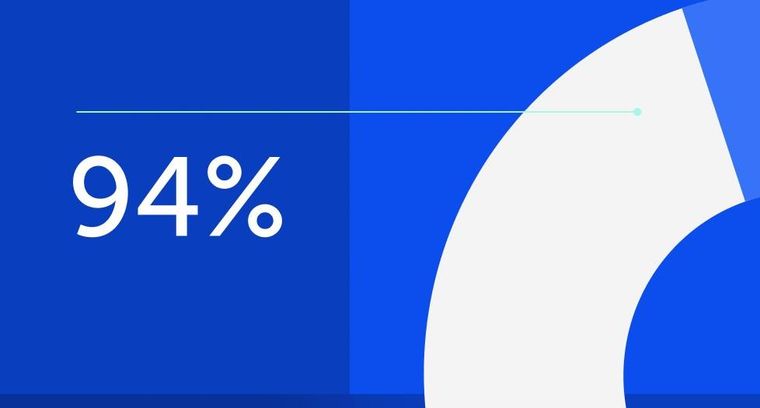
94% of researchers rate our articles as excellent or good
Learn more about the work of our research integrity team to safeguard the quality of each article we publish.
Find out more
REVIEW article
Front. Cell Dev. Biol., 15 November 2019
Sec. Stem Cell Research
Volume 7 - 2019 | https://doi.org/10.3389/fcell.2019.00285
This article is part of the Research TopicImmunomodulatory Properties of Adult Stem Cells: Implications for the Niche Microenvironment in Homeostasis and DiseaseView all 9 articles
Mesenchymal stem cells (MSCs) have a potently immunosuppressive capacity in both innate and adaptive immune responses. Consequently, MSCs transplantation has emerged as a potential beneficial therapy for autoimmune diseases even though the mechanisms underlying the immunomodulatory activity of MSCs is incompletely understood. Transplanted MSCs from healthy individuals with no known history of autoimmune disease are immunosuppressive in systemic lupus erythematosus (SLE) patients and can ameliorate SLE disease symptoms in those same patients. In contrast, autologous MSCs from SLE patients are not immunosuppressive and do not ameliorate disease symptoms. Recent studies have shown that MSCs from SLE patients are dysfunctional in both proliferation and immunoregulation and phenotypically senescent. The senescent phenotype has been attributed to multiple genes and signaling pathways. In this review, we focus on the possible mechanisms for the defective phenotype and function of MSCs from SLE patients and summarize recent research on MSCs in autoimmune diseases.
Systemic autoimmune disease is caused by abnormal immune reactivity and antibody production to self-antigens with subsequent inflammation and damage to host tissues or target organs such as skin, kidney, joints, and muscles (Fanouriakis et al., 2019). Systemic lupus erythematosus (SLE) is a systemic autoimmune disease characterized by aberrant activation of lymphocytes and autoantibody production (Marion and Postlethwaite, 2014). Prominent among the autoantibody specificities in SLE are nuclear antigens including DNA, RNA, ribonuclear proteins, and histones. Pathogenesis in SLE is notoriously heterogeneous and may involve multiple connective tissues, skin, and organ systems. Similarly, the disease heterogeneity complicates diagnosis (Petri et al., 2012). Anti-inflammatory and immunosuppressive drugs have improved survival and prognosis of SLE patients (Bernatsky et al., 2006) and notably in China, according to the statistics of the Chinese SLE Treatment and Research group (CSTAR) (Zhao et al., 2016). Renal disease has the highest standardized mortality ratio in SLE, although patients who fail to respond to conventional therapies or whose disease is accompanied with pulmonary arterial hypertension (PAH) or other serious complications, also have high mortality.
Recently, mesenchymal stem cell (MSC) transplantation has emerged as a promising therapy in refractory SLE patients because of MSCs’ strong immunosuppressive potential (Barbado et al., 2018). MSCs are multipotential, self-replicating stem cells that may differentiate into different mature specialized cells (Weissman and Shizuru, 2008). MSCs can modulate both the adaptive and innate immune system in patients with autoimmune diseases (Munir and McGettrick, 2015), although the intricate details by which MSCs exert their therapeutic effects are not fully understood.
Bone marrow-derived MSCs (BM-MSCs) were effective in clinical trials for steroid-resistant graft-versus-host disease (GVHD), a complication in allogeneic bone marrow transplantation (Le Blanc et al., 2008). The MSCs elicited a suppressive immunoregulatory response that was thought to involve multiple immunosuppressive mechanisms. A 6 years follow-up observational study has provided evidence for the safety and efficacy of allogeneic umbilical cord-derived MSCs transplantation in refractory SLE patients (Wang et al., 2017b). Although a large proportion of the refractory SLE patients attained clinical remission or reduced disease activity after transplantation of allogeneic MSCs from healthy, non-autoimmune individuals, SLE patients benefit little from autologous stem cell transplantation (Barbado et al., 2018), implying that the capabilities of MSCs from SLE patients may be impaired. In this review, we aim to further explore the potential mechanisms that account for the apparent dysfunction of MSCs derived from SLE patients by referring to relevant literature.
Stem cells are a class of undifferentiated cells in multicellular organisms that are pluripotential and self-replicating. Through unequal cell division, stem cells produce one daughter cell with multiple differentiation potential and one identical daughter cell for self-renewal (Weissman and Shizuru, 2008). There are two major types of stem cells in mammals. One is the embryonic stem cell from the inner cell mass of blastocysts that can form cells from all three embryonic germ layers, endoderm, mesoderm, and ectoderm (Thomson et al., 1998). The other is the adult stem cell, which can selectively replenish dying cells and regenerate damaged tissues, for example hematopoietic stem cells (HSC) in bone marrow and intestinal stem cells in the small intestine (van der Flier and Clevers, 2009).
In 1976, multipotential stromal precursor cells were identified by Friedenstein et al. (1976) since the bone marrow cells they cultured differentiated into bone-like and chondrocyte-like cells both in vitro and in vivo. The concept that the multipotential stromal precursor cells, MSC, identified by Friedenstein et al., could be used to therapeutic benefit was raised in 1991 (Caplan, 1991). Bone marrow MSCs can be expanded 104–108-fold in vitro with a Hayflick’s limit of 13–25 doublings (Wagner et al., 2008). In vitro cultured MSC retain multipotent stromal cell potential and may differentiate into multiple mature cell types from mesodermal lineage such as lipocytes, osteoblasts and chondrocytes. The latter potential is what provides them with potential for regenerative and traumatic medicine (see Figure 1).
Figure 1. The multipotentiality and immunomodulatory effects of MSCs. The figure illustrates the multitasking capabilities of MSC. Those capabilities include self-renewal, damaged tissue repair, and multipotential differentiation into multiple mesodermal cell types. MSCs also have immunoregulatory function with potential to inhibit or suppress autoimmune and chronic inflammatory reactions by direct cell contact, paracrine release and secretion, and/or cytokine secretion.
The International Society for Cellular Therapy proposed minimal criteria to define human MSC in 2006 (Dominici et al., 2006). Cultured MSC must be plastic-adherent and capable of differentiating into adipocytes, osteoblasts, and chondroblasts in vitro. Phenotypically, MSC must express CD73, CD90, and CD105 but none of the hematopoietic differentiation markers CD11b, CD14, CD34, CD45, CD19, CD79α, or HLA-DR. Bone marrow was the first identified and, historically, most frequently utilized source of MSCs. More recently, MSCs have been identified in and isolated from umbilical cord, adipose tissue, molar cells, urine, amniotic fluid, and connective tissue (Dong et al., 2018; Xie and Shen, 2018).
MSCs have aroused widespread interest because they are capable of differentiating into both mesenchymal and non-mesenchymal lineages after isolation from several tissues and in vitro expansion. MSCs are considered promising reagents in regenerative medicine and cell-based therapies because of their self-renewal and multilineage potential (Squillaro et al., 2016). The culture environment to which stem cells are exposed is especially relevant for their differentiation. The specific lineages into which naïve MSCs will differentiate and the morphology and phenotypes they will display depend upon differential in vitro culture conditions that may vary among individual donors (Engler et al., 2006; Lin et al., 2017; Stojanovic et al., 2018). The proliferative and differentiative capabilities of MSCs decline with donor age and passage number of MSC cultures in vitro (Infante and Rodriguez, 2018). To obtain functionally differentiated cells or tissues, stem cells have been cultivated in vitro under controlled conditions. The environmental cues (Dinsmore et al., 1996; Liu et al., 2017) that control MSC differentiation include special culture media, various chemical, biological and physical factors, and mechanical stimuli. For instance, osteogenic stimuli such as dexamethasone, ascorbic acid, and β-glycerophosphate can promote the osteogenic differentiation of cultured MSCs. Osteogenic differentiation can be distinguished by the ALP activity, deposition of extracellular calcium, and expression of osteogenic genes. Furthermore, studies revealed that miRNAs and several signaling pathways may affect the regulation of MSC differentiation (Sun X.K. et al., 2017).
MSCs have immunoregulatory effects on multiple immune system cells and functions (see Figure 2). MSCs mediate their immunoregulatory effect by secreting soluble factors or directly interacting with a variety of immune effector cells (Gebler et al., 2012), and it should be emphasized that MSCs are not always immunosuppressive. MSCs may have different properties and immunoregulatory effects depending on the inflammatory milieu and disease setting (Djouad et al., 2005; Zhou et al., 2013; Dorraji et al., 2018). MSCs can suppress proliferation of both CD4 + and CD8 + T lymphocytes in vitro in a dose-dependent, non-apoptotic-induced manner, and the immunosuppressive properties against T cells varies among different MSC sources (Di Nicola et al., 2002; Castro-Manrreza et al., 2014). Transforming growth factor-β (TGF-β), prostaglandin E2 (PGE2), nitric oxide (NO), and indoleamine 2,3-dioxygenase (IDO) were reported to be involved in the MSC-mediated T cell suppression (Aggarwal and Pittenger, 2005; Groh et al., 2005; Sato et al., 2007; Li W. et al., 2012). MSCs can also exert immunoregulatory effects by release of microvesicles (MVs) (Di Trapani et al., 2016) although several studies have substantiated the in vitro superiority 0f MSCs over their MVs for antiproliferation effects on T cells (Gouveia de Andrade et al., 2015; Di Trapani et al., 2016). For that reason, infusion of MVs may not be the ideal substitute for MSCs in affecting immune-mediated disorders where T cells are predominate.
Figure 2. MSC immunoregulatory activities. MSCs can inhibit the proliferation and activation of B and T lymphocytes and NK cells and increase or restore the ratio of Tregs to Th effector cells. MSCs can also promote a switch from pro-inflammatory to anti-inflammatory phenotype and cytokine secretion by T cells, dendritic cells, and natural killer cells.
