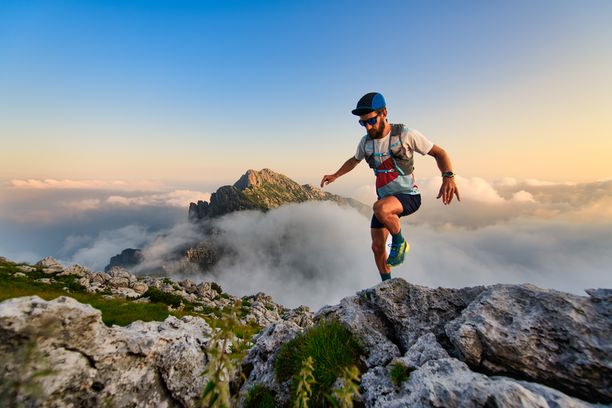
94% of researchers rate our articles as excellent or good
Learn more about the work of our research integrity team to safeguard the quality of each article we publish.
Find out more
ORIGINAL RESEARCH article
Front. Built Environ., 10 January 2025
Sec. Construction Materials
Volume 10 - 2024 | https://doi.org/10.3389/fbuil.2024.1504953
This article is part of the Research TopicAdvancements in Intelligent and Sustainable Construction Materials (ISCM): Experimental Investigation, Computational Analysis, and Real-World ApplicationView all 4 articles
In recent times, increasing demand for raw aggregate has emphasized the need for construction industry to adopt sustainable practices by exploring alternative materials, such as agro-waste, to address resource depletion and reduce environmental pollution., This current study investigated the suitability of combining discarded periwinkle shell (PWKS) and palm kernel shell (PMKS) at low-replacement volumes to partially substitute granite in ecofriendly load-bearing cement-based interlocking paver units for use on medium-duty traffic roads. 108 paver samples were produced, targeting a 28-day strength of 30 MPa, using a mix proportion of 1:1:2 (cement: sand: granite) and water-cement ratio of 0.50. Physical and chemical compositions of materials are examined, while the produced paver samples were tested for their water absorption, compressive and split-tensile strengths properties. The influence of curing (immersion and open air) on the strength development was evaluated. Findings showed that the shells recorded low physical properties but increased water intake tendency. A gradual decrease in the strength performances of the pavers was observed as the amount of PWKS and PMKS increases in the mixes. The water absorption tendency increases with increasing PMKS and PWKS levels. Pavers cured by complete immersion in water recorded good strength and achieved the 30 MPa compared to open air cured pavers. This study recommends load-bearing pavers of up to 30 MPa can be made by combining PMKS and PWKS at a low-replacement level of 5% deployed for medium-traffic roads, but with adequate curing technique. Outcomes showed the viability of incorporating periwinkle and palm kernel shells as aggregate in making standard paver units of adequate strength and resistance to water to promote sustainable construction practices.
In recent times, there are global concerns on the continuous depletion of the world’s natural resources due to the growing demand for natural aggregate (Mehta and Monteiro, 2006; Dang et al., 2021), owing to factors such as industrialization, urbanization, and population growth (Bilodeau and Malhotra, 2000; Wilson and Webster, 2017). In a study by Huang et al. (2020), it was estimated that the use of materials for construction has increased from 6.7 billion tons to about 17.5 billion tons within a space of 17 years (2000–2017). Concrete, whose main components are aggregates (sand and granite) and cement, is one of the most used material for construction, suggesting that increasing demand for concrete relatively means rising demand for cement and aggregates (Lv et al., 2022). Meanwhile, Prusty and Patro (Prusty and Patro, 2015) projected the yearly demand for concrete will increase to about 18 billion tons by 2050. This point to the possibility of a continuous rise in the demand for aggregates, which account for up to 60%–80% of the volume of concrete (Bamigboye et al., 2020; Egamana and Sule, 2017). The making of concrete has been reported to have a consequential influence on the environment because of its huge raw resources and energy demand during production, as well as environmental damages (Lv et al., 2022). For instance, cement production is reported to be responsible for the emission of about 30% carbon dioxide (CO2) into the atmosphere (Chen et al., 2021). Likewise, the increasing demand for concrete has resulted in the actual dwindling of sand (used as fine aggregate in concrete) in sufficient quantities (Amin et al., 2020). Moreover, Mo et al. (2016) pointed out that with the continuous rise in construction activities in many nations, especially in emerging countries, immoderate mining of natural resources will increase, resulting in more ecological imbalances, pollution and environmental losses. Such serious demand for aggregates and consequences are now drawing attention to the need to conserve natural resources. Recently, the focus of the global community is now on achieving sustainable development which is parts of the sustainable development goals (SDGs) (Calkins, 2009). The United States Environmental Protection Agency report (United States Environmental Protection Agency, 2023), as updated in 2022, highlighted that one of the common strategies that is widely accepted and is applied to all aspects of society for achieving sustainability is to reduce, reuse, and recycle (3R). The report further mentioned that the 3R can help achieve circularity as part of sustainable materials management in a circular economy. Studies by Singh et al. (2016); Scrivenera et al. (2018) opined that formulating policies that will enhance the use of alternative materials to substitute aggregate and cement by the building industry partially should be adopted to protect the environment, preserve the natural resources and avoid energy depletion.
Furthermore, another global issue of concern to the environment is the poor handling of solid waste from households and industries (Heriyanto et al., 2018). Sharba (2019) opined that effective solid waste management is one of the persevering subjects currently confronting several emerging countries. Pappua et al. (2007) projected an increase in the waste from households and industry to about 19 billion tonnes annually by 2025, while (Beede and Bloom, 1995), in their report, projected about 27 billion tons yearly by 2050. One such industry is the agricultural industry, where generated agricultural waste (also known as agro-waste) is often discriminately disposed into the environment, resulting in environmental pollution (Azunna, 2019). However, with more focus on effective waste management and resource conservation, the reuse and recycling of agro-waste can help the construction industry effectively contribute to and achieve the conservation of natural resources, energy savings, and reduction in the cost of building materials.
