- 1Pontificia Universidad Católica del Perú (PUCP), GERDIS Research Group, Lima, Peru
- 2Pontificia Universidad Católica del Perú (PUCP), IPDESUR Research Group, Lima, Peru
Introduction: In urban areas exposed to high-magnitude earthquakes, the drinking water supply would be severely damaged, and domestic services would be disrupted for a large part of the population in the event of an earthquake. The Lima-Callao metropolitan area in Peru, South America, is expected to experience an 8.8 Mw earthquake, and it is estimated that approximately 90% of the population would not have immediate access to emergency water in the case of such an event. The main objective of this paper is to define criteria for a spatial analysis method to guide the design criteria for an Emergency Water Supply System (EWaSS).
Methods: This paper combines territorial, urban resilience and participatory approaches and presents the results of an interdisciplinary research with social impact. Thus, it examines the urban territory at macro-, meso- and micro scales; physical-spatial variables indicating risk levels and possible public spaces to implement the system; and socio-spatial variables regarding the population, risk perception and participation in management to strengthen urban resilience. Normative tools and the Geographic Information System are used to spatialize and systematize quantitative and qualitative information.
Results and discussion: The EWaSS is an alternative for safe water supply in a post-disaster situation that would provide immediate and autonomous operation during the first 72 h of the emergency. The results show the physical-spatial and social viability of urbanized areas and the system design criteria that guide local actors in making decisions at the three levels of emergency management.
1 Introduction
Assisting populations affected by high-intensity earthquakes poses significant challenges. These include the lack of domestic water supply due to the failure of the distribution system (ALA, 2001; Liu et al., 2020; Alberto et al., 2022), which increases sanitary risks and can lead to additional adverse effects and even loss of life due to the immediate unavailability of water. Since the 2000s, earthquakes ranging from 6.7 to 9.1 magnitude have shown that the average time to restore water supply service in Latin American countries is between 45 and 60 days. In comparison, in developed countries, the average time to restore service is estimated at 33 days, only a relative reduction in the context of an emergency (Alberto and De la Llera, 2016; Balaei et al., 2019; Takemoto et al., 2021). It is important to consider that “in disaster situations, the number of people demanding access to water and sanitation services is much higher than the number of dead, injured, displaced, or in need of medical care” (PAHO/WHO, UNISDR, UNICEF, & IFRC, 2006; cited by IDB, 2019).
According to international experience, public management expects emergency response to occur, on average, 72 h after a disaster (FEMA 2004; EPA, 2011). This delay can lead to further social risks, especially in urban areas with pre-existing water insecurity and poor capacity to attend the emergency. To prevent such situations, regulations and academic contributions are aimed at controlling social risks (Lavell, 2005, Simioni, 2003), including three new Disaster Risk Management, DRM, approaches 1) risks related to civil rights (IPCC, 2012) and person-centered (Scolobig, Prior, Schröter, Jörin, Patt, 2015); 2) interdisciplinarity and articulation of different levels of government, private entities and civil society; and 3) participation to improve risk perception and assume responsibility at the community level (IPCC, 2012). These approaches aim to improve the response capacity of the different actors during an extreme natural or anthropogenic event (Simioni, 2003).
A contribution to the traditional visions is the inclusion of an integral knowledge of structural as well as non-structural measures in DRM based on the risk perception of the community, for which it is proposed to prioritize the knowledge of risks in an educational process at different levels and with the collaborative participation of the relevant actors of society (Lavell, 2005; Ferradas, 2008; Balaei et al., 2019; Takemoto et al., 2021). The spatial analysis framework with Geographic Information Systems facilitates multi-criteria assessment for a coherent and practical characterization of the territory, allowing interrelationship between the main aspects of vulnerability and resilience (Barredo, 1996; Bocco and Urquijo, 2013; Aguilera, Rodriguez and Monserrat, 2018; Moschella, 2018). Likewise, the spatial qualitative dimension is included to make visible the experiences and knowledge of local actors (Sepúlveda, 2018).
Despite these contributions and approaches, dealing with emergencies at different levels of public management remains challenging. One reason is that most DRM practitioners lack the urban-spatial perspective and have different visions of risk knowledge and emergency response. Moreover, these visions focus on objective and quantifiable assessments, prioritizing structural measures over non-structural ones.
This paper proposes a spatial analysis method for defining design criteria in earthquake-emergency water provisioning to contribute to the management of the first 72 h of a high-intensity earthquake scenario. The proposed method is the result of the systematization of experiences for spatial analysis, urban design and planning in Andean cities of intermediate size with a DRM approach that was incorporated consistently (Santa Cruz et al., 2016; Fernández de Córdova et al., 2017; Vilela and de Córdova, 2017; Vilela and Moschella, 2017). The main contribution of these experiences consisted of integrating three approaches: the articulation of spatial urban scales, collaborative participation and interdisciplinarity. Most of these experiences materialized in public investment projects or were incorporated into specific urban plans and local DRM.
The method is based on an Emergency Water Supply System (EWaSS) to face the first moments of the emergency in heterogeneous urban areas with pre-existing water insecurity (Santa-Cruz et al., 2024). The EWaSS is a network of local autonomous Spatial Supply Units, SSU, defined as the minimal part of the EwaSS. The SSU consists of one or more Points of Distribution (PoD) of water, the neighborhood’s community and urban infrastructure. The PoDs are located in safe public spaces like parks and squares.
The innovation of this research lies in the interrelation of the following approaches: a) territorial, in the articulation of macro-, meso- and micro scales with their respective normative tools; b) urban resilience, for risk diagnosis and identifying a feasible solution, based on the physical-spatial variables related to the characterization of damage levels, seismic microzonation and the quality of public spaces; as well as the socio-spatial variables related to population density, socioeconomic strata and risk perception by the population and their participation in its management; c) participatory, by involving local actors in the co-production of alternatives of solution through collaborative activities during the research process.
The application of the method is demonstrated through a case study of the design of an EWaSS, capable of operating autonomously from the first moments after the occurrence of an earthquake in the Lima-Callao metropolitan area where a magnitude 8.8 Mw earthquake is predicted (IGP, 2020). This case study was elaborated in the framework of an interdisciplinary and social responsibility research project. From an operational point of view, the criteria of social responsibility and those of DRM are applied following common objectives, with contributions from civil engineering, architecture, geography and sociology, and with the participation of various actors of local management.
2 Disaster risk management for safe water in the first moments of an earthquake emergency
The Sendai Framework for Disaster Risk Reduction 2015–2030 (PCM, 2021) outlines the new approach to disaster risk management. This approach prioritizes prevention, preparedness, and mitigation in the face of impending disasters and emphasizes addressing people’s vulnerabilities to reduce existing disaster risks, prevent the emergence of new risks, and strengthen community resilience.
2.1 Experiences in past events
International experience with the effects of extreme earthquakes shows that the infrastructure of the domestic water supply system suffers massive damage. As a result, services are interrupted, basic needs are limited, and the health risks of the affected population are increased. Faced with such a scenario, preparedness and post-disaster response measures recommend strengthening the self-sufficiency capacity of the affected population in the first days of the emergency (Balaei et al., 2019; Takemoto et al., 2021).
For example, in New Zealand and Chile, DRM agencies recommend having a supply of bottled water that can last 72 h after a disaster, agree that the amount of water needed per person is 4 L per day, and distinguish personal use from other uses. With regard to the situations that hindered or facilitated the continuity of water supply after a high-intensity earthquake, the following experiences were noted:
- Structural aspects (characteristics of the infrastructure): As a result of the earthquakes in New Zealand (2011) and Chile (2010), the drinking water network collapsed in some sectors, resulting in service interruptions, flooding, and other negative impacts (Balaei et al., 2019). In contrast, in the case of the earthquake in Japan (2011), water utilities invested in earthquake-resistant ductile iron pipes, a system that was not damaged and allowed for service continuity. Another solution was the implementation of underground water tanks (in the city of Sendai, Japan) for use in emergencies (Takemoto et al., 2021). Recurrent solutions focus on delivering water in bottles or by tanker trucks and flexible tanks. In such cases, distribution is not always immediate, as it depends on the availability and accessibility of the necessary elements.
- Non-structural aspects (socio-cultural behaviors): The experiences of New Zealand and Chile show that confidence in the efficiency of institutions to distribute water promptly helped to avoid violence over access to water. Active neighborhood participation facilitated water distribution to the most affected areas (Balaei et al., 2019). In Japan, discipline, calm maintenance, public trust in state institutions, and planned scientific management contributed to minimizing conflicts over access to water and avoiding long queues (Zaré and Afrouz, 2012).
Recommendations from international organizations related to DRM (UN, 2015) are aligned with the Sendai framework approach:
- The World Health Organization (WHO) recommends the rational use of safe water sources, the design of mechanisms to transport water from these sources, and the decentralized management of the water system. Inspectors, NGOs, and organized communities must take responsibility for the water supply in their area during emergency scenarios, which require specialized training from the state.
- The Inter-American Development Bank (IDB) points out that post-disaster preparedness and response processes should integrate decentralization and equity in water management and implement alternative mechanisms to ensure immediate continuity of water supply. The IDB recommends effective strategies to adapt to emergency scenarios through redundancy of water supply and the formulation, dissemination, and application of response plans for water distribution.
