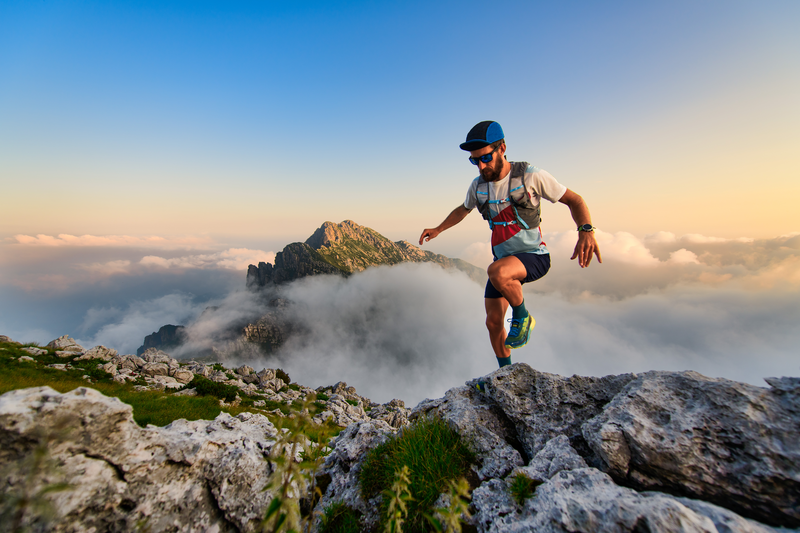
95% of researchers rate our articles as excellent or good
Learn more about the work of our research integrity team to safeguard the quality of each article we publish.
Find out more
MINI REVIEW article
Front. Cell Death , 23 July 2024
Sec. Cellular Stress and Survival: Crosslinks, Intersections, and Pathways
Volume 3 - 2024 | https://doi.org/10.3389/fceld.2024.1422224
This article is part of the Research Topic Women in Cell Death Research View all articles
In addition to many industrial activities that release pollutants in coastal areas, numerous human behaviors contribute to climate change, inducing global warming, which can also reshape the environmental impacts of some pollutants. Therefore, it is extremely important to develop new tools that can detect pollutants and environmental changes quickly and easily with high levels of sensitivity. The sea urchin embryo is a well-known model used worldwide in many research fields, including marine ecotoxicology, as a huge range of contaminants can affect its embryonic development with species-specific sensitivity. Morphological abnormalities are already considered biomarkers to evaluate the effects of pollutants, and, indeed, the sea urchin has long been used as one of the key species in a battery of bioassays to assess the toxicity of many pollutants and dredged sediments. At the cellular level, the molecular mechanisms activated against a stress agent constitute what is known as the “cell stress response,” analyzed here within a whole organism, namely, the sea urchin embryo. In this minireview, we have reported the available molecular biomarkers linked to morphological abnormalities and the genes affected by environmental changes and emerging pollutants, highlighting those studies that use high-throughput screening approaches to evaluate the effects of environmental conditions on sea urchin embryos.
In 1962, Ritossa published a discovery regarding “new and unknown factors” synthesized by Drosophila cells in response to elevated temperatures (Ritossa, 1962). This study, not well-acknowledged at that time, opened the way to heat shock protein (HSP) research and, more generally, to cell stress response studies that had an unexpected impact on many research fields (De Maio et al., 2012). Nowadays, it is well known that HSPs play key roles in many cellular processes as well as in many human diseases and cancer (Hu et al., 2022). Considering marine invertebrates, HSP expression is often tightly coupled to environmental thermal conditions (Tomanek, 2010) as well as to pollutant exposure (Magesky and Pelletier, 2018). In nature, embryos and larvae are part of the zooplankton and must cope with many adverse environmental conditions, such as food deprivation, temperature, UV radiation, and pollutants (always as mixtures), that can affect their development, and, consequently, the successful transition to the adult stage (Hamdoun and Epel, 2007). The sea urchin embryo represents an acknowledged model organism in ecotoxicology (Morroni et al., 2023). It is able to activate a variety of actions to counteract external and internal harmful signals, which constitute the cell stress response (Goldstone et al., 2006; Bonaventura and Matranga, 2017), similarly to many other organisms, from the lowest to the highest evolutionary levels. Many studies used sea urchin embryos to analyze the molecular mechanisms adopted to cope with various pollutants, as, for example, metals (Bonaventura et al., 2015; Martino et al., 2017); climate change factors, such as CO2-increase, acidification, and ocean warming (Stumpp et al., 2011; Layous et al., 2021); UV radiation (Bonaventura et al., 2005; Russo et al., 2010); nanoparticles (Della Torre et al., 2014; Gambardella et al., 2016); and microplastics (Rendell-Bhatti et al., 2021; Paganos et al., 2023). For example, a survey for the homologs of gene families involved in the protection against chemical stressors (“chemical defensome”) identified more than 400 genes in the sea urchin genome (Goldstone et al., 2006). Following exposure to pollutants such as heavy metals, physical factors, and toxic natural compounds, the sea urchin embryo can activate apoptosis or autophagic processes (Di Tuccio et al., 2023), depending on the intensity of the stress stimuli.
Here, we have highlighted studies using high-throughput screening approaches, with techniques such as qPCR, cDNA microarray, NanoString nCounter technology, and RNA-seq. Indeed, these approaches have been increasingly applied to deeply analyze the molecular response to pollutants, in addition to chemical and physical stresses, in embryos of different sea urchin species.