According to (Prusty and Patro, 2015), agricultural wastes in the form of aggregate in concrete and mortar composites can benefit society towards achieving a sustainable environment and circular economy. A study by Obi et al. (2016) referred to agricultural waste as the byproducts of handling agricultural products. Some examples of agro-waste include coconut shells, corn cob, palm kernel shells, pulp from fruits, stalks, date seeds, husks, bagasse, sawdust, rice husks, maize husks, and coconut shells and palm kernel shells. Due to their resilient nature when exposed to aggressive environmental conditions, these agro-waste materials can serve as an alternative replacement or admixture for some of these conventional natural construction materials (Adekunle et al., 2015). Moreover, using these materials, which in most cases are locally sourced materials as sustainable alternative substitutes for the various conventional components without compromising strength in collaboration with simple and low-cost technologies to produce durable but low-cost building components (Olofinnade and Adeyinka, 2024; Ogundipe et al., 2020). There are also agro-waste materials that are marine-based waste. Studies have shown that shell waste, such as oyster shells, scallop shells, cockle shells, periwinkle shells, and mussel shells can be used as alternative materials to substitute natural aggregates partially. It was reported that due to their hardness property, they can produce good quality concrete but with more cement content (Prusty and Patro, 2015), (Ogundipe et al., 2021).
Prominent among these agro-waste materials in Nigeria are both periwinkle shell (PWKS) and palm kernel shell (PMKS). According to (Peter and Ikechukwu, 2018), periwinkle, also known as Nodilittorina radiata, is a form of seafood prevalent in many coastal and riverine communities in Nigeria. The outer shells are discarded as waste once the edible parts are eaten. These shells possess a spiral conical, almost V-shape with a rough outer layer and are greenish-blue with a circle-shaped opening (Omisande and Onugba, 2020). The discarded hard shells constitute an apparent nuisance to the environment due to their foul odour and unpleasant sight in dump sites (Dahiru et al., 2018). Various studies have examined the possible use of PWKS as constituent material in concrete. A study by Ikponmwosa et al. (2019) reported that PWKS possess pozzolanic properties. The study also showed a notable decline in the mixes’ flow consistency and density of hardened concrete as the shell content increased. Agbede and Manasseh (2009) also reported a reduction in workability as the PWKS increases in the mixes. In a review work by Prusty and Patro (2015), it was stated that PWKS is a good material for substituting aggregate in concrete and can be used as lightweight aggregate, while (Adewuyi and Adegoke, 2008) opined that PWKS will be a suitable alternative aggregate to substitute gravel in normal strength concrete partially. A recent study by (Olofinnade et al., 2023) advocated using crushed PWKS for making non-load-bearing lightweight hollow sandcrete blocks. Likewise, (Peter and Ikechukwu, 2018), opined that both PWKS and PMKS materials possess most of the chemical constituents similar to standard cement, hence suggesting both materials can be combined or used individually with additives in cement production. The utilization of PWKS as an alternative construction material has the potential to reduce the over-dependent on conventional aggregate in the construction industry and also assist in eliminating the issues of indiscriminate disposal (Olofinnade et al., 2023; Otunyo et al., 2013). Studies have also shown the possibility of combining PWKS with other agro-wastes, like sawdust, palm kernel or coconut shells, in composites material production (Ogundipe et al., 2020). Odeyemi et al. (2020) demonstrated this by combining crushed PWKS with sawdust material in particle-board production.
Furthermore, PMKS from agricultural waste are another material that can be a suitable alternative aggregate to natural aggregate (Azunna, 2019). The hard-core shell (endocarp) component is left after the extraction of palm oil, fibres, and nuts from the palm kernel nut, also known as Elaeis guineensis. Palm oil is a major ingredient used in the making of many diverse products such as lipstick, bread, shampoo, detergent, chocolate, ice cream and biodiesel, and it was reported that the demand for palm oil is expected to grow by 2050 (McCarthy, 2020). The oil palm tree is mainly cultivated in countries with tropical climates, such as Malaysia, Thailand, Indonesia, Ghana, Brazil, Nigeria, Guatemala, etc. Reports indicated that Indonesia produced about 42.5 million tonnes (58% of global production), Malaysia had about 19 million tonnes (26% of the global output), while others, such as Thailand, Colombia and Nigeria, supplied less than three million tons annually (McCarthy, 2020). Nigeria is one of the world’s top consumers and producers of palm oil, hence, generating a steady vast amount of palm kernel shell waste (Ikubanni et al., 2020). These shell wastes are mostly heaped or stacked indiscriminately in dump sites, causing a nuisance to the environment. Sometimes, these shells are utilised to fill portholes in rural roads due to their hardness property or used as local fuel with firewood for cooking, thereby polluting the environment (Eziefula et al., 2018). Many research studies have extensively examined the use of PMKS materials for various applications, such as water purification, bio-fertilizer and biomass, supercapacitor electrode, reinforcing addictive in composites, energy storage and so on (Ikubanni et al., 2020; Eziefula et al., 2018; Osei, 2013; Obi, 2015; Ishola et al., 2017; Salawu et al., 2019), and also used in soil stabilization (Rahgozar et al., 2018). A review study by Prusty and Patro (2015) extensively reported on the beneficial use of the PMKS in concrete. Ikubanni et al. (2020) in their study noted that the mechanical qualities, shock and abrasion resilience of PMKS are exceptional compared to other agro-waste materials. Results showed that concrete mixes incorporating PMKS exhibited sufficient workability and density enough to achieve lightweight concrete (Olusola and Babafemi, 2013; Alengaram et al., 2013). The PMKS was reported to be suitable for partial substitution of natural coarse aggregate in concrete mixes with increasing strength as the curing age increases (Olanipekun et al., 2006; Osei and Jackson, 2012). In a recent study by Jamaludin et al. (2023), it was recommended on the potential utilization of crushed palm oil clinker at 10% replacement level to partially substitute fine aggregate in mortar. A study by Olusola and Babafemi (2013) suggested the use of PMKS for producing lightweight concrete at an optimum limit range of 25%–50% as a replacement for the coarse aggregate component. Recently, Ogundipe et al. (2021) demonstrated the possible combinination of PMKS and PWKS in making eco-friendly lightweight concrete for affordable housing.
