- Tarbiat Modares University, Tehran, Iran
The problems of today’s world, particularly climate change and air pollution, require the search for sustainable and green buildings in urban environments and the people who inhabit them. General population and construction industry has emitted pollutants such as carbon dioxide to the atmosphere due to urbanization and rise in vehicles. In addition to that, the products employed in construction express a caliber of polluting the air. Urban facades and the materials used for construction in cities can often have a significant impact on aggravating air pollution. The implementation of green walls on building’s facade and other structures can be considered as the proper substitute to utilizing the stone and cement as those materials minimize the emission of air pollutants and add the better aesthetics to the city environment. The main objectives of this research are to describe and introduce a new method of applying green facades for buildings and structures using plants which have even greater abilities at absorbing pollutants and are simpler in application and management in comparison with other systems of green walls. In the first stage, the known green wall systems and along with suitable plants were introduced and studied through library studies and accordingly the most compatible plants for the studied area, considering the climate of Iran, specially Tehran which is the most polluted city in Iran, were identified. The ENVI-met application was used subsequently to determine the most appropriate model or plant to combat the pollutants in the environment.
1 Introduction
Pollution of our environment particularly through air has become a real issue that has taken a toll on human lives. Urban centers have played a major role in heightening this problem though it is expected that the proportion of the global populace living in cities will rise from 54 percent in 2015 to 60 percent in 2030 and to 66 percent in 2050 (WHO, 2016). Urbanization has amplified together with the scarcity and high cost of land for housing so buildings are significantly constructing in city centers and air becomes trapped among these youthful urban constructions (Junior et al., 2022). The global population increase, also complementary utilization of the fossil fuel needed for several activities such as transport, leads to heightened levels of air pollution in cities (Rezazadeh et al., 2022). To mitigate and prevent the production of emissions in the atmosphere, the following interventions have been recommended which includes forecasting the manufacturing industries to outskirts, restricting the usage of automobiles, increasing the number of plants in cities. However, even with the help of these measures, progress is limited (Lelieveld et al., 2015).
About 40% of all the carbon dioxide emissions to the atmosphere originate from construction especially from building materials and this increases air pollution globally (Wieser et al., 2021). Several environmental problems are a consequence of the poor-quality construction materials applied to the facades in urban areas (Andersen et al., 2024). Such materials are also less durable than conventional ones, which means that they degrade more quickly; as a result, they need repair or replacement more frequently. This leads to even higher resource consumption and levels of waste (Wieser et al., 2021). Besides, such materials may also lack sufficient insulating properties, meaning that they necessitate the use of more energy, especially for regulating indoor temperatures. In addition, dark and heat-concentrated materials worsen the so-called “urban heat island” and increase temperatures in the urban environment. In summary, the given situations imply that the inferior construction materials chosen for the urban facade are responsible for not only the unsightly look of the buildings and their poor stability but also the significant contribution to the biological contamination and deterioration of the air.
Introduction of vegetation through green walls has a positive impact on air movement given that there are usually little or no plant growth in such areas (Viecco et al., 2021). Some pollutants are directly deposited on the surfaces of the plants and some are even taken up by the plants through their leaves (Morakinyo et al., 2016) and roots, further, any remaining particles are dispersed by the flow of air surrounding and through the wall (Pettit et al., 2021). Nonetheless, the implications of green walls on air circulation are not absolute since they are influenced by the following factors (Pugh et al., 2012). This intellectual judgment indicates that the characteristics of the vegetative media, the structure of the green wall, and its position affect the efficiency with which it can reroute or confine airflow to lower pollutant concentrations (Abhijith and Kumar, 2020). Sometimes, there could be some other constituents like close buildings or structures that may hinder the wall from facilitating the flow of air in certain directions (Abhijith et al., 2017; Ahmadi et al., 2022; Ghanbari et al., 2022). In general, it can be stated that green walls are environmentally friendly interventions to enhance air quality in cities with the help of plants’ innate ability to clean the air (Al-Dabbous and Kumar, 2014). They can assist in improving the movement of air and dilution of pollutants and gases; however, their efficiency is relatively inclined by the prevailing aspects of design and settings (Baradaran Motie et al., 2023; Grote et al., 2016).
Green infrastructure in cities can be used as an nature-based solution to combat air pollution in urban areas and the urban walkways (Janhäll, 2015). Some of the green solutions are as follows: tree planting on the boulevard; green strip which is used to segregate pedestrian and the vehicles’ way, green walls (GW), and roof gardens (RG) (Ysebaert et al., 2021).
1.1 Green wall
The green wall is one of the modern technologies that are gradually becoming integrated into emerging and innovative cities across the globe (Othman and Sahidin, 2016). A green wall involves a structure, which can be independent or integrated into the building envelope, with foliage growth on it (Pérez-Urrestarazu et al., 2015). Economically, the green walls enhance the property value through: averting the expansion of green land, shielding building frameworks from harm (Baldauf, 2017), and adding beauty to the buildings (Baraldi et al., 2019). Also, they act as insulators by saving energy used in heating and cooling throughout the year, thus a conservation of energy costs (Gallagher et al., 2015). Drip irrigation systems and water recycling that are incorporated in green walls ensure that water consumption is efficient making them environmentally beneficial (Minks et al., 2017).
1.2 The role of the green wall in the sustainability of architecture and urban planning
More specifically, green walls perform a significant function in the enhancement of sustainability in architecture and particularly within the urban planning context along several perspectives (Vélez-Landa et al., 2021). They can help reduce effects of urban heat island by providing shade and evaporative cooling to reduce the micro climate of the surrounding urban area (Perini et al., 2017a; Sharbafian et al., 2024). Besides, green walls enhance the quality of air within the surrounding environment through trapping of the pollutants and the particulate matters that cause unhealthiness of the urban areas (Shishegar, 2014). They are vital in energy efficiency too as they act as insulators, preventing the need for a heated room in winter or a cooled room in summer leading to decreased energy requirements by building, and hence reducing the carbon footprint (Tramontin et al., 2012). Also, green walls play a significant role in storm water control since they can store and filter rainwater and thus lighten the load of urban drainage systems and the likelihood of floods (Ashtari et al., 2021; Hop and Hiemstra, 2013). It is possible to point on aesthetic values as well: green walls improve the look of buildings and urban environment, positively influencing people’s psychological state (Abhijith et al., 2017). Finally, because they foster urban wildlife, green walls promote stability of natural ecosystems and align with sustainability concerns in city planning. The incorporation of green walls in the layout and design of cities is therefore seen as a positive forward thinking strategy for developing healthy resilient, sustainable cities globally (McConnaha, 2003).