In particular, we focused our attention on the gene modulations following different exposure conditions of the sea urchin embryo, considering the genes involved in the cellular stress response as well as in the developmental program, which could be exploited as biomarkers of pollutants with harmful effects in the marine biomonitoring field. Biomarkers are quantifiable parameters at the cellular, biochemical, molecular, and physiological levels of an organism, which, thanks to their changes in response to pollution stress, are used as indicators of the presence of pollutants in the environment. Biomarkers can be specific to a single pollutant and must be sensitive enough to promptly reveal environmental changes according to the pollutant levels considered harmful for biomonitoring.
The key role of the sea urchin embryo as a study model, first in embryology and afterward in many other fields, is beyond doubt (Ernst, 2011).
In ecotoxicological studies, the morphological abnormalities of sea urchin embryos exposed to different classes of contaminants are a fundamental first step of analysis and can be used as biomarkers of pollution stress (Gambardella et al., 2021).
In recent decades, a deep understanding of gene regulatory networks (GRNs) has been achieved, describing the specification of embryonic territories under physiological conditions (Davidson et al., 2002; McClay et al., 2016). This deep understanding of the molecular mechanisms governing sea urchin embryo development has allowed an evolution in the type of approaches used in ecotoxicological studies. However, when embryos are treated with a stress agent in “in vitro” experiments, the first step of analysis usually remains the evaluation of their morphologies. The embryonic skeleton, both its formation and patterning, is one of the main targets affected by different pollutants (Matranga et al., 2011). This target structure is damaged, for example, by UV radiation (UVR) and cadmium, as well as by their combination (Bonaventura et al., 2015), by lithium, nickel, and zinc (Bonaventura et al., 2018; Russo et al., 2018) or PVC leachates (Rendell-Bhatti et al., 2021; Paganos et al., 2023). The well-known skeletal marker msp130, a glycoprotein specifically expressed on the surface of skeletogenic cells (Anstrom et al., 1987), has been examined in all these studies. For example, the analysis of this skeletal marker was recently used to evaluate and discriminate the different effects of various types of plastics on skeleton development, linking the most evident skeletal defects to the presence of PVC leachates in the embryo cultures (Rendell-Bhatti et al., 2021).
Additional markers specific for other embryonic structures are useful for assessing the damage suffered, both revealed through specific antibodies (to visualize the corresponding proteins) and specific mRNA probes (to evaluate gene expression changes), such as myosin heavy chain (MHC) and Endo1 proteins, or gcm and pks1 (Wessel and McClay, 1985; Bonaventura et al., 2018; Paganos et al., 2023).
Many cell type-specific markers of the nervous system are also available, such as the pan-neuronal marker Sp-syt1 (Synaptotagmin B) (Burke et al., 2006; Paganos et al., 2023), serotonin (Rendell-Bhatti et al., 2021), and the ciliary band markers β-tubulin (Paganos et al., 2021; 2023), all potential markers of damages from pollutants to the embryonic nervous system.
A crucial question in ecology is how organisms survive environmental changes. Nowadays, it is clearly established that climate changes, together with ionizing radiation, noise, and vibration (Solé et al., 2023), are affecting the marine environment and its inhabitants. Here, we provide an overview of climate change and ionizing radiation studies describing the transcriptomic responses of the sea urchin embryo.
Due to anthropogenic CO2 emissions and depending on their future magnitude, seawater pCO2 is expected to increase significantly in the coming years compared to current values, leading to reductions in pH and carbonate ion concentrations of seawater (a phenomenon known as ocean acidification, OA) (Melzner et al., 2020). Therefore, an important new research priority in marine sciences is understanding the mechanisms by which marine organisms respond to rapidly changing oceans. Significant progress has been made in the OA research field recently, with the use of various techniques, leading to the identification of highly sensitive species and others quite resistant to the changes associated with OA. Even in this research field, the sea urchin embryo represents a suitable model organism, and much information on its response to environmental changes has been obtained mainly from laboratory studies by combining morphological and molecular analyses. The differences in the species and developmental stages analyzed and the methodologies used make it difficult to compare transcriptomic results between studies as well as summarize the key findings obtained (Strader et al., 2020). Even if fundamental molecular responses to OA appear common (including differential regulation of metabolic pathways and skeletogenic-specific genes, activation of cellular stress response, and regulation of acid–base ion transport mechanisms), a diversity in the ability to tolerate different pH environments can be observed between different species and across different life stages within the same species. The diversity of biological responses was highlighted in early studies by RT-qPCR, specifically showing ATP-synthase and Na+/K+-ATPase upregulation and sm30b (a biomineralization gene) downregulation (Stumpp et al., 2011) and by microarray-based transcriptomic analyses on 1,057 genes (Todgham and Hofmann, 2009) (Table 1). A genomics-based approach using transcriptomics is a recent tool applied in the attempt to identify the physiological capacity of sea urchins to respond and/or adapt to OA, as described in the following few studies (limited due to space). The transcriptomic response to OA has been measured in the Heliocidaris erythrogramma species in controlled laboratory experiments. A predicted future ocean pH condition (7.6) was compared to the current one (8.0) at three developmental stages. In addition to highlighting changes in the gene expression at all the different conditions (particularly of genes involved in biomineralization, msp130, the defensome, hsp70, and genes encoding for calcium toolkit and chaperone proteins), the authors measured the variance and changes in the shape of gene expression profiles, suggesting the importance of examining diverse aspects of the molecular response to OA to understand its complexity (Devens et al., 2020) (Table 1). A similar approach was applied to study the OA effects on Strongylocentrotus droebachiensis, that is, RNA-seq together with gene set variation analyses on 14-day-old larvae cultured at pH 8 and 7.6 (Runcie et al., 2016). In addition to changes in gene expression similar to those observed in H. erythrogramma, increased expression of genes involved in immune responses has been described in S. droebachiensis embryos, larvae, and juveniles. This study also highlighted the complexity of molecular responses to OA, discussing the importance of transcriptomic and quantitative genetics approaches to understand the biological impact of climate change. Recently, a single-embryo RNA-seq analysis of H. crassispina grown at three pH conditions (pH 8.0, 7.7, and 7.4) reported gene expression changes to OA at an individual and family level (Fung et al., 2023) (Table 1). The transcriptomic profiles of every single embryo showed differences in the expression of genes involved in biomineralization (ets1), development (univin, nodal, and lefty), and metabolism (gfpt1 and E2F family genes) induced by pH conditions.
