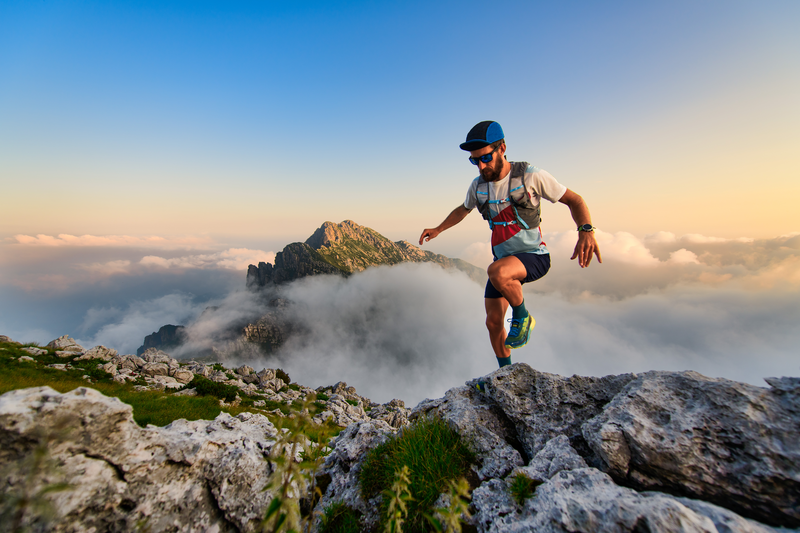
94% of researchers rate our articles as excellent or good
Learn more about the work of our research integrity team to safeguard the quality of each article we publish.
Find out more
ORIGINAL RESEARCH article
Front. Built Environ. , 25 October 2024
Sec. Indoor Environment
Volume 10 - 2024 | https://doi.org/10.3389/fbuil.2024.1494237
This article is part of the Research Topic Indoor Air Quality: Monitoring, Pollutants, and Remedial Strategies View all 6 articles
Introduction: Buildings that constitute cultural heritage and that are the identity of a defined geographical area are increasingly being restored to offer the community historical places to enjoy. Often the restoration preserves the original structure and building materials, which are usually natural stones. In this study, a radioprotection protocol dedicated to this kind of built environment was proposed and validated.
Methods: After identifying the two predominant types of building material stones (Rosso ammonitico and Pietra Serena), radiometric measurements for natural gamma-emitting radionuclides (Ra-226, Th-232, and K-40) and measurements of the emanation coefficient and calculation of the exhalation rate of radon gas were carried out.
Results: The two types of stone have a content of natural radionuclides that do not exceed the levels recommended by the regulations. The difference between the two types of stone is of an order of magnitude indicating that the red ammonite has a greater radiological impact than the pietra serena.
Discussion: The results, in addition to ensuring the radioprotection of the population, highlighted the need to increase the number of this kind of investigations to implement scientific knowledge and serve the stakeholders involved.
Indoor air quality management is constantly updated given the growing interest in protecting the health of humans who spend their time in confined environments. Among the sources of pollutants are building materials (BMs), among which, those of natural origin involve exposure to ionizing radiation (European Commission, 1999; IAEA, 2023). Radionuclides in BMs are sources of exposure to both external and internal radiation. External gamma radiation is caused by natural radionuclides, such as uranium (U-238), thorium (Th-234) and their decay products, and potassium (K-40). Internal exposure, on the other hand, is caused by the short-lived decay products of radon gas (Rn-222) that are exhaled from BMs into the indoor air (UNSCEAR, 2000; BEIR, 2006; ICRP, 2007; Kuluöztürk, 2019). Rn-222 is classified by WHO as second cause of lung cancer (WHO, 2009), and by IARC as a carcinogenic agent to humans (Group 1) (IARC, 1988). For this reason, it is important to carry out radiometric characterization ensuring the optimization and effectiveness of radiation protection. Even more so if we consider the constant recovery of historic buildings intended to accommodate a large number of people for occasional events or for events that require assiduous and long-lasting attendance over time.
To date, international references and legislation (European Union, 2013) and national Italian implementation (Repubblica Italiana, 2020) concern the classification of BMs in the first phase of production and sale, in order to obtain radiological suitability and fall within the standards of European regulation n. 305/2011 (European Union, 2011), which establishes harmonized conditions for the marketing of construction products. However, none of the aforementioned documents reports indications and is related to existing situations and even less in cases where ancient buildings constructed with natural stones are restored.