1.3 Types of green walls
There are different types of green walls, from the simplest and most basic to the most complex and advanced implementation systems. They are divided into two main groups based on the type of plants and maintenance system: green facade (GF) and living wall (LW) (Pérez-Urrestarazu et al., 2015).
1.3.1 Green facade
Green facades can be divided into two groups based on the behavior of plants that can be hanging or creeping: direct system (DGS) and indirect system (IDGS) (see Figure 1) (Ottelé et al., 2011). In direct green walls, plants are directly connected to the wall due to the adhesion property of some plants without the need for additional structures, and the roots of the plants are either placed on the ground or in flower-shaped boxes (Figure 2). However, not all plant species have this adhesive property, so they need additional support systems to maintain their position and grow along the wall. This issue is also clearly evident in the case of the indirect system, where the rooting space is also placed either in the ground or in the potting boxes. The indirect green wall also acts as a two-shell facade, which creates an air gap between the facade of the building and the plants (Jim, 2015).
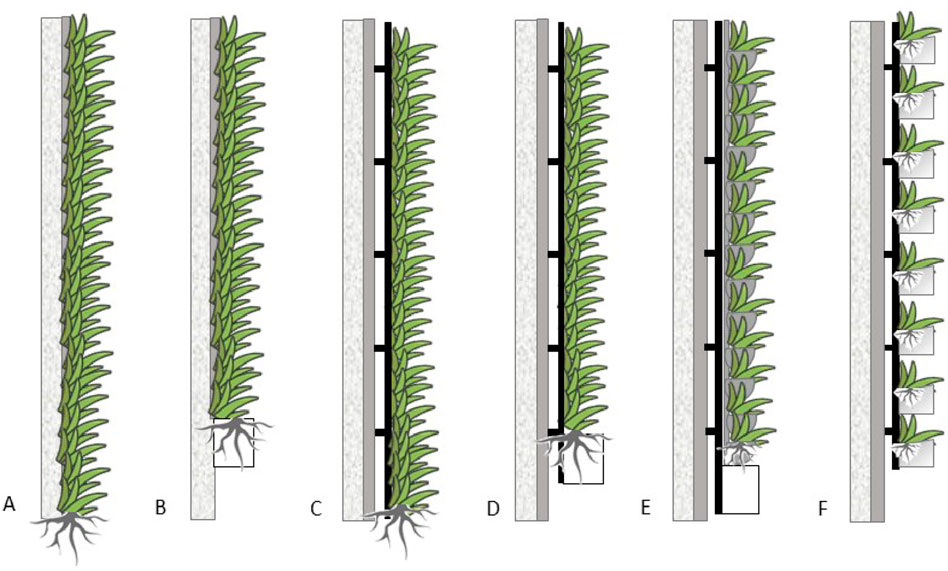
Figure 1. Diagrams of green wall and living wall systems: (A) Direct greening (planted into the soil), (B) Direct greening (planted into planter box), (C) Indirect greening (planted into the soil), (D) Indirect greening (planted into planter box), (E) Hydroponic living wall, (F) Tray system.
1.3.2 Living wall
Living walls are considered noir in the wall-covering field and generally have a more complex structure than other green coverings. In living walls, the roots of plants are connected to the wall, and creeping plants are usually used for this structure Unlike green walls, living walls allow rapid coverage of large areas and more uniform growth in height. Living walls are divided into two categories: continuous system and modular system (Chang and Chang, 2022). A French botanist named Patrick Blanc first invented a continuous living wall, vertical garden, or hydroponic system (see Figure 1). This system consists of two layers of felt on a metal chassis with a waterproof layer against moisture to protect the facade of the building from moisture caused by watering the plants; in this method, the plants are first planted in small bags or pockets and then installed on felt. Nutrients and water are injected into the roots of plants through an irrigation system (Palermo and Turco, 2020). Modular living walls are different in composition and weight; this type of living wall consists of modular pots (trays) that can be connected vertically or at a certain angle to the back structure according to the design, then plants in bags small ones are placed in pots, this method requires regular watering and fertilization. An automatic system usually does it; also, various plants with high density can be used in this method. With this method, the green wall can create 90% coverage from the beginning of planting, unlike the continuous green wall (Table 1).
1.4 The effect of green walls on air pollution
Green walls are one of the sustainable approaches to increasing vegetation in areas with high pollution without using up valuable city space. They give environmental advantage and beautification value, which makes them a noble offer for today’s urban beautification and city preservation initiatives. It also can create a healthy-lifestyle pattern (Perini et al., 2017b). Research conducted on a number of different investigations suggest that green infrastructure in cities is a concept of optimizing the quality of air and the sustainability of the urban environment. Information provided (see Table 2) shows that green walls and greenery contribute to improvements of wellbeing of the masses and standard of the urban environment. Since these green barriers, therefore referred to as green walls, are porous materials that influence air pollution emissions, they can be a vent or filter for air. Hence, the adoption of this strategy can be regarded as one of the key factors that contribute to the availability of a clean environment (Jim, 2015).
1.5 The impact of different types of vegetation on green walls
With climbing plants like ivy, the roots fix into the structure or the back wall which provides a patchy facing of the green wall (Baldauf, 2017). These plants are usually very stable and can tolerate different atmospheric factors like excessive heat, strong winds, and rain that prevail in severe climates (Currie and Bass, 2008). It is mounted vertically on exterior walls and is very robust, which is why it can be recommended for high-rise constructions (Köhler, 2008). In the normal circumstances it is expected to cover a wall with climbing plants within 3–5 years depending with the prevailing environmental factors (Grabowiecki et al., 2017). However, this can take a relatively longer period of time in some cases. Faster growing climbers may receive excellent coverage in just a couple of seasons or even 2 years, while slower growing plants may take about or more than 2 years to ground cover a corresponding area (Niklas, 2011).
Many strategies have been underdeveloped to mitigate and prevent air pollution (see Table 2), and interest groups are also trying to regulate pollutant levels and enhance the quality of the air in cities as a global problem like urbanism. In conclusion, a number of researches have indicated green walls and green façade as an optimal proposal in maintaining the AQI and PM concentrations in urban environment which would have positive effects on people’s health as well. However, there are some issues which are yet to be addressed despite working with other researches indicating that green spaces can be effectively used to alleviate polluted air situations and especially in the urban neighborhoods. First, the greening pattern of the literature published in the last decade on the application of green walls to reducing pollution intensity in urbanized areas has not been clearly declared because in many cases it is impossible to cover most of the building’s facade. It is very appropriate to provide an efficient pattern in the process.
