulation of inflammatory cytokine, but also augments RANKL expression (Narimiya et al., 2019). We presumed the animal model in the present study is sufficient to observe the effect of HPP-E on RANKL-mediated osteoclastogenesis in vivo.
RANKL-mediated osteoclastogenesis uses ROS for intracellular signaling (Ha et al., 2004), which signifying that the interference against intracellular ROS would be possible anti-osteoclastogenic activity. Indeed, we reported that the activation of anti-oxidation via activation of Nrf2-mediated anti-oxidative enzymes clearly inhibited osteoclastogenesis (Kanzaki et al., 2013, 2014, 2015, 2017; Katsumata et al., 2018; Yamaguchi et al., 2018). Another groups also reported anti-oxidant Quercetin exhibited relevant anti-oxidant properties by high radical scavenging activity, and inhibited osteoclastogenesis (Forte et al., 2017). Taken together, the interference against intracellular ROS can be an anti-osteoclastogenic activity.
Reactive oxygen species causes several diseases by cytotoxic effects, such as peroxidation of lipids and oxidative damage to protein and DNA (Esterbauer et al., 1991; Wells et al., 2009). Cells have several protective mechanisms against this oxidative stress (Kensler et al., 2007). A major one is the transcriptional factor Nrf2, which induces antioxidant enzymes, such as HMOX1, NQO1, and GCS (Favreau and Pickett, 1991; Wild et al., 1998; Alam et al., 1999), making activation of Nrf2 a promising molecular drug target for several diseases (Kwon et al., 2007; Eggler et al., 2008). We previously reported that RANKL induced the nuclear translocation of Bach1 and decreased nuclear Nrf2, attenuated Nrf2-mediated expression of antioxidant enzymes, and augmented osteoclastogenesis (Kanzaki et al., 2017).
Bach1 is a member of the BTB and CNC transcriptional regulator family that binds to ARE sequences as heterodimeric complexes with small Maf proteins and suppresses antioxidant enzyme genes by binding to their ARE sequences (Oyake et al., 1996). Therefore Bach1 and Nrf2 compete with each other to occupy ARE sequences (Suzuki et al., 2003). A previous study demonstrated that the Bach1 inhibitor, HPP-4382, increased Nrf2-imediated antioxidant enzyme genes, such as HMOX1 and NQO1 in normal human lung fibroblasts (Attucks et al., 2014). HPPs were able to modulate the transcriptional repression of Bach1 of ARE genes by binding of HPPs to Bach1. We previously also reported that induction of nuclear export of Bach1 by the combination of ALA and SFC increased nuclear transport of Nrf2, which augmented antioxidant enzyme genes. Furthermore, we reported that the induction of nuclear export of Bach1 augmented nuclear translocation of Nrf2 detected by immunofluorescent analysis and western blotting using nuclear protein samples. Bach1 inhibition by ALA/SFC augmented anti-oxidation and inhibited RANKL-mediated osteoclastogenesis from primary peritoneal macrophages. Consistent with these reports, our present study also demonstrated that a Bach1 inhibitor, HPP-E, increased the expression of HMOX1 and NQO1 in RAW 264.7 cells. Inflammatory stimulation has been reported to induce Bach1 expression, which promotes attenuation of HMOX1 in leukemic cells (Miyazaki et al., 2010). Furthermore, Bach1-deficient mouse bone marrow-derived macrophages were resistant to RANKL-dependent HMOX1 reduction and impaired osteoclastogenesis (Hama et al., 2012). These findings concur with our observations that Bach1 inhibitors increase the expression of HMOX1 and NQO1 in osteoclast precursors, attenuate RANKL-mediated intracellular ROS signaling, and thereby inhibit osteoclastogenesis. Further confirmatory experiments using primary macrophages are necessary whether Bach1 inhibition by HPPs inhibit osteoclastogenesis.