The only radiation protection requirement relates to the monitoring of indoor radon gas activity concentration for workers and representative persons. In addition, most regulations provide for a National Radon Action Plan (NRAP) (Repubblica Italiana, 2024), a management tool developed around three particular strategic axes: 1) measuring and identifying the priority areas; 2) acting through the implementation of remedial actions in buildings with activity concentration values higher than the reference level; 3) involving, educating and informing the population about radon and its risks.
In this work, a part of the NRAP has been considered, adapting it to a peculiar context, such as an ancient Tuscan furnace restored and with a change of intended use as a cultural space, to validate a methodological approach that can contribute to implement the work of the stakeholders involved (Ambrosino et al., 2024). After an analysis of the territory on a geological and lithological basis (Action 1.2), the identification and radiological characterization of the BMs has been performed, also considering the radon exhalation rate (Action 2.3).
The study and measurement site was the Municipality of Impruneta, (coordinates 43°41′07.53″N 11°15′15.82″E) south of Florence, in Tuscany, Central Italy. The entire area is known to have clay deposits that have been exploited by numerous furnaces, buildings intended for the firing of clay artifacts.
From a geological point of view (Figure 1) the Impruneta area and in particular the site where the furnace is located is straddled between a chaotic complex of entirely disorganized masses and exotic olistoliths in a clay matrix, and a complex made up of nappa debris.
Figure 1. Extract of geological map of Impruneta, detail of the location area of the furnace (Courtesy of Public Works Service, Municipality of Impruneta).
From a geological hazard point of view, however, the site is in a medium-high risk zone with the addition of landslide hazard (Figure 2) This cosideration is relevant given the close correlation with the nature of the soil, its structure and the exhalation of radon gas into the atmosphere, with a potential risk of accumulation in indoor environments. (Sabbarese et al., 2020; Benà et al., 2022; Ahmed et al., 2020).
Figure 2. Extract of geological hazard map of Impruneta, detail of the location area of the furnace (Courtesy of Public Works Service, Municipality of Impruneta).
The investigated furnace has a historical and economic significance for the entire area, and has recently been renovated to be used as a multipurpose space open to the public. The building, dating back to the end of the 17th century, is located on a hill and is divided into three levels: ground floor, first and second floor (Figure 3). Its position in relation to the floor level is peculiar: in fact, on one side the ground floor emerges outside, on the opposite side it is buried up to the first floor. The walls of the building are made of terracotta bricks and natural stones such as ammonites and sandstones. The roof of the building has a wooden structure, a terracotta brick floor and a roof made of tiles and pantiles (Figure 4)
Figure 3. Design of the furnace structure and distribution of the floors (Photo courtesy of Public Works Service, Municipality of Impruneta).
Figure 4. Internal structure of the furnace with the building materials used (Photo courtesy of Public Works Service, Municipality of Impruneta).
A dutiful mention is made of the BMs, a mix of terracotta bricks and a prevalence of natural Tuscan stones and widely spread throughout the region (Fratini and Rescic, 2014): Rosso Ammonitico (a marine limestone), and Pietra Serena (a quartz-feldspar sandstone), already previously characterized from a chemical-physical point of view in La Verde et al. (2022). Being a place of historical and cultural interest, it is not possible to apply standard sampling procedures, but the stones were sampled in the locations indicated by the superintendence office, which deals specifically with the protection of the archaeological, architectural, artistic and landscape heritage of the city, in a number that we considered to be representative based on the abundance of each type of stone.
Five samples for each of the two types of rocks were collected from the furnace site. After collection, the samples were prepared according to the UNI EN ISO 18589–2:2015 standard (ISO, 18589-2:2015, 2015).
The stones were reduced to fine powder using a PM 100 Retsch grinder, and then sieved. The resulting powder was dried in the DIGITRONIC Selecta 2005141 oven at 105°C for 2 hours. Finally, the sample was weighed and hermetically sealed in a Marinelli Becker for 30 days to allow the secular equilibrium between Ra-226 and its daughters (La Verde et al., 2021a; La Verde et al., 2021b).