Second, it is also highly significant and effective to propose appropriate plants that can lower the concentrations of pollutants. However, there is insufficient literature focusing on and comparing plants that are suitable for the various climatic zones and also plants whose characteristics correlate with the climatic conditions of the regions to which they are suitable for and which can be put in the urban facades. In this study, first of all, it is tried to determine the target area for the implementation of green facade and analyze this area in terms of climate. Next, green wall plants which could be used for green walls and that are suitable for the climate are described. Following that, patterns for greening are presented. Last, with the help of an ENVI-met software, the selected patterns and plants are examined in order to establish the most fit pattern and the proposed plant species.
2 Methodology
Using plants on urban facades and walls can provide many benefits for residents and buildings. These benefits include reducing air pollution, enhancing visual appeal, providing thermal and sound insulation, promoting biodiversity, controlling indoor temperature, and reducing indoor dust levels. The primary and most important goal of this research is to evaluate and investigate various plants and planting patterns for urban walls and to provide suitable alternatives for urban facades. This study examined four types of plants suitable for green walls and the climate of Tehran, along with nine greening patterns, using ENVI-met software to select the most appropriate plants and patterns for urban walls (see Figure 3).
2.1 Case study: Central fabric of tehran, Iran
2.1.1 Location and background
Geographically, Tehran is located between 35.6892° North latitude and 51.3890° East longitudes. It has an average elevation of 1,200 m above sea level and occupies a total area of 730 km2. It is Iran’s capital city and serves as the political, cultural, economic, and administrative center of the country. Tehran is divided into 22 district and the selected area is located in District 11, more precisely in Zone 5 of Tehran (see Figure 4). In view of the commercial, cultural, historical, and educational functions of the buildings located here, this area is believed to be the most crowded in Tehran. The use of numerous approaches including limiting road usage by vehicles could not help minimize the pollutants entrenched in this area.Thus, this area can be suitable to assess and study the effectiveness of green walls in countering air pollution.
2.1.2 Climate
Tehran has a semi-arid climate that entails hot summers and cool winters. Average temperature vary from about 4°C to about 22°C it is also important to note that most of the times low altitude areas receive more rainfall than high altitude areas. 5°C (40°F) in January reaching to 33°C in July. Average minimum temperature 5°C (92.3°F) in July (see Table 3). Rainfall (see Figure 5) is fairly low and averages to around 233 mm or 9.2 inches per year and it is well distributed from November to April. Seasonal fluctuation is also recorded concerning with relative humidity is likely to experience high levels during winter while summer records low levels which in general is an arid region.
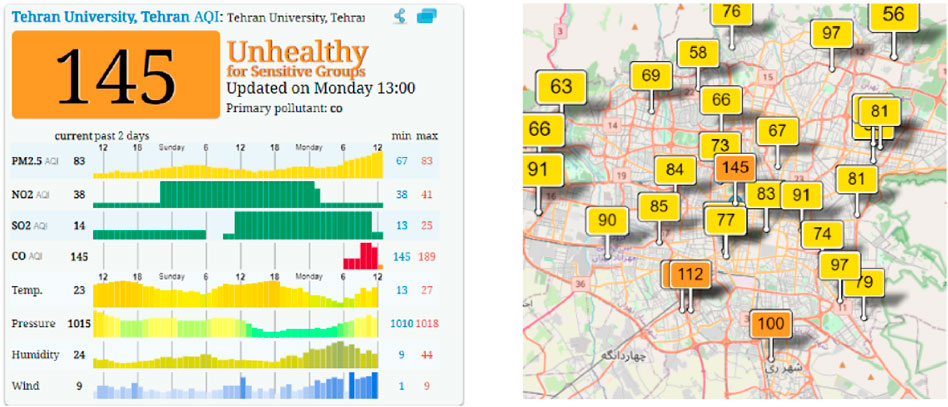
Figure 5. Average yearly precipitation (mm), sunshine (hours), and Temperature (°C) of Tehran, Iran.
Specifically, air pollution challenges are acute in Tehran due to the location of this city and climate conditions. It is a large city located in a high have altitude that is also surrounded by the Alborz Mountains that prevent air pollution from dispersing (Taghizadeh et al., 2023). Warm air becomes trapped on top of cool denser air at the surface resulting into pollution of the air during many months of the winter. Another statistical feature is seasonal periodicity for all type of pollutants, although it is minor for sulfur dioxide and nitrogen oxides which are higher in the winter for PM2.5 and PM10 (see Figure 6).
The left panel provides the air quality data of air pollution monitoring station located at Tehran University in more detail. It is presented as 145 to reflect “Unhealthy for Sensitive Groups” AQI with carbon monoxide (CO) as the primary pollution agent. The panel also provides a breakdown of additional pollutants over the past 2 days, including PM2.5, NO2, SO2, and CO levels, alongside meteorological data such as temperature, pressure, humidity, and wind speed.
2.2 Tools and software
Micro-scale modeling and simulation were performed by using ENVI-met which is a Comprehensive non-hydrostatic 3D model, in order to investigate the impact of plants on air pollution. Simulations employed the Monde module in ENVI-met with GIS map employed by Bitmap so that the streets, heights of buildings, and vegetation cover were as real as possible. The extracted information and maps were used to set up the situation by importing them into ENVI-met to assess the effects of different greenery patterns. This software does not model the precise shapes of plants, including leaves and branches though it identifies these features by parameters. ENVI-met evaluates plants based on two parameters: These include a concept defined as the density of the leaf area, “Leaf Area Density (LAD)” and “Leaf Area Index (LAI)” described. This paper aims to analyze the effect of plants and in this context the factors that need to be taken into consideration are LAD, temperature, humidity and airflow. This software focuses on the imitation of the mechanisms of the soil to measure the impact, while the introduction of the LAD and the diameter of the broadleaf, and the needle-leaf trees assess the velocity of the deposition. LAD determines the canopy configuration and plant height while LAI explains the mass of the plants, though not the same for every plant.
2.3 Analysis parameters in ENVI-met software
2.3.1 Pollution
The pollutant dispersion toward the environment computed by the ENVI-met software directly addresses the Eulerian approach, which is suitable for both gaseous pollutants and particle systems. This research is concerned with the accumulation of PM2.5 in the air, These are so small that they can easily enter the human respiratory system and cause respiratory problems. As such, their concentration is highly harmful to human health and can cause great threats to wellbeing of the citizens. These pollutants consist of fuel emissions, suspended solid particulate matters from incomplete combustion those are hydrocarbon compounds (HC), nitrogen oxides (NOx) and black carbon (BC). In ENVI-met, the mobile source of pollution from vehicles and traffic is shown as line sources. The modeling that is done within this software includes a dispersion and deposition model and this predicts the manoeuvre of particles and pollutants by calculating the concentration of each gas or Pollutant by the use of standard atmospheric dispersion equations or Eulerian Approach. A new point source is established along the primary direction. The source has a diameter of 10 µm, a height of 0.3 m, and a constant speed of 3 m*s/µg. This value is taken from the STREET s’ASPA database and represents the cross-section of a moderately used street during a period consisting of 7–8 a.m.
