- 1Institute of Fundamental Medicine and Biology, Kazan Federal University, Kazan, Russia
- 2Nottingham Breast Cancer Research Centre, Division of Cancer and Stem Cells, School of Medicine, University of Nottingham Biodiscovery Institute, Nottingham, United Kingdom
Cytokine-based immunotherapy is a promising field in the cancer treatment, since cytokines, as proteins of the immune system, are able to modulate the host immune response toward cancer cell, as well as directly induce tumor cell death. Since a low dose monotherapy with some cytokines has no significant therapeutic results and a high dose treatment leads to a number of side effects caused by the pleiotropic effect of cytokines, the problem of understanding the influence of cytokines on the immune cells involved in the pro- and anti-tumor immune response remains a pressing one. Immune system cells carry CD makers on their surface which can be used to identify various populations of cells of the immune system that play different roles in pro- and anti-tumor immune responses. This review discusses the functions and specific CD markers of various immune cell populations which are reported to participate in the regulation of the immune response against the tumor. The results of research studies and clinical trials investigating the effect of cytokine therapy on the regulation of immune cell populations and their surface markers are also discussed. Current trends in the development of cancer immunotherapy, as well as the role of cytokines in combination with other therapeutic agents, are also discussed.
Immune System and Cancer
The immune system is a complex system consisting of immune cells, compliment proteins and cytokines which provide communication and coordinated cell functions. The coordinated functioning of the immune system allows the maintenance of the body’s homeostasis, eliminating foreign antigens or proteins (Eyileten et al., 2016). The immune system can protect the body against virus-induced tumors by limiting or terminating viral infection. Also, the well-timed elimination of pathogens by the immune system and the rapid termination of inflammation prevents the formation of inflammatory sites which are favorable for tumor development. Finally, the immune system can identify and eliminate tumor cells relying on the recognition of tumor-specific antigens. This process of identifying transformed cells and eliminating them before the tumor forms is referred to as immunological surveillance (Terme and Tanchot, 2017). However, recent discoveries in human immunology also confirm the involvement of immune cells in the maintenance of tumor development and growth (Duan et al., 2014).
Depending on speed and specificity of the immune response innate and adoptive immune responses can be identified. Innate immunity is assumed to be rapid but non-specific response mediated by phagocytes (neutrophils, monocytes, and macrophages), natural killer (NK) cells and complement system (Liu and Zeng, 2012). On the contrary, adaptive immune response takes time to mature naive lymphocytes, T- and B cells, into effector T-cells and secreting B cells (Chaplin, 2010).
It is known that innate and adoptive immune responses are involved in surveying tumor development which can be divided into three stages: elimination, equilibrium, and escape (Dunn et al., 2004; O’Sullivan et al., 2012). With elimination, the innate immune system is able to identify and destroy a nascent tumor using a number of inflammatory cells and signaling molecules (Ricciardi et al., 2015). However, if the tumor cell with a rare and aggressive mutation survives at the elimination stage, the process proceeds to the next stage of equilibrium in which excessive growth of the tumor is prevented by immunological mechanisms. At this second equilibrium stage, the tumor cells are not completely destroyed, but the tumor growth is controlled by the immune system (Ricciardi et al., 2015). The equilibrium stage is the longest of the three stages and can last up to 20 years, from the initial transforming event to the clinical manifestation of the tumor (Miliotou and Papadopoulou, 2018). The escape stage occurs when tumor cells which have survived both elimination and equilibrium become resistant to immunological surveillance allowing the tumor to grow and become clinically detectable (Dunn et al., 2004).
Tumors inherently are characterized by low immunogenicity since they arise from the body’s normal cells. The tumor microenvironment (TME), which in addition to the tumor cells contains a large number of other cell populations (Chulpanova et al., 2018b; Kitaeva et al., 2019) inhibits the tumor killing capacities of immune cells (Borst et al., 2018). Thus, there is a close relationship between the tumor and immune system cells, which is the key to understanding the processes that lead to the elimination of the tumor or its progression. The majority of immune system cells and signaling molecules involved in immunological surveillance will be comprehensively discussed in this review. Understanding the role of immune cells expressing specific surface markers typical for cell populations with pro- or anti-tumor properties, and their identification will allow researchers to develop new methods for predicting tumor progression as well as to identify new targets and possible immune system modulation mechanisms for optimal drug selection. In particular, the success of cytokine-based cancer therapies in modulating various populations of immune system cells and the prospects for the further development of this approach for cancer therapy will be discussed.
NK Cells
Natural killer cells are important participants in the antitumor immunity, as they detect the major histocompatibility complex (MHC) presented on the surface of all nuclear cells of the body. NK cells secrete perforins and granzymes to induce apoptosis of cells that have abnormal or altered MHCI expression (Brodbeck et al., 2014). Typically, NK cells express CD56, in the absence of CD3 (T-cell receptor). Depending on CD56 surface-density expression NK cells can be divided into two populations – CD56bright (or CD56high) and CD56dim (or CD56low) NK cells (Poli et al., 2009).
CD56low NK cells, which also have high expression of CD16 (CD16high), exhibit cytotoxic function and contain large amounts of perforin (Angelo et al., 2015). CD56high CD16± NK cells are characterized by low perforin levels and mainly specialize in the production of cytokines, predominately IFN-γ, which is necessary for the maturation of dendritic cells (DCs) (Stabile et al., 2017). TME can significantly affect population distribution and the function of tumor-infiltrating NK cells (TINKs). For example, a high number of CD56high perforinlow NK cells are observed in breast and lung cancers compared with normal tissues. High accumulation of CD56high perforinlow NK cells is associated with the secretion of specific chemokine (C-X-C motif) ligand 9 (CXCL9) and CXCL10, which support the migration of non-cytotoxic CD56high NK cells in TME (Carrega et al., 2014). The population of CD56high NK cells also prevails among patients within breast, melanoma, colon cancer (Levi et al., 2015), non-small lung cancer and has a pro-angiogenic effect, thereby promoting tumor growth (Bruno et al., 2013). However, CD56low NK cells found in the lymph nodes infiltrated with tumor cells were highly cytotoxic against autologous melanoma (Ali et al., 2014). Probably, tumor-related soluble factors [e.g., interleukin (IL)10, indoleamine-pyrrole 2,3-dioxygenase (IDO), prostaglandin E2 (PGE2)] and TME cells are responsible for phenotypic and functional changes in NK cells (Stabile et al., 2017) and help tumors to recruit NK cells.
Unlike B and T-cells, NK cells do not undergo gene rearrangements to generate the repertoire of cell surface receptors. Instead, they use germline-encoded inhibiting and activating receptors (Carrillo-Bustamante et al., 2016). NK cells possess the ability to distinguish between normal and transformed cells based on the expression of MHCI on the cell surface. MHCI molecules, which are largely expressed in normal cells, bind to the inhibitory receptors on the surface of NK cells, which leads to NK cell inactivation. In addition to aberrant MHCI expression, transformed cells also acquire stress-induced ligands for activating NK cell receptors (Caligiuri, 2008). The most important activating NK cell receptors are natural cytotoxicity receptors (NKp46, NKp30, and NKp44), C-type lectin natural killer group 2D receptor (NKG2D), DNAX accessory molecule 1 (DNAM1) and immunoglobulin-like killer receptors (KIR2DS and KIR3DS) (Martinet and Smyth, 2015). Inhibitory receptors that can bind to human leukocyte antigen (HLA) class I (HLA-I) or HLA-I-like molecules include two different classes: immunoglobulin-like killer receptors (KIR2DL and KIR3DL) and C-type lectin receptors NKG2A/B (Campbell and Purdy, 2011).
In order to avoid an NK cell mediated immune response, tumor cells secrete various immunosuppressive factors that regulate the expression or functional activity of NK cell receptors. For example, the binding of proliferating cell nuclear antigen (PCNA) to the NKp44 receptor leads to activation of the constitutively inactive immunoreceptor tyrosine-based inhibition motif (ITIM) in the cytoplasmic domain of the receptor, which inhibits the cytotoxic function of NK cells (Rosental et al., 2011). Transforming growth factor-β (TGF-β) and IL10 produced by tumor cells and immune cells of TME can inhibit NKG2D expression (Schiavoni et al., 2013). Other TME participants, tumor-associated fibroblasts, can also inhibit the expression of NKp44, NKp30, and DNAM-1 receptors due to PGE2 secretion, which suppress the antitumor activity of NK cells (Balsamo et al., 2009). As expected, the reduced expression of activating receptors, in particular NKG2D, NKp30, NKp46, DNAM1, is associated with poor prognosis in patients with pancreatic cancer, gastric cancer, colorectal cancer and melanoma (Peng et al., 2013; Mirjacic Martinovic et al., 2014). Whilst the increased expression of inhibitory receptors KIR2DL1 and KIR2DL2/3 negatively correlates with the cytotoxicity of NK cells and enhances the melanoma progression (Naumova et al., 2007). Overexpression of the NKG2A inhibitory receptor is also associated with poor prognosis in patients with breast and colorectal cancer (Balsamo et al., 2009).
Neutrophils
Neutrophils, polymorphonuclear and granulocytic cells, consist approximately 50–70% of the total immune cell population and act as the first responders to infection and injury (Singel and Segal, 2016). Neutrophils mature in the bone marrow and enter the bloodstream as terminally differentiated cells, carrying CD11b, CD66b, CD16, Ly6G, C-X-C chemokine receptor type 2 (CXCR2) on their surface (Coffelt et al., 2016; Lakschevitz et al., 2016). The role of neutrophils in tumor immunity has long remained unclear, since it was thought that neutrophil lifespan is too short to influence cancer progression. However, neutrophil half-life increases from 7 h in normal conditions to 17 h in cancer (Ocana et al., 2017) which may allow them to further contribute to tumor progression. The effect of neutrophils on tumor progression remains controversial, and N1/N2 nomenclature has been proposed, where N1 are neutrophils that support tumor progression, whilst N2 are those that suppress tumor progression (Fridlender et al., 2009). However, this nomenclature seems to be too simplified and there is not enough information regarding different functions and CD markers to identify N1/N2 neutrophils. A previous study declares that neutrophils can lead to tumor regression (Stoppacciaro et al., 1993). However, most of the information suggests that neutrophils promote tumor growth, mainly by the stimulation of angiogenesis (Nozawa et al., 2006; Jablonska et al., 2010). Neutrophils are also able to suppress the immune response against tumor cells including the secretion of nitric oxide synthase (iNOS), or arginase 1 (ARG1) which suppress CD8+ T lymphocyte antitumor response (Fridlender et al., 2009). It is also worth noting that several studies have shown neutrophils to have a pro-tumor effect, while in others no effect has been found (Granot et al., 2011; Li Z. et al., 2012).
Macrophages
Monocytes originate from circulating peripheral blood monocytes and play an important role in maintaining homeostasis (Wynn et al., 2013). Macrophages are traditionally classified into M1 and M2 macrophages depending on their function in the immune system (Mills et al., 2000). Classically activated macrophages (M1) protect the body from external pathogens and destroy tumor cells by secreting the pro-inflammatory cytokines IL6, IL12, IL23, and tumor necrosis factor-α (TNF-α), as well as releasing reactive oxygen/nitrogen species (Atri et al., 2018). Phenotypically, M1 express CD68, CD80, CD86, CD206low, and HLA-II DR isotype (HLA-DR)high (Li W. et al., 2012; Bertani et al., 2017).
The alternatively activated macrophages (M2) are involved in the resolution of inflammation by producing anti-inflammatory IL10 and TGF-β (Aras and Zaidi, 2017). M2 macrophages are positive for CD163 (Hu et al., 2017), CD68, CD206, negative for CD80 and have a low level of HLA-DR expression (Bertani et al., 2017). However, it is worth noting that such a classification is regarded as too simplified, and now the M1< –…– >M2 spectrum concept is considered to be more preferable. According to this concept, macrophages do not differentiate strictly into stable M1 and M2 subsets, but form complex mixed phenotypes depending on the combination of received external stimuli [discussed in detail in Murray et al. (2014)].
Tumor-associated macrophages (TAMs) are close to M2-polarized type. The cells of tumor and TME secrete CCL2 and macrophage colony-stimulating factor (M-CSF) (Qian et al., 2011) and attract a large number of inflammatory monocytes to tumor sites where they differentiate into TAMs due to secretion of IL4, IL10, and IL13 (Wang and Joyce, 2010; Chuang et al., 2016). TAMs can be identified by CD163, CD200R, CD204, CD206, and HLA-DRlow expression (Yang et al., 2015; Cope et al., 2016; Kubota et al., 2017). The recruited macrophages represent a significant part of the TME and provide significant support to the tumor. TAMs secrete pro-angiogenic factors, such as vascular endothelial growth factor (VEGF) family members, and provoke neovascularization and lymphovascularisation of the tumor (Linde et al., 2012; Werchau et al., 2012). The secretion of matrix metalloproteinases (MMPs) and cathepsins supports tumor cell invasion and metastasis (Gocheva et al., 2010; He et al., 2016). TAMs are also able to inhibit the CD8+ T-cell immune response through direct interaction with T-cells, for example, using programmed cell death 1 ligand 1 (PD-L1) (Kubota et al., 2017) or by secretion of immunosuppressive molecules, such as IL10, TGF-β, ARG1, and PGEs (Kubota et al., 2017; Takahashi et al., 2017).
Gamma-Delta (γδ) T-Cells
T lymphocytes can be divided into two subtypes: αβ and γδ T-cells. αβ T-cells represent up to 95% of the CD3+ cell population and recognize the antigen presented on MHCI or MHCII (Zou et al., 2017) (since αβ T-cells is a larger population, we will use “T-cells” to denote αβ T lymphocytes). The γδ T-cells form the remaining T lymphocyte population, but do not need conventional MHC-dependent antigen presentation (Tanaka et al., 1995). Most often, γδ T-cells are classified by the type of variable chains (γ and δ) in the T-cell receptor (TCR). The vast majority of γδ T lymphocytes (75%) in peripheral-blood express Vδ2 chain and co-express Vγ9 chain (Dimova et al., 2015). Such Vγ9Vδ2 T-cells are able to directly lyse tumor cells due to the secretion of perforin/granzymes (Mattarollo et al., 2007). They can also induce apoptosis of tumor cells through the TNF-related apoptosis-inducing ligand (TRAIL)/TRAIL receptor (TRAILR) system (Zou et al., 2017). Such cytotoxic Vγ9Vδ2 T-cells can be identified by increased NKG2D (natural killer group 2D) (Todaro et al., 2009), CD56 (Alexander et al., 2008), and CD16 expression (Lafont et al., 2001). However, Vγ9Vδ2 T-cells may also have antigen-presenting cell-like activity, which is accompanied by the expression of CD86, CD80, CD40, and MHCII (Pang et al., 2012). Other T-cells that have a Vδ1 chain prevail in tissues and are able to recognize malignant epithelial cells (Maeurer et al., 1996). Both subgroups express CD6 (Zhou et al., 2012). Both subgroups can also be classified by the expression of CD45RA and CD27 on CD45RA+ CD27+ naïve, CD45RA– CD27+ central memory, CD45RA– CD27– effector memory and CD45RA+ CD27– effector memory T cells [for more information see Pang et al. (2012)]. However, in TME, under the influence of secreted factors, γδ T-cells can be polarized; i.e., can shift from one phenotype to another, in forkhead box P3 (FOXP3)+ regulatory γδ T-cells (γδ Tregs) which display regulatory/immunosuppressive activity (Casetti et al., 2009) or in CD30+ γδ T17 cells, which secrete large amounts of IL17 and can provide the accumulation of immunosuppressive cells in the tumor and stimulate angiogenesis (Wu et al., 2014; Patil et al., 2016).
T-Cells
T-cells with αβ TCR are the main participants in the adaptive immune response and are usually classified by polarization (functional subtypes) or degree of differentiation (naïve, activated, memory) (Geginat et al., 2014). T-cells can be grouped into functional subsets depending on the expression of CD markers. In the process of maturation in the thymus, T-cells acquire CD4 or CD8 markers (Kurd and Robey, 2016). CD4+ T-cells (helper T-cells) are divided into different subsets: Th (T helper) 1, Th2, Th9, Th17, Th22, Tregs (regulatory T cells), and Tfh (follicular helper T-cells), which are characterized by different CD expression profiles and their functions in the immune system (Raphael et al., 2015). Th1 can enhance the priming and expansion of CD8 T-cells or inhibit angiogenesis in IFN-γ-dependent manner (Qin and Blankenstein, 2000; Chraa et al., 2019). Most Th1 cells are CXCR3+ and CCR4–, and also express CCR2, CCR5 and CXCR6 in the activated state (Kim et al., 2001) (activation and memory states are discussed below).
The effect of Th2 on tumor growth is not so clear. IL10, expressed by Th2, inhibits the processing and presentation of antigens by DCs (Steinbrink et al., 2002) and also activates Tregs (Levings et al., 2002). At the same time, another Th2 cytokine IL4 increases tumor infiltration with eosinophils and macrophages that can kill tumor cells (Tepper et al., 1992). Almost all Th2 cells are CCR4+ CXCR3– (Kim et al., 2001), and can also express CCR3 (Sallusto et al., 1997) and CCR8 on their surface (Zingoni et al., 1998).
Th17 cells are found in a large number of different tumors, but their effect remains controversial (Asadzadeh et al., 2017). On one hand, Th17 cells are able to secrete various cytokines and chemokines, such as IL17, IL23, CCL20 which promote tumor growth (Tartour et al., 1999; Langowski et al., 2006). On the other hand, IL17 supports the recruitment of NK cells (Lv et al., 2011) and stimulates the production of cytokines by stromal cells, which finally results in the recruitment and activation of neutrophils (Witowski et al., 2000). The interaction of Th17 cells and the tumor is comprehensively discussed in Asadzadeh et al. (2017). Basically, Th17 cells are determined by the CCR6+ marker, then two populations of CCR6+ Th17 cells can de distinguished. CCR6+ CCR4+ Th17 cells produce more IL17 as well as IL22 (Acosta-Rodriguez et al., 2007b) and are able to suppress the activity of CD8+ T-cells (Greten et al., 2012). Another population, CCR6+ CXCR3+ Th17 cells, produce less IL17, but also synthesizes IFN-γ (Acosta-Rodriguez et al., 2007b). Th17 cells have also increased expression of IL1, IL6, and IL23 receptors, which are necessary for their differentiation (Acosta-Rodriguez et al., 2007a; Chung et al., 2009).
Tfh are characterized by expression of CD28, CD40L, CXCR5 (or CD185), inducible T-cell costimulator (ICOS), and programmed cell-death protein 1 (PD-1) (Deenick and Ma, 2011; Ame-Thomas et al., 2012) which appear during the process of Tfh differentiation after stimulation by DCs. Tfh cells seem to support antitumor immunity (Jia et al., 2015). Their main function is to promote B cells differentiation into antibody-secreting cells in secondary lymphoid organs (Kim and Cantor, 2014). Tfh cells also secrete IL21, which can stimulate CD8+ T-cells (Shi W. et al., 2018). However, Tfh can stimulate growth and survival of some lymphoid tumors. For example, these cells can promote the proliferation of chronic lymphocytic leukemia (CLL) from secondary lymphoid tissue (Pascutti et al., 2013), support follicular lymphoma (FL) (Ame-Thomas et al., 2012).
Tregs are one of the most common T-cell phenotypes in TME (20–30%) (Quezada et al., 2006). They can be detected by the expression of CD25 and FOXP3 transcription factor (Sharma et al., 2005). Tumor cells recruit Tregs from lymphoid organs (Malchow et al., 2013) by the expression of specific chemokines such as CCL2 or CCL21 (Curiel et al., 2004; Shields et al., 2010). Tregs in turn provide suppression of antitumor immunity through the increased expression of PD-1 and cytotoxic T-lymphocyte (CTL)-associated antigen 4 (CTLA-4) (Zappasodi et al., 2018). A number of studies have also been shown that CD39+ and/or CD73+ Tregs have higher immunosuppressive properties due to the synthesis of adenosine (Borsellino et al., 2007; Deaglio et al., 2007).
Th9 cells are another T-cell population that produces a large amount of IL9 and plays an important role in the antitumor immune response (Rivera Vargas et al., 2017). Th9 cells can both directly lyse tumor cells due to the secretion of granzyme B (GrzmB) (Purwar et al., 2012), and induce TRAIL-mediated apoptosis (Fang et al., 2015). Indirect antitumor activity is mediated by the regulation of DCs and CD8+ T-cells which is discussed in detail in Rivera Vargas et al. (2017). Th9 cells express functional CCR3, CCR6, CXCR3 (Kara et al., 2013), and IL17B receptors as well as secrete IL4, TGF-β essential for their differentiation (Veldhoen et al., 2008; Angkasekwinai et al., 2010). Also, since IL1β can induce secretion of IL9 in Th9, thereby increasing antitumor activity (Vegran et al., 2014), it can be concluded that IL1β receptors have been also presented on the surface of Th9.
