- 1Peter the Great St. Petersburg Polytechnic University St. Petersburg, Saint Petersburg, Russia
- 2Moscow State University of Civil Engineering, Moscow, Russia
- 3Peoples' Friendship University of Russia, Department of Civil Engineering, Moscow, Russia
This study investigates on the impact of basalt fiber reinforcement concrete in protected building and structures. Basalt fibers, derived from the melting of basalt rock at temperatures ranging from 1,500 to 1700°C, are recognized as sustainable and environmentally friendly fiber materials. Various studies have revealed differing optimal percentages of basalt fibers for enhancing the mechanical and chemical properties of concrete. The objectives of this paper are to investigate the effects of basalt fibre reinforcement on mechanical properties like tensile, compressive, and bending strengths. Additionally, performance indicators like void content, water absorption, chloride ion permeability, alkali and slag resistance, temperature stability, shrinkage characteristics, and abrasion resistance will be evaluated. Basalt fibre is typically utilised to increase the mechanical properties and durability of concrete, which has an impact in the effect on protected buildings and structures. The findings indicate that the most effective percentage range for improving mechanical properties lies between 0.1% and 0.3% of basalt fibers. Notably, concrete reinforced with basalt fibers demonstrates superior mechanical and chemical performance in alkaline environments compared to other fiber types. Moreover, the addition of 0.5% basalt fibers to concrete has been shown to significantly reduce chloride ion penetration, as evidenced by a decrease in RCPT load from 2,500 (C) to 1900 (C), indicative of enhanced chloride resistance. Reinforced concrete containing basalt fibers exhibits remarkable temperature resistance, withstanding temperatures exceeding 800°C due to its high-water absorption capacity. Additionally, basalt fibers exhibit resilience at temperatures up to 200°C. However, it is noted that the introduction of 0.14% basalt fibers leads to a slight increase in water absorption from 4.08 to 4.28. In general, basalt fibres are beneficial to many aspects of concrete; they strengthen resistance to temperature, alkali, acid exposure, and chloride while also improving mechanical qualities such as bending and tensile strength. The development of basalt fibres that extend building lifespans and improve concrete quality for structural engineering applications is making encouraging strides, according to all the results.
1 Introduction
One of the most advanced engineering materials of this decade is basalt fibre reinforcement concrete, which is adept at boosting strength, ductility, and durability in building structure uses. The basalt fiber naturally originates from the melting of basalt rock in the form of frozen lava at temperatures ranging from 1,500°C to 1700°C. The Moscow Research Institute of Glass and Plastic first created the fibre in 1953–1954, utilising an industrial furnace designed for melting basalt rock to create the fiber’s shape. Moreover, the first industrial production was carried out by the Ukraine Fibre Laboratory in 1985, with an emphasis on basalt fibre research (Usage of Chopped Basalt Fibres in Concrete Composites: A Review, 2017; Zhou et al., 2020).The sustainable characteristics of basalt fiber are effective factors for replacing this type of fiber with others, such as glass and steel fibers (Fiore et al., 2015). Basalt fiber is a green material known for its high efficiency and lack of toxic and non-carcinogenic effects. Notably, basalt fiber is an environmentally friendly option with a cost comparable to other fiber types. Moreover, it enhances temperature resistance, corrosion resistance, chemical properties, and mechanical properties (Karacor and Ozcanli, 2022; Shoaib et al., 2022; Tamás-Bényei and Sántha, 2023).
The construction sectors are currently adopting more green materials in an effort to protect the environment (Esparham et al., 2023). In this approach, the chemical and mechanical qualities of concrete are enhanced by the use of basalt fibre, a green reinforcement made of lava rock. Because basalt fibres can absorb high-energy electromagnetic radiation and withstand both hot and cold temperatures, adding them to concrete can protect buildings and structures from many forms of electromagnetic radiation. Basalt fibre reinforced concrete could improve building protection from various angles due to its high output energy, high tensile strength, and excellent corrosion resistance.
Basalt fibers exhibit high insulation and anti-corrosion properties, making them applicable in railway and road engineering (Di Ludovico et al., 2010). The outperform steel in mitigating chloride damage and corrosion, offering a more economically viable solution (Angst et al., 2009). Moreover, research suggests that basalt fibers surpass glass and carbon fibers in resisting corrosion due to saltwater (Wu et al., 2015). However, it is noteworthy that the strength of basalt fibers decreases by more than 80% after 90 days, although this effect is less pronounced than observed in glass and carbon fibers (Sim et al., 2005).
Basalt fiber is widely used in civil engineering, especially in the production of reinforcing materials. One investigation, for instance, considered using basalt fiber polymer bars to reinforce a timber beam, which led to an incredible increase in maximum loading of more than 16% (Gand et al., 2018).
Similarly, the reinforced timber beam is made up of several sorts of bars, such as steel, basalt, and glass. Their results showed that steel bars increased maximum loading by 57%, basalt bars by 32%, and glass bars by 27% (Wdowiak-Postulak et al., 2023). When basalt rebar was used to determine a beam’s maximum loading capacity, it was discovered that the capacity was increased by ribbed, fiber-free basalt rebar (Li et al., 2024). Additionally, researchers investigated the use of fiber-wrapped ribbed basalt fiber polymer rebar for reinforcing sea-sand concrete. Their results showed how crucial geometric parameters are to achieve the best outcomes. In addition, bond strength increased in the expanded clay concrete reinforced with basalt fiber 15 from 13.13 MPa to 20.34 MPa. It is understood that the reinforced concrete can increase the compressive strength more than 66%. The effects of adding basalt fiber-reinforced polymer (BFRP) and glass fiber-reinforced polymer (GFRP) to a concrete mixture design that included metakaolinite and zeolite were investigated in a related study (Krassowska et al., 2023).
The results indicated a 20% improvement in adhesion stress with GFRP and an increase of more than 15% with BFRP. The study evaluated how the mechanical characteristics of tailing mortar were affected by basalt and polyacrylonitrile fibers (W et al., 2023). The researchers found that the compressive, splitting tensile, and flexural strengths were raised by adding 0.5% polyacrylonitrile fiber and 25% basalt fiber. Using basalt fibers to improve the mechanical properties of pea gravel concrete is yet another illustration (Sun and Xu, 2009). It was discovered that the addition of 0.4% of 12-mm-long basalt fibers to pea gravel concrete increased the concrete’s compressive strength by over 44%. Another study examined adding nanomaterial cellulose pellets and chopped basalt fiber with a cementitious matrix to find new mechanical properties (Zhang et al., 2018). It is understood that mechanical properties such as compressive strength increased more than their prediction. Based on their findings, basalt fiber minibars can enhance tensile strength by more than 4.18–6 times and flexural strength by more than 12–26 times (Fu et al., 2021).
To evaluate changes in the mechanical properties of recycled concrete, basalt fiber dosages of 0, 0.1, 0.3, and 0.5% are incorporated into the reinforced recycled concrete with basalt fibre (Chen and Chen, 2023). The findings indicated that concrete with 0.5% basalt fiber and 100% recycled aggregates exhibited the best compressive strength and a 90% improvement in tensile strength. Other studies have explored hybrid-reinforced concrete, combining basalt and various fibers, to analyze mechanical and durability aspects in salty environments. For the study of reinforced concrete with basalt fibers with recycled aggregates, the results show that the dose of basalt fibers with 1% is the optimal percentage for flexural strength. Compared to the control concrete containing 100% recycled aggregates and without basalt fibers, 1% basalt fibers resulted in a 31.6% decreasing in drying shrinkage (Jiao et al., 2022). The results demonstrated that hybrid basalt–polypropylene fiber-reinforced concrete outperformed conventional concrete (Zhang, 2017; Zhang et al., 2018; Sun et al., 2019; Fu et al., 2021; Klyuev et al., 2023).
The research indicates that adding a significant percentage of basalt fiber, around 1%, enhances the performance of recycled aggregate concrete. Moreover, research has been done on the effects of basalt fiber on recycled aggregate concrete (CSA S806-12 Design and Construction of Building Structures with Fibre-Reinforced Polymers, 2012). It is concluded from the results that basalt fiber enhances the ductility of concrete while simultaneously improving its microstructure and interfacial transition zone. According to Monaldo et al. (Monaldo et al., 2019), basalt fiber rebar could cut anthropogenic emissions by over 62% when compared to steel rebar. Additionally, concrete reinforced with basalt fiber rebar reduces shear resistance and crack width (ACI 440.1R-15, 2015 Guide for Design and Construction of Structural Concrete Reinforced with Fiber-Reinforced Polymer FRP Bars, 2015; CSA S806-12 Design and Construction of Building Structures with Fibre-Reinforced Polymers, 2012).