Alpha spectrometry was performed through an electrostatic collection chamber, a cylindrical steel chamber, of length and diameter of 10 cm and a volume of 0.8 L. The cylinder body is sealed and is equipped with a metal tray covered by a tight meshed metal grid at the bottom to hold the sample and to ensure a homogeneous electric field. A surface barrier silicon detector (model BU 019300100 by Ametek) is positioned at the top of the chamber to detect alpha particles, and it is insulated from the chamber’s body, which holds a voltage of 3500 V. The detector’s resolution is 19 keV (FWHM) for alpha particles and 14 keV for beta, with an active area of 300 mm2 and a sensitive zone depth of 100 µm. During the experiment, the detector is polarized with a 50 V tension.
The chamber electrostatic field directs the positively-charged decay products of radon towards the detector where they further decay emitting alpha particles which are then detected. The high resolution of the detector, along with minimal energy degradation, allows for precise differentiation of peaks from Rn-222 and Rn-220 decay products. This separation is vital because knowing the exact activity concentrations allows for a better consideration of health risks associated with these two radionuclides.
The multichannel analyzer provides the complete alpha spectrum of the decay products, identifying peaks for Po-218 (6.01 keV) and Po-214 (7.68 keV) from Rn-222, as well as Po-216 (6.77 keV), Bi-212 (6.09 keV), and Po-212 (8.77 keV) from Rn-220. Using these peaks along with an accurate calibration factor, the concentrations of Rn-222 and Rn-220 are calculated once equilibrium with their progenitors is achieved. The sealed chamber ensures that radon emitted by the sample accumulates in the chamber’s free volume, increasing in concentration until equilibrium is reached between the radon released and its decaying progeny. More detail on the calibration process can be found in Ambrosino et al. (2024).
High-resolution gamma spectrometry was carried out using a coaxial High-Purity Germanium detector (HPGe ORTEC®), model GMX-45P4ST, equipped with beryllium windows. The detector offers a relative efficiency of 48% and an energy resolution of 2.16 keV at 1.33 MeV FWHM. The minimum detectable activity (MDA) was calculated at a 95% confidence level.
Spectral data collection was managed through the Ortec DSPEC-LF system and the MCA Emulator software (Maestro-32), with subsequent analysis conducted with the software GammaVision Spectrum Analysis (v. 7.01). Measurements of the background spectra were taken and subtracted from the sample data to remove any noise contribution. A counting time of approximately 172,800 s (48 h) was used for each sample, while 259,200 s (72 h) were set for background measurements, in order to ensure statistical robustness.
The gamma-ray analysis targeted the following transition energies from the natural decay chains:
• U-238: 63.2 keV and 92.5 keV for Th-234, 186 keV for Ra-226, 46.50 keV for Pb-210.
• Th-232: 911.1 keV and 968.9 keV for Ac-228.
• K-40: 1461 keV.
These were used to calculate the Index I, a screening tool for the identification of BMs of radiological significance concerning external gamma radiation exposure, adopted by RP112 (European Commission, 1999), by Euratom 59/2013 Annex VIII (European Union, 2013) and finally implemented by Italian Decree 101/2020 Annex II (Repubblica Italiana, 2020).
If the Index I value is equal to or less than 1 (I ≤ 1), the material is deemed suitable for unrestricted use. If the Index I exceeds 1 (I > 1), a more detailed assessment of the dose from gamma exposure becomes necessary. Should the calculated dose surpass the reference level of 1 mSv/y, the material is considered unsuitable for construction in civil engineering, particularly in residential or high-occupancy structures.
Index I was calculated from the activity concentrations (Bq/kg) obtained through gamma spectrometry using the following Formula 1:
Where CRa-226, CTh-232, and CK-40 indicate respectively the activity concentration of Ra-226, Th-232, and K-40 in the BM. More detail can be found in Markkanen (1995).
Radon emanation refers to the fraction of radon atoms that retain sufficient kinetic energy to escape the material in which they were originated. Exhaled radon is the portion of these atoms that reaches the porous volume of said material and then diffuses into the outside air, potentially entering living spaces where people are exposed to its radioactivity.
