- 1Institut National de la Santé et de la Recherche Médicale, UMR-S 1193, Université Paris-Sud, Université Paris-Saclay, Châtenay-Malabry, France
- 2Laboratoire de Biochimie-Hormonologie, Hôpital Antoine Béclère, AP-HP, Clamart, France
- 3Département de Génétique, Institut de la Vision, Université Pierre et Marie Curie Paris 06, Sorbonne Universités, Institut National de la Santé et de la Recherche Médicale UMR-S 968, Centre National de la Recherche Scientifique UMR 7210, Paris, France
Since the initial discovery of septin family GTPases, the understanding of their molecular organization and cellular roles keeps being refined. Septins have been involved in many physiological processes and the misregulation of specific septin gene expression has been implicated in diverse human pathologies, including neurological disorders and cancer. In this minireview, we focus on the importance of the subunit composition and subcellular localization of septins relevant to tumor initiation, progression, and metastasis. We especially underline the importance of septin polymer composition and of their association with the plasma membrane, actin, or microtubules in cell functions involved in cancer and in resistance to cancer therapies. Through their scaffolding role, their function in membrane compartmentalization or through their protective function against protein degradation, septins also emerge as critical organizers of membrane-associated proteins and of signaling pathways implicated in cancer-associated angiogenesis, apoptosis, polarity, migration, proliferation, and in metastasis. Also, the question as to which of the free monomers, hetero-oligomers, or filaments is the functional form of mammalian septins is raised and the control over their spatial and temporal localization is discussed. The increasing amount of crosstalks identified between septins and cellular signaling mediators reinforces the exciting possibility that septins could be new targets in anti-cancer therapies or in therapeutic strategies to limit drug resistance.
Introduction
In humans, 13 septin proteins have been identified, which can be clustered into four groups according to their gene structure homology (Kinoshita, 2003; Hall et al., 2005). A plethora of isoforms has been described, and for SEPT4, 8 and 9, long and short N-terminal extensions have been identified (see Hall and Russell, 2012). Such diversity allows a large repertoire of septin assemblies, which could explain the multiplicity of septin subcellular localizations and functions. Septins can oligomerize into repeated and ordered hexamers or octamers, which can organize into higher-order structures, including rings or filaments. Ring structures have been well described in the context of mitotic completion or in differentiated cell structures like at the base of primary cilia or in the spermatozoid annulus (for reviews, Caudron and Barral, 2009; Saarikangas and Barral, 2011; Spiliotis and Gladfelter, 2012). Septins are also observed as rods and filaments, which associate with actin, microtubules (MTs) or membranes and are thus considered as a fourth component of the cytoskeleton (Mostowy and Cossart, 2012). Septins function as scaffolds for protein-protein interactions, or as diffusion barriers for protein compartmentalization, not only during cell division, but also in an increasing number of processes in interphase cells (for reviews, Mostowy and Cossart, 2012; Fung et al., 2014; Montagna et al., 2015).
Septins have initially been identified as fusion partners with MLL in leukemia (Osaka et al., 1999; Cerveira et al., 2011). Their gene expression is also deregulated in tumors. SEPT2, 8, 9, and 11 genes are consistently up-regulated, while SEPT4 and 10 are down-regulated in many cancer cells (Liu et al., 2010; Montagna et al., 2015). A colon cancer diagnosis method based on the quantification of circulating methylated SEPT9 DNA has even been proposed (for review, Song and Li, 2015). Septin isoform expression (mainly focused on SEPT9 isoforms) has also been studied in a broad range of solid tumors (Scott et al., 2006; Connolly et al., 2011, 2014; Shen et al., 2012; Gilad et al., 2015). Here, we focus on the links between the modulation of septin polymer composition, their differential subcellular localization, and the molecular and cellular pathophysiological mechanisms they affect in cancer, both in mitotic and interphase cells.
Roles of Septins in Mitosis
By forming highly organized rings at cell division sites, septins have been found to play a crucial role in the spatio-temporal control of yeast budding, and the mechanisms that control septin assembly, remodeling and functions in this context are still thoroughly explored in the yeast model. In mammalian cells, septins have also been identified as one of the contributors of mitosis, and could potentially be implicated in a variety of cancers. Indeed, after Cdk1-mediated phosphorylation, long SEPT9 isoforms become a substrate of the prolyl-isomerase Pin1, and their isomerization is required for cytokinesis completion (Estey et al., 2013). Like other oncogenes and tumor suppressors controlled by Pin1 (for review, Zhou and Lu, 2016), specific SEPT9 isoforms may thus participate in oncogenesis. Also, septins contribute to fulfill proper chromosome congression and correct segregation during the anaphase. In this context, the SEPT2/6/7 complexes seem to be important for the recruitment of the kinesin family protein CENP-E (Spiliotis et al., 2005), which participates in the mitotic checkpoint, and for chromosome movement along MTs during the anaphase. At the onset of telophase, septins concentrate at the central spindle region where they interact with the actomyosin contractile ring via the partner protein anillin (for reviews, Fung et al., 2014; Menon and Gaestel, 2015). Recent data indicate that the anillin-septin ring promotes the intercellular bridge ingression, elongation and narrowing. These steps occur prior to septin and anillin relocalization to the central stem body and to sites of MT constriction. There, the septin ring facilitates the recruitment of Chmp4B, allowing the assembly of the ESCRT III complex, which actually mediates the abcission step (Renshaw et al., 2014). A recent study on the effects of chrysotile fibers (responsible of mesothelioma, lung cancer, and asbestos) points out the role of cytokinesis failure mediated by an overexpression of SEPT2 and by anillin and SEPT9 mislocalizations, in causing aneuploidy, centrosome amplification, and multipolar mitoses (Cortez et al., 2016), which are frequent in cancer cells.
Localization-Dependent Roles of Septins in Interphase Cells
Out of the cell division context, septin contribution to cancer may also involve interphase cells, in a way that is linked to their subcellular location, as described hereafter and summarized in Figure 1.