Self-consolidating concrete (SCC) used in bridge decks was examined for the effects of basalt fibre (Sadrmomtazi et al., 2018). It was found that basalt fiber greatly increases shear resistance (Hassan et al., 2000). Looked at the usage of basalt fiber to reinforce recycled concrete aggregates. Their study involved replacing recycled aggregates with 0%, 50%, and 100%. Additionally, basalt fiber was added at 0 kg/m3, 2 kg/m3, and 4 kg/m3. The results indicated an increase in maximum loading capacity with the rising content of basalt fiber. However, beyond 2 kg/m3 to 4 kg/m3, the maximum capacity of concrete decreased, according to their findings.
Research indicates that basalt fiber can enhance the chemical properties of concrete, exhibiting robust resistance against corrosion, heat, alkali, and acid (Sim et al., 2005). The three-dimensional irregular network formed by basalt fibers is interconnected with the cement matrix and aggregates (Monaldo et al., 2019). Studies reveal that concrete reinforced with basalt fibers demonstrates superior bonding strength compared to steel rebar in alkaline environments (Taha et al., 2020). Basalt fibers exhibit enduring chemical stability when immersed in mild alkali conditions (Lee et al., 2014). To analyze the durability of agricultural waste walnut shells reinforced by basalt fiber (Militk et al., 2002). Results showed that adding chopped basalt fiber with a dose of 0.6% and a length of 6 mm to a mortar with a compressive strength of over 13%, 48%, and 43%, respectively, improved splitting tensile strength and flexural strength.
The addition of basalt fibers to concrete is shown to increase electrical resistance and improve chemical properties. The Rapid Chloride Penetration Test (RCPT) is employed to assess the chemical properties of concrete (Sadrmomtazi et al., 2018). The utilisation of basalt fibres in concrete is a crucial factor in improving its porosity (Hrynyk and Frydrych, 2015). Additionally, (Novitskii, 2004), discovered that basalt fibres are more resistant to chloride than polypropylene fibres. Moreover, (Buratti et al., 2015), examined the basalt fibre concrete’s resistance to attacks by the sulfate-magnesium combination. The flexural strength of the concrete was raised by more than 16% when 0.5% of basalt fiber was added, as per their findings. Also, the use of basalt fibers in concrete spacecraft limits the propagation of cracks, and the sulfate attack in concrete is also reduced, which increases its durability. Certain studies examined reinforced concrete with basalt fibers to boost the concrete’s resilience to heat treatment. The assessment of the addition of basalt fiber is necessary in order to gain further insight into the heat resistance of concrete. The flexural strength of basalt fiber reinforced concrete is affected by the reduction in basalt fiber size that occurs after 180 min of exposure to a temperature of 300°C. For instance, (Li et al., 2020) investigate how heat treatment improves the mechanical parameters of basalt fiber-reinforced concrete, such as flexural strength, when compared to other samples.
Basalt fiber presents numerous advantages in engineering and civil engineering. Literature supports the idea that basalt fiber can enhance both mechanical and chemical properties. This study examines the impact of basalt fibers on concrete and points to its capacity to change mechanical properties, including compressive, tensile, and bending strengths as well as chemical resistance. The aim of the research is to demonstrate how adding basalt fibres to concrete can strengthen its mechanical and chemical qualities, hence improving the structure’s protection. To enhance mechanical and chemical quality, basalt fibre addition in the right amount is therefore essential.
2 Methods
In this study, the focus initially centered on the examination of basalt fibers. The fundamental characteristics and potential uses of basalt fibers, specifically in the field of civil engineering, were important areas of research in the beginning stage. Subsequently, the investigation delved into assessing the impact of basalt fibers on concrete. The following phase examined various aspects of concrete performance, as illustrated in Figure 1, such as void content, water absorption, chloride ion permeability, resistance to corrosion and alkali, resistance to shrinkage, abrasion resistance, and mechanical qualities. Collectively, this study sheds light on both the intrinsic properties of basalt fibers and their influence on concrete. Figure 1 demonstrates the way this study uses basalt fibres to improve the quality of concrete, which increases the life expectancy of buildings and structures that are protected.
3 Basalt fiber
3.1 The nature of basalt fiber
Basalt fiber is made by melting the natural volcanic basalt fiber rock at a temperature between 1,450 and 1,500°C. For the first time, the Moscow Research Institute of Glass and Plastic have used the basalt fibers. Melted basalt rock must be passed through crucible bushing consisting of rhodium and platinum to produce basalt fiber. Due to the distinctive properties of basalt fiber, the chopped form of this fiber has been utilized in building materials and various industrial applications. Moreover, basalt fiber can serve as a replacement for other types of fibers, such as glass and carbon fibers. Other uses of basalt fiber include electrical insulation, automotive parts, sports items, fire protection, and so on (Dhand et al., 2015). Tables 1, 2 illustrate the mechanical and chemical properties of basalt fibers. One advantage of basalt fiber is its reasonable cost, especially when compared to glass and carbon fibers, considering their chemical properties (Banibayat and Patnaik, 2014; Sadrmomtazi et al., 2018). Basalt fiber is utilized in the production of personal protective equipment due to its high thermal resistance, ranging from −260°C to +800°C, and its low moisture absorption. Additionally, basalt fiber exhibits resistance to alkalis and acids. Moreover, it possesses non-flammable properties and can withstand exposure to flames (Militk et al., 2002; Hrynyk and Frydrych, 2015; Miskiewicz et al., 2019).
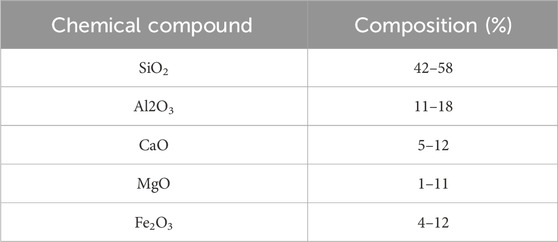
Table 1. Basalt fiber chemical properties (Banibayat and Patnaik, 2014; Sadrmomtazi et al., 2018).
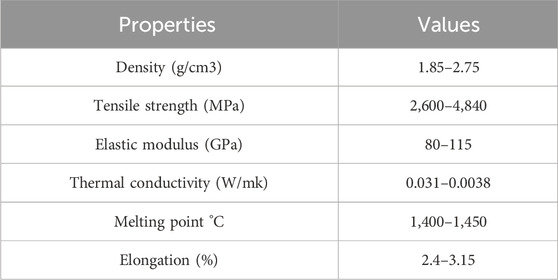
Table 2. Basalt fiber mechanical properties (Banibayat and Patnaik, 2014; Sadrmomtazi et al., 2018).
3.2 The application of basalt fiber in civil engineering
Due to its capacity to improve the mechanical and chemical properties of materials, basalt fibers have found extensive applications in the field of civil engineering. These fibers serve various functions, including insulation, sound absorption, and augmenting concrete’s resistance to heat, among other properties pertinent to concrete, owing to their use as additives (Novitskii, 2004; Buratti et al., 2015). For instance, (Wang et al., 2020) demonstrated that the incorporation of 0.4% basalt fiber content stabilizes soil when combined with cementitious materials. Their findings suggest that the addition of basalt fiber and cement offers a novel approach to soil stabilization in geotechnical engineering.
In a similar vein, (Saloni et al., 2020), investigated the effects of different proportions of basalt fiber (ranging from 0% to 100% by binder mass) added to geopolymer paste as a replacement for rice husk ash silica. The research aimed to evaluate the geopolymer paste’s ensuing mechanical qualities. Notably, the chemical composition of basalt fiber primarily comprises SiO2 and CaO. The synergistic interaction between SiO2 and CaO is believed to enhance the mechanical properties of geopolymer paste, particularly its compressive strength. The outcomes revealed that basalt fiber not only enhances mechanical properties such as bulk density, compressive strength, and flexural strength but also improves the chemical resistance of geopolymer paste.
Basalt fiber has demonstrated favorable effects on the mechanical properties of asphalt. (Mohaghegh et al., 2018) investigated the impact of basalt fiber on the interfacial bond of asphalt binder, concluding that basalt fiber positively influences mechanical properties. Similarly, (Wang et al., 2019), determined the optimal basalt fiber length and percentage for porous asphalt mixture to be 9 mm and 0.3%, respectively. Also, basalt fiber is used in textile-reinforced concrete (TRC), which increases the hardness of the cement matrix. The incorporation of chopped basalt fiber improves TRC’s load-bearing capacity due to its inherent brittleness, complementing the brittleness of concrete (Du et al., 2018; Li et al., 2019). The author (Tumadhir, 2013), introduced basalt fiber to enhance compressive strength, finding that 0.3% basalt fiber content yielded optimal results, albeit with a slight reduction in compressive strength upon fiber addition. The influence of basalt fibers extends to the mechanical and chemical properties of concrete, including compressive, tensile, and flexural strengths (Hasanzadeh et al., 2022; Hematibahar et al., 2022; Kharun et al., 2022).