MSCs secrete numerous cytokines, chemokines, and hormones to exert paracrine effects on adjacent immune cells to modulate their proliferation, differentiation, migration, and adhesion functions under injury conditions (Meirelles Lda et al., 2009). Paracrine effects of MSC have been referred to as trophic effects, which can be divided into immunosuppressive, anti-fibrogenic, anti-apoptotic, pro-angiogenic, and pro-mitogenic functions (Caplan and Dennis, 2006). Several chemokine receptors including CXCR5, CCR1, CCR4, CCR7, and CCR10 are expressed on MSCs, which might be involved in their homeostatic and tissue-specific recruitment (Von Luttichau et al., 2005). In addition, MSCs secret PD-L1 and PD-L2, two ligands of the programmed death-1 (PD-1) receptor, that inhibit activation and proliferation of T cells, suppress T cell effector function, and modulate peripheral tolerance (Davies et al., 2017). The ability of MSCs to be therapeutic without engraftment or differentiation into tissue-specific cells may expand their range of clinical applications.
Different subpopulations of T cells are differentially affected by the immunoregulatory potential of MSCs. IFN-γ and TNF-α synergistically enhanced the ability of MSCs to adhere to Th17 in vitro, inhibited the differentiation of naïve T cells into Th17, and promoted the expression of Foxp3 so as to facilitate differentiation of Th17 toward regulatory T cells (Tregs) that in turn inhibited CD4+ Th effector cell activation (Ghannam et al., 2010). MSCs can inhibit T follicular helper (Tfh) differentiation and IL-21 cytokine production in vitro and in autoimmune MRL-MPlpr/lpr mice in vivo. The in vivo MSCs infusion prolonged life and alleviated lupus nephritis in the autoimmune mice (Yang et al., 2018). Autologous MSCs from two SLE patients were able to inhibit lymphocyte activation in vitro and, when respectively infused, increased the circulating Treg cell number in vivo but had no effect on disease activity (Carrion et al., 2010). In lupus-prone mice, infusion of BM-MSCs did not significantly affect serum anti-dsDNA autoantibody but did result in improved renal histopathology, including reduced immune complex deposition, glomerular proliferation, and lymphocytic infiltration (Schena et al., 2010), all of which are consistent with a potential role for MSCs in the prevention of glomerular damage. Further, the renoprotective effect has been shown to be partially mediated by paracrine effects in mice (Togel et al., 2005). An imbalance in Th1 and Th2 cytokine profiles is suggested to play an important role in the pathogenesis of GVHD, SLE, and other autoimmune diseases. While it is debatable how MSCs modulate and balance the differentiation of the Th1 and Th2 lymphocyte subpopulations, most research on MSC function in immunosuppression has revealed that MSCs exert an immunomodulatory effect by activating or increasing Treg and Th2 and inhibiting or decreasing proinflammatory Th1 and Th17 cells both in vivo and in vitro (Ghannam et al., 2010; Luz-Crawford et al., 2013; Campanati et al., 2017; Sun Y. et al., 2017; Yan et al., 2017). Results from a few studies indicate that MSCs showed no significant effects on the proliferation or secretory function of Th1 or Th2 subsets individually but did cause a global decrease in the ratio of Th1/Th2 cells (Lim et al., 2014; Choi et al., 2016). In contrast at least one study in arthritic mice indicated that MSC transplantation promoted accumulation of Th1 (Gonzalo-Gil et al., 2016). Nevertheless, in general, MSCs can inhibit proinflammatory cytokine secretion and reduce Th1/Th2 ratios.
Currently, there are contradictory viewpoints regarding immunomodulatory properties of MSCs on B lymphocyte proliferation and activation (Corcione et al., 2006; Tabera et al., 2008; Schena et al., 2010). Corcione et al. (2006) observed that MSCs induced inhibitory effects on B cell proliferation, antibody secretion, and chemotactic function. MSCs blocked proliferation of B cells in the G0 or G1 phase of the cell cycle, but they were not induced to apoptosis (Corcione et al., 2006; Tabera et al., 2008). Schena et al. (2010) found that MSCs inhibited the proliferation of mature murine splenic B cells in a dose-dependent and cell-to-cell contact-dependent manner but failed to affect B cell differentiation to plasma cells. The immunoregulatory effects of MSCs on B cells are mediated, at least in part, by secreted cytokines. The immunosuppressive activity on B cells in vivo maybe partially, if not mostly, due to the inhibition of Th cells by MSCs.
As for innate immune cells, MSCs also play a key role in modulating the maturation and function of dendritic cells (DC) (Zhang et al., 2004), potent antigen presenting cells. MSCs alter cytokine secretion from DC1 and DC2, stimulating a decrease in the secretion of TNF-α by DC1 and an increase in IL-10 from DC2 (Aggarwal and Pittenger, 2005). MSCs have been shown to mediate the polarization of macrophages by skewing macrophages toward the M2 lineage (Cho et al., 2014), an anti-inflammatory macrophage phenotype. In addition, MSCs can also inhibit the proliferation, cytokine secretion, and cytotoxicity of natural killer (NK) cells (Li et al., 2015).
MSCs exhibited two distinct, non-MHC-restricted immunomodulatory functions that depended on the relative numbers of MSCs and local inflammatory conditions. Low numbers of MSCs had less inhibitory effects and sometimes enhanced lymphocyte proliferation, whereas large doses of MSCs always exerted a suppressive effect (Le Blanc et al., 2003; Bocelli-Tyndall et al., 2009). MSCs provided no clinical amelioration in murine collagen induced arthritis (CIA), and in fact, even increasing the number of MSCs could not reduce affected paw swelling in the respective CIA mice due to the high level of proinflammatory cytokines, especially TNF-α (Djouad et al., 2005). In contrast, other in vivo studies of MSC effects on CIA indicated that MSCs can effectively inhibit CIA inflammation and joint pathology (Park et al., 2017; Sun Y. et al., 2017). Indeed, preliminary evidence for MSC efficacy has been reported in some RA patients (Alvaro-Gracia et al., 2017). MSCs could also suppress autophagy of activated T cells induced by respiratory mitochondrial metabolism in SLE patients (Chen et al., 2016) and, consequently, reduce T cell apoptosis and maybe play a crucial role in SLE treatment.
Overall, these findings strongly suggest a crucial therapeutic role for MSCs in regulating the proliferation and functional activation of lymphocytes and other immune cells in chronic inflammatory disease. Nevertheless, the relative numbers of MSCs and the proinflammatory cytokine environment may profoundly affect the immunoregulatory effect of MSC therapy.
SLE is a heterogeneous autoimmune disease with clinical manifestations ranging from butterfly erythema and mild arthritis to severe lupus nephritis and lupus encephalopathy (Marion and Postlethwaite, 2014; Fanouriakis et al., 2019). SLE patients often have familial association, for example, monozygotic twins and siblings with a family history are more likely to suffer with lupus compared to siblings in families with no history of systemic autoimmune diseases (Cooper et al., 1999). Genome wide association studies (GWAS) have identified many genetic loci that associate with lupus (Sanchez et al., 2011; Bentham et al., 2015). Together the familial associations and GWAS indicate that SLE is a heterogeneous disease with strong, but complicated genetic background. Given that MSCs from SLE patients have dysfunctional immunomodulatory effect in vitro, genetic factors that contribute to, albeit heterogeneous, development of SLE may contribute to the dysfunction of autologous MSCs from SLE patients.
The human leukocyte antigen (HLA) complex encodes the major histocompatibility complex (MHC) proteins that regulate the immune system in humans (Bodis et al., 2018). Autoimmune diseases such as SLE, RA, ankylosing spondylitis (AS), and Behcet’s disease (BD) all have known associations with particular HLA alleles. SLE was found to have significant association with HLA-DMA and DMB alleles (Yen et al., 1999), but neither DMA nor DMB was correlated with disease activity. RA susceptibility is linked to HLA-DRB1 alleles (Raychaudhuri et al., 2012), and there is a strong linkage between ankylosing spondylitis (AS) and HLA-B27 (Brewerton et al., 1973). BD has a relatively strong correlation with HLA-B51 (Ohno et al., 1982).
HLA-G molecules are mainly expressed in human placental tissue (Curigliano et al., 2013). HLA-G generates seven alternative mRNAs encoding four membrane-bound isoforms (mHLA-G: HLA-G1, G2, G3, and G4) on the cellular surface and three soluble HLA-Gs (sHLA-G: HLA-G5, G6, and G7). HLA-G5 is one of the HLA-G family of non-classical MHC class I molecules that is secreted by MSCs. HLA-G5 was found to be critical for the immunomodulatory function of MSCs by inhibiting reactivity and cytolytic function of alloreactive T cells in vitro (Riteau et al., 2001; Selmani et al., 2008). The HLA-G5 alloprotective activity of the MSCs was mediated both by cell contact and soluble HLA-G5, and HLA-G5 secretion was enhanced by cell-cell contact between alloreactive T cells and MSCs. HLA-G5 functions in the initial cell contact between MSCs and stimulated, alloreactive T cells and contributes to the suppression of T cell proliferation and subsequent T cell differentiation toward Tregs (Selmani et al., 2008). HLA-G5 expression by MSCs was enhanced by IL-10 in a dose-dependent relationship. The latter finding is important since IL-10 is increased in SLE patients compared to healthy individuals. The association between IL-10 and HLA-G5 secretion by MSCs notwithstanding, BM-MSCs from SLE patients have a proinflammatory and senescence-associated phenotype mediated by a mitochondrial antiviral signaling protein (MAVS) that induces an IFN-β feedback loop (Gao et al., 2017). MAVS, IFN-β promoter stimulator protein 1, was significantly increased in SLE MSCs as were IFN-β-induced messenger RNAs. Notably, silencing of MAVS could downregulate IFN-β, p53, and p16 proteins and alter cytokine production in SLE MSCs. This newly identified pathway may provide critical insight about cellular mechanism that contribute to lupus autoimmunity and, as such, may define new potential therapeutic targets.
BM-MSCs are gradually gaining attention because of their multidirectional differentiation potential that in turn may offer broad application prospects in clinical treatment of autoimmune diseases (Munir and McGettrick, 2015) and regenerative medicine (Mahla, 2016). However, MSCs from SLE patients possess a very limited proliferation potential in vitro and present a morphological appearance of senescence characterized by inflated volume, deeply stained nucleolus, and disordered cytoskeletal organization (Gao et al., 2017; Ji et al., 2017). The in vitro proliferative rate is decreased, and the proportion of apoptotic cells increased with MSCs from SLE patients compared with those from healthy, normal individuals.