Table 1. Genes affected by different exposure conditions in sea urchin embryos and larvae: potential biomarkers of embryo toxicity.
In nature, environmental changes occur in a multi-stressor context, with a combination of lower pH levels, higher temperatures, and lower oxygen concentrations than normal conditions. Typically, multi-stressor transcriptomic studies include only two stress factors, that is, low pH and high temperature, while other factors, such as oxygen or salinity levels, have not been considered as often. Recently, a transcriptomic analysis has been performed to investigate the influence of both temperature and pCO2 levels on the early development of Mesocentrotus franciscanus (Wong and Hofmann, 2021). The transcriptomic patterns varied between the two developmental stages analyzed, especially between the two treatment conditions. The warmer temperature mainly induced cellular stress response and increased expression of genes related to transmembrane transport at the gastrula stage, whereas pCO2 treatment had a smaller effect, and both factors together did not significantly affect embryos. At the prism stage, warmer temperature caused an upregulation of genes related to transmembrane transport (ion binding, ion transmembrane transporter, cation channel, and sodium and potassium ion transmembrane transporters), indicating osmoregulation and homeostasis maintenance and genes related to energetic processes, probably to meet higher energetic demand. These results suggest a plasticity at 17°C, which may not be present under more severe warming conditions. Furthermore, sea urchin embryos appear to be more vulnerable to increased pCO2 levels and, therefore, to reduced seawater pH levels than to warming temperatures.
The impossibility of replicating the full range of variables that naturally occur within the ecosystems is one of the major limitations of controlled laboratory experiments. Thus, the need arose to verify whether the responses of marine organisms extrapolated from laboratory exposures were consistent with those naturally occurring in ecologically relevant sites. Natural vent sites have been identified that release CO2 gas into the surrounding seawater, creating generally hostile habitats for animals. These sites are considered ideal “natural laboratories” for studying future OA conditions. Until now, field studies on sea urchin embryos/larvae grown at natural CO2-vent sites are very few, probably due to the difficulty of organizing the correct experimental setup. In addition to evaluating the relationships between abnormal development of sea urchin larvae and elevated pCO2/reduced pH of the environment (Lamare et al., 2016) as well as temperature, oxygen concentration, salinity, and food availability of the sites (Foo et al., 2020), few field studies examined their genetic response to OA by investigating genes and functional groups (Uthicke et al., 2019). In addition, some recent studies reported interactive effects between marine acidification and pollutants, such as pharmaceutical compounds or metals, on sea urchin embryos, but no molecular data are available (Dorey et al., 2018; Pusceddu et al., 2022).
All these phenomena can impact food availability, which is extremely important for the vital processes of marine animals. Therefore, reduced food availability can have further negative effects on their survival strategies. Depending on food availability (microalgae), sea urchin embryos and larvae can experience nutritional stress, recently studied through a transcriptome approach (RNA-seq) analyzing the gene expression signature linked to food deprivation. In particular, starved larvae of S. droebachiensis showed upregulated genes involved in lipid transport, environmental sensing, and defense, that is, elk, foxj1, creb, nf-kb, the neural excitatory amino acid transporter eaat, and the translation inhibitors 4ebp and foxo (Carrier et al., 2015) (Table 1). Recently, Li et al. (2023) (Table 1) identified 14 biomarker genes belonging to different categories in unfed larvae of S. purpuratus: membrane transporter, hsp, extracellular matrix, immune response, and receptor and gene regulation. Of these, the gene LOC575259 coding for the neutral amino acid transporter (aat) was the most highly expressed, suggesting that unfed larvae use organic matter dissolved in the seawater as an energy source. Both studies, performed under different feeding regimes, highlighted that sea urchin larvae can respond to unfavorable environmental conditions by delaying or arresting their development and metabolic activities and modulating their gene expression patterning, a phenomenon known as “developmental plasticity,” which is necessary to survive.
In addition to OA and warming, global climate change has the potential to partially worsen ozone layer depletion in the Arctic, increasing the transmission of solar UVR through the atmosphere to the ocean (Day and Neale, 2002; Barnes et al., 2022). UVR is known to affect sea urchin embryos living in shallow water, which in turn can activate UVR molecular defense systems at the transcriptional, translational, and post-translational levels. Various aspects should be taken into consideration, looking for molecular biomarkers related to UVR stress response, such as different sensitivity to UVR related to the sea urchin species, developmental stages (early or late), duration (short or long-term) of exposure, and doses chosen for the UVR (Bonaventura and Matranga, 2017). Few studies have examined this question, and of them, very few reported field data (Lesser, 2010; Lister et al., 2010). Meanwhile, laboratory experiments have evaluated developmental abnormalities, survival, oxidative stress, DNA damage, and activation of antioxidant defense mechanisms, and only some addressed the molecular aspect by focusing on the up- or downregulation or delocalization of gene expression involved in UVR stress (Russo et al., 2010; 2014).