2.3.2 plant species
Depending on its social, environmental, financial, and economical values and functions, the urban green spaces are among the important assets of urban management and city development (Morakinyo and Lam, 2016). Different kinds of plants that can be used in green walls like climber plants, flowers, or seasonal plants have specific characteristics regarding their compatibility with the climate conditions and water consumption in Tehran (see Figure 7). They also differ in the extent to which they perform some of the expected functions of urban green spaces, beauty, and air purification and procreation of oxygen. Therefore, this research is to review the criteria for choosing and assessing plants and look into the best criteria for Tehran green areas. Choosing plant species that can be planted on living walls (see Figure 8) was done based on certain criterion such as adaptability to Tehran’s climate, water requirement, the aesthetic value, and oxygen-emitting capacity (see Table 4). To do this, the authors compiled a list of plants that can be planted in GW and LW through the collaboration with several firms located in Tehran, Iran that design and install GW and LW. Based on the existing plant species in Tehran and the plant species that can be planted on urban facades, four plant species of Pittosporum, Rosemarinus, Hedera helix, and Berberis were selected as the main samples of this study.
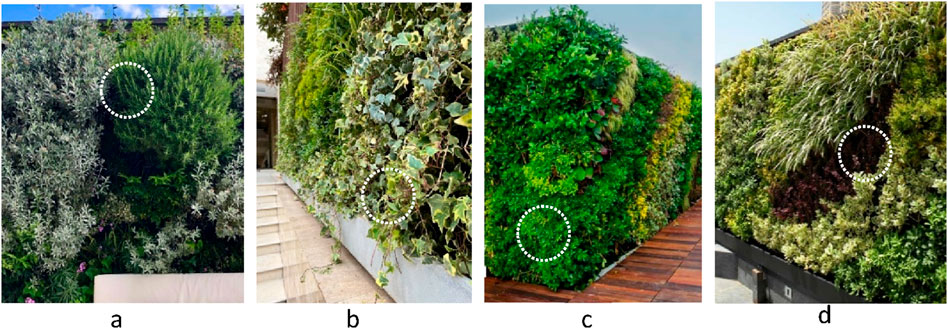
Figure 8. (A) Rosmearinus, (B) Hedera helix, (C) Pittosporum, (D) Berberis (images: ©Zia Eco, outdoor living walls, Tehran, Iran).
2.3.3 Greening pattern
There is another factor that might affect the decrease of air pollution and it is the greening pattern (Morakinyo et al., 2016). While facades may be clad over with vegetation, portions of them can be turned into Living Walls (LW) or Green Walls (GW). This approach is considered because managing, constructing, and establishing vast green walls are expensive for the residents. Moreover, green walls can be integrated with the facade materials to improve the aesthetic of the city. Hence, in this research, nine greening scenarios have been developed, each of which includes thirty 1 m * 1 m pixels (see Figure 9).
First, the facade of a building or any structure in the zone is examined with the following features being important. When having modeled the buildings within the ENVI-met software applying the greening scenarios, their facades are depicted as green (see Figure 10). For each of the greening patterns, assessments and analysis are conducted on the four plant species for the aim of identifying the best pattern and plant for efficient green facade concerning the absorption of pollutants (Figures 11, 12).
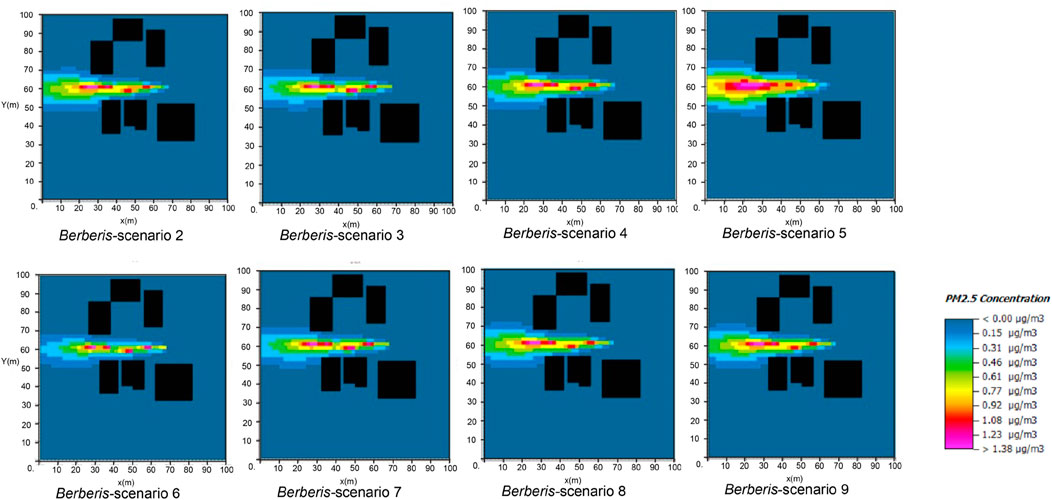
Figure 11. Spatial distribution of PM2.5 concentration (plant: Berberis). Leonardo maps extracted from ENVI-met.
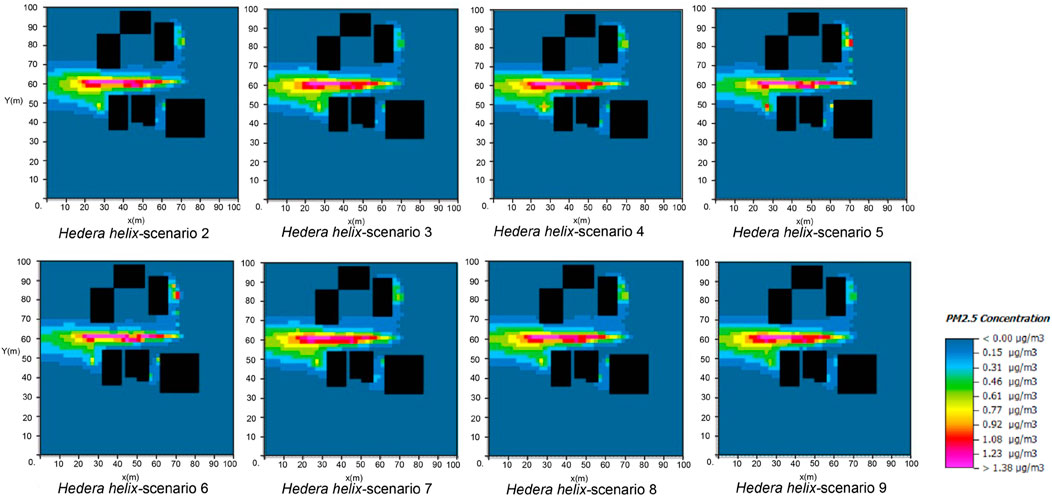
Figure 12. Spatial distribution of PM2.5 concentration (plant: Hedera helix). Leonardo maps extracted from ENVI-met.