The need for understanding the strength parameters of combining PMKS and PWKS for achieving sustainable load-bearing interlocking concrete paver units created a gap in its demand application in the construction industry. Nevertheless, most of the reported studies on the reuse of both the PMKS and PWKS wastes are mostly used as constituent materials in structural lightweight sandcrete and normal strength concrete production. Besides other reported applications, it is necessary to expand knowledge further on the possible reuse of these waste shells as an alternative to natural aggregate in making load-bearing interlocking concrete paver block units for possible use in light- and medium-duty roadways. This study investigated the strength parameters of combining discarded PWKS and PMKS at low replacement levels to partially to substitute conventional coarse aggregate in making eco-friendly load-bearing cement-based interlocking paver block units. The objectives are to investigate the physio-checmical properties of the shells, and determine their influence on the strength and water absorption tendency of the produced interlocking paver units. Interlocking paver units are sometimes preferred for paving roads due to their low maintenance cost, as well as their ability to be adapted to any environmental situation in rural areas (Olofinnade et al., 2023). The motivation of this study is investigate the suitability of utilizing PMKS and PWKS as alternative aggregate to traditional granite stone for raw resource conservation.
The finding is expected to assist construction industry professionals, government policymakers, and housing developers to overcome issues around depletion of natural resource, reduction in carbon dioxide (CO2) emission and environmental concern regarding concrete production by embracing the use PWKS and PMKS as substitute for natural coarse aggregate. This is because an increased demand for non-renewable raw aggregates suggests continuous depletion of natural resources, increase energy demand, and increased greenhouse gas emissions, causing a considerable impact on the environment and society. Nonetheless, this research examined the potential of deploying discarded PWKS and PMKS from agricultural waste as alternatives to natural aggregate in making interlocking concrete pavers. This helps to possibly conserve some significant amount of raw aggregate to achieve responsible consumption, in addition to creating sustainable infrastructure, as well as achieving eco-friendly structures from waste materials which aligns with sustainable cities and communities.
The materials utilized in this research for the production of the interlocking concrete paver blocks were;
i. Periwinkle shells (PWKS);
ii. Palm kernel shells (PMKS);
iii. Portland cement;
iv. Sand;
v. Granite; and Portable water
The sand and granite materials were obtained from quarry sites in Abeokuta, Ogun State. The maximum nominal size for the granite used as coarse aggregate in the mixes ranges between 10–13.2 mm. The PWKS and PMKS shown in Figures 1A, B were obtained as waste from agricultural waste collection point located in Badagry area, Lagos state. The shells were first properly washed with warm water to remove dirt and any surface impurities, then the shells were sufficiently openly air-dried in the sun before their utilization in the mixes. The particle size distribution of the sand, granite and shell materials was evaluated in line with BS 812 (1990). Physical parameters such as bulk density, water absorption, specific gravity, moisture content, aggregate impact value (AIV) and aggregate crushing value (ACV) of the sand, granite as well as that of the PWKS and PMKS materials were evaluated based on relevant standards. These tests are important to show the suitability of these PWKS and PMKS as alternative aggregates to conventional aggregates in concrete paver block production. The Portland cement used was of grade 42.5N, bought from a vendor outlet store located in Ota town, Ogun State. The cement conforms to type 1 Portland cement for general-purpose construction (BS EN 197-1, 2000). Portable water was used in this research for mixing. The oxide compounds in the cement, PMKS, and PWKS materials were determined using the x-ray fluorescence (XRF).
The batching proportioning of the various components for making the block samples was performed by volume in three (Bilodeau and Malhotra, 2000) batches adopting a mixing proportion of 1:1:2 (cement: sand: granite) at constant water-cement (w/c) of 0.50. A minimum targeted strength of 30 MPa was expected to be achieved by the produced paver samples after 28-day period of curing. The first batch was the control mix sample, and the second batch involved partially replacing the granite component separately with PWKS and PMKS at percentage replacement of 5% and 10% in the concrete mixes. The third batch involved combining both PMKS and PWKS materials in the same mixes and used to partially replace the granite component in the concrete mixes using the same percentage replacement levels of 5% and 10% at a constant w/c ratio of 0.5. Table 1 shows the adopted mix proportioning of the constituents in the production of the interlocking concrete paver block samples. Each constituent material was weighed, and the materials were carefully and evenly mixed to achieve uniform distribution. The mixing procedure was done manually, and a slump test was used to determine the mix’s workability. The recorded slump values were observed to be in the S3 class (100–150 mm) for a 0.50 w/c ratio, thus implying that the achieved mixes are sufficiently moist and fluid for making concrete paver blocks that can be deployed for structural uses.
An H-type shape mould was used in this research to make the interlocking paver block units, as shown in Figure 2. Each of the interlocking paver block units produced was 215 × 135 × 80 mm in dimensions. The 80 mm thickness follows the guidance of ASTM C936 (2002) on the minimum thickness for paver block units for possible deployment on street roads or light traffic. A total of 108 paver block samples were produced, and casting was carried out in three layers in a well-oiled plastic mould. Compaction of the concrete during casting was done manually with a tapping rod to compact each layer twenty-five times and a vibrating table to remove pore spaces within the concrete. Afterwards, these paver samples were demoulded after 24 h and curing of the samples was done using two different curing approaches that included; total immersion in water using a water curing tank and open-air curing at temperature ranges of 30°C–31°C. The hardened paver samples were cured for 7 and 28 days before testing.
Compressive strength is one of the critical properties of paver block to determine its capacity to withstand load. The test was carried out on the hardened interlocking concrete paver block samples according to BS 6717 (2001) after 7 and 28 days of curing to determine their axial load-carrying capacity. Three (Bilodeau and Malhotra, 2000) samples were tested at a constant loading rate of 2.4 MPa/min using the Yes-2000 kN capacity compression machine. The compressive strength was estimated using Equation 1:
The split tensile strength test was also carried out on the hardened interlocking concrete paver block samples according to BS 6717 (BS 6717, 2001) after 7 and 28 days of curing to determine their tensile strength capacity. Three (Bilodeau and Malhotra, 2000) samples were tested at a constant loading capacity range of 2.4 MPa/min using the Yes-2000 kN capacity machine. The split tensile strength was calculated using Equation 2:
where P is the measured applied load at failure in kN, S is the area of failure plane in m2 and k is the correction coefficient for paver thickness of 80 mm.
The water absorption rate of the paver samples containing PWKS and PMKS at 28 days was determined relative to the control samples by evaluating the reduction in the weight of wet paver samples and dry paver samples after oven drying the samples to a constant weight at the temperature of 105°C in accordance to ASTM C642-06 (2008). The water absorption was calculated with Equation 3:
where W1 = weight of dry paver sample, W2 = weight of wet paver sample.