4 Basalt fiber results on concrete
4.1 Workability
The workability of concrete significantly impacts its placement and overall composite performance. However, the addition of fibers can lead to a decrease in concrete workability, with the extent of reduction dependent on fiber dosage and length (Li and Li, 2013; De Figueiredo and Ceccato, 2015). For instance, (Niu et al., 2020) observed a decrease in concrete slump with the addition of basalt fiber. Their experiments revealed that as the basalt fiber dosage increased (0.05%, 1%, 0.15%, and 0.2%), the slump decreased by more than 26%, 51%, 58%, and 67%, respectively. This reduction can be attributed to the high surface area of basalt fiber, which reduces cohesion between concrete components, consequently increasing the viscosity of the concrete matrix (Katkhuda and Shatarat, 2017). Similarly, (Borhan, 2012), demonstrated that adding 0.5% of basalt fiber led to a reduction in concrete slump. Furthermore, Kabay (Kabay, 2014) investigated the effect of basalt fiber length (12 mm and 24 mm) and dosage (0.07% and 0.14%) on concrete slumps. When the basalt fiber length was 12 mm, the slump was 110 mm and 150 mm for 0.07% and 0.14% fiber percentages, respectively. However, for fiber lengths exceeding 24 mm, the slump exceeded 100 mm for both fiber percentages. Additionally, (Sadrmomtazi et al., 2018), analyzed the influence of basalt fiber dosage on cementitious mixtures, noting a reduction in mortar matrix flowability with increased fiber dosage. Comparatively, other fiber types such as steel, polypropylene (PP), and polyvinyl alcohol (PVA) also decrease concrete workability when added to cementitious mixtures (Jiang et al., 2014; Bouziadi et al., 2016; Afroughsabet et al., 2018). For instance, (MO et al., 2019), studied the effect of PVA fibers on concrete workability, observing slump reductions of more than 5.3%, 20.2%, and 29.8% with the addition of 1%, 1.5%, and 2% PVA fibers, respectively. Notably, workability reductions with the addition of basalt fibers were much more significant, surpassing 29.7% and 40.8% for 1% and 2% basalt fiber additions, respectively. Table 3 provides a summary of slump results from various studies in this section (Kabay, 2014; Katkhuda and Shatarat, 2017; Niu et al., 2020).
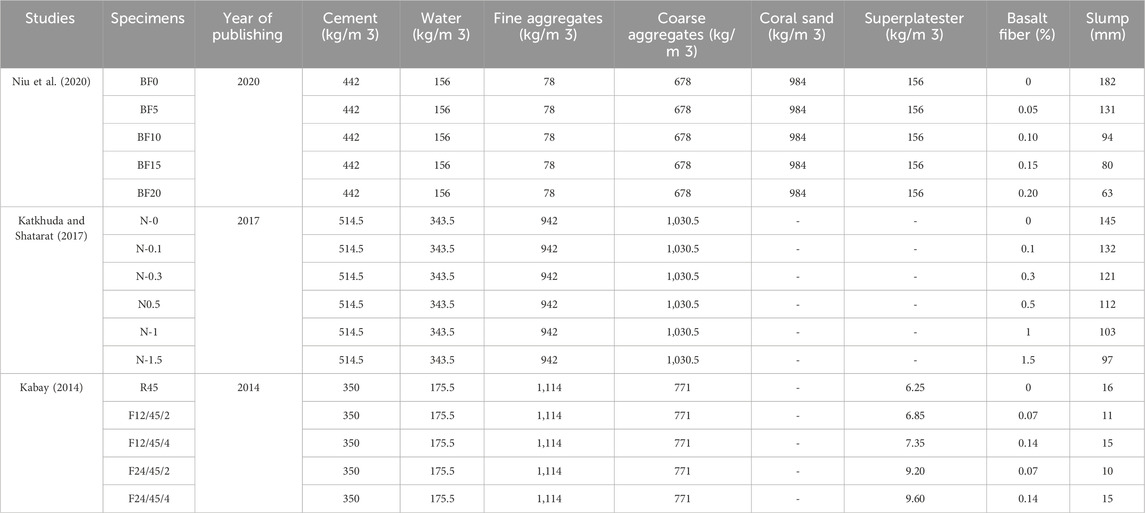
Table 3. The Slump results of basalt fiber reinforced concrete (Kabay, 2014; Katkhuda and Shatarat, 2017; Niu et al., 2020).
4.2 Void content of basalt fiber concrete
The addition of fibers to concrete introduces voids and porosity into the cementitious matrix (Guo et al., 2019). Observed that the inclusion of basalt fiber in concrete led to an increase in porosity within the cementitious matrix. This increase in voids and porosity due to basalt fiber may contribute to a decrease in workability. In contrast, (Zheng et al., 2022) noted that as the percentage of basalt fiber increased, the voids within the concrete slightly decreased.
4.3 Water absorption
It has been discovered that increasing the dose of basalt fiber helps the cementitious matrix absorb more water, which is a crucial component (Behfarnia and Behfarnia, 2014). For instance, when the water absorption of the concrete was raised from 3.2% to 4.3% by adding 0.07% and 0.14% of 12 mm-long basalt fiber, respectively. Comparable results were also obtained, indicating that the water absorption of concrete reinforced with 1% and 1.5% basalt fiber was 33% and 57% higher, respectively, than that of unreinforced concrete.
The addition of basalt fiber to self-consolidating concrete (SCC) increased water absorption was studied (Algin and Ozen, 2018). Considering an increase in water penetration with the addition of basalt fiber, attributing it to the surface characteristics of the basalt fiber and the ITZ.
In contrast to PP fibers, basalt fiber could increase water absorption by opening voids in the cementitious matrix. Research indicates that the optimal dosage for adding PP fiber to concrete is 0.7 kg/m³ (Ramezanianpour et al., 2013; Behfarnia and Behfarnia, 2014). According to (Afroughsabet and Ozbakkaloglu, 2015), steel fibers can decrease the amount of water that concrete absorbs, giving outcomes that are comparable to those of PP fibers. Table 4 presents the water absorption results of this section (Kabay, 2014; Afroughsabet and Ozbakkaloglu, 2015). Water absorption of concrete reinforced with basalt fibers can improve the ability of concrete against high temperature and fire conditions in the building, so adding basalt fibers in concrete improves the protection of buildings against fire and high temperature.
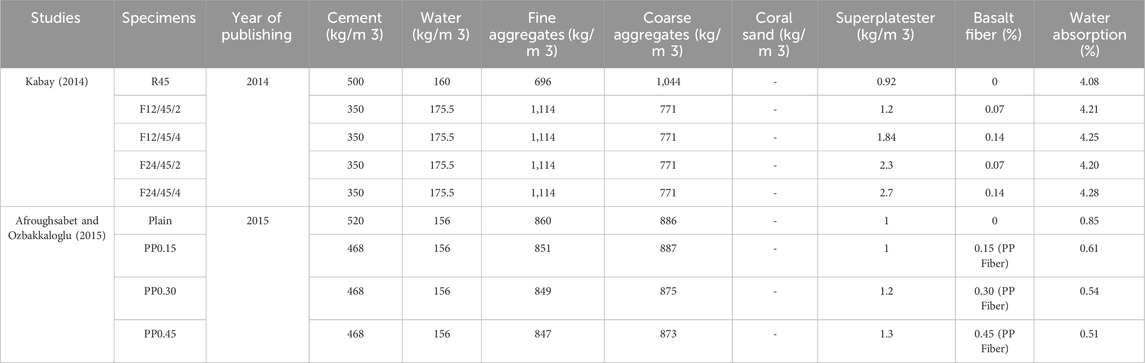
Table 4. The basalt fiber reinforced concrete water absorption (%) (Kabay, 2014; Afroughsabet and Ozbakkaloglu, 2015).
4.4 Chloride ion permeability
The rapid chloride penetration test (RCPT) is an effective method for assessing chloride ion penetration in cementitious composites, conducted in accordance with ASTM 1202 (ASTM, Standard Test Method for Electrical Indication of Concrete’s Ability to Resist Chloride, C 1202-07, 2009). This test measures the total charge passing through a cubic concrete specimen over 6 hours.
The impact of adding basalt fiber to concrete on RCPT results has been investigated (Guo et al., 2019). According to the study, hydration caused the charge communicated to drop over time, even though it initially rose due to voids in the concrete. However, the addition of basalt fiber had a negative impact on the charge transmitted, ultimately leading to low chloride ion penetrability, particularly evident in concrete samples cured for 28 and 56 days. Similar outcomes were obtained when basalt fiber was added to SCC; a higher dosage of basalt fiber resulted in a lower level of chloride permeability (Algin and Ozen, 2018). The water absorption findings for this region are shown in Table 5 (Guo et al., 2019).