In particular, a high-throughput screening approach has been applied to P. lividus embryos exposed in vitro to UVR to characterize the molecular responses adopted to cope with this stressor (Bonaventura et al., 2021). Using NanoString nCounter technology, 127 genes encoding for regulatory and structural proteins were analyzed in embryos 48 h post irradiation and showed dysregulation of the expression of some genes, including hsp70-II, jun, gal-8, sox9, and Hbox12, and genes related to neuronal development, such as sspo (or SCO-spondin precursor) (Table 1). The modulation of sspo gene expression caused by UVR intriguingly suggests putative effects on the mechanisms regulating the sea urchin embryo’s nervous development (Bonaventura et al., 2021).
In recent decades, environmental pollution has increased in tandem with the increase in human activities. The marine ecosystem is distressed by a wide variety of new anthropogenic pollutants that include many substances called “emerging contaminants” (ECs) released when industrial, agricultural, hospital, and domestic wastes are discarded in the sea (Landrigan et al., 2020; González-Gaya, 2021). Differently from the classical “pollutants” (pesticides, hydrocarbons, and heavy metals), the ECs include compounds such as pharmaceutical drugs, personal care products, microplastics, and nanoparticles. They can cause toxic effects even in trace amounts, thus representing a high-risk factor to a single organism and a threat to the entire marine biota, as well as to human health via food chains, due to their persistence in the environment and accumulation in the living tissues (Pereira et al., 2015).
Plastic pollution and its effects on marine ecosystems are of particular concern for the scientific community and for civil society as well. Organisms at all levels of the food chain, from top predators to plankton, and even in remote places, have experienced plastic pollution in the form of ingestion, suffocation, and chemical contamination (Tekman et al., 2023). The world of plastic is variegated in its polymer compositions and consequently in the related waste, known to be classified by size as macro (>25 mm), meso (25–5 mm), large micro (5–1 mm), small micro (1 mm–20 μm), and nano (1,000–1 nm) plastics (Nayanathara Thathsarani Pilapitiya and Ratnayake, 2024).
Studies have evaluated the effects of micro- (MPs) and nano- (NPs) plastics on sea urchin embryos (Gambardella et al., 2021), although the effects attributed to MPs actually appear due to leaching chemicals (additive and absorbed chemicals) released by MPs rather than to MP particles themselves (Manzo and Schiavo, 2022), as reported in detail by the two recent reviews cited. This was reported by a study comparing leachate obtained from plastic particles collected from a beach and industrial ones (polyvinyl chloride, PVC), showing that abnormal morphologies in P. lividus embryos were linked to chemicals, persistent organic pollutant (PAHs and PCBs) leached from industrial PVC (Rendell-Bhatti et al., 2021). The molecular response of S. purpuratus embryos and larvae to PVC leachates was deeply analyzed by a differential RNA sequencing (deRNA-seq) approach at 48- and 72-hours post fertilization (hpf) (Paganos et al., 2023). Thanks to a single-cell type atlas, transcriptomic data highlighted an increased number of PVC leachate downregulated genes (86% at 48 hpf and 77% at 72 hpf) belonging to the anterior neuroectoderm/apical plate, aboral ectoderm, neurons, immune, and skeletal cells, while the upregulated genes mainly belonged to gut-derived cluster cells. Furthermore, authors characterized the deregulated key genes in detail, considering them both for their role in the cell type specification GRNs (i.e., pax6 and soxc, involved in neural development, and ets1, involved in biomineralization) and as markers of distinct cell types (i.e., gcm and pks1 expressed in immune cells) (Table 1). These genes might potentially be taken into consideration as biomarkers of PVC leachate impact.
The gene expression effects of metals such as Cd (Bonaventura et al., 2021), Li, Ni, and Zn (Bonaventura et al., 2022) have been evaluated in P. lividus embryos (Table 1) using NanoString nCounter technology. Cd exposure caused the upregulation of stress genes, such as metallothionein (MT), hsp70-II, and hsp60, and the downregulation of pax6, sox9/soxE, and two nervous-related genes, onecut/hnf6 and sspo, also downregulated in embryos exposed to UVB and to the combination Cd/UV-B (Bonaventura et al., 2021). Although the sublethal concentrations of Li, Ni, and Zn used were not environmentally relevant, also due to the short period of metal exposure (0–48 h), the study by Bonaventura et al. gave some indications about the genes that were sensitive to Li, Ni, and Zn that can be used as potential biomarkers of impact (Bonaventura et al., 2022). Among them at 48 hpf, 14-3-3epsilon (adapter protein), hsp70-II, gata1/2/3 (zinc finger TF), nectin (extracellular matrix protein), vegfr (biomineralization), and chordin and pax2/5/8 (nervous system) genes were affected by all the three metals. In addition, some other potential biomarker genes could be considered, such as carbonic anhydrase (can/ca) (biomineralization), hox7, foxA, pks1 (immunity), irxa, sox9 (myogenesis), and wnt16, as they are greatly affected by one or more of these metals.
In this minireview, we have reported an update, although not-exhaustive, of the current knowledge of the sea urchin embryo genes involved in the cellular stress response following exposure to different environmental conditions and different types of pollutants.