Selected samples from the crushed paver samples were further analysed with the scanning electron microscope (SEM) using the Phenom ProX model. This was carried out to examine the surface microstructure of the tested hardened samples. A small fragment collected from the fractured selected paver samples was mounted on the SEM stub without any coating applied on the samples. The selected concrete fragments are from the crushed 28-day samples with good strength performance under the applied load to further view their surface morphology at the micro-scale.
Table 2 depicts the chemical compositions present in the PMKS and PWKS materials. The result showed no presence of toxic compounds in both materials, which is similar to the outcomes of Ogundipe et al. (2021). Both PMKS and PWKS materials contain a sufficient amount of silica (SiO2), while there is a relatively high amount of calcium oxide (CaO) content in the PWKS. The findings show that the shells material do not contain toxic compounds similar to the outcomes by Ogundipe et al. (2020); Adewuyi and Adegoke (2008). Table 3 show the results on the tested physical properties of the constituent materials. The results show that the specific gravity values recorded for both river sand and granite are within the defined standard of 2.5–3.0 required for aggregate (BS EN ISO 10545, 1997). However, the recorded values for the specific gravity values for PMKS and PWKS were less, indicating that both materials could be regarded as lightweight aggregate. The water absorption tendency simply shows that the PMKS material has a higher tendency to take in more water. Further, the recorded Los Angeles abrasion values and aggregate impact values showed that PWKS failed to meet wearing surface criteria compared to PMKS which shows some degree of resistance to impact and abrasion. The Los Angeles abrasion and aggregate impact values should not exceed 30% for aggregates used for wearing surfaces. Figure 3 shows the particle size distribution curve for natural sand, granite, PMKS and PWKS aggregate materials.
Figures 4A, B depict the influence of incorporating the PMKS and PWKS materials as partial substitutes for coarse aggregate on the compressive strength of the fabricated interlocking concrete pavers. A steady decreasing trend was observed in the compressive strength values of the paver blocks with the increase in the replacement levels of granite with PMKS and PWKS, and a combination of both PMKS and PWKS in the mixes compared to the control. For instance, the recorded outcomes for the 28-day average compressive strength for the control paver samples are 39.85 MPa and 27.70 MPa for immersion and open-air curing, respectively. For paver samples containing 5%PWKS as granite replacement, the recorded strength was 35.72 MPa and 25.07 MPa for curing by immersion and open-air, respectively, implying a 10% strength reduction. Further increase in the PWKS content to 10% (10%PWKS) as granite replacement also showed a 15% reduction in strength with compressive strength values of 34.02 MPa and 23.82 MPa for curing by immersion and open-air, respectively. Similarly, for paver samples containing 5%PMKS content, the recorded compressive strength values were 34.61 MPa and 24.23 MPa, which indicate a 13% strength reduction for curing by immersion and open-air respectively. However, about 26% strength reduction was achieved for paver block samples containing 10%PMKS. The obtained compressive strength values were 29.54 MPa and 20.68 MPa for immersion and open-air curing, respectively. For concrete paver samples produced from mixes containing the combination of both shell materials at varying percentages, a combination of 5%PMKS & 5%PWKS (31.43 MPa and 22.43 MPa), 5%PMKS & 10%PWKS (32.95 MPa and 23.68 MPa), 10%PMKS & 5%PWKS (30.12 MPa and 21.70 MPa), and 10%PMKS & 10%PWKS (28.32 MPa and 19.66 MPa) as coarse aggregate replacement, the achieved 28-day compressive strengths are for immersion and open-air curing are as indicated respectively. The percentage reduction in strength was 20%, 17%, 24% and 29% respectively. The decrease in the compressive strength becomes more pronounced as the replacement levels increase beyond 10%. This can be attributed to the physical properties of the PMKS and PWKS materials compared to granite materials. The shell material for instance, has a lesser weight compared to the granite, and its shape often resulted in a possible increase of pore spaces within the concrete matrix (Osei, 2013). Generally, there was a steady increase in the compressive strength of all samples to the curing age. However, the results depict the impact of the two curing methods (immersion and open-air) adopted on the strength development of the tested hardened samples. The open-air curing is simply openly exposing the paver samples without covering but with occasional wetting with water as the usual practice in most paver blocks production sites. The plots showed that hardened paver samples cured by immersion achieved the highest compressive strengths for the control and pavers containing PMKS and PWKS compared to the paver blocks cured in the open, indicating that water immersion is a more effective means of curing paver block samples. The outcomes also indicated that mixes containing 5%PWKS and 5%PMKS performed relatively better compared to others. These findings corroborate the reported findings of (Prusty and Patro, 2015; Azunna, 2019; Ogundipe et al., 2021; Adewuyi and Adegoke, 2008; Odeyemi et al., 2020) on how effective PWKS and PMKS materials are as possible alternative aggregate. As shown in Figure 4A, the pavers cured by water immersion were able to meet the minimum compressive strength of 30 MPa as recommended by BS 6717 (2001); IS 15658 (2006) for interlocking concrete pavers used for light load carriageway and non-traffic applications, car parking spaces and building premises, while ESS 4382 (2004) recommended for normal and medium duty loads. However, the obtained compressive strength fails to meet the 40–55 MPa for heavy traffic and duty applications (BS 6717, 2001; IS 15658, 2006). Samples cured in open air failed to meet any of these minimum strength requirements, but can be deployed for walkways (Figure 4B).
Figure 4. (A) Strength development for paver samples cured by immersion. (B) Strength development for paver samples cured by open air.