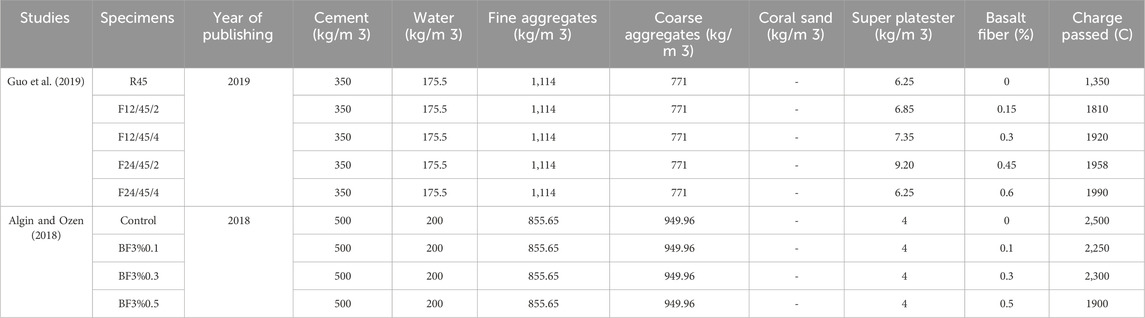
Table 5. The basalt fiber reinforced concrete water absorption (%) (Algin and Ozen, 2018; Guo et al., 2019).
4.5 Alkali and corrosion resistance
While there is no specific evidence directly linking basalt fiber to alkali environmental resistance, some studies suggest that basalt fiber may enhance resistance to alkali environments (Ramachandran et al., 1981; Wang et al., 2008). The concrete reinforced with basalt fiber exhibited decreased mass and strength when immersed in a 1 M sodium hydroxide solution (Lee et al., 2014). The prolonged immersion of basalt fiber in sodium hydroxide solution resulted in surface flaking was studied in (Sim et al., 2005). The studies conducted by (Ramachandran et al., 1981) indicated that basalt fiber demonstrated more excellent resistance to alkali environments compared to acid environments. The modified basalt fiber to enhance its resistance to alkali and chemical environments was considered (Li, et al., 2020). Some studies have explored chemical treatment methods to improve the chemical properties of basalt fiber concrete (Lipatov, et al., 2015; Li, et al., 2020). The addition of modified basalt fiber increased the flexural strength of concrete was observed (Nováková et al., 2018). The coated basalt fiber with zirconia has increased its corrosion resistance properties (Yousefieh et al., 2017). Overall, incorporating basalt fiber in concrete to enhance corrosion resistance yielded superior results compared to other fiber types, such as steel and carbon fiber (Wu et al., 2015). The results of alkali and corrosion resistance show that basalt fibers can improve the protection of concrete buildings and structures against alkali resistance.
4.6 Temperature resistance
Basalt fiber can be utilized in concrete exposed to high temperatures (Cerny et al., 2009; Wu et al., 2015). Its incorporation in concrete enhances the material’s temperature stability. Basalt fibers strengthen the concrete’s resistance to high temperatures by increasing its crystallinity (Li, et al., 2020). Study findings showed that, at 250°C, concrete with 2% more basalt fibers was lighter than concrete without any basalt fibers (Felekoglu et al., 2007). Additionally, basalt fiber-reinforced concrete exhibited fewer cracks than unreinforced concrete when exposed to temperatures exceeding 800°C (Shaikh and Taweel, 2015). For instance, the basalt fiber concrete significantly enhanced fire resistance for temperatures exceeding 600°C (Nováková et al., 2004). Furthermore, basalt fiber exhibits superior stability in fire resistance compared to steel and glass fibers.
4.7 Shrinkage properties
Short fibers can effectively mitigate the impact of shrinkage in cementitious matrices when dispersed evenly throughout the material (Yousefieh et al., 2017; Adesina, 2020). A study on concrete reinforced with basalt fibers found that integrating 3% of 25 mm basalt fibers resulted in a considerable reduction in plastic shrinkage (Al-Majidi, et al., 2017). The study found that the percentage and length of basalt fibers correspond with a reduction in plastic shrinkage. Furthermore, the bridging effect of basalt fibers in concrete helps to reduce concrete plastic shrinkage strain. Similarly, basalt fibers provide a bridging action that lessens drying shrinkage and helps to control volumetric changes (Ramachandran et al., 1981). Research has also looked into using different fibre types, such as polypropylene (PP), to mitigate drying shrinkage—for example, the study of the use of PP fibers to reduce drying shrinkage (Li et al., 2002). Several doses of PP fibers were used, including 0.45 kg/m3, 0.9 kg/m3, and 1.35 kg/m3, resulting in drying shrinkage reductions of more than 43.0%, 51.9%, and 68.3%, respectively.
4.8 Abrasion resistance
The Bohme test, as outlined in EN 1338, serves as a standardized method for assessing abrasion resistance (Classification et al., 2013). Research indicates that the abrasion resistance of concrete is influenced by the water-cement ratio when basalt fibers are added. Specifically, a water-cement ratio of 0.6 tends to decrease abrasion resistance, whereas a ratio of 0.45 enhances it. Additionally, regardless of the water-cement ratio, the dosage of basalt fibers plays a crucial role in improving abrasion resistance. Overall, basalt fibers exhibit superior performance in terms of abrasion resistance compared to steel and polypropylene (PP) fibers (Felekoglu et al., 2007; Sun and Xu, 2009; Ganesan et al., 2015; Kharun et al., 2020).
4.9 Mechanical properties
4.9.1 Compressive strength
Numerous studies have investigated the impact of basalt fiber on the compressive strength of concrete, revealing divergent findings regarding its effectiveness. While some research suggests that specific percentages of basalt fiber enhance compressive strength (Algin and Ozen, 2018; Hassani Niaki et al., 2018; Guo et al., 2019), others indicate a decrease (Kabay, 2014; Sadrmomtazi et al., 2018; Guo et al., 2019; Li, et al., 2020; Sarkar and Hajihosseini, 2020). For example, after basalt fibre reinforcement, a certain amount of basalt fibre can raise the compressive strength of the concrete (Du et al., 2020). The varying fiber lengths and percentages can positively influence compressive strength, as depicted in Figure 2, where compressive strength increased with the addition of 12 mm fibers at a 0.5% percentage (Algin and Ozen, 2018). According to their findings, the optimal percentage of basalt fiber is 0.1%.
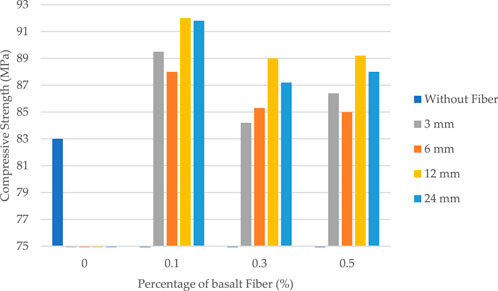
Figure 2. Compressive strength results (based on data from Algin and Ozen, 2018).
Compressive strength was observed to increase at levels greater than 0.1% while decreasing at lower concentrations (Katkhuda and Shatarat, 2017). Compressive strength was observed to increase at levels greater than 0.1% while decreasing at lower concentrations (Ramachandran et al., 1981). The addition of basalt fiber to high-performance concrete (HPC) with 12 mm and 22 mm fibers was studied, and 0.2% was shown to be the ideal proportion for reaching maximum compressive strength (Li and Li, 2013).
The assessment of concrete’s compressive strength with the inclusion of both basalt fiber and modified basalt fiber in alkaline environments, as illustrated in Figure 3 (Li, et al., 2020). Notably, the addition of modified basalt fiber led to an increase in the compressive strength of concrete. The optimal percentage of modified basalt fiber was determined to be 0.1%, resulting in a compressive strength of 56.7 MPa.
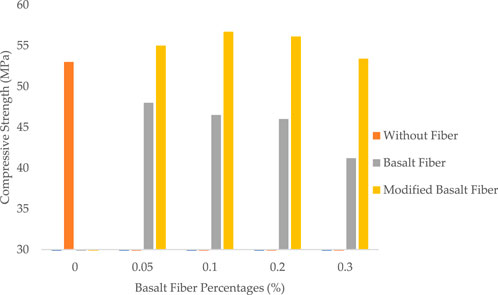
Figure 3. Compressive strength results (based on data from Li et al., 2020).