2.2 Criteria for implementation of an emergency water provision system in urban areas
The guidelines of the Sendai Framework (2015–2030) aim to reduce and prevent new disaster risks through measures that integrate social, economic, political, legal, cultural, educational, environmental and institutional factors. These measures aim to reduce vulnerability to disasters by improving preparedness, response and recovery, i.e., by strengthening resilience.
Resilience is understood as the ability of a system to reorganize the relationships between its elements in order to withstand an external perturbation without collapsing and then returning to its initial state (Bruneau, Chang and Eguchi, 2003; Sharifi and Yamagata, 2018; Yepes, 2020). In urban planning, resilience refers to the ability of communities and urban infrastructure to withstand, adapt to, and recover from emergencies and disasters (UN, 2015).
A precision made by INDECI (2020) regarding resilient communities is relevant. A community is interpreted as a set of people who share customs, resources and culture; the effects generated by disasters, good practices, adequate organization, support networks and coexistence. When a community is resilient, it is constantly self-organizing to know how to assimilate, adapt, resist and recover from the impact of a natural or anthropogenic event that puts its lives and assets at risk.
A resilient city considers four interrelated dimensions (Bruneau et al., 2003; Yepes, 2020):
1. Technical dimension: The ability of urban physical systems to function at an acceptable or desirable level when affected by extreme events, based on four attributes:
Robustness: The system has no or few physical vulnerabilities (essentially structural), which allows it to withstand the impact of a critical event and provide an immediate response to the emergency scenario.
Redundancy: If major components of the system are severely damaged by the critical event (e.g., disruption of the domestic water supply), the system can use other elements that can replace the function of the damaged components.
Speed: The system can recover its functions after the disaster and provide an efficient response immediately or in the short term, which is particularly important because drinking water supply systems are typically only partially functional after 3 days, which is the maximum period of survival without water in the most densely populated urban areas.
Self-sufficiency: If the main components on which the system depends are severely damaged by the extreme event and their proper functioning is interrupted, other independent or alternative components of the system can function autonomously and temporarily provide sufficient water to the population.
2. Organizational dimension: The ability of the social organizations that manage the city’s critical infrastructure to respond immediately to the emergency in a self-sufficient and innovative manner.
3. Social dimension: Consists of actions specifically designed to reduce the impact of extreme events in the city that limit the provision of basic needs and generate greater inequality between social groups.
4. Economic dimension: Refers to the ability to reduce direct and indirect economic losses caused by extreme city events.
Meeting the emergency water needs of the population will be a major challenge for the humanitarian response. Existing plans do not define parameters for providing safe or drinking water in emergencies. Among the many parameters proposed by international institutions, the Sphere Project (2011) and the UN Refugee Agency (2023) are preferred references for calculating the minimum requirements in terms of quantity, distance and waiting time. Their recommendations for providing water to the affected people during the earthquake emergency are presented below:
- The maximum distance to the nearest water point should be 500 m.
- The waiting time at the water point should not exceed 30 min.
- The maximum number of people queuing should be 250 per tap at a flow rate of 7.5 L/min and can be up to 500 people at a flow rate of 17 L/min.
- As a reference, the minimum amount of water for cooking, personal hygiene and drinking is estimated to be 15 L/person/day.
- The maximum distance to the water point should be 200 m.
- The minimum amount of water for survival is estimated to be 7 L/person/day.
- It is recommended that each water point should be able to serve 80 to 100 people.
More information about water supply technologies is provided by Coerver et al. (2021).
The DRM policy guidelines reinforce the idea that providing safe water to the population in a disaster scenario should be organized in a system at the local government level. This system should articulate different levels of local government, public and private institutions, and therefore different urban scales, and guarantee the autonomy of neighborhood organizations in safe water distribution.
International experience shows that building trust in public institutions and knowledge of emergency plans and protocols are necessary to strengthen neighborhood participation. However, it is recognized that not all levels of government are articulated, and not all local governments have emergency response plans. Therefore, an urgent need is to provide alternative water supply in emergencies. These alternatives should prioritize social organizations as initiators and decision-makers for response actions. It is recommended that the implementation of urban resilience actions be balanced in its technical, organizational, and social dimensions, as well as in structural and non-structural measures.
3 Methodology for the design of the EWaSS
The proposal for a system for the emergency water supply system, EWaSS, is based on an earthquake scenario (magnitude, location, and fault-rupture geometry, USGS, 2023). The spatial analysis and design criteria for the components of the SSU consider the technical, organizational and social dimensions of the resilient city approach in three spatial scales: macro, meso and micro (Figure 1).
At the macro scale, public spaces are assigned as possible points of distribution, PoD. According to their feasibility, they are classified as optimal, viable, or non-viable. The level of concentration or dispersion of feasible public spaces is analyzed to define the conditions of service of the EWaSS.
Thematic layers of the urban and socio-spatial structure, quantitative and qualitative information (e.g., population density, socioeconomic strata, roads and land uses, level of probable damage, seismic micro-zoning and public spaces) are superimposed, spatialized, and systematized with GIS (Arc View, Google Earth, Street View) to determine the macro strategies for emergency attention. This process is complemented by qualitative tools such as a risk perception survey. Participatory activities with decision-making actors, such as work tables, workshops, and in-depth interviews, are mandatory.
In addition to the spatial analysis, it is recommended that agreements for providing safe water and budget allocation be made at public management levels. It is also necessary to review the adjustments or creation of standards and other regulations that allow for the effective implementation of a safe water supply system in emergencies.
At the meso scale, a first approximation of the SSU networks in urban sectors (for example, districts) is identified. SSUs are delimited by the superposition of thematic urban information: main roads, population density, land uses, and the PoD classification done in the macro-scale analysis. International recommendations for the maximum distance to the PoD, the minimum provision of water, and the minimum waiting times are considered to define the SSU area in an iterative process to fulfill the technical and urban aspects.
It is recommended that the EWaSS be articulated with the existing DRM plans, the location of road infrastructure and shelters. Earthquake drills must include the operation of the EWaSS. Special attention must be paid to the areas lacking feasible public spaces.
The micro-scale phase consists of the design of the SSU physical components: reservoirs, meeting points, paths to the PoD, and the public spaces to install the PoD. These structural measures are complemented by non-structural measures composed of protocols for activating the EWaSS and audiovisual materials for communication and dissemination. The autonomous functioning of the SSU is based on the willingness and capacity of neighborhood organizations to actively participate and assume responsibilities in the operation in the event of an emergency.
Complementary elements are included for autonomous operation: signage, alternative lighting, furniture, equipment and materials for use and maintenance. The SSUs are designed according to the characteristics of the possible public spaces (optimal, viable, or not viable), following current regulations and considering that these can be adapted to the particular needs of the neighborhoods.
4 Case study spatial analysis in the metropolitan area of Lima-Callao
According to census data, the city has a population of 9,569 468 and an urban area of 101,170 ha (INEI, 2017). In this area, the density varies in three ranges: up to 150 inhabitants/ha, 151 to 400 inhabitants/ha, and over 400 inhabitants/ha. In the central area, the density is beginning to be lower than in the peripheral expansions due to the predominance of smaller lot sizes and the recent process of high-rise housing and condominium construction in the peripheral expansions. The Lima-Callao metropolitan area is a political-administrative division of the province of Lima. Lima has 43 districts, and the Constitutional Province of Callao has 7.
Since the 1990s, the conformation of the urban territory of the metropolitan area of Lima-Callao has been conditioned by (a) the political, economic and social changes brought about by the Structural Adjustment Program of 1990, which followed the guidelines of the neoliberal model, and (b) the characteristics of its location in a coastal area with foothills in the Andes and facing the Pacific Ring of Fire, where the Nazca and South American plates interact in a subduction process that causes high seismic activity.
Under these conditions, the urban structure of the metropolitan area shows accelerated growth due to important private investments that combine formal and informal urbanization processes. The diffuse morphology of the city shows clear physical and functional differences between the central zone and the expansion zone towards the periphery. Its main road axes exceed 100 km from north to south and 60 km from east to west (Figure 3). Several smaller radial axes emerge from these main axes, making the city sprawling, low-density, and with few service centers. This expansion occupies unmitigated risk areas due to the soil quality and the buildings built on it (sandy areas, more than 20% slopes, use of adobe and non-resistant materials, and precarious construction).
4.1 DRM in Lima-Peru
In Peru, there have been positive advances in DRM that would make it possible to plan for alternative systems to provide safe water in the post-disaster emergency of a major earthquake and for affected populations to be prepared and take responsibility in such a scenario. The National Policy for Disaster Risk Management 2050 (PCM, 2021) follows the Sendai Framework’s guidelines for prospective, corrective and reactive management. The National Center for the Estimation, Prevention and Reduction of Disaster Risk (CENEPRED) and the National Institute of Civil Defense (INDECI) are responsible for preparedness, response and recovery, and reconstruction, respectively.
