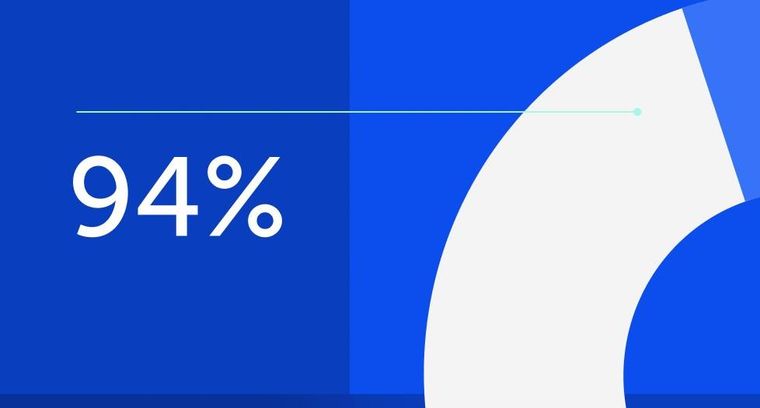
94% of researchers rate our articles as excellent or good
Learn more about the work of our research integrity team to safeguard the quality of each article we publish.
Find out more
BRIEF RESEARCH REPORT article
Front. Built Environ., 25 March 2024
Sec. Geotechnical Engineering
Volume 10 - 2024 | https://doi.org/10.3389/fbuil.2024.1389576
This article is part of the Research TopicRising Stars in Geotechnical EngineeringView all 7 articles
The fragility curve expresses the probability that an asset exceeds some serviceability state for a given level of environmental perturbation or other loadings. It is an important component in the quantitative risk analysis and resilience evaluation of infrastructure exposed to natural hazards. Incidences of over-settlement of embankments are increasingly reported due to more intense and longer-duration rainfall events. This paper develops fragility curves for the rainfall-induced embankment settlement. For this purpose, an embankment incorporating enhanced seepage and displacement analysis within unsaturated soil conditions is modelled based on a reported case history. A Monte Carlo simulation is used for rainfall infiltration and embankment deformation analysis under various rainfall scenarios. Probability values are obtained to achieve three levels of damage states in terms of road embankment settlement. The parametric analysis produces the exceedance probability curves for various rainfall intensities, saturated permeabilities and embankment slope angles. This work offers an efficient tool for assessing fragility to rainfall-induced excessive settlement of embankments.
Embankment structures have been increasingly reported to have experienced various levels of deformation during rainfall events, such as the 2014 Bosnia and Herzegovina rainfall and the 2012 Southern Europe rainfall (Winter et al., 2020; Martinović et al., 2018). These occurrences present significant challenges to transportation infrastructure, disrupting the seamless operation of roads, railways, and other essential components of the transport network (Wang et al., 2022). During the 2013–2014 heavy rainfalls in the United Kingdom, extensive damage to rail lines across the southwest was observed (Thorne, 2014), resulting in the disruption of access along these routes.
Historic events have demonstrated that rainfall may induce embankment damage including cracking, excessive settlement, and lateral spreading (Reeves et al., 2019; Winter et al., 2020). The damage mechanism of excessive settlement results from the reduction of near surface soil suctions due to infiltrating water, along with the downward movement of the wetting front (Martinović et al., 2018; Chen et al., 2021). Besides, changes in climate and the environmental conditions surrounding the slopes are commonly insufficiently considered in design. Therefore, it is of paramount importance to consider the environmental, weather and climate change effects on embankment performance (Reeves et al., 2019; Achillopoulou et al., 2020).
The fragility curve acts as an efficient tool that can be implemented in the risk and resilience analysis (Chen et al., 2023; Xu et al., 2023; Lu et al., 2024). Fragility curves represent the probability of the studied objective exceeding a specific damage state (DS). In road embankment seismic analysis, fragility curves have been developed for embankments on soft and liquefiable soils (Argyroudis and Kaynia, 2015; Zhou et al., 2023). Compared with the seismic fragility analysis of embankments, the assessment of embankments under the rainfall within the framework of fragility curves is limited (Argyroudis et al., 2019). Considering the increasing rainfall-induced embankment damage, assessment of the risk resistance of transportation infrastructure becomes imperative. Enhancing the resilience of embankments against rainfall hazards is vital for ensuring uninterrupted mobility and sustaining socio-economic development.