Various molecules can activate Nrf2 (Jana and Mandlekar, 2009; Nakamura and Miyoshi, 2010; Dao et al., 2015), with dimethyl fumarate (DMF) being one of the most well-known (Talalay et al., 1988; Nelson et al., 1999; Lehmann et al., 2007; Ooi et al., 2011; Yore et al., 2011). Kelch-like ECH-associated protein 1 (Keap1) negatively regulates Nrf2-dependent transcription of cytoprotective enzymes by inhibiting nuclear translocation of Nrf2. DMF protects Nrf2 from ubiquitination and degradation triggered by Keap1 via alkylation of Keap1, which subsequently activates the Nrf2-mediated gene expression. We previously reported that DMF also reduced RANKL-mediated intracellular ROS generation, which resulted in the inhibition of osteoclastogenesis (Yamaguchi et al., 2018). Our present study demonstrated that some HPPs exhibited cytotoxicity except for HPP-E. Anti-osteoclastogenic activity of HPP-A, -B, and -C might partly by this cytotoxicity. HPPs have the same core structure but each HPPs exhibited different property from the point of anti-osteoclastogenic activity and cytotoxicity. We are now investigating how the difference of the side chain structures affect anti-osteoclastogenic activity and cytotoxicity. We previously reported that the cytotoxicity of DMF for RAW 264.7 cells were seen at 100 μM, though HPPs-A, -B, and -C exhibited cytotoxicity even at 400 nM. Further investigations are required to directly compare Bach1 inhibitors and DMF in their effect on osteoclastogenesis.
As to the possible affected stage by HPPs during osteoclastogenesis, we presumed the early stage would be affected due to the reduction of the size and nuclear in the multi-nucleated cells under HPPs treatment, and the reduction of DC-STAMP, which is known as cell-cell fusion molecule during osteoclastogenesis (Kukita et al., 2004), were significantly reduced by HPPs, especially by HPP-E.
Figure 8 summarizes our interpretative competitive regulatory mechanism by Bach1 and Nrf2 during osteoclastogenesis. RANKL stimulation gives rise to induce nuclear translocation of Bach1, which antagonizes to Nrf2 and excludes nuclear Nrf2, promoting osteoclastogenesis by downregulating the anti-oxidation. Bach1 inhibitor promotes nuclear export of Bach1, and induces Nrf2 nuclear accumulation, which augments anti-oxidation that results in the inhibition of osteoclastogenesis.
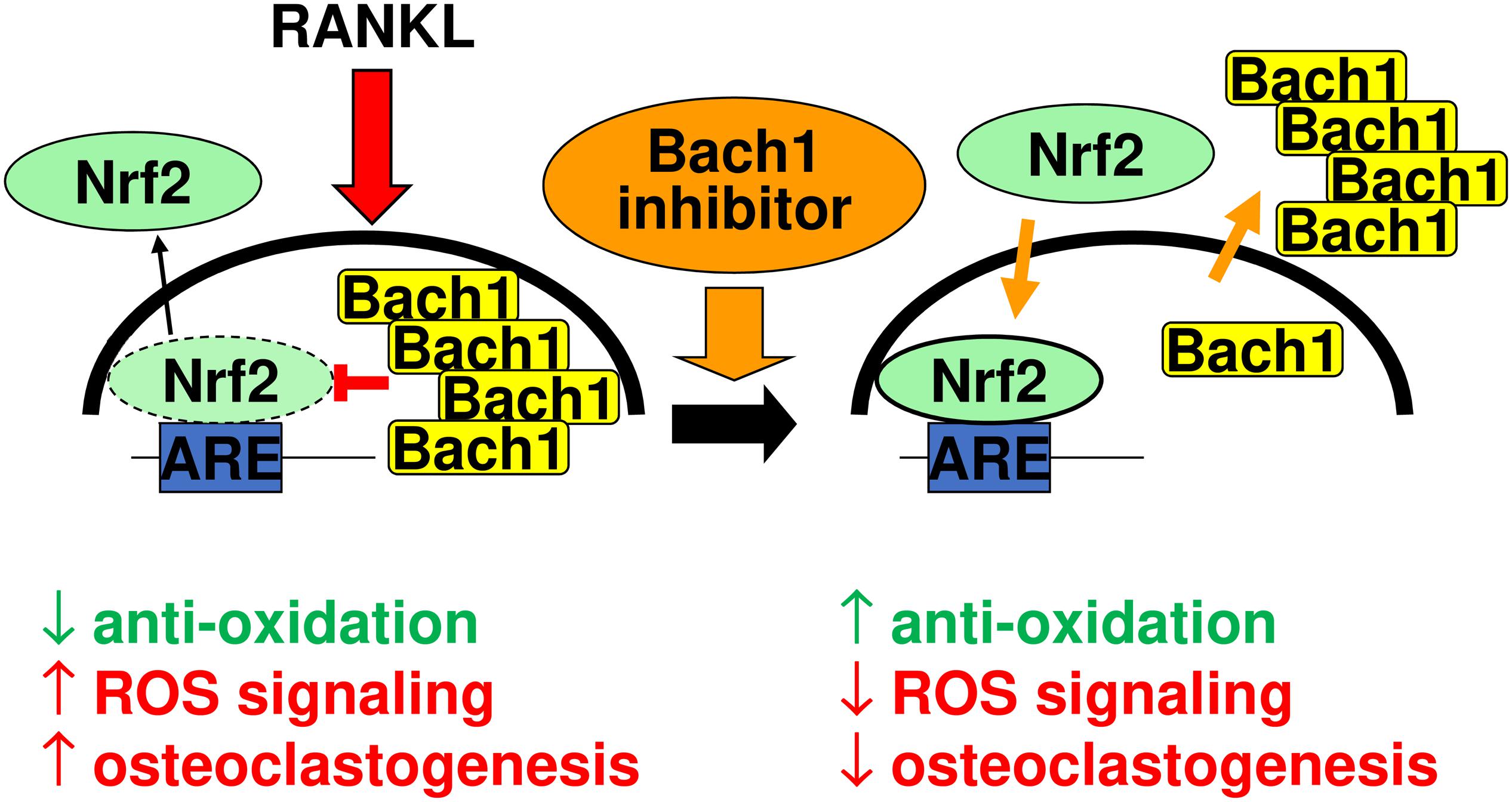
Figure 8. Interpretative competitive regulatory mechanism by Bach1 and Nrf2 during osteoclastogenesis. RANKL stimulation gives rise to induce nuclear translocation of Bach1, which antagonizes to Nrf2 and exclude nuclear Nrf2, promoting osteoclastogenesis by downregulating the anti-oxidation. Bach1 inhibitor promotes nuclear export of Bach1, and induces Nrf2 nuclear accumulation, which augments anti-oxidation that results in the inhibition of osteoclastogenesis.