Figures 5A, B show the results of the tensile strength conducted on the paver blocks samples. The result indicates that utilization of PWKS, PMKS, and a combination of both shell materials as granite substitutes slightly decreases the tensile strength of the concrete pavers as the shells content increases in the mixes. This trend is like the findings seen for compressive strength. However, the tensile strength values for the mixes containing 5% PMKS and PWKS are relatively close compared to the value recorded for the control. The reduction in the tensile strength could be a result of a possible loss of bonding within the concrete that may have resulted in less resistance to splitting (Yerramala and Ramachandrudu, 2012). Moreover, the granite aggregate is denser than both PMKS and PWKS, which could have possibly caused an increase in void spaces in the mixes resulting in a decrease in the tensile strength performance (Azunna, 2019). The plots also depict the effect of curing methods on the tensile strength development of the paver samples. Again, it was noticed that curing by immersion achieved better tensile strength values after 28 days of curing compared to the open-air curing. The recorded outcomes for the 28-day average split tensile strength for the control samples were 2.68 MPa and 2.37 MPa for immersion and open-air curing respectively. For mixes containing only 5%PWKS as granite replacement, the 28-day tensile strength was 2.61 MPa for water immersion and 2.29 MPa for open-air curing, while at 10%PWKS, the results show the tensile strength values to be 2.53 MPa for water immersion and 2.09 MPa for open-air curing. For mixes containing only 5%PMKS as granite replacement, the 28-day split tensile strength was 2.53 MPa for water immersion and 2.28 MPa for open-air curing, while at 10%PMKS, the results were 2.50 MPa for water immersion and 2 MPa for open-air curing. Similar trends were noticed indicating curing with water immersion achieved better tensile strength performance compared to open-air curing for mixes containing the combination of both shell materials as granite replacement. However, it was noticed that the reduction in the tensile strength for some mixes containing the combination of both shell materials is minimal.
Figure 5. (A) Tensile strength for paver samples by immersion curing. (B) Tensile strength for paver samples by open-air curing.
Figure 6 shows the correlation regression linear models between the 28-day compressive strength and tensile strength magnitudes obtained for the concrete paver block samples relative to the curing methods used. The model establishes the best fit by adjusting the relationship between compressive strength and tensile strength variables. The coefficient of determination (R) of these models establishes that the dependent variable, compressive strength was predictable at R2 = 0.73 for samples cured by water immersion, while for samples cured by open air, the compressive strength was predictable at R2 = 0.80.
Water absorption is a relevant attribute that influences the long-term performance of concrete pavers. Low water absorption would imply good resistance to damages caused by water infiltration and environmental effects (Abid et al., 2022). Figure 7 depicts the results of the water absorption of the hardened paver block, showing a proportional increase in water absorption rate with an increase in PMKS and PWKS content. A similar increase was noticed for concrete pavers with combined PMKS and PWKS materials compared to the control. The interlocking concrete paver blocks cured by open air recorded the highest water absorption values compared to those cured by water immersion. The figure showed that pavers with combined shells (10%PMKS/10%PWKS) recorded the highest water absorption values of 6.19% (water immersion curing) and 7.7% (open air curing), compared to 5.26% (water immersion curing) and 6.5% (open air curing) for the control. The findings agree with the recent work of (Olofinnade et al., 2023) on increasing water absorption of composite containing PMKS and PWKS, respectively at increasing content. This was attributed to the high-water absorption capacity of the shells and the possible presence of more numbers of pores in the hardened paver samples, especially those cured in the open air. Similarly, ASTM C936 (2002) and IS 15658 (2006) standards recommended that the water absorption limit for concrete paving units should not be more than 5% and 6% respectively, while (BS 6717, 2001) suggested 7%. However the outcomes showed that all concrete paver units containing PMKS and PWKS cured in open air exceeded the recommended limits, whereas those cured by complete water immersion fell within the 5%–7% recommended for interlocking paving units. The relationship between 28-day compressive strength and water absorption values at 28 days for water immersion and open-air curing is shown in Figure 8. The plot depicts low compression strength values to high water absorption recorded for open-air cured samples. The compressive strength of paver samples cured by water immersion exhibited a more moderate water absorption capacity. The coefficient of determination (R2) of the models showed that the compressive strength was predictable at R2 = 0.9323 for samples cured by water immersion, while for samples cured by open air, the compressive strength was predictable at R2 = 0.7642. It can be said that samples cured by water immersion performed better than openly cured samples.
Selected concrete fragments from the crushed 28-day samples cured by water immersion were further analysed using the scanning electron microscope (SEM) to view the surface morphology at the micro-scale. The selection was limited to control mix samples and mixes containing 5%PWKS and combined 5% PMKS/10%PWKS (these are mixes that recorded maximum strength performance under applied load). Figures 9A–C show the outcome of the SEM analysis. The SEM micrograph for the control mix (Figure 9A) revealed a dense packing interface with the presence of few micro-pores, thus implying a well-compacted concrete mix with somehow good bonding between the aggregates and cement matrix. This explained the high strength exhibited by the control mix. Additionally, Portlandite (Ca(OH)2) crystals were noticed to be arbitrarily dispersed through the control sample. Figure 9B depicts the SEM image for the mix sample containing 5%PWKS. The SEM revealed the presence of excess micro-pores, with possible weak interfaces due to the existence of pores within the concrete internal structure. The SEM image suggests that the presence of possibly fragmented PWKS in the mixes may have resulted in poor development of the concrete internal structure pores and increased water intake tendency, causing decreased strength. Moreover, the SEM image for concrete mix with the combined shell material (5%PMKS/10%PWKS), as shown in Figure 9C also displayed a similar presence of excess tiny micro-pores on the concrete interface, coupled with the revealing of weak bond and micro-cracks at the interfacial zone.
In this study, the suitable utilization of discarded PMKS and PWKS as alternative aggregates to granite in the making of eco-friendly interlocking paving block units for load-bearing applications was experimentally investigated. The study limits its scope to evaluating the shell’s properties, and their influences on the strength performance and water absorption capacity of the manufactured interlocking paving blocks cured under two separate curing conditions. The following are the conclusions deduced;
i. The results depicted that the PMKS and PWKS has lower physical properties but a high water absorption tendency compared to the granite aggregate, thus the materials can be classified as lightweight aggregate. The outcomes of the physical properties and chemical compositions of the shells therefore indicated the suitability of using these shells as good alternative construction materials to partially substitute coarse aggregate to achieve lightweight interlocking pavers of near comparable strength to regular interlocking concrete pavers.
ii. A steady decline in the strength of the paver samples was noticed as the replacement volume of PMKS, and PWKS increased in the mixes. However, the study was able to show that both the PMKS and PWKS can be good usable materials for partial replacement of natural coarse aggregate in interlocking paving blocks.