The National Strategic Planning Center (CEPLAN) coordinates the integration of DRM into the National Development Strategic Plan. It includes the participation of private and civil society organizations responsible for responding to emergencies. The plan also emphasizes that participation is both an obligation and a right, so all private natural or legal persons should align their actions with the national DRM policy. This plan also includes the participation of the regional and local governments that make up the National Disaster Risk Management System (SINAGERD).
In Peru, the Ministry of Environment, the Ministry of Defense, the Ministry of Housing, Construction and Sanitation (MCVS), INDECI, CENEPRED, municipalities and service providers are the main institutions responsible for preparedness and response actions for water supply. Therefore, they must be articulated to maintain the continuity of the water supply or restore its operation during the emergency scenario, as stated in the Regulations for the Identification, Evaluation and Risk Management of Critical National Assets (Supreme Decree No. 106-2017-PCM, 2017).
The guidelines of the National Policy for DRM 2050 integrate public and private institutions and focus actions on the restoration of the traditional drinking water system. The recommendations of the WHO and the IDB are incorporated in the immediate or short-term measures of the Peruvian regulations. The National Emergency Operations Plan (PNOE) (PCM, 2022) indicates that public institutions are responsible for providing alternative means of access to water, which should be coordinated with INDECI. These alternatives include bottled water and water purification tablets, among others. The PNOE indicates that coordination should be articulated, although not all levels of government have a specific plan for the first emergency response after a disaster.
DRM guidelines are being incorporated into laws and regulations so that the central, regional and local levels of government have the necessary means to coordinate management actions, such as water supply in emergencies. The National DRM System is in charge of formulating and approving standards and plans and evaluating, directing, organizing, supervising, monitoring, controlling, and executing processes following DRM-related laws and regulations. Specifically, the following legal framework (Table 1) will be taken into account to identify sites for the location of the alternative water supply system:
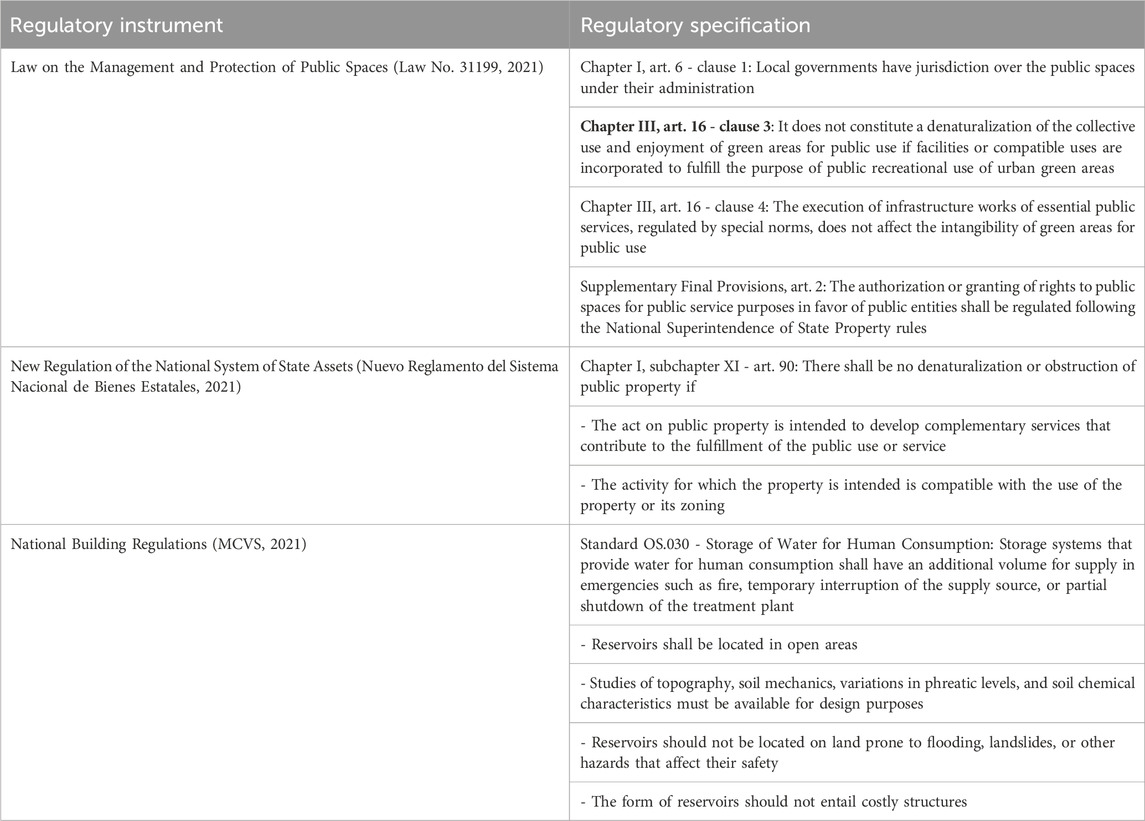
Table 1. Legal framework for the design and implementation of the Emergency Water Supply System (EWaSS) in public spaces.
The guidelines for the implementation of the Emergency Water Supply and Response Protocol for the Affected Population are based on the Emergency Operations Plan for the Province of Lima 2021–2023 (MML, 2021a) and the Contingency Plan for a Large-Scale Earthquake Followed by a Tsunami in the Province of Lima 2021–2023 (MML, 2021b) (which does not include the Constitutional Province of Callao). Based on these guidelines, the phases for implementing immediate or short-term measures for water supply after a large earthquake are outlined (INDECI, 2015) (Table 2).
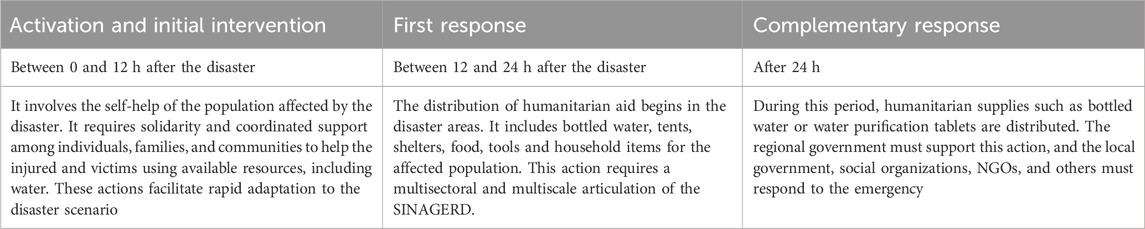
Table 2. Phases of emergency response after an earthquake (INDECI, 2015).
As part of its emergency plan, the city water company SEDAPAL has some facilities (static tanks, reservoirs and wells) that would be operational after an 8.8 Mw earthquake to supply water to the nearby population, independently of the regular supply network, which would be interrupted due to infrastructure damage. In the scenario of a possible disaster leading to a shortage of drinking water, SEDAPAL would be responsible for delivering bottled drinking water to the population in need within 24–72 h. Subsequently, bladders or flexible tanks should be installed within a period ranging from 24 h after the earthquake to the seventh day, as indicated by the Metropolitan Municipality of Lima (MML, 2014).
4.2 Earthquake scenario
According to the Institute of Geophysics of Peru, there is enough accumulated energy to generate an earthquake of magnitude Mw 8.8 (CENEPRED, 2020) in front of the Lima-Callao coast. In this scenario, 45% of its population would experience a high level of damage, 33% a medium level of damage, and 25% a low level of damage (CISMID, 2013). In this area, 41% of the urban area would collapse or suffer severe damage to its buildings, which is considered a high level of damage; 33.43% would suffer moderate damage; and 25.37% would suffer light or no damage. The grouping of high, moderate and light corresponds to the five levels of damage (CENEPRED, 2020), as shown in Table 3.
In the case of the Lima-Callao metropolitan area, the emergency facilities of SEDAPAL would be able to serve only 10% of the population living near them (an estimated 500 m walk). Meanwhile, 90% of the metropolitan area would be left without safe water after the 8.8 scenario. In this area, 74.79% of the population lives in places with buildings that would likely collapse or be highly or moderately damaged by the impact of a seismic event, and only 25% of the population lives in places with buildings that would suffer only light damage (Figure 2).
4.3 Spatial analysis of the macroscale: the metropolitan area
Currently, a large part of the city would face a serious situation after a major earthquake. These unserved areas of the city correspond to the neighborhoods with the largest number of inhabitants. In Figure 4D, the red areas are unserved zones corresponding to recently densified urban settlements.
At the metropolitan scale, the criteria for the location of the EWaSS have been identified using the local management standards, according to which it is feasible to occupy public green spaces (Law No. 31199, 2021). The viability of use for water supply is determined based on the characterization of the urbanized area in terms of seismic microzonation, level of housing damage, population density and socioeconomic strata. There are three conditions for public green spaces: optimal, viable and non-viable (Figure 3; Table 4). The area to be supplied has a radius of 500 m from the household, which is a feasible distance to cover carrying the weight of water, according to the Sphere Project (2011).