The emanation coefficient η is defined as the ratio between the radon that is emitted into the material’s porosity and the total amount of radon contained in the sample. For the 222Rn isotope η was calculated using the following Formula 2:
where
To measure
where
Radon exhalation rate (E) is defined as the quantity of radon released from the material per unit of time. Assuming a constant E over time, which is reasonable when the chamber volume is significantly larger than the sample volume, and considering that the sample thickness is negligible compared to the mean free path of radon diffusion (thus preventing back diffusion), the exhalation rates for Rn-222 and Rn-220 can be expressed as reported in Equations 6, 7:
Time of the measurements was continuous acquisition cycles of 1 h for 4 weeks (time required for the secular equilibrium of Ra-226 and of Th-232 and their daughters)
Results of the measurements performed on the 10 samples are reported in Table 1, indicating the minimum, maximum and average value. Furthermore, although it is not a legislative requirement, the index I was calculated using the average values to better label the typology of the two BMs. Indeed, the index I is required in the production and sale phase and not for BMs in existing buildings. This aspect is a critical issue since the realistic exposure scenario of a representative person inside a building is not considered.
Table 1. Minimum, maximum and average values of activity concentration of Ra-222, Th-232 and K-40 for samples of both types of stones. Index I was calculated using the mean value of activity concentration of each radionuclide.
From these results it is evident that the two types of stones are not comparable and that Rosso Ammonitico has an index I of 0.82 close to unity (reference limit of the legislation). Minimum Detectable Activity (MDA) of each measurement can be assumed below 5% of the value for both gamma and alpha measurements (La Verde et al., 2021a; La Verde et al., 2021b).
Tables 2, 3 show the results of the 10 samples considering a constant volume of 0.00008 m3 and a mass of 0.068 kg and 0.071 kg for Rosso Ammonitico and Pietra Serena, respectively.
Table 2. Activity concentration of Rn-222 per unit volume (Bq L-1), emanation coefficient (η) and exhalation rate E (E) calculated for samples of both types of stones. The mean value of η and E is not the mean of the values calculated for each sample but the result using the mean value of Ra-226 activity concentration reported in Table 1.
Table 3. Activity concentration of Rn-220 per unit volume (Bq L-1), emanation coefficient (η) and exhalation rate (E) calculated for samples of both types of stones. The mean value of η and E is not the mean of the values calculated for each sample but the result using the mean value of Th-232 activity concentration reported in Table 1.
If emanation depends mainly on the activity concentration of Ra-226 and Th-232, exhalation depends on multiple factors such as porosity, humidity (Janik et al., 2015), size of the granules, (Immè et al., 2014), distribution of radionuclides with respect to the surfaces of the granule, along the matrix-internal cavity interface (Sakoda et al., 2011). To confirm this, comparing the mean values of the two types of stones, it emerges that CRn-222 ema and ȠRn-222 of Rosso Ammonitico are 49% and 80% lower than the values of Pietra Serena, respectively. On the contrary, E average values are comparable.
For Rn-220 in Rosso Ammonitico, the CRn-220 ema and ȠRn-220 are 38% and 92% lower than the values of Pietra Serena, respectively. In addition, E mean value is 62% lower than that of Pietra Serena. From the results obtained it is reasonable to deduce that Rn-220 contributions may exceed the corresponding Rn-222 values, since in the indoor environment Rn-220 could be more likely to come from the BMs exhalation rather than from soils, due to its shorter half-life.
The first criticality is the limited amount of information available from the literature, given that the topic concerns a restricted circle of experts. In fact, the data can be compared only with validated and verified data from official and resonant documents such as the ISTISAN Report, a database updated to 2017 in which all the radiometric information from the international literature is collected, (Nuccetelli, et al., 2017). This comparison is possible associating Rosso Ammonitico and Pietra Serena to the geological category they belong to: Limestone and Sandstone, respectively. Table 4 shows the radiometric data of the gamma-emitting radionuclides.
Table 4. Ra-226, Th-232 and K-40 activity concentration in BMs of Italian section in ISTISAN Report 17/36 (Nuccetelli et al., 2017).
Table 5 shows the data relating to η and E for Rn-222. No data found for Rn-220.
Table 5. Rn-222 emanation coefficient (η) and exhalation rate (E)in BMs of Italian section in ISTISAN Report 17/36 (Nuccetelli et al., 2017).