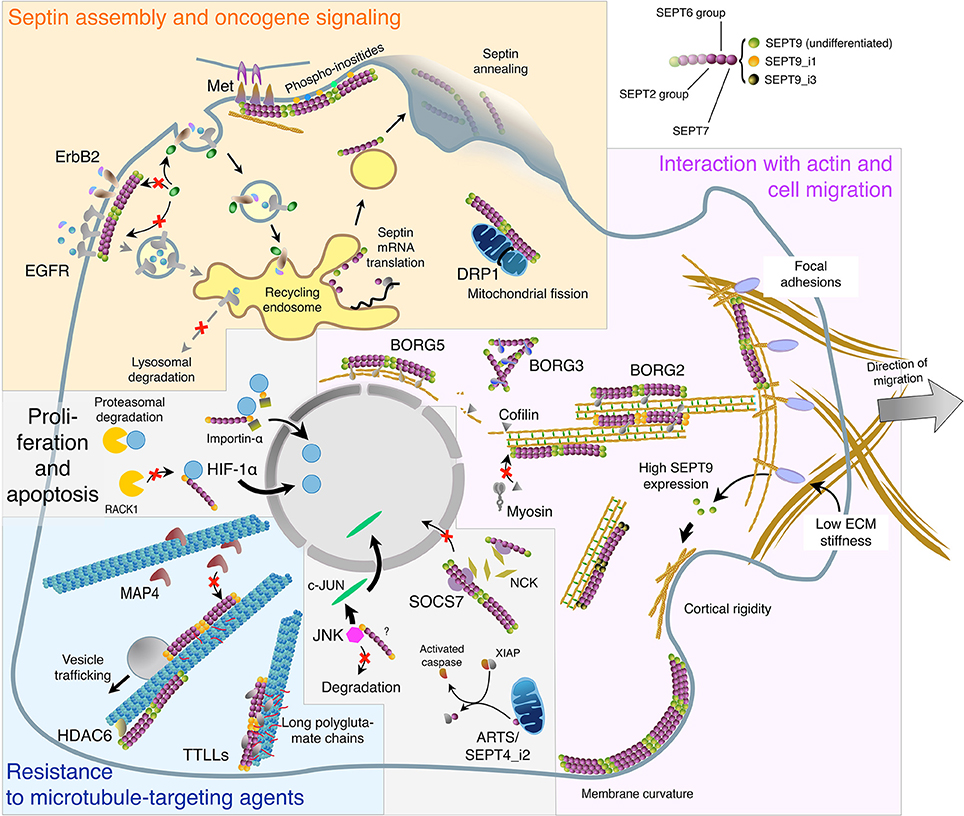
Figure 1. Subcellular localizations and functions of septins in interphase cells in relation with oligomer composition and binding partners. This virtual cell summarizes the main topics described in the text regarding: Septin biosynthesis, annealing, and association with the plasma membrane. Role of septin filaments in oncogene receptor signaling and dynamics, and in mitochondria fission (Yellow panel). Septin localization to the actin cytoskeleton: direct binding or involvement of SEPT9 isoforms and BORG proteins. Implication of septin filaments in actin filament bundling and organization, cell migration, cortical rigidity, and membrane curvature sensing (Magenta panel). Septin binding to microtubules and the links with tubulin post-translational modifications and MAPs in vesicular trafficking and in cell resistance to microtubule-targeting agents (Cyan panel). Septin roles in the nuclear import of signaling factors involved in cell proliferation and in apoptosis. It is not known whether SEPT9 interacts alone or as part of septin oligomers with HIF-1α, importin-α and JNK (Light gray panel). The color code of septin monomers is indicated on the top right of the figure.
Membrane-Associated Septins
Septin self-assembly into filaments and higher-order structures occur by diffusion-driven annealing on the plasma membrane (Bridges et al., 2014). In return, large septin filament arrays stably interacting with the plasma membrane may modify cortical morphogenesis by imposing membrane curvature (Tanaka-Takiguchi et al., 2009), and affect the cortical rigidity of migrating cells (Tooley et al., 2009), thus contributing to tumor metastasis.
Besides their role in membrane compartmentalization, septins have been implicated in the misregulation of growth factor receptors involved in cancer progression. They can cluster and stabilize plasma membrane proteins (Caudron and Barral, 2009; Hagiwara et al., 2011) including receptor tyrosine kinases. Indeed, membrane-associated SEPT9 prevents CIN85 binding to the ubiquitin ligase Cbl, resulting in reduced ubiquitin-dependent EGFR degradation (Diesenberg et al., 2015). Also, septins are involved in the abnormal persistence of ErbB2 at the plasma membrane of cancer cells via decreased ubiquitylation and degradation (Marcus et al., 2016). In addition, the surface distribution of the c-Met protooncogene is regulated in opposite ways by SEPT2 and 11, but both participate in its interaction with the ligand and anchorage to the actin cytoskeleton (Mostowy et al., 2011), illustrating the importance of the subunit composition of septin filaments in controlling their biological functions.
Septins may also associate with other membrane-bound organelles. SEPT2 and 7, by interacting with DRP1 would concentrate it at the sites of mitochondrial constriction and facilitate their fission (Pagliuso et al., 2016; Sirianni et al., 2016). Mitochondria-associated septins also comprise the isoform 2 of SEPT4 (SEPT4_i2) also called ARTS, which upon pro-apoptotic stimuli, is released in the cytosol where it binds to the XIAP proteins to release the inhibition of caspases and thus promote apoptosis (Edison et al., 2012). ARTS expression has been shown to drop in acute lymphoid leukemias and in lymphomas, and would be implicated in controlling the number of normal stem cells (García-Fernández et al., 2010). This has led to propose ARTS as a valuable therapeutic target against cancer stem cells (Elhasid and Larisch, 2011). Also, septins are implicated in autophagosome formation upon nutrient deprivation in yeast (Barve et al., 2016). These studies provide new clues to understand the role of septins in cancerogenesis and/or adaptation to tumoral environment.
Actin-Associated Septins
In many cell types, septin filaments coalign with actin in subcortical regions or along stress fibers (Kinoshita et al., 1997). Actin loss causes septins to form free cytoplasmic rings (Kinoshita et al., 2002). Conversely, SEPT2 depletion attenuates actin bundling (Kinoshita et al., 2002) and disrupts stress fibers (Schmidt and Nichols, 2004). Knocking down SEPT6 and 7 (Kremer et al., 2007) produce a similar loss of actin bundling along with the disruption of cell polarity. These effects may involve septin binding partners that crosslink septins to actin like BORG2 and BORG5, which are two of the five Cdc42-effector proteins of the BORG family (Liu et al., 2014; Calvo et al., 2015). Stress fiber disruption may also involve septin partners that directly regulate actin like the protein Wdpcp (Cui et al., 2013).