CD8+ T-cells are effector group of T-cells that can kill tumor cells by granule exocytosis and Fas ligand (FasL) (CD95)-mediated apoptosis (Farhood et al., 2019). Activation of naïve CD8+ T-cells begins with the binding of CD3 on the T-cell surface with the MHCI-protein complex on the surface of antigen presenting cell (APC). In addition, CD28 replaced on the surface of CD8+ T-cell recognizes co-stimulatory B7 proteins (CD80 and CD86) of APC (usually DCs), and CXCR3 expressed in T-cell binds to CXCL9 and CXCL10 chemokines produced by DC (Spranger and Gajewski, 2018). Having bonded with DCs, CD8+ T-cells begin to express lymphocyte-function-associated protein 1 (LFA-1) adhesion molecules to enhance binding (Semmrich et al., 2005). After the antigen presentation, CD8+ T-cells begin to secrete IL2 and also express its receptor (CD25), thus stimulating proliferation by themselves (Cheng et al., 2002). Following IL2 stimulation, activated CD8+ T-cells begin to express lysosomal-associated membrane protein 1 (LAMP-1 or CD107a) which is related to T-cell cytotoxicity (Aktas et al., 2009). Hours or days after activation, CD8+ T-cells start expressing PD-1 and CTLA-4, which can form part of a mechanism by which cancer cells would suppress immune responses (Topalian et al., 2016). The relationships of CD8+ T-cells and tumors is discussed in detail in Farhood et al. (2019).
Another approach to classify T-cells is by the degree of differentiation. In the process of the differentiation mature, naïve carrying TCR T-cells emerge from the thymus and are able to differentiate into effector and memory cells (Restifo and Gattinoni, 2013). At different stages of differentiation, T-cells carry many different markers on their surface. From the perspective of this article we consider surface markers that are essential for CD-based identification of T-cell populations in vitro, but provide links to articles that address these issues in more detail. Naïve T-cells (CD45RA+ CD45RO–) carry on their surface CD27, CD28 which are essential for interaction with APC (Chen and Flies, 2013). Naïve T-cells are also CCR7+ and CD62L+ (l-selectin), which ensures their ability to migrate toward secondary lymphoid organs (Picker et al., 1993; Campbell et al., 2001). After antigen presentation, T-cells acquire an effector phenotype (activation) and migrate to the sites of tumor localization. Activated T-cells lose almost all CD markers of naïve T-cells and start de novo expressing CD95, which provides either co-stimulating or pro-apoptotic signals (Siegel et al., 2000). Other markers also appear during the activation process, the earliest activation markers (12 h) are CD69 and CD25, the α subunit of the IL2 receptor (Salgado et al., 2002). Expression of CD38 and HLA-DR is associated with late (1 day and 3–5 days, respectively) activation and proliferation of subset of mature T-cells (Amlot et al., 1996; Sandoval-Montes and Santos-Argumedo, 2005). CD134, also known as tumor necrosis factor receptor superfamily, member 4 (TNFRSF4 or OX40), is a specific marker of CD4+ T-cells activation, which increases their survival (Ladanyi et al., 2004). CD137, also called 4-1BB, stimulates survival and enhances the cytotoxic function of CD8+ T-cells which infiltrate the tumor (Zhu and Chen, 2014). Isolated from the tumor site CD137+ T cells can inhibit tumor cell growth in vivo (Ye et al., 2014).
T-cells can also differentiate into memory cells, which provide a quick immune response upon reinfection (Mahnke et al., 2013) and also contribute to the antitumor immunity. Traditionally, the classification into different memory populations takes place in the context of CD8+ T-cells. Memory cells can be located in the secondary lymphoid organs (central memory cells, TCM) or in recently infected tissues – effector memory cells, TEM cells (Opata and Stephens, 2013). TEM cells (CD45RA–, CD45RO+, CD62L–, CCR7–) provide a more rapid cytotoxic response during infections than TCM (CD45RA–, CD45RO+, CD62L+, CCR7+) (Mahnke et al., 2013). However, in the context of a tumor, TCM can more effectively suppress tumor growth compared to TEM (Klebanoff et al., 2005). Another population of memory cells is resident memory T-cells (TRM) (CD103+, CD69+, CD49a+, CD62L–, CCR7–), which are located in various tissues of the body (Cheuk et al., 2017; Reading et al., 2018). Although their role in antitumor immunity is not fully understood, an increased number of CD8+ CD103+ T-cells are associated with prolonged patient survival (Dumauthioz et al., 2018). There are several different theories, which phenotype, effector or memory, appears first, the relationships between effector cells and memory cells which are comprehensively discussed in Restifo and Gattinoni (2013).
NKT-Cells
Natural Killer T-cells are a group of cells that play an important role to link the innate and adaptive immunity since they have the characteristics of both conventional T-cells and NK cells (Kumar et al., 2017). NKT-cells mature in the thymus and acquire specific TCRs that can recognize lipid, but not protein, molecules represented by MHCI-like CD1d molecules (Metelitsa et al., 2001). Usually, NKT-cells are classified in two large groups depending on the structure of TCR chains and its ability to bind to lipid molecules. Type I NKT-cells bind to a common lipid prototype, α-galactosylceramide (α-GalCer) (Horikoshi et al., 2012). Since these cells express many typical NK cell and T-cell markers on their surface, fluorescent dye-conjugated α-GalCer lipid tetramers that will selectively bind to TCR are primarly used to detect NKT-cells (Kawano et al., 1997). Antibodies to specific fragments of TCR chains, such as Vα24 (especially clone 6B11) and Vβ11 are also used to identify these cells (Montoya et al., 2007). After interaction with α-GalCer type I NKT-cells activate (Kawano et al., 1997) and acquire typical activation markers, such as CD69 (Kitayama et al., 2016), CD38 (Chan et al., 2013), CD25 (Chan et al., 2013). Activated type I NKT-cells are capable of killing CD1d+ tumor cells in a CD1d-dependent manner (Ni et al., 2015). However, they will most likely mediate antitumor activity through activation of downstream immune effector cells. Secretion of large amounts of IFN-γ leads to the formation of tumor-specific CD8+ CTLs (Nishimura et al., 2000) and rapid activation of NK cells (Ishihara et al., 2000; Escriba-Garcia et al., 2017). A noteworthy detail is that type I NKT-cells express a variable number of CD4 and CD8 receptors depending on the donor. For example, neonatal NKT-cells are predominantly (>90%) CD4+, whereas adult peripheral blood NKT-cells are either CD4+ or CD4–. The same can be said about typical markers of NK cells, CD56 and CD161, which are expressed on NKT-cells in varying numbers. For example CD4– cells express CD56 in 17–70% of cases and have a more significant cytotoxic function in the activated condition (Eger et al., 2006). However, the anti-cancer effect of the individual NKT-cell populations has not yet been investigated.
Another large group of NKT-cells is type II NKT-cells which do not have any specific receptors and do not respond to α-GalCer (Dasgupta and Kumar, 2016), but can recognize many other lipids, including sulfatides and lipopolysaccharides (LPS) (Chang et al., 2008; Blomqvist et al., 2009). For lack of any specific markers, it is sulfatide-loaded CD1d multimers with a fluorescent label that are often used to identify these cells (Zhang et al., 2011). The antitumor function of these cells is ambiguous, where most studies indicate that type II NKT-cells support tumor immunosurveillance by secreting IL13 (Terabe et al., 2003; Ambrosino et al., 2007; Fichtner-Feigl et al., 2008). However, Th1-like type II NKT cells are also able to suppress tumor growth due to IFN-γ secretion (Zhao et al., 2014).
In general, NKT-cells play an important role in the antitumor immune response, but their identification and clustering using surface markers is rather difficult due to small amount of cumulative knowledge.
B Cells
Despite the fact a lot of attention is paid to the role of T-cells in the antitumor immune response, B cells play an equally important role in carcinogenesis and tumor progression (Tsou et al., 2016). B cells mature in the bone marrow and enter the peripheral blood as transitional B cells, which carry typical B cell markers CD19 and CD20 as well as are CD5+, CD38high, CD27– (LeBien and Tedder, 2008). This population of B cells can differentiate into follicular (FO), marginal zone (MZ), and regulatory B cells (Bregs) (Clavarino et al., 2016). FO B cells (CD19+, CD20+, CD21+, CD22+, CD23+, CD24+, CD10–, CD27–) can activates after stimulation by antigen and differentiate into effector cells (Sarvaria et al., 2017). After binding to the antigen, B cells bind to Th1 cells via the MHC-peptide complex, B7 and CD40 on the surface of B cells (Watanabe et al., 2017). Such activated B cells (CD19+, CD20+, CD25+, CD27+, CD30+, CD69+, CD80+, CD88+) can become short-lived plasma cells (CD19low, CD20–, CD27+, CD38high, CD69+, CD138+), that secrete specific antibodies or form germinal centers (GCs) in lymph nodes and the spleen (De Silva and Klein, 2015). GCs are temporary formations in which activated B cells (CD10+, CD19+, CD20+, CD27–, CD33+, CD38high) continue their maturation and can develop into long-lived memory cells (CD19+, CD20+, CD27+, CD38–, CD40+, CD23low).
Another significant B cell population is MZ B cells (CD1c+, CD19+, CD20–, CD21high, CD27var), which recognize T-cell-independent carbohydrate and phospholipid antigens and produce multireactive IgM antibodies (Descatoire et al., 2014), providing a rapid response to T-cell- independent antigens.
Tumor-infiltrating B cells can have both pro- and antitumor effects (Yuen et al., 2016). B cells are capable of producing antibodies that target tumor intracellular antigens, for example, against aberrantly exposed β-actin (Hansen et al., 2001) or p53 (Kumar et al., 2009), the presence of the antibodies correlates with a favorable outcome of the disease. B cells can also act as APCs and stimulate the T-cell-mediated immune response (Bruno et al., 2017) or directly induce TRAIL/Apo-2L-mediated death of tumor cells (Kemp et al., 2004). However, B cells can also contribute to tumor progression due to the secretion of lymphotoxin (Ammirante et al., 2010) and Bregs, whose functions are discussed below.
The immunophenotype and functions of another B cell population have been studied more widely. Bregs seem to evolve from transitional B cells, however, it is suggested that mature B cells and plasmoblasts also have the ability to differentiate into IL10-producing Bregs (Rosser and Mauri, 2015). The function of IL10-producing CD1dhigh CD5+ B cells, that suppress anti-tumor immunity by stimulating the development of Tregs (Liu and Zeng, 2012) and suppressing CD8+ T-cells is best described (Wei et al., 2016). The same properties to suppress CD4+ T-cell proliferation and effector function have been described for CD19+ CD24high CD38high Bregs (Bouaziz et al., 2010; Flores-Borja et al., 2013; Zhang et al., 2017). The various Bregs populations and their functions are described in Sarvaria et al. (2017). The role of B-cells in antitumor immunity is Janus-faced, as well as the one of T-cells, although they have not been investigated as intensively as T-cells. Apart from Bregs, pro- and antitumor properties are mainly determined for general CD19+ CD20+ B cell population, therefore, the role of each individual B cell population in the tumor immune response remains to be investigated.
Dendritic Cells
The presentation of the antigen to the cells of an adaptive immunity is an essential for providing the antitumor immune response. Conventional population of DCs is one of the main sources of processed tumor antigens for T-cells (Gardner and Ruffell, 2016). DCs can be divided into two populations, DC1 and DC2, which develop depending on the activation of different transcription factors (Murphy et al., 2016). Both of these populations express CD11c and MHCII, with DC1 expressing X-C motif chemokine receptor 1 (XCR1) and dendritic cell NK lectin group receptor-1 [DNGR-1, also called C-type lectin domain family 9 member A (CLEC9A)] and CD141 (blood dendritic cell antigen 3, BDCA3) (Poulin et al., 2010). DC1 provide the processing of tumor antigens, migrate via CCR7 chemotaxis to the lymphoid organs and ensure antigen presentation to T-cells (Roberts et al., 2016). DC1 also express IFN-α receptor 1 (IFNAR1) because type I IFNs (IFN-α, IFN-β) promote their activation, migration and cross-presentation (Diamond et al., 2011). DC2 can be separated from DC1 by expression of CD11b, CD1c (BDCA1) and CD172a (Veglia and Gabrilovich, 2017), however, they are very difficult to distinguish from CD11c+ MHCII+ macrophages and monocyte-derived DCs (moDCs), so understanding the role of DC2 in tumor immunity remains limited.
A population of moDCs, also known as inflammatory DCs, are formed from CD14high monocytes as a result of inflammation (Segura and Amigorena, 2013). These cells carry a large number of common markers of myeloid cells, namely CD1a, CD11c, BDCA1, CD172a, CD206, and HLA-DR (Veglia and Gabrilovich, 2017). However, they can be identified by the expression of CD64 or the receptor of granulocyte-macrophage colony-stimulating factor (GM-CSFR), since GM-CSF is necessary for the development of moDCs from monocytes (Hiasa et al., 2009). It is GM-CSF-generated moDCs that are used to create antitumor vaccines. In vivo differentiated moDC-based vaccines are safe but not effective in a large number of clinical trials in patients with various tumors (Anguille et al., 2014; Chulpanova et al., 2018a). The failure of moDCs-based vaccines to stimulate an antitumor immune response in patients may be due to the compromised functionality of immune cells isolated from cancer patients (Shinde et al., 2018). In the human body, moDCs are able to present antigen, which leads to the simulation of CD8+ T-cells, inhibition of tumor growth (Kuhn et al., 2015) and Th17 cell stimulation (Segura et al., 2013).
Another population with a described role in antitumor immunity is plasmacytoid DCs (pDCs), which are characterized by a high level of type I IFN secretion (Facchetti et al., 2003). These cells mature in the bone marrow and have a plasma cell-like morphology; typically, pDCs express CD4, HLA-DR, CD123, BDCA2 (CD303), BDCA4 (CD304) and do not express CD11c (Veglia and Gabrilovich, 2017). Through the secretion of IFN-α and other pro-inflammatory cytokines, pDCs promote innate immune responses via the induction of NK cell migration and stimulation of macrophages and dendritic cells (Mitchell et al., 2018). pDCs can also regulate the T-cell mediated immune response and act as APCs [for more details see Mitchell et al. (2018)]. Direct GrzmB and TRAIL-mediated antitumor activity of pDCs has also been reported in some studies (Tel et al., 2012; Lombardi et al., 2015). However, a number of studies of pDCs isolated from cancer patients show a tendency toward the formation of immunological tolerance of the tumor due to pDCs (Maldonado and von Andrian, 2010). TME appears to suppress IFN-α secretion in pDCs and stimulate the development of Tregs (Faith et al., 2007; Sisirak et al., 2013).
Markers of various populations of DCs and their role in the tumor immunity, which varies depending on the population, have been widely investigated. Antitumor properties of moDCs have been actively explored, however, the active use of DCs as a platform for the development of anticancer vaccines has not led to significant success in the treatment of cancer (Chulpanova et al., 2018c).
The functions and CD markers of above populations of immune cells are summarized in Table 1 and Figure 1.
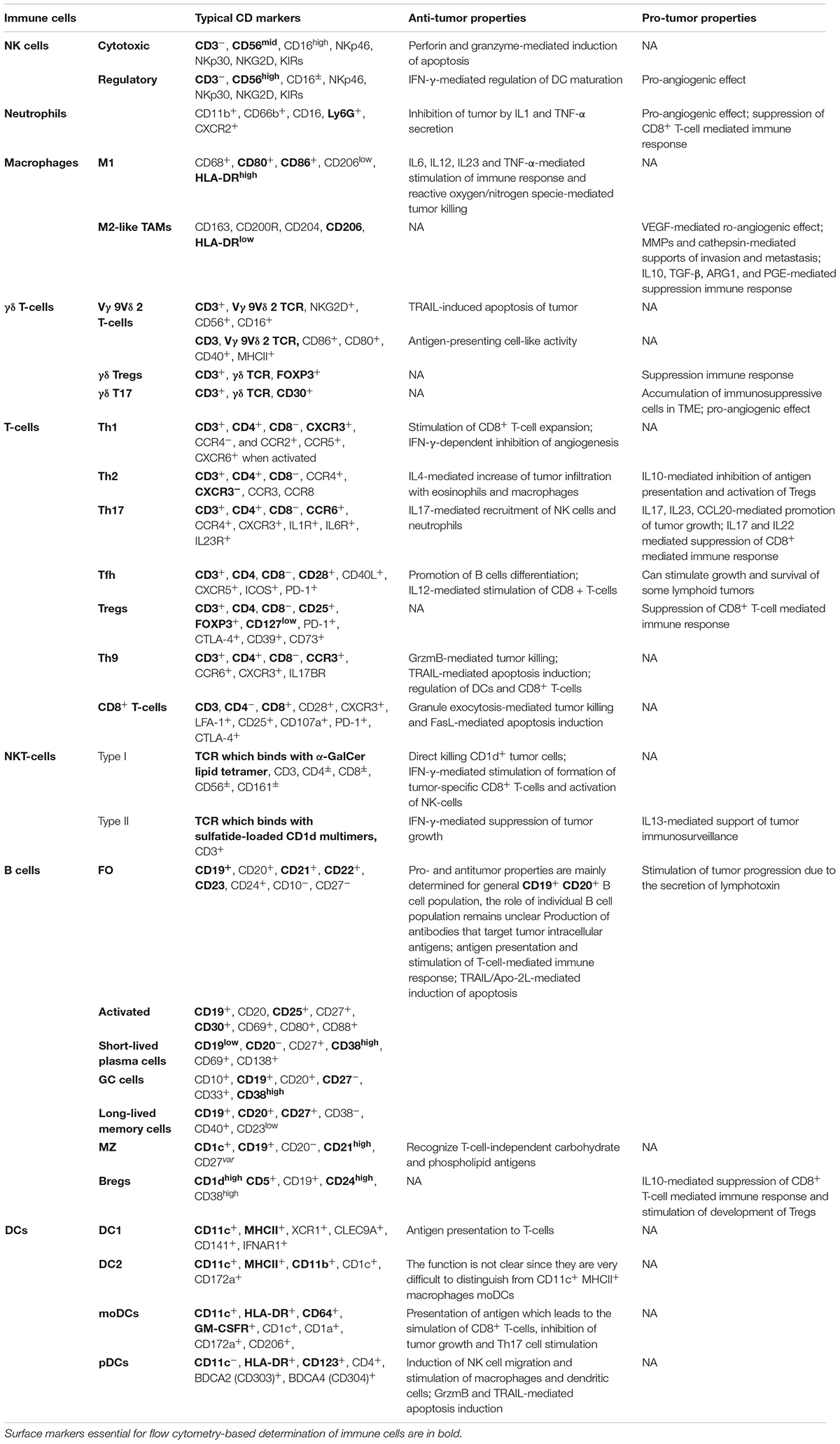
Table 1. Typical CD markers and functions in tumor immunity of various populations of human immune cells.
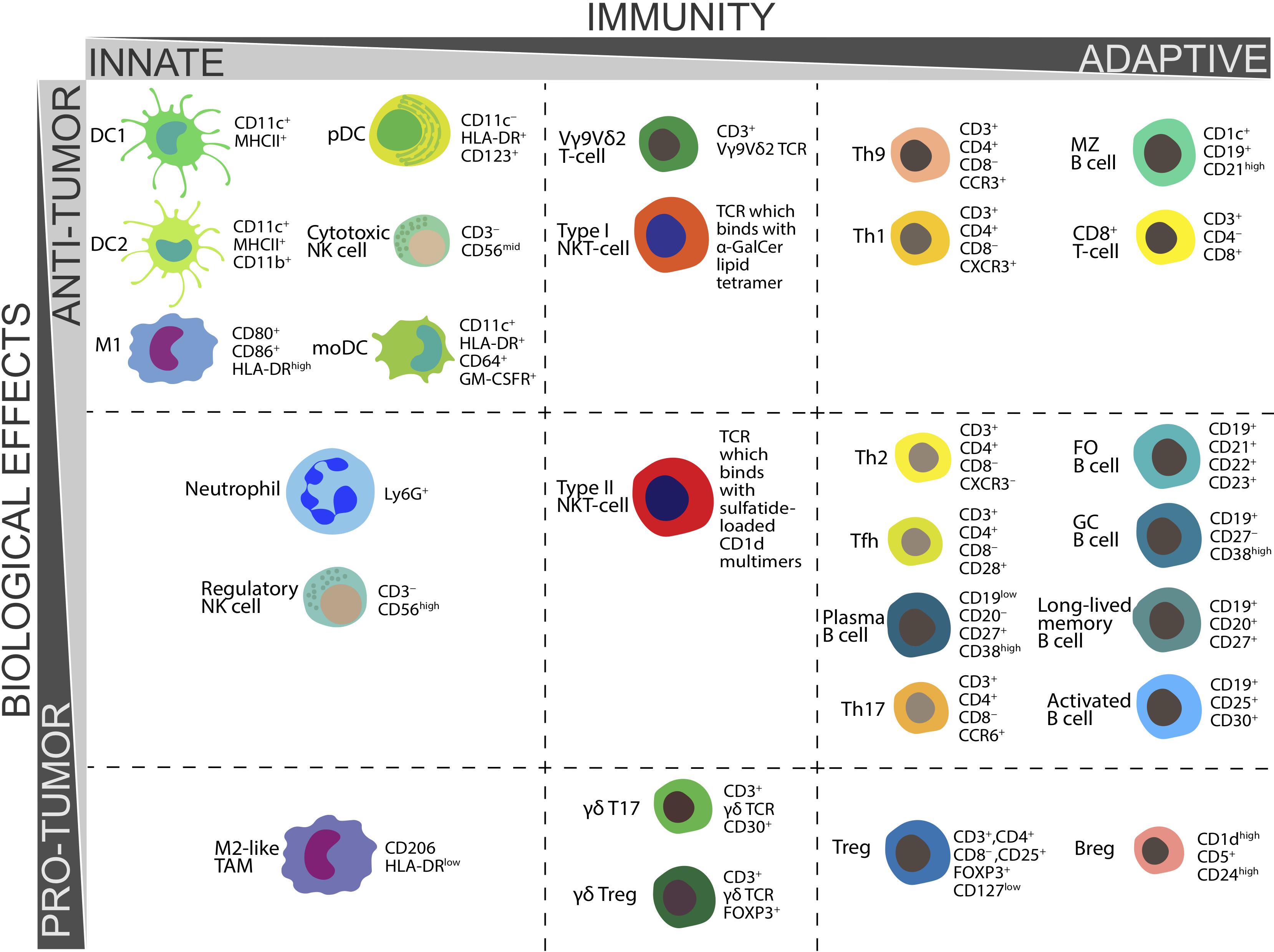
Figure 1. Biological functions in tumor immunity and CD markers essential for ex vivo determination of various populations of human immune cells.