3 Results and discussions
In measuring the level of air quality in this study, the Air Quality Index (AQI) (see Table 5) was used. AQI is a tool used to inform the public on the status of air quality. This scale on the use of different colors depicts the healthiness or hazardousness of the air. When pollutant concentrations elevate, the air quality degrades. In particular, there are normally eight basic pollutants taken into account for this index, of which PM2.5 particles. Thus, using the AQI as a reference, it is possible to assess the air quality in this case. The AQI is further broken into six bands, each having a color and corresponding to a set of number. These numbers can be directly correlated to pollution levels as well as the effects these have on health. When the AQI is above 100 the pollutant will be above EPA limits, which will be deemed unhealthy for all residents.
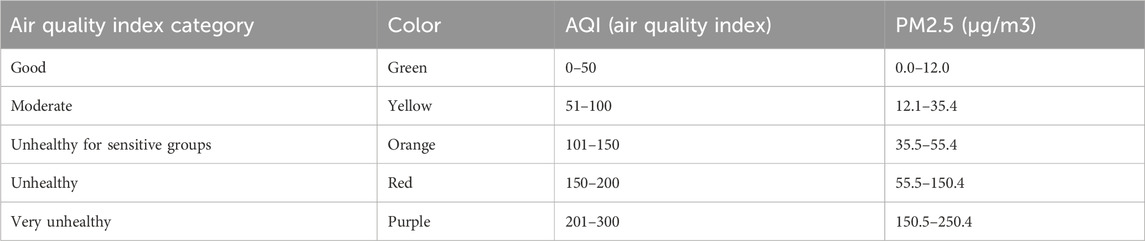
Table 5. Air Quality Index classification (Pm, 2019).
In this study, the outcomes are expressed in terms of two-dimensional maps employing the Leonardo software in ENVI-met. The AQI index was used to measure the effectiveness of the greening patterns and plants used in the green walls and also to compare the improvement of different factors. The results are examined separately in the following components (Figure 13).
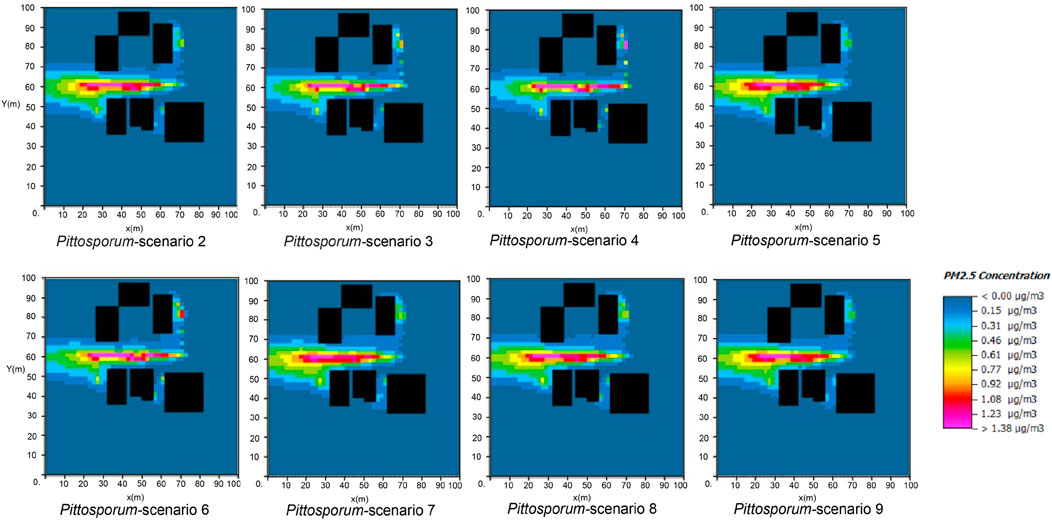
Figure 13. Spatial distribution of PM2.5 concentration (plant: Pittosporum). Leonardo maps extracted from ENVI-met.
3.1 The impact of plant type on reducing pollutants in the air
In this simulation, the facade of the buildings that has frontages on pedestrian zones was converted into green walls. These facades were most notably covered with four kinds of plants that can grow in Tehran instead of numerous stones, cements, and glasses. Based on the data received and analyzed with the help of ENVI-met software, one can conclude that changing conventional materials of building facades and applying planting instead of it in the form of green or living walls may have a positive impact on the reduction of particulate matter concentration. In accordance with what has been shown in Figure 14, it can be therefore postulated that out of all the plants that have been employed in the research, Rosemarinus was the most effective in immediately filtering out the most amounts of air pollutants. Thus, the integration of Rosemarinus on building facades lowered the Particulate Matter (PM2.5 pollutant) density has reduced (see Table 6). To elaborate, this figure fluctuated with the changes in various green coverage patterns. However, the plant Berberis had comparatively lower effectiveness in the mitigation and dispersion of PM2. Five pollutants than other plants.” Furthermore, plants like Pittosporum or Rosemarinus which are green permanently are considered adequate for facade greenery as the plants will cover the facade throughout the seasons, unlike deciduous plants like Berberis or H. helix which may lose leaves in autumn and winter or change their color (Figure 15). Hence, according to this study, employing specific varieties of plants, more importantly evergreens like Rosemarinus on building elevations, plays a major role in decreasing air pollution and hence improving the quality of air in the urban environment.