Septins control actin remodeling during cell migration and may thus contribute to metastatic cancer cell dissemination and invasion. Septin filaments bind to and stabilize the transverse arc and radial stress fibers in the lamellipodia of migrating cells (Dolat et al., 2014). Such stabilization could involve SEPT9-mediated prevention of actin depolymerisation by myosin and cofilin (Smith et al., 2015). Septins also contribute to the stabilization of nascent focal adhesions (Dolat et al., 2014), which is necessary for their turnover and thus for effective migration. Sept9-knockout mouse embryonic fibroblasts indeed migrate more slowly than wild-type cells (Füchtbauer et al., 2011). Also, SEPT9_i4 is involved in the control of migration directionality in MCF7 breast cancer cells (Chacko et al., 2005). Persistent directional migration is further dependent on SEPT7 and on BORG5, which maintain proper actin filament organization (Liu et al., 2014).
Cell migration and invasion requires epithelial-mesenchymal transition (EMT), which involves the formation of cell protrusions and changes in the way cells interact with the extracellular matrix (ECM). Depletion of SEPT9 in various metastatic cancer cells allows the reversion of EMT and reduces cell spreading, migration and invasion (Shankar et al., 2010). SEPT1 also participates in the spreading of squamous cell carcinoma DJM-1 cells (Mizutani et al., 2013).
Tumor progression also requires neoangiogenesis, which implies the migration of leader cells of the tumor microenvironment. Interestingly, septins are involved in both processes. Indeed, Yeh et al. (2012) proposed that the ECM stiffness controls SEPT9 expression of endothelial cells via integrin signaling, and regulates cell proliferation and peripheral distribution of actin assembly. Regarding tumor-associated fibroblast migration, Calvo et al. (2015) showed that the cohesion between actin fibers and septins is increased due to elevated expression of the crosslinking protein BORG2. This in turn leads to matrix remodeling, favors the activation of highly contractile cancer-associated fibroblasts and promotes cancer cell invasion, angiogenesis, and tumor growth.
Microtubule-Associated Septins
In a few cell types, septin filaments co-align with MTs (Surka et al., 2002; Nagata et al., 2003; for review, Silverman-Gavrila and Silverman-Gavrila, 2008). They compete with the MT-stabilizing protein MAP4 to associate with the MT lattice and reduce MT stability (Kremer et al., 2005; Spiliotis et al., 2008). By contrast, septin-decorated MTs also exhibit lower MT dynamics in MDCK cells (Bowen et al., 2011) although septins do not colocalize with stabilized acetylated or detyrosinated MTs (Spiliotis et al., 2008). However, the relationship between septins and MT acetylation is still unclear as, in dendrites, SEPT7 was found to interact with the tubulin deacetylase HDAC6 (Ageta-Ishihara et al., 2013). In addition, septin filaments interact with polyglutamylated MTs and favor vesicle trafficking along these tracks to maintain the polarity of MDCK cells (Spiliotis et al., 2008).
Cancer chemotherapy often makes use of MT-targeting agents (MTA), which do not only act during mitosis but also interfere with MT dynamics during the interphase. MT-associated septins by modulating the MT environment may therefore modulate MT-based activities. Several septins have been proposed to participate in cancer cell resistance to MTA. Low SEPT10 expression level would promote paclitaxel resistance (Xu et al., 2012), while resistance to paclitaxel and to 2-methyl-estradiol involves SEPT9_i1 overexpression in several cancer cell lines (Amir and Mabjeesh, 2007). Also, misregulations of SEPT9_i1 and i4 have been linked to bad prognosis and resistance to MTA in prostate (Gilad et al., 2015) and breast cancers (Chacko et al., 2012). Consistently, paclitaxel-resistant MDA-MB 231 breast cancer cells display increased SEPT2, 8, 9, 11 levels (Froidevaux-Klipfel et al., 2011). In these cells, septins are displaced from actin fibers to MTs, where they restore higher level of MT dynamics by acting as scaffolding proteins to recruit tubulin polyglutamylation enzymes. Septin recruitment to polyglutamylated MTs result in increased binding of MT modulators that play key roles in controlling catastrophe and rescue events (Froidevaux-Klipfel et al., 2015).
Septins not only form a diffusion barrier at the base of the primary cilium (Hu and Nelson, 2011), but also associate with acetylated MTs in the axonema of RPE-1 cells in which the SEPT2/7/9 complex controls ciliary length (Ghossoub et al., 2013). The loss of TTLL3 activity, a polyglycylase required for robust primary cilium formation has been involved in colon cancer development (Rocha et al., 2014). By analogy with the finding that septin filaments recruit tubulin polyglutamylases on MTs (Froidevaux-Klipfel et al., 2015), the axonema-associated septins could perhaps function to scaffold TTLL3 on ciliary MTs, as it belongs to the same family of enzymes.
Other Roles of Septins in Ill-Defined Locations
As observed for membrane receptors, septins, and more precisely SEPT9_i1, stabilize other signaling proteins like JNK (Gonzalez et al., 2009) or HIF-1α (Amir et al., 2009) by preventing their degradation. However, these studies used total cell extracts and gave no indication of where these events take place in the cell. In both cases, it is not known whether septins function as filaments. By stabilizing JNK, SEPT9_i1 promotes longer JNK signaling, c-Jun phosphorylation, and cyclin D1 expression, leading to enhanced proliferation (Gonzalez et al., 2009). Septins are also involved in the nucleo-cytoplasmic distribution of proteins. By binding to SOCS7, which contains the nuclear import/export signals, the SEPT2/6/7 complex maintains SOCS7 together with the adapter protein NCK in the cytoplasm, thus perturbing cell cycle arrest induced by DNA damage (Kremer et al., 2007). Also, SEPT9_i1 was evidenced to physically bind to importin-α and HIF-1α to promote HIF-1α nuclear translocation and subsequent transcriptional activation (Golan and Mabjeesh, 2013).
Molecular Determinants of Septin Filament Localization
The high diversity of septin isoform expression and the variety of their assembly into oligomers and higher-order structures suggest a molecular basis for their multiple localizations and functions. The intimate determinants of subcellular septin fate are most often enigmatic but some targeting and/or interaction mechanisms have been described (see Figure 1).
One intriguing mechanism has been revealed in fungi and could potentially be generalized to other organisms. In polarized fungal hyphae, septins are locally translated and assembled into heteromeric complexes on the surface of shuttling endosomes for an efficient long-distance transport and deposition at growth poles (Baumann et al., 2013; Zander et al., 2016). Consistent with such a model, the majority of septin molecules found in the cytosol are already assembled into octamers, while the septin complexes recovered at the plasma membrane are composed of multiple octamers that can anneal (Bridges et al., 2014). Septin rod association into filaments would be driven by interactions with the plasma membrane (Bridges et al., 2014). Such interactions involve the binding to membrane-specific phosphoinositides like PIP2 (Zhang et al., 1999), PI4,5bis-phosphate (Bertin et al., 2010) or PI5P (Akil et al., 2016) and the local curvature of the membrane, which can be efficiently discriminated by septin rods (Bridges et al., 2016).