Molecular Aspects of Cytokine-Based Cancer Therapy
Interleukin 2
The history of the use of cytokines as agents for the treatment of various diseases, including cancer, began in the mid-1990s when the anticancer effect of High-dose (HD) IL2 therapy was first demonstrated (West, 1989). IL2 is predominantly produced by antigen-stimulated CD4+ T-cells (Choudhry et al., 2018), as well as NKT-cells, CD8+ T-cells, mast cells and DCs (Paliard et al., 1988; Granucci et al., 2001; Yui et al., 2004; Hershko et al., 2011). IL2 can stimulate the proliferation of antigen-activated CD8+ T-cells (Cornish et al., 2006), treatment with endogenous IL2 leads to an increase in the expression of CD25, IL2 receptor, which in turn stimulates the proliferation of CD8+ T-cells (Hinrichs et al., 2008). IL2 increases the expression of LAMP-1 on the surface of CD8+ T-cells (Hromadnikova et al., 2016), decreases the expression of PD-1, an immunosuppressive receptor (Thommen et al., 2018), thereby mediating the cytotoxic activity of CD8+ T-cells. IL2 can stimulate the expansion and activation of NK cells with CD56high CD16– receptor (Caligiuri et al., 1993), with the cytotoxic function of this population is increased after activation (Fehniger et al., 2003). This cytokine also enhances the cytotoxic effect of γδ T-cells, increasing CD69 and degranulation marker CD107a expression and IFN-γ secretion (Ribot et al., 2014). Furthermore, IL2 can also promote the expansion of NKT-cells in cancer patients (Euhus et al., 1997; Engel et al., 1998). IL2-based therapy has several significant drawbacks, particularly the stimulation of Tregs, which are associated with the suppression of the antitumor immune response (Boyman et al., 2006). Stimulation with IL2 leads to increased expression of CD25, CTLA-4, and HLA-DR (Hirakawa et al., 2016) on the surface of CD3+ T-cells. IL2 treatment leads to an increase in the expression of FOXP3 in CD3+CD25+ T-cells and the formation of the Tregs phenotype, including during low-dose therapy (Zorn et al., 2006). However, recent discoveries in understanding the functioning of Tregs have opened up new possibilities for the use of IL2 in combination with Treg inhibitors, such as anti-CTLA-4 and anti-PD-1 (West et al., 2013). The combination of IL12 (Prochazkova et al., 2012) and IL21 (Attridge et al., 2012) has also been shown to block IL2-induced Treg activation.
Currently, IL2 has found its place in cancer immunotherapy for the expansion of immune cells such as NK cells, T-cells, NKT-cells, cytokine-induced killer (CIK) cells (Chung et al., 2014; Boyiadzis et al., 2017; Exley et al., 2017; Yoshida et al., 2017). IL2 is also used as an adjuvant in the treatment of patients with melanoma, advanced colorectal cancer or ovarian cancer with autologous dendritic cells stimulated by autologous tumor lysate (Baek et al., 2015; Greene et al., 2016; Liu et al., 2016), as well as in the treatment with viral vaccines (Oudard et al., 2011). The addition of IL2 to the treatment regimen can increase the effectiveness of therapy due to the induction of expansion of tumor antigen presented T-cell (Oudard et al., 2011).
Interleukin 2 is also effective to treat patients with melanoma when combined with dacarbazine monotherapy or anti-VEGF monoclonal antibody (mAb) monotherapy, but do not increase the effectiveness of neuroblastoma therapy in combination with dinutuximab (anti-GD2 mAb) (Ladenstein et al., 2018; Tarhini et al., 2018; Weide et al., 2019). Several clinical trials have also evaluated the effectiveness of the combination of radiotherapy and IL2, however, this approach has not been further developed (Oudard et al., 2011; Ridolfi et al., 2014; van den Heuvel et al., 2015).
One of the newest approaches to cancer immunotherapy is the combination of immune checkpoint inhibitors and recombinant IL2 for the treatment of melanoma and renal cell carcinoma. Such a combination may improve the activation of the immune system (West et al., 2013) and potentially enhance clinical efficacy. HD IL2 increased overall survival (OS) in patients with renal cell carcinoma and progressive melanoma who was previously treated with a PD-1 or PD-L1 inhibitor compared to patients without pretreatment with anti-PD-1/PD-L1 (Buchbinder et al., 2019). The combination of IL2 and ipilimumab (CTLA-4 checkpoint inhibitor) for the treatment of patients with melanoma led to large side effects expected from single agent treatment, with an increase in the number of peripheral IFN-γ producing CD8+ T-cells in most patients, which indicated the effectiveness of IL2 and ipilimumab combination to stimulate the immune response (Ray et al., 2016; Weide et al., 2017; Silk et al., 2019).
Interleukin 12
Interleukin 12 is another cytokine with well-studied antitumor activity, which is mainly mediated by stimulation of IFN-γ production in cytotoxic cells (CD8 T-cells and NK cells) and Th1 cells (Lasek et al., 2014). Treatment with IL12 leads with varying degrees of success to an increase in the number of CD56+ NK cells (Agaugue et al., 2008), as well as CD2 and LFA-1 expression (Robertson et al., 1999), which ultimately results in the increased cytotoxicity of IL12-treated NK cells (Lehmann et al., 2014; Martinovic et al., 2015). IL12 also stimulates the production of IFN-γ in cytotoxic CD8+ T-cells (Yang et al., 2016), enhances their proliferative activity (Mukhopadhyay et al., 2019) and cytotoxicity, probably due to increased expression of GrzmB (Rubinstein et al., 2015; Li et al., 2017). In addition, IL12-stimulated CD8 T-cells are able to reduce the number of Tregs in TME by Fas-mediated apoptosis (Kilinc et al., 2009; Kerkar et al., 2010). IL12 is also involved in the differentiation of naïve Th cells in Th1 cells (Kennedy et al., 1994). Promising results in cell and animal models have prompted clinical studies of IL12. However, the future of IL12 in the treatment of cancer was overshadowed by the significant side effects that were observed in the first clinical trials. The established protocol in the Phase I study schedule of IL12 administration was slightly amended (patients in Phase 2 trial received daily IL12 dosing without the single injection of IL12 2 weeks earlier that was employed in the Phase 1 study) that led to the development of severe IFN-γ-mediated toxicity, resulting in 12 patients being hospitalized and two patients dying (Leonard et al., 1997). Clinical trials of IL12 have continued, but with more caution, however, ultimately IL12 has not been shown particular efficacy, either alone or in combination with various therapeutic agents, with the exception of patients with cutaneous T-cell lymphoma (CTCL), with non-Hodgkin’s B-cell lymphoma, and with acquired immune deficiency syndrome (AIDS)-associated Kaposi sarcoma (Lasek et al., 2014).
To avoid the high IL12-mediated systemic toxicity, regulated plasmids have been developed in which IL12 expression is induced by an activator. The use of a plasmid in which the expression of IL12 is regulated by a promoter sensitive to nuclear factor of activated T-cells (NFAT) for the genetic modification of TILs has allowed a reduction in the number of cells necessary for significant response by 10–100 times in comparison with standard treatment protocols. However, serum IL12 levels were unpredictable and significant toxicity was observed in patients (Zhang et al., 2015). In another study, a regulated plasmid was injected at the site of tumor resection, and IL12 expression was then activated. After this therapy OS rates were encouraged compared to historical controls (Chiocca et al., 2019).
In combination with chemotherapy (Anwer et al., 2013) or mAbs (McMichael et al., 2019) IL12 did not show significant toxicity, as well as promising result indicating the usefulness of IL12.
An actively developing approach is the use of IL12 in combination with oncolytic viruses (OVs), which selectively replicate in and kill cancer cells. The integration of the IL12 gene in the virus genome can enhance the virus-mediated immune response, while avoiding systemic toxicity (Nguyen et al., 2020). OVs with IL12 showed safety and promising efficacy in mouse models compared with OS without IL12 (Cheema et al., 2013; Alessandrini et al., 2019), however, the results of clinical trials on humans have not yet been presented (Patel et al., 2016) (NCT02555397, NCT00406939, NCT03281382, NCT00849459, and NCT01397708).
Interleukin 15
Another cytokine that belongs to the same family as IL2 and has many overlapping functions is IL15 (Waldmann, 2018). Since IL15 and IL2 use several identical receptor components, IL2/IL15Rβ and γc, and trigger the common molecules of Janus kinase (JAK) 1/3 and signal transducer and activator of transcription (STAT) 3/5 pathways they have overlapping functions (Robinson and Schluns, 2017). The administration of IL15 leads to an increase in the expansion of CD8+ T-cell, as well as expression of CD38 and HLA-DR activation markers (Conlon et al., 2015). This cytokine also enhances the proliferation of NK cells in cancer patients with greater stimulation of CD56high NK cells (Dubois et al., 2017). IL15 can also stimulate the cytotoxic functions of CD56high NK cells (Wagner et al., 2017), while increasing the expression of CD16 and CX3C chemokine receptor 1 (CX3CR1), which are mainly expressed on CD56low NK cells (Dubois et al., 2017). A similar effect (enhanced expansion and cytotoxicity) IL15 has on γδ T-cells (Ribot et al., 2014; Conlon et al., 2015). Despite similar functions with IL2, IL15 therapy does not stimulate the expansion of Tregs and does not cause capillary leak syndrome. However, recombinant IL15 therapy can lead to general systemic toxicity by increasing the production of pro-inflammatory cytokines or by stimulating autoimmune-like responses (Cooley et al., 2019).
Phase I clinical trials of subcutaneous recombinant human IL15 (rhIL15) therapy showed that rhIL15 was well tolerated and caused expansion of circulating NK cells, especially CD56bright subset, and to a lesser degree CD8+ T-cells (Miller et al., 2018). Since IL15 is trans-presented to CD8+ T and NK cells in a complex with the IL15α receptor in order to increase its immunomodulating activity, the ALT-803 therapeutic complex has been developed, which is IL15 with IL15α receptor fused to human IgG1 dimer Fc, which maintains stability and extends the half-life of the entire complex (Knudson et al., 2019). ALT-803 showed safety and the ability to increase the number of NK cells after subcutaneous administration to patients with progressive solid tumors in phase I clinical trials (Margolin et al., 2018). Clinical effect demonstration in single-agent phase I trials is rare, and has not been observed in these trials. However, several clinical trials to evaluate the therapeutic potential of rhIL15 in combination with other therapeutic agents are ongoing (NCT03905135, NCT03388632).
ALT-803 in combination with nivolumab (anti-PD-1 mAb) may repeatedly elicit objective responses to anti-PD-1 immunotherapy after relapse or treatment failure in patients with non-small cell lung cancer (NSCLC) (Wrangle et al., 2018).
Interleukin 15 is actively used to activate and expand NK cells, which are then transplanted to patients with leukemia (Vela et al., 2018) or solid tumors (Perez-Martinez et al., 2015), as well as to stimulate the proliferation of chimeric antigen receptor (CAR) T-cells in combination with IL2 (Wang et al., 2019). In a recent clinical trial, anti-CD19 CAR-NK cells encoding the human IL15 gene were used to treat patients with recurrent or refractory CD19-positive cancer. Most patients had a response to treatment with CAR-NK cells without the development of major toxic effects (Liu et al., 2020).
Mouse models also showed the efficacy of using IL15/IL15R in combination with an autologous vaccine to stimulate antitumor immunity against acute myeloid leukemia (Shi Y. et al., 2018). However, these studies have not yet reached human clinical trials. It is most likely that in the near future combinations of IL15 with various therapeutic agents, such as immune checkpoint inhibitors, will be actively investigated.
Interleukin 21
Interleukin 21, another member of the IL2 family, is one of the last cytokines investigated for the clinical use in cancer treatment (Santegoets et al., 2013). One of the most important functions of IL21 is to stimulate the proliferation of germinal center (GC) B cells (Zotos et al., 2010), as well as to induce the differentiation CD40L-stimulated B cells in plasma cells (Ding et al., 2013). However, IL21 treatment also results in an increase in the number of B10 cells as well as the levels of IL10 that they produce (Yoshizaki et al., 2012). The increase in IL10 secretion after IL21 stimulation is also observed in CD4+, CD8+ T-cells (Spolski et al., 2009). IL21 is also able to activate NK cells by stimulating the expression of CD69 and the natural cytotoxicity receptor NKp46 and increasing cytotoxic activity (Skak et al., 2008). IL21 stimulates differentiation of naïve CD4+ T-cells in Th17 cells, inducing expression of IL17, retinoic-acid-receptor-related orphan nuclear receptor gamma (RORγt) transcription factor and IL23R (Korn et al., 2007). Also, this cytokine plays an important role in the autocrine stimulation of proliferation and differentiation (expression of inducible T-cell costimulator (ICOS) is increased) of Tfh (Vogelzang et al., 2008). It is also worth noting that IL21 negatively regulates homeostasis of CD4+ CD25+ FOXP3+ Tregs (Attridge et al., 2012). However, in clinical trials the therapeutic effect of IL21 is evaluated by analyzing the secretion of soluble CD25, the level of which increases during the activation of T-cells and NK cells (Thompson et al., 2008; Davis et al., 2009). Whilst the effect of IL21 therapy on the remaining immune system cell populations of cancer patients remains unexplored.
Clinical trials have shown that rIL21 is able to increase the number of CD3+ CD56 NKT-like cells in patients with stage IV malignant melanoma (Coquet et al., 2013), and also activate T and NK cells in patients with stage IV colorectal cancer (Steele et al., 2012). The combination of IL21 with various mAbs showed that the combination of IL21 with rituximab (anti-CD20 mAb) or sorafenib (anti-VEGF mAb) was well tolerated and had antitumor activity (Timmerman et al., 2012; Bhatia et al., 2014). However, the contribution of IL21 into the shown antitumor activity has not been fully determined.
Interleukin 21, like other members of its family, is used to activate and expand T-cell and NK cells (Chapuis et al., 2016; Ciurea et al., 2017). There are several completed and active clinical trials where IL21 is combined with checkpoint inhibitors or with anti-CD19 CAR T-cells (NCT01629758, NCT04093648). There is insufficient clinical data on the use of this cytokine. However, it is likely that the use of IL21 in cancer immunotherapy will be investigated in this direction.
Interferons
Another group of cytokines, interferons, has also been shown to be effective in cancer immunotherapy. IFNs are divided into three types depending on the function and the target receptor: type I (α, β, ε, κ, and ω), type II (γ), and type III (λ) (Budhwani et al., 2018). IFN-α2a was the first approved cytokine for the treatment of chronic myeloid leukemia (Italian Cooperative Study Group on Chronic Myeloid Leukemia, Tura et al., 1994), since type I IFNs have broad immunomodulatory activity. IFN-α can stimulate differentiation of CD14+ monocytes in DCs jointly with GM-CSF (Gabriele et al., 2004) which will be discussed below. IFN-α/GM-CSF stimulation leads to the increased expression of HLA-DR, CD11c, CD83, B7 costimulatory molecules CD80 and CD86, including on DCs of cancer patients, and such DCs are able to efficiently present an antigen to CD4+ and CD8+ T-cells (Paquette et al., 2002; Jin et al., 2017). However, IFN-β reduces the ability of mature DCs to stimulate T-cell proliferation and differentiate into IFN-γ-producing Th1 cells (Yen et al., 2010). In addition to its apparent effect on T-cells via DCs, IFN-α is also able to stimulate the effector functions of pre-activated CD8+ T-cell (which have already interacted with antigen and costimulatory molecules), leading to increased number of activation markers CD38 and CD25 and raised expression of GrzmB, TRAIL, FasL, and IFN-γ (Sikora et al., 2009; Hervas-Stubbs et al., 2010; Lu et al., 2019). Type I IFNs have different effects on the differentiation of CD4+ T-cells, supporting the polarization into Th1 cells and inhibiting the formation of Th2 and Th17 T-cell phenotypes (Huber et al., 2010; Huber and Farrar, 2011). Type I IFNs also play an important role in the regulation of NK cell cytotoxicity, however, low cytotoxicity in the absence of type I IFN stimulation can be overcomed by stimulation with IL2 (Muller et al., 2017).
IFN-γ is a type II IFN cytokine which can both regulate the antitumor immune response and directly induce apoptosis of tumor cells (Zaidi, 2019). Here we focus on the regulation of the immune system cells. It was shown that IFN-γ therapy leads to a significant increase in the number of CD14high CD16+ monocytes and a rise in MHCII expression on all monocytes (Kirkwood et al., 1997) in patients with various types of tumors. The number of activated NK cells also increases (expression of NK receptor activation marker NKp30 was increased on both CD56high and CD56low NK cells) (Zibelman et al., 2017) (NCT02614456). In addition, a number of investigations in mouse models has been shown that IFN-γ is able to modulate polarization toward CD86+ iNOS+ M1 macrophages, which can inhibit tumor cell growth by releasing NO (Ren et al., 2014; Muller et al., 2018). Nevertheless, IFN-γ stimulates differentiation of CD4+CD25– T-cells in CD4+ Tregs in a mouse model of experimental autoimmune encephalomyelitis (Wang et al., 2006) as well inhibiting the proliferation of Th2 cells (Gajewski and Fitch, 1988; Oriss et al., 1997). However, the effect of IFN-γ on various T-cell populations in cancer patients requires more detailed investigation. In addition, there is an evidence of the pro-tumor effect of IFN-γ [for more details see Zaidi (2019)] which also requires the attention of researchers.
Type I interferons are actively combined with various therapeutic agents in clinical trials (NCT03112590). In the number of clinical trials, IFN-α or IFN-β were used to stimulate the immune response in patients who received therapy with DC vaccines (Schwaab et al., 2009; Duggan et al., 2016) or tumor-specific antigens (Elkord et al., 2015; Hawkins et al., 2016; Shima et al., 2019). Vaccination itself did not always lead to the increase in OS or showed some encouraging results (Shima et al., 2019). However, IFN-α administration was sometimes able to significantly increase OS compared to a single vaccine (Duggan et al., 2016; Sheng et al., 2020).
Interferons have also been combined with chemotherapy and mAbs for the treatment of various types of tumors (Dijkgraaf et al., 2015). The combination of IFN-β with temozolomide did not show any promising results in patients with glioblastoma (Wakabayashi et al., 2018). Also, the combination of IFNs with bevacizumab (anti-VEGF mAb) did not show much benefit compared to the combination of bevacizumab + everolimus, which is usually used to treat metastatic renal cell carcinoma (Ravaud et al., 2015).
In general, there are not many clinical trials that are ongoing to evaluate the efficacy of combination of IFNs with immune checkpoint inhibitors, most often IFN-α monotherapy is used as a reference to evaluate the effectiveness of the inhibitors for the treatment of advanced melanoma (Li et al., 2020; Tarhini et al., 2020). However, the clinical trials of IFN-α and pembrolizumab have shown the safety but had limited antitumor activity of the IFN-α + pembrolizumab combination (Atkins et al., 2018). Several clinical trials of IFN-γ with PD-1 inhibitors are ongoing (NCT02614456, NCT03063632).
Granulocyte-Macrophage Colony-Stimulating Factor
Granulocyte-Macrophage Colony-Stimulating Factor plays an important role in the regulation of proliferation and differentiation of myeloid cells (Bhattacharya et al., 2015). One of the main functions of GM-CSF that is actively used for cancer immunotherapy is its ability to regulate the maturation of DCs from myeloid progenitors (Ushach and Zlotnik, 2016). Stimulation of CD14high or CD133+ monocytes with GM-CSF together with IL4 and/or type I IFNs leads to differentiation in moDCs expressing MHCII, CD80, CD83, and CD86 costimulatory molecules (Moldenhauer et al., 2010; Blyszczuk et al., 2013). This cytokine has been used in a large number of clinical trials of antitumor vaccines (Chang et al., 2000; Slingluff et al., 2003). However, such moDCs are able to stimulate Th17 due to the secretion of IL1b and IL6, as already noted above (Ko et al., 2014), and also provoke the formation of FOXP3+ Tregs (Gopisetty et al., 2013). GM-CSF is also involved in the programming of M1 macrophages, and may promote M2 to CD45+ CD11b+ F4/80 MHCII+ CD163– CD206– M1 polarization of macrophages in TME (Eubank et al., 2009; Brenot et al., 2018; Benner et al., 2019). The ability of GM-CSF to stimulate the proliferation of activated CD54+ neutrophils has also been described, which may be useful for immunotherapy, since neutropenia is one of the most common symptoms of cytokine-based immunotherapy in cancer patients (Yu and Hua, 2018). However, the effect of neutrophils on tumor progression is still under discussion, and GM-CSF can stimulate the expression of PD-L1, an immunosuppressive molecule, on the surface of neutrophils (Wang et al., 2017). Despite the pronounced stimulation of differentiation of DCs, the effect of GM-CSF on other populations of the innate immune system cells remains insufficiently explored, especially in cancer patients. The use of this cytokine requires broader inquiry, because, despite active investigations, GM-CSF-based therapeutic vaccines have not shown the expected effectiveness.