MSCs from SLE patients also exhibit impaired capabilities for differentiation, migration, and immune regulation (Gao et al., 2017; Gu et al., 2016). MSCs from SLE patients have abnormalities in F-actin cytoskeleton accompanied by increased levels of intracellular reactive oxygen species (ROS) and MAVS, when compared to MSCs from normal, healthy individuals (Gao et al., 2017). The endoplasmic reticulum stress response (ERS) is involved in the senescence of MSCs from SLE patients and accounts for the dilated, distorted, and swollen morphology of SLE patient MSCs (Gu et al., 2015) detected by electron microscopy. The endoplasmic reticulum (ER) is an intracellular organelle that performs essential cellular functions including protein synthesis, post-translational modification, and protein folding (Ma and Hendershot, 2004). The endoplasmic reticulum stress response (ERS) occurs when chaperone proteins in the ER perceive and respond to abnormalities in normal ER function, such as protein folding (Ma and Hendershot, 2004). The ERS induces apoptosis and autophagy if the conditions that initiated the ERS are not resolved. However, the mechanisms that control the abnormal phenotype(s) of MSCs in SLE patients, including increased senescence and apoptosis, remain incompletely understood.
MSCs from SLE patients have elevated MAVS, TGF-β, ROS, telomerase activity, DNA damage, and increase expression of senescence associated genes that block the cell cycle (Nie et al., 2010; Gao et al., 2017). MSCs from SLE patients also have up-regulated immunoregulatory factors such as TGF-β, IDO-1, and LIF (Shi et al., 2014; Ji et al., 2017). MAVS is the only adaptor protein between retinoic acid-inducible gene I (RIG-I)-like receptors (RLRs) and NF-κB and the downstream IRF-3/7 signaling pathways (Vazquez and Horner, 2015). Thus, MAVS plays an indispensable role in the innate immune signaling pathway and induces IFN expression, especially IFN-β. A MAVS-IFN-β positive feedback loop essentially provides feed-forward stimulation for increased IFN-β in MSCs from SLE patients. Further, MSCs from SLE patients exhibited proinflammatory and aging features mediated by ROS induced as a consequence of the MAVS-IFN-β positive feedback loop (Shi et al., 2014; Gao et al., 2017; Ji et al., 2017). Growth restriction, enhanced β-gal activity, and impaired migration capacity are also consequences of the MAVS-IFN-β positive feedback loop. IFN-β triggers DNA signaling pathways by inducing chemical modification of p53, ROS that affects the transcriptional activity and function of P53, all of which interfere with the tumor inhibition pathways controlled by p53 that also trigger cellular aging and senescence (Moiseeva et al., 2006). Senescence-associated secretory phenotype–related gene expression, including IL-6, IL-8, and granulocyte–macrophage colony-stimulating factor (GM-CSF), is significantly increased in MSCs from SLE patients (Gao et al., 2017). In contrast, Bcl-2, which is important for inhibiting apoptosis (Li X. et al., 2012), is markedly reduced in SLE patient MSCs. Furthermore, abnormal activation of several signaling pathways including JAK-STAT, p53/p21, PTEN/Akt, PI3K/Akt, and Wnt/beta-catenin (Gu et al., 2014; Chen et al., 2015; Tan et al., 2015; Ji et al., 2017) are involved in development of the senescence phenotype in SLE patient MSCs. Inhibition or knockout of the expression of these pathways could reverse the senescent phenotype of MSCs from SLE patients, upregulate immunomodulatory cytokines such as TGF-β and IL-10, and downregulate proinflammatory cytokines, such as IFN-β, IL-17, and IL-6 (Tan et al., 2015; Gao et al., 2017). Thus, we speculated that the senescence of MSCs might be both a part of or an indicator for regulatory abnormalities in SLE patients, which may be related to the underlying SLE pathogenesis. Of note, IFN-β has a higher affinity to the type I IFN receptor than IFN-α (Schreiber and Piehler, 2015).
Autoimmune diseases create microenvironments of chronic inflammation as a consequence of immunological dysregulation that leads to excessive innate and adaptive immune stimulation. As a classic model systemic autoimmune disease, SLE can be characterized by the loss of peripheral immune tolerance, increased lymphocyte numbers and activation, and other immune cell activation, and autoantibody production, all of which contribute to pathogenic chronic inflammation (Marion and Postlethwaite, 2014). The homeostatic regulatory balance that generally prevents and controls autoimmunity is lost. Key among the regulatory elements lost are Tregs. Tregs function by downregulating the activation and proliferation of effector T cells (Li and Rudensky, 2016), and critical to SLE, Treg numbers are decreased in SLE patients, especially in active disease (Tselios et al., 2014).
In addition to inhibiting the secretion of proinflammatory cytokines, MSCs can also induce naïve CD4+T cells to differentiate into Tregs (Luz-Crawford et al., 2013; Wang et al., 2017a), indicating that allogeneic MSC transplantation may be able to restore the balance between Treg and Th in SLE patients. Working against that potential are antigen presenting cells, particularly plasmacytoid dendritic cells (pDC), that are the primary producers of IFN-α (Swiecki and Colonna, 2015). In SLE patients, pDC drive the differentiation of immature B cells to plasmablasts but fail to induce Bregs, and this compromised cross-talk with pDC and B cells has been associated with increased production of IFN-α (Menon et al., 2016). Additionally, the proinflammatory effect of IFN-α will not only promote T cell activation but will also stimulate more differentiation of CD4+ T cells to become effector cells, Tfh, Th1, and Th17, rather than iTregs further tipping the balance away from the immunosuppressive function of Tregs and loss of peripheral tolerance in SLE patients (Yan et al., 2008; Golding et al., 2010; Ambrosi et al., 2012).
The immunophenotype and immunoregulatory function of MSCs may be altered by microenvironments as a consequence of the local pro-inflammatory cytokine milieu (Djouad et al., 2005). For example, in SLE, aberrant accumulation and activation of immune cells and overexpression proinflammatory cytokines causes pivotal change in MSCs. The immunomodulatory function of MSCs stimulated with both IL-1β and TNF-α was pro-inflammatory and enhanced CD4+ T cell proliferation and differentiation to Th effector cell subsets rather than the anti-inflammatory immunomodulatory function of MSCs not stimulated with TNF-α and IL-1β (Dorraji et al., 2018). In fact, the role of TNF-α in MSCs remains controversial as TNF-α may exert different effects on MSC migration under different conditions. Normally, TNF-α upregulates MSC migration by activation of the NF-κB signaling pathway via IKK-2 (Haasters et al., 2013), a key regulatory enzyme of the NF-κB pathway. However, the significantly increased TNF-α in SLE patient serum inhibited the migration capacity of MSCs revealing what seems to be an impaired phenotype in the TNF-α-dependent migration of bone marrow-derived MSCs from SLE patients (Geng et al., 2014).
IDO, mainly secreted by DCs and macrophages, is an enzyme that mediates tryptophan degradation into immunosuppressive metabolites. Lipopolysaccharide and cytokines especially, IFN-γ, can induce the expression of IDO during inflammation or infection (Pallotta et al., 2011). Studies have demonstrated that IDO plays an indispensable role in allogenic MSC-mediated inhibition of T cell proliferation in lupus patients, which could be enhanced by IFN-γ (Wang et al., 2014). Intriguingly, MSCs from active SLE patients exhibited defective IDO production under IFN-γ stimulation (Wang et al., 2014). Consequently, modulation of IDO activity might be a novel therapeutic way to restore the defective properties of SLE patient MSCs. In contrast, mouse MSCs require nitric oxide synthase (NOS) instead of IDO to catalyze production of NO to mediate their immunosuppressive function (Sato et al., 2007; Ren et al., 2008). There are three isozyme subtypes of NOS, including neuronal nitric oxide synthase (nNOS) and endothelial nitric oxide synthase (eNOS) expressed under normal conditions, and inducible nitric oxide (iNOS) induced by injury (Forstermann and Sessa, 2012). MSCs were able to inhibit Tfh cells in lupus-prone mice by producing NO, and iNOS was an important mediator in the process since L-NMMA, a specific inhibitor of iNOS, could partially restore the generation of Tfh cells inhibited by MSCs in vitro (Sato et al., 2007; Zhang et al., 2017).
There is another reason why transplanted autologous MSCs may fail to suppress the excessive and damaging immune reactions in SLE patients. The number of autologous immunosuppressive MSCs present in relevant organs and tissues subject to chronic inflammation after autologous transplantaion may be too low to exert an efficient immunosuppressive effect. Compared with MSCs from healthy individuals, MSCs from SLE patients are morphologically biased toward senescent cells with reduced proliferative and migratory capabilities (Geng et al., 2014; Gao et al., 2017). Whether this abnormality is an inherent MSC defect alone or in addition to effects from drug treatment requires further research. As discussed above, previous studies showed that inhibition or reversal of the MSC aging-associated genes or signaling pathways could partially or fully reverse the senescent phenotype and immunoregulatory function of SLE patient MSCs (Gu et al., 2014; Chen et al., 2015; Tan et al., 2015; Ji et al., 2017). Consequently, reversing MSC senescence may allow autologous MSCs to be an effective therapy for SLE.
SLE is a systemic autoimmune disorder involving a multitude of autoantibodies that are produced by over-activated B cells that circulate in peripheral blood and deposit in organs (Marion and Postlethwaite, 2014). The autoantibodies are produced by B cells activated in germinal centers to produce isotype-switched, somatically mutated IgG autoantibodies most notably specific for nuclear antigens but other autoantigens as well. SLE is more prevalent in women of childbearing age and shows a significant gender bias with a male to female ratio of 1:9, although men and children tend to have more severe disease (Bernatsky et al., 2006; Aggarwal and Srivastava, 2015; Hwang et al., 2015). Most SLE patients show a chronic remission-relapse course except for a small number of patients that can achieve long-term remission (Fanouriakis et al., 2019). SLE is a potentially fatal autoimmune disease that can affect multiple tissues and organ with lupus nephritis being one of the most common and severe complications (Marion and Postlethwaite, 2014). Among all the complications, renal involvement carries substantial mortality and morbidity.