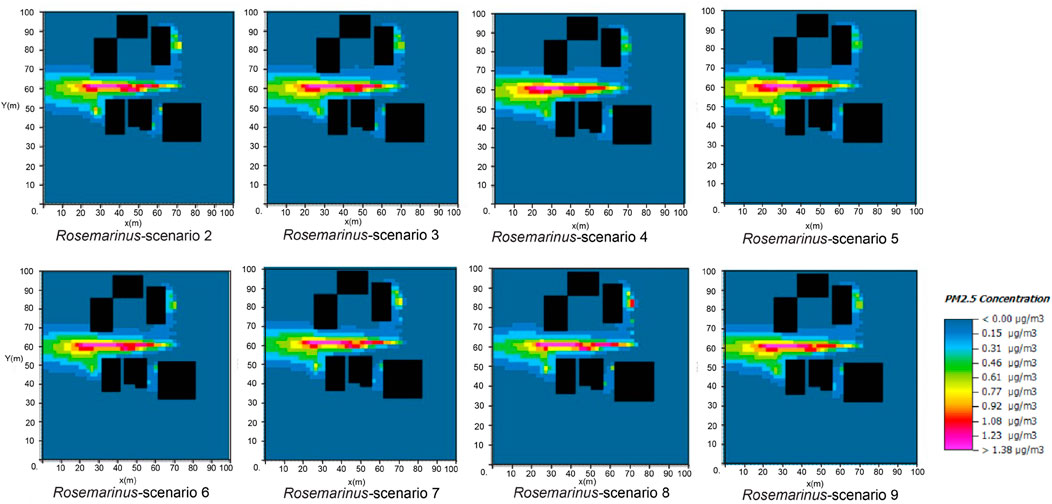
Figure 14. Spatial distribution of PM2.5 concentration (plant: Rosemarinus). Leonardo maps extracted from ENVI-met.
3.2 The impact of green coverage patterns on reducing pollutants in the air
Hence, when studying the maps and the obtained data, it can be concluded that green coverage of type, denser configuration, where the pixels are closer to each other, such as patterns 6 and 4, have contributed greatly to the reduction of PM2.5 concentrations of pollutants in the air. If both green blocks (pixels) are close to each other, then more significant impact was reported on spreading pollutants. However, the patterns which have greater distances between green blocks have demonstrated the lesser influence on dispersing pollutants. This implies that where green coverage is denser, PM2.5 is well contained or in other words, green coverage reduces PM2.5 pollutants because they offer more extensive and the persistent coverage across the building exteriors. Thus, according to this study, the increase in Densities or compactness of the green coverage by bringing the green blocks closer together can afford great contribution in mitigating air pollutants like PM2.5, improving air quality in the growing metropolitan regions.
4 Conclusion
Urbanization and high population density make cities vulnerable to several risks. Cultivable lands are shrinking and population from the rural areas is shifting to the urban areas and urban heat island is continent. Due to emergence of new climate changes, global warming, and issues related to urban sprawl, the new ideas concerning the construction business in terms of minimizing the impact on the nature should be developed. Thus, for the leadership of the environment and welfare of the society, it is possible to note that the application of green walls both in the new and existing constructions will contribute to the positive changes of the environmental parameters and prevent such threats that the modern world faces today, including climate change, the increase of the temperature in cities, carbon footprint, greenhouse effect, and pollution of the atmosphere (Figures 16, 17). Using ENVI-met software, the effectiveness of green walls in the reduction of particulate matter dispersion was evaluated and the results revealed the positive potential of the design strategy in reducing PM2.5. Such species as Pittosporum and Rosemarinus are examples of plants that can be used for green walls as they provide benefits for the look of cities and effective filtering of particles and pollutants across the year. However, using all the building facades or changing the facade materials with the green or living walls is often not possible because of implementation as well as maintenance costs. This way, applying a segment of building facades (30%) densely or applying suitable green patterns, as it is described in this research, can help enhance the quality of air in polluted cities and metropolises and make the living environment healthier and better looking for people.
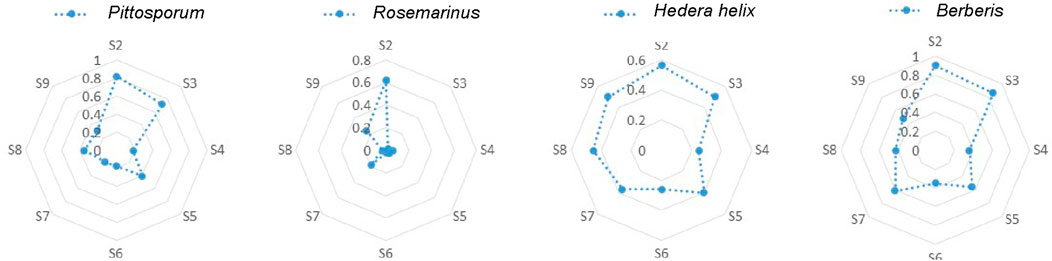
Figure 16. The concentration of Particulate Matters (PM2.5) in each scenario based on plant species (simulation result).
Data availability statement
The raw data supporting the conclusions of this article will be made available by the authors, without undue reservation.
Author contributions
MB: Data curation, Formal Analysis, Investigation, Resources, Software, Visualization, Writing–original draft. MY: Conceptualization, Methodology, Project administration, Supervision, Validation, Writing–review and editing.
Funding
The author(s) declare that no financial support was received for the research, authorship, and/or publication of this article.
Conflict of interest
The authors declare that the research was conducted in the absence of any commercial or financial relationships that could be construed as a potential conflict of interest.
Publisher’s note
All claims expressed in this article are solely those of the authors and do not necessarily represent those of their affiliated organizations, or those of the publisher, the editors and the reviewers. Any product that may be evaluated in this article, or claim that may be made by its manufacturer, is not guaranteed or endorsed by the publisher.