Despite this, the number of clinical trials of antitumor vaccines that are used in combination with GM-CSF remains unchanged. Over the past 5 years (2015–2020), 58 new clinical trials have been registered, whilst between 2010 and 2015 69 clinical trials of antitumor vaccines were registered1. GM-CSF is added as an adjuvant to DCs for both DC-based vaccines and autologous tumor cell vaccines (Dillman et al., 2018; Clifton et al., 2019). Tumor cells can also be modified to overexpress GM-CSF to generate the vaccines (Gray et al., 2018). Interestingly, the administration of GM-CSF led to an increase in anti-GM-CSF neutralizing antibodies (Nabs), which, however, correlated with improved relapse-free survival (RFS) and OS (Butterfield et al., 2017).
Granulocyte-Macrophage Colony-Stimulating Factor was combined with radiation therapy to stimulate the maturation of DCs that could present antigens released by radiation-damaged cells. The combined therapy caused objective abscopal responses in some patients with metastatic solid tumors (Golden et al., 2015). The efficacy of T-vec oncolytic virus encoding the GM-CSF gene for the treatment of patients with melanoma was also shown (Andtbacka et al., 2016).
As with other cytokines, there is a tendency to combine cell-based and DNA-based vaccines + GM-CSF with immune checkpoint inhibitors (NCT04013672, NCT03600350) in order to achieve a significant therapeutic effect. In clinical trials, immune checkpoint inhibitors are combined with oncolytic viruses containing GM-CSF (NCT02977156, NCT04197882, NCT03206073, and NCT03003676). All of these clinical trials are now ongoing.
The effect of cytokine therapy on various populations of immune cells is summarized in Table 2.
Conclusion and Future Perspectives
Undoubtedly, cytokines have proven to be effective in cancer therapy, however, the effect of some promising targets on various immune cell populations remains poorly understood. The same situation is with respect to surface CD markers. For well-studied populations, such as T-cells, the set and functions of surface receptors are fairly well defined, and researchers use approximately the same sets of CD markers to identify populations using flow cytometry. However, less studied are the population of immune cells, the more diverse are the sets of determined receptors, and the more difficult it is to compare the results to identify persistent patterns. The study of changes in the function and surface marker expression of each individual immune system cell population after immunotherapy will simplify and unify the assessment of the effectiveness of therapy, as well as allow predicting the effectiveness of immunotherapy by analyzing surface markers of immune cells of cancer patients.
Despite the fact that this review focuses on a detailed description of the functions and surface markers of immune system cells in cancer and after cytokine-based immunotherapy, a review of this topic requires discussion of the prospects of cytokine-based cancer treatment. One of the main features of cytokines, as regulators of the immune response, is its pleiotropic effect. Each cytokine regulates many different populations of the immune cells that can support both anti-tumor and pro-tumor responses. Therefore, the future perspectives of cytokine-based cancer therapy will depend on the production of combined schemes aimed at enhancing the antitumor response and suppressing immune cells that support tumor growth. Also, other significant problems encountered are the short half-life and systemic toxicity (pro-inflammatory and autoimmune reactions) of high doses of cytokines which are necessary to elicit a significant response in cancer patients. New approaches that improve targeting of cytokines and alter their pharmacokinetics might be useful (such cell based or other vector delivery, chemically modified recombinant proteins, etc.) to overcome limitations of different cytokine therapies. Current trends in the development of cancer immunotherapy indicate that cytokines may find their greatest role in therapy when administered in combination with other agents, such as immune checkpoint inhibitors, oncolytic viruses or as a component of DC-based and tumor cell-based vaccines.
Author Contributions
DC wrote the manuscript and made the tables. KK created the figure. AG edited the manuscript. DC, VS, and AR conceived the idea and edited the manuscript and tables.
Funding
This study was supported by the Russian Government Program of Competitive Growth of Kazan Federal University and the Russian Science Foundation grant 18-74-10044.
Conflict of Interest
The authors declare that the research was conducted in the absence of any commercial or financial relationships that could be construed as a potential conflict of interest.
References
Acosta-Rodriguez, E. V., Napolitani, G., Lanzavecchia, A., and Sallusto, F. (2007a). Interleukins 1beta and 6 but not transforming growth factor-beta are essential for the differentiation of interleukin 17-producing human T helper cells. Nat. Immunol. 8, 942–949. doi: 10.1038/ni1496
Acosta-Rodriguez, E. V., Rivino, L., Geginat, J., Jarrossay, D., Gattorno, M., Lanzavecchia, A., et al. (2007b). Surface phenotype and antigenic specificity of human interleukin 17-producing T helper memory cells. Nat. Immunol. 8, 639–646. doi: 10.1038/ni1467
Agaugue, S., Marcenaro, E., Ferranti, B., Moretta, L., and Moretta, A. (2008). Human natural killer cells exposed to IL-2, IL-125 IL-18, or IL-4 differently modulate priming of naive T cells by monocyte-derived dendritic cells. Blood 112, 1776–1783. doi: 10.1182/blood-2008-02-135871
Aktas, E., Kucuksezer, U. C., Bilgic, S., Erten, G., and Deniz, G. (2009). Relationship between CD107a expression and cytotoxic activity. Cell. Immunol. 254, 149–154. doi: 10.1016/j.cellimm.2008.08.007
Alessandrini, F., Menotti, L., Avitabile, E., Appolloni, I., Ceresa, D., Marubbi, D., et al. (2019). Eradication of glioblastoma by immuno-virotherapy with a retargeted oncolytic HSV in a preclinical model. Oncogene 38, 4467–4479. doi: 10.1038/s41388-019-0737-2
Alexander, A. A., Maniar, A., Cummings, J. S., Hebbeler, A. M., Schulze, D. H., Gastman, B. R., et al. (2008). Isopentenyl pyrophosphate-activated CD56+ {gamma}{delta} T lymphocytes display potent antitumor activity toward human squamous cell carcinoma. Clin. Cancer Res. 14, 4232–4240. doi: 10.1158/1078-0432.CCR-07-4912
Ali, T. H., Pisanti, S., Ciaglia, E., Mortarini, R., Anichini, A., Garofalo, C., et al. (2014). Enrichment of CD56(dim)KIR + CD57 + highly cytotoxic NK cells in tumour-infiltrated lymph nodes of melanoma patients. Nat. Commun. 5:5639. doi: 10.1038/ncomms6639
Ambrosino, E., Terabe, M., Halder, R. C., Peng, J., Takaku, S., Miyake, S., et al. (2007). Cross-regulation between type I and type II NKT cells in regulating tumor immunity: a new immunoregulatory axis. J. Immunol. 179, 5126–5136. doi: 10.4049/jimmunol.179.8.5126
Ame-Thomas, P., Le Priol, J., Yssel, H., Caron, G., Pangault, C., Jean, R., et al. (2012). Characterization of intratumoral follicular helper T cells in follicular lymphoma: role in the survival of malignant B cells. Leukemia 26, 1053–1063. doi: 10.1038/leu.2011.301
Amlot, P. L., Tahami, F., Chinn, D., and Rawlings, E. (1996). Activation antigen expression on human T cells. I. Analysis by two-colour flow cytometry of umbilical cord blood, adult blood and lymphoid tissue. Clin. Exp. Immunol. 105, 176–182. doi: 10.1046/j.1365-2249.1996.d01-722.x
Ammirante, M., Luo, J. L., Grivennikov, S., Nedospasov, S., and Karin, M. (2010). B-cell-derived lymphotoxin promotes castration-resistant prostate cancer. Nature 464, 302–305. doi: 10.1038/nature08782
Andtbacka, R. H., Ross, M., Puzanov, I., Milhem, M., Collichio, F., Delman, K. A., et al. (2016). Patterns of clinical response with talimogene laherparepvec (T-VEC) in patients with melanoma treated in the OPTiM phase III clinical trial. Ann. Surg. Oncol. 23, 4169–4177. doi: 10.1245/s10434-016-5286-0
Angelo, L. S., Banerjee, P. P., Monaco-Shawver, L., Rosen, J. B., Makedonas, G., Forbes, L. R., et al. (2015). Practical NK cell phenotyping and variability in healthy adults. Immunol. Res. 62, 341–356. doi: 10.1007/s12026-015-8664-y
Angkasekwinai, P., Chang, S. H., Thapa, M., Watarai, H., and Dong, C. (2010). Regulation of IL-9 expression by IL-25 signaling. Nat. Immunol. 11, 250–256. doi: 10.1038/ni.1846
Anguille, S., Smits, E. L., Lion, E., van Tendeloo, V. F., and Berneman, Z. N. (2014). Clinical use of dendritic cells for cancer therapy. Lancet Oncol. 15, e257–e267. doi: 10.1016/S1470-2045(13)70585-0
Anwer, K., Kelly, F. J., Chu, C., Fewell, J. G., Lewis, D., and Alvarez, R. D. (2013). Phase I trial of a formulated IL-12 plasmid in combination with carboplatin and docetaxel chemotherapy in the treatment of platinum-sensitive recurrent ovarian cancer. Gynecol. Oncol. 131, 169–173. doi: 10.1016/j.ygyno.2013.07.081
Aras, S., and Zaidi, M. R. (2017). TAMeless traitors: macrophages in cancer progression and metastasis. Br. J. Cancer 117, 1583–1591. doi: 10.1038/bjc.2017.356
Asadzadeh, Z., Mohammadi, H., Safarzadeh, E., Hemmatzadeh, M., Mahdian-Shakib, A., Jadidi-Niaragh, F., et al. (2017). The paradox of Th17 cell functions in tumor immunity. Cell. Immunol. 322, 15–25. doi: 10.1016/j.cellimm.2017.10.015
Atkins, M. B., Hodi, F. S., Thompson, J. A., McDermott, D. F., Hwu, W. J., Lawrence, D. P., et al. (2018). Pembrolizumab plus pegylated interferon alfa-2b or ipilimumab for advanced melanoma or renal cell carcinoma: dose-finding results from the phase Ib KEYNOTE-029 study. Clin. Cancer Res. 24, 1805–1815. doi: 10.1158/1078-0432.CCR-17-3436
Atri, C., Guerfali, F. Z., and Laouini, D. (2018). Role of human macrophage polarization in inflammation during infectious diseases. Int. J. Mol. Sci. 19:1801. doi: 10.3390/ijms19061801
Attridge, K., Wang, C. J., Wardzinski, L., Kenefeck, R., Chamberlain, J. L., Manzotti, C., et al. (2012). IL-21 inhibits T cell IL-2 production and impairs Treg homeostasis. Blood 119, 4656–4664. doi: 10.1182/blood-2011-10-388546
Baek, S., Kim, Y. M., Kim, S. B., Kim, C. S., Kwon, S. W., Kim, Y., et al. (2015). Therapeutic DC vaccination with IL-2 as a consolidation therapy for ovarian cancer patients: a phase I/II trial. Cell. Mol. Immunol. 12, 87–95. doi: 10.1038/cmi.2014.40
Balsamo, M., Scordamaglia, F., Pietra, G., Manzini, C., Cantoni, C., Boitano, M., et al. (2009). Melanoma-associated fibroblasts modulate NK cell phenotype and antitumor cytotoxicity. Proc. Natl. Acad. Sci. U.S.A. 106, 20847–20852. doi: 10.1073/pnas.0906481106
Benner, B., Scarberry, L., Suarez-Kelly, L. P., Duggan, M. C., Campbell, A. R., Smith, E., et al. (2019). Generation of monocyte-derived tumor-associated macrophages using tumor-conditioned media provides a novel method to study tumor-associated macrophages in vitro. J. Immunother. Cancer 7:140. doi: 10.1186/s40425-019-0622-0
Bertani, F. R., Mozetic, P., Fioramonti, M., Iuliani, M., Ribelli, G., Pantano, F., et al. (2017). Classification of M1/M2-polarized human macrophages by label-free hyperspectral reflectance confocal microscopy and multivariate analysis. Sci. Rep. 7:8965. doi: 10.1038/s41598-017-08121-8
Bhatia, S., Curti, B., Ernstoff, M. S., Gordon, M., Heath, E. I., and Miller, W. H. Jr. et al. (2014). Recombinant interleukin-21 plus sorafenib for metastatic renal cell carcinoma: a phase 1/2 study. J. Immunother. Cancer 2:2. doi: 10.1186/2051-1426-2-2
Bhattacharya, P., Thiruppathi, M., Elshabrawy, H. A., Alharshawi, K., Kumar, P., and Prabhakar, B. S. (2015). GM-CSF: an immune modulatory cytokine that can suppress autoimmunity. Cytokine 75, 261–271. doi: 10.1016/j.cyto.2015.05.030
Blomqvist, M., Rhost, S., Teneberg, S., Lofbom, L., Osterbye, T., Brigl, M., et al. (2009). Multiple tissue-specific isoforms of sulfatide activate CD1d-restricted type II NKT cells. Eur. J. Immunol. 39, 1726–1735. doi: 10.1002/eji.200839001
Blyszczuk, P., Behnke, S., Luscher, T. F., Eriksson, U., and Kania, G. (2013). GM-CSF promotes inflammatory dendritic cell formation but does not contribute to disease progression in experimental autoimmune myocarditis. Biochim. Biophys. Acta 1833, 934–944. doi: 10.1016/j.bbamcr.2012.10.008
Borsellino, G., Kleinewietfeld, M., Di Mitri, D., Sternjak, A., Diamantini, A., Giometto, R., et al. (2007). Expression of ectonucleotidase CD39 by Foxp3+ Treg cells: hydrolysis of extracellular ATP and immune suppression. Blood 110, 1225–1232. doi: 10.1182/blood-2006-12-064527
Borst, J., Ahrends, T., Babala, N., Melief, C. J. M., and Kastenmuller, W. (2018). CD4(+) T cell help in cancer immunology and immunotherapy. Nat. Rev. Immunol. 18, 635–647. doi: 10.1038/s41577-018-0044-0
Bouaziz, J. D., Calbo, S., Maho-Vaillant, M., Saussine, A., Bagot, M., Bensussan, A., et al. (2010). IL-10 produced by activated human B cells regulates CD4(+) T-cell activation in vitro. Eur. J. Immunol. 40, 2686–2691. doi: 10.1002/eji.201040673
Boyiadzis, M., Agha, M., Redner, R. L., Sehgal, A., Im, A., Hou, J. Z., et al. (2017). Phase 1 clinical trial of adoptive immunotherapy using “off-the-shelf” activated natural killer cells in patients with refractory and relapsed acute myeloid leukemia. Cytotherapy 19, 1225–1232. doi: 10.1016/j.jcyt.2017.07.008
Boyman, O., Kovar, M., Rubinstein, M. P., Surh, C. D., and Sprent, J. (2006). Selective stimulation of T cell subsets with antibody-cytokine immune complexes. Science 311, 1924–1927. doi: 10.1126/science.1122927
Brenot, A., Knolhoff, B. L., DeNardo, D. G., and Longmore, G. D. (2018). SNAIL1 action in tumor cells influences macrophage polarization and metastasis in breast cancer through altered GM-CSF secretion. Oncogenesis 7:32. doi: 10.1038/s41389-018-0042-x
Brodbeck, T., Nehmann, N., Bethge, A., Wedemann, G., and Schumacher, U. (2014). Perforin-dependent direct cytotoxicity in natural killer cells induces considerable knockdown of spontaneous lung metastases and computer modelling-proven tumor cell dormancy in a HT29 human colon cancer xenograft mouse model. Mol. Cancer 13:244. doi: 10.1186/1476-4598-13-244
Bruno, A., Focaccetti, C., Pagani, A., Imperatori, A. S., Spagnoletti, M., Rotolo, N., et al. (2013). The proangiogenic phenotype of natural killer cells in patients with non-small cell lung cancer. Neoplasia 15, 133–142. doi: 10.1593/neo.121758
Bruno, T. C., Ebner, P. J., Moore, B. L., Squalls, O. G., Waugh, K. A., Eruslanov, E. B., et al. (2017). Antigen-presenting intratumoral B cells affect CD4(+) TIL phenotypes in non-small cell lung cancer patients. Cancer Immunol. Res. 5, 898–907. doi: 10.1158/2326-6066.CIR-17-0075
Buchbinder, E. I., Dutcher, J. P., Daniels, G. A., Curti, B. D., Patel, S. P., Holtan, S. G., et al. (2019). Therapy with high-dose Interleukin-2 (HD IL-2) in metastatic melanoma and renal cell carcinoma following PD1 or PDL1 inhibition. J. Immunother. Cancer 7:49. doi: 10.1186/s40425-019-0522-3
Budhwani, M., Mazzieri, R., and Dolcetti, R. (2018). Plasticity of Type I interferon-mediated responses in cancer therapy: from anti-tumor immunity to resistance. Front. Oncol. 8:322. doi: 10.3389/fonc.2018.00322
Butterfield, L. H., Zhao, F., Lee, S., Tarhini, A. A., Margolin, K. A., White, R. L., et al. (2017). Immune correlates of GM-CSF and melanoma peptide vaccination in a randomized trial for the adjuvant therapy of resected high-risk melanoma (E4697). Clin. Cancer Res. 23, 5034–5043. doi: 10.1158/1078-0432.CCR-16-3016
Caligiuri, M. A. (2008). Human natural killer cells. Blood 112, 461–469. doi: 10.1182/blood-2007-09-077438
Caligiuri, M. A., Murray, C., Robertson, M. J., Wang, E., Cochran, K., Cameron, C., et al. (1993). Selective modulation of human natural-killer-cells invivo after prolonged infusion of low-dose recombinant interleukin-2. J. Clin. Invest. 91, 123–132. doi: 10.1172/Jci116161
Campbell, J. J., Murphy, K. E., Kunkel, E. J., Brightling, C. E., Soler, D., Shen, Z., et al. (2001). CCR7 expression and memory T cell diversity in humans. J. Immunol. 166, 877–884. doi: 10.4049/jimmunol.166.2.877
Campbell, K. S., and Purdy, A. K. (2011). Structure/function of human killer cell immunoglobulin-like receptors: lessons from polymorphisms, evolution, crystal structures and mutations. Immunology 132, 315–325. doi: 10.1111/j.1365-2567.2010.03398.x
Carrega, P., Bonaccorsi, I., Di Carlo, E., Morandi, B., Paul, P., Rizzello, V., et al. (2014). CD56(bright)perforin(low) noncytotoxic human NK cells are abundant in both healthy and neoplastic solid tissues and recirculate to secondary lymphoid organs via afferent lymph. J. Immunol. 192, 3805–3815. doi: 10.4049/jimmunol.1301889
Carrillo-Bustamante, P., Kesmir, C., and de Boer, R. J. (2016). The evolution of natural killer cell receptors. Immunogenetics 68, 3–18. doi: 10.1007/s00251-015-0869-7
Casetti, R., Agrati, C., Wallace, M., Sacchi, A., Martini, F., Martino, A., et al. (2009). Cutting edge: TGF-beta1 and IL-15 Induce FOXP3+ gammadelta regulatory T cells in the presence of antigen stimulation. J. Immunol. 183, 3574–3577. doi: 10.4049/jimmunol.0901334
Chan, W. K., Rujkijyanont, P., Neale, G., Yang, J., Bari, R., Das Gupta, N., et al. (2013). Multiplex and genome-wide analyses reveal distinctive properties of KIR+ and CD56+ T cells in human blood. J. Immunol. 191, 1625–1636. doi: 10.4049/jimmunol.1300111
Chang, A. E., Li, Q., Bishop, D. K., Normolle, D. P., Redman, B. D., and Nickoloff, B. J. (2000). Immunogenetic therapy of human melanoma utilizing autologous tumor cells transduced to secrete granulocyte-macrophage colony-stimulating factor. Hum. Gene Ther. 11, 839–850. doi: 10.1089/10430340050015455
Chang, D. H., Deng, H., Matthews, P., Krasovsky, J., Ragupathi, G., Spisek, R., et al. (2008). Inflammation-associated lysophospholipids as ligands for CD1d-restricted T cells in human cancer. Blood 112, 1308–1316. doi: 10.1182/blood-2008-04-149831