This study aims to develop a set of fragility curves to assess the fragility of embankments to rainfall-induced settlement. An embankment model is constructed using the finite difference method FLAC 3D (Itasca Consulting Group, 2017). The model accounts for various parameters, including rainfall intensities, slope angles, and saturated permeability levels, to quantify the potential risk associated with rainfall-induced settlement. A detailed analysis of the relevant curve characteristics is conducted to gain insights into the interrelationships among these factors and the fragility of embankments. The outcomes of this study provide an efficient and reliable tool for assessing the fragility of embankments to excessive settlement triggered by rainfall, thereby contributing to informed decision-making in the design, construction, and maintenance of road infrastructure.
A finite difference method FLAC 3D (Itasca Consulting Group, 2017; Zheng et al., 2024a) is adopted to simulate the embankment in this study. A railroad embankment located in Qingdao, Shandong province, China, was reported by Chen et al. (2014) to investigate the embankment settlement in site for an unsaturated soil. Embankments with a height of 7.53 m, a slope of 1.5 H: 1 V and a 6.25 m wide crest are constructed on the soft soil layers. One-half of the embankments are modelled, and symmetrical boundary conditions are applied along the lateral boundaries, while both the horizontal and vertical displacements are fixed along the bottom boundary. The lateral boundaries of the model are simulated with rollers, while pins provide support to the bottom. The numerical model parameters are chosen to be the same as Chen et al. (2014), as shown in Table 1. The behavior of the embankment and soft soils is simulated using a linearly elastic-perfectly plastic Mohr-Coulomb constitutive model (Huang and Han, 2009; Zheng et al., 2019; Zhou et al., 2024). A staged construction is applied to represent the embankment load, and a 0.5 m high filling is constructed in each stage. With regard to hydraulic boundary conditions, a zero-pore pressure boundary condition is applied at the top of the soft clay layers and hence it is permeable (Zheng et al., 2024b). At the bottom of the mesh, an impervious boundary condition is employed. An impermeable boundary condition is applied to the left and right-hand side vertical boundaries because they are far from the loaded area. The excess pore pressures developed near these vertical boundaries are nearly zero throughout the analysis.
Probabilistic techniques are well-suited for analyzing rainfall-induced damages as both the load (i.e., disturbing force induced by rainfall) and the resistance (depends on soil suction) vary over time due to climatic factors (Babu and Murthy, 2005; Xue and Gavin, 2007; Gavin and Xue, 2009; Reale et al., 2016b). A framework is presented for interpreting serviceability state than traditional deterministic design which assumes fixed point values (Reale et al., 2015). If the probability distributions of both the limiting tolerable displacement and the calculated displacement S are known, then a fully reliability-based serviceability evaluation can be conducted. The reliability evaluation would be analogous to that of the serviceability limit evaluation. The performance function g(X) for the serviceability limit can be written as the difference between an embankment capacity C) and its demand D), as shown in Eq. 1:
where g(X) > 0 defines a satisfactory performance region and g(X) < 0 defines an unsatisfactory performance region. The main steps to derive fragility curves based on numerical modelling are the following.
1. Derive a model including the studied embankment and the imposed hazard effects (i.e., rainfall), and perform numerical analysis for increased hazard intensities. An infiltration analysis was carried out to analyze the influx of water into the embankment with time. The progression of the wetting front over time is tracked by monitoring the time taken for each element to reach its residual suction.
2. Define damage states (DSs) based on the range of damage values (i.e., Minor damage, Moderate damage, Extensive damage). A Monte Carlo simulation (MCS) is used to model how the embankment settlement distributions change as the wetting front progresses.
3. The resultant probability of failure data is plotted with time to form the fragility curves. For each rainfall intensity and duration combination considered, three realisations of probability of exceedance and reliability index are determined.