Septins have been found to bind actin directly (Mavrakis et al., 2014; Smith et al., 2015). The N-terminal tail of SEPT9 was recently evidenced to cross-link actin filaments by binding to three different sites on F-actin (Smith et al., 2015). While anillin has been evidenced to bridge septin filaments with actin during mitosis, BORG2 was recently shown to play a similar role in non-dividing cells (Calvo et al., 2015). BORG1, 2 and 3 were evidenced to directly bind to septin oligomers that comprise SEPT6, and this interaction is negatively regulated by Cdc42 (Joberty et al., 2001; Sadian et al., 2013). Gic1, one of the yeast homologs of human BORG, has been shown to stabilize long septin filaments by binding to the Cdc10 (a septin of the group 3) subunit (Sadian et al., 2013). In contrast, activated BORG3, by strongly binding to the SEPT6/7 interface (Sheffield et al., 2003), causes uncontrolled septin bundling and thus filament loss (Joberty et al., 2001; Kinoshita et al., 2002). More recently, BORG5 was shown to control the localization of septin filaments along the perinuclear actin fibers (Liu et al., 2014). The spatiotemporal control of septin targeting to the actin cytoskeleton may thus depend on the presence of binding partners in the cytoplasm, excluding the participation of anillin, which is sequestered in the nucleus during the interphase (Field and Alberts, 1995). Regulation of BORG availability may also exist, as BORG2 expression increased in response to cancer cell-derived soluble factors and is consistently increased in the stromal compartment of breast cancers (Calvo et al., 2015).
SEPT9, in particular SEPT9_i1, has been linked to many cancers of bad prognosis, to cell resistance to MTA and was found to be overexpressed during the interphase (G1 and S) in breast cancer (Gonzalez et al., 2009). Long SEPT9 isoforms (SEPT9_i1, i2, and i3), which are preferentially incorporated into higher-order structures than short ones (_i4-5; Sellin et al., 2011), contribute to localize septin filaments along interphase MTs (Sellin et al., 2012; Bai et al., 2013; Mizutani et al., 2013). Thus, the localization of SEPT9-containing filaments to actin in the cytoplasm and to MTs in the cilium of RPE-1 cells (Ghossoub et al., 2013), may also rely on the expression of specific SEPT9 splice variants. By directly binding in vitro to MTs through its GTP-binding domain (Nagata et al., 2003), SEPT9_i1 was proposed to target septin filaments to MTs in interphase cells (Surka et al., 2002). The repeated basic motifs in the N-terminal regions of the long SEPT9 isoforms (SEPT9_i1-3) were shown to interact with the acidic regions of tubulin (Bai et al., 2013). However, a more recent study demonstrated that the whole N-terminal domain of mammalian SEPT9_i1 also contains an interaction domain with F-actin (Smith et al., 2015). These findings indicate that other molecular determinants on septins or on their target organelles may play a role in septin association with either cytoskeleton element. Tubulin posttranslational modifications are one of these determinants as highlighted by the differential septin filament locations between sensitive and Taxol®-resistant breast cancer cells, which both express long SEPT9 isoforms (Froidevaux-Klipfel et al., 2015). As already observed in MDCK cells, septin filaments coalign with polyglutamylated MT tracks (Spiliotis et al., 2008). Long lateral polyglutamate chains on tubulin even enhance this association in Taxol®-resistant breast cancer cells, while septins remain associated with actin in their Taxol®-sensitive counterpart (Froidevaux-Klipfel et al., 2015). This differential septin filament localization between MTs and actin filaments correlates with a high level of SEPT9_i1 in resistant cells, while SEPT9_i3 is the main SEPT9 isoform found in the septin filaments of sensitive cells. By bearing 5 more positive charges than SEPT9_i3, SEPT9_i1 would have more affinity for the acidic tail of tubulin, and even more when it bears long polyglutamate chains.
Concluding Remarks
Although, septin roles in cancer have been largely documented, the understanding of how the spatial and temporal dynamics of septin subcellular localization is regulated remains to be fully addressed. As described above, some clues are emerging, as the incorporation of specific SEPT9 isoforms into oligomers was found to orient the final destination of septin filaments inside the cell. Other septins are also involved, as the SEPT5-containing complexes are enriched in the lamellipodia of squamous cell carcinoma DJM-1 cells, while complexes recovered along MTs exclude this septin (Mizutani et al., 2013).
Besides filament composition, much remains to be learned about the potential roles of septin monomers vs. oligomers and vs. filaments. Indeed, while SEPT2, 7, and 11 are required at the early stages of cytokinesis, only the SEPT9 deletion impairs the final separation of daughter cells (Estey et al., 2010), suggesting a role for SEPT9 even when it is not incorporated into a filament. Also, not only filaments but septin hexamers can mediate actin bending and bundling during actin remodeling at the furrow canal in Drosophila embryos (Mavrakis et al., 2014). Therefore, caution must be taken when overexpressing a single septin because it might perturb the original septin filament location and/or function, as observed for some SEPT9 isoforms. Also, a strong expression of individual septins may form homo-oligomers (see Fung et al., 2014; Sellin et al., 2014) that have nothing to do with physiological septin filaments. Furthermore, posttranslational modifications (phosphorylation, acetylation, and sumoylation) may impact the way septins assemble into higher-order structures as described in fungi and will deserve further investigation in the context of cancer (Hernández-Rodriguez and Momany, 2012).
Regarding cancer therapy or cell adaptation and resistance to chemotherapies, the direct targeting of septins could have many side effects. Nevertheless, septins have been proposed as molecular targets in solid tumors, where they are required for cytokinesis completion as opposed to hematopoietic cells (Menon et al., 2014). Alternatively, research of novel therapeutic targets might focus on the perturbation of the subcellular localization of septin filaments. As such, by dampening the lateral interactions between parallel septin filaments at the anaphase spindle midline, alternating electric fields used for the treatment of recurrent glioblastoma induce mitotic catastrophe and subsequent apoptosis (Gera et al., 2015). Septin relocalization in interphase cells might as well be achieved by the targeting of their membrane- or cytoskeletal-binding partners. Understanding the underlying mechanisms of septin subcellular localization therefore deserves more interest, and will be important to focus on in the years to come.
Author Contributions
CP, LK, and AB designed and wrote the manuscript.
Funding
This work was supported by INSERM and by Univ. Paris-Sud.