Chaplin, D. D. (2010). Overview of the immune response. J. Allergy Clin. Immunol. 125(2 Suppl. 2), S3–S23. doi: 10.1016/j.jaci.2009.12.980
Chapuis, A. G., Lee, S. M., Thompson, J. A., Roberts, I. M., Margolin, K. A., Bhatia, S., et al. (2016). Combined IL-21-primed polyclonal CTL plus CTLA4 blockade controls refractory metastatic melanoma in a patient. J. Exp. Med. 213, 1133–1139. doi: 10.1084/jem.20152021
Cheema, T. A., Wakimoto, H., Fecci, P. E., Ning, J., Kuroda, T., Jeyaretna, D. S., et al. (2013). Multifaceted oncolytic virus therapy for glioblastoma in an immunocompetent cancer stem cell model. Proc. Natl. Acad. Sci. U.S.A. 110, 12006–12011. doi: 10.1073/pnas.1307935110
Chen, L., and Flies, D. B. (2013). Molecular mechanisms of T cell co-stimulation and co-inhibition. Nat. Rev. Immunol. 13, 227–242. doi: 10.1038/nri3405
Cheng, L. E., Ohlen, C., Nelson, B. H., and Greenberg, P. D. (2002). Enhanced signaling through the IL-2 receptor in CD8+ T cells regulated by antigen recognition results in preferential proliferation and expansion of responding CD8+ T cells rather than promotion of cell death. Proc. Natl. Acad. Sci. U.S.A. 99, 3001–3006. doi: 10.1073/pnas.052676899
Cheuk, S., Schlums, H., Gallais Serezal, I., Martini, E., Chiang, S. C., Marquardt, N., et al. (2017). CD49a expression defines tissue-resident CD8(+) T cells poised for cytotoxic function in human skin. Immunity 46, 287–300. doi: 10.1016/j.immuni.2017.01.009
Chiocca, E. A., Yu, J. S., Lukas, R. V., Solomon, I. H., Ligon, K. L., Nakashima, H., et al. (2019). Regulatable interleukin-12 gene therapy in patients with recurrent high-grade glioma: results of a phase 1 trial. Sci. Transl. Med. 11:eaaw5680. doi: 10.1126/scitranslmed.aaw5680
Choudhry, H., Helmi, N., Abdulaal, W. H., Zeyadi, M., Zamzami, M. A., Wu, W., et al. (2018). Prospects of IL-2 in cancer immunotherapy. Biomed. Res. Int. 2018:9056173. doi: 10.1155/2018/9056173
Chraa, D., Naim, A., Olive, D., and Badou, A. (2019). T lymphocyte subsets in cancer immunity: friends or foes. J. Leukoc. Biol. 105, 243–255. doi: 10.1002/JLB.MR0318-097R
Chuang, Y., Hung, M. E., Cangelose, B. K., and Leonard, J. N. (2016). Regulation of the IL-10-driven macrophage phenotype under incoherent stimuli. Innate Immun. 22, 647–657. doi: 10.1177/1753425916668243
Chulpanova, D. S., Kitaeva, K. V., James, V., Rizvanov, A. A., and Solovyeva, V. V. (2018a). Therapeutic prospects of extracellular vesicles in cancer treatment. Front. Immunol. 9:1534. doi: 10.3389/fimmu.2018.01534
Chulpanova, D. S., Kitaeva, K. V., Tazetdinova, L. G., James, V., Rizvanov, A. A., and Solovyeva, V. V. (2018b). Application of mesenchymal stem cells for therapeutic agent delivery in anti-tumor treatment. Front. Pharmacol. 9:259. doi: 10.3389/fphar.2018.00259
Chulpanova, D. S., Solovyeva, V. V., Kitaeva, K. V., Dunham, S. P., Khaiboullina, S. F., and Rizvanov, A. A. (2018c). Recombinant viruses for cancer. Ther. Biomed. 6:94. doi: 10.3390/biomedicines6040094
Chung, M. J., Park, J. Y., Bang, S., Park, S. W., and Song, S. Y. (2014). Phase II clinical trial of ex vivo-expanded cytokine-induced killer cells therapy in advanced pancreatic cancer. Cancer Immunol. Immunother. 63, 939–946. doi: 10.1007/s00262-014-1566-3
Chung, Y., Chang, S. H., Martinez, G. J., Yang, X. O., Nurieva, R., Kang, H. S., et al. (2009). Critical regulation of early Th17 cell differentiation by interleukin-1 signaling. Immunity 30, 576–587. doi: 10.1016/j.immuni.2009.02.007
Ciurea, S. O., Schafer, J. R., Bassett, R., Denman, C. J., Cao, K., Willis, D., et al. (2017). Phase 1 clinical trial using mbIL21 ex vivo-expanded donor-derived NK cells after haploidentical transplantation. Blood 130, 1857–1868. doi: 10.1182/blood-2017-05-785659
Clavarino, G., Delouche, N., Vettier, C., Laurin, D., Pernollet, M., Raskovalova, T., et al. (2016). Novel strategy for phenotypic characterization of human B lymphocytes from precursors to effector cells by flow cytometry. PLoS One 11:e0162209. doi: 10.1371/journal.pone.0162209
Clifton, G. T., Peace, K. M., Holmes, J. P., Vreeland, T. J., Hale, D. F., Herbert, G. S., et al. (2019). Initial safety analysis of a randomized phase II trial of nelipepimut-S+GM-CSF and trastuzumab compared to trastuzumab alone to prevent recurrence in breast cancer patients with HER2 low-expressing tumors. Clin. Immunol. 201, 48–54. doi: 10.1016/j.clim.2019.02.011
Coffelt, S. B., Wellenstein, M. D., and de Visser, K. E. (2016). Neutrophils in cancer: neutral no more. Nat. Rev. Cancer 16, 431–446. doi: 10.1038/nrc.2016.52
Conlon, K. C., Lugli, E., Welles, H. C., Rosenberg, S. A., Fojo, A. T., Morris, J. C., et al. (2015). Redistribution, hyperproliferation, activation of natural killer cells and CD8 T cells, and cytokine production during first-in-human clinical trial of recombinant human interleukin-15 in patients with cancer. J. Clin. Oncol. 33, 74–82. doi: 10.1200/JCO.2014.57.3329
Cooley, S., He, F., Bachanova, V., Vercellotti, G. M., DeFor, T. E., Curtsinger, J. M., et al. (2019). First-in-human trial of rhIL-15 and haploidentical natural killer cell therapy for advanced acute myeloid leukemia. Blood Adv. 3, 1970–1980. doi: 10.1182/bloodadvances.2018028332
Coquet, J. M., Skak, K., Davis, I. D., Smyth, M. J., and Godfrey, D. I. (2013). IL-21 modulates activation of NKT cells in patients with stage IV malignant melanoma. Clin. Transl. Immunol. 2:e6. doi: 10.1038/cti.2013.7
Cornish, G. H., Sinclair, L. V., and Cantreli, D. A. (2006). Differential regulation of T-cell growth by IL-2 and IL-15. Blood 108, 600–608. doi: 10.1182/blood-2005-12-4827
Curiel, T. J., Coukos, G., Zou, L., Alvarez, X., Cheng, P., Mottram, P., et al. (2004). Specific recruitment of regulatory T cells in ovarian carcinoma fosters immune privilege and predicts reduced survival. Nat. Med. 10, 942–949. doi: 10.1038/nm1093
Dasgupta, S., and Kumar, V. (2016). Type II NKT cells: a distinct CD1d-restricted immune regulatory NKT cell subset. Immunogenetics 68, 665–676. doi: 10.1007/s00251-016-0930-1
Davis, I. D., Brady, B., Kefford, R. F., Millward, M., Cebon, J., Skrumsager, B. K., et al. (2009). Clinical and biological efficacy of recombinant human interleukin-21 in patients with stage IV malignant melanoma without prior treatment: a phase IIa trial. Clin. Cancer Res. 15, 2123–2129. doi: 10.1158/1078-0432.CCR-08-2663
De Silva, N. S., and Klein, U. (2015). Dynamics of B cells in germinal centres. Nat. Rev. Immunol. 15, 137–148. doi: 10.1038/nri3804
Deaglio, S., Dwyer, K. M., Gao, W., Friedman, D., Usheva, A., Erat, A., et al. (2007). Adenosine generation catalyzed by CD39 and CD73 expressed on regulatory T cells mediates immune suppression. J. Exp. Med. 204, 1257–1265. doi: 10.1084/jem.20062512
Deenick, E. K., and Ma, C. S. (2011). The regulation and role of T follicular helper cells in immunity. Immunology 134, 361–367. doi: 10.1111/j.1365-2567.2011.03487.x
Descatoire, M., Weller, S., Irtan, S., Sarnacki, S., Feuillard, J., Storck, S., et al. (2014). Identification of a human splenic marginal zone B cell precursor with NOTCH2-dependent differentiation properties. J. Exp. Med. 211, 987–1000. doi: 10.1084/jem.20132203
Diamond, M. S., Kinder, M., Matsushita, H., Mashayekhi, M., Dunn, G. P., Archambault, J. M., et al. (2011). Type I interferon is selectively required by dendritic cells for immune rejection of tumors. J. Exp. Med. 208, 1989–2003. doi: 10.1084/jem.20101158
Dijkgraaf, E. M., Santegoets, S. J., Reyners, A. K., Goedemans, R., Wouters, M. C., Kenter, G. G., et al. (2015). A phase I trial combining carboplatin/doxorubicin with tocilizumab, an anti-IL-6R monoclonal antibody, and interferon-alpha2b in patients with recurrent epithelial ovarian cancer. Ann. Oncol. 26, 2141–2149. doi: 10.1093/annonc/mdv309
Dillman, R. O., Cornforth, A. N., Nistor, G. I., McClay, E. F., Amatruda, T. T., and Depriest, C. (2018). Randomized phase II trial of autologous dendritic cell vaccines versus autologous tumor cell vaccines in metastatic melanoma: 5-year follow up and additional analyses. J. Immunother. Cancer 6:19. doi: 10.1186/s40425-018-0330-1
Dimova, T., Brouwer, M., Gosselin, F., Tassignon, J., Leo, O., Donner, C., et al. (2015). Effector Vgamma9Vdelta2 T cells dominate the human fetal gammadelta T-cell repertoire. Proc. Natl. Acad. Sci. U.S.A. 112, E556–E565. doi: 10.1073/pnas.1412058112
Ding, B. B., Bi, E., Chen, H., Yu, J. J., and Ye, B. H. (2013). IL-21 and CD40L synergistically promote plasma cell differentiation through upregulation of Blimp-1 in human B cells. J. Immunol. 190, 1827–1836. doi: 10.4049/jimmunol.1201678
Duan, Z., Zheng, H., Xu, S., Jiang, Y., Liu, H., Li, M., et al. (2014). Activation of the Ig Ialpha1 promoter by the transcription factor Ets-1 triggers Ig Ialpha1-Calpha1 germline transcription in epithelial cancer cells. Cell. Mol. Immunol. 11, 197–205. doi: 10.1038/cmi.2013.52
Dubois, S., Conlon, K. C., Muller, J. R., Hsu-Albert, J., Beltran, N., Bryant, B. R., et al. (2017). IL15 Infusion of cancer patients expands the subpopulation of cytotoxic CD56(bright) NK cells and increases NK-cell cytokine release capabilities. Cancer Immunol. Res. 5, 929–938. doi: 10.1158/2326-6066.CIR-17-0279
Duggan, M. C., Jochems, C., Donahue, R. N., Richards, J., Karpa, V., Foust, E., et al. (2016). A phase I study of recombinant (r) vaccinia-CEA(6D)-TRICOM and rFowlpox-CEA(6D)-TRICOM vaccines with GM-CSF and IFN-alpha-2b in patients with CEA-expressing carcinomas. Cancer Immunol. Immunother. 65, 1353–1364. doi: 10.1007/s00262-016-1893-7
Dumauthioz, N., Labiano, S., and Romero, P. (2018). Tumor resident memory T cells: new players in immune surveillance and therapy. Front. Immunol. 9:2076. doi: 10.3389/fimmu.2018.02076
Dunn, G. P., Old, L. J., and Schreiber, R. D. (2004). The three Es of cancer immunoediting. Annu. Rev. Immunol. 22, 329–360. doi: 10.1146/annurev.immunol.22.012703.104803
Eger, K. A., Sundrud, M. S., Motsinger, A. A., Tseng, M., Van Kaer, L., and Unutmaz, D. (2006). Human natural killer T cells are heterogeneous in their capacity to reprogram their effector functions. PLoS One 1:e50. doi: 10.1371/journal.pone.0000050
Elkord, E., Burt, D. J., Sundstedt, A., Nordle, O., Hedlund, G., and Hawkins, R. E. (2015). Immunological response and overall survival in a subset of advanced renal cell carcinoma patients from a randomized phase 2/3 study of naptumomab estafenatox plus IFN-alpha versus IFN-alpha. Oncotarget 6, 4428–4439. doi: 10.18632/oncotarget.2922
Engel, B. C., Laws, H. J., Buttlies, B., Kahn, T., Gobel, U., and Burdach, S. E. (1998). Induction of a CD3+/CD56+ lymphocyte population following gene therapy with transgenic IL-2 secreting fibroblasts in a child with peripheral neuroectodermal malignancy. Med. Pediatr. Oncol. 31, 56–60.
Escriba-Garcia, L., Alvarez-Fernandez, C., Tellez-Gabriel, M., Sierra, J., and Briones, J. (2017). Dendritic cells combined with tumor cells and alpha-galactosylceramide induce a potent, therapeutic and NK-cell dependent antitumor immunity in B cell lymphoma. J. Transl. Med. 15:115. doi: 10.1186/s12967-017-1219-3
Eubank, T. D., Roberts, R. D., Khan, M., Curry, J. M., Nuovo, G. J., Kuppusamy, P., et al. (2009). Granulocyte macrophage colony-stimulating factor inhibits breast cancer growth and metastasis by invoking an anti-angiogenic program in tumor-educated macrophages. Cancer Res. 69, 2133–2140. doi: 10.1158/0008-5472.CAN-08-1405
Euhus, D. M., Kimura, L., and Arnold, B. (1997). Expansion of CD3+CD56+ lymphocytes correlates with induction of cytotoxicity by interleukin-2 gene transfer in human breast tumor cultures. Ann. Surg. Oncol. 4, 432–439.
Exley, M. A., Friedlander, P., Alatrakchi, N., Vriend, L., Yue, S., Sasada, T., et al. (2017). Adoptive transfer of invariant NKT cells as immunotherapy for advanced melanoma: a phase I clinical trial. Clin. Cancer Res. 23, 3510–3519. doi: 10.1158/1078-0432.CCR-16-0600
Eyileten, C., Majchrzak, K., Pilch, Z., Tonecka, K., Mucha, J., Taciak, B., et al. (2016). Immune cells in cancer therapy and drug delivery. Mediators Inflamm. 2016:5230219. doi: 10.1155/2016/5230219
Facchetti, F., Vermi, W., Mason, D., and Colonna, M. (2003). The plasmacytoid monocyte/interferon producing cells. Virchows Arch. 443, 703–717. doi: 10.1007/s00428-003-0918-8
Faith, A., Peek, E., McDonald, J., Urry, Z., Richards, D. F., Tan, C., et al. (2007). Plasmacytoid dendritic cells from human lung cancer draining lymph nodes induce Tc1 responses. Am. J. Respir. Cell Mol. Biol. 36, 360–367. doi: 10.1165/rcmb.2006-0284OC
Fang, Y., Chen, X., Bai, Q., Qin, C., Mohamud, A. O., Zhu, Z., et al. (2015). IL-9 inhibits HTB-72 melanoma cell growth through upregulation of p21 and TRAIL. J. Surg. Oncol. 111, 969–974. doi: 10.1002/jso.23930
Farhood, B., Najafi, M., and Mortezaee, K. (2019). CD8(+) cytotoxic T lymphocytes in cancer immunotherapy: a review. J. Cell. Physiol. 234, 8509–8521. doi: 10.1002/jcp.27782
Fehniger, T. A., Cooper, M. A., Nuovo, G. J., Cella, M., Facchetti, F., Colonna, M., et al. (2003). CD56(bright) natural killer cells are present in human lymph nodes and are activated by T cell-derived IL-2: a potential new link between adaptive and innate immunity. Blood 101, 3052–3057. doi: 10.1182/blood-2002-09-2876
Fichtner-Feigl, S., Terabe, M., Kitani, A., Young, C. A., Fuss, I., Geissler, E. K., et al. (2008). Restoration of tumor immunosurveillance via targeting of interleukin-13 receptor-alpha 2. Cancer Res. 68, 3467–3475. doi: 10.1158/0008-5472.CAN-07-5301
Flores-Borja, F., Bosma, A., Ng, D., Reddy, V., Ehrenstein, M. R., Isenberg, D. A., et al. (2013). CD19+CD24hiCD38hi B cells maintain regulatory T cells while limiting TH1 and TH17 differentiation. Sci. Transl. Med. 5:173ra123. doi: 10.1126/scitranslmed.3005407
Fridlender, Z. G., Sun, J., Kim, S., Kapoor, V., Cheng, G., Ling, L., et al. (2009). Polarization of tumor-associated neutrophil phenotype by TGF-beta: “N1” versus “N2” TAN. Cancer Cell 16, 183–194. doi: 10.1016/j.ccr.2009.06.017
Gabriele, L., Borghi, P., Rozera, C., Sestili, P., Andreotti, M., Guarini, A., et al. (2004). IFN-alpha promotes the rapid differentiation of monocytes from patients with chronic myeloid leukemia into activated dendritic cells tuned to undergo full maturation after LPS treatment. Blood 103, 980–987. doi: 10.1182/blood-2003-03-0981
Gajewski, T. F., and Fitch, F. W. (1988). Anti-proliferative effect of IFN-gamma in immune regulation. I. IFN-gamma inhibits the proliferation of Th2 but not Th1 murine helper T lymphocyte clones. J. Immunol. 140, 4245–4252.
Gardner, A., and Ruffell, B. (2016). Dendritic cells and cancer immunity. Trends Immunol. 37, 855–865. doi: 10.1016/j.it.2016.09.006
Geginat, J., Paroni, M., Maglie, S., Alfen, J. S., Kastirr, I., Gruarin, P., et al. (2014). Plasticity of human CD4 T cell subsets. Front. Immunol. 5:630. doi: 10.3389/fimmu.2014.00630
Gocheva, V., Wang, H. W., Gadea, B. B., Shree, T., Hunter, K. E., Garfall, A. L., et al. (2010). IL-4 induces cathepsin protease activity in tumor-associated macrophages to promote cancer growth and invasion. Genes Dev. 24, 241–255. doi: 10.1101/gad.1874010
Golden, E. B., Chhabra, A., Chachoua, A., Adams, S., Donach, M., Fenton-Kerimian, M., et al. (2015). Local radiotherapy and granulocyte-macrophage colony-stimulating factor to generate abscopal responses in patients with metastatic solid tumours: a proof-of-principle trial. Lancet Oncol. 16, 795–803. doi: 10.1016/S1470-2045(15)00054-6
Gopisetty, A., Bhattacharya, P., Haddad, C., Bruno, J. C. Jr., Vasu, C., Miele, L., et al. (2013). OX40L/Jagged1 cosignaling by GM-CSF-induced bone marrow-derived dendritic cells is required for the expansion of functional regulatory T cells. J. Immunol. 190, 5516–5525. doi: 10.4049/jimmunol.1202298
Granot, Z., Henke, E., Comen, E. A., King, T. A., Norton, L., and Benezra, R. (2011). Tumor entrained neutrophils inhibit seeding in the premetastatic lung. Cancer Cell 20, 300–314. doi: 10.1016/j.ccr.2011.08.012
Granucci, F., Vizzardelli, C., Pavelka, N., Feau, S., Persico, M., Virzi, E., et al. (2001). Inducible IL-2 production by dendritic cells revealed by global gene expression analysis. Nat. Immunol. 2, 882–888. doi: 10.1038/ni0901-882
Gray, J. E., Chiappori, A., Williams, C. C., Tanvetyanon, T., Haura, E. B., Creelan, B. C., et al. (2018). A phase I/randomized phase II study of GM.CD40L vaccine in combination with CCL21 in patients with advanced lung adenocarcinoma. Cancer Immunol. Immunother. 67, 1853–1862. doi: 10.1007/s00262-018-2236-7
Greene, J. M., Schneble, E. J., Jackson, D. O., Hale, D. F., Vreeland, T. J., Flores, M., et al. (2016). A phase I/IIa clinical trial in stage IV melanoma of an autologous tumor-dendritic cell fusion (dendritoma) vaccine with low dose interleukin-2. Cancer Immunol. Immunother. 65, 383–392. doi: 10.1007/s00262-016-1809-6
Greten, T. F., Zhao, F., Gamrekelashvili, J., and Korangy, F. (2012). Human Th17 cells in patients with cancer: Friends or foe? Oncoimmunology 1, 1438–1439. doi: 10.4161/onci.21245
Hansen, M. H., Nielsen, H., and Ditzel, H. J. (2001). The tumor-infiltrating B cell response in medullary breast cancer is oligoclonal and directed against the autoantigen actin exposed on the surface of apoptotic cancer cells. Proc. Natl. Acad. Sci. U.S.A. 98, 12659–12664. doi: 10.1073/pnas.171460798
Hawkins, R. E., Gore, M., Shparyk, Y., Bondar, V., Gladkov, O., Ganev, T., et al. (2016). A randomized phase II/III study of naptumomab estafenatox + IFNalpha versus IFNalpha in renal cell carcinoma: final analysis with baseline biomarker subgroup and trend analysis. Clin. Cancer Res. 22, 3172–3181. doi: 10.1158/1078-0432.CCR-15-0580
He, N., Jin, Q., Wang, D., Huang, Y., Wu, K., Shi, L., et al. (2016). [Effect of tumor-associated macrophages on invasion and metastasis of gastric cancer cells]. Zhonghua Wei Chang Wai Ke Za Zhi 19, 793–797.