The selection of appropriate damage states is crucial, and various DSs have been previously considered for road transport infrastructure. Criteria such as horizontal displacements at embankments and traffic lanes, maximum settlement of the embankment surface, lateral displacement rate, and maximum subsidence rate have been used to classify DSs in previous studies. Following Pitilakis et al. (2014) and Argyroudis and Kaynia (2015), this study adopts the mean settlement value (measured at the edge and middle of the embankment/cut surface) at the railroad embankment crest and delineates three DSs (minor, moderate, and extensive) to depict damage levels. Expert judgment-based descriptions of these damage levels are as follows: DS1 indicates slight surface sliding or minor cracks on the road surface (corresponding to an embankment settlement of 0.03 m), with vehicle passage unaffected; DS2 denotes the need for partial road closure due to medium settlement or cracks (corresponding to 0.08 m), signalling a maintenance state; and DS3 signifies extensive cracks (corresponding to 0.20 m), requiring full road closure for reconstruction or partial closure during significant repairs (Pitilakis et al., 2014).
In this study, rainfall (of a given intensity and duration) is taken as an intensity measure. Rainfall duration is depicted on the x-axis of the fragility curves (Xue and Gavin, 2008). Given that this study assumes a rainfall intensity of 10 mm/h as a baseline case, the total rainfall can be acquired by multiplying rainfall intensity and rainfall duration. The fragility curves for minor, moderate, and extensive damage states are developed. The coefficients of variation (COV) are assigned for probabilistic calculations. The geotechnical strength parameters and unit weight are defined using normal distributions as recommended by Phoon (2008), and Phoon and Kulhawy (1999). The mean values of embankment materials are summarised in Table 1. The COVs assigned to the unit weight, angle of internal friction, cohesion, and compression modulus are 0.1, 0.3, 0.5, and 0.5, respectively. A Monte Carlo probabilistic analysis was conducted. This section analyzes the fragility curve of the embankment considering the influence of the rainfall intensity, the fill saturated permeability and the embankment slope angles.
Figure 1 illustrates the fragility curves corresponding to rainfall intensities of 5, 10 and 18 mm/h, respectively. These curves indicate that within the initial 5 hours of rainfall, the likelihood of surpassing any damage state is nearly negligible. However, for longer rainfall durations, the probability of exceedance increases progressively, accompanied by extended time intervals between the damage states. When the duration of rainfall is 8 h, all the probabilities of three rainfall intensities exceeding the limit state of the minor damage are approximately 0%. The embankment is unlikely to be affected by rainfall at this level. When the rainfall duration is 28 h, the probabilities of the rainfall intensities of 5, 10 and 18 mm/h exceeding the minor damage state are 6.4%, 18.1% and 96.9%. The studied embankment is likely to be slightly damaged at this rainfall duration. For very serious rainfall, for example, when the rainfall duration is 64 h, the extensive damage probability of the rainfall intensity of 18 mm/h reaches 27.2%. The embankment has a high fragility at extreme rainfall (e.g., rainfall durations longer than 96 h), and it may suffer instability.
Figure 2 illustrates the influence of embankment saturated permeability on the fragility curve, and the saturated permeability values of 0.77 × 10−6 m/s and 5.77 × 10−6 m/s are considered (Wu, 2011). The red curve represents the large permeability, while the black curve represents the low permeability. For the rainfall duration of 40 h, the probabilities of the saturated permeability values of 0.77 × 10−6 m/s and 5.77 × 10−6 m/s exceeding the minor damage state are 47.4% and 81.9%, respectively. At this rainfall duration, the embankment is prone to minor damage. In the case of a longer rainfall duration, such as 72 h, the probability of extensive damage for the saturated permeability of 5.77 × 10−6 m/s reaches 19.6%, indicating a high fragility to extreme rainfall events.
Figure 3 presents the influence of the embankment slope angle on the fragility curves. The slope angles corresponding to 2H: 1V and 1.5H: 1V are represented by the black and red lines, respectively. When the rainfall duration extends to 32 h, the probability of exceeding the minor damage state stands at 24.2% for slope angles of 2H: 1V and 37.1% for 1.5H: 1V. This indicates a propensity for minor damage in the studied embankment under such conditions. For a longer rainfall duration of 72 h, the probability of extensive damage for a slope angle of 1.5H: 1V is 20.4%. That emphasizes the increased susceptibility of the embankment to severe damage under extreme rainfall conditions.