The genome sequence of S. purpuratus has been available since 2006 (Sodergren et al., 2006), and recently, the sequences of other Echinoderms (Arshinoff et al., 2022), including P. lividus (Marlétaz et al., 2023; Costantini et al., 2024), have become available and are of great support in many different fields of research. As highlighted, the genomic data collected in this review suggest that exposure to different insults related to climate change and pollution causes lethal consequences in sea urchin embryos, affecting both structural and functional genes and so demonstrating the high sensitivity of this marine invertebrate. These data can be very useful in providing new approaches to address ecotoxicological studies, encouraging new possible monitoring and intervention strategies with the potential to be exploited as an alarm bell of both climatic and pollution changes in the marine environment. However, some challenges need to be addressed before this new approach can be fully applied in ecotoxicology. For example, many genes related to the formation of the embryonic skeleton and the nervous and immune systems could be used as potential biomarkers of deleterious effects (Deidda et al., 2021). In this direction, our review focused on highlighting genes related to dangerous exposure, with the aim of facilitating the identification of panels of modulated genes to be potentially exploited as biomarkers for the evaluation of embryotoxicity.
Integrative approaches linking morphological defects to biochemical and physiological measures, as well as gene expression signatures and GRN analyses, are needed for a deep understanding of how sea urchins and other marine organisms respond to a wide variety of environmental conditions.
Furthermore, multidisciplinary approaches should be encouraged, which, in addition to molecular biology research, should also include field analyses and studies on the interactions between different pollutants.
A last but not least important aspect to consider is that sea urchins are keystone species of sublittoral ecosystems. Some of them, including P. lividus, have a high economic value as their gonads are edible (Boudouresque and Verlaque, 2020). Therefore, understanding how future ocean conditions (including physical and chemical changes as well as environmental pollution) will affect the overall fitness and adaptive potential of sea urchins in nature, as well as how they will respond, is needed to support fisheries conservation and management strategies.
ID: writing–original draft and writing–review and editing. RR: writing–review and editing. NL: writing–review and editing. FZ: writing–original draft and writing–review and editing. RB: conceptualization, writing–original draft, and writing–review and editing.
The authors declare that no financial support was received for the research, authorship, and/or publication of this article.
The authors declare that the research was conducted in the absence of any commercial or financial relationships that could be construed as a potential conflict of interest.
All claims expressed in this article are solely those of the authors and do not necessarily represent those of their affiliated organizations, or those of the publisher, the editors, and the reviewers. Any product that may be evaluated in this article, or claim that may be made by its manufacturer, is not guaranteed or endorsed by the publisher.
Anstrom, J. A., Chin, J. E., Leaf, D. S., Parks, A. L., and Raff, R. A. (1987). Localization and expression of msp130, a primary mesenchyme lineage-specific cell surface protein in the sea urchin embryo. Development 101, 255–265. doi:10.1242/dev.101.2.255
Arshinoff, B. I., Cary, G. A., Karimi, K., Foley, S., Agalakov, S., Delgado, F., et al. (2022). Echinobase: leveraging an extant model organism database to build a knowledgebase supporting research on the genomics and biology of echinoderms. Nucleic Acids Res. 50, D970–D979. doi:10.1093/nar/gkab1005
Barnes, P. W., Robson, T. M., Neale, P. J., Williamson, C. E., Zepp, R. G., Madronich, S., et al. (2022). Environmental effects of stratospheric ozone depletion, UV radiation, and interactions with climate change: UNEP Environmental Effects Assessment Panel, Update 2021. Photochem. Photobiol. Sci. 21, 275–301. doi:10.1007/s43630-022-00176-5
Bonaventura, R., Costa, C., Deidda, I., Zito, F., and Russo, R. (2022). Gene expression analysis of the stress response to lithium, nickel, and zinc in Paracentrotus lividus embryos. Toxics 10, 325. doi:10.3390/toxics10060325
Bonaventura, R., and Matranga, V. (2017). Overview of the molecular defense systems used by sea urchin embryos to cope with UV radiation. Mar. Environ. Res. 128, 25–35. doi:10.1016/j.marenvres.2016.05.019
Bonaventura, R., Poma, V., Costa, C., and Matranga, V. (2005). UVB radiation prevents skeleton growth and stimulates the expression of stress markers in sea urchin embryos. Biochem. Biophys. Res. Commun. 328, 150–157. doi:10.1016/j.bbrc.2004.12.161