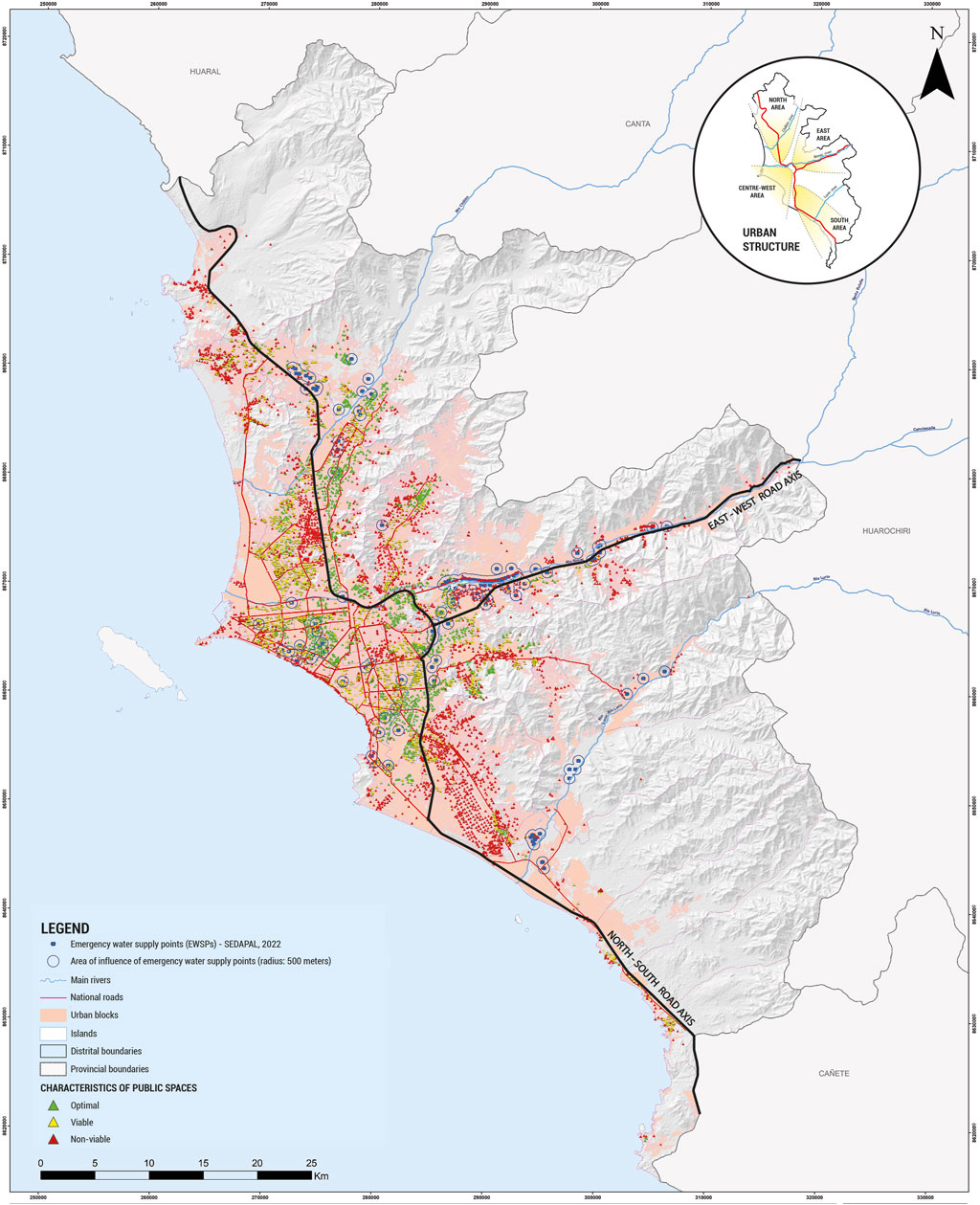
Figure 3. Classification of public spaces into optimal, viable and non-viable for the location of EWaSS water supply points.
According to Table 4, the metropolitan area is characterized in terms of the location of water supply points:
a) Non-viable public spaces (36.2% of the total public spaces identified). They are predominant in the peripheral expansion zones. Based on the north-south and east-west road axes, the characteristics of these spaces are described (Figure 3).
- Towards the north and northeast, a clear concentration of non-viable public spaces is identified, forming extensive but non-continuous patches, especially at the edges of the urban sprawl. In these areas, the population density varies, and the lower socioeconomic class predominates. In the northern end, with low density, the main reason for the non-viability of public spaces is the damage that would be caused to the surrounding houses due to the low quality of the buildings. In the northeastern zone, of medium and low density, located on the edges of the urban sprawl, the main reason for the non-viability is the low quality of the soil, the slopes of more than 20% and the possible damage to the housing. Moreover, as for the high-density northern zone near the center, non-viable public spaces are predominantly located in areas of severe damage and collapse (Figure 4D).
- To the east, the distribution of non-viable public spaces is scattered in the ravines of the hills. These are places with a high density on the left bank of the Rimac River (Figure 4C), a medium density on the right bank, and a low socioeconomic stratum. According to the seismic microzoning (Figure 4A), the soil is of type I. It should be noted that the non-viable public spaces in the ravines of the Rimac River are exposed to the risk of landslides and mudslides.
- Towards the south, there is a high concentration of these spaces, which form an extensive patch in an area consisting of sandbanks, with a high density of land occupation and a low socioeconomic stratum. The main causes of the non-viability of these public spaces are the seismic microzonation, since soils of type III and IV predominate, and the levels of serious damage and collapse due to the low quality of housing construction. In addition, some areas on the coast are exposed to soil liquefaction.
- Towards the center and west, the public spaces of the non-viable classification form smaller patches than in the previous zones and are scattered, especially near the Pacific Ocean, on the “Costa Verde” (Green Coast). A high socioeconomic class predominates, with a lower presence of the middle socioeconomic class. The seismic microzonation is mainly type I soil. The level of damage varies: the moderate level predominates, with areas where the damage would be superficial and light; however, in the historical areas, the damage would be severe, and some areas are at risk of collapse.
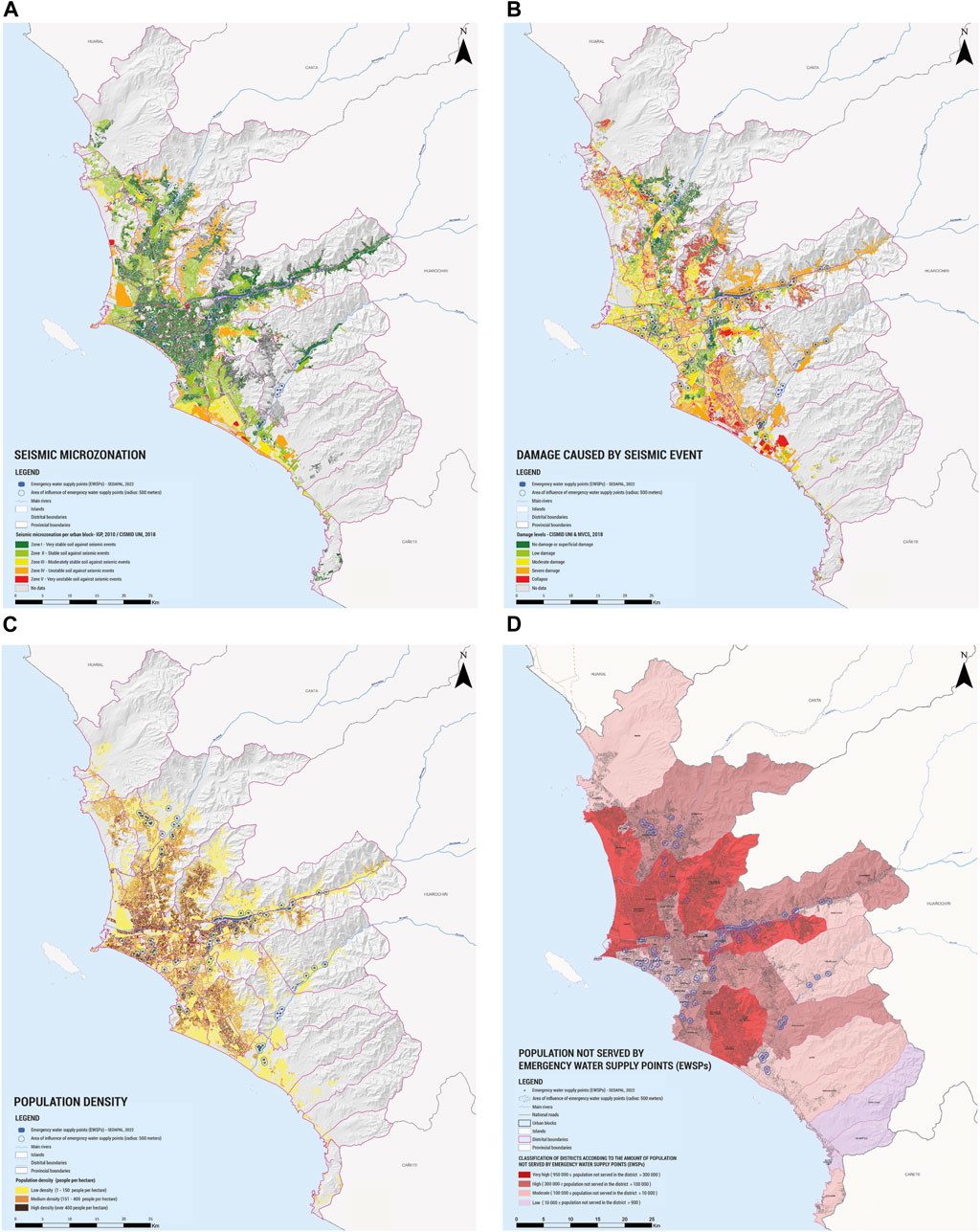
Figure 4. Spatial analysis using thematic map overlays (A) Seismic microzonation. (B) Damage caused by a seismic of 8.8 Mw. (C) Population density. (D) Classification of districts according to the amount of population not served by emergency water supply points.