The influence of rainfall intensity, embankment saturated permeability, and slope angle varies in shaping the fragility curve. As shown in Figure 1, the fragility curves for the lower-intensity rainfall events shift towards higher probabilities, reflecting the extended development of the wetting front. Once the degradation process begins, the curves assume a consistent shape for different rainfall intensities. This is because the rainfall intensity has a negligible effect on the infiltration process post-wetting front development. However, the infiltration process post-wetting front development primarily hinges on the saturated permeability value and geometric parameters. As shown in Figure 2, the curve for the large permeability is steeper, indicating the embankment of saturated permeability values of 5.77 × 10−6 m/s is more sensitive to rainfall. A large permeability results in great wetting front progression and large probabilities of exceeding all three damage states, leading to steeper fragility curves. The results highlight the pivotal role of saturated permeability in shaping embankment fragility curves and consequently determining the risk associated with damage states. As the damage state transitions from minor to extensive, the difference in slope between the curves widens, emphasizing varying wetting front progression. Additionally, a high-angle embankment slope results in a steep fragility curve trend, as shown in Figure 3, suggesting increased susceptibility to settlement triggered by rainfall. A lower slope angle reduces the advancement of the wetting front, leading to decreased probabilities of exceeding all three damage states. Conversely, a higher slope angle accelerates downward seepage, resulting in more pronounced fragility curves. These findings emphasize the substantial impact of the slope angles on embankment fragility curves and their implications for assessing risks associated with damage states.
This paper establishes rainfall-induced fragility curves for embankments on silty soils to improve the understanding of the rainfall-induced risk assessment of road embankment infrastructure. Nonlinear creep and deformation analyses using Monte Carlo simulations are carried out with reference to geometrical schemes and site conditions corresponding to the embankment in site from prior literature. The embankment performance is estimated using the DS in terms of the settlement of the embankment crest.
Fragility curves show that embankments subjected to higher rainfall intensity are more vulnerable to rainfall-induced deformation. That is captured by the increase in damage probability across all states with higher rainfall intensity. For extreme rainfall of 18 mm/h, when the rainfall duration is 64 h, the extensive damage probability reaches 27.2%. The fragility curves for high-intensity rainfall events indicate the accelerated progression of the wetting front. Once the degradation process begins, the curves show a consistent shape for different rainfall intensities.
The key role of saturated permeability and slope angle is highlighted in shaping embankment fragility curves and consequently determining the risk associated with various damage states. Higher saturated permeability and slope angle contribute to steeper fragility curves due to reduced soil suction and a lower wetting front. Fragility curves serve as valuable tools for transport network operators, enabling them to make informed decisions about service closures based on detailed weather forecasts and the assessment of damage risk exceeding permissible limits.
Note that the analyses that have been presented show that the shape of the fragility curve depends on environmental loading (rainfall intensity and duration), soil parameters and geometric parameters. The fragility is also influenced by the initial soil suction, soil-water characteristic curve (SWCC) and other parameters, which are expected to be considered in future work.
The original contributions presented in the study are included in the article/supplementary material, further inquiries can be directed to the corresponding author.
HZ: Writing–review and editing, Supervision, Methodology, Conceptualization, Writing–original draft. FM: Writing–original draft, Software, Methodology, Data curation. XY: Writing–review and editing, Writing–original draft, Methodology, Formal Analysis, Conceptualization. GZ: Writing–review and editing, Methodology, Conceptualization.
The author(s) declare that financial support was received for the research, authorship, and/or publication of this article. The research reported in this paper was supported by the National Natural Science Foundation of China (No. 52322809, No. 52078337 and No. 52208363) and the Natural Science Foundation of Hebei, China (No. E2021202215).
The authors declare that the research was conducted in the absence of any commercial or financial relationships that could be construed as a potential conflict of interest.
All claims expressed in this article are solely those of the authors and do not necessarily represent those of their affiliated organizations, or those of the publisher, the editors and the reviewers. Any product that may be evaluated in this article, or claim that may be made by its manufacturer, is not guaranteed or endorsed by the publisher.