Considering the literature and experimental data, it was found that it was impossible to make a reasonable comparison, for a first and fundamental reason: the different origin of the samples. In fact, Righi and Bruzzi, 2006 used samples from northern Italy, while Rizzo et al. (2001) from southern Italy (Sicily), the samples of this study come from central Italy, therefore gamma emitting radionuclides activity concentrations are varied.
For η and E, measured and calculated from the same samples as in the Table 4, in addition to the different geological origin, the different measurement methodology must be considered. Righi and Bruzzi (2006) used the E-PERM electret ion chambers (Kotrappa and Jester, 1993), Rizzo et al. (2001) adopted a theoretical model (Man and Yeung, 1999), in this work an electrostatic collection chamber described in 2.5 was used.
A radiometric characterization of peculiar BMs of a historical building intended for public use was conducted to ensure radiation protection. The building, an ancient furnace, is located in a geologically peculiar area mainly made up of clay, which represented the raw material for the production of the artifacts. After a thorough renovation, while preserving the ancient BMs used, radiometric surveys were conducted on the two main types of BMs: Rosso Ammonitico (a marine limestone) and Pietra Serena (a sandstone). In particular, measurements were carried out of gamma-emitting radionuclides activity concentrations (Ra-226, Th-232 and K-40), and measurement of the exhalation coefficient and calculation of the exhalation rate of radon gas.
The methodological approach adopted was that of the current national legislation (Repubblica Italiana, 2020), although not having to fulfill any obligation. In fact, gamma spectrometry measurements and calculation of the Index I are mandatory only during the production phase of particular BM (such as tuff, pozzolana, granite) in order to verify their suitability for marketing and use. For values of I > 1 the BM is not suitable. In this case, although they are not BMs of radioprotection interest, the aforementioned radiometric evaluations were carried out, discovering that Rosso Ammonitico unexpectedly has an Index I of 0.82, very close to unity although not causing any concern, while Pietra Serena has an I of 0.08.
Another indication adopted in this study was that of the National Radon Action Plan (Repubblica Italiana, 2024), aimed at reducing exposure to indoor radon through actions promoted by the authorities. This document highlights the need to calculate radon exhalation rate (E) from BMs that could contribute to the gas accumulation indoor. The emanation coefficient (η) was therefore measured, both of Rn-222 and of its isotope Rn-220 to calculate E. The Rosso Ammonitico and the Pietra Serena have ERn-222 of 0.004 ± 0.001 (Bq kg−1 h−1) and 0.007 ± 0.001(Bq kg−1 h−1), for the ERn-220 mean values were 70 ± 12 (Bq kg−1 h−1) and 113 ± 20 (Bq kg−1 h−1), respectively.
Results discussion highlighted two critical issues: the paucity of information in the scientific literature regarding a specific lithological typology, considering that the geogenesis processes are often specific to each geographical area, and the diversity of measurement methodologies that make comparisons of the results complex and often impossible. Therefore, it would be desirable to validate a standardized protocol for a holistic radiometric characterization of BMs for radiation protection not only in the specific environments identified by the legislation, but also in restored buildings and with a new intended use that includes the attendance of members of the population.
The raw data supporting the conclusions of this article will be made available by the authors, without undue reservation.
GL: Conceptualization, Data curation, Formal Analysis, Investigation, Methodology, Project administration, Validation, Visualization, Writing–original draft, Writing–review and editing. AR: Resources, Supervision, Writing–review and editing. EO: Writing–review and editing. FA: Data curation, Formal Analysis, Investigation, Writing–review and editing. MP: Conceptualization, Funding acquisition, Project administration, Resources, Supervision, Validation, Visualization, Writing–review and editing.
The author(s) declare that no financial support was received for the research, authorship, and/or publication of this article.
The authors would like to thank the kindness of the Public Works Service of the Municipality of Impruneta for having made available the site called “ex fornace Agresti” as a site for the radiometric characterization.
The authors declare that the research was conducted in the absence of any commercial or financial relationships that could be construed as a potential conflict of interest.
All claims expressed in this article are solely those of the authors and do not necessarily represent those of their affiliated organizations, or those of the publisher, the editors and the reviewers. Any product that may be evaluated in this article, or claim that may be made by its manufacturer, is not guaranteed or endorsed by the publisher.