In this scenario, it has been determined that non-viable public spaces, where it is not feasible to install a reservoir, represent 36.2% of the total number of public park spaces in the metropolitan area of Lima-Callao. Therefore, it is necessary to integrate the population with non-viable public spaces into the system through nearby viable SSUs. Alternatively, if this is not possible, other alternative solutions must be found to provide water during emergencies.
b) Viable public spaces (54.5% of the total public spaces identified). They are the most common in the metropolitan area, forming large and continuous areas. They are predominant along the north-south road axis, where the socioeconomic strata are medium, low and high, and the density is relatively high. Towards the center-west road axis, the high socioeconomic class predominates, with a lower presence of the middle class and high and medium densities. In both sectors, viable public spaces are located in seismic microzoning zones I and II; however, towards the coast (where the soil presents the possibility of liquefaction), there are zones of type IV. The level of damage is mostly moderate. The proportion of viable public spaces decreases towards the east and south axes.
Viable public spaces make up 54.5% of the total public spaces in parks. Even in this classification, specifics should be examined in order to achieve adequate emergency water SSUs for their inhabitants. It was found that this main category is scattered in the metropolitan area and in close proximity to non-viable public spaces. It provides an opportunity to provide water to residents who do not have a viable public space, although the distance may be greater than the recommended 500 m.
c) Optimal public spaces (9.3% of the identified public spaces). They are representative of the north and the center-west road axes.
- Towards the north and northeast, the concentrations of optimal public spaces form medium-sized and scattered patches. In these places, the predominant socioeconomic stratum is low, with a lower presence of the middle stratum and very high density. The microzoning corresponds to zones I and II, and the damage levels are minor or no damage.
- To the east, optimal public spaces are concentrated in a single sector with small patches, where the socioeconomic stratum is high and medium, and the population density is medium. The seismic microzonation corresponds to zones I and II, and the levels of damage are light and no damage.
- Towards the center-west, the concentration of optimal public spaces is significantly higher than in the previous road axes. In this area, the socioeconomic stratum is predominantly high, and the density is medium, with high-density sectors. Regarding seismic microzoning, the soils correspond to zone I, which favors light damage and no damage levels.
Optimal public spaces represent only 9.3% of the public park spaces for the location of water supply points in the metropolitan area of Lima-Callao. They correspond to places of middle and high socioeconomic strata, where the levels of damage are light and null, and their density is medium and high. All these conditions must be taken into account when making decisions. This small category is scattered throughout the metropolitan area, which requires studies on a smaller scale to determine the possibility of serving the residents of nearby non-viable public spaces.
In summary, the results at the metropolitan level indicate that it is possible to locate SSUs in different locations along the north-south and east-west road axes. This will facilitate a balanced distribution of SSUs. However, the classification corresponding to the optimal public spaces is clearly small, while the classification corresponding to the viable public spaces, although predominant, requires specific studies to be carried out to ensure the proper operation of the SSUs. Both offer the possibility of serving residents whose public spaces are not viable, thus serving vulnerable sectors exposed to greater damage in the event of a major earthquake.
In this macro-spatial scenario of an 8.8 Mw earthquake, a survey was conducted among the metropolitan area’s inhabitants to identify aspects related to non-structural measures (Figure 5). The features of the survey are shown in the appendix. The survey highlighted the following results:
a) According to the respondents, the most important activities in the first moments after the earthquake are caring for the injured (64%) and seeking shelter (59%), followed by food (51%) and water (37%).
b) Regarding the people who could help the most quickly to get water after an earthquake, the police, army and firefighters were mentioned first (35.7%), followed by neighbors (35.4%), SEDAPAL (40%), the municipality and INDECI.
c) When asked about the best places to store and provide water, the respondents mentioned parks, green areas and sports fields.
These results align with the previously mentioned problem of low-risk perception about not having water during the 3 days following an earthquake, which was not mentioned as a top priority. Regarding the participation of local actors, the responses are mixed. These results show that the SSUs of the EWaSS can function as a local system in decision-making and implementation and that they function autonomously in water distribution during the emergency, managed by the neighbors. Similarly, public spaces are prioritized for water storage and distribution.
It is worth noting that residents’ responses are directly or indirectly related to the different levels of government, risk management institutions, and civil society. It aligns with the processes outlined in the National Policy for Disaster Risk Management 2050 (PCM, 2021), embedded in various regional and local urban planning regulatory instruments.
Local actors in a participatory workshop held in October 2022 pointed out that the city’s diversity must be considered in the EWaSS design. A meeting with the Board of SEDAPAL was held at the beginning of this project to establish ways of collaboration for population risk reduction.
4.4 Mesoscale spatial analysis: districts
The mesoscale analysis examines the spatial conditions that make it possible to identify SSU networks at the district level. For this purpose, two binding conditions are prioritized: (a) accessibility (road hierarchy, availability and proximity of public spaces) and (b) social vulnerability (organizations in urban sectors, population density and level of damage per block). The case of the district of San Miguel is presented, a zone that, based on the macroanalysis, has viable and non-viable public spaces for the location of water supply points (Table 5; Table 6; Figure 6).
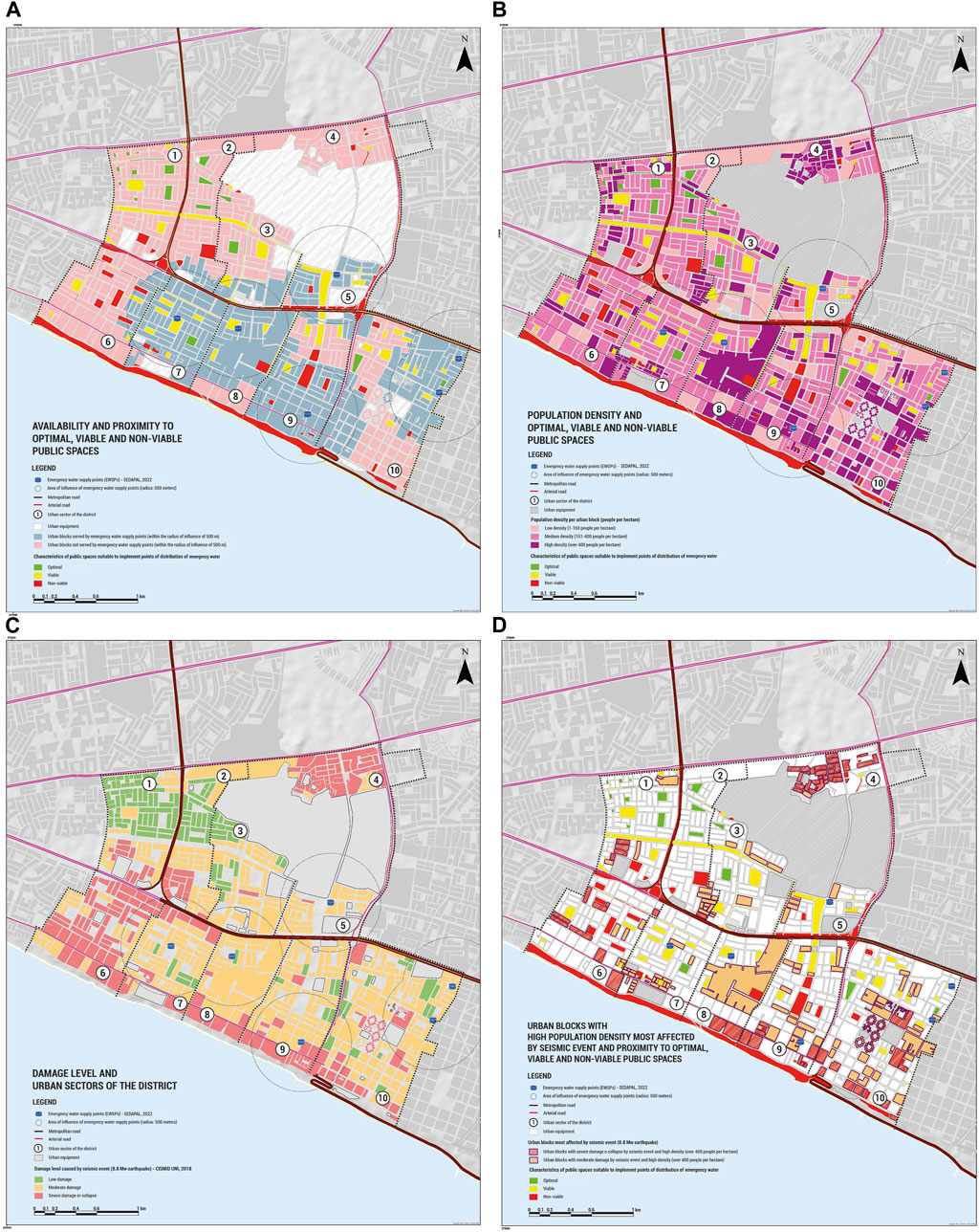
Figure 6. Mesoscale spatial analysis using thematic map overlays (A) Availability and proximity to optimal, viable, and non-viable public spaces. (B) Population density and optimal, viable and non-viable public spaces. (C) Damage level and urban sectors of the district. (D) Urban blocks with high population density most affected by seismic event and proximity to optimal viable and non-viable public spaces.