Treatment of SLE is challenging because of clinical heterogeneity and unpredictable disease flares. The current guideline for treating moderate to severe lupus nephritis is a two-stage treatment regimen including an initial induction phase and a prolonged maintenance phase (Fanouriakis et al., 2019). Induction therapy with intensive immunosuppressive agents, for example, high-dose methylprednisolone combined with cyclophosphamide (CTX) intravenous infusion, is adopted at the initial stage to control autoantibody production and lymphocyte activation, restore organ function and inhibit tissue damage (Austin et al., 1986; Grootscholten et al., 2006). To consolidate disease remittance and reduce recurrence, long-term maintenance treatment with less intense and moderate side effects such as low-dose prednisone and mycophenolate mofetil (MMF) is recommended (Contreras et al., 2004; Ruiz-Irastorza et al., 2010). Although most lupus patients respond well to the conventional treatment of steroid and immunosuppressive agents such as CTX, tacrolimus, and MMF, there are remarkable and potentially serious side effects associated with each including infection, metabolic disorders, ischemic osteonecrosis, gastrointestinal adverse reactions, liver and renal toxicity, gonadal inhibition, and myelosuppression (Kamanamool et al., 2010; Ishii et al., 2015; Kishi et al., 2018; Fanouriakis et al., 2019). Conventional therapy usually requires the use of multiple immunosuppressive agents for several years or even for the lifetime of a patient. Long-term complex prescriptions and tapering methods, regular follow-up and routine blood tests not only plague the patient’s daily life, but also aggravate the patient’s financial and psychological burdens (Barber and Clarke, 2017; Zhao et al., 2018). Even worse are refractory patients who fail to response to conventional therapy and have persistently active disease (Nikpour et al., 2009). Therefore, there is a strong, urgent need to develop a new treatment for SLE that not only can effectively control disease flares with acceptable side effects, but also reduce the patient’s burden for continuous medication and extend the follow-up time. B cell depletion therapies have engendered hope for availability and effectiveness of new biologics to treat, if not cure, lupus (Looney, 2010). Results from both the EXPLORER (Looney et al., 2010) and LUNAR (Rovin et al., 2012) clinical trials of the B cell targeting monoclonal antibody rituximab were disappointing since neither trial achieved the predetermined endpoint for success. Belimumab trials have been much more promising with significant improvement in immunologic parameters, but clinical disease improvement was still only moderate compared to placebo (Navarra et al., 2011; Manzi et al., 2012; Stohl et al., 2012). New approaches and new therapies are desperately needed for SLE. The more recent success of B cell depletion with anti-CD19 CAR-T (chimeric antigen receptor-T) cells in lupus-prone mice may offer an alternative, more successful approach for B cell depletion therapy for SLE in humans (Kansal et al., 2019). MSC may be the much-needed new approach to therapy.
For patients who respond poorly to conventional therapy, MSC therapy has shown satisfactory efficacy with acceptable treatment-related adverse events (Wang et al., 2017b; Barbado et al., 2018; Liang et al., 2018). Data from North and South American transplantation centers have indicated that the 3 years MSC transplantation-related mortality (TRM) was no more than 5% (Pasquini et al., 2012), while in a Chinese long-term retrospective study, the TRM was 0.2% (1/404) (Liang et al., 2018).
In recent years, various countries or regions have attached great importance to stem cell research and clinical translation. The number of clinical trials for stem cell-based therapies for autoimmune diseases registered in www.clinicaltrials.gov website reached 212 cases worldwide as of July 4, 2019. Moreover, there are 14 different stem cell-associated products approved for therapy1, most of which are MSCs or HSCs.
Anecdotal case reports have reported that total remission can be achieved after HSC transplantation in refractory SLE patients with severe disease and who were resistant to conventional therapies (Traynor and Burt, 1999; Rosen et al., 2000). In a 5 year follow-up study, most of the refractory SLE patients who underwent autologous HSC infusion, after immunoablation and depletion of mononuclear cells, attained durable clinical and serological remission (Burt et al., 2018). In another clinical study, autologous HSC transfusion also restored Treg numbers and immunosuppressive function in SLE patients (Zhang et al., 2009). Autologous HSC transfusion in conjunction with non-myeloablative immunoablation with CTX, rituximab, and thymoglobulin (rATG) may be therapeutically effective in SLE since the autoreactive immune cell clones are eliminated, and the reconstituted immune competent cells develop with normal, effective self-tolerance. The most common adverse events in the treatment with HSC are fever, infection, and infusion reactions, but most adverse events were determined to be unrelated to infusion (Liang et al., 2018). The success of autologous HSC therapies has encouraged a possible therapeutic use of MSCs to treat SLE patients.
MSCs are non-hematopoietic, multipotent progenitor stem cells that possess immunomodulatory capabilities. Several clinical studies have been performed to evaluate the potential clinical efficacy for MSC transplantation as an alternative therapeutic approach to the current pharmacologic therapy for SLE. Results from those studies indicate that MSC transplantation is a safe and effective therapy (Munir and McGettrick, 2015; Squillaro et al., 2016; Wang et al., 2017b) that can ameliorate multiorgan injuries and induce long-term disease remission in active and refractory SLE patients (Liang et al., 2010; Deng et al., 2017; Barbado et al., 2018). MSCs were able to re-establish the defective osteoblastic niche in lupus-prone mice and effectively reverse multiorgan dysfunction, especially glomerulonephritis, in mice and patients compared with CTX (Sun et al., 2009; Choi et al., 2016). Unlike anti-CD20 and TNF inhibitor biological agents, MSC could restore the ratio of Treg/Tfh cells in CIA mice (Sun Y. et al., 2017). Finally, experiments in mice have evaluated the effect of combination therapy with MSCs and five kinds of drugs including prednisone, dexamethasone, cyclosporine A, mycophenolate mofetil, and rapamycin for their effects on T cell subpopulations. The results indicated that MSCs could enhance the anti-inflammatory effects of the drugs and attenuate the cytotoxic side effects of the immunosuppressants (Hajkova et al., 2017). Hence the combination of MSCs and immunosuppressants may become a more ideal therapeutic strategy to treat autoimmune diseases, especially SLE, compared to pharmacologic immunosuppression alone.
Our review shows that, although allogeneic MSCs are promising candidates for treating SLE, autologous MSCs may not be therapeutically useful because of their defects in both immunomodulatory function and regenerative characteristics. MSCs from SLE patients present a morphological appearance of senescence with impaired capabilities of differentiation, migration and immune regulation (Gu et al., 2016; Gao et al., 2017; Ji et al., 2017). B cells contribute to pathogenesis in SLE through both autoantibody-dependent and autoantibody-independent mechanisms. Abnormally activated B cells in SLE patients affect the function of MSCs, and depletion of B cells may help to restore the potential immunosuppressive of autologous MSCs. Notably, previous studies have shown that MSCs are capable of inhibiting the proliferation and differentiation of B cells (Corcione et al., 2006) and hence may have a promising efficacy in treating SLE. On the other hand, MSCs could enhance proliferation and differentiation into immunoglobulin-secreting cells of naïve and transitional B lymphocytes from SLE patients in vitro (Traggiai et al., 2008) raising concerns regarding the therapeutic use of MSC to suppress B lymphocytes. These results suggest caution in considering and monitoring MSC therapy to treat SLE. Further studies are needed to uncover the underlying regulation between MSC and immune cells and how those effects may affect disease in SLE patients.
Likely, no single factor can account for the intricate mechanisms of the MSCs-mediated immunosuppressive effect on autoimmune diseases. There are several hypothetical mechanisms why MSCs from SLE patients appear defective (see Figure 3). GWAS have indicated that autoimmune diseases, including SLE, are associated with numerous, heterogeneous genetic loci. MSCs from SLE patients are characterized by morphological and phenotypic changes associated with aging. These include functional changes modulated by expression of several senescence-associated genes and signaling pathways that decrease proliferative potential. Likely, the proinflammatory microenvironment in patients with lupus alters the immunosuppressive potential of MSCs from those patients. Similar results were found in patients with other inflammatory diseases such as abdominal aortic aneurysm (AAA). MSCs isolated from human AAA wall display a dysregulated immunosuppressive effect on peripheral blood mononuclear cells (PBMCs) proliferation (Ciavarella et al., 2015).
Figure 3. Possible mechanism that may contribute to MSC dysfunction in SLE. The figure depicts several hypothetical mechanisms to explain why MSCs are defective in SLE patients. Both genetic factors and the immune system environment, particularly pro-inflammatory, are expected to contribute to the immunosuppressive dysfunction of MSCs from SLE patients. The morphological changes associated with aging of MSCs from SLE patients are the consequence of several senescence-associated genes and signaling pathways. The proinflammatory niche created by immune system dysfunction in SLE synergistically contributes to the abnormalities in MSC.
In conclusion, murine models and clinical trials have produced evidence for the therapeutic potential of MSCs for SLE. Our review shows that although allogeneic MSCs are promising candidates for treating SLE, autologous MSCs may not be eligible to treat SLE patients because of their defective immunomodulatory function and poor regenerative characteristics. Moreover, whether the immunological rejection of allogeneic stem cell transplantation will influence the efficacy of MSC therapies or have long-term effects on recipients is not known. If the causes of MSC dysfunction for MSCs from SLE patients can be better understood in the future, maybe modification or transformation of SLE patient MSCs to restore immunosuppressive and regenerative function can yield a therapeutically beneficial treatment. In fact, recent research has indicated that MSCs transfected with an etanercept-encoding vector can successfully produce the drug in vitro and had superior suppressive effects in CIA mice compared to non-modified MSCs (Park et al., 2017). Prospects for MSCs as immunosuppressive therapy in other rheumatic diseases are being and should continue to be explored. However, only through further research and clinical trials can we completely resolve this mystery of why MSCs are defective in SLE patients. Studying the mechanism of MSC defects in SLE patients can provide new ideas for the pathogenesis of SLE, and provide a new theoretical corroborate for cellular therapy that may be of great significance for clinical application in rheumatic diseases.
R-JC wrote the original manuscript. A-JX and Y-HL organized sections of the manuscript. S-YP and Q-PZ prepared the figures. YZ, YL, and TM revised the manuscript.
The present work was supported by the National Key Research and Development Program of China (Project no. 2016YFC0906201) to YL, 1.3.5 project for disciplines of excellence, West China Hospital, Sichuan University (Project no. ZYGD18015) to YL, and Department of Science and Technology of Sichuan Province (Project no. 2019YJ0099) to Y-HL.
The authors declare that the research was conducted in the absence of any commercial or financial relationships that could be construed as a potential conflict of interest.