iii. The obtained results on the strength development indicated that curing of the concrete paver blocks by complete immersion in water appeared to be the preferred method for curing than open air curing. The recorded values indicated that hardened paver samples cured by water immersion performed better in terms of strength and water absorption than those cured by the open air method. The mixes containing PWKS and PMKS at an optimum percentage level of 5% and combined percentage of 5% PMKS and 10% PWKS were observed to achieve good compressive strength and tensile strength values nearly comparable to the control.
iv. The water intake of the pavers increases with increasing amounts of PMKS, PWKS and a combination of both in the mixes. The control pavers cured by water immersion recorded the lowest water absorption value. Pavers containing low replacement levels of shells cured by water immersion also recorded low water absorption values compared to those cured by open air. Findings showed that all the pavers containing PMKS and PWKS cured in open air exceeded the recommended water absorption limits, but those cured by complete water immersion fell within the 5%–7% limits recommended for interlocking paving blocks.
v. The SEM images revealed excess micro-pores and weak bonds between the shells and mortar matrix at the interfacial zone compared to a more compacted interface exhibited by the control. Hence, suggesting a continuous increase in the shell content in the concrete mixes will relatively result in continuous loss of strength.
The models show that the amount of PMKS and PWKS and curing by water immersion had a significant effect on the response, which is the strength and water absorption of the paver samples. The models indicated that the target strength of 30 MPa can be achieved with a low replacement level of 5% and ensure adequate curing of the produce pavers. However, it should be mentioned that the appreciable performance of concrete mix with 10% volume replacement was noted.
The results showed that the interlocking paver blocks of sufficient thickness, strength and resistance to water that meets specified standard requirements can be made by incorporating periwinkle and palm kernel shells as aggregate. These types of pavers can be deployed for roads carrying normal medium-duty loads such as light load carriageways, light traffic and non-traffic applications (walkways), spaces for car parking and gardening walkway and building premises. This study further demonstrated one of the major roles the construction industry can play in achieving raw resource conservation and a sustainable built environment, which can engender a circular economy. The study depicts the suitability of adopting PMKS and PWKS as alternative materials to granite at a suggested low percentage of 5% replacement to produce cement-based interlocking concrete paving units.
In the future, it is necessary to research on evaluating the environmental impact of using these shells material in paver blocks production through the lifecycle assessment studies (LCA) to have more insights into the potential offered by these materials. In addition, other areas for future works should be to further ascertain the economic viability of using these materials, possible standardization, scalability and industrial applications.
The original contributions presented in the study are included in the article, further inquiries can be directed to the corresponding authors.
OO: Conceptualization, Formal Analysis, Investigation, Supervision, Visualization, Writing–original draft, Writing–review and editing. PA: Supervision, Writing–review and editing. KE: Investigation, Methodology, Writing–original draft. KO: Methodology, Writing–review and editing.
The author(s) declare that no financial support was received for the research, authorship, and/or publication of this article.
The authors appreciate the Structures and Materials Laboratory of the department of Civil Engineering, Covenant University, Ota, Nigeria.
The authors declare that the research was conducted in the absence of any commercial or financial relationships that could be construed as a potential conflict of interest.
The author(s) declared that they were an editorial board member of Frontiers, at the time of submission. This had no impact on the peer review process and the final decision.
The authors declare that no Generative AI was used in the creation of this manuscript.
All claims expressed in this article are solely those of the authors and do not necessarily represent those of their affiliated organizations, or those of the publisher, the editors and the reviewers. Any product that may be evaluated in this article, or claim that may be made by its manufacturer, is not guaranteed or endorsed by the publisher.
Abid, R., Kamoun, N., Jamoussi, F., and El Feki, H. (2022). Fabrication and properties of compressed earth brick from local Tunisian raw materials. Bol. la Soc. Española Cerámica Vidr. 61 (5), 397–407. doi:10.1016/j.bsecv.2021.02.001
Adekunle, A. J., Adedeji, O. E., and Foyeke, O. T. (2015). The untapped potential of agro-industrial wastes in developing country: a case study of Nigeria. Am. Res. J. Agric. 1 (4), 1–13.
Adewuyi, A. P., and Adegoke, T. (2008). Exploratory study of periwinkle shells as coarse aggregates in concrete works. J. Eng. Appl. Sci. 3 (6), 1–5.
Agbede, O. I., and Manasseh, J. (2009). Suitability of periwinkle shell as partial replacement for river gravel in concrete. Leonard Electron. J. Pract. Technol. 15, 59–66.
Alengaram, U. J., Muhit, B. A. A., and Jumaat, M. Z. B. (2013). Utilization of oil palm kernel shell as lightweight aggregate in concrete – a review. Constr. Build. Mater. 38, 161–172. doi:10.1016/j.conbuildmat.2012.08.026
Amin, M., Tayeh, B. A., and Agwa, I. S. (2020). Effect of using mineral admixtures and ceramic wastes as coarse aggregates on properties of ultra-high-performance concrete. J. Clean. Prod. 273, 123073. doi:10.1016/j.jclepro.2020.123073
Astm C127-04 - standard test method for density, relative density (specific gravity), and absorption of coarse aggregate. American Society for Testing and Materials (ASTM) International, West Conshohocken, PA, (2023).
ASTM C 131 (2020) “ASTM C 131 – standard test method for resistance to degradation of small-size coarse,” in Aggregate by abrasion and impact in the Los Angeles machine. West Conshohocken, PA: American Society for Testing and Materials (ASTM) International.
ASTM C29/C29M (2017). Standard test method for bulk density (unit weight) and voids in aggregate. West Conshohocken, PA: American Society for Testing and Materials (ASTM) International.
ASTM C642-06 (2008). Standard test method for density, absorption, and voids in hardened concrete. West Conshohocken, PA: American Society for Testing and Materials (ASTM) International, 11–13.
ASTM C936 (2002). Standard specification for solid concrete interlocking paving units. West Conshohocken, USA.
Azunna, S. U. (2019). Compressive strength of concrete with palm kernel shell as a partial replacement for coarse aggregate. SN Appl. Sci. 1, 342. doi:10.1007/s42452-019-0334-6
Bamigboye, G. O., Okara, O., Bassey, D. E., Jolayemi, K. J., and Ajimalofin, D. (2020). The use of Senilia senilis seashells as a substitute for coarse aggregate in eco-friendly concrete. J. Build. Eng. 32, 101811. doi:10.1016/j.jobe.2020.101811
Beede, D. N., and Bloom, D. E. (1995). The economics of municipal solid waste. World Bank Res. Observer 10 (2), 113–150. doi:10.1093/wbro/10.2.113
Bilodeau, A., and Malhotra, V. M. (2000). High volume fly ash system: concrete solution for sustainable development. Am. Concr. Inst. Mater. J. 1, 41–47. doi:10.14359/804
BS 6717 (2001). “Precast, unreinforced concrete paving blocks,” in Requirement for and test methods. London: BIS.