Based on these characteristics, three groups of urban sectors (division of the district into sectors with more or less homogeneous urban characteristics) are identified with delegates elected by the residents. The delegates are responsible for guiding the decisions of local management actors and residents, aiming to form and articulate SSU networks that function as a local system. This objective articulates aspects of non-structural measures of local management, for which the regulations of the Disaster Risk Management Plan will be taken into account, in accordance with the Urban Development Plans and the Law on the Management and Protection of Public Spaces, as well as those of other relevant public bodies.
Common criteria will be applied to heterogeneous sectors, considering their prevailing characteristics. SSUs will serve their residents and, if necessary, the nearby population that does not have viable public spaces.
Considering the density population is 75 inhabitants per Ha, the provision is 2.5 L per person per day for 3 days (72 h of operation during the first moments of the earthquake emergency) and a maximum distance of 200 m to the PoD, the volume of the reservoir is estimated as 9.5 m3 approximately. This number should be adjusted in the micro-scale analysis considering the actual conditions of the public space and the surroundings, such as soil type and existing urban equipment.
4.5 Microscale spatial analysis: neighborhoods
The microscale spatial analysis provides the conditions for a precise location within the public space and the criteria for designing the SSUs at the neighborhood level, shown in Table 6. The aim is to move from theoretical design to the necessary adjustments for practical implementation involving local stakeholders, including local management and neighbors. The physical and usage characteristics of public spaces, users and existing neighborhood organizations are identified. In the spatial analysis, structural and non-structural measures are linked through technical, organizational and social dimensions (dimensions of a resilient city). Fieldwork and participatory–collaborative activities with local actors complement the desk work. The spatial analysis allows the research team to define the following SSU components:
(a) Meeting points are defined in public spaces that neighbors recognize as having safe physical characteristics and modes of use.
b) Access routes and signage to safe water supply points, which coincide with evacuation routes in the event of an earthquake. They are defined as streets and avenues whose physical characteristics and those of the surrounding buildings are safe.
c) Water supply points are located in parks and coincide with the safe areas of these public spaces. They preserve the design of recreational spaces and green areas and contribute to their improvement.
For the case study at the microscale, urban sector No. 4 in the district of San Miguel was selected as a priority and divided into two contiguous subsectors with public spaces of non-viable and viable classification. A third subsector was selected in the district of Lima, adjacent to sector No. 4, with optimal public spaces (Figure 7).
Accessibility, location and type of safe water supply points are three criteria for the design of SSU components. A relevant condition for creating the design proposal was the need to respect the spatial composition of public spaces so that the inclusion of the EWaSS would contribute to improving these spaces.
a) For accessibility at the microscale, the physical conditions of public road spaces and their hierarchies, the function of recreational and green spaces, and how different users use them are studied. Meeting points, evacuation routes, and safe areas with safe water supply points are identified on this basis.
In practice, the spaces, even if they are optimal, do not always meet the appropriate conditions for accessibility, as there may be streets closed by security fences, occupied by poorly parked vehicles and street vendors, lacking maintenance, with overhead electrical wires, and the like (Figure 8). Therefore, proposals should be made for actions that combine structural and non-structural measures to enable the future implementation and use of meeting points, evacuation routes and safe areas.
(b) The location of the water supply point and the signage at the microscale considers the existence of active and non-active neighborhood organizations, in addition to what has been indicated in the macro-and mesoscales regarding the hierarchy of roads and the classification of public space. It aims to contribute to non-structural measures so that the EWaSS can have an autonomous operation run by neighbors during an emergency. According to the National DRM Policy, citizen participation can occur through neighborhood committees, parish committees, sports associations, educational institutions and other similar bodies in the area. The signage should be visible day and night in public spaces, businesses, and other local landmarks in the neighborhood. It is recommended that official DRM signage be used and supplemented with EWaSS signage to facilitate access and use of the SSU (Figure 9).
In the case of neighborhoods where public spaces are not viable, the closest possible space will be identified, even if its distance exceeds the 500 m recommended for the location of the water supply point. Similarly, recommendations should be made to the local government to improve the design of pedestrian crossings and the reinforcement of structures facing evacuation routes that could be damaged and impede access to the water supply point. These recommendations should be applied in neighborhoods in non-viable areas where neighbors have access to water in the nearest viable area due to the impossibility of having a safe water supply in their neighborhood. In general, it should also be taken into account that the physical conditions and the use of meeting points and evacuation routes do not always have the qualities required to facilitate access to safe water supply points (Figure 9).
c) Type of safe water supply point. Considering that there will be no electricity or alternative energy sources during the first hours after the earthquake, using reservoirs with pumps, as in the case of buried tanks, is excluded. Therefore, it is suggested that the reservoirs be elevated, above ground and semi-buried, with water distribution mechanisms that are clearly visible and manual to facilitate access.
The future implementation of the SSU requires that its facilities (reservoirs, signage, solar panels, storage compartments) be part of viable and recreational public spaces, that they function day and night, and that they do not interfere with daily uses (Figure 10). In addition, local authorities are required to take measures to improve the structure of some facilities. They must also train neighbors to use the facilities autonomously, take care of the equipment, and understand the protocols for use in an emergency. This includes the provision of water containers, waiting times, amount of water per person per day, solidarity assistance, and other procedures for access to safe water.
The link between the research, the USR course, and the participation of the citizens was key to knowing and jointly defining the criteria for the location and design of the SSU components. Likewise, the fieldwork shows that each neighborhood has specificities in its technical, organizational and social conditions, regardless of whether the public spaces are optimal, viable or non-viable. These specificities are related to information that cannot be captured in official sources, such as details on population density, distances, and physical aspects of public spaces that can facilitate or hinder the collective use of meeting points, evacuation routes, and safe areas as a system.
Neighborhood organizations also contribute to the definition of structural measures, such as the size of reservoirs, the number of supply points, maintenance, and the organization of distribution during emergencies. Strengthening active and non-active neighborhood organizations is essential for effective neighborhood behavior during an emergency.
From an academic point of view, feedback from the results of the social impact research, the USR course, and the social actors is fundamental to the development of scientific knowledge at the microscale. Equally important at the microscale, these experiences strengthen society’s trust in public services and science. A closer approach to the specificities of each place and each social group is essential for DRM in the emergency of providing safe water to the affected population, as it will contribute to ensuring water privision and distribution during the first 72 h immediately after a high-intensity earthquake.
4.6 Discussion
Based on the analysis at the metropolitan (macro) level, a solidary and equitable solution to the emergency is proposed among the districts of the metropolis, regardless of socioeconomic status, population density and the state of public space. Ninety percent of the population would not have a domestic water supply in the first 72 h of the emergency, and one-third of the urbanized territory of Lima-Callao does not have the conditions for installing an EWaSS. Moreover, most of this territory consists of residential areas that are highly vulnerable, both physically-spatially and socio-economically. Therefore, it is proposed that areas with viable and optimal public spaces can share water with non-viable areas. To this end, public management actors must plan actions to ensure emergency response.
At the district level (meso), in the municipality of San Miguel, collaborative participation activities with local management actors made it possible to arrive at criteria consistent with DRM plans and relevant to urban conditions. In addition, the municipal authorities recognized the need to strengthen DRM capacities based on the proposed vision of spatial analysis and the feasibility of including it in their budgets. Based on this analysis, the conformation of EWaSS networks is proposed according to political-administrative subsectors, urban structure, and the availability of optimal public spaces, whether viable or not.
At the microscale (neighborhood), the design of the SSUs transforms the theoretical approach into a concrete and specific reality that may differ from the results at the meso and macro levels, even between adjacent neighborhoods at the microscale. For example, it is worth considering that the optimal and viable spaces were hampered by improper occupation of the streets and an irregular urban layout that hindered accessibility. These situations could be seen in the fieldwork carried out at the microscale. The application at the microscale allows the adjustment of the radius of influence of 500 m of the public spaces classified as viable and optimal to polygons defined by the hierarchy of the street structure, the organization of the neighbors in the neighborhoods, and the dimension and availability of public spaces.
Similarly, through participatory activities at the microscale, decision-makers and neighbors recognize their low level of resilience. At the same time, they show interest and commitment to participate in the future implementation of the EWaSS. In this project, it is proposed that the protocols be designed in a participatory way, based on the interest of the neighbors, valuing their knowledge of their neighborhoods and that these protocols be included in the emergency plans of the DRM.