Conflict of Interest Statement
The authors declare that the research was conducted in the absence of any commercial or financial relationships that could be construed as a potential conflict of interest.
References
Ageta-Ishihara, N., Miyata, T., Ohshima, C., Watanabe, M., Sato, Y., Hamamura, Y., et al. (2013). Septins promote dendrite and axon development by negatively regulating microtubule stability via HDAC6-mediated deacetylation. Nat. Commun. 4, 2532. doi: 10.1038/ncomms3532
Akil, A., Peng, J., Omrane, M., Gondeau, C., Desterke, C., Marin, M. E. L., et al. (2016). Septin 9 induces lipid droplets growth by a phosphatidylinositol-5-phosphate and microtubule-dependent mechanism hijacked by HCV. Nat. Commun. 7, 1–19. doi: 10.1038/ncomms12203
Amir, S., and Mabjeesh, N. J. (2007). SEPT9_V1 protein expression is associated with human cancer cell resistance to microtubule-disrupting agents. Cancer Biol. Ther. 6, 1926–1931. doi: 10.4161/cbt.6.12.4971
Amir, S., Wang, R., Simons, J. W., and Mabjeesh, N. J. (2009). SEPT9_v1 Up-regulates Hypoxia-inducible Factor 1 by Preventing Its RACK1-mediated Degradation. J. Biol. Chem. 284, 1–10. doi: 10.1074/jbc.M808348200
Bai, X., Bowen, J. R., Knox, T. K., Zhou, K., Pendziwiat, M., Kuhlenbäumer, G., et al. (2013). Novel septin 9 repeat motifs altered in neuralgic amyotrophy bind and bundle microtubules. J. Cell Biol. 203, 895–905. doi: 10.1083/jcb.201308068
Barve, G., Sridhar, S., Aher, A., Singh, S., Lakshmeesha, K. N., and Manjithaya, R. (2016). Septins are involved at the early stages of macroautophagy. BioRXiv. doi: 10.1101/043133. [Epub ahead of print].
Baumann, S., König, J., Koepke, J., and Feldbrügge, M. (2013). Endosomal transport of septin mRNA and protein indicates local translation on endosomes and is required for correct septin filamentation. EMBO Rep. 15, 94–102. doi: 10.1002/embr.201338037
Bertin, A., McMurray, M. A., Thai, L., Garcia, G., Votin, V., Grob, P., et al. (2010). Phosphatidylinositol-4,5-bisphosphate promotes budding yeast septin filament assembly and organization. J. Mol. Biol. 404, 711–731. doi: 10.1016/j.jmb.2010.10.002
Bowen, J. R., Hwang, D., Bai, X., Roy, D., and Spiliotis, E. T. (2011). Septin GTPases spatially guide microtubule organization and plus end dynamics in polarizing epithelia. J. Cell Biol. 194, 187–197. doi: 10.1083/jcb.201102076
Bridges, A. A., Jentzsch, M. S., Oakes, P. W., Occhipinti, P., and Gladfelter, A. S. (2016). Micron-scale plasma membrane curvature is recognized by the septin cytoskeleton. J. Cell Biol. 213, 23–32. doi: 10.1083/jcb.201512029
Bridges, A. A., Zhang, H., Mehta, S. B., Occhipinti, P., Tani, T., and Gladfelter, A. S. (2014). Septin assemblies form by diffusion-driven annealing on membranes. Proc. Natl. Acad. Sci. U.S.A. 111, 2146–2151. doi: 10.1073/pnas.1314138111
Calvo, F., Ranftl, R., Hooper, S., Farrugia, A. J., Moeendarbary, E., Bruckbauer, A., et al. (2015). Cdc42EP3/BORG2 and septin network enables mechano-transduction and the emergence of cancer-associated fibroblasts. Cell Rep. 13, 2699–2714. doi: 10.1016/j.celrep.2015.11.052
Caudron, F., and Barral, Y. (2009). Septins and the lateral compartmentalization of eukaryotic membranes. Dev. Cell 16, 493–506. doi: 10.1016/j.devcel.2009.04.003
Cerveira, N., Bizarro, S., and Teixeira, M. R. (2011). MLL-SEPTIN gene fusions in hematological malignancies. Biol. Chem. 392, 1–12. doi: 10.1515/BC.2011.072
Chacko, A. D., Hyland, P. L., McDade, S. S., Hamilton, P. W., Russell, S. H., and Hall, P. A. (2005). SEPT9_v4 expression induces morphological change, increased motility and disturbed polarity. J. Pathol. 206, 458–465. doi: 10.1002/path.1794
Chacko, A. D., McDade, S. S., Chanduloy, S., Church, S. W., Kennedy, R., Price, J., et al. (2012). Expression of the SEPT9_i4 isoform confers resistance to microtubule-interacting drugs. Cell. Oncol. 35, 85–93. doi: 10.1007/s13402-011-0066-0
Connolly, D., Hoang, H. G., Adler, E., Tazearslan, C., Simmons, N., Bernard, V. V., et al. (2014). Septin 9 amplification and isoform-specific expression in peritumoral and tumor breast tissue. Biol. Chem. 395, 157–167. doi: 10.1515/hsz-2013-0247
Connolly, D., Yang, Z., Castaldi, M., Simmons, N., Oktay, M. H., Coniglio, S., et al. (2011). Septin 9 isoform expression, localization and epigenetic changes during human and mouse breast cancer progression. Breast Cancer Res. 13, R76. doi: 10.1186/bcr2924
Cortez, B. A., Rezende-Teixeira, P., Redick, S., Doxsey, S., and Machado-Santelli, G. M. (2016). Multipolar mitosis and aneuploidy after chrysotile treatment: a consequence of abscission failure and cytokinesis regression. Oncotarget 7, 8979–8992. doi: 10.18632/oncotarget.6924
Cui, C., Chatterjee, B., Lozito, T. P., Zhang, Z., Francis, R. J., Yagi, H., et al. (2013). Wdpcp, a PCP protein required for ciliogenesis, regulates directional cell migration and cell polarity by direct modulation of the actin cytoskeleton. PLoS Biol. 11:e1001720. doi: 10.1371/journal.pbio.1001720
Diesenberg, K., Beerbaum, M., Fink, U., Schmieder, P., and Krauss, M. (2015). SEPT9 negatively regulates ubiquitin-dependent downregulation of EGFR. J. Cell Sci. 128, 397–407. doi: 10.1242/jcs.162206