References
Abhijith, K. V., and Kumar, P. (2020). Quantifying particulate matter reduction and their deposition on the leaves of green infrastructure. Environ. Pollut. 265, 114884. doi:10.1016/j.envpol.2020.114884
Abhijith, K. V., Kumar, P., Gallagher, J., McNabola, A., Baldauf, R., Pilla, F., et al. (2017). Air pollution abatement performances of green infrastructure in open road and built-up street canyon environments – a review. Atmos. Environ. 162, 71–86. doi:10.1016/j.atmosenv.2017.05.014
Ahmadi, S., Yeganeh, M., Motie, M. B., and Gilandoust, A. (2022). The role of neighborhood morphology in enhancing thermal comfort and resident’s satisfaction. Energy Rep. 8, 9046–9056. doi:10.1016/J.EGYR.2022.07.042
Al-Dabbous, A. N., and Kumar, P. (2014). The influence of roadside vegetation barriers on airborne nanoparticles and pedestrians exposure under varying wind conditions. Atmos. Environ. 90, 113–124. doi:10.1016/j.atmosenv.2014.03.040
Alemardan, A., Asadi, W., Rezaei, M., Tabrizi, L., and Mohammadi, S. (2013). “Cultivation of iranian seedless barberry (berberis integerrima ’bidaneh’): a medicinal shrub,” in Industrial crops and products. doi:10.1016/j.indcrop.2013.07.061
Andersen, C. E., Hoxha, E., Nygaard Rasmussen, F., Grau Sørensen, C., and Birgisdóttir, H. (2024). Evaluating the environmental performance of 45 real-life wooden buildings: a comprehensive analysis of low-impact construction practices. Build. Environ. 250, 111201. doi:10.1016/j.buildenv.2024.111201
Ashtari, B., Yeganeh, M., Bemanian, M., and Fakhr, B. V. (2021). A conceptual review of the potential of cool roofs as an effective passive solar technique: elaboration of benefits and drawbacks. doi:10.3389/fenrg.2021.738182
Baldauf, R. (2017). Roadside vegetation design characteristics that can improve local, near-road air quality. Transp. Res. Part D Transp. Environ. 52, 354–361. doi:10.1016/j.trd.2017.03.013
Baradaran Motie, M., Yeganeh, M., and Bemanian, M. (2023). Assessment of greenery in urban canyons to enhance thermal comfort and air quality in an integrated seasonal model. Appl. Geogr. 151, 102861. doi:10.1016/J.APGEOG.2022.102861
Baraldi, R., Neri, L., Costa, F., Facini, O., Rapparini, F., and Carriero, G. (2019). Ecophysiological and micromorphological characterization of green roof vegetation for urban mitigation. Urban For. Urban Green. 37, 24–32. doi:10.1016/j.ufug.2018.03.002
Chang, L. T., and Chang, F. C. (2022). Study of Living Wall Systems’ (LWSs) Support system for improving LWSs Life cycle performance and noise reduction potential. Build. Environ. 216, 109007. doi:10.1016/j.buildenv.2022.109007
Chung, Y. S., Nam, Y., and Lee, J. H. (2018). Feasibility of ivy (hedra helix) for landscaping in seoul, korea. Ornam. Hortic. 24, 424–428. doi:10.14295/oh.v24i4.1250
Currie, B. A., and Bass, B. (2008). Estimates of air pollution mitigation with green plants and green roofs using the UFORE model. Urban Ecosyst. 11, 409–422. doi:10.1007/s11252-008-0054-y
Gallagher, J., Baldauf, R., Fuller, C. H., Kumar, P., Gill, L. W., and McNabola, A. (2015). Passive methods for improving air quality in the built environment: a review of porous and solid barriers. Atmos. Environ. 120, 61–70. doi:10.1016/j.atmosenv.2015.08.075
Ghanbari, S., Yeganeh, M., and Reza bemanian, M. (2022). Architecture typology of rural plain houses based on formal features, case study: (talesh, Iran). Front. Built Environ. 8. doi:10.3389/fbuil.2022.856567
Grabosky, J. (2018). Arboriculture: integrated management of landscape trees, shrubs, and vines. HortTechnology 9, 297–298. doi:10.21273/horttech.9.2.297
Grabowiecki, K., Jaworski, A., Niewczas, T., and Belleri, A. (2017). Green solutions-climbing vegetation impact on building -energy balance element. Energy Procedia 111, 377–386. doi:10.1016/j.egypro.2017.03.199
Grote, R., Samson, R., Alonso, R., Amorim, J. H., Cariñanos, P., Churkina, G., et al. (2016). Functional traits of urban trees: air pollution mitigation potential. Front. Ecol. Environ. 14, 543–550. doi:10.1002/fee.1426
Hop, M. E. C. M., and Hiemstra, J. A. (2013). Contribution of green roofs and green walls to ecosystem services of urban green. Acta Hortic., 475–480. doi:10.17660/ActaHortic.2013.990.61
Janhäll, S. (2015). Review on urban vegetation and particle air pollution - deposition and dispersion. Atmos. Environ. 105, 130–137. doi:10.1016/j.atmosenv.2015.01.052
Jayasooriya, V. M., Ng, A. W. M., Muthukumaran, S., and Perera, B. J. C. (2017). Green infrastructure practices for improvement of urban air quality. Urban For. Urban Green. 21, 34–47. doi:10.1016/j.ufug.2016.11.007
Jim, C. Y. (2015). Greenwall classification and critical design-management assessments. Ecol. Eng. 77, 348–362. doi:10.1016/j.ecoleng.2015.01.021
Joshi, S., Rao, A., Lehmler, H. J., Knutson, B. L., and Rankin, S. E. (2014). Interfacial molecular imprinting of Stöber particle surfaces: a simple approach to targeted saccharide adsorption. J. Colloid Interface Sci. 428, 101–110. doi:10.1016/j.jcis.2014.04.041
Junior, D. P. M., Bueno, C., and da Silva, C. M. (2022). The effect of urban green spaces on reduction of particulate matter concentration. Bull. Environ. Contam. Toxicol. 108, 1104–1110. doi:10.1007/s00128-022-03460-3
Köhler, M. (2008). Green facades-a view back and some visions. Urban Ecosyst. 11, 423–436. doi:10.1007/s11252-008-0063-x
Kookhaie, T., and Masnavi, M. R. (2014). Environmental design for ecological infrastructure of urban landscape through aggregate with outlier principle (awop) in order to enhance the quality of urban life (case of District Two, Tehran City). J. Environ. Stud.
Lelieveld, J., Evans, J. S., Fnais, M., Giannadaki, D., and Pozzer, A. (2015). The contribution of outdoor air pollution sources to premature mortality on a global scale. Nature 525, 367–371. doi:10.1038/nature15371
McConnaha, W. E. (2003). “Assessment of coho salmon habitat in an urban stream using species-habitat analysis,” in Environmental Sciences and resources.