Hershko, A. Y., Suzuki, R., Charles, N., Alvarez-Errico, D., Sargent, J. L., Laurence, A., et al. (2011). Mast cell interleukin-2 production contributes to suppression of chronic allergic dermatitis. Immunity 35, 562–571. doi: 10.1016/j.immuni.2011.07.013
Hervas-Stubbs, S., Riezu-Boj, J. I., Gonzalez, I., Mancheno, U., Dubrot, J., Azpilicueta, A., et al. (2010). Effects of IFN-alpha as a signal-3 cytokine on human naive and antigen-experienced CD8(+) T cells. Eur. J. Immunol. 40, 3389–3402. doi: 10.1002/eji.201040664
Hiasa, M., Abe, M., Nakano, A., Oda, A., Amou, H., Kido, S., et al. (2009). GM-CSF and IL-4 induce dendritic cell differentiation and disrupt osteoclastogenesis through M-CSF receptor shedding by up-regulation of TNF-alpha converting enzyme (TACE). Blood 114, 4517–4526. doi: 10.1182/blood-2009-04-215020
Hinrichs, C. S., Spolski, R., Paulos, C. M., Gattinoni, L., Kerstann, K. W., Palmer, D. C., et al. (2008). IL-2 and IL-21 confer opposing differentiation programs to CD8(+) T cells for adoptive immunotherapy. Blood 111, 5326–5333. doi: 10.1182/blood-2007-09-113050
Hirakawa, M., Matos, T., Liu, H. Y., Koreth, J., Kim, H. T., Paul, N. E., et al. (2016). Low-dose IL-2 selectively activates subsets of CD4(+) Tregs and NK cells. JCI Insight 1:e89278. doi: 10.1172/jci.insight.89278
Horikoshi, M., Goto, D., Segawa, S., Yoshiga, Y., Iwanami, K., Inoue, A., et al. (2012). Activation of Invariant NKT cells with glycolipid ligand alpha-galactosylceramide ameliorates glucose-6-phosphate isomerase peptide-induced arthritis. PLoS One 7:e51215. doi: 10.1371/journal.pone.0051215
Hromadnikova, I., Li, S., Kotlabova, K., and Dickinson, A. M. (2016). Influence of In Vitro IL-2 or IL-15 alone or in combination with Hsp 70 derived 14-Mer Peptide (TKD) on the expression of NK cell activatory and inhibitory receptors on peripheral blood T cells, B cells and NKT cells. PLoS One 11:e0151535. doi: 10.1371/journal.pone.0151535
Hu, J. M., Liu, K., Liu, J. H., Jiang, X. L., Wang, X. L., Chen, Y. Z., et al. (2017). CD163 as a marker of M2 macrophage, contribute to predicte aggressiveness and prognosis of Kazakh esophageal squamous cell carcinoma. Oncotarget 8, 21526–21538. doi: 10.18632/oncotarget.15630
Huber, J. P., and Farrar, J. D. (2011). Regulation of effector and memory T-cell functions by type I interferon. Immunology 132, 466–474. doi: 10.1111/j.1365-2567.2011.03412.x
Huber, J. P., Ramos, H. J., Gill, M. A., and Farrar, J. D. (2010). Cutting edge: type I IFN reverses human Th2 commitment and stability by suppressing GATA3. J. Immunol. 185, 813–817. doi: 10.4049/jimmunol.1000469
Ishihara, S., Nieda, M., Kitayama, J., Osada, T., Yabe, T., Kikuchi, A., et al. (2000). Alpha-glycosylceramides enhance the antitumor cytotoxicity of hepatic lymphocytes obtained from cancer patients by activating CD3-CD56+ NK cells in vitro. J. Immunol. 165, 1659–1664. doi: 10.4049/jimmunol.165.3.1659
Italian Cooperative Study Group on Chronic Myeloid Leukemia, Tura, S., Baccarani, M., Zuffa, E., Russo, D., Fanin, R., et al. (1994). Interferon alfa-2a as compared with conventional chemotherapy for the treatment of chronic myeloid leukemia. N. Engl. J. Med. 330, 820–825. doi: 10.1056/NEJM199403243301204
Jablonska, J., Leschner, S., Westphal, K., Lienenklaus, S., and Weiss, S. (2010). Neutrophils responsive to endogenous IFN-beta regulate tumor angiogenesis and growth in a mouse tumor model. J. Clin. Invest. 120, 1151–1164. doi: 10.1172/JCI37223
Jia, Y., Zeng, Z., Li, Y., Li, Z., Jin, L., Zhang, Z., et al. (2015). Impaired function of CD4+ T follicular helper (Tfh) cells associated with hepatocellular carcinoma progression. PLoS One 10:e0117458. doi: 10.1371/journal.pone.0117458
Jin, Z., Fan, J., Zhang, Y., Yi, Y., Wang, L., Yin, D., et al. (2017). Comparison of morphology, phenotypes and function between cultured human IL4DC and IFNDC. Mol. Med. Rep. 16, 7345–7354. doi: 10.3892/mmr.2017.7581
Kara, E. E., Comerford, I., Bastow, C. R., Fenix, K. A., Litchfield, W., Handel, T. M., et al. (2013). Distinct chemokine receptor axes regulate Th9 cell trafficking to allergic and autoimmune inflammatory sites. J. Immunol. 191, 1110–1117. doi: 10.4049/jimmunol.1203089
Kawano, T., Cui, J., Koezuka, Y., Toura, I., Kaneko, Y., Motoki, K., et al. (1997). CD1d-restricted and TCR-mediated activation of valpha14 NKT cells by glycosylceramides. Science 278, 1626–1629. doi: 10.1126/science.278.5343.1626
Kemp, T. J., Moore, J. M., and Griffith, T. S. (2004). Human B cells express functional TRAIL/Apo-2 ligand after CpG-containing oligodeoxynucleotide stimulation. J. Immunol. 173, 892–899. doi: 10.4049/jimmunol.173.2.892
Kennedy, M. K., Picha, K. S., Shanebeck, K. D., Anderson, D. M., and Grabstein, K. H. (1994). Interleukin-12 regulates the proliferation of Th1, but not Th2 or Th0, clones. Eur. J. Immunol. 24, 2271–2278. doi: 10.1002/eji.1830241002
Kerkar, S. P., Muranski, P., Kaiser, A., Boni, A., Sanchez-Perez, L., Yu, Z., et al. (2010). Tumor-specific CD8+ T cells expressing interleukin-12 eradicate established cancers in lymphodepleted hosts. Cancer Res. 70, 6725–6734. doi: 10.1158/0008-5472.CAN-10-0735
Kilinc, M. O., Rowswell-Turner, R. B., Gu, T., Virtuoso, L. P., and Egilmez, N. K. (2009). Activated CD8+ T-effector/memory cells eliminate CD4+ CD25+ Foxp3+ T-suppressor cells from tumors via FasL mediated apoptosis. J. Immunol. 183, 7656–7660. doi: 10.4049/jimmunol.0902625
Kim, C. H., Rott, L., Kunkel, E. J., Genovese, M. C., Andrew, D. P., Wu, L., et al. (2001). Rules of chemokine receptor association with T cell polarization in vivo. J. Clin. Invest. 108, 1331–1339. doi: 10.1172/JCI13543
Kim, H. J., and Cantor, H. (2014). CD4 T-cell subsets and tumor immunity: the helpful and the not-so-helpful. Cancer Immunol. Res. 2, 91–98. doi: 10.1158/2326-6066.CIR-13-0216
Kirkwood, J. M., Bryant, J., Schiller, J. H., Oken, M. M., Borden, E. C., and Whiteside, T. L. (1997). Immunomodulatory function of interferon-gamma in patients with metastatic melanoma: results of a phase II-B trial in subjects with metastatic melanoma, ECOG study E 4987. Eastern cooperative oncology group. J Immunother. 20, 146–157.
Kitaeva, K. V., Prudnikov, T. S., Gomzikova, M. O., Kletukhina, S. K., James, V., Rizvanov, A. A., et al. (2019). Analysis of the interaction and proliferative activity of adenocarcinoma, peripheral blood mononuclear and mesenchymal stromal cells after co-cultivation in vitro. BioNanoScience 9, 502–509. doi: 10.1007/s12668-019-00625-z
Kitayama, S., Zhang, R., Liu, T. Y., Ueda, N., Iriguchi, S., Yasui, Y., et al. (2016). Cellular adjuvant properties, direct cytotoxicity of re-differentiated Valpha24 invariant NKT-like cells from human induced pluripotent stem cells. Stem Cell Rep. 6, 213–227. doi: 10.1016/j.stemcr.2016.01.005
Klebanoff, C. A., Gattinoni, L., Torabi-Parizi, P., Kerstann, K., Cardones, A. R., Finkelstein, S. E., et al. (2005). Central memory self/tumor-reactive CD8+ T cells confer superior antitumor immunity compared with effector memory T cells. Proc. Natl. Acad. Sci. U.S.A. 102, 9571–9576. doi: 10.1073/pnas.0503726102
Knudson, K. M., Hicks, K. C., Alter, S., Schlom, J., and Gameiro, S. R. (2019). Mechanisms involved in IL-15 superagonist enhancement of anti-PD-L1 therapy. J. Immunother. Cancer 7:82. doi: 10.1186/s40425-019-0551-y
Ko, H. J., Brady, J. L., Ryg-Cornejo, V., Hansen, D. S., Vremec, D., Shortman, K., et al. (2014). GM-CSF-responsive monocyte-derived dendritic cells are pivotal in Th17 pathogenesis. J. Immunol. 192, 2202–2209. doi: 10.4049/jimmunol.1302040
Korn, T., Bettelli, E., Gao, W., Awasthi, A., Jager, A., Strom, T. B., et al. (2007). IL-21 initiates an alternative pathway to induce proinflammatory T(H)17 cells. Nature 448, 484–487. doi: 10.1038/nature05970
Kubota, K., Moriyama, M., Furukawa, S., Rafiul, H., Maruse, Y., Jinno, T., et al. (2017). CD163(+)CD204(+) tumor-associated macrophages contribute to T cell regulation via interleukin-10 and PD-L1 production in oral squamous cell carcinoma. Sci. Rep. 7:1755. doi: 10.1038/s41598-017-01661-z
Kuhn, S., Yang, J., and Ronchese, F. (2015). Monocyte-derived dendritic cells are essential for CD8(+) T cell activation and antitumor responses after local immunotherapy. Front. Immunol. 6:584. doi: 10.3389/fimmu.2015.00584
Kumar, A., Suryadevara, N., Hill, T. M., Bezbradica, J. S., Van Kaer, L., and Joyce, S. (2017). Natural killer T cells: an ecological evolutionary developmental biology perspective. Front. Immunol. 8:1858. doi: 10.3389/fimmu.2017.01858
Kumar, S., Mohan, A., and Guleria, R. (2009). Prognostic implications of circulating anti-p53 antibodies in lung cancer–a review. Eur. J. Cancer Care 18, 248–254. doi: 10.1111/j.1365-2354.2008.01019.x
Kurd, N., and Robey, E. A. (2016). T-cell selection in the thymus: a spatial and temporal perspective. Immunol. Rev. 271, 114–126. doi: 10.1111/imr.12398
Ladanyi, A., Somlai, B., Gilde, K., Fejos, Z., Gaudi, I., and Timar, J. (2004). T-cell activation marker expression on tumor-infiltrating lymphocytes as prognostic factor in cutaneous malignant melanoma. Clin. Cancer Res. 10, 521–530.
Ladenstein, R., Potschger, U., Valteau-Couanet, D., Luksch, R., Castel, V., Yaniv, I., et al. (2018). Interleukin 2 with anti-GD2 antibody ch14.18/CHO (dinutuximab beta) in patients with high-risk neuroblastoma (HR-NBL1/SIOPEN): a multicentre, randomised, phase 3 trial. Lancet Oncol. 19, 1617–1629. doi: 10.1016/S1470-2045(18)30578-3
Lafont, V., Liautard, J., Liautard, J. P., and Favero, J. (2001). Production of TNF-alpha by human V gamma 9V delta 2 T cells via engagement of Fc gamma RIIIA, the low affinity type 3 receptor for the Fc portion of IgG, expressed upon TCR activation by nonpeptidic antigen. J. Immunol. 166, 7190–7199. doi: 10.4049/jimmunol.166.12.7190
Lakschevitz, F. S., Hassanpour, S., Rubin, A., Fine, N., Sun, C., and Glogauer, M. (2016). Identification of neutrophil surface marker changes in health and inflammation using high-throughput screening flow cytometry. Exp. Cell Res. 342, 200–209. doi: 10.1016/j.yexcr.2016.03.007
Langowski, J. L., Zhang, X., Wu, L., Mattson, J. D., Chen, T., Smith, K., et al. (2006). IL-23 promotes tumour incidence and growth. Nature 442, 461–465. doi: 10.1038/nature04808
Lasek, W., Zagozdzon, R., and Jakobisiak, M. (2014). Interleukin 12: still a promising candidate for tumor immunotherapy? Cancer Immunol. Immunother. 63, 419–435. doi: 10.1007/s00262-014-1523-1
LeBien, T. W., and Tedder, T. F. (2008). B lymphocytes: how they develop and function. Blood 112, 1570–1580. doi: 10.1182/blood-2008-02-078071
Lehmann, D., Spanholtz, J., Sturtzel, C., Tordoir, M., Schlechta, B., Groenewegen, D., et al. (2014). IL-12 directs further maturation of ex vivo differentiated NK cells with improved therapeutic potential. PLoS One 9:e87131. doi: 10.1371/journal.pone.0087131
Leonard, J. P., Sherman, M. L., Fisher, G. L., Buchanan, L. J., Larsen, G., Atkins, M. B., et al. (1997). Effects of single-dose interleukin-12 exposure on interleukin-12-associated toxicity and interferon-gamma production. Blood 90, 2541–2548.
Levi, I., Amsalem, H., Nissan, A., Darash-Yahana, M., Peretz, T., Mandelboim, O., et al. (2015). Characterization of tumor infiltrating natural killer cell subset. Oncotarget 6, 13835–13843. doi: 10.18632/oncotarget.3453
Levings, M. K., Bacchetta, R., Schulz, U., and Roncarolo, M. G. (2002). The role of IL-10 and TGF-beta in the differentiation and effector function of T regulatory cells. Int. Arch. Allergy Immunol. 129, 263–276. doi: 10.1159/000067596
Li, L., Jay, S. M., Wang, Y., Wu, S. W., and Xiao, Z. (2017). IL-12 stimulates CTLs to secrete exosomes capable of activating bystander CD8(+) T cells. Sci. Rep. 7:13365. doi: 10.1038/s41598-017-14000-z
Li, T., Jia, D. D., and Teng, L. S. (2020). Adjuvant pembrolizumab versus high-dose interferon alpha-2b for Chinese patients with resected stage III melanoma: a retrospective cohort study. Invest. New Drugs. doi: 10.1007/s10637-020-00913-6
Li, W., Katz, B. P., and Spinola, S. M. (2012). Haemophilus ducreyi-induced interleukin-10 promotes a mixed M1 and M2 activation program in human macrophages. Infect. Immun. 80, 4426–4434. doi: 10.1128/IAI.00912-12
Li, Z., Pang, Y., Gara, S. K., Achyut, B. R., Heger, C., Goldsmith, P. K., et al. (2012). Gr-1+CD11b+ cells are responsible for tumor promoting effect of TGF-beta in breast cancer progression. Int. J. Cancer 131, 2584–2595. doi: 10.1002/ijc.27572
Linde, N., Lederle, W., Depner, S., van Rooijen, N., Gutschalk, C. M., and Mueller, M. M. (2012). Vascular endothelial growth factor-induced skin carcinogenesis depends on recruitment and alternative activation of macrophages. J. Pathol. 227, 17–28. doi: 10.1002/path.3989
Liu, E., Marin, D., Banerjee, P., Macapinlac, H. A., Thompson, P., Basar, R., et al. (2020). Use of CAR-transduced natural killer cells in CD19-positive lymphoid tumors. N. Engl. J. Med. 382, 545–553. doi: 10.1056/NEJMoa1910607
Liu, K. J., Chao, T. Y., Chang, J. Y., Cheng, A. L., Ch’ang, H. J., Kao, W. Y., et al. (2016). A phase I clinical study of immunotherapy for advanced colorectal cancers using carcinoembryonic antigen-pulsed dendritic cells mixed with tetanus toxoid and subsequent IL-2 treatment. J. Biomed. Sci. 23:64. doi: 10.1186/s12929-016-0279-7
Liu, Y., and Zeng, G. (2012). Cancer and innate immune system interactions: translational potentials for cancer immunotherapy. J. Immunother. 35, 299–308. doi: 10.1097/CJI.0b013e3182518e83
Lombardi, V. C., Khaiboullina, S. F., and Rizvanov, A. A. (2015). Plasmacytoid dendritic cells, a role in neoplastic prevention and progression. Eur. J. Clin. Invest. 45(Suppl. 1), 1–8. doi: 10.1111/eci.12363
Lu, C., Klement, J. D., Ibrahim, M. L., Xiao, W., Redd, P. S., Nayak-Kapoor, A., et al. (2019). Type I interferon suppresses tumor growth through activating the STAT3-granzyme B pathway in tumor-infiltrating cytotoxic T lymphocytes. J. Immunother. Cancer 7:157. doi: 10.1186/s40425-019-0635-8
Lv, L., Pan, K., Li, X. D., She, K. L., Zhao, J. J., Wang, W., et al. (2011). The accumulation and prognosis value of tumor infiltrating IL-17 producing cells in esophageal squamous cell carcinoma. PLoS One 6:e18219. doi: 10.1371/journal.pone.0018219
Maeurer, M. J., Martin, D., Walter, W., Liu, K., Zitvogel, L., Halusczcak, K., et al. (1996). Human intestinal Vdelta1+ lymphocytes recognize tumor cells of epithelial origin. J. Exp. Med. 183, 1681–1696. doi: 10.1084/jem.183.4.1681
Mahnke, Y. D., Brodie, T. M., Sallusto, F., Roederer, M., and Lugli, E. (2013). The who’s who of T-cell differentiation: human memory T-cell subsets. Eur. J. Immunol. 43, 2797–2809. doi: 10.1002/eji.201343751
Malchow, S., Leventhal, D. S., Nishi, S., Fischer, B. I., Shen, L., Paner, G. P., et al. (2013). Aire-dependent thymic development of tumor-associated regulatory T cells. Science 339, 1219–1224. doi: 10.1126/science.1233913
Maldonado, R. A., and von Andrian, U. H. (2010). How tolerogenic dendritic cells induce regulatory T cells. Adv. Immunol. 108, 111–165. doi: 10.1016/B978-0-12-380995-7.00004-5
Margolin, K., Morishima, C., Velcheti, V., Miller, J. S., Lee, S. M., Silk, A. W., et al. (2018). Phase I Trial of ALT-803, a novel recombinant IL15 complex, in patients with advanced solid tumors. Clin. Cancer Res. 24, 5552–5561. doi: 10.1158/1078-0432.CCR-18-0945
Martinet, L., and Smyth, M. J. (2015). Balancing natural killer cell activation through paired receptors. Nat. Rev. Immunol. 15, 243–254. doi: 10.1038/nri3799
Martinovic, K. M., Babovic, N., Dzodic, R., Jurisic, V., Matkovic, S., and Konjevic, G. (2015). Favorable in vitro effects of combined IL-12 and IL-18 treatment on NK cell cytotoxicity and CD25 receptor expression in metastatic melanoma patients. J. Transl. Med. 13:120. doi: 10.1186/S12967-015-0479-Z
Mattarollo, S. R., Kenna, T., Nieda, M., and Nicol, A. J. (2007). Chemotherapy and zoledronate sensitize solid tumour cells to Vgamma9Vdelta2 T cell cytotoxicity. Cancer Immunol. Immunother. 56, 1285–1297. doi: 10.1007/s00262-007-0279-2
McMichael, E. L., Benner, B., Atwal, L. S., Courtney, N. B., Mo, X., Davis, M. E., et al. (2019). A phase I/II trial of cetuximab in combination with interleukin-12 administered to patients with unresectable primary or recurrent head and neck squamous cell carcinoma. Clin. Cancer Res. 25, 4955–4965. doi: 10.1158/1078-0432.CCR-18-2108
Metelitsa, L. S., Naidenko, O. V., Kant, A., Wu, H. W., Loza, M. J., Perussia, B., et al. (2001). Human NKT cells mediate antitumor cytotoxicity directly by recognizing target cell CD1d with bound ligand or indirectly by producing IL-2 to activate NK cells. J. Immunol. 167, 3114–3122. doi: 10.4049/jimmunol.167.6.3114
Miliotou, A. N., and Papadopoulou, L. C. (2018). CAR T-cell therapy: a new era in cancer immunotherapy. Curr. Pharm. Biotechnol. 19, 5–18. doi: 10.2174/1389201019666180418095526
Miller, J. S., Morishima, C., McNeel, D. G., Patel, M. R., Kohrt, H. E. K., Thompson, J. A., et al. (2018). A first-in-human phase i study of subcutaneous outpatient recombinant human IL15 (rhIL15) in adults with advanced solid tumors. Clin. Cancer Res. 24, 1525–1535. doi: 10.1158/1078-0432.CCR-17-2451
Mills, C. D., Kincaid, K., Alt, J. M., Heilman, M. J., and Hill, A. M. (2000). M-1/M-2 macrophages and the Th1/Th2 paradigm. J. Immunol. 164, 6166–6173. doi: 10.4049/jimmunol.164.12.6166
Mirjacic Martinovic, K. M., Babovic, N., Dzodic, R. R., Jurisic, V. B., Tanic, N. T., and Konjevic, G. M. (2014). Decreased expression of NKG2D, NKp46, DNAM-1 receptors, and intracellular perforin and STAT-1 effector molecules in NK cells and their dim and bright subsets in metastatic melanoma patients. Melanoma Res. 24, 295–304. doi: 10.1097/CMR.0000000000000072
Mitchell, D., Chintala, S., and Dey, M. (2018). Plasmacytoid dendritic cell in immunity and cancer. J. Neuroimmunol. 322, 63–73. doi: 10.1016/j.jneuroim.2018.06.012
Moldenhauer, L. M., Keenihan, S. N., Hayball, J. D., and Robertson, S. A. (2010). GM-CSF is an essential regulator of T cell activation competence in uterine dendritic cells during early pregnancy in mice. J. Immunol. 185, 7085–7096. doi: 10.4049/jimmunol.1001374
Montoya, C. J., Pollard, D., Martinson, J., Kumari, K., Wasserfall, C., Mulder, C. B., et al. (2007). Characterization of human invariant natural killer T subsets in health and disease using a novel invariant natural killer T cell-clonotypic monoclonal antibody, 6B11. Immunology 122, 1–14. doi: 10.1111/j.1365-2567.2007.02647.x
Mukhopadhyay, A., Wright, J., Shirley, S., Canton, D. A., Burkart, C., Connolly, R. J., et al. (2019). Characterization of abscopal effects of intratumoral electroporation-mediated IL-12 gene therapy. Gene Ther. 26, 1–15. doi: 10.1038/s41434-018-0044-5
Muller, E., Speth, M., Christopoulos, P. F., Lunde, A., Avdagic, A., Oynebraten, I., et al. (2018). Both type I and type II interferons can activate antitumor M1 macrophages when combined with TLR stimulation. Front. Immunol. 9:2520. doi: 10.3389/fimmu.2018.02520
Muller, L., Aigner, P., and Stoiber, D. (2017). Type I interferons and natural killer cell regulation in cancer. Front. Immunol. 8:304. doi: 10.3389/fimmu.2017.00304
Murphy, T. L., Grajales-Reyes, G. E., Wu, X., Tussiwand, R., Briseno, C. G., Iwata, A., et al. (2016). Transcriptional control of dendritic cell development. Annu. Rev. Immunol. 34, 93–119. doi: 10.1146/annurev-immunol-032713-120204
Murray, P. J., Allen, J. E., Biswas, S. K., Fisher, E. A., Gilroy, D. W., Goerdt, S., et al. (2014). Macrophage activation and polarization: nomenclature and experimental guidelines. Immunity 41, 14–20. doi: 10.1016/j.immuni.2014.06.008
Naumova, E., Mihaylova, A., Ivanova, M., and Mihailova, S. (2007). Impact of KIR/HLA ligand combinations on immune responses in malignant melanoma. Cancer Immunol. Immunother. 56, 95–100. doi: 10.1007/s00262-006-0151-9
Nguyen, H. M., Guz-Montgomery, K., and Saha, D. (2020). Oncolytic virus encoding a master pro-inflammatory cytokine interleukin 12 in cancer immunotherapy. Cells 9:400. doi: 10.3390/cells9020400
Ni, C., Wu, P., Wu, X., Zhang, T., Zhang, T., Wang, Z., et al. (2015). Thymosin alpha1 enhanced cytotoxicity of iNKT cells against colon cancer via upregulating CD1d expression. Cancer Lett. 356(2 Pt B), 579–588. doi: 10.1016/j.canlet.2014.10.002
Nishimura, T., Kitamura, H., Iwakabe, K., Yahata, T., Ohta, A., Sato, M., et al. (2000). The interface between innate and acquired immunity: glycolipid antigen presentation by CD1d-expressing dendritic cells to NKT cells induces the differentiation of antigen-specific cytotoxic T lymphocytes. Int. Immunol. 12, 987–994. doi: 10.1093/intimm/12.7.987
Nozawa, H., Chiu, C., and Hanahan, D. (2006). Infiltrating neutrophils mediate the initial angiogenic switch in a mouse model of multistage carcinogenesis. Proc. Natl. Acad. Sci. U.S.A. 103, 12493–12498. doi: 10.1073/pnas.0601807103
Ocana, A., Nieto-Jimenez, C., Pandiella, A., and Templeton, A. J. (2017). Neutrophils in cancer: prognostic role and therapeutic strategies. Mol. Cancer 16:137. doi: 10.1186/s12943-017-0707-7
Opata, M. M., and Stephens, R. (2013). Early decision: effector and effector memory T cell differentiation in chronic infection. Curr. Immunol. Rev. 9, 190–206. doi: 10.2174/1573395509666131126231209
Oriss, T. B., McCarthy, S. A., Morel, B. F., Campana, M. A., and Morel, P. A. (1997). Crossregulation between T helper cell (Th)1 and Th2: inhibition of Th2 proliferation by IFN-gamma involves interference with IL-1. J. Immunol. 158, 3666–3672.