Aggarwal, A., and Srivastava, P. (2015). Childhood onset systemic lupus erythematosus: how is it different from adult SLE? Int. J. Rheum. Dis. 18, 182–191. doi: 10.1111/1756-185X.12419
Aggarwal, S., and Pittenger, M. F. (2005). Human mesenchymal stem cells modulate allogeneic immune cell responses. Blood 105, 1815–1822. doi: 10.1182/blood-2004-04-1559
Alvaro-Gracia, J. M., Jover, J. A., Garcia-Vicuna, R., Carreno, L., Alonso, A., Marsal, S., et al. (2017). Intravenous administration of expanded allogeneic adipose-derived mesenchymal stem cells in refractory rheumatoid arthritis (Cx611): results of a multicentre, dose escalation, randomised, single-blind, placebo-controlled phase Ib/IIa clinical trial. Ann. Rheum. Dis. 76, 196–202. doi: 10.1136/annrheumdis-2015-208918
Ambrosi, A., Espinosa, A., and Wahren-Herlenius, M. (2012). IL-17: a new actor in IFN-driven systemic autoimmune diseases. Eur. J. Immunol. 42, 2274–2284. doi: 10.1002/eji.201242653
Austin, H. A. III, Klippel, J. H., Balow, J. E., le Riche, N. G., Steinberg, A. D., Plotz, P. H., et al. (1986). Therapy of lupus nephritis. Controlled trial of prednisone and cytotoxic drugs. N. Engl. J. Med. 314, 614–619. doi: 10.1056/NEJM198603063141004
Barbado, J., Tabera, S., Sanchez, A., and Garcia-Sancho, J. (2018). Therapeutic potential of allogeneic mesenchymal stromal cells transplantation for lupus nephritis. Lupus 27, 2161–2165. doi: 10.1177/0961203318804922
Barber, M. R. W., and Clarke, A. E. (2017). Socioeconomic consequences of systemic lupus erythematosus. Curr. Opin. Rheumatol. 29, 480–485. doi: 10.1097/BOR.0000000000000416
Bentham, J., Morris, D. L., Graham, D. S. C., Pinder, C. L., Tombleson, P., Behrens, T. W., et al. (2015). Genetic association analyses implicate aberrant regulation of innate and adaptive immunity genes in the pathogenesis of systemic lupus erythematosus. Nat. Genet. 47, 1457–1464. doi: 10.1038/ng.3434
Bernatsky, S., Boivin, J. F., Joseph, L., Manzi, S., Ginzler, E., Gladman, D. D., et al. (2006). Mortality in systemic lupus erythematosus. Arthritis Rheum. 54, 2550–2557. doi: 10.1002/art.21955
Bocelli-Tyndall, C., Bracci, L., Schaeren, S., Feder-Mengus, C., Barbero, A., Tyndall, A., et al. (2009). Human bone marrow mesenchymal stem cells and chondrocytes promote and/or suppress the in vitro proliferation of lymphocytes stimulated by interleukins 2, 7 and 15. Ann. Rheum. Dis. 68, 1352–1359. doi: 10.1136/ard.2008.094003
Bodis, G., Toth, V., and Schwarting, A. (2018). Role of human leukocyte antigens (HLA) in autoimmune diseases. Rheumatol. Ther. 5, 5–20. doi: 10.1007/s40744-018-0100-z
Brewerton, D. A., Hart, F. D., Nicholls, A., Caffrey, M., James, D. C., and Sturrock, R. D. (1973). Ankylosing spondylitis and HL-A 27. Lancet 1, 904–907.
Burt, R. K., Han, X., Gozdziak, P., Yaung, K., Morgan, A., Clendenan, A. M., et al. (2018). Five year follow-up after autologous peripheral blood hematopoietic stem cell transplantation for refractory, chronic, corticosteroid-dependent systemic lupus erythematosus: effect of conditioning regimen on outcome. Bone Marrow Transplant. 53, 692–700. doi: 10.1038/s41409-018-0173-x
Campanati, A., Orciani, M., Lazzarini, R., Ganzetti, G., Consales, V., Sorgentoni, G., et al. (2017). TNF-alpha inhibitors reduce the pathological Th1 -Th17 /Th2 imbalance in cutaneous mesenchymal stem cells of psoriasis patients. Exp. Dermatol. 26, 319–324. doi: 10.1111/exd.13139
Caplan, A. I. (1991). Mesenchymal stem cells. J. Orthop. Res. 9, 641–650. doi: 10.1002/jor.1100090504
Caplan, A. I., and Dennis, J. E. (2006). Mesenchymal stem cells as trophic mediators. J. Cell. Biochem. 98, 1076–1084. doi: 10.1002/jcb.20886
Carrion, F., Nova, E., Ruiz, C., Diaz, F., Inostroza, C., Rojo, D., et al. (2010). Autologous mesenchymal stem cell treatment increased T regulatory cells with no effect on disease activity in two systemic lupus erythematosus patients. Lupus 19, 317–322. doi: 10.1177/0961203309348983
Castro-Manrreza, M. E., Mayani, H., Monroy-Garcia, A., Flores-Figueroa, E., Chavez-Rueda, K., Legorreta-Haquet, V., et al. (2014). Human mesenchymal stromal cells from adult and neonatal sources: a comparative in vitro analysis of their immunosuppressive properties against T cells. Stem Cells Dev 23, 1217–1232. doi: 10.1089/scd.2013.0363
Chen, H., Shi, B., Feng, X., Kong, W., Chen, W., Geng, L., et al. (2015). Leptin and neutrophil-activating peptide 2 promote mesenchymal stem cell senescence through activation of the phosphatidylinositol 3-Kinase/Akt pathway in patients with systemic lupus erythematosus. Arthritis Rheumatol. 67, 2383–2393. doi: 10.1002/art.39196
Chen, J., Wang, Q., Feng, X., Zhang, Z., Geng, L., Xu, T., et al. (2016). Umbilical cord-derived mesenchymal stem cells suppress autophagy of T cells in patients with systemic lupus erythematosus via transfer of mitochondria. Stem Cells Int. 2016:4062789. doi: 10.1155/2016/4062789
Cho, D. I., Kim, M. R., Jeong, H. Y., Jeong, H. C., Jeong, M. H., Yoon, S. H., et al. (2014). Mesenchymal stem cells reciprocally regulate the M1/M2 balance in mouse bone marrow-derived macrophages. Exp. Mol. Med. 46:e70. doi: 10.1038/emm.2013.135
Choi, E. W., Lee, M., Song, J. W., Shin, I. S., and Kim, S. J. (2016). Mesenchymal stem cell transplantation can restore lupus disease-associated miRNA expression and Th1/Th2 ratios in a murine model of SLE. Sci. Rep. 6:38237. doi: 10.1038/srep38237
Ciavarella, C., Alviano, F., Gallitto, E., Ricci, F., Buzzi, M., Velati, C., et al. (2015). Human vascular wall mesenchymal stromal cells contribute to abdominal aortic aneurysm pathogenesis through an impaired immunomodulatory activity and increased levels of matrix metalloproteinase-9. Circ. J. 79, 1460–1469. doi: 10.1253/circj.CJ-14-0857
Contreras, G., Pardo, V., Leclercq, B., Lenz, O., Tozman, E., O’Nan, P., et al. (2004). Sequential therapies for proliferative lupus nephritis. N. Engl. J. Med. 350, 971–980. doi: 10.1056/NEJMoa031855
Cooper, G. S., Miller, F. W., and Pandey, J. P. (1999). The role of genetic factors in autoimmune disease: implications for environmental research. Environ. Health Perspect. 107(Suppl. 5), 693–700. doi: 10.1289/ehp.99107s5693
Corcione, A., Benvenuto, F., Ferretti, E., Giunti, D., Cappiello, V., Cazzanti, F., et al. (2006). Human mesenchymal stem cells modulate B-cell functions. Blood 107, 367–372. doi: 10.1182/blood-2005-07-2657
Curigliano, G., Criscitiello, C., Gelao, L., and Goldhirsch, A. (2013). Molecular pathways: human leukocyte antigen G (HLA-G). Clin. Cancer Res. 19, 5564–5571. doi: 10.1158/1078-0432.CCR-12-3697
Davies, L. C., Heldring, N., Kadri, N., and Le Blanc, K. (2017). Mesenchymal stromal cell secretion of programmed death-1 ligands regulates T cell mediated immunosuppression. Stem Cells 35, 766–776. doi: 10.1002/stem.2509
Deng, D., Zhang, P., Guo, Y., and Lim, T. O. (2017). A randomised double-blind, placebo-controlled trial of allogeneic umbilical cord-derived mesenchymal stem cell for lupus nephritis. Ann. Rheum. Dis. 76, 1436–1439. doi: 10.1136/annrheumdis-2017-211073
Di Nicola, M., Carlo-Stella, C., Magni, M., Milanesi, M., Longoni, P. D., Matteucci, P., et al. (2002). Human bone marrow stromal cells suppress T-lymphocyte proliferation induced by cellular or nonspecific mitogenic stimuli. Blood 99, 3838–3843.
Di Trapani, M., Bassi, G., Midolo, M., Gatti, A., Kamga, P. T., Cassaro, A., et al. (2016). Differential and transferable modulatory effects of mesenchymal stromal cell-derived extracellular vesicles on, T., B and NK cell functions. Sci. Rep. 6:24120. doi: 10.1038/srep24120
Dinsmore, J., Ratliff, J., Deacon, T., Pakzaban, P., Jacoby, D., Galpern, W., et al. (1996). Embryonic stem cells differentiated in vitro as a novel source of cells for transplantation. Cell Transplant. 5, 131–143.
Djouad, F., Fritz, V., Apparailly, F., Louis-Plence, P., Bony, C., Sany, J., et al. (2005). Reversal of the immunosuppressive properties of mesenchymal stem cells by tumor necrosis factor alpha in collagen-induced arthritis. Arthritis Rheum. 52, 1595–1603. doi: 10.1002/art.21012
Dominici, M., Le Blanc, K., Mueller, I., Slaper-Cortenbach, I., Marini, F., Krause, D., et al. (2006). Minimal criteria for defining multipotent mesenchymal stromal cells. The international society for cellular therapy position statement. Cytotherapy 8, 315–317. doi: 10.1080/14653240600855905
Dong, L., Pu, Y., Zhang, L., Qi, Q., Xu, L., Li, W., et al. (2018). Human umbilical cord mesenchymal stem cell-derived extracellular vesicles promote lung adenocarcinoma growth by transferring miR-410. Cell Death Dis. 9, 218. doi: 10.1038/s41419-018-0323-5
Dorraji, S. E., Hovd, A. K., Kanapathippillai, P., Bakland, G., Eilertsen, G. O., Figenschau, S. L., et al. (2018). Mesenchymal stem cells and T cells in the formation of tertiary lymphoid structures in lupus nephritis. Sci. Rep. 8:7861. doi: 10.1038/s41598-018-26265-z
Engler, A. J., Sen, S., Sweeney, H. L., and Discher, D. E. (2006). Matrix elasticity directs stem cell lineage specification. Cell 126, 677–689. doi: 10.1016/j.cell.2006.06.044
Fanouriakis, A., Kostopoulou, M., Alunno, A., Aringer, M., Bajema, I., Boletis, J. N., et al. (2019). 2019 update of the EULAR recommendations for the management of systemic lupus erythematosus. Ann. Rheum. Dis. 78, 736–745. doi: 10.1136/annrheumdis-2019-215089
Forstermann, U., and Sessa, W. C. (2012). Nitric oxide synthases: regulation and function. Eur. Heart J. 33, 837a–837d. doi: 10.1093/eurheartj/ehr304
Friedenstein, A. J., Gorskaja, J. F., and Kulagina, N. N. (1976). Fibroblast precursors in normal and irradiated mouse hematopoietic organs. Exp. Hematol. 4, 267–274.