5 Conclusion
The spatial analysis method proposed to define design criteria in the context of an emergency response manages to integrate territorial, resilience and participatory approaches, yielding results that improve the response capacity at different spatial scales and levels of decision-making of local public management actors and neighbors. The method provides a comprehensive vision in DRM for planning and managing an emergency response in case of high-intensity earthquakes. It also helps to control the rise of new social risks during the emergency. In addition, collaborative participation enables neighbors to exercise their civic rights and responsibilities, move beyond low-risk perceptions, reduce vulnerability, and increase resilience.
The following steps for this spatial analysis method are to transform it into an interactive and accessible tool that will be the basis for developing DRM plans for safe water supply in accordance with the participatory approach. Likewise, these actions can be linked to other local DRM management plans for emergency prevention and response.
Data availability statement
The raw data supporting the conclusion of this article will be made available by the authors, without undue reservation.
Author contributions
SS-C: Conceptualization, Data curation, Formal Analysis, Funding acquisition, Investigation, Methodology, Project administration, Resources, Supervision, Validation, Visualization, Writing–original draft, Writing–review and editing. GC: Conceptualization, Data curation, Formal Analysis, Investigation, Methodology, Supervision, Validation, Writing–original draft, Writing–review and editing. MV: Conceptualization, Data curation, Formal Analysis, Investigation, Methodology, Supervision, Validation, Writing–original draft, Writing–review and editing. JP: Conceptualization, Formal Analysis, Investigation, Methodology, Software, Visualization, Writing–original draft, Writing–review and editing. MS-M: Data curation, Formal Analysis, Software, Visualization, Writing–original draft. KM: Data curation, Formal Analysis, Software, Visualization, Writing–original draft.
Funding
The author(s) declare that financial support was received for the research, authorship, and/or publication of this article. Project funded by Vicerrectorado de Investigación–Pontificia Universidad Católica del Perú, contract PI0841.
Acknowledgments
The microanalysis was developed by students in the course on University Social Responsibility USR Topics 1: Risk Management in the Project Process, Faculty of Architecture and Urbanism, PUCP.
Conflict of interest
The authors declare that the research was conducted in the absence of any commercial or financial relationships that could be construed as a potential conflict of interest.
Publisher’s note
All claims expressed in this article are solely those of the authors and do not necessarily represent those of their affiliated organizations, or those of the publisher, the editors and the reviewers. Any product that may be evaluated in this article, or claim that may be made by its manufacturer, is not guaranteed or endorsed by the publisher.
Supplementary material
The Supplementary Material for this article can be found online at: https://www.frontiersin.org/articles/10.3389/fbuil.2024.1385476/full#supplementary-material
References
Aguilera, F., Rodríguez, V., and Gómez, M. (2018). Definición de infraestructuras verdes: una propuesta metodológica integrada mediante análisis espacial. Doc. d’Anàlisi Geogràfica 64 (2), 313–337. doi:10.5565/rev/dag.419
Alberto, Y., and De la Llera, J. (2016). “Damage to the water system in the earthquakes of Chile 2010 and Japan 2011,” in 1st international conference on natural hazards & infrastructure (ICONHIC), chania, crete. [Conference].
Alberto, Y., De la Llera, J.C., Aguirre, P., Monsalve, M., and Molinos, M. (2022). Comparative qualitative and quantitative analyses of the seismic performance of water networks during the maule 2010, christchurch 2010–2011, and tohoku 2011 earthquakes. J. Water Resour. Plann. Manage. 148(4), 04022004. doi:10.1061/(ASCE)WR.1943-5452.0001520
American Lifelines Alliance (ALA) (2001). “Seismic fragility formulations for water systems,” in ASCE and FEMA (American Lifelines Alliance).
Balaei, B., Wilkinson, S., Potangaroa, R., Adamson, C., and Alavi-Shoshtari, M. (2019). Social factors affecting water supply resilience to disasters. Int. J. Disaster Risk Reduct. 37 (8), 101187. doi:10.1016/j.ijdrr.2019.101187
Barredo, J. (1996). Sistema de información geográfica y evaluación multicriterio en la ordenación del territorio. Editorial RA-MA, Spain.
Bocco, G., and Urquijo, P. (2013). Geografía ambiental: reflexiones teóricas y práctica institucional. Región Soc. 25 (56), 75–102. doi:10.22198/rys.2013.56.a100
Bruneau, M., Chang, S. E., Eguchi, R. T., Lee, G. C., O'Rourke, T. D., Reinhorn, A. M., et al. (2003). A framework to quantitatively assess and enhance the seismic resilience of communities. Earthq. Spectra 19 (4), 733–752. doi:10.1193/1.1623497
Coerver, A., Ewers, L., Fewster, E., Galbraith, D., Gensch, R., Matta, J., et al. (2021). Compendium of water supply technologies in emergencies. Berlin. Germany: German WASH Network, University of Applied Sciences and Arts Northwestern Switzerland, Global WASH Cluster and Sustainable Sanitation Alliance.
EPA - United States Environmental Protection Agency (2011). EPA600/R-11/054 www.epa.gov/research Planning for an Emergency Drinking Water Supply.
Federal Emergency Management Agency (FEMA) (2004). “Food and Water in an Emergency.” [Pamphlet.] Federal Emergency Management Agency and American Red Cross. FEMA 477 A5055. http://www.fema.gov/pdf/library/f&web.pdf.
Fernandez de Cordova, G. D. C., Vilela, M. R., and y Peña, R. M. (2017). “Aportes al anteproyecto de ciclovía en el Jr. Santa Ana, Purrumpampa, Huamachuco,” in Transversal acciones de integración en el territorio peruano (LIMA, Peru: Fondo Editorial PUCP), 531–536.
Inter-American Development Bank (IDB) (2019). Guía para la gestión del riesgo en sistemas de agua y saneamiento ante amenazas naturales. in Guide to risk management in water and sanitation systems in the face of natural hazards. Editors D. Arteaga, J. Ordóñez, and M. Basani Available at: https://publications.iadb.org/es/guia-para-la-gestion-del-riesgo-en-sistemas-de-agua-y-saneamiento-ante-amenazas-naturales.
Intergovernmental Panel on Climate Change (IPCC) (2012). Informe especial del grupo intergubernamental de expertos sobre el cambio climático. [Special report of the intergovernmental group of experts on climate change] (Technical report). https://www.ipcc.ch/site/assets/uploads/2018/09/AC6_brochure_es.pdf.
Lavell, A. (2005). Los conceptos, estudios y práctica en torno al tema de los riesgos y desastres en América Latina: evolución y cambio, 1980-2004: el rol de la red, sus miembros y sus instituciones de apoyo. Costa Rica: FLACSO-Secretaría General. Available at: https://biblioteca-repositorio.clacso.edu.ar/handle/CLACSO/724.
Law No. 31199 (2021). Ley de Gestión y Protección de los Espacios Públicos [Law on the Management and Protection of Public Spaces]. Available at: https://busquedas.elperuano.pe/dispositivo/NL/1955580-1.
Liu, W., Song, Z., Ouyang, M., and Jie, L. (2020). Recovery-based seismic resilience enhancement strategies of water distribution networks. Reliab. Eng. Syst. Saf. 203, 107088. doi:10.1016/j.ress.2020.107088
Metropolitan Municipality of Lima (MML) (2014). Decreto de Alcaldía N.º 008. Protocolo de actuación para respuestas a emergencias en agua, saneamiento e higiene en Lima Metropolitana [Mayoral Decree No. 008. Action protocol for emergency response to water, sanitation and hygiene emergencies in Metropolitan Lima]. Available at: https://cdn.www.gob.pe/uploads/document/file/2583530/2014-Decreto%20de%20Alcaldia%20008.pdf.pdf.
Metropolitan Municipality of Lima (MML) (2021b). Plan de contingencia ante un terremoto de gran escala seguido de tsunami en la provincia de Lima 2021-2023 [Contingency plan for a large-scale earthquake followed by a tsunami in the province of Lima 2021-2023]. Available at: https://www.munlima.gob.pe/images/PC_Ante_Sismo_y_Tsunami_MML-_V_11-5-2021_-Vfinal_VB-SDC.pdf.
Metropolitan Municipality of Lima (MML) (2021a). Plan de operaciones de emergencia de la provincia de Lima, 2021-2023 [Emergency operations plan for the province of Lima, 2021-2023]. Munic. Lima. Available at: https://smia.munlima.gob.pe/uploads/documento/79d3c24a92900743.pdf.
Ministry of Housing, Construction and Sanitation (MVCS) (2021). Reglamento Nacional de Edificaciones (RNE). National Building Regulations. Available at: https://www.gob.pe/institucion/vivienda/informes-publicaciones/2309793-reglamento-nacional-de-edificaciones-rne.
Moschella, P. (2018). Peri-urbanization and land management sustainability in peruvian cities. Dr. thesis, Univ. de Strasbourg. Hal Open Sci. Available at: https://theses.hal.science/tel-02144701.