Ahmed, K. A., Khan, S., Sultan, M., Nisar, U. B., Mughal, M. R., and Qureshi, S. N. (2020). Landslides assessment using geophysical and passive radon exhalation detection techniques in Murree Hills, northern Pakistan: implication for environmental hazard assessment. J. Earth Syst. Sci. 129, 53. doi:10.1007/s12040-019-1327-y
Ambrosino, F., La Verde, G., Gagliardo, G., Mottareale, R., Della Peruta, G., Imparato, C., et al. (2024). Radon exhalation rate: a metrological approach for radiation protection. Sensors 24, 3633. doi:10.3390/s24113633
Baskaran, M. (2016). “Physical, chemical and nuclear properties of radon: an introduction,” in Radon: a tracer for geological, geophysical and geochemical studies (Cham: Springer Geochemistry. Springer). doi:10.1007/978-3-319-21329-3_1
Beir, V. I. I. (2006). “Health risks from exposure to low levels of ionizing radiation,” in Committee to assess health risks from exposure to low levels of ionizing radiation. Washington, DC: National Academies Press US: National Research Council.
Benà, E., Ciotoli, G., Ruggiero, L., Coletti, C., Bossew, P., Massironi, M., et al. (2022). Evaluation of tectonically enhanced radon in fault zones by quantification of the radon activity index. Sci. Rep. 12, 21586. doi:10.1038/s41598-022-26124-y
European Commission (1999). “Radiation protection 112,” in Radiological protection principles concerning the natural radioactivity of building materials.
European Union (2011). Regulation 305/2011 of the European Parliament and of the Council of 9 March 2011 laying down harmonized conditions for the marketing of construction products and repealing Council Directive 89/106/EEC
European Union (2013). Council Directive 2013/59/Euratom of 5 December 2013 laying down basic safety standards for protection against the dangers arising from exposure to ionising radiation, and repealing Directives 89/618/Euratom, 90/641/Euratom, 96/29/Euratom, 97/43/Euratom and 2003/122/Euratom.
Fratini, F., and Rescic, S. (2014). The stone materials of the historical architecture of Tuscany, Italy. Geol. Soc. Lond. Spec. Publ. 391 (1), 71–92. doi:10.1144/SP391.5
IARC (1988). Monographs on the evaluation of carcinogenic risks to humans. Man-Made Mineral Fibres Radon 43, 33–171.
ICRP (2007). The 2007 recommendations of the international commission on radiological protection. ICRP Publ. 103. Ann. ICRP 37 (2-4).
Immè, G., Catalano, R., Mangano, G., and Morelli, D. (2014). Radon exhalation measurements for environmental and geophysics study. Radiat. Phys. Chem. 95, 349–351. doi:10.1016/j.radphyschem.2013.02.033
International Atomic Energy Agency (IAEA) (2023). “Regulatory control of exposure due to radionuclides,” in Building materials and construction materials, safety reports series No. 117 (Vienna: IAEA).
ISO 18589-2:2015 (2015). Measurement of radioactivity in the environment - soil -Part 2: guidance for the selection of the sampling strategy, sampling and pre-treatment of samples. Technical Committee: ISO/TC 85/SC 2.
Janik, M., Omori, Y., and Yonehara, H. (2015). Influence of humidity on radon and thoron exhalation rates from building materials. Appl. Radiat. Isot. 95, 102–107. doi:10.1016/j.apradiso.2014.10.007
Kotrappa, P., and Jester, W. A. (1993). Electret ion chamber radon monitors measure dissolved 222Rn in water. Health Phys. 64 (4), 397–405. doi:10.1097/00004032-199304000-00007
La Verde, G., Artiola, V., Raulo, A., D’Avino, V., Paternoster, G., Roca, V., et al. (2022). Radiological impact of building material: characterization of a village entirely built out of stone in Tuscany, Italy. IOP Conf. Ser. Earth Environ. Sci. 1046 (1), 012002. doi:10.1088/1755-1315/1046/1/012002
La Verde, G., Raulo, A., D’Avino, V., Paternoster, G., Roca, V., La Commara, M., et al. (2021a). Pietra leccese and other natural stones in puglia region: a new category of building materials for radiation protection? Int. J. Environ. Res. Public Health 18, 11213. doi:10.3390/ijerph182111213
La Verde, G., Raulo, A., D’Avino, V., Roca, V., de Asmundis, R., and Pugliese, M. (2021b). Green tuff: gamma index in a typical building material of ciglio area (ischia island). Solid State Phenom. 319, 24–29. doi:10.4028/www.scientific.net/SSP.319.24
Man, C. K., and Yeung, H. S. (1999). Modeling and measuring the indoor radon concentrations in high-rise buildings in Hong Kong. Appl. Radiat. Isot. 50, 1131–1135. doi:10.1016/s0969-8043(98)00128-6
Markkanen, M. (1995). Radiation dose assessments for materials with elevated natural radioactivity, Report STUK-B-STO 32. Radiation and Nuclear Safety Authority-STUK.