Minks, V., Sutton, R. K., Ottelé, M., Perini, K., Fraaij, A. L. A., Haas, E. M., et al. (2017). Acoustics evaluation of vertical greenery systems for building walls. Build. Environ. 45, 411–420. doi:10.1016/j.buildenv.2009.06.017
Morakinyo, T. E., and Lam, Y. F. (2016). Simulation study of dispersion and removal of particulate matter from traffic by road-side vegetation barrier. Environ. Sci. Pollut. Res. 23, 6709–6722. doi:10.1007/s11356-015-5839-y
Morakinyo, T. E., Lam, Y. F., and Hao, S. (2016). Evaluating the role of green infrastructures on near-road pollutant dispersion and removal: modelling and measurement. J. Environ. Manag. 182, 595–605. doi:10.1016/j.jenvman.2016.07.077
Niklas, K. J. (2011). “Climbing plants: attachment and the ascent for light,” in Current biology. doi:10.1016/j.cub.2011.01.034
Othman, A. R., and Sahidin, N. (2016). Vertical greening wall as sustainable approach. Asian J. Qual. Life 1, 39. doi:10.21834/ajqol.v1i3.32
Ottelé, M., Perini, K., Fraaij, A. L. A., Haas, E. M., and Raiteri, R. (2011). Comparative life cycle analysis for green façades and living wall systems. Energy Build. 43, 3419–3429. doi:10.1016/j.enbuild.2011.09.010
Ottelé, M., van Bohemen, H. D., and Fraaij, A. L. A. (2010). Quantifying the deposition of particulate matter on climber vegetation on living walls. Ecol. Eng. 36, 154–162. doi:10.1016/j.ecoleng.2009.02.007
Palermo, S. A., and Turco, M. (2020). Green Wall systems: where do we stand? IOP Conf. Ser. Earth Environ. Sci. 410, 012013. doi:10.1088/1755-1315/410/1/012013
Pérez-Urrestarazu, L., Fernández-Cañero, R., Franco-Salas, A., and Egea, G. (2015). Vertical greening systems and sustainable cities. J. Urban Technol. 22, 65–85. doi:10.1080/10630732.2015.1073900
Perini, K., Bazzocchi, F., Croci, L., Magliocco, A., and Cattaneo, E. (2017a). The use of vertical greening systems to reduce the energy demand for air conditioning. Field monitoring in Mediterranean climate. Energy Build. 143, 35–42. doi:10.1016/j.enbuild.2017.03.036
Perini, K., Ottelé, M., Giulini, S., Magliocco, A., and Roccotiello, E. (2017b). Quantification of fine dust deposition on different plant species in a vertical greening system. Ecol. Eng. 100, 268–276. doi:10.1016/j.ecoleng.2016.12.032
Pettit, T., Torpy, F. R., Surawski, N. C., Fleck, R., and Irga, P. J. (2021). Effective reduction of roadside air pollution with botanical biofiltration. J. Hazard. Mater. 414, 125566. doi:10.1016/j.jhazmat.2021.125566
Pugh, T. A. M., MacKenzie, A. R., Whyatt, J. D., and Hewitt, C. N. (2012). Effectiveness of green infrastructure for improvement of air quality in urban street canyons. Environ. Sci. Technol. 46, 7692–7699. doi:10.1021/es300826w
Rezazadeh, H., Salahshoor, Z., Ahmadi, F., and Nasrollahi, F. (2022). Reduction of carbon dioxide by bio-façades for sustainable development of the environment. Environ. Eng. Res. 27, 200583–200590. doi:10.4491/eer.2020.583
Sharbafian, M., Yeganeh, M., and Baradaran Motie, M. (2024). Evaluation of shading of green facades on visual comfort and thermal load of the buildings. Energy Build. 317, 114303. doi:10.1016/j.enbuild.2024.114303
Shishegar, N. (2014). The impact of green areas on mitigating urban heat island effect: a review. Int. J. Environ. Sustain. 9, 119–130. doi:10.18848/2325-1077/CGP/v09i01/55081
Srbinovska, M., Andova, V., Mateska, A. K., and Krstevska, M. C. (2021). The effect of small green walls on reduction of particulate matter concentration in open areas. J. Clean. Prod. 279, 123306. doi:10.1016/j.jclepro.2020.123306
Sternberg, T., Viles, H., Cathersides, A., and Edwards, M. (2010). Dust particulate absorption by ivy (Hedera helix L) on historic walls in urban environments. Sci. Total Environ. 409, 162–168. doi:10.1016/j.scitotenv.2010.09.022
Taghizadeh, F., Mokhtarani, B., and Rahmanian, N. (2023). Air pollution in Iran: the current status and potential solutions. Environ. Monit. Assess. 195, 737. doi:10.1007/s10661-023-11296-5
Tong, Z., Baldauf, R. W., Isakov, V., Deshmukh, P., and Max Zhang, K. (2016). Roadside vegetation barrier designs to mitigate near-road air pollution impacts. Sci. Total Environ. 541, 920–927. doi:10.1016/j.scitotenv.2015.09.067
Tramontin, V., Loggia, C., and Trois, C. (2012). “Strategies for stustainable building design and retrofit in developing countries. New goals for green buildings in South Africa,” in Journal of construction.
Vélez-Landa, L., Hernández-De León, H. R., Pérez-Luna, Y. D. C., Velázquez-Trujillo, S., Moreira-Acosta, J., Berrones-Hernández, R., et al. (2021). Influence of light intensity and photoperiod on the photoautotrophic growth and lipid content of the microalgae verrucodesmus verrucosus in a photobioreactor. Sustain. Switz. 13, 6606. doi:10.3390/su13126606
Viecco, M., Jorquera, H., Sharma, A., Bustamante, W., Fernando, H. J. S., and Vera, S. (2021). Green roofs and green walls layouts for improved urban air quality by mitigating particulate matter. Build. Environ. 204, 108120. doi:10.1016/j.buildenv.2021.108120
Who, W. H. O. (2016). Ambient Air Pollution: a global assessment of exposure and burden of disease. Clean Air J.
Wieser, A. A., Scherz, M., Passer, A., and Kreiner, H. (2021). Challenges of a healthy built environment: air pollution in construction industry. Sustain. Switz. 13, 10469. doi:10.3390/su131810469
Keywords: green wall, reduction of air pollution, urban wall, plants, pattern of greenery
Citation: Borna M and Yeganeh M (2024) Analysis of the impact of growing green walls based on the reduction of PM2.5 particles in the resilient central urban fabric. Front. Built Environ. 10:1443554. doi: 10.3389/fbuil.2024.1443554
Received: 04 June 2024; Accepted: 13 August 2024;
Published: 26 September 2024.
Edited by:
Valentina Zaffaroni Caorsi, Earth and Environmental Sciences, University of Milan Bicocca, ItalyReviewed by:
Hasim Altan, Prince Mohammad bin Fahd University, Saudi ArabiaChng Saun Fong, Institute for Advanced Studies, Universiti Malaya, Malaysia
Copyright © 2024 Borna and Yeganeh. This is an open-access article distributed under the terms of the Creative Commons Attribution License (CC BY). The use, distribution or reproduction in other forums is permitted, provided the original author(s) and the copyright owner(s) are credited and that the original publication in this journal is cited, in accordance with accepted academic practice. No use, distribution or reproduction is permitted which does not comply with these terms.
*Correspondence: Mansour Yeganeh, yeganeh@modares.ac.ir