Dolat, L., Hunyara, J. L., Bowen, J. R., Karasmanis, E. P., Elgawly, M., Galkin, V. E., et al. (2014). Septins promote stress fiber-mediated maturation of focal adhesions and renal epithelial motility. J. Cell Biol. 207, 225–235. doi: 10.1083/jcb.201405050
Edison, N., Reingewertz, T.-H., Gottfried, Y., Lev, T., Zuri, D., Maniv, I., et al. (2012). Peptides mimicking the unique ARTS-XIAP binding site promote apoptotic cell death in cultured cancer cells. Clin. Cancer Res. 18, 2569–2578. doi: 10.1158/1078-0432.CCR-11-1430
Elhasid, R., and Larisch, S. (2011). ARTS-based anticancer therapy: taking aim at cancer stem cells. Future Oncol. 7, 1185–1194. doi: 10.2217/fon.11.96
Estey, M. P., Di Ciano-Oliveira, C., Froese, C. D., Bejide, M. T., and Trimble, W. S. (2010). Distinct roles of septins in cytokinesis: SEPT9 mediates midbody abscission. J. Cell Biol. 191, 741–749. doi: 10.1083/jcb.201006031
Estey, M. P., Di Ciano-Oliveira, C., Froese, C. D., Fung, K. Y. Y., Steels, J. D., Litchfield, D. W., et al. (2013). Mitotic regulation of SEPT9 protein by cyclin-dependent kinase 1 (Cdk1) and pin1 protein is important for the completion of cytokinesis. J. Biol. Chem. 288, 30075–30086. doi: 10.1074/jbc.M113.474932
Field, C. M., and Alberts, B. M. (1995). Anillin, a contractile ring protein that cycles from the nucleus to the cell cortex. J. Cell Biol. 131, 165–178. doi: 10.1083/jcb.131.1.165
Froidevaux-Klipfel, L., Poirier, F., Boursier, C., Crépin, R., Poüs, C., Baudin, B., et al. (2011). Modulation of septin and molecular motor recruitment in the microtubule environment of the Taxol-resistant human breast cancer cell line MDA-MB-231. Proteomics 11, 3877–3886. doi: 10.1002/pmic.201000789
Froidevaux-Klipfel, L., Targa, B., Cantaloube, I., Ahmed-Zaïd, H., Poüs, C., and Baillet, A. (2015). Septin cooperation with tubulin polyglutamylation contributes to cancer cell adaptation to taxanes. Oncotarget 6, 36063–36080. doi: 10.18632/oncotarget.5373
Füchtbauer, A., Lassen, L. B., Jensen, A. B., Howard, J., Quiroga Ade, S., Warming, S., et al. (2011). Septin9 is involved in septin filament formation and cellular stability. Biol. Chem. 392, 769–777. doi: 10.1515/BC.2011.088
Fung, K. Y., Dai, L., and Trimble, W. S. (2014). Cell and molecular biology of septins. Int. Rev. Cell Mol. Biol. 310, 289–339. doi: 10.1016/B978-0-12-800180-6.00007-4
García-Fernández, M., Kissel, H., Brown, S., Gorenc, T., Schiele, A. J., Rafii, S., et al. (2010). SEPT4/ARTS is required for stem cell apoptosis and tumor suppression. Genes Dev. 24, 2282–2293. doi: 10.1101/gad.1970110
Gera, N., Yang, A., Holtzman, T. S., Lee, S. X., Wong, E. T., and Swanson, K. D. (2015). Tumor treating fields perturb the localization of septins and cause aberrant mitotic exit. PLoS ONE 10:e0125269. doi: 10.1371/journal.pone.0125269
Ghossoub, R., Hu, Q., Failler, M., Rouyez, M. C., Spitzbarth, B., Mostowy, S., et al. (2013). Septins 2, 7 and 9 and MAP4 colocalize along the axoneme in the primary cilium and control ciliary length. J. Cell Sci. 126, 2583–2594. doi: 10.1242/jcs.111377
Gilad, R., Meir, K., Stein, I., German, L., Pikarsky, E., and Mabjeesh, N. J. (2015). High SEPT9_i1 protein expression is associated with high-grade prostate cancers. PLoS ONE 10:e0124251. doi: 10.1371/journal.pone.0124251
Golan, M., and Mabjeesh, N. J. (2013). SEPT9_i1 is required for the association between HIF-1α and importin-α to promote efficient nuclear translocation. Cell Cycle 12, 2297–2308. doi: 10.4161/cc.25379
Gonzalez, M. E., Makarova, O., Peterson, E. A., Privette, L. M., and Petty, E. M. (2009). Up-regulation of SEPT9_v1 stabilizes c-Jun-N-Terminal kinase and contributes to its pro-proliferative activity in mammary epithelial cells. Cell. Signal. 21, 477–487. doi: 10.1016/j.cellsig.2008.11.007
Hagiwara, A., Tanaka, Y., Hikawa, R., Morone, N., Kusumi, A., Kimura, H., et al. (2011). Submembranous septins as relatively stable components of actin-based membrane skeleton. Cytoskeleton (Hoboken) 68, 512–525. doi: 10.1002/cm.20528
Hall, P. A., and Russell, S. E. H. (2012). Mammalian septins: dynamic heteromers with roles in cellular morphogenesis and compartmentalization. J. Pathol. 226, 287–299. doi: 10.1002/path.3024
Hall, P. A., Todd, C. B., Hyland, P. L., McDade, S. S., Grabsch, H., Dattani, M., et al. (2005). The septin-binding protein anillin is overexpressed in diverse human tumors. Clin. Cancer Res. 11, 6780–6786. doi: 10.1158/1078-0432.CCR-05-0997
Hernández-Rodriguez, Y., and Momany, M. (2012). Posttranslational modifications and assembly of septin heteropolymers and higher-order structures. Curr. Opin. Microbiol. 15, 660–668. doi: 10.1016/j.mib.2012.09.007
Hu, Q., and Nelson, W. J. (2011). Ciliary diffusion barrier: the gatekeeper for the primary cilium compartment. Cytoskeleton (Hoboken) 68, 313–324. doi: 10.1002/cm.20514
Joberty, G., Perlungher, R. R., Sheffield, P. J., Kinoshita, M., Noda, M., Haystead, T., et al. (2001). Borg proteins control septin organization and are negatively regulated by Cdc42. Nat. Cell Biol. 3, 861–866. doi: 10.1038/ncb1001-861
Kinoshita, M. (2003). Assembly of mammalian septins. J. Biochem. 134, 491–496. doi: 10.1093/jb/mvg182
Kinoshita, M., Field, C. M., Coughlin, M. L., Straight, A. F., and Mitchison, T. J. (2002). Self- and actin-templated assembly of mammalian septins. Dev. Cell 3, 1–12. doi: 10.1016/S1534-5807(02)00366-0