When compared to PP fiber, basalt fiber possessed more of an effect on compressive strength (Jiang et al., 2014). While PP fiber enhanced the resistance to crack formation, the addition of PP fiber to concrete resulted in a decrease in compressive strength. Similarly, (Ramezanianpour et al., 2013) demonstrated a reduction in compressive strength upon the incorporation of PP fiber into concrete.
4.9.2 Splitting tensile strength
The splitting tensile strength, as per ASTM C 496 (ASTM C496/C496M-17, Standard Test Method for Splitting Tensile Strength of Cylindrical Concrete Specimens, 2017) standard, has been extensively investigated to assess the impact of fiber addition on concrete and cementitious composites. Numerous studies have indicated optimal percentages of basalt fiber that lead to enhanced tensile strength (Li et al., 2002; Al-Majidi, et al., 2017; Momoh and Osofero, 2021). Research findings suggest that the tensile strength can experience a notable increase with the addition of more than 1.5% of basalt fiber, by over 25% (Katkhuda and Shatarat, 2017). The incorporation of basalt fiber can bolster the tensile strength owing to its effect on the cementitious matrix (Ramachandran et al., 1981). Furthermore, the tensile strength tends to improve with the augmentation of both dosage and length of basalt fiber. Borhan (Borhan, 2012) noted that the addition of 0.3% basalt fiber yielded the most significant improvement in tensile strength, attributed to its bridging effect on the concrete.
Figure 4 shows the results of tests that included adding basalt fiber to SCC (Algin and Ozen, 2018). According to their results, the optimal percentage of basalt fiber was found to be between 0.5% and 0.30% and the optimal length was 12 mm and 24 mm.
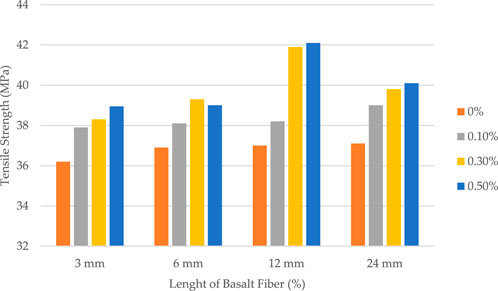
Figure 4. Tensile strength results (based on data from Algin and Ozen, 2018).
In a separate investigation, the utilization of 2% basalt fiber was found to enhance the mechanical properties of concrete. According to the study (Hassani Niaki et al., 2018), the basalt fibre content of 2% in concrete produced the best tensile strength findings at 18.5 MPa. However, exceeding this threshold by adding more than 2% basalt fiber to the specimens led to a decline in the mechanical properties of the concrete. The test results are illustrated in Figure 5.
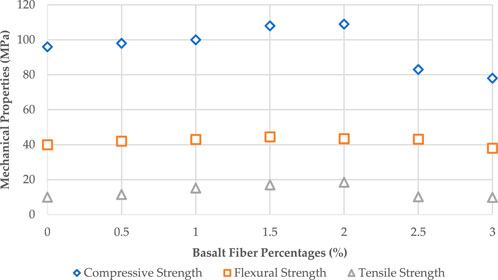
Figure 5. Tensile strength results (based on data from Hassani Niaki et al., 2018).
Furthermore, it has been observed that adding basalt fiber to concrete exposed to alkaline environments increases its tensile strength by more than 15%; in particular, tensile strength increased by 15% when 0.3% of 10 mm-long basalt fiber was added to the concrete (Li, et al., 2020).
4.9.3 Flexural strength
The flexural strength of concrete reinforced with basalt fibers was examined by (Katkhuda and Shatarat, 2017). In incorporated varying percentages of basalt fiber—0.1%, 0.5%, 1%, and 1.5%—and observed improvements in flexural strength of more than 8%, 34%, 39%, and 75%, respectively.
More dosages and longer lengths of basalt fiber are correlated with improved flexural strength, according to the findings of (Jiang et al., 2014). Similarly, (ACI 440.1R-15 Guide for Design and Construction of Structural Concrete Reinforced with Fiber-Reinforced Polymer FRP Bars, 2015), observed a 60% improvement in flexural strength with a dosage of 1.5% basalt fiber in the cementitious matrix. The dosages of 0.5% basalt fiber at lengths of 36 mm and 50 mm increased flexural strength by more than 22% and 26%, respectively (Jiang et al., 2014). The optimal length and percentage of basalt fiber to enhance flexural strength are 30 mm and 0.3%, respectively (Wang and Zhang, 2010). As to the review, increasing bending strength is the most efficient way to utilise basalt fibres. It is worth mentioning that the load bearing capacity of concrete beams in buildings can be enhanced by the incorporation of basalt fibres.
The length of basalt fiber does not significantly affect flexural strength, while optimal percentages are found at 0.3% and 0.5% additional basalt fiber as shown in Figure 6 (Algin and Ozen, 2018). Research also indicates improvements in flexural strength of more than 20% with the addition of 0.1% basalt fiber to concrete in alkali environments (Li, et al., 2020). The effect of basalt fiber can stabilize flexural strength in geopolymer concrete compared to cementitious concrete (Dias, and Thaumaturgo, 2007).
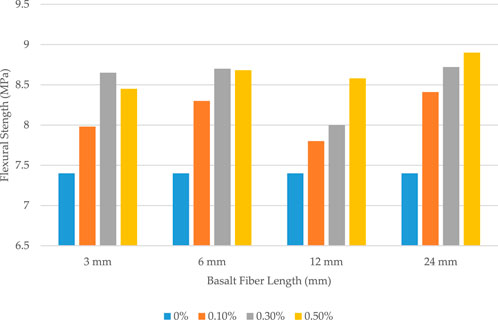
Figure 6. Flexural strength results (based on data from Algin and Ozen, 2018).
Figure 7 presents the effectiveness of various percentages and dosages of basalt fiber in improving the flexural strength of concrete. According to the findings, an optimal percentage of 0.6% basalt fiber is recommended (Guo et al., 2019).
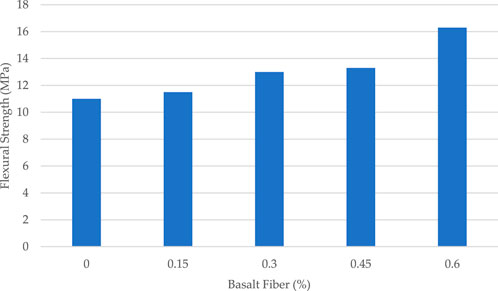
Figure 7. Flexural strength results (based on data from Guo et al., 2019).
4.10 Summary of finding
According to Table 6, when basalt fibers are added to any type of concrete, the flexural and tensile strength increases, while the compressive strength decreases or at least increases. Table 6 shows that the optimal percentage of basalt fibers for adding concrete is 0.5% to improve bending and tensile strength and for compressive strength is between 0.05% and 0.15%. Another aspect of basalt fiber addition is the reduction of chloride ion resistance due to the formation of harmful pores (200 nm) from the basalt fibers.
Figure 8 shows that when 0.1% basalt fibers are added to concrete, the compressive strength increases, but when 0.3% basalt fibers are added to concrete, the compressive strength decreases, Table 6 also proves these results. According to Table 6, the compressive strength increases with certain percentages of basalt fibers and decreases with the addition of basalt fibers. While the addition of more PP and steel fibers improved the compressive strength.
Differnces between compressive strength and flexural strength when basalt fiber added to concrete is increasing flexural strength with adding basalt fiber, while when basalt fiber add to concrete the compressive strength decrease. When 1.5% of basalt fiber was added to concrete, the flexural strength increased by more than 41%; when 0.55 of basalt fiber was added to concrete, the flexural strength improved by more than 12%, as shown in Figure 9.
Despite compressive and flexural strengths, tensile strength has a different situation, as shown in Figure 10. Adding 0.1%, 0.3%, and 0.6% of basalt fiber to concrete had maximum tensile strength (Algin and Ozen, 2018). Adding 0.3% and 0.5% of basalt fiber were optimal percentages for improvement of tensile strength (Guo et al., 2019).
5 Conclusion
This article summarized the research results on the mechanical and chemical properties of basalt fibers in reinforced concrete led to the following key conclusions. Research on basalt fibres still needed to address a few issues that remain to be addressed. The following could be used to sum up the current issues and potential future paths for basalt fibre research:
• In civil engineering applications, basalt fibres present a competitive alternative to typical fibres due to their superior mechanical and chemical qualities and affordability. Basalt fibres improve the performance of concrete, according to testing studies, albeit the ideal dosage differs depending on the investigation.
• Basalt fibers exhibit a substantial chemical composition characterized by high levels of SiO2, Al2O3, and CaO. Furthermore, the basalt fibers have a mechanical properties, with tensile strength ranging from 2,600 to 4,840 MPa and elasticity modulus between 80 and 115 GPa. Increasing the dosage of basalt fibers in concrete leads to a reduction in efficiency and an increase in void content. The introduction of basalt fibers may also introduce air into the concrete, potentially diminishing its effectiveness.