Bonaventura, R., Russo, R., Zito, F., and Matranga, V. (2015). Combined effects of cadmium and UVB radiation on sea urchin embryos: skeleton impairment parallels p38 MAPK activation and stress genes overexpression. Chem. Res. Toxicol. 28, 1060–1069. doi:10.1021/acs.chemrestox.5b00080
Bonaventura, R., Zito, F., Chiaramonte, M., Costa, C., and Russo, R. (2018). Nickel toxicity in P. lividus embryos: dose dependent effects and gene expression analysis. Mar. Environ. Res. 139, 113–121. doi:10.1016/j.marenvres.2018.05.002
Bonaventura, R., Zito, F., Russo, R., and Costa, C. (2021). A preliminary gene expression analysis on Paracentrotus lividus embryos exposed to UVB, Cadmium and their combination. Aquat. Toxicol. 232, 105770. doi:10.1016/j.aquatox.2021.105770
Boudouresque, C. F., and Verlaque, M. (2020). “Paracentrotus lividus,” in Developments in aquaculture and fisheries science (London, United Kingdom: Elsevier B.V.), 447–485. doi:10.1016/B978-0-12-819570-3.00026-3
Burke, R. D., Angerer, L. M., Elphick, M. R., Humphrey, G. W., Yaguchi, S., Kiyama, T., et al. (2006). A genomic view of the sea urchin nervous system. Dev. Biol. 300, 434–460. doi:10.1016/j.ydbio.2006.08.007
Carrier, T. J., King, B. L., and Coffman, J. A. (2015). Gene expression changes associated with the developmental plasticity of sea urchin larvae in response to food availability. Biol. Bull. 228, 171–180. doi:10.1086/BBLv228n3p171
Costantini, M., Esposito, R., Ruocco, N., Caramiello, D., Cordella, A., Ventola, G. M., et al. (2024). De novo assembly of the genome of the sea urchin Paracentrotus lividus (lamarck 1816). Int. J. Mol. Sci. 25, 1685. doi:10.3390/ijms25031685
Davidson, E. H., Rast, J. P., Oliveri, P., Ransick, A., Calestani, C., Yuh, C. H., et al. (2002). A provisional regulatory gene network for specification of endomesoderm in the sea urchin embryo. Dev. Biol. 246, 162–190. doi:10.1006/dbio.2002.0635
Day, T. A., and Neale, P. J. (2002). Effects of UV-B radiation on terrestrial and aquatic primary producers. Annu. Rev. Ecol. Syst. 33, 371–396. doi:10.1146/annurev.ecolsys.33.010802.150434
Deidda, I., Russo, R., Bonaventura, R., Costa, C., Zito, F., and Lampiasi, N. (2021). Neurotoxicity in marine invertebrates: an update. Biol. (Basel). 10, 161–227. doi:10.3390/biology10020161
Della Torre, C., Bergami, E., Salvati, A., Faleri, C., Cirino, P., Dawson, K. A., et al. (2014). Accumulation and embryotoxicity of polystyrene nanoparticles at early stage of development of sea urchin embryos Paracentrotus lividus. Environ. Sci. Technol. 48, 12302–12311. doi:10.1021/es502569w
De Maio, A., Gabriella Santoro, M., Tanguay, R. M., and Hightower, L. E. (2012). Ferruccio Ritossa’s scientific legacy 50 years after his discovery of the heat shock response: a new view of biology, a new society, and a new journal. Cell Stress Chaperones 17, 139–143. doi:10.1007/s12192-012-0320-z
Devens, H. R., Davidson, P. L., Deaker, D. J., Smith, K. E., Wray, G. A., and Byrne, M. (2020). Ocean acidification induces distinct transcriptomic responses across life history stages of the sea urchin Heliocidaris erythrogramma. Mol. Ecol. 29, 4618–4636. doi:10.1111/mec.15664
Di Tuccio, V., De Luca, P., and Romano, G. (2023). Programmed cell death in sea urchins: a review. J. Mar. Sci. Eng. 11, 956. doi:10.3390/jmse11050956
Dorey, N., Martin, S., Oberhänsli, F., Teyssié, J. L., Jeffree, R., and Lacoue-Labarthe, T. (2018). Ocean acidification modulates the incorporation of radio-labeled heavy metals in the larvae of the Mediterranean sea urchin Paracentrotus lividus. J. Environ. Radioact. 191, 20–30. doi:10.1016/j.jenvrad.2018.04.017
Ernst, S. G. (2011). Offerings from an urchin. Dev. Biol. 358, 285–294. doi:10.1016/j.ydbio.2011.06.021
Foo, S. A., Koweek, D. A., Munari, M., Gambi, M. C., Byrne, M., and Caldeira, K. (2020). Responses of sea urchin larvae to field and laboratory acidification. Sci. Total Environ. 723, 138003. doi:10.1016/j.scitotenv.2020.138003
Fung, C. W., Chau, K. Y., Tong, D. C. S., Knox, C., Tam, S. S. T., Tan, S. Y., et al. (2023). Parentage influence on gene expression under acidification revealed through single-embryo sequencing. Mol. Ecol. 32, 6796–6808. doi:10.1111/mec.17148
Gambardella, C., Ferrando, S., Gatti, A. M., Cataldi, E., Ramoino, P., Aluigi, M. G., et al. (2016). Review: Morphofunctional and biochemical markers of stress in sea urchin life stages exposed to engineered nanoparticles. Environ. Toxicol. 31, 1552–1562. doi:10.1002/tox.22159
Gambardella, C., Marcellini, F., Falugi, C., Varrella, S., and Corinaldesi, C. (2021). Early-stage anomalies in the sea urchin (Paracentrotus lividus) as bioindicators of multiple stressors in the marine environment: overview and future perspectives. Environ. Pollut. 287, 117608. doi:10.1016/j.envpol.2021.117608
Goldstone, J. V., Hamdoun, A., Cole, B. J., Howard-Ashby, M., Nebert, D. W., Scally, M., et al. (2006). The chemical defensome: environmental sensing and response genes in the Strongylocentrotus purpuratus genome. Dev. Biol. 300, 366–384. doi:10.1016/j.ydbio.2006.08.066