Gao, L., Bird, A. K., Meednu, N., Dauenhauer, K., Liesveld, J., Anolik, J., et al. (2017). Bone marrow-derived mesenchymal stem cells from patients with systemic lupus erythematosus have a senescence-associated secretory phenotype mediated by a mitochondrial antiviral signaling protein-interferon-beta feedback loop. Arthritis Rheumatol. 69, 1623–1635. doi: 10.1002/art.40142
Gebler, A., Zabel, O., and Seliger, B. (2012). The immunomodulatory capacity of mesenchymal stem cells. Trends Mol. Med. 18, 128–134. doi: 10.1016/j.molmed.2011.10.004
Geng, L., Li, X., Feng, X., Zhang, J., Wang, D., Chen, J., et al. (2014). Association of TNF-alpha with impaired migration capacity of mesenchymal stem cells in patients with systemic lupus erythematosus. J. Immunol. Res. 2014:169082. doi: 10.1155/2014/169082
Ghannam, S., Pene, J., Moquet-Torcy, G., Jorgensen, C., and Yssel, H. (2010). Mesenchymal stem cells inhibit human Th17 cell differentiation and function and induce a T regulatory cell phenotype. J. Immunol. 185, 302–312. doi: 10.4049/jimmunol.0902007
Golding, A., Rosen, A., Petri, M., Akhter, E., and Andrade, F. (2010). Interferon-alpha regulates the dynamic balance between human activated regulatory and effector T cells: implications for antiviral and autoimmune responses. Immunology 131, 107–117. doi: 10.1111/j.1365-2567.2010.03280.x
Gonzalo-Gil, E., Perez-Lorenzo, M. J., Galindo, M., Diaz de la Guardia, R., Lopez-Millan, B., Bueno, C., et al. (2016). Human embryonic stem cell-derived mesenchymal stromal cells ameliorate collagen-induced arthritis by inducing host-derived indoleamine 2,3 dioxygenase. Arthritis Res. Ther. 18:77. doi: 10.1186/s13075-016-0979-0
Gouveia de Andrade, A. V., Bertolino, G., Riewaldt, J., Bieback, K., Karbanova, J., Odendahl, M., et al. (2015). Extracellular vesicles secreted by bone marrow- and adipose tissue-derived mesenchymal stromal cells fail to suppress lymphocyte proliferation. Stem Cells Dev. 24, 1374–1376. doi: 10.1089/scd.2014.0563
Groh, M. E., Maitra, B., Szekely, E., and Koc, O. N. (2005). Human mesenchymal stem cells require monocyte-mediated activation to suppress alloreactive T cells. Exp. Hematol. 33, 928–934. doi: 10.1016/j.exphem.2005.05.002
Grootscholten, C., Ligtenberg, G., Hagen, E. C., van den Wall Bake, A. W., de Glas-Vos, J. W., Bijl, M., et al. (2006). Azathioprine/methylprednisolone versus cyclophosphamide in proliferative lupus nephritis. A randomized controlled trial. Kidney Int. 70, 732–742. doi: 10.1038/sj.ki.5001630
Gu, Z., Meng, Y., Tao, T., Guo, G., Tan, W., Xia, Y., et al. (2015). Endoplasmic reticulum stress participates in the progress of senescence of bone marrow-derived mesenchymal stem cells in patients with systemic lupus erythematosus. Cell Tissue Res. 361, 497–508. doi: 10.1007/s00441-015-2131-x
Gu, Z., Tan, W., Feng, G., Meng, Y., Shen, B., Liu, H., et al. (2014). Wnt/beta-catenin signaling mediates the senescence of bone marrow-mesenchymal stem cells from systemic lupus erythematosus patients through the p53/p21 pathway. Mol. Cell. Biochem. 387, 27–37. doi: 10.1007/s11010-013-1866-5
Gu, Z., Tan, W., Ji, J., Feng, G., Meng, Y., Da, Z., et al. (2016). Rapamycin reverses the senescent phenotype and improves immunoregulation of mesenchymal stem cells from MRL/lpr mice and systemic lupus erythematosus patients through inhibition of the mTOR signaling pathway. Aging 8, 1102–1114. doi: 10.18632/aging.100925
Haasters, F., Prall, W. C., Westphal, I., Bocker, W., Padula, D., Mutschler, W., et al. (2013). Overexpression of dnIKK in mesenchymal stem cells leads to increased migration and decreased invasion upon TNFalpha stimulation. Biochem. Biophys. Res. Commun. 436, 265–270. doi: 10.1016/j.bbrc.2013.05.091
Hajkova, M., Hermankova, B., Javorkova, E., Bohacova, P., Zajicova, A., Holan, V., et al. (2017). Mesenchymal stem cells attenuate the adverse effects of immunosuppressive drugs on distinct T cell subopulations. Stem Cell Rev. 13, 104–115. doi: 10.1007/s12015-016-9703-3
Hwang, J., Lee, J., Ahn, J. K., Park, E. J., Cha, H. S., and Koh, E. M. (2015). Clinical characteristics of male and female Korean patients with systemic lupus erythematosus: a comparative study. Korean J. Intern. Med. 30, 242–249. doi: 10.3904/kjim.2015.30.2.242
Infante, A., and Rodriguez, C. I. (2018). Osteogenesis and aging: lessons from mesenchymal stem cells. Stem Cell Res. Ther. 9:244. doi: 10.1186/s13287-018-0995-x
Ishii, S., Miwa, Y., Otsuka, K., Nishimi, S., Nishimi, A., Saito, M., et al. (2015). Influence of renal complications on the efficacy and adverse events of tacrolimus combination therapy in patients with systemic lupus erythematosus (SLE) during a maintenance phase: a single-centre, prospective study. Lupus Sci. Med. 2:e000091. doi: 10.1136/lupus-2015-000091
Ji, J., Wu, Y., Meng, Y., Zhang, L., Feng, G., Xia, Y., et al. (2017). JAK-STAT signaling mediates the senescence of bone marrow-mesenchymal stem cells from systemic lupus erythematosus patients. Acta Biochim. Biophys. Sin. 49, 208–215. doi: 10.1093/abbs/gmw134
Kamanamool, N., McEvoy, M., Attia, J., Ingsathit, A., Ngamjanyaporn, P., and Thakkinstian, A. (2010). Efficacy and adverse events of mycophenolate mofetil versus cyclophosphamide for induction therapy of lupus nephritis: systematic review and meta-analysis. Medicine 89, 227–235. doi: 10.1097/MD.0b013e3181e93d00
Kansal, R., Richardson, N., Neeli, I., Khawaja, S., Chamberlain, D., Ghani, M., et al. (2019). Sustained B cell depletion by CD19-targeted CAR T cells is a highly effective treatment for murine lupus. Sci. Transl. Med. 11:eaav1648. doi: 10.1126/scitranslmed.aav1648
Kishi, C., Motegi, S. I., Yasuda, M., and Ishikawa, O. (2018). Therapeutic efficacy and adverse events of hydroxychloroquine administration in Japanese systemic/cutaneous lupus erythematosus patients. J. Dermatol. 45, 1020–1022. doi: 10.1111/1346-8138.14512
Le Blanc, K., Frassoni, F., Ball, L., Locatelli, F., Roelofs, H., Lewis, I., et al. (2008). Mesenchymal stem cells for treatment of steroid-resistant, severe, acute graft-versus-host disease: a phase II study. Lancet 371, 1579–1586. doi: 10.1016/S0140-6736(08)60690-X
Le Blanc, K., Tammik, L., Sundberg, B., Haynesworth, S. E., and Ringden, O. (2003). Mesenchymal stem cells inhibit and stimulate mixed lymphocyte cultures and mitogenic responses independently of the major histocompatibility complex. Scand. J. Immunol. 57, 11–20.