BS 812 (1990). Methods for sampling and testing of mineral aggregates, sands and fillers. London: British Standard Institution.
BS EN 197-1 (2000). Composition, specification and conformity criteria for common cement. London: British Standard Institute.
BS EN ISO 10545 (1997). Determination of water absorption, apparent porosity, apparent relative density and bulk-density. London: British Standard Institution.
Calkins, M. (2009). Materials for sustainable sites: a complete guide to the evaluation, selection, and use of sustainable construction materials. Hoboken, NJ: Wiley.
Chen, J. J., Li, B. H., Ng, P. L., and Kwan, A. K. H. (2021). Adding granite polishing waste to reduce sand and cement contents and improve performance of mortar. J. Clean. Prod. 279, 123653. doi:10.1016/j.jclepro.2020.123653
Dahiru, D., Yusuf, U. S., and Paul, N. J. (2018). Characteristics of concrete produced with periwinkle and palm kernel shells as aggregates. FUTY J. Environ. 12 (1), 42–61.
Dang, V. Q., Ogawa, Y., Bui, P. T., and Kawai, K. (2021). Effects of chloride ions on the durability and mechanical properties of sea sand concrete incorporating supplementary cementitious materials under an accelerated carbonation condition. Constr. Build. Mater. 274, 122016. doi:10.1016/j.conbuildmat.2020.122016
Egamana, S., and Sule, S. (2017). Optimization of compressive strength of periwinkle shell aggregate concrete. Niger. J. Technol. 36 (1), 32–38. doi:10.4314/njt.v36i1.5
Eziefula, U. G., Opara, E. H., and Anya, U. C. (2018). Mechanical properties of palm kernel shell concrete in comparison with periwinkle shell concrete. Malays. J. Civ. Eng. 29 (1), 1–14. doi:10.11113/mjce.v29.15585
Heriyanto, , Pahlevani, F., and Sahajwalla, V. (2018). From waste glass to building materials – an innovative solution for waste glass. J. Clean. Prod. 191, 192–206. doi:10.1016/j.jclepro.2018.04.214
Huang, B., Gao, X., Xu, X., Geng, Y., Fishman, T., Kua, H., et al. (2020). A life cycle thinking framework to mitigate the environmental impact of building materials. One Earth 3 (5), 564–573. doi:10.1016/j.oneear.2020.10.010
Ikponmwosa, E. E., Ehikhuenmen, S. O., and Irene, K. K. (2019). Comparative study and empirical modelling of pulverized coconut shell, periwinkle shell, palm kernel shell as a pozzolan in concrete. Acta Polytech. 59 (6), 560–572. doi:10.14311/AP.2019.59.0560
Ikubanni, P. P., Oki, M., Adeleke, A. A., Adediran, A. A., and Adesina, O. S. (2020). Influence of temperature on the chemical compositions and microstructural changes of ash formed from palm kernel shell. Results Eng. 8, 100173. doi:10.1016/j.rineng.2020.100173
IS 15658 (2006). Precast concrete blocks for paving-specification. New Delhi: Bureau of Indian Standards.
Ishola, M., Oladimeji, O., and Paul, K. (2017). Development of ecofriendly automobile brake pad using different grade sizes of palm kernel shell powder. Curr. J. Appl. Sci. Technol. 23, 1–14. doi:10.9734/cjast/2017/35766
Jamaludin, N. F. A., Muthusamy, K., Mohd Nasir, M. S., and Hilal, N. N. (2023). Enhancing mortar properties with crushed palm oil clinker as a partial fine aggregate replacement. Construction 3 (2), 293–299. doi:10.15282/construction.v3i2.9933
Lv, Z., Jiang, A., and Liang, B. (2022). Development of eco-efficiency concrete containing diatomite and iron ore tailings: mechanical properties and strength prediction using deep learning. Constr. Build. Material 327, 126930. doi:10.1016/j.conbuildmat.2022.126930
McCarthy, N. (2020). Which countries produce the most palm oil? Forbes Bus. Available at: https://www.forbes.com/sites/niallmccarthy/2020/10/02/which-countries-produce-the-most-palm-oil-infographic/?sh=420fbf9f1e42 April 26, 2023).
Mehta, K. P., and Monteiro, P. J. M. (2006). Concrete: microstructure, properties, and materials. New York: McGraw-Hill.
Mo, K. H., Alengaram, U. J., Jumaat, M. Z., Yap, S. P., and Lee, S. C. (2016). Green concrete partially comprised of farming waste residues: a review. J. Clean. Prod. 117, 122–138. doi:10.1016/j.jclepro.2016.01.022
Obi, F. O., Ugwuishiwu, O. B., and Nwakarire, N. J. (2016). Agricultural waste concept, generation, utilization and management. Niger. J. Technol. 35 (4), 957–964. doi:10.4314/njt.v35i4.34
Obi, O. F. (2015). Evaluation of the physical properties of composite briquette of sawdust and palm kernel shell. Biomass Convers. Biorefinery 5, 271–277. doi:10.1007/s13399-014-0141-7
Odeyemi, S. O., Abdulwahab, R., Adeniyi, A. G., and Atoyebi, O. D. (2020). Physical and mechanical properties of cement-bonded particle board produced from African balsam tree (Populous Balsamifera) and periwinkle shell residues. Results Eng. 3, 1–12. doi:10.1016/j.rineng.2020.100126
Ogundipe, K. E., Ajao, A. M., Ogunbayo, B. F., and Adeyi, A. S. (2020). “Impact of partial replacement of granite with periwinkle and palm kernel shells on concrete strength,” in In. Proceedings of international structural engineering and construction; holistic overview of structural design and construction. Limassol, Cyprus.