In conclusion, we have demonstrated that Bach1 inhibitor suppressed RANKL-mediated osteoclastogenesis and bone destruction via attenuation of intracellular ROS signaling by augmenting antioxidant enzymes. Therefore, Bach1 inhibitors have potential utility in bone destructive diseases, such as osteoporosis, periodontitis, and rheumatoid arthritis.
Data Availability Statement
The datasets generated for this study are available on request to the corresponding author.
Ethics Statement
The animal study was reviewed and approved by the Institutional Animal Care and Use Committee, Tsurumi University (approval number; 28A030).
Author Contributions
SW and HK wrote the main manuscript text. OA, YN, and HT reviewed and modified the manuscript text. SW, YK, YY, and TN performed the experiments. SW, HK, and OA prepared the figures. All authors reviewed the manuscript.
Funding
This work was supported in part by the NEXT KAKENHI grant numbers JP23689081, JP25670841, JP15K11376, and JP15K11356.
Conflict of Interest
OA was employed by company vTv Therapeutics LLC.
The remaining authors declare that the research was conducted in the absence of any commercial or financial relationships that could be construed as a potential conflict of interest.
References
Alam, J., Stewart, D., Touchard, C., Boinapally, S., Choi, A. M., and Cook, J. L. (1999). Nrf2, a Cap‘n’Collar transcription factor, regulates induction of the heme oxygenase-1 gene. J. Biol. Chem. 274, 26071–26078. doi: 10.1074/jbc.274.37.26071
Attucks, O. C., Jasmer, K. J., Hannink, M., Kassis, J., Zhong, Z., Gupta, S., et al. (2014). Induction of heme oxygenase I (HMOX1) by HPP-4382: a novel modulator of Bach1 activity. PLoS One 9:e101044. doi: 10.1371/journal.pone.0101044
Bax, B. E., Alam, A. S., Banerji, B., Bax, C. M., Bevis, P. J., Stevens, C. R., et al. (1992). Stimulation of osteoclastic bone resorption by hydrogen peroxide. Biochem. Biophys. Res. Commun. 183, 1153–1158. doi: 10.1016/s0006-291x(05)80311-0
Dao, V. T., Casas, A. I., Maghzal, G. J., Seredenina, T., Kaludercic, N., Robledinos-Anton, N., et al. (2015). Pharmacology and clinical drug candidates in redox medicine. Antioxid. Redox Signal. 23, 1113–1129. doi: 10.1089/ars.2015.6430
Dhakshinamoorthy, S., Jain, A. K., Bloom, D. A., and Jaiswal, A. K. (2005). Bach1 competes with Nrf2 leading to negative regulation of the antioxidant response element (ARE)-mediated NAD(P)H:quinone oxidoreductase 1 gene expression and induction in response to antioxidants. J. Biol. Chem. 280, 16891–16900. doi: 10.1074/jbc.m500166200
Eggler, A. L., Gay, K. A., and Mesecar, A. D. (2008). Molecular mechanisms of natural products in chemoprevention: induction of cytoprotective enzymes by Nrf2. Mol. Nutr. Food Res. 52(Suppl), 1, S84–S94.