• The mechanical properties of concrete are intricately linked to the dosage of basalt fibers, with optimal percentages ranging between 0.1% and 0.2% for compressive strength, 0.2% for splitting tensile strength, and 0.3%–0.5% for flexural strength. Concrete water absorption exhibits marginal increases with the addition of 0.14% basalt fibers, while the incorporation of 0.45% PP fibers results in a significant reduction in water absorption. Basalt fiber concrete demonstrates exceptional temperature resistance, exceeding 800°C. Results from rapid chloride penetration tests (RCPT) indicate that the addition of 0.5% basalt fibers can reduce charge transmission from 2500 C to 1900°C, thereby enhancing the impermeability of concrete. The present work demonstrates that the addition of basalt fibres into concrete enhances its chemical and mechanical properties, suggesting that such concrete could potentially find application in specially designed protected buildings and structures.
• Basalt fibers confer improvements in the mechanical properties of concrete, particularly in alkaline environments, demonstrating resistance to alkali exposure. In conclusion, the findings of this study underscore the significant potential of basalt fibers in enhancing the performance and durability of concrete structures. However, further research is warranted to address remaining knowledge gaps and optimize the utilization of basalt fibers in concrete applications. Future investigations should focus on elucidating the mechanisms underlying the observed effects and exploring innovative approaches to harness the full potential of basalt fibers in civil engineering. The research on basalt fibres is currently restricted to laboratory analysis. In order to bring together the laboratory study and apply it to real-world engineering, more investigation and development are therefore required.
• The addition of basalt fibres to any kind of concrete results in an increase in its flexural, compressive, and tensile strengths, according to a summary of each study that highlights the key findings. This article analysed the effects of basalt fibres on the flexural, compressive, and tensile strengths of several types of concrete and summarised research findings related to this topic. Research on basalt fibres still needed to address a few issues that remained to be resolved.
Author contributions
NV: Conceptualization, Funding acquisition, Methodology, Project administration, Resources, Supervision, Validation, Visualization, Writing–review and editing. MH: Conceptualization, Data curation, Formal Analysis, Investigation, Methodology, Resources, Software, Supervision, Visualization, Writing–original draft. TG: Data curation, Formal Analysis, Investigation, Methodology, Software, Supervision, Validation, Visualization, Writing–review and editing.
Funding
The author(s) declare that financial support was received for the research, authorship, and/or publication of this article. This research was funded by the Ministry of Science and Higher Education of the Russian Federation within the framework of the state assignment No. 075-03-2022-010 dated 14 January 2022 and No. 075-01568-23-04 dated 28 March 2023 (Additional agreement 075-03-2022-010/10 dated 09 November 2022, Additional agreement 075-03-2023-004/4 dated 22 May 2023), FSEG-2022-0010.
Conflict of interest
The authors declare that the research was conducted in the absence of any commercial or financial relationships that could be construed as a potential conflict of interest.
Publisher’s note
All claims expressed in this article are solely those of the authors and do not necessarily represent those of their affiliated organizations, or those of the publisher, the editors and the reviewers. Any product that may be evaluated in this article, or claim that may be made by its manufacturer, is not guaranteed or endorsed by the publisher.
References
ACI 440.1R-15 (2015) Guide for design and construction of structural concrete reinforced with fiber-reinforced polymer (FRP) bars. Farmington Hills, MI, USA: American Concrete Institute.
Adesina, A. (2020). Performance of fibre reinforced alkali-activated composites – a review. Materialia 12, 100782. doi:10.1016/j.mtla.2020.100782
Afroughsabet, V., Biolzi, L., and Monteiro, P. J. M. (2018). The effect of steel and polypropylene fibers on the chloride diffusivity and drying shrinkage of high-strength concrete. Compos. B Eng. 139, 84–96. doi:10.1016/j.compositesb.2017.11.047
Afroughsabet, V., and Ozbakkaloglu, T. (2015) Mechanical and durability properties of high strength concrete containing steel and polypropylene fibers, 73–82.
Algin, Z., and Ozen, M. (2018). The properties of chopped basalt fibre reinforced self-compacting concrete. Constr. Build. Mater. 186, 678–685. doi:10.1016/j.conbuildmat.2018.07.089
Al-Majidi, M. H., Lampropoulos, A., and Cundy, A. B. (2017). Tensile properties of a novel fibre reinforced geopolymer composite with enhanced strain hardening characteristics. Compos. Struct. 168, 402–427. doi:10.1016/j.compstruct.2017.01.085
Angst, U., Elsener, B., Larsen, C. K., and Vennesland, Ø. (2009). Critical chloride content in reinforced concrete—a review. Cem. Concr. Res. 39, 1122–1138. doi:10.1016/j.cemconres.2009.08.006
ASTM (2009) Standard test method for electrical indication of concrete's ability to resist chloride, C, 1202–1207.
ASTM C496/C496M-17 (2017). Standard test method for splitting tensile strength of cylindrical concrete specimens. West Conshohocken: ASTM International.
Banibayat, P., and Patnaik, A. (2014). Variability of mechanical properties of basalt fiber reinforced polymer bars manufactured by wet-layup method. Mater. Des. 56, 898–906. doi:10.1016/j.matdes.2013.11.081
Behfarnia, K., and Behfarnia, A. (2014). Application of high performance polypropylene fibers in concrete lining of water tunnels. Mater. Des. 55, 274–279. doi:10.1016/j.matdes.2013.09.075
Borhan, T. M. (2012). Properties of glass concrete reinforced with short basalt fibre. Mater. Des. 42, 265–271. doi:10.1016/j.matdes.2012.05.062
Bouziadi, F., Boulekbache, B., and Hamrat, M. (2016). The effects of fibres on the shrinkage of high-strength concrete under various curing temperatures. Constr. Build. Mater. 114, 40–48. doi:10.1016/j.conbuildmat.2016.03.164
Buratti, C., Moretti, E., Belloni, E., and Agosti, F. (2015). Thermal and acoustic performance evaluation of new basalt fiber insulation panels for buildings. Energy Procedia 78, 303–308. doi:10.1016/j.egypro.2015.11.648
Carrillo, F., Colom, X., Suñol, J. J., and Saurina, J. (2004). Structural FTIR analysis and thermal characterisation of lyocell and viscose-type fibres. Eur. Polym. J. 40, 2229–2234. doi:10.1016/j.eurpolymj.2004.05.003
Cerny, M., Glogar, P., Sucharda, Z., Chlup, Z., and Kotek, J. (2009). Partially pyrolyzed composites with basalt fibres – mechanical properties at laboratory and elevated temperatures. Compos. A Appl. Sci. Manuf. 40, 1650–1659. doi:10.1016/j.compositesa.2009.08.002
Chen, W., and Chen, X. (2023). Experimental study and mathematical modeling of mechanical properties of basalt fiber-reinforced recycled concrete containing a high content of construction waste. Constr. Mater 3, 462–473. doi:10.3390/constrmater3040030
Classification, P., Burridge, J., Franklin, R. E., Erntroy, H. E., and Marsh, B. K. (2013). B.S. En, British standards institution BSI, British standard, British standards. Concrete paving blocks–requirements and test methods, Br. Stand. 76s.
CSA S806-12 (2012). Design and construction of building structures with fibre-reinforced polymers. Mississauga, ON, Canada: Canadian Standards Association:.