Achillopoulou, D. V., Mitoulis, S. A., Argyroudis, S. A., and Wang, Y. (2020). Monitoring of transport infrastructure exposed to multiple hazards: a roadmap for building resilience. Sci. total Environ. 746, 141001. doi:10.1016/j.scitotenv.2020.141001
Argyroudis, S., and Kaynia, A. M. (2015). Analytical seismic fragility functions for highway and railway embankments and cuts. Earthq. Eng. Struct. Dyn. 44 (11), 1863–1879. doi:10.1002/eqe.2563
Argyroudis, S. A., Mitoulis, S. Α., Winter, M. G., and Kaynia, A. M. (2019). Fragility of transport assets exposed to multiple hazards: state-of-the-art review toward infrastructural resilience. Reliab. Eng. Syst. Saf. 191, 106567. doi:10.1016/j.ress.2019.106567
Babu, G. S., and Murthy, D. S. (2005). Reliability analysis of unsaturated soil slopes. J. geotechnical geoenvironmental Eng. 131 (11), 1423–1428. doi:10.1061/(asce)1090-0241(2005)131:11(1423)
Chen, K., Liang, F., and Wang, C. (2021). A fractal hydraulic model for water retention and hydraulic conductivity considering adsorption and capillarity. J. Hydrology 602, 126763. doi:10.1016/j.jhydrol.2021.126763
Chen, K., Pang, R., and Xu, B. (2023). Stochastic dynamic response and seismic fragility analysis for high concrete face rockfill dams considering earthquake and parameter uncertainties. Soil Dyn. Earthq. Eng. 167, 107817. doi:10.1016/j.soildyn.2023.107817
Chen, W. Z., Jiang, G. L., Zhao, H. S., Wu, L. J., and Li, A. H. (2014). Analysis of nonlinear settlement for an unsaturated soil under stage continuous loading. J. Central South Univ. 21, 4690–4697. doi:10.1007/s11771-014-2478-2
Gavin, K., and Xue, J. (2009). Use of a genetic algorithm to perform reliability analysis of unsaturated soil slopes. Géotechnique 59 (6), 545–549. doi:10.1680/geot.8.t.004
Huang, J., and Han, J. (2009). 3D coupled mechanical and hydraulic modeling of a geosynthetic-reinforced deep mixed column-supported embankment. Geotext. Geomembranes 27 (4), 272–280. doi:10.1016/j.geotexmem.2009.01.001
Itasca Consulting Group (2017). Flac 3D – fast Lagrangian analysis of continua in 3 dimensions, ver. 6.0. Minneapolis, Minnesota: Itasca.
Lu, Y., Pang, R., Du, M., and Xu, B. (2024). Simulation of non-stationary ground motions and its applications in high concrete faced rockfill dams via direct probability integral method. Eng. Struct. 298, 117034. doi:10.1016/j.engstruct.2023.117034
Martinović, K., Reale, C., and Gavin, K. (2018). Fragility curves for rainfall-induced shallow landslides on transport networks. Can. Geotechnical J. 55 (6), 852–861. doi:10.1139/cgj-2016-0565
K. K. Phoon (2008). Reliability-based design in geotechnical engineering: computations and applications. New York, NY, United States: (Taylor and Francis).
Phoon, K. K., and Kulhawy, F. H. (1999). Characterization of geotechnical variability. Can. Geotechnical J. 36 (4), 612–624. doi:10.1139/cgj-36-4-612
Pitilakis, K., Crowley, H., and Kaynia, A. M. (2014). SYNER-G: typology definition and fragility functions for physical elements at seismic risk. Geotechnical, Geol. Earthq. Eng. 27, 1–28. doi:10.1007/978-94-007-7872-6
Reale, C., Xue, J., and Gavin, K. (2016b). System reliability of slopes using multimodal optimisation. Géotechnique 66 (5), 413–423. doi:10.1680/jgeot.15.p.142
Reale, C., Xue, J. F., Pan, Z., and Gavin, K. (2015). Deterministic and probabilistic multimodal analysis of slope stability. Comput. Geotechnics 66 (5), 172–179. doi:10.1016/j.compgeo.2015.01.017
Reeves, S., Winter, M., Leal, D., and Hewitt, A. (2019a). Rail: an industry guide to enhancing resilience. The Resilience Shift and TRL: London, UK.