O’Sullivan, T., Saddawi-Konefka, R., Vermi, W., Koebel, C. M., Arthur, C., White, J. M., et al. (2012). Cancer immunoediting by the innate immune system in the absence of adaptive immunity. J. Exp. Med. 209, 1869–1882. doi: 10.1084/jem.20112738
Oudard, S., Rixe, O., Beuselinck, B., Linassier, C., Banu, E., Machiels, J. P., et al. (2011). A phase II study of the cancer vaccine TG4010 alone and in combination with cytokines in patients with metastatic renal clear-cell carcinoma: clinical and immunological findings. Cancer Immunol. Immunother. 60, 261–271. doi: 10.1007/s00262-010-0935-9
Paliard, X., de Waal Malefijt, R., Yssel, H., Blanchard, D., Chretien, I., Abrams, J., et al. (1988). Simultaneous production of IL-2, IL-4, and IFN-gamma by activated human CD4+ and CD8+ T cell clones. J. Immunol. 141, 849–855.
Pang, D. J., Neves, J. F., Sumaria, N., and Pennington, D. J. (2012). Understanding the complexity of gammadelta T-cell subsets in mouse and human. Immunology 136, 283–290. doi: 10.1111/j.1365-2567.2012.03582.x
Paquette, R. L., Hsu, N., Said, J., Mohammed, M., Rao, N. P., Shih, G., et al. (2002). Interferon-alpha induces dendritic cell differentiation of CML mononuclear cells in vitro and in vivo. Leukemia 16, 1484–1489. doi: 10.1038/sj.leu.2402602
Pascutti, M. F., Jak, M., Tromp, J. M., Derks, I. A., Remmerswaal, E. B., Thijssen, R., et al. (2013). IL-21 and CD40L signals from autologous T cells can induce antigen-independent proliferation of CLL cells. Blood 122, 3010–3019. doi: 10.1182/blood-2012-11-467670
Patel, D. M., Foreman, P. M., Nabors, L. B., Riley, K. O., Gillespie, G. Y., and Markert, J. M. (2016). Design of a phase I clinical trial to evaluate M032, a genetically engineered HSV-1 expressing IL-12, in patients with recurrent/progressive glioblastoma multiforme, anaplastic astrocytoma, or gliosarcoma. Hum. Gene Ther. Clin. Dev. 27, 69–78. doi: 10.1089/humc.2016.031
Patil, R. S., Shah, S. U., Shrikhande, S. V., Goel, M., Dikshit, R. P., and Chiplunkar, S. V. (2016). IL17 producing gammadeltaT cells induce angiogenesis and are associated with poor survival in gallbladder cancer patients. Int. J. Cancer 139, 869–881. doi: 10.1002/ijc.30134
Peng, Y. P., Zhu, Y., Zhang, J. J., Xu, Z. K., Qian, Z. Y., Dai, C. C., et al. (2013). Comprehensive analysis of the percentage of surface receptors and cytotoxic granules positive natural killer cells in patients with pancreatic cancer, gastric cancer, and colorectal cancer. J. Transl. Med. 11:262. doi: 10.1186/1479-5876-11-262
Perez-Martinez, A., Fernandez, L., Valentin, J., Martinez-Romera, I., Corral, M. D., Ramirez, M., et al. (2015). A phase I/II trial of interleukin-15–stimulated natural killer cell infusion after haplo-identical stem cell transplantation for pediatric refractory solid tumors. Cytotherapy 17, 1594–1603. doi: 10.1016/j.jcyt.2015.07.011
Picker, L. J., Treer, J. R., Ferguson-Darnell, B., Collins, P. A., Buck, D., and Terstappen, L. W. (1993). Control of lymphocyte recirculation in man. I. Differential regulation of the peripheral lymph node homing receptor L-selectin on T cells during the virgin to memory cell transition. J. Immunol. 150, 1105–1121.
Poli, A., Michel, T., Theresine, M., Andres, E., Hentges, F., and Zimmer, J. (2009). CD56bright natural killer (NK) cells: an important NK cell subset. Immunology 126, 458–465. doi: 10.1111/j.1365-2567.2008.03027.x
Poulin, L. F., Salio, M., Griessinger, E., Anjos-Afonso, F., Craciun, L., Chen, J. L., et al. (2010). Characterization of human DNGR-1+ BDCA3+ leukocytes as putative equivalents of mouse CD8alpha+ dendritic cells. J. Exp. Med. 207, 1261–1271. doi: 10.1084/jem.20092618
Prochazkova, J., Pokorna, K., and Holan, V. (2012). IL-12 inhibits the TGF-beta-dependent T cell developmental programs and skews the TGF-beta-induced differentiation into a Th1-like direction. Immunobiology 217, 74–82. doi: 10.1016/j.imbio.2011.07.032
Purwar, R., Schlapbach, C., Xiao, S., Kang, H. S., Elyaman, W., Jiang, X., et al. (2012). Robust tumor immunity to melanoma mediated by interleukin-9-producing T cells. Nat. Med. 18, 1248–1253. doi: 10.1038/nm.2856
Qian, B. Z., Li, J., Zhang, H., Kitamura, T., Zhang, J., Campion, L. R., et al. (2011). CCL2 recruits inflammatory monocytes to facilitate breast-tumour metastasis. Nature 475, 222–225. doi: 10.1038/nature10138
Qin, Z., and Blankenstein, T. (2000). CD4+ T cell–mediated tumor rejection involves inhibition of angiogenesis that is dependent on IFN gamma receptor expression by nonhematopoietic cells. Immunity 12, 677–686.
Quezada, S. A., Peggs, K. S., Curran, M. A., and Allison, J. P. (2006). CTLA4 blockade and GM-CSF combination immunotherapy alters the intratumor balance of effector and regulatory T cells. J. Clin. Invest. 116, 1935–1945. doi: 10.1172/JCI27745
Raphael, I., Nalawade, S., Eagar, T. N., and Forsthuber, T. G. (2015). T cell subsets and their signature cytokines in autoimmune and inflammatory diseases. Cytokine 74, 5–17. doi: 10.1016/j.cyto.2014.09.011
Ravaud, A., Barrios, C. H., Alekseev, B., Tay, M. H., Agarwala, S. S., Yalcin, S., et al. (2015). RECORD-2: phase II randomized study of everolimus and bevacizumab versus interferon alpha-2a and bevacizumab as first-line therapy in patients with metastatic renal cell carcinoma. Ann. Oncol. 26, 1378–1384. doi: 10.1093/annonc/mdv170
Ray, A., Williams, M. A., Meek, S. M., Bowen, R. C., Grossmann, K. F., Andtbacka, R. H., et al. (2016). A phase I study of intratumoral ipilimumab and interleukin-2 in patients with advanced melanoma. Oncotarget 7, 64390–64399. doi: 10.18632/oncotarget.10453
Reading, J. L., Galvez-Cancino, F., Swanton, C., Lladser, A., Peggs, K. S., and Quezada, S. A. (2018). The function and dysfunction of memory CD8(+) T cells in tumor immunity. Immunol. Rev. 283, 194–212. doi: 10.1111/imr.12657
Ren, F., Fan, M., Mei, J., Wu, Y., Liu, C., Pu, Q., et al. (2014). Interferon-gamma and celecoxib inhibit lung-tumor growth through modulating M2/M1 macrophage ratio in the tumor microenvironment. Drug Des. Devel. Ther. 8, 1527–1538. doi: 10.2147/DDDT.S66302
Restifo, N. P., and Gattinoni, L. (2013). Lineage relationship of effector and memory T cells. Curr. Opin. Immunol. 25, 556–563. doi: 10.1016/j.coi.2013.09.003
Ribot, J. C., Ribeiro, S. T., Correia, D. V., Sousa, A. E., and Silva-Santos, B. (2014). Human gamma delta thymocytes are functionally immature and differentiate into cytotoxic type 1 effector T cells upon IL-2/IL-15 signaling. J. Immunol. 192, 2237–2243. doi: 10.4049/jimmunol.1303119
Ricciardi, M., Zanotto, M., Malpeli, G., Bassi, G., Perbellini, O., Chilosi, M., et al. (2015). Epithelial-to-mesenchymal transition (EMT) induced by inflammatory priming elicits mesenchymal stromal cell-like immune-modulatory properties in cancer cells. Br. J. Cancer 112, 1067–1075. doi: 10.1038/bjc.2015.29
Ridolfi, L., de Rosa, F., Ridolfi, R., Gentili, G., Valmorri, L., Scarpi, E., et al. (2014). Radiotherapy as an immunological booster in patients with metastatic melanoma or renal cell carcinoma treated with high-dose Interleukin-2: evaluation of biomarkers of immunologic and therapeutic response. J. Transl. Med. 12:262. doi: 10.1186/s12967-014-0262-6
Rivera Vargas, T., Humblin, E., Vegran, F., Ghiringhelli, F., and Apetoh, L. (2017). TH9 cells in anti-tumor immunity. Semin. Immunopathol. 39, 39–46. doi: 10.1007/s00281-016-0599-4
Roberts, E. W., Broz, M. L., Binnewies, M., Headley, M. B., Nelson, A. E., Wolf, D. M., et al. (2016). Critical role for CD103(+)/CD141(+) dendritic cells bearing CCR7 for tumor antigen trafficking and priming of T cell immunity in melanoma. Cancer Cell 30, 324–336. doi: 10.1016/j.ccell.2016.06.003
Robertson, M. J., Cameron, C., Atkins, M. B., Gordon, M. S., Lotze, M. T., Sherman, M. L., et al. (1999). Immunological effects of interleukin 12 administered by bolus intravenous injection to patients with cancer. Clin. Cancer Res. 5, 9–16.
Robinson, T. O., and Schluns, K. S. (2017). The potential and promise of IL-15 in immuno-oncogenic therapies. Immunol. Lett. 190, 159–168. doi: 10.1016/j.imlet.2017.08.010
Rosental, B., Brusilovsky, M., Hadad, U., Oz, D., Appel, M. Y., Afergan, F., et al. (2011). Proliferating cell nuclear antigen is a novel inhibitory ligand for the natural cytotoxicity receptor NKp44. J. Immunol. 187, 5693–5702. doi: 10.4049/jimmunol.1102267
Rosser, E. C., and Mauri, C. (2015). Regulatory B cells: origin, phenotype, and function. Immunity 42, 607–612. doi: 10.1016/j.immuni.2015.04.005
Rubinstein, M. P., Su, E. W., Suriano, S., Cloud, C. A., Andrijauskaite, K., Kesarwani, P., et al. (2015). Interleukin-12 enhances the function and anti-tumor activity in murine and human CD8(+) T cells. Cancer Immunol. Immunother. 64, 539–549. doi: 10.1007/s00262-015-1655-y
Salgado, F. J., Lojo, J., Fernandez-Alonso, C. M., Vinuela, J., Cordero, O. J., and Nogueira, M. (2002). Interleukin-dependent modulation of HLA-DR expression on CD4and CD8 activated T cells. Immunol. Cell Biol. 80, 138–147. doi: 10.1046/j.1440-1711.2002.01055.x
Sallusto, F., Mackay, C. R., and Lanzavecchia, A. (1997). Selective expression of the eotaxin receptor CCR3 by human T helper 2 cells. Science 277, 2005–2007. doi: 10.1126/science.277.5334.2005
Sandoval-Montes, C., and Santos-Argumedo, L. (2005). CD38 is expressed selectively during the activation of a subset of mature T cells with reduced proliferation but improved potential to produce cytokines. J. Leukoc. Biol. 77, 513–521. doi: 10.1189/jlb.0404262
Santegoets, S. J., Turksma, A. W., Powell, D. J. Jr., Hooijberg, E., and de Gruijl, T. D. (2013). IL-21 in cancer immunotherapy: At the right place at the right time. Oncoimmunology 2:e24522. doi: 10.4161/onci.24522
Sarvaria, A., Madrigal, J. A., and Saudemont, A. (2017). B cell regulation in cancer and anti-tumor immunity. Cell. Mol. Immunol. 14, 662–674. doi: 10.1038/cmi.2017.35
Schiavoni, G., Gabriele, L., and Mattei, F. (2013). The tumor microenvironment: a pitch for multiple players. Front. Oncol. 3:90. doi: 10.3389/fonc.2013.00090
Schwaab, T., Schwarzer, A., Wolf, B., Crocenzi, T. S., Seigne, J. D., Crosby, N. A., et al. (2009). Clinical and immunologic effects of intranodal autologous tumor lysate-dendritic cell vaccine with Aldesleukin (Interleukin 2) and IFN-{alpha}2a therapy in metastatic renal cell carcinoma patients. Clin. Cancer Res. 15, 4986–4992. doi: 10.1158/1078-0432.CCR-08-3240
Segura, E., and Amigorena, S. (2013). Inflammatory dendritic cells in mice and humans. Trends Immunol. 34, 440–445. doi: 10.1016/j.it.2013.06.001
Segura, E., Touzot, M., Bohineust, A., Cappuccio, A., Chiocchia, G., Hosmalin, A., et al. (2013). Human inflammatory dendritic cells induce Th17 cell differentiation. Immunity 38, 336–348. doi: 10.1016/j.immuni.2012.10.018
Semmrich, M., Smith, A., Feterowski, C., Beer, S., Engelhardt, B., Busch, D. H., et al. (2005). Importance of integrin LFA-1 deactivation for the generation of immune responses. J. Exp. Med. 201, 1987–1998. doi: 10.1084/jem.20041850
Sharma, S., Yang, S. C., Zhu, L., Reckamp, K., Gardner, B., Baratelli, F., et al. (2005). Tumor cyclooxygenase-2/prostaglandin E2-dependent promotion of FOXP3 expression and CD4+ CD25+ T regulatory cell activities in lung cancer. Cancer Res. 65, 5211–5220. doi: 10.1158/0008-5472.CAN-05-0141
Sheng, L., Chen, X., Wang, Q., Lyu, S., and Li, P. (2020). Interferon-alpha2b enhances survival and modulates transcriptional profiles and the immune response in melanoma patients treated with dendritic cell vaccines. Biomed. Pharmacother. 125:109966. doi: 10.1016/j.biopha.2020.109966
Shi, W., Dong, L., Sun, Q., Ding, H., Meng, J., and Dai, G. (2018). Follicular helper T cells promote the effector functions of CD8(+) T cells via the provision of IL-21, which is downregulated due to PD-1/PD-L1-mediated suppression in colorectal cancer. Exp. Cell Res. 372, 35–42. doi: 10.1016/j.yexcr.2018.09.006
Shi, Y., Dincheva-Vogel, L., Ayemoba, C. E., Fung, J. P., Bergamaschi, C., Pavlakis, G. N., et al. (2018). IL-15/IL-15Ralpha/CD80-expressing AML cell vaccines eradicate minimal residual disease in leukemic mice. Blood Adv. 2, 3177–3192. doi: 10.1182/bloodadvances.2018019026
Shields, J. D., Kourtis, I. C., Tomei, A. A., Roberts, J. M., and Swartz, M. A. (2010). Induction of lymphoidlike stroma and immune escape by tumors that express the chemokine CCL21. Science 328, 749–752. doi: 10.1126/science.1185837
Shima, H., Tsurita, G., Wada, S., Hirohashi, Y., Yasui, H., Hayashi, H., et al. (2019). Randomized phase II trial of survivin 2B peptide vaccination for patients with HLA-A24-positive pancreatic adenocarcinoma. Cancer Sci. 110, 2378–2385. doi: 10.1111/cas.14106
Shinde, P., Fernandes, S., Melinkeri, S., Kale, V., and Limaye, L. (2018). Compromised functionality of monocyte-derived dendritic cells in multiple myeloma patients may limit their use in cancer immunotherapy. Sci. Rep. 8:5705. doi: 10.1038/s41598-018-23943-w
Siegel, R. M., Chan, F. K., Chun, H. J., and Lenardo, M. J. (2000). The multifaceted role of Fas signaling in immune cell homeostasis and autoimmunity. Nat. Immunol. 1, 469–474. doi: 10.1038/82712
Sikora, A. G., Jaffarzad, N., Hailemichael, Y., Gelbard, A., Stonier, S. W., Schluns, K. S., et al. (2009). IFN-alpha enhances peptide vaccine-induced CD8+ T cell numbers, effector function, and antitumor activity. J. Immunol. 182, 7398–7407. doi: 10.4049/jimmunol.0802982
Silk, A. W., Kaufman, H. L., Curti, B., Mehnert, J. M., Margolin, K., McDermott, D., et al. (2019). High-dose ipilimumab and high-dose interleukin-2 for patients with advanced melanoma. Front. Oncol. 9:1483. doi: 10.3389/fonc.2019.01483
Singel, K. L., and Segal, B. H. (2016). Neutrophils in the tumor microenvironment: trying to heal the wound that cannot heal. Immunol. Rev. 273, 329–343. doi: 10.1111/imr.12459
Sisirak, V., Faget, J., Vey, N., Blay, J. Y., Menetrier-Caux, C., Caux, C., et al. (2013). Plasmacytoid dendritic cells deficient in IFNalpha production promote the amplification of FOXP3(+) regulatory T cells and are associated with poor prognosis in breast cancer patients. Oncoimmunology 2:e22338. doi: 10.4161/onci.22338
Skak, K., Frederiksen, K. S., and Lundsgaard, D. (2008). Interleukin-21 activates human natural killer cells and modulates their surface receptor expression. Immunology 123, 575–583. doi: 10.1111/j.1365-2567.2007.02730.x
Slingluff, C. L. Jr., Petroni, G. R., Yamshchikov, G. V., Barnd, D. L., Eastham, S., Galavotti, H., et al. (2003). Clinical and immunologic results of a randomized phase II trial of vaccination using four melanoma peptides either administered in granulocyte-macrophage colony-stimulating factor in adjuvant or pulsed on dendritic cells. J. Clin. Oncol. 21, 4016–4026. doi: 10.1200/JCO.2003.10.005
Spolski, R., Kim, H. P., Zhu, W., Levy, D. E., and Leonard, W. J. (2009). IL-21 mediates suppressive effects via its induction of IL-10. J. Immunol. 182, 2859–2867. doi: 10.4049/jimmunol.0802978
Spranger, S., and Gajewski, T. F. (2018). Impact of oncogenic pathways on evasion of antitumour immune responses. Nat. Rev. Cancer 18, 139–147. doi: 10.1038/nrc.2017.117
Stabile, H., Fionda, C., Gismondi, A., and Santoni, A. (2017). Role of distinct natural killer cell subsets in anticancer response. Front. Immunol. 8:293. doi: 10.3389/fimmu.2017.00293
Steele, N., Anthony, A., Saunders, M., Esmarck, B., Ehrnrooth, E., Kristjansen, P. E., et al. (2012). A phase 1 trial of recombinant human IL-21 in combination with cetuximab in patients with metastatic colorectal cancer. Br. J. Cancer 106, 793–798. doi: 10.1038/bjc.2011.599
Steinbrink, K., Graulich, E., Kubsch, S., Knop, J., and Enk, A. H. (2002). CD4(+) and CD8(+) anergic T cells induced by interleukin-10-treated human dendritic cells display antigen-specific suppressor activity. Blood 99, 2468–2476. doi: 10.1182/blood.v99.7.2468
Stoppacciaro, A., Melani, C., Parenza, M., Mastracchio, A., Bassi, C., Baroni, C., et al. (1993). Regression of an established tumor genetically modified to release granulocyte colony-stimulating factor requires granulocyte-T cell cooperation and T cell-produced interferon gamma. J. Exp. Med. 178, 151–161. doi: 10.1084/jem.178.1.151
Takahashi, H., Sakakura, K., Kudo, T., Toyoda, M., Kaira, K., Oyama, T., et al. (2017). Cancer-associated fibroblasts promote an immunosuppressive microenvironment through the induction and accumulation of protumoral macrophages. Oncotarget 8, 8633–8647. doi: 10.18632/oncotarget.14374
Tanaka, Y., Morita, C. T., Tanaka, Y., Nieves, E., Brenner, M. B., and Bloom, B. R. (1995). Natural and synthetic non-peptide antigens recognized by human gamma delta T cells. Nature 375, 155–158. doi: 10.1038/375155a0
Tarhini, A. A., Frankel, P., Ruel, C., Ernstoff, M. S., Kuzel, T. M., Logan, T. F., et al. (2018). NCI 8628: A randomized phase 2 study of ziv-aflibercept and high-dose interleukin 2 or high-dose interleukin 2 alone for inoperable stage III or IV melanoma. Cancer 124, 4332–4341. doi: 10.1002/cncr.31734
Tarhini, A. A., Lee, S. J., Hodi, F. S., Rao, U. N. M., Cohen, G. I., Hamid, O., et al. (2020). Phase III study of adjuvant ipilimumab (3 or 10 mg/kg) versus high-dose interferon Alfa-2b for resected high-risk melanoma: north american intergroup E1609. J. Clin. Oncol. 38, 567–575. doi: 10.1200/JCO.19.01381
Tartour, E., Fossiez, F., Joyeux, I., Galinha, A., Gey, A., Claret, E., et al. (1999). Interleukin 17, a T-cell-derived cytokine, promotes tumorigenicity of human cervical tumors in nude mice. Cancer Res. 59, 3698–3704.