Esterbauer, H., Schaur, R. J., and Zollner, H. (1991). Chemistry and biochemistry of 4-hydroxynonenal, malonaldehyde and related aldehydes. Free Radic. Biol. Med. 11, 81–128. doi: 10.1016/0891-5849(91)90192-6
Favreau, L. V., and Pickett, C. B. (1991). Transcriptional regulation of the rat NAD(P)H:quinone reductase gene. Identification of regulatory elements controlling basal level expression and inducible expression by planar aromatic compounds and phenolic antioxidants. J. Biol. Chem. 266, 4556–4561.
Forte, L., Torricelli, P., Boanini, E., Rubini, K., Fini, M., and Bigi, A. (2017). Quercetin and alendronate multi-functionalized materials as tools to hinder oxidative stress damage. J. Biomed. Mater. Res. Part A 105, 3293–3303. doi: 10.1002/jbm.a.36192
Ha, H., Kwak, H. B., Lee, S. W., Jin, H. M., Kim, H. M., Kim, H. H., et al. (2004). Reactive oxygen species mediate RANK signaling in osteoclasts. Exp. Cell Res. 301, 119–127. doi: 10.1016/j.yexcr.2004.07.035
Hama, M., Kirino, Y., Takeno, M., Takase, K., Miyazaki, T., Yoshimi, R., et al. (2012). Bach1 regulates osteoclastogenesis in a mouse model via both heme oxygenase 1-dependent and heme oxygenase 1-independent pathways. Arthrit. Rheum. 64, 1518–1528. doi: 10.1002/art.33497
Harada, S., and Rodan, G. A. (2003). Control of osteoblast function and regulation of bone mass. Nature 423, 349–355. doi: 10.1038/nature01660
Hyeon, S., Lee, H., Yang, Y., and Jeong, W. (2013). Nrf2 deficiency induces oxidative stress and promotes RANKL-induced osteoclast differentiation. Free Radic. Biol. Med. 65, 789–799. doi: 10.1016/j.freeradbiomed.2013.08.005
Jana, S., and Mandlekar, S. (2009). Role of phase II drug metabolizing enzymes in cancer chemoprevention. Curr. Drug Metab. 10, 595–616. doi: 10.2174/138920009789375379
Kanzaki, H., Shinohara, F., Itohiya, K., Yamaguchi, Y., Katsumata, Y., Matsuzawa, M., et al. (2017). RANKL induces Bach1 nuclear import and attenuates Nrf2-mediated antioxidant enzymes, thereby augmenting intracellular reactive oxygen species signaling and osteoclastogenesis in mice. FASEB J. 31, 781–792. doi: 10.1096/fj.201600826r
Kanzaki, H., Shinohara, F., Itohiya-Kasuya, K., Ishikawa, M., and Nakamura, Y. (2015). Nrf2 activation attenuates both orthodontic tooth movement and relapse. J. Dent. Res. 94, 787–794. doi: 10.1177/0022034515577814
Kanzaki, H., Shinohara, F., Kajiya, M., Fukaya, S., Miyamoto, Y., and Nakamura, Y. (2014). Nuclear Nrf2 induction by protein transduction attenuates osteoclastogenesis. Free Radic. Biol. Med. 77, 239–248. doi: 10.1016/j.freeradbiomed.2014.09.006
Kanzaki, H., Shinohara, F., Kajiya, M., and Kodama, T. (2013). The Keap1/Nrf2 protein axis plays a role in osteoclast differentiation by regulating intracellular reactive oxygen species signaling. J. Biol. Chem. 288, 23009–23020. doi: 10.1074/jbc.m113.478545