De Figueiredo, A. D., and Ceccato, M. R. (2015). Workability analysis of steel fiber reinforced concrete using slump and ve-be test. Mater. Res. 18, 1284–1290. doi:10.1590/1516-1439.022915
Dhand, V., Mittal, G., Rhee, K. Y., Park, S.-J., and Hui, D. (2015). A short review on basalt fiber reinforced polymer composites. Compos. B Eng. 73, 166–180. doi:10.1016/j.compositesb.2014.12.011
Dias, D. P., and Thaumaturgo, C. (2007). Fracture toughness of geopolymeric concretes reinforced with basalt fibers. Cem. Concr. Compos. 27, 49–54. doi:10.1016/j.cemconcomp.2004.02.044
Di Ludovico, M., Prota, A., and Manfredi, G. (2010). Structural upgrade using basalt fibers for concrete confinement. J. Compos. Constr. 14, 541–552. doi:10.1061/(asce)cc.1943-5614.0000114
Du, Q., Cai, C., Lv, J., Wu, J., Pan, T., and Zhou, J. (2020). Experimental investigation on the mechanical properties and microstructure of basalt fiber reinforced engineered cementitious composite. Mater. (Basel) 3796, 3796. doi:10.3390/ma13173796
Du, Y., Zhang, X., Liu, L., Zhou, F., Zhu, D., and Pan, W. (2018). Flexural behaviour of carbon textile-reinforced concrete with prestress and steel fibres. Polym. (Basel) 10, 98. doi:10.3390/polym10010098
Esparham, A., Nikolai, I. V., Makhmud, K., and Mohammad, H. (2023). A study of modern eco-friendly composite (Geopolymer) based on blast furnace slag compared to conventional concrete using the life cycle assessment approach. Infrastructures 8(3), 58. doi:10.3390/infrastructures8030058
Felekoglu, B., Türkel, S., and Altuntas, Y. (2007). Effects of steel fiber reinforcement on surface wear resistance of self-compacting repair mortars. Cem. Concr. Compos. 29, 391–396. doi:10.1016/j.cemconcomp.2006.12.010
Fiore, V., Scalici, T., Di Bella, G., and Valenza, A. (2015). A review on basalt fibre and its composites. Compos. B Eng. 74, 74–94. doi:10.1016/j.compositesb.2014.12.034
Fu, Q., Bu, M., Xu, W., Chen, L., Chen, L., Li, H., et al. (2021). Comparative analysis of dynamic constitutive response of hybrid fibre-reinforced concrete with different matrix strengths. Int. J. Impact Eng. 148, 103763. doi:10.1016/j.ijimpeng.2020.103763
Gand, A. K., Yeboah, D., Khorami, M., Olubanwo, A. O., and Lumor, R. (2018). Behaviour of strengthened timber beams using near surface mounted basalt fibre reinforced polymer (BFRP) rebars. Eng. Solid Mech., 341–352. doi:10.5267/j.esm.2018.7.001
Ganesan, N., Abraham, R., and Deepa Raj, S. (2015). Durability characteristics of steel fibre reinforced geopolymer concrete. Constr. Build. Mater. 93, 471–476. doi:10.1016/j.conbuildmat.2015.06.014
Guo, Y., Hu, X., and Lv, J. (2019). Experimental study on the resistance of basalt fibre-reinforced concrete to chloride penetration. Constr. Build. Mater. 223, 142–155. doi:10.1016/j.conbuildmat.2019.06.211
Hasanzadeh, A., Ivanovich Vatin, N., Hematibahar, M., Kharun, M., and Shooshpasha, I. (2022). Prediction of the mechanical properties of basalt fiber reinforced high-performance concrete using machine learning techniques. Materials 15, 7165. doi:10.3390/ma15207165
Hassan, K. E., Cabrera, J. G., and Maliehe, R. S. (2000). The effect of mineral admixtures on the properties of high-performance concrete. Cem. Concr. Compos. 22, 267–271. doi:10.1016/s0958-9465(00)00031-7
Hassani Niaki, M., Fereidoon, A., and horbanzadeh Ahangari, A. (2018). Experimental study on the mechanical and thermal properties of basalt fiber and nanoclay reinforced polymer concrete. Compos. Struct. 191, 231–238. doi:10.1016/j.compstruct.2018.02.063
Hematibahar, M., Ivanovich Vatin, N., A. Alaraza, H., Khalilavi, A., and Kharun, M. (2022). The prediction of compressive strength and compressive stress-strain of basalt fiber reinforced high-performance concrete using classical programming and logistic map algorithm. Materials 15, 6975. doi:10.3390/ma15196975
Hrynyk, R., and Frydrych, I. (2015). Study on textile assemblies with aluminized basalt fabrics destined for protective gloves. Int. J. Cloth. Sci. Technol. 27, 705–719. doi:10.1108/ijcst-09-2014-0112
Jiang, C., Fan, K. E., Wu, F., and Chen, D. A. (2014). Experimental study on the mechanical properties and microstructure of chopped basalt fibre reinforced concrete, 187–193.
Jiao, C., Zhang, X., Chen, W., and Chen, X. (2022). Mechanical property and dimensional stability of chopped basalt fiber-reinforced recycled concrete and modeling with fuzzy inference system. Buildings 97.
Kabay, N. (2014). Abrasion resistance and fracture energy of concretes with basalt fiber. Constr. Build. Mater. 50, 95–101. doi:10.1016/j.conbuildmat.2013.09.040
Karacor, B., and Ozcanli, M. (2022). Thermal and mechanical characteristic investigation of the hybridization of basalt fiber with aramid fiber and carbon fiber. Polym. Compos 43, 8529–8544. doi:10.1002/pc.27022
Katkhuda, H., and Shatarat, N. (2017). Improving the mechanical properties of recycled concrete aggregate using chopped basalt fibers and acid treatment. Constr. Build. Mater. 140, 328–335. doi:10.1016/j.conbuildmat.2017.02.128
Kharun, M., Koroteev, D. D., Hemati Bahar, M., Huang, J., and Kamrunnaher, M. (2020). Behavior of conventional and fibered concrete shear walls during earthquake, an analytical simulation in SAP2000. J. Phys. Conf. Ser. 12033, 012033. doi:10.1088/1742-6596/1687/1/012033
KharunAlaraza, M. A. H., Hematibahar, M., Al Daini, R., and Manoshin, A. (2022). “Experimental study on the effect of chopped basalt fiber on the mechanical properties of high-performance concrete,” in Proceedings of the International Conference on Engineering Research 2021 (ICER 2021), Moscow, Russia, 20-22 October 2021.
Klyuev, S. V., Antsiferov, S. I., Klyuev, A. V., Shorstova, E. S., Karachevtseva, A. V., and Popov, V. P. (2023). Extrusion of mixtures for the construction industry by 3D printing. Russ. Eng. Res. Russ. Eng. Res. 43, 107–110. doi:10.3103/s1068798x23020168
Krassowska, J., Wolka, P., Protchenko, K., and Vidales-Barriguete, A. (2023). Testing the influence of metakaolinite and zeolite on the adhesion of BFRP and GFRP bars to concrete. Materials 16, 7435. doi:10.3390/ma16237435
Lee, J. J., Song, J., and Kim, H. (2014). Chemical stability of basalt fiber in alkaline solution. Fibers Polym. 15, 2329–2334. doi:10.1007/s12221-014-2329-7
Li, D., Ding, Y., Wang, Q., Zhang, Y., Azevedo, C., and Zhang, Y. (2019). Hybrid effect of fibre mesh and short fibres on the biaxial bending behaviour of TRC. Mag. Concr. Res. 71, 869–880. doi:10.1680/jmacr.17.00284
Li, M., Gong, F., and Wu, Z. (2020). Study on mechanical properties of alkali-resistant basalt fiber reinforced concrete. Constr. Build. Mater. 245, 118424. doi:10.1016/j.conbuildmat.2020.118424
Li, M. O., and Li, V. C. (2013). Rheology, fiber dispersion, and robust properties of engineered cementitious composites. Mater. Struct. 46, 405–420. doi:10.1617/s11527-012-9909-z
Li, V. C., Wu, C., Wang, S., Ogawa, A., and Saito, T. (2002). Interface tailoring for strain hardening polyvinyl alcohol-engineered cementitious composite (PVA-ECC). ACI Mater. J., 463–472.
Li, Y.-F., Hung, J.-F., Syu, J.-Y., Chen, S.-H., Huang, C.-H., Chang, S.-M., et al. (2024). Effect of the sizing removal methods of fiber surface on the mechanical performance of basalt fiber-reinforced concrete. Fibers 12, 10. doi:10.3390/fib12010010
Lipatov, Y. V., Gutnikov, S. I., Manylov, M. S., Zhukovskaya, E. S., and Lazoryak, B. I. (2015). High alkali-resistant basalt fiber for reinforcing concrete. Mater. Des. 73, 60–66. doi:10.1016/j.matdes.2015.02.022
Militk, J., Kovacic, V., and Rubnerowá, J. (2002). Influence of thermal treatment on tensile failure of basalt fibers. Eng. Fract. Mech. 69, 1025–1033. doi:10.1016/s0013-7944(01)00119-9
Miskiewicz, P., Frydrych, I., Pawlak, W., and Cichocka, A. (2019). Modification of surface of basalt fabric on protecting against high temperatures by the method of magnetron sputtering. Autex Res. J. 19, 36–43. doi:10.1515/aut-2018-0025
Mo, K. H., Loh, Z. P., An, C. G., and Eo, S. H. (2019). Mechanical characteristics and flexural behaviour of fibre-reinforced cementitious composite containing PVA and basalt fibres. Sa dhana.