Tel, J., Smits, E. L., Anguille, S., Joshi, R. N., Figdor, C. G., and de Vries, I. J. (2012). Human plasmacytoid dendritic cells are equipped with antigen-presenting and tumoricidal capacities. Blood 120, 3936–3944. doi: 10.1182/blood-2012-06-435941
Tepper, R. I., Coffman, R. L., and Leder, P. (1992). An eosinophil-dependent mechanism for the antitumor effect of interleukin-4. Science 257, 548–551. doi: 10.1126/science.1636093
Terabe, M., Matsui, S., Park, J. M., Mamura, M., Noben-Trauth, N., Donaldson, D. D., et al. (2003). Transforming growth factor-beta production and myeloid cells are an effector mechanism through which CD1d-restricted T cells block cytotoxic T lymphocyte-mediated tumor immunosurveillance: abrogation prevents tumor recurrence. J. Exp. Med. 198, 1741–1752. doi: 10.1084/jem.20022227
Terme, M., and Tanchot, C. (2017). [Immune system and tumors]. Ann. Pathol. 37, 11–17. doi: 10.1016/j.annpat.2016.12.004
Thommen, D. S., Koelzer, V. H., Herzig, P., Roller, A., Trefny, M., Dimeloe, S., et al. (2018). A transcriptionally and functionally distinct PD-1(+) CD8(+) T cell pool with predictive potential in non-small-cell lung cancer treated with PD-1 blockade. Nat. Med. 24, 994–1004. doi: 10.1038/s41591-0.18-0057-z
Thompson, J. A., Curti, B. D., Redman, B. G., Bhatia, S., Weber, J. S., Agarwala, S. S., et al. (2008). Phase I study of recombinant interleukin-21 in patients with metastatic melanoma and renal cell carcinoma. J. Clin. Oncol. 26, 2034–2039. doi: 10.1200/JCO.2007.14.5193
Timmerman, J. M., Byrd, J. C., Andorsky, D. J., Yamada, R. E., Kramer, J., Muthusamy, N., et al. (2012). A phase I dose-finding trial of recombinant interleukin-21 and rituximab in relapsed and refractory low grade B-cell lymphoproliferative disorders. Clin. Cancer Res. 18, 5752–5760. doi: 10.1158/1078-0432.CCR-12-0456
Todaro, M., D’Asaro, M., Caccamo, N., Iovino, F., Francipane, M. G., Meraviglia, S., et al. (2009). Efficient killing of human colon cancer stem cells by gammadelta T lymphocytes. J. Immunol. 182, 7287–7296. doi: 10.4049/jimmunol.0804288
Topalian, S. L., Taube, J. M., Anders, R. A., and Pardoll, D. M. (2016). Mechanism-driven biomarkers to guide immune checkpoint blockade in cancer therapy. Nat. Rev. Cancer 16, 275–287. doi: 10.1038/nrc.2016.36
Tsou, P., Katayama, H., Ostrin, E. J., and Hanash, S. M. (2016). The emerging role of B cells in tumor immunity. Cancer Res. 76, 5597–5601. doi: 10.1158/0008-5472.CAN-16-0431
Ushach, I., and Zlotnik, A. (2016). Biological role of granulocyte macrophage colony-stimulating factor (GM-CSF) and macrophage colony-stimulating factor (M-CSF) on cells of the myeloid lineage. J. Leukoc. Biol. 100, 481–489. doi: 10.1189/jlb.3RU0316-144R
van den Heuvel, M. M., Verheij, M., Boshuizen, R., Belderbos, J., Dingemans, A. M., De Ruysscher, D., et al. (2015). NHS-IL2 combined with radiotherapy: preclinical rationale and phase Ib trial results in metastatic non-small cell lung cancer following first-line chemotherapy. J. Transl. Med. 13:32. doi: 10.1186/s12967-015-0397-0
Veglia, F., and Gabrilovich, D. I. (2017). Dendritic cells in cancer: the role revisited. Curr. Opin. Immunol. 45, 43–51. doi: 10.1016/j.coi.2017.01.002
Vegran, F., Berger, H., Boidot, R., Mignot, G., Bruchard, M., Dosset, M., et al. (2014). The transcription factor IRF1 dictates the IL-21-dependent anticancer functions of TH9 cells. Nat. Immunol. 15, 758–766. doi: 10.1038/ni.2925
Vela, M., Corral, D., Carrasco, P., Fernandez, L., Valentin, J., Gonzalez, B., et al. (2018). Haploidentical IL-15/41BBL activated and expanded natural killer cell infusion therapy after salvage chemotherapy in children with relapsed and refractory leukemia. Cancer Lett. 422, 107–117. doi: 10.1016/j.canlet.2018.02.033
Veldhoen, M., Uyttenhove, C., van Snick, J., Helmby, H., Westendorf, A., Buer, J., et al. (2008). Transforming growth factor-beta ‘reprograms’ the differentiation of T helper 2 cells and promotes an interleukin 9-producing subset. Nat. Immunol. 9, 1341–1346. doi: 10.1038/ni.1659
Vogelzang, A., McGuire, H. M., Yu, D., Sprent, J., Mackay, C. R., and King, C. (2008). A fundamental role for interleukin-21 in the generation of T follicular helper cells. Immunity 29, 127–137. doi: 10.1016/j.immuni.2008.06.001
Wagner, J. A., Rosario, M., Romee, R., Berrien-Elliott, M. M., Schneider, S. E., Leong, J. W., et al. (2017). CD56bright NK cells exhibit potent antitumor responses following IL-15 priming. J. Clin. Invest. 127, 4042–4058. doi: 10.1172/JCI90387
Wakabayashi, T., Natsume, A., Mizusawa, J., Katayama, H., Fukuda, H., Sumi, M., et al. (2018). JCOG0911 INTEGRA study: a randomized screening phase II trial of interferonbeta plus temozolomide in comparison with temozolomide alone for newly diagnosed glioblastoma. J. Neurooncol. 138, 627–636. doi: 10.1007/s11060-018-2831-7
Waldmann, T. A. (2018). Cytokines in cancer immunotherapy. Cold Spring Harb. Perspect. Biol. 10:a028472. doi: 10.1101/cshperspect.a028472
Wang, H. W., and Joyce, J. A. (2010). Alternative activation of tumor-associated macrophages by IL-4: priming for protumoral functions. Cell Cycle 9, 4824–4835. doi: 10.4161/cc.9.24.14322
Wang, T. T., Zhao, Y. L., Peng, L. S., Chen, N., Chen, W., Lv, Y. P., et al. (2017). Tumour-activated neutrophils in gastric cancer foster immune suppression and disease progression through GM-CSF-PD-L1 pathway. Gut 66, 1900–1911. doi: 10.1136/gutjnl-2016-313075
Wang, Y., Yu, W., Zhu, J., Wang, J., Xia, K., Liang, C., et al. (2019). Anti-CD166/4-1BB chimeric antigen receptor T cell therapy for the treatment of osteosarcoma. J. Exp. Clin. Cancer Res. 38:168. doi: 10.1186/s13046-019-1147-6
Wang, Z., Hong, J., Sun, W., Xu, G., Li, N., Chen, X., et al. (2006). Role of IFN-gamma in induction of Foxp3 and conversion of CD4+ CD25- T cells to CD4+ Tregs. J. Clin. Invest. 116, 2434–2441. doi: 10.1172/JCI25826
Watanabe, M., Fujihara, C., Radtke, A. J., Chiang, Y. J., Bhatia, S., Germain, R. N., et al. (2017). Co-stimulatory function in primary germinal center responses: CD40 and B7 are required on distinct antigen-presenting cells. J. Exp. Med. 214, 2795–2810. doi: 10.1084/jem.20161955
Wei, X., Jin, Y., Tian, Y., Zhang, H., Wu, J., Lu, W., et al. (2016). Regulatory B cells contribute to the impaired antitumor immunity in ovarian cancer patients. Tumour Biol. 37, 6581–6588. doi: 10.1007/s13277-015-4538-0
Weide, B., Eigentler, T., Catania, C., Ascierto, P. A., Cascinu, S., Becker, J. C., et al. (2019). A phase II study of the L19IL2 immunocytokine in combination with dacarbazine in advanced metastatic melanoma patients. Cancer Immunol. Immunother. 68, 1547–1559. doi: 10.1007/s00262-019-02383-z
Weide, B., Martens, A., Wistuba-Hamprecht, K., Zelba, H., Maier, L., Lipp, H. P., et al. (2017). Combined treatment with ipilimumab and intratumoral interleukin-2 in pretreated patients with stage IV melanoma-safety and efficacy in a phase II study. Cancer Immunol. Immunother. 66, 441–449. doi: 10.1007/s00262-016-1944-0
Werchau, S., Toberer, F., Enk, A., Dammann, R., and Helmbold, P. (2012). Merkel cell carcinoma induces lymphatic microvessel formation. J. Am. Acad. Dermatol. 67, 215–225. doi: 10.1016/j.jaad.2011.09.002
West, E. E., Jin, H. T., Rasheed, A. U., Penaloza-Macmaster, P., Ha, S. J., Tan, W. G., et al. (2013). PD-L1 blockade synergizes with IL-2 therapy in reinvigorating exhausted T cells. J. Clin. Invest. 123, 2604–2615. doi: 10.1172/JCI67008
West, W. H. (1989). Continuous infusion recombinant interleukin-2 (rIL-2) in adoptive cellular therapy of renal carcinoma and other malignancies. Cancer Treat. Rev. 16(Suppl. A), 83–89.
Witowski, J., Pawlaczyk, K., Breborowicz, A., Scheuren, A., Kuzlan-Pawlaczyk, M., Wisniewska, J., et al. (2000). IL-17 stimulates intraperitoneal neutrophil infiltration through the release of GRO alpha chemokine from mesothelial cells. J. Immunol. 165, 5814–5821. doi: 10.4049/jimmunol.165.10.5814
Wrangle, J. M., Velcheti, V., Patel, M. R., Garrett-Mayer, E., Hill, E. G., Ravenel, J. G., et al. (2018). ALT-803, an IL-15 superagonist, in combination with nivolumab in patients with metastatic non-small cell lung cancer: a non-randomised, open-label, phase 1b trial. Lancet Oncol. 19, 694–704. doi: 10.1016/S1470-2045(18)30148-7
Wu, P., Wu, D., Ni, C., Ye, J., Chen, W., Hu, G., et al. (2014). gammadeltaT17 cells promote the accumulation and expansion of myeloid-derived suppressor cells in human colorectal cancer. Immunity 40, 785–800. doi: 10.1016/j.immuni.2014.03.013
Wynn, T. A., Chawla, A., and Pollard, J. W. (2013). Macrophage biology in development, homeostasis and disease. Nature 496, 445–455. doi: 10.1038/nature12034
Yang, L., Wang, F., Wang, L., Huang, L., Wang, J., Zhang, B., et al. (2015). CD163+ tumor-associated macrophage is a prognostic biomarker and is associated with therapeutic effect on malignant pleural effusion of lung cancer patients. Oncotarget 6, 10592–10603. doi: 10.18632/oncotarget.3547
Yang, S. X., Wei, W. S., Ouyan, Q. W., Jiang, Q. H., Zou, Y. F., Qu, W., et al. (2016). Interleukin-12 activated CD8(+) T cells induces apoptosis in breast cancer cells and reduces tumor growth. Biomed. Pharmacother. 84, 1466–1471. doi: 10.1016/j.biopha.2016.10.046
Ye, Q., Song, D. G., Poussin, M., Yamamoto, T., Best, A., Li, C., et al. (2014). CD137 accurately identifies and enriches for naturally occurring tumor-reactive T cells in tumor. Clin. Cancer Res. 20, 44–55. doi: 10.1158/1078-0432.CCR-13-0945
Yen, J. H., Kong, W., and Ganea, D. (2010). IFN-beta inhibits dendritic cell migration through STAT-1-mediated transcriptional suppression of CCR7 and matrix metalloproteinase 9. J. Immunol. 184, 3478–3486. doi: 10.4049/jimmunol.0902542
Yoshida, Y., Naito, M., Yamada, T., Aisu, N., Kojima, D., Mera, T., et al. (2017). Clinical study on the medical value of combination therapy involving adoptive immunotherapy and chemotherapy for stage IV colorectal cancer (COMVI Study). Anticancer Res. 37, 3941–3946. doi: 10.21873/anticanres.11777
Yoshizaki, A., Miyagaki, T., DiLillo, D. J., Matsushita, T., Horikawa, M., Kountikov, E. I., et al. (2012). Regulatory B cells control T-cell autoimmunity through IL-21-dependent cognate interactions. Nature 491, 264–268. doi: 10.1038/nature11501
Yu, W. L., and Hua, Z. C. (2018). Evaluation of effectiveness of granulocyte-macrophage colony-stimulating factor therapy to cancer patients after chemotherapy: a meta-analysis. Oncotarget 9, 28226–28239. doi: 10.18632/oncotarget.24890
Yuen, G. J., Demissie, E., and Pillai, S. (2016). B lymphocytes and cancer: a love-hate relationship. Trends Cancer 2, 747–757. doi: 10.1016/j.trecan.2016.10.010
Yui, M. A., Sharp, L. L., Havran, W. L., and Rothenberg, E. V. (2004). Preferential activation of an IL-2 regulatory sequence transgene in TCR gamma delta and NKT cells: subset-specific differences in IL-2 regulation. J. Immunol. 172, 4691–4699.
Zaidi, M. R. (2019). The interferon-gamma paradox in cancer. J. Interferon Cytokine Res. 39, 30–38. doi: 10.1089/jir.2018.0087
Zappasodi, R., Budhu, S., Hellmann, M. D., Postow, M. A., Senbabaoglu, Y., Manne, S., et al. (2018). Non-conventional inhibitory CD4(+)Foxp3(-)PD-1(hi) T cells as a biomarker of immune checkpoint blockade activity. Cancer Cell 33, 1017–1032.e7. doi: 10.1016/j.ccell.2018.05.009
Zhang, G., Nie, H., Yang, J., Ding, X., Huang, Y., Yu, H., et al. (2011). Sulfatide-activated type II NKT cells prevent allergic airway inflammation by inhibiting type I NKT cell function in a mouse model of asthma. Am. J. Physiol. Lung. Cell. Mol. Physiol. 301, L975–L984. doi: 10.1152/ajplung.00114.2011
Zhang, L., Morgan, R. A., Beane, J. D., Zheng, Z., Dudley, M. E., Kassim, S. H., et al. (2015). Tumor-infiltrating lymphocytes genetically engineered with an inducible gene encoding interleukin-12 for the immunotherapy of metastatic melanoma. Clin. Cancer Res. 21, 2278–2288. doi: 10.1158/1078-0432.CCR-14-2085
Zhang, L., Tai, Y. T., Ho, M., Xing, L., Chauhan, D., Gang, A., et al. (2017). Regulatory B cell-myeloma cell interaction confers immunosuppression and promotes their survival in the bone marrow milieu. Blood Cancer J. 7:e547. doi: 10.1038/bcj.2017.24
Zhao, J., Bagchi, S., and Wang, C. R. (2014). Type II natural killer T cells foster the antitumor activity of CpG-oligodeoxynucleotides. Oncoimmunology 3:e28977. doi: 10.4161/onci.28977
Zhou, J., Kang, N., Cui, L., Ba, D., and He, W. (2012). Anti-gammadelta TCR antibody-expanded gammadelta T cells: a better choice for the adoptive immunotherapy of lymphoid malignancies. Cell. Mol. Immunol. 9, 34–44. doi: 10.1038/cmi.2011.16
Zhu, Y., and Chen, L. (2014). CD137 as a biomarker for tumor-reactive T cells: finding gold in the desert. Clin. Cancer Res. 20, 3–5. doi: 10.1158/1078-0432.CCR-13-2573
Zibelman, M. R., Macfarlane, A., Alpaugh, R. K., Dulaimi, E., Costello, K., O’Neill, J., et al. (2017). Effect of exogenous interferon-gamma (IFN-gamma) on peripheral blood immune markers as part of a phase I clinical trial of combined IFN-gamma with nivolumab (Nivo) in patients (pts) with select solid tumors. J. Clin. Oncol. 35:97.
Zingoni, A., Soto, H., Hedrick, J. A., Stoppacciaro, A., Storlazzi, C. T., Sinigaglia, F., et al. (1998). The chemokine receptor CCR8 is preferentially expressed in Th2 but not Th1 cells. J. Immunol. 161, 547–551.
Zorn, E., Nelson, E. A., Mohseni, M., Porcheray, F., Kim, H., Litsa, D., et al. (2006). IL-2 regulates FOXP3 expression in human CD4(+)CD25(+) regulatory T cells through a STAT-dependent mechanism and induces the expansion of these cells in vivo. Blood 108, 1571–1579. doi: 10.1182/blood-2006-02-004747
Zotos, D., Coquet, J. M., Zhang, Y., Light, A., D’Costa, K., Kallies, A., et al. (2010). IL-21 regulates germinal center B cell differentiation and proliferation through a B cell-intrinsic mechanism. J. Exp. Med. 207, 365–378. doi: 10.1084/jem.20091777
Keywords: cancer immunotherapy, cytokines, anticancer immune responses, immune cell markers/populations, tumor microenvironment
Citation: Chulpanova DS, Kitaeva KV, Green AR, Rizvanov AA and Solovyeva VV (2020) Molecular Aspects and Future Perspectives of Cytokine-Based Anti-cancer Immunotherapy. Front. Cell Dev. Biol. 8:402. doi: 10.3389/fcell.2020.00402
Received: 27 November 2019; Accepted: 01 May 2020;
Published: 03 June 2020.
Edited by:
Hasan Korkaya, Augusta University, United StatesReviewed by:
Cristina Maccalli, Sidra Medicine, QatarJoanna Julia Domagala-Kulawik, Medical University of Warsaw, Poland
Copyright © 2020 Chulpanova, Kitaeva, Green, Rizvanov and Solovyeva. This is an open-access article distributed under the terms of the Creative Commons Attribution License (CC BY). The use, distribution or reproduction in other forums is permitted, provided the original author(s) and the copyright owner(s) are credited and that the original publication in this journal is cited, in accordance with accepted academic practice. No use, distribution or reproduction is permitted which does not comply with these terms.
*Correspondence: Valeriya V. Solovyeva, solovyovavv@gmail.com