Li, M. O., and Rudensky, A. Y. (2016). T cell receptor signalling in the control of regulatory T cell differentiation and function. Nat. Rev. Immunol. 16, 220–233. doi: 10.1038/nri.2016.26
Li, W., Ren, G., Huang, Y., Su, J., Han, Y., Li, J., et al. (2012). Mesenchymal stem cells: a double-edged sword in regulating immune responses. Cell Death. Differ. 19, 1505–1513. doi: 10.1038/cdd.2012.26
Li, X., Liu, L., Meng, D., Wang, D., Zhang, J., Shi, D., et al. (2012). Enhanced apoptosis and senescence of bone-marrow-derived mesenchymal stem cells in patients with systemic lupus erythematosus. Stem Cells Dev. 21, 2387–2394. doi: 10.1089/scd.2011.0447
Li, Y., Qu, Y. H., Wu, Y. F., Liu, L., Lin, X. H., Huang, K., et al. (2015). Bone marrow mesenchymal stem cells suppressing activation of allogeneic cytokine-induced killer/natural killer cells either by direct or indirect interaction. Cell Biol. Int. 39, 435–445. doi: 10.1002/cbin.10404
Liang, J., Zhang, H., Hua, B., Wang, H., Lu, L., Shi, S., et al. (2010). Allogenic mesenchymal stem cells transplantation in refractory systemic lupus erythematosus: a pilot clinical study. Ann. Rheum. Dis. 69, 1423–1429. doi: 10.1136/ard.2009.123463
Liang, J., Zhang, H., Kong, W., Deng, W., Wang, D., Feng, X., et al. (2018). Safety analysis in patients with autoimmune disease receiving allogeneic mesenchymal stem cells infusion: a long-term retrospective study. Stem Cell Res. Ther. 9:312. doi: 10.1186/s13287-018-1053-4
Lim, J. Y., Park, M. J., Im, K. I., Kim, N., Jeon, E. J., Kim, E. J., et al. (2014). Combination cell therapy using mesenchymal stem cells and regulatory T-cells provides a synergistic immunomodulatory effect associated with reciprocal regulation of TH1/TH2 and th17/treg cells in a murine acute graft-versus-host disease model. Cell Transplant. 23, 703–714. doi: 10.3727/096368913X664577
Lin, T. H., Gibon, E., Loi, F., Pajarinen, J., Cordova, L. A., Nabeshima, A., et al. (2017). Decreased osteogenesis in mesenchymal stem cells derived from the aged mouse is associated with enhanced NF-kappaB activity. J. Orthop. Res. 35, 281–288. doi: 10.1002/jor.23270
Liu, J., Chen, B., Yan, F., and Yang, W. (2017). The influence of inflammatory cytokines on the proliferation and osteoblastic differentiation of MSCs. Curr. Stem Cell Res. Ther. 12, 401–408. doi: 10.2174/1574888X12666170509102222
Looney, R. J. (2010). B cell-targeted therapies for systemic lupus erythematosus: an update on clinical trial data. Drugs 70, 529–540. doi: 10.2165/11535420-000000000-00000
Looney, R. J., Anolik, J., and Sanz, I. (2010). A perspective on B-cell-targeting therapy for SLE. Mod. Rheumatol. 20, 1–10. doi: 10.1007/s10165-009-0213-x
Luz-Crawford, P., Kurte, M., Bravo-Alegria, J., Contreras, R., Nova-Lamperti, E., Tejedor, G., et al. (2013). Mesenchymal stem cells generate a CD4+CD25+Foxp3+ regulatory T cell population during the differentiation process of Th1 and Th17 cells. Stem Cell Res. Ther. 4:65. doi: 10.1186/scrt216
Ma, Y., and Hendershot, L. M. (2004). ER chaperone functions during normal and stress conditions. J. Chem. Neuroanat. 28, 51–65. doi: 10.1016/j.jchemneu.2003.08.007
Mahla, R. S. (2016). Stem cells applications in regenerative medicine and disease therapeutics. Int. J. Cell. Biol. 2016:6940283. doi: 10.1155/2016/6940283
Manzi, S., Sanchez-Guerrero, J., Merrill, J. T., Furie, R., Gladman, D., Navarra, S. V., et al. (2012). Effects of belimumab, a B lymphocyte stimulator-specific inhibitor, on disease activity across multiple organ domains in patients with systemic lupus erythematosus: combined results from two phase III trials. Ann. Rheum. Dis. 71, 1833–1838. doi: 10.1136/annrheumdis-2011-200831
Marion, T. N., and Postlethwaite, A. E. (2014). Chance, genetics, and the heterogeneity of disease and pathogenesis in systemic lupus erythematosus. Semin. Immunopathol. 36, 495–517. doi: 10.1007/s00281-014-0440-x
Meirelles Lda, S., Fontes, A. M., Covas, D. T., and Caplan, A. I. (2009). Mechanisms involved in the therapeutic properties of mesenchymal stem cells. Cytokine Growth. Factor. Rev 20, 419–427. doi: 10.1016/j.cytogfr.2009.10.002
Menon, M., Blair, P. A., Isenberg, D. A., and Mauri, C. (2016). A regulatory feedback between plasmacytoid dendritic cells and regulatory B cells is aberrant in systemic lupus erythematosus. Immunity 44, 683–697. doi: 10.1016/j.immuni.2016.02.012
Moiseeva, O., Mallette, F. A., Mukhopadhyay, U. K., Moores, A., and Ferbeyre, G. (2006). DNA damage signaling and p53-dependent senescence after prolonged beta-interferon stimulation. Mol. Biol. Cell 17, 1583–1592. doi: 10.1091/mbc.e05-09-0858
Munir, H., and McGettrick, H. M. (2015). Mesenchymal stem cell therapy for autoimmune disease: risks and rewards. Stem Cells Dev. 24, 2091–2100. doi: 10.1089/scd.2015.0008
Navarra, S. V., Guzman, R. M., Gallacher, A. E., Hall, S., Levy, R. A., Jimenez, R. E., et al. (2011). Efficacy and safety of belimumab in patients with active systemic lupus erythematosus: a randomised, placebo-controlled, phase 3 trial. Lancet 377, 721–731. doi: 10.1016/S0140-6736(10)61354-2
Nie, Y., Lau, C., Lie, A., Chan, G., and Mok, M. (2010). Defective phenotype of mesenchymal stem cells in patients with systemic lupus erythematosus. Lupus 19, 850–859. doi: 10.1177/0961203309361482
Nikpour, M., Urowitz, M. B., Ibanez, D., and Gladman, D. D. (2009). Frequency and determinants of flare and persistently active disease in systemic lupus erythematosus. Arthritis Rheum. 61, 1152–1158. doi: 10.1002/art.24741
Ohno, S., Ohguchi, M., Hirose, S., Matsuda, H., Wakisaka, A., and Aizawa, M. (1982). Close association of HLA-Bw51 with Behcet’s disease. Arch. Ophthalmol. 100, 1455–1458.
Pallotta, M. T., Orabona, C., Volpi, C., Vacca, C., Belladonna, M. L., Bianchi, R., et al. (2011). Indoleamine 2,3-dioxygenase is a signaling protein in long-term tolerance by dendritic cells. Nat. Immunol. 12, 870–878. doi: 10.1038/ni.2077
Park, N., Rim, Y. A., Jung, H., Kim, J., Yi, H., Kim, Y., et al. (2017). Etanercept-synthesising mesenchymal stem cells efficiently ameliorate collagen-induced arthritis. Sci. Rep. 7:39593. doi: 10.1038/srep39593
Pasquini, M. C., Voltarelli, J., Atkins, H. L., Hamerschlak, N., Zhong, X., Ahn, K. W., et al. (2012). Transplantation for autoimmune diseases in north and South America: a report of the center for international blood and marrow transplant research. Biol. Blood Marrow Transplant. 18, 1471–1478. doi: 10.1016/j.bbmt.2012.06.003
Petri, M., Orbai, A. M., Alarcon, G. S., Gordon, C., Merrill, J. T., Fortin, P. R., et al. (2012). Derivation and validation of the Systemic Lupus International Collaborating Clinics classification criteria for systemic lupus erythematosus. Arthritis Rheum. 64, 2677–2686. doi: 10.1002/art.34473
Raychaudhuri, S., Sandor, C., Stahl, E. A., Freudenberg, J., Lee, H. S., Jia, X., et al. (2012). Five amino acids in three HLA proteins explain most of the association between MHC and seropositive rheumatoid arthritis. Nat. Genet. 44, 291–296. doi: 10.1038/ng.1076
Ren, G., Zhang, L., Zhao, X., Xu, G., Zhang, Y., Roberts, A. I., et al. (2008). Mesenchymal stem cell-mediated immunosuppression occurs via concerted action of chemokines and nitric oxide. Cell Stem Cell 2, 141–150. doi: 10.1016/j.stem.2007.11.014
Riteau, B., Rouas-Freiss, N., Menier, C., Paul, P., Dausset, J., and Carosella, E. D. (2001). HLA-G2,-G3, and-G4 isoforms expressed as nonmature cell surface glycoproteins inhibit NK and antigen-specific CTL cytolysis. J. Immunol. 166, 5018–5026. doi: 10.4049/jimmunol.166.8.5018
Rosen, O., Thiel, A., Massenkeil, G., Hiepe, F., Haupl, T., Radtke, H., et al. (2000). Autologous stem-cell transplantation in refractory autoimmune diseases after in vivo immunoablation and ex vivo depletion of mononuclear cells. Arthritis Res. 2, 327–336. doi: 10.1186/ar107
Rovin, B. H., Furie, R., Latinis, K., Looney, R. J., Fervenza, F. C., Sanchez-Guerrero, J., et al. (2012). Efficacy and safety of rituximab in patients with active proliferative lupus nephritis: the lupus nephritis assessment with rituximab study. Arthritis Rheum. 64, 1215–1226. doi: 10.1002/art.34359
Ruiz-Irastorza, G., Ramos-Casals, M., Brito-Zeron, P., and Khamashta, M. A. (2010). Clinical efficacy and side effects of antimalarials in systemic lupus erythematosus: a systematic review. Ann. Rheum. Dis. 69, 20–28. doi: 10.1136/ard.2008.101766
Sanchez, E., Nadig, A., Richardson, B. C., Freedman, B. I., Kaufman, K. M., Kelly, J. A., et al. (2011). Phenotypic associations of genetic susceptibility loci in systemic lupus erythematosus. Ann. Rheum. Dis. 70, 1752–1757. doi: 10.1136/ard.2011.154104
Sato, K., Ozaki, K., Oh, I., Meguro, A., Hatanaka, K., Nagai, T., et al. (2007). Nitric oxide plays a critical role in suppression of T-cell proliferation by mesenchymal stem cells. Blood 109, 228–234. doi: 10.1182/blood-2006-02-002246
Schena, F., Gambini, C., Gregorio, A., Mosconi, M., Reverberi, D., Gattorno, M., et al. (2010). Interferon-gamma-dependent inhibition of B cell activation by bone marrow-derived mesenchymal stem cells in a murine model of systemic lupus erythematosus. Arthritis Rheum. 62, 2776–2786. doi: 10.1002/art.27560
Schreiber, G., and Piehler, J. (2015). The molecular basis for functional plasticity in type I interferon signaling. Trends Immunol. 36, 139–149. doi: 10.1016/j.it.2015.01.002
Selmani, Z., Naji, A., Zidi, I., Favier, B., Gaiffe, E., Obert, L., et al. (2008). Human leukocyte antigen-G5 secretion by human mesenchymal stem cells is required to suppress T lymphocyte and natural killer function and to induce CD4+CD25highFOXP3+ regulatory T cells. Stem Cells 26, 212–222. doi: 10.1634/stemcells.2007-0554
Shi, D., Li, X., Chen, H., Che, N., Zhou, S., Lu, Z., et al. (2014). High level of reactive oxygen species impaired mesenchymal stem cell migration via overpolymerization of F-actin cytoskeleton in systemic lupus erythematosus. Pathol. Biol. 62, 382–390. doi: 10.1016/j.patbio.2014.07.009
Squillaro, T., Peluso, G., and Galderisi, U. (2016). Clinical trials with mesenchymal stem cells: an update. Cell Transplant. 25, 829–848. doi: 10.3727/096368915X689622
Stohl, W., Hiepe, F., Latinis, K. M., Thomas, M., Scheinberg, M. A., Clarke, A., et al. (2012). Belimumab reduces autoantibodies, normalizes low complement levels, and reduces select B cell populations in patients with systemic lupus erythematosus. Arthritis Rheum. 64, 2328–2337. doi: 10.1002/art.34400
Stojanovic, S., Najman, S., and Korac, A. (2018). Stem cells derived from lipoma and adipose tissue-similar mesenchymal phenotype but different differentiation capacity governed by distinct molecular signature. Cells 7:E260. doi: 10.3390/cells7120260
Sun, L., Akiyama, K., Zhang, H., Yamaza, T., Hou, Y., Zhao, S., et al. (2009). Mesenchymal stem cell transplantation reverses multiorgan dysfunction in systemic lupus erythematosus mice and humans. Stem Cells 27, 1421–1432. doi: 10.1002/stem.68
Sun, X. K., Zhou, J., Zhang, L., Ma, T., Wang, Y. H., Yang, Y. M., et al. (2017). Down-regulation of Noggin and miR-138 coordinately promote osteogenesis of mesenchymal stem cells. J. Mol. Histol. 48, 427–436. doi: 10.1007/s10735-017-9740-5
Sun, Y., Kong, W., Huang, S., Shi, B., Zhang, H., Chen, W., et al. (2017). Comparable therapeutic potential of umbilical cord mesenchymal stem cells in collagen-induced arthritis to TNF inhibitor or anti-CD20 treatment. Clin. Exp. Rheumatol. 35, 288–295.