Nuccetelli, C., Risica, S., Onisei, S., Leonardi, F., and Trevisi, R. (2017). “Natural radioactivity in building materials in the European Union: a database of activity concentrations, radon emanations and radon exhalation rates,” in Istituto Superiore di Sanità (Roma). Rapporti ISTISAN 17/36.
Repubblica Italiana (2024). Adozione del Piano Nazionale D’azione per il Radon 2023–2032. GU Ser. Gen. n.43 del 21-02-2024-Suppl. Ordin. N. 10. Available at: https://www.gazzettaufficiale.it/eli/id/2024/02/21/24A00877/sg (Accessed September 2, 2024.
Righi, S., and Bruzzi, L. (2006). Natural radioactivity and radon exhalation in building materials used in Italian dwellings. J. Environ. Radioact. 88, 158–170. doi:10.1016/j.jenvrad.2006.01.009
Rizzo, S., Brai, M., Basile, S., Bellia, S., and Hauser, S. (2001). Gamma activity and geochemical features of building materials: estimation of gamma dose rate and indoor radon levels in Sicily. Appl. Radiat. Isot. 55, 259–265. doi:10.1016/S0969-8043(00)00384-5
Sabbarese, C., Ambrosino, F., Chiodini, G., Giudicepietro, F., Macedonio, G., Caliro, S., et al. (2020). Continuous radon monitoring during seven years of volcanic unrest at Campi Flegrei caldera (Italy). Sci. Rep. 10, 9551. doi:10.1038/s41598-020-66590-w
Sakoda, A., Ishimori, Y., and Yamaoka, K. A. (2011). A comprehensive review of radon emanation measurements for mineral, rock, soil, mill tailing and fly ash. Appl. Radiat. Isot. 69 (10), 1422–1435. doi:10.1016/j.apradiso.2011.06.009
UNSCEAR (2000). SOURCES and effects of ionizing radiation united nations scientific committee on the effects of atomic radiation UNSCEAR 2000 report to the general assembly, with scientific annexes volume I. New York: SOURCES UNITED NATIONS.
Keywords: building material, measurements, ionizing radiation, cultural heritage, radiation protection, indoor environment
Citation: La Verde G, Ricciardelli A, Ognibene E, Ambrosino F and Pugliese M (2024) Radiation protection and natural building materials in cultural heritage. Front. Built Environ. 10:1494237. doi: 10.3389/fbuil.2024.1494237
Received: 10 September 2024; Accepted: 17 October 2024;
Published: 25 October 2024.
Edited by:
Hasim Altan, Prince Mohammad bin Fahd University, Saudi ArabiaReviewed by:
Aydın Büyüksaraç, Çanakkale Onsekiz Mart University, TürkiyeCopyright © 2024 La Verde, Ricciardelli, Ognibene, Ambrosino and Pugliese. This is an open-access article distributed under the terms of the Creative Commons Attribution License (CC BY). The use, distribution or reproduction in other forums is permitted, provided the original author(s) and the copyright owner(s) are credited and that the original publication in this journal is cited, in accordance with accepted academic practice. No use, distribution or reproduction is permitted which does not comply with these terms.
*Correspondence: Giuseppe La Verde, Z2l1c2VwcGUubGF2ZXJkZUB1bmluYS5pdA==
Disclaimer: All claims expressed in this article are solely those of the authors and do not necessarily represent those of their affiliated organizations, or those of the publisher, the editors and the reviewers. Any product that may be evaluated in this article or claim that may be made by its manufacturer is not guaranteed or endorsed by the publisher.
Research integrity at Frontiers
Learn more about the work of our research integrity team to safeguard the quality of each article we publish.