Reeves, S., Winter, M., Leal, M., and Hewitt, D. (2019b). Roads: an industry guide to enhancing resilience. London, UK: TRL and the Resilience Shift.
Thorne, C. (2014). Geographies of UK flooding in 2013/4. Geogr. J. 180 (4), 297–309. doi:10.1111/geoj.12122
Wang, C., Yuan, Y., Liang, F., and Tao, J. (2022). Experimental investigation of local scour around cylindrical pile foundations in a double-layered sediment under current flow. Ocean. Eng. 251, 111084. doi:10.1016/j.oceaneng.2022.111084
Winter, M. G., Reeves, S. J., Smajlović, S., Ghataora, G., Šehić, D., and Zejnić, H. (2020). The Kosova landslide, Bosnia and Herzegovina. Q. J. Eng. Geol. Hydrogeology 53 (4), 523–529. doi:10.1144/qjegh2019-097
Wu, L. J. (2011). Study on compressibility and consolidation of unsaturated soil and reinforced technique on high-speed railway. Chengdu, China: Southwest Jiaotong University.
Xu, M., Pang, R., Zhou, Y., and Xu, B. (2023). Seepage safety evaluation of high earth-rockfill dams considering spatial variability of hydraulic parameters via subset simulation. J. Hydrology 626, 130261. doi:10.1016/j.jhydrol.2023.130261
Xue, J., and Gavin, K. (2008). Effect of rainfall intensity on infiltration into partly saturated slopes. Geotechnical Geol. Eng. 26, 199–209. doi:10.1007/s10706-007-9157-0
Xue, J. F., and Gavin, K. (2007). Simultaneous determination of critical slip surface and reliability index for slopes. J. geotechnical geoenvironmental Eng. 133 (7), 878–886. doi:10.1061/(asce)1090-0241(2007)133:7(878)
Zheng, G., Guo, Z., Zhou, H., and He, X. (2024a). Design method for wall deformation and soil movement of excavations with inclined retaining walls in sand. Int. J. Geomechanics 24 (4), doi:10.1061/ijgnai.gmeng-9029
Zheng, G., Guo, Z., Zhou, H., Tan, Y., Wang, Z., and Li, S. (2024b). Multibench-retained excavations with inclined–vertical framed retaining walls in soft soils: observations and numerical investigation. J. Geotechnical Geoenvironmental Eng. 150 (5), doi:10.1061/jggefk.gteng-11943
Zheng, G., Yang, X., Zhou, H., and Chai, J. (2019). Numerical modeling of progressive failure of rigid piles under embankment load. Can. Geotechnical J. 56 (1), 23–34. doi:10.1139/cgj-2017-0613
Zhou, H., Liu, X., Tan, J., Zhao, J., and Zheng, G. (2023). Seismic fragility evaluation of embankments on liquefiable soils and remedial countermeasures. Soil Dyn. Earthq. Eng. 164, 107631. doi:10.1016/j.soildyn.2022.107631
Keywords: embankments, rainfall, probabilistic analysis, settlement, fragility curves
Citation: Zhou H, Ma F, Yu X and Zheng G (2024) Fragility assessment for the rainfall-induced embankments on silty soils. Front. Built Environ. 10:1389576. doi: 10.3389/fbuil.2024.1389576
Received: 21 February 2024; Accepted: 14 March 2024;
Published: 25 March 2024.
Edited by:
Jie Han, University of Kansas, United StatesCopyright © 2024 Zhou, Ma, Yu and Zheng. This is an open-access article distributed under the terms of the Creative Commons Attribution License (CC BY). The use, distribution or reproduction in other forums is permitted, provided the original author(s) and the copyright owner(s) are credited and that the original publication in this journal is cited, in accordance with accepted academic practice. No use, distribution or reproduction is permitted which does not comply with these terms.
*Correspondence: Xiaoxuan Yu, eXV4aWFveHVhbkB0anUuZWR1LmNu
Disclaimer: All claims expressed in this article are solely those of the authors and do not necessarily represent those of their affiliated organizations, or those of the publisher, the editors and the reviewers. Any product that may be evaluated in this article or claim that may be made by its manufacturer is not guaranteed or endorsed by the publisher.
Research integrity at Frontiers
Learn more about the work of our research integrity team to safeguard the quality of each article we publish.