Swiecki, M., and Colonna, M. (2015). The multifaceted biology of plasmacytoid dendritic cells. Nat. Rev. Immunol. 15, 471–485. doi: 10.1038/nri3865
Tabera, S., Perez-Simon, J. A., Diez-Campelo, M., Sanchez-Abarca, L. I., Blanco, B., Lopez, A., et al. (2008). The effect of mesenchymal stem cells on the viability, proliferation and differentiation of B-lymphocytes. Haematologica 93, 1301–1309. doi: 10.3324/haematol.12857
Tan, W., Gu, Z., Shen, B., Jiang, J., Meng, Y., Da, Z., et al. (2015). PTEN/Akt-p27(kip1) Signaling Promote the BM-MSCs Senescence and Apoptosis in SLE Patients. J. Cell. Biochem. 116, 1583–1594. doi: 10.1002/jcb.25112
Thomson, J. A., Itskovitz-Eldor, J., Shapiro, S. S., Waknitz, M. A., Swiergiel, J. J., Marshall, V. S., et al. (1998). Embryonic stem cell lines derived from human blastocysts. Science 282, 1145–1147.
Togel, F., Hu, Z., Weiss, K., Isaac, J., Lange, C., and Westenfelder, C. (2005). Administered mesenchymal stem cells protect against ischemic acute renal failure through differentiation-independent mechanisms. Am. J. Physiol. Renal Physiol. 289, F31–F42. doi: 10.1152/ajprenal.00007.2005
Traggiai, E., Volpi, S., Schena, F., Gattorno, M., Ferlito, F., Moretta, L., et al. (2008). Bone marrow-derived mesenchymal stem cells induce both polyclonal expansion and differentiation of B cells isolated from healthy donors and systemic lupus erythematosus patients. Stem Cells 26, 562–569. doi: 10.1634/stemcells.2007-0528
Traynor, A., and Burt, R. K. (1999). Haematopoietic stem cell transplantation for active systemic lupus erythematosus. Rheumatology 38, 767–772. doi: 10.1093/rheumatology/38.8.767
Tselios, K., Sarantopoulos, A., Gkougkourelas, I., and Boura, P. (2014). CD4+CD25highFOXP3+ T regulatory cells as a biomarker of disease activity in systemic lupus erythematosus: a prospective study. Clin. Exp. Rheumatol. 32, 630–639.
van der Flier, L. G., and Clevers, H. (2009). Stem cells, self-renewal, and differentiation in the intestinal epithelium. Annu. Rev. Physiol. 71, 241–260. doi: 10.1146/annurev.physiol.010908.163145
Vazquez, C., and Horner, S. M. (2015). MAVS coordination of antiviral innate immunity. J. Virol. 89, 6974–6977. doi: 10.1128/JVI.01918-14
Von Luttichau, I., Notohamiprodjo, M., Wechselberger, A., Peters, C., Henger, A., Seliger, C., et al. (2005). Human adult CD34- progenitor cells functionally express the chemokine receptors CCR1, CCR4, CCR7, CXCR5, and CCR10 but not CXCR4. Stem Cells Dev. 14, 329–336. doi: 10.1089/scd.2005.14.329
Wagner, W., Horn, P., Castoldi, M., Diehlmann, A., Bork, S., Saffrich, R., et al. (2008). Replicative senescence of mesenchymal stem cells: a continuous and organized process. PLoS One 3:e2213. doi: 10.1371/journal.pone.0002213
Wang, D., Feng, X., Lu, L., Konkel, J. E., Zhang, H., Chen, Z., et al. (2014). A CD8 T cell/indoleamine 2,3-dioxygenase axis is required for mesenchymal stem cell suppression of human systemic lupus erythematosus. Arthritis Rheumatol. 66, 2234–2245. doi: 10.1002/art.38674
Wang, D., Huang, S., Yuan, X., Liang, J., Xu, R., Yao, G., et al. (2017a). The regulation of the Treg/Th17 balance by mesenchymal stem cells in human systemic lupus erythematosus. Cell. Mol. Immunol. 14, 423–431. doi: 10.1038/cmi.2015.89
Wang, D., Niu, L., Feng, X., Yuan, X., Zhao, S., Zhang, H., et al. (2017b). Long-term safety of umbilical cord mesenchymal stem cells transplantation for systemic lupus erythematosus: a 6-year follow-up study. Clin. Exp. Med. 17, 333–340. doi: 10.1007/s10238-016-0427-0
Weissman, I. L., and Shizuru, J. A. (2008). The origins of the identification and isolation of hematopoietic stem cells, and their capability to induce donor-specific transplantation tolerance and treat autoimmune diseases. Blood 112, 3543–3553. doi: 10.1182/blood-2008-08-078220
Xie, Y., and Shen, G. (2018). MicroRNA-139-5p elevates skeletal myogenic differentiation of human adult dental pulp stem cells through Wnt/beta-catenin signaling pathway. Exp. Ther. Med. 16, 2835–2842. doi: 10.3892/etm.2018.6585
Yan, B., Ye, S., Chen, G., Kuang, M., Shen, N., and Chen, S. (2008). Dysfunctional CD4+,CD25+ regulatory T cells in untreated active systemic lupus erythematosus secondary to interferon-alpha-producing antigen-presenting cells. Arthritis Rheum. 58, 801–812. doi: 10.1002/art.23268
Yan, M., Liu, X., Dang, Q., Huang, H., Yang, F., and Li, Y. (2017). Intra-articular injection of human synovial membrane-derived mesenchymal stem cells in murine collagen-induced arthritis: assessment of immunomodulatory capacity in vivo. Stem Cells Int. 2017:9198328. doi: 10.1155/2017/9198328
Yang, X., Yang, J., Li, X., Ma, W., and Zou, H. (2018). Bone marrow-derived mesenchymal stem cells inhibit T follicular helper cell in lupus-prone mice. Lupus 27, 49–59. doi: 10.1177/0961203317711013
Yen, J. H., Chen, C. J., Tsai, W. C., Tsai, J. J., Ou, T. T., and Liu, H. W. (1999). HLA-DMA and HLA-DMB genotyping in patients with systemic lupus erythematosus. J. Rheumatol. 26, 1930–1933.
Zhang, L., Bertucci, A. M., Ramsey-Goldman, R., Burt, R. K., and Datta, S. K. (2009). Regulatory T cell (Treg) subsets return in patients with refractory lupus following stem cell transplantation, and TGF-beta-producing CD8+ Treg cells are associated with immunological remission of lupus. J. Immunol. 183, 6346–6358. doi: 10.4049/jimmunol.0901773
Zhang, W., Ge, W., Li, C., You, S., Liao, L., Han, Q., et al. (2004). Effects of mesenchymal stem cells on differentiation, maturation, and function of human monocyte-derived dendritic cells. Stem Cells Dev. 13, 263–271. doi: 10.1089/154732804323099190
Zhang, Z., Feng, R., Niu, L., Huang, S., Deng, W., Shi, B., et al. (2017). Human umbilical cord mesenchymal stem cells inhibit T follicular helper cell expansion through the activation of iNOS in lupus-prone B6.MRL-Fas(lpr) Mice. Cell Transplant. 26, 1031–1042. doi: 10.3727/096368917x694660
Zhao, J., Bai, W., Zhu, P., Zhang, X., Liu, S., Wu, L., et al. (2016). Chinese SLE treatment and research group (CSTAR) registry VII: prevalence and clinical significance of serositis in Chinese patients with systemic lupus erythematosus. Lupus 25, 652–657. doi: 10.1177/0961203315625460
Zhao, Q., Chen, H., Yan, H., He, Y., Zhu, L., Fu, W., et al. (2018). The correlations of psychological status, quality of life, self-esteem, social support and body image disturbance in Chinese patients with Systemic Lupus Erythematosus. Psychol. Health Med. 23, 779–787. doi: 10.1080/13548506.2018.1434214
Keywords: systemic lupus erythematosus, mesenchymal stem cells, dysfunction, senescence, immunoregulatory
Citation: Cheng R-J, Xiong A-J, Li Y-H, Pan S-Y, Zhang Q-P, Zhao Y, Liu Y and Marion TN (2019) Mesenchymal Stem Cells: Allogeneic MSC May Be Immunosuppressive but Autologous MSC Are Dysfunctional in Lupus Patients. Front. Cell Dev. Biol. 7:285. doi: 10.3389/fcell.2019.00285
Received: 13 September 2019; Accepted: 04 November 2019;
Published: 15 November 2019.
Edited by:
Gianluca Carnevale, University of Modena and Reggio Emilia, ItalyReviewed by:
Gianandrea Pasquinelli, University of Bologna, ItalyCopyright © 2019 Cheng, Xiong, Li, Pan, Zhang, Zhao, Liu and Marion. This is an open-access article distributed under the terms of the Creative Commons Attribution License (CC BY). The use, distribution or reproduction in other forums is permitted, provided the original author(s) and the copyright owner(s) are credited and that the original publication in this journal is cited, in accordance with accepted academic practice. No use, distribution or reproduction is permitted which does not comply with these terms.
*Correspondence: Yi Liu, eWkyMDA2bGl1QDE2My5jb20=
Disclaimer: All claims expressed in this article are solely those of the authors and do not necessarily represent those of their affiliated organizations, or those of the publisher, the editors and the reviewers. Any product that may be evaluated in this article or claim that may be made by its manufacturer is not guaranteed or endorsed by the publisher.
Research integrity at Frontiers
Learn more about the work of our research integrity team to safeguard the quality of each article we publish.