Kinoshita, M., Kumar, S., Mizoguchi, A., Ide, C., Kinoshita, A., Haraguchi, T., et al. (1997). Nedd5, a mammalian septin, is a novel cytoskeletal component interacting with actin-based structures. Genes Dev. 11, 1535–1547. doi: 10.1101/gad.11.12.1535
Kremer, B. E., Adang, L. A., and MacAra, I. G. (2007). Septins regulate actin organization and cell-cycle arrest through nuclear accumulation of NCK mediated by SOCS7. Cell 130, 837–850. doi: 10.1016/j.cell.2007.06.053
Kremer, B. E., Haystead, T., and MacAra, I. G. (2005). Mammalian septins regulate microtubule stability through interaction with the microtubule-binding protein MAP4. Mol. Biol. Cell 16, 4648–4659. doi: 10.1091/mbc.E05-03-0267
Liu, M., Shen, S., Chen, F., Yu, W., and Yu, L. (2010). Linking the septin expression with carcinogenesis. Mol. Biol. Rep. 37, 3601–3608. doi: 10.1007/s11033-010-0009-2
Liu, Z., Vong, Q. P., Liu, C., and Zheng, Y. (2014). Borg5 is required for angiogenesis by regulating persistent directional migration of the cardiac microvascular endothelial cells. Mol. Biol. Cell 25, 841–851. doi: 10.1091/mbc.E13-09-0543
Marcus, E. A., Tokhtaeva, E., Turdikulova, S., Capri, J., Whitelegge, J. P., Scott, D. R., et al. (2016). Septin oligomerization regulates persistent expression of ErbB2/HER2 in gastric cancer cells. Biochem. J. 473, 1703–1718. doi: 10.1042/BCJ20160203
Mavrakis, M., Azou-Gros, Y., Tsai, F.-C., Alvarado, J., Bertin, A., Iv, F., et al. (2014). Septins promote F-actin ring formation by crosslinking actin filaments into curved bundles. Nat. Cell Biol. 16, 322–334. doi: 10.1038/ncb2921
Menon, M. B., and Gaestel, M. (2015). Sep(t)arate or not - how some cells take septin-independent routes through cytokinesis. J. Cell Sci. 128, 1877–1886. doi: 10.1242/jcs.164830
Menon, M. B., Sawada, A., Chaturvedi, A., Mishra, P., Schuster-Gossler, K., Galla, M., et al. (2014). Genetic deletion of SEPT7 reveals a cell type-specific role of septins in microtubule destabilization for the completion of cytokinesis. PLoS Genet. 10:e1004558. doi: 10.1371/journal.pgen.1004558
Mizutani, Y., Ito, H., Iwamoto, I., Morishita, R., Kanoh, H., Seishima, M., et al. (2013). Possible role of a septin, SEPT1, in spreading in squamous cell carcinoma DJM-1 cells. Biol. Chem. 394, 281–290. doi: 10.1515/hsz-2012-0258
Montagna, C., Sagie, M., and Zechmeister, J. (2015). Mammalian septins in health and disease. Res. Rep. Biochem. 5, 59–14. doi: 10.2147/RRBC.S59060
Mostowy, S., and Cossart, P. (2012). Septins: the fourth component of the cytoskeleton. Nat. Rev. Mol. Cell. Biol. 13, 183–194. doi: 10.1038/nrm3284
Mostowy, S., Janel, S., Forestier, C., Roduit, C., Kasas, S., Pizarro-Cerdá, J., et al. (2011). A role for septins in the interaction between the listeria monocytogenes invasion protein InlB and the met receptor. Biophys. J. 100, 1949–1959. doi: 10.1016/j.bpj.2011.02.040
Nagata, K. I., Kawajiri, A., Matsui, S., Takagishi, M., Shiromizu, T., Saitoh, N., et al. (2003). Filament formation of MSF-A, a mammalian septin, in human mammary epithelial cells depends on interactions with microtubules. J. Biol. Chem. 278, 18538–18543. doi: 10.1074/jbc.M205246200
Osaka, M., Rowley, J. D., and Zeleznik-Le, N. J. (1999). MSF (MLL septin-like fusion), a fusion partner gene of MLL, in a therapy-related acute myeloid leukemia with a t(11;17)(q23;q25). Proc. Natl. Acad. Sci. U.S.A. 96, 6428–6433. doi: 10.1073/pnas.96.11.6428
Pagliuso, A., Tham, T. N., Stevens, J. K., Lagache, T., Persson, R., Salles, A., et al. (2016). A role for septin 2 in Drp1-mediated mitochondrial fission. EMBO Rep. 17, 858–873. doi: 10.15252/embr.201541612
Renshaw, M. J., Liu, J., Lavoie, B. D., and Wilde, A. (2014). Anillin-dependent organization of septin filaments promotes intercellular bridge elongation and Chmp4B targeting to the abscission site. Open Biol. 4, 130190–130190. doi: 10.1098/rsob.130190
Rocha, C., Papon, L., Cacheux, W., Marques Sousa, P., Lascano, V., Tort, O., et al. (2014). Tubulin glycylases are required for primary cilia, control of cell proliferation and tumor development in colon. EMBO J. 33, 2247–2260. doi: 10.15252/embj.201488466
Saarikangas, J., and Barral, Y. (2011). The emerging functions of septins in metazoans. EMBO Rep. 12, 1118–1126. doi: 10.1038/embor.2011.193
Sadian, Y., Gatsogiannis, C., Patasi, C., Hofnagel, O., Goody, R. S., Farkašovský, M., et al. (2013). The role of Cdc42 and Gic1 in the regulation of septin filament formation and dissociation. eLife 2, 8274–8226. doi: 10.7554/eLife.01085
Schmidt, K., and Nichols, B. J. (2004). Functional interdependence between septin and actin cytoskeleton. BMC Cell Biol. 5:43. doi: 10.1186/1471-2121-5-43
Scott, M., McCluggage, W. G., Hillan, K. J., Hall, P. A., and Russell, S. E. H. (2006). Altered patterns of transcription of the septin gene, SEPT9, in ovarian tumorigenesis. Int. J. Cancer 118, 1325–1329. doi: 10.1002/ijc.21486
Sellin, M. E., Sandblad, L., Stenmark, S., and Gullberg, M. (2011). Deciphering the rules governing assembly order of mammalian septin complexes. Mol. Biol. Cell 22, 3152–3164. doi: 10.1091/mbc.E11-03-0253
Sellin, M. E., Stenmark, S., and Gullberg, M. (2012). Mammalian SEPT9 isoforms direct microtubule-dependent arrangements of septin core heteromers. Mol. Biol. Cell 23, 4242–4255. doi: 10.1091/mbc.E12-06-0486
Sellin, M. E., Stenmark, S., and Gullberg, M. (2014). Cell type-specific expression of SEPT3-homology subgroup members controls the subunit number of heteromeric septin complexes. Mol. Biol. Cell 25, 1594–1607. doi: 10.1091/mbc.E13-09-0553
Shankar, J., Messenberg, A., Chan, J., Underhill, T. M., Foster, L. J., and Nabi, I. R. (2010). Pseudopodial actin dynamics control epithelial-mesenchymal transition in metastatic cancer cells. Cancer Res. 70, 3780–3790. doi: 10.1158/0008-5472.CAN-09-4439
Sheffield, P. J., Oliver, C. J., Kremer, B. E., Sheng, S., Shao, Z., and MacAra, I. G. (2003). Borg/septin interactions and the assembly of mammalian septin heterodimers, trimers, and filaments. J. Biol. Chem. 278, 3483–3488. doi: 10.1074/jbc.M209701200
Shen, S., Liu, M., Wu, Y., Saiyin, H., Liu, G., and Yu, L. (2012). Involvement of SEPT4_i1 in hepatocellular carcinoma: SEPT4_i1 regulates susceptibility to apoptosis in hepatocellular carcinoma cells. Mol. Biol. Rep. 39, 4519–4526. doi: 10.1007/s11033-011-1242-z
Silverman-Gavrila, R., and Silverman-Gavrila, L. (2008). Septins: new microtubule interacting partners. Sci. World J. 8, 611–620. doi: 10.1100/tsw.2008.87
Sirianni, A., Krokowski, S., Lobato-Márquez, D., Buranyi, S., Pfanzelter, J., Galea, D., et al. (2016). Mitochondria mediate septin cage assembly to promote autophagy of Shigella. EMBO Rep. 17, 1029–1043. doi: 10.15252/embr.201541832
Smith, C., Dolat, L., Angelis, D., Forgacs, E., Spiliotis, E. T., and Galkin, V. E. (2015). Septin 9 exhibits polymorphic binding to f-actin and inhibits myosin and cofilin activity. J. Mol. Biol. 427, 3273–3284. doi: 10.1016/j.jmb.2015.07.026
Song, L., and Li, Y. (2015). SEPT9: a specific circulating biomarker for colorectal cancer. Adv. Clin. Chem. 72, 171–204. doi: 10.1016/bs.acc.2015.07.004
Spiliotis, E. T., and Gladfelter, A. S. (2012). Spatial guidance of cell asymmetry: septin GTPases show the way. Traffic 13, 195–203. doi: 10.1111/j.1600-0854.2011.01268.x
Spiliotis, E. T., Hunt, S. J., Hu, Q., Kinoshita, M., and Nelson, W. J. (2008). Epithelial polarity requires septin coupling of vesicle transport to polyglutamylated microtubules. J. Cell Biol. 180, 295–303. doi: 10.1083/jcb.200710039
Spiliotis, E. T., Kinoshita, M., and Nelson, W. J. (2005). A mitotic septin scaffold required for Mammalian chromosome congression and segregation. Science 307, 1781–1785. doi: 10.1126/science.1106823
Surka, M. C., Tsang, C. W., and Trimble, W. S. (2002). The mammalian septin MSF localizes with microtubules and is required for completion of cytokinesis. Mol. Biol. Cell 13, 3532–3545. doi: 10.1091/mbc.E02-01-0042
Tanaka-Takiguchi, Y., Kinoshita, M., and Takiguchi, K. (2009). Septin-mediated uniform bracing of phospholipid membranes. Curr. Biol. 19, 140–145. doi: 10.1016/j.cub.2008.12.030
Tooley, A. J., Gilden, J., Jacobelli, J., Beemiller, P., Trimble, W. S., Kinoshita, M., et al. (2009). Amoeboid T lymphocytes require the septin cytoskeleton for cortical integrity and persistent motility. Nat. Cell Biol. 11, 17–26. doi: 10.1038/ncb1808
Xu, M., Takanashi, M., Oikawa, K., Nishi, H., Isaka, K., Yoshimoto, T., et al. (2012). Identification of a novel role of Septin 10 in paclitaxel-resistance in cancers through a functional genomics screen. Cancer Sci. 103, 821–827. doi: 10.1111/j.1349-7006.2012.02221.x
Yeh, Y.-T., Hur, S. S., Chang, J., Wang, K.-C., Chiu, J.-J., Li, Y.-S., et al. (2012). Matrix stiffness regulates endothelial cell proliferation through septin 9. PLoS ONE 7:e46889. doi: 10.1371/journal.pone.0046889
Zander, S., Baumann, S., Weidtkamp-Peters, S., and Feldbrügge, M. (2016). Endosomal assembly and transport of heteromeric septin complexes promote septin cytoskeleton formation. J. Cell Sci. 129, 2778–2792. doi: 10.1242/jcs.182824
Zhang, J., Kong, C., Xie, H., McPherson, P. S., Grinstein, S., and Trimble, W. S. (1999). Phosphatidylinositol polyphosphate binding to the mammalian septin H5 is modulated by GTP. Curr. Biol. 9, 1458–1467. doi: 10.1016/S0960-9822(00)80115-3
Keywords: septin, cancer, plasma membrane, actin cytoskeleton, microtubules
Citation: Poüs C, Klipfel L and Baillet A (2016) Cancer-Related Functions and Subcellular Localizations of Septins. Front. Cell Dev. Biol. 4:126. doi: 10.3389/fcell.2016.00126
Received: 31 August 2016; Accepted: 24 October 2016;
Published: 08 November 2016.
Edited by:
Manoj B. Menon, Hannover Medical School, GermanyReviewed by:
Li-Wha Wu, National Cheng Kung University, TaiwanOlga Vagin, University of California, Los Angeles, USA
Copyright © 2016 Poüs, Klipfel and Baillet. This is an open-access article distributed under the terms of the Creative Commons Attribution License (CC BY). The use, distribution or reproduction in other forums is permitted, provided the original author(s) or licensor are credited and that the original publication in this journal is cited, in accordance with accepted academic practice. No use, distribution or reproduction is permitted which does not comply with these terms.
*Correspondence: Anita Baillet, anita.baillet@u-psud.fr