Katsumata, Y., Kanzaki, H., Honda, Y., Tanaka, T., Yamaguchi, Y., Itohiya, K., et al. (2018). Single local injection of epigallocatechin gallate-modified gelatin attenuates bone resorption and orthodontic tooth movement in mice. Polymers 10:1384. doi: 10.3390/polym10121384
Kensler, T. W., Wakabayashi, N., and Biswal, S. (2007). Cell survival responses to environmental stresses via the Keap1-Nrf2-ARE pathway. Annu. Rev. Pharmacol. Toxicol. 47, 89–116. doi: 10.1146/annurev.pharmtox.46.120604.141046
Kukita, T., Wada, N., Kukita, A., Kakimoto, T., Sandra, F., Toh, K., et al. (2004). RANKL-induced DC-STAMP is essential for osteoclastogenesis. J. Exp. Med. 200, 941–946. doi: 10.1084/jem.20040518
Kwon, K. H., Barve, A., Yu, S., Huang, M. T., and Kong, A. N. (2007). Cancer chemoprevention by phytochemicals: potential molecular targets, biomarkers and animal models. Acta Pharmacol. Sin. 28, 1409–1421. doi: 10.1111/j.1745-7254.2007.00694.x
Lai, T. H., Shieh, J. M., Tsou, C. J., and Wu, W. B. (2015). Gold nanoparticles induce heme oxygenase-1 expression through Nrf2 activation and Bach1 export in human vascular endothelial cells. Int. J. Nanomed. 10, 5925– 5939.
Lehmann, J. C., Listopad, J. J., Rentzsch, C. U., Igney, F. H., von Bonin, A., Hennekes, H. H., et al. (2007). Dimethylfumarate induces immunosuppression via glutathione depletion and subsequent induction of heme oxygenase 1. J. Invest. Dermatol. 127, 835–845. doi: 10.1038/sj.jid.5700686
Liu, D., Duan, X., Dong, D., Bai, C., Li, X., Sun, G., et al. (2013). Activation of the Nrf2 pathway by inorganic arsenic in human hepatocytes and the role of transcriptional repressor Bach1. Oxid. Med. Cell. Longev. 2013: 984546.
Miyazaki, T., Kirino, Y., Takeno, M., Samukawa, S., Hama, M., Tanaka, M., et al. (2010). Expression of heme oxygenase-1 in human leukemic cells and its regulation by transcriptional repressor Bach1. Cancer Sci. 101, 1409–1416. doi: 10.1111/j.1349-7006.2010.01550.x
Nakamura, Y., and Miyoshi, N. (2010). Electrophiles in foods: the current status of isothiocyanates and their chemical biology. Biosci. Biotechnol. Biochem. 74, 242–255. doi: 10.1271/bbb.90731
Narimiya, T., Kanzaki, H., Yamaguchi, Y., Wada, S., Katsumata, Y., Tanaka, K., et al. (2019). Nrf2 activation in osteoblasts suppresses osteoclastogenesis via inhibiting IL-6 expression. Bone Rep. 11:100228. doi: 10.1016/j.bonr.2019.100228
Nelson, K. C., Carlson, J. L., Newman, M. L., Sternberg, P. Jr., Jones, D. P., Kavanagh, T. J., et al. (1999). Effect of dietary inducer dimethylfumarate on glutathione in cultured human retinal pigment epithelial cells. Invest. Ophthalmol. Vis. Sci. 40, 1927–1935.