González-Gaya, B. (2021). Invisible Pollution: Emerging marine pollutants. Mètode Sci. Stud. J. 11, 183–191. doi:10.7203/metode.11.16976
Hamdoun, A., and Epel, D. (2007). Changing world protection strategies in early development: a general strategy. Pnas 104, 1745–1750. doi:10.1073/pnas.0610108104
Hu, C., Yang, J., Qi, Z., Wu, H., Wang, B., Zou, F., et al. (2022). Heat shock proteins: biological functions, pathological roles, and therapeutic opportunities. MedComm 3, 1611–e239. doi:10.1002/mco2.161
Lamare, M. D., Liddy, M., and Uthicke, S. (2016). In situ developmental responses of tropical sea urchin larvae to ocean acidification conditions at naturally elevated pCO2 vent sites. Proc. R. Soc. B Biol. Sci. 283, 20161506. doi:10.1098/rspb.2016.1506
Landrigan, P., Stegeman, J., Fleming, L., Allemand, D., Backer, L., Brucker-davis, F., et al. (2020). Human health and ocean pollution. Ann. Glob. Health Ubiquity Press 86 (1), 151–164. doi:10.5334/aogh.2831
Layous, M., Khalaily, L., Gildor, T., and de-Leon, S. B. T. (2021). The tolerance to hypoxia is defined by a time-sensitive response of the gene regulatory network in sea urchin embryos. Dev 148, 195859. doi:10.1242/dev.195859
Lesser, M. P. (2010). Depth-dependent effects of ultraviolet radiation on survivorship, oxidative stress and DNA damage in sea urchin (Strongylocentrotus droebachiensis) embryos from the Gulf of Maine. Photochem. Photobiol. 86, 382–388. doi:10.1111/j.1751-1097.2009.00671.x
Li, N., Griffith, A. W., and Manahan, D. T. (2023). Integrative biological analyses of responses to food deprivation reveal resilience mechanisms in sea urchin larvae. Mol. Ecol. 33, 171200–e17217. doi:10.1111/mec.17120
Lister, K. N., Lamare, M. D., and Burritt, D. J. (2010). Oxidative damage in response to natural levels of UV-B radiation in larvae of the tropical sea urchin tripneustes gratilla. Photochem. Photobiol. 86, 1091–1098. doi:10.1111/j.1751-1097.2010.00779.x
Magesky, A., and Pelletier, É. (2018). Cytotoxicity and physiological effects of silver nanoparticles on marine invertebrates. Adv. Exp. Med. Biol. 1048, 285–309. doi:10.1007/978-3-319-72041-8_17
Manzo, S., and Schiavo, S. (2022). Physical and chemical threats posed by micro(nano)plastic to sea urchins. Sci. Total Environ. 808, 152105. doi:10.1016/j.scitotenv.2021.152105
Marlétaz, F., Couloux, A., Poulain, J., Labadie, K., Da Silva, C., Mangenot, S., et al. (2023). Analysis of the P. lividus sea urchin genome highlights contrasting trends of genomic and regulatory evolution in deuterostomes. Cell Genomics 3, 100295. doi:10.1016/j.xgen.2023.100295
Martino, C., Bonaventura, R., Byrne, M., Roccheri, M., and Matranga, V. (2017). Effects of exposure to gadolinium on the development of geographically and phylogenetically distant sea urchins species. Mar. Environ. Res. 128, 98–106. doi:10.1016/j.marenvres.2016.06.001
Matranga, V., Bonaventura, R., Costa, C., Karakostis, K., Pinsino, A., Russo, R., et al. (2011). Echinoderms as blueprints for biocalcification: regulation of skeletogenic genes and matrices. Prog. Mol. Subcell. Biol. 52, 225–248. doi:10.1007/978-3-642-21230-7_8
McClay, D. R., Martik, M. L., and Lyons, D. C. (2016). Developmental gene regulatory networks in sea urchins and what we can learn from them. F1000Research 5. doi:10.12688/f1000research.7381.1
Melzner, F., Mark, F. C., Seibel, B. A., and Tomanek, L. (2020). Ocean acidification and coastal marine invertebrates: tracking CO2 effects from seawater to the cell. Ann. Rev. Mar. Sci. 12, 499–523. doi:10.1146/annurev-marine-010419-010658
Morroni, L., Rakaj, A., Grosso, L., Flori, G., Fianchini, A., Pellegrini, D., et al. (2023). Echinoderm larvae as bioindicators for the assessment of marine pollution: sea urchin and sea cucumber responsiveness and future perspectives. Environ. Pollut. 335, 122285. doi:10.1016/j.envpol.2023.122285
Nayanathara Thathsarani Pilapitiya, P. G. C., and Ratnayake, A. S. (2024). The world of plastic waste: a review. Clean. Mater. 11, 100220. doi:10.1016/j.clema.2024.100220
Paganos, P., Ullmann, C. V., Gaglio, D., Bonanomi, M., Salmistraro, N., Arnone, M. I., et al. (2023). Plastic leachate-induced toxicity during sea urchin embryonic development: insights into the molecular pathways affected by PVC. Sci. Total Environ. 864, 160901. doi:10.1016/j.scitotenv.2022.160901
Paganos, P., Voronov, D., Musser, J., Arendt, D., and Arnone, M. I. (2021). Single cell rna sequencing of the strongylocentrotus purpuratus larva reveals the blueprint of major cell types and nervous system of a nonchordate deuterostome. Elife 10, e70416–e70429. doi:10.7554/eLife.70416
Pereira, L. C., de Souza, A. O., Bernardes, M. F. F., Pazin, M., Tasso, M. J., Pereira, P. H., et al. (2015). A perspective on the potential risks of emerging contaminants to human and environmental health. Environ. Sci. Pollut. Res. 22, 13800–13823. doi:10.1007/s11356-015-4896-6
Pusceddu, F. H., Guimarães, M. M., Lopes, L. O., Souza, L. S., Cortez, F. S., Pereira, C. D. S., et al. (2022). Biological effects of the antihypertensive losartan under different ocean acidification scenarios. Environ. Pollut. 292, 118329. doi:10.1016/j.envpol.2021.118329