Mohaghegh, M., Silfwerbrand, A., and Årskog, V. (2018). Shear behavior of high-performance basalt fiber concrete—Part I: laboratory shear tests on beams with macro fibers and bars. Struct. Concr. 19, 246–254. doi:10.1002/suco.201700208
Momoh, E. O., and Osofero, A. I. (2021). Behaviour of oil palm broom fibres (OPBF) reinforced concrete. Constr. Build. Mater. 221, 745–761. doi:10.1016/j.conbuildmat.2019.06.118
Monaldo, E., Nerilli, F., and Vairo, G. (2019). Basalt-Based fiber-reinforced materials and structural applications in civil engineering. Compos. Struct. 214, 246–263. doi:10.1016/j.compstruct.2019.02.002
Niu, D., Su, L. I., Luo, S. U., Huang, D., and Luo, D. (2020). Experimental study on mechanical properties and durability of basalt fiber reinforced coral aggregate concrete. Constr. Build. Mater. 237, 117628. doi:10.1016/j.conbuildmat.2019.117628
Nováková, I., Þórhallsson, E. R., and Bodnárová, L. (2017). “Behaviour of basalt fibre reinforced concrete exposed to elevated temperatur,” in 5th Int. RILEM Work. Concr. Spalling Due to Fire Expo.
Novitskii, A. G. (2004). High-temperature heat-insulating materials based on fibers from basalt-type rock materials. Refract. Ind. Ceram. 45, 144–146. doi:10.1023/b:refr.0000029624.43008.ef
Ramachandran, B. E., Velpari, V., and Balasubramanian, N. (1981). Chemical durability studies on basalt fibres. J. Mater Sci. 16, 3393–3397. doi:10.1007/bf00586301
Ramezanianpour, A. A., Esmaeili, M., Ghahari, S. A., and Najafi, M. H. (2013). Laboratory study on the effect of polypropylene fiber on durability, and physical and mechanical characteristic of concrete for application in sleepers. Constr. Build. Mater. 44, 411–418. doi:10.1016/j.conbuildmat.2013.02.076
Sadrmomtazi, A., Tahmouresi, B., and Saradar, A. (2018). Effects of silica fume on mechanical strength and microstructure of basalt fiber reinforced cementitious composites (BFRCC). Constr. Build. Mater. 162, 321–333. doi:10.1016/j.conbuildmat.2017.11.159
Saloni, P., Pham, M., and Pham, T. M. (2020). Enhanced properties of high-silica rice husk ash-based geopolymer paste by incorporating basalt fibers. Constr. Build. Mater. 245, 118422. doi:10.1016/j.conbuildmat.2020.118422
Sarkar, A., and Hajihosseini, M. (2020). The effect of basalt fibre on the mechanical performance of concrete pavement. Road Mater. Pavement Des. 21, 1726–1737. doi:10.1080/14680629.2018.1561379
Shaikh, F. U. A., and Taweel, M. (2015). Compressive strength and failure behaviour of fibre reinforced concrete at elevated temperatures. Adv. Concr. Constr. 3, 283–293. doi:10.12989/acc.2015.3.4.283
Shoaib, M., Jamshaid, H., Alshareef, M., Alharth, F. A., Ali, M., and Waqas, M. (2022). Exploring the potential of alternate inorganic fibers for automotive composites. Polymers 14(22), 4946. doi:10.3390/polym14224946
Sim, J., Park, C., and Moon, D. Y. (2005). Characteristics of basalt fiber as a strengthening material for concrete structures. Compos. B Eng. 36, 504–512. doi:10.1016/j.compositesb.2005.02.002
Sun, X., Gao, Z., Cao, P., and Zhou, C. (2019). Mechanical properties tests and multiscale numerical simulations for basalt fiber reinforced concrete. Constr. Build. Mater 202, 58–72. doi:10.1016/j.conbuildmat.2019.01.018
Sun, Z., and Xu, Q. (2009). Microscopic, physical and mechanical analysis of polypropylene fiber reinforced concrete. Mater. Sci. Eng. 527, 198–204. doi:10.1016/j.msea.2009.07.056
Taha, A., Alnahhal, W., and Alnuaimi, N. (2020). Bond durability of basalt FRP bars to fiber reinforced concrete in a saline environment. Compos. Struct. 243, 112277. doi:10.1016/j.compstruct.2020.112277
Tamás-Bényei, P., and Sántha, P. (2023). Potential applications of basalt fibre composites in thermal shielding. J. Therm. Anal. Calorim. 148, 271–279. doi:10.1007/s10973-022-11799-2
Tumadhir, M. (2013). Thermal and mechanical properties of basalt fibre reinforced concrete. Int. J. Civ. Environ. Eng. 7, 334–337.
Usage of Chopped Basalt Fibers in Concrete Composites: A Review (2017). Usage of chopped basalt fibers in concrete composites: a review. Int. J. Eng. Res. Technol. 6. doi:10.17577/IJERTV6IS090164
Wang, D., Ju, Y., Shen, H., and Xu, L. (2019). Mechanical properties of high performance concrete reinforced with basalt fiber and polypropylene fiber. Constr. Build. Mater. 197, 464–473. doi:10.1016/j.conbuildmat.2018.11.181
Wang, D., Wang, H., Larsson, S., Benzerzour, M., Maherzi, W., and Amar, M. (2020). Effect of basalt fiber inclusion on the mechanical properties and microstructure of cement-solidified kaolinite. Constr. Build. Mater. 241, 118085. doi:10.1016/j.conbuildmat.2020.118085
Wang, J., and Zhang, Y. (2010). “Experimental research on mechanical and working properties of non-dipping chopped basalt fiber reinforced concret,” in Proc. - 3rd Int. Conf. Inf. Manag. Innov. Manag. Ind. Eng. ICIII 2010.
Wang, M., Zhang, Z., Li, Y., Li, M., and Sun, Z. (2008). Chemical durability and mechanical properties of alkali-proof basalt fiber and its reinforced epoxy composites. J. Reinf. Plast. Compos. 27, 393–407. doi:10.1177/0731684407084119
Wdowiak-Postulak, A., Gocál, J., Bahleda, F., and Prokop, J. (2023). Load and deformation analysis in experimental and numerical studies of full-size wooden beams reinforced with prestressed FRP and steel bars. Appl. Sci. 13178, 13178. doi:10.3390/app132413178
Wen, B., Gao, G., Huang, D., and Zheng, H. (2023). Adhesion and sliding constitutive relationship between basalt–polypropylene hybrid fiber-reinforced concrete and steel bars. Appl. Sci. 13(22), 12108. doi:10.3390/app132212108
Wu, G., Wang, X., Wu, Z., Dong, Z., and Zhang, G. (2015). Durability of basalt fibers and composites in corrosive environments. J. Compos. Mater. 49, 873–887. doi:10.1177/0021998314526628
Yousefieh, N., Joshaghani, A., Hajibandeh, E., and Shekarchi, M. (2017). Influence of fibers on drying shrinkage in restrained concrete. Constr. Build. Mater. 148, 833–845. doi:10.1016/j.conbuildmat.2017.05.093
Zhang, H., Wang, L., Zheng, K., Bakura, T. J., and Totakhil, P. C. (2018). Research on compressive impact dynamic behavior and constitutive model of polypropylene fiber reinforced concrete. Constr. Build. Mater 187, 584–595. doi:10.1016/j.conbuildmat.2018.07.164
Zhang, J. (2017). Experimental study on preparation and mechanical properties of basalt-polypropylene hybrid fiber concrete with high durability. China: Xi'an University of Architecture and Technology.
Zheng, Y. X., Zhou, J. B., Zhang, P., and Ma, M. (2022). Mechanical properties and meso-microscopic mechanism of basalt fiber-reinforced recycled aggregate concrete. J. Clean. Prod. 370, 133555. doi:10.1016/j.jclepro.2022.133555
Keywords: basalt fiber, building protection, chemical properties, water absorption, chloride ion permeability, alkali and carrions resistance, shrinkage properties, abrasion resistance
Citation: Vatin NI, Hematibahar M and Gebre TH (2024) Impact of basalt fiber reinforced concrete in protected buildings: a review. Front. Built Environ. 10:1407327. doi: 10.3389/fbuil.2024.1407327
Received: 26 March 2024; Accepted: 01 May 2024;
Published: 21 May 2024.
Edited by:
T. Jothi Saravanan, Indian Institute of Technology Bhubaneswar, IndiaReviewed by:
Tushar Bansal, Sharda University, IndiaTrushna Jena, Indian Institute of Technology Bhubaneswar, India
Pawan Kumar, Indian Institute of Technology Bhubaneswar, India
Copyright © 2024 Vatin, Hematibahar and Gebre. This is an open-access article distributed under the terms of the Creative Commons Attribution License (CC BY). The use, distribution or reproduction in other forums is permitted, provided the original author(s) and the copyright owner(s) are credited and that the original publication in this journal is cited, in accordance with accepted academic practice. No use, distribution or reproduction is permitted which does not comply with these terms.
*Correspondence: Nikolai Ivanovich Vatin, vatin@mail.ru