Ooi, A., Wong, J. C., Petillo, D., Roossien, D., Perrier-Trudova, V., Whitten, D., et al. (2011). An antioxidant response phenotype shared between hereditary and sporadic type 2 papillary renal cell carcinoma. Cancer Cell 20, 511–523. doi: 10.1016/j.ccr.2011.08.024
Oyake, T., Itoh, K., Motohashi, H., Hayashi, N., Hoshino, H., Nishizawa, M., et al. (1996). Bach proteins belong to a novel family of BTB-basic leucine zipper transcription factors that interact with MafK and regulate transcription through the NF-E2 site. Mol. Cell. Biol. 16, 6083–6095. doi: 10.1128/mcb.16.11.6083
Sakamoto, H., Sakai, E., Fumimoto, R., Yamaguchi, Y., Fukuma, Y., Nishishita, K., et al. (2012). Deltamethrin inhibits osteoclast differentiation via regulation of heme oxygenase-1 and NFATc1. Toxicol. In Vitro 26, 817–822. doi: 10.1016/j.tiv.2012.05.005
Suzuki, H., Tashiro, S., Sun, J., Doi, H., Satomi, S., and Igarashi, K. (2003). Cadmium induces nuclear export of Bach1, a transcriptional repressor of heme oxygenase-1 gene. J. Biol. Chem. 278, 49246–49253. doi: 10.1074/jbc.m306764200
Takayanagi, H. (2007). Osteoimmunology: shared mechanisms and crosstalk between the immune and bone systems. Nat. Rev. Immunol. 7, 292–304. doi: 10.1038/nri2062
Talalay, P., Long, M.J. De, and Prochaska, H. J. (1988). Identification of a common chemical signal regulating the induction of enzymes that protect against chemical carcinogenesis. Proc. Natl. Acad. Sci. U.S.A. 85, 8261–8265. doi: 10.1073/pnas.85.21.8261
Taubman, M. A., Valverde, P., Han, X., and Kawai, T. (2005). Immune response: the key to bone resorption in periodontal disease. J. Periodontol. 76(Suppl 11), 2033–2041. doi: 10.1902/jop.2005.76.11-s.2033
Thimmulappa, R. K., Mai, K. H., Srisuma, S., Kensler, T. W., Yamamoto, M., and Biswal, S. (2002). Identification of Nrf2-regulated genes induced by the chemopreventive agent sulforaphane by oligonucleotide microarray. Cancer Res. 62, 5196–5203.
Wells, P. G., McCallum, G. P., Chen, C. S., Henderson, J. T., Lee, C. J., Perstin, J., et al. (2009). Oxidative stress in developmental origins of disease: teratogenesis, neurodevelopmental deficits, and cancer. Toxicol. Sci. 108, 4–18. doi: 10.1093/toxsci/kfn263
Wild, A. C., Gipp, J. J., and Mulcahy, T. (1998). Overlapping antioxidant response element and PMA response element sequences mediate basal and beta-naphthoflavone-induced expression of the human gamma-glutamylcysteine synthetase catalytic subunit gene. Biochem. J. 332(Pt 2), 373–381. doi: 10.1042/bj3320373
Yamaguchi, Y., Kanzaki, H., Katsumata, Y., Itohiya, K., Fukaya, S., Miyamoto, Y., et al. (2018). Dimethyl fumarate inhibits osteoclasts via attenuation of reactive oxygen species signalling by augmented antioxidation. J. Cell Mol. Med. 22, 1138–1147.
Yore, M. M., Kettenbach, A. N., Sporn, M. B., Gerber, S. A., and Liby, K. T. (2011). Proteomic analysis shows synthetic oleanane triterpenoid binds to mTOR. PLoS One 6:e22862. doi: 10.1371/journal.pone.0022862
Keywords: Bach1, osteoclastogenesis, ROS, antioxidant enzymes, Nrf2
Citation: Wada S, Kanzaki H, Katsumata Y, Yamaguchi Y, Narimiya T, Attucks OC, Nakamura Y and Tomonari H (2020) Bach1 Inhibition Suppresses Osteoclastogenesis via Reduction of the Signaling via Reactive Oxygen Species by Reinforced Antioxidation. Front. Cell Dev. Biol. 8:740. doi: 10.3389/fcell.2020.00740
Received: 07 May 2020; Accepted: 16 July 2020;
Published: 04 August 2020.
Edited by:
Claudia Fiorillo, University of Florence, ItalyReviewed by:
Teun J. De Vries, Academic Centre for Dentistry Amsterdam, VU University Amsterdam, NetherlandsHelen Knowles, University of Oxford, United Kingdom
Raj Gopalakrishnan, University of Minnesota, United States
Copyright © 2020 Wada, Kanzaki, Katsumata, Yamaguchi, Narimiya, Attucks, Nakamura and Tomonari. This is an open-access article distributed under the terms of the Creative Commons Attribution License (CC BY). The use, distribution or reproduction in other forums is permitted, provided the original author(s) and the copyright owner(s) are credited and that the original publication in this journal is cited, in accordance with accepted academic practice. No use, distribution or reproduction is permitted which does not comply with these terms.
*Correspondence: Hiroyuki Kanzaki, kanzaki-h@tsurumi-u.ac.jp