Ogundipe, K. E., Ogunbayo, B. F., Olofinnade, O. M., Amusan, L. M., and Aigbavboa, C. O. (2021). Affordable housing issue: experimental investigation on properties of eco-friendly lightweight concrete produced from incorporating periwinkle and palm kernel shells. Results Eng. 9, 100193. doi:10.1016/j.rineng.2020.100193
Olanipekun, E. A., Olusola, K. O., and Ata, O. (2006). A comparative study of concrete properties using coconut shell and palm kernel shell as coarse aggregates. Build. Environ. 41 (3), 297–301. doi:10.1016/j.buildenv.2005.01.029
Olofinnade, O., and Adeyinka, O. (2024). The utilization of pulverized waste tire rubber in a soil–cement composite for sustainable compressed earth brick production. Discov. Civ. Eng. 1, 69. doi:10.1007/s44290-024-00075-x
Olofinnade, O. M., Anwulidiunor, J. U., Ogundipe, K. E., and Ajimalofin, D. A. (2023). Recycling of periwinkle shell waste as partial substitute for sand and stone dust in lightweight hollow sandcrete blocks towards environmental sustainability. Materials 16, 1853. doi:10.3390/ma16051853
Olusola, K. O., and Babafemi, A. J. (2013). Effect of Coarse Aggregate sizes and Replacement levels on the strength of palm kernel shell (PKS) Concrete. Civ. Eng. Dimens. 15 (1), 43–50. doi:10.9744/ced.15.1.43-50
Omisande, A. L., and Onugba, A. M. (2020). Sustainable concrete using periwinkle shell as coarse aggregate – a review. IOSR J. Mech. Civ. Eng. 17 (6), 17–22. doi:10.9790/1684-1706031722
Osei, D. Y. (2013). Pozzolana and palm kernel shells replace Portland cement and crushed granite in concrete. Int. J. Eng. Invent. 2, 1–5.
Osei, D. Y., and Jackson, E. N. (2012). Experimental study on palm kernel shells as coarse aggregates in concrete. Int. J. Sci. Eng. Res. 3 (8), 1–6.
Otunyo, A. W., Friday, I. U., and Israel, T. A. (2013). Exploratory study of crushed periwinkle shell as partial replacement for fine aggregates in concrete. J. Emerg. Trends Eng. Appl. Sci. 4 (6), 823–827.
Pappua, A., Saxenaa, M., and Asolekar, S. R. (2007). Solid wastes generation in India and their recycling potential in building materials. Build. Environ. 42 (6), 2311–2320. doi:10.1016/j.buildenv.2006.04.015
Peter, U. C., and Ikechukwu, O. G. (2018). Production of cement from mixture of palm kernel and periwinkle shell. Indian J. Eng. 15, 166–179.
Prusty, J. K., and Patro, S. K. (2015). Properties of fresh and hardened concrete using agro-waste as partial replacement of coarse aggregate – a review. Constr. Build. Mater. 82, 101–113. doi:10.1016/j.conbuildmat.2015.02.063
Rahgozar, M. A., Saberian, M., and Li, J. (2018). Soil stabilization with non-conventional ecofriendly agricultural waste materials: an experimental study. Transp. Geotech. 14, 52–60. doi:10.1016/j.trgeo.2017.09.004
Salawu, E. Y., Ajayi, O. O., Inegbenebor, A., Akinlabi, S., and Akinlabi, E. (2019). Influence of pulverized palm kernel and eggshell additives on the hardness, coefficient of friction and grey cast iron material microstructure for advanced applications. Results Eng. 3 (100025), 1–3. doi:10.1016/j.rineng.2019.100025
Scrivenera, K. L., John, V. M., and Gartner, E. M. (2018). Eco-efficient cements: potential economically viable solutions for a low-CO2 cement-based materials industry. Cem. Concr. Res. 114, 2–26. doi:10.1016/j.cemconres.2018.03.015
Sharba, A. A. (2019). The efficiency of steel slag and recycled concrete aggregate on the strength properties of concrete. KSCE J. Civ. Eng. 23 (11), 4846–4851. doi:10.1007/s12205-019-0700-3
Singh, S., Khan, S., Khandelwal, R., Chugh, A., and Nagar, R. (2016). Performance of sustainable concrete containing granite cutting waste. J. Clean. Prod. 119, 86–98. doi:10.1016/j.jclepro.2016.02.008
United States Environmental Protection Agency (2023). Reduce, reuse, recycle, United States environmental protection agency. Available at: https://www.epa.gov/recycle (Accessed April 25, 2023).
Wilson, D. C., and Webster, M. (2017). Building capacity for community waste management in low-and-middle income countries. Waste Manag. & Res. J. a Sustain. Circular Econ. 36, 1–2. doi:10.1177/0734242x17748535
Keywords: interlocking concrete paver, agro-wastes management, compressive strength, sustainable built environment, resources conservation
Citation: Olofinnade O, Awoyera P, Edemirukewa K and Ogundipe K (2025) Utilization of periwinkle and palm kernel shells in load-bearing ecofriendly interlocking concrete paver. Front. Built Environ. 10:1504953. doi: 10.3389/fbuil.2024.1504953
Received: 01 October 2024; Accepted: 12 December 2024;
Published: 10 January 2025.
Edited by:
Zengping Zhang, Chang’an University, ChinaReviewed by:
Mohammad K. Najjar, Federal University of Rio de Janeiro, BrazilCopyright © 2025 Olofinnade, Awoyera, Edemirukewa and Ogundipe. This is an open-access article distributed under the terms of the Creative Commons Attribution License (CC BY). The use, distribution or reproduction in other forums is permitted, provided the original author(s) and the copyright owner(s) are credited and that the original publication in this journal is cited, in accordance with accepted academic practice. No use, distribution or reproduction is permitted which does not comply with these terms.
*Correspondence: Oluwarotimi Olofinnade, cm90aW1pLm9sb2Zpbm5hZGVAY292ZW5hbnR1bml2ZXJzaXR5LmVkdS5uZw==; Paul Awoyera, YXdvcGF1bDIwMDJAZ21haWwuY29t
Disclaimer: All claims expressed in this article are solely those of the authors and do not necessarily represent those of their affiliated organizations, or those of the publisher, the editors and the reviewers. Any product that may be evaluated in this article or claim that may be made by its manufacturer is not guaranteed or endorsed by the publisher.
Research integrity at Frontiers
Learn more about the work of our research integrity team to safeguard the quality of each article we publish.