National Center for the Estimation, Prevention and Reduction of Disaster Risk (CENEPRED) (2020). Escenario de riesgo por sismo y tsunami para Lima y Callao [Earthquake and tsunami risk scenario for Lima and Callao]. Available at: http://sigrid.cenepred.gob.pe/sigridv3/storage/biblioteca/10354_escenario-de-riesgo-por-sismo-y-tsunami-para-lima-y-callao.pdf.
National Institute of Civil Defense (INDECI) (2015). Informe de identificación de rutas de evacuación y zonas seguras ante tsunamis, 2015 [Report on the identification of evacuation routes and tsunami safe zones, 2015]. Available at: https://portal.indeci.gob.pe/wp-content/uploads/2019/01/201710241613271-1.pdf.
National Institute of Civil Defense (INDECI) (2020). Caminando hacia la resiliencia comunitaria frente a desastres: Guía práctica para autoridades y líderes comunitarios [Moving towards community resilience to disasters: A practical guide for authorities and community leaders]. INDECI. Available at: https://cdn.www.gob.pe/uploads/document/file/1289119/GUIA%20DE%20RESILIENCIA%20COMUNITARIA%20-%20INDECI.pdf.
National Institute of Statistics and Informatics (INEI) (2017). Resultados definitivos de los Censos Nacionales de 2017, provincia de Lima [Final results of the 2017 National Census, province of Lima]. Available at: https://www.inei.gob.pe/media/MenuRecursivo/publicaciones_digitales/Est/Lib1583/.
Nuevo Reglamento del Sistema Nacional de Bienes Estatales (2021). New regulation of the national system of state assets. Available at: https://busquedas.elperuano.pe/dispositivo/NL/1942689-4.
Peruvian Geophysical Institute (IGP) (2020). Deformación cortical y potencial sísmico en la zona de subducción del Perú. Contribución de la Geodesia [Cortical deformation and seismic potential in the Peruvian subduction zone. Contribution of Geodesy]. Lima: MINAM.
Peruvian-Japanese Center for Seismic Research and Disaster Mitigation (CISMID) (2016). Mapa de microzonificación sísmica de la ciudad de Lima. Actualizado al 2016 [Seismic microzonation map of the city of Lima. Updated to 2016]. Available at: https://sigrid.cenepred.gob.pe/sigridv3/documento/3803.
Presidency of the Ministerial Council (PCM) (2021). Política nacional de gestión del riesgo de desastres al 2050. National policy for disaster risk management 2050. Available at: https://www.gob.pe/institucion/pcm/informes-publicaciones/1892252-politica-nacional-de-gestion-del-riesgo-de-desastres-al-2050.
Presidency of the Ministerial Council (PCM) (2022). Plan Nacional de Operaciones de Emergencia (PNOE) [National Emergency Operations Plan (PNOE)], Peru. Available at: https://cdn.www.gob.pe/uploads/document/file/3582947/PLAN%20DE%20OPERACIONES%20-%20PNOE.pdf.pdf.
Santa-Cruz, S., Fernández de Córdova, G., Vilela, M., Blondet, M., Pajuelo, J., Santa-María, M., et al. (2024). “Interactive guide for post-disaster water supply in urban areas,” in Proceedings of the 18th WCEE World conference on earthquake engineering, Italy. (Accepted for publication).
Santa-Cruz, S. C., Fernandez de Cordova, G. D. C., Rivera, M. R., Vilela, M. R., Arana, V. E., and Palomino, J. (2016). Social sustainability dimensions in the seismic risk reduction of public schools: a case study of Lima, Peru. Sustain. Sci. Pract. Policy 12 (1), 1–13. doi:10.1080/15487733.2016.11908152
Santa-Cruz, S. C., Fernandez de Cordova, G. D. C., and Vilela, M. R. (2018). “Innovation in the course disaster risk management to improve the university students competence for multidisciplinary and participatory work,” in En 2018 ASEE annual conference & exposition (Salt Lake City, Utah: ASEE. Recuperado de), 1–17. Available at: http://peer.asee.org/30665.
Scolobig, A., Prior, T., Schröter, D., Jörin, J., and Patt, A. (2015). Towards people-centred approaches for effective disaster risk management: balancing rhetoric with reality. Int. J. Disaster Risk Reduct. 12, 202–212. doi:10.1016/j.ijdrr.2015.01.006
Sepúlveda, U. (2018). Recovering the spatiality of subject: qualitative spatial analysis methodologies, a model of topes, landscapes and technologies. Investig. Geográficas (96). Available at: https://www.investigacionesgeograficas.unam.mx/index.php/rig/article/view/59551/53104. doi:10.14350/rig.59551
Sharifi, A., and Yamagata, I. (2018). Resilient urban form: a conceptual framework. Lect. Notes Energy 65, 167–179. doi:10.1007/978-3-319-75798-8_9
Simioni, D. (2003). Ciudad y desastres naturales: planificación y vulnerabilidad urbana [City and natural disasters: urban vulnerability and urban planning]. In Economic Commission for Latin America and the Caribbean (ECLAC) (Ed.), Cuadernos de la CEPAL: La Ciudad Inclusiva [ECLAC's Notebooks: The inclusive city] (pp. 297–305). United Nations.
Sphere Project (2011). Carta humanitaria y normas mínimas para la respuesta humanitaria [Humanitarian charter and minimum standards for humanitarian action]. United Kingdom: Sphere Project. Available at: https://www.acnur.org/fileadmin/Documentos/Publicaciones/2011/8206.pdf.
Supreme Decree No. 106-2017-PCM (2017). “Decreto Supremo que aprueba el Reglamento para la Identificación,” in Evaluación y Gestión de Riesgos de los Activos Críticos Nacionales (ACN) [Supreme Decree approving the Regulations for the Identification, Evaluation and Risk Management of Critical National Assets (ACN)]. Available at: https://busquedas.elperuano.pe/dispositivo/NL/1585361-1.
Takemoto, S., Shibuya, N., and Sakoda, K. (2021). Learning from megadisasters: a decade of lessons from the great East Japan earthquake. The World Bank.
United Nations (UN) (2015). “Marco de Sendai para la reducción del riesgo de desastres 2015-2030. 69,” in periodo de sesiones de la Asamblea General de la ONU [Sendai Framework for Disaster Risk Reduction 2015-2030. 69th session of the UN General Assembly].
UN Refugee Agency (2023). Normas sobre el abastecimiento de agua en situaciones de emergencia [Rules on water supply in emergencies]. Available at: https://emergency.unhcr.org/es/emergency-assistance/water-hygiene-and-energy/normas-sobre-el-abastecimiento-de-agua-en-situaciones-de-emergencia.
USGS (US Geological Survey) (2023). https://earthquake.usgs.gov/scenarios/.
Vale, L., Campanella, J., and Thomas, J. (2005). The resilient city: how modern cities recover from disaster. Oxford University Press.
Vilela, M. R., and Fernandez de Cordova, G. D. C. (2016). “Transversal, Processus participatif pour l'aménagement du territoire á San Pedro de Lloc et Huamachuco, Pérou,” in En Lieuxdits#10 Urbanisme: equal opportunities human rights inclusion diversity (LOUVAIN: Université Catholique de Louvain), 20–21.
Vilela, M. R., and Fernandez de Cordova, G. D. C. (2017). “La participación como innovación en la co-producción de proyectos urbanos en el diseño de espacios públicos en ciudades intermedias,” in Transversal acciones de integración en el territorio peruano (LIMA, Peru: Fondo Editorial PUCP), 155–166.
Vilela, M. R., and Moschella, P. (2017). Paisaje y expansión urbana sobre espacios naturales en ciudades intermedias. El caso de Purrumpampa en Huamachuco, La Libertad, Perú. Bull. l'Institut français d'études Andin. 3 (46), 529–550. doi:10.4000/bifea.9003
Yepes, V. (2020). La resiliencia de las infraestructuras [Infrastructure resilience]. Available at: https://victoryepes.blogs.upv.es/2020/09/17/la-resiliencia-de-las-infraestructuras/.
Keywords: large magnitude earthquake, safe water supply, emergency response, urban resilience, emergency planning and response
Citation: Santa-Cruz S, Fernández de Córdova G, Vilela M, Pajuelo J, Santa-María M and Muñoz K (2024) Spatial analysis for water supply in seismic emergencies: the Lima-Callao metropolitan area. Front. Built Environ. 10:1385476. doi: 10.3389/fbuil.2024.1385476
Received: 12 February 2024; Accepted: 14 May 2024;
Published: 18 June 2024.
Edited by:
Solomon Tesfamariam, University of Waterloo, CanadaReviewed by:
Mohammad K. Najjar, Federal University of Rio de Janeiro, BrazilNimesh Chettri, Royal University of Bhutan, Bhutan
Copyright © 2024 Santa-Cruz, Fernández de Córdova, Vilela, Pajuelo, Santa-María and Muñoz. This is an open-access article distributed under the terms of the Creative Commons Attribution License (CC BY). The use, distribution or reproduction in other forums is permitted, provided the original author(s) and the copyright owner(s) are credited and that the original publication in this journal is cited, in accordance with accepted academic practice. No use, distribution or reproduction is permitted which does not comply with these terms.
*Correspondence: Sandra Santa-Cruz, c3NhbnRhY3J1ekBwdWNwLmVkdS5wZQ==