Rendell-Bhatti, F., Paganos, P., Pouch, A., Mitchell, C., D’Aniello, S., Godley, B. J., et al. (2021). Developmental toxicity of plastic leachates on the sea urchin Paracentrotus lividus. Environ. Pollut. 269, 115744. doi:10.1016/j.envpol.2020.115744
Ritossa, F. (1962). A new puffing pattern induced by temperature shock and DNP in drosophila. Experientia 18, 571–573. doi:10.1007/bf02172188
Runcie, D. E., Dorey, N., Garfield, D. A., Stumpp, M., Dupont, S., and Wray, G. A. (2016). Genomic characterization of the evolutionary potential of the sea urchin Strongylocentrotus droebachiensis facing ocean acidification. Genome Biol. Evol. 8, 3672–3684. doi:10.1093/gbe/evw272
Russo, R., Bonaventura, R., Chiaramonte, M., Costa, C., Matranga, V., and Zito, F. (2018). Response to metals treatment of Fra1, a member of the AP-1 transcription factor family, in P. lividus sea urchin embryos. Mar. Environ. Res. 139, 99–112. doi:10.1016/j.marenvres.2018.05.003
Russo, R., Bonaventura, R., and Matranga, V. (2014). Time- and dose-dependent gene expression in sea urchin embryos exposed to UVB. Mar. Environ. Res. 93, 85–92. doi:10.1016/j.marenvres.2013.08.006
Russo, R., Zito, F., Costa, C., Bonaventura, R., and Matranga, V. (2010). Transcriptional increase and misexpression of 14-3-3 epsilon in sea urchin embryos exposed to UV-B. Cell Stress Chaperones 15, 993–1001. doi:10.1007/s12192-010-0210-1
Sodergren, E., Weinstock, G., Davidson, E., Al, E., Cameron, R. A., Gibbs, R. A., et al. (2006). The genome of the sea urchin Strongylocentrotus purpuratus. Science 314, 941–952. doi:10.1126/science.1133609
Solé, M., Kaifu, K., Mooney, T. A., Nedelec, S. L., Olivier, F., Radford, A. N., et al. (2023). Marine invertebrates and noise. Front. Mar. Sci. 10, 1–34. doi:10.3389/fmars.2023.1129057
Strader, M. E., Wong, J. M., and Hofmann, G. E. (2020). Ocean acidification promotes broad transcriptomic responses in marine metazoans: a literature survey. Front. Zool. 17, 7–23. doi:10.1186/s12983-020-0350-9
Stumpp, M., Dupont, S., Thorndyke, M. C., and Melzner, F. (2011). CO2 induced seawater acidification impacts sea urchin larval development II: gene expression patterns in pluteus larvae. Comp. Biochem. Physiol. - A Mol. Integr. Physiol. 160, 320–330. doi:10.1016/j.cbpa.2011.06.023
Tekman, M. B., Walther, B. A., Peter, C., Gutow, L., Bergmann, M., and Bauske, B. (2023). The effects of plastic pollution on marine ecosystems. Res. Mar. Sci. 8, 173–186.
Todgham, A. E., and Hofmann, G. E. (2009). Transcriptomic response of sea urchin larvae Strongylocentrotus purpuratus to CO2-driven seawater acidification. J. Exp. Biol. 212, 2579–2594. doi:10.1242/jeb.032540
Tomanek, L. (2010). Variation in the heat shock response and its implication for predicting the effect of global climate change on species’ biogeographical distribution ranges and metabolic costs. J. Exp. Biol. 213, 971–979. doi:10.1242/jeb.038034
Uthicke, S., Deshpande, N. P., Liddy, M., Patel, F., Lamare, M., and Wilkins, M. R. (2019). Little evidence of adaptation potential to ocean acidification in sea urchins living in “Future Ocean” conditions at a CO2 vent. Ecol. Evol. 9, 10004–10016. doi:10.1002/ece3.5563
Wessel, G. M., and McClay, D. R. (1985). Sequential expression of germ-layer specific molecules in the sea urchin embryo. Dev. Biol. 111, 451–463. doi:10.1016/0012-1606(85)90497-X
Keywords: echinoderm, invertebrates, emerging pollutants, stress response, transcripts, biomarkers, environmental change
Citation: Deidda I, Russo R, Lampiasi N, Zito F and Bonaventura R (2024) The sea urchin embryo and the cell stress responses: new perspectives. Front. Cell. Death 3:1422224. doi: 10.3389/fceld.2024.1422224
Received: 23 April 2024; Accepted: 19 June 2024;
Published: 23 July 2024.
Edited by:
Adrian Ting, Mayo Clinic, United StatesReviewed by:
Nikolay Popgeorgiev, Université de Lyon, FranceCopyright © 2024 Deidda, Russo, Lampiasi, Zito and Bonaventura. This is an open-access article distributed under the terms of the Creative Commons Attribution License (CC BY). The use, distribution or reproduction in other forums is permitted, provided the original author(s) and the copyright owner(s) are credited and that the original publication in this journal is cited, in accordance with accepted academic practice. No use, distribution or reproduction is permitted which does not comply with these terms.
*Correspondence: Irene Deidda, aXJlbmUuZGVpZGRhQGlyaWIuY25yLml0; Rosa Bonaventura, cm9zYS5ib25hdmVudHVyYUBpcmliLmNuci5pdA==
Disclaimer: All claims expressed in this article are solely those of the authors and do not necessarily represent those of their affiliated organizations, or those of the publisher, the editors and the reviewers. Any product that may be evaluated in this article or claim that may be made by its manufacturer is not guaranteed or endorsed by the publisher.
Research integrity at Frontiers
Learn more about the work of our research integrity team to safeguard